- 1Donghu Experimental Station of Lake Ecosystems, State Key Laboratory of Freshwater Ecology and Biotechnology of China, Institute of Hydrobiology, Chinese Academy of Sciences, Wuhan, China
- 2Laboratory for Marine Fisheries Science and Food Production Processes, Qingdao National Laboratory for Marine Science and Technology, Qingdao, China
- 3College of Advanced Agricultural Sciences, University of Chinese Academy of Sciences, Beijing, China
- 4Bren School of Environmental Science & Management, University of California, Santa Barbara, Santa Barbara, CA, United States
- 5Organismal and Evolutionary Biology, University of Helsinki, Helsinki, Finland
- 6Center for Advanced Systems Understanding (CASUS), Görlitz, Germany
- 7Helmholtz-Zentrum Dresden-Rossendorf (HZDR), Dresden, Germany
- 8College of Fisheries, Huazhong Agricultural University, Hubei Provincial Engineering Laboratory for Pond Aquaculture, Freshwater Aquaculture Collaborative Innovation Center of Hubei Province, Wuhan, China
Functional diversity is critical to ecosystem stability and resilience to disturbances as it supports the delivery of ecosystem services on which human societies rely. However, changes in functional diversity over space and time, as well as the importance of particular marine fish species to functional space are less known. Here, we reported a temporal change in the functional diversity of marine capture fisheries from all coastal provinces in China from 1989 to 2018. We suggested that both functional evenness (FEve) and functional divergence (FDiv) changed substantially over time, especially with considerable geographic variation in FEve in the detected patterns. Even within the same sea, the relative contributions of fishes with various water column positions and trophic levels in different waters have different patterns. Together these results underline the need of implementing specific climate-adaptive functional diversity conservation measures and sustainable fisheries management in different waters.
1 Introduction
Marine fisheries and ecosystems are threatened by climate change and human activities worldwide, rising issues such as habitat degradation or resource overexploitation (Brierley and Kingsford, 2009; Halpern et al., 2015; Hilborn, 2016; Hughes et al., 2017). Despite conservation efforts to restore habitat and resources, biodiversity loss continues at regional and global scales in diverse ecosystems (Vitousek et al., 1997; Cardinale et al., 2006; Maclean and Wilson, 2011; Worm and Tittensor, 2011; Mouillot et al., 2013). As a critical part of biodiversity, functional diversity indices have been increasingly applied to fish communities to explore the connection between biodiversity and ecosystem function, indicate the community structure changes, and assess the effect of multiple pressures (Villéger et al., 2010; Mouillot et al., 2013; Stuart-Smith et al., 2013; Belley and Snelgrove, 2016; Villéger et al., 2017; Rolim et al., 2022; Zhao et al., 2022). However, only a few studies so far report the functional diversity of marine fish communities.
China is the world’s largest producer of capture fisheries, accounting for approximately 15% of total global captures in 2018 (FAO, 2020). Thus, China’s sustainable marine capture fishery industry is critical to the global seafood supply, food security, and seafood trade (Smith et al., 2010; Blomeyer et al., 2012; Su et al., 2020). Zhao et al. (2022) recently used China’s reported marine catch data and a comprehensive set of functional traits to explore how the functional diversity of commercial fish communities responds to climate change and changes in fishing pressure. They showed that climate warming and fishing significantly and oppositely affect the functional diversity (i.e.,FEve and FDiv), partly causing functional diversity fluctuations in China’s seas. FEve describes the regularity of species abundance distribution in the functional space and reflects the overall utilization of resources by species. FDiv measures how species abundances diverge from the center of the functional space and reflects the competitive pressures among species in communities (Mason et al., 2005; Villéger et al., 2008). Functional diversity is a crucial facet of biodiversity, but how it changes over space and time is less known than taxonomic diversity (Trindade-Santos et al., 2020).
In recent years, Chinese government has invested extensive efforts into curbing overfishing and developing sustainable fisheries, and prioritized fishery resource conservation in marine fishery management (Han et al., 2018; Su et al., 2020; Zhao et al., 2021). Zhao et al., 2021 indicated that China’s aquaculture is transforming into a sustainable development pattern with low input and high output, reducing its dependence on marine wild fishery resources. Along with the development of Chinese sustainable capture fisheries and increasing climate change in recent decades, an enhanced understanding of how biodiversity changes in space and time could improve decision-making about establishing protected areas, sustainable fishery management, etc. Here, China’s marine catch data and functional traits reported by Zhao et al. (2022) were used to explore the temporal characteristics of functional diversity changes in fish communities caught by each province and the patterns of species’ contributions to the functional space. This study could help guide targeted biodiversity conservation policies and marine fishery management under multiple pressures across various provinces and seas.
2 Materials and methods
2.1 Data set
2.1.1 Catch data
Marine catch data of 11 coastal province administrative regions (Tianjin, Hebei, Liaoning, Shanghai, Jiangsu, Zhejiang, Fujian, Shandong, Guangdong, Guangxi, and Hainan) from 1989 to 2018 were collated from the National Fishery Statistics Yearbook (Ministry of Agriculture and Rural Affairs of the People's Republic of China, 1990-2019). From 1989, 16 species or species groups (four individual species and 12 species groups) have been reported continuously and account for more than 84% of the total reported fish catch (Supplementary Table S1). In the present study, all catches in coastal regions were from the Bohai Sea, Yellow Sea, East China Sea, and South China Sea. Catch data for all seas were obtained by combining provincial catches based on their main fishing waters (Supplementary Table S2). Although the catch data for Chinese fisheries have been a subject of scrutiny for many years, the main concern is that China may over-report its catch (Watson and Pauly, 2001; Pauly et al., 2014; Pauly and Zeller, 2016). However, scaling up or down the reported catch should not introduce bias into FEve and FDiv estimation (Zhao et al., 2022), and we used relative catch data to calculate them.
Starting from the time records began in 2003, the proportions of power between different fishing methods remain relatively stable with no drastic overall changes in all provinces (Supplementary Figure S1). Over the past three decades, trawls, gillnets, and stow nets represent the three major fishing gears, accounting for approximately 81% of the total catch in China. Despite some variations in power proportions of trawling and gillnet vessels in Tianjin, Hebei, Jiangsu, and Shanghai, the poor selectivity of trawls and gillnet characterizes Chinese fishing activities indicates that the catch comprises many species and a large yield of bycatch without specific bias towards any species. In general, China’s marine fishing practices have been exhaustive and largely nonselective, with minimal discard over the past decades (Costello et al., 2016; Costello, 2017; Szuwalski et al., 2017; Zhang et al., 2020; Zhao et al., 2021). Moreover, the proportions of China’s annual catch from different fishing methods remained relatively stable from 1989 to 2018, suggesting that no single fishing method changed disproportionately in catch over the past three decades (Zhao et al., 2022). Thus, relative catch could represent a good proxy for the compositional community changes occurring in the ocean.
2.1.2 Functional traits
The selection of traits to be incorporated into functional analyses depends on the research question. This study focused on the functional diversity characteristics of commercial marine fish communities in all coastal provinces under multiple environmental pressures. Thus, the functional trait database, which encompasses seven traits (water column position, mouth position, diet, mean preferred temperature, trophic level, maximum body length, and body shape) was obtained from Zhao et al. (2022) for four individual species and 12 species groups (species–group mean quantitative traits were used), including food acquisition, locomotion, ecological adaptation, and population dynamics (Supplementary Tables S2, S3). China’s fisheries record lumps a group of species with similar characteristics into one category. Many fish species occupy a large range, lacking differentiation between the communities according to the sea boundaries. In general, selected species that are similar in the ecological niche for each species group (Zhao et al., 2022). Thus, it is still appropriate to calculate functional diversity indices with the species–group mean quantitative traits. An underlying assumption of using a single estimate for each trait for a species or species group is that trait values are fixed and do not change for a particular species in time, space, or as individuals grow.
Subsequently, we followed the procedure described by Laliberté and Legendre (2010). First, a trait matrix for the complete set of species and species groups was constructed and then converted into a Gower dissimilarity matrix, which is a non-Euclidean measure appropriate for datasets comprising a combination of categorical and continuous traits with missing values. Then, principal coordinate analysis (PCoA) was performed on the dissimilarity matrix, and the first four axes of PCoA were selected as the new traits in accordance with Zhao et al. (2022).
2.2 Functional diversity indices
Different functional metrics provide distinct and often complementary perspectives on functional diversity (Villéger et al., 2008; Mouillot et al., 2013). Here, two complementary indices (FEve and FDiv) were calculated to assess the functional diversity of commercial marine fish communities for each province. The ecological effects of a species were well-documented to be generally proportional to its abundance or biomass (Grime, 1998). Compared to diversity metrics based on a simple count or inventory of species present within a broader geographic region, abundance-weighted functional diversity more accurately reflects community functional structure (Stuart-Smith et al., 2013). In the present study, the functional diversity indices were calculated based on the new traits (i.e., four axes of PCoA on Gower dissimilarity matrix) and the relative catch of species or species groups (Zhao et al., 2022). All functional diversity metrics were computed using the “FD” package in R.
2.3 Statistical analysis
First, locally estimated scatterplot smoothing regression was used to assess each province’s temporal nonlinearity in functional diversity indices. Then, relative contribution of each species or species group was calculated by sequentially removing species or species groups from the dataset and then recalculating the functional diversity indices and computing the percent change in diversity value before and after species disappear (Pool et al., 2014). Here, the fishing water of each province’s fishing fleets was assumed to have not changed over the past three decades. All commercial species or species groups have been reported in the Bohai and Yellow Seas, the East China Sea and the South China Sea. Thus, several species or species groups were unreported in some years, which should be too little in catches. Relative catches for unreported species or species groups were counted as 0. As the four axes of PCoA was used to construct the trait space, unreported species or species groups could still affect the relative positions of all species or species groups in the functional space when removed. Here, the relative contributions of species or species groups with catch count of 0 were generally small but still retained. Besides, FEve and FDiv were related to changes in species abundance rather than richness. Counting the catch of unreported species or species groups as 0 would cause little bias, and we didn’t consider them here. Interquartile range method was used to detect outliers in the resulting relative contribution dataset (Rousseeuw and Hubert, 2011; Vinutha et al., 2018). The “wilcox.test” function from the “stats” package (R Development Core Team, 2020) was used to conduct Wilcoxon rank-sum tests and compare the relative contributions of fish species or species groups with different water column positions (demersal and pelagic) and trophic levels (with 3.5 as the threshold) to FD. All statistical analyses were performed using R version 4.1.1 for Windows (R Development Core Team, 2020).
3 Results
3.1 Dynamics of functional diversity across all provinces
Across all provinces, FEve fluctuated stronger than FDiv over the past three decades, except for the Guangdong (Figure 1). The functional diversity indices of five provinces (Tianjin, Hebei, Liaoning, Jiangsu, and Shandong) that mainly fished in the Bohai and Yellow Seas were the most unstable, followed by three provinces (Shanghai, Zhejiang, and Fujian) that mainly fished in the East China Sea. Three provinces (Guangdong, Guangxi, and Hainan) that mainly fished in the South China Sea exhibited a considerably stable functional diversity over time for both functional diversity indices.
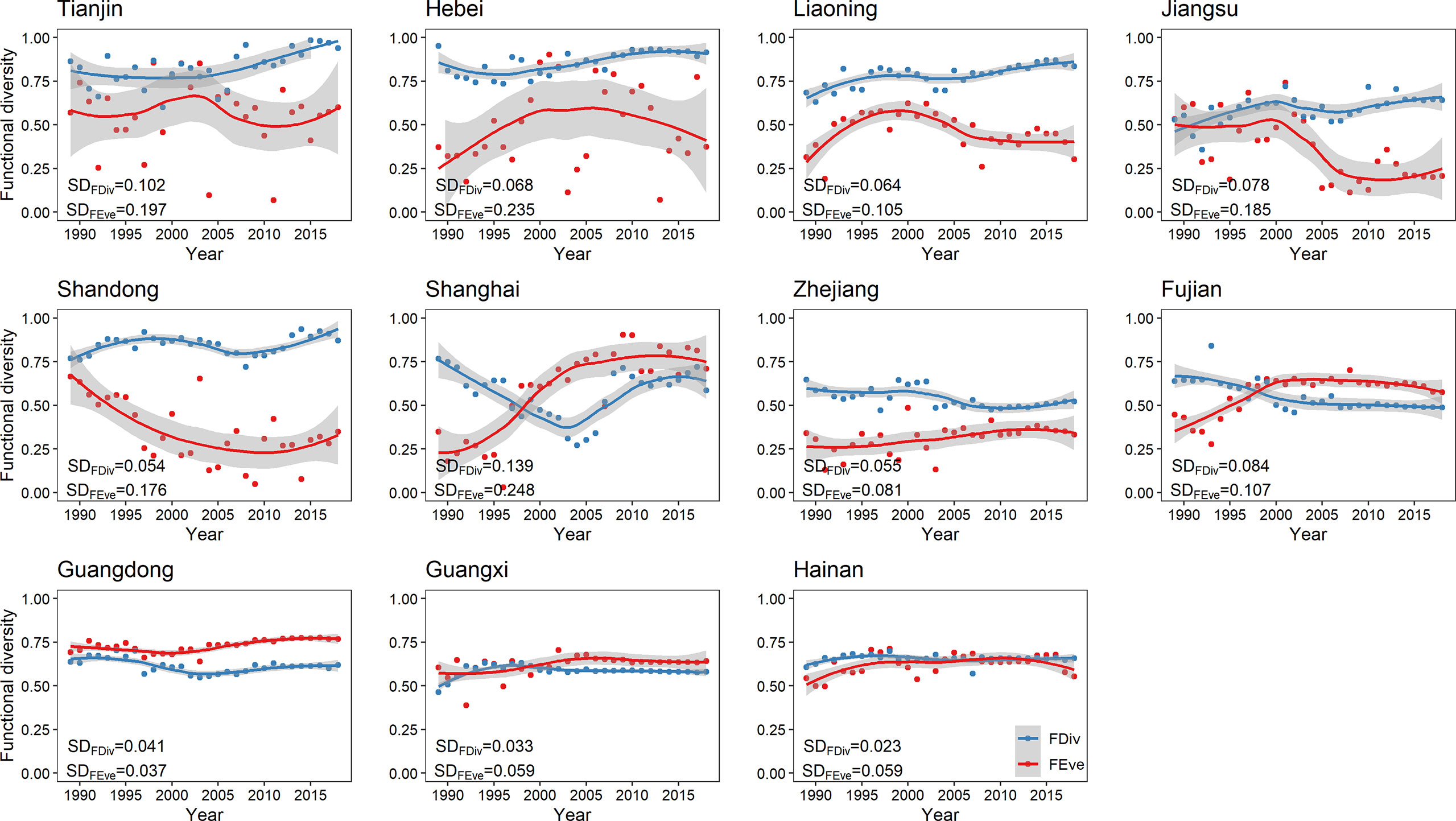
Figure 1 Locally estimated scatterplot smoothing regression curves of functional diversity for each coastal province from 1989 to 2018. The shaded gray column indicates 95% confidence interval. SDFEve, standard deviation of functional evenness; SDFDiv, standard deviation of functional divergence. (FEve, functional evenness; FDiv, functional divergence).
3.2 Relative contribution of fishes to functional space
The relative contributions of fishes in the Bohai and Yellow Seas and the South China Sea were more concentrated than that in the East China Sea (Figure 2). The relative contributions of fishes in the South China Sea to both functional diversity indices were lower than that in the Bohai and Yellow Seas and East China Sea, except for FDiv in the Bohai and Yellow Seas (Figure 2). In most provinces, the relative contributions of fishes with different trophic levels to both functional diversity indices varied greatly. Only few provinces had significant differences in the relative contributions of fishes with different water column positions (Figures 3, 4). In all provinces, the contribution of low-trophic-level fish to functional diversity was higher than that of high-trophic-level fish, except for Shanghai and Guangxi (Figure 3). In Liaoning, Shandong, Fujian, and Hainan, demersal fish contributed more to FEve, while pelagic fish contributed more to FDiv in Guangdong, Guangxi, and Hainan (Figure 4).
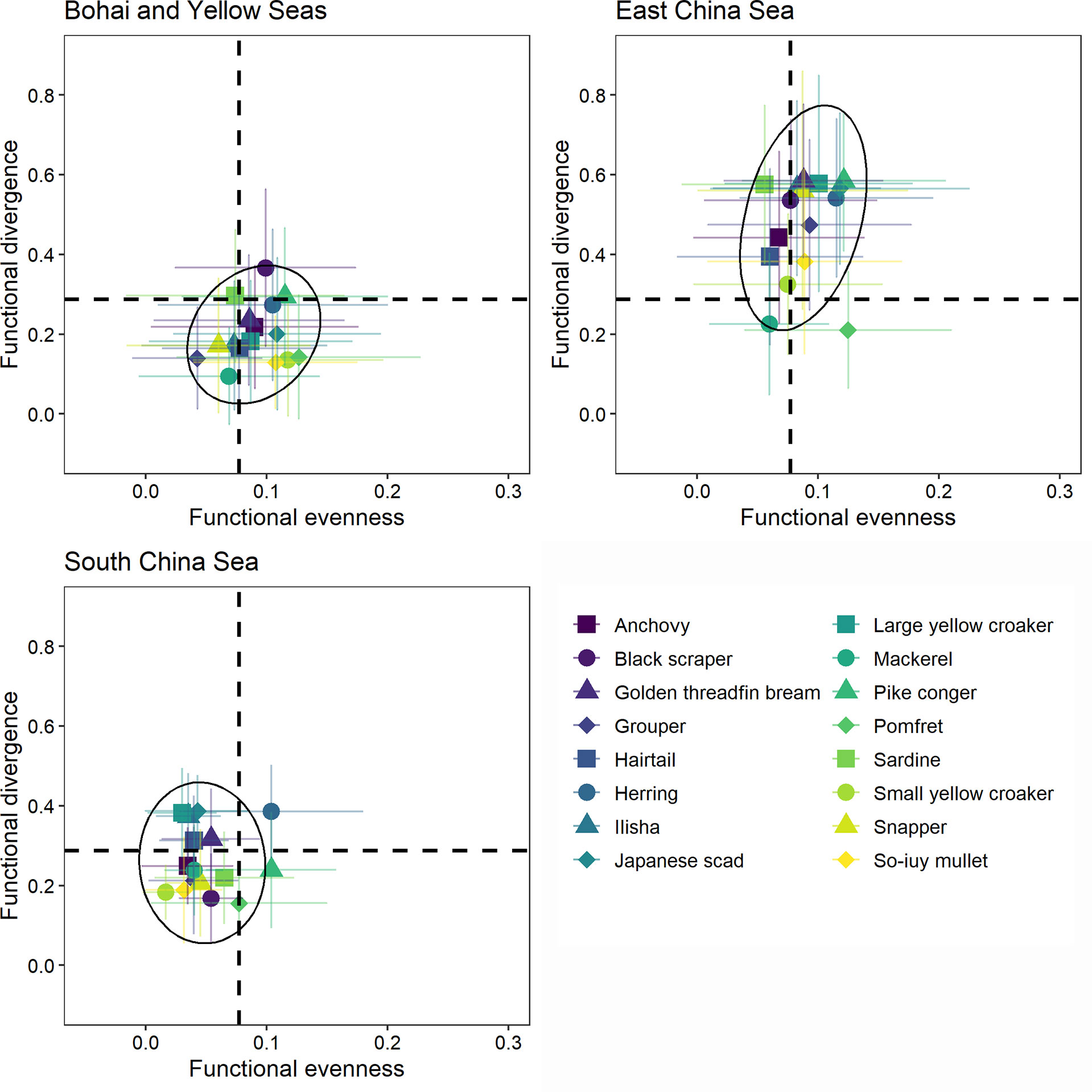
Figure 2 Contribution of 16 species or species groups to functional diversity in different seas. The dashed line indicates the average contribution of all species across three seas. The black circle is 95% confidence level ellipse for a multivariate t-distribution.
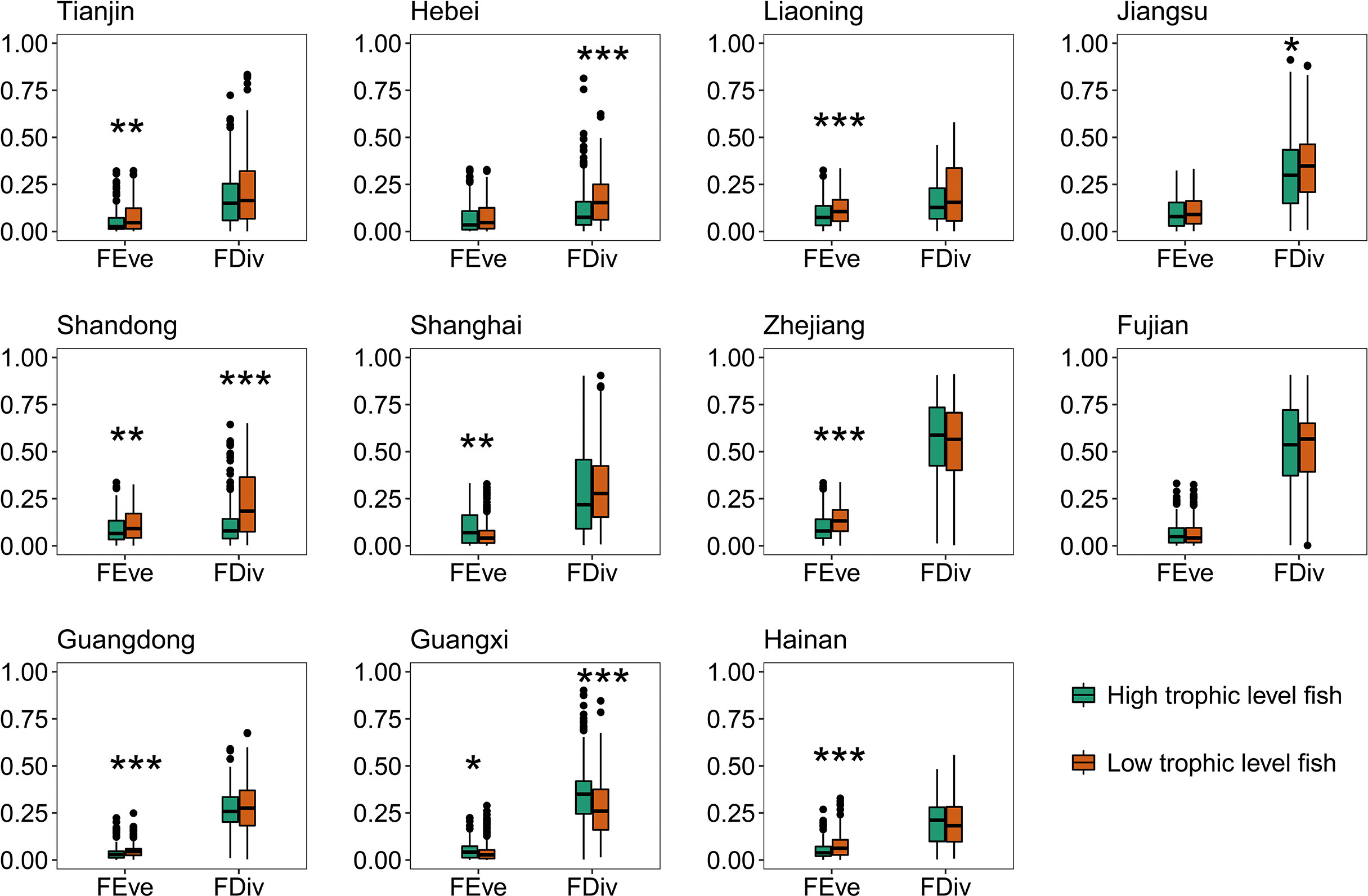
Figure 3 Comparison of relative contributions of fishes with different trophic levels to functional diversity for 11 provinces. The results of Wilcoxon rank-sum tests are shown upon each plotted item (*p < 0.05; **p < 0.01; ***p < 0.001; Supporting Information Table S5 shows the complete Wilcoxon rank-sum tests. FEve, functional evenness; FDiv, functional divergence).
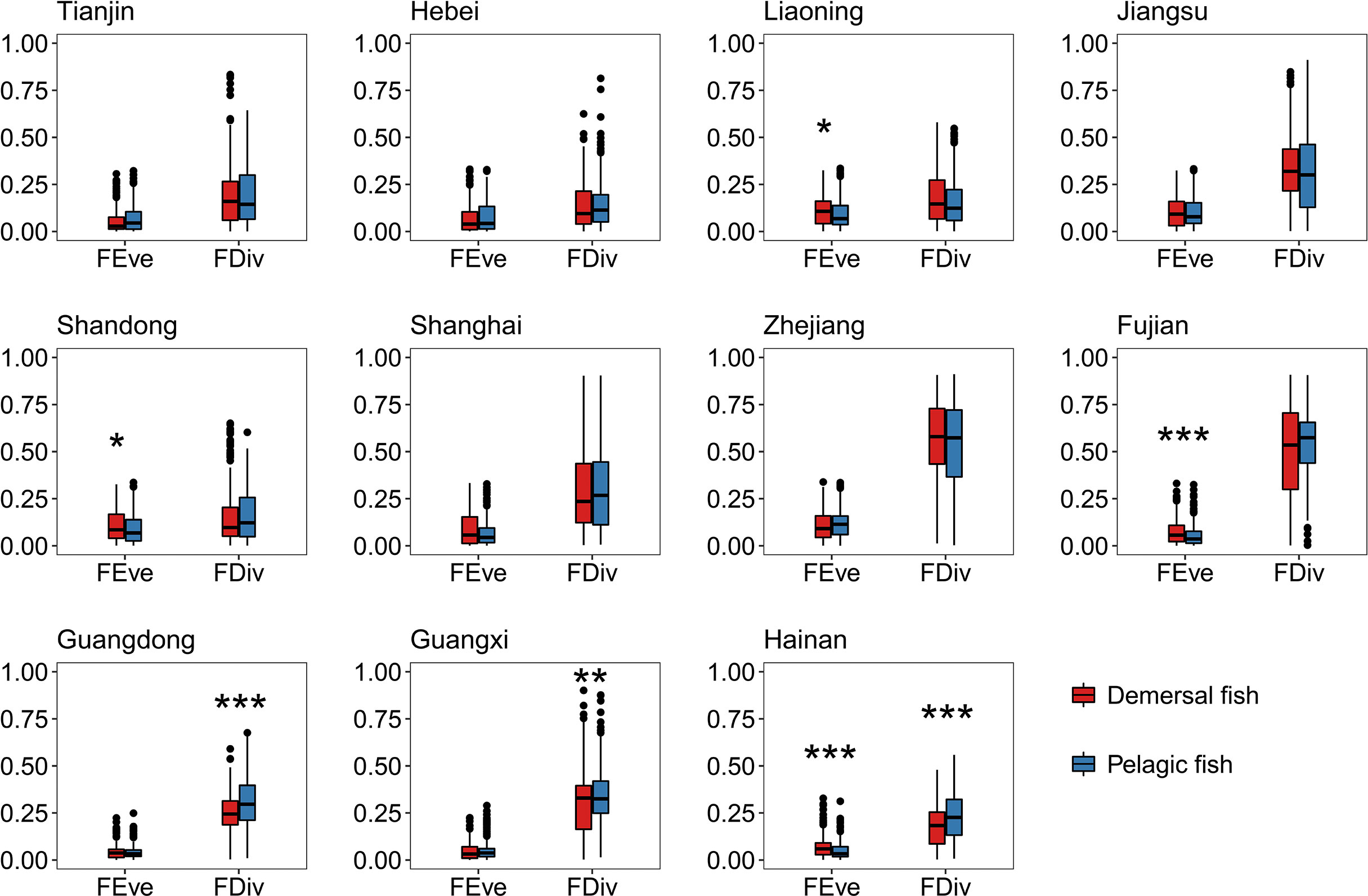
Figure 4 Comparison of relative contributions of fishes with different water column positions to functional diversity for 11 provinces. The results of Wilcoxon rank-sum tests are shown upon each plotted item (*p < 0.05; **p < 0.01; ***p < 0.001; Supporting Information Table S5 shows the complete Wilcoxon rank-sum tests. FEve, functional evenness; FDiv, functional divergence).
4 Discussion
Functional diversity is a key facet of biodiversity that accounts for the diversity of biological and ecological features of organisms related to their responses to the environment and their effects on ecosystem processes (Su et al., 2022). The functional diversity of marine fish communities is facing pressure from habitat degradation, human activities, climate change, and overfishing (Villéger et al., 2010; D'Agata et al., 2014; Brandl et al., 2016; Zhao et al., 2022). In China, marine fishery resources and functional diversity have been affected by various factors, such as overfishing and climate change (Blasiak et al., 2017; Costello, 2017; Zhao et al., 2022). However, evaluating the functional diversity of marine fish communities and the influencing factors is insufficient. The results of the present study showed that the functional diversity of different provinces had different performance characteristics under multiple disturbances, and the most considerable temporal fluctuations were found in provinces that fished in the Bohai and Yellow Seas and East China Sea (Figure 1). This finding indicated that the structure of the fish communities fished by Tianjin, Hebei, Liaoning, Jiangsu, and Shanghai fluctuated wildly, and these communities were probably more vulnerable in terms of resistance to external multiple pressures than those fished by Guangdong, Guangxi, and Hainan.
Our results showed that the relative contributions of fish species in the Bohai and Yellow Seas and the East China Sea were generally higher than that in the South China Sea (Figure 2 and Supplementary Figure S2). Moreover, in the past three decades, the functional diversity of provinces that fished in the Bohai and Yellow Seas and the East China Sea fluctuated greatly (Figure 1). If a particular species disappears [e.g., redistribution following the gradients of habitat suitability by their temperature preferences and limits or even local disappearance as temperature limits are exceeded (Cheung et al., 2010; Jones and Cheung, 2015; Cheung et al., 2016)] in the Bohai and Yellow Seas and the East China Sea, it would lead to more remarkable changes in the functional space and increased vulnerability to external environmental disturbance. Moreover, fish and other animals have already moved poleward and such movements are expected to continue or accelerate (Poloczanska et al., 2013; Cheung et al., 2016; Pinsky et al., 2020; Melbourne-Thomas et al., 2021). Thus, even in the South China Sea, where functional diversity is more stable, fish communities structure may change greatly in the future.
Meanwhile, low-trophic-level fish generally showed greater relative contributions to functional diversity (Figures 3, 4). This finding could be supported laterally by the substantial changes in the domestic marine catch composition, from the predominance of a few medium to relatively large-sized, high-valued, high-trophic-level demersal species to multiple small, low-valued, short-lived, low-trophic-level ones over the past six decades (Liu and De Mitcheson, 2008; Shen and Heino, 2014; Cao et al., 2017; Zhang et al., 2020). This phenomenon also happens worldwide, fishing down the marine food webs (Pauly et al., 1998). The extensive reduction in low-trophic-level forage fish (e.g., anchovy, sardine, and herring) generate concerns that commercial forage fish resources have not been merely overharvested but caused severe effects on the diversity of marine fish communities (Zhang et al., 2020; Zhao et al., 2022; Zhao et al., 2021; Zheng et al., 2014). Moreover, even within the same sea, no fixed pattern of the relative contributions of fishes existed with different water column positions and trophic levels in different waters (Figures 3, 4). Thus, designating climate-adaptive conservation schemes in accordance with different fish community features are necessary to improve functional diversity conservation.
At present, the Chinese government is vigorous in controlling marine overfishing (Han et al., 2018; Su et al., 2020; Zhao et al., 2021), but climate change could be difficult to control in the short term. Meanwhile, controlling overfishing alone will not conserve functional diversity of commercial marine fish communities under climate change (Zhao et al., 2022). Along with the increasing climate warming, the productivity of fish stocks is predicted to decrease in tropical and temperate regions and increase towards the poles (Lotze et al., 2019). A shifting fish stock exacerbates existing fisheries and sustainable management challenges need to be more concerned, and all coastal provinces should strengthen cooperation. Cooperation should extend beyond data sharing to inform genuinely collaborative management, which demands an emphasis on periodically acquiring reliable assessment of species shifts and changes in community structures. Finally, China should develop specific climate-adaptive sustainable fishery management in different waters, which is necessary to consider functional diversity.
Data availability statement
The original contributions presented in the study are included in the article/Supplementary Material, further inquiries can be directed to the corresponding author/s.
Author contributions
PZ and KZ conceived the study. PZ, MZ, and KZ collected and analyzed the data. KZ and MZ drafted the manuscript. All authors checked and edited the final manuscript. All authors contributed to the article and approved the submitted version.
Funding
This research was also supported by the International Cooperation Project of the Chinese Academy of Sciences (Grant No. 152342KYSB20190025) and the National Natural Science Foundations of China (Grant No. 31872687).
Acknowledgments
A special thanks to Professor Jun Xu, Jorge Garcı́a Molinos and Steven D. Gaines for the suggestions to some earlier data processing.
Conflict of interest
The authors declare that the research was conducted in the absence of any commercial or financial relationships that could be construed as a potential conflict of interest.
Publisher’s note
All claims expressed in this article are solely those of the authors and do not necessarily represent those of their affiliated organizations, or those of the publisher, the editors and the reviewers. Any product that may be evaluated in this article, or claim that may be made by its manufacturer, is not guaranteed or endorsed by the publisher.
Supplementary material
The Supplementary Material for this article can be found online at: https://www.frontiersin.org/articles/10.3389/fmars.2022.970218/full#supplementary-material
References
Belley R., Snelgrove P. V. (2016). Relative contributions of biodiversity and environment to benthic ecosystem functioning. Front. Mar. Sci. 3, 242. doi: 10.3389/fmars.2016.00242
Blasiak R., Spijkers J., Tokunaga K., Pittman J., Yagi N., Österblom H. (2017). Climate change and marine fisheries: Least developed countries top global index of vulnerability. PLoS One 12, e0179632. doi: 10.1371/journal.pone.0179632
Blomeyer R., Goulding I., Pauly D., Sanz A., Stobberup K. (2012). “The role of China in world fisheries,” in Directorate general for internal policies. policy department b (European Union: Structural and Cohesion Policies. European Parliament).
Brandl S. J., Emslie M. J., Ceccarelli D. M., Z.T. R. (2016). Habitat degradation increases functional originality in highly diverse coral reef fish assemblages. Ecosphere 7, e01557. doi: 10.1002/ecs2.1557
Brierley A. S., Kingsford M. J. (2009). Impacts of climate change on marine organisms and ecosystems. Curr. Biol. 19, R602–R614. doi: 10.1016/j.cub.2009.05.046
Cao L., Chen Y., Dong S., Hanson A., Huang B., Leadbitter D., et al. (2017). Opportunity for marine fisheries reform in China. Proc. Natl. Acad. Sci. 114, 435–442. doi: 10.1073/pnas.1616583114
Cardinale B. J., Srivastava D. S., Duffy J. E., Wright J. P., Downing A. L., Sankaran M., et al. (2006). Effects of biodiversity on the functioning of trophic groups and ecosystems. Nature 443, 989–992. doi: 10.1038/nature05202
Cheung W. W., Lam V. W., Sarmiento J. L., Kearney K., Watson R., Zeller D., et al. (2010). Large-Scale redistribution of maximum fisheries catch potential in the global ocean under climate change. Global Change Biol. 16, 24–35. doi: 10.1111/j.1365-2486.2009.01995.x
Cheung W. W., Reygondeau G., Frölicher T. L. (2016). Large Benefits to marine fisheries of meeting the 1.5 c global warming target. Science 354, 1591–1594. doi: 10.1126/science.aag2331
Costello C. (2017). Fish harder; catch more? Proc. Natl. Acad. Sci. 114, 1442–1444. doi: 10.1073/pnas.1620731114
Costello C., Ovando D., Clavelle T., Strauss C. K., Hilborn R., Melnychuk M. C., et al. (2016). Global fishery prospects under contrasting management regimes. Proc. Natl. Acad. Sci. 113, 5125–5129. doi: 10.1073/pnas.1520420113
D'Agata S., Mouillot D., Kulbicki M., Andrefouet S., Bellwood D. R., Cinner J. E., et al. (2014). Human-mediated loss of phylogenetic and functional diversity in coral reef fishes. Curr. Biol. 24, 555–560. doi: 10.1016/j.cub.2014.01.049
FAO (2020). The state of world fisheries and aquaculture (Rome Italy: Food and Agriculture Organization of the United Nations), 40–41.
Grime J. (1998). Benefits of plant diversity to ecosystems: immediate, filter and founder effects. J. Ecol. 86, 902–910. doi: 10.1046/j.1365-2745.1998.00306.x
Halpern B. S., Frazier M., Potapenko J., Casey K. S., Koenig K., Longo C., et al. (2015). Spatial and temporal changes in cumulative human impacts on the world’s ocean. Nat. Commun. 6, 1–7. doi: 10.1038/ncomms8615
Han D., Shan X. J., Zhang W. B., Chen Y. S., Wang Q. Y., Li Z. J., et al. (2018). A revisit to fishmeal usage and associated consequences in Chinese aquaculture. Rev. Aquaculture. 10, 493–507. doi: 10.1111/raq.12183
Hilborn R. (2016). Policy: Marine biodiversity needs more than protection. Nature 535, 224–226. doi: 10.1038/535224a
Hughes T. P., Barnes M. L., Bellwood D. R., Cinner J. E., Cumming G. S., Jackson J. B. C., et al. (2017). Coral reefs in the anthropocene. Nature 546, 82–90. doi: 10.1038/nature22901
Jones M. C., Cheung W. W. (2015). Multi-model ensemble projections of climate change effects on global marine biodiversity. ICES. J. Mar. Sci. 72, 741–752. doi: 10.1093/icesjms/fsu172
Laliberté E., Legendre P. (2010). A distance-based framework for measuring functional diversity from multiple traits. Ecology 91, 299–305. doi: 10.1890/08-2244.1
Liu M., De Mitcheson Y. S. (2008). Profile of a fishery collapse: why mariculture failed to save the large yellow croaker. Fish. Fisheries. 9, 219–242. doi: 10.1111/j.1467-2979.2008.00278.x
Lotze H. K., Tittensor D. P., Bryndum-Buchholz A., Eddy T. D., Cheung W. W., Galbraith E. D., et al. (2019). Global ensemble projections reveal trophic amplification of ocean biomass declines with climate change. Proc. Natl. Acad. Sci. 116, 12907–12912. doi: 10.1073/pnas.1900194116
Maclean I. M., Wilson R. J. (2011). Recent ecological responses to climate change support predictions of high extinction risk. Proc. Natl. Acad. Sci. 108, 12337–12342. doi: 10.1073/pnas.1017352108
Mason N. W. H., Mouillot D., Lee W. G., Wilson J. B. (2005). Functional richness, functional evenness and functional divergence: the primary components of functional diversity. Oikos 111, 112–118. doi: 10.1111/j.0030-1299.2005.13886.x
Melbourne-Thomas J., Audzijonyte A., Brasier M.J., et al. Poleward bound: adapting to climate-driven species redistribution. Rev Fish Biol Fisheries 32, 231–251 (2022). doi: 10.1007/s11160-021-09641-3
Ministry of Agriculture and Rural Affairs of the People's Republic of China (1990-2019). Chinese National fishery statistics yearbook (China Agriculture Press).
Mouillot D., Graham N. A. J., Villéger S., Mason N. W. H., Bellwood D. R. (2013). A functional approach reveals community responses to disturbances. Trends Ecol. Evol. 28, 167–177. doi: 10.1016/j.tree.2012.10.004
Pauly D., Belhabib D., Blomeyer R., Cheung W., Cisneros-Montemayor A. M., Copeland D., et al. (2014). China's distant-water fisheries in the 21st century. Fish. Fisheries. 15, 474–488. doi: 10.1111/faf.12032
Pauly D., Christensen V., Dalsgaard J., Froese R., Torres F. (1998). Fishing down marine food webs. Science 279, 860. doi: 10.1126/science.279.5352.860
Pauly D., Zeller D. (2016). Catch reconstructions reveal that global marine fisheries catches are higher than reported and declining. Nat. Commun. 7, 10244. doi: 10.1038/ncomms10244
Pinsky M. L., Selden R. L., Kitchel Z. J. (2020). Climate-driven shifts in marine species ranges: Scaling from organisms to communities. Annu. Rev. Mar. Sci. 12, 153–179. doi: 10.1146/annurev-marine-010419-010916
Poloczanska E. S., Brown C. J., Sydeman W. J., Kiessling W., Schoeman D. S., Moore P. J., et al. (2013). Global imprint of climate change on marine life. Nat. Climate Change 3, 919–925. doi: 10.1038/nclimate1958
Pool T. K., Grenouillet G., Villéger S., Ricciardi A. (2014). Species contribute differently to the taxonomic, functional, and phylogenetic alpha and beta diversity of freshwater fish communities. Diversity Distributions. 20, 1235–1244. doi: 10.1111/ddi.12231
R Development Core Team (2020). R: A language and environment for statistical computing, 3.6.3 (Vienna, Austria: R Foundation for Statistical Computing).
Rolim F. A., Langlois T., Motta F., Castro G., Abieri M. L., Gadig O. B. F., et al. (2022). Habitat and marine reserve status drive reef fish biomass and functional diversity in the largest south Atlantic coral reef system (Abrolhos, Brazil). Front. Mar. Sci. 9, 678. doi: 10.3389/fmars.2022.701244
Rousseeuw P. J., Hubert M. (2011). Robust statistics for outlier detection. Wiley interdisciplinary reviews. Data Min. Knowledge. Discovery 1, 73–79. doi: 10.1002/widm.2
Shen G., Heino M. (2014). An overview of marine fisheries management in China. Mar. Policy 44, 265–272. doi: 10.1016/j.marpol.2013.09.012
Smith M. D., Roheim C. A., Crowder L. B., Halpern B. S., Turnipseed M., Anderson J. L., et al. (2010). Sustainability and global seafood. Science 327, 784–786. doi: 10.1126/science.1185345
Stuart-Smith R. D., Bates A. E., Lefcheck J. S., Duffy J. E., Baker S. C., Thomson R. J., et al. (2013). Integrating abundance and functional traits reveals new global hotspots of fish diversity. Nature 501, 539–542. doi: 10.1038/nature12529
Su S., Tang Y., Chang B., Zhu W., Chen Y. (2020). Evolution of marine fisheries management in China from 1949 to 2019: How did China get here and where does China go next? Fish. Fisheries. 21, 435–452. doi: 10.1111/faf.12439
Su G., Tedesco P. A., Toussaint A., Villéger S., Brosse S. (2022). Contemporary environment and historical legacy explain functional diversity of freshwater fishes in the world rivers. Global Ecol. Biogeography. 31, 700–713. doi: 10.1111/geb.13455
Szuwalski C. S., Burgess M. G., Costello C., Gaines S. D. (2017). High fishery catches through trophic cascades in China. Proc. Natl. Acad. Sci. United. States America 114, 717–721. doi: 10.1073/pnas.1612722114
Trindade-Santos I., Moyes F., Magurran A. E. (2020). Global change in the functional diversity of marine fisheries exploitation over the past 65 years. Proc. R. Soc. B. 287, 20200889. doi: 10.1098/rspb.2020.0889
Villéger S., Brosse S., Mouchet M., Mouillot D., Vanni M. J. (2017). Functional ecology of fish: current approaches and future challenges. Aquat. Sci. 79, 783–801. doi: 10.1007/s00027-017-0546-z
Villéger S., Mason N. W., Mouillot D. (2008). New multidimensional functional diversity indices for a multifaceted framework in functional ecology. Ecology 89, 2290–2301. doi: 10.1890/07-1206.1
Villéger S., Miranda J. R., Hernández D. F., Mouillot D. (2010). Contrasting changes in taxonomic vs. functional diversity of tropical fish communities after habitat degradation. Ecol. Appl. 20, 1512–1522. doi: 10.1890/09-1310.1
Vinutha H., Poornima B., Sagar B. (2018). Detection of outliers using interquartile range technique from intrusion dataset, information and decision sciences. Springer pp, 511–518. doi: 10.1007/978-981-10-7563-6_53
Vitousek P. M., Mooney H. A., Lubchenco J., Melillo J. M. (1997). Human domination of earth's ecosystems. Science 277, 494–499. doi: 10.1126/science.277.5325.494
Watson R., Pauly D. (2001). Systematic distortions in world fisheries catch trends. Nature 414, 534–536. doi: 10.1038/35107050
Worm B., Tittensor D. P. (2011). Range contraction in large pelagic predators. Proc. Natl. Acad. Sci. 108, 11942–11947. doi: 10.1073/pnas.1102353108
Zhang W. B., Liu M., de Mitcheson Y. S., Cao L., Leadbitter D., Newton R., et al. (2020). Fishing for feed in China: Facts, impacts and implications. Fish. Fisheries. 21, 47–62. doi: 10.1111/faf.12414
Zhao K., Gaines S. D., García Molinos J., Zhang M., Xu J. (2022). Climate change and fishing are pulling the functional diversity of the world's largest marine fisheries to opposite extremes. Global Ecol. Biogeography.doi: 10.1111/geb.13534
Zhao K., Zhang M., Wang K., Zhu K., Xu C., Xie J., et al. (2021). Aquaculture impacts on china’s marine wild fisheries over the past 30 years. Front. Mar. Sci. 31(8):1616–1629. 10.3389/fmars.2021.710124
Keywords: functional diversity, biodiversity conservation, fisheries management, marine ecosystem, sustainable development
Citation: Zhao K, He Y, Su G, Xu C, Xu X, Zhang M and Zhang P (2022) Implications for functional diversity conservation of China’s marine fisheries. Front. Mar. Sci. 9:970218. doi: 10.3389/fmars.2022.970218
Received: 15 June 2022; Accepted: 09 September 2022;
Published: 23 September 2022.
Edited by:
Angel Borja, Technology Center Expert in Marine and Food Innovation (AZTI), SpainCopyright © 2022 Zhao, He, Su, Xu, Xu, Zhang and Zhang. This is an open-access article distributed under the terms of the Creative Commons Attribution License (CC BY). The use, distribution or reproduction in other forums is permitted, provided the original author(s) and the copyright owner(s) are credited and that the original publication in this journal is cited, in accordance with accepted academic practice. No use, distribution or reproduction is permitted which does not comply with these terms.
*Correspondence: Min Zhang, emhtNzg3NUBtYWlsLmh6YXUuZWR1LmNu; Peiyu Zhang, emhhbmdwZWl5dUBpaGIuYWMuY24=