- 1National Institute of Water and Atmospheric Research Ltd., Nelson, New Zealand
- 2Institute for Marine and Antarctic Studies, University of Tasmania, Hobart, TAS, Australia
- 3National Institute of Water and Atmospheric Research Ltd., Wellington, New Zealand
The grenadiers Macrourus caml and M. whitsoni form a significant bycatch component of longline fisheries for Antarctic toothfish (Dissostichus mawsoni) in the Southern Ocean. A lack of species-level biological and catch data has to date hindered the development of quantitative assessments for these deepwater species. This paper examines species- and sex-specific life histories of M. caml and M. whitsoni in the Ross Sea region, Antarctica, from samples collected from the commercial fishery and research collections. Macrourus caml was found to live longer, grow slower, and attain a larger maximum length than M. whitsoni, reaching at least 65 years of age and 97 cm total length (TL) vs. 43 years and 78 cm TL for M. whitsoni. In addition, M. caml attains a larger length for a given age and a greater weight for a given length. For each species, females of a given age were larger and reached a greater maximum age than males. Assuming that selectivity of the fishing gear is related to fish size, greater fishing pressure on females than males is likely, and was evidenced by female-biased sex ratios of both species. Estimates of natural and fishing mortality rates were low for both species. Despite having a shorter lifespan and attaining a smaller maximum length, M. whitsoni matured later in life and at larger lengths than M. caml. Although sampling opportunities are constrained seasonally due to sea ice cover, gonad staging and interannual patterns in gonadosomatic indices suggest prolonged spawning for both species, with available data indicating peak spawning during austral summer. The differences in life history observed between these two closely related and morphologically similar species illustrates the importance of understanding species-specific life histories to infer responses to exploitation, provide key biological inputs to inform parameters for future risk assessments and ecosystem models as well as baseline information for comparative work over both time and space, contribute to greater clarity in managing fisheries that interact with these two species, and further our understanding of grenadier life history.
Introduction
Grenadiers (Family Macrouridae) are a large and diverse family of fishes, with around 400 species found throughout the world’s oceans (Iwamoto, 2008). Grenadiers are mostly euryphagous predators and scavengers, occurring from the upper continental slope down to the abyssal depths (Cohen et al., 1990; Pinkerton et al., 2012). While the biology of many grenadier species is largely uncertain, available information suggests they possess life-history traits characteristic of deep-sea fish, including high longevity, slow growth, late maturation, and low rates of natural mortality, making them sensitive to fishing (Bergstad, 1990; Kelly et al., 1997; Rodríguez-Marín et al., 2002).
Grenadiers form significant target and bycatch components of a number of the world’s fisheries. In particular, grenadiers are the main bycatch species in bottom longline fisheries for Antarctic and Patagonian toothfish (Dissostichus mawsoni and D. eleginoides) in the Southern Ocean (Delegations of France and Australia, 2020; Moore and Parker, 2021). Fisheries for these species began in the Ross Sea region (RSR) in the western Pacific sector of the Southern Ocean in the late 1990s, principally targeting D. mawsoni, before expanding to other areas around Antarctica (Hanchet et al., 2015). These fisheries are managed according to the Convention for the Conservation of Antarctic Marine Living Resources with a specific requirement to minimize the effect of fishing on associated species and the overall ecosystem. To meet these goals, toothfish fisheries have high observer coverage, with at least one national and Scheme of International Scientific Observation (SISO) observer onboard every vessel, and high sampling rates of both target and bycatch species for each fishing haul to provide the information necessary for management.
Historically, grenadier catches in the RSR toothfish fishery were considered to predominantly comprise three species: the ridge scaled grenadier (Macrourus carinatus), bigeye grenadier (M. holotrachys), and Whitson’s grenadier (M. whitsoni), with M. whitsoni considered the most commonly observed species (Hanchet et al., 2008). However, genetic examination revealed that what was previously regarded as M. whitsoni was comprised of two distinct, albeit morphologically similar, species, now recognized as M. caml (Caml grenadier) and M. whitsoni (Whitson’s grenadier) (Smith et al., 2011; McMillan et al., 2012). Macrourus caml and M. whitsoni exhibit overlapping circumpolar distributions in Antarctic waters (Gon et al., 2021). Macrourus caml and M. whitsoni comprise almost 75% of the total bycatch of the RSR toothfish fishery by abundance, with the vast majority of landings being retained for processing (Moore and Parker, 2021). In addition, the two species play a crucial role in ecosystem functioning of the RSR, being the major prey species of D. mawsoni, the top piscine predator in the region (Stevens et al., 2014).
Pinkerton et al. (2013) provide a preliminary examination of the life history and distribution of M. caml and M. whitsoni in the RSR, based on samples collected from the commercial longline fishery for Antarctic toothfish. Life history parameters examined included age and growth, length- and age-at-female maturity, as well as diets, trophic ecology and depth distributions of the two species. Results indicated that the two species have contrasting life histories and thus vulnerabilities to fishing, with M. caml living longer and reaching a greater maximum length than M. whitsoni. Their analyses, however, were limited in time and space, and sample size, being restricted to data collected from New Zealand fishing vessels in a single fishing season (December 2011 to February 2012). Moreover, as the RSR toothfish fishery typically selects for larger individuals of both M. caml and M. whitsoni, small fish were largely missing from their dataset, constraining or preventing the determination of several key population parameter estimates. As such, there is a need to revisit the biology of these two cryptic species to provide more accurate estimates of biological parameters to strengthen future risk assessments and management efforts.
In this paper, we provide an in-depth examination of the biology of M. caml and M. whitsoni in the RSR, taking advantage of data and samples collected through multiple years of species-level sampling by scientific observers, two out-of-season fishery surveys, and two fisheries-independent trawl surveys. We combine biological data collected from these sources to compare and contrast length-length and length-weight relationships, sex ratios, age and growth relationships, mortality rates, length- and age-at-maturity, and spawning seasonality between the two species. Results from this study provide key biological inputs to inform parameters for future risk assessments and ecosystem models and as baseline information for comparative work over both time and space, and further contribute to our understanding of grenadier life history.
Materials and methods
Study region
The RSR covers the Commission for the Conservation of Antarctic Marine Living Resources (CCAMLR) Subarea 88.1 and Small-Scale Research Units (SSRUs) 88.2A–B, encompassing an area of roughly 2 million km2 and extending south of 60° S and between 150° E and 150° W (Figure 1). The RSR can be divided into three subregions: the continental shelf (hereafter RSR shelf), the continental slope (hereafter RSR slope), and the northern RSR characterized by the presence of numerous seamounts.
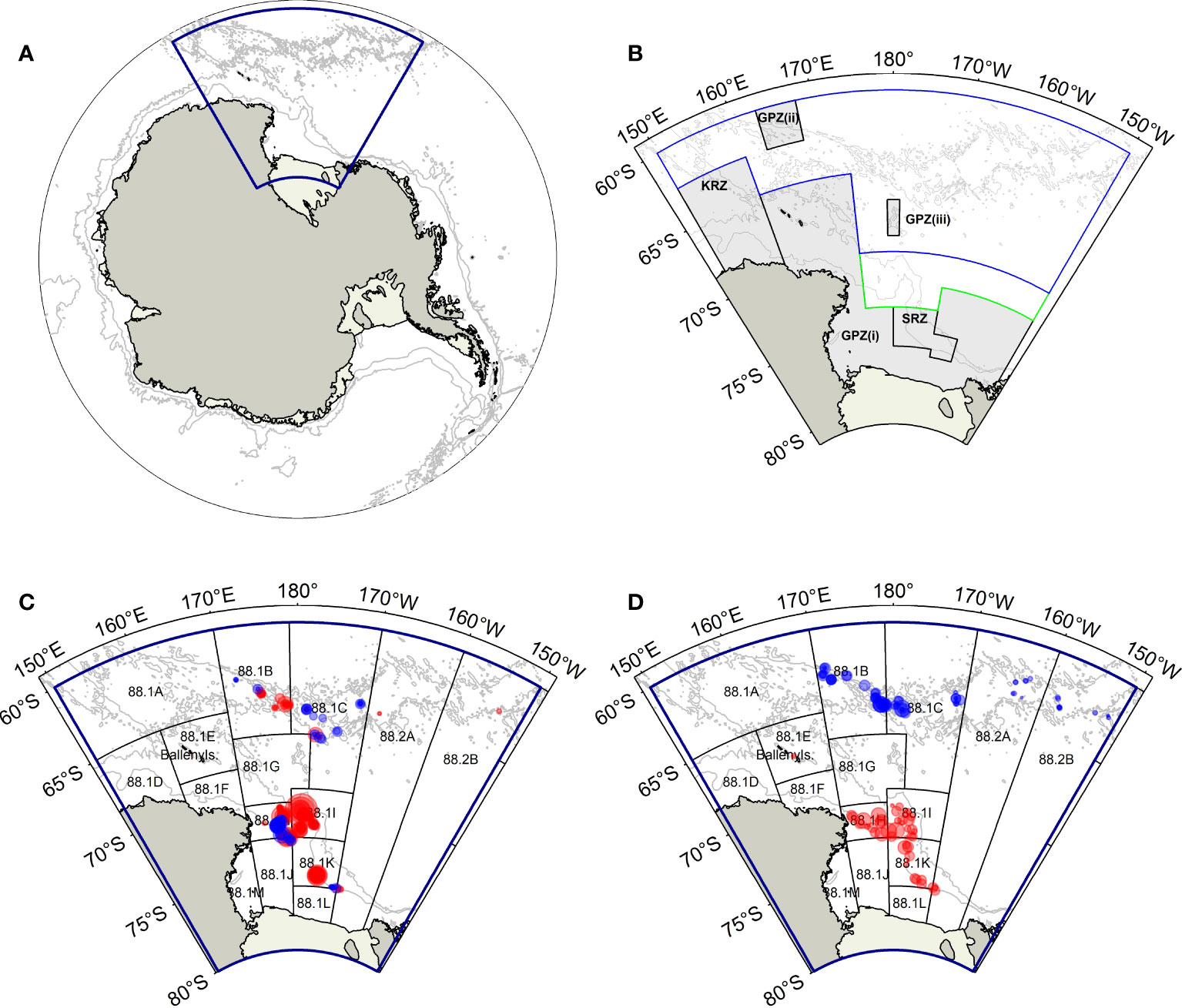
Figure 1 (A) The Antarctic continent, showing the Ross Sea region (RSR; blue polygon); (B) The RSR, showing the Ross Sea region Marine Protected Area (General Protection Zones (GPZ) (i)–(iii), the Krill Research Zone (KRZ), and the Special Research Zone (SRZ); in dark grey shade). The blue polygon delineates the N70 (North of 70°S) management area, while the green polygon delineates the S70 (South of 70°S) management area; (C) Sampling locations of Macrourus caml and M. whitsoni from the commercial fishery between 2014–2021 (red circles) and Pinkerton et al. (2013) (blue circles); (D) Sampling locations of research trawl survey hauls in 2015 and 2019 (red circles) and the 2016 and 2019 winter surveys (blue circles). Also shown are the depth contours of 1000 m and 3000 m (all panels) and the CCAMLR small-scale research units (SSRUs) (panels C, D). Circles sizes are proportional to sample sizes, with the largest circle representing ~200 sampled individuals.
In 2016, CCAMLR adopted the Ross Sea region Marine Protected Area (RSrMPA) (CCAMLR, 2016), which came into effect at the start of the 2018 fishing season (fishing seasons are defined as starting in December, e.g., the 2018 fishing season started on 1st December 2017.) The RSrMPA has three zones (Figure 1): the General Protection Zone (GPZ, including GPZ(i), GPZ(ii) and GPZ(iii)) where fishing is prohibited; the Special Research Zone (SRZ) permits limited fishing for toothfish; and the Krill Research Zone (KRZ) permits limited fishing for krill (CCAMLR, 2016). As a result of the implementation of the RSrMPA, for management purposes the RSR is now divided into four management areas: the GPZ, SRZ, N70 (North of 70°S), and S70 (South of 70°S) management areas (Figure 1). The implementation of the RSrMPA has resulted in slight changes in the distribution of fishing effort, with fishing effort more concentrated in SSRUs 88.1H and 88.1I, on the RSR slope (Grüss et al., 2021; Moore and Parker, 2021).
Sample collection
Fisheries-dependent sampling
Data and samples of M. caml and M. whitsoni were collected by scientific observers onboard New Zealand-flagged commercial longline fishing vessels targeting D. mawsoni in the RSR (Figure 1) from the 2014 to 2021 fishing seasons, commencing when observer training in M. caml and M. whitsoni species identification was implemented. Observers sampled at least 10 macrourids per haul, where available (range = 1 to 30). For each fish sampled, pre-anal length (PAL) and total length (TL) were measured to the nearest cm, and whole weight (W) was measured to the nearest 10 g. Gonad stage was assessed using a 5-stage classification system as detailed in the CCAMLR SISO scientific observer’s manual (CCAMLR, 2020a), where 1 = Immature, 2 = Maturing virgin or resting (for females)/Developing or resting (for males), 3 = Developing (for females)/Developed (for males), 4 = Gravid (for females)/Ripe (for males), and 5 = Spent. Sagittal otoliths (hereafter simply referred to as otoliths) were extracted from at least five fish per set (where available), cleaned, dried and stored in paper envelopes for age estimation. These data were supplemented by samples collected during the 2016 and 2019 winter fishing surveys (n = 463 and 209 individuals, respectively) conducted using commercial longline fishing vessels (see Parker et al., 2019 for details), and by samples collected by the commercial longline fishery from the RSR during the 2011/12 fishing season (n = 568; see Pinkerton et al., 2013).
Fisheries-independent sampling
Additional samples of both species were collected during fisheries-independent research trawl surveys of the RV Tangaroa in 2015 and 2019 predominantly from the RSR slope (see O'Driscoll, 2015; O'Driscoll et al., 2019 for a full description of the gear, procedures used, and the outcomes of the surveys); the core area of the commercial fishery (Figure 1). During these surveys, all fish captured were measured to the nearest mm TL and PAL (2015 survey only) and weighed to at least the nearest 5 g. Sex and gonad stage were identified macroscopically, with gonad stage of these samples assessed using a 7-stage classification system, where 1 = Immature, 2 = Resting, 3 = Ripening, 4 = Ripe, 5 = Running ripe, 6 = Partially spent, and 7 = Spent. Otoliths were extracted from a subsample of fish from the 2019 survey, cleaned, dried and stored in paper envelopes. Inclusion of samples from the research surveys greatly increased sample sizes, particularly of individuals smaller than the lengths selected by the commercial fishing gear (see Results).
Age estimation
All otoliths from the RV Tangaroa surveys, and a subset of otoliths collected by scientific observers from the commercial fishery, were selected for ageing. A random length- and sex-stratified approach was used to select otoliths collected by observers, ensuring a good spread of samples across lengths, sexes, prospective age classes and core fishery areas.
Otolith processing and interpretation generally followed the thin-section approach outlined in Marriott et al. (2006). This approach was considered the most appropriate method for these species following a re-examination of both thin- and thick-section prepared otoliths (using terminology of Marriott et al., 2006). Otoliths selected for ageing were embedded in epoxy resin and sectioned transversely through the primordium perpendicular to the sulcus acusticus, using a low-speed saw with a single diamond-edged wafering blade (as opposed to the twin-blade approach used in Marriott et al., 2006). Otolith sections were cut at ~320–350 μm to optimize increment clarity. Sections were mounted on glass slides with a cover slip using clear two-part epoxy resin. Opaque increments on each otolith section were counted using a compound microscope and a combination of reflected and transmitted light. Increments were counted primarily along an axis from the primordium to the otolith margin on the ventral rim of the sulcus acusticus, following Marriott et al. (2006), as this was typically the axis with the most well-defined increments. Other axes were examined when opaque increments were unclear on this primary axis. Although the periodicity of increment deposition has not been validated for either M. caml or M. whitsoni, increment deposition has been demonstrated to occur annually in other grenadier species (e.g., Gordon and Swan, 1996). Thus, it was assumed that one opaque and one translucent zone represents a single year’s growth. Each otolith was read at least once by a single reader (PMM) experienced in age estimation of these species, and whose accuracy was tested against a reference set of M. caml and M. whitsoni otoliths. Age estimations from this reader were considered valid on the basis of a consistent agreement with those of the reference set (Supplementary Figure S1). A subset of otoliths (n = 104 for M. caml and n = 99 for M. whitsoni) were read twice to examine intra-reader variability. All otoliths were read without prior knowledge of the length or sex of the Individual or any prior age estimations. Results indicated close agreement between the primary and secondary reads within this subset (Supplementary Figure S2). Accordingly, the primary reads from all otoliths were used in the final analyses. Following Marriott et al. (2006), final ages were interpreted as the count of the opaque increments with no adjustment made for the birth date or month of capture, as all aged fish were collected within a short time span (i.e., between the start of December and early February) around the assumed peak spawning period (see Results).
Data analysis
Morphology, length and age distributions and sex ratios
Relationships between PAL and TL were determined for all samples combined and for each species separately using the equation TL = a + bPAL, where a is the intercept and b is the gradient of the slope. Relationships between TL and W were determined for all samples combined and for each species using the equation W = aTLb, where a is the intercept and b is the allometric coefficient. Values for a and b in the PAL-TL and TL-W relationships were estimated by fitting generalized linear models (GLMs) with a Gaussian error distribution to PAL and TL data, and log-transformed TL and weight data, respectively. Outliers were identified visually and removed prior to final modelling. Support for species-specific PAL-TL and TL-W relationships was evaluated by comparing the Akaike Information Criterion (AIC) for small sample sizes (i.e., AICc; Burnham and Anderson, 2002) of models fitted with and without an effect of species using the AICcmodavg package in R. The Akaike weight (w) of each model i was calculated to assess the weight of evidence for model i being the actual best model of those available (Burnham and Anderson, 2002). Models with AICc values within two of that of the best approximating model (lowest AICc) were considered to describe the data equally well (Burnham and Anderson, 2002). A similar process was conducted to evaluate sex-specific differences in PAL-TL and TL-W relationships for each species. Where necessary, the best approximating models were then used to predict missing values for TL and W.
Length (TL) and age frequency distributions were constructed for M. caml and M. whitsoni. Differences in length and age frequency distributions between species, and between sexes and collection methods (i.e., longline, including commercial longlines and longline surveys, vs. fisheries-independent research trawl surveys) for each species, were assessed using Kolmogorov-Smirnov (K-S) tests. Sex ratios for each species and collection method were plotted against TL to examine trends with size. Deviation from an expected species and sex ratios of 1:1 was examined using χ2 goodness-of-fit tests.
Growth
Growth of female and male M. caml and M. whitsoni was modelled using the von Bertalanffy growth function (VBGF) fitted by nonlinear least-squares regression of TL on age using the nls package in R. The VBGF was employed due to its near-ubiquitous use and to facilitate comparisons with other studies of grenadier growth. The form of the VBGF used to model length-at-age data was:
where Lt is the length-at-age t, L∞ is the hypothetical asymptotic length, k is the growth coefficient, and t0 is the hypothetical age at which fish would have zero length. Outliers were identified visually and removed prior to final modelling. Corresponding 95% confidence intervals for each parameter were derived by bootstrap resampling using 1000 iterations. Due to a lack of young fish, datasets for both species and sexes were supplemented by the inclusion of unsexed, immature juveniles of ≤ 2 years of age collected from the RSR in 2004 from Marriott et al. (2006). A second series of VBGFs models were run where t0 was constrained to zero. Due to differences in otolith preparation methods between studies, data from Pinkerton et al. (2013) were not included in the length-at-age analyses.
Formal support for sex-specific growth curves was evaluated for each species using the approach of Kimura (2008), whereby sex was added as a covariate on each of the three VBGF parameters of the unconstrained growth curve, such that:
where xi is a dummy variable for sex (F or M) for the ith fish, and β0 and β1 are covariates for each of the three VBGF parameters. To assess sex-specific differences in growth, a growth model fitted to all data without the effect of sex was compared with a growth model fitted to all data with sex as a covariate using AICc, as described for the PAL-TL and TL-W analyses.
Geographical variation in growth was explored using generalized additive mixed effect models (GAMMs; Lin and Zhang, 1999). Models examined the effects of latitude and depth on the residual length-at-age data from the sex-specific unconstrained VBGF models for each species. These terms were explored separately, as preliminary results found latitude and depth to be colinear (R2 = 0.66, p< 0.05). A random effect of fishing haul was added to each model, to account for the potential non-independence of fish in a given haul. GAMMs were fitted with a low number of basis functions (k = 4) and a gamma of two, to avoid over-fitting and preserve the ecological interpretability of functional relationships (Pèron et al., 2016). Significance of the latitude and depth terms was evaluated by comparing the AICs of GAMMs including and excluding these terms. Models with AIC values within two of that calculated for the best approximating model (lowest AIC) were considered to be equivalent (Burnham and Anderson, 2002; Williams et al., 2017).
Mortality
To ensure the otolith selection process did not bias mortality estimates, a resampling procedure was undertaken, whereby samples with age estimates from the commercial fishery (for each species separately) from each 2 cm TL bin were selected approximately proportionally to their occurrence in the raw length frequency distributions, with the caveat that the number of samples in each length bin (where available) was at least one. Samples were randomly selected within individual length bins. From these selections, the instantaneous rate of total mortality (Z) for M. caml and M. whitsoni was estimated using the generalized linear mixed model (GLMM) estimator of Millar (2015). For each species, random-intercept Poisson log-linear GLMMs were fitted separately for females and males, and both sexes combined, with the frequency of fish in each age class as the response variable, age as a fixed factor, and a random intercept, following the approach of Moore (2019; 2022). Models were fitted to data for age classes equal to or greater than the modal age. The negative of the maximum likelihood estimator of the slope coefficient from the model was taken as an estimate of Z.
Natural mortality (M) of M. caml and M. whitsoni was estimated using three methods. The first method consisted of calculating M as:
based on the theoretical assumption that 1% of individuals in the study region survive to the maximum observed age (Amax) (Quinn and Deriso, 1999). Maximum ages of 65 years for M. caml and 43 years for M. whitsoni were cautiously applied, reflecting the maximum age class observed for the species (see Results). In Methods 2 and 3, values of M were also calculated using the empirical mortality equations for fish of Hoenig (1983) and Then et al. (2015), respectively: (Hoenig, 1983)
Estimates of fishing mortality (F) were then calculated as the difference between Z and M, based on M estimates derived using the approach of Hoenig (1983).
Maturity and spawning season
Due to differences in gonad classification schemes between the fishery and survey data a common staging scheme was developed, where 1 = Immature, 2 = Resting, 3 = Developing/Ripening, 4 = Ripe/Running Ripe, and 5 = Partially spent/Spent. GLMs were used to estimate the lengths and ages at which 50% and 95% of female and male M. caml and M. whitsoni are mature (TL50 and TL95 and A50 and A95, respectively). Maturity state [i.e., ‘immature’ (fish with gonads in stage 1) or ‘mature’ (fish with gonads in stages 3–5)], as determined from macroscopic staging data from research activities and experienced observers, was treated as a binomial response variable and modelled as a function of either TL or age with a logit link. Following Pinkerton et al. (2013), fish with gonads in stage 2 were excluded from the maturity analyses, as it is unclear from macroscopic inspection whether these individuals are immature and developing towards sexual maturity, or are already mature and resting. The unsexed, immature juveniles used in the age and growth analyses were similarly included in the maturity analyses to improve the fits of the logistic functions for each species. Data from Pinkerton et al. (2013) were included in the length-at-maturity analyses, but were excluded from age-based analyses due to differences in ageing approaches between studies as outlined above.
Species-specific monthly trends in the proportion of mature females in reproductive stages 2–5 and the gonadosomatic index (GSI: gonad weight/W*100) of females across sampled months were examined to determine the seasonality of spawning of M. caml and M. whitsoni in the RSR. For stage 2 fish, only those individuals whose length was equal to or greater than the length at 50% maturity were included in the analyses, as there is a high probability that these fish were mature with gonads in resting stage (as opposed to being immature, as discussed above). GSI analyses were limited to those individuals with gonads of stages 3–5, as weights of gonads in stages ≤2 collected at sea are often near the resolution of the weighing balances used (SJP pers. obs.). GSI and gonad staging data were grouped by calendar month, pooling all sampling years, as sample numbers in individual years were generally too small to detect reproductive patterns.
Unless stated, to prevent potential biases resulting from misidentification of species, only those individuals collected by scientific observers that were predicted to be correctly identified to species level in the first instance using the approach of Moore et al. (2022), obtained from research surveys (including research trawl and winter surveys), or identified during the study of Pinkerton et al. (2013), were used in biological analyses. While this reduce sample sizes in the analyses, it yielded a dataset for which there was a high degree of confidence in the species assignments for these individuals. All biological analyses were conducted in R version 4.0.5 (R Core Team, 2021). The nls function in the stats package was used for non-linear modelling of the extended VBGF. All GLMs were performed using the glm function in the stats package. GAMMs were fitted using the gam function in the mgcv package (Wood, 2017).
Results
Morphology, length and age distributions and sex ratios
There was evidence for differences in the PAL-TL and TL-W relationships between M. caml and M. whitsoni, as well as between sexes within each species, with models including terms for species or sex having lower AICc values and higher w values than models excluding terms for species or sex (Table 1, see also Figure 2). The estimated parameters of the TL-W relationship indicated that growth of female M. caml and M. whitsoni is positively allometric (b > 3), whereby their weight increases disproportionately to length, while growth of males of both species is negatively allometric (b< 3) (Table 2).
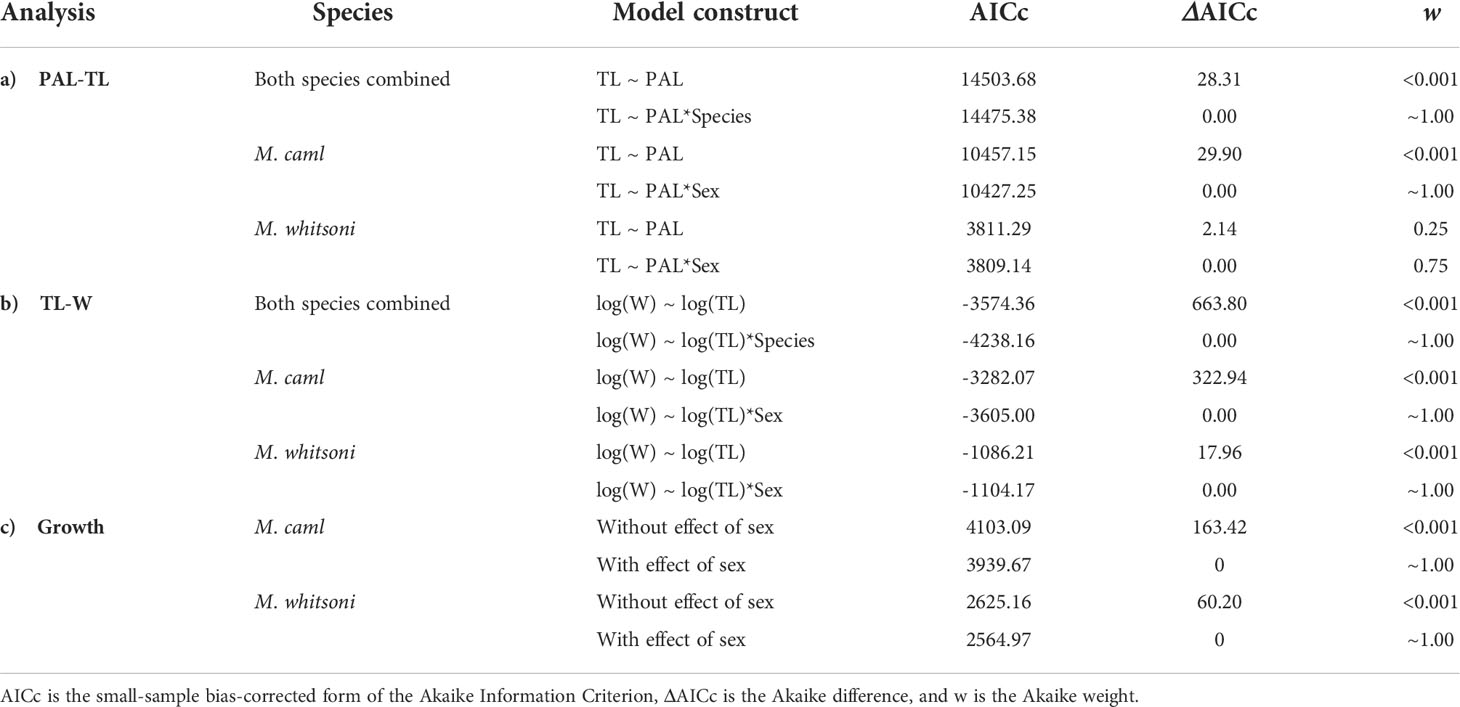
Table 1 Summary of fits of models used to examine the effects of species and/or sex on a) the relationship between pre-anal length (PAL, cm) and total length (TL, cm), b) TL and weight (W, kg) and c) growth for Macrourus caml and M. whitsoni in the Ross Sea region.
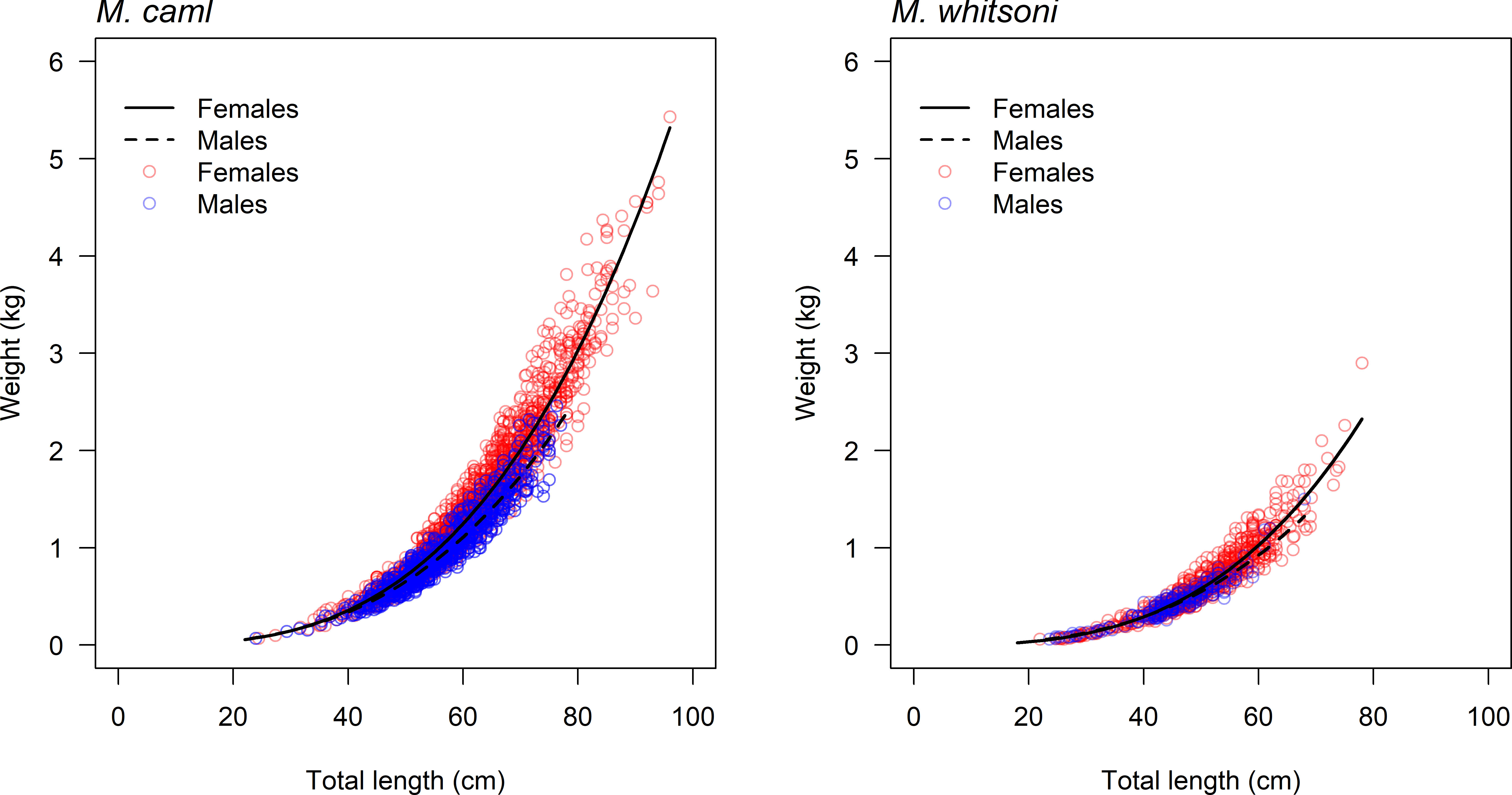
Figure 2 Weight-at-length data and fitted length-weight curves for female and male Macrourus caml (left) and M. whitsoni (right) in the Ross Sea region.
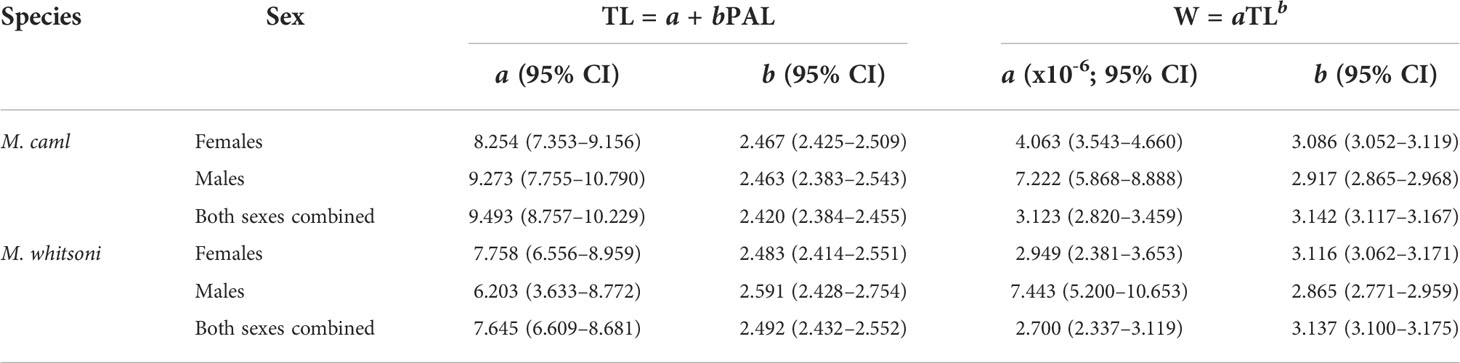
Table 2 Summary of coefficients (and 95% confidence intervals; CI) of the relationship between pre-anal length (PAL, cm) and total length (TL, cm), and TL and weight (W, kg), for Macrourus caml and M. whitsoni in the Ross Sea region.
Macrourus caml was significantly more prevalent (χ2 goodness-of-fit tests, p< 0.001) than M. whitsoni in both the longline- and research trawl survey-derived samples, with 2366 M. caml and 1128 M. whitsoni sampled from longline catches, and 767 M. caml and 187 M. whitsoni sampled from the research trawl surveys (Figure 3). Significant differences in both length (D = 0.41, p< 0.01) and age (D = 0.22, p< 0.01) frequency distributions were observed between M. caml and M. whitsoni from combined longline and research trawl survey data (Supplementary Table S1), with sampled M. caml being on average larger and older than M. whitsoni (Figures 3, 4). Significant differences in length frequency distributions were also observed between sexes and collection method (longline vs. research trawl) for each species, and between collection method for each species-sex combination (all p< 0.01; Supplementary Table S1). The largest female M. caml was 97.0 cm TL (= 36.0 cm PAL), while the largest male M. caml was 77.0 cm TL (= 27.5 cm PAL). The largest female M. whitsoni was 78.0 cm TL (= 28.3 cm PAL), while the largest male M. whitsoni was 68.0 cm TL (= 23.9 cm PAL) (Figure 3). Age frequency distributions differed significantly between collection methods for both M. caml and M. whitsoni, although showed no difference between sexes for either species (Supplementary Table S1). The research trawl surveys generally caught a larger proportion of smaller, younger individuals (< 40 cm TL,< 10 years) of both species compared to longlines (Figures 3, 4). The oldest female M. caml was 65 years, while the oldest male M. caml was 53 years. The oldest female M. whitsoni was 43 years, while the oldest male M. whitsoni was 41 years (Figure 4).
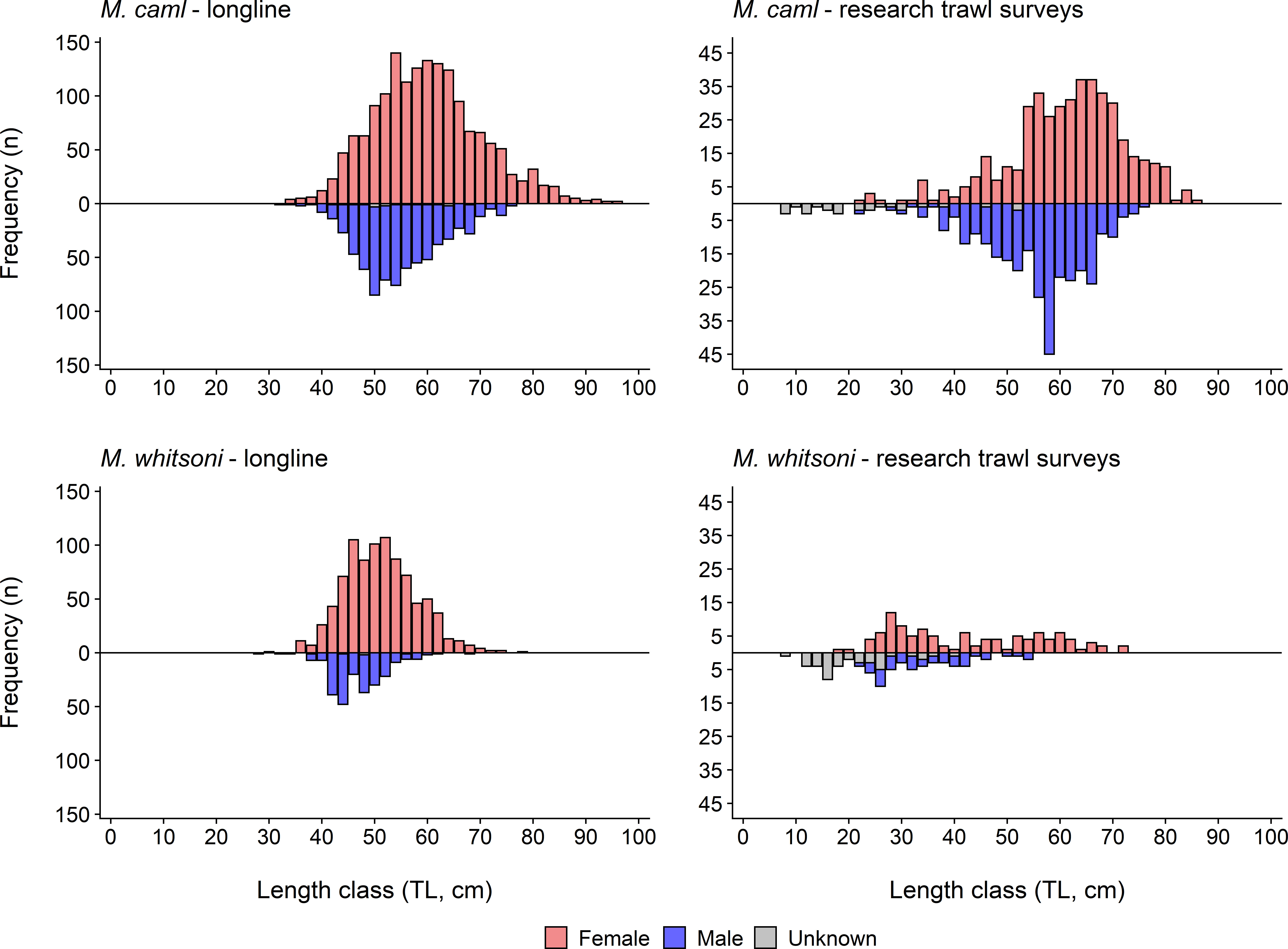
Figure 3 Length frequency distributions for female, male and unsexed Macrourus caml (top row) and M. whitsoni (bottom row) sampled from the Ross Sea region by longline (i.e., the commercial fishery and the 2016 and 2019 winter surveys) (left column), and fisheries-independent research trawl surveys in 2015 and 2019 (right column). Note the different y-axis scales between longline and research trawl plots.
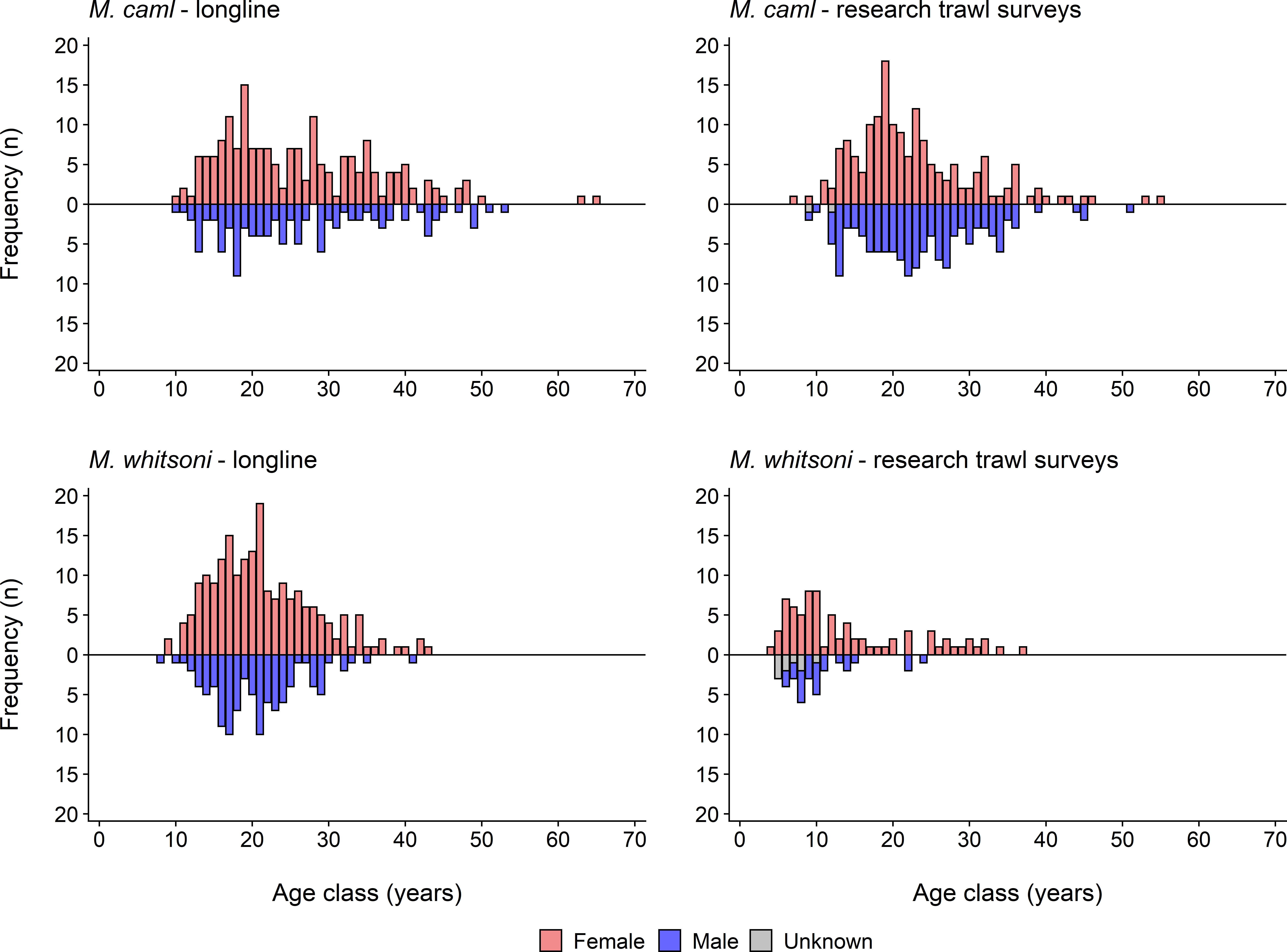
Figure 4 Age frequency distributions for female, male and unsexed Macrourus caml (top row) and M. whitsoni (bottom row) sampled from the Ross Sea region by the commercial longline fishery (left column), and fisheries-independent research trawl surveys in 2019 (right column).
Sampled populations were significantly female-biased for both M. caml (χ2 = 383.92, p< 0.001) and M. whitsoni (χ2 = 410.46, p< 0.001) (Figure 3), particularly at greater length classes (Figure 5). The sex ratios of M. caml and M. whitsoni from the fishery-sourced samples were 2.38 females to 1 male, and 3.82 females to 1 male, respectively. Sex ratios from the research surveys were 1.43 females to 1 male for M. caml, and 2.59 females to 1 male for M. whitsoni.
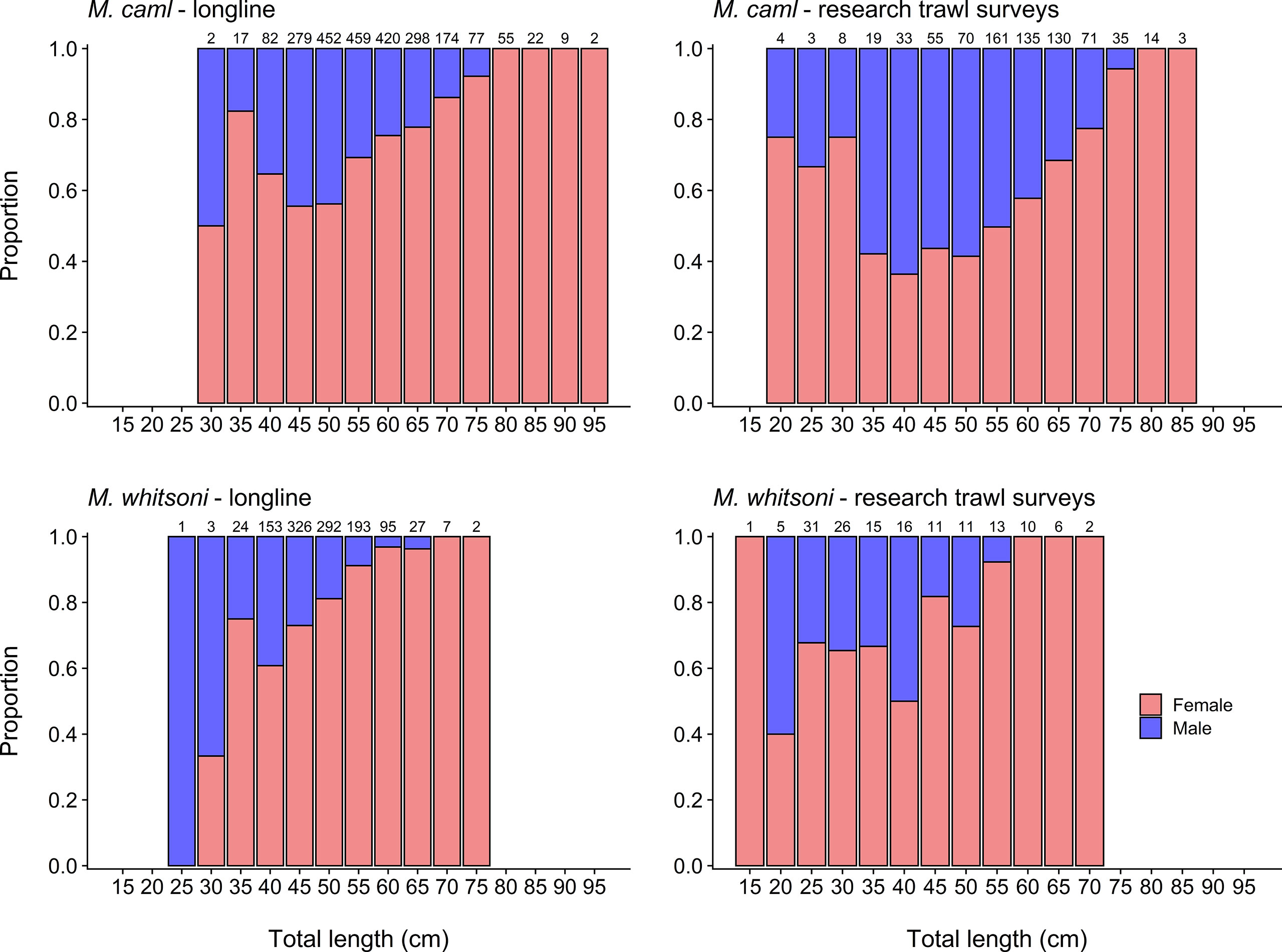
Figure 5 Proportion of female and male Macrourus caml (top row) and M. whitsoni (bottom row) by 5 cm total length (TL) class sampled from the Ross Sea region by longline (i.e., the commercial fishery and the 2016 and 2019 winter surveys) (left column) and fisheries-independent research trawl surveys in 2015 and 2019 (right column). Sample sizes of each length class are indicated above each bar.
Growth
Sex-specific growth was supported for both species with differences of 163.42 and 60.20 between the AICc from the growth models fitted with and without sex as a covariate on each of the three VBGF parameters for M. caml and M. whitsoni, respectively (Table 1). For both species, females of a given age were larger than males, particularly for ages of 10 years and older (Figure 6). The predicted mean asymptotic length of females was approximately 17 cm and 12 cm larger than males for M. caml and M . whitsoni, respectively (Table 3). For a given sex, M. caml attained a greater length-at-age than M. whitsoni, with the predicted mean asymptotic length of female and male M. caml approximately 22 cm and 16 cm larger than the predicted mean asymptotic length for female and male M. whitsoni, respectively (Table 3; Figure 6; see also Supplementary Table S2 for sex-aggregated VBGF parameters).
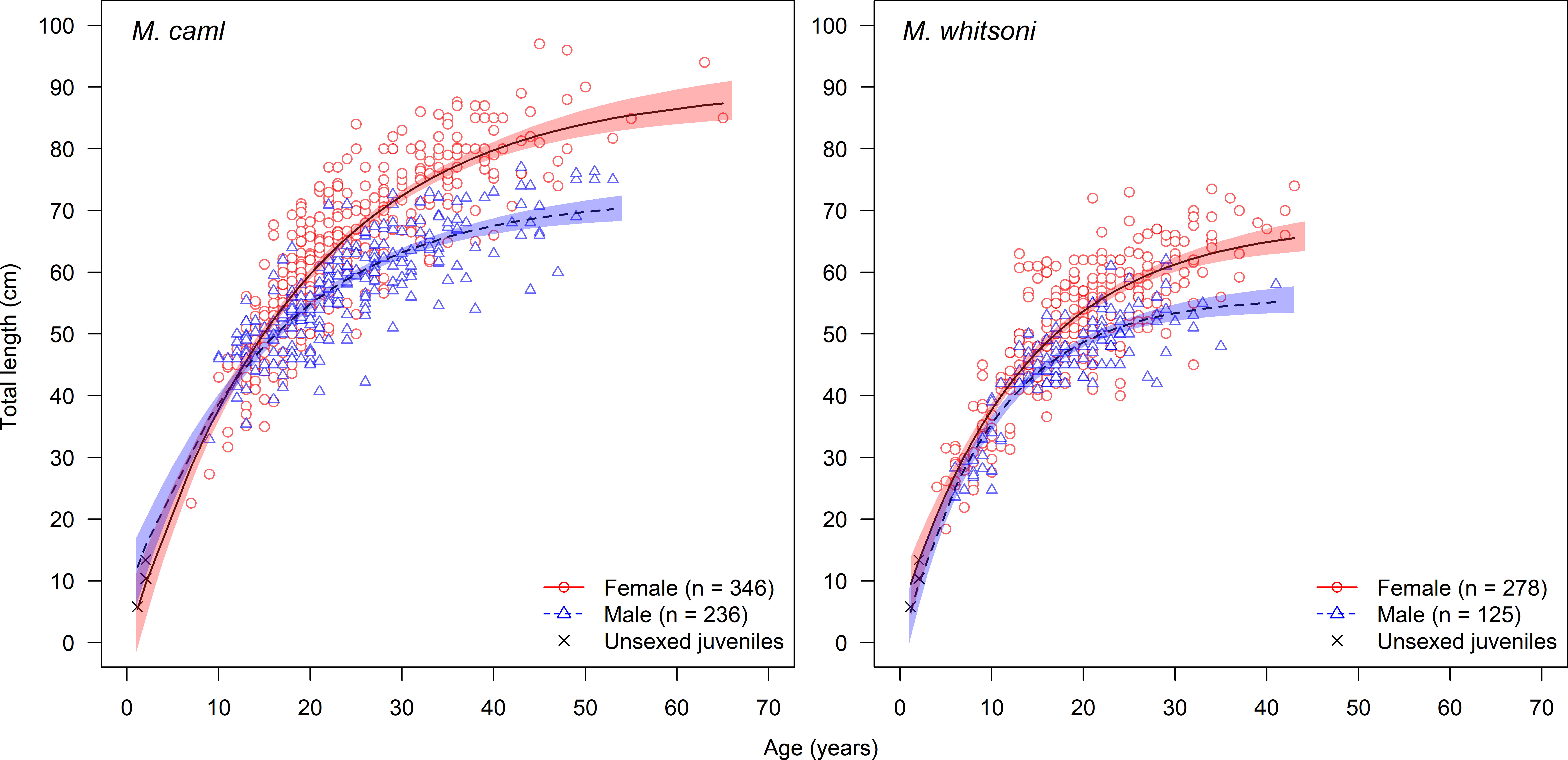
Figure 6 Length-at-age data and fitted unconstrained von Bertalanffy growth curves for female and male Macrourus caml (left) and M. whitsoni (right) in the Ross Sea region. Shaded areas indicate 95% confidence intervals. Total sample sizes for each sex are indicated in parentheses.
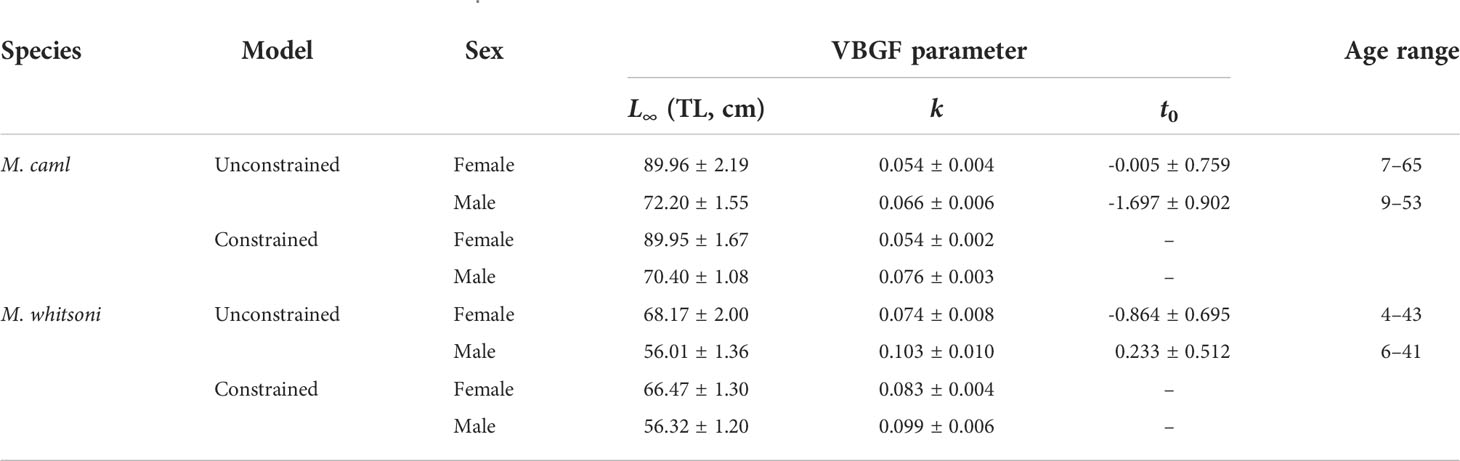
Table 3 von Bertalanffy growth function (VBGF) parameter estimates ± standard error for unconstrained and constrained (t0 = 0) models and observed age ranges for Macrourus caml and M. whitsoni in the Ross Sea region.
A significant effect of latitude on residual length-at-age was evident for female M. caml, with smaller fish than predicted at more northerly locations (Figure 7; Table 4). There was no effect of latitude on the residual length-at-age for male M. caml, or for either sex of M. whitsoni, with models including these terms having AIC values within 2 of models excluding these terms (Table 4). While no effect of depth on residual length-at-age was evident for M. caml, there was a strong positive relationship between the residual length-at-age and depth for both female and male M. whitsoni, with larger individuals observed than predicted by the model at greater depths (Figure 7; Table 4).
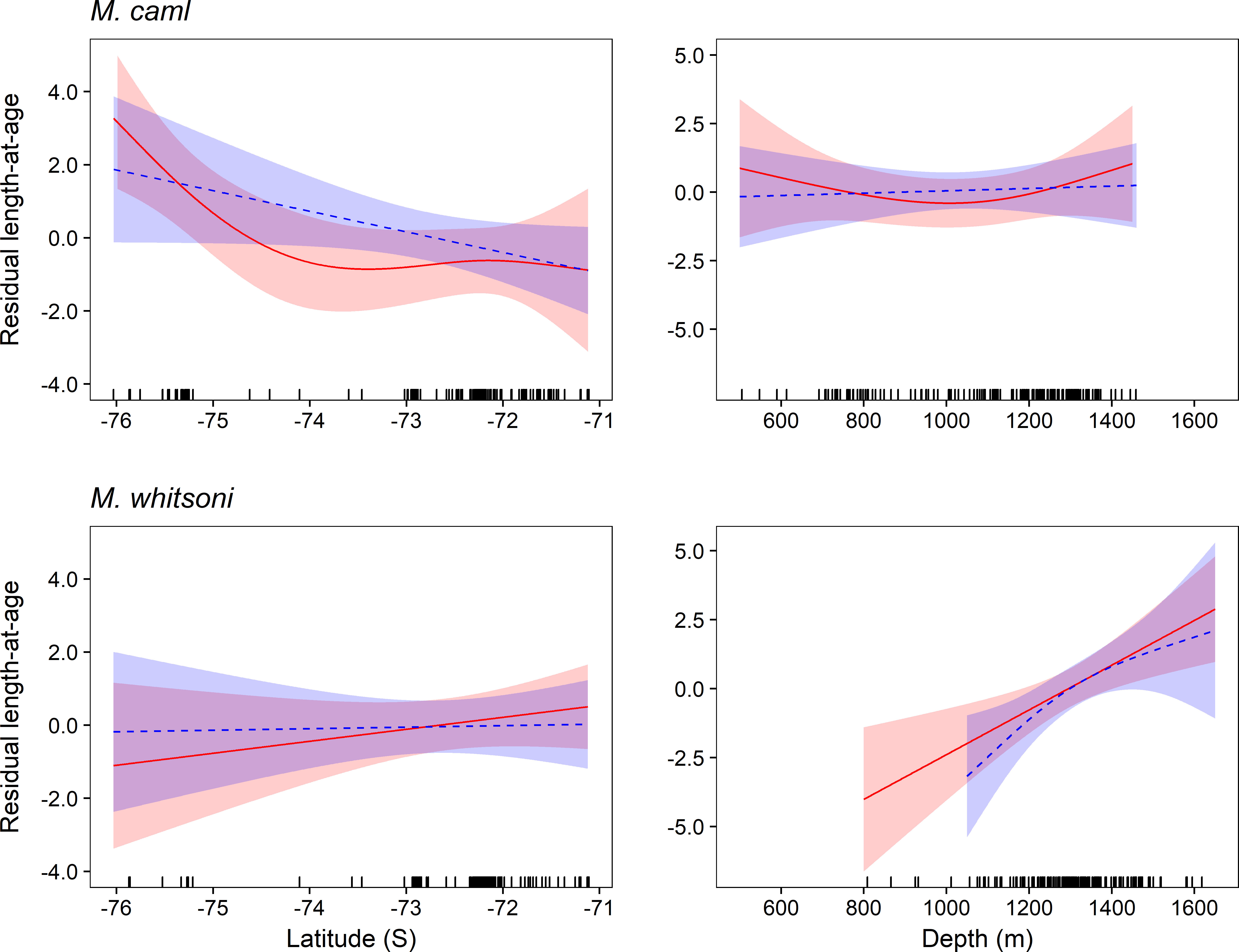
Figure 7 Sex-specific trends in residual length-at-age for Macrourus caml (top row) and M. whitsoni (bottom row) in the Ross Sea region by latitude (left column) and depth (right column).
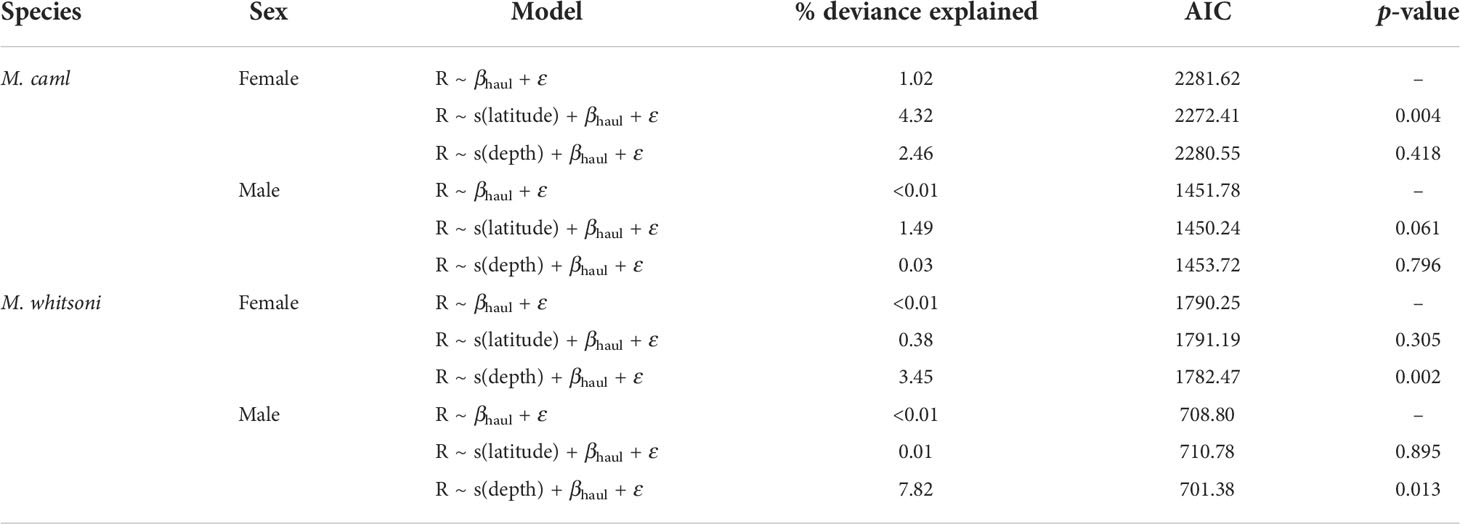
Table 4 Parameters and fit of generalized additive mixed effect models exploring the effect of latitude and depth on residual length-at-age for Macrourus caml and M. whitsoni in the Ross Sea region.
Mortality
The estimated instantaneous rate of total mortality (Z) was higher for M. whitsoni than M. caml, and similar between sexes for both species (Table 5). Estimates of natural mortality were lower for M. caml than M. whitsoni for each of the three methods used (Table 5). The M estimates resulting from the equations of Quinn and Deriso (1999) and Then et al. (2015) occasionally (in the case of Quinn and Deriso, 1999), or in all instances [in the case of Then et al. (2015)] yielded values of F which were below zero, and therefore, were not considered biologically plausible. Based on M estimates from the Hoenig (1983) equation, estimates of F were low for both species, and estimated as 0.024 and 0.046 for M. caml and M. whitsoni, respectively (Table 5).
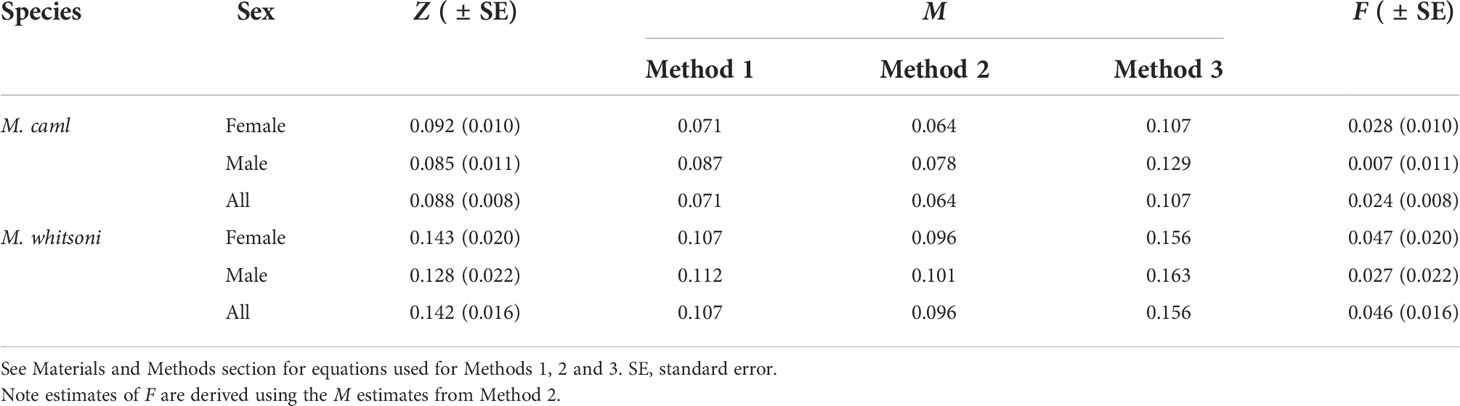
Table 5 Estimates of total mortality (Z), natural mortality (M), and fishing mortality (F) of Macrourus caml and M. whitsoni in the Ross Sea region.
Maturity and spawning season
The length and age at which 50% of individuals reached maturity was slightly larger for females than males for both species, and slightly larger for female M. whitsoni than female M. caml (Table 6 and Figure 8). For M. caml, the estimated length and age at which 50% of females reached maturity was 49.8 cm TL (= 16.8 cm PAL) and 15.9 years, while 50% of males were estimated to be mature at 38.0 cm TL (= 11.7 cm PAL) and 4.8 years (although there was a general lack of young immature males to inform the lower end of the assumed logistic relationship). The estimated length and age at which 50% of female M. whitsoni reached maturity was 54.7 cm TL (= 18.9 cm PAL) and 22.7 years, while 50% of male M. whitsoni were estimated to be mature at 44.1 cm TL (= 14.6 cm PAL) and 17.0 years (Table 6).
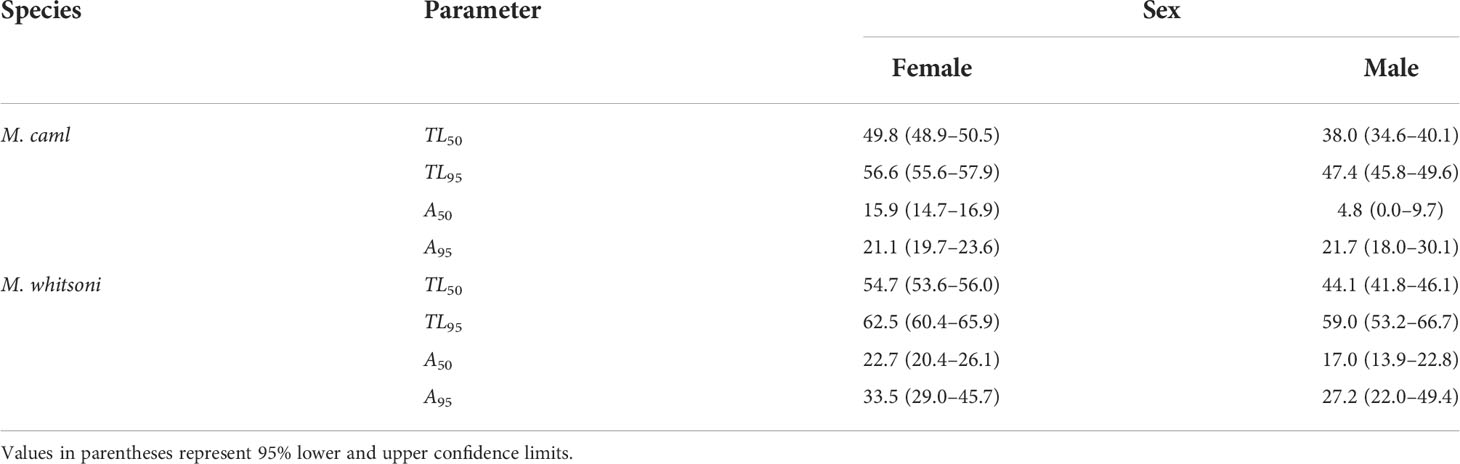
Table 6 Total lengths (TL, cm) and ages (A) by which 50% and 95% (TL50, TL95, A50, A95) of female and male Macrourus caml and M. whitsoni in the Ross Sea region have attained sexual maturity.
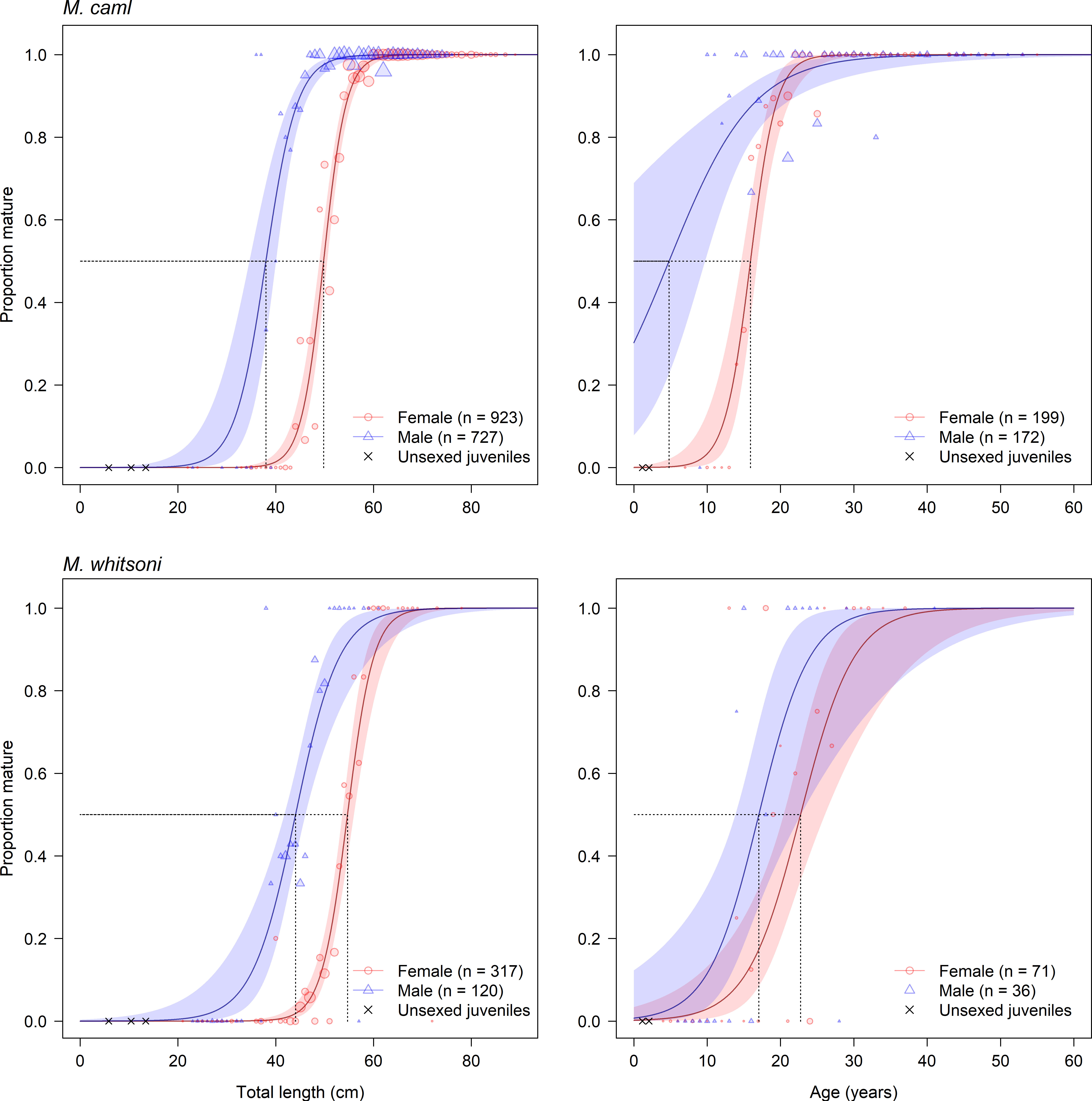
Figure 8 Predicted proportion of mature male and female Macrourus caml (top row) and M. whitsoni (bottom row) by length (left column) and age (right column) in the Ross Sea region. Shaded areas indicate 95% confidence intervals. Points represent the proportion of mature fish per 2 cm length class or age class, and have been scaled relative to sample sizes (largest point = 44 individuals, smallest point = one individual). Total sample sizes for each sex are indicated in parentheses.
Monthly trends in GSI and staging data suggested potential similarities in the timing of spawning between M. caml and M. whitsoni (Figure 9). For M. caml, mean GSI values were highest between December and February, peaking in late austral summer. Fish in ripe/running ripe condition were observed in most months, with the highest proportion of these individuals observed in January (Figure 9), and were observed from both northern seamounts and the RSR slope (Supplementary Figure S3). Spent M. caml were observed only in January (Figure 9), and only from the RSR slope (Supplementary Figure S3). Mean GSI values for M. whitsoni were generally low (<6) across all months, but were highest in December. Few ripe/running ripe or spent M. whitsoni were observed. Ripe/running ripe M. whitsoni were observed in January and February, from the RSR slope, while spent M. whitsoni were observed only in December and January (Figure 9), both from northern seamounts and the RSR slope (Supplementary Figure S3).
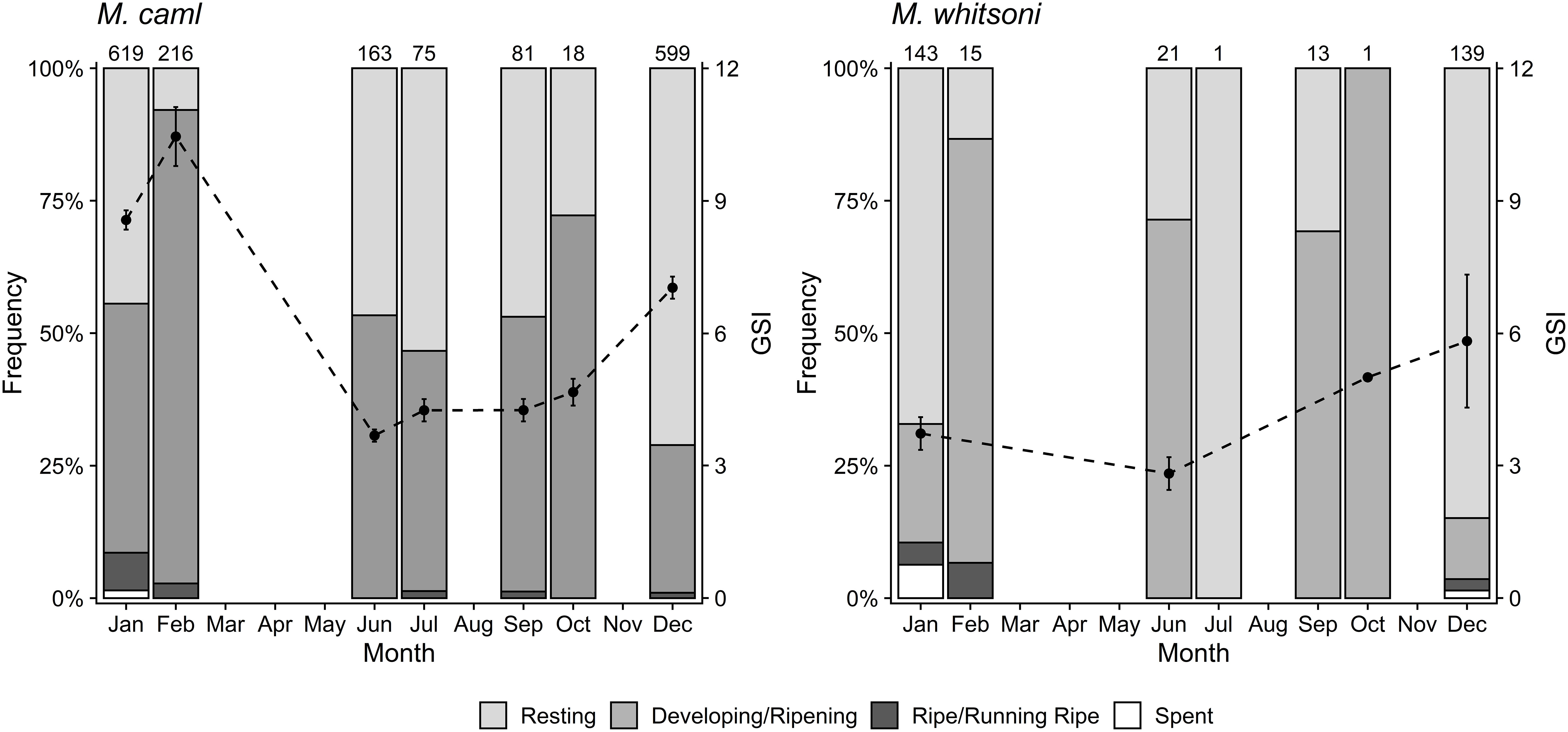
Figure 9 The percentage of females with gonads at stages 2–5 and mean monthly gonadosomatic indices (GSI ± standard error) of gonads in stages 3–5 for Macrourus caml (left) and M. whitsoni (right) in the Ross Sea region. Numbers above sampling bins represent sample sizes for staging data in each month.
Discussion
Results from this study support preliminary work by Pinkerton et al. (2013) that demonstrate significant variation in life history between M. caml and M. whitsoni, and between sexes for each species. Reaching at least 97 cm TL (= 36.0 cm PAL) and at least 65 years of age, M. caml is a large-bodied species with extended longevity and slow growth to exploited size classes. By comparison, M. whitsoni attain shorter maximum lengths and ages [~78 cm TL (= 28.3 cm PAL) and 43 years, respectively, in our study], but mature at larger lengths and later in life. Estimates of natural and fishing mortality were low for both species, although slightly greater for M. whitsoni than M. caml. Sex ratios of M. caml and M. whitsoni from both longline and research trawl surveys were significantly female biased, despite both species being gonochoristic. The observed differences in life history between these morphologically-similar species demonstrate the importance of accurate species identification, provide the foundation for the development of the first species-specific risk assessment models for M. caml and M. whitsoni, and highlight the need to consider species- and sex-specific demographic parameters in future assessments for these species.
In the current study, females of M. caml and M. whitsoni were found to reach a larger length-at-age than males. Similar results have been observed previously for these species in the RSR (Pinkerton et al., 2013), as well as for other grenadier species elsewhere [e.g., Macrourus carinatus (Lee et al., 2019), M. berglax (Orlov et al., 2018)], and has been hypothesized to be a strategy to increase female reproductive output in depauperate deep-sea environments (Laptikhovsky, 2005). Under this hypothesis, the larger length-at-age of females potentially reflects a trade-off between reproductive potential and mortality. The energetic costs associated with egg production are higher than for sperm (Hayward and Gillooly, 2011), therefore, for females to reach a larger length-at-age than males, they would likely require a higher food intake and/or greater foraging activity, exposing them to a greater mortality risk due to increased encounters with predators. Improved understanding of the species- and sex-specific feeding rates, diets, and trophic status of M. caml and M. whitsoni are required to explore this hypothesis.
The longevity of M. caml (65 years) observed in the current study is similar to that previously reported for the species by Pinkerton et al. (2013) (62 years), and the age ranges estimated for other large grenadiers. Lee et al. (2019) reported a maximum age of 53 years for both females and males of the closely-related M. carinatus from the Falkland Islands in the south-west Atlantic Ocean. Radiometric validated age for the Pacific grenadier (Coryphaenoides acrolepis) confirmed longevity of at least 56 years and possibly as great as 73 years for individuals off the west coast of the United States (Andrews et al., 1999). Roundnose grenadier (C. rupestris) were reported to live for up to 60 years in the north Atlantic (Bergstad, 1990; Kelly et al., 1997), while giant grenadier (Albatrossia pectoralis) were reported to live for up to 58 years in the north Pacific (Rodgveller et al., 2010).
The positive association observed between residual length-at-age and increasing depth for both female and male M. whitsoni potentially reflects differing environmental productivity with depth, or decreased inter-specific competition for prey items. While M. caml and M. whitsoni exhibit overlapping depth distributions, a greater relative proportion of M. caml are encountered in shallower waters, with an increasing relative proportion of M. whitsoni in deeper waters (Pinkerton et al., 2013). While species-specific studies into the diets of M. caml and M. whitsoni are limited, both species are considered to be euryphagous predators and scavengers, likely feeding on a broad range of invertebrates and small fishes (Pinkerton et al., 2012; Münster et al., 2016). As such, some overlap in prey items between the two species is likely. Accordingly, individual M. whitsoni at greater depths may be subject to reduced dietary competition from the larger M. caml than individuals in shallower environments, allowing them to reach a greater length-at-age than expected. Further research is needed to understand the diets of M. caml and M. whitsoni, and how patterns in species biology, including length, age, and length-at-age, vary across both time and space.
It should be noted that annual increment deposition in otoliths has not been previously directly validated for either M. caml or M. whitsoni, and thus the age estimates and associated age-based parameter estimates presented here should be treated with a degree of caution. However, indirect validation approaches, such as examination of patterns in otolith edge material over time, have demonstrated that increment deposition likely occurs annually for a range of grenadier species (Gordon and Swan, 1996), supporting this assumption for M. caml and M. whitsoni. The high rates of post-capture mortality of both species are likely to limit the success of conventional chemical-based tag-recapture approaches to age validation. The application of radiometric analysis to otolith material may provide an alternative means of validating age estimates for these species, with this approach having been used successfully on other grenadier species (e.g., C. acrolepis, Andrews et al., 1999).
Parameter estimates of the VBGF, and particularly k and t0, can be sensitive to the distribution of age data at the lower end of the size range (Ferreira and Russ, 1994). For both M. caml and M. whitsoni, we were generally lacking sex and age information from small individuals (~< 20 cm TL), with individuals of both species entering the commercial longline fishery from approximately 30 cm TL. While small fish were encountered during the research trawl surveys, sex information and otoliths were not available for these individuals. The collection, speciation, sex determination, and ageing of small individuals from future trawl surveys or sampled from toothfish stomachs would greatly improve the reliability of growth parameter estimates for both species.
The maturity estimates presented here are largely similar to previous estimates developed for these species. Using a subset of the data used here, Pinkerton et al. (2013) estimated the length at 50% female maturity to be 46 cm TL (16 cm PAL) for M. caml and 52 cm TL (18 cm PAL) for M. whitsoni. Based on macroscopic assessments of gonads, length at 50% maturity was estimated to be around 18 cm PAL for female M. caml and 22 cm PAL for both female and male M. whitsoni for fish sampled between 63°S and 67°S in East Antarctica (Delegations of France and Australia, 2020). The slightly larger lengths at 50% maturity estimated for both species in East Antarctic likely reflects differing productivities between regions, potentially mediated by differences in water temperature, although differences in maturity stage determination from macroscopic observations cannot be ruled out from available data. Additional sampling of small immature individuals, confirmation of maturity schedules via histology and further comparative work into how the biology of both M. caml and M. whitsoni varies across their distribution would be of considerable benefit in managing fisheries that interact with these species.
Information on the spawning season for M. caml and M. whitsoni is limited across their geographical range, and the current study only partially elucidated this aspect of the species’ life history. This was in part due to the short temporal span of the toothfish fishery in the RSR, which presently operates from 1st December to ca. mid-January in the following year (Grüss et al., 2021), limiting the bulk of sample collection opportunities to these months. Macroscopic gonad staging data suggested the likely occurrence of a late-spring to summer peak spawning period for both species. Pinkerton et al. (2013) concluded that the spawning period for both species may be quite long, commencing in late spring and extending beyond the end of the austral summer. For other grenadier species, protracted spawning seasons have been documented, and females of several species have been reported with ripe oocytes nearly year-round (Massutí et al., 1995; Coggan et al., 1999; Morley et al., 2004). Further collection of samples during other times of the year, combined with histology, is required to further elucidate the spawning time and behavior of these species.
Management of M. caml and M. whitsoni catches in Antarctic waters is enacted inter alia through the setting of regional catch limits (e.g., Conservation Measure 41-09; CCAMLR, 2020b). Catch limits of grenadiers in the RSR were developed before the identification of M. caml and were thus established using biological parameters derived from an amalgamated set of samples of both species. The differences in productivity and longevity of the two species observed in the current study suggests the two species likely respond differently to exploitation, and that catch limits should be re-evaluated to incorporate these differences. The implementation of species-specific catch limits, however, is likely to be impractical, owing to the species' morphological similarity and broadly overlapping distributions. As an alternate approach, the generation of area-specific catch limits weighted to the productivity of the dominant species present in the area may be feasible, but would require an improved understanding of the distribution and relative catch rates of the two species.
Concluding remarks
Macrourus caml and M. whitsoni were found to be long-lived and slow-growing, with late maturity and low rates of natural mortality. These traits, characteristic of many deep-sea fish species, indicate that both M. caml and M. whitsoni are vulnerable to exploitation, albeit to differing degrees. Furthermore, the fact that both species are main prey of the target species of toothfish fisheries in the Southern Ocean implies that both will also be subject to predation release. This complexity emphasizes the need to account for differing productivity between the two species in developing a precautionary approach to management. Of particular importance to the development of such an approach is an improved understanding of the regional stock structure of both M. caml and M. whitsoni to define the appropriate scale of assessment and management of these species, as well as improved species identification in catch reporting. Combined with the biological parameters derived in the present study, this information will provide essential inputs for improved estimates of abundance, population status and predation release.
Data availability statement
The fishery and observer data used here are confidential and not publicly available. The biological datasets generated in this study are available on reasonable request to the corresponding author.
Ethics statement
All fish used in these analyses were caught via commercial fishing operations or from research surveys. Research survey-sampled fish were collected by research trawl under permits issued by the New Zealand Ministry for Primary Industries to NIWA pursuant to the Antarctic Marine Living Resources (AMLR) Act 1981. Special permits were granted under Section 5 of the AMLR Act to authorize taking aquatic life for the purpose of investigative research. Special permits by research voyage: AMLR/14/04/Tangaroa/ZMFR (Voyage TAN1502); AMLR/18/R05/Tangaroa/ZMFR (voyage TAN1901). There were no ethical considerations. No fish were CITES listed.
Author contributions
BRM: Conceptualization, Methodology, Investigation, Data curation, Formal analysis, Writing – original draft preparation, and Visualization. PMM: Laboratory work, Writing – review and editing. CS: Laboratory work. SJP and MHP: Conceptualization, Methodology, Writing – review and editing, Project administration and Funding acquisition. All authors contributed to the article and approved the submitted version.
Funding
This project was funded by the New Zealand Ministry for Primary Industries under project ANT2019/01 and the New Zealand Ministry of Business, Innovation and Employment through the Endeavour Fund Ross Sea Research and Monitoring Programme (Ross-RAMP; MBIE contract C01X1710).
Acknowledgments
The authors thank the scientific observers and the staff onboard the New Zealand commercial fishing vessels, and the staff onboard the RV Tangaroa, who collected data and samples. Jennifer Devine (NIWA), Arnaud Grüss (NIWA) and the CCAMLR Secretariat assisted with the initial extraction of the commercial fishery data. We also thank members of the New Zealand Antarctic Working Group for helpful discussions and input into this paper. Arnaud Grüss, Nathan Walker (New Zealand Ministry for Primary Industries) and three reviewers provided constructive feedback on earlier drafts of the manuscript.
Conflict of interest
The authors declare that the research was conducted in the absence of any commercial or financial relationships that could be construed as a potential conflict of interest.
Publisher’s note
All claims expressed in this article are solely those of the authors and do not necessarily represent those of their affiliated organizations, or those of the publisher, the editors and the reviewers. Any product that may be evaluated in this article, or claim that may be made by its manufacturer, is not guaranteed or endorsed by the publisher.
Supplementary material
The Supplementary Material for this article can be found online at: https://www.frontiersin.org/articles/10.3389/fmars.2022.968848/full#supplementary-material
References
Andrews A. H., Cailliet G. M., Coale K. H. (1999). Age and growth of the Pacific grenadier (Coryphaenoides acrolepis) with age estimate validation using an improved radiometric ageing technique. Can. J. Fish. Aquat. Sci. 56, 1339–1350. doi: 10.1139/f99-054
Bergstad O. A. (1990). Distribution, population structure, growth and reproduction of the roundnose grenadier Coryphaenoides rupestris (Pisces: Macrouridae) in the deep waters of the Skagerrak. Mar. Biol. 107, 25–39. doi: 10.1007/BF01313239
Burnham K. P., Anderson D. R. (2002). Model selection and multimodel inference: A practical information-theoretic approach, second edition (New York: Springer).
CCAMLR (2016). Ross Sea Region marine protected area, Conservation Measure 91–05 (2016) (Hobart, Australia: CCAMLR).
CCAMLR (2020a). Scheme of international scientific observation scientific observer's manual finfish fisheries version 2020 (Hobart, Australia: CCAMLR).
CCAMLR (2020b). Limits on the exploratory fishery for Dissostichus mawsoni in statistical subarea 88.1 in the 2020/21 season, Conservation Measure 41-09 (2020) (Hobart, Australia: CCAMLR).
Coggan R. A., Gordon J. D. M., Merrett N. R. (1999). Aspects of the biology of Nezumia aequalis from the continental slope west of the British Isles. J. Fish Biol. 54, 152–170. doi: 10.1111/j.1095-8649.1999.tb00619.x
Cohen D. M., Inada T., Iwamoto T., Scialabba N. (1990). FAO species catalogue. vol 10. Gadiform fishes of the world (Order Gadiformes). An annotated and illustrated catalogue of cods, grenadiers and other gadiform fishes known to date (Rome: FAO).
Delegations of France and Australia (2020). Report on fish by-catch in the exploratory toothfish fishery in Divisions 58.4.1 and 58.4.2 between 2014 and 2020, SC-CAMLR-39/BG/44 (Hobart, Australia: CCAMLR), 33.
Ferreira B. P., Russ G. R. (1994). Age validation and estimation of growth rate in the coral trout, Plectropomus leopardus (Lacepede 1802) from Lizard Island, Northern Great Barrier Reef. Fish Bull. 92, 46–57.
Gon O., Miya T., Mcmillan P., Leslie R. (2021). The distribution of four species of the genus Macrourus (Gadiformes: Macrouridae) from the Southern Ocean based on samples from the toothfish longline fishery. Zootaxa 4903, 105–116. doi: 10.11646/zootaxa.4903.1.6
Gordon J. D. M., Swan S. C. (1996). Validation of age readings from otoliths of juvenile roundnose grenadier, Coryphaenoides rupestris, a deep-water macrourid fish. J. Fish Biol. 49, 289–297. doi: 10.1111/j.1095-8649.1996.tb06082.x
Grüss A., Devine J., Parker S. (2021). Characterisation of the toothfish fishery in the Ross Sea region through 2020/21. WG-FSA-2021/24 (Hobart, Australia: CCAMLR).
Hanchet S., Dunn A., Parker S., Horn P., Stevens D., Mormede S. (2015). The Antarctic toothfish (Dissostichus mawsoni): biology, ecology, and life history in the Ross Sea region. Hydrobiologia 761, 397–414. doi: 10.1007/s10750-015-2435-6
Hanchet S., O'Driscoll R., Ballara S., Dunn A. (2008). “Grenadier bycatch in the toothfish longline fishery in the Ross Sea, Antarctica”, in Grenadiers of the world oceans: Biology, stock assessment, and fisheries. Eds. Orlov A. M., Iwamoto T. (Maryland: American Fisheries Society), 451–462.
Hayward A., Gillooly J. F. (2011). The cost of sex: Quantifying energetic investment in gamete production by males and females. PloS One 6, e16557. doi: 10.1371/journal.pone.0016557
Hoenig J. M. (1983). Empirical use of longevity data to estimate mortality rates. Fish. Bull. 82, 898–903.
Iwamoto T. (2008). “A brief taxonomic history of grenadiers”, in Grenadiers of the world oceans: Biology, stock assessment and fisheries. Eds. Orlov A. M., Iwamoto T. (Maryland: American Fisheries Society), 3–13.
Kelly C. J., Connolly P. L., Bracken J. J. (1997). Age estimation, growth, maturity and distribution of the roundnose grenadier from the Rockall trough. J. Fish Biol. 50, 1–17. doi: 10.1111/j.1095-8649.1997.tb01336.x
Kimura D. K. (2008). Extending the von Bertalanffy growth model using explanatory variables. Can. J. Fish. Aquat. Sci. 65, 1879–1891. doi: 10.1139/F08-091
Laptikhovsky V. V. (2005). A trophic ecology of two grenadier species (Macrouridae, Pisces) in deep waters of the Southwest Atlantic. Deep Sea Res. I: Oceanogr. Res. Pap. 52, 1502–1514. doi: 10.1016/j.dsr.2005.03.003
Lee B., Cockroft K., Arkhipkin A., Wing S. R., Randhawa H. S. (2019). Age, growth and mortality estimates for the ridge-scaled grenadier Macrourus carinatus (Günther 1878) in the south-western Atlantic. Fish. Res. 218, 174–185. doi: 10.1016/j.fishres.2019.05.012
Lin X., Zhang D. (1999). Inference in generalized additive mixed models by using smoothing splines. J. R. Stat. Soc Ser. B Stat. Methodol. 61, 381–400. doi: 10.1111/1467-9868.00183
Marriott P., Manning M. J., Horn P. L. (2006). Age estimation and maturity of the ridge-scaled macrourid (Macrourus whitsoni) from the Ross Sea. CCAMLR Sci. 13, 291–303.
Massutí E., Morales-Nin B., Stefanescu C. (1995). Distribution and biology of five grenadier fish (Pisces: Macrouridae) from the upper and middle slope of the northwestern Mediterranean. Deep Sea Res. I: Oceanogr. Res. Pap. 42, 307–330. doi: 10.1016/0967-0637(95)00003-O
McMillan P., Iwamoto T., Stewart A., Smith P. J. (2012). A new species of grenadier, genus Macrourus (Teleostei, Gadiformes, Macrouridae) from the southern hemisphere and a revision of the genus. Zootaxa 3165, 1–24. doi: 10.11646/zootaxa.3165.1.1
Millar R. B. (2015). A better estimator of mortality rate from age-frequency data. Can. J. Fish. Aquat. Sci. 72, 364–375. doi: 10.1139/cjfas-2014-0193
Moore B. R. (2019). Age-based life history of humpback red snapper, Lutjanus gibbus, in New Caledonia. J. Fish Biol. 95, 1374–1384. doi: 10.1111/jfb.14142
Moore B. R. (2022). Age-based life history of Pacific longnose parrotfish Hipposcarus longiceps from New Caledonia. J. Fish Biol. 100, 997–1008. doi: 10.1111/jfb.15004
Moore B. R., Parker S. J. (2021). Catches and data available on bycatch species from the toothfish fishery in the Ross Sea region (Subarea 88.1 and SSRUs 88.2A-b) through 2020-2021. WG-FSA-2021/32 (Hobart, Australia: CCAMLR).
Moore B. R., Parker S., Pinkerton M. H. (2022). Otolith shape as a tool for species identification of the grenadiers Macrourus caml and M. whitsoni. Fish. Res 253:106370. doi: 10.1016/j.fishres.2022.106370
Morley S. A., Mulvey T., Dickson J., Belchier M. (2004). The biology of the bigeye grenadier at South Georgia. J. Fish Biol. 64, 1514–1529. doi: 10.1111/j.0022-1112.2004.00405.x
Münster J., Kochmann J., Klimpel S., Klapper R., Kuhn T. (2016). Parasite fauna of Antarctic Macrourus whitsoni (Gadiformes: Macrouridae) in comparison with closely related macrourids. Parasites Vectors 9, 403. doi: 10.1186/s13071-016-1688-x
O'Driscoll R. L. (2015). New Zealand-Australia Antarctic ecosystems voyage. WG-EMM-15/56 (Hobart, Australia: CCAMLR).
O'Driscoll R. L., Bowden D., Pinkerton M. H. (2019). Ross Sea environment and ecosystem voyage 2019. WG-EMM-2019/38 (Hobart, Australia: CCAMLR).
Orlov A. M., Vedishcheva E. V., Trofimova A. O., Orlova S. Y. (2018). Growth and age of the roughhead grenadier Macrourus berglax in waters off southwest Greenland. J. Ichthyol. 58, 389–395. doi: 10.1134/S0032945218030098
Parker S. J., Stevens D. W., Ghigliotti L., La Mesa M., Di Blasi D., Vacchi M. (2019). Winter spawning of Antarctic toothfish Dissostichus mawsoni in the Ross Sea region. Antarct. Sci. 31, 243–253. doi: 10.1017/S0954102019000282
Pèron C., Welsford D., Ziegler P., Lamb T., Gasco N., Chazeau C., et al. (2016). Modelling spatial distribution of Patagonian toothfish through life-stages and sex and its implications for the fishery on the Kerguelen Plateau. Prog. Oceanogr. 141, 81–95. doi: 10.1016/j.pocean.2015.12.003
Pinkerton M. H., Forman J., Stevens D. W., Bury S. J., Brown J. (2012). Diet and trophic niche of Macrourus spp. (Gadiformes, Macrouridae) in the Ross Sea region of the Southern Ocean. J. Ichthyol. 52, 787–799. doi: 10.1134/S0032945212100104
Pinkerton M. H., McMillan P., Forman J., Marriott P., Horn P., Bury S., et al. (2013). Distribution, morphology and ecology of Macrourus whitsoni and M. caml (Gadiformes, Macrouridae) in the Ross Sea region. CCAMLR Sci. 20, 37–61.
R Core Team (2021). R: A language and environment for statistical computing. 4.0.2 edn (Vienna: R Foundation for Statistical Computing).
Rodgveller C. J., Clausen D. M., Nagler J. J., Hutchinson C. (2010). Reproductive characteristics and mortality of female giant grenadiers in the Northern Pacific Ocean. Mar. Coast. Fish. 2, 73–82. doi: 10.1577/C09-028.1
Rodríguez-Marín E., Ruiz M., Sarasua A. (2002). Validation of roughhead grenadier (Macrourus berglax) otolith reading. J. Appl. Ichthyol. 18, 70–80. doi: 10.1046/j.1439-0426.2002.00330.x
Smith P. J., Steinke D., McMillan P. J., Stewart A. L., McVeagh S. M., Diaz de Astarloa J. M., et al. (2011). DNA barcoding highlights a cryptic species of grenadier Macrourus in the Southern Ocean. J. Fish Biol. 78, 355–365. doi: 10.1111/j.1095-8649.2010.02846.x
Stevens D. W., Dunn M. R., Pinkerton M. H., Forman J. S. (2014). Diet of Antarctic toothfish (Dissostichus mawsoni) from the continental slope and oceanic features of the Ross Sea region, Antarctica. Antarctic Sci. 26, 502–512. doi: 10.1017/S095410201300093X
Then A. Y., Hoenig J. M., Hall N. G., Hewitt D. A. (2015). Evaluating the predictive performance of empirical estimators of natural mortality rate using information on over 200 fish species. ICES J. Mar. Sci. 72, 82–92. doi: 10.1093/icesjms/fsu136
Williams A. J., Wakefield C. B., Newman S. J., Vourey E., Abascal F. J., Halafihi T., et al. (2017). Oceanic, latitudinal and sex-specific variation in demography of a tropical deepwater snapper across the Indo-Pacific region. Front. Mar. Sci. 4. doi: 10.3389/fmars.2017.00382
Keywords: Macrouridae, age, growth, maturity, deepwater fisheries, Antarctica
Citation: Moore BR, Parker SJ, Marriott PM, Sutton C and Pinkerton MH (2022) Comparative biology of the grenadiers Macrourus caml and M. whitsoni in the Ross Sea region, Antarctica. Front. Mar. Sci. 9:968848. doi: 10.3389/fmars.2022.968848
Received: 14 June 2022; Accepted: 19 August 2022;
Published: 06 October 2022.
Edited by:
Cristina Porcu, University of Cagliari, ItalyReviewed by:
Andrea Bellodi, University of Cagliari, ItalyBrendon Lee, Falkland Islands Fisheries Department, Falkland Islands
Massimiliano Bottaro, Anton Dohrn Zoological Station, Italy
Copyright © 2022 Moore, Parker, Marriott, Sutton and Pinkerton. This is an open-access article distributed under the terms of the Creative Commons Attribution License (CC BY). The use, distribution or reproduction in other forums is permitted, provided the original author(s) and the copyright owner(s) are credited and that the original publication in this journal is cited, in accordance with accepted academic practice. No use, distribution or reproduction is permitted which does not comply with these terms.
*Correspondence: Bradley R. Moore, YnJhZGxleS5tb29yZUBuaXdhLmNvLm56