- 1School of Ecology and Environment, Anhui Normal University, Wuhu, China
- 2Collaborative Innovation Center of Recovery and Reconstruction of Degraded Ecosystem in Wanjiang Basin Co-founded by Anhui Province and Ministry of Education, Anhui Normal University, Wuhu, China
- 3Provincial Key Laboratory of Biotic Environment and Ecological Safety in Anhui, Anhui Normal University, Wuhu, China
- 4College of Animal Sciences, Zhejiang University, Hangzhou, China
Berberine, an isoquinoline alkaloid found in some traditional medicinal plants, such as Berberis vulgaris and Coptis Chinensis, has been considered as an effective drug in treating obesity, hypertension, type-2 diabetes, hyperlipidemia, and gout in humans and animals. It currently has certain applications in fish nutrition and health, mainly because it has strong biological and pharmacological properties, such as antioxidative, anti-inflammation, antidiarrheal, analgesic, antimicrobial, anticancer, hepatoprotective, and lipid- and glucose-lowering properties. Recent studies revealed that berberine supplementation in different fish diets could alleviate liver pathological changes, intestinal histological and microbiota alterations caused by high lipid and carbohydrate diets, as well as improve growth performance, antioxidative and immune status, and stress resistance ability of fish. However, the beneficial effects of berberine vary with fish species, basal diet, feeding modes, supplementation level, and etc. This review highlights the bioavailability and toxicity of berberine, and its mechanisms in lipid and glucose metabolism, antioxidation, anti-inflammation, and protection of intestinal health, as well as the other findings on supplementing berberine in the fish diet. Moreover, this review provides future perspectives on berberine application in fish nutrition and health.
Introduction
Aquaculture contributes to more than 50% of seafood production in the world and is responsible for increasing human protein consumption (FAO, 2018). Fish is a better source of protein, micronutrients (e.g., minerals and vitamins), and essential fatty acids compared with that of farm animals (Kiczorowska et al., 2017). However, the rapid expansion of aquaculture is confronted by limited land source, and most aquaculture species are under stress in intensive farming conditions, leading to the outbreak of diseases. To reduce financial losses, chemicals and antibiotics are generally used to maintain good health condition and promote the growth of fish. Nevertheless, the overuse of antibiotics could increase the emergence of antibiotic-resistant bacteria, weaken the natural immunity of farmed fish, increase host susceptibility, and cause residue accumulation in fish tissues and water, thus threatening the environment and human health (Gangwar et al., 2013; Nm et al., 2018; Abdel-Latif et al., 2020). For the sake of reducing unnecessary use of antibiotics in aquaculture, herbal components are supplemented in aquatic feed due to their low toxicity, high safety, and environment-friendly advantage, in addition to their effects in enhancing disease resistance, immune and antioxidative status, promoting growth, and treating metabolic disorders (Zhu, 2020). Recently, the application of natural compounds has been widely explored in feed to replace antibiotics in monogastric animals, fish, and poultry (Alagawany et al., 2021; Tadese et al., 2022).
Berberine is a yellow odorless crystalline powder with an extremely bitter taste. Its molecular formula is (16,17-dimethoxy-5,7-dioxa-13-azoniapentacyclo[11.8.0.02,10.04,8.015,20]henicosa-1(13),2,4(8),9,14,16,18,20-octaene) with a molecular weight of 336.37g/mol (Figure 1). It can easily dissolve in hot ethanol, but slowly dissolves in water, and is almost insoluble in low-polar organic solvents (Xu et al., 2021). It is an isoquinoline alkaloid that widely exists in the rhizome, roots, and stems of some traditional medicinal plants, such as Berberis aristata DC, Coptis Chinensis Franch, and Berberis vulgaris L. The berberine contents in these plants range from 0.05 mg/g to 96.10 mg/g, while Berberis is the richest natural source of berberine (Xu et al., 2021). Presently, chemical synthesis is a more productive way to obtain berberine, and berberine sulfate salt and chloride are commonly used in the clinic because they are relatively more soluble than berberine (Kumar et al., 2015).
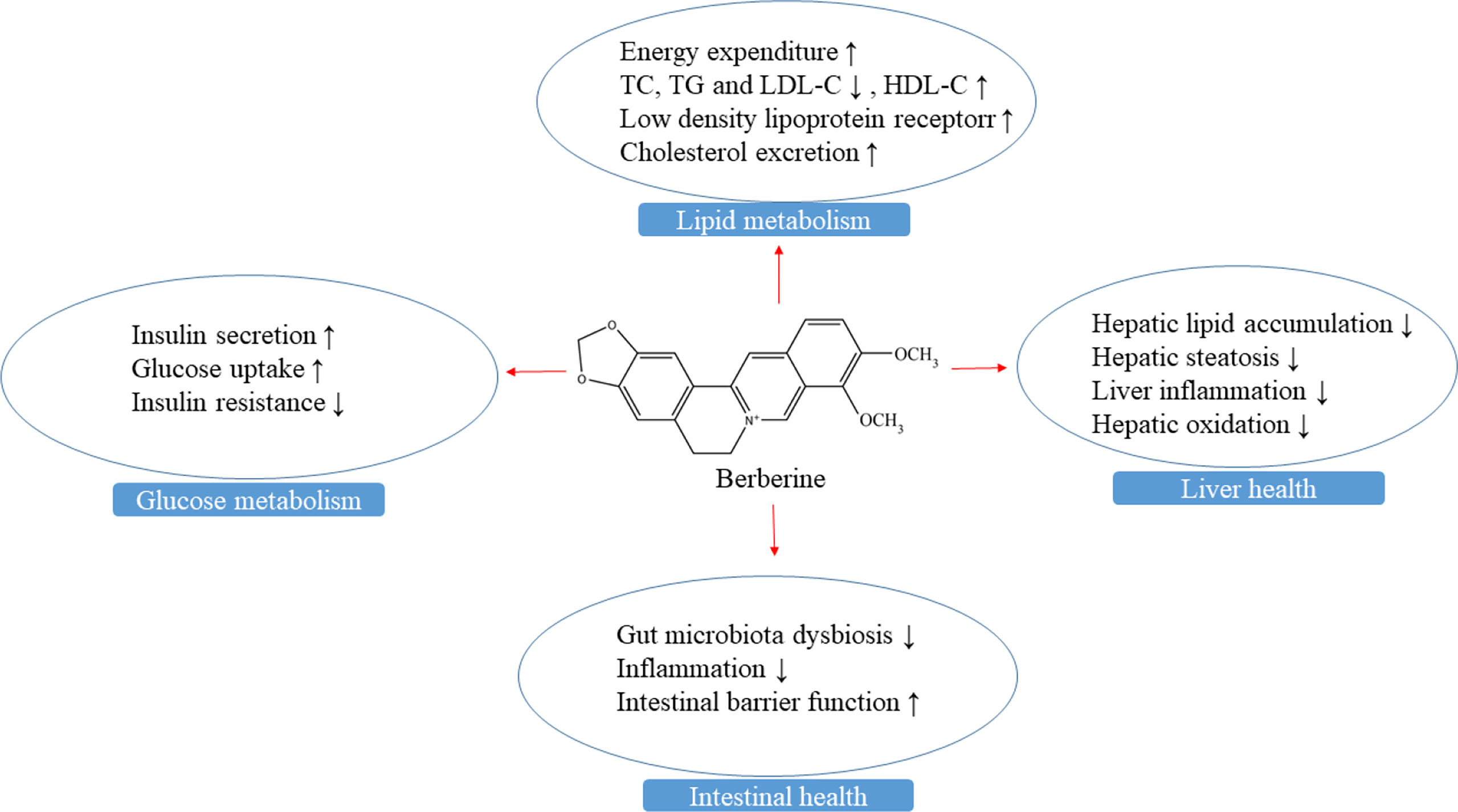
Figure 1 The mechanisms of berberine in maintaining liver and intestine health, and glucose and lipid metabolism.
There is a long history of using berberine in Chinese and Ayurvedic medicinal systems (Kumar et al., 2015). In traditional Chinese medicine, berberine is widely used for treating diarrhea, dysentery, fatty liver, hypertension, obesity, and type-2 diabetes (Liu et al., 2016; Wang et al., 2020a), owing to its strong antioxidant, anti-inflammation, antidiarrheal, analgesic, anticancer, and antimicrobial activities, as well as lipid- and glucose-lowering properties (Kumar et al., 2015; Pirillo and Catapano, 2015; Liu et al., 2016; Xu et al., 2021).
In fish feed, carbohydrate and lipid are widely used because of their “protein-sparing” effect, as well as they are important nutrients for fish. Suitable levels of dietary lipid and carbohydrate can promote growth performance, improve feed efficiency, reduce dietary protein requirement, reduce disease susceptibility, and maintain the health condition of fish (Ai et al., 2004; Wu et al., 2016; Kamalam et al., 2017; Wang et al., 2019). However, the lipid and carbohydrate levels in fish feed sometimes exceed the requirements because they are cheaper energy sources than protein. Excessive lipid and carbohydrate in the fish diet causes lipid accumulation in the liver, impairs liver functions, reduces the antioxidative and immune status of fish, and eventually leads to low yields and economic losses (Wu et al., 2016; Lin et al., 2018; Cao et al., 2019; Yang et al., 2019). The liver is a metabolic organ in charge of lipid and glucose homeostasis, plasma protein production, degradation of toxins, and bile synthesis (Zhou et al., 2021). Thus, maintenance of liver normal function is vital for fish health and growth. Previously, some herbal compounds were proven to attenuate liver dysfunction caused by high lipid (HL) diet and high carbohydrate (HC) diet (e.g., xylooligosaccharides (Abasubong et al., 2018), resveratrol (Shi et al., 2018), and curcumin (Bao et al., 2022)). Berberine has immense potential to be a novel feed additive in treating liver dysfunction caused by HL and HC diets in fish, due to its strong effects in treating metabolic disorders, such as type-2 diabetes, fatty liver, hyperlipidemia, obesity, and non-alcoholic steatohepatitis (NASH) in clinical and animal studies, suggesting its important role in glucose and lipid metabolism (Xu et al., 2021). Besides, berberine supplementation also promoted growth performance, intestinal health, antioxidative capacity, and immune status of fish in several studies (Doan et al., 2020; Yu et al., 2020).
This review paper aims to summarize the bioavailability and toxicity of berberine, and its mechanisms in lipid and glucose modulation, antioxidant and anti-inflammatory effects, and regulation of intestinal microbiota demonstrated in clinical and animal studies. We also review the current berberine-related studies in fish health to increase its potential application in aquaculture.
Bioavailability
Feng et al. (2015) proposed that the gut microbiota converts insoluble berberine into dihydroberbreine (dhBBR), which showed a five times faster absorption rate than that of berberine in the intestine of rats. DhBBR is subsequently oxidized to berberine and enters blood circulation. Although intestinal microbiota contributes to the absorption of berberine, the bioavailability of berberine is still lower than 1% after oral administration (Imenshahidi and Hosseinzadeh, 2016). Several factors affect the intestinal absorption of berberine: 1) Intestinal first-pass elimination. Liu et al. (2010) reported that almost half of the intragastric administrated berberine disposed in the small intestine of rats due to the intestinal first-pass elimination, which caused very low oral bioavailability (0.36%); 2) Berberine is in the ion form and easily occurs self-aggregates in the stomach and upper intestine, with acidic environment (Spinozzi et al., 2014); 3) Berberine exhibits low permeability across the intestinal mucous membrane of rats, and is identified as a low permeable drug (Liu et al., 2010); 4) The re-excretion of berberine from the hepatoenteral circulation process inhibits its absorption (Imenshahidi and Hosseinzadeh, 2016; Liu et al., 2016); 5) Berberine is a substrate of P-glycoprotein (P-gp), which is a ATP-binding cassette transporter that located in the apical membrane of the epithelial layer of the gut wall, and is in charge of maintaining the integrity of intestinal barrier to keep body away from many drugs and exogenous toxins, thus P-gp is a crucial factor that influences bioavailability of orally administered drugs in the intestine (Chen et al., 2014). P-glycoprotein reducing berberine absorption by transporting berberine out of cells has been widely reported in vivo and in vitro (Maeng et al., 2002; Shitan et al., 2007; Pirillo and Catapano, 2015). Besides, P-gp expression in the intestine increased with increasing berberine levels and berberine exposure time, indicating the high berberine level or long-term administration of berberine may result in poor berberine absorption (Benet et al., 1999; Maeng et al., 2002). Similarly, Kheir et al. (2010) observed that a low concentration of berberine is rapidly absorbed by the intestine, whereas a high level of berberine caused absorption limitation in rats. Some studies have reported that P-gp antagonists, such as silymarin (Di Pierro et al., 2013), tetrandrine (Shan et al., 2013a), and cationized chitosan (Fratter and Servi, 2015) treatments significantly improved intestinal berberine absorption. Therefore, oral administration of berberine normally shows low bioavailability.
Toxicity and side effects
Generally, berberine has very low side effects and toxicity, and the toxicity is related to administration methods (Pang et al., 2015). A study in mice found that the LD50 of intraperitoneal (IP) and intravenous (IV) injections were 57.6 g/kg and 9.0 g/kg, respectively, whereas intragastric administration did not obtain LD50, which might be due to the low oral bioavailability of berberine (Kheir et al., 2010). Rad et al. (2017) concluded that the toxicity of berberine varies with dosage, animal species, and routes of administration, and the oral route is less toxic than IP and IV injections. Similarly, mice fed 156 mg berberine/kg/day for 30 days did not cause any death (Yi et al., 2013). In a clinical study, the gastrointestinal adverse effects were only presented in the first four weeks in 34.5% of patients when treated with 0.5g berberine (twice a day) for 13 weeks (Yin et al., 2008). Thus, both clinical and animal studies have demonstrated that berberine exhibits low side effects and toxicity, hence potentially a safe drug.
Liver protection, antioxidative and immunomodulatory properties
The liver is the main accumulation organ of berberine after oral administration, followed by the kidney, muscle, heart, and pancreas in mice (Pirillo and Catapano, 2015). The high hepatic accumulation of berberine may account for its lipid-lowering effect on the liver (Liu et al., 2016). Berberine displays a potent hepatoprotective effect that has been extensively studied, which may be ascribed to its regulation of glucose and lipid metabolism, antioxidative and immune systems (Figure 1).
Regulation of glucose metabolism
Numerous studies demonstrated that berberine has anti-diabetic activity, and the main mechanisms are: 1) Promotion of insulin secretion. Glucagon-like peptide-1 (GLP-1) is produced by enteroendocrine L cells in the gut which is sensitive to nutrient ingestion and in charge of the overall insulin response to glucose ingestion (Chen et al., 2014). Besides, berberine can improve insulin production and secretion through the insulin/insulin-like growth factor-1 signaling cascade in 3T3-L1 adipocytes (Ko et al., 2005). 2) Alleviating insulin resistance (IR). Berberine reduces IR by the protein kinase C (PKC)-dependent upregulation of insulin receptor substrate-2 (IRS-2) in rats (Xing et al., 2011; Yang et al., 2011; Yan et al., 2015; Zhao et al., 2017). In addition, berberine lessened IR by improving the activity of AMP-activated protein kinase (AMPK) in 3T3-L1 adipocytes (Lee et al., 2006). 3) Inhibiting gluconeogenesis. Glucose-6-phosphatase (G6Pase) and phosphoenolpyruvate carboxykinase (PEPCK) are two vital enzymes in the gluconeogenesis pathway. Berberine inhibited the expressions of PEPCK and G6Pase by upregulating the expressions of liver kinase B1 (LKB1) and AMPK, thus reducing the translocation of cAMP response element-binding protein (CREB)-regulated transcription coactivator 2 (TORC2) into the cell nucleus in STZ-induced diabetic rats (Jiang et al., 2015). 4) Promotion of glucose uptake. A study in 3T3-L1 adipocytes proved that berberine promoted glucose uptake through activating adenosine monophosphate-activated protein kinase, and increasing glucose transporter 1 (GLUT 1) activity (Zhou et al., 2007). Besides, berberine can increase glucose transporter 4 (GLUT4) translocation into the plasma membrane to improve glucose uptake through the insulin receptor substrate-1-phosphoinositide 3 Kinase-Akt (IRS1-PI3-Kinase-Akt) and insulin signaling pathways in 3T3-L1 adipocytes (Ko et al., 2005). 5) Promoting glycolysis. Berberine can inhibit the mitochondrial respiratory chain complex I to reduce ATP production, then elevate AMP/ATP ratio and activate AMPK, thus inhibiting gluconeogenesis and improving glycolysis in HepG2 hepatocytes and C2C12 myotubes (Xu et al., 2014). 6) Berberine reduces intestinal glucose digestion and absorption by inhibiting α-glucosidase activity, which is in charge of carbohydrates digestion and monosaccharides production (Pang et al., 2015) (Figure 2).
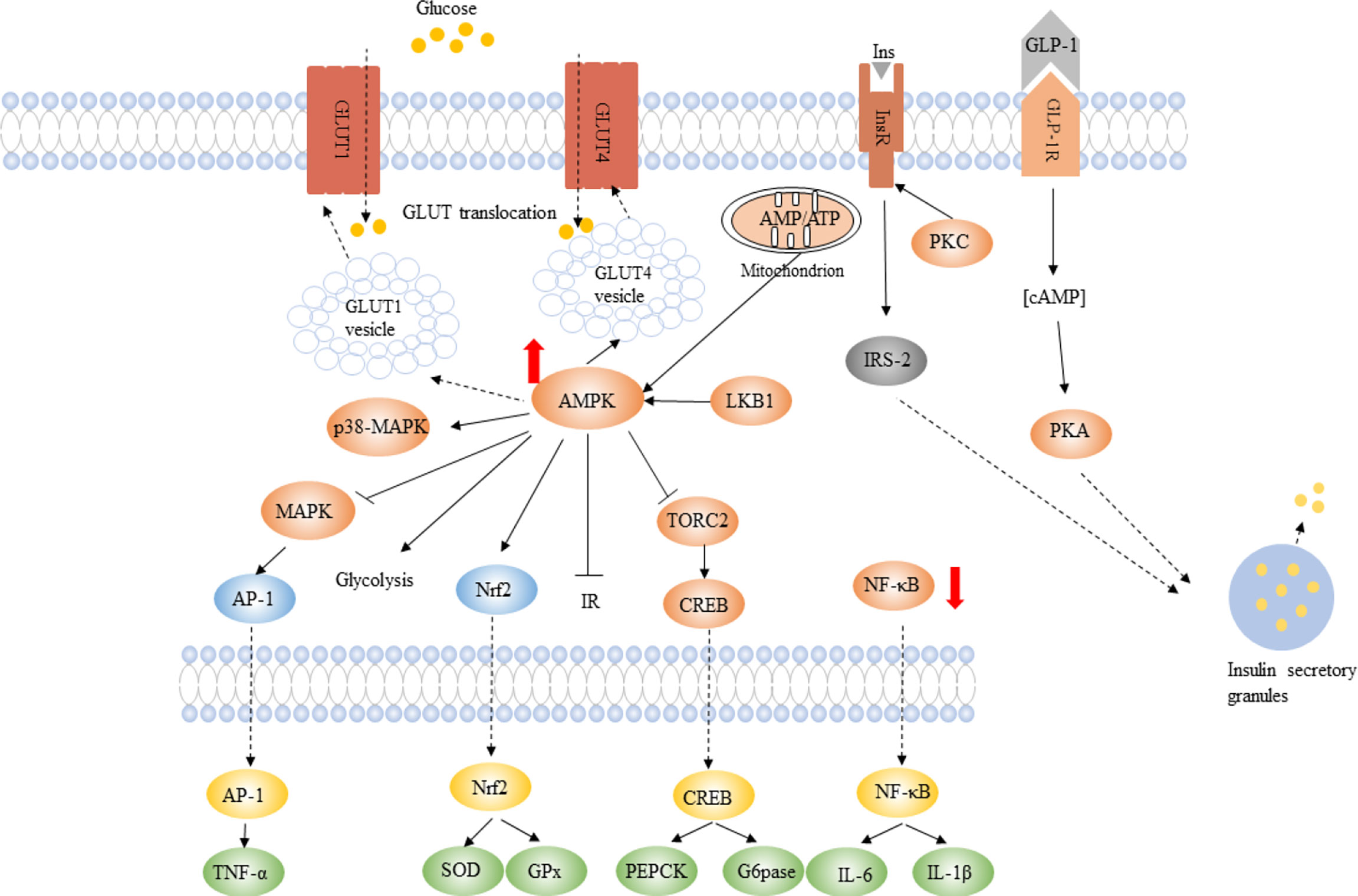
Figure 2 The potential mechanisms of berberine in regulating glucose metabolism, improving antioxidative and immune status. Berberine can enhance insulin sensitivity and improve insulin secretion. BBR induces glycolysis via activating AMPK pathway and increasing GLUT1 and GLUT4 translocation. BBR reduces the expression of PEPCK and G6Pase genes to suppress gluconeogenesis. BBR decreases the production of pro-inflammatory cytokines, for example, TNF-α. Up arrows indicate an increase or activation, and down arrows indicate a decrease or suppression. Modified from Xu et al. (2021) and Zhou et al. (2021).
Regulation of lipid metabolism
Berberine exhibits an anti-hyperlipidemia effect by reducing triglyceride (TG), total cholesterol (TC), and low-density lipoprotein cholesterol (LDL-C) contents in the plasma of rats (Tang et al., 2006), as well as a hepatoprotective effect by reducing hepatic TG and TC contents (Zhou et al., 2021). The mechanisms of berberine regulating lipid metabolism are mainly: 1) Inhibition of lipid uptake. Sun et al. (2017) reported that berberine inhibited intestinal bile salt hydrolase secretion, increased taurocholic acid discharge, activated intestinal farnesoid X receptor (FXR) pathway, and reduced hepatic fatty-acid translocase Cd36 expression, which together inhibited long-chain fatty acid uptake of the mice liver. 2) Promoting lipid oxidation and inhibiting lipogenesis. Berberine can activate the AMPK signaling pathway, which is a target for metabolic diseases and is the upstream gene of many lipid-producing genes. Berberine promotes phosphorylation of AMPK and increases expression of fibroblast growth factor 21 (FGF21) to increase hepatic energy metabolism in primary mouse hepatocytes (Zhou et al., 2018). Besides, it also promotes phosphorylation of sterol-regulatory element binding protein-1c (SREBP-1c) to suppress the fatty acid synthase (FAS) and stearoyl-CoA desaturase (SCD) expressions, thus improving hepatic steatosis and reducing hepatic TG synthesis in mice (Zhu et al., 2019). In addition, Sirtuin 3 (SIRT3) is also activated by phosphorylation of AMPK, which affects carnitine palmitoyltransferase-1A (CPT-1A) and phosphorylation-acetyl-CoA carboxylase (ACC) expressions to increase liver steatosis of rats (Zhang et al., 2019). 3) Increasing lipid transportation. Excessive cholesterol intake generally causes massive cholesterol accumulation in the liver, which activates nonparenchymal cells and leads to liver metabolic abnormalities. Low-density lipoprotein (LDL) transports cholesterol from the liver to peripheral tissue cells to alleviate liver damage (Zhou et al., 2021). Berberine can increase low-density lipoprotein receptor (LDLR) expression in the liver, and it also works on the 5′ proximal section of LDLR mRNA 3′ UTR to improve the stability of LDLR mRNA, which is achieved by activating the signaling cascade of AMPK/Raf-1/MEK/ERK in human hepatoma cells (Kong et al., 2004). 4) Maintenance of the normal function of mitochondria. Gomes et al. (2012) reported that berberine alleviated mitochondrial dysfunction caused by hyperglycemia and the high fat (HF) diet partly via increasing mitochondrial biogenesis modulated by sirtuins 1 gene through the AMPK pathway in the skeletal muscle of rats. 5) The alleviation of IR by berberine also contributes to lipid metabolism (Zhou et al., 2021) (Figure 3).
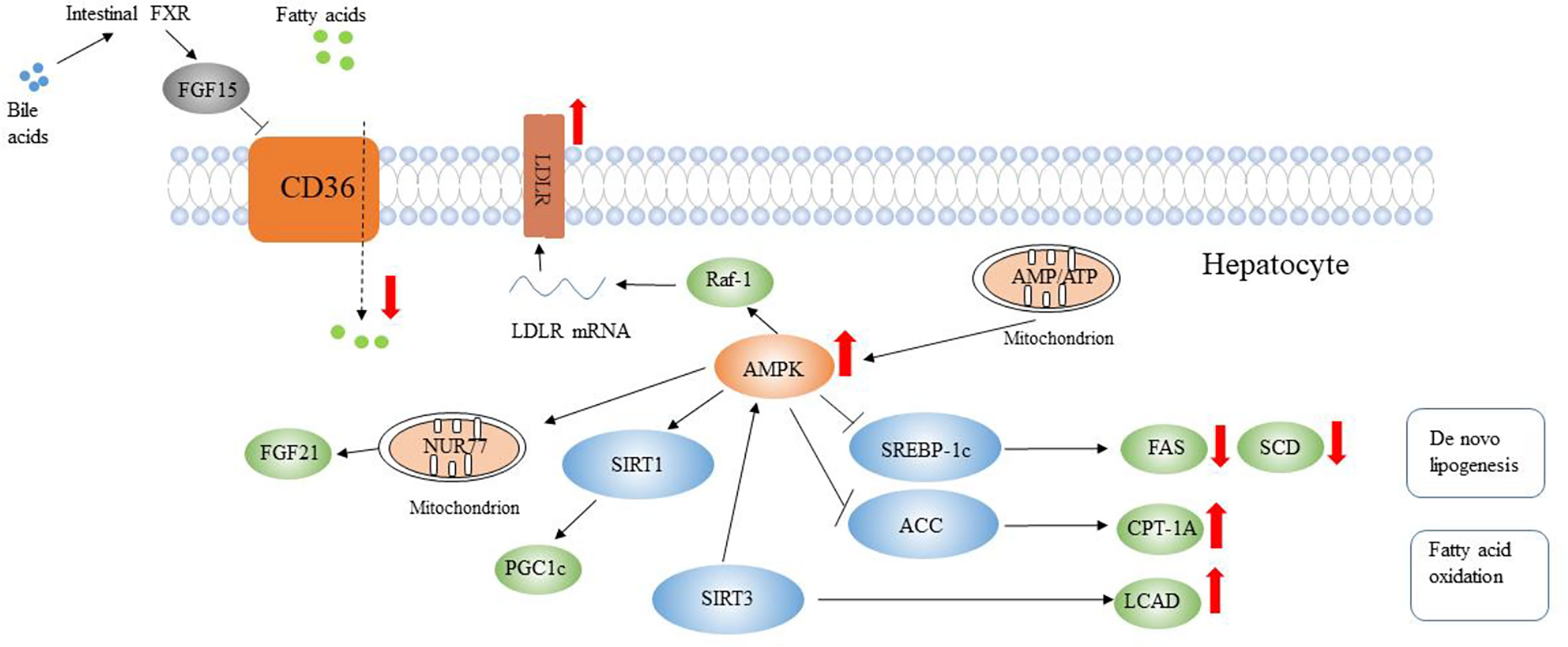
Figure 3 The potential mechanisms of berberine in reducing liver lipid accumulation. Berberine inhibits hepatic lipid absorption by the intestinal microbiota-bile acid-FXR pathway. Berberine inhibits de novo lipogenesis but improves fatty acid oxidation via activation of AMPK. Berberine increases LDLR expression and LDLR mRNA stabilization. Up arrows indicate an increase or activation, and down arrows indicate a decrease or suppression. Modified from Xu et al. (2021) and Zhou et al. (2021).
Antioxidative and anti-inflammatory effects
Berberine and its derivatives have strong antioxidant activity, and both in vivo and in vitro studies revealed that berberine is able to inhibit reactive oxygen species (ROS) production (Shan et al., 2011; Siow et al., 2011). Firstly, berberine can quench nitric oxide (NO), superoxide anion ( ) and the precariously reactive molecule, peroxynitrite (OONO-) directly (Siow et al., 2011). A study in rats found that oral administration of berberine alleviated the decline of catalase (CAT), superoxide dismutase (SOD), and glutathione peroxidase (GPx) activities in serum by scavenging ONOO-, NO, and stress (Yokozawa et al., 2010). Besides, berberine can inhibit ROS-producing oxidase enzymes. Sarna et al. (2010) observed that berberine effectively inhibited the production of intracellular superoxide in LPS-stimulated macrophages by selectively inhibiting gp91phox expression. Similarly, berberine activated the AMP-activated protein kinase sensitive pathway and reduced iNOS expression to inhibit NO production in LPS treated murine macrophages (Jeong et al., 2009). Hur et al. (2009) also reported that berberine inhibited the production of ROS through the p38 mitogen-activated protein kinase (MAPK) pathway, and activated caspase-3 in the human hematoma HepG2 cells. Furthermore, berberine can modulate the activities of several endogenous antioxidant enzymes. For example, a study in macrophages revealed that pretreatment with berberine significantly increased SOD activity (Sarna et al., 2010).
Berberine can directly downregulate the expressions of pro-inflammatory cytokines, such as interleukin-6 (IL-6), tumor necrosis factor-α (TNF-α), and interleukin-1β (IL-1β), as well as indirectly affect the expressions of activator protein 1 (AP-1), nuclear factor-kappa B (NF-κB), AMPK, Rho GTPase signaling pathways, and MAPK- mediated pathways to decrease inflammation response in human macrophages (Chen et al., 2014). Berberine mitigated non-alcoholic steatohepatitis (NASH) by the C-X-C chemokine receptor type 4 (CXCR4) signaling pathway, and significantly reduced the expressions of interleukin-8 (IL-8), NF-κB, and phosphoinositide 3-kinase in mice (Yang et al., 2017). Likewise, berberine treatment increased the survival rate, anti-inflammatory cytokines (IFN-γ and IL-10) expressions, and downregulated proinflammatory cytokines (IL-17A, IL-6, IL-17F, and IL-1β) expressions in the liver and spleen of ducks infected by Riemerella anatipestifer (Fernandez et al., 2017). Besides, berberine also enhanced macrophage function through increasing apoptosis by caspase-3 activation in the DDS-induced colitis mice (Yan et al., 2012).
Protection of the intestine and regulation of intestinal microbiota
Due to the low bioavailability of berberine, more than 90% of oral-administrated berberine remains in the intestine, causing the intestine to become the main working site of berberine (Wang et al., 2017; Pan et al., 2019). Gu et al. (2015) reported that a stronger lipid-lowering effect was found in intragastrically administered hamsters than that of intraperitoneally administered hamsters, even though the former showed much lower bioavailability than the latter, indicating the gastrointestinal tract was the main target for the hypolipidemic effect of berberine.
The intestine is regarded as the first line of defense against antigens. The intestinal physical barrier determines the absorption capacity of nutrients, and its integrity is vital for animal health (Lin et al., 2020; Wang et al., 2020b). Berberine was demonstrated to restore barrier function and maintain epithelial gut permeability in diseased intestines. Berberine reduced the TNF-α-NF-κB-MLCK pathway by increasing tumor necrosis factorα-induced protein 3 (TNFAIP3) expression to protect intestinal epithelial tight junction and repaired the intestinal epithelial barrier in diarrhea-predominant irritable bowel syndrome rats (Hou et al., 2019). Besides, Gu et al. (2011) reported that pretreatment of berberine prevented the disruption of tight junction in intestinal epithelium caused by lipopolysaccharide (LPS) in mice, which might be linked to the down-regulating of the NF-κB and myosin light chain kinase pathway.
It is well-known that berberine has antidiarrheal activity, mainly because it has a direct bactericidal effect. Berberine was demonstrated to reduce bacterial adherence to the epithelial or mucosal surface, and decrease intestinal secretion of electrolytes and water caused by microbial enterotoxins (Chen et al., 2014). Besides, a wide range of animal experiments revealed that berberine has noteworthy effects on intestinal microbiota composition. Berberine could kill or repress some harmful intestinal bacteria, such as gram-negative bacteria (e.g., K. pneumonia, P. mirabilis, and E. coli) (Čerňáková and Košťálová, 2002), and promote the growth and reproduction of some beneficial bacteria (e.g., Lactobacillus acidophilus and Bifidobacterium adolescentis) (Chen et al., 2014). The antimicrobial activity of berberine also contributes to curing metabolic disorders. For example, Firmicutes and Bacteroidetes are two dominant gut microbiota, and a higher Firmicutes/Bacteroidetes (F/B) ratio may be an indication of more intestinal energy harvest (Jumpertz et al., 2011; Cao et al., 2016). Xie et al. (2011) found berberine supplementation in a HF diet increased fecal Bacteroidetes and reduced Firmicutes richness, along with reduced blood glucose and lipid of rats. A similar observation of intestinal F/B ratio was reported in rats when berberine was co-administrated with a high-fat diet (Sun et al., 2016). Berberine also reduced insulin resistance, plasma lipid contents and endogenous glucose production of rats fed a HF diet by regulation of the microbiota-gut-brain axis (Sun et al., 2016). In mammals, berberine regulated bacterial bile salt hydrolase and 7α-dehydroxylases activities, thus changing the bile acid pool, FXR signaling, and alleviating metabolic disease (Gu et al., 2015a; Sun et al., 2017), which is an important mechanism of regulating energy and immune by berberine. Moreover, berberine can promote intestinal butyrate-producing bacteria (e.g., Ruminococcus, Butyricimonas, and Coprococcus) growth to produce short-chain fatty acids (SCFAs) (Xu et al., 2021), which is beneficial for alleviating inflammation, improving gut barrier functions, creating a nonpermissive environment for pathogens, and helpful for obesity and insulin resistance-related metabolic abnormalities (Pang et al., 2015).
The role of berberine in fish nutrition
The effects of supplementing berberine in different fish diets are shown in Table 1. It includes a total of 18 papers, which reported the roles of berberine in protecting liver and intestine functions, improving growth performance, antioxidative and immune status, as well as increasing disease and stress resistance ability. Many of the berberine functions observed in fish have been reported in rodent animals and in vitro, but there are still some discrepancies with the previous findings.
Optimal supplementation level and feeding mode of berberine
Qin (2014) studied the pharmacokinetics of berberine hydrochloride in Nile tilapia (Oreochromis niloticus). After oral administration of 30 mg/kg body weight of berberine hydrochloride, the berberine concentrations in different tissues were liver > kidney > muscle > plasma. After intraperitoneal injection of 10 mg/kg body weight berberine hydrochloride, the berberine levels were kidney > liver > muscle > plasma. Oral administration of berberine hydrochloride showed slower absorption and elimination rates but wider distribution than that of intraperitoneal injection. These pharmacokinetic patterns were similar to the results in other animals (Li et al., 2005; Feng et al., 2015; Kumar et al., 2015; Pirillo and Catapano, 2015).
As shown in Table 1, the supplementation level of berberine in fish feed ranged from 30 to 9000 mg/kg in different fish species. In Chinese Fisheries Pharmacopoeia, 30 mg/kg berberine is the highest level for controlling bacterial diseases in fish (Pan et al., 2019). A study in blunt snout bream (Megalobrama amblycephala) reported that supplementation of 50 mg/kg berberine in a HF diet (15% fat) increased weight gain (WG) and specific growth rate (SGR) significantly, while 100 mg/kg berberine did not influence the growth of fish, which might be because 100 mg/kg berberine reduced the palatability of the diet since feed intake (FI) was significantly reduced, the authors observed some fish spat out the feed after eating the 100 mg/kg berberine supplemented HF diet due to its bitter taste. Thus 50 mg/kg berberine was recommended as a suitable level in fish feed (Zhou et al., 2019), and widely applied in the studies on blunt snout bream (Chen et al., 2016; Lu et al., 2016; Xu et al., 2017; Yu et al., 2020; He et al., 2021) and black sea bream (Acanthopagrus schlegelii) (Wang et al., 2020a; Wang et al., 2021). However, Doan et al. (2020) supplemented 1, 3, 6, and 9 g/kg berberine in the Nile tilapia diet, but the authors did not report the feed utilization data. And the reduction of FI in black sea bream was only observed in the 50 mg/kg berberine supplemented normal diet group, not in the berberine supplemented HL diet group (Wang et al., 2021). On the contrary, 400 mg/kg berberine supplementation in a HC diet significantly improved FI of largemouth bass (Chen et al., 2022). Besides, 50 mg/kg berberine supplemented in the HC diet did not affect the FI of blunt snout bream (He et al., 2021) and black sea bream (Wang et al., 2020a). Thus, the bitter taste of berberine may influence feed palatability in some cases, which varies with supplementation level, fish species, and basal diet composition.
Nile tilapia were fed 1, 3, 6, and 9 g/kg berberine supplemented diets for 8 weeks, and the lowest supplementation level (1g/kg) obtained the lowest feed conversion ratio (FCR), with the highest WG, SGR, and immune parameters (Doan et al., 2020). Xu et al. (2017) explored the effects of supplementing berberine in 5% and 10% fat diets with different feeding modes (continuous, 2-week interval, and 4-week interval modes) on the growth and immunity of blunt snout bream. They found that the low-fat diet with 50 mg/kg berberine at 2-week interval mode obtained the best growth performance of fish, while fish fed berberine supplemented high-fat diet at 2-week interval or 4-weeks interval feeding mode showed similar or even better growth performance than that of the continuous feeding mode. These results indicate that high dietary berberine level or long berberine exposure time may reduce the function of berberine. One hypothesis is that the intestinal Pg-p expression being upregulated by high dosage, or long-term administration of berberine, which inhibits the absorption of berberine by the intestine as reported in mammals (Maeng et al., 2002; Shan et al., 2013b). However, this mechanism in fish has not been reported and deserves further study. Thus, supplementing a relatively low level of berberine in the diet with a discontinuous feeding mode may be an effective and economical way in fish feed. Considering the high discrepancy of berberine supplementation levels in the previous studies, the effective and economical dietary berberine levels of different fish species should be investigated. Furthermore, even 9 g/kg berberine supplementation of berberine enhanced the growth performance of Nile tilapia (Doan et al., 2020), indicating that berberine is a safe feed additive in fish.
Effects on growth
Enhanced growth performance was reported in blunt snout bream fed a 50 mg/kg berberine-supplemented normal diet (Chen et al., 2016; Xu et al., 2017), a 50 mg/kg berberine-supplemented HC diet (Yu et al., 2020), a 50 mg/kg berberine-supplemented HL diet (Zhou et al., 2019), Nile tilapia fed the 1, 3, 6, and 9 g/kg berberine-supplemented normal diet (Doan et al., 2020), and yellow drum (Nibea albiflora) fed 50 mg/kg berberine-supplemented soybean oil-based diet (Tan et al., 2022). The growth promotion effect of berberine might be ascribed to its hepatoprotective effect, like some other Chinese herbs (Zhou et al., 2015; Zhou et al., 2019). Doan et al. (2020) proposed that the modulation of the gut microbiota (especially SUFA producing bacteria) by berberine might be a contributing factor for the enhanced growth performance of fish. Tan et al. (2022) hypothesized that the hepatic ribosome biogenesis might be the main reason accounting for improved growth performance of yellow drum fed berberine supplemented soybean oil-based diet, since ribosome biogenesis in eukaryotes was the most significantly enriched-KEGG pathway based on RNA sequencing. Largemouth bass fed 1000 mg/kg and 2000 mg/kg berberine for eight weeks obtained significantly improved growth performance, while 500 mg/kg did not significantly influence growth of fish (Xia et al., 2022b). Nevertheless, a nonsignificant effect on growth performance was reported in different species: black sea bream fed 50 mg/kg berberine supplemented in normal, high starch, and HL diets (Wang et al., 2020a; Wang et al., 2021); blunt snout bream fed a 50 mg/kg berberine supplemented HF diet (Xu et al., 2017; Yu et al., 2020) and a 50 mg/kg berberine supplemented HC diet (He et al., 2021); largemouth bass fed the 100 mg/kg and 400 mg/kg berberine supplemented HC diet (Chen et al., 2022), and grass carp (Ctenopharyngodon idella) fed a 30 mg/kg berberine supplemented normal diet (Pan et al., 2019). While studies in rats and humans revealed that chronic administration of berberine caused growth inhibition due to increased energy expenditure, alleviated growth of adipose tissue, and prevented obesity (Lee et al., 2006; Kim et al., 2009; Hu et al., 2012; Ilyas et al., 2020). Thus, the growth promotion effect of berberine may vary with fish species, basal diet composition, feeding mode, and other factors yet to be determined.
Lipid metabolism
The liver is the main organ for nutrient metabolism, and it also plays an important role in plasma protein production, bile synthesis, degradation of toxins, as well as lipid and glucose homeostasis in the body (Zhou et al., 2021). Liver diseases normally occur in fish partially because of excessive lipid and/or carbohydrate in the diet. Berberine was demonstrated to alleviate excessive hepatic lipid accumulation and pathologic changes caused by HL and HC diets in black sea bream (Wang et al., 2020a; Wang et al., 2021), blunt snout bream (Lu et al., 2017; Zhou et al., 2019; He et al., 2021), and zebrafish (Chen et al., 2020). Zhou et al. (2019) reported that HF diet caused excessive glycogen droplets, stricture of the hepatic sinus, inordinate and obscure hepatic cords, irregular arrangement of hepatocytes, diffused lipid vacuolization, large and electron-dense fat droplets in the liver of blunt snout bream, while 50 and 100 mg/kg berberine supplementation alleviated these hepatic abnormalities. Moreover, increased mitochondria density and repaired mitochondrial respiratory chain in hepatocytes by berberine inclusion were reported in blunt snout bream (Lu et al., 2017) and black sea bream (Wang et al., 2020a), which might be related to the improved liver energy expenditure and/or maintenance of mitochondria function. Besides, increased serum TG and TC contents are signs of liver dysfunction and steatosis and are regarded as poor health condition symbols (Wang et al., 2021). Reduced serum TG and TC contents by berberine treatment were reported in grass carp (Pan et al., 2019), zebrafish (Han et al., 2015), and blunt snout bream (He et al., 2021). The mechanisms of berberine alleviating hepatic lipid accumulation in fish are similar to those found in other animals, mainly by increasing lipid oxidation and transportation, reducing lipogenesis and fatty acids uptake. Berberine was demonstrated to downregulate hepatic lipogenesis genes, such as acetyl-CoA carboxylase α (ACCα), SREBP-1, 6-phosphogluconate dehydrogenase (6PGD), glucose 6-phosphate dehydrogenase (G6PD), and peroxisome proliferator-activated receptors γ (PPARγ) expressions in black sea bream (Wang et al., 2021); and hepatic FAS and ACCα expressions in blunt snout bream (He et al., 2021). In addition, berberine upregulated the expressions of hepatic lipid β-oxidation genes, such as carnitine palmitoyltransferase 1a (CPT1a) and hormone-sensitive lipase (HSL) expressions in black sea bream (Wang et al., 2021); CPT 1 in blunt snout bream (He et al., 2021); CPT 1 and PPARα in blunt snout bream (Lu et al., 2016); as well as Acyl-CoA oxidase (AOX), apolipoprotein B (ApoB), Apolipoprotein E (ApoE), peroxisome proliferator-activated receptor-gamma coactivator-1α (PGC-1α) and peroxisome proliferator-activated receptor alpha (PPARα) in blunt snout bream (Zhou et al., 2019). Furthermore, the fatty acid uptake and transportation genes including fatty acid transport protein (FATP), lipoprotein lipase (LPL), and low-density lipoprotein receptor (LDLR) were all upregulated by berberine supplementation in blunt snout bream (Zhou et al., 2019). However, some hepatoprotective mechanisms have not been reported in fish studies, such as if berberine can stabilize LDLR mRNA and regulate intestinal microbiota thus affecting fatty acid uptake.
Glucose metabolism
It is well known that fish show prolonged hyperglycemia after ingestion of HC diet, which is due to limited insulin secretion, low amount of insulin receptors in muscle, poor ability to use glucose in white muscle, endogenous glucose production, and low ability of lipogenesis from glucose (Kamalam et al., 2017). Therefore, fish exposed to HC diet may cause metabolic disorders and eventually threaten health status (He et al., 2021). Berberine supplementation in the HC diets reduced serum glucose in grass carp (Pan et al., 2019), black sea bream (Wang et al., 2020a), largemouth bass (Chen et al., 2022; Xia et al., 2022a; Xia et al., 2022b), and blunt snout bream (He et al., 2021). He et al. (2021) investigated the mechanisms of berberine regulating glucose metabolism in the liver. Berberine upregulated hepatic insulin receptor substrate (IRS), phosphatidylinositol 3-kinase (PI3K), and protein kinase B (AKT) expressions, but downregulated forkhead transcription factor 1 (Foxo1) expression. The IRS/PI3K/AKT pathway is a major insulin signaling pathway, indicating that berberine activates the insulin pathway to reduce plasma glucose. The similar mechanism was also reported in largemouth bass fed 100 mg/kg and 400 mg/kg berberine supplemented HC diet (Chen et al., 2022). Besides, berberine reduced the expressions of hepatic gluconeogenesis genes: PEPCK, G6Pase, and glycogen synthase (GS), but upregulated pyruvate kinase (PK), and glucose transporter 2 (GLUT2) expressions. In largemouth bass also reported that berberine improved hepatic glycolytic enzymes activities and gene expression (HK (hexokinase) and PK), whereas gluconeogenic enzymes (G6Pase and PEPCK) activities and gene expression were reduced (Xia et al., 2022b). Thus, berberine inhibited hepatic gluconeogenesis and glycogen synthesis, activated insulin signaling, and promoted glucose transport and glycolysis, which in all contributed to reduced plasma glucose, liver glycogen, and lipid contents of some fish species.
Antioxidative and immune status
Berberine also shows strong antioxidant activity in fish. Chen et al. (2016) observed that 50 mg/kg berberine supplementation in a HL diet reduced hepatic malondialdehyde (MDA), lipid peroxide (LPO), and protein carbonyl (PC) contents of blunt snout bream through elevating superoxide dismutase (SOD) activity and total sulfhydryl (T-SH) levels. Another study in blunt snout bream found that HF (15% fat) diet damaged mitochondria normal structure and induced more ROS production in the liver, while berberine supplementation reduced hepatic MDA content along with increased glutathione (GSH) activity via upregulating Sirtuin 3 expression and increasing the complex I and II activities (Lu et al., 2017). Xu et al. (2017) also reported that berberine (50 mg/kg) supplementation in the normal and HF diets of blunt snout bream improved hepatic CAT and SOD activities, with a decreased MDA content, a similar result was found in largemouth bass fed the berberine-supplemented high starch diets (Xia et al., 2022b). Besides, berberine supplementation in a high starch diet of black sea bream increased hepatic GPx activity, and reduced MDA content after the ammonia challenge (Wang et al., 2020a). Chen et al. (2020) demonstrated that berberine could eliminate hepatic ROS production and reduce DNA damage caused by the high cholesterol diet of zebrafish. Chen et al. (2022) reported that berberine carried out its antioxidative capacity in largemouth bass through the Nrf2/Keap1 pathway, which is the same as that found in mammals. Up to now, the specific mechanisms of the antioxidative effect of berberine remain to be better illustrated in fish, especially the pathways (e.g., NF-κB and Nrf2) found in mammals.
Zhou et al. (2016) reported that common carp (Cyprinus carpio) fed a 0.78 g/kg berberine supplemented diet for 21 days significantly increased respiratory burst, lysozyme (LYZ), myeloperoxidase, and phagocytic activities, as well as serum complement C3 level. Besides, berberine inclusion downregulated IL-10 expression but upregulated the TNF-α, IL-1β, lysozyme-c, and C3 expressions in the head kidney. The survival rate of fish infected with Aeromonas hydrophila was also enhanced by berberine supplementation. These results indicated that short-term berberine ingestion could enhance non-specific immunity and disease resistance ability, which might account for its immunomodulatory property. Likewise, plasma LYZ, acid phosphatase (ACP) activities, and alternative complement C3 and C4 contents were all improved by 50 mg/kg berberine supplementation in a HF diet of blunt snout bream (Chen et al., 2016). Berberine supplementation also reduced soybean oil-induced hepatic proinflammatory response mainly by decreasing cytokine–cytokine receptor interaction pathway-related genes expression in yellow drum (Tan et al., 2022). However, Xu et al. (2017) found the immune parameters (ACP, LYZ, C3, and C4) of blunt snout bream fed the 50 mg/kg berberine supplemented normal and HF diets at 2-week and 4-week interval feeding modes were similar or even higher than that of the continuous feeding mode. In addition, after being challenged by Aeromonas hydrophila, the cumulative mortality of fish fed the normal diet with 50 mg/kg berberine inclusion at the 2-week interval feeding mode was the lowest, while the other treatments showed no significant difference. The authors proposed that feeding fish berberine supplemented diet for the long term might cause immunosuppression or immunity fatigue, just like some other immunostimulants (e.g., β-glucan (Bai et al., 2010) and fructooligosaccharide (Zhang et al., 2014)). Besides, Nile tilapia fed the 1, 3, 6, and 9 g/kg berberine supplemented normal diets for 8 weeks all showed enhanced skin mucus lysozyme and peroxidase activities, as well as improved serum lysozyme, peroxidase, alternative complement (ACH50), phagocytosis, and respiratory burst activities. While the 1g/kg berberine supplemented group obtained the best immune status, and the highest survival rate after Streptococcus agalactiae infection (Doan et al., 2020). These results indicate that low dietary berberine supplementation level and discontinuous feeding mode may be more beneficial for the immune status of fish. A study on black sea bream found that fish fed a 50 mg/kg berberine supplemented high starch diet continuously for 8 weeks did not influence serum C3, C4, and IgM contents, as well as LYZ activity (Wang et al., 2020a). Yu et al. (2020) also observed that plasma IgG and IgM contents of blunt snout bream were not affected by berberine supplementation in the HC or HF diet.
Berberine supplementation also benefits intestinal immune function. Yu et al. (2020) reported that berberine reduced intestinal TNF-α and IL-6 expressions upregulated by HF or HC diet in blunt snout bream, and it also upregulated intestinal major histocompatibility complex class gene expression. In addition, several in vitro studies revealed that berberine shows a strong ability against pathogenic bacteria in fish. Berberine hydrochloride effectively inhibited Escherichia coli, Aeromonas hydrophila, Vibrio vulnificus, Pseudomonas fluorescens, Edwardsiella ictaluri, and Streptococcus agalactiae, and it showed a synergistic bactericidal effect with enrofloxacin against these fish pathogenic bacteria (Zhang et al., 2010). Ji et al. (2012) found that pretreatment with berberine hydrochloride activated the complement system and significantly enhanced serum bactericidal activity of grass carp against Edwardsiella ictaluri.
Regulation of intestinal integrity and microbiota
Even though most studies in fish concerned the hepatoprotective effect of berberine, several studies investigated the effects of berberine supplementation on intestinal health and microbiota composition. Modulating of intestinal microbiota may be the main mechanism of regulating energy mechanism and immune system by berberine since most ingested berberine remains in the intestine and may regulate intestinal microbiota composition and metabolism, as reported in mammals. Pan et al. (2019) reported that berberine supplementation in a normal diet increased intestinal microbiota diversity and decreased the Firmicutes to Bacteroidetes ratio of grass carp. Based on Spearman’s rank correlation, serum glucose content was negatively related to the 32 berberine-operational taxonomic units, indicating the glucose-lowering effect of berberine might be correlated with the regulation of intestinal microbiota composition. Berberine supplementation in the HC and normal diets improved intestinal microbiota diversity of blunt snout bream, whereas a reduced diversity and richness of intestinal microbiota were found in the berberine supplemented HF diet group (Yu et al., 2020). Moreover, berberine supplementation induced a high abundance of phyla Proteobacteria, Cyanobacteria, Firmicutes, and Bacteroidetes. It reduced intestinal Verrucomicrobia, Planctomycetes, Dependentiae, and Chloroflexi abundance increased by the HF diet, as well as reduced Verrucomicrobia, Planctomycetes, and Chloroflexi abundance elevated by the HC diet in blunt snout bream (Yu et al., 2020). Tian et al. (2022) demonstrated that the supplementation of Gly-β-MCA (an intestine-specific FXR inhibitor) alleviated the lipid-lowering effect of berberine of grass carp, which demonstrated intestinal FXR is the main working site of berberine. Similarly, berberine supplementation (500 mg/kg, 1000 mg/kg, and 2000mg/kg) significantly improved the expression levels of intestinal FXR and FGF-19 of largemouth bass, which led to improved glycolysis and inhibited gluconeogenesis (Xia et al., 2022a; Xia et al., 2022b). Currently, the bile acids-FXR axis has been demonstrated to regulate lipid and glucose metabolism, immune and antioxidative systems in many fish species (Du et al., 2018; Du et al., 2020; Du et al., 2021; Tian et al., 2021; Wen et al., 2021; Xu et al., 2022; Zhang et al., 2022). Thus, the modulation of intestinal bile acids metabolizing bacteria may account for the multiple functions of berberine in fish. Besides, berberine supplementation alleviated intestinal villus length reduction, intestinal integrity impairment, expanded lamina propria, decreased goblet cell count and mucosal folds, and elevated plasma diamine oxidase (DAO) and D-lactate (D-LA) contents caused by HF and HC diets in blunt snout bream (Yu et al., 2020). Modulation of intestinal microbiota by berberine is also related to nutrient utilization. Xia et al. (2022b) proposed that the enhanced intestinal digestive enzymes by berberine supplementation might be linked to the modulation of intestinal microbiota. Chen et al. (2022) reported that elevated intestinal abundance of Cetobacterium induced by berberine was contributing to the improved glucose utilization of largemouth bass.
Therefore, berberine supplementation is beneficial for maintaining intestinal structure and health, and its regulation of intestinal microbiota may be the important mechanism that exert various functions. And the relationship between berberine functions and its regulation of the specific intestinal microbiota is not clear enough.
Other applications
Recent studies have shown that dietary berberine decreased the cumulative mortality of blunt snout bream (Chen et al., 2016), and elevated the glucose level of black sea bream (Wang et al., 2020a) after acute ammonia challenge. These results might be due to improved liver and intestine health, as well as enhanced antioxidative and immune status of fish by berberine supplementation. Besides, injection of crucian carp (Carassius auratus gibelio) with berberine inhibited cytochrome P4501A (cyp1a) and cyp3a expression, which belong to the superfamily of monooxygenases, and play a vital role in drug metabolism in animal species. The authors proposed that when berberine is co-administrated with other drugs, it can reduce the dosage and increase the efficiency of other drugs, thus diminishing the pollution (Zhou et al., 2011). Besides, berberine also showed a strong antibacterial effect in fish via attenuating viral gene expression and host inflammatory response simultaneously. Berberine could inhibit cyprinid herpesvirus 2 (CyHV-2) replication and viral gene transcription in vitro, and single oral administration of berberine could against CyHV-2 infection in a dose-depend manner in crucian carp (Su et al., 2021). Su et al. (2022) reported that berberine could attenuate NF-κB signaling induced by acute CyHV-2 infection in crucian carp in a dosage-dependent manner, thus suppressing the expression of inflammatory cytokines.
Conclusion and perspectives
Berberine has potential lipid- and glucose-lowering, anti-inflammatory, antioxidant, antimicrobial, and anti-stress activities in animals. Based on human and animal studies of rodents, berberine may be a safe, effective, and relatively cheap feed additive in the fish diet to protect the liver and intestine, and maintain good health condition. However, berberine also has limitations such as low bioavailability, and negatively influences feed palatability. Indeed, a major risk of berberine supplementation could be its negative impact on feed intake and growth performance during prolonged periods. In addition, some human and rodents studies also revealed that berberine promotes energy expenditure thus reducing body weight eventually. This discrepancy result with the studies in fish also needs further investigation.
Further studies may focus on improving the bioavailability of berberine in the feed, which can learn from studies in vivo and other animals. For example, Shan et al. (2013b) found that pseudoberberine (IMB-Y53), a berberine analogue, has a much lower ability to increase intestinal P-gp expression than berberine, but it showed a strong glucose-lowering effect similar to berberine in rats. A study in rabbits revealed that administration of nanoparticles berberine led to higher berberine in blood circulation and higher pharmacokinetic parameters, as well as a better liver protection effect compared with regular berberine (Sahibzada et al., 2021). Besides, intestinal absorption promoters may be beneficial for intestinal permeability. For example, sodium caprate has been approved by the FDA as a food additive for humans (Liu et al., 2016). Moreover, P-gp inhibitors such as silymarin (Wei et al., 2020), and tetrandrine (Shan et al., 2013b) were demonstrated to improve intestinal berberine absorption significantly. Additionally, using lipid microparticles to transport berberine could increase its solubility and permeability in the gastrointestinal tract, and enhance transportation to the lymphatic system (Liu et al., 2016). On the other side, many studies indicate that the main mechanism of berberine exerts its multiple functions is by modulating intestinal microbiota since most ingested berberine is highly accumulated in the intestine, as reviewed by Habtemariam (2020). Thus, the hidden link between berberine and intestinal microbiota may be a hot topic in the future.
The hepatoprotective effect of berberine has been well demonstrated in some fish species. Further studies should concern the effective and economical dietary berberine level to cope with different dietary levels of lipid and carbohydrate in different fish species. Berberine has strong antioxidant and immune regulation properties, which are beneficial for fish species under intensive aquaculture systems. However, long-term berberine feeding or high dietary berberine level may cause immune fatigue of immunosuppression. Thus, optimal dietary berberine levels with suitable feeding modes for different fish species deserve further study. Besides, most fish have limited ability to use dietary carbohydrates, especially carnivorous fishes, which are considered to have poorer ability than herbivorous and omnivorous fishes because of their slower blood glucose clearance and poorer intestinal glucose uptake rate (Kamalam et al., 2017). Previous studies have proved that berberine could improve insulin secretion, accelerate blood glucose clearance, and enhance glucose utilization. However, the beneficial effect of berberine supplementation on improving dietary carbohydrate utilization was only reported in blunt snout bream (herbivorous fish). Further research is needed about berberine on carnivorous and omnivorous fish.
From the economical aspect, supplementation of purified berberine may induce more economic costs for feed companies. However, berberine-enriched plant organs, such as Forsythia suspensa extract (Zhang et al., 2013), barberry root (Ramezanzadeh et al., 2020; Ramezanzadeh et al., 2021), barberry fruit (Shekarabi et al., 2022), fibrous root of Rhizoma Coptidis (Zhou et al., 2016), also showed considerable beneficial effects for animals and can be an economical choice.
The current studies of berberine are mainly conducted in rodents and humans. Though it shows excellent features in treating some diseases, there is still a long way for it to be widely applied in fish feed due to the limited studies. The application of herbal components is drawing more and more attention, and berberine is a promising candidate for fish feed additive in the future.
Author contributions
All authors have read and agreed to the published version of the manuscript. LW wrote the paper under the supervision of QS and YY; CG, BW, and CW helped collect data. GS revised the manuscript. All authors contributed to the article and approved the submitted version.
Funding
This work was funded by the National Natural Science Foundation of China (No.31872251), the Special Fund for Anhui Agriculture Research System (Anhui Agricultural Science letter No. (2021)711), and the Natural Science Foundation of Universities of Anhui Province (Project No: KJ2021A0105).
Conflict of interest
The authors declare that the research was conducted in the absence of any commercial or financial relationships that could be construed as a potential conflict of interest.
Publisher’s note
All claims expressed in this article are solely those of the authors and do not necessarily represent those of their affiliated organizations, or those of the publisher, the editors and the reviewers. Any product that may be evaluated in this article, or claim that may be made by its manufacturer, is not guaranteed or endorsed by the publisher.
References
Abasubong K. P., Li X.-F., Zhang D.-D., Jia E.-T., Xiang-Yang Y., Xu C., et al. (2018). Dietary supplementation of xylooligosaccharides benefits the growth performance and lipid metabolism of common carp (Cyprinus carpio) fed high-fat diets. Aquacult. Nutr. 24, 1416–1424. doi: 10.1111/anu.12678
Abdel-Latif H. M. R., Soliman A. A., Sewilam H., Almeer R., Van Doan H., Alagawany M., et al. (2020). The influence of raffinose on the growth performance, oxidative status, and immunity in Nile tilapia (Oreochromis niloticus). Aquacult. Rep. 18, 100457. doi: 10.1016/j.aqrep.2020.100457
Ai Q., Mai K., Li H., Zhang C., Zhang L., Duan Q., et al. (2004). Effects of dietary protein to energy ratios on growth and body composition of juvenile Japanese seabass, Lateolabrax japonicus. Aquaculture 230, 507–516. doi: 10.1016/j.aquaculture.2003.09.040
Alagawany M., Farag M. R., Abdelnour S. A., Dawood M. A. O., Elnesr S. S., Dhama K. (2021). Curcumin and its different forms: A review on fish nutrition. Aquaculture 532, 736030. doi: 10.1016/j.aquaculture.2020.736030
Bai N., Zhang W., Mai K., Wang X., Xu W., Ma H. (2010). Effects of discontinuous administration of β-glucan and glycyrrhizin on the growth and immunity of white shrimp Litopenaeus vannamei. Aquaculture 306, 218–224. doi: 10.1016/j.aquaculture.2010.06.017
Bao X., Chen M., Yue Y., Liu H., Yang Y., Yu H., et al. (2022). Effects of dietary nano-curcumin supplementation on growth performance, glucose metabolism, and endoplasmic reticulum stress in juvenile largemouth bass, Micropterus salmoides. Front. Mar. Sci. 9. doi: 10.3389/fmars.2022.924569
Benet L. Z., Izumi T., Zhang Y., Silverman J. A., Wacher V. J. (1999). Intestinal MDR transport proteins and p-450 enzymes as barriers to oral drug delivery. J. Controlled Release. 62, 25–31. doi: 10.1016/S0168-3659(99)00034-6
Cao X.-F., Liu W.-B., Zheng X.-C., Yuan X.-Y., Wang C.-C., Jiang G.-Z. (2019). Effects of high-fat diets on growth performance, endoplasmic reticulum stress and mitochondrial damage in blunt snout bream Megalobrama amblycephala. aquacult. Nutr. 25, 97–109. doi: 10.1111/anu.12834
Cao Y., Pan Q., Cai W., Shen F., Chen G. Y., Xu L. M., et al. (2016). Modulation of gut microbiota by berberine improves steatohepatitis in high-fat diet-fed BALB/C mice. Arch. Iran. Med. 19, 197–203. doi: 0161903/AIM.008
Čerňáková M., Košťálová D. (2002). Antimicrobial activity of berberine–a constituent of Mahonia aquifolium. folia. Microbiol 47, 375–378. doi: 10.1007/BF02818693
Chen S., Jiang X., Liu N., Ren M., Wang Z., Li M., et al. (2022). Effects of dietary berberine hydrochloride inclusion on growth, antioxidant capacity, glucose metabolism and intestinal microbiome of largemouth bass (Micropterus salmoides). Aquaculture 552, 738023. doi: 10.1016/j.aquaculture.2022.738023
Chen Q.-Q., Liu W.-B., Zhou M., Dai Y.-J., Xu C., Tian H.-Y., et al. (2016). Effects of berberine on the growth and immune performance in response to ammonia stress and high-fat dietary in blunt snout bream Megalobrama amblycephala. Fish Shellfish Immunol. 55, 165–172. doi: 10.1016/j.fsi.2016.05.023
Chen C., Yu Z., Li Y., Fichna J., Storr M. (2014). Effects of berberine in the gastrointestinal tract — a review of actions and therapeutic implications. Am. J. Chin. Med. 42, 1053–1070. doi: 10.1142/S0192415X14500669
Chen B., Zheng Y.-M., Zhang M.-Q., Han Y., Zhang J.-P., Hu C.-Q. (2020). Microarray expression profiling and raman spectroscopy reveal anti-fatty liver action of berberine in a diet-induced larval zebrafish model. Front. Pharmacol. 10, 1504. doi: 10.3389/fphar.2019.01504
Di Pierro F., Putignano P., Villanova N., Montesi L., Moscatiello S., Marchesini G. (2013). Preliminary study about the possible glycemic clinical advantage in using a fixed combination of Berberis aristata and Silybum marianum standardized extracts versus only Berberis aristata in patients with type 2 diabetes. Clin. Pharm. Adv. Appli. 5, 167–174. doi: 10.2147/CPAA.S54308
Doan H. V., Hoseinifar S. H., Jaturasitha S., Dawood M. A. O., Harikrishnan R. (2020). The effects of berberine powder supplementation on growth performance, skin mucus immune response, serum immunity, and disease resistance of Nile tilapia (Oreochromis niloticus) fingerlings. Aquaculture 520, 734927. doi: 10.1016/j.aquaculture.2020.734927
Du J., Chen Q., Li Y., Xiang X., Xu W., Mai K., et al. (2020). Activation of the farnesoid X receptor (FXR) suppresses linoleic acid-induced inflammation in the large yellow croaker (Larimichthys crocea). J. Nutr. 150, 2469–2477. doi: 10.1093/jn/nxaa185
Du J., Xiang X., Li Y., Ji R., Xu H., Mai K., et al. (2018). Molecular cloning and characterization of farnesoid X receptor from large yellow croaker (Larimichthys crocea) and the effect of dietary CDCA on the expression of inflammatory genes in intestine and spleen. Comp. Biochem. Physiol.B Biochem. Mol. Bio. 216, 10–17. doi: 10.1016/j.cbpb.2017.09.007
Du J., Xiang X., Xu D., Zhang J., Fang W., Xu W., et al. (2021). FXR, a key regulator of lipid metabolism, is inhibited by ER stress-mediated activation of JNK and p38 MAPK in large yellow croakers (Larimichthys crocea) fed high fat diets. Nutrients 13, 4343. doi: 10.3390/nu13124343
FAO (2018). The state of world fisheries and aquaculture 2018-meeting the sustainable development goals. fisheries and aquaculture department, food and agriculture organization of the united nations, Rome.
Feng R., Shou J.-W., Zhao Z.-X., He C.-Y., Ma C., Huang M., et al. (2015). Transforming berberine into its intestine-absorbable form by the gut microbiota. Sci. Rep. 5, 12155. doi: 10.1038/srep12155
Fernandez C. P., Afrin F., Flores R. A., Kim W. H., Jeong J., Kim S., et al. (2017). Downregulation of inflammatory cytokines by berberine attenuates Riemerella anatipestifer infection in ducks. Dev. Comp. Immunol. 77, 121–127. doi: 10.1016/j.dci.2017.07.027
Fratter A., Servi B. (2015). New oral delivery system to improve absorption of berberine: Likely interaction of cationized chitosan with PG-p pump. Int. J. Drug Deliv. 5, 33–42.
Gangwar R. K., Tomar G. B., Dhumale V. A., Zinjarde S., Sharma R. B., Datar S. (2013). Curcumin conjugated silica nanoparticles for improving bioavailability and its anticancer applications. J. Agric. Food Chem. 61, 9632–9637. doi: 10.1021/jf402894x
Gomes A. P., Duarte F. V., Nunes P., Hubbard B. P., Teodoro J. S., Varela A. T., et al. (2012). Berberine protects against high fat diet-induced dysfunction in muscle mitochondria by inducing SIRT1-dependent mitochondrial biogenesis. BBA Mole. Basis Dis. 1822, 185–195. doi: 10.1016/j.bbadis.2011.10.008
Gu S., Cao B., Sun R., Tang Y., Paletta J. L., Wu X., et al. (2015). A metabolomic and pharmacokinetic study on the mechanism underlying the lipid-lowering effect of orally administered berberine. Mol. Biosyst. 11, 463–474. doi: 10.1039/C4MB00500G
Gu L., Li N., Gong J., Li Q., Zhu W., Li J. (2011). Berberine ameliorates intestinal epithelial tight-junction damage and down-regulates myosin light chain kinase pathways in a mouse model of endotoxinemia. J. Infect. Dis. 203, 1602–1612. doi: 10.1093/infdis/jir147
Habtemariam S. (2020). Berberine pharmacology and the gut microbiota: A hidden therapeutic link. Pharmacol. Res. 155, 104722. doi: 10.1016/j.phrs.2020.104722
Han B., Kou S.-M., Chen B., Peng Y.-Z., Wang Y., Han Y.-L., et al. (2015). Efficient and rapid liquid reduction animal model. China J. Chin. Mate. Med. 40, 4446–4451. doi: 10.4268/cjcmm20152223
He C., Jia X., Zhang L., Gao F., Jiang W., Wen C., et al. (2021). Dietary berberine can ameliorate glucose metabolism disorder of Megalobrama amblycephala exposed to a high-carbohydrate diet. Fish Physiol. Biochem. 47, 499–513. doi: 10.1007/s10695-021-00927-8
Hou Q., Zhu S., Zhang C., Huang Y., Guo Y., Li P., et al. (2019). Berberine improves intestinal epithelial tight junctions by upregulating A20 expression in IBS-d mice. Biomed. Pharmacother. 118, 109206. doi: 10.1016/j.biopha.2019.109206
Hu Y., Ehli E. A., Kittelsrud J., Ronan P. J., Munger K., Downey T., et al. (2012). Lipid-lowering effect of berberine in human subjects and rats. Phytomedicine 19, 861–867. doi: 10.1016/j.phymed.2012.05.009
Hur J.-M., Hyun M.-S., Lim S.-Y., Lee W.-Y., Kim D. (2009). The combination of berberine and irradiation enhances anti-cancer effects via activation of p38 MAPK pathway and ROS generation in human hepatoma cells. J. Cell. Biochem. 107, 955–964. doi: 10.1002/jcb.22198
Ilyas Z., Perna S., Al-thawadi S., Alalwan T. A., Riva A., Petrangolini G., et al. (2020). The effect of berberine on weight loss in order to prevent obesity: A systematic review. Biomed. Pharmacother. 127, 110137. doi: 10.1016/j.biopha.2020.110137
Imenshahidi M., Hosseinzadeh H. (2016). Berberis vulgaris and berberine: An update review. Phytother. Res. 30, 1745–1764. doi: 10.1002/ptr.5693
Jeong H. W., Hsu K. C., Lee J.-W., Ham M., Huh J. Y., Shin H. J., et al. (2009). Berberine suppresses proinflammatory responses through AMPK activation in macrophages. Am. J. Physiology-Endocrinology Metab. 296, E955–E964. doi: 10.1152/ajpendo.90599.2008
Jiang S.-J., Dong H., Li J.-B., Xu L.-J., Zou X., Wang K.-F., et al. (2015). Berberine inhibits hepatic gluconeogenesis via the LKB1-AMPK-TORC2 signaling pathway in streptozotocin-induced diabetic rats. World J. Gastroenterol. 21, 7777–7785. doi: 10.3748/wjg.v21.i25.7777
Ji C., Zhang D. F., Li A. H., Gong X. N. (2012). Effect of berberine hydrochloride on grass carp Ctenopharyngodon idella serum bactericidal activity against Edwardsiella ictaluri. Fish Shellfish Immunol. 33, 143–145. doi: 10.1016/j.fsi.2012.03.022
Jumpertz R., Le D. S., Turnbaugh P. J., Trinidad C., Bogardus C., Gordon J. I., et al. (2011). Energy-balance studies reveal associations between gut microbes, caloric load, and nutrient absorption in humans. Ame. J. Clin. Nutri. 94, 58–65. doi: 10.3945/ajcn.110.010132
Kamalam B. S., Medale F., Panserat S. (2017). Utilisation of dietary carbohydrates in farmed fishes: New insights on influencing factors, biological limitations and future strategies. Aquaculture 467, 3–27. doi: 10.1016/j.aquaculture.2016.02.007
Kheir M. M., Wang Y., Hua L., Hu J., Li L., Lei F., et al. (2010). Acute toxicity of berberine and its correlation with the blood concentration in mice. Food Chem. Toxicol. 48, 1105–1110. doi: 10.1016/j.fct.2010.01.033
Kiczorowska B., Samolińska W., Al-Yasiry A. R. M., Kiczorowski P., Winiarska-Mieczan A. (2017). The natural feed additives as immunostimulants in monogastric animal nutrition – a review. An. Ani. Sci. 17, 605–625. doi: 10.1515/aoas-2016-0076
Kim W. S., Lee Y. S., Cha S. H., Jeong H. W., Choe S. S., Lee M.-R., et al. (2009). Berberine improves lipid dysregulation in obesity by controlling central and peripheral AMPK activity. Ame. J. Physiol. Endocrinol. Met. 296, E812–E819. doi: 10.1152/ajpendo.90710.2008
Ko B. S., Choi S. B., Park S. K., Jang J. S., Kim Y. E., Park S. (2005). Insulin sensitizing and insulinotropic action of berberine from Cortidis rhizoma. biol. Pharm. Bull. 28, 1431–1437. doi: 10.1248/bpb.28.1431
Kong W., Wei J., Abidi P., Lin M., Inaba S., Li C., et al. (2004). Berberine is a novel cholesterol-lowering drug working through a unique mechanism distinct from statins. Nat. Med. 10, 1344–1351. doi: 10.1038/nm1135
Kumar A., Ekavali, Chopra K., Mukherjee M., Pottabathini R., Dhull D. K. (2015). Current knowledge and pharmacological profile of berberine: An update. Eur. J. Pharmacol. 761, 288–297. doi: 10.1016/j.ejphar.2015.05.068
Lee Y. S., Kim W. S., Kim K. H., Yoon M. J., Cho H. J., Shen Y., et al. (2006). Berberine, a natural plant product, activates AMP-activated protein kinase with beneficial metabolic effects in diabetic and insulin-resistant states. Diabetes 55, 2256. doi: 10.2337/db06-0006
Lin S.-M., Shi C.-M., Mu M.-M., Chen Y.-J., Luo L. (2018). Effect of high dietary starch levels on growth, hepatic glucose metabolism, oxidative status and immune response of juvenile largemouth bass, Micropterus salmoides. Fish Shellfish Immunol. 78, 121–126. doi: 10.1016/j.fsi.2018.04.046
Lin S.-M., Zhou X.-M., Zhou Y.-L., Kuang W.-M., Chen Y.-J., Luo L., et al. (2020). Intestinal morphology, immunity and microbiota response to dietary fibers in largemouth bass, Micropterus salmoide. Fish Shellfish Immunol. 103, 135–142. doi: 10.1016/j.fsi.2020.04.070
Liu Y.-T., Hao H.-P., Xie H.-G., Lai L., Wang Q., Liu C.-X., et al. (2010). Extensive intestinal first-pass elimination and predominant hepatic distribution of berberine explain its low plasma levels in rats. Drug Metab. Disposition. 38, 1779–1784. doi: 10.1124/dmd.110.033936
Liu C., Zheng Y., Zhang Y., Long X. (2016). Research progress on berberine with a special focus on its oral bioavailability. Fitoterapia 109, 274–282. doi: 10.1016/j.fitote.2016.02.001
Li Z., Wei Y., Chu T. (2005). Radioiodination, biodistribution and pharmacokinetics of berberine in mice. J. Radioanal. Nucle. Chem. 265, 355–359. doi: 10.1007/s10967-005-0832-4
Lu K., Wang L., Zhang D., Liu W., Xu W. (2017). Berberine attenuates oxidative stress and hepatocytes apoptosis via protecting mitochondria in blunt snout bream Megalobrama amblycephala fed high-fat diets. Fish Physiol. Biochem. 43, 65–76. doi: 10.1007/s10695-016-0268-5
Lu K.-L., Zhang D.-D., Wang L.-N., Xu W.-N., Liu W.-B. (2016). Molecular characterization of carnitine palmitoyltransferase IA in Megalobrama amblycephala and effects on its expression of feeding status and dietary lipid and berberine. Comp. Biochem. Physiol.B Biochem. Mol. Bio. 191, 20–25. doi: 10.1016/j.cbpb.2015.08.010
Maeng H.-J., Yoo H.-J., Kim I.-W., Song I.-S., Chung S.-J., Shim C.-K. (2002). P-glycoprotein–mediated transport of berberine across caco-2 cell monolayers. J. Pharm. Sci. 91, 2614–2621. doi: 10.1002/jps.10268
Nm J., Joseph A., Maliakel B., Im K. (2018). Dietary addition of a standardized extract of turmeric (TurmaFEEDTM) improves growth performance and carcass quality of broilers. J. Ani. Sci. Technol. 60, 8. doi: 10.1186/s40781-018-0167-7
Pang B., Zhao L.-H., Zhou Q., Zhao T.-Y., Wang H., Gu C.-J., et al. (2015)2015. Application of berberine on treating type 2 diabetes mellitus. Int. J. Endocrinol. 905749. doi: 10.1155/2015/905749
Pan H., Li Z., Xie J., Liu D., Wang H., Yu D., et al. (2019). Berberine influences blood glucose via modulating the gut microbiome in gass carp. Front. Microbiol. 10, 1066. doi: 10.3389/fmicb.2019.01066
Pirillo A., Catapano A. L. (2015). Berberine, a plant alkaloid with lipid- and glucose-lowering properties: From in vitro evidence to clinical studies. Atherosclerosis 243, 449–461. doi: 10.1016/j.atherosclerosis.2015.09.032
Qin Q. (2014). Studies on pharmacokinetics and residues of berberine hydrochloride in tilapia (Oreochromis niloticus) (Zhanjiang: Guangdong Ocean University Zhanjiang).
Rad S. Z. K., Rameshrad M., Hosseinzadeh H. (2017). Toxicology effects of Berberis vulgaris (barberry) and its active constituent, berberine: A review. Iran. J. Basic Med. Sci. 20, 516–529. doi: 10.22038/IJBMS.2017.8676
Ramezanzadeh S., Abedian Kenari A., Esmaeili M. (2020). Immunohematological parameters of rainbow trout (Oncorhynchus mykiss) fed supplemented diet with different forms of barberry root (Berberis vulgaris). Comp. Clin. Pathol. 29, 177–187. doi: 10.1007/s00580-019-03032-8
Ramezanzadeh S., Abedian Kenari A., Esmaeili M., Rombenso A. (2021). Effects of different forms of barberry root (Berberis vulgaris) on growth performance, muscle fatty acids profile, whole-body composition, and digestive enzymes of rainbow trout (Oncorhynchus mykiss). J. World Aquacult. Soc 52, 284–302. doi: 10.1111/jwas.12722
Sahibzada M. U. K., Zahoor M., Sadiq A., Ur Rehman F., Al-Mohaimeed A. M., Shahid M., et al. (2021). Bioavailability and hepatoprotection enhancement of berberine and its nanoparticles prepared by liquid antisolvent method. Saudi J. Biol. Sci. 28, 327–332. doi: 10.1016/j.sjbs.2020.10.006
Sarna L. K., Wu N., Hwang S.-Y., Siow Y. L., O K. (2010). Berberine inhibits NADPH oxidase mediated superoxide anion production in macrophages. Can. J. Physiol. Pharmacol. 88, 369–378. doi: 10.1139/Y09-136
Shan W.-J., Huang L., Zhou Q., Meng F.-C., Li X.-S. (2011). Synthesis, biological evaluation of 9-n-substituted berberine derivatives as multi-functional agents of antioxidant, inhibitors of acetylcholinesterase, butyrylcholinesterase and amyloid-β aggregation. Eur. J. Med. Chem. 46, 5885–5893. doi: 10.1016/j.ejmech.2011.09.051
Shan Y.-Q., Ren G., Wang Y.-X., Pang J., Zhao Z.-Y., Yao J., et al. (2013b). Berberine analogue IMB-Y53 improves glucose-lowering efficacy by averting cellular efflux especially p-glycoprotein efflux. Metabolism 62, 446–456. doi: 10.1016/j.metabol.2012.09.009
Shan Y.-Q., Zhu Y.-P., Pang J., Wang Y.-X., Song D.-Q., Kong W.-J., et al. (2013a). Tetrandrine potentiates the hypoglycemic efficacy of berberine by inhibiting p-glycoprotein function. Biol. Pharm. Bull. 36, 1562–1569. doi: 10.1248/bpb.b13-00272
Shekarabi S. P. H., Mehrgan M. S., Ramezani F., Dawood M. A. O., Van Doan H., Moonmanee T., et al. (2022). Effect of dietary barberry fruit (Berberis vulgaris) extract on immune function, antioxidant capacity, antibacterial activity, and stress-related gene expression of Siberian sturgeon (Acipenser baerii). Aquacult. Rep. 23, 101041. doi: 10.1016/j.aqrep.2022.101041
Shitan N., Tanaka M., Terai K., Ueda K., Yazaki K. (2007). Human MDR1 and MRP1 recognize berberine as their transport substrate. Biosci. Biotechnol. Biochem. 71, 242–245. doi: 10.1271/bbb.60441
Shi H.-J., Xu C., Liu M.-Y., Wang B.-K., Liu W.-B., Chen D.-H., et al. (2018). Resveratrol improves the energy sensing and glycolipid metabolism of blunt snout bream Megalobrama amblycephala fed high-carbohydrate diets by activating the AMPK–SIRT1–PGC-1α network. Front. Physiol. 9, 1258. doi: 10.3389/fphys.2018.01258
Siow Y. L., Sarna L., O K. (2011). Redox regulation in health and disease — therapeutic potential of berberine. Food Res. Int. 44, 2409–2417. doi: 10.1016/j.foodres.2010.12.038
Spinozzi S., Colliva C., Camborata C., Roberti M., Ianni C., Neri F., et al. (2014). Berberine and its metabolites: Relationship between physicochemical properties and plasma levels after administration to human subjects. J. Nat. Prod. 77, 766–772. doi: 10.1021/np400607k
Su M., Lu C., Tang R., Zhang X., Lu L. (2022). Downregulation of NF-кB signaling is involved in berberine-mediated protection of crucian carp (Carassius auratus gibelio) from cyprinid herpesvirus 2 infection. Aquaculture 548, 737713. doi: 10.1016/j.aquaculture.2021.737713
Sun H., Wang N., Cang Z., Zhu C., Zhao L., Nie X., et al. (2016). Modulation of microbiota-gut-brain axis by berberine resulting in improved metabolic status in high-fat diet-fed rats. Obes. Facts. 9, 365–378. doi: 10.1159/000449507
Sun R., Yang N., Kong B., Cao B., Feng D., Yu X., et al. (2017). Orally administered berberine modulates hepatic lipid metabolism by altering microbial bile acid metabolism and the intestinal FXR signaling pathway. Mol. Pharmacol. 91, 110–122. doi: 10.1124/mol.116.106617
Su M., Tang R., Wang H., Lu L. (2021). Suppression effect of plant-derived berberine on cyprinid herpesvirus 2 proliferation and its pharmacokinetics in crucian carp (Carassius auratus gibelio). Antiviral Res. 186, 105000. doi: 10.1016/j.antiviral.2020.105000
Tadese D. A., Song C., Sun C., Liu B., Liu B., Zhou Q., et al. (2022). The role of currently used medicinal plants in aquaculture and their action mechanisms: A review. Rev. Aquac. 14, 816–847. doi: 10.1111/raq.12626
Tang L.-Q., Wei W., Chen L.-M., Liu S. (2006). Effects of berberine on diabetes induced by alloxan and a high-fat/high-cholesterol diet in rats. J. Ethnopharmacol. 108, 109–115. doi: 10.1016/j.jep.2006.04.019
Tan P., Wang L., Chen R., Xu D. (2022). Berberine chloride supplementation ameliorates excessive hepatic lipid deposition and proinflammatory gene upregulation in the soybean-oil-based diet of juvenile yellow drum (Nibea albiflora). Aquacult. Nutr. 2022, 1–14. doi: 10.1155/2022/8690138
Tian J., Jin Y., Yu E., Sun J., Xia Y., Zhang K., et al. (2021). Farnesoid X receptor is an effective target for modulating lipid accumulation in grass carp, Ctenopharyngodon idella. Aquaculture 534, 736248. doi: 10.1016/j.aquaculture.2020.736248
Tian J.-J., Jin Y.-Q., Yu E.-M., Sun J.-H., Xia Y., Zhang K., et al. (2022). Intestinal farnesoid X receptor mediates the effect of dietary berberine on lipid accumulation in grass carp (Ctenopharyngodon idella). Aquaculture 553, 738055. doi: 10.1016/j.aquaculture.2022.738055
Wang Y., Shou J. W., Li X. Y., Zhao Z. X., Fu J., He C. Y., et al. (2017). Berberine-induced bioactive metabolites of the gut microbiota improve energy metabolism. Met. Clin. Experim. 70, 72–84. doi: 10.1016/j.metabol.2017.02.003
Wang L., Sun Y., Xu B., Sagada G., Chen K., Xiao J., et al. (2020a). Effects of berberine supplementation in high starch diet on growth performance, antioxidative status, immune parameters and ammonia stress response of fingerling black sea bream (Acanthopagrus schlegelii). Aquaculture 527, 735473. doi: 10.1016/j.aquaculture.2020.735473
Wang L., Xu B., Sagada G., Ng W. K., Chen K., Zhang J., et al. (2021). Dietary berberine regulates lipid metabolism in muscle and liver of black sea bream (Acanthopagrus schlegelii) fed normal or high-lipid diets. Br. J. Nutr. 125, 481–493. doi: 10.1017/S0007114520003025
Wang L., Zhang W., Gladstone S., Ng W.-K., Zhang J., Shao Q. (2019). Effects of isoenergetic diets with varying protein and lipid levels on the growth, feed utilization, metabolic enzymes activities, antioxidative status and serum biochemical parameters of black sea bream (Acanthopagrus schlegelii). Aquaculture 513, 734397. doi: 10.1016/j.aquaculture.2019.734397
Wang Y., Zheng J., Hou H., Zhang J., Qi S., Li Y., et al. (2020b). Effects of berberine on intestinal flora of non-alcoholic fatty liver induced by high-fat diet through 16S rRNA gene segmentation. J. King Saud Uni. Sci. 32, 2603–2609. doi: 10.1016/j.jksus.2020.04.020
Wei L., Wu P., Zhou X.-Q., Jiang W.-D., Liu Y., Kuang S.-Y., et al. (2020). Dietary silymarin supplementation enhanced growth performance and improved intestinal apical junctional complex on juvenile grass carp (Ctenopharyngodon idella). Aquaculture 525, 735311. doi: 10.1016/j.aquaculture.2020.735311
Wen J., Mercado G. P., Volland A., Doden H. L., Lickwar C. R., Crooks T., et al. (2021). Fxr signaling and microbial metabolism of bile salts in the zebrafish intestine. Sci. Adv. 7, eabg1371. doi: 10.1126/sciadv.abg1371
Wu C., Ye J., Gao J. E., Chen L., Lu Z. (2016). The effects of dietary carbohydrate on the growth, antioxidant capacities, innate immune responses and pathogen resistance of juvenile black carp Mylopharyngodon piceus. Fish Shellfish Immunol. 49, 132–142. doi: 10.1016/j.fsi.2015.12.030
Xia Y., Wang Y., Xie J., Yu E., Tian J., Li Z., et al. (2022b). Effects of BBR on growth performance, serum and hepatic biochemistry parameters, hepatic morphology and gene expression levels related to glucose metabolism in largemouth bass, Micropterus salmoides. Aquacult. Res. 53, 3807-3817. doi: 10.1111/are.15887
Xia Y., Wang G., Yu E., Tian J., Li Z., Zhang K., et al. (2022a). Addition of berberine to formulated feed changes the glucose utilisation, intestinal microbiota and serum metabolites of largemouth bass (Micropterus salmoides). Aquacult. Rep. 23, 101018. doi: 10.1016/j.aqrep.2022.101018
Xie W., Gu D., Li J., Cui K., Zhang Y. (2011). Effects and action mechanisms of berberine and Rhizoma coptidis on gut microbes and obesity in high-fat diet-fed C57BL/6J mice. PloS One 6, e24520. doi: 10.1371/journal.pone.0024520
Xing L.-J., Zhang L., Liu T., Hua Y.-Q., Zheng P.-Y., Ji G. (2011). Berberine reducing insulin resistance by up-regulating IRS-2 mRNA expression in nonalcoholic fatty liver disease (NAFLD) rat liver. Eur. J. Pharmacol. 668, 467–471. doi: 10.1016/j.ejphar.2011.07.036
Xu W.-N., Chen D.-H., Chen Q.-Q., Liu W.-B. (2017). Growth performance, innate immune responses and disease resistance of fingerling blunt snout bream, Megalobrama amblycephala adapted to different berberine-dietary feeding modes. Fish Shellfish Immunol. 68, 458–465. doi: 10.1016/j.fsi.2017.07.051
Xu J., He G., Chen L., Xie S., Chi S., Zhang S., et al. (2022). Farnesoid X receptor (FXR) and G protein-coupled bile acid receptor 1 (TGR5) signaling pathways improved the hepatic lipid metabolism in hybrid grouper. Aquacult. Rep. 22, 100997. doi: 10.1016/j.aqrep.2021.100997
Xu M., Xiao Y., Yin J., Hou W., Yu X., Shen L., et al. (2014). Berberine promotes glucose consumption independently of AMP-activated protein kinase activation. PloS One 9, e103702. doi: 10.1371/journal.pone.0103702
Xu X., Yi H., Wu J., Kuang T., Zhang J., Li Q., et al. (2021). Therapeutic effect of berberine on metabolic diseases: Both pharmacological data and clinical evidence. Biomed. Pharmacother. 133, 110984. doi: 10.1016/j.biopha.2020.110984
Yang Q.-H., Hu S.-P., Zhang Y.-P., Xie W.-N., Li N., Ji G.-Y., et al. (2011). Effect of berberine on expressions of uncoupling protein-2 mRNA and protein in hepatic tissue of non-alcoholic fatty liver disease in rats. Chin. J. Integr. Med. 17, 205–211. doi: 10.1007/s11655-011-0668-4
Yang J., Ma X. J., Li L., Wang L., Chen Y. G., Liu J., et al. (2017). Berberine ameliorates non−alcoholic steatohepatitis in ApoE-/- mice. Exp. Ther. Med. 14, 4134–4140. doi: 10.3892/etm.2017.5051
Yang S.-S., Yu C.-B., Luo Z., Luo W.-L., Zhang J., Xu J.-X., et al. (2019). Berberine attenuates sodium palmitate-induced lipid accumulation, oxidative stress and apoptosis in grass carp (Ctenopharyngodon idella) hepatocyte in vitro. Fish Shellfish Immunol. 88, 518–527. doi: 10.1016/j.fsi.2019.02.055
Yan F., Wang L., Shi Y., Cao H., Liu L., Washington M. K., et al. (2012). Berberine promotes recovery of colitis and inhibits inflammatory responses in colonic macrophages and epithelial cells in DSS-treated mice. Ame. J. Physiol. Gastroint. Liver Physiol. 302, G504–G514. doi: 10.1152/ajpgi.00312.2011
Yan H.-M., Xia M.-F., Wang Y., Chang X.-X., Yao X.-Z., Rao S.-X., et al. (2015). Efficacy of berberine in patients with non-alcoholic fatty liver disease. PloS One 10, e0134172. doi: 10.1371/journal.pone.0134172
Yin J., Xing H., Ye J. (2008). Efficacy of berberine in patients with type 2 diabetes mellitus. Metabolism 57, 712–717. doi: 10.1016/j.metabol.2008.01.013
Yi J., Ye X., Wang D., He K., Yang Y., Liu X., et al. (2013). Safety evaluation of main alkaloids from Rhizoma coptidis. J Ethnopharmacol. 145, 303–310. doi: 10.1016/j.jep.2012.10.062
Yokozawa T., Ishida A., Cho E. J., Kim H. Y., Kashiwada Y., Ikeshiro Y. (2010). Coptidis rhizoma: protective effects against peroxynitrite-induced oxidative damage and elucidation of its active components. J. Pharm. Pharmacol. 56, 547–556. doi: 10.1211/0022357023024
Yu C., Zhang J., Qin Q., Liu J., Xu J., Xu W. (2020). Berberine improved intestinal barrier function by modulating the intestinal microbiota in blunt snout bream (Megalobrama amblycephala) under dietary high-fat and high-carbohydrate stress. Fish Shellfish Immunol. 102, 336–349. doi: 10.1016/j.fsi.2020.04.052
Zhang Y.-P., Deng Y.-J., Tang K.-R., Chen R.-S., Liang S., Liang Y.-J., et al. (2019). Berberine ameliorates high-fat diet-induced non-alcoholic fatty liver disease in rats via activation of SIRT3/AMPK/ACC pathway. Cur. Med. Sci. 39, 37–43. doi: 10.1007/s11596-019-1997-3
Zhang Y., Feng H., Liang X.-F., He S., Lan J., Li L. (2022). Dietary bile acids reduce liver lipid deposition via activating farnesoid X receptor, and improve gut health by regulating gut microbiota in Chinese perch (Siniperca chuatsi). Fish Shellfish Immunol. 121, 265–275. doi: 10.1016/j.fsi.2022.01.010
Zhang C.-N., Li X.-F., Jiang G.-Z., Zhang D.-D., Tian H.-Y., Li J.-Y., et al. (2014). Effects of dietary fructooligosaccharide levels and feeding modes on growth, immune responses, antioxidant capability and disease resistance of blunt snout bream (Megalobrama amblycephala). Fish Shellfish Immunol. 41, 560–569. doi: 10.1016/j.fsi.2014.10.005
Zhang D., Li A., Xie J., Ji C. (2010). In vitro antibacterial effect of berberine hydrochloride and enrofloxacin to fish pathogenic bacteria. Aquacult. Res. 41, 1095–1100. doi: 10.1111/j.1365-2109.2009.02399.x
Zhang H. Y., Piao X. S., Zhang Q., Li P., Yi J. Q., Liu J. D., et al. (2013). The effects of forsythia suspensa extract and berberine on growth performance, immunity, antioxidant activities, and intestinal microbiota in broilers under high stocking density. Poult Sci. 92, 1981–1988. doi: 10.3382/ps.2013-03081
Zhao L., Cang Z., Sun H., Nie X., Wang N., Lu Y. (2017). Berberine improves glucogenesis and lipid metabolism in nonalcoholic fatty liver disease. BMC Endo. Dis. 17, 13. doi: 10.1186/s12902-017-0165-7
Zhou F., Bai M., Zhang Y., Zhu Q., Zhang L., Zhang Q., et al. (2018). Berberine-induced activation of AMPK increases hepatic FGF21 expression via NUR77. Biochem. Biophys. Res. Commun. 495, 1936–1941. doi: 10.1016/j.bbrc.2017.12.070
Zhou M., Deng Y., Liu M., Liao L., Dai X., Guo C., et al. (2021). The pharmacological activity of berberine, a review for liver protection. Eur. J. Pharmacol. 890, 173655. doi: 10.1016/j.ejphar.2020.173655
Zhou C., Li X.-C., Fang W.-H., Yang X.-L., Hu L.-L., Zhou S., et al. (2011). Inhibition of CYP450 1A and 3A by berberine in crucian carp Carassius auratus gibelio. comp. Biochem. Physiol. C Toxico. Pharm. 154, 360–366. doi: 10.1016/j.cbpc.2011.07.005
Zhou J., Li C., Wang L., Ji H., Zhu T. (2015). Hepatoprotective effects of a Chinese herbal formulation, yingchen decoction, on olaquindox-induced hepatopancreas injury in jian carp (Cyprinus carpio var. Jian). Fish Physiol. Biochem. 41, 153–163. doi: 10.1007/s10695-014-0013-x
Zhou X., Peng Y., Li L., He K., Huang T., Mou S., et al. (2016). Effects of dietary supplementations with the fibrous root of Rhizoma coptidis and its main alkaloids on non-specific immunity and disease resistance of common carp. Vet. Immunol. Immunopathol. 173, 34–38. doi: 10.1016/j.vetimm.2016.03.014
Zhou W., Rahimnejad S., Lu K., Wang L., Liu W. (2019). Effects of berberine on growth, liver histology, and expression of lipid-related genes in blunt snout bream (Megalobrama amblycephala) fed high-fat diets. Fish Physiol. Biochem. 45, 83–91. doi: 10.1007/s10695-018-0536-7
Zhou L., Yang Y., Wang X., Liu S., Shang W., Yuan G., et al. (2007). Berberine stimulates glucose transport through a mechanism distinct from insulin. Metabolism 56, 405–412. doi: 10.1016/j.metabol.2006.10.025
Zhu F. (2020). A review on the application of herbal medicines in the disease control of aquatic animals. Aquaculture 526, 735422. doi: 10.1016/j.aquaculture.2020.735422
Keywords: Berberine, fish health, lipid metabolism, antioxidant, immune
Citation: Wang L, Sagada G, Wang C, Gao C, Wang B, Shao Q and Yan Y (2022) Berberine in fish nutrition: Impact on hepatoenteric health, antioxidative and immune status. Front. Mar. Sci. 9:967748. doi: 10.3389/fmars.2022.967748
Received: 13 June 2022; Accepted: 16 August 2022;
Published: 05 September 2022.
Edited by:
Min Jin, Ningbo University, ChinaReviewed by:
Kang-le Lu, Jimei University, ChinaMohamed M. Abdel-Daim, Suez Canal University, Egypt
Ermeng Yu, Pearl River Fisheries Research Institute (CAFS), China
Copyright © 2022 Wang, Sagada, Wang, Gao, Wang, Shao and Yan. This is an open-access article distributed under the terms of the Creative Commons Attribution License (CC BY). The use, distribution or reproduction in other forums is permitted, provided the original author(s) and the copyright owner(s) are credited and that the original publication in this journal is cited, in accordance with accepted academic practice. No use, distribution or reproduction is permitted which does not comply with these terms.
*Correspondence: Lei Wang, bGVpd2FuZ0BhaG51LmVkdS5jbg==; Qingjun Shao, cWpzaGFvQHpqdS5lZHUuY24=; Yunzhi Yan, eWFueXVuemhpQGFobnUuZWR1LmNu