- 1School of Marine Science, University of Gibraltar, Gibraltar, Gibraltar
- 2School of Marine Science and Engineering, University of Plymouth, Plymouth, United Kingdom
- 3Ocean BioGeosciences, National Oceanography Centre, Southampton, United Kingdom
The presence of marine litter is of concern in submarine canyons, although research in this area is still in its infancy. A critical synthesis and literature review selecting studies with primary data of benthic marine litter at depths of over 50 m revealed important gaps in the knowledge, with information on the impact of macroplastics in deep-sea environments still scarce. Less than 1% of medium to large submarine canyons mapped have been studied in any measure for marine litter, with over 91% of the canyon studies located in European waters. Imaging techniques are now the main tools used for sampling, overtaking trawling methods despite the continued growth of the latter for marine litter deep-sea research. Enumeration of litter was diverse with over 75% using abundance for quantification. Despite the existence of litter protocols available for deep-sea environments, over 73% of studies did not use any. There was no standardization in the implementation of established classification protocols, which were either not used in full or were customized in part. Fishing-related categories do not feature as a top-level category in the classification hierarchy in any of the protocols, yet over 50% of publications featured fishing materials as a main category, pointing to a more intuitive activity-based categorization of litter instead of a materials-led approach from the established protocols. Furthermore, interactions between litter and the surrounding environment and biota are very much underreported with little or no consensus between how the data are analyzed and expressed. There were no discernible patterns between litter density, composition and broad geographical location of canyons, with individual topographical characteristics, hydrodynamic regimes and anthropogenic activities being determining factors in how submarine canyons are affected by litter. Overall, there is no apparent framework to allow comparison of studies and due to the different methods of identifying, enumerating, quantifying and classifying marine litter, or lack of data on position and morphological setting within the canyon system. The evidence provided within this study highlights a ‘call to action’ for an urgent need to standardize and unify methodologies with new or established protocols to fully understand the impact of marine litter in submarine canyons.
Introduction
Marine litter
Marine litter, also known as marine debris, is defined by the UNEP as “any persistent, manufactured or processed solid material discarded, disposed of, or abandoned in the marine and coastal environment” (UNEP, 2009), and is found in all seas, at all depths, and regardless of proximity to densely populated regions. It has economic, cultural, public health and safety impacts, adding additional pressures to ecosystems and habitats already affected by other anthropogenic pressures. The terms ‘marine litter’ and ‘marine debris’ can be used interchangeably; the former, however, can infer greater attributability on littering behaviors by consumers instead of surging plastic production and/or waste mismanagement by wealthier nations. The term ‘marine litter’ is used in overarching programs such as the UNEP and within legal texts in Europe, such as the MSFD, where it is included in the list of descriptors to achieve GES (Good Environmental Status) by the European Commission, with the deep seafloor considered one of the largest litter accumulation zones in the ocean (European Commission, 2010; Galgani et al., 2013; Pham et al., 2014).
Most marine litter studies, thus far, have focused on the impacts on sea surface biota, beach ecosystem services, floating or coastal litter, and knowledge of benthic macrolitter (>25 mm in diameter) beyond coastal habitats is sparse (Spengler and Costa, 2008; Barboza et al., 2018; Consoli et al., 2018a; Napper and Thompson, 2019; Sinopoli et al., 2020; Suaria and Alani, 2014; Canals et al., 2021). Marine sources of litter are linked to shipping routes and activities (commercial and recreational), fishing activities, marine traffic, military activities, aquaculture, offshore installations, and dumping at sea (Ramirez-Llodra et al., 2013; Woodall et al., 2015). Sources of land-based litter include agriculture, recreational coastal use, ports, tourism, or shipyards, sewage outflows or river discharge, making the exact source of any item of litter almost impossible to identify (Stefatos et al., 1999; Galgani et al., 2000; Pham et al., 2014; Angiolillo, 2019). Land-based sources of marine litter, however, have been found to provide the major fraction of litter found on the coastline (Canals et al., 2021).
Plastics are the most prevalent type of litter; they are inexpensive, strong, lightweight, resistant to corrosion and taking many forms, with global production rising from 5 Mt per year in the 1950s to 438 Mt per year in 2017 and is projected to increase to 1.1 billion tonnes per year by 2050 (Jambeck et al., 2015; Napper and Thompson, 2019; Geyer, 2020). It has been estimated that 19 to 23 million metric tons (Mt) (11%, of plastic waste generated globally) entered aquatic ecosystems in 2016 alone, and taking into consideration ambitious commitments by some governments this could rise to 53 Mt per year by 2030, or to over 90 Mt per year under the current ‘business as usual scenario’ (Borrelle et al., 2020). There are thought to be over 5.25 trillion plastic particles > 5 mm in diameter floating on the sea surface alone, weighing over 240,000 tonnes (Eriksen et al., 2014). Plastics are long-lasting with yet unknown persistence timescales which require more data to be assessed effectively, but are largely dependent on the polymer properties aided by additives (Barnes et al., 2009; Ward and Reddy, 2020). In deep-sea benthic environments where oxygen levels are reduced and light required for photooxidation is absent, timescales could be considerably larger than on the surface (Barnes et al., 2009; Spedicato et al., 2019).
Impacts on biota
Ingestion is one of the main direct impacts that marine litter has on a wide range of biota, causing potential internal injuries, blockage in the digestive tract, decreased nutrient uptake or false sense of satiation, harm by toxicity such as deleterious effects on sexual reproduction and survival, or even death by accumulation (Kühn et al., 2015). Other threats include physical harm by entanglement, smothering, abrasion, breakage, necrosis, and suffocation. Observations of mechanical damage by fishing lines have been documented on sessile organisms in deep-sea environments, such as cold-water corals (CWCs) and sponges, and when they die the affecting debris can be released if structures do not remain intact, free to repeat the cycle on other organisms (Pham et al., 2013; Bo et al., 2014; Angiolillo et al., 2015; Cau et al., 2017; Angiolillo, 2019). Alien invasive species can use non-degradable litter objects as vectors for transportation horizontally over large distances (Kühn et al., 2015).
Derelict fishing gear (DFG) is one of the most abundant types of litter in rocky environments, and is responsible for ghost fishing; a type of entanglement that entraps and causes the death of a wide range of organisms by hindering their ability to breathe, feed or escape from predators (Lopez-Lopez et al., 2017; Melli et al., 2017; Angiolillo, 2019). The overall amount of DFG is unknown, although crude approximations point to 10% of all litter entering the marine environment consisting of ghost nets (Macfadyen et al., 2009). Other types of fishing gear such as traps and pots may also continue to “fish” passively for years after settlement in the environment (Ramirez-Llodra et al., 2011; Galgani et al., 2013; Consoli et al., 2020). It is estimated that DFG may contribute up to 98% of total litter where fishing activities represent an important economic sector (Angiolillo et al., 2015; Cau et al., 2017; Consoli et al., 2018b; Consoli et al., 2019). Impacts which may not necessarily be considered to be negative are also observed through increases in habitat and spatial heterogeneity at small scales, use as a substratum by some organisms to grow, refugia by prey and predator species, and even becoming biodiversity hotspots boosting population extensions of sessile and some free-living invertebrates (Watters et al., 2010; Miyake et al., 2011; Mordecai et al., 2011; Bergmann and Klages, 2012; Schlining et al., 2013; Woodall et al., 2015; Song et al., 2021).
Transport of litter into the deep sea
The buoyancy of plastics is highly variable and while it is estimated that 50% of plastics from municipal waste have a density greater than seawater and will sink quickly, the rest can float on the surface for large distances before changing in density by being fouled, waterlogged, leaching of additives or amassing too much fouling epibiota (Ye and Andrady, 1991; Barnes et al., 2009; Andrady, 2011; Engler, 2012; Pham et al., 2014; Avio et al., 2017; Fortibuoni et al., 2019; van Sebille et al., 2020). It is thought that 70% of floating litter eventually sinks although rates are largely unknown, with arrival on the seafloor potentially delayed for long periods of time (UNEP, 2005; Pham et al., 2014; Tekman et al., 2017). Spatial distribution of litter and accumulation are uneven and dependent on localised factors such as the route and amount of litter, the marine environment, local wind and hydrographic conditions, anthropogenic activities, population density, temporal environmental variations or catastrophic events, such as the Tohoku earthquake where litter density on the continental slope of north Japan increased substantially after the event, or flash floods in Sicily causing the highest litter accumulations in submarine canyons (and in the deep sea) recorded to date (Keller et al., 2010; Mordecai et al., 2011; Pham et al., 2013; Ramirez-Llodra et al., 2013; Pham et al., 2014; Tubau et al., 2015; Goto and Shibata, 2015; Shimanaga and Yanagi, 2016; Pierdomenico et al., 2020). Litter can also become trapped in areas of low circulation, high sedimentation, and high rugosity morphological settings (Galgani et al., 2000; Eriksen et al., 2014).
Hydrodynamic processes such as gravity currents, thermohaline currents, bottom currents, seafloor gyres and downwelling processes, are important for the transport and redistribution of marine litter on the seafloor, with entrainment of litter possible in turbulent flows that can cause higher litter densities in submarine canyons in comparison to the continental slope (Galgani et al., 2000; Ramirez-Llodra et al, 2011; Ioakeimidis et al., 2014; Tubau et al., 2015; Woodall et al., 2015; Buhl Mortensen and Buhl Mortensen, 2017; Kane and Clare, 2019; Pierdomenico et al., 2019b, Kane et al., 2020; Pierdomenico et al., 2020). Downwelling processes have been found to responsible for the vertical transport of litter in the South China Sea, where fishing activity is a notable source of pollution (Zhang et al., 2020). A substantial portion of plastic litter entering the ocean is now found deposited and/or buried in ocean sediments with marine sediments being a major sink of plastic pollution in the ocean (Kane and Fildani, 2021; Martin et al., 2022).
Submarine canyons
To date 9,477 medium-to-large-sized submarine canyons with a depth range of at least 1,000 m, a width/depth ratio of less than 150:1 and a canyon incision of at least 100 m, have been mapped on all continental margins, occupying a global ocean surface area of 11.2% of the continental slope (Harris and Whiteway, 2011; Harris et al., 2014). Submarine canyons are preferential conduits for nutrients, organic matter, and sediments to deep-sea environments, connecting the shallower continental shelf with deeper basins (Canals et al., 2006). They exhibit greater abundances and species richness when compared to adjacent slope areas as higher levels of associated primary productivity can lead to canyons to be hotspots of faunal productivity in the deep sea (De Leo et al., 2010). As such, submarine canyons are important regional sources of marine biodiversity and ecosystem function, and provide a wide range of ecosystem services (Fernandez-Arcaya et al., 2017). Sedimentary gravity flows such as turbidity currents, density currents, and oceanographic processes such as internal waves and upwelling/downwelling circulation patterns in shelf-incising canyons are thought to facilitate the transport of marine litter hundreds of kilometers from the coast at times, turning canyons into sinks that act as passive accumulation areas (Watters et al., 2010; Ramirez-Llodra et al., 2013; Schlining et al., 2013; Pham et al., 2014; Tubau et al., 2015; Fernandez-Arcaya et al., 2017; Pierdomenico et al., 2019b). There is no discernible pattern regarding the source of litter in submarine canyons, although marine sources are responsible for litter on the open slope close to major shipping routes and fishing lanes, with correlations established between these and the presence of heavy, fast-sinking objects (Ramirez-Llodra et al., 2013; Fiorentino et al., 2015; García-Rivera et al., 2017; Tekman et al., 2017; Parga Martinez et al., 2020).
Monitoring marine litter
Most marine litter protocols and frameworks are better suited to record floating, beach and coastal litter (Canals et al., 2021). Some have been designed to monitor marine litter regionally during fisheries surveys using trawling as their method of sampling, such as the MEDITS and OSPAR protocols (MEDITS, 2017; OSPAR Commission, 2017). The latter incorporates the International Bottom Trawling Survey that has been running yearly surveys since 1970 in the North Sea, and the Baltic International Trawl Survey. The MEDITS protocol oversees collection of data at depths between 10 m and 800 m in five different strata on a yearly basis. The MSFD recommends Member States to use existing regional sea conventions, such as OSPAR (NE Atlantic Ocean), Barcelona Convention (Mediterranean Sea), Helsinki Convention (Baltic Sea), and the Bucharest Convention (Black Sea) as institutional cooperation structures to build upon existing programs and activities to achieve cohesion and coordination of strategies and even standardizes the OSPAR and MEDITS protocol within its overarching framework (European Commission, 2010; Galgani et al., 2013; MEDITS, 2017; OSPAR Commission, 2017). The MSFD has a large master list of litter categories and subcategories which are suitable for use in different environments, such as the sea surface, beaches, or the seabed, caters for item size in its classification system, is the only major official protocol that caters for imaging technology, and has an online photo catalogue to aid identification of litter types (Galgani et al., 2013; Fleet et al., 2021). Few protocols exist that specifically target macrolitter, benthic or deep-sea environments that can be used to assess the impacts of marine litter in submarine canyons. Alignment, synergies, and divergences in non-coastal benthic litter classification between the MSFD, MEDITS and OSPAR can be found in Supplementary Table 1.
This review aims to identify gaps in the knowledge to this effect, with one of the main objectives being to determine whether comparisons and coherent patterns can be derived in the identification, classification, enumeration, and quantification of marine litter in existing studies, as well as possible comparisons in litter trends in the literature. Another aim is to evaluate the need for unified and standardized parameters and practices when carrying out litter surveys in submarine canyons (although there is a need for a unified and standardized observational protocol for litter in the deep-sea as a whole), so that the true extent of litter pollution in submarine canyons can be fully understood, the transport, deposition, and biotic interactions can be investigated, and individual studies can be made compatible and comparable.
Materials and methods
A literature review and critical synthesis was conducted using Google Scholar (GS) and Science Direct (SD) and structured based on the PRISMA framework (Page et al., 2021). Using both discovery engines is supported by standard infometric methodologies as a way of countering bias from paywall services, with 95% of Web of Science citations and 92% of Scopus citations found on GS, which can be considered a superset of these services with substantially increased coverage (Moed et al., 2016; Halevi et al., 2017; Martín-Martín et al., 2018). Lexical units were selected from preliminary subject reading, forming two distinct groupings: seafloor features and habitats (SFH) and marine litter impacts (MLI), (Table 1). Searches per lexical unit were conducted for the period 1981-2020 at yearly intervals. Adequacy of using only GS was established for subsequent searches (Supplementary Table 2), and lexical units from both groups were combined for the discrete period 2001-2020 in a string of two parts using quotation marks around the components for greater specificity, to focus on the last two decades when most of the literature related to marine litter was seen to appear and was taken as the main limiting factor. A heatmap was produced to examine the weight of MLI in relation to SFH. Searches were carried out to determine the most mentioned submarine canyons using the term “submarine canyon” and a list of broad geographical areas (Table 1) between 2001-2020. Submarine canyons found in the top 100 search results were then searched by canyon name (allowing for syntax differences and name equivalences) combined with a selection of MLI terms (Table 1).
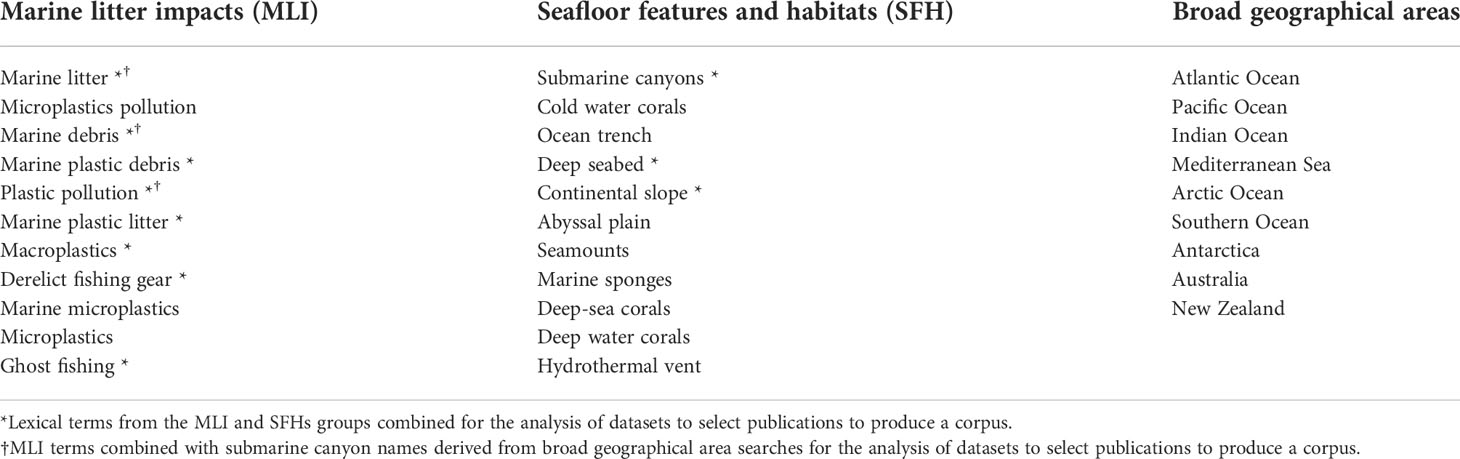
Table 1 List of marine litter impacts, seafloor features and habitats and broad geographical areas used to conduct searches.
Exhaustive analyses of datasets produced from combinations of selected terms as indicated from Table 1 were carried out to produce a collection of studies on marine litter based on the criteria below, henceforth referred to as the corpus. The period 2001-2020 was used for the inclusion of data since this was taken as the limiting factor when combining lexical terms and made for a more efficient focus on the corpus analysis. The criteria for literature selection were:
• Inclusion of primary data on macrolitter identification, classification and quantification in submarine canyons or the seabed at depths > 50 m.
• Exclusion of data on beach, estuarine, riverine, floating litter, or microplastics.
Review publications and the LITTERBASE database were also queried for analysis of litter distribution and composition in the literature (Pham et al., 2014; Angiolillo, 2019; LITTERBASE, 2020).
Data were extracted from the corpus publications for geographical area and study sites, data type, acquisition methodology, morphological setting, impacts on biota, enumeration, quantification, litter protocols used, depth ranges, litter density and litter categories used (hierarchical tiers and nomenclature). Litter categories used were standardized to the minimum number of broad categories possible for the comparison of litter composition and classification systems, by grammatically harmonizing for use of singular and plural, use of punctuation, syntax variations of the same object or term, and incorrect terminology, thus reducing the number of categories and subcategories while maintaining maximum diversity of discrete items where contextually and lexically possible. Categories and subcategories were not based on existing litter protocols pre-2020 since their top-level classification systems were insufficient to list all the main types of litter found in the corpus. Contextual groupings were made with categories that had evident links in type of material composition to determine the main types of litter found, such as plastic bottle, plastic sheet or plastic bag grouped into a wider artificial polymer group.
Geographical areas were standardized and grouped to establish links and patterns with study sites extracted with the corresponding number of surveys per site. If canyon and non-canyon features were cited for the same geographical area, these were listed separately. Number of surveys per site, differentiated between seafloor and submarine canyons were plotted in a map. Litter densities per canyon were recalculated to provide a mean value where canyon data were provided by more than one study (providing the data were in comparable formats, e.g. abundance per spatial area) or when data were combined in studies to give a single density figure for canyons in a broad geographical area (such as the Gulf of Lyon canyons), taking into account standard deviation and standard error. Interactions between litter and benthic fauna were also extracted from the corpus studies. The schematic representation of the overall analysis is summarized in Figure 1.
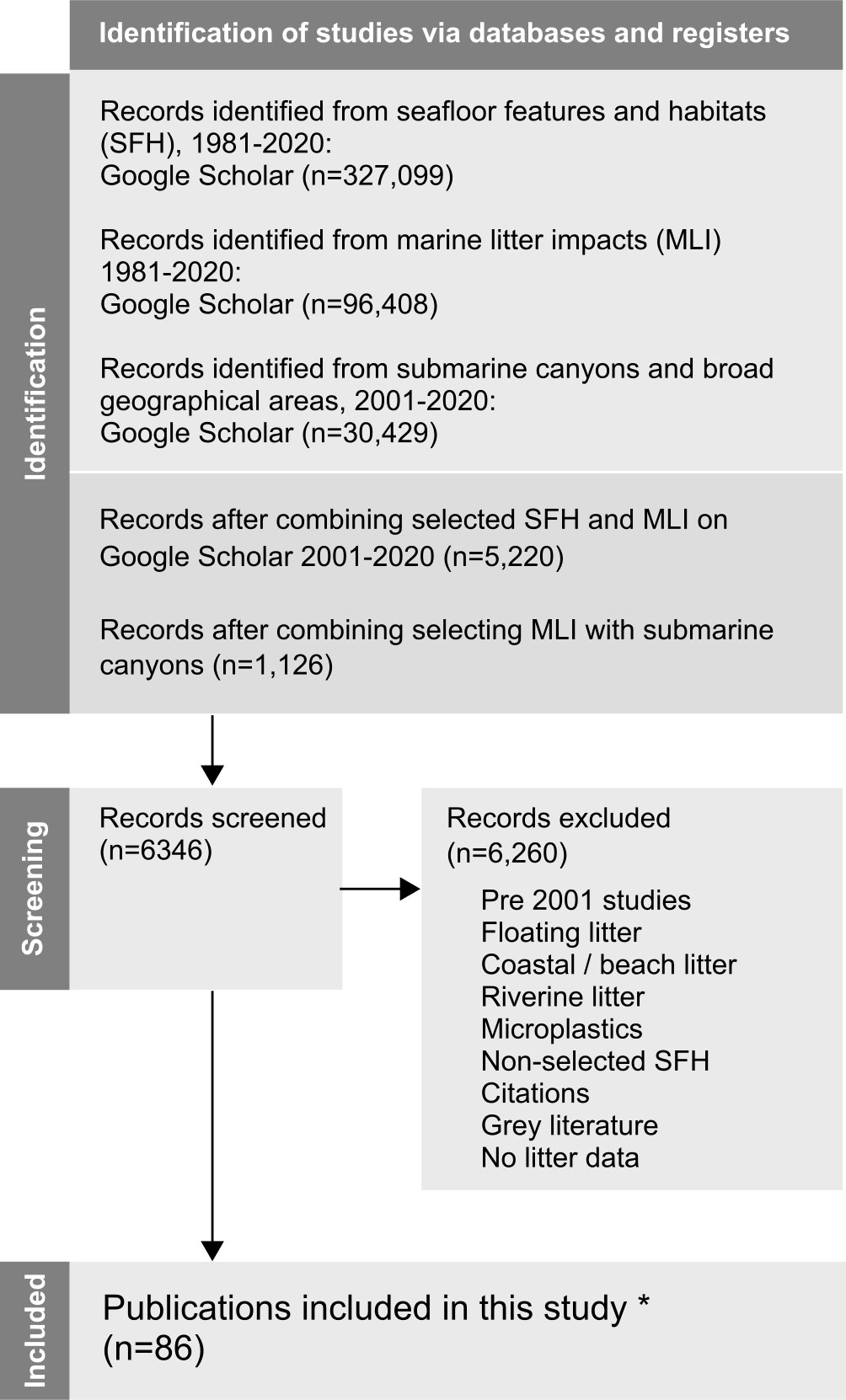
Figure 1 Schematic representation of the literature review and critical synthesis to produce a corpus.* Jeong et al., 2005; Lee at al., 2006; Koutsodendris et al., 2008; Barnes et al., 2009; Keller et al., 2010; Topçu and Öztürk, 2010; Watters et al., 2010; Miyake et al., 2011; Mordecai et al., 2011; Bergmann and Klages, 2012; Wei et al., 2012; Güven et al., 2013; Lefkaditou et al., 2013; Mifsud et al., 2013; Pham et al., 2013; Purser et al., 2013; Ramirez-Llodra et al., 2013; Sánchez et al., 2013; Schlining et al., 2013; Bo et al., 2014; Debrot et al., 2014; Eryaşar et al., 2014; Fabri et al., 2014; Ioakeimidis et al., 2014; Pham et al., 2014; Angiolillo et al., 2015; Fiorentino et al., 2015; Goto and Shibata, 2015; Ioakeimidis et al., 2015; Neves et al., 2015; Oliveira et al., 2015; Papadopoulou et al., 2015; Strafella et al., 2015; Tubau et al., 2015; Vieira et al., 2015; Woodall et al., 2015; Yamakita et al., 2015; Lastras et al., 2016; Moriarty et al., 2016; Pasquini et al., 2016; Shimanaga and Yanaghi., 2016; Buhl-Mortensen and Buhl-Mortensen, 2017; Cau et al., 2017; García-Rivera et al., 2017; Lopez-Lopez et al., 2017; Louald et al., 2017; Rodríguez and Pham, 2017; Tekman et al., 2017; van den Beld., 2017; Alvito et al., 2018; Baudrier et al., 2018; Cau et al., 2018; Chiba et al., 2018; Consoli et al., 2018b; Domingiez Carrió, 2018; Ferrigno et al., 2018; García-Rivera et al., 2018; Grøsvik et al., 2018; Kammann et al., 2018; Maes et al., 2018; Olguner et al., 2018; Urban-Malinga et al., 2018; Consoli et al., 2019; Fortibuoni et al., 2019; Gerigny et al., 2019; Giusti et al., 2019; Peng et al., 2019; Pierdomenico et al., 2019a; Pierdomenico et al., 2019b; Rhinane and Loulad, 2019; Spedicato et al., 2019; Strafella et al., 2019; Zablotski and Kraak, 2019; Alomar et al., 2020; Amon et al., 2020; Botero, et al., 2020; Consoli et al., 2020; Crocetta et al., 2020; Du Preez et al., 2020; Enrichetti et al., 2020; Garofalo et al., 2020; Grinyó et al., 2020; Kuroda et al., 2020; Mecho et al., 2020; Pierdomenico et al., 2020 and Ryan et al., 2020
Results
Lexical term search results 1981-2020
Lexical groups showed a marked difference in the number of publications and the rate at which they appear over the forty-year period studied. The rate of increase in the number of publications was steady for most terms in the SFH group (Figure 2), in particular for the terms ‘continental slope’, ‘seamounts’, ‘marine sponge’ and ‘hydrothermal vents’ since the year 2000, with the latter having registered an increase of over 37% between 2019 and 2020, to 3,630 results returned by GS. Synonyms for CWCs were analyzed individually and cumulatively, showing a five-fold increase in results from 2003 to 1,197 results returned by GS in 2020. Number of results displayed for ‘marine sponge’ increased noticeably between 2010 and 2014 and showed almost three times as many results (3,270) as the three variations of CWCs combined, which may be indicative of the connection of CWC terms with deep-sea habitats whereas research on marine sponges could be from shallow waters. Progression in results on ‘submarine canyons’, ‘deep seabed’/’deep seafloor’, and ‘abyssal plain’ showed similar trajectories but with much smaller increases until 2019, with ‘submarine canyons’ showing over 48% more results (1,510) in 2020 than in the previous year.
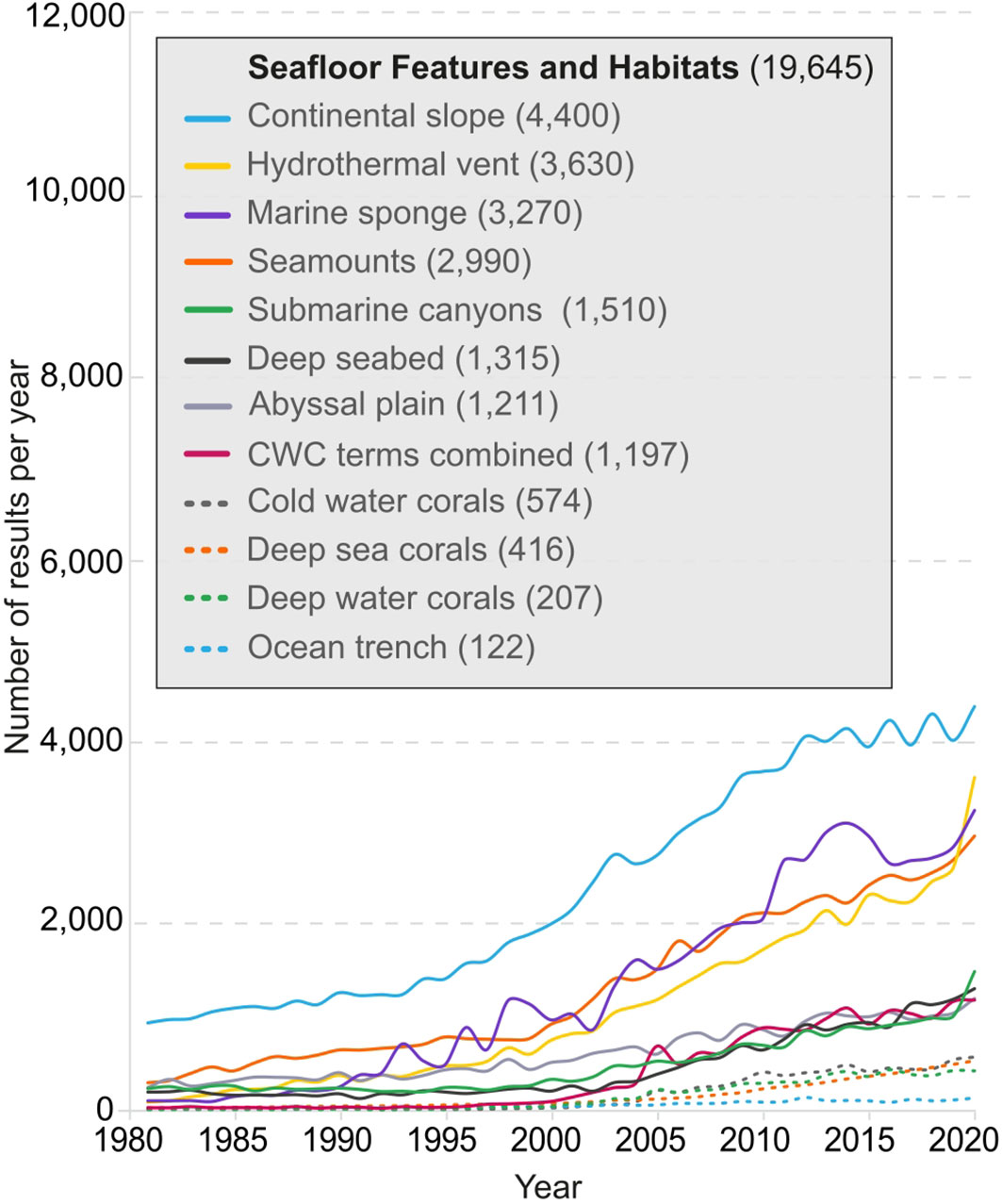
Figure 2 Number of publications per year listed in GS for the SFH lexical group, between 1981-2020. CWC terms (deep-water corals, deep-sea corals, cold-water corals) were plotted individually and as a combined count.
The rate of increase in results shown by GS in the MLI group grew dramatically per year from 2013, in large part driven by research into microplastics and plastic pollution related terms (Figure 3), with an almost sixteen-fold increase between 2010 and 2020 to 29,494 results, suggesting an increase into the perception of topicality of MLI. Publications related to ‘ghost fishing’ and ‘derelict fishing gear’ show the least amount of growth. Even though these are amply referred to in the corpus as one of the biggest MLI in the sea, there are few dedicated research programs on these impacts specifically.
Lexical group combinations
A summary matrix was produced by combining terms from the MLI and SFH groups to depict and evaluate contextual clusters and hotspots from the lexical unit combinations for the period 2001-2020 and were compared to the results for single lexical units in the MLI and SFH groups for the same period. Some SFH terms such as ‘submarine canyons’ (13.0%), or CWCs (16.4%), showed a much higher proportion of publications discussing MLI than others such as ‘continental slope’ (3.2%), ‘hydrothermal vent’ (1.5%) or ‘marine sponge’ (0.4%), despite these terms having the highest number of results per year when searched individually (Figure 4). Terms in the MLI group showed much higher proportions as a total of individual results relating to SFH than in reverse, especially in terms such as ‘ghost fishing’ (43.3%) and ‘derelict fishing gear’ (48.7%).
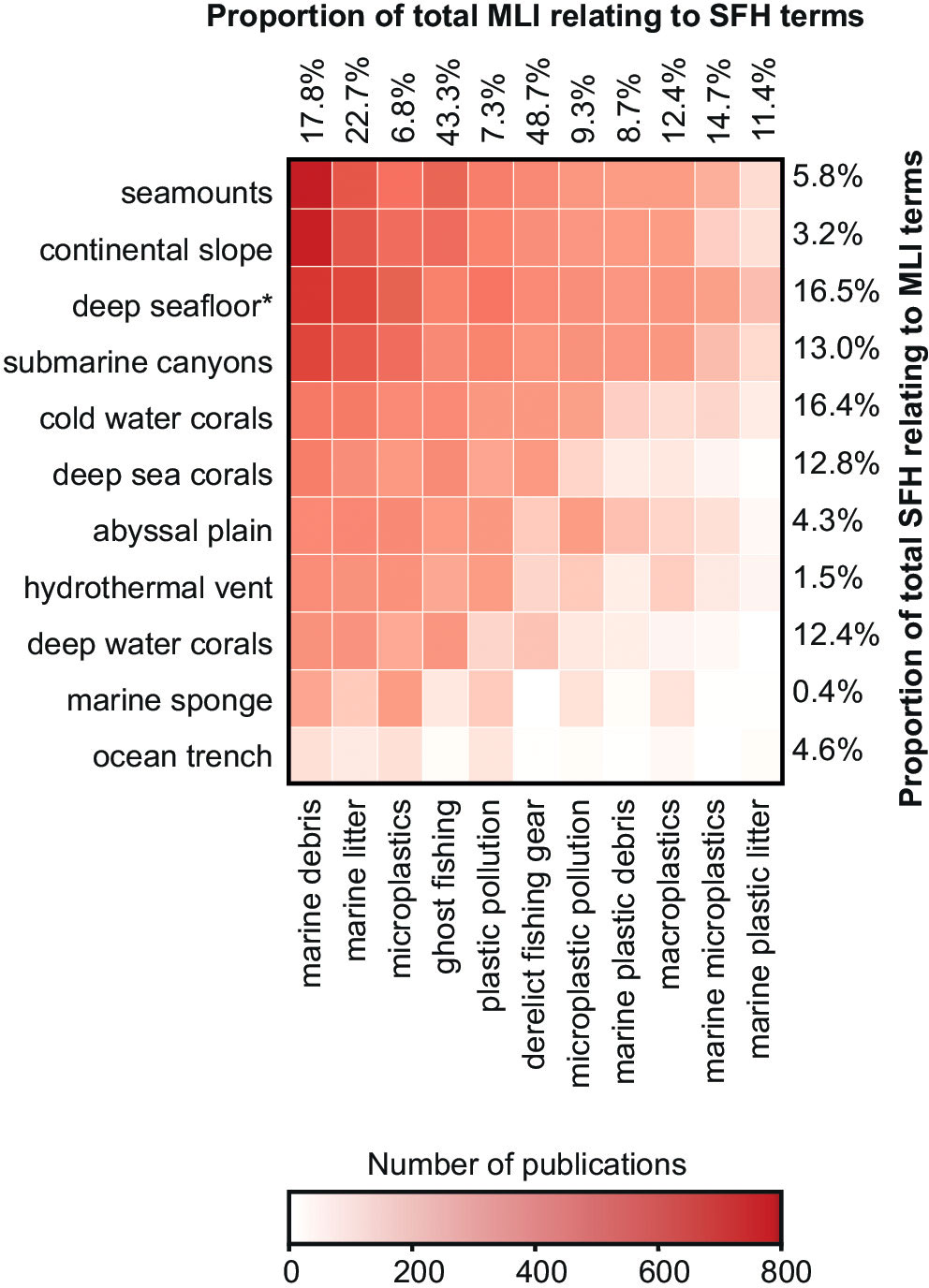
Figure 4 Heatmap for the cumulative number of publications for lexical unit combinations between lexical groupings, for the period 2001-2020 in Google Scholar. Proportion percentages were calculated as: MLI and SFH lexical unit combinations results 2001-2020 divided by single MLI or SFH lexical unit results 2001-2020. *Term equivalent for deep seafloor or deep seabed.
Submarine canyons in the literature
Refining litter related searches to include canyon names shows a very high variability in the number of publications (Table 2). The volume of these results can be somewhat misleading as they do not represent studies of MLI in submarine canyons per se, but mentions of litter within the publication, including references. The canyons in the Gulf of Mexico (Green Canyon and Mississippi Canyon) have some of the lowest MLI percentages despite showing the highest number of overall results, whereas some of the canyons with the highest MLI ratios have much fewer total publications under their name. In contrast, the Monterey Canyon, has one of the highest counts by name and in numbers of MLI results, but is not within the top positions of MLIs as a percentage, indicative of a heavily researched canyon in other areas.
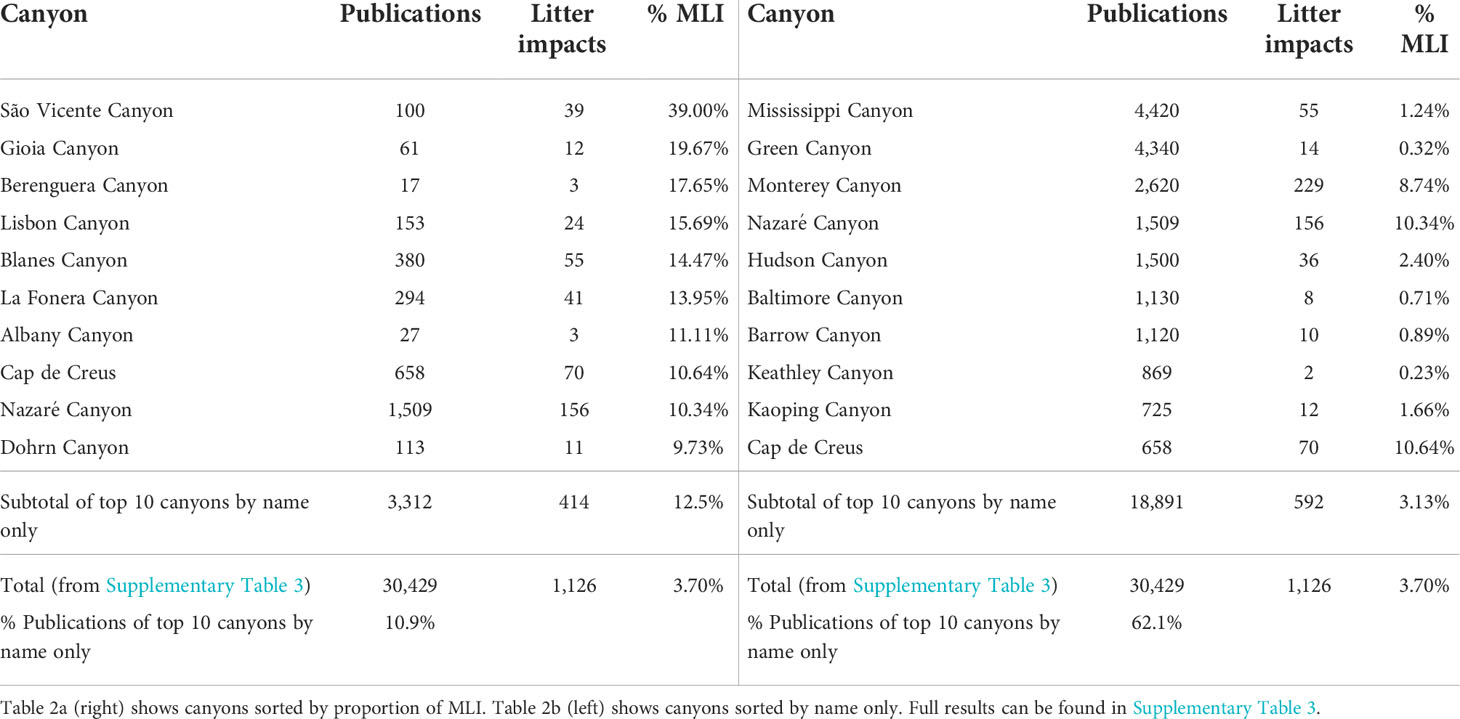
Table 2 A and B Submarine canyons most studied showing number of publications in Google Scholar when searched by name only for the period 2001-2020, and in combination with selected marine litter impacts terms (‘marine litter’, ‘marine debris’, ‘plastic pollution’).
Corpus analysis
There were 86 publications (85 papers from scientific journals, one PhD thesis) identified using the selection criteria for inclusion in the corpus (Figure 5), of which 18 had data of litter in submarine canyons (either specifically or as part of a wider study with other non-canyon sites) and 68 had data solely on other seafloor features; 10 publications were specifically focussed on litter, with the other 76 including litter data among other data provided, 5 publications had other foci such as ecological surveys, and 3 publications had data reanalyzed for the purpose of quantifying marine litter in studies with data spanning a number of years from MEDITS trawls. It was not generally possible to ascertain whether publications that had marine litter as the focus acquired the data specifically for the purpose of analyzing litter, or whether the data were part of a larger contingent or multidisciplinary analysis, repackaged as a litter-focused study.
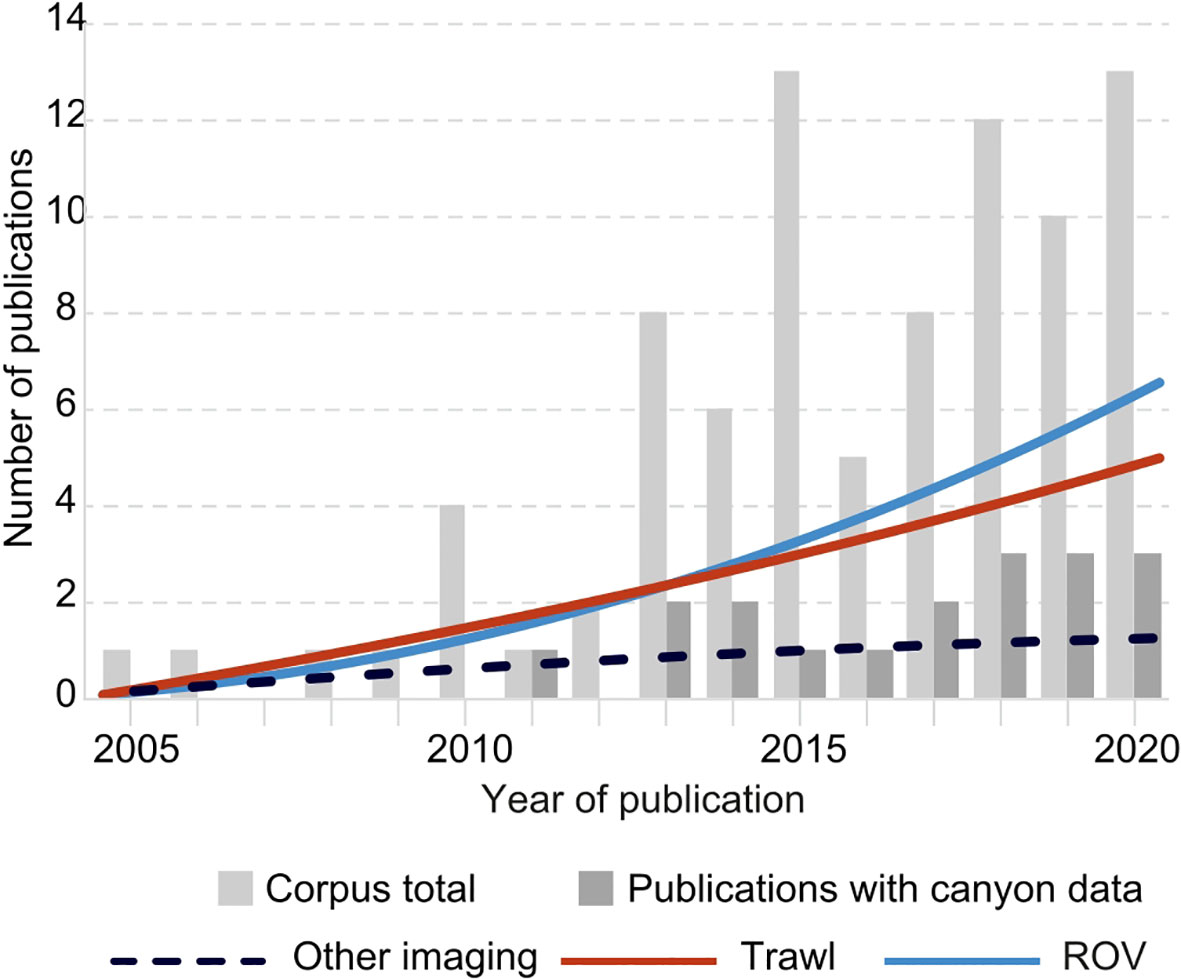
Figure 5 Total number of publications in the corpus per year for the period 2001-2020, and publications with canyon data, with polynomial trend lines (order 2) for different data acquisition methods. “Other imaging” techniques include submersible, TC and dropframe camera.
Trawled physical litter samples (henceforth referred to as ‘samples’) were used in 39 (45.3%) studies, equivalent to the number of studies that used trawls as the data acquisition method. Video and image data were used in conjunction in 7 (8.1%) studies despite the different ways used to acquire that data (ROV, drop camera, TC, or submersible). Samples, photographs, and video data were used in conjunction in 1 (1.2%) study.
Abundance of litter was used by 65 (75.7%) studies in different forms, such as abundance per area, per linear distance, per number of photographic frames or with no other criteria (Figure 6). Some studies used more than one way to acquire, record, and enumerate marine litter, with 17 (19.8%) studies recording items both as weight and abundance in any of its different forms.
Litter protocols, classification systems and interactions
Official litter protocols were not used by 63 (73.3%) publications. Of those that did, 8 (34.5%) used MEDITS, 8 (34.5%) used the MSFD, 5 (20.7%) used OSPAR, with the remaining publications using other minor litter protocols as classification systems. Classification systems in litter protocols were not followed fully with substantial differences between protocols. Studies that followed MEDITS used on average 78.6% ± 38.2 of categories available in the protocol, and most included a ‘general’ or ‘miscellaneous’ category not listed in the protocol. Studies that followed OSPAR had the greatest amount of classification fidelity with 91.7% ± 16.7, and those that followed the MSFD had the least fidelity and greatest variance, with 44.6% ± 42.1. The number of subcategories used from the protocols were much lower in comparison with 24.6% ± 37.9 for MEDITS, 3.1% ± 8.8 for the MSFD and 32.7% ± 42.7 for OSPAR. There were 23 publications that had fishing gear subcategories under other broad categories, such as artificial polymer, metal, rubber, general, or even wood, but not under its own broad ‘fishing materials’ (or similar) category (Figure 7).
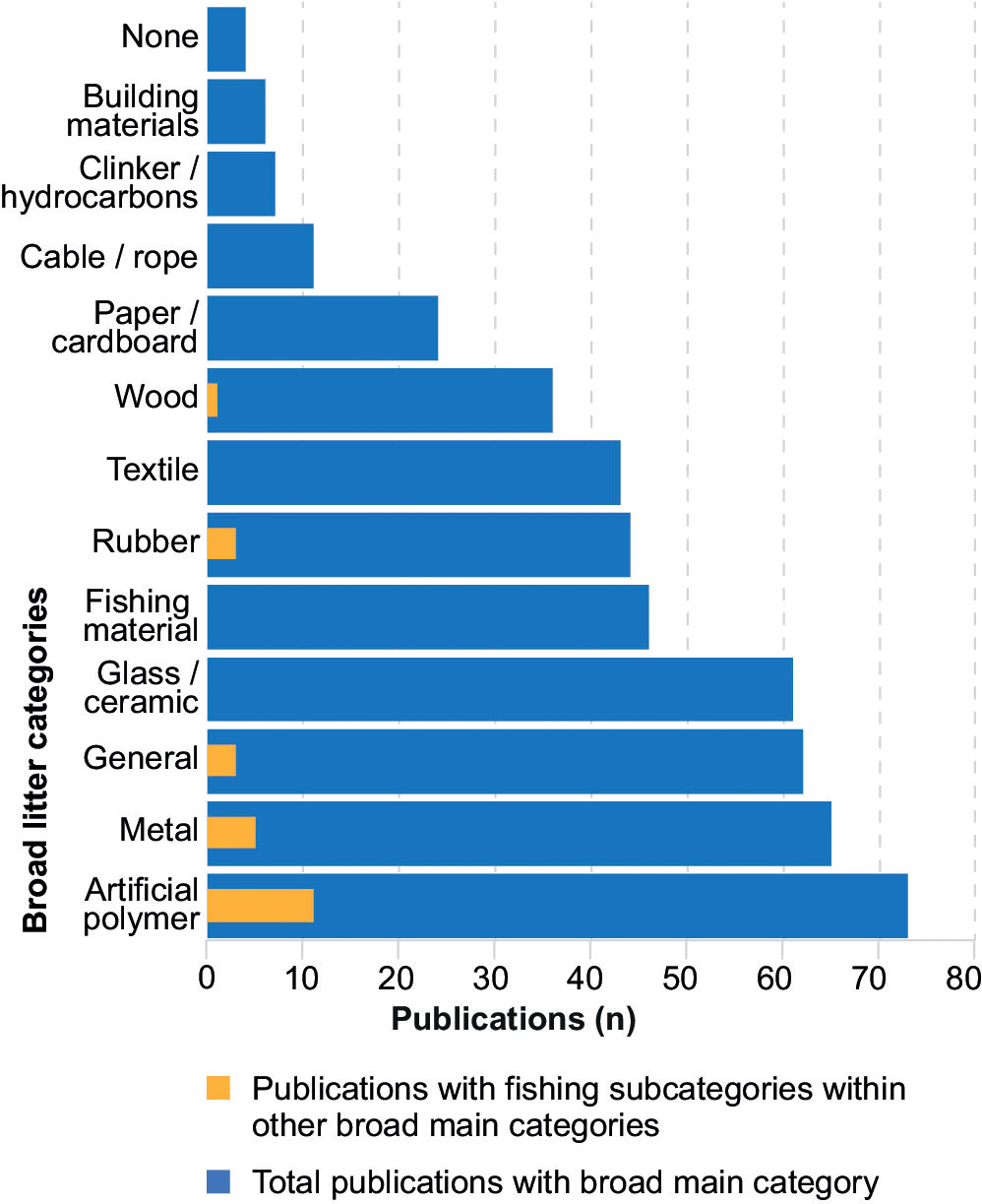
Figure 7 Number of publications in the corpus (n=86) that record data in each of the broad litter classification groupings (blue). Number of publications that included fishing materials as subcategories in other broad litter classification groups (yellow).
The resolution of litter classification systems was highly variable, with 54 (62.8%) having a primary classification system (categories) only, 28 (32.6%) including a secondary classification system (categories and subcategories) and 4 (4.7%) having neither. Additionally, 49 (56.9%) had <12 litter categories with no subcategories, and 33 (38.4%) had >12 categories and/or subcategories. The mean number of categories where a classification system existed was 7.3 ± 3.6 (n=82), and the mean number of hierarchical subcategories was 18.6 ± 11.6 (n=28).
Interactions between litter and organisms or the surrounding environment were mentioned in 25 (29.1%) publications. Entanglement was the most prevalent type of interaction with 13 (18.3%) of the total (Figure 8). Only 1 (1.4%) publication had an instance of ‘not observed’ and 4 publications (5.6%) included ‘no impact’ as an option, which in both cases can be taken as recording data of absence.
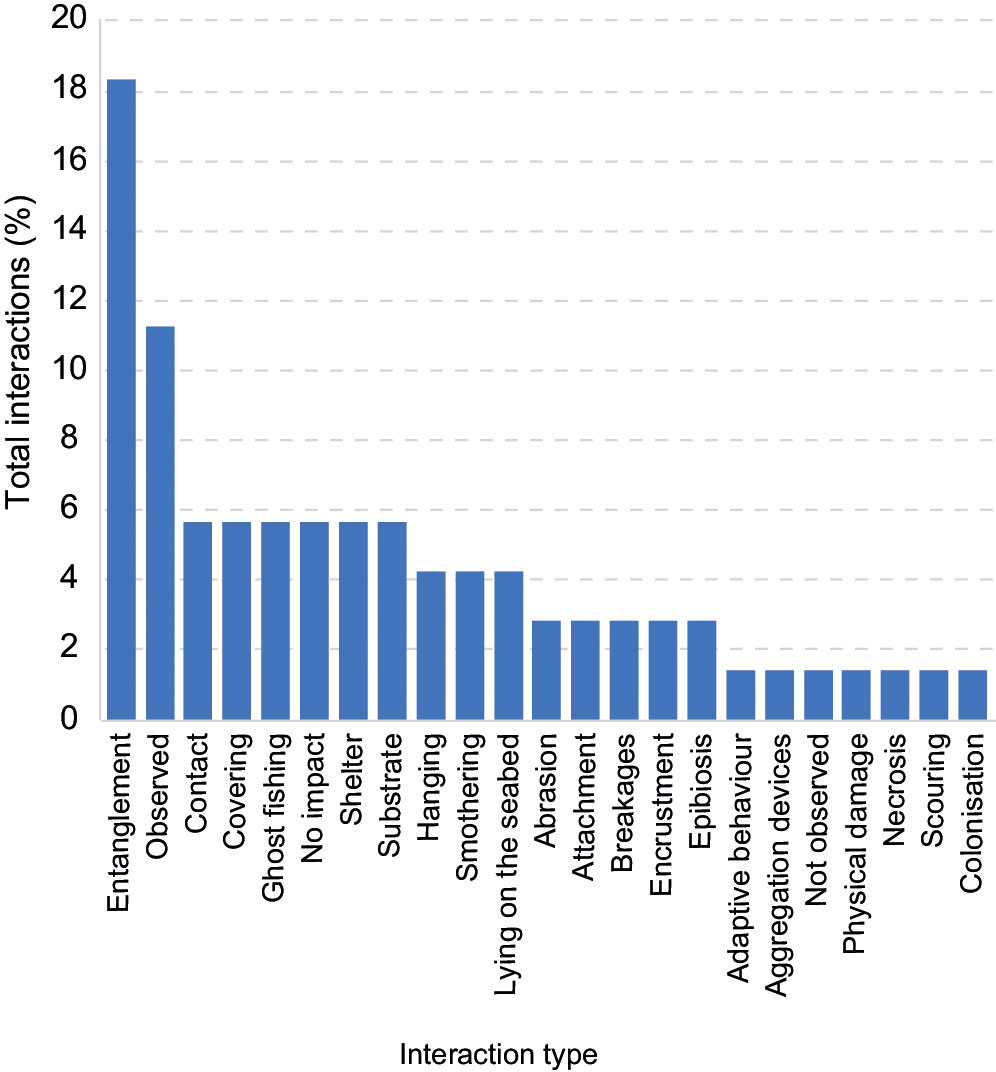
Figure 8 Instances of litter interactions (n=71) listed in the literature (25 out of 86 total publications).
Marine litter surveys, litter density and composition
Surveys in the corpus were conducted in 291 distinct sites with the Mediterranean basin accounting for 64.5% of the total. Submarine canyons encompassed 31.3% of the sites with 91.2% of these located in European waters (Figure 9). The high number of surveys in relation to number of sites is due to most publications having data on at least more than one survey over periods of time, or in different locations, with publications featuring over 600 surveys in the Gulf of Lyon, the Ligurian Sea and Corsica over a 24-year period (Gerigny et al., 2019).
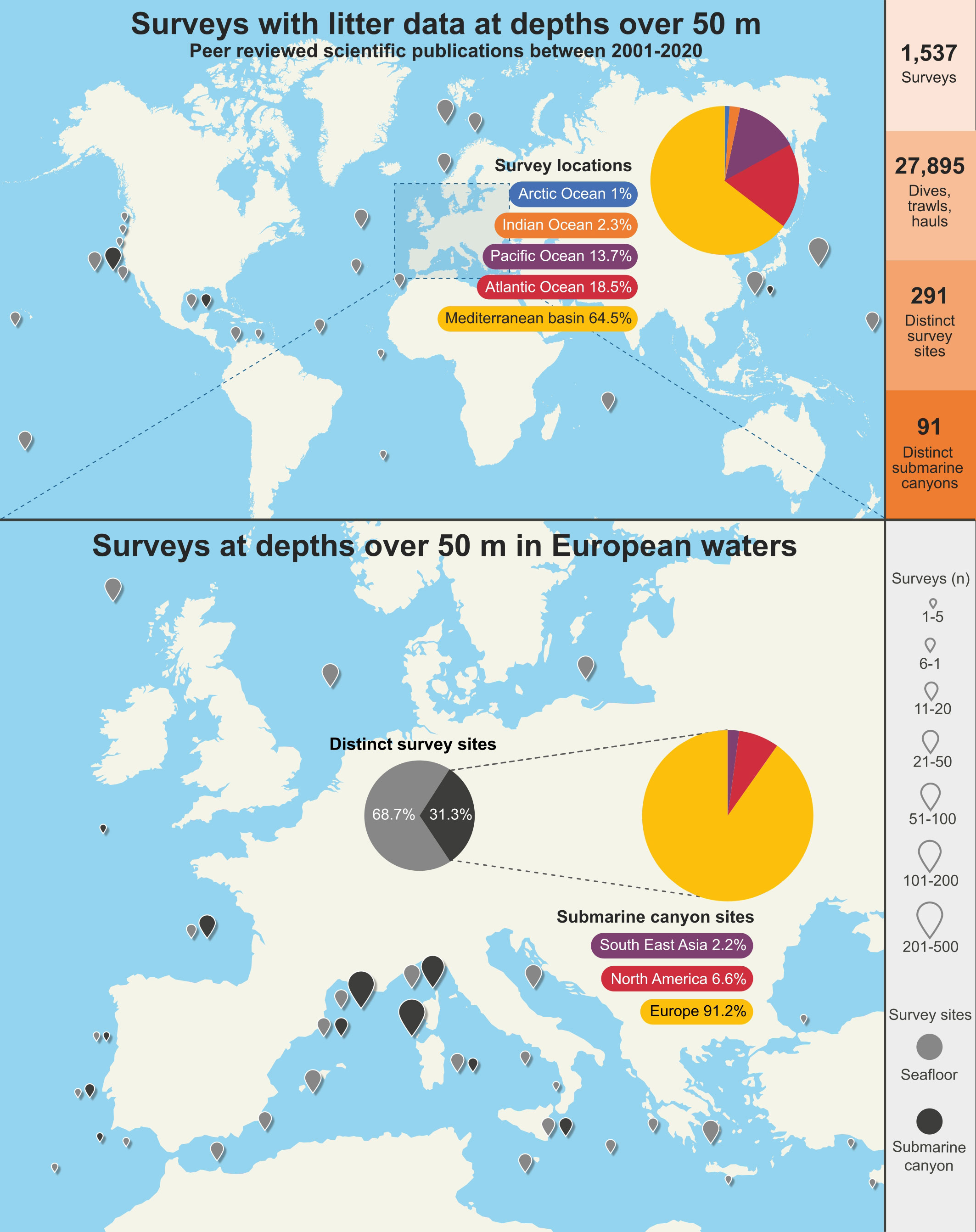
Figure 9 Surveys in the literature that contain marine litter data at depths >50 m worldwide and in European waters. Data in pie charts are representative of worldwide figures.
The highest reported litter density by far was found in four channels of the Messina Canyon, with a mean density of 521,250 ± 117,100 items km-2, over ten times higher than the next highest density recorded in the SY82 tributary canyon of the Xisha Trough (Peng et al., 2019; Pierdomenico et al., 2019b). Other heavily littered canyons in the western Mediterranean Sea and some of the most impacted canyons in the Bay of Biscay showed litter densities >10,000 items km-2. There was much variability between canyons located in the same broad geographical area indicating localised factors driving these differences. Global budgets and per broad geographical region were calculated by standardizing the canyon means with a 95% confidence to remove outliers and provide conservative estimates. The number of canyons and mean canyon area per broad geographical region from Harris et al., 2014, were used to derive global and regional total litter value ranges. Global conservative budgets of 22488 ± 6897 items km-2 in the global ocean canyons were calculated using data from the Mediterranean Sea, North Atlantic Ocean, and North Pacific Ocean, giving a range of 7.0 to 13.7 million items of macrolitter in any one canyon, and a total figure of 68 to 128 billion items of macrolitter found as a total of all the ocean’s canyons.
Litter composition was reduced to the lowest possible thematic resolution (based on the broad categories used in Figure 7), only including those groups of litter found in the canyon data (Figure 10). There are no discernible patterns between broad geographical area and litter composition, or with depth ranges either (Figure 11). Hydrographical and topographical features of individual canyons are the determining factors in the typology and distribution of litter. The Cap de Creus Canyon featured in different studies and had very different litter composition data; one study found a predominance of artificial polymers, and another found it to be fishing gear (Dominguez-Carrió, 2018; Gerigny et al., 2019).
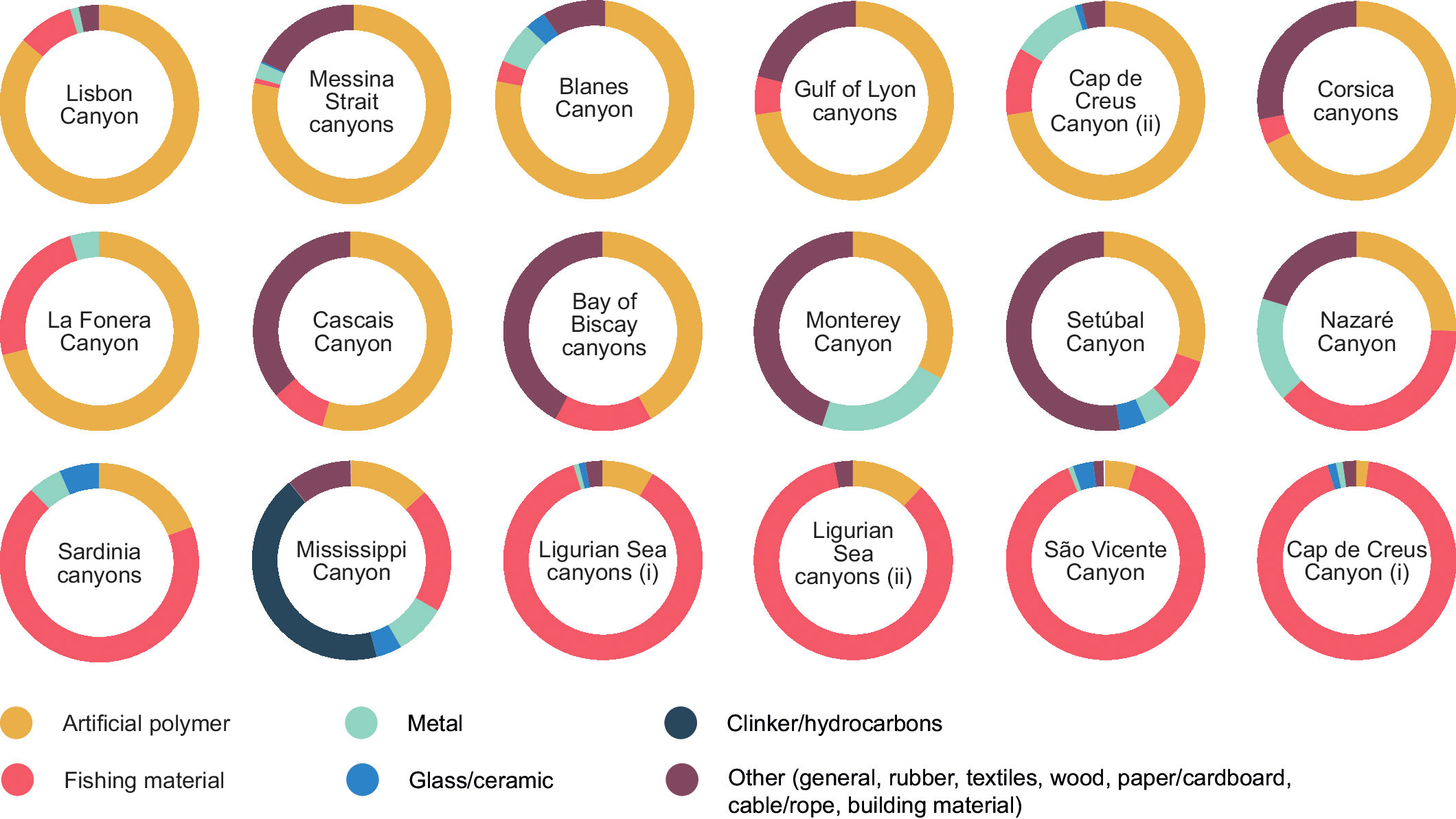
Figure 10 Low resolution litter composition in submarine canyons ordered by proportion of artificial polymer, adapted from the standardized custom 12 broad categories in Figure 7, where ‘Other’ represents building material, cable/rope, paper/cardboard, textile, rubber, wood and general/miscellaneous, for brevity and clarity. Ligurian Sea canyons were detailed in two separate studies targeting different canyons within that broad geographical area. Cap de Creus studies were kept separate as they display distinctly different litter typologies for the same site.
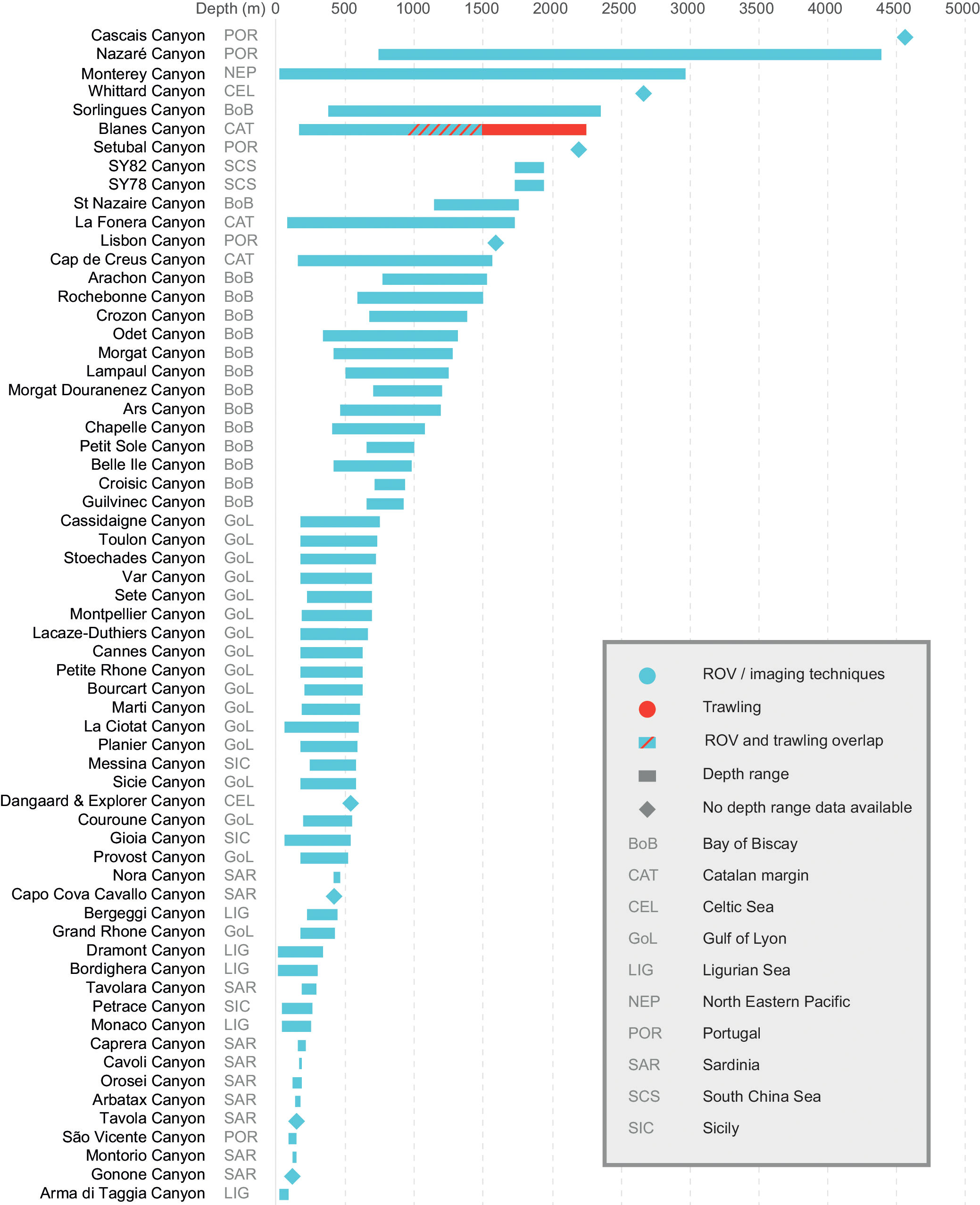
Figure 11 Depth ranges of litter surveys within canyons. Broad geographical areas for each canyon were added (codes can be found in the legend) including the main sampling technique used.
Depth ranges available for litter studies in canyons in Figure 11 reveal a clear split between shallower ranges in the Mediterranean Canyons and those in the Atlantic Ocean especially. The exceptions are the canyons in the Catalan margin which have been sampled to considerably greater depths than other Mediterranean canyons, and the São Vicente Canyon which was sampled at much shallower depths than the other Portuguese canyons. Several studies only gave data on the mean survey depth which have been included for comparative reasons and labelled accordingly.
Discussion
Analysis of canyon literature search results
Literature searches 1981-2020
There was a general increase in SFH literature over time, with CWC related publications increasing as from 2003, possibly due to technological developments making it easier to study these geomorphologically complex ecosystems. The inclusion of CWCs and marine sponges as a vulnerable marine ecosystem in UN resolution 61/105 in 2006 may have also prompted dedicated funding programs (e.g. EU framework programs) that have facilitated research into these areas (UN General Assembly, 2006). Results for submarine canyons showed a similar trajectory than CWCs, possibly for similar reasons stemming from the development of new technologies making them more attractive and feasible to study.
Searches into marine litter impacts in seafloor features and habitats
The high proportion of ‘ghost fishing’ or ‘derelict fishing gear’ publications that relate to SFH terms in Figure 4 (43% and 49% respectively) could be attributed to studies about their deleterious and nefarious consequences on habitats, and the damage to fishing gears associated with deep-sea trawling. The latter has been quantified for losses of over 1% of revenue from all fishing activities in the EU alone, (61.67M €), and although there is limited data on the damage of litter to fisheries, revenue lost from this economic activity could well be a driver for results in this area (Terzi and Seyhan, 2020). The hotspot around CWC terms combined with ‘marine litter’, ‘marine debris’ or ‘ghost fishing’ could be attributed to many studies focusing on the damage caused to CWCs by fishing activities, and because CWCs can snag litter and fishing gear (Purser et al., 2013; Lastras et al., 2016; Giusti et al., 2019; Pierdomenico et al., 2019a).
Research on deep-sea benthic macrolitter is not as prominent as other types of litter studies (such as floating, beach, or microlitter), and can be somewhat indicative of anthropocentric perspectives regarding impacts on the environment, with little regard for the effects on species and habitats far removed from direct view. Finding data on benthic deep-sea macrolitter is challenging and could benefit from promoting a central repository for recording data, such as the JAMSTEC Deep-Sea Debris database, or the LITTERBASE developed by the Alfred Wegener Institute, even if the former is more regional in nature (JAMSTEC, 2020; LITTERBASE, 2020).
Canyon searches
The top ten canyons mentioned in the literature (Table 2B) accounted for over 62% of all canyon publications found, in line with other findings where a small number of canyons dominated most of the canyon research with uneven geographical and thematical distribution (Matos et al., 2018). While the São Vicente Canyon had a sizeable proportion of the literature dedicated to selected MLI (39%), the mean overall canyon MLI proportion was 3%. This is supported by observations that some canyons have specific research foci (Matos et al., 2018) Blanes Canyon is mostly studied for anthropogenic effects (including marine litter), Cap de Creus Canyon for CWCs, density gradients and sedimentation, Nazaré Canyon for biodiversity, or the Monterey Canyon for hydrodynamic modelling. Typically, once a canyon has been studied in a field, the accumulation of background information makes it easier to continue detailed studies in the same field, as patterns derived from a single canyon may not be easy to extrapolate to others (Huvenne and Davies, 2014; Matos et al., 2018).
The type of study conducted could be crucial for whether litter data is available, given that litter is not usually the primary focus of studies in submarine canyons. Thus, given that different canyons tend to be the focus of different research areas, it could help explain the geographical disproportion in litter studies in canyons to date, such as those in European waters versus submarine canyons elsewhere. The acquisition of data of high enough resolution to enumerate litter is dependent on the purpose of the survey. For example, a geological study using acoustic techniques to characterize the seafloor will not acquire imagery or sample data to allow quantification of litter, although acoustic surveys have been used to assess and recover large items of litter such as DFG using stakeholder cooperation (Havens et al., 2011; Sullivan et al., 2019). Biological or ecological studies, however, may conduct trawl surveys or use camera systems which could allow for identification and enumeration of litter even if the purpose of the study was another.
Litter study sites in submarine canyons and in the deep sea
As can be seen in Figure 9, a sizeable majority of deep-sea litter surveys occurred in European waters, especially in the Mediterranean Sea, with a disproportionate number carried out on the northern side (European waters) which is much more densely populated and industrialized than the southern side (African waters), possibly due to the explicit inclusion of marine litter as an indicator of GES in the MSFD. Regional litter protocols such as MEDITS that allow for litter data to be collected as part of fisheries assessments and of a time series may have also been a factor. Additionally, submarine canyons studied in the European side of the Mediterranean basin tend to be close to coastal areas and connected to well-known fishing grounds, which may make them logistically easier to study for marine litter. It could be argued that developed nations have greater resources for litter studies yet there is a general scarcity of data. In North American waters only canyons in the Monterey area and the Mississippi Canyon in the Gulf of Mexico have been surveyed for marine litter (Bo et al., 2014; Enrichetti et al., 2020). In the central South China Sea basin, only two tributary canyons in the Xisha Trough have been studied (Peng et al., 2019). Overall, there is a very large gap in the knowledge, with <1% of 9,477 mapped medium-to-large canyons studied for marine litter presence and impact (Harris et al., 2014).
Data type and data acquisition
There was alignment in data types between studies that used trawling and samples, and those that used equipment for visual analysis and photographs or video. There were several instances of overlaps using data from multiple methods such as ROV and trawls in studies that consolidated data from different sites and/or sources (Pham et al., 2014; Chiba et al., 2018; Gerigny et al., 2019; Strafella et al., 2019). Most studies that used ROVs also used video data, with only 20% using photographs. During surveys photographs are taken at regular intervals, unlike video data which is continuous. Even though an adequate and representative photographic sampling scheme should be as accurate as video data, there could be litter items missed along the transect leading to an underestimation of the true amount of litter if not enough ground is covered, often a limitation in deep-sea surveys. Additionally, the camera setup may also cause issues with the ability to compare data, as oblique angled cameras may introduce an error in area estimates and should be taken into account for comparative studies.
There are associated difficulties and costs to deep-sea sampling (logistics and equipment) when compared to shallower or coastal areas, as well as advantages and disadvantages for each sampling method (Cau et al., 2018). Image sampling is non-invasive and occurs in-situ, can detect more types of litter and smaller sized items, is not harmful to benthic environments and communities and allows for direct observation of the interactions between debris and epifauna, allows for identification of smaller-scale distribution/accumulation patterns (unlike trawls), and does not remove litter that can be assessed over time (Watters et al., 2010; Eryaşar et al., 2014; Ioakeimidis et al., 2015; Canals et al., 2021). Image-based techniques can thus be used to assess the impacts of interactions and entanglement by litter, especially DFG on areas dominated by sessile suspension feeders (also known as marine animal forests) and sponges (Galgani et al., 2018; Parga Martinez et al., 2020). Another advantage of ROVs and other imaging techniques (camera frames, drop frame cameras, or TCs) is that they are not limited to use on soft bottoms or by depth like trawls are, and have been used to detect litter in the deepest parts of the ocean (Miyake et al., 2011; Chiba et al., 2018; Peng et al., 2019). Disadvantages of using imaging technology include the limitation of transect length and higher costs in comparison to trawling, with the latter usually combined with fishing assessments to reduce overheads. Additionally, videos and photographs cannot detect items which are buried beneath the surface, can be of limited use in turbid conditions and the minimum size of litter that can be observed is dependent on the resolution of the cameras (Ioakeimidis et al., 2015; Canals et al., 2021).
Trawls have their own set of challenges, from damage to seafloor environments to loss of litter due the mesh size and opening of the cod end when retrieving the trawl net (Spengler and Costa, 2008). The lower depth limit of fishing trawls used to survey litter (currently 1,500 m), the inconclusive efficacy for sampling different types of litter, their limited spatial range conducted within fishing lanes, or not being able to be used on rocky bottoms or steep bottom slopes, can lead to possible underestimations of litter densities in these morphologies (Watters et al., 2010; Harris and Whiteway, 2011; Consoli et al., 2018b; Galgani et al., 2019; Garofalo et al., 2020). Large amounts of litter can be removed by commercial bottom trawling with underestimations and unexpected low instances of litter and/or fishing gear possible due to its continuous removal by these fishing activities (Ioakeimidis et al., 2015; Alomar et al., 2020). Correlations have been found between greater presence of litter (including fishing material) and slopes with gradients >40˚ out of reach of bottom trawls (Schlining et al., 2013) There has also been a shift in types of sampling method over time. A review of twenty-six litter studies in 2008 found trawling to be the most common type of sampling method (Spengler and Costa, 2008). The corpus in this review shows that even though use of trawling has increased through time, the use of ROVs is more widespread to date (Figure 5), whereas other imaging techniques have not increased much through the years. Regardless of the techniques used to acquire deep-sea litter data, it is noteworthy that only 12% of studies in the corpus were focussed on marine litter, which is in accordance with the view that generally, litter tends to be studied opportunistically and not in its own right, relying on methodologies designed for other areas of research, such as the assessment of fish stocks (using bottom trawling), or image/video data from ecological or geological surveys that have been reanalyzed (Canals et al., 2021).
Enumeration of litter data
Over 75% of studies used abundance in some form for enumeration with the most frequent variation being per spatial area unit (n km-2, n ha-1). Abundance can be expressed when using trawls and imaging techniques, reflected in the large number of studies that use trawls and enumerated litter in both abundance and weight, similar to how biological data can be measured. Weight, however, is limited to trawling as it cannot be determined by visual means alone and was most frequently used per spatial area unit (kg km-2), with some studies arguing that weight may be better overall for quantifying of marine litter (Zablotski and Kraak, 2019). This can be true when there are too many small pieces to count, but comparisons between studies if they use different parameters (such as weight per spatial area, or weight per haul) can be difficult (Canals et al., 2021).
Abundance per spatial area unit can be considered to be the most flexible type of enumeration allowing for direct comparisons between studies even with different area units. Linear units (e.g. n km-1) may be used when the width of the field of view of the camera cannot be determined especially if there are no laser scales or when large debris items, such as fishing gears, appear to extend for hundreds of meters leading to possible overestimations in litter density if a conventional transformation to an area unit is performed (Dominguez Carrió, 2018). Comparisons using weight and abundance data are limited to using the same data types, although for monitoring purposes both can be treated as complementary. Coupling information from trawling (as a by-product of fishing operations or stock assessment) and imaging techniques, covering different substrates and depths within the same location, could give a more complete picture of the state of littering (Gerigny et al., 2019). The different ways of enumerating data given the difficulties in comparability between them denotes a lack of harmonization to the detriment of deep-sea research communities that could benefit from being able to compare and share data. Lack of standardization in litter quantification and distribution may be preventing hidden sinks and the true extent of litter impacts from being identified (Tekman et al., 2017). Thus, providing data in as many formats as possible especially since the practical problems in the comparability of data are yet to be solved, is particularly important as this research area is mostly at the baseline stage, with baseline concentrations yet to be agreed upon (Canals et al., 2021).
Litter densities in submarine canyons
Litter densities of over 100,000 items km-2 have historically been reported in areas of the Mediterranean Sea with high population density and close to anthropogenic inputs, influenced by a combination of currents, surface circulation variability and areas of high sedimentation rates (Galgani et al., 2000; Galgani, 2019). Caution must be exercised when looking at the density values in Table 3, as the data may only be representative of part of a canyon and not the whole site. A minority of studies detail where the transects took place and where samples were taken from, such as the canyon floor, thalweg, flank, or head. Most, however, do not provide that information, even if there are data from more than one dive or transect, or the depth of dives as a numerical value or site locations on a bathymetric map are provided. Conservative global budgets of up to 128 billion items of macrolitter have been estimated (Table 3) with the Mediterranean Sea canyons in total potentially having up to 46% (11.2 billion items) as much litter as the canyons in the North Pacific, which is a disproportionate amount given the size difference of the two geographical areas. Possible reasons for this could be the enclosed nature of the Mediterranean Sea and having a large number of canyons close to highly urbanized areas. These estimates, while useful, do come with important caveats, such as being based on a reduced number (36) of canyons out of 9,477 with data that was in a format of abundance per spatial area that was comparable, and the figures being representative of the entirety of the canyon areas.
The complex topographies, different morphologies, localised hydrodynamic processes, and pressures from marine litter impacts could explain the high degree of variability of litter densities, even within the same broad geographical area. The Messina Canyon shows the highest amount of litter density of all canyons studied with 521,500 items km-2 calculated from data provided from four different channels within the canyon area, ranging from a minimum of 121,000 items km-2 in the Sant’Agata channel (already twice the density of the next most littered canyon, SY82 Canyon, in Table 3) to one of the Tremestieri channels with over 1.3 million items km-2, two orders of magnitude higher than even the other most littered canyons in the Mediterranean Sea. Localised conditions could be responsible for these extraordinary densities, such as the occurrence of violent flash floods in fiumara (short but steep ephemeral streams), that are exacerbated by a densely urbanized coastal area and poor or no waste management, generating sedimentary submarine gravity flows, seafloor erosion and slope failures that can transport large numbers of litter items (Pierdomenico et al., 2019b).
Moreover, sediments in submarine canyons are transient and in flux, with episodic movements spanning tens to thousands of years before reaching their end points, which could help explain somewhat the density variability within canyons and between those in the same geographical area (Kane and Fildani, 2021). Recent studies looking at microplastics in deep-sea sediments point to intermediate depths (200 - 2000 m) and the continental rise (2000 – 4000 m) having 10 times greater loads of litter density than deeper parts of the seafloor, although trenches are known to be litter accumulation zones (Martin et al., 2022). These findings agree with other studies in two North Atlantic canyons (Norfolk Canyon and Baltimore Canyon) which show litter (both microplastics and macroplastics) to be more prevalent in the higher and intermediate sections of the canyons suggesting that macroplastics may be deposited earlier than microplastics by down-canyon currents (Jones et al., 2022). Given the timescales involved and that most canyons featured in this study (except for the Cascais Canyon and Nararé Canyon) have not been sampled at depths greater than 4000 m, it is yet unknown whether the results from studies on microplastics can be extrapolated to deeper areas of submarine canyons to show whether they remain less affected, accumulate less macroplastics or do so less rapidly than the intermediate continental slope, given the strong gravity flows that are known to occur in canyons (Kane et al., 2020).
Other localised hydrographic factors can translocate litter, as is the case of Corsica Island, that is affected by currents in the Gulf of Lyon, Ligurian Sea and Tyrrhenian Sea (Gerigny et al., 2019). The current reaching the north Tyrrhenian Sea creates a mesoscale eddy dividing the oceanic flow into two currents with opposing orientations (north and south), creating a litter accumulation zone to the north of the island (which can be aggravated by seasonal tourism activities) that cannot drain to Ligurian waters due natural topographic barriers on the seabed, whereas the southern current drains part of the litter debris to the south of Corsica (Gerigny et al., 2019). Off the French Mediterranean coast, low litter densities have been found on the continental shelf contrasting canyons adjacent to heavily urbanized areas such as Marseilles, suggesting localised hydrodynamic conditions possibly influenced by the discharge of the Rhône River, removing litter from the continental shelf into deeper areas (Galgani et al., 1996). In contrast, river flow may explain the relatively low litter densities generally recorded in some Gulf of Lyon canyons, such as high sedimentation rates in adjacent canyons of the Var River burying items of litter thus preventing them from being observed on imagery data (Galgani et al., 1996; Fabri et al., 2014; Pham et al., 2014; Gerigny et al., 2019). River discharge can be responsible for relatively high densities in some canyons in the Bay of Biscay, where the Belle-île Canyon shows the highest mean density and is believed to be heavily influenced by input from the Loire River despite being located on the widest part of the continental shelf of the Armorican margin (van den Beld et al., 2017).
In the Monterey Canyon hydrographic regimes are also thought to be responsible for litter accumulation zones, where debris swept off the continental shelf into the canyon is restricted by topographical barriers and is coupled with sediment transport processes that carry debris into deep habitats that characterize canyons as conduits for sediments and litter (Canals et al., 2006; Schlining et al., 2013). Similar patterns in movements have been observed in the South China Sea where large litter dumps are found in terrace scarps in the upper headless canyons of the Xisha trough, located approximately 150 km from land and with a favorable topography for litter to accumulate (Peng et al., 2019). The area is subject to frequent turbidity currents, receiving litter and sediment delivered by along-shelf currents, with turbidity currents having been found to transfer plastic down the canyon to the deeper parts of the ocean floor which may then be redistributed into accumulation zones (Zhong and Peng., 2021)
Strong bottom currents and periodic dense shelf water cascading events affect the Cap de Creus Canyon and could be responsible for temporal variations by moving and redistributing large amounts of litter to deeper areas where the canyon floor becomes an accumulation zone (Canals et al., 2006). Mean densities of 8,090-42,100 items km-2 in different studies have been recorded, where in one instance densities of >28,000 items km-2 were recorded at depths >1000 m, in another study the highest densities were observed at depths <400 m (Tubau et al., 2015; Dominguez-Carrió, 2018). These differences serve to demonstrate that there can be substantial variations within a canyon due to data being acquired at different depths, time periods where redistribution of litter may be taking place, or geomorphological settings within the canyon. Bottom currents have been shown to control the distribution of microplastics on the seafloor, especially those transported via submarine channels linked to terrestrial sources in the Tyrrhenian Sea, with stronger gravity currents in deeper areas capable of flushing them down the canyon even further, with greater implications for the translocation of macroplastics (Kane et al., 2020).
Litter composition in submarine canyons
There is no clear geographical separation in the litter composition affecting different canyons. Differences in enumeration were not a limiting factor when comparing litter composition between sites, as publications that used abundance in all forms, or weight as their measuring unit could be included. The distribution of DFG has been found to have a negative and non-linear effect on other types of benthic litter, dependent on the location of fishing grounds, type of fishing activities, and whether litter removal and redistribution to rocky bottoms outside of sampling capabilities takes place (Lopez-Lopez et al., 2017; Angiolillo, 2019).
As with litter density there can be marked variations in composition even within the same canyon, such as for the Cap de Creus Canyon in Figure 10 (Tubau et al., 2015; Dominguez Carrió, 2018). The land area adjacent to the canyon is a national park and is relatively unpopulated although there is considerable commercial fishing activity in the canyon with fishing concentrating along the flank areas at depths of 300–700 m. This may explain the high presence of DFG at shallower depths (Cap de Creus(i) in Figure 10), and a much greater proportion of plastic litter than fishing gear sampled at much greater depths (Cap de Creus (ii) in Figure 10), with trawling removing small items of litter from shallower depths coupled with deposition at greater depths by bottom currents and dense shelf water cascading events (Tubau et al., 2015; Dominguez Carrió, 2018). Other factors could include discharge from the Rhône River, despite being 160 km away from the canyon area, and aeolian transport by the Tramuntana winds, which can transport light items of litter from land onto the sea and cause litter accumulation in this canyon (Galgani et al., 2000; Tubau et al., 2015).
Socio-economic factors and anthropogenic activities must also be taken into account when considering differences in litter abundance and composition. Despite the relative geographical closeness of the Gulf of Lyon canyons to land, they were more affected by higher densities from fishing debris and trawling. Canyons in the Ligurian Sea, where there are smaller and more artisanal fishing fleets, saw higher densities of litter sourced from shipping and recreational activities (Fabri et al., 2014; Giusti et al., 2019). The presence of fisheries adjacent to or in canyon areas exerts a large influence on fishing litter found especially at shallower depths where fishing activities are unimpeded by depth or complex topographical terrains. This is observed in Sardinian canyons which are surrounded by a relatively low coastal population and thus a lower land-based litter input, yet small scale professional fisheries can account for up to three times higher DFG density when compared to other types of litter (Cau et al., 2017). Similarly, the “Sagres Bank” as it is known among local fishermen, can be found by the São Vicente Canyon where there is a high fishing activity correlating with a large proportion of DFG found in the canyon, although the sampling depth in this canyon was much shallower than the other Portuguese canyons (Figure 11) (Oliveira et al., 2015). The Lisbon and Setubal canyons were found to be markedly more impacted by plastic litter, due to their closer proximity to densely populated and highly industrialized areas, also receiving direct input from the Tagus River. The Nazaré Canyon appears to be much less affected by land-based litter and shows higher levels of fishing litter, in part due to the greater distance surveyed within the canyon from the coast, and greater maritime activity (Mordecai et al., 2011). Despite the Bay of Biscay being an area of high fishing activity there is a greater prevalence of plastic litter and categories combined under “other” in Figure 10. This can be partly explained by the large influence of land-based sources of litter from the Loire River and Gironde estuary, as well as the increased maritime activities from seasonal tourism in the area (Galgani et al., 2000). There is also evidence that unlike plastic litter, fishing litter is much less likely to be displaced by currents due to its size and weight since it is not found to preferentially aggregate around corals or complex habitats (van den Beld et al., 2017).
Depth ranges in canyon studies
Depth of surveys (when made available) showed a large overall range and a clear geographical separation (with some exceptions) between canyons sampled at shallower depths and those with deeper data (Figure 11). Except for the Blanes Canyon, all canyons where depth was provided were sampled using ROVs using video data mainly. An otter trawl and Agassiz trawl were used in the Blanes Canyon to depths of 2,700 m which were used for sampling megafauna, and not as part of fishing surveys as is common with other studies that use trawling on the continental shelf (Ramirez-Llodra et al., 2013). Depth ranges are largely dependent on the study in which they are conducted with some studies from the Sardinian and Ligurian Sea canyons sampling at shallower depths, whereas those off the coast of Portugal (except for São Vicente Canyon which was sampled on the canyon head and was not part of the same study) were sampled at great depths (Cau et al., 2017; Giusti et al., 2019; Mordecai et al., 2011). Caution must be exercised when trying to establish patterns between litter densities, composition, and depth ranges in different canyons unless the data are segmented accordingly, which is often not the case.
Marine litter interactions with biota
Over 29% of studies recorded interactions of marine litter with biota or the surrounding environment, with the level of observational detail varying greatly. Some studies detailed types of interactions and impacts observed (mainly studies that focussed on benthic biodiversity), whereas others only acknowledged that interactions were observed with no further comparative data provided, with little or no consistency between studies. It could be argued that studies that use trawling cannot directly view interactions that litter may have with its surroundings in-situ, unlike ROVs or other visual data-capture techniques, although some interactions like colonization, epibiosis or encrustation can still be observed on litter items collected.
Characteristics of biota, especially of coral skeletons in terms of flexibility or resistance to friction, can also play a role in the impacts endured, such as entanglement, smothering or partial necrosis (Bo et al., 2014; Kühn et al., 2015). The upright and rugose structure of biota such as corals and sponges, may render them particularly vulnerable to entanglement or smothering by litter, hence why are considered sentinels (Galgani et al., 2018). Entanglement has been observed as an impact on thirty-four taxa (mostly cnidarians), predominantly caused by fishing gears, with abrasion being the most common action that can cause breakage of biotic structures and progressive removal of tissues, making them more vulnerable to disease and covering by epibionts (Angiolillo and Fortibuoni, 2020). Coral species that are breakable are frequently observed as having debris on the surrounding area following interaction with marine litter or fishing activities (Angiolillo et al., 2015). Interactions between benthic fauna and DFG and the consequences of these (such as ghost fishing, smothering or coverage) can cause significant modifications to the structure and functioning of reef ecosystems (Angiolillo and Fortibuoni, 2020). Indeed, there is a paucity of studies at population level over time assessing how many organisms in a population are affected by litter, providing valuable information, such as a study carried out at the HAUSGARTEN observatory in the Arctic Ocean (Parga Martinez et al., 2020). Interactions with microplastics and macroplastics under controlled conditions have also been found to be species specific, with potential implications for large changes within coral community composition and subsequent biodiversity in submarine canyons and deep-sea environments (Mouchi et al., 2019)
Negative interactions may be very much underestimated, and the pressure exerted by litter on the environment may be greater than thought, outweighing any positive interactions that may occur. Of the studies that used imaging data, over 54% did not list any impacts or interactions (or lack of thereof), suggesting an important gap in the data even when interaction identification is potentially possible. Having data on litter interactions can be as important as knowing how much litter is on the seafloor. Recording interactions helps identify stresses that debris could be exerting and can help determine long-term effects that are yet relatively unknown. Other interactions that may seem advantageous at first glance, such as increased substrate heterogeneity, increased availability as substrate for colonization, shelter, or even enhancing the presence of some species, may be less so since they involve changes in diversity patterns, making the true impact of litter on benthic fauna very difficult to quantify (Mordecai et al., 2011).
Litter classification systems and marine litter protocols for submarine canyons
Studies that did not use a protocol to classify litter were in the majority, with less than 27% doing so. The main official protocols available are regional in nature and are intended for use in European waters. Official litter protocols are much more than mere classification systems, with OSPAR and MEDITS detailing the methodology for sampling (trawling), use of equipment, identification, and enumeration (MEDITS, 2017, OSPAR Commission, 2017). These two protocols also prioritize using fishing expeditions that were originally designed to provide fish stock assessments to save costs, and is cheaper than acquiring visual data (OSPAR Commission, 2017; Spedicato et al., 2020). Trawling is no longer recommended for studying submarine canyons as it can alter natural sedimentary environments, cause sediment resuspension events and can be destructive towards benthic environments. MEDITS also establishes a framework for the annual sampling of macrolitter and collection of data providing temporal and spatial comparability (Ramirez-Llodra, et al., 2013; MEDITS, 2017; Paradis et al., 2017). Temporal scales of studies are important to determine whether marine litter is increasing or decreasing and by how much, with scales of ten years or more observed in eleven studies, of which only two were in submarine canyons, although not all the timespans observed in the studies from the corpus represented continuous intervals of data (Schlining et al, 2013; Gerigny et al., 2019).
Studies that encompass large time ranges can provide a wider knowledge of litter distribution, density, patterns, and trends required for a better understanding of the pressures of marine litter on the environment (Alomar et al., 2020). Most studies outside of European waters did not follow any litter protocols, possibly due to a lack of regional protocols suitable for deep-sea litter analysis or a lack of requirement like there is in European waters with the MSFD.
Litter classification systems were used by over 95% of studies in the corpus, with over 65% using a one-tier classification system only (categories), and over 34% using a two-tier system (categories and subcategories). The mean number of subcategories shows a much higher variability (18.6 ± 11.6) than categories, whereas the mean number of categories used (7.3 ± 3.6) is lower than the twelve standardized top-level category groups derived in this review based on observation from studies in the corpus to include all main litter types found (Figure 7). The number of categories used in the official protocols is lower than the mean in the corpus (7 for MSFD, 7 for MEDITS and 6 for OSPAR), although the mean number of subcategories in the protocols was substantially higher than in the corpus (52 for MSFD, 28 for MEDITS and 39 for OSPAR) (Galgani et al., 2013; MEDITS, 2017; OSPAR Commission, 2017). The higher variability in the corpus subcategories used indicates that whereas most publications used few or no subcategories, the minority that did follow the established protocols used an elevated number of subcategories, with some studies that used the OSPAR protocol listing up to 39 litter subcategories in their classification system. High variabilities can also be observed in the publications that did follow a protocol, seen in the number of studies that used fragments of the classification system instead of the full classification systems provided. Possible reasons could be that nothing was recorded in some of the categories/subcategories, in which case there would be an underreporting of data of absence, or because items could not be identified. Many publications also added custom categories such as ‘general’ or ‘miscellaneous’ to those supplied in the protocols, especially those that followed the MSFD, indicating difficulty in placing some items of litter in any of the categories provided.
Fishing-specific top-level categories were included in over 40% of publications in the corpus (Figure 7), although fishing gear is not used as a category grouping in any of the official protocols and is usually included as a subcategory in plastics, metals, rubber, or general/miscellaneous categories. Over 10% of artificial polymer, metal and rubber subcategories in the corpus belong to fishing gear items, indicative of studies that follow the official protocols and how they methodically classify litter, with the first level classifying by material and the second level by item type. Corpus studies that do not follow any protocols may be categorizing litter in a more intuitive but less systematic manner. The discrepancy between how official protocols and how many corpus studies classify litter could point towards a difference in questions asked or prioritized. A more methodical materials-led approach may be more beneficial if trying to evaluate the physiochemical or toxicological impacts of litter. A more intuitive approach led by type of litter may be more appropriate if trying to assess possible sources or impacts derived from specific anthropogenic activities. Another possibility could be that the official protocol classification systems may not be sufficiently fitting in practice for the identification of the main types of litter found in the corpus studies, given the low adherence to the protocol classification systems available and the large number of studies that do not follow them. A separate and unified litter classification system independent of the litter sampling methods in the protocols could be used for deep-sea environments, irrespective of geographical location, maintaining a degree of flexibility to include new litter subcategories if required for types of litter that may be found in specific locations, as a solution to bridge the disconnect between the classification systems in the protocols and those used in studies.
The variety, variability, and resolution of classification systems in the corpus studies, and the loose adherence of classification systems provided by the protocols, could pose the question of the usefulness of using high-resolution classification systems, and whether doing so at a lower resolution could lead to easier litter classification. Higher-resolution data, however, allows types of litter pressures to be identified to a much greater precision allowing for specific problems caused by marine litter to be identified even if they are highly localised. At larger scales, lower resolutions may be more useful to allow comparability between studies if they are using the same, or at least similar classification systems. Recording litter data at a higher resolution, allows conversion to lower resolutions or reclassification depending on the criteria of the study, such as assessing the amount and impacts of fishing gear. Using a high-resolution system would allow for relevant subcategories within groups such as artificial polymers, metals, or rubber to be regrouped for this purpose to convert from a more methodical materials led approach to a more intuitive activity led approach. If, however, the initial recording is more intuitively led by associated activity (such as fishing gear) then it would be impossible to reclassify to estimate the amount of any given material on the seafloor, such as plastics. The lack of standardization to this respect could continue to make comparisons difficult, although having high resolution data allows for comparison between sites, such as in Figure 10 where lowering the overall thematic resolution allowed for comparison of litter composition in different submarine canyons.
Conclusions and recommendations
It is very difficult to ascertain how litter is affecting submarine canyons in different geographical areas when <1% have been studied for marine litter and only 36 canyons have data that can be used for comparative purposes to establish regional and global canyon litter budgets. Another difficulty lies in the accessibility of the data, where many studies did not comply with FAIR (Findability, Accessibility, Interoperability and Reuse) data principles, nor is data provided in accessible or comparable formats on many occasions. This is compounded by numerous knowledge gaps and lack of information of where the data was taken from within the submarine canyons, being incongruent with the acceptance of their varied topographic profiles. The following recommendations are put forward:
Standardization in classification
There is a need to standardize identification, enumeration, quantification, and classification of marine litter in deep-sea environments generally and especially within submarine canyons. At present there is no consensus on how data are reported or presented, making comparisons between studies very difficult which may preclude the true extent of litter impacts from being quantified or identified. Most studies seem to use litter classification systems based on their own findings and not based on any existing marine litter protocols available, with a dichotomy in litter classification systems. Established protocols take a more materials-based approach evident in the inclusion of fishing gear as subcategories of plastics, metal, and rubber, whereas many studies take a more intuitive functionality-based approach having fishing gear as a main category based on its prevalence and impact on benthic environments. This raises the point of whether the classification systems in the official protocols are useful or suitable for marine litter studies beyond floating, beach or coastal areas. It is not within the scope of this review to evaluate different litter protocols to this effect although having a unified and standardized approach when identifying and classifying litter that considers different resolutions of litter identification, the impact of fishing gears and the materials they are composed of, could be very beneficial.
Standardization in enumeration and quantification
Enumeration methods are varied and diverse which can be problematic when attempting to derive spatial and temporal patterns and impacts. The most flexible form of enumeration for the purpose of comparison is that of abundance in a spatial area unit, although one potential pitfall is a possible underestimation of large fishing gear, where volume could give a more appropriate estimation. A unified method of enumerating benthic litter that takes into consideration the relative size of items to account for large fishing gears would be beneficial. Studies that use weight can also give data based on abundance and the coupling of both formats could be seen as complimentary and not mutually exclusive. Indeed, until standards are introduced and harmonization across studies is improved, it would be recommended to report data in as many dimensions as possible.
Litter interactions and impacts
Interactions between marine litter and the surrounding environment/biota are very much underreported and an aspect of the research that needs to be developed further. The relatively small number of studies that have data on litter interactions and the differences between these denote an urgent requirement for standardization in this area. While recognizing that the scope of many studies may not be identification of species or biological in nature, listing interactions with benthic fauna and or abiotic structures would be of much use when assessing the impacts and any possible underestimation of negative impacts. It would be advantageous to take a sentinel approach for monitoring purposes, and if studies rely on imagery, to quantify interactions at population level where possible. Unifying the types of interactions and impacts possible would be advantageous so that the extent of these impacts, temporally and spatially, can be evaluated.
Considerations when evaluating benthic marine litter
Submarine canyons are very diverse topographic structures and as such require careful consideration when trying to understand how affected they are by marine litter. Density and composition should be considered together in submarine canyons at least, as the complex processes that take place within and in different parts of canyons can determine the amount and type of litter found. When evaluating benthic litter in canyons it is important to consider that while canyons are regarded as conduits for the transport sediments and nutrients connecting the continental shelf with deeper basins, each canyon structure is unique and is affected by a set of circumstances that may not be transposable to another canyon, even if located in a close geographic setting. Their complexities require a holistic approach to study different parts within a canyon to have a greater understanding of litter impact on the canyon as a whole. Having a single density or composition data figure from a canyon may be useful at first glance especially when comparing with other sites but does not give a full or realistic picture, especially if it is not known which part of the canyon the data is pertinent to. When evaluating litter density and composition in canyons the following factors could be considered:
a. Hydrographic regimes that occur within the canyon and in the geographical area that may be responsible for the transport of litter. River discharge even at distance may have a considerable impact on litter in canyons and must be considered.
b. Seabed barriers and topography that may prevent litter from redistributing and create accumulation zones, related to hydrographic regimes.
c. The standard canyon geomorphic settings (head, flanks, thalweg, floor, mouth) as well as depth, for more accurate segmented results.
d. Proximity of submarine canyons to urban areas of high population density and deficiencies in waste management.
e. Shipping lanes and sources of litter (land or marine).
f. Fishing activities and seasonal variations that may explain the litter composition.
g. Conservation zones or marine protected areas close to submarine canyons and their enforcement.
Data availability statement
The original contributions presented in the study are included in the article/Supplementary Material. Further inquiries can be directed to the corresponding author.
Author contributions
IH, conceptualization, methodology, formal analysis, investigation, writing – original draft, and visualization. JD, conceptualization, methodology, validation, formal analysis, investigation, resources, data curation, writing – review and editing, supervision, and project administration. VH, conceptualization, methodology, validation, formal analysis, resources, writing – review and editing, supervision, and project administration. AD, conceptualization, methodology, validation, resources, writing – review and editing, supervision, and project administration. All authors contributed to the article and approved the submitted version.
Conflict of interest
The authors declare that the research was conducted in the absence of any commercial or financial relationships that could be construed as a potential conflict of interest.
Publisher’s note
All claims expressed in this article are solely those of the authors and do not necessarily represent those of their affiliated organizations, or those of the publisher, the editors and the reviewers. Any product that may be evaluated in this article, or claim that may be made by its manufacturer, is not guaranteed or endorsed by the publisher.
Supplementary material
The Supplementary Material for this article can be found online at: https://www.frontiersin.org/articles/10.3389/fmars.2022.965612/full#supplementary-material
Abbreviations
CWCs, Cold-water corals; DFG, Derelict fishing gear; EU, European Union; GES, Good Environmental Status; GS, Google Scholar; JAMSTEC, Japan Agency for Marine-Earth Science and Technology; LITTERBASE, Online Portal for Marine Litter; MEDITS, Mediterranean International Bottom Trawl Survey; MLI, Marine litter impacts; MSFD, Marine Strategy Framework Directive; OSPAR, Oslo Paris Convention; ROV, Remotely operated vehicle; SD, Science Direct; SFH, Seafloor features and habitats; TC, Towed camera; UN, United Nations; UNEP, United Nations Environmental Programme.
References
Alomar C., Compa M., Deudero S., Guijarro B. (2020). Spatial and temporal distribution of marine litter on the seafloor of the Balearic islands (western Mediterranean Sea). Deep-Sea Res. Part I: Oceanogr. Res. Papers 155, 103178. doi: 10.1016/j.dsr.2019.103178
Alvito A., Bellodi A., Cau A., Moccia D., Mulas A., Palmas F., et al. (2018). Amount and distribution of benthic marine litter along sardinian fishing grounds (CW Mediterranean Sea). Waste Manage. 75, 131–140. doi: 10.1016/j.wasman.2018.02.026
Amon D. J., Kennedy B. R. C., Cantwell K., Suhre K., Glickson D., Shank T. M., et al. (2020). Deep-Sea debris in the central and Western pacific ocean. Front. Mar. Sci. 7. doi: 10.3389/fmars.2020.00369
Andrady A. L. (2011). Microplastics in the marine environment. Mar. Pollut. Bull. 62 (8), 1596–1605. doi: 10.1016/j.marpolbul.2011.05.030
Angiolillo M. (2019). Chapter 14 - Debris in Deep Water,World Seas.Volume III: Ecological Issues and Environmental Impacts, (Second Edition), Academic Press, (pp. 251–268). ISBN 9780128050521. doi: 10.1016/B978-0-12-805052-1.00015-2
Angiolillo M., Fortibuoni T. (2020). Impacts of marine litter on Mediterranean Reef Systems: From shallow to deep waters. Frontiers in Marine Science, 7. doi: 10.3389/fmars.2020.581966
Angiolillo M., Lorenzo B., Farcomeni A., Bo M., Bavestrello G., Santangelo G., et al. (2015). Distribution and assessment of marine debris in the deep tyrrhenian Sea (NW Mediterranean Sea, Italy). Mar. Pollut. Bull. 92 (1–2), 149–159. doi: 10.1016/j.marpolbul.2014.12.044
Avio C. G., Gorbi S., Regoli F. (2017). Plastics and microplastics in the oceans: From emerging pollutants to emerged threat. Mar. Environ. Res. 128, 2–11. doi: 10.1016/j.marenvres.2016.05.012
Barboza L. G. A., Cózar A., Gimenez B. C. G., Barros T. L., Kershaw P. J., Guilhermino L. (2018). +. In: Sheppard C. (eds) World Seas: An Environmental Evaluation Volume III: Ecological Issues and Environmental Impacts, Academic Press (pp. 305–328), ISBN 9780128050521. doi: 10.1016/B978-0-12-805052-1.00019-X
Barnes D. K. A., Galgani F., Thompson R. C., Barlaz M. (2009). Accumulation and fragmentation of plastic debris in global environments. Philos. Trans. R. Soc. B: Biol. Sci. 364 (1526), 1985–1998. doi: 10.1098/rstb.2008.0205
Baudrier J., Lefebvre A., Galgani F., Saraux C., Doray M. (2018). Optimising French fisheries surveys for marine strategy framework directive integrated ecosystem monitoring. Mar. Policy 94, 10–19. doi: 10.1016/j.marpol.2018.04.024
Bergmann M., Klages M. (2012). Increase of litter at the Arctic deep-sea observatory HAUSGARTEN. Mar. Pollut. Bull. 64 (12), 2734–2741. doi: 10.1016/j.marpolbul.2012.09.018
Bo M., Bava S., Canese S., Angiolillo M., Cattaneo-Vietti R., Bavestrello G. (2014). Fishing impact on deep Mediterranean rocky habitats as revealed by ROV investigation. Biol. Conserv. 171, 167–176. doi: 10.1016/j.biocon.2014.01.011
Borrelle S. B., Ringma J., Law K. L., Monnahan C. C., Lebreton L., McGivern A., et al. (2020). Predicted growth in plastic waste exceeds efforts to mitigate plastic pollution. Science 369 (6510), 1515–1518. doi: 10.1126/science.aba3656
Botero C. M., Zielinski S., Pereira C. I., León J. A., Dueñas L. F., Puentes V. (2020). The first report of deep-sea litter in the south-Western Caribbean Sea. Mar. Pollut. Bull. 157, 111327. doi: 10.1016/j.marpolbul.2020.111327
Buhl-Mortensen L., Buhl-Mortensen P. (2017). Marine litter in the Nordic seas: Distribution composition and abundance. Mar. Pollut. Bull. 125 (1–2), 260–270. doi: 10.1016/j.marpolbul.2017.08.048
Canals M., Pham C. K., Bergmann M., Gutow L., Hanke G., Van Sebille E., et al. (2021). The quest for seafloor macrolitter: a critical review of background knowledge, current methods and future prospects. Environ. Res. Lett. 16, 023001. doi: 10.1088/1748-9326/abc6d4
Canals M., Puig P., de Madron X. D., Heussner S., Palanques A., Fabres J. (2006). Flushing submarine canyons. Nature 444 (7117), 354–357. doi: 10.1038/nature05271
Cau A., Alvito A., Moccia D., Canese S., Pusceddu A., Rita C., et al. (2017). Submarine canyons along the upper sardinian slope (Central Western Mediterranean) as repositories for derelict fishing gears. Mar. Pollut. Bull. 123 (1–2), 357–364. doi: 10.1016/j.marpolbul.2017.09.010
Cau A., Bellodi A., Moccia D., Mulas A., Pesci P., Cannas R., et al. (2018). Dumping to the abyss: single-use marine litter invading bathyal plains of the sardinian margin (Tyrrhenian Sea). Mar. Pollut. Bull. 135, 845–851. doi: 10.1016/j.marpolbul.2018.08.007
Chiba S., Saito H., Fletcher R., Yogi T., Kayo M., Miyagi S., et al. (2018). Human footprint in the abyss: 30 year records of deep-sea plastic debris. Mar. Policy 96, 204–212. doi: 10.1016/j.marpol.2018.03.022
Company J. B., Ramirez-llodra E., Aguzzi J., Puig P., Canals M., Calafat A., et al. (2012) Submarine canyons in the Catalan Sea (NW mediterranean): megafaunal biodiversity patterns and anthropogenic threats. Mediterranean submarine canyons: Ecology and governance. Available at: https://www.researchgate.net/publication/235277627.
Consoli P., Andaloro F., Altobelli C., Battaglia P., Campagnuolo S., Canese S., et al. (2018b). Marine litter in an EBSA (Ecologically or biologically significant area) of the central Mediterranean Sea: Abundance, composition, impact on benthic species and basis for monitoring entanglement. Environ. Pollut. 236, 405–415. doi: 10.1016/j.envpol.2018.01.097
Consoli P., Falautano M., Sinopoli M., Perzia P., Canese S., Esposito V., et al. (2018a). Composition and abundance of benthic marine litter in a coastal area of the central Mediterranean Sea. Mar. Pollut. Bull. 136, 243–247. doi: 10.1016/j.marpolbul.2018.09.033
Consoli P., Romeo T., Angiolillo M., Canese S., Esposito V., Salvati E., et al. (2019). Marine litter from fishery activities in the Western Mediterranean Sea: The impact of entanglement on marine animal forests. Environ. Pollut. 249, 472–481. doi: 10.1016/j.envpol.2019.03.072
Consoli P., Sinopoli M., Deidun A., Canese S., Berti C., Andaloro F., et al. (2020). The impact of marine litter from fish aggregation devices on vulnerable marine benthic habitats of the central Mediterranean Sea. Mar. Pollut. Bull. 152, 110928. doi: 10.1016/j.marpolbul.2020.110928
Crocetta F., Riginella E., Lezzi M., Tanduo V., Balestrieri L., Rizzo L. (2020). Bottom-trawl catch composition in a highly polluted coastal area reveals multifaceted native biodiversity and complex communities of fouling organisms on litter discharge. Mar. Environ. Res. 155, 104875. doi: 10.1016/j.marenvres.2020.104875
Debrot A. O., Vinke E., van der Wende G., Hylkema A., Reed J. K. (2014). Deepwater marine litter densities and composition from submersible video-transects around the ABC-islands, Dutch Caribbean. Mar. Pollut. Bull. 88 (1–2), 361–365. doi: 10.1016/j.marpolbul.2014.08.016
Dominguez Carrió C. (2018) ROV-based ecological study and management proposals for the offshore marine protected area of cap de creus (NW Mediterranean). Available at: www.tdx.cat.
de Leo F. C., Smith C. R., Rowden A. A., Bowden D. A., Clark M. R. (2010). Submarine canyons: Hotspots of benthic biomass and productivity in the deep sea. Proceedings of the Royal Society B: Biological Sciences 277 (1695), 2783–2792. doi: 10.1098/rspb.2010.0462
du Preez C., Swan K. D., Curtis J. M. R. (2020). Cold-water corals and other vulnerable biological structures on a north pacific seamount after half a century of fishing. Front. Mar. Sci. 7. doi: 10.3389/fmars.2020.00017
Engler R. E. (2012). The complex interaction between marine debris and toxic chemicals in the ocean. Environ. Sci. Technol. 46 (22), 12302–12315. doi: 10.1021/es3027105
Enrichetti F., Dominguez-Carrió C., Toma M., Bavestrello G., Canese S., Bo M. (2020). Assessment and distribution of seafloor litter on the deep ligurian continental shelf and shelf break (NW Mediterranean Sea). Mar. Pollut. Bull. 151, 110872. doi: 10.1016/j.marpolbul.2019.110872
Eriksen M., Lebreton L. C. M., Carson H. S., Thiel M., Moore C. J., Borerro J. C., et al. (2014). Plastic pollution in the world’s oceans: More than 5 trillion plastic pieces weighing over 250,000 tons afloat at Sea. PloS One 9 (12), e111913. doi: 10.1371/journal.pone.0111913
Eryaşar A. R., Özbilgin H., Gücü A. C., Sakinan S. (2014). Marine debris in bottom trawl catches and their effects on the selectivity grids in the north eastern Mediterranean. Mar. Pollut. Bull. 81 (1), 80–84. doi: 10.1016/j.marpolbul.2014.02.017
European Commission (2010). ‘COMMISSION DECISION of 1 September 2010 on criteria and methodological standards on good environmental status of marine waters’, (2010/477/Eu) Commission Decision, (2008), p. 11. https://eur-lex.europa.eu/LexUriServ/LexUriServ.do?uri=OJ:L:2010:232:0014:0024:EN:PDF
Fabri M. C., Pedel L., Beuck L., Galgani F., Hebbeln D., Freiwald A. (2014). Megafauna of vulnerable marine ecosystems in French Mediterranean submarine canyons: Spatial distribution and anthropogenic impacts. Deep-Sea Res. Part II: Topical Stud. Oceanogr 104, 184–207. doi: 10.1016/j.dsr2.2013.06.016
Fernandez-Arcaya U., Ramirez-Llodra E., Aguzzi J., Allcock A. L., Davies J. S., Dissanayake A., et al. (2017). Ecological role of submarine canyons and need for canyon conservation: A review. Front. Mar. Sci. 4. doi: 10.3389/fmars.2017.00005
Ferrigno F., Appolloni L., Russo G. F., Sandulli R. (2018). Impact of fishing activities on different coralligenous assemblages of gulf of Naples (Italy). J. Mar. Biol. Assoc. U K 98 (1), 41–50. doi: 10.1017/S0025315417001096
Fiorentino F., Gancitano V., Giusto G. B., Massi D., Sinacori G., Titone A., et al. (2015). Marine litter on trawlable bottoms of the Strait of Sicily/Rifiuti antropici marini sui fondi strascicabili dello strettottotto di Sicilia. Biologia Marina Mediterranea 22 (1), 225–228. https://www.proquest.com/docview/1819112565
Fleet D., Vlachogianni T., Hanke G. (2021). A joint list of litter categories for marine macrolitter monitoring (Luxembourg, European Union: EUR 30348 EN Publications Office of the European Union, JRC121708), 52. doi: 10.2760/127473
Fortibuoni T., Ronchi F., Mačić V., Mandić M., Mazziotti C., Peterlin M., et al. (2019). A harmonized and coordinated assessment of the abundance and composition of seafloor litter in the Adriatic-Ionian macroregion (Mediterranean Sea). Mar. Pollut. Bull. 139, 412–426. doi: 10.1016/j.marpolbul.2019.01.017
Galgani F. (2019). Litter in the Mediterranean Sea, In: Komatsu T., Ceccaldi H. J., Yoshida J., Prouzet P., Henocque Y. (eds) Oceanography Challenges to Future Earth: Human and Natural Impacts on our Seas (Springer International Publishing), 55–67. doi: 10.1007/978-3-030-00138-4_6
Galgani F., Hanke G., Werner S., Oosterbaan L., Nilsson P., Fleet D., et al. (2013). Guidance on Monitoring of Marine Litter in European Seas. Joint Research Centre, Institute for Environment and Sustainability, Publications Office. doi: 10.2788/99475
Galgani F., Leaute J. P., Moguedet P., Souplet A., Verin Y., Carpentier A., et al. (2000). Litter on the sea floor along European coasts. Mar. Pollut. Bull. 40 (6), 516–527. doi: 10.1016/S0025-326X(99)00234-9
Galgani F., Pham C. K., Claro F., Consoli P. (2018). Marine animal forests as useful indicators of entanglement by marine litter. Mar. Pollut. Bulletin Elsevier 135 (June), 735–738. doi: 10.1016/j.marpolbul.2018.08.004
Galgani F., Souplet A., Cadious Y. (1996). Accumulation of debris on the deep sea floor off the French Mediterranean coast. Mar. Ecol. Prog. Ser. 142, 225–234. doi: 10.3354/meps142225
García-Rivera S., Lizaso J. L. S., Millán J. M. B. (2017). Composition, spatial distribution and sources of macro-marine litter on the gulf of alicante seafloor (Spanish Mediterranean). Mar. Pollut. Bull. 121 (1–2), 249–259. doi: 10.1016/j.marpolbul.2017.06.022
García-Rivera S., Lizaso J. L. S., Millán J. M. B. (2018). Spatial and temporal trends of marine litter in the Spanish Mediterranean seafloor. Mar. Pollut. Bull. 137, 252–261. doi: 10.1016/j.marpolbul.2018.09.051
Garofalo G., Quattrocchi F., Bono G., di Lorenzo M., di Maio F., Falsone F., et al. (2020). What is in our seas? assessing anthropogenic litter on the seafloor of the central Mediterranean Sea. Environ. Pollut. 266, 115213. doi: 10.1016/j.envpol.2020.115213
Gerigny O., Brun M., Fabri M. C., Tomasino C., le Moigne M., Jadaud A., et al. (2019). Seafloor litter from the continental shelf and canyons in French Mediterranean water: Distribution, typologies and trends. Mar. Pollut. Bull. 146, 653–666. doi: 10.1016/j.marpolbul.2019.07.030
Geyer R. (2020). Chapter 2 - Production, use, and fate of synthetic polymers. In: Letcher T. M. (eds) Plast. Waste Recycling Elsevier, 13–32. Academic Press. doi: 10.1016/B978-0-12-817880-5.00002-5
Giusti M., Canese S., Fourt M., Bo M., Innocenti C., Goujard A., et al. (2019). Coral forests and derelict fishing gears in submarine canyon systems of the ligurian Sea. Prog. Oceanogr 178, 102186 doi: 10.1016/j.pocean.2019.102186
Goto T., Shibata H. (2015). Changes in abundance and composition of anthropogenic marine debris on the continental slope off the pacific coast of northern Japan, after the march 2011 tohoku earthquake. Mar. Pollut. Bull. 95 (1), 234–241. doi: 10.1016/j.marpolbul.2015.04.011
Grøsvik B. E., Prokhorova T., Eriksen E., Krivosheya P., Horneland P. A., Prozorkevich D. (2018). Assessment of marine litter in the barents Sea, a part of the joint Norwegian-Russian ecosystem survey. Front. Mar. Sci. 5 (MAR). doi: 10.3389/fmars.2018.00072
Grinyó J., lo Iacono C., Pierdomenico M., Conlon S., Corbera G., Gràcia E. (2020). Evidences of human impact on megabenthic assemblages of bathyal sediments in the alboran Sea (western Mediterranean). Deep Sea Res. Part I: Oceanogr. Res. Papers 165, 103369. doi: 10.1016/j.dsr.2020.103369
Güven O., Gülyavuz H., Deval M. C. (2013). Antalya körfezi (doğu akdeniz) batial zonunda bentik çöp birikimi. Turkish J. Fish Aquat. Sci. 13 (1), 43–49. doi: 10.4194/1303-2712-v13_1_06
Halevi G., Moed H., Bar-Ilan J. (2017). Suitability of Google scholar as a source of scientific information and as a source of data for scientific evaluation–review of the literature. J. Informetrics 11, 823–834. doi: 10.1016/j.joi.2017.06.005
Harris P. T., Macmillan-Lawler M., Rupp J., Baker E. K. (2014). Geomorphology of the oceans. Mar. Geol. 352, 4–24. doi: 10.1016/j.margeo.2014.01.011
Harris P. T., Whiteway T. (2011). Global distribution of large submarine canyons: Geomorphic differences between active and passive continental margins. Mar. Geol. 285 (1–4), 69–86. doi: 10.1016/j.margeo.2011.05.008
Havens K., Bilkovic D. M., Stanhope D., Angstadt K. (2011). Fishery failure, unemployed commercial fishers, and lost blue crab pots: An unexpected success story. Environ. Sci. Policy Elsevier Ltd 14(4) pp, 445–450. doi: 10.1016/j.envsci.2011.01.002
Huvenne V. A. I., Davies J. S. (2014). Towards a new and integrated approach to submarine canyon research. Deep Sea Res. Part II: Topical Stud. Oceanogr 104, 1–5. doi: 10.1016/j.dsr2.2013.09.012
Ioakeimidis C., Papatheodorou G., Fermeli G., Streftaris N., Papathanassiou E. (2015). Use of ROV for assessing marine litter on the seafloor of saronikos gulf (Greece): A way to fill data gaps and deliver environmental education. SpringerPlus, 4, 463. doi: 10.1186/s40064-015-1248-4
Ioakeimidis C., Zeri C., Kaberi H., Galatchi M., Antoniadis K., Streftaris N., et al. (2014). A comparative study of marine litter on the seafloor of coastal areas in the Eastern Mediterranean and black seas. Mar. Pollut. Bull. 89 (1–2), 296–304. doi: 10.1016/j.marpolbul.2014.09.044
Jambeck J. R., Geyer R., Wilcox C., Siegler T. R., Perryman M., Andrady A., et al. (2015). Plastic waste inputs from land into the ocean. Science 347 (6223), 768–771. doi: 10.1126/science.1260352
JAMSTEC (2020) Deep Sea debris database. Available at: http://www.godac.jamstec.go.jp/catalog/dsdebris/e/.
Jeong S.-B., Lee D.-I., Cho H. S., Kim Y.-J. (2005). Characteristics of marine litters distribution on the seabed of the East China Sea. J. Korean Soc. Mar. Environ. Eng. 8 (4), 220–226.
Jones E. S., Ross S. W., Robertson C. M., Young C. M. (2022). Distributions of microplastics and larger anthropogenic debris in Norfolk Canyon, Baltimore Canyon, and the adjacent continental slope (Western North Atlantic Margin, U.S.A.). Marine Pollution Bulletin 174, 113047. doi: 10.1016/j.marpolbul.2021.113047
Kammann U., Aust M. O., Bahl H., Lang T. (2018). Marine litter at the seafloor - abundance and composition in the north Sea and the Baltic Sea. Mar. Pollut. Bull. 127, 774–780. doi: 10.1016/j.marpolbul.2017.09.051
Kane I. A., Clare M. A. (2019). Dispersion, accumulation, and the ultimate fate of microplastics in deep-marine environments: A review and future directions. In Frontiers in Earth Science, 7. doi: 10.3389/feart.2019.00080
Kane I. A., Clare M. A., Miramontes E., Wogelius R., Rothwell J. J., Garreau P., et al. (2020). Seafloor microplastic hotspots controlled by deep-sea circulation. Science 368 (6495), 1140–1145. doi: 10.1126/science.aba5899
Kane I. A., Fildani A. (2021). Anthropogenic pollution in deep-marine sedimentary systems–a geological perspective on the plastic problem. Geol. 49 (5), 607–608. doi: 10.1130/focus052021.1
Keller A. A., Fruh E. L., Johnson M. M., Simon V., McGourty C. (2010). Distribution and abundance of anthropogenic marine debris along the shelf and slope of the US West coast. Mar. Pollut. Bull. 60 (5), 692–700. doi: 10.1016/j.marpolbul.2009.12.006
Koutsodendris A., Papatheodorou G., Kougiourouki O., Georgiadis M. (2008). Benthic marine litter in four gulfs in Greece, Eastern mediterranean; abundance, composition and source identification. Estuarine Coast. Shelf Sci. 77 (3), 501–512. doi: 10.1016/j.ecss.2007.10.011
Kühn S., Bravo Revolledo E., van Franeker J. (2015). Deleterious effects of litter on marine life. In: Bergmann M., Gutow L., Klages &M. (eds) Marine Anthropogenic Litter (75–116). Springer, Cham. doi: 10.1007/978-3-319-16510-3
Kuroda M., Uchida K., Tokai T., Miyamoto Y., Mukai T., Imai K., et al. (2020). The current state of marine debris on the seafloor in offshore area around Japan. Mar. Pollut. Bull. 161, 111670. doi: 10.1016/j.marpolbul.2020.111670
Lastras G., Canals M., Ballesteros E., Gili J. M., Sanchez-Vidal A. (2016). Cold-water corals and anthropogenic impacts in la fonera submarine canyon head, northwestern Mediterranean Sea. PloS One 11 (5), e0155729. doi: 10.1371/journal.pone.0155729
Lee D. I., Cho H. S., Jeong S. B. (2006). Distribution characteristics of marine litter on the seabed of the East China Sea and the south Sea of Korea. Estuarine Coast. Shelf Sci. 70 (1–2), 187–194. doi: 10.1016/j.ecss.2006.06.003
Lefkaditou E., Karkani M., Anastasopoulou A., Kavadas S., Christidis G., Mytilineou C. (2013). Litter composition on the shelf and upper slope of the argosaronikos region and the eastern Ionian Sea, as evidenced by MEDITS surveys 1995-2008. ICES CM 2013/A 3357, 1–3. Available at: https://www.ices.dk/sites/pub/CM%20Doccuments/CM-2013/Theme%20Session%20A%20contributions/A0613.pdf
LITTERBASE (2020) Online portal for marine litter. Available at: https://litterbase.awi.de/litter.
Lopez-Lopez L., González-Irusta J. M., Punzón A., Serrano A. (2017). Benthic litter distribution on circalittoral and deep sea bottoms of the southern bay of Biscay: Analysis of potential drivers. Continental Shelf Res. 144, 112–119. doi: 10.1016/j.csr.2017.07.003
Loulad S., Houssa R., Rhinane H., Boumaaz A., Benazzouz A. (2017). Spatial distribution of marine debris on the seafloor of Moroccan waters. Mar. Pollut. Bull. 124 (1), 303–313. doi: 10.1016/j.marpolbul.2017.07.022
Macfadyen G., Huntington T., Cappell R. (2009) Abandoned, lost or otherwise discarded fishing gear, FAO consutlants, lymington. united kingdom of great Britain and northern Ireland, FAO fisheries and aquaculture technical paper. Available at: http://www.unep.org/regionalseas/marinelitter/publications/default.asp.
Maes T., Barry J., Leslie H. A., Vethaak A. D., Nicolaus E. E. M., Law R. J., et al. (2018). Below the surface: Twenty-five years of seafloor litter monitoring in coastal seas of north West europe 1992–2017). Sci. Total Environ. 630, 790–798. doi: 10.1016/j.scitotenv.2018.02.245
Martín-Martín A., Orduna-Malea E., Thelwall M., Delgado López-Cózar E. (2018). Google Scholar, web of science, and scopus: A systematic comparison of citations in 252 subject categories. J. Informetrics 12 (4), 1160–1177. doi: 10.1016/j.joi.2018.09.002
Martin C., Young C. A., Valluzzi L., Duarte C. M. (2022). Ocean sediments as the global sink for marine micro- and mesoplastics. Limnology Oceanogr Lett. 7 (3), 235–243. doi: 10.1002/lol2.10257
Matos F. L., Ross S. W., Huvenne V. A. I., Davies J. S., Cunha M. R. (2018). Canyons pride and prejudice: Exploring the submarine canyon research landscape, a history of geographic and thematic bias. Prog. Oceanogr 169, 6–19. doi: 10.1016/j.pocean.2018.04.010
Mecho A., Francescangeli M., Ercilla G., Fanelli E., Estrada F., Valencia J., et al. (2020). Deep-sea litter in the gulf of cadiz (Northeastern Atlantic, Spain). Mar. Pollut. Bull. 153, 110969. doi: 10.1016/j.marpolbul.2020.110969
MEDITS-Handbook. Version 9 (2017) Instruction manual of Mediterranean international bottom trawl survey. Available at: http://www.sibm.it/MEDITS2011/principaleproject.htm.
Melli V., Angiolillo M., Ronchi F., Canese S., Giovanardi O., Querin S., et al. (2017). The first assessment of marine debris in a site of community importance in the north-western Adriatic Sea (Mediterranean Sea). Mar. Pollut. Bull. 114 (2), 821–830. doi: 10.1016/j.marpolbul.2016.11.012
Mifsud R., Dimech M., Schembri P. J. (2013). Marine litter from circalittoral and deeper bottoms off the Maltese islands (Central Mediterranean). Mediterr. Mar. Sci. 14 (2), 298–308. doi: 10.12681/mms.413
Miyake H., Shibata H., Furushima Y. (2011). Deep-sea litter study using deep-sea observation tools. In: Omori K., Guo X., Yoshie N., Fujii N., Handoh I. C., Isobe A., et al (eds) Interdisciplinary Studies on Environmental Chemistry - Marine Environmental Modeling and Analysis, 261–269. TERRAPUB. https://www.researchgate.net/publication/266245893_Deep-Sea_Litter_Study_Using_Deep-Sea_Observation_Tools.
Moed H. F., Bar-Ilan J., Halevi G. (2016). A new methodology for comparing Google scholar and scopus. J. Informetrics 10 (2), 533–551. doi: 10.1016/j.joi.2016.04.017
Mordecai G., Tyler P. A., Masson D. G., Huvenne V. A. I. (2011). Litter in submarine canyons off the west coast of Portugal. Deep Sea Res. Part II: Topical Stud. Oceanogr 58 (23–24), 2489–2496. doi: 10.1016/j.dsr2.2011.08.009
Moriarty M., Pedreschi D., Stokes D., Dransfeld L., Reid D. G. (2016). Spatial and temporal analysis of litter in the celtic Sea from groundfish survey data: Lessons for monitoring. Mar. Pollut. Bull. 103 (1–2), 195–205. doi: 10.1016/j.marpolbul.2015.12.019
Mouchi V., Chapron L., Per E., Prusk A. M., Meistertzhei A. L., Vétio G., et al. (2019). Long-term aquaria study suggests species-specific responses of two cold-water corals to macro-and microplastics exposure. Environ. Pollut 253 pp, 322–329. doi: 10.1016/j.envpol.2019.07.024
Napper I. E., Thompson R. C. (2019). Marine plastic pollution: Other than microplastic. In: Letcher T. M., Vallero D. A. (eds) Waste (Second Edition), 425–442. Academic Press. doi: 10.1016/b978-0-12-815060-3.00022-0
Neves D., Sobral P., Pereira T. (2015). Marine litter in bottom trawls off the Portuguese coast. Mar. Pollut. Bull. 99 (1–2), 301–304. doi: 10.1016/j.marpolbul.2015.07.044
Olguner M. T., Olguner C., Mutlu E., Deval M. C. (2018). Distribution and composition of benthic marine litter on the shelf of antalya in the eastern Mediterranean. Mar. Pollut. Bull. 136, 171–176. doi: 10.1016/j.marpolbul.2018.09.020
Oliveira F., Monteiro P., Bentes L., Henriques N. S., Aguilar R., Gonçalves J. M. S. (2015). Marine litter in the upper são Vicente submarine canyon (SW portugal): Abundance, distribution, composition and fauna interactions. Mar. Pollut. Bull. 97 (1–2), 401–407. doi: 10.1016/j.marpolbul.2015.05.060
OSPAR Commission (2017) ‘CEMP Guidelines on Litter on the Seafloor’, OSPAR Agreement 2017-06. EIHA 17/9/1 Annex 12. https://www.ospar.org/documents?d=37515
Page M. J., McKenzie J. E., Bossuyt P. M., Boutron I., Hoffmann T. C., Mulrow C. D., et al. (2021). The PRISMA 2020 statement: an updated guideline for reporting systematic reviews. BMJ 372, n71. doi: 10.1136/bmj.n71
Papadopoulou K.-N., Smith C. J., Apostolidis C. H., Karachle P. K. (2015). Trawled up marine litter, first observations from Heraklion Bay. 11th Panhellenic Symposium on Oceanography and Fisheries, 373–376. https://www.researchgate.net/publication/281118473
Paradis S., Puig P., Masqué P., Juan-Díaz X., Martín J., Palanques A. (2017). Bottom-trawling along submarine canyons impacts deep sedimentary regimes. Scientific Reports, 7 (1), 43332. doi: 10.1038/srep43332
Parga Martínez K. B., Tekman M. B., Bergmann M. (2020). Temporal trends in marine litter at three stations of the HAUSGARTEN observatory in the Arctic deep Sea. Front. Mar. Science 7(May) pp. doi: 10.3389/fmars.2020.00321
Pasquini G., Ronchi F., Strafella P., Scarcella G., Fortibuoni T. (2016). Seabed litter composition, distribution and sources in the northern and central Adriatic Sea (Mediterranean). Waste Manage. 58, 41–51. doi: 10.1016/j.wasman.2016.08.038
Peng X., Dasgupta S., Zhong G., Du M., Xu H., Chen M., et al. (2019). Large Debris dumps in the northern south China Sea. Mar. Pollut. Bull. 142, 164–168. doi: 10.1016/j.marpolbul.2019.03.041
Pham C. K., Gomes-Pereira J. N., Isidro E. J., Santos R. S., Morato T. (2013). Abundance of litter on condor seamount (Azores, Portugal, northeast Atlantic) (Deep-Sea Research Part II: Topical Studies in Oceanography, 98(PA), 204–208). doi: 10.1016/j.dsr2.2013.01.011
Pham C. K., Ramirez-Llodra E., Alt C. H. S., Amaro T., Bergmann M., Canals M., et al. (2014). …Marine litter distribution and density in European seas, from the shelves to deep basins. PloS One 9 (4), e95839. doi: 10.1371/journal.pone.0095839
Pierdomenico M., Cardone F., Carluccio A., Casalbore D., Chiocci F., Maiorano P., et al. (2019a). Megafauna distribution along active submarine canyons of the central Mediterranean: Relationships with environmental variables. Prog. Oceanogr 171, 49–69. doi: 10.1016/j.pocean.2018.12.015
Pierdomenico M., Casalbore D., Chiocci F. L. (2019b). Massive benthic litter funnelled to deep sea by flash-flood generated hyperpycnal flows. Sci. Rep. 9 (1), 5330. doi: 10.1038/s41598-019-41816-8
Pierdomenico M., Casalbore D., Chiocci F. L. (2020). The key role of canyons in funnelling litter to the deep sea: A study of the gioia canyon (Southern tyrrhenian Sea). Anthropocene 30, 100237. doi: 10.1016/j.ancene.2020.100237
Purser A., Orejas C., Gori A., Tong R., Unnithan V., Thomsen L. (2013). Local variation in the distribution of benthic megafauna species associated with cold-water coral reefs on the Norwegian margin. Continental Shelf Res. 54, 37–51. doi: 10.1016/j.csr.2012.12.013
Ramirez-Llodra E., de Mol B., Company J. B., Coll M., Sardà F. (2013). Effects of natural and anthropogenic processes in the distribution of marine litter in the deep Mediterranean Sea. Prog. Oceanogr 118, 273–287. doi: 10.1016/j.pocean.2013.07.027
Ramirez-Llodra E., Tyler P. A., Baker M. C., Bergstad O. A., Clark M. R., Escobar E., et al. (2011). Man and the last great wilderness: Human impact on the deep Sea. PLoS One 6 (8), e22588. doi: 10.1371/journal.pone.0022588
Rhinane H., Houssa R., Loulad S. (2019). The seafloor marine debris on the north and the central part of the Moroccan Atlantic waters from tangier (35n) to sidi ifni (29n): composition, abundance, spatial distribution, sources and movement (Remote Sensing and Spatial Information Sciences: ISPRS - International Archives of the Photogrammetry). doi: 10.5194/isprs-archives-xlii-4-w19-377-2019
Rodríguez Y., Pham C. K. (2017). Marine litter on the seafloor of the faial-pico passage, Azores archipelago. Mar. Pollut. Bull. 116 (1–2), 448–453. doi: 10.1016/j.marpolbul.2017.01.018
Ryan P. G., Weideman E. A., Perold V., Durholtz D., Fairweather T. P. (2020). A trawl survey of seafloor macrolitter on the south African continental shelf (150: Marine Pollution Bulletin). doi: 10.1016/j.marpolbul.2019.110741
Sánchez P., Masó M., Sáez R., De Juan S., Muntadas A., Demestre M.. (2013). Estudio de referencia sobre la distribución de basura marina en fondos blandos asociados a caladeros de pesca de arrastre en el Mediterráneo norte. Scientia Marina 77 (2), 247–255. doi: 10.3989/scimar03702.10A
Schlining K., von Thun S., Kuhnz L., Schlining B., Lundsten L., Jacobsen Stout N., et al. (2013). Debris in the deep: Using a 22-year video annotation database to survey marine litter in Monterey canyon, central California, USA. Deep-Sea Res. Part I: Oceanogr. Res. Papers 79, 96–105. doi: 10.1016/j.dsr.2013.05.006
Shimanaga M., Yanagi K. (2016). The Ryukyu trench may function as a “depocenter”. anthropogenic Mar. litterJ. Oceanogr 72 (6), 895–903. doi: 10.1007/s10872-016-0388-7
Sinopoli M., Cillari T., Andaloro F., Berti C., Consoli P., Galgani F., et al. (2020). Are FADs a significant source of marine litter? assessment of released debris and mitigation strategy in the Mediterranean Sea (253: Journal of Environmental Management). doi: 10.1016/j.jenvman.2019.109749
Song X., Lyu M., Zhang X., Ruthensteiner B., Ahn I., Pastorino G., et al. (2021). Large Plastic debris dumps: New biodiversity hot spots emerging on the deep-Sea floor. Environ. Sci. Technol. Letters 8(2), pp. 148–154. doi: 10.1021/acs.estlett.0c00967
Spedicato M. T., Massutí E., Mérigot B., Tserpes G., Jadaud A., Relini G. (2020). The MEDITS trawl survey specifications in an ecosystem approach to fishery management. Scientia Marina 83 (S1), 9. doi: 10.3989/scimar.04915.11X
Spedicato M. T., Zupa W., Carbonara P., Fiorentino F., Follesa M. C., Galgani F., et al. (2019). Spatial distribution of marine macro-litter on the seafloor in the northern Mediterranean Sea: The MEDITS initiative. Scientia Marina 83 (S1), 257–270. doi: 10.3989/scimar.04987.14A
Spengler A., Costa M. F. (2008). Methods applied in studies of benthic marine debris. Mar. Pollut. Bull. 56 (2), 226–230. doi: 10.1016/j.marpolbul.2007.09.040
Stefatos A., Charalampakis M., Papatheodorou G., Ferentinos G. (1999). Marine debris on the seafloor of the Mediterranean Sea: Examples from two enclosed gulfs in Western Greece. Mar. Pollut. Bull. 38 (5), 389–393. doi: 10.1016/S0025-326X(98)00141-6
Strafella P., Fabi G., Despalatovic M., Cvitković I., Fortibuoni T., Gomiero A., et al. (2019). Assessment of seabed litter in the northern and central Adriatic Sea (Mediterranean) over six years. Mar. Pollut. Bull. 141, 24–35. doi: 10.1016/j.marpolbul.2018.12.054
Strafella P., Fabi G., Spagnolo A., Grati F., Polidori P., Punzo E., et al. (2015). Spatial pattern and weight of seabed marine litter in the northern and central Adriatic Sea. Mar. Pollut. Bull. 91 (1), 120–127. doi: 10.1016/j.marpolbul.2014.12.018
Suaria G., Aliani S. (2014). Floating debris in the Mediterranean Sea. Mar. Pollut. Bull. 86 (1–2), 494–504. doi: 10.1016/j.marpolbul.2014.06.025
Sullivan M., Straub P., Reding M., Robinson N., Zimmermann E. (2019). Identification, recovery, and impact of ghost fishing gear in the mullica river-great bay estuary (New Jersey, USA): Stakeholder-driven restoration for smaller-scale systems. Mar. Pollut. Bulletin Elsevier 138(November 2018) pp, 37–48. doi: 10.1016/j.marpolbul.2018.10.058
Tekman M. B., Krumpen T., Bergmann M. (2017). Marine litter on deep Arctic seafloor continues to increase and spreads to the north at the HAUSGARTEN observatory. Deep-Sea Res. Part I: Oceanogr. Res. Papers 120(June 2016), 88–99. doi: 10.1016/j.dsr.2016.12.011
Terzi Y., Syhan K. (2020). Marine plastics in the fishing grounds in the Black Sea. In: Aytan Ü., Pogojeva M., Simeonova A. (eds) Marine Litter in the Black Sea (pp 151–160. Turkish Marine Research Foundation (TUDAV) Publication No: 56, Istanbul, Turkey, ISBN 978-975-8825-48-6
Topçu E. N., Öztürk B. (2010). Abundance and composition of solid waste materials on the western part of the Turkish black sea seabed. Aquat. Ecosystem Health Manage. 13 (3), 301–306. doi: 10.1080/14634988.2010.503684
Tubau X., Canals M., Lastras G., Rayo X., Rivera J., Amblas D. (2015). Marine litter on the floor of deep submarine canyons of the northwestern Mediterranean Sea: The role of hydrodynamic processes. Prog. Oceanogr 134, 379–403. doi: 10.1016/j.pocean.2015.03.013
UN General Assembly (2006). Resolution 61/105 ‘Sustainable fisheries, including through the 1995 agreement for the implementation of the provisions of the united nations convention on the law of the Sea of 10 December 1982 relating to the conservation and management of straddling fish stocks and highly migratory fish stocks, and related instruments. pp1–p21. https://documents-dds-ny.un.org/doc/UNDOC/GEN/N06/500/73/PDF/N0650073.pdf?OpenElement
UNEP (2005). Marine litter: An analytical overview. intergovernmental oceanographic commission of the united nations educational, scientific and cultural organisation Vol. 58 (Nairobi: UNEP).
UNEP (2009). ‘Marine litter: A global challenge’. Regional Seas U Nations Environ. Programme p, 13. Available at: https://stg-wedocs.unep.org/bitstream/handle/20.500.11822/31632/MLAGC.pdf
Urban-Malinga B., Wodzinowski T., Witalis B., Zalewski M., Radtke K., Grygiel W. (2018). Marine litter on the seafloor of the southern Baltic. Mar. Pollut. Bull. 127, 612–617. doi: 10.1016/j.marpolbul.2017.12.052
van den Beld I. M. J., Guillaumont B., Menot L., Bayle C., Arnaud-Haond S., Bourillet J.-F. (2017). Marine litter in submarine canyons of the bay of Biscay. Deep Sea Res. Part II: Topical Stud. Oceanogr 145, 142–152. doi: 10.1016/j.dsr2.2016.04.013
van Sebille E., Aliani S., Law K. L., Maximenko N., Alsina J. M., Bagaev A., et al. (2020). The physical oceanography of the transport of floating marine debris the physical oceanography of the transport of floating marine debris. Environ. Res. Letters 15(2) p, 023003. doi: 10.1088/1748-9326/ab6d7d
Vieira R. P., Raposo I. P., Sobral P., Gonçalves J. M. S., Bell K. L. C., Cunha M. R. (2015). Lost fishing gear and litter at gorringe bank (NE Atlantic). J. Sea Res. 100, 91–98. doi: 10.1016/j.seares.2014.10.005
Ward C. P., Reddy C. M. (2020). We need better data about the environmental persistence of plastic goods. Proc. Natl. Acad. Sci. U S A 117 (26), 14618–14621. doi: 10.1073/pnas.2008009117
Watters D. L., Yoklavich M. M., Love M. S., Schroeder D. M. (2010). Assessing marine debris in deep seafloor habitats off California. Mar. Pollut. Bull. 60 (1), 131–138. doi: 10.1016/j.marpolbul.2009.08.019
Wei C. L., Rowe G. T., Nunnally C. C., Wicksten M. K. (2012). Anthropogenic “Litter” and macrophyte detritus in the deep northern gulf of Mexico. Mar. Pollut. Bull. 64 (5), 966–973. doi: 10.1016/j.marpolbul.2012.02.015
Woodall L. C., Robinson L. F., Rogers A. D., Narayanaswamy B. E., Paterson G. L. J. (2015). Deep-sea litter: A comparison of seamounts, banks and a ridge in the Atlantic and Indian oceans reveals both environmental and anthropogenic factors impact accumulation and composition. Front. Mar. Sci. 2 (FEB). doi: 10.3389/fmars.2015.00003
Yamakita T., Yamamoto H., Yokoyama Y., Sakamoto I., Tsuchida S., Lindsay D., et al. (2015). Distribution of the marine debris on seafloor from the primary report of five cruises after the great East Japan earthquake 2011. In: Ceccaldi H. J., Hénocque Y., Koike Y., Komatsu T., Stora G., Tusseau-Vuillemin M. H., et al (eds) Marine Productivity: Perturbations and Resilience of Socio-ecosystems (pp. 101–109). Springer, Cham. doi: 10.1007/978-3-319-13878-7_11
Ye S., Andrady A. L. (1991). Fouling of floating plastic debris under Biscayne bay exposure conditions. Mar. Pollut. Bull. 22 (12), 608–613. doi: 10.1016/0025-326X(91)90249-R
Zablotski Y., Kraak S. B. M. (2019). Marine litter on the Baltic seafloor collected by the international fish-trawl survey. Mar. Pollut. Bull. 141, 448–461. doi: 10.1016/j.marpolbul.2019.02.014
Zhang F., Yao C., Xu J., Zhu L., Peng G., Li D. (2020). Composition, spatial distribution and sources of plastic litter on the East China Sea floor. Sci. Total Environ Elsevier B.V 742, 140525. doi: 10.1016/j.scitotenv.2020.140525
Keywords: submarine canyons, marine litter, plastic pollution, marine debris, macrolitter, benthic litter, deep-sea litter, deep-sea debris
Citation: Hernandez I, Davies JS, Huvenne VAI and Dissanayake A (2022) Marine litter in submarine canyons: A systematic review and critical synthesis. Front. Mar. Sci. 9:965612. doi: 10.3389/fmars.2022.965612
Received: 09 June 2022; Accepted: 04 August 2022;
Published: 02 September 2022.
Edited by:
Clara F. Rodrigues, University of Aveiro, PortugalReviewed by:
Fantina Madricardo, National Research Council (CNR), ItalyMelanie Bergmann, Alfred Wegener Institute Helmholtz Centre for Polar and Marine Research (AWI), Germany
Michela Angiolillo, Istituto Superiore per la Protezione e la Ricerca Ambientale (ISPRA), Italy
Copyright © 2022 Hernandez, Davies, Huvenne and Dissanayake. This is an open-access article distributed under the terms of the Creative Commons Attribution License (CC BY). The use, distribution or reproduction in other forums is permitted, provided the original author(s) and the copyright owner(s) are credited and that the original publication in this journal is cited, in accordance with accepted academic practice. No use, distribution or reproduction is permitted which does not comply with these terms.
*Correspondence: Awantha Dissanayake, QXdhbnRoYS5EaXNzYW5heWFrZUB1bmlnaWIuZWR1Lmdp
†These authors have contributed equally to this work and share senior authorship