- 1Pritzker Laboratory for Molecular Systematics and Evolution, The Field Museum, Chicago, IL, United States
- 2Delaware Biotechnology Institute, Center for Bioinformatics & Computational Biology, University of Delaware, Newark, DE, United States
- 3Research and Conservation, Georgia Aquarium, Atlanta, GA, United States
Public aquaria showcase aquatic life while raising awareness and educating the public on biodiversity and the need for conservation. Recently, aquaria have followed in the footsteps of zoos by taking more directed approaches in species conservation as well as leveraging animals in their collection to fill biological knowledge gaps through research. Similar to zoos, aquaria are able to house animals that are not feasible to care for in traditional academic settings, allowing important life history information to be gained. In particular, our understanding of reproductive aspects of large migratory fishes such as elasmobranchs (sharks, skates and rays) have benefited from information gleaned in aquarium settings that would otherwise be difficult or impossible to study in the natural environment. For example, the ability of elasmobranchs to reproduce parthenogenetically was discovered through observation of sharks maintained in public aquaria. Since its discovery, parthenogenesis has been observed for many ex situ shark and ray species. Aquaria have made other important contributions to characterizing elasmobranch reproductive biology such as understanding reproductive cycling and trialing assisted reproductive techniques, among others. Here, we review the role aquaria have played in the field of elasmobranch reproductive biology and discuss how zoos and aquariums can continue to contribute to this field in the future.
Introduction
Elasmobranchs (sharks, skates, and rays) are an important group of mostly marine organisms that comprise approximately 1,200 species (White and Last, 2012). Although they are a charismatic group of fishes, playing important predatory roles in marine food webs, research on this taxon has lagged behind that of other vertebrates. Elasmobranchs tend to be wide-ranging, cryptic animals that are challenging to sample and this likely contributes to their underrepresentation in the literature. As such, there is still much to learn regarding elasmobranch species-specific life history characteristics. In particular, certain biological aspects (such as reproduction) can only be studied in situ as single point-in-time measurements, resulting in patterns being surmised from sampling many individuals once over the course of a study. While this information is valuable, noise in the dataset due to individual variability may hamper deeper understanding of complex processes in the natural environment.
Public aquaria (herein referred to as “aquaria”) have recently followed in the footsteps of zoos by taking more directed approaches in species conservation as well as using animals in their care to fill biological knowledge gaps through research. Indeed, aquaria are able to house animals that are difficult or impossible to care for in traditional academic settings, allowing important life history information to be gained. Importantly, aquaria can study the same individual(s) over time, allowing researchers to examine both seasonal and age-related effects on physiology.
One of the more important aspects of elasmobranch life history that has benefited from aquarium collections is reproductive biology. Some of the first observations on egg laying in elasmobranchs came from the Brighton Aquarium in the late 1800s (Koob, 2004). Aquaria have also played an important role in characterizing mating behavior in elasmobranchs. Clark (1963) described premating behavior in Lemon Sharks (Negaprion brevirostris) at the Seaquarium in Miami, Florida. Other studies have described ex situ mating behavior in more detail (Uchida et al., 1990; Henningsen et al., 2004) and these behaviors have been confirmed in wild populations (Nordell, 1994; Pratt and Carrier, 2001; Whitney et al., 2004). Aquaria have played an unparalleled role in their ability to track reproductive behavior and physiology year-round for extended periods of time on the same individual(s) (Henningsen et al., 2008; Nozu et al., 2018; Sheldon et al., 2018; Murakumo et al., 2020; Wyffels et al., 2020a; Wyffels et al., 2022). For example, regular physical examinations have enabled hormone cycling fluctuations to be paired with associated changes in reproductive organs (e.g. ovaries, testes) using ultrasonography (Nau et al., 2018; Wyffels et al., 2020a; Wyffels et al., 2022). Aquaria also provide a platform for the development and in-house testing of new technologies that can then be applied in the field (Payne et al., 2015; Dove et al., 2021). Here, we focus on the contributions of research conducted in an aquarium setting to the understanding of the unique and varied reproductive biology of elasmobranchs, much of which would be nearly impossible to carry out on free-ranging in situ wild populations. Because mating behavior has been extensively reviewed, it will not be covered here in detail; rather, the discussion will be centered on reproductive physiology patterns that are now understood from studying animals ex situ.
Areas of advancement
Reproductive cycling
Traditionally, in situ reproductive biology of elasmobranch species has been studied as single points in time, either destructively (Wourms, 1977; Pratt, 1988; Natanson and Gervelis, 2013) or non-lethally (Sulikowski et al., 2007; Awruch et al., 2008; Wyffels et al., 2020a). While both approaches provide important information for describing basic biology, they cannot capture temporal changes within individuals, leading to inference being used to estimate natural reproductive cycling based on observations from the sum of individuals sampled within a natural population. This approach underrepresents natural variation among individuals, and can, at times, lead to discrepancies in accurately describing the reproductive cycle of a species. For example, in the Smalltooth Sawfish (Pristis pectinata) reproduction was estimated to occur on a biennial cycle in situ (Carlson and Simpfendorfer, 2015; Feldheim et al., 2017), but was observed to occur annually ex situ (Flowers et al., 2020), indicating that females are physiologically capable of reproducing every year. Animals held in human care have access to ample food resources and, potentially, reduced stressors, which could remove energetic obstacles that may result in animals having prolonged time between breeding cycles in situ. Importantly, Smalltooth Sawfish are critically endangered, and their biennial reproduction could be a result of resource limitation(s) such as food or mates in situ (Flowers et al., 2020), the latter of which may contribute to why parthenogenesis has been observed in this wild population of elasmobranchs (Fields et al., 2015). Nevertheless, documentation of this important life history discrepancy through information gleaned from aquaria has important implications for recovery and management of this severely impacted species. If Smalltooth Sawfish are capable of reproducing every year, instead of every other year, the ability of wild stocks to recover may be better than previously thought, assuming the obstacles preventing them from reproducing biennially are removed.
Unexpected observations of fetal habits have been documented thanks to pregnancy and parturition events in aquaria. For example, through repeated ultrasound, it was discovered that embryos of the Tawny Nurse Shark (Nebrius ferrugineus) move between uteri during gestation (Tomita et al., 2019). At parturition, the transition in respiration from in utero buccal pumping to ram ventilation was observed for the Tiger Shark (Galeocerdo cuvier; Tomita et al., 2018). These and other interesting but ephemeral characteristics of elasmobranch reproduction are only possible through cooperation and collaboration with aquaria.
Similarly, rest periods between reproductive events are nearly impossible to confirm in the wild. Data from individuals held in aquaria have allowed scientists to estimate the reproductive timing of species, which is important life history information for species management in situ. For example, Sand Tiger Sharks (Carcharias taurus) were assumed to reproduce every year using traditional lethal methods of research (Gilmore, 1993) and hypothesized to reproduce biennially or triennially based on photo-identification and residency patterns (Bansemer and Bennett, 2008; Bansemer and Bennett, 2009). However, recent examinations of female reproductive cycling in aquaria unequivocally shows reproduction every other or every two years, with resting between cycles (Wyffels et al., 2022). Similarly, embryonic development across gestation was described in a pair of ex situ Reef Manta Rays (Mobula alfredi), providing baseline information that can be used to guide gestational staging in the field (Murakumo et al., 2020). Obtaining these critical reproductive timelines would be impossible to achieve otherwise and is important to inform and guide management of protected species.
Routine examination of animals while in human care can also enable research to determine what environmental cues (e.g. changes in temperature, photoperiod, and/or salinity) may be important for reproduction (Smale et al., 2004). Considering that many aquaria hold environmental conditions constant, removing these natural cycles reveals which species are more sensitive to these cues to properly time reproductive activity and which species are more adaptable (Henningsen et al., 2004). For example, Sand Tiger Sharks have traditionally been difficult to breed in aquarium settings, but recent successes appear to be closely related to providing both seasonally fluctuating light and temperature (Wyffels et al., 2020b). Similarly, because some aquaria keep animals in different conditions, it provides a powerful platform to compare and contrast among institutions that would be difficult to do otherwise for large, charismatic megafauna. For instance, previous work by the Churaumi Aquarium has shown season-dependent cycling of female Zebra Shark (Stegostoma tigrinium) reproductive hormones and egg laying patterns with natural fluctuations in sea water temperature (Nozu et al., 2018). This approach is in contrast to many North American institutions that keep constant temperatures and have less synchronized breeding cycles (Watson and Janse, 2017; Wyffels and Lyons, pers. obs.). For example, continuous egg laying is observed year-round for some Zebra Shark females held in aquaria (Adams et al., 2022), a pattern that is likely atypical from a natural cycle where there would be a distinct reproductive period. Current research is being conducted to determine what effect (if any) the lack of environmental cues has on influencing reproductive cycling in this species. By contrast, other species appear to have no challenges breeding in managed settings regardless of environmental cues, such as Cownose Rays (Rhinoptera bonasus; Sheldon et al., 2018), Southern Stingrays (Hypanus americana; Henningsen, 2000) and carpet sharks (Chiloscyllium spp.; Wyffels et al., 2021). The variety of species held in human care provides a rich platform to understand the mechanisms underpinning species reproductive biology and the factors that drive reproductive cycling.
Mating in aquaria has also shed light on female choice in elasmobranchs (Lyons et al., 2021). For example, female Sand Tiger Sharks exhibit retaliatory biting of males that try to copulate with them (Gordon, 1993). They also practice “shielding” where they swim very close to the substrate (Gordon, 1993). Shielding has also been shown in female Epaulette Sharks (Hemiscyllium ocellatum; West and Carter, 1990). A female Sand Tiger Shark at UnderWater World SEA LIFE Mooloolaba, Australia, was observed wedging her body between rocky outcrops on or near the bottom to avoid unwanted male copulation (Willson and Smith, 2017). These behaviors have the effect of preventing mating, thus demonstrating females employing pre-copulatory mate choice. Because aquaria are able to track and dictate group composition within habitats, there are unique opportunities for aquaria to take the lead on understanding the role of female choice for elasmobranch mating systems.
Corresponding to observation of reproductive behaviors, aquaria can take more deliberate steps to genetically test offspring. This information can play a role in linking reproductive behavior with genetic outcomes to further our understanding of female choice and multiple paternity (Heist and Feldheim, 2004), a widespread phenomenon across elasmobranch species both in situ (reviewed in Bester-van der Merwe et al., 2022) and ex situ (Janse et al., 2013; Townsend et al., 2015). Performing parentage analysis will provide institutions with an indication of which individuals are genetically overcontributing or undercontributing to the regional studbook and will enable facilities to coordinate breeding efforts effectively (Janse et al., 2013; Hook, 2019). Thus, coordinated breeding efforts informed by genetic parentage can both serve to help sustainably source animals for display within the aquarium community, reducing the need to source animals from the wild, as well as lay the groundwork for possible in situ conservation work (i.e. reinforcement or reintroduction programs).
Reproductive life history parameters
Along with reproductive cycling, other important reproductive life history parameters have been described for a number of species maintained in aquarium collections, several aspects of which are only obtainable through long-term monitoring of individuals across collections. For example, some of the most basic life history parameters (e.g. age at maturity, litter size, longevity, senescence, etc.) are unknown for most shark species. By leveraging the expert husbandry provided to animals in aquaria, individuals can be tracked for many years, sometimes across multiple decades, elucidating some of these life history parameters. At times, information gathered through aquarium collections can corroborate what has been estimated in the field. For example, Zebra Sharks obtained at 6 months of age reached reproductive maturity at approximately 6 years of age for females and 7.5 years of age for the male (Watson and Janse, 2017), which corroborates what is estimated for their in situ population (Ebert et al., 2021). For other species, age/size at maturity can, in essence, only be gathered through aquarium collections because the animals are rare and/or extremely difficult to study in situ. For example, sexual maturity was recently described for a male Reef Manta Ray born at Okinawa Churaumi Aquarium and a resident Whale Shark (Rhincodon typus), where aquarists were able to monitor blood hormones and correlate increased titers with clasper morphology to confirm maturity, as well as document when sex-specific behaviors (i.e. mating) commenced (Nozu et al., 2017; Matsumoto et al., 2019). Information collected by this research group provided essential baseline biological information for species that are challenging to study in situ at the same level of detail.
One outstanding parameter that is not well understood in elasmobranchs, yet is critically important for species management, is longevity. Current methods and tools available often require lethal sampling to obtain hard structures (e.g. vertebrae, eye lens, etc.; Cailliet, 2015) in an attempt to use growth markers to estimate age. However, many of these methods are undesirable because obtaining sufficient data across a range of size classes is difficult or impossible, and many of these methods have shown to be rather inaccurate (Burke et al., 2020) or unable to be validated (Nielsen et al., 2016). For example, vertebral “growth ring” aging of Sand Tiger Sharks estimated lifespan to reach 34 years for males and 40 years for females; however, the authors state outright the inadequacy of the method to accurately age this species beyond 12 years (Passerotti et al., 2014). Individual Sand Tiger Sharks that have been mature and in aquaria collections for 30 years or more will undoubtedly provide more accurate information on the maximum potential longevity of this species (Wyffels et al., 2022). Determination of maximum lifespan in ex situ animals will allow for current and future aging methods to be validated so that in situ animals can be aged more accurately and determine if they reach similar lifespans as their ex situ counterparts.
For oviparous species, in particular, aspects of their reproductive life history (such as fecundity and embryo development time) are particularly difficult to estimate in the field but are crucially important. Despite the limitations of field samples, fecundity is a fairly reliable metric that can be estimated for viviparous species because the number of young can directly be associated with an individual female, assuming she does not spontaneously abort prior to sampling (Wosnick et al., 2018). For oviparous species, point-in-time sampling to quantify fecundity is not possible since females lay clutches of eggs over a reproductive period that can last for months (Wyffels et al., 2021; Adams et al., 2022). Furthermore, variance in fecundity is possibly more variable in oviparous species than viviparous species because the former do not have a physical limitation on available body space to carry young. For example, ex situ egg laying activity of the Barndoor Skate (Dipturus laevis) demonstrated these animals laid variable numbers of eggs in consecutive years, but also confirmed year-round laying activity as well as a longer incubation time than previously thought (Parent et al., 2008). Likewise, reproductive output of individual female Zebra Sharks has been documented from aquaria worldwide (Watson and Janse, 2017). Alongside fecundity, gestation for viviparous species and incubation period for oviparous species, are important reproductive life history parameters. Gestation time can be estimated for synchronously reproducing in situ species, but incubation time requires temporal observations of the same egg cases over time to monitor embryo development, which is easily accomplished in aquaria (Luer and Gilbert, 1985; Castro et al., 1988; Wyffels et al., 2006; Harahush et al., 2007; Rodda and Seymour, 2008). Furthermore, by observing animals in managed care, the impact of global warming and other anthropogenic environmental changes on development time can be investigated (Pistevos et al., 2015; Musa et al., 2020; Ripley et al., 2021; Wheeler et al., 2021). For oviparous species, leveraging aquarium collections to describe fundamental life history characteristics is important for proper species management, and at times, can only be obtained through observations in aquaria.
Reproductive pathologies
The ability to observe the same individuals throughout complete reproductive cycles in the controlled setting of an aquarium, versus unpredictable field conditions, in combination with the availability of advanced diagnostic tools including endoscopy, radiology and ultrasonography, has led to the discovery of reproductive pathologies among elasmobranchs in aquaria (George et al., 2017). Overall, the incidence of reproductive pathologies is relatively low (2.4%) compared to other causes of death among elasmobranchs in aquaria (Garner, 2013).
Viviparous sharks and rays that readily reproduce in aquaria sometimes suffer from dystocia, a difficult or obstructed labor, which is life threatening for the female and the fetus. The cause of dystocia is typically attributed to abnormal fetal positioning, anatomy, and/or an oversized fetus, but insufficient cervical dilation or congestion in the birth canal can also prevent a successful birth. An oversized fetus may be a consequence of delayed parturition whereby the fetus has more time to accumulate size and mass before birth. Over-conditioning (i.e. ample food provisioning) of ex situ females may lead to high birth-weights and exacerbate dystocia (Jung-Schroers et al., 2015).
Over-gestation and dystocia were suspected for a Sand Tiger Shark that gave birth (unassisted) to a female that was either stillborn or died soon after birth (Wyffels et al., 2020a). The neonate was longer in length and heavier in mass than that reported for the smallest free-swimming conspecifics in situ, suggesting the large size of the fetus may have contributed to its death (Wyffels et al., 2020a) and explain why stillborn, full-term young have been reported repeatedly for Sand Tiger Sharks in aquaria (Henningsen et al., 2017; Willson and Smith, 2017). A similar case in a Blacktip Reef Shark (Carcharhinus melanopterus) ended with negative consequences for the oversized young, which died in utero, and the female, which succumbed to infection two days after a cesarean delivery (Jung-Schroers et al., 2015). Other sharks in managed care that have been affected by dystocia include a Bonnethead Shark (Sphyrna tiburo; Stoskopf, 2017) and a Blacktip Shark (Carcharhinus limbatus; Mylniczenko et al., 2017).
Dystocia has been observed on multiple occasions for Cownose Rays in aquaria (Cavalcante et al., 2016; George et al., 2017), and it has also been suggested as a cause of mortality associated with capture for in situ rays (Leite et al., 2020). Procedures for cesarean delivery and monitoring have been developed for Cownose Rays, and, if adopted, should minimize neonate and female loss for animals in aquaria. Prevention of dystocia due to neonate size requires the gestation period of the species to be documented, and that the start of gestation be known, so that intervention can be planned for the end of gestation to ensure survival of the female and offspring (George et al., 2017). Insufficient cervical dilation and congestion in the birth canal necessitated an assisted birth for a Whitespotted Eagle Ray (Aetobatus narinari; Mahon et al., 2004). The neonate was delivered, but its tail was entangled with gills and required amputation in order for the newborn to swim and thus respire (Mahon et al., 2004). Three previous pregnancies for this female resulted in stillborn young that were fully developed and term, suggesting dystocia may have been a contributing factor.
Southern Stingrays in aquaria are prone to reproductive disease that may be related to age and/or their lack of reproduction (Mylniczenko et al., 2019). Southern Stingrays are prolific and readily reproduce in aquaria (Henningsen, 2000), and because of this, they are often maintained in aquaria as single-sex groups in an effort to limit reproduction. It is not known if the disease develops because of a lack of reproduction or other factors such as advanced age, but disease was not observed among the smaller but mature and actively reproducing in situ rays examined (Mylniczenko et al., 2019). The reproductive disease manifests in the ovary, which becomes enlarged, congested, and comprised of a large number of ovarian cysts and variably sized follicles. The uterus and vestigial right uterus of rays with severe disease is often enlarged, sometimes with cysts in the uterine wall, and filled with copious fluid, presumed to be histotroph despite their non-pregnant reproductive state (Mylniczenko et al., 2019). The increase in size of reproductive organs translates to less space for digestive organs and eventually the rays become emaciated and succumb to the disease.
Female Sand Tiger Sharks are prone to another type of reproduction-related complication known as egg-retention metritis (George et al., 2017). Females in aquaria readily ovulate during their reproductive cycle, but if they do not become pregnant, the egg cases sometimes remain in the uterus for much longer than the gestation period (Wyffels et al., 2022). Egg cases are a rich source of nutrition and if they are not spontaneously released, this can lead to bacterial metritis that may result in septicemia and death (George et al., 2017). Fortunately, with routine reproductive monitoring to follow the cycle of females and understand the possible timeframe for egg-retention, uterine lavage is an effective way to remove inspissated and degrading egg cases and prevent more serious complications (George et al., 2017; Wyffels et al., 2022).
Although not life-threatening, the reproductive cycle of male elasmobranchs may also be impacted by managed care, as evidenced by the comparatively poor quality of semen for aquarium versus in situ Sand Tiger Sharks. Low sperm number and poor motility in ejaculates from aquarium sharks may contribute to the lack of successful reproduction observed for this species in managed care (Wyffels et al., 2020a), and demonstrates the need to more thoroughly understand the sensitivity of individual species to environmental cues or other factors missing ex situ, such as nutrition.
Contraception
While the ability to have successfully breeding individuals in aquaria can provide important information about basic life history parameters, it is not always desirable because it can compromise the ability to manage populations both within and among institutions. Maintaining single-sex collections is not always possible nor are resources always available to support the young generated from indiscriminate breeding, especially for species that are relatively fecund or cycle frequently. Furthermore, there can be a need to control reproduction in certain segments of the ex situ population if a few animals are genetically overrepresented (Janse and Luten, 2019), which could lead to future inbreeding. Therefore, aquaria have been some of the first to trial modern methods to limit reproduction in elasmobranch fishes.
Three general options are available for facilities to reduce undesired procreation: physical separation, surgical, and hormonal. Physical separation of sexes may be managed within an institution as well as through relocation. Individual animals can be transferred to other institutions, ideally in coordination through species studbook managers (see below); however, availability of monetary and logistical resources may hamper this option as a reliable means of managing collection species, especially for large-bodied elasmobranchs. Therefore, employing contraception methods, with permanent or temporary sterilization, aims to achieve stable collection populations. Since little research has been performed in this arena, it leaves the field open for a number of future research pathways. For studies based in surgery, ovariectomy has been used successfully in Southern Stingrays with few complications when animals were selected at appropriate sizes for the procedure (George et al., 2017). However, it should be noted that ovariectomy as a means of birth control was an indirect result of maintaining a single-sex population where animals suffered from reproductive disease that may have been caused by or have been exacerbated by the lack of pregnancy cycles that are a part of their normal life history (see above section). To our knowledge, similar procedures to control reproduction from the male perspective have not been performed, and represents another avenue of exploration.
While limited, more work has been conducted using hormonal means to suppress reproduction, possibly due to lower risk of life-threatening complications associated with implanting or injecting hormones compared with more invasive surgical techniques (George et al., 2017). Hormonal methods have the advantage of a potential reversal to enable future reproduction, if and when desired. The hypothalamic–pituitary–gonadal axis represents the endocrine pathway that hormonally regulates reproduction (Awruch, 2013), and most studies have targeted the first step in this cascade by limiting the release of gonadotropin-releasing hormone (GnRH) in the hypothalamus. Although elasmobranchs appear to use several forms of GnRH (Lovejoy et al., 1992), one of these may be mammalian GnRH (Calvin et al., 1993), which is significant, as several agonistic and antagonistic pharmaceuticals have already been manufactured and implemented successfully in mammals to suppress reproduction (Agnew et al., 2021). Thus, employing similar pharmaceutical hormone therapies in sharks and rays may prove beneficial. The most commonly trialed form of hormonal contraception in male and female elasmobranchs has been Suprelorin® implants (deslorelin, a GnRH agonist), with mixed success. For example, pregnancy was suppressed for 22 months in a single female Eagle Ray (Aetobatus ocellatus) given a deslorelin implant, despite copulation and conspecifics reproducing successfully in the same system (Janse and Luten, 2019). By contrast, deslorelin implants were unsuccessful in male Zebra Sharks and Short-tailed Stingrays (Bathytoshia brevicaudata), as well as female Largespot River Stingrays (Potamotrygon falkneri; Daly and Jones, 2017). Antide, a GnRH antagonist, has also been trialed in male Sand Tiger Sharks and resulted in decreased, although variable, reproductive hormone levels (Henningsen et al., 2015); however, whether these reductions were associated with significantly reduced semen quality and ultimately prevented reproduction was not investigated.
Overall, contraception of elasmobranchs as a reliable method to regulate ex situ populations remains unknown. Of the few published studies, sample sizes are limited to one or a handful of individuals, which does not have the statistical power to test if these methods are effective at preventing reproduction. In addition, the longevity of implants and how frequently they need to be administered is also unclear, which is necessary in order to have effective contraception year-round, likely necessary at a species-specific level. Aquaria have the ability to leverage their diverse collections to conduct well-designed studies aimed at answering these questions.
Parthenogenesis
Parthenogenesis is the process whereby an embryo develops from a female gamete without contribution of male sperm (Lampert, 2008). The ability of elasmobranchs to reproduce unisexually was first recognized through observations in aquaria, where females were noted producing young in the absence of males (Chapman et al., 2007). Since it first being noted in a Bonnethead Shark, parthenogenesis has been documented for many elasmobranch species using a variety of reproductive modes (Table 1). The underlying mechanisms leading females to reproduce parthenogenetically are unknown; however, aquarium collections play an important role in understanding the processes behind this interesting biological phenomenon, which is extremely difficult, if not near impossible, to study in situ.
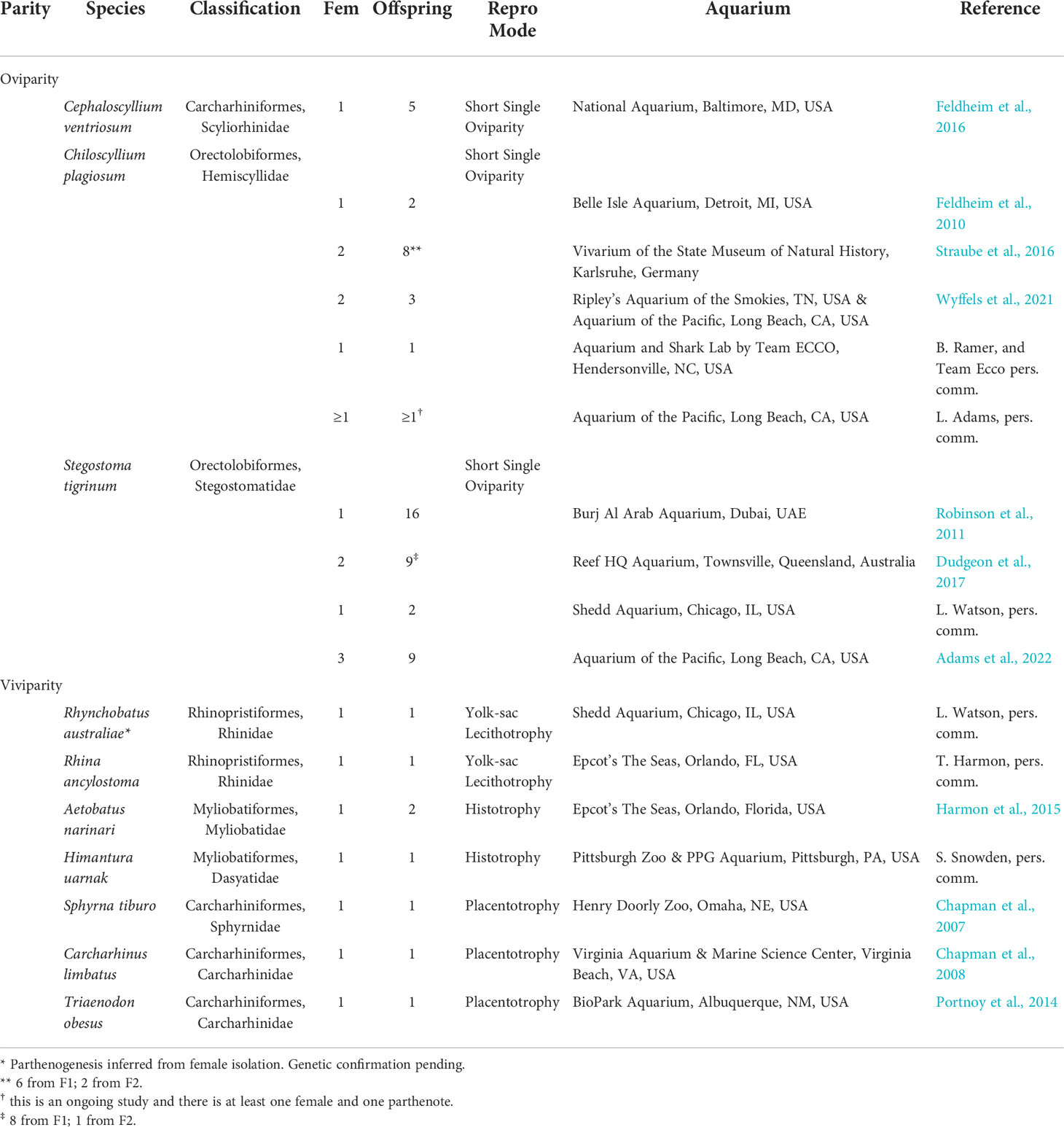
Table 1 Cases of parthenogenesis from aquaria. Fem indicates the number of females in that study that gave birth via parthenogenesis. Offspring represents the number of parthenotes in the study. Repro Mode is the reproductive mode of the species.
Parthenogenesis has been documented across a variety of shark and ray species, and at times, independently in the same species at multiple aquaria. For example, parthenogenesis in Zebra Sharks has been observed in three different countries across the world (Australia, United Arab Emirates, and United States) and has been documented in animals producing parthenotes yearly (Robinson et al., 2011) and switching between sexual and unisexual reproduction (Dudgeon et al., 2017; Adams et al., 2022). Likewise, parthenogenesis has been observed on several, independent occasions in the Whitespotted Bamboo Shark (Chiloscyllium plagiosum; Feldheim et al., 2010; Straube et al., 2016; Wyffels et al., 2021). Reproduction by parthenogenesis across multiple years is not restricted to oviparous species. In the Whitespotted Eagle Ray, a female gave birth to parthenogenic offspring in consecutive years (Harmon et al., 2015).
Considering how often this phenomenon has been described simply as a byproduct of females laying fertile eggs in the absence of males, it raises questions about how often parthenogenesis occurs when it is explicitly studied. For instance, parthenogenesis has been documented to occur alongside sexual reproduction in the Whitespotted Bamboo Shark (Wyffels et al., 2021) and the Zebra Shark (Adams et al., 2022); however, it is likely that the only reason why parthenogenesis was detected at all in these studies is that every hatchling was genotyped to determine the success of artificial insemination techniques, not to determine rates of parthenogenesis itself. Thus, in general, the impetus for examining young born in aquaria for parthenogenesis usually only occurs when young are produced at a time when females are maintained as a single sex population and/or when they have not been housed with males for an extended period of time. However, two independent artificial insemination studies (Wyffels et al., 2021; Adams et al., 2022) demonstrate that even in the presence of semen, females still reproduced parthenogenetically. Therefore, it is likely that the known instances of parthenogenesis represent only a small portion of its true occurrence for elasmobranchs in aquaria and in situ populations.
Sperm storage
Sperm storage is a common trait in many animal taxa (Holt and Lloyd, 2010; Holt and Fazeli, 2016) and serves the purpose of uncoupling copulation from ovulation, allowing females flexibility as to when they fertilize their eggs. Some elasmobranchs store sperm in specialized tubules of the oviducal gland (Pratt, 1993; Dutilloy and Dunn, 2020; Jordan et al., 2021). Although the occurrence of sperm storage is known for some species, the duration sperm remain viable once stored by females can only be ascertained in an aquarium setting. To date, only a few studies have addressed this question. Luer et al. (2007) inseminated Clearnose Skate (Rostroraja eglanteria) females with freshly collected and extended semen and found females were able to use this sperm for insemination over the next ten weeks; however, genetic testing was not performed and sireship was only assumed based on the successful development of an embryo. In a similar study, Wyffels et al. (2021) found Whitespotted Bamboo Shark females were able to fertilize eggs (genetically confirmed paternity) up to 17 weeks after insemination.
The longest duration of sperm storage documented for an elasmobranch occurred at the Steinhart Aquarium when a female Brown-Banded Bamboo Shark (Chiloscyllium punctatum) laid an egg that resulted in a viable hatchling after 45 months of isolation from a male. Importantly, the authors genotyped the putative mothers and the hatchling and discovered the offspring had levels of heterozygosity similar to those of its mother, consistent with sexual reproduction (Bernal et al., 2014). This indicates that sperm can remain viable in a female’s reproductive tract for up to four years in this species. The duration of viable sperm storage has been speculated in wild-caught specimens (Pratt, 1993); however, ex situ monitoring is the only way to truly quantify the length of time females store sperm for future use. With the ability to definitively document when males and females were together, combined with access to subsequent reproductive products (i.e. eggs or live-birth young), aquaria are well poised to quantitatively monitor duration of sperm storage in a variety of elasmobranch species.
Artificial insemination
Despite being practiced in terrestrial mammals for more than two centuries (Foote, 2002), artificial insemination (AI) is a relatively new technology used to support elasmobranch ex situ breeding. In other taxa, AI has been used to maintain genetic diversity or introduce novel genes into threatened populations (Hildebrandt et al., 2012; Howard et al., 2016; Fox et al., 2018). In addition to maintaining genetic diversity, aquaria can also use AI in elasmobranchs to avoid logistically challenging and expensive transport of individuals between institutions. AI allows for the maintenance of female-only groups, eliminating the risk of injury from male harassment. This approach has the additional benefit of reducing the requirement to maintain extra animals on reserve for display when animals in main exhibits need to be rehabilitated behind-the-scenes, thus, decreasing husbandry costs (Wildt, 2000).
Use of AI in any species, including elasmobranchs, requires detailed knowledge of the reproductive biology of the target species, including the potential for female sperm storage, effective dose of sperm for successful insemination, whether or not the species reproduces seasonally, timing and control of ovulation, and genetic compatibility with potential mates (i.e. inbreeding avoidance). In addition, safe animal handling procedures are needed to minimize potential stress during AI. To date, AI has been successfully used to produce young in the following species: Cloudy Catshark (Scyliorhinus torazame; Masuda et al., 2003), Whitespotted Bamboo Sharks (Masuda et al., 2005; Wyffels et al., 2021), Clearnose Skate (Luer et al., 2007) and Zebra Sharks (Adams et al., 2022). In addition, there are anecdotal reports from Brown-Banded Bamboo Sharks (Daly and Jones, 2017; Lance Adams, pers. comm.) and most recently Sand Tiger Sharks (Robert George, pers. comm.). However, not all of these studies performed genetic testing of offspring to confirm paternity, which is important considering that sexual reproduction via AI can occur concurrently with parthenogenesis (see above; Wyffels et al., 2021; Adams et al., 2022). Furthermore, use of AI has been generally limited to oviparous species, with little exploration in viviparous systems, which have more complex reproductive biology. Considering the diversity of elasmobranchs across aquarium collections, AI technologies should also be applied to a broader range of species to determine the viability of this technique to maintain genetically diverse ex situ populations.
Coordinated breeding programs
Zoos and aquaria have a pivotal role to play in maintaining sustainable populations of ex situ elasmobranchs as well as contributing to in situ conservation efforts (Janse et al., 2017). In 2017, it was determined that elasmobranch species with an IUCN status of “threatened,” or greater, were overrepresented in zoo and aquarium collections with respect to the number of threatened species globally in situ compared to other vertebrate taxa (Buckley et al., 2018). In other words, zoos and aquaria demonstrate bias in their collections toward displaying elasmobranch species of conservation concern. While these animals act as ambassadors for their species (or genera), this bias raises questions about sustainable practices and ethical sourcing of animals, especially for species where removal from the wild could impact already struggling populations. Buckley et al. (2018) describe methods for evaluating species sustainability in zoos and aquaria as well as outline the need for regular assessments as part of the permitting process for collecting animals from the wild for public display. In essence, zoos and aquaria should hold themselves to a higher accountability standard when proposing to source animals from the wild to maintain their collections.
The development of ex situ breeding programs would not only enable self-sustaining managed populations, but also could help restock wild populations (Conde et al., 2011; Tlusty et al., 2017). Coordination across institutions to facilitate such efforts are required and can be aided through efforts of Taxon Advisory Groups (TAGs) and the creation and maintenance of regularly curated studbooks. Amongst the five major zoological associations throughout the world, only two, the European Association of Zoos and Aquariums (EAZA) and the American Association of Zoos and Aquariums (AZA), have actively maintained studbooks for species at risk in order to manage and inform breeding efforts (Da Silva et al., 2019). Therefore, increasing the number of advisory groups or studbooks worldwide for threatened and endangered species and expanding to include all chondrichthyans in managed care might enable more coordinated breeding efforts and possibly lead to more sustainable collections. These efforts can be aided by databases such as Zoological Information Management System (ZIMS, 2017), maintained by Species360, and the Captive Elasmobranch Census, conducted by the American Elasmobranch Society (AES, 2008), that may allow users to identify other institutions with conspecifics which may facilitate coordinated breeding efforts. Furthermore, centralized databases that help facilitate interinstitutional connections may also encourage knowledge-sharing about reproductive behaviors and observations across the industry, which will only further benefit our understanding about elasmobranch reproductive biology (Janse et al., 2017; Da Silva et al., 2019).
Future directions
Knowledge of elasmobranch reproductive biology has made great strides over the past few decades, due in part to the contribution of aquaria providing information that would be nearly impossible to glean naturally. However, there are a number of areas where ex situ research can continue to advance knowledge about mating systems and reproduction across a diverse array of elasmobranch species.
Basic knowledge of reproductive parameters remains unknown for a large number of elasmobranchs species. Important life history information such as fecundity, maturity, lifespan, among others, are all important parameters that have implications not only for ex situ management but also for management and conservation of species in situ. Aquaria have a role to play in providing this much needed data by monitoring their diverse elasmobranch populations. For example, the highly collaborative StAR project, which aims to re-wild Zebra Sharks to Indonesia where this species has been functionally extirpated, relied on biological information collected by zoos and aquaria in order to perform a Population Viability Analysis (Traylor-Holzer, 2021) that was crucial to convincing government officials that this project was worth conducting. As aquaria move towards more conservation-based efforts, leveraging basic reproductive information collected ex situ will become important for determining the success of these projects.
Parthenogenesis in the wild has only been documented once in the Smalltooth Sawfish, a critically endangered species (Fields et al., 2015). The low numbers of naturally occurring sawfish may mimic ex situ conditions where parthenogenesis has been observed in other species and where females are kept isolated from males. Aquaria have a unique role to play in understanding the triggers and factors that contribute to the occurrence of parthenogenesis that will contribute to the fundamental understanding of reproductive biology in elasmobranchs that would be impossible to study in situ.
The development of reproductive technologies is another discipline where aquaria can make a significant contribution towards understanding elasmobranch reproductive biology (Daly and Jones, 2017). While AI has been performed with some success, it is almost exclusively limited to oviparous species. Expansion of this technique to viviparous species of elasmobranchs represents a field ripe for exploration. Gamete preservation, short term via cold storage or long term using cryopreservation, is a nascent area of study in the field of elasmobranch reproductive biology that could have significant implications for both ex situ and in situ populations. Cryopreservation of sperm removes the requirement of male and female reproductive synchronization and increases the amount of time viable sperm are available for AI. Limited success (measured by survival of sperm after thawing) has been reported for several ray and shark species (Daly and Jones, 2017; García-Salinas et al., 2021). This contrasts with the majority of sperm surviving cryopreservation for the Bluntnose Sixgill Shark (Hexanchus griseus), a benthic species already adapted to survive cold temperatures, which may account for the success of cryopreservation (García-Salinas et al., 2021). The variability in cryopreservation success between elasmobranch species highlights the need for development of species-specific protocols that account for and leverage the reproductive physiology and ecology of each species. Already, transfer of semen between aquaria alongside short-term storage has proven feasible (Wyffels et al., 2021) and opens the door for longer storage and longer duration transports to take place between aquaria worldwide, including between in situ and ex situ populations. Exchange of gametes between ex situ and in situ populations has the potential to help keep smaller aquarium populations genetically diverse via introduction of new genes.
The diversity and resources of aquaria, in terms of their species collection, knowledge of husbandry, and unique ability to gather important life history data, represent a mostly untapped potential to make substantial contributions to in situ elasmobranch species conservation. For example, zoos have had a significant role in the continued recovery of wild populations of Black-Footed Ferrets (Mustela nigripes; Jachowski and Lockhart, 2009) and Whooping Cranes (Grus americana; Horwich, 2001); however, much work is required to officially “down list” conservation-dependent species that would indicate true recovery of imperiled species. Aquarium contributions to this realm have thus far been limited, despite comparable resources. In the future, aquaria should take a more substantial role in leveraging their elasmobranch populations towards understanding basic elasmobranch biology and applying that knowledge to support in situ conservation. In particular, creating new programs for collaborative breeding and reintroduction of imperiled species is an arena where aquaria can play a leading role. For example, these efforts are being trialed by the StAR project that represents a multi-institutional effort spanning aquaria, academia, government, for-profit, and non-profit institutions to re-wild this species in the Indo-Pacific. If successful, this project could lay the ground work for other critically endangered species such as the Bowmouth Guitarfish (Rhina ancylstoma; Hanna and Hazeres, 2021), and sawfish (Pritis species; Flowers et al., 2020) where breeding has occurred.
Author contributions
KF, JW, and KL conceived the study and contributed to writing and editing equally. All authors approved the submitted version.
Conflict of interest
The authors declare that the research was conducted in the absence of any commercial or financial relationships that could be construed as a potential conflict of interest.
Publisher’s note
All claims expressed in this article are solely those of the authors and do not necessarily represent those of their affiliated organizations, or those of the publisher, the editors and the reviewers. Any product that may be evaluated in this article, or claim that may be made by its manufacturer, is not guaranteed or endorsed by the publisher.
References
Adams L. A., Lyons K., Larkin E., Leier N., Monday J., Plante C., et al. (2022). Artificial insemination and parthenogenesis in the zebra shark Stegostoma tigrinum. Front. Mar. Sci. 9, 886616. doi: 10.3389/fmars.2022.886616
AES (2008). The American elasmobranch society (AES) international captive elasmobranch census (AES CEC) 2008 (Dade County: The American Elasmobranch Society).
Agnew M. K., Asa C. S., Franklin A. D., McDonald M. M., Cowl V. B. (2021). Deslorelin (Suprelorin®) use in north American and European zoos and aquaria: Taxonomic scope, dosing, and efficacy. J. Zoo Wildl. Med. 52, 427–436. doi: 10.1638/2020-0217
Awruch C. A. (2013). Reproductive endocrinology in chondrichthyans: The present and the future. Gen. Comp. Endocrinol. 192, 60–70. doi: 10.1016/j.ygcen.2013.05.021
Awruch C. A., Frusher S. D., Pankhurst N. W., Stevens J. D. (2008). Non-lethal assessment of reproductive characteristics for management and conservation of sharks. Mar. Ecol. Prog. Ser. 355, 277–285. doi: 10.3354/meps07227
Bansemer C. S., Bennett M. B. (2008). Multi-year validation of photographic identification of grey nurse sharks, Carcharias taurus, and applications for non-invasive conservation research. Mar. Freshw. Res. 59, 322–331. doi: 10.1071/MF07184
Bansemer C., Bennett M. (2009). Reproductive periodicity, localised movements and behavioural segregation of pregnant Carcharias taurus at wolf rock, southeast Queensland, Australia. Mar. Ecol. Prog. Ser. 374, 215–227. doi: 10.3354/meps07741
Bernal M. A., Sinai N. L., Rocha C., Gaither M. R., Dunker F., Rocha L. A. (2014). Long-term sperm storage in the brownbanded bamboo shark Chiloscyllium punctatum. J. Fish. Biol. 86, 1171–1176. doi: 10.1111/jfb.12606
Bester-van der Merwe A., Lyons K., Kacev D., Feldheim K. (2022). “Elasmobranch mating systems,” in Biology of sharks and their relatives, 3rd ed. Eds. Carrier J. C., Simpfendorfer C. A., Heithaus M. R., Yopak K. E. (Boca Raton, FL: CRC Press), 203–229.
Buckley K. A., Crook D. A., Pillans R. D., Smith L., Kyne P. M. (2018). Sustainability of threatened species displayed in public aquaria, with a case study of Australian sharks and rays. Rev. Fish. Biol. Fish. 28, 137–151. doi: 10.1007/s11160-017-9501-2
Burke P. J., Raoult V., Natanson L. J., Murphy T. D., Peddemors V., Williamson J. E. (2020). Struggling with age: Common sawsharks (Pristiophorus cirratus) defy age determination using a range of traditional methods. Fish. Res. 231, 105706. doi: 10.1016/j.fishres.2020.105706
Cailliet G. M. (2015). Perspectives on elasmobranch life-history studies: A focus on age validation and relevance to fishery management. J. Fish. Biol. 87, 1271–1292. doi: 10.1111/jfb.12829
Calvin J. L., Slater C. H., Bolduc T. G., Laudano A. P., Sower S. A. (1993). Multiple molecular forms of gonadotropin-releasing hormone in the brain of an elasmobranch: Evidence for IR-lamprey GnRH. Peptides 14, 725–729. doi: 10.1016/0196-9781(93)90104-O
Carlson J. K., Simpfendorfer C. A. (2015). Recovery potential of smalltooth sawfish, Pristis pectinata, in the united states determined using population viability models. Aquat. Conserv.: Mar. Freshwat. Ecosyst. 25, 187–200. doi: 10.1002/aqc.2434
Castro J. I., Bubucis P. M., Overstrom N. A. (1988). The reproductive biology of the chain dogfish, Scyliorhinus retifer. Copeia 1988, 740–746. doi: 10.2307/1445396
Cavalcante D. G., Gallo H., de Sousa O. M., de Azevedo V. G., Sanches E. G. (2016). Aspectos da reprodução e primeiro registro de distocia em raiaticonha (Rhinoptera bonasus). Bol. Inst. Pesca. São. Paulo. 42, 710–718. doi: 10.20950/1678-2305.2016v42n3p710
Chapman D. D., Firchau B., Shivji M. S. (2008). Parthenogenesis in a large-bodied requiem shark, the blacktip Carcharhinus limbatus. J. Fish. Bio. 73, 1473–1477. doi: 10.1111/j.1095-8649.2008.02018.x
Chapman D. D., Shivji M. S., Louis E., Sommer J., Fletcher H., Prodöhl P. A. (2007). Virgin birth in a hammerhead shark. Biol. Lett. 3, 425–427. doi: 10.1098/rsbl.2007.0189
Clark E. (1963). “The maintenance of sharks in captivity, with a report on their instrumental conditioning,” in Sharks and survival. Ed. Gilbert P. W. (Boston, MA: D.C. Health & Co), 115–149.
Conde D. A., Flesness N., Colchero F., Jones O. R., Scheuerlein A. (2011). An emerging role of zoos to conserve biodiversity. Science 331, 1390–1391. doi: 10.1126/science.1200674
Daly J., Jones R. (2017). “Use of reproductive technologies in breeding programs for elasmobranchs in aquaria,” in Elasmobranch husbandry manual II: Recent advances in the care of sharks, rays and their relatives. Eds. Smith M., Warmolts D., Thoney D., Hueter R., Murray M., Ezcurra J. (Columbus, OH: Ohio Biological Survey), 363–374.
Da Silva R., Pearce-Kelly P., Zimmerman B., Knott M., Foden W., Conde D. A. (2019). Assessing the conservation potential of fish and corals in aquariums globally. J. Nat. Conserv. 48, 1–11. doi: 10.1016/j.jnc.2018.12.001
Dove A. D. M., Matsumoto R., Schreiber C., Murakumo K., Coco C., Yanagisawa M., et al. (2021). “Lessons from care of whale sharks in public aquaria,” in Whale sharks: Biology, ecology, and conservation. Eds. Dove A. D. M., Pierce S. J. (Boca Raton, FL: CRC Press), 181–198.
Dudgeon C. L., Coulton L., Bone R., Ovenden J. R., Thomas S. (2017). Switch from sexual to parthenogenetic reproduction in a zebra shark. Sci. Rep. 7, 1–8. doi: 10.1038/srep40537
Dutilloy A., Dunn M. R. (2020). Observations of sperm storage in some deep-sea elasmobranchs. Deep. Sea. Res. Part I. Oceanogr. Res. Pap. 166, 103405. doi: 10.1016/j.dsr.2020.103405
Ebert D. A., Dando M., Fowler S. (2021). Sharks of the world: A complete guide, second edition (Oxford: Princeton University Press).
Feldheim K. A., Chapman D. D., Sweet D., Fitzpatrick S., Prodöhl P. A., Shivji M. S., et al. (2010). Shark virgin birth produces multiple, viable offspring. J. Hered. 101, 374–377. doi: 10.1093/jhered/esp129
Feldheim K. A., Clews A., Henningsen A., Todorov L., McDermott C., Meyers M., et al. (2016). Multiple births by a captive swellshark Cephaloscyllium ventriosum via facultative parthenogenesis. J. Fish. Bio. 90, 1047–1053. doi: 10.1111/jfb.13202
Feldheim K. A., Fields A. T., Chapman D. D., Scharer R., Poulakis G. P. (2017). Insights into reproduction and behavior of the smalltooth sawfish Pristis pectinata. endanger. Species. Res. 34, 463–471. doi: 10.3354/esr00868
Fields A. T., Feldheim K. A., Poulakis G. R., Chapman D. D. (2015). Facultative parthenogenesis in a critically endangered wild vertebrate. Curr. Biol. 25, R446–R447. doi: 10.1016/j.cub.2015.04.018
Flowers K. I., Chapman D. D., Kemp T., Wert D., Feldheim K. A. (2020). Annual breeding in a captive smalltooth sawfish, Pristis pectinata. J. Fish. Bio 97, 1586–1589. doi: 10.1111/jfb.14523
Foote R. H. (2002). The history of artificial insemination: Selected notes and notables. J. Anim. Sci. 80, 1–10. doi: 10.2527/animalsci2002.80E-Suppl_21a
Fox G., Darolti J., Hibbitt J., Preziosi R. F., Fitzpatrick J. L., Rowntree J. K. (2018). Bespoke markers for ex-situ conservation: Application, analysis and challenges in the assessment of a population of endangered undulate rays. J. Zoo Aquar. Res. 6, 50–56. doi: 10.19227/jzar.v6i2.299
García-Salinas P., Gallego V., Asturiano J. F. (2021). Development of sperm cryopreservation protocols for sharks and rays: New tools for elasmobranch conservation. Front. Mar. Sci. 8, 1–13. doi: 10.3389/fmars.2021.689089
Garner M. M. (2013). A retrospective study of disease in elasmobranchs. Vet. Pathol. 50, 377–389. doi: 10.1177/0300985813482147
George R. H., Steeil J., Baine K. (2017). “Diagnosis and treatment of common reproductive problems in elasmobranchs,” in Elasmobranch husbandry manual II: Recent advances in the care of sharks, rays and their relatives. Eds. Smith M., Warmolts D., Thoney D., Hueter R., Murray M., Ezcurra J. (Columbus, OH: Ohio Biological Survey), 357–362.
Gilmore R. G. (1993). Reproductive biology of lamnoid sharks. Environ. Biol. Fishes. 38, 95–114. doi: 10.1007/BF00842907
Gordon I. (1993). Pre-copulatory behaviour of captive sandtiger sharks, Carcharias taurus. Environ. Biol. Fishes. 38, 159–164. doi: 10.1007/BF00842912
Hanna J., Hazeres J. (2021). “Bowmouth guitarfish – an evolution of species management from local to international applications within the aquarium and zoo industry,” in Report on the American elasmobranch society global wedgefish & guitarfish symposium 2021 Sarasota, FL: American Elasmobranch Society.
Harahush B. K., Fischer A. B. P., Collin S. P. (2007). Captive breeding and embryonic development of Chiloscyllium punctatum Muller & henle 1838 (Elasmobranchii: Hemiscyllidae). J. Fish. Biol. 71, 1007–1022. doi: 10.1111/j.1095-8649.2007.01569.x
Harmon T. S., Kamerman T. Y., Corwin A. L., Sellas A. B. (2015). Consecutive parthenogenetic births in a spotted eagle ray Aetobatus narinari. J. Fish. Biol. 88, 741–745. doi: 10.1111/jfb.12819
Heist E. J., Feldheim K. A. (2004). “Elasmobranch genetics and captive management,” in The elasmobranch husbandry manual: Captive care of sharks, rays and their relatives. Eds. Smith M., Warmolts D., Thorney D., Hueter R. (Columbus, OH: Ohio Biological Survey), 249–260.
Henningsen A. D. (2000). Notes on reproduction in the southern stingray, Dasyatis americana (Chondrichthyes: Dasyatidae), in a captive environment. Copeia 2000, 826–828. doi: 10.1643/0045-8511(2000)000[0826:NORITS]2.0.CO;2
Henningsen A. D., Murru F. L., Rasmussen L. E. L., Whitaker B. R., Violetta G. C. (2008). Serum levels of reproductive steroid hormones in captive sand tiger sharks, Carcharias taurus (Rafinesque), and comments on their relation to sexual conflicts. Fish. Physiol. Biochem. 34, 437–446. doi: 10.1007/s10695-008-9202-9
Henningsen A. D., Smale M. J., Gordon I., Garner R., Marin-Osorno R., Kinnunen N. (2004). “Captive breeding and sexual conflict in elasmobranchs,” in The elasmobranch husbandry manual: Captive care of sharks, rays and their relatives. Eds. Smith M., Warmolts D., Thorney D., Hueter R. (Columbus, OH: Ohio Biological Survey), 237–248.
Henningsen A., Street E. P., Claus E., Street E. P., Littlehale D., Choromanski J., et al. (2017). “Reproduction of the sand tiger sharks, Carcharias taurus, in aquaria: A framework for a managed breeding program,” in Elasmobranch husbandry manual II: Recent advances in the care of sharks, rays and their relatives. Eds. Smith M., Warmolts D., Thoney D., Hueter R., Murray M., Ezcurra J. (Columbus, OH: Ohio Biological Survey), 375–390.
Henningsen A. D., Whitaker B. R., Kight K., Hess D. L., Hadfield C., Zohar Y. (2015). The use of a gonadotropin releasing hormone antagonist in captive sand tiger sharks, Carcharias taurus, and the serum levels of the antagonist and reproductive steroid hormones. J. Zoo Aquar. Res. 3, 107–115. doi: 10.19227/jzar.v3i3.120
Hildebrandt T. B., Hermes R., Saragusty J., Potier R., Schwammer H. M., Balfanz F., et al. (2012). Enriching the captive elephant population genetic pool through artificial insemination with frozen-thawed semen collected in the wild. Theriogenology 78, 1398–1404. doi: 10.1016/j.theriogenology.2012.06.014
Holt W. V., Fazeli A. (2016). Sperm storage in the female reproductive tract. Annu. Rev. Anim. Biosci. 4, 291–310. doi: 10.1146/annurev-animal-021815-111350
Holt W. V., Lloyd R. E. (2010). Sperm storage in the vertebrate female reproductive tract: how does it work so well? Theriogenology 73, 713–722. doi: 10.1016/j.theriogenology.2009.07.002
Hook S. A. (2019). The application of genetics and proteomics for the conservation of sharks and their relatives (Manchester (United Kingdom: University of Manchester).
Horwich R. H. (2001). “Developing a migratory whooping crane flock,” in Proceedings of the eighth north American crane workshop. Ed. Ellis D. H. (Seattle, WA: North American Crane Working Group), 85–95.
Howard J. G., Lynch C., Santymire R. M., Marinari P. E., Wildt D. E. (2016). Black-footed ferret gene restoration. Anim. Conserv. 19, 102–111. doi: 10.1111/acv.12229
Jachowski D. S., Lockhart J. M. (2009). Reintroducing the black-footed ferret Mustela nigripes to the great plains of north America. Small. Carniv. Conserv. 41, 58–64.
Janse M., Kappe A. L., Van Kuijk B. L. M. (2013). Paternity testing using the poisonous sting in captive white-spotted eagle rays Aetobatus narinari: A non-invasive tool for captive sustainability programmes. J. Fish. Biol. 82, 1082–1085. doi: 10.1111/jfb.12038
Janse M., Luten H. A. (2019). Contraception within an elasmobranch captive breeding programme: A case report on the eagle ray aetobatus ocellatus using gonadotrophin-releasing hormone (GnRH) agonist deslorelin (Suprelorin). J. Fish. Biol. 94, 196–199. doi: 10.1111/jfb.13872
Janse M., Zimmerman B., Geerlings L., Brown C., Nagelkerke L. A. (2017). Sustainable species management of the elasmobranch populations within European aquariums: A conservation challenge. J. Zoo Aquar. Res. 5, 172–181. doi: 10.19227/jzar.v5i4.313
Jordan R. P., Graham C. T., Minto C., Anderson A. C. (2021). Assessment of sperm storage across different reproductive modes in the elasmobranch fishes. Environ. Biol. Fish. 104, 27–39. doi: 10.1007/s10641-020-01051-x
Jung-Schroers V., Hellmann J., Steinhagen D. (2015). Birth complications and cesarean section in a blacktip reef shark (Carcharhinus melanopterus). Austin. J. Vet. Sci. Anim. Husb. 2, 1017.
Koob T. J. (2004). “Elasmobranchs in the public aquarium: 1860 to 1930,” in The elasmobranch husbandry manual: Captive care of sharks, rays and their relatives. Eds. Smith M., Warmolts D., Thorney D., Hueter R. (Columbus, OH: Ohio Biological Survey), 1–14.
Lampert K. P. (2008). Facultative parthenogenesis in vertebrates: reproductive error or chance? Sex Dev. 2, 290–301. doi: 10.1159/000195678
Leite R. D., Wosnick N., Giareta E. P., Freire C. A. (2020). Evidence of dystocia in a free-ranging cownose ray Rhinoptera bonasus (Elasmobranchii, rhinopteridae). Bol. Lab. Hidrobiol. 30, 1–6. doi: 10.18764/1981-6421e2020.13
Lovejoy D. A., Fischer W. H., Ngamvongchon S., Craig A. G., Nahorniak C. S., Peter R. E., et al. (1992). Distinct sequence of gonadotropin-releasing hormone (GnRH) in dogfish brain provides insight into GnRH evolution. Proc. Natl. Acad. Sci. 89, 6373–6377. doi: 10.1073/pnas.89.14.6373
Luer C. A., Gilbert P. W. (1985). Mating behavior, egg deposition, incubation period, and hatching in the clearnose skate, Raja eglanteria. Environ. Biol. Fishes. 13, 161–171. doi: 10.1007/BF00000926
Luer C. A., Walsh C. I., Bodine A. B., Wyffels J. (2007). Normal embryonic development in the clearnose skate, Raja eglanteria, with experimental observations on artificial insemination. Environ. Biol. Fishes. 80, 239–255. doi: 10.1007/s10641-007-9219-4
Lyons K., Kacev D., Mull C. G. (2021). An inconvenient tooth: Evaluating female choice in multiple paternity using an evolutionarily and ecologically important vertebrate clade. Mol. Ecol. 30, 1574–1593. doi: 10.1111/mec.15844
Mahon J., Chua F., Newman P. (2004). “Successful spotted eagle ray (Aetobatis narinari) breeding program and details of an assisted birth,” in The elasmobranch husbandry symposium. Eds. Mohan P., Smith M. (Columbus, Ohio: Ohio Biological Survey), 104–107.
Masuda M., Izawa Y., Kametuta S., Ikuta H., Isogai T. (2003). Artificial insemination of the cloudy catshark. J. Jpn. Assoc. Zool. Gard. Aquaria. 44, 39–43.
Masuda M., Izawa Y., Kametuta S., Ikuta H., Isogai T. (2005). Artificial insemination of the white-spotted bamboo shark, Chiloscyllium plagiosum. J. Jpn. Assoc. Zool. Gard. Aquaria. 46, 91–96.
Matsumoto R., Matsumoto Y., Ueda K., Suzuki M., Asahina K., Sato K. (2019). Sexual maturation in a male whale shark (Rhincodon typus) based on observations made over 20 years of captivity. Fish. Bull. 117, 78–86. doi: 10.7755/FB.117.1-2.9
Murakumo K., Matsumoto R., Tomita T., Matsumoto Y., Ueda K. (2020). The power of ultrasound: observation of nearly the entire gestation and embryonic developmental process of captive reef manta rays (Mobula alfredi). Fish. Bull. 118, 1–7. doi: 10.7755/FB.118.1.1
Musa S. M., Ripley D. M., Moritz T., Shiels H. A. (2020). Ocean warming and hypoxia affect embryonic growth, fitness and survival of small-spotted catsharks, Scyliorhinus canicula. J. Fish. Biol. 97, 257–264. doi: 10.1111/jfb.14370
Mylniczenko N. D., Culpepper E. E., Clauss T. (2017). “Diagnostic imaging of elasmobranchs: updates and case examples,” in Elasmobranch husbandry manual II: Recent advances in the care of sharks, rays and their relatives. Eds. Smith M., Warmolts D., Thoney D., Hueter R., Murray M., Ezcurra J. (Columbus, OH: Ohio Biological Survey), 303–324.
Mylniczenko N. D., Sumigama S., Wyffels J. T., Wheaton C. J., Guttridge T. L., Dirocco S., et al. (2019). Ultrasonographic and hormonal characterization of reproductive health and disease in wild, semiwild, and aquarium-housed southern stingrays (Hypanus americanus). Am. J. Vet. Res. 80, 931–942. doi: 10.2460/ajvr.80.10.931
Natanson L. J., Gervelis B. J. (2013). The reproductive biology of the common thresher shark in the Western north Atlantic ocean. Trans. Am. Fish. Soc 142, 1546–1562. doi: 10.1080/00028487.2013.811099
Nau M. R., O’Brien J. K., Schmitt T. L., Nollens H. H., Robeck T. R. (2018). Diagnostic assessment of reproductive status in white-spotted bamboo sharks (Chiloscyllium plagiosum). Anim. Reprod. Sci. 197, 48–57. doi: 10.1016/j.anireprosci.2018.08.005
Nielsen J., Hedeholm R. B., Heinemeier J., Bushnell P. G., Christiansen J. S., Olsen J., et al. (2016). Eye lens radiocarbon reveals centuries of longevity in the Greenland shark (Somniosus microcephalus). Science 353, 702–704. doi: 10.1126/science.aaf1703
Nordell S. E. (1994). Observations of the mating behavior and dentition of the round stingray, Urolophus halleri. Environ. Biol. Fishes. 39, 219–229. doi: 10.1007/BF00005124
Nozu R., Murakumo K., Matsumoto R., Matsumoto Y., Yano N., Nakamura M., et al. (2017). High-resolution monitoring from birth to sexual maturity of a male reef manta ray, Mobula alfredi, held in captivity for 7 years: Changes in external morphology, behavior, and steroid hormones levels. BMC Zool. 2, 14. doi: 10.1186/s40850-017-0023-0
Nozu R., Murakumo K., Yano N., Furuyama R., Matsumoto R., Yanagisawa M., et al. (2018). Changes in sex steroid hormone levels reflect the reproductive status of captive female zebra sharks (Stegostoma fasciatum). Gen. Comp. Endocrinol. 265, 174–179. doi: 10.1016/j.ygcen.2018.03.006
Parent S., Pépin S., Genet J. P., Misserey L., Rojas S. (2008). Captive breeding of the barndoor skate (Dipturus laevis) at the Montreal biodome, with comparison notes on two other captive-bred skate species. Zoo Biol. 27, 145–153. doi: 10.1002/zoo.20170
Passerotti M. S., Andrews A. H., Carlson J. K., Wintner S. P., Goldman K. J., Natanson L. J. (2014). Maximum age and missing time in the vertebrae of sand tiger shark (Carcharias taurus): Validated lifespan from bomb radiocarbon dating in the western north Atlantic and southwestern Indian oceans. Mar. Freshwat. Res. 65, 674–687. doi: 10.1071/MF13214
Payne N. L., Snelling E. P., Fitzpatrick R., Seymour J., Courtney R., Barnett A., et al. (2015). A new method for resolving uncertainty of energy requirements in large water breathers: The ‘mega-flume’seagoing swim-tunnel respirometer. Methods Ecol. Evol. 6, 668–677. doi: 10.1111/2041-210X.12358
Pistevos J. C. A., Nagelkerken I., Rossi T., Olmos M., Connell S. D. (2015). Ocean acidification and global warming impair shark hunting behaviour and growth. Sci. Rep. 5, 16293. doi: 10.1038/srep16293
Portnoy D. S., Hollenbeck C. M., Johnston J. S., Casman H. M., Gold J. R. (2014). Parthenogenesis in a whitetip reef shark Triaenodon obesus involves a reduction in ploidy. J. Fish. Biol. 85, 502–508. doi: 10.1111/jfb.12415
Pratt H. L. Jr. (1988). Elasmobranch gonad structure: A description and survey. Copeia 1988, 719–729. doi: 10.2307/1445394
Pratt H. L. Jr. (1993). “The storage of spermatozoa in the oviducal glands of western north Atlantic sharks,” in The reproduction and development of sharks, skates, rays and ratfishes. Eds. Demski L. S., Wourms J. P. (Dordrecht: Springer), 139–149.
Pratt H. L. Jr., Carrier J. C. (2001). A review of elasmobranch reproductive behavior with a case study on the nurse shark, Ginglymostoma cirratum. Environ. Biol. Fishes. 60, 157–188. doi: 10.1023/A:1007656126281
Ripley D. M., De Giorgio S., Gaffney K., Thomas L., Shiels H. A. (2021). Ocean warming impairs the predator avoidance behaviour of elasmobranch embryos. Conserv. Physiol. 9, coab045. doi: 10.1093/conphys/coab045
Robinson D. P., Baverstock W., Al-Jaru A., Hyland K., Khazanehdari K. A. (2011). Annually recurring parthenogenesis in a zebra shark Stegostoma fasciatum. J. Fish. Biol. 79, 1376–1382. doi: 10.1111/j.1095-8649.2011.03110.x
Rodda K. R., Seymour R. S. (2008). Functional morphology of embryonic development in the port Jackson shark Heterodontus portusjacksoni(Meyer). J. Fish. Biol. 72, 961–984. doi: 10.1111/j.1095-8649.2007.01777.x
Sheldon J. D., Allender M. C., George R. H., Bulman F., Abney K. (2018). Reproductive hormone patterns in male and female cownose rays (Rhinoptera bonasus in an aquarium setting and correlation to ultrasonographic staging. J. Zoo Wildl. Med. 49, 638–647. doi: 10.1638/2017-0247.1
Smale M. J., Jones R. T., Correia J. P., Henningsen A. D., Crow G. L., Garner R. (2004). “Research on elasmobranchs in public aquaria,” in The elasmobranch husbandry manual: Captive care of sharks, rays and their relatives. Eds. Smith M., Warmolts D., Thorney D., Hueter R. (Columbus, OH: Ohio Biological Survey), 533–541.
Stoskopf M. K. (2017). “Shark health management,” in Elasmobranch husbandry manual II: Recent advances in the care of sharks, rays and their relatives. Eds. Smith M., Warmolts D., Thoney D., Hueter R., Murray M., Ezcurra J. (Columbus, OH: Ohio Biological Survey), 245–254.
Straube N., Lampert K. P., Geiger M. F., Weiß J. D., Kirchhauser J. X. (2016). First record of second-generation facultative parthenogenesis in a vertebrate species, the whitespotted bambooshark Chiloscyllium plagiosum. J. Fish. Biol. 88, 668–675. doi: 10.1111/jfb.12862
Sulikowski J. A., Driggers W. B., Ingram G. W., Kneebone J., Ferguson D. E., Tsang P. C. (2007). “Profiling plasma steroid hormones: A non-lethal approach for the study of skate reproductive biology and its potential use in conservation management,” in Biology of skates. Eds. Ebert D. A., Sulikowski J. A. (Dordrecht: Springer), 179–186.
Tlusty M. F., Baylina N., Rhyne A. L., Brown C., Smith M. (2017). “Public aquaria,” in Marine ornamental species aquaculture. Eds. Calado R., Olivotto I., Oliver M. P., Holt G. J. (Hoboken: John Wiley & Sons), 611–621.
Tomita T., Murakumo K., Ueda K., Ashida H., Furuyama R. (2019). Locomotion is not a privilege after birth: Ultrasound images of viviparous shark embryos swimming from one uterus to the other. Ethology 125, 122–126. doi: 10.1111/eth.12828
Tomita T., Touma H., Murakumo K., Yanagisawa M., Yano N., Oka S. I., et al. (2018). Captive birth of tiger shark (Galeocerdo cuvier) reveals a shift in respiratory mode during parturition. Copeia 106, 292–296. doi: 10.1643/CI-17-683
Townsend R., Stow A., Asmyhr M., Momigliano P. (2015). Multiple paternity in captive grey nurse sharks (Carcharias taurus): Implications for the captive breeding of this critically endangered species. Pac. Conserv. Biol. 21, 122–125. doi: 10.1071/PC14909
Traylor-Holzer K. (2021). Population viability analysis (PVA) report for population augmentation of zebra sharks (Stegostoma tigrinum) in raja ampat, Indonesia (Apple Valley, MN: IUCN SSC Conservation Planning Specialist Group).
Uchida S., Toda M., Kamei Y. (1990). “Reproduction of elasmobranchs in captivity,” in Elasmobranchs as living resources: Advances in biology, ecology, systematics and status of the fisheries. Eds. Pratt H. L. Jr., Gruber S. H., Taniuchi T. (NOAA Technical Report NMFS 90), 211–237. (Silver Spring, MD: National Marine Fisheries Service)
Watson L., Janse M. (2017). “Reproduction and husbandry of zebra sharks, stegostoma fasciatum, in aquaria,” in Elasmobranch husbandry manual II: Recent advances in the care of sharks, rays and their relatives. Eds. Smith M., Warmolts D., Thoney D., Hueter R., Murray M., Ezcurra J. (Columbus, OH: Ohio Biological Survey), 421–432.
West J. G., Carter S. (1990). Observations on the development and growth of the epaulette shark, Hemiscyllium ocellatum (Bonnaterre) in captivity. J. Aquaric. Aquat. Sci. 5, 111–117.
Wheeler C. R., Rummer J. L., Bailey B., Lockwood J., Vance S., Mandelman J. W. (2021). Future thermal regimes for epaulette sharks (Hemiscyllium ocellatum): Growth and metabolic performance cease to be optimal. Sci. Rep. 11, 454. doi: 10.1038/s41598-020-79953-0
White W. T., Last P. R. (2012). A review of the taxonomy of chondrichthyan fishes: A modern perspective. J. Fish. Biol. 80, 901–917. doi: 10.1111/j.1095-8649.2011.03192.x
Whitney N. M., Pratt H. L. Jr., Carrier J. C. (2004). Group courtship, mating behaviour and siphon sac function in the whitetip reef shark, Triaenodon obesus. Anim. Behav. 68, 1435–1442. doi: 10.1016/j.anbehav.2004.02.018
Wildt D. E. (2000). Genome resource banking for wildlife research, management, and conservation. ILAR. J. 41, 228–234. doi: 10.1093/ilar.41.4.228
Willson K., Smith M. (2017). “Reproduction of the sand tiger shark, Carcharias taurus (Rafinesque 1810), at UnderWater world SEA LIFE mooloolaba from 1992 – 2012,” in Elasmobranch husbandry manual II: Recent advances in the care of sharks, rays and their relatives. Eds. Smith M., Warmolts D., Thoney D., Hueter R., Murray M., Ezcurra J. (Columbus, OH: Ohio Biological Survey), 391–401.
Wosnick N., Adams K. R., Freire C. A. (2018). Ultrasonography as a promising methodology to indicate captured-induced abortion in viviparous elasmobranchs. J. Fish. Biol. 93, 1033–1037. doi: 10.1111/jfb.13746
Wourms J. P. (1977). Reproduction and development in chondrichthyan fishes. Am. Zool. 17, 379–410. doi: 10.1093/icb/17.2.379
Wyffels J. T., Adams L. M., Bulman F., Fustukjian A., Hyatt M. W., Feldheim K. A., et al. (2021). Artificial insemination and parthenogenesis in the whitespotted bamboo shark Chiloscyllium plagiosum. Sci. Rep. 11, 9966. doi: 10.1038/s41598-021-88568-y
Wyffels J., Coco C., Schreiber C., Palmer D., Clauss T., Bulman F., et al. (2020b). Natural environmental conditions and collaborative efforts provide the secret to success for sand tiger shark Carcharias taurus reproduction in aquaria. Zoo Biol. 39, 355–363. doi: 10.1002/zoo.21558
Wyffels J. T., George R., Adams L., Adams C., Clauss T., Newton A., et al. (2020a). Testosterone and semen seasonality for the sand tiger shark Carcharias taurus. Biol. Reprod. 102, 876–887. doi: 10.1093/biolre/ioz221
Wyffels J. T., George R., Christiansen E. F., Clauss T. M., Newton A. L., Hyatt M. W., et al. (2022). Reproductive cycle and periodicity of in situ and aquarium female sand tiger sharks Carcharias taurus from the western north Atlantic. Front. Mar. Sci. 9, 925749. doi: 10.3389/fmars.2022.925749
Wyffels J., Masuda M., Sakai J., Itoh Y. (2006). Characteristics of Scyliorhinus torazame egg case jelly. J. Japanese. Soc Elasmobranch. Stud. 45, 1–7.
Keywords: ex situ, artificial insemination, mating behavior, parthenogenesis, contraception, reproductive pathology
Citation: Feldheim KA, Wyffels JT and Lyons K (2022) The role of aquaria in the advancement of elasmobranch reproductive biology. Front. Mar. Sci. 9:963542. doi: 10.3389/fmars.2022.963542
Received: 07 June 2022; Accepted: 08 July 2022;
Published: 04 August 2022.
Edited by:
Steven T. Kessel, Shedd Aquarium, United StatesReviewed by:
Max Janse, Burgers’ Zoo, NetherlandsMark Smith, Royal Zoological Society of South Australia, Australia
Copyright © 2022 Feldheim, Wyffels and Lyons. This is an open-access article distributed under the terms of the Creative Commons Attribution License (CC BY). The use, distribution or reproduction in other forums is permitted, provided the original author(s) and the copyright owner(s) are credited and that the original publication in this journal is cited, in accordance with accepted academic practice. No use, distribution or reproduction is permitted which does not comply with these terms.
*Correspondence: Kevin A. Feldheim, a2ZlbGRoZWltQGZpZWxkbXVzZXVtLm9yZw==