- 1Coastal Oceanography and Climate Change Research Center, Faculty of Environmental Management, Prince of Songkla University, Songkhla, Thailand
- 2Marine and Coastal Resources Research Center, Lower Gulf of Thailand, Songkhla, Thailand
- 3Department of Aquatic Science, Faculty of Science, Burapha University, Chonburi, Thailand
- 4Department of Marine Science, Faculty of Science, Chulalongkorn University, Bangkok, Thailand
In February and March 2022, a large amount of pumice stone appeared along the shoreline of Thailand. Pumice is a type of extrusive volcanic rock, and since there are no volcanoes in the Gulf of Thailand, an interesting question was where the pumice stones originated from. Another question was whether the pumice could be a vehicle for microplastics (MPs) which could then journey across the ocean until reaching the coast of Thailand. A preliminary study was begun, which randomly collected samples from seven beaches in five provinces along the coast of Thailand. Grayish-green pumice stones are tiny, porous, and lightweight, ranging from 0.3 to 5.0 cm in size. The examination found 5.7–12.6 MP items per pumice stone. Most of the MP particles observed were less than 1 mm in length. From Fourier transform infrared spectroscopy (FTIR) analysis, the MPs were characterized as polystyrene, polypropylene, poly (ethylene terephthalate) (PET), rayon, and nylon. The MP could have entered the holes in pumice stones while floating on the water surface over long periods. From the seasonal flow patterns, it was revealed that pumice from the South China Sea was more likely to have floated with surface currents into the Gulf of Thailand
Introduction
Plastic waste in aquatic ecosystems has become a global issue because of its toxic effects on marine animals and humans. In recent years, the production of plastic has increased rapidly in conjunction with continued socioeconomic development, urbanization, and industrialization. It has been estimated that 370 million tons of plastic particles have been produced around the world (Plastics Europe, 2020) and around 4.8–12.7 million tons are released annually into marine environments (Haward, 2018) through river runoff and atmospheric deposition (Chen et al., 2021; Muanyaneza et al., 2022). Plastics are easily broken and fragmented into smaller-sized pieces under the effect of environmental forces, yet they take a long time to degrade. Microplastics (MPs) are usually defined as plastic particles less than 5 mm in size and can be categorized as primary and secondary according to their origin (Bradney et al., 2019; Park and Park, 2021). The greatest concern regarding MPs in the environment is their association with toxic chemicals and the transfer of those chemicals into marine organisms that ingest the debris. It has been found that MP debris can adsorb heavy metals (Brennecke et al., 2016; Pradit et al., 2021; Goh et al., 2022), as well as organic pollutants such as polycyclic aromatic hydrocarbons, polychlorinated biphenyls (PCBs), and DDTs (Jimeenez-Skrzypek et al., 2021), which could be transferred to higher trophic levels and could potentially affect human health (Carbery et al., 2018). Additionally, the impact of MP ingestion on various marine animals has been studied in different parts of the world (Ahrendt et al., 2020; Barboa et al., 2020; Hue et al., 2021; Pradit et al., 2022a).
Pumice is a type of volcanic rock that is created when magma suddenly depressurizes and cools during volcanic eruptions (Whitham and Sparks, 1986). Pumice is characterized as porous and siliceous and has a sponge-like appearance (Sarkar et al., 2017). The composition of pumice is primarily silicon dioxide (SiO2) and aluminum oxide (Al2O3), with trace amounts of other oxides (Manurung et al., 2022). Pumice has been reported to have low bulk density, ranging from 0.35 to 0.65 g cm−3, high porosity (64%–85% by volume), and large pore size (Ersoy et al., 2010; Cekova et al., 2013). The skeleton structure of pumice allows molecules and particles to enter and remain within the pores. Therefore, it is possible that pumice could be a source and sink of MPs and affect the environment where it is found. Pumice has been found in many parts of the world but is most commonly associated with volcanic areas such as Turkey, Italy, Greece, Japan, and Indonesia (Bolen, 2008; Lowensten et al., 2018). Recently, numerous pumice rocks were found on the beaches along the lower Gulf of Thailand in Pattani, Narathiwat, Songkhla, and Chumphon provinces in February 2022 and in Rayong province (eastern part of Thailand) in March 2022 Pumice from volcanic eruption found on southern beaches off Gulf of Thailand, 2022. Many researchers believe they could have originated from a volcanic eruption in Indonesia or Japan (Pumice from volcanic eruption found on southern beaches off Gulf of Thailand, 2022) and were transported across the sea, partly due to the effects of the monsoon and seasonal ocean currents (Yoshida et al., 2022).
If pumice can capture and transport MPs, it could affect ecological systems wherever it is found. Most of the previous research conducted along the coast of Thailand has studied MPs in seawater, sediment, and marine species (Azad et al., 2018; Pradit et al., 2020a; Pradit et al., 2020b; Goh et al., 2021; Jualaong et al., 2021; Pradit et al., 2022b). Therefore, this study was the first to investigate MPs in pumice along the coast of Thailand. It examined the abundance, distribution, and types of MPs, and the findings can be used as baseline data for the presence of MPs in pumice stones throughout this region.
Materials and methods
Pumice stone samples (Figure 1) were collected in February and March 2022 from seven beaches in five provinces in the Gulf of Thailand (Supplementary Figure S1). These beaches were Narathiwat province, Ao Manoa Beach (NTNW, 6.4313°N 101.8498°E), Pattani province, Panare Beach (PNTC, 6.9506°N 101.2864°E), Songkhla province, Bo-it Beach (SKBI, 7.1147°N 100.6654°E), Songkhla province, Maharat Beach (SKMH, 7.4762°N 100.4454°E), Songkhla province, Pak Trae Beach (SKPT, 7.7784°N 100.3693°E), Chumphon province, Thung Wua Laen Beach (CPTW, 10.5627°N 99.2740°E), and Rayong province, Pak Nam Rayong Beach (RYPN, 12.6551°N 101.2773°E). Samples were manually collected by picking approximately 1 kg of pumice stones from each beach. The samples were put in new, clean, plastic zip lock bags and brought back to the laboratory for analysis.
To avoid MP contamination, all devices were flushed with distilled water before use. A blank control was created using distilled water poured into a Petri dish and left in the laboratory near the stereomicroscope. At the end of the experiment, no MPs were found in the Petri dish. Furthermore, all sample processing was performed in a clean fume chamber. The pumice samples were rinsed three to five times with clean water to remove sand debris and sediments that could be from the beach environment. In total, 110 pumice stone samples were randomly selected, with a gross weight of approximately 550 g (one stone typically weighs 3–5 g while a small pumice stone typically weighs 0.3–0.5 g). It was expected that the pumice pebbles swarming Thai beaches of several provinces were probably caused by an undersea volcanic eruption and made a long-distance journey across the South China Sea before arrival at Thai beaches. The suspended pumice stones were thoroughly mixed with suspended MPs according to the long-distance journey with long marine residence times and therefore physically homogenized all the stones of pumice containing MPs. This means that the collected pumice of each stone represents one replicate. For our study, approximately 20 stones (replicates) from each province were selected, except for Songkhla province, from which 30 stones (replicates) were required. For samples from provinces where the samples were very small (0.3–0.5 g), the researchers weighed multiple pumice stones with a combined weight of 5 g to equal a single larger stone (5 g). The samples were dried in an oven at 50°C for 3–5 h. Each pumice stone was then ground using a mortar and pestle and put in a 500-ml beaker. MPs were extracted from the pumice stones using a density separation method based on NaCl, which is a widely used technique (Wang et al, 2020; Chinfak et al., 2021; Jiwarungrueangkul et al., 2021). The 250-ml saturated NaCl solution (1.2 g/cm3) was filtered through GF/C filter paper before being added to the ground pumice samples. They were then mixed by stirring the content with a stirring rod for 3–5 min, covered with aluminum foil, and left for 24 h. Subsequently, the samples were poured through a 20-µm mesh filter cloth, and the samples that remained on the filter cloth were transferred to a Petri dish before being oven-dried at 50°C for 3–5 h.
Next, the samples remaining in the Petri dish underwent MP identification by visual examination using a stereomicroscope (Olympus SZ61, Olympus Group, Shinjuku, Tokyo, Japan). The Hidalgo-Ruz et al. (2012) rules for identifying MPs were used to assist in identifying most of the MP particles which were encountered during this analysis. These rules consist of Rule 1: No cellular or organic structures visible; Rule 2: Fibers should be equally thick throughout their entire length; and Rule 3: Particles should exhibit homogenous color throughout the item. All the MPs found were recorded for amount, size [based on three class sizes (>1 mm; 500 µm–1 mm; <500 µm)] (modified from Karbalaei et al., 2019), color, and shape (fiber, fragment, or other) (Li et al., 2016; Jiwarungrueangkul et al., 2021). Polymer type identification was performed using FTIR (Spectrum Two with Spotlight 200i, Perkin Elmer Inc., Waltham, MA, USA). The wavelengths used were 4,000–400 cm−1, using an attenuated total reflection mode. The types of polymer analyzed were compared with the library attached to the FTIR. Data analysis of MP abundance, size, color, and shape was performed using MS Excel 2007 (Office Professional Plus 2019, Microsoft Corp., Redmond, WA, USA). The quantity of MPs within an approximately 5-g pumice stone was calculated to the number of items/stone. Statistical analysis to compare the addressed parameters between sampling sites was performed using the R program.
Results
Pumice stones are porous, light, and can float in water. The pumice stones used in the experiment were approximately around 0.3–5.0 cm in length. Pumice stones are mainly grayish-green, with some stones found with shells within them. From the identification of MPs through visual observation by stereomicroscope, it was found that the MPs found in RYPN = 8.9 items/stone, NTNW = 8.2 items/stone, PNTC = 6.8 items/stone, SKBI = 6.5 items/stone, SKMH = 5.9 items/stone, SKPT = 5.7, and CPTW = 12.6 items/stone. The most common type of MPs found was fiber (Figure 2A) (CPTW>NTNW>PNPT>RYPN>SKPT) except at SKBI and SKMH. The most common color found was blue, followed by white, black, and transparent (Figure 2B), with most being less than 1 mm in size (Figure 2C). FTIR was used to identify the type of polymer. The MP content was characterized as polystyrene, polypropylene, PET, rayon, and nylon, with an example spectrum shown in Supplementary Figure S2.
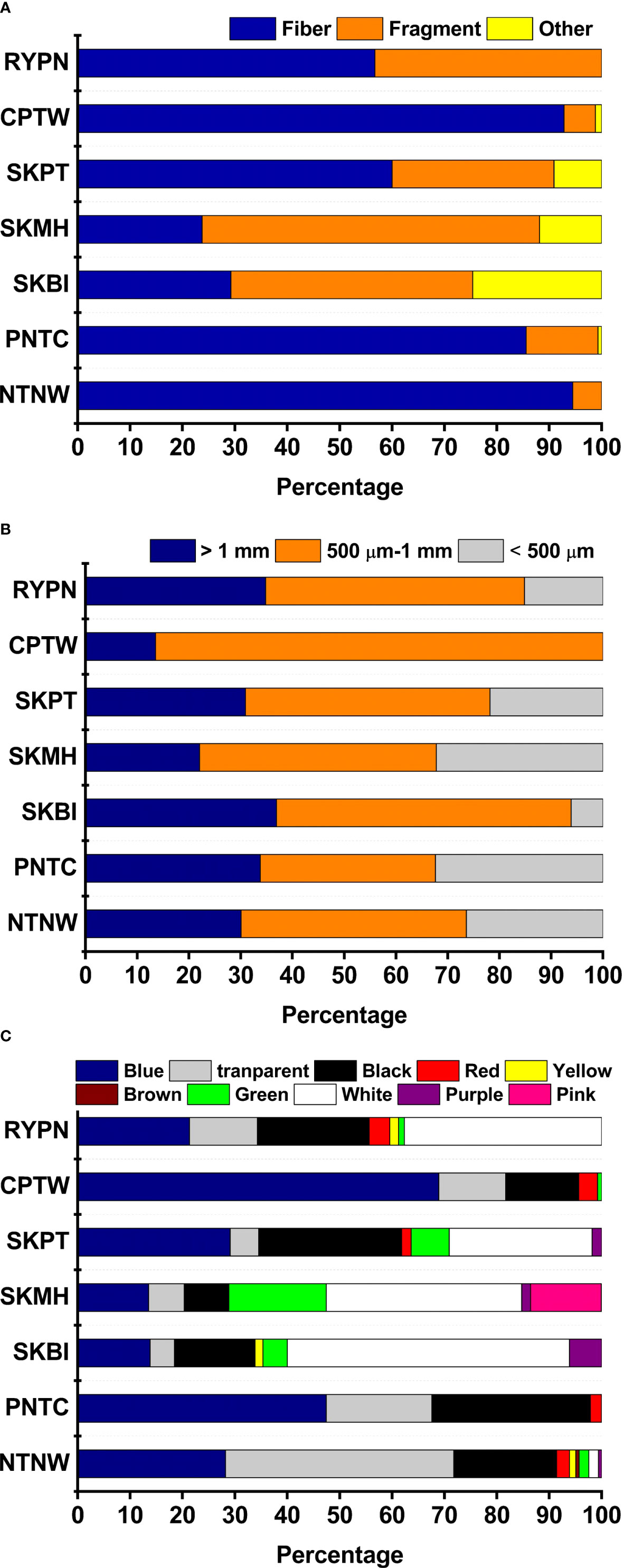
Figure 2 The graphs show (A) MP type found in pumice stone, (B) MP size found in pumice stone, and (C) MP color found in pumice stone.
Discussion
Between February and March 2022, a large quantity of pumice stones was carried by ocean currents and deposited on beaches along the Gulf of Thailand. Pumice is a type of extrusive volcanic rock that is spongy and light and is produced when lava, with a very high content of water and gases, is discharged from volcanoes. When this lava cools and hardens, the result is a very light rock that is filled with tiny bubbles and gas. Based on the results of the analyses, fibers were found to be more predominant in the samples, and this structure type was also very common in previous MP research. Studies suggest that microfibers constitute up to 91% of the entire plastics collected in global seawater samples and are the most ubiquitous type found in ocean surface waters (Barrows et al., 2018). Fibers usually appear to be yarn or threadlike and are either found crumpled or as single threads and are considered degradable plastic waste (Pirc et al., 2016). Commonly, fibers originate from fishing nets, ropes, lines, laundry, and urban waste (Hossain et al., 2020; Severini et al., 2020). Chi-square analysis revealed that the color between the sampling sites was significantly different (p < 0.01), which means that blue was mostly found at CPTW and PNTC. White was largely observed at RYPN, SKBI, SKMH, and SKPT, and black was mostly found at SPKT. The most common color found was blue (mostly fiber shape). It would probably be part of the net or fishing gear used in the region. The pumice stones found in RYPN were smaller than the larger and more porous stones found in the other provinces. From one-way ANOVA, size and shape were significantly different (p < 0.01), whereas the amount of MPs was not significantly different (p > 0.05) among the stations. Most MPs are less dense than seawater and tend to float at the sea surface. Rayon fiber is commonly used to produce artificial silk and other textiles (Pradit et al., 2021). Several broad classes of plastics are used in packaging polyethylene, polypropylene, polystyrene, and PET, while PET and nylons are also used heavily in fishing gear applications (Timmers et al., 2005).
An interesting question is where the pumice stones came from since there are no volcanoes in the Gulf of Thailand. However, February and March mark the end of the southeast monsoon season, which can transport pumice stones from the South China Sea via currents in the Gulf of Thailand. It is therefore highly likely that the pumice stones were carried and driven by ocean currents and wind to the Thai coastline (Figure 3). Ocean currents reach the coastlines of the lower and central Gulf of Thailand (NTNW, PNTC, SKBI, SKMH, SKPT, and CPTW) before changing direction toward the eastern side of the Gulf of Thailand (RYPN). This likely caused pumice stones to be found on the beaches of the southern region earlier in February, and then later (March 2022) in the eastern region of Thailand. By the end of 2021, a massive quantity of pumice stones had been found on the Japanese coasts, which were blown out by the early 2021 eruption of a submarine volcano in the Ogasawara Islands, administratively part of Tokyo and 1,400 km due east of Okinawa. As a consequence, it is possible that the pumice stones found in Thailand originated from the eruption of the undersea volcano in Japan and were then swept by ocean currents in the South China Sea to the Gulf of Thailand.
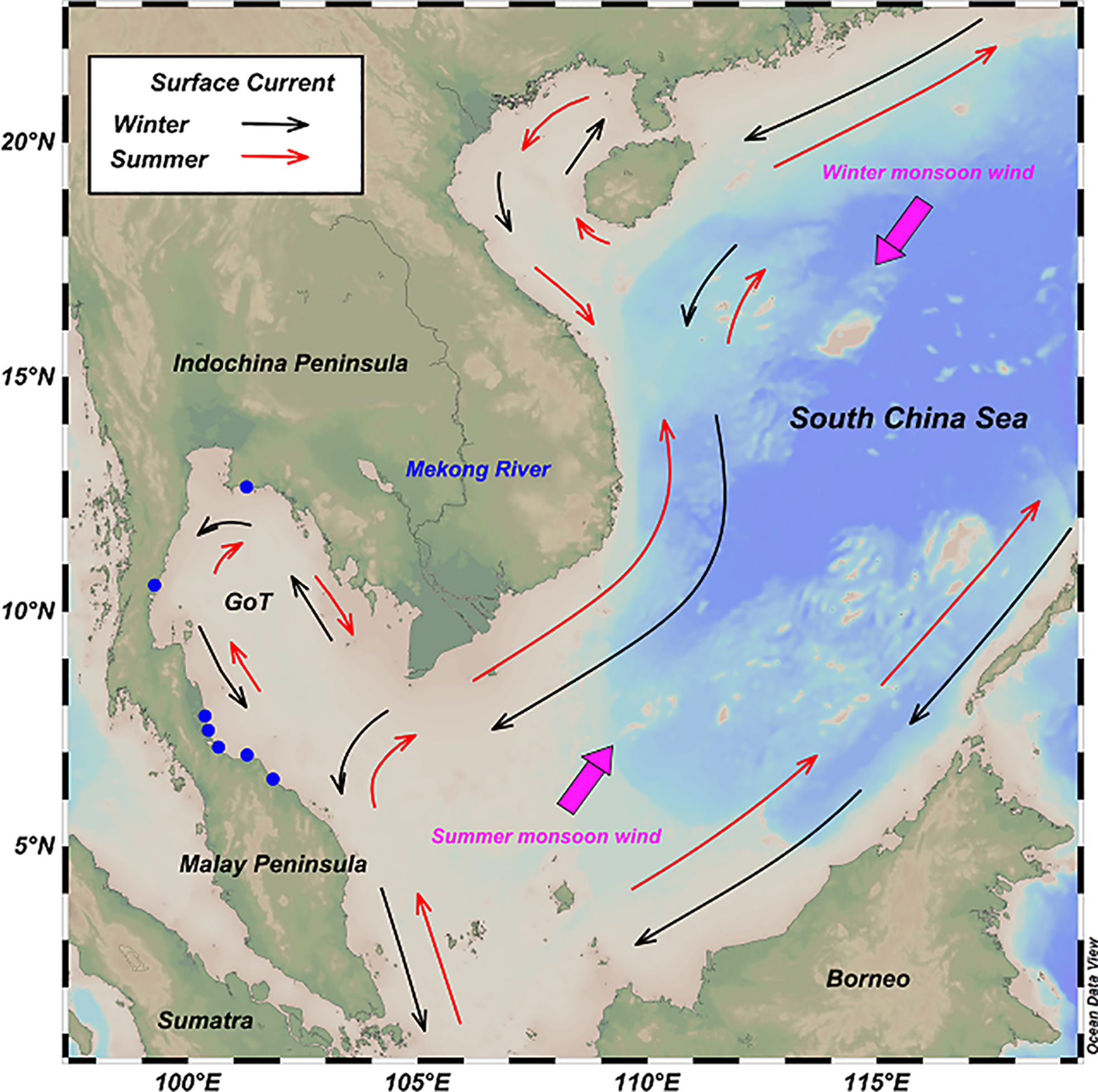
Figure 3 Gulf of Thailand (GoT) surface current (modified from Liu et al., 2016). Surface currents in winter (black) and summer (red) surface currents. The blue solid circle represents a sampling station from the bottom–up, namely NTNW, PNTC, SKBI, SKMH, SKPT, CPTW, and RYPN.
Ocean currents in Southeast Asia change seasonally due to the influence of the northeast and southwest monsoons during different times of the year. Seasonal variations of the influences of the South China Sea on the water in the central Gulf of Thailand have been reported (Yanagi et al., 2001). Subsurface water intrusion from the South China Sea has been found to develop in the summer during the transition from the northeast to the southwest monsoons, in addition to during the southwest monsoon due to surface heating and Ekman transport (Buranapratheprat et al., 2016). Upwelling along the west coast also occurs during the southwest monsoon season. Strong southwest winds induce the flow of water mass from the Gulf of Thailand to the South China Sea, lowering the mean sea level in the Gulf of Thailand (Higuchi et al., 2020). The northeast monsoon season is the period during which the Gulf of Thailand is influenced by the surface current from the South China Sea according to the wind direction. The mean sea level in the gulf then rises, generating overflowing water levels at the head of the inner Gulf of Thailand. The current flows clockwise during the northeast monsoon and counterclockwise during the southwest monsoon in the Gulf of Thailand (Liu et al., 2016). This water circulation weakens during the transition periods between the monsoons. From the seasonal flow patterns, it has been revealed that pumice stones from the South China Sea are more likely to float along with surface currents into the Gulf of Thailand during the northeast monsoon season, than during the southwest monsoon season.
It is also highly possible that the pumice stones were from the eruption of the undersea volcano in Japan, mixed with MPs floating at the surface of the Western Pacific Ocean, and were carried from the South China Sea, a hotspot of floating MPs (Liu et al., 2021). Basin flow, currents, or regional sea-level processes, such as mesoscale eddies and upwelling, may affect the horizontal and vertical transportation and the distribution of MPs. It is well known that the vesicular texture of pumice rock certainly provides a large number of suitable voids for trapping all suspended tiny particles, making it ideal for use as a filter media in the treatment of municipal and industrial effluents. The low specific gravity and high porosity of pumice make it ideal for these applications and treatment processes. Thus, the floating porous pumice stones immersed in the ocean with MPs under the influence of waves, tides, and currents will definitely provide suitable voids for being bombarded and eventually entered by MPs. Therefore, it is highly likely that they will become a place for small animals that require adhesion, such as shipworms or shellfish, to live (Velasquez et al., 2018). According to the experiment, it can be seen that MPs adhere to pumice stones (Figure 4). Surprisingly, a considerable amount of MP debris could be recovered from destroying the pumice structure by grinding with a mortar and pestle. This means that the MP particles were physically trapped inside the vesicular pumice texture and could not be easily washed away from the pumice surface. Once MP debris is trapped inside the pumice structure, it is not easily released into the environment until the pumice structure is weathered. It will have a very long residence time inside the pumice stone since it takes a few hundred years for the pumice structure to break down and release the trapped MPs into the environment.
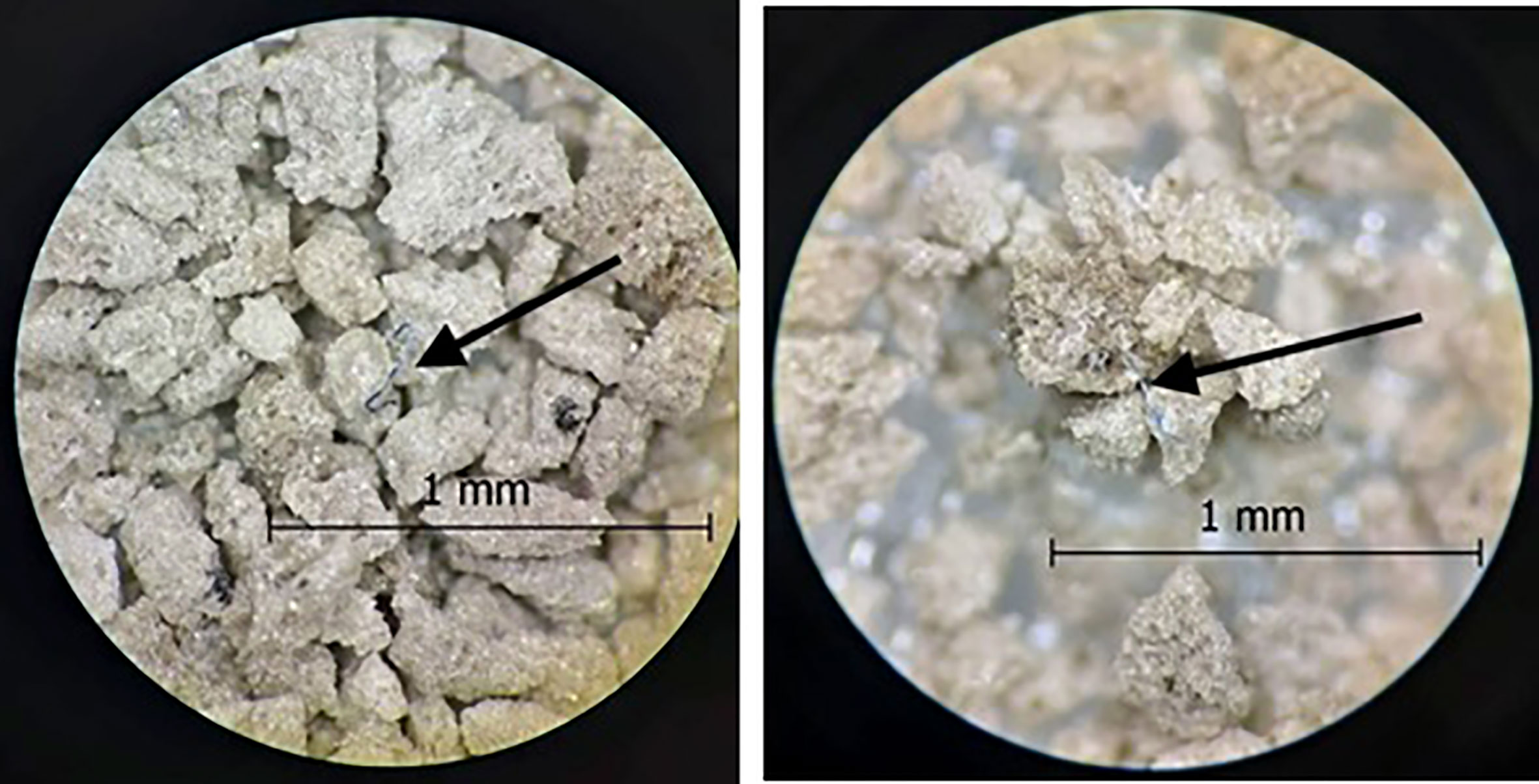
Figure 4 Examples of MPs attached to pumice stone (left, right). Note the light blue fiber in the middle of the circle.
This is the preliminary report on the presence of MPs in pumice stones found along the coast of Thailand. MPs were found to be attached inside the stones, and it could be said that pumice stones act as a floating home for MPs and, in a way, remove it from the ocean. The polymers found were common types seen in surface water and include polystyrene, polypropylene, PET, rayon, and nylon. It was revealed that volcanic rocks from the South China Sea were more likely to float along surface currents into the Gulf of Thailand. Considering that the MPs found in the pumice stones were tiny (<1 mm), it is very likely that MPs entered the pores of the pumice stones. It is possible that pumice could be a distribution source and sink of MPs. Thus, this amazing feature of the pumice structure probably makes the pumice stone an excellent scavenger of the MP debris suspended in seawater. Although the occurrence of pumice around coastal lines normally has adverse impacts on fishing, transport, ports, and tourism, the appearance of pumice stone at sea will probably lessen the amount of MP contaminants in the ocean since the trapped MP debris in pumice stone will have a lower chance of being released and entering the food chain. This accident investigation finding from the natural field experiment obtained from the collected pumice stone drifting in the South China Sea from our study provides valuable information that terrestrial pumice could probably be employed as a filter medium for removing MP particles from drinking water, and after its service life, it can easily be disposed of by using it as a soil conditioner for growing plants, and the trapped MP particles will weather away before the pumice structure breaks down. Thus, no MP debris will be certainly released into the environment from the disposal of pumice filter waste.
Data availability statement
The original contributions presented in the study are included in the article/Supplementary Material. Further inquiries can be directed to the corresponding author.
Author contributions
SP and RP contributed to the conception and design of the study. RP, PN, and SP contributed to sample collection and laboratory work. SP, AB, and PS performed the data analysis. SP wrote the first draft of the manuscript. PS, RP, AB, PN, and SP wrote sections of the manuscript. All authors contributed to manuscript revision, read and approved the submitted version.
Funding
The funding was provided by the Coastal Oceanography and Climate Change Research Center (COCC), Faculty of Environmental Management, Prince of Songkla University.
Acknowledgments
The authors would like to thank Kittiwara Sornplang and Preyanuch Jitkaew for their assistance with the laboratory work and Associate Dr. Prawit Towatana for a valuable suggestion and comment on this research.
Conflict of interest
The authors declare that the research was conducted in the absence of any commercial or financial relationships that could be construed as a potential conflict of interest.
Publisher’s note
All claims expressed in this article are solely those of the authors and do not necessarily represent those of their affiliated organizations, or those of the publisher, the editors and the reviewers. Any product that may be evaluated in this article, or claim that may be made by its manufacturer, is not guaranteed or endorsed by the publisher.
Supplementary material
The Supplementary Material for this article can be found online at: https://www.frontiersin.org/articles/10.3389/fmars.2022.961729/full#supplementary-material
References
Ahrendt C., Perez-Venegas D. J., Urbinad M., Gonzalezf C., Echevestee P., Aldanah M., et al. (2020). Microplastic ingestion cause intestinal lesions in the intertidal fish girella laevifrons. Mar. Pollut. Bull. 151, 110795. doi: 10.1016/j.marpolbul.2019.110795
Azad S. M. O., Towatana P., Pradit S., Patricia B. G., Hue H. T. (2018). Ingestion of microplastics by some commercial fishes in the lower gulf of Thailand: A preliminary approach to ocean conservation. Int. J. Agric. Technol. 14, 1017–1032. Availabe at: http://www.scopus.com/inward/record.url?eid=2-s2.0-85111690969&partnerID=MN8TOARS
Barboa L. G. A., Lopes C., Oliveira P., Bessa F., Otero V., Henriques B., et al. (2020). Microplastics in wild fish from north East Atlantic ocean and its potential for causing neurotoxic effects, lipid oxidative damage, and human health risks associated with ingestion exposure. Sci. Total Environ. 717, 134625. doi: 10.1016/j.scitotenv.2019.134625
Barrows A. P. W., Cathey S. E., Petersen C. W. (2018). Marine environment microfiber contamination: global patterns and the diversity of microparticle origins. Environ. Pollut. 237, 275–284. doi: 10.1016/j.envpol.2018.02.062
Bolen W. P. (2008). Pumice and pumicite, minerals yearbook Vol. vol 130 (Virginia, USA: U.S. Geological Survey).
Bradney L., Wijesekara H., Palansooriya K. N., Obadamudalige N., Bolan N. S., Ok Y. S., et al. (2019). Particulate plastics as a vector for toxic trace-element uptake by aquatic and terrestrial organisms and human health risk. Environ. Int. 131, 104937. doi: 10.1016/j.envint.2019.104937
Brennecke D., Duarte B., Paiva F., Caçador I., Canning-Clode J. (2016). Microplastics as vector for heavy metal contamination from the marine environment. Estuar. Coast. Shelf Sci. 178, 189–195. doi: 10.1016/j.ecss.2015.12.003
Buranapratheprat A., Luadnakrob P., Yanagi T., Morimoto A., Qiao F. (2016). The modification of water column conditions in the Gulf of Thailand by the influences of the south China Sea and monsoonal winds. Cont. Shelf Res. 118, 100–110. doi: 10.1016/j.csr.2016.02.016
Carbery M., O’Connor W., Thavamani P. (2018). Trophic transfer of microplastics and mixed contaminants in the marine food web and implications for human health. Environ. Int. 115, 400–409. doi: 10.1016/j.envint.2018.03.007
Cekova B., Pavlovski B., Spasev D., Reka A. (2013). “Structural examinations of natural raw materials pumice and trepel from republic of Macedonia,” in Proceedings of the XV Balkan mineral processing congress(Sozopol, Bulgaria), 12–16, 73–75.
Chen J., Wang W., Liu H., Xu X., Xia J. (2021). A review on the occurrence, distribution, characteristics, and analysis methods of microplastic pollution in ecosystems. Env. Pollut. Bioavail. 33, 227–246. doi: 10.1080/26395940.2021.1960198
Chinfak N., Sompongchaiyakul P., Charoenpong C., Shi H., Yeemin T., Abundance J. (2021). Composition, and fate of microplastics in water, sediment, and shellfish in the tapi-phumduang river system and bandon bay, Thailand. Sci. Total Environ. 781, 146700. doi: 10.1016/j.scitotenv.2021.146700
Ersoy B., Sariisik A., Dikmen S., Sariisik G. (2010). Characterization of acidic pumice and determination of its electrokinetic properties in water. Powder Technol. 197, 129–135. doi: 10.1016/j.powtec.2009.09.005
Goh P. B., Pradit S., Towatana P., Khokkiatiwong B., Chuan O. M. (2022). Laboratory experiment on copper and lead adsorption ability of microplastics. Sains Malaysiana 51 (4), 993–1004. doi: 10.17576/jsm-2022-5104-04
Goh P. B., Pradit S., Towatana P., Khokkiatiwong S., Kongket B., Moh J. H. Z. (2021). Microplastic abundance in blood cockles and shrimps from fishery market, songkhla province, southern Thailand. Sains Malaysiana 50 (10), 2899–2911. doi: 10.17576/jsm-2021-5010-05
Haward M. (2018). Plastic pollution of the world’s seas and oceans as a contemporary challenge in ocean governance. Nat. Commun. 9, 667–669. doi: 10.1038/s41467-018-03104-3
Hidalgo-Ruz V., Gutow L., Thompson R. C., Thiel M. (2012). Microplastics in the marine environment: A review of the methods used for identification and quantification. Environ. Sci. Technol. 46, 3060–3075. doi: 10.1021/es2031505
Higuchi M., Anongponyoskun M., Phaksopa J., Onishi H. (2020). Influence of monsoon-forced ekman transport on sea surface height in the gulf of Thailand. Agric. Nat. Resour. 54, 205–210. doi: 10.34044/j.anres.2020.54.2.12
Hossain M. S., Rahman M. S., Uddin M. N. (2020). Microplastic contamination in penaeid shrimp from the northern bay of Bengal. Chemosphere. 238, 124688–124697. doi: 10.1016/j.chemosphere.2019.124688
Hue H. T. T., Dong L. K., Hien T. T., Nguyen T. N., Pradit S. (2021). Assessment of microplastics contamination in commercial clams in Vietnam’s coast zone. Appl. Ecol. Envrionmental. 18 (4), 5461–5474. doi: 10.15666/aeer/1804_54615474
Jimeenez-Skrzypek G., Hernandez-Sanchez C., Ortega-Zamora C., Gonzalez-Salamo J., Gonzalez-Curbelo M. A., Hernandez-Borges J. (2021). Microplastic-adsorbed organic contaminants: Analytical methods and occurrence. Trends Anal. Chem. 136, 116186. doi: 10.1016/j.trac.2021.116186
Jiwarungrueangkul T., Phaksopa J., Sompongchaiyakul P., Tipmanee D. (2021). Seasonal microplastic variations in estuarine sediments from urban canal on the West coast of Thailand: A case study in phuket province. Mar. Pollut. Bull. 168, 112452. doi: 10.1016/j.marpolbul.2021.112452
Jualaong S., Pransilpa M., Pradit S., Towatana P. (2021). Type and distribution of microplastics in beach sediment along the coast of the Eastern gulf of Thailand. J. Mar. Sci. Eng. 9, 1405. doi: 10.3390/jmse9121405
Karbalaei S., Golieskardi A., Hamzah H. B., Abdulwahid S., Hanachi P., Walker T. R., et al. (2019). Abundance and characteristics of microplastics in commercial marine fish from Malaysia. Mar. Pollut. Bull. 148, 5–15. doi: 10.1016/j.marpolbul.2019.07.072
Liu Z., Zhao Y., Colin C., Stattegger K., Wiesner M. G., Huh C.-A., et al. (2016). Source-to-sink transport processes of fluvial sediments in the South China Sea. Earth-Sci. Rev. 153, 238–273. doi: 10.1016/j.earscirev.2015.08.005
Li J., Qu X., Su L., Zhang W., Yang D., Kolandhasamy P., et al. (2016). Microplastics in mussels along the coastal waters of China. – Environ. Pollut. 214, 177–184. doi: 10.1016/j.envpol.2016.04.012
Liu M., Ding Y., Huang P., Zheng H., Wang W., Ke H., et al. (2021). Microplastics in the western pacific and south China Sea: Spatial variations reveal the impact of kuroshio intrusion. Environ. Pollut. 288, 117745. doi: 10.1016/j.envpol.2021.117745
Lowensten J. B., Hinsberg V. V., Berlo K., Liesegang M., Lacovino K., Bindeman L. N., et al. (2018). Opal-a in glassy pumice, acid alteration, and the 1817 phreatomagnetic eruption at kawah ljen (Java), Indonesia. Front. Earth Sci. 6. doi: 10.3389/feart.2018.00011
Manurung P., Ginting E. S., Ginting E., Suprihatin (2022). Synthesis and characterisation of nano-silica based on pumice using NaOH. J. Phys. Sci. 33, 17–28. doi: 10.21315/jps2022.33.1.2
Muanyaneza J., Jia Q., Qaraah F. A., Hossain M. F., Wu C., Zhen H., et al. (2022). Review of atmospheric microplastics pollution: In-depth sighting of sources, analytical methods, physiognomies, transport and risks. Sci. Total Environ. 822, 153339. doi: 10.1016/j.scitotenv.2022.153339
Park H., Park B. (2021). Review of microplastic distribution, toxicity, analysis methods, and removal technologies. Water. 13, 2736. doi: 10.3390/w13192736
Pirc U., Vidmar M., Mozer A., Krzan A. (2016). Emissions of microplastic fibers from microfiber fleece during domestic washing. Environ. Sci. Pollut. Res. 23 (21), 22206–222111. doi: 10.1007/s11356-016-7703-0
Plastics Europe (2020) Plastics–the facts. plastic Europe. Available at: https://plasticseurope.org/knowledge-hub/ (Accessed 5 November 2021).
Pradit S., Nitiratsuwan T., Towatana P., Jualaong S., Jirajarus M., Sonrnplang K., et al. (2020a). Marine debris accumulation on the beach in libong, a small island in Andaman Sea, Thailand. Appl. Ecol. Environmental. 18 (4), 5461–5474. doi: 10.15666/aeer/1804_54615474
Pradit S., Noppradit P., Goh B. P., Sornplang K., Ong M. C., Towatana P. (2021). Occurrence of microplastics and trace metals in fish and shrimp from songkhla lake, Thailand during the covid-19 pandemic. Appl. Ecol. Environ. Res. 19, 1085–1106. doi: 10.15666/aeer/1902_10851106
Pradit S., Noppradit P., Loh P.-S., Nitiratsuwan T., Quynh T. P., Qeurng C. O., et al. (2022b). The occurrence of microplastics in sediment cores from two mangrove areas in southern Thailand. J. Mar. Sci. Eng. 10, 418. doi: 10.3390/jmse10030418
Pradit S., Noppradit P., Sengloyluan K., Nitiratsuwan T., Chuan O. M., Towatana P. (2022a). Low occurrence of microplastic contamination in anchovies, a transboundary species, in Thai waters. Scienceasia 48 (4), 456–466. doi: 10.2306/scienceasia1513-1874.2022.069
Pradit S., Towatana P., Nitiratsuwan T., Jualaong S., Jirajarus M., Sonrnplang K., et al. (2020b). Occurrence of microplastics on beach sediment at libong, a pristine island in Andaman Sea, Thailand. ScienceAia 46 (3), 336–343. doi: 10.2306/scienceasia1513-1874.2020.042
Pumice from volcanic eruption found on southern beaches off Gulf of Thailand (2022). Available at: https://thethaiger.com/news/south/songkhla/pumice-from-volcanic-eruption-found-on-southern-beaches-off-gulf-of-thailand (Accessed 10 February 2022).
Sarkar B., Basak B. B., Sarkar S., Mandal S., Bhaduri D. (2017). “Use of soil amendments in an integrated framework for adaptive resource management in agriculture and forestry,” in Adaptive soil management: From theory to practices (Springer, Singapore), 143–159. doi: 10.1007/978-981-10-3638-5
Severini M. D. F., Buzzi N. S., López A. D. F., Colombo C. V., Sartor G. L. C., Rimondino G. N., et al. (2020). Chemical composition and abundance of microplastics in the muscle of commercial shrimp pleoticus muelleri at an impacted coastal environment (Southwestern Atlantic). Mar. Pollut. Bull. 161, 111700. doi: 10.1016/j.marpolbul.2020.111700
Timmers M., Kistner C., Donohue M. (2005). Marine debris of the northwestern Hawaiian islands (Honolulu: Ghost Net Identification). doi: 10.13140/RG.2.1.1057.5207
Velasquez E., Bryan S. E., Ekins M., Cook A. G., Hurrey L., Firn J. (2018). Age and area predict patterns of species richness in pumice rafts contingent on oceanic climatic zone encountered. Ecol. Evol. 8 (10), 5034–5046. doi: 10.1002/ece3.3980
Wang Y., Zou X., Peng C., Qiao S., Wang T., Yu W., et al. (2020). Occurrence and distribution of microplastics in surface sediments from the Gulf of Thailand. Mar. Pollut. Bull. 152, 110916. doi: 10.1016/j.marpolbul.2020.110916
Yanagi T., Sachoemar S. I., Takao T., Fujiwara S. (2001). Seasonal variation of stratification in the gulf of Thailand. J. Oceanogr. 57, 461–470. doi: 10.1023/A:1021237721368
Keywords: microplastics, marine debris, volcano, monsoon season, South China Sea
Citation: Pradit S, Puttapreecha R, Noppradit P, Buranapratheprat A and Sompongchaiyakul P (2022) The first evidence of microplastic presence in pumice stone along the coast of Thailand: A preliminary study. Front. Mar. Sci. 9:961729. doi: 10.3389/fmars.2022.961729
Received: 05 June 2022; Accepted: 21 July 2022;
Published: 15 August 2022.
Edited by:
Tanveer Adyel, Deakin University, AustraliaReviewed by:
Anish Kumar Warrier, Manipal Academy of Higher Education, IndiaWajid Umar, Hungarian University of Agricultural and Life Sciences, Hungary
Copyright © 2022 Pradit, Puttapreecha, Noppradit, Buranapratheprat and Sompongchaiyakul. This is an open-access article distributed under the terms of the Creative Commons Attribution License (CC BY). The use, distribution or reproduction in other forums is permitted, provided the original author(s) and the copyright owner(s) are credited and that the original publication in this journal is cited, in accordance with accepted academic practice. No use, distribution or reproduction is permitted which does not comply with these terms.
*Correspondence: Siriporn Pradit, siriporn.pra@psu.ac.th