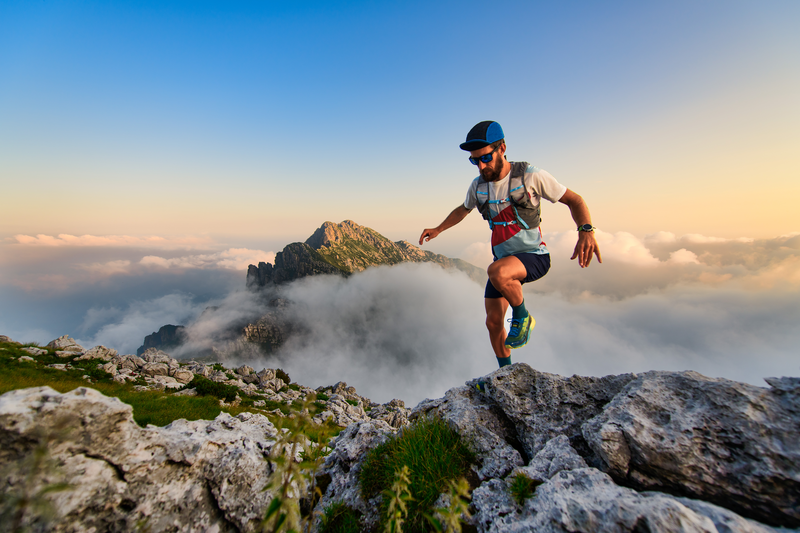
94% of researchers rate our articles as excellent or good
Learn more about the work of our research integrity team to safeguard the quality of each article we publish.
Find out more
ORIGINAL RESEARCH article
Front. Mar. Sci. , 28 July 2022
Sec. Aquatic Physiology
Volume 9 - 2022 | https://doi.org/10.3389/fmars.2022.960746
This article is part of the Research Topic Nutritional Physiology of Aquacultured Species View all 12 articles
The study of fish digestive biochemistry is essential to understand factors that affect the net efficiency of food transformation and growth, and therefore aquaculture profitability. The aim of the present study was to assess the activity and functional characteristics of key digestive enzymes in juveniles of greater amberjack (Seriola dumerili), as well as the possible modulation of their relative importance by water temperature. For that, a combination of biochemical assays and substrate-SDS-PAGE were used. Under physiological conditions pepsin activity was negligible. Chymotrypsin was the most active enzyme in the digestive tract of the greater amberjack, while lipase was the enzyme with lower activity, though both enzymes in addition to trypsin were responsive to water temperature as revealed by discriminant analysis. Seriola dumerili showed to have pH-sensitive and, except for chymotrypsin, thermally robust proteases. Inhibition assays showed the major importance of serine proteases and revealed inverse trypsin and chymotrypsin responses to environmental temperature, with higher trypsin contribution in 26°C-fish while higher chymotrypsin contribution in 18°C-fish. Zymograms revealed three isotrypsin and three isochymotrypsin enzymes, with no variation in the presence of particular isoforms among rearing temperatures. However, they confirmed the role of chymotrypsin activity in providing digestive plasticity, with one of the isoforms being more active at lower temperatures. Thus, results indicate that variation in the relative contribution of chymotrypsin isoenzymes to a particular environmental temperature occurs due to different physic-chemical features of isoforms as a source of functional flexibility. This study assessed for the first time the effects of rearing temperature on greater amberjack digestive enzymes, increasing the knowledge on its digestive biochemistry, and aiding in the improvement of management practices for this species industrialization.
Temperature is likely the major physical environmental factor affecting the fish physiology through its influence on overall metabolism and energy balance, including significant effects on the first steps of energy acquisition such as ingestion rate, activity of digestive enzymes, digestibility, absorption and evacuation rate (Volkoff and Rønnestad, 2020). Therefore, temperature is one of the main factors driving evolutionary adaptations (Nitzan et al., 2019). The tissue-specific responses to stressful temperatures have been addressed in different fish species such as zebrafish (Danio rerio) and tilapia (Oreochromis niloticus) (Hu et al., 2016). Within species, differences in tolerance to low temperature have been also revealed, involving metabolic pathways such as glycolysis and gluconeogenesis in the gills, and biosynthesis of aminoacids in the liver (Nitzan et al., 2019).
Within the temperature tolerance range, many studies have been also performed on the effects of temperature on growth, physiological responses, and health of farmed fish, including the effects of seasonal thermal changes (Pelusio et al., 2021). However, for relatively novel species for the aquaculture industry this information is rather limited. That is the case of the greater amberjack, Seriola dumerili, a pelagic teleost with great interest for the diversification of marine fish aquaculture due to its high grow rates and flesh quality (Sicuro and Luzzana, 2016; Navarro-Guillén et al., 2019; Monge-Ortiz et al., 2020). There is scarce information related to temperature effects on the physiology of S. dumerili. The optimal rearing temperature for the species has been determined to be 26°C, with a valid range down to 22°C without affecting growth or feed utilization. The minimum temperature was set at 17°C, in which deleterious effects on growth and shape were observed (Fernández-Montero et al., 2018; Fernández-Montero et al., 2020). Nevertheless, fry and juveniles seeded in cages for on-growing often face seawater temperatures which, in the case of the Mediterranean Sea, range between 15-28°C, approximately. This may have seasonal effects on feeding and growth efficiencies, with relevant economic and environmental impacts.
The study of fish digestive biochemistry is essential to understand one of the key factors affecting the net efficiency of food utilization and growth, and therefore aquaculture profitability. In this context, the types and functional features of the digestive enzymes involved in the hydrolysis of the main nutrients are basic information to understand digestive capacities of species of interest. To the best of our knowledge, digestive enzymes in Seriola dumerili have been not characterized, with the exception of the study published by Oliveira et al. (2017) analyzing trypsin and chymotrypsin activities in digestive viscera for industrial purposes. In related species, information is only available for trypsin in the Japanese amberjack (Seriola quinqueradiata) (Kishimura et al., 2006). The relationship between digestive enzymes and temperature in Seriola spp. have been also poorly studied, despite this allow a better understanding of its phenotypic/digestive flexibility in response to an environmental factor of ecological and aquaculture relevance. In the Japanese amberjack and yellowtail kingfish (Seriola lalandi), intestinal enzymes activity levels were higher at lower temperatures, possibly in response to the increased gut transit time at reduced water temperatures (Kofuji et al., 2005; Miegel et al., 2010).
Moreover, while most studies on digestive biochemistry of fish have reported several isoenzymes for different enzymes, only a handful of studies have investigated the adaptive value of this isoenzyme richness. The occurrence of multiple isoforms for digestive proteases is thought to provide an adaptive advantage for insects feeding on plants containing inhibitors (Díaz-Mendoza et al., 2005), for crustaceans feeding on a broad diet (Saborowski et al., 2012; Perera et al., 2015), and as an adaptation to environmental temperature in only few fish species (Gelman et al., 2008; Sandholt et al., 2019). In the Atlantic salmon (Salmo salar), trypsin isoenzymes have been thoroughly studied (Torrissen, 1987; Rungruangsak-Torrissen, 2012) and related to differences in the absorption and transport of free amino acids (Torrissen et al., 1994; Rungruangsak-Torrissen and Male, 2000). However, digestive and growth efficiencies are not always related to trypsin-specific activity or isoenzyme composition, but to the activity ratio of trypsin to chymotrypsin (Rungruangsak-Torrissen, 2012).
Therefore, the specific aims of the present study were to assess i) the activity and functional characteristics of key digestive enzymes in greater amberjack juveniles (i.e., pepsin, trypsin, chymotrypsin, leucine aminopeptidase and lipase), ii) their possible modulation by water temperature, and iii) the adaptive value of protease isoenzymes with respect to environmental temperature. The overall objective is to aid in the improvement of management practices for this species industrialization.
Greater amberjack juveniles were supplied by Futuna Blue España S.L. (Puerto de Santa María, Cádiz, Spain) and transferred to the Institute of Marine Sciences of Andalusia (Puerto Real, Cádiz, Spain) facilities. Juveniles were randomly distributed in three 1 m3 cylindroconical tanks (8 fish tank-1), each tank belonging to an independent recirculating aquaculture system (RAS), and acclimated to final experimental temperatures during one week. During the period of acclimatation water temperature in one tank decreased at a rate of 0.5°C per day up to 18°C, in other tank it increased at a rate of 0.5°C per day up to 26°C, while in the remaining tank was kept constant at 22°C. Acclimated juveniles with 60.9 ± 19.6 g of mean wet body weight were reared under a light/dark cycle (12 h light: 12 h dark) at the three temperatures. Water salinity was 34.1 ± 1.2 g L-1, oxygen level was above 90%, pH was8.0 ± 0.1 and NH4< 0.25 mg L-1. The fish were fed a commercial diet (Skretting, Burgos, Spain) to satiety by hand five times a day (09:00, 10:30, 12:30, 14:00 and 16:00h, local time). After 2 weeks under these conditions, fish were sampled at 16:00h to ensure all fish were in the same feeding status (chyme present in all digestive tract sections), as previous results revealed a slowing down of intestinal transit time at low water temperatures. During sampling, fish were euthanized with overdose of 2-phenoxyethanol (Sigma-Aldrich, Merck, Germany) and the whole digestive tract was removed and separated in sections: stomach, pyloric caeca, and anterior, middle and posterior intestine (Figure 1). Dissected digestive tracts were stored at -20°C until analysis.
Figure 1 Digestive tract of a Seriola dumerili juvenile indicating the dissected regions for digestive enzymes analysis.
All experimental procedures complied with the Guidelines of the European Union Council (2010/63/EU) for the use and experimentation of laboratory animals and were reviewed and approved by the Spanish National Research Council (CSIC) bioethical committee for project THERMODIGEST (RTI2018-096134-B-I00).
Samples were mechanically homogenized in distilled water (1:5 v:w) using an Ultra-Turrax® Homogenizer (IKA®-Werke, Germany) and centrifuged for 20 min at 10000 g at 4°C (Eppendorf 5417R, Germany). The supernatants from stomach and pyloric caeca samples were used as source of enzymes for pepsin and alkaline enzymes characterization, respectively, for which enzymatic assays were performed at 37°C. However, to evaluate the distribution of digestive enzyme activity through the digestive tract and the effect of temperature on activity levels, the supernatants from all digestive sections were used and the enzymatic reactions were performed at the temperature the fish providing each sample was adapted to (18, 22 or 26°C).
Pepsin activity in stomach extracts was determined by the method of Anson (1938): 15 µL of extract was mixed to 0.8 mL of 0.5% acid denatured bovine hemoglobin diluted in 0.2 M HCl-glycine buffer pH 3. After incubation for 30 min, the enzymatic reaction was stopped by adding 0.3 mL of 20% trichloroacetic acid (TCA), cooled at 4°C for 15 min and then centrifuged at 8000 g for 15 min. The absorbance of resulting supernatant was measured at 280 nm. Blanks were constructed by adding the enzyme extracts just after TCA to the reaction mixture. A standard curve was prepared with increasing concentrations of tyrosine to determine the molar extinction coefficient of tyrosine at 280 nm. One unit of activity was defined as the amount of enzyme required to produce 1 µg of tyrosine per minute.
Trypsin and chymotrypsin assays were performed as described before (Perera et al., 2008). Trypsin activity was assayed using 1.25 mM N-benzoyl-DL-argininep-nitroanilide (BApNA) in 200 mM Tris–HCl, 20 mM CaCl2, 0.3M NaCl, pH 7.5 (referred hence-forth as the assay buffer). Chymotrypsin activity was measured with 0.1 mM Suc-Ala-Ala-Pro-Phe-p-nitroanilide (SApNA) in the same buffer. Substrate stock solutions of BApNA and SApNA were prepared in DMSO and diluted up to working concentration with buffer prior the assays. Lipase and leucine aminopeptidase were measured as before (Perera and Yúfera, 2017). Lipase activity was measured using 1 mM 4-nitrophenyl palmitate (4NPP) in assay buffer containing 0.3% Triton X-100. Substrate stock solution was prepared in 1:4 v:v acetonitrile/isopropanol. Leucine aminopeptidase activity was assessed by the hydrolysis of 0.5 mM L-Leucine-p-nitroanilide (LeupNA) in assay buffer. Substrate stock solution was prepared in methanol. For all these alkaline enzymes, enzymatic reactions started in 96-well microplates by the addition of 10 µL of the enzyme extract to 200 µL of the respective substrate in buffer, and then, the liberation of p-nitroaniline and p-nitrophenol were kinetically followed at 405 nm in a microplate reader (ELx808 BioTek, BioTek Instrument, Inc.).
The effect of NaCl and CaCl2 on the activity of the digestive enzymes was examined by using different concentrations of NaCl (0, 0.1, 0.5, 1 and 1.5 M) and CaCl2 (0, 20, 50, 100 and 200mM) in the assay buffer. The optimum concentrations of NaCl or CaCl2 were always used in subsequent assays.
The effect of pH on enzyme activities was evaluated using the following buffer solutions: 50 mM glycine-HCl (pH 2-4), 50 mM sodium cacodylate (pH 5-6), 50 mM Tris-HCl (pH 7-8) and 50 mM glycine-NaOH (pH 9-10). Excluding the assay buffer, enzyme activities were measured as described above. Optimal temperature for each enzyme was determined by evaluating the activity of the enzymes at the optimum pH over the range 10°C to 80°C. The effects of pH and temperature on the stability of the digestive enzymes were assessed by pre-incubating the enzyme extracts at different pH and temperature for 15, 30 and 60 min prior to the enzyme assays at optimum pH and 37°C. The stability results were expressed as residual activity (% of the maximum activity).
Classes of alkaline proteases in the digestive tract of fish, using the pyloric caeca as the source of enzymes, were characterized by assessing the effect of protease inhibitors on caseinolytic activity (see section 3.5. Effect of protease inhibitors on caseinolytic activity). Inhibitors employed were soybean trypsin inhibitor (SBTI) for serine-proteases, EDTA for metalloproteases, Nα-p-tosyl-L-lysine chloromethyl ketone (TLCK) for trypsin, and N-tosyl-L-phenylalanine chloromethyl ketone (TPCK) for chymotrypsin. In addition, the specific inhibitors TLCK and TPCK were used to inhibit trypsin and chymotrypsin activities on BApNA and SApNA, respectively. For the assays, enzymatic extracts were incubated with the inhibitors for 60 min at 37°C and then assayed using the appropriate substrate. A preliminary trial revealed no increase on inhibitory capacity at concentrations higher than those used in this work. Enzyme extracts incubated with buffer instead of inhibitors were used as controls and referred to as 100% of enzyme activity.
Substrate-SDS-PAGE (5% stacking gel, 13% separating gel) was used to determine the composition of proteases in the digestive tract (Perera et al., 2008). Gels were run at 30 mA constant current and 4°C in a vertical electrophoresis device (Mini-PROTEAN 3, Bio-Rad, USA). The gel was then immersed in a 3% casein solution for 30 min at 4°C to allow the diffusion of casein into the gel and incubated at 37°C for 45 min to allow the proteases to digest gel-embedded casein. The gel was washed with tap water and stained with 0.1% Coomassie Brilliant Blue in 45% methanol with 10% acetic acid and finally distained in 10% methanol with 10% acetic acid solution. The gel stains blue because of the presence of casein except in areas with proteolytic activity. Unreduced molecular weight markers (14.4 - 116kDa, Thermo Scientific, USA) were used to determine apparent molecular weights. For protease classification, the same substrate-SDS-PAGE procedure was used, but enzyme extracts were incubated with inhibitors for 60 min at 37°C before electrophoresis. Inhibitors used were TLCK, TPCK, PMSF, SBTI and EDTA. The absence of bands in the presence of specific inhibitors indicates a specific type of protease. Given the limited efficiency of some inhibitors to completely abrogate the activity of fish enzymes, reduced intensity of band was also taken as indicative of type of protease, as the same amount of protein were always loaded into the gel (4.5 µg of protein).
To gain more insights on the effects of temperature on digestive efficiency, and the putative contribution of different isoenzymes in response to differences in rearing temperature, the thermal sensitivity (Q10 value) and activation energy (Ea) were calculated. Q10 values were determined using the van ‘t Hoff equation as follows:
where A1 and A2 are activity levels at temperatures T1 and T2 (when T1< T2). The activation energy (Ea) was obtained from slopes in Arrhenius plots:
where R is the gas constant (8.314 J K-1 mol-1). The values were calculated for the range from 30 to 50°C for Q10 and from 30 to 60°C for Ea of lipase and pepsin and from 10 to 60°C for Ea of trypsin, chymotrypsin and leucine aminopeptidase.
All descriptive statistics are expressed as mean ± standard deviation of the mean (SD). For each enzyme, statistical differences in total activity among temperatures were assessed through one-way ANOVA and, whenever significant differences were identified, means were compared by the Post hoc multiple comparisons Tukey’s test. Differences among temperatures were considered significant at P< 0.05. Before analyses, the ANOVA assumptions of normality and homogeneity of variance were tested using the Shapiro-Wilk and Levene’s tests, respectively.
A forward stepwise discriminant analysis using the activity of all enzymes studied at the different regions of the digestive tract was performed. This analysis was carried out to understand the combination of variables that better explain the differences in digestive biochemistry among fish reared at different temperatures. For the analysis, it was considered F to enter as 0.01, F to remove at 0.0, and tolerance of 0.01. After significant functions development, the relative importance of the original variables was gauged by standardized values. All analyses were performed with SPSS 26 software (IBM, New York, USA).
Total enzyme secretion was assessed by performing the analyses at the optimal pH for each enzyme and 37°C. Chymotrypsin was the most abundant enzyme and lipase the enzyme with the lowest activity (Figure 2A). Significant differences in total activity levels were found for all enzymes among the rearing temperatures, with fish reared at 22 and 26°C showing higher activity levels than at 18°C for all intestinal enzymes except for chymotrypsin, for which activity levels were only statistically different between fish reared at 26 and 18°C (Figure 2A). Total pepsin activity levels were higher in fish reared at 22 and 26°C than at 18°C (Supplementary Table 1). Under real conditions of temperature (18, 22 or 26°C) and physiological pH, the general activity pattern in pyloric caeca was similar but activity levels much lower (Supplementary Table 1). This was particularly noticeable for pepsin and lipase. Lipase activity was undetectable in extracts from fish reared at 22 and 18°C, and pepsin activity was not detected in any extract, independently of rearing temperature (Supplementary Table 1).
Figure 2 (A) Total activity of digestive enzymes in the intestinal tract of Seriola dumerili juveniles reared at 18 (blue), 22 (green) and 26°C (orange). (B) Total activity of digestive enzymes present in the gut sections of Seriola dumerili reared at 22°C. PC – pyloric caeca, AI – anterior intestine, MI – middle intestine, PI – posterior intestine. Chymotrypsin activity is represented in the right Y axis. TRY, trypsin; CHY, chymotrypsin; LAP, leucine aminopeptidase; LIP, lipase. Data is presented as means ± SD (n = 8). Different letters indicate significant differences for each enzyme among rearing temperatures (P< 0.05).
Extracts from fish reared at 22°C were chosen to describe the overall distribution of activities along the digestive tract. At the moment of sampling (after last meal), all digestive enzymes analyzed were more abundant in the middle and posterior intestine (Figure 2B). Interestingly, the transit of the enzymes along the tract was not identical, as most chymotrypsin activity was already in the posterior intestine, while for trypsin a significant amount of activity was still in the middle intestine at this time (Figure 2B).
Extracts from fish reared at all the three temperatures (18, 22, and 26°C) were further used to depict the effect of rearing temperature on the relative relevance of the enzymes at particular sections of the tract, by performing a discriminant analysis. Two significant discriminant functions could be developed from the activity of digestive enzymes in all the analyzed sections. These functions collectively accounted for 100% of the total variance (Table 1). The activity of lipase in pyloric caeca and posterior intestine, total lipase activity, trypsin in pyloric caeca, and chymotrypsin in almost all segments of the tract were the variables with the highest relevance in the first discriminant function (Table 1), which accounted for 78.3% of the total variance (Table 1). In this function, 18/22°C and 26°C adapted fish showed opposed central values (Figure 3, axis x). However, the second discriminant function, although explained only 21.7% of the total variance, discriminated among all the three temperatures (Figure 3, axis y), with chymotrypsin in the pyloric caeca being by far the variable with the highest relevance, although trypsin in the pyloric caeca and chymotrypsin in the middle intestine also significantly contributed (Table 1). Conversely, their activity in other sections of the tract and their total activities had poor importance in this function, as well as the activity of the other enzymes studied.
Table 1 (A) Summary of the canonical discrimination analysis for discriminant functions used to identified differences in digestive biochemistry among Seriola dumerili juveniles reared at different temperatures. (B) Standardized function coefficients for each of the significant discriminant functions used to identify differences in digestive biochemistry.
Figure 3 Plot of the two axes from the forward stepwise discriminant function analysis of digestive enzymes total activities in Seriola dumerili juveniles reared at 18 (blue), 22 (green) and 26°C (orange). Group centroids are represented by blue squares.
Optimal concentrations of NaCl and CaCl2 for the activity of the enzymes studied were assessed (Table 2). Notably, serine proteases such as trypsin and chymotrypsin required 10 times more CaCl2 for optimal activity than leucine aminopeptidase and lipase (Table 2). Optimal pH and temperature for the activity of the enzymes studied were similar among fishes adapted to different temperatures. Optimal pH was 2.5 for pepsin, 10 for trypsin, 9 for chymotrypsin, 7.5-8 for leucine aminopeptidase, and 11 for lipase. Optimal temperature was 60-70°C for pepsin, greater than or equal to 70°C for trypsin and chymotrypsin, 50-60°C for leucine aminopeptidase, and 65-75°C for lipase (Table 2). However, slight differences were noted such as higher optimal temperature for pepsin and leucine aminopeptidase in extracts coming from fish adapted to the higher temperature (i.e., 26°C), and higher optimal temperature for lipase in extracts coming from fish adapted to 22°C (Table 2).
Table 2 Optimal pH, temperature, ionic strength and calcium for digestive enzymes activities in the greater amberjack (Seriola dumerili) adapted to different rearing temperatures (18, 22 and 26°C).
Enzymatic activities shared a similar pattern of pH stability, being affected by all pH values assayed even after short incubation time (Figure 4). The most notable difference among enzymes is that lipase activity was less stable facing all pH assayed, losing more than 50% of activity after 15 min at physiological pH (i.e., pH8) and with pH 3 completely abrogating the activity after this incubation time (Figure 4H). Pepsin activity was stable for 1 h in the range of pH 3 to 8 (Figure 4I).
Figure 4 Stability of trypsin (TRY), chymotrypsin (CHY), leucine aminopeptidase (LAP), lipase (LIP) and pepsin (PP) activities from pyloric caeca and stomach of S. dumerili incubated at different pH. Leucine aminopeptidase, lipase and pepsin graphs represent mean values from all treatments. Individual graphs of pH stability for each enzyme in extracts coming from the different rearing temperatures are shown in the Supplementary Figure 1.
Regarding the effect of rearing temperatures on pH stability of fish enzymes, differences were observed only for trypsin and chymotrypsin. For instance, while for all fish most alkaline enzymes studied (trypsin, chymotrypsin, leucine aminopeptidase, and lipase) lost about a 50%of activity after 15 min at all pH values assayed (pH 3, 5, 8, 11), trypsin activity was stable at pH 11 for 1h only in extracts from 18°C-adapted fish (Figure 4A). In addition, trypsin activity in extracts from 26°C-adapted fish was the most affected by pH 3, losing about 80% of activity after 30 min, while in extracts from fish from the other two water temperatures, trypsin activity remained above 40% at this time (Figures 4A-C). Also, chymotrypsin activity was more stable in the range pH 5 to 11 in extracts coming from 26°C-adapted fish than in those from the other two groups (Figure 4F). Conversely, stability patterns of leucine aminopeptidase, lipase, and pepsin activities were nearly identical in fish adapted to the three experimental temperatures (Figures 4G-I).
Differences were evident in the thermal stability of studied enzymes (Figure 5). The most stable enzyme was trypsin, whose activity was stable up to 60°C for at least 1 h (Figure 5G). The next more stable enzyme was leucine aminopeptidase, which was fairly stable at 40°C for 1 h and kept more than 50% of activity after this period at 60°C (Figures 5A-C). Pepsin was also stable at 40°C for 1 h, although its activity decreased more than 50% after 30 min at 60°C (Figure 5I). Conversely, chymotrypsin activity was thermally sensitive, losing 20% of activity at 40°C and more than 80% of activity at 60°C after just 15 min (Figure 5H). However, the most thermally sensitive enzyme was lipase; 60°C was able to completely abrogate activity after just 15 min (Figures 5D–F).
Figure 5 Stability of leucine aminopeptidase (LAP), lipase (LIP), trypsin (TRY), chymotrypsin (CHY) and pepsin (PP) activities from pyloric caeca and stomach of S. dumerili incubated at different temperatures. Trypsin, chymotrypsin and pepsin graphs represent mean values from all treatments. Individual graphs of thermal stability for each enzyme in extracts coming from the different rearing temperatures are shown in the Supplementary Figure 2.
Regarding the influence of rearing temperature on the thermal stability of fish digestive enzymes, variations for leucine aminopeptidase and lipase were observed. Leucine aminopeptidase activity was more stable in extracts from fish adapted to 22°C (Figure 5B), retaining 80% of activity after 1 h at 60°C, whereas only 40-60% of activity was maintained under these conditions in extracts from fish from 18°C or 26°C (Figures 5A, C). Likewise, lipase activity in extracts from 26°C-adapted fish was more stable than that from fish maintained at lower temperatures (Figure 5F). Conversely, thermal stability pattern of trypsin, chymotrypsin, and pepsin activities was nearly identical among extracts from fish adapted to the different temperatures (Figures 5G–I).
Given the key role of serine proteases in protein digestion, classes of proteases in the extracts were further assessed by using specific protease inhibitors to inhibit casein hydrolysis. The serine protease inhibitor SBTI abrogated 37-47% of caseinolytic activity in the extracts (Table 3). The trypsin inhibitor TLCK inhibited 20-38% of the activity, while 13-28% of proteolysis was abrogated by the chymotrypsin inhibitor TPCK (Table 3). Interestingly, the chelating agents EDTA inhibited caseinolytic activity by 17-23% (Table 3).
Table 3 Effect of proteinase inhibitors on caseinolytic activity of pyloric caeca of S. dumerili adapted to different rearing temperatures (18, 22 and 26°C).
Regarding differences among fish rearing temperatures on the relative contribution of the different types of proteolytic enzymes to overall protein digestion, we found a reverse trend with trypsin and chymotrypsin inhibitors (Table 3). Concomitantly with water temperature increase, inhibition of caseinolytic activity by TLCK increased while inhibition by TPCK decreased (Table 3).
To further evaluate the relative contribution of trypsin and chymotrypsin in fish reared at different temperatures, we used the same inhibitors (TLCK and TPCK) to inhibit trypsin and chymotrypsin activities, this time through the use of specific substrates (BApNA and SApNA, respectively). TLCK inhibited 80% of trypsin activity on BApNA irrespective of the thermal origin of fish (Figure 6A), whereas TPCK inhibition pattern was reverse to water temperature. In extracts coming from 18°C-adapted fish, TPCK inhibited 80% of chymotrypsin activity, while only 40% of the activity was inhibited in fish kept at the highest temperature (26°C) (Figure 6B).
Figure 6 Effect of TLCK (A) and TPCK (B) inhibitors on trypsin and chymotrypsin activities, respectively, of pyloric caeca of S. dumerili adapted to different rearing temperatures (18, 22 and 26°C).
Zymograms revealed 6 active zones of caseinolytic activity (Figure 7A, control lane). All bands were inhibited by SBTI and EDTA, indicating they are serine proteases depending on divalent cations, presumably calcium, for their activity (Figure 7A). Conversely, PMSF, which is other serine protease inhibitor, did not inhibit activity bands (Figure 7A). Enzymes with the lowest and the highest electrophoretic mobility, and that of about 30 kDa were total or partially inhibited by TLCK in addition to SBTI, revealing that these bands correspond to trypsin enzymes (Figure 7A). Three very active bands of similar molecular weight (around 18 kDa) were inhibited by SBTI but not by TLCK, thus being more likely chymotrypsins, although not affected by TPCK (Figure 7A).
Figure 7 (A) 13% substrate SDS-PAGE showing caseinolytic activity bands in a pool containing pyloric caeca extracts of S. dumerili adapted to different rearing temperatures (18, 22 and 26°C) (Ctrl) and inhibition by specific inhibitors for trypsin (TLCK), chymotrypsin (TPCK), serine proteinases (PMSF and SBTI) and metalloproteases (EDTA). Type of proteases is indicated in the control lane as follows: trypsin like proteinases (→) and chymotrypsin like proteinases (►). (B) 13% substrate SDS-PAGE showing caseinolytic activity bands in independent pools of pyloric caeca extracts of S. dumerili from each rearing temperature (18, 22 and 26°C) and incubated at 37°C or at the temperature the fish providing each sample was adapted to (18, 22 and 26°C).
We further studied the variations in isoenzymes composition and/or activity in extracts coming from fish adapted to different temperatures (Figure 7B). When incubation was performed at a standard high temperature (37°C), no differences in activity or isoenzymes composition were noticed among extracts from fish adapted to the different temperatures (Figure 7B). However, when incubations were done at the temperature in which the fish were reared, clear differences were observed. Activity levels, inferred from band intensity, decreased as incubation temperature did for all bands except for the chymotrypsin of the higher electrophoretic mobility. This band exhibited a high activity at 18°C, similar to that observed when incubated at higher temperatures (Figure 7B), revealing differences in temperature dependence for activity among chymotrypsin isoforms.
Q10 values were similar for all enzymes studied and no major differences were observed among extracts coming from fish reared at different temperatures (Table 4). No break point in the Arrhenius plots for the studied enzymes was found. However, values of Ea for trypsin, chymotrypsin and leucine aminopeptidase activities decreased as fish rearing temperature increased (Table 4). For pepsin and lipase Ea values were more variable, with the maximum values observed in fish at 18 for both enzymes, while the minimum values were recorded in fish at 26 and 22°C for pepsin and lipase, respectively (Table 4).
Table 4 Q10 value and activation energy (Ea) for digestive enzymes of Seriola dumerili at different rearing temperatures.
Chymotrypsin was by far the most abundant enzyme in the digestive tract. The contribution of trypsin and chymotrypsin activities in fish is rather variable. For example, in scaleless carp (Gymnocypris przewalskii) the activity of trypsin is much higher than that of chymotrypsin (Tian et al., 2019), while in the Pacific bluefin tuna (Thunnus orientalis) chymotrypsin activity contributes more to digestion than trypsin activity (de la Parra et al., 2007). Even so, our observations in general agree with the notion that fish with carnivorous preferences show high proteolytic enzyme activities to digest high-protein animals, while herbivorous species exhibit higher carbohydrase activities to digest the storage carbohydrates of macroalgae (German et al., 2004). Indeed, no detectable amylase activity in any segment of the tract was found in the present study, using a colorimetric method that was suited to measure this activity in other fish species such as gilthead seabream (Sparus aurata) (Perera and Yúfera, 2017). This result is in line with observations in other carnivore fish regarding low or undetectable amylase activity. A previous study assessing amylase activity in fish with different feeding habits linked low amylase activity to carnivorous fish species with a stomach, whereas stomachless omnivorous fish trend to show higher amylase activity levels (Hidalgo et al., 1999). These authors reported low amylase activity levels for gilthead seabream, rainbow trout (Oncorhynchus mykiss) and European eel (Anguilla anguilla). Likewise, almost undetectable levels of amylase activity have been reported for the carnivorous fish Glyptosternum maculatum (Xiong et al., 2011). On the other hand, total pepsin activity, measured at the enzyme optimal pH and 37°C, is a good estimation for the availability of pepsinogen, but not for active pepsin. pH in the fish stomach does not always reach the levels required for pepsin activation, explaining the negligible pepsin activity levels observed in the present study when measured at physiological conditions. A relatively poor acidification capacity, and thus, limited pepsin activation, have been previously reported for gilthead seabream juveniles (Gilannejad et al., 2018; Gilannejad et al., 2021), Atlantic salmon (Krogdahl et al., 2015) and Senegalese sole juveniles (Yúfera and Darias, 2007), suggesting that functional pepsin activity is frequently over estimated.
Temperature is well documented to significantly modify transit time in fish (Yúfera et al., 2019; Volkoff and Rønnestad, 2020). The observed overall distribution of enzymes along the digestive tract, with higher activities toward the posterior segments, seems to be a result of the time of sampling after feeding, and corroborates the results of previous studies using inert markers on the rapid transit time in this species under the same feeding regimen (Navarro-Guillén et al., 2021). The results of the discriminant analysis revealed a clear effect of rearing temperature on the digestive function of the greater amberjack. For instance, lipase and chymotrypsin activities through nearly all segments of the tract are responsive to high environmental temperature, suggesting a shift in the digestion capacity from 18/22°C to 26°C. In fact, in a previous trial, greater amberjack reared at 26°C showed higher growth rate and feed efficiency than fish reared at 22 and 18°C (Soriano, 2021), and also in consonance with the study by Fernández-Montero et al. (2018). However, it is interesting that both, trypsin and chymotrypsin activities in pyloric caeca are the better discriminants of the environmental temperature the fish are acclimated to. The fact that this occurs well after feeding, and not as a response to recent feed ingestion, strongly suggests an adaptation to ambient temperature also at the level of synthesis and secretion of enzymes, likely as preparation for next feeding, and/or different retention time of these enzymes in the pyloric caeca. Indeed, in yellowtail kingfish (Seriola lalandi), a greater proportion of nutrient digestion occurred during winter in the stomach, pyloric caeca and anterior intestine, while it occurred in the posterior intestine in summer, probably due to the slower gut motility during winter (Miegel et al., 2010). Globally, our multivariate analysis supports that fish digestion plasticity regarding environmental temperature largely relies on trypsin and chymotrypsin activities, being chymotrypsin the most responsive enzyme, though lipid digestion seems also to be under temperature control.
Most of the digestive enzymes of greater amberjack share optimal conditions with those from other fish species (Kishimura et al., 2006; de la Parra et al., 2007; Candiotto et al., 2018) and crustaceans (Perera et al., 2008; Chávez-Rodríguez et al, 2020; Muhlia-Almazán and Fernández-Gimenez, 2022), pointing out the relative high thermal optimum of alkaline proteases, although a previous study described lower thermal optimum for trypsin and chymotrypsin of greater amberjack (Oliveira et al., 2017). Also, the enzymes studied are all susceptible to acidic conditions, with lipase being the most sensitive enzyme, probably as a result of a more complex tridimensional architecture and structural requirements for activity as reported for other lipases (Perera et al., 2008). Nevertheless, while proteases such as pepsin, trypsin and leucine aminopeptidase of greater amberjack are thermally robust, chymotrypsin activity is thermally sensitive, indicating a clear difference in the effects of temperature over chymotrypsin and all other proteases. This finding also points to chymotrypsin enzymes as putative drivers of the effects of temperature on the digestive process. Similar results of chymotrypsin thermal stability have been described for other fish species such as the Brazilian flounder (Paralichthys orbignyanus) and gilthead seabream (Alarcón et al., 1998; Liang et al., 2011; Candiotto et al., 2018).
The fact that the activity of most enzymes decreased up to a certain level and then remained relatively stable over time strongly suggests the presence of isoforms with differences in susceptibility to the assayed conditions. Moreover, while thermal stability patterns were nearly identical among most proteases studied regardless of rearing temperature (i.e., trypsin, chymotrypsin, pepsin), stability of trypsin and chymotrypsin under varied pH conditions suggests differences among rearing temperatures in isoforms composition or contribution of particular isoenzymes. Trypsin activity was more stable at highly alkaline pH values in extracts from 18°C-adapted fish, while the same enzyme was more affected by acidic conditions in extracts from 26°C-adapted fish. On the other hand, chymotrypsin activity was more stable in the range pH 5 to 11 in extracts coming from 26°C-adapted fish than in extracts coming from fish reared at 18/22°C. Isoforms for digestive proteases in fish often differ in biochemical features (Klomklao et al., 2009), thus our results were not unexpected in this regard, but the changes in the relative contribution of those isoforms driven by differences among fish rearing temperatures have not been reported before.
Our results on the inhibition of caseinolytic activity, as indirect indicative of overall protein digestion capacity, corroborate the key role of serine proteases in digestion, particularly trypsin and chymotrypsin activities, as occur in other fish species (Natalia et al., 2004; Candiotto et al., 2018). Moreover, we found a clear dependence of these enzymes on divalent cations, in agreement with that described for other fish species (Kishimura et al., 2006; Klomklao et al., 2009).
Results on the effects of trypsin and chymotrypsin inhibitors on caseinolytic activity suggest a shift in the contribution of these activities as the rearing temperature of fish varies. The higher inhibition of overall proteolysis by TPCK in extracts from fish adapted to 18°C suggests that chymotrypsin may have a more important role at lower temperatures. In Atlantic salmon (Salmo salar), the role of chymotrypsin in low temperature adaptation is well documented. Chymotrypsin activity increased when there was a reduction in growth rate because of external factors such as low temperature, thus fish compensated to a certain extent by lowering the trypsin to chymotrypsin (T/C) ratio (Rungruangsak-Torrissen et al., 2006).
We further addressed this issue by using specific substrates for these enzymes and observed that the contribution of activities changed for chymotrypsin but not for trypsin. Inhibition of chymotrypsin by TPCK showed a reverse pattern with water temperature, with inhibition increasing from 40% to 80% as fish rearing temperature decreased. The most plausible explanation for these results is a change in the relative contribution of chymotrypsin isoenzymes, with an increase in the contribution of particular chymotrypsin isoenzymes at lower temperatures that are more sensitive to TPCK. Unfortunately, while trypsin isoenzymes were clearly identified in activity gels, with apparent molecular weights similar to those reported for other fish trypsins (Natalia et al., 2004; Kurtovic et al., 2006; Klomklao et al., 2009), our attempts to identify chymotrypsin isoenzymes in the zymogram gels were unsuccessful using TPCK as done in the tube assays. Other chymotrypsin inhibitor was used, carbobenzoxy-Phe chloromethyl ketone (ZPCK, data not shown), but it did not work either. Problems to identify fish chymotrypsins in gels are recurrent in the literature. For gilthead seabream and common dentex (Dentex dentex), no inhibitory effect was observed for TPCK in gels, though ZPCK partially deleted two bands in zymograms from both species (Alarcón et al., 1998). Similarly, in the Pacific red snapper (Lutjanus peru), TPCK had no inhibitory effect in zymograms of alkaline proteases (Peña-Marín et al., 2021). However, in the present study, three bands were strongly inhibited by SBTI but not by TLCK, thus assuming to be chymotrypsins.
When casein zymograms were performed using pools of extracts coming from fishes adapted to the different temperatures, or with extracts from individual fishes (Supplementary Figure 3), no variation in the presence/absence of these three isoforms was noticed among rearing temperatures. Only a small individual variation was observed, likely due to low-frequency individual polymorphism, with one of these isoforms (the one of intermediate electrophoretic mobility) being absent or less represented in few individuals irrespective of rearing temperature. Thus, results obtained in this study regarding the changing role of chymotrypsin activity at low temperature could not be explained by a shift in particular isoforms. Therefore, a feasible hypothesis is that variation in the relative contribution of chymotrypsin isoenzymes to a particular environmental temperature occurs due to different physic-chemical features among isoforms as suggested by results from our stability assays.
To gain insight on the in vivo contribution of chymotrypsin isoenzymes under different ambient temperatures, we further analyzed by zymograms the changes in chymotrypsin isoenzymes pattern and activity in extracts from fish adapted to different temperatures and revealed the activity in gels at 37°C and at the corresponding temperatures. Activity of isoforms at 37°C, inferred from band intensity, was identical for extracts from fishes adapted to the different temperatures, corroborating that the amount of enzyme loaded into the gel was the same among treatments, and that there are no changes in synthesis and secretion of particular isoforms among temperatures. However, activity decreased as incubation temperature did for all bands (including the three trypsins and two chymotrypsin isoforms) except for the chymotrypsin of the higher electrophoretic mobility. This chymotrypsin isoenzyme exhibited high activity levels at 18°C, being similar to that observed when incubated at higher temperatures (Figure 7B). Thus, it is demonstrated that the isoenzyme richness and the biochemical differences among isoforms revealed in this study, lead to different digestion performance under realistic temperature conditions. Although in only few species, there is some evidence of the impact of protease isoforms with different biochemical features on fish performance at different temperatures (Rungruangsak-Torrissen et al., 1998; Rungruangsak-Torrissen and Male, 2000; Gelman et al., 2008). However, whether the presence of this chymotrypsin isoenzyme in the greater amberjack compensates for decrease activity of other proteases, and to what extent, remains unknown. This will be difficult to assess in vivo as all individuals hold this isoenzyme and thus, no performance comparisons can be made at low temperatures between individuals having or not the isoenzyme, as assessed in the Atlantic salmon (Rungruangsak-Torrissen et al., 1998; Rungruangsak-Torrissen and Male, 2000). Yet, the fact that all individuals analyzed presented this isoform may suggest that it has an adaptive value for the species. The analysis of a higher number of individuals and from different origins, to broad the genetic variability of the population studied, would shed some light on this issue.
On average, Q10 and Ea values were similar for all studied enzymes of greater amberjack. The Ea found for the studied enzymes were lower than those described for silver carp, common carp, sheatfish, gilthead seabream, redfish and turbot (Jónás et al., 1983; Munilla-Morán and Saborido-Rey, 1996; Alarcón et al., 1998), though this last study used a proteinaseous substrate for alkaline activity, while specific substrates were used in the current work for individual enzymes. In any case, enzymes of the greater amberjack seem to be more efficient that those of the above-mentioned fish, as the lower the Ea value the higher the efficiency of the enzyme.
Moreover, we found no break point in the Arrhenius plots for the studied enzymes, which is interpreted as an adaptation to a wide temperature range, as described for redfish and turbot (Munilla-Morán and Saborido-Rey, 1996). In other fish species, break points are evident, indicating changes in digestion efficiency. For gilthead seabream, Munilla-Morán and Saborido-Rey (1996) described a break point at 20°C for gastric acid proteases, being Ea below this temperature about six times higher than above the break point, indicating that this species seems to have a more efficient acidic digestion of protein in a warm environment. By contrast, a later study on the same species did not find such a break point for acid proteases (Alarcón et al., 1998).
In addition to the absence of break points, we observed a slight decrease in Ea for proteases (trypsin, chymotrypsin and leucine aminopeptidase) as rearing temperature increased, with lower values at 26°C. These observations agree with the notion of different biochemical features among isoenzymes. Increased efficiency at higher environmental temperature would aid the fish to deal with an increased feed intake and/or counteract an increase in transit time. By contrast, lipase Ea pattern was not linked to temperature gradient. The lowest Ea for lipase was found at 22°C (standard temperature for the species culture), while the highest was at 18°C. The more reasonable hypothesis for these results is again the presence of isoforms. However, due to the technical difficulty of performing zymograms for lipases (Kurtovic et al., 2010), we are not able for the moment to confirm this assumption.
In conclusion, the present study describes the functional characteristics of greater amberjack digestive enzymes, showing that they have biochemical features similar to those of other fish species. It is of particular interest that some specific enzymes and isoforms, particularly chymotrypsins, may play a key role in providing certain phenotypic flexibility regarding environmental temperature, being this study one of the few providing experimental evidence of an adaptive value of digestive enzyme polymorphism in farmed fish. More investigation is needed on this issue since a better understanding of the balance among the different factors affecting digestion efficiency in the greater amberjack would lead to optimized feeding protocols depending on the environmental temperature, wherever in sea cages or in-land facilities.
The original contributions presented in the study are included in the article/supplementary material. Further inquiries can be directed to the corresponding author.
The animal study was reviewed and approved by the Spanish National Research Council Bioethical Committee.
CN-G: conceptualization, methodology, formal analysis, writing—original draft preparation. MY: conceptualization, writing—review and editing, project administration, and funding acquisition. EP: conceptualization, methodology, formal analysis, supervision, writing—original draft preparation, writing—review and editing. All authors contributed to the article and approved the submitted version.
This research was funded by the project RTI208-096134-B-I00 from the Spanish Ministry of Science, Innovation and Universities (MCIU), the State Research Agency (AEI) and the European Regional Development Fund (FEDER/ERDF) granted to MY. CN-G was supported by the researcher contract DOC_01203 funded by the Andalusian Plan for Research Development and Innovation (PAIDI 2020).
The authors declare that the research was conducted in the absence of any commercial or financial relationships that could be construed as a potential conflict of interest.
All claims expressed in this article are solely those of the authors and do not necessarily represent those of their affiliated organizations, or those of the publisher, the editors and the reviewers. Any product that may be evaluated in this article, or claim that may be made by its manufacturer, is not guaranteed or endorsed by the publisher.
The Supplementary Material for this article can be found online at: https://www.frontiersin.org/articles/10.3389/fmars.2022.960746/full#supplementary-material
Alarcón F. J., Díaz M., Moyano F. J., Abellán E. (1998). Characterization and functional properties of digestive proteases in two sparids; gilthead seabream (Sparus aurata) and common dentex (Dentex dentex). Fish Physiol. Biochem. 19 (3), 257–267. doi: 10.1023/A:1007717708491
Anson M. L. (1938). The estimation of pepsin, trypsin, papain, and cathepsin with hemoglobin. J. Gen. Physiol. 22 (1), 79–89. doi: 10.1085/jgp.22.1.79
Candiotto F. B., Freitas-Júnior A. C. V., Neri R. C. A., Bezerra R. S., Rodrigues R. V., Sampaio L. A., et al. (2018). Characterization of digestive enzymes from captive Brazilian flounder Paralichthys orbignyanus. Braz. J. Biol. 78 (2), 281–288. doi: 10.1590/1519-6984.06616
Chávez-Rodríguez L., Rodríguez-Viera L., Montero-Alejo V., Perdomo-Morales R., Mancera J. M., Perera E. (2020). A very sctive α-amylase and an inhibitor-based control of proteinases are key features of digestive biochemistry of the omnivorous Caribbean king crab Maguimithrax spinosissimus. J. Evol. Biochem. Physiol. 56 (6), 550–564. doi: 10.1134/S0022093020060083
de la Parra A. M., Rosas A., Lazo J. P., Viana M. T. (2007). Partial characterization of the digestive enzymes of pacific bluefin tuna Thunnus orientalis under culture conditions. Fish Physiol. Biochem. 33 (3), 223–231. doi: 10.1007/s10695-007-9134-9
Díaz-Mendoza M., Ortego F., García de Lacoba M., Magaña C., de la Poza M., Farinós G. P., et al. (2005). Diversity of trypsins in the Mediterranean corn borer Sesamia nonagrioides (Lepidoptera: Noctuidae), revealed by nucleic acid sequences and enzyme purification. Insect Biochem. Mol. Biol. 35, 1005–1020. doi: 10.1016/j.ibmb.2005.04.003
Fernández-Montero A., Caballero M. J., Torrecillas S., Tuset V. M., Lombarte A., Ginés R., et al. (2018). Effect of temperature on growth performance of greater amberjack (Seriola dumerili risso 1810) juveniles. Aquac. Res. 49, 908–918. doi: 10.1111/are.13537
Fernández-Montero A., Torrecillas S., Tort L., Ginés R., Acosta F., Izquierdo M. S., et al. (2020). Stress response and skin mucus production of greater amberjack (Seriola dumerili) under different rearing conditions. Aquaculture 520, 735005. doi: 10.1016/j.aquaculture.2020.735005
Gelman A., Kuz'mina V., Drabkin V., Glatman L. (2008). “Temperature adaptation of digestive enzymes in fish,” in In feeding and digestive functions in fishes. Eds. Cyrino J. E. P., Bureau D. P., Kapoor B. (Enfield, NH, USA: Science Publishers), 155–225.
German D. P., Horn M. H., Gawlicka A. (2004). Digestive enzyme activities in herbivorous and carnivorous prickleback fishes (Teleostei: Stichaeidae): ontogenetic, dietary, and phylogenetic effects. Physiol. Biochem. Zool. 77 (5), 789–804. doi: 10.1086/422228
Gilannejad N., Martínez-Rodríguez G., Yúfera M., Moyano F. J. (2018). Modelling digestive hydrolysis of nutrients in fish using factorial designs and desirability function. PloS One 13 (11), e0206556. doi: 10.1371/journal.pone.0206556
Gilannejad N., Moyano F. J., Martínez-Rodríguez G., Yúfera M. (2021). The digestive function of gilthead seabream juveniles in relation to feeding frequency. Aquaculture 531, 735867. doi: 10.1016/j.aquaculture.2020.735867
Hidalgo M. C., Urea E., Sanz A. (1999). Comparative study of digestive enzymes in fish with different nutritional habits. proteolytic and amylase activities. Aquaculture 170 (3), 267–283. doi: 10.1016/S0044-8486(98)00413-X
Hu P., Liu M., Liu Y., Wang J., Zhang D., Niu H., et al. (2016). Transcriptome comparison reveals a genetic network regulating the lower temperature limit in fish. Sci. Rep. 6, 28952. doi: 10.1038/srep28952
Jónás E., Rágyanszki M., Oláh J., Boross L. (1983). Proteolytic digestive enzymes of carnivorous (Silurus glanis l.), herbivorous (Hypophthalmichthys molitrix val.) and omnivorous (Cyprinus carpio l.) fishes. Aquaculture 30, 145–154. doi: 10.1016/0044-8486(83)90158-8
Kishimura H., Hayashi K., Miyashita Y., Nonami Y. (2006). Characteristics of trypsins from the viscera of true sardine (Sardinops melanostictus) and the pyloric ceca of arabesque greenling (Pleuroprammus azonus). Food Chem. 97 (1), 65–70. doi: 10.1016/j.foodchem.2005.03.008
Klomklao S., Kishimura H., Nonami Y., Benjakul S. (2009). Biochemical properties of two isoforms of trypsin purified from the intestine of skipjack tuna (Katsuwonus pelamis). Food Chem. 115 (1), 155–162. doi: 10.1016/j.foodchem.2008.11.087
Kofuji P. Y. M., Akimoto A., Hosokawa H., Masumoto T. (2005). Seasonal changes in proteolytic enzymes of yellowtail Seriola quinqueradiata (Temminck & schlegel; carangidae) fed extruded diets containing different protein and energy levels. Aquac. Res. 36 (7), 696–703. doi: 10.1111/j.1365-2109.2005.01276.x
Krogdahl Å., Sundby A., Holm H. (2015). Characteristics of digestive processes in Atlantic salmon (Salmo salar). Enzyme pH optima, chyme pH, and enzyme activities. Aquaculture 449, 27–36. doi: 10.1016/j.aquaculture.2015.02.032
Kurtovic I., Marshall S. N., Simpson B. K. (2006). Isolation and characterization of a trypsin fraction from the pyloric ceca of chinook salmon (Oncorhynchus tshawytscha). Comp. Biochem. Phys. B 143 (4), 432–440. doi: 10.1016/j.cbpb.2005.12.020
Kurtovic I., Marshall S. N., Zhao X., Simpson B. K. (2010). Purification and properties of digestive lipases from Chinook salmon (Oncorhynchus tshawytscha) and new Zealand hoki (Macruronus novaezelandiae). Fish Physiol. Biochem. 36 (4), 1041–1060. doi: 10.1007/s10695-010-9382-y
Liang Z., Suzanne M. B., Abdel E. G., Marianne S. B., Deepika D. (2011). Extraction, purification and characterization of fish chymotrypsin: a review. Am. J. Biochem. Biotechnol. 7 (3), 104–123. doi: 10.3844/ajbbsp.2011.104.123
Miegel R. P., Pain S. J., Wettere W. H. E. J., Howarth G. S., Stone D. A. J. (2010). Effect of water temperature on gut transit time, digestive enzyme activity and nutrient digestibility in yellowtail kingfish (Seriola lalandi). Aquaculture 308 (3/4), 145–151. doi: 10.1016/j.aquaculture.2010.07.036
Monge-Ortiz R., Martínez-Llorens S., Lemos-Neto M. J., Falcó-Giaccaglia S. L., Pagán M. J., Godoy-Olmos S., et al. (2020). Growth, sensory and chemical characterization of Mediterranean yellowtail (Seriola dumerili) fed diets with partial replacement of fish meal by other protein sources. Aquac. Rep. 18, 100455. doi: 10.1016/j.aqrep.2020.10046
Muhlia-Almazán A. T., Fernández-Gimenez A. V. (2022). Understanding the digestive peptidases from crustaceans: from their biochemical basis and classical perspective to the biotechnological approach. Mar. Biotechnol. 18, 480–491. doi: 10.1007/s10126-022-10122-2
Munilla-Morán R., Saborido-Rey F. (1996). Digestive enzymes in marine species. i. proteinase activities in gut from redfish (Sebastes mentella), seabream (Sparus aurata) and turbot (Scophthalmus maximus). Comp. Biochem. Phys. B 113, 395–402. doi: 10.1016/0305-0491(95)02057-8
Natalia Y., Hashim R., Ali A., Chong A. (2004). Characterization of digestive enzymes in a carnivorous ornamental fish, the Asian bony tongue Scleropages formosus (Osteoglossidae). Aquaculture 233 (1), 305–320. doi: 10.1016/j.aquaculture.2003.08.012
Navarro-Guillén C., Conceição L. E. C., Pinto W., Siguero I., Urrutia P., Moyano F. J., et al. (2019). Fast growing greater amberjack post-larvae require a high energy-high protein weaning diet. Aquaculture 499, 195–202. doi: 10.1016/j.aquaculture.2018.09.037
Navarro-Guillén C., Gilannejad N., Martínez-Rodríguez G., Yúfera M. (2021). Water temperature affects feeding behavior and gut transit time in greater amberjack juveniles (Seriola dumerili) fed multiple daily meals. Eur. Aquac. Soc. 867–868
Nitzan T., Kokou F., Doron-Faigenboim A., Slosman T., Biran J., Mizrahi I., et al. (2019). Transcriptome analysis reveals common and differential response to low temperature exposure between tolerant and sensitive blue tilapia (Oreochromis aureus). Front. Genet. 10. doi: 10.3389/fgene.2019.00100
Oliveira V., Nascimento T. P., Assis C. R. D., Bezerra R. S., Porto A. L. F. (2017). Study on enzymes of industrial interest in digestive viscera: Greater amberjack (Seriola dumerili). J. Coast. Life Med. 5, 233–238. doi: 10.12980/jclm.5.2017J6-300
Pelusio N. F., Scicchitano D., Parma L., Dondi F., Brini E., D’Amico F., et al. (2021). Interaction between dietary lipid level and seasonal temperature changes in gilthead sea bream Sparus aurata: effects on growth, fat deposition, plasma biochemistry, digestive enzyme activity, and gut bacterial community. Front. Mar. Sci. 8. doi: 10.3389/fmars.2021.664701
Peña-Marín E. S., Ibarra-Castro L., Martínez-Brown J. M., Hernández-López I. A., Tovar-Ramírez D., Pérez-Urbiola J. C., et al. (2021). Partial characterization of digestive proteases in pacific red snapper Lutjanus peru nichols & murphy 1922 (Perciformes: Lutjanidae). Lat. Am. J. Aquat. Res. 49, 442–450. doi: 10.3856/vol49-issue3-fulltext-2624
Perera E., Moyano F. J., Díaz M., Perdomo-Morales R., Montero-Alejo V., Alonso E., et al. (2008). Polymorphism and partial characterization of digestive enzymes in the spiny lobster Panulirus argus. comp. Biochem. Phys. B 150 (3), 247–254. doi: 10.1016/j.cbpb.2008.03.009
Perera E., Rodríguez-Viera L., Perdomo-Morales R., Montero-Alejo V., Moyano F. J., Martínez-Rodríguez G., et al. (2015). Trypsin isozymes in the lobster Panulirus argus (Latreille 1804): from molecules to physiology. J. Comp. Physiol. B. 185 (1), 17–35. doi: 10.1007/s00360-014-0851-y
Perera E., Yúfera M. (2017). Effects of soybean meal on digestive enzymes activity, expression of inflammation-related genes, and chromatin modifications in marine fish (Sparus aurata l.) larvae. Fish Physiol. Biochem. 43 (2), 563–578. doi: 10.1007/s10695-016-0310-7
Rungruangsak-Torrissen K. (2012). “Trypsin and its implementations for growth, maturation, and dietary quality assessment,” in Trypsin: structure, biosynthesis and functions. Eds. Weaver K., Kelley C. (New York: Nova Science Publishers, Inc.), 1–59.
Rungruangsak-Torrissen K., Male R. (2000). “Trypsin isozymes: development, digestion and structure,” in Seafood enzymes, utilization and influence on postharvest seafood quality. Eds. Haard N. F., Simpson B. K. (New York: Marcel Dekker, Inc.).
Rungruangsak-Torrissen K., Moss R., Andresen L. H., Berg A., Waagbø R. (2006). Different expressions of trypsin and chymotrypsin in relation to growth in Atlantic salmon (Salmo salar l.). Fish Physiol. Biochem. 32 (1), 7–23. doi: 10.1007/s10695-005-0630-5
Rungruangsak-Torrissen K., Pringle G. M., Moss R., Houlihan D. F. (1998). Effects of varying rearing temperatures on expression of different trypsin isozymes, feed conversion efficiency and growth in Atlantic salmon (Salmo salar l.). Fish Physiol. Biochem. 19, 247–255. doi: 10.1023/A:1007731717021
Saborowski R., Schatte J., Gimenez L. (2012). Catalytic properties and polymorphism of serine endopeptidases from the midgut gland of the brown shrimp Crangon crangon (Decapoda, caridea) mar. Biol 159 (5), 1107–1118. doi: 10.1007/s00227-012-1890-0
Sandholt G. B., Stefansson B., Scheving R., Gudmundsdottir Á. (2019). Biochemical characterization of a native group III trypsin ZT from Atlantic cod (Gadus morhua). Int. J. Biol. Macromol. 15, 847–855. doi: 10.1016/j.ijbiomac.2018.12.099
Sicuro B., Luzzana U. (2016). The state of seriola spp. other than yellowtail (S. quinqueradiata) farming in the world. Rev. Fish. Sci. Aquac. 24, 314–325. doi: 10.1080/23308249.2016.1187583
Soriano P. (2021). Influencia de la temperatura sobre el crecimiento y metabolismo de la seriola (Seriola dumerili). [bachelor thesis] (Spain: University of Cádiz).
Tian H., Meng Y., Li C., Zhang L., Xu G., Shi Y., et al. (2019). A study of the digestive enzyme activities in scaleless carp (Gymnocypris przewalskii) on the qinghai-Tibetan plateau. Aquac. Rep. 13, 100174. doi: 10.1016/j.aqrep.2018.10.002
Torrissen K. R. (1987). Genetic variation of trypsin-like isozymes correlated to fish size of Atlantic salmon (Salmo salar). Aquaculture 62, 1–10. doi: 10.1016/0044-8486(87)90180-3
Torrissen K. R., Lied E., Espe M. (1994). Differences in digestion and absorption of dietary protein in Atlantic salmon (Salmo salar) with genetically different trypsin isozymes. J. Fish Biol. 45, 1087–1104. doi: 10.1111/j.1095-8649.1994.tb01075.x
Volkoff H., Rønnestad I. (2020). Effects of temperature on feeding and digestive processes in fish. Temperature 7, 307–320. doi: 10.1080/23328940.2020.1765950
Xiong D. M., Xie C. X., Zhang H. J., Liu H. P. (2011). Digestive enzymes along digestive tract of a carnivorous fish Glyptosternum maculatum (Sisoridae, siluriformes). J. Anim. Physiol. Anim. Nutr. 95 (1), 56–64. doi: 10.1111/j.1439-0396.2009.00984.x
Yúfera M., Darias M. J. (2007). Changes in the gastrointestinal pH from larvae to adult in Senegal sole (Solea senegalensis). Aquaculture 267, 94–99. doi: 10.1016/j.aquaculture.2007.02.009
Keywords: chymotrypsin, digestive enzymes, enzyme characterization, functional flexibility, isoforms, Seriola dumerili, water temperature
Citation: Navarro-Guillén C, Yúfera M and Perera E (2022) Biochemical features and modulation of digestive enzymes by environmental temperature in the greater amberjack, Seriola dumerili. Front. Mar. Sci. 9:960746. doi: 10.3389/fmars.2022.960746
Received: 03 June 2022; Accepted: 05 July 2022;
Published: 28 July 2022.
Edited by:
Mohamed Salah Azaza, National Institute of Marine Science and Technology, TunisiaReviewed by:
Saichiro Yokoyama, Kagoshima University, JapanCopyright © 2022 Navarro-Guillén, Yúfera and Perera. This is an open-access article distributed under the terms of the Creative Commons Attribution License (CC BY). The use, distribution or reproduction in other forums is permitted, provided the original author(s) and the copyright owner(s) are credited and that the original publication in this journal is cited, in accordance with accepted academic practice. No use, distribution or reproduction is permitted which does not comply with these terms.
*Correspondence: Carmen Navarro-Guillén, Y2FybWVuLm5hdmFycm9AY3NpYy5lcw==
Disclaimer: All claims expressed in this article are solely those of the authors and do not necessarily represent those of their affiliated organizations, or those of the publisher, the editors and the reviewers. Any product that may be evaluated in this article or claim that may be made by its manufacturer is not guaranteed or endorsed by the publisher.
Research integrity at Frontiers
Learn more about the work of our research integrity team to safeguard the quality of each article we publish.