- 1Fisheries Resources Division, National Marine Fisheries Service, Southwest Fisheries Science Center, La Jolla, CA, United States
- 2Fisheries Ecology Division, National Marine Fisheries Service, Southwest Fisheries Science Center, Arcata, CA, United States
- 3Department of Fisheries Biology, Humboldt State University, Arcata, CA, United States
- 4Environmental Research Division, National Marine Fisheries Service, Southwest Fisheries Science Center, Monterey, CA, United States
- 5Cooperative Institute for Marine Resources Studies, Oregon State University Hatfield Marine Science Center, Newport, OR, United States
- 6Fisheries Ecology Division, National Marine Fisheries Service, Southwest Fisheries Science Center, Santa Cruz, CA, United States
- 7California Sea Grant, Scripps Institution of Oceanography, University of California (UC) San Diego, La Jolla, CA, United States
- 8Farallon Institute, Petaluma, CA, United States
- 9Scripps Acoustic Ecology Lab, Scripps Institution of Oceanography, University of California, San Diego, CA, United States
- 10Pacific States Marine Fisheries Commission, Hatfield Marine Science Center, Newport, OR, United States
- 11Marine Physical Laboratory, Scripps Institution of Oceanography, University of California, San Diego, CA, United States
- 12Fish Ecology Division, National Marine Fisheries Service, Northwest Fisheries Science Center, Seattle, WA, United States
- 13Integrative Oceanography Division, Scripps Institution of Oceanography, University of California, San Diego, CA, United States
- 14Department of Fisheries, Wildlife, and Conservation Sciences, Oregon State University, Hatfield Marine Science Center, Newport, OR, United States
- 15U.S Geological Survey, Western Ecological Research Center, Santa Cruz Field Station, Santa Cruz, CA, United States
- 16Fish Ecology Division, National Marine Fisheries Service, Northwest Fisheries Science Center, Hatfield Marine Science Center, Newport, OR, United States
- 17Physical Sciences Laboratory, National Oceanic and Atmospheric Administration, Boulder, CO, United States
- 18Point Blue Conservation Science, Petaluma, CA, United States
- 19Ocean Sciences Department, University of California, Santa Cruz, CA, United States
- 20Marine Mammal Laboratory, National Marine Fisheries Service, Alaska Fisheries Science Center, Seattle, WA, United States
- 21Cooperative Institute for Marine Ecosystems and Climate, Humboldt State University, Arcata, CA, United States
- 22Climate, Atmospheric Science and Physical Oceanography Division, Scripps Institution of Oceanography, University of California, San Diego, CA, United States
In late 2020, models predicted that a strong La Niña would take place for the first time since 2013, and we assessed whether physical and biological indicators in 2021 were similar to past La Niñas in the California Current Ecosystem (CCE). The Pacific Decadal Oscillation and Oceanic Niño Index indeed remained negative throughout 2021; the North Pacific Gyre Oscillation Index, however, remained strongly negative. The seventh largest marine heatwave on record was unexpectedly present from April to the end of 2021; however, similar to past La Niñas, this mass of warm water mostly remained seaward of the continental shelf. As expected from past La Niñas, upwelling and chlorophyll were mostly high and sea surface temperature was low throughout the CCE; however, values were close to average south of Point Conception. Similar to past La Niñas, abundances of lipid-rich, northern copepods off Oregon increased. In northern California, unlike past La Niñas, the body size of North Pacific krill (Euphausia pacifica) was close to average. Predictably, overall krill abundance was above average in far northern California but, unexpectedly, below average south of Cape Mendocino. Off Oregon, similar to past La Niñas, larval abundances of three of six coastal species rose, while five of six southern/offshore taxa decreased in 2021. Off California, as expected based on 2020, Northern Anchovy (Engraulis mordax) were very abundant, while Pacific Sardine (Sardinops sagax) were low. Similar to past La Niñas, market squid (Doryteuthis opalescens) and young of the year (YOY) Pacific Hake (Merluccius pacificus), YOY sanddabs (Citharichthys spp.), and YOY rockfishes (Sebastes spp.) increased. Southern mesopelagic (e.g., Panama lightfish Vinciguerria lucetia, Mexican lampfish Triphoturus mexicanus) larvae decreased as expected but were still well above average, while northern mesopelagic (e.g., northern lampfish Stenobrachius leucopsarus) larvae increased but were still below average. In line with predictions, most monitored bird species had above-average reproduction in Oregon and California. California sea lion (Zalophus californianus) pup count, growth, and weight were high given the abundant Anchovy forage. The CCE entered an enduring La Niña in 2021, and assessing the responses of various ecosystem components helped articulate aspects of the system that are well understood and those that need further study.
Introduction
Eastern boundary upwelling systems (EBUS) are characterized by equatorward currents transporting cool, fresh, and oxygen- and nutrient-rich water along the west coast of a continent. The four major EBUS include the California Current off the west coast of North America, the Humboldt Current off western South America, the Iberian/Canary Current off northwest Africa, and the Benguela Current off southwest Africa (Garcia-Reyes et al., 2015). These ecosystems are extraordinarily productive when wind-driven upwelling infuses nutrients toward surface waters that fuel phytoplankton blooms. Indeed, although EBUS geographically comprise less than 1% of ocean waters, they can produce up to 20% of the global fishery catch via potentially hyperabundant coastal pelagic fishes such as anchovies and sardines (Pauly and Christensen, 1995). However, fishery production can vary by orders of magnitude annually, and thus, predicting the status of physical and biological components of EBUS is paramount for effective ecosystem-based management.
Off the west coast of North America, the California Current Ecosystem stretches from southern Vancouver Island, Canada, to Baja California Sur, Mexico. The CCE originates where the eastward-flowing North Pacific Current (NPC) abuts the west coast of North America at latitudes varying interannually between 42°N and 52°N (Sydeman et al., 2011). Upon hitting land, the NPC bifurcates into the northward-flowing Alaska Current and equatorward California Current. The California Current (CC) flows south into Baja California before veering westward at between 15°N and 25°N (Checkley and Barth, 2009). The CC transports relatively cool, fresh, nutrient-rich, and high-oxygen water from subarctic to subtropical locations (Sydeman et al., 2011). In addition to the CC water, the CCE comprises several distinct water masses. Near the coast, cold, saline, low-oxygen, and high-nutrient upwelled water fuels high primary production. Along the coastline, the California Undercurrent transports warm, saline, low-nutrient, and low-oxygen water poleward (Checkley and Barth, 2009). Seaward from the CC, Central Pacific water is also relatively warm, saline, and low in nutrients and oxygen (McClatchie, 2014). The CCE has been categorized into a northern region encompassing the northern extent of the CC to Cape Mendocino in northern California, a central section from Cape Mendocino to Point Conception, and a southern area from Point Conception to the southern extent of the CC (Checkley and Barth, 2009). The distribution of each major water mass is highly dynamic due in part to El Niño and La Niña oceanographic conditions, and biological characteristics often vary greatly between years (McClatchie, 2014).
The CCE is a particularly well-studied EBUS (Peña and Bograd, 2007). The California Cooperative Oceanic Fisheries Investigations (CalCOFI) program has systematically monitored the CCE since 1951 (McClatchie, 2014). The value of long-term observations, as demonstrated by CalCOFI, has motivated many additional ocean monitoring programs, including various annual surveys spanning large extents of the CCE (often with a stock-assessment motivation) and high-frequency coastal transects, such as the Newport Hydrographic Line and Trinidad Head Line that effectively resolve seasonal transitions (Gallo et al., 2022). The breadth of information about the CCE and other EBUS allows us to generate near-term hypotheses of how physical and biological components are expected to play out within EBUS based on basin-scale oceanographic predictions. The U.S. National Oceanic and Atmospheric Administration’s Climate Prediction Center regularly provides 6-month forecasts of whether the Pacific Ocean will experience La Niña, El Niño, or Niño-neutral conditions. Based on the forecast, it is then possible to hypothesize how specific ecosystem components will react in the upcoming year (Bond et al., 2008). Despite decades of observing relationships between physical and biological responses in the CCE, unexpected events [i.e., ecological surprises; sensu Filbee-Dexter et al. (2017)] still regularly occur. For example, although Northern Anchovy (Engraulis mordax; Anchovy) abundance increased under cool and Pacific Sardine (Sardinops sagax; Sardine) under warm ocean conditions in the 1900s, Anchovy increased greatly during the warmest conditions on record from 2014 to 2016, while Sardine remained low (Thompson et al., 2022). In addition, whereas high rockfish (Sebastes spp.) recruitment was typically associated with cool, La Niña conditions in central California (Ralston et al., 2015), young of the year pelagic rockfish abundances were at near-record highs during the 2014–2016 marine heatwave (MHW; Schroeder et al., 2019). By identifying when ecological responses do and, importantly, do not conform to expectations, we can come closer to a mechanistic understanding of the processes that drive species population dynamics.
Subsequent to 2014, the CCE was largely characterized by abnormally warm water. Although conditions were relatively cool in early 2014 and had been cool in the majority of the years from 1999 to 2013 (Thompson et al., 2017a), a mass of anomalously warm, relatively shallow water that originated in the Gulf of Alaska subsumed most of the CCE by mid-2014, leading to the largest marine heatwave (by area) on record by fall of 2014 (Leising et al., 2015). This warm “Blob” persisted into 2015 when more warm water that extended deeper into the water column entered the system via an El Niño (McClatchie et al., 2016b). El Niño conditions persisted into mid-2016 when yet another surface warming event formed in the Gulf of Alaska later in 2016. Collectively, the 2014–2016 Blob and El Niño produced the longest and largest continuous MHW on record in the CCE (Jacox et al., 2018). Although the CCE cooled off in 2017, the biological impacts of the 2014–2016 MHW lingered (Wells et al., 2017). Large MHWs formed again in 2018–2020; however, these tended to occur offshore and thus had a less biological impact on the CCE than the 2014–2016 MHW (Thompson et al., 2018; Thompson et al., 2019b; Weber et al., 2021). Moving into 2021, however, NOAA’s Climate Prediction Center projected that La Niña conditions would manifest in winter 2021 (cpc.ncep.noaa.gov/products/analysis_monitoring/enso_advisory/ensodisc.shtml).
Expectations for 2021
Given the likely onset of cooler conditions in 2021, past observations of the CCE during La Niñas (Hayward et al., 1999; Wells et al., 2013), and our knowledge of biological conditions in 2020 that may carry over to 2021 (e.g., Anchovy were very abundant; Weber et al., 2021), we generated a suite of expectations about physical and biological conditions at the basin to local spatial scales in 2021 (please see Table 1 for a reference to each prediction). At the basin scale, NOAA’s Climate Prediction Center predicted that water temperature would be anomalously cool at and around the equator and in the northeast Pacific Ocean, resulting in low Oceanic Niño Index (ONI) and Pacific Decadal Oscillation (PDO) values. When the ONI and PDO are low, upwelling is often high, and the North Pacific Gyre Oscillation (NPGO) has been highly correlated with upwelling strength (Di Lorenzo et al., 2008). As such, we expected that the NPGO would be high in 2021 after being almost exclusively low since 2014.
At the regional scales, we predicted that upwelling would be anomalously high throughout the CCE. Because upwelling infuses nutrients into the system, we expected that primary production (chlorophyll) would also be high. At the primary consumer trophic level, we hypothesized that the high primary production would induce an increase in northern, lipid-rich copepods and krill; increase body sizes of North Pacific krill (Euphausia pacifica); decrease southern, lipid-poor copepods/krill; and decrease pelagic invertebrate species richness as southern invertebrates are more specious than northern counterparts (Peterson et al., 2014).
At the next higher trophic level, we expected that northern zooplankton species would provide a robust forage base for yearling salmon that are leaving rivers and that yearling salmon abundance would be high in the northern CCE. By contrast, we expected that water temperature would be too cold for Pacific Pompano (Peprilus simillimus) and market squid (Doryteuthis opalescens) and that these species would decrease off Washington and Oregon (Thompson et al., 2019b). Off Newport, Oregon, we predicted that larvae of coastal taxa such as Pacific Sandlance (Ammodytes hexaptarus) would increase under cool conditions, while southern, offshore taxa such as Anchovy and Sardine (which were at record highs during the 2014–2016 MHW) would decrease (Auth et al., 2018). Off California, we hypothesized that young of the year (YOY) rockfishes, YOY sanddabs (Citharichthys sordidus plus C. stigmaeus), YOY Pacific Hake (Merluccius productus), and market squid would increase, while adult Sardine, adult Anchovy, and mesopelagic species (e.g., Myctophidae) would decrease (Ralston et al., 2015; Santora et al., 2021a; Santora et al., 2021b). Given that Anchovy recruitment was very high and Sardine recruitment was low in recent years (Thompson et al., 2019b), however, we expected that adult Anchovy would remain high in 2021 despite the cool conditions and that adult Sardine abundances would be low based on persistence from 2020. In southern California, among coastal pelagic species, we hypothesized that larval Sardine and Jack mackerel (Trachurus symmetricus) would decrease, while Pacific Hake and Anchovy would increase (Thompson et al., 2022). Pacific Mackerel (Scomber japonicus) do not display a clear response to warming/cooling, so we did not generate a hypothesis for this species. Among mesopelagic fishes, we expected those with southern distributions (e.g., Panama Lightfish, Vinciguerria lucetia) to decrease and those with northern distributions (e.g., Northern Lampfish, Stenobrachius leucopsarus) (Thompson et al., 2022) to increase. For common groundfishes, we expected sanddabs to decrease and rockfishes to increase (Thompson et al., 2022).
Among top predators, given the anticipated high forage associated with a La Niña, we predicted high seabird reproduction success (Wells et al., 2013; Santora et al., 2021b). In central California, we expected that at-sea abundances of a resident bird, common murre (Uria aalge) would be low because under conditions of high forage, the birds spend more time resting on the colony instead of searching for food at sea (Harding et al., 2007). By contrast, we expected the at-sea density of the cold-water migrant, sooty shearwater (Ardenna grisea) to be high in response to high prey availability. Off southern California, we predicted the at-sea abundance of a migratory bird associated with warm, sub-tropical waters, the black-vented sheerwater (Puffinus opisthomelas), to be low and the cool-water sooty shearwater to be high (Hyrenbach and Veit, 2003). In addition, we anticipated that the high abundance of forage would attract mobile marine mammals such as the humpback whales Megaptera novaeangliae and the Pacific white-sided dolphins Lagenorhynchus obliquidens. Finally, because sea lion Zalophus californianus pup condition is highly, positively correlated with the abundance of forage fishes such as Sardine and Anchovy (McClatchie et al., 2016a), and Anchovy were very abundant in 2020 (Weber et al., 2021), we expected pup numbers, weight, and growth rate to be high in 2021.
Methods
To evaluate the physical and biological characteristics of the CCE and surrounding regions, we compile data from various long-running surveys (Gallo et al., 2022). In 2021, data were available from much of the northern and central portions of the CCE. For the southern CCE, we had robust data from southern California but only limited information from Mexico. Although the southern CCE extends into Baja California, data from Mexico were unfortunately not available in 2021 [the State of the California Current Report typically obtains data from the Investigaciones Mexicanas de la Corriente de California (IMECOCAL) program, but the survey did not run in 2021].
Large-scale physical oceanography and chlorophyll a
At the basin scale, we evaluated three widely used indices: the PDO, ONI, and NPGO. The PDO is a function of sea surface temperature (SST) in the Pacific Ocean north of 20°N latitude. The ONI is also based on SST but from the equatorial Pacific 5°S–5°N, 120°–170°W. The NPGO is a climate pattern that emerges as the second dominant mode of sea surface height variability (second empirical orthogonal function of sea surface height) in the Northeast Pacific (25°–62°N, 180°–250°E) (Di Lorenzo et al., 2008). PDO, ONI, and NPGO values were accessed from the California Current Integrated Ecosystem Assessment website (https://oceanview.pfeg.noaa.gov/dashboard/).
To visualize the distribution of SST and wind strength anomalies relative to 1980–2020, we plotted satellite images throughout the Pacific Ocean in fall 2020 and winter, spring, and summer 2021. We obtained wind data from the NCEP/NCAR Reanalysis and the NOAA Extended Reconstructed SST V5 data from http://www.esrl.noaa.gov.
Next, we created image plots of satellite-derived SST averaged from the coast to 75 km offshore throughout the entire potential geographic extent of the CCE [Baja California Sur, Mexico (24°N) to British Columbia, Canada (51°N)] from January 2017 to September 2021. SST data were downloaded from https://coastwatch.pfeg.noaa.gov/erddap/griddap/ncdcOisst2Agg. In addition, we constructed image plots of the Bakun upwelling index over the same period of time and spatial extent (https://oceanview.pfeg.noaa.gov/erddap/tabledap/). Finally, we plotted satellite images onto a 0.1° × 0.1° grid of spring (March–May) chlorophyll a anomalies from 2019–2021 relative to 2003–2021. We obtained chlorophyll a data from https://coastwatch.pfeg.noaa.gov/erddap/griddap/erdMH1chlamday.
Regional physical oceanography and chlorophyll a
Data collected at the local scales are from marine monitoring surveys described in Gallo et al. (2022) and the previous State of the California Current Reports (e.g., Weber et al., 2021). We present data that are commonly collected in multiple surveys. For example, temperature, salinity, and chlorophyll a are sampled with conductivity temperature depth (CTD) instruments in most modern marine surveys. However, additional physical information (e.g., nutrients) can be specific to a given survey, and we relegate this and other information to the supplemental appendix. More details about each survey (e.g., make of CTD instruments, mesh size of nets) can be found in accompanying references. For all subsequently described surveys, we present the time series of seasonal or monthly anomalies relative to long-term means (see figure captions for the duration over which means were calculated). The locations of all surveys are shown in Figure 1.
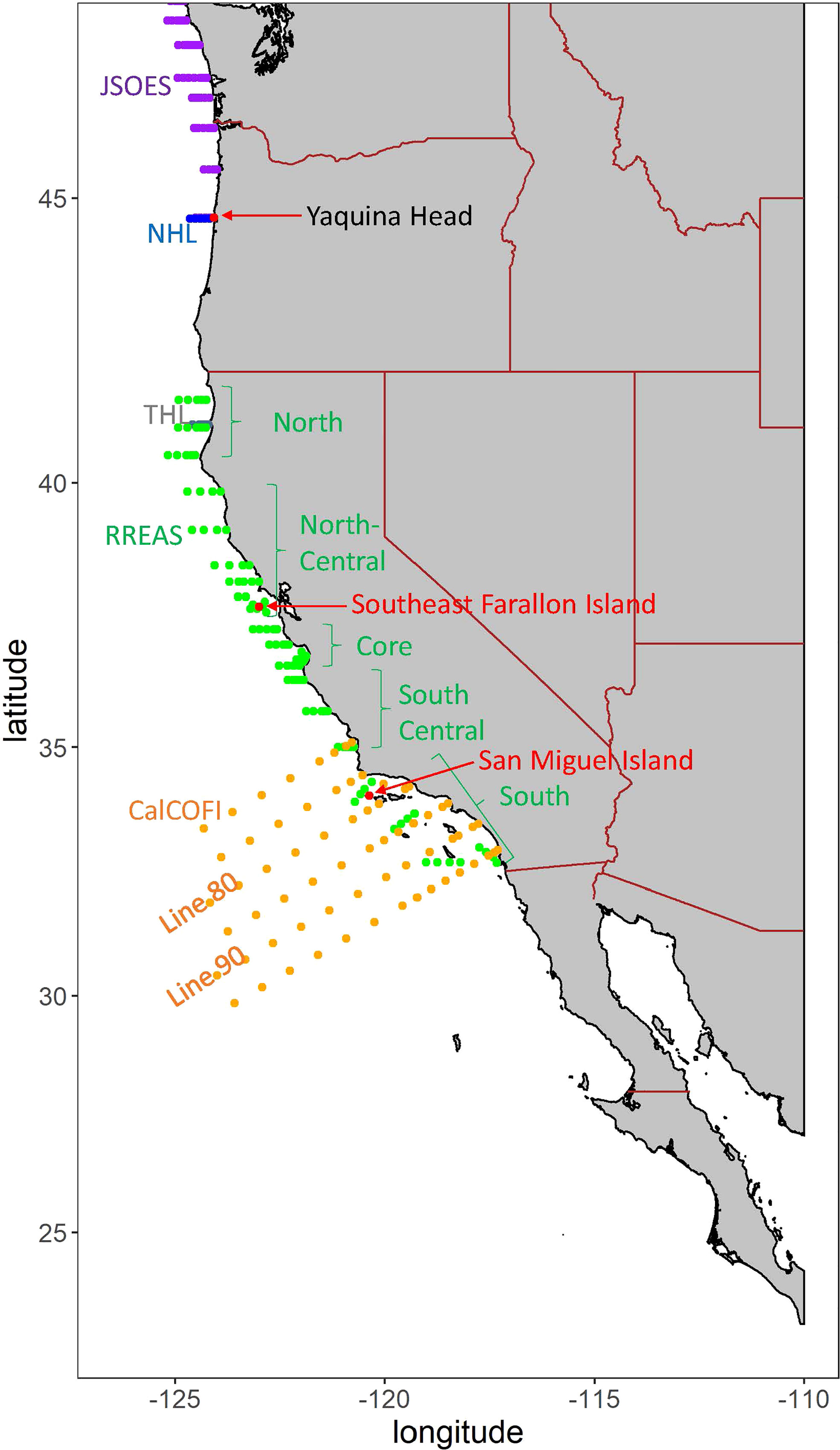
Figure 1 Locations of the surveys. Land-based locations are in red. The Juvenile Salmon and Ocean Ecosystem Surveys (JSOES) is in purple, the Newport Hydrographic Line (NHL) in blue, the Trinidad Head Line (THL) in gray, the Rockfish Recruitment and Ecosystem Assessment Survey (RREAS) in green, and the California Cooperative Oceanic Fisheries Investigation (CalCOFI) in orange.
We sampled temperature and salinity at a depth of 50 m at the Newport Hydrological Line (NHL) station NHL05 and at 150 m at NHL25. In addition, chlorophyll a concentration was measured from surface water collected at NHL05 (Auth et al., 2011). Along the Trinidad Head Line, temperature and salinity were recorded at 15 and 65 m, and chlorophyll a was integrated from 2 to 30 m at station TH02 (Robertson and Bjorkstedt, 2020). Off southern California, salinity, temperature, and chlorophyll a were measured and averaged within the mixed layer at each of the 66 core CalCOFI stations (Scripps Institution of Oceanography (SIO), 2012).
Regional zooplankton
Zooplankton were collected at NHL05 using a vertically hauled ring net from 2 m off the bottom (60 m) to the sea surface. We then quantified the abundances of northern and southern copepods and copepod species richness (Fisher et al., 2015). Off the Trinidad Head Line (THL), zooplankton were sampled with an obliquely towed Bongo net deployed to 100 m at TH04 and TH05 (the two most offshore stations), and the body sizes of E. pacifica were measured in the laboratory (Robertson and Bjorkstedt, 2020). Krill, which are dominated by the combined sums of E. pacifica and Thysanoessa spinifera were collected throughout California by the Rockfish Recruitment and Ecosystem Analysis Survey from transects within five geographic regions (north, north central, core, south central, south) using a midwater (30 m) trawl that was towed for 15 min (Sakuma et al., 2016). A subsample of krill was counted at sea and total abundance was extrapolated to the total volume of krill.
Regional fish and squid
We examined data from multiple surveys that target various life stages of fishes and market squid in the CCE. Furthest north, the Juvenile Salmon and Ocean Ecosystem Survey (JSOES) conducted trawl surveys in the upper 18 m during daytime with the primary purpose of monitoring yearling salmon (Thompson et al., 2019a). In addition to salmon, JSOES quantified species that reside close to the surface during the day such as the Pacific Pompano and market squid. Off the NHL, bongo samples were collected weekly (weather permitting) from January to March on NHL stations 5 to 25, and we evaluated the changes in the density of common southern and coastal fish larvae (Auth et al., 2018). Further south, the Rockfish Recruitment and Ecosystem Assessment Survey (RREAS) has conducted 15-min-long midwater trawls at a depth of 30 m from fixed transects in a region centered on Monterey Bay since 1983 and throughout all of California since 2004 (Sakuma et al., 2006). Depending on the species, we created time series for common young of the year or adult fishes and market squid. Finally, we examined common mesopelagic, coastal pelagic, and groundfish larval fish from spring CalCOFI oblique net tows from 1951 to the present (Thompson et al., 2017b).
Regional top predators
We monitored seabird colonies at two locations, Yaquina Head, Oregon (Porquez et al., 2021), and Southeast Farallon Island (SEFI) (Warzybok et al., 2018), California, from May through August. At both colonies, the reproductive success of common murre (U. aalge) was quantified via plot-based monitoring. Additionally, the common murre diet was measured by observing prey items brought to chicks. At SEFI, annual seabird monitoring for most species has been conducted continuously since 1971 (Warzybok et al., 2018). The time series at Yaquina Head was initiated in 1998; however, the colony has been systematically monitored annually since 2007 (Porquez et al., 2021). At Yaquina Head, we also recorded disturbances to murres by bald eagles (Haliaeetus leucocephalus).
Seabirds have also been quantified at sea through visual observation during daylight hours from central California by the Farallon Institute in cooperation with the RREAS (1996–2021) and summer CalCOFI cruises (1987–2021) (Hyrenbach and Veit, 2003; Santora et al., 2017; Santora et al., 2021b). From the RREAS, we report at-sea densities of resident common murres and migrant, sooty shearwaters. In southern California, we present densities of warm-water, migratory black-vented sheerwater and cool-water, migratory sooty shearwater. In addition, RREAS records marine mammal abundances, and we present the time series of humpback whale and Pacific white-sided dolphin sightings.
Finally, California sea lion reproduction has been visually monitored on San Miguel Island since 1997 (Melin et al., 2010). Here, we report the number of pups born, pup growth rate, and pup weight.
Results
Large-scale physical oceanography and chlorophyll a
As predicted in late 2020, the ONI and PDO remained negative until 2021 (Table 1; Figure 2A). The NPGO, however, did not adhere to previous La Niña conditions (Figure 2A). Whereas the NPGO tended to be positive during La Niñas over the past four decades, it was strongly negative in 2014–2020 and remained negative throughout 2021.
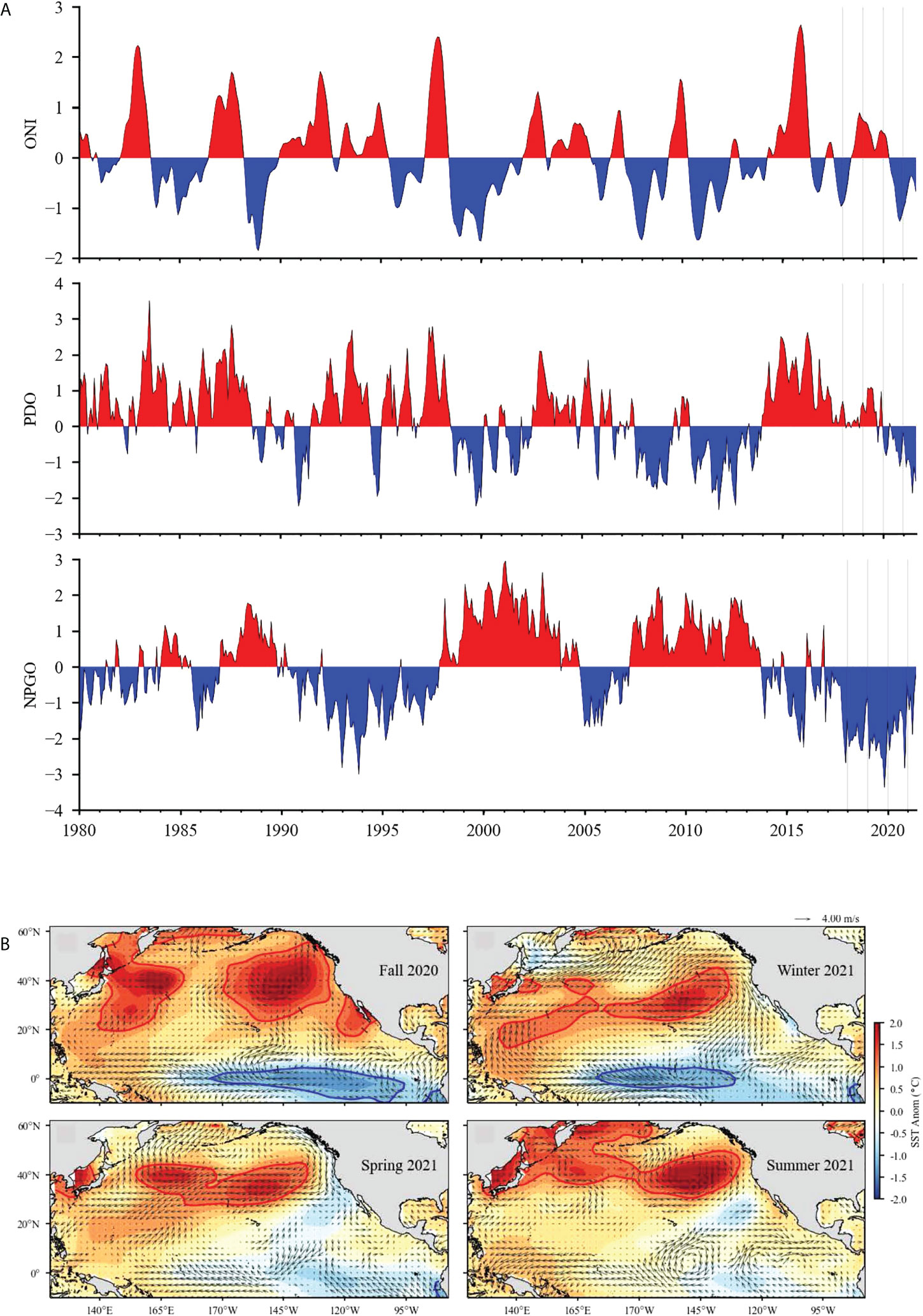
Figure 2 (A) Time series of monthly values for three ocean indices especially relevant to the California Current: Oceanic Niño Index (ONI), Pacific Decadal Oscillation (PDO), and North Pacific Gyre Oscillation (NPGO). Vertical lines mark January 2017–2021. (B) Surface wind velocity and sea surface temperature anomalies in the North Pacific Ocean for fall (September–November) 2020, winter (December–February) 2021, spring (March–May) 2021, and summer (June–August) 2021. Arrows denote the magnitude and direction of wind anomaly (scale arrow at the top). Contours denote temperature anomaly. Shading interval is 0.25°C and contour intervals at ±1°C are shown in red and blue, respectively.
At a basin scale, SST reflected La Niña conditions as the temperature was highly negatively anomalous near the equator in late 2020 and early 2021 (Figure 2B). Although the equatorial SST anomaly increased somewhat in spring and summer 2021, it was still mainly negative. By contrast, anomalously warm surface water was consistently persistent in the north Pacific from late 2020 throughout 2021, and the threshold for marine heatwave categorization was exceeded during almost the entire period (https://www.integratedecosystemassessment.noaa.gov/regions/california-current). In late 2020, the MHW conditions reached the shoreline in the southern CCE (Figure 2B). In 2021, MHWs transitioned to the northwest and were offshore from the U.S. Exclusive Economic Zone (370 km from shore) (Figure 2B).
Entering 2021, we predicted that low SST, high upwelling, and high chlorophyll a would occur throughout the CCE (Table 1). This expectation was largely met as SST was consistently below average and upwelling was above average within 75 km of shore north of Point Conception (approximately 34°N) (Figure 3A). South of Point Conception, however, SST was mainly above average and upwelling only average to slightly above average in 2021. Spring chlorophyll a anomalies largely followed upwelling and were extremely high north of Monterey Bay in spring 2021 (Figure 3B). Around Point Conception, chlorophyll a was mostly below average, and within southern California, it was largely close to average although it was below average in the Santa Barbara basin and above average off Ventura, California (Figure 3C).
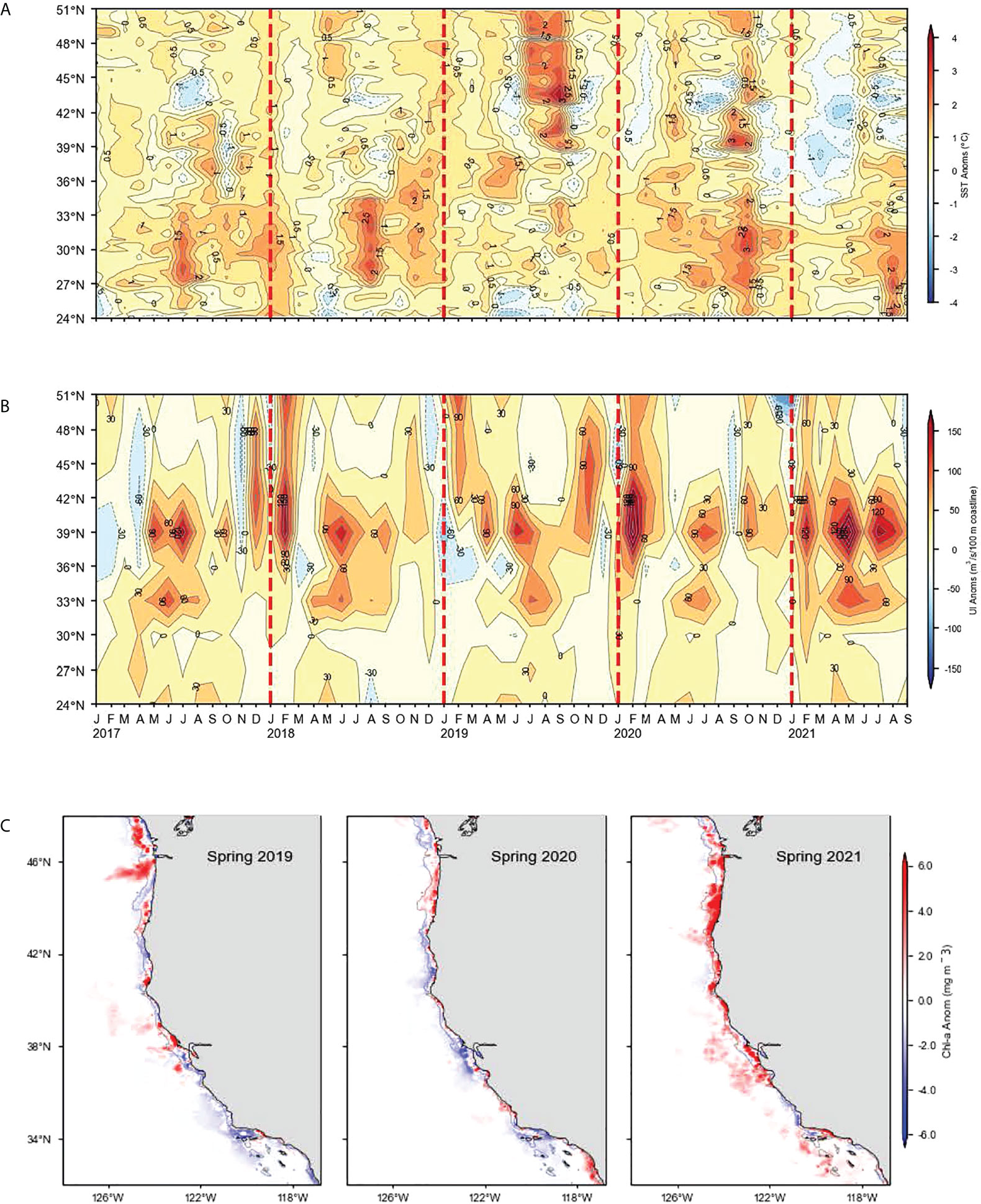
Figure 3 (A) Monthly sea surface temperature anomalies averaged from the coast to 75 km offshore. (B) Bakun upwelling index (UI) anomalies. (C) Spring (March–May) chlorophyll a anomalies averaged onto a 0.1° × 0.1° grid.
Regional physical oceanography and chlorophyll a
At regional scales, environmental conditions mostly met the expectation (Table 1) of low temperature, high salinity, and high chlorophyll a. Off Newport, Oregon, the temperature at 50 m was well below normal and salinity was well above normal for most of the year and only reverted to average in October (Figure 4A). This upwelled water apparently created optimal conditions for primary production as chlorophyll a rose from normal values in February to the highest values ever recorded by September 2021. Temperature and salinity at 150 m generally followed the same patterns as at 50 m, but the variability was much more subdued at depth. On the THL, sampling was not conducted between December 2020 and May 2021 due to coronavirus disease (COVID), and we thus missed recording conditions during the spring when upwelling is typically the strongest (Figure 4B). By mid-2021, the temperature was close to average and was within approximately 1°C for the remainder of 2021 (Figure 4B; note that the data here go through February 2022). Salinity fluctuated more than the temperature in 2021 and was below average in June 2021 and then above average in fall. Fluorescence was near average in mid-2021. In southern California, the temperature in the mixed layer was slightly below average in winter and then very close to average in spring and summer of 2021 (Figure 4C). Salinity was essentially average in winter and summer and slightly above average in spring. The average chlorophyll a was above the long-term, seasonally adjusted mean in winter, spring, and summer of 2021.
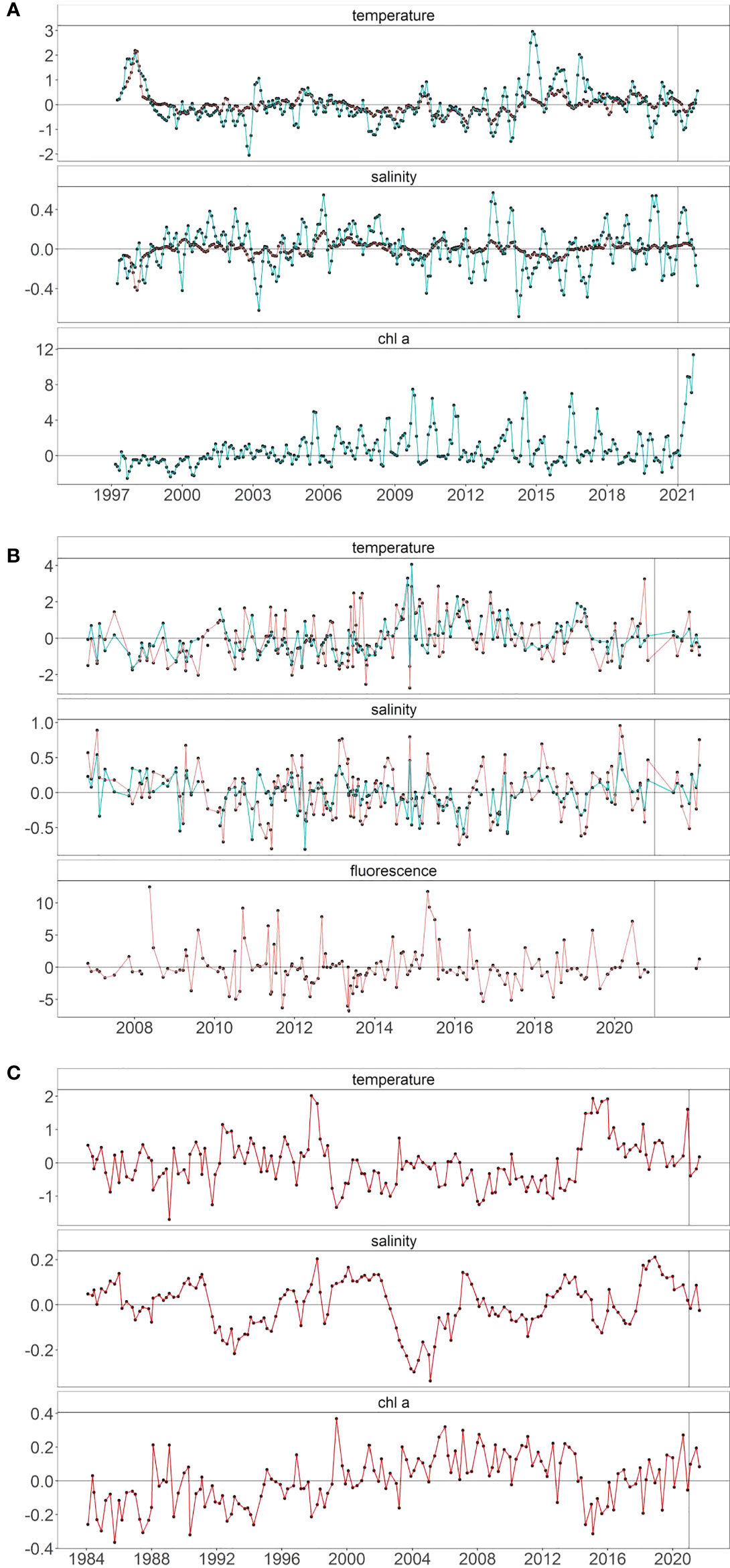
Figure 4 Seasonally adjusted temperature, salinity, and chlorophyll a anomalies at (A) Newport Hydrographic stations NHL5 (50 m, blue line) and NHL25 (150 m, red line), (B) Trinidad Head Line station 2 at 15 m (blue line) and 65 m (red line) with chlorophyll values integrated from 2 to 30 m, and (C) within the mixed layer averaged across the 66 core CalCOFI stations.
Regional zooplankton
Similar to past La Niñas (Table 1), the biomass anomalies of northern copepods on the NHL in the beginning of 2021 were the highest, and southern species the lowest, since the onset of the 2014–2016 MHW (Figure 5A). By the end of 2021, however, both northern and southern species were closer to average relative to the 25-year time series. Copepod species richness tracked the copepod biomass anomalies, with species richness being low through most of 2021, reflecting a community with cold-water affinities, returning to average, and in late 2021, when the biomass anomalies were also closer to neutral. In northern California, however, the body sizes of the dominant krill species, E. pacifica, collected on the TH line were average in mid-2021 (Figure 5B). Total krill abundance (largely driven by E. pacifica) from RREAS surveys was above average only in the northern region, north of Cape Mendocino (Figure 5C). South of Cape Mendocino, total krill abundance was below average in all regions (Figure 5C).
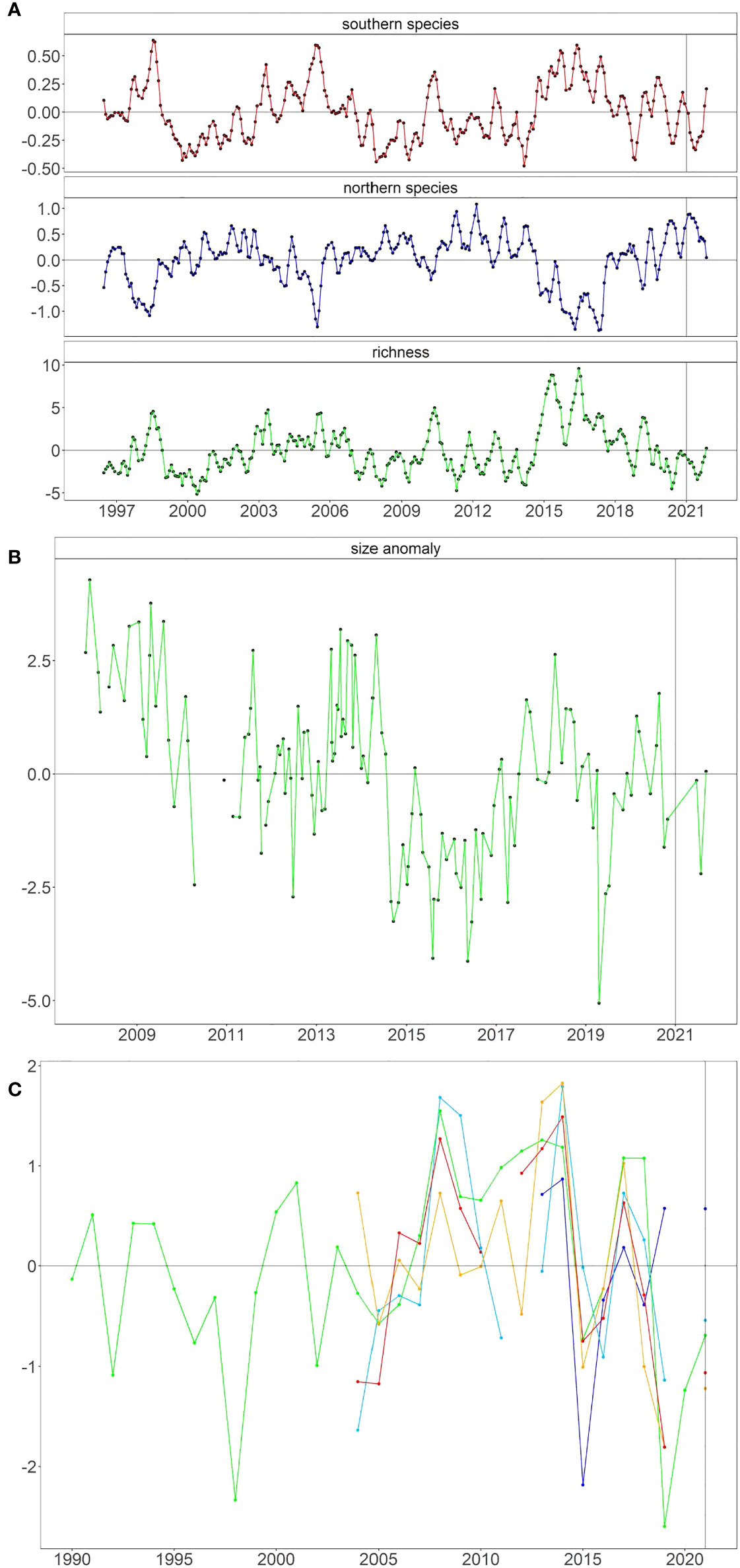
Figure 5 Seasonally adjusted anomalies of planktonic invertebrates from (A) Newport Hydrographic Line off Oregon, (B) Euphausia pacifica from Trinidad Head Line off northern California, and (C) total abundance of krill from the Rockfish Recruitment and Ecosystem Assessment Survey off various parts of California.
Regional fish and squid
As expected (Table 1), Pacific Pompano and market squid decreased off Oregon and Washington in 2021 (Figure 6A). In addition, as predicted, both Coho and Chinook Salmon rose to near-average abundances. Predictions were mostly met at NHL as four of the five species classified as offshore or southern declined to below average in 2021, although rockfishes continued to be very abundant (Figure 6B). The response of coastal species was mixed as three of the six species were above average (Figure 6B). Off California, predictions of species abundance patterns from RREAS midwater trawls were mostly met. Market squid increased in all regions but were still below average in the north central and south central regions (Figure 6C). Similarly, myctophids decreased throughout California and were below average in all regions. In addition, as expected, adult Anchovy were very high throughout California and were at record levels in the north central, south central, and south. Adult Sardine abundance, as expected, was also low throughout most of California. As predicted, YOY Hake and rockfishes increased uniformly in all regions. Regional changes of YOY sanddabs were mixed as abundances increased in the north and were low in southern California. YOY Anchovy were below average in all RREAS regions except southern California. YOY Sardine were mixed with very low abundances from Monterey Bay south into southern California but above average in the northern parts of California. In southern California, the dynamics of larval abundances in 2021 were mixed relative to our simple predictions (Figure 6D). Among species with pelagic adult habitats, as expected, market squid increased (to average levels), Sardine remained low, and Jack Mackerel decreased to very low abundances. Hake, which typically increase under cooler conditions, however, remained low. Pacific Mackerel, which had not shown clear relationships with warming or cooling in the past did not change much in 2021 and were close to average abundance. Anchovy larval abundance reflected elevated adult abundance and was the highest since the 1960s. Northern mesopelagics increased relative to recent years but were still well below-average levels, while southern mesopelagics displayed exactly the opposite patterns. Groundfishes adhered to predictions as sanddabs were low and rockfishes high in 2021.
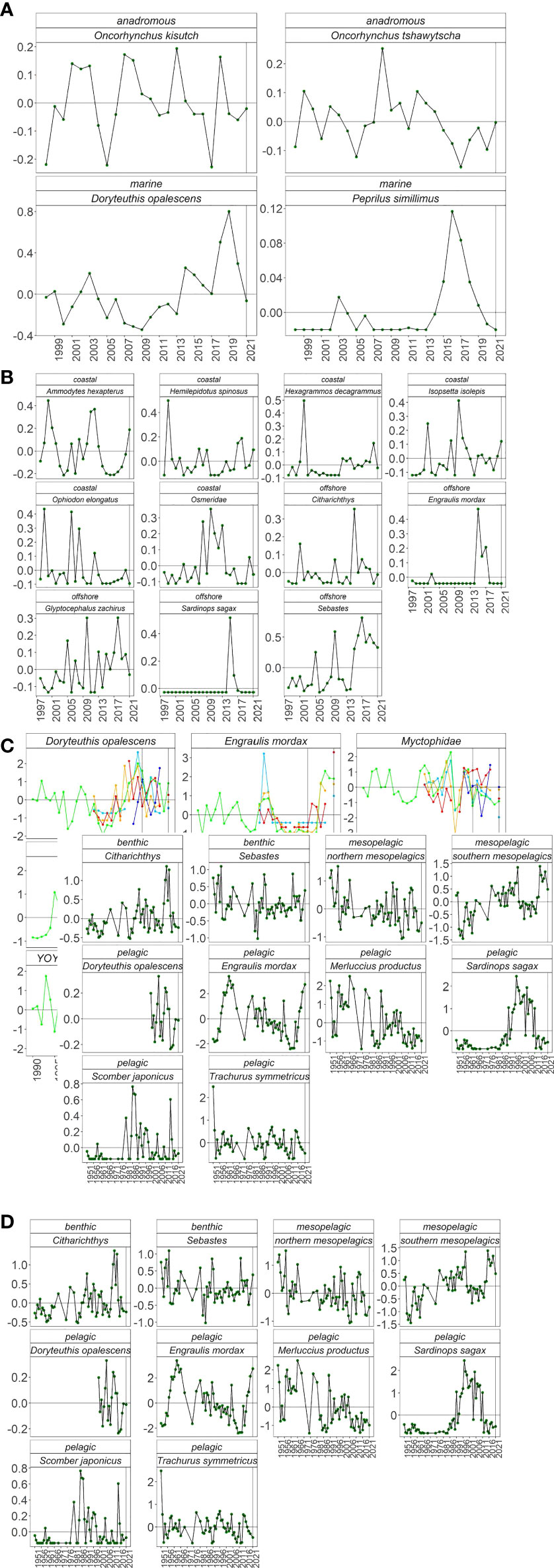
Figure 6 Anomalies of fish abundance from (A) JSOES, (B) NHL, (C) RREAS, and (D) CalCOFI. Panels within surveys depict different fishes. For RREAS, colors depict the survey region as in Figure 1: dark blue is north, light blue is north central, green is core, orange is south central, and red is south.
Regional top predators
As predicted (Table 1), the reproductive output of Brandt’s and pelagic cormorants were high in 2021, and common murre reproductive success was average at the coastal colony located at Yaquina Head (Figure 7A). Reproduction by common murre, which fed on a mix of sand lance, Clupeid fishes, and herring (Figure 7C), was likely tempered by eagle disturbances, as there was a strong, negative relationship between rates of eagle disturbance and murre reproduction (Figure 7B, adjusted R2 = 0.56) at Yaquina Head, and the disturbance level was approximately average in 2021. On Southeast Farallon Island (an offshore colony where eagle disturbance is not an issue), seabird reproductive success was above average with the exception of common murre (Figure 7D). Although murre productivity increased relative to the past 2 years, reproduction was still slightly below the long-term average. The common murre diet was similar to 2020 and consisted mostly of Anchovy followed by YOY rockfishes (Figure 7E).
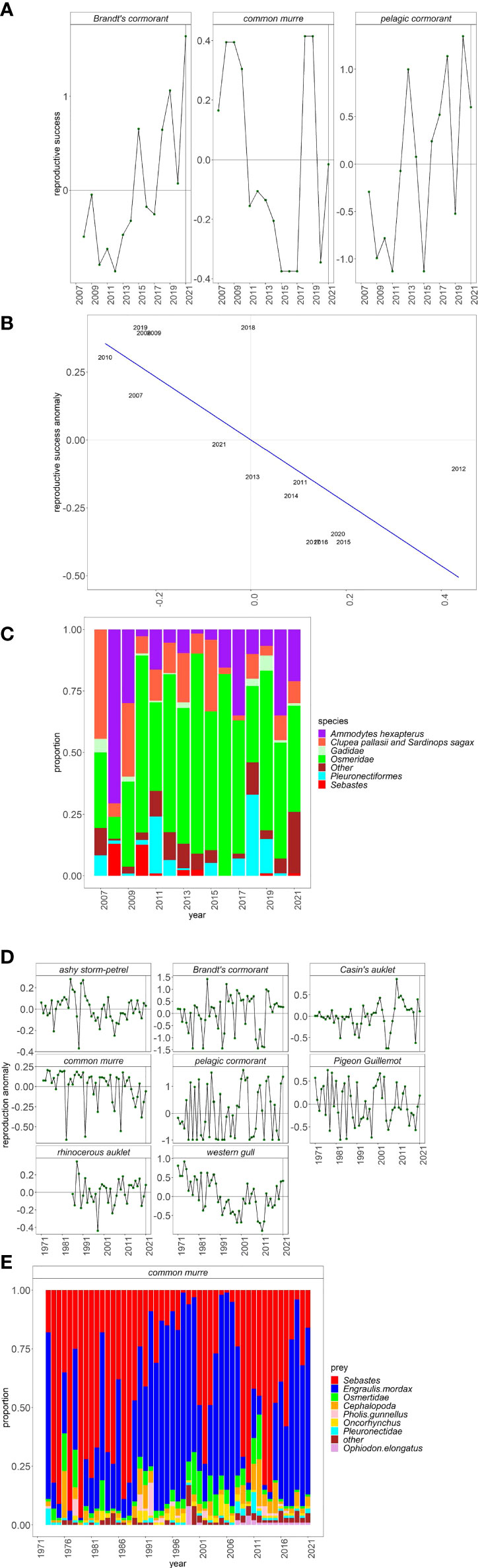
Figure 7 (A) Anomalies of seabird reproduction through time, (B) relationship between common murre reproduction and eagle disturbance (reproduction anomaly ~ −1.16 * disturbance anomaly + 2.561e−10; adjusted R2 = 0.56, p < 0.001), and (C) common murre diet through time at Yaquina Head and (D) seabird reproduction and (E) common murre diet at Southeast Farallon Island.
Hypothesized changes of seabirds at sea (Table 1) did not fully meet the expectations in central California as the density of both migrant sooty sheerwater and resident common murre was high in 2021 (Figure 8A). Similarly, predictions were only partially realized for the migrant bird in southern California, as cool-water sooty sheerwater was low (Figure 8B). Warm-water black-vented sheerwater decreased relative to 2020 and recent warm years (2005–2019), but it was still above the long-term mean, as predicted.
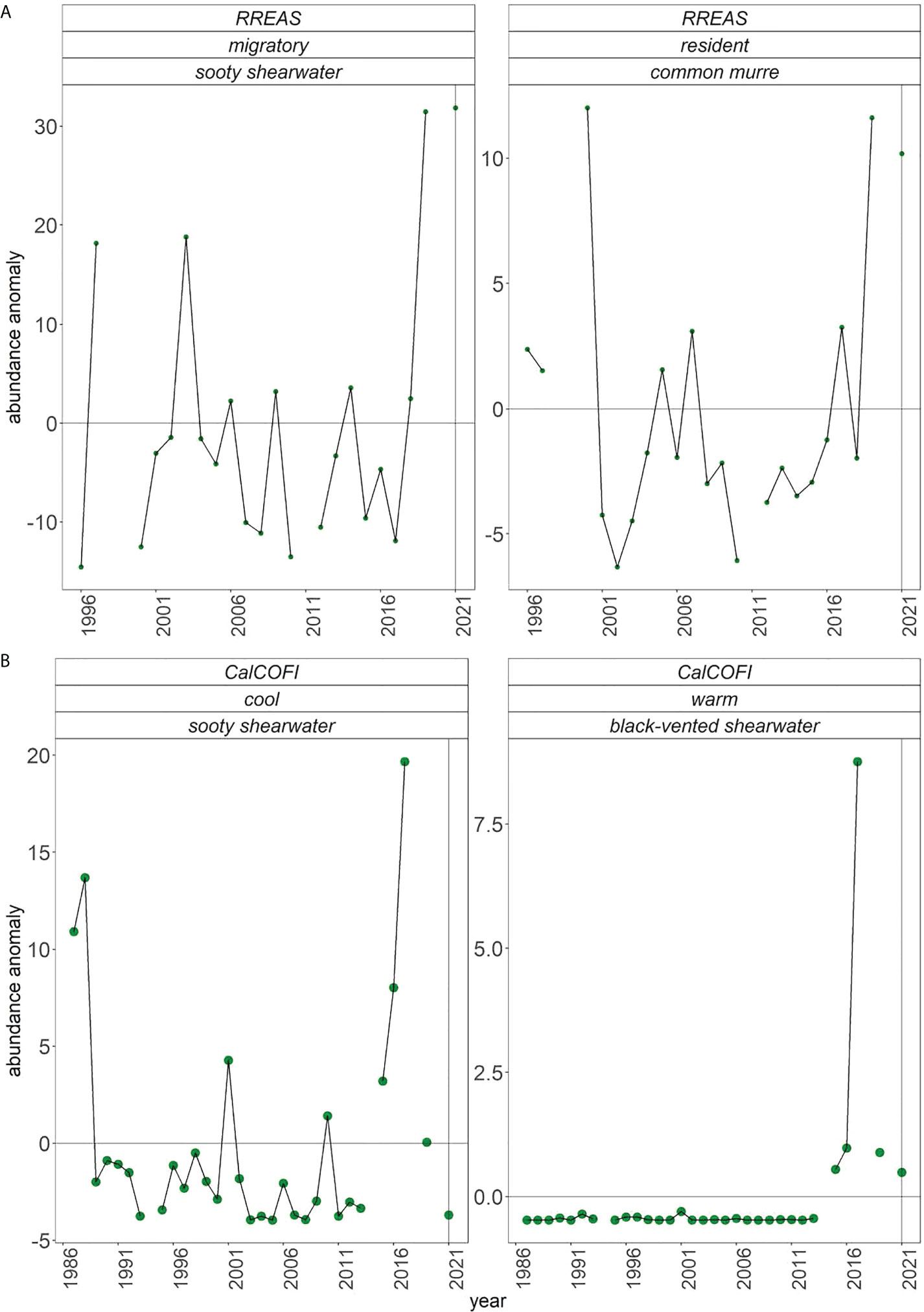
Figure 8 (A) Anomalies of at-sea sightings of migratory and resident sea birds from RREAS and (B) cool- and warm-water birds from summer CalCOFI.
We predicted that marine mammal sightings would increase (Table 1). However, within central California, sightings of humpback whales and Pacific white-sided dolphins decreased in 2021 (Figure 9A).
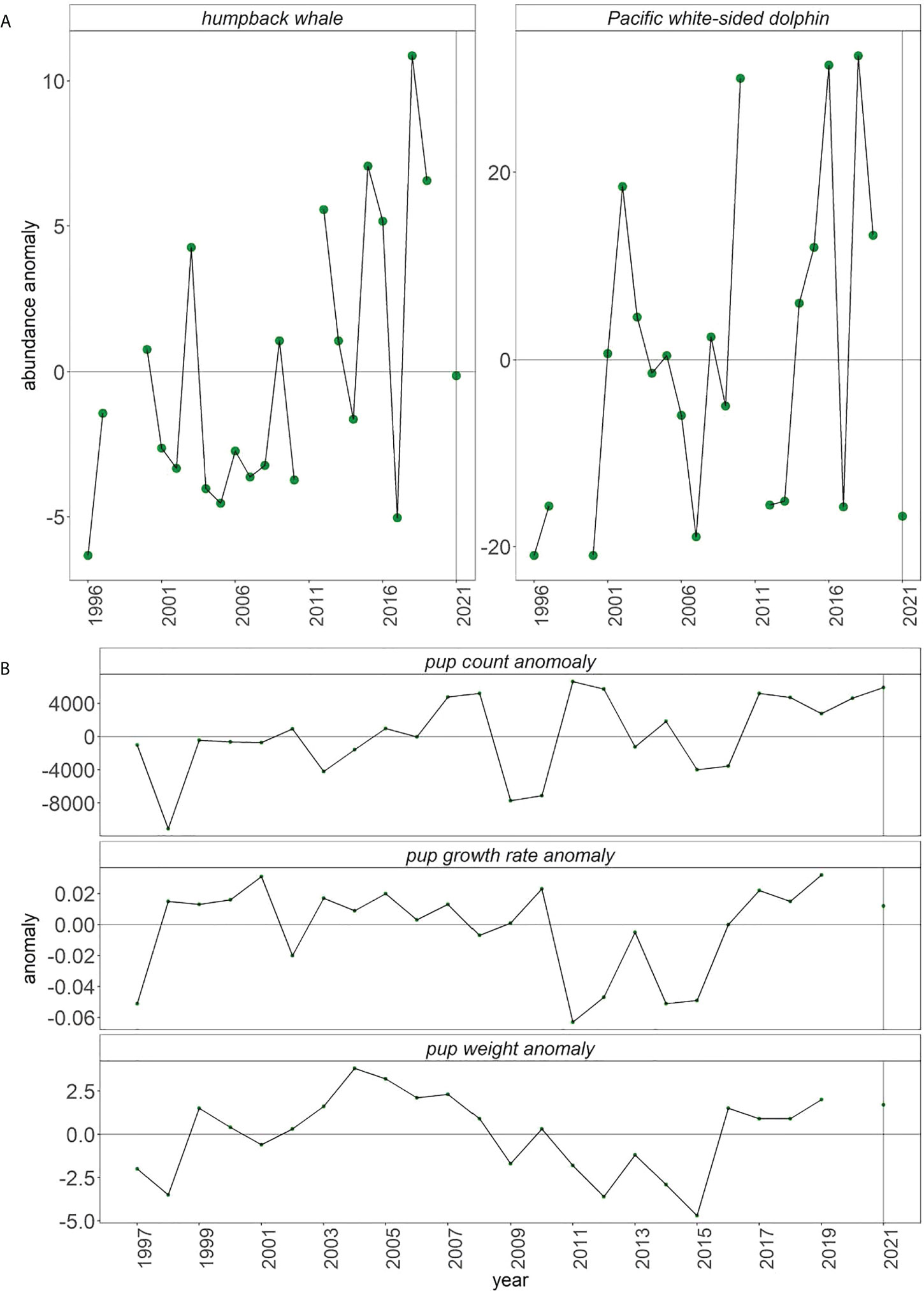
Figure 9 (A) Anomalies of sighting of marine mammals from RREAS and (B) sea lion pup count, pup weight, and pup growth anomalies from San Miguel Island (bottom).
Our expectation that sea lion pup indices would be high was fully realized (Figure 9B). Pup count and growth rate anomaly was above average for the fifth consecutive year. Pup weight anomaly was above average for the sixth consecutive year.
Discussion
The overarching objective of this paper was to evaluate whether physical and biological conditions in the California Current Ecosystem were similar to past La Niñas. As forecasted by NOAA in late 2020, a strong La Niña formed in early 2021 and wound up persisting through the entire year. While many of the measured parameters adhered to predictions based on previous observations of La Niñas, we did encounter several ecological surprises. Here, we discuss the ecological implications of major events that took place in 2021 and why they did or did not differ from a priori predictions.
Another marine heatwave
Although we did not make an explicit prediction about MHWs going into 2021, it is important to state that a large MHW formed offshore of the U.S. Exclusive Economic Zone (200 nm). Although it is not particularly rare for there to be a mass of offshore warm water during a La Niña (Wells et al., 2013), the 2021 MHW was unusual as it was very long (began in April and lasted into December; >230 days long) and large (~4.5M km2). Ultimately, it turned out to be the seventh largest heatwave in this region on record since 1982. In addition, it was closer to shore than most warm-water masses during La Niñas (e.g., Wells et al., 2013) although cool, productive waters near shore mostly kept it from affecting coastal regions. However, the 2021 MHW did briefly reach the central CA coast during a relatively short period of upwelling relaxation in mid-June. Overall, MHWs have been consistently present in the north Pacific for 7 of the past 8 years (Thompson et al., 2019b; Weber et al., 2021). Moving into the future, when MHW conditions are predicted to become more prevalent (Jacox et al., 2022), it will be interesting to see if MHWs impact coastal regions even in La Niña years.
Basin-scale indicators
At a basin scale, physical conditions that characterize negative PDO and negative ONI largely aligned with a typical La Niña, as it was cool near the equator and in the northeast Pacific along the coast, with warmer waters in the central NE Pacific/Gulf of Alaska. The NPGO, however, was negative, which is atypical during La Niñas over the past 40 years (e.g., Wells et al., 2013). Indeed, the NPGO and PDO were both negative in all months of 2021 for the first time since at least 1990. The NPGO is typically high when the flow of the California Current and the upwelling are strong, and has major implications for biological production in the central and southern CCE (Sydeman et al., 2011; Schroeder et al., 2019). A decoupling between basin and regional physical conditions began in 1989, and the degree of differentiation is increasing under climate change (Litzow et al., 2020). As such, data from 2021 further show that attempting to correlate large-scale indices such as the NPGO against biological dynamics at regional scales may be problematic. Further investigation is warranted to determine why the NPGO has been consistently negative since 2014 despite widely variable physical and biological conditions in the CCE.
Invertebrates
Northern copepods, which are lipid rich in comparison to their southern counterparts, were very abundant off Oregon in 2021. This finding is fully in line with expectations as copepod communities in the CCE tend to be comprised of southern species under warm, low-nutrient, and low phytoplankton conditions and northern species when it is cool and nutrients and phytoplankton are abundant (Du and Peterson, 2018). The high biomass of northern copepods in 2021 has important ecosystem implications as it indicates favorable feeding conditions and increased survival potential of juvenile salmon first entering the ocean relative to recent years (Peterson et al., 2014). Off California, however, the response of krill to cool, productive conditions was mostly unexpected. Euphausiid abundance was below average in all regions off California except the far north, and euphausiid body size in the north off Trinidad Head was average or below average. In recent years, diminished euphausiid abundance and size were attributed primarily to low primary productivity (Robertson and Bjorkstedt, 2020). In 2021, however, some unknown factor must have driven down euphausiids. A possible explanation is that the extraordinarily high Anchovy abundance south of Cape Mendocino exerted top-down control and significantly culled euphausiid size and abundance. Although the impact of grazers on planktonic communities has been recognized in the CCE (Suchman et al., 2008), the broad role of top-down control on secondary productivity in the CCE is poorly understood and needs further study.
Fishes and market squid
For the most part, as observed in the past during La Niñas, fishes associated with cool water increased, while those with warm water decreased throughout the CCE. Off Oregon, coastal fishes such as Pacific Sand Lance are important prey for salmon, and the biomass of coastal taxa [Index of Coastal Prey Biomass (ICPB)] is utilized as an index of prey availability and quality for salmon migrating from rivers in late spring and early summer (Daly et al., 2021). In 2021, the ICPB was the sixth highest since the survey began in 1998 and the highest since 2012. Hence, indices based on both zooplankton and ichthyoplankton suggest above-average forage conditions for salmon in the northern CCE in 2021.
The response of Anchovy and Sardine to warming/cooling in the CCE is very different now than it was in the 1900s. Throughout the twentieth century, Sardine tended to thrive when it was warm, while Anchovy abundance rose when it was cold (Chavez et al., 2003). This facile paradigm began to fail in the twenty-first century, as Anchovy did not appreciably increase during the mostly cool years from 1999 to 2014 (Thompson et al., 2017a; Sydeman et al., 2020). Over the same period, Sardine also began to decline, and by 2013, the abundance of both Sardine and Anchovy was very low (Thompson et al., 2018). In mid-2014, the CCE abruptly warmed as the Pacific Marine Heatwave spread south from the Gulf of Alaska (Jacox et al., 2018). Unexpectedly, this marked the beginning of prolonged, strong Anchovy recruitment as young of the year abundance was very high within and around Monterey Bay in 2014 and then throughout most of California in 2015 (Thompson et al., 2019b). Subsequent strong recruitment classes augmented the adult Anchovy population from 2016 to the present, and in 2021, adult Anchovy abundance was the highest since at least the 1960s. Meanwhile, despite the MHW and subsequent warm events (Weber et al., 2021), Sardine recruitment and adult population size were consistently low through 2021. The surprising response of Anchovy and Sardine to warming in the twenty-first century stresses the need to resolve mechanistic forces impacting the recruitment of coastal pelagic species in the CCE (Checkley et al., 2017).
Market squid responded predictably to the 2021 La Niña as abundance increased throughout most of the surveyed areas. These cephalopods are highly affected by SST as growth rates of paralarvae are strongly, negatively correlated with water temperatures between 14°C and 24°C (Reiss et al., 2004). During the 2014–2016 MHW, market squid distribution shifted markedly to the north (Chasco et al., 2022), and large numbers turned up as far north as the Gulf of Alaska (Burford et al., 2022). Market squid by volume is the most valuable fishery off the west coast of the United States, and the northern shift significantly decreased overall fishing revenue in California from 2014 to 2019. In 2021, however, market squid decreased to below-average abundances off Oregon but increased throughout California and was above average off Monterrey and southern California. These trends may induce a boost in market squid catch in California in 2021 and upcoming years.
Rockfishes are also commercially and ecologically valuable in the CCE (Love et al., 2002), and the abundance of both larval and young of the year rockfishes mostly increased or was high in 2021 throughout the CCE. Larval rockfish abundance is driven by the number and age of adult females and environmental conditions. Larval abundances will be high if adults are abundant, females are older, and females are well fed (Hixon et al., 2013). It appears that all of these conditions were met in 2021 as rockfish age and biomass increased throughout the 2000s as a result of strong year classes and favorable environmental conditions (Field et al., 2010; Thompson et al., 2017a), and productive environmental conditions in 2021 likely produced ample food for females. The rates of larval survival determine the young of the year rockfish abundance, but overall larval abundance is typically not related to recruitment strength (Cury et al., 2014). However, Schroeder et al. (2019) found that rockfish recruitment was high when parents were exposed to Pacific subarctic (i.e., California Current) water in the months preceding sampling of pelagic juvenile rockfish. The volume of Pacific subarctic water tends to be higher in the CCE during La Niñas, and it is likely that optimal physical conditions facilitated high rockfish recruitment in 2021.
Birds
The reproductive success of resident-breeder seabirds tends to increase during La Niñas (Santora et al., 2021b) and was predictably high in 2021. The availability of appropriate forage is a main driver of seabird reproduction, and the capacity of a bird to thrive on a particular prey item can be species-specific (Santora et al., 2014). In 2021, our data showed that many species of potential bird prey such as the young of the year rockfishes and Hake were abundant off Southeastern Farallon Island, and this robust prey base likely augmented shore bird reproduction. Unlike all other species, however, common murre experienced below-average reproductive success at Southeast Farallon Island while primarily consuming Anchovy. The mechanisms driving low common murre reproduction are not entirely clear. It is possible that abundant adult but low YOY Anchovy abundance in central California impeded common murre reproduction as adults provide chicks with whole fish and adult Anchovy are too large for chicks to consume (Thompson et al., 2018). By contrast, a species such as Brandt’s cormorant can feed its chicks regurgitated Anchovy or young of the year rockfishes (Nur and Sydeman, 1999), and reproductive success has been above average since 2013 when either or both Anchovy or rockfishes were very abundant. Similarly, the western gull is a generalist predator and has had average to above-average reproductive success in 2021 and recent years after experiencing reproductive failure in 2011 when the abundance of many forage fishes was low. Cassin’s auklet, which feeds primarily on krill (Santora et al., 2014), also had above-average reproductive success in 2021, despite below-average overall krill abundance in central California. Overall krill abundance, however, is mostly driven by E. pacifica, and at-sea observations (Jarrod Santora, personal observation) indicated that upwelling-induced high abundance of T. spinifera likely provided adequate forage for Cassin’s auklet. Moreover, because the caloric value of T. spinifera is higher than E. pacifica (Ju et al., 2009; Färber Lorda and Murcia-Riaño, 2021), an increase in T. spinera could have compensated as an energy source for predators for a reduction in E. pacifica. In contrast with Southeast Farallon Island, common murre had average reproductive success at Yaquina Head. Given the forage base off Oregon, we expected common murre reproduction to be well above average in 2021. However, disturbance by eagles was approximately average and probably reduced reproductive success. Although common murre reproduction was only average at Yaquina Head in 2021, it was much higher than in 2020 when eagle harassment was very intense. The high eagle activity in 2020 may have been due to the lack of people visiting the region when it was closed to the public because of the COVID pandemic. In 2021, park visitation was closer to normal, and this likely reduced eagle disturbance (Rachel Orban, personal observation). Similarly, increased harassment by eagles and augmented murre egg mortality occurred due to COVID-induced reduction in human activity in the Baltic Sea in 2021 (Hentati-Sundberg et al., 2021). Overall, the documented bottom-up and top-down forces largely accurately predicted seabird reproduction in 2021.
Seabird abundance and distribution patterns at sea mostly did not adhere to our expectations. Off central California, the cool-water-associated sooty shearwater was high, likely in response to the abundant forage base and cool conditions (Santora et al., 2011; Santora et al., 2017). Unexpectedly, the at-sea abundance of common murre was also high, which is surprising as this species tends to spend more time on the colony when prey is locally abundant (Harding et al., 2007). However, the low common murre reproductive success at Southeastern Farallon Island suggests that local prey abundance was suboptimal in 2021. It is thus likely that common murre were forced to forage away from land which explains the high at-sea abundance. In southern California, the abundance of warm- and cool-water migrants did not meet our expectations. Unlike most of the CCE, summer SST was above average which may have driven cool-water-associated sooty sheerwater further north. In addition, while warm-water black-vented sheerwater decreased relative to recent years, this species was mostly absent from southern California subsequent to the 2014–2016 MHW. Hence, slightly above-average abundances of black-vented sheerwater may have been facilitated by moderately high SST. Overall, while at-sea bird abundances did not adhere to our a priori predictions, the patterns make sense in light of observed environmental and prey base conditions.
Marine mammals
As expected going into 2021, sea lion reproductive success was high. However, our prediction for sea lion reproduction was based on the continued high abundance of Anchovy rather than the onset of La Niña conditions. Pup count and growth rate have been above average from 2017 to 2021 and pup weight from 2016 to 2021. This is a sharp contrast to 2013–2015 when pups were starving, and an unusual mortality event (UME) was investigated by the National Marine Fisheries Service (Laake et al., 2018). The UME and abrupt recovery are well explained by the dynamics of Anchovy and Sardine (McClatchie et al., 2016a). One of the few periods in the past 70 years when both Anchovy and Sardine had very low abundances was during 2013–2015. By mid-2016, Anchovy born in the high recruitment year of 2015 began to spawn (Thompson et al., 2022) and were thus large enough to be available as prey for nursing adult female sea lions (Wells et al., 2017). The influx of high-quality Anchovy prey in 2016 augmented pup weight, and by 2017, forage was plentiful both during and after sea lion pregnancy. The 6-year run of above average is the second longest of the time series to a 7-year period from 2002 to 2008. Given that Anchovy abundance was at close to an all-time high in 2021 and that Anchovy recruitment was still high in southern California in 2021, it is likely that the 7-year streak of above-average sea lion condition will be matched in 2022.
Southern California current ecosystem
Although the southernmost biological survey in 2021 was north of the U.S./Mexico border, the CCE extends south into Mexican waters. The latitude of the southernmost portion of the CC varies annually, but the CC typically moves westward away from the coast in southern Baja California Sur (Checkley and Barth, 2009; Contreras-Catala et al., 2021). In Baja California Sur, Bahia Magdalena (24.5°N) is a biogeographic barrier separating temperate/subtropical species from tropical species (Contreras-Catala et al., 2021). During strong El Niños, however, tropical fish and invertebrates will move north of Bahia Magdalena but rapidly diminish from northern areas upon cessation of anomalously warm conditions (Funes-Rodríguez et al., 2006; Funes-Rodríguez et al., 2011). In 2021, we, therefore, expected that the southern CC biological assemblage would comprise largely of temperate/subtropical species. Unfortunately, the Mexican counterpart to CalCOFI, Investigaciones Mexicanas de la Corriente de California (IMECOCAL), did not sample the southern CC in 2021. If sampling resumes in 2022, we will have further opportunities to evaluate the impacts of La Niña on the full CCE.
Suggestions for the future and conclusion
EBUS are some of the most productive marine systems in the world and affect the livelihood of millions of people (Pauly and Christensen, 1995). As climate change accelerates, and non-analogous oceanographic conditions manifest (Jacox et al., 2022), the biological components of EBUS may change in unpredictable ways (Checkley et al., 2017). Continuous monitoring of EBUS is thus critical to manage ecosystems to the best of our capacity. Here, we document major physical and biological indices in the CCE. A suggestion for future work is to assess the ecosystem status of not just the CCE but all major EBUS concurrently to evaluate differences and commonalities within and among EBUS.
In conclusion, climate indices, including the ONI and PDO index were negative, indicating a shift to cooler conditions. As predicted, the shift to cooler conditions was reflected in the lower temperature, higher salinity, and higher chlorophyll a throughout much of the CCE, although this response was somewhat muted south of Point Conception. Generally, northern species such as northern copepods and northern mesopelagic larval fish species increased. A robust prey base augmented shore bird and sea lion reproductive success. In addition, many species displayed patterns in abundance that varied regionally, such as krill, adult market squid, YOY Hake, and YOY Anchovy (Figure 10). The difference among regions in the CCE could be related to the relative importance of changes in local processes and dynamics, such as the variability in alongshore wind, wind stress curl, and eddies (Fewings, 2017; Xiu et al., 2018; Fewings and Brown, 2019). As such, there is still much more to understand about the CCE, and going through this exercise provides an opportunity to more holistically assess our knowledge related to the ecosystem dynamics of the entire CCE.
Looking forward, the NOAA Climate Prediction Center has forecasted that La Niña conditions will persist in 2022. As such, we predict that physical and biological conditions will be similar to 2021. However, several unexpected events occurred in 2021, and it remains to be seen what surprises the CCE will have in store for us in 2022. With continual monitoring, we will determine if we need to change our expectations moving into the future.
Data availability statement
The datasets presented in this study can be found in online repositories. The names of the repository/repositories and accession number(s) can be found in the article/Supplementary Material.
Ethics statement
The animal study was reviewed and approved by Scripps Institution of Oceanography.
Author contributions
All authors contributed to the article and approved the submitted version.
Conflict of interest
The authors declare that the research was conducted in the absence of any commercial or financial relationships that could be construed as a potential conflict of interest.
Publisher’s note
All claims expressed in this article are solely those of the authors and do not necessarily represent those of their affiliated organizations, or those of the publisher, the editors and the reviewers. Any product that may be evaluated in this article, or claim that may be made by its manufacturer, is not guaranteed or endorsed by the publisher.
Supplementary material
The Supplementary Material for this article can be found online at: https://www.frontiersin.org/articles/10.3389/fmars.2022.958727/full#supplementary-material
References
Auth T. D., Brodeur R. D., Soulen H. L., Ciannelli L., Peterson W. T (2011). The response of fish larvae to decadal changes in environmental forcing factors off the Oregon coast. Fisheries Oceanography 20, 314–328.
Auth T. D., Daly E. A., Brodeur R. D., Fisher J. L. (2018). Phenological and distributional shifts in ichthyoplankton associated with recent warming in the northeast pacific ocean. Global Change Biol. 24, 259–272. doi: 10.1111/gcb.13872
Bond N. A., Batchelder H. P., Bograd S. J. (2008). Forecasting northeastern pacific ecosystem responses to la niña. Eos Trans. Am. Geophys Union 89, 321–322. doi: 10.1029/2008EO350002
Burford B. P., Wild L. A., Schwarz R., Chenoweth E. M., Sreenivasan A., Elahi R., et al. (2022). Rapid range expansion of a marine ectotherm reveals the demographic and ecological consequences of short-term variability in seawater temperature and dissolved oxygen. Am. Nat. 199, 523–550. doi: 10.1086/718575
Chasco B. E., Hunsicker M. E., Jacobson K. C., Welch O. T., Morgan C. A., Muhling B. A., et al. (2022). Evidence of temperature-driven shifts in market squid doryteuthis opalescens densities and distribution in the California current ecosystem. Mar. Coast. Fisheries 14, e10190. doi: 10.1002/mcf2.10190
Chavez F. P., Ryan J., Lluch-Cota S. E., Niquen M. (2003). From anchovies to sardines and back: Multidecadal change in the pacific ocean. Science 299, 217–221. doi: 10.1126/science.1075880
Checkley D. M. Jr, Asch R. G., Rykaczewski R. R. (2017). Climate, anchovy, and sardine. Annu. Rev. Mar. Sci. 9, 469–493. doi: 10.1146/annurev-marine-122414-033819
Checkley D. M., Barth J. A. (2009). Patterns and processes in the California current system. Prog. Oceanogr 83, 49–64. doi: 10.1016/j.pocean.2009.07.028
Contreras-Catala F., Beier E. J., Sánchez-Velasco L., Godínez V. M., Sánchez-Pérez E. D., Barton E. D. (2021). Water masses and larval fish habitats in the pacific tropical-subtropical convergence off Mexico. Continental Shelf Res. 230, 104575. doi: 10.1016/j.csr.2021.104575
Cury P. M., Fromentin J. M., Figuet S., Bonhommeau S. (2014). Resolving hjort’s dilemma: How is recruitment related to spawning stock biomass in marine fish? Oceanography 27, 42–47. doi: 10.5670/oceanog.2014.85
Daly E. A., Brodeur R. D., Morgan C. A., Burke B. J., Huff D. H. (2021). Prey selectivity and diet partitioning of juvenile salmon in coastal waters in relation to prey biomass and implications for salmon early marine survival. North Pacific Anadromous Fish Commission Tech. Rep. 17, 53–56.
Di Lorenzo E., Schneider N., Cobb K. M., Franks P. J. S., Chhak K., Miller A. J., et al. (2008). North pacific gyre oscillation links ocean climate and ecosystem change. Geophys Res. Lett. 35, L08607. doi: 10.1029/2007GL032838
Du X., Peterson W. T. (2018). Phytoplankton community structure in 2011–2013 compared to the extratropical warming event of 2014–2015. Geophys Res. Lett. 45, 1534–1540. doi: 10.1002/2017GL076199
Färber Lorda J., Murcia-Riaño M. (2021). Trophic position and partitioning differences between euphausiid species in the southern California current and eastern tropical north pacific using stable isotope ratios (δ13C and δ15N), elemental composition, and lipid contents. Mar. Biol. 168, 121. doi: 10.1007/s00227-021-03928-6
Fewings M. R. (2017). Large-Scale structure in wind forcing over the California current system in summer. Monthly Weather Rev. 145, 4227–4247. doi: 10.1175/MWR-D-17-0106.1
Fewings M. R., Brown K. S. (2019). Regional structure in the marine heat wave of summer 2015 off the Western united states. Front. Mar. Sci. 6. doi: 10.3389/fmars.2019.00564
Field J. C., Maccall A. D., Ralston S., Love M. S., Miller E. F. (2010). Bocaccionomics: The effectiveness of pre-recruit indices for assessment and management of bocaccio. CalCOFI Rep. 51, 1–14.
Filbee-Dexter K., Pittman J., Haig H. A., Alexander S. M., Symons C. C., Burke M. J. (2017). Ecological surprise: concept, synthesis, and social dimensions. Ecosphere 8, e02005. doi: 10.1002/ecs2.2005
Fisher J., Casini M., Frank K. T., Möllmann C., Leggett W. C., Daskalov G. (2015). The importance of within-system spatial variation in drivers of marine ecosystem regime shifts. Philos. Trans. R. Soc. B: Biol. Sci. 370, 20130271. doi: 10.1098/rstb.2013.0271
Funes-Rodríguez R., Hinojosa-Medina A., Aceves-Medina G., Jiménez-Rosenberg S. P. A., Jesús Bautista-Romero J. (2006). Influences of El niño on assemblages of mesopelagic fish larvae along the pacific coast of Baja California sur. Fisheries Oceanogr 15, 244–255. doi: 10.1111/j.1365-2419.2005.00388.x
Funes-Rodríguez R., Zárate-Villafranco A., Hinojosa-Medina A., González-Armas R., Hernández-Trujillo S. (2011). Mesopelagic fish larval assemblages during El niño-southern oscillation, (1997–2001) in the southern part of the California current. Fisheries Oceanogr 20, 329–346. doi: 10.1111/j.1365-2419.2011.00587.x
Gallo N. D., Bowlin N. M., Thompson A. R., Satterthwaite E. V., Brady B., Semmens B. X. (2022). Fisheries surveys are essential ocean observing programs in a time of global change: A synthesis of oceanographic and ecological data from U.S. West coast fisheries surveys. Front. Mar. Sci. 9, 757124. doi: 10.3389/fmars.2022.757124
Garcia-Reyes M., Sydeman W. J., Schoeman D. S., Rykaczewski R. R., Black B. A., Smit A. J., et al. (2015). Under pressure: Climate change, upwelling, and Eastern boundary upwelling ecosystems. Front. Mar. Sci. 2. doi: 10.3389/fmars.2015.00109
Harding A. M. A., Piatt J. F., Schmutz J. A., Shultz M. T., Van Pelt T. I., Kettle A. B., et al. (2007). Prey density and the behavioral flexibility of a marine predator: The common murre (Uria aalge). Ecology 88, 2024–2033. doi: 10.1890/06-1695.1
Hayward T. L., Durazo R., Murphree T., Baumgartner T. R., Gaxiola-Castro G., Schwing F. B., et al. (1999). The state of the California current in 1998-1999: Transition to cool-water conditions. CalCOFI Rep. 40, 29–62.
Hentati-Sundberg J., Berglund P.-A., Hejdström A., Olsson O. (2021). COVID-19 lockdown reveals tourists as seabird guardians. Biol. Conserv. 254, 108950. doi: 10.1016/j.biocon.2021.108950
Hixon M. A., Johnson D. W., Sogard S. M. (2013). BOFFFFs: on the importance of conserving old-growth age structure in fishery populations. ICES J. Mar. Sci. 71, 2171–2185. doi: 10.1093/icesjms/fst200
Hyrenbach K. D., Veit R. R. (2003). Ocean warming and seabird communities of the southern California current system, (1987–98): Response at multiple temporal scales. Deep Sea Res. Part II: Topical Stud. Oceanogr 50, 2537–2565. doi: 10.1016/S0967-0645(03)00123-1
Jacox M. G., Alexander M. A., Amaya D., Becker E., Bograd S. J., Brodie S., et al. (2022). Global seasonal forecasts of marine heatwaves. Nature 604, 486–490. doi: 10.1038/s41586-022-04573-9
Jacox M. G., Alexander M. A., Mantua N. J., Scott J. D., Hervieux G., Webb R. S., et al. (2018). Forcing of multiyear extreme ocean temperatures that impacted California current living marine resources in 2016. Bull. Am. Meteorol Soc. 99, S27–S33. doi: 10.1175/BAMS-D-17-0119.1
Ju S.-J., Kang H.-K., Kim W. S., Harvey H. R. (2009). Comparative lipid dynamics of euphausiids from the Antarctic and northeast pacific oceans. Mar. Biol. 156, 1459–1473. doi: 10.1007/s00227-009-1186-1
Laake J. L., Lowry M. S., Delong R. L., Melin S. R., Carretta J. V. (2018). Population growth and status of california sea lions. J. Wildlife Manage. 82, 583–595. doi: 10.1002/jwmg.21405
Leising A. W., Schroeder I. D., Bograd S. J., Abell J., Durazo R., Gaxiola-Castro G., et al. (2015). State of the California current 2014-2015: Impacts of the warm-water “Blob”. CalCOFI Rep. 56, 31–68.
Litzow M. A., Hunsicker M. E., Bond N. A., Burke B. J., Cunningham C. J., Gosselin J. L., et al. (2020). The changing physical and ecological meanings of north pacific ocean climate indices. Proc. Natl. Acad. Sci. 117, 7665–7671. doi: 10.1073/pnas.1921266117
Love M. S., Yoklavich M., Thorsteinson L. (2002). The rockfishes of the northeast pacific (Berkely, CA: University of California Press).
McClatchie S. (2014). Regional fisheries oceanography of the California current system and the CalCOFI program (Springer).
McClatchie S., Field J. C., Thompson A. R., Gerrodette T., Lowry M., Fiedler P. C., et al. (2016a). Food limitation of sea lion pups and the decline of forage off central and southern California. R. Soc. Open Sci. 3, 150628. doi: 10.1098/rsos.150628
McClatchie S., Goericke R., Leising A. L., Auth T. D., Bjorkstedt E., Robertson R. R., et al. (2016b). State of the California current 2015–16: Comparisons with the 1997–98 El niño. CalCOFI Rep. 57, 1–57.
Melin S. R., Orr A. J., Harris J. D., Laake J. L., Delong R. L., Gulland F. M., et al. (2010). Unprecedented mortality of California sea lion pups associated with anomalous oceanographic conditions along the central California coast in 2009. CalCOFI Rep. 51, 182–194.
Nur N., Sydeman W. J. (1999). Survival, breeding probability and reproductive success in relation to population dynamics of brandt’s cormorants phalacrocorax penicillatus. Bird Study 46, S92–S103. doi: 10.1080/00063659909477236
Pauly D., Christensen V. (1995). Primary production required to sustain global fisheries. Nature 374, 255–257. doi: 10.1038/374255a0
Peña M. A., Bograd S. J. (2007). Time series of the northeast pacific. Prog. Oceanogr 75, 115–119. doi: 10.1016/j.pocean.2007.08.008
Peterson W. T., Fisher J. L., Peterson J. O., Morgan C. A., Burke B. J., Fresh K. L. (2014). Applied fisheries oceanography: Ecosystem indicators of ocean conditions inform fisheries management in the California current. Oceanography 27, 80–89. doi: 10.5670/oceanog.2014.88
Porquez J. M., Klunis L., Kim E., Cox A., Dlinajec N., Orben R. A. (2021). Yaquina head seabird colony: 2021 season summary (Department of Fisheries, Wildlife, and Conservation Sciences, Oregon State University). Available at: https://hmsc.oregonstate.edu/sites/hmsc.oregonstate.edu/files/yhona_finalreport2021.pdf.
Ralston S., Jc F., Km S. (2015). Long-term variation in a central California pelagic forage assemblage. J. Mar. Syst. 146, 26–37. doi: 10.1016/j.jmarsys.2014.06.013
Reiss C. S., Maxwell M. R., Hunter J. R., Henry A. (2004). Investigating environmental effects on population dynamics of Loligo opalescens in the southern California bight. CalCOFI Rep. 45, 87–97.
Robertson R. R., Bjorkstedt E. P. (2020). Climate-driven variability in euphausia pacifica size distributions off northern California. Prog. Oceanogr 188, 102412. doi: 10.1016/j.pocean.2020.102412
Sakuma K. M., Ralston S., Wespestad V. G (2006). Interannual and spatial variation in the distribution of young-of-the-year rockfish (Sebastes spp.): expanding and coordinating a survey sampling frame. CalCOFI Rep. 47, 127–139.
Sakuma K. M., Field J. C., Mantua N. J., Ralston S., Marinovich B. B., Carrion C. N. (2016). Anomalous epipelagic micronekton assemblage patterns in the neritic waters of the california current in spring 2015 during a period of extreme ocean conditions. CalCOFI Rep. 57, 163–183.
Santora J. A., Hazen E. L., Schroeder I. D., Bograd S. J., Sakuma K. M., Field J. C. (2017). Impacts of ocean climate variability on biodiversity of pelagic forage species in an upwelling ecosystem. Mar. Ecol. Prog. Ser. 580, 205–220. doi: 10.3354/meps12278
Santora J. A., Rogers T. L., Cimino M. A., Sakuma K. M., Hanson K. D., Dick E. J., et al. (2021a). Diverse integrated ecosystem approach overcomes pandemic-related fisheries monitoring challenges. Nat. Commun. 12, 6492. doi: 10.1038/s41467-021-26484-5
Santora J. A., Schroeder I. D., Bograd S. J., Chavez F. P., Cimino M., Fiechter J., et al. (2021b). Pelagic biodiversity, ecosystem function, and services: An integrated observing and modeling approach. Oceanography 34, 16–37. doi: 10.5670/oceanog.2021.212
Santora J. A., Schroeder I. D., Field J. C., Wells B. K., Sydeman W. J. (2014). Spatio-temporal dynamics of ocean conditions and forage taxa reveal regional structuring of seabird–prey relationships. Ecol. Appl. 24, 1730–1747. doi: 10.1890/13-1605.1
Santora J. A., Sydeman W. J., Schroeder I. D., Wells B. K., Field J. C. (2011). Mesoscale structure and oceanographic determinants of krill hotspots in the California current: Implications for trophic transfer and conservation. Prog. Oceanogr 91, 397–409. doi: 10.1016/j.pocean.2011.04.002
Schroeder I. D., Santora J. A., Bograd S. J., Hazen E. L., Sakuma K. M., Moore A. M., et al. (2019). Source water variability as a driver of rockfish recruitment in the California current ecosystem: implications for climate change and fisheries management. Can. J. Fisheries Aquat. Sci. 76, 950–960. doi: 10.1139/cjfas-2017-0480
Scripps Institution of Oceanography (SIO) (2012). Data report, CalCOFI cruise 1110. CC ref. 12-05 (La Jolla, CA:Scripps Institution of Oceanography), 59 pp.
Suchman C. L., Daly E. A., Keister J. E., Peterson W. T., Brodeur R. D. (2008). Feeding patterns and predation potential of scyphomedusae in a highly productive upwelling region. Mar. Ecol. Prog. Ser. 358, 161–172. doi: 10.3354/meps07313
Sydeman W. J., Dedman S., García-Reyes M., Thompson S. A., Thayer J. A., Bakun A., et al. (2020). Sixty-five years of northern anchovy population studies in the southern California current: A review and suggestion for sensible management. ICES J. Mar. Sci. 77, 486–499. doi: 10.1093/icesjms/fsaa004
Sydeman W. J., Thompson S. A., Field J. C., Peterson W. T., Tanasichuk R. W., Freeland H. J., et al. (2011). Does positioning of the north pacific current affect downstream ecosystem productivity? Geophys Res. Lett. 38, L12606. doi: 10.1029/2011gl047212
Thompson A. R., Ben-Aderet N. J., Bowlin N. M., Kacev D., Swalethorp R., Watson W. (2022). Putting the pacific marine heatwave into perspective: The response of larval fish off southern California to unprecedented warming in 2014–2016 relative to the previous 65 years. Global Change Biol. 28, 1766–1785. doi: 10.1111/gcb.16010
Thompson A. R., Chen D. C., Guo L. W., Hyde J. R., Watson W. (2017a). Larval abundances of rockfishes that were historically targeted by fishing increased over 16 years in association with a large marine protected area. R. Soc. Open Sci. 4, 170639. doi: 10.1098/rsos.170639
Thompson A. R., Harvey C. J., Sydeman W. J., Barceló C., Bograd S. J., Brodeur R. D., et al. (2019a). Indicators of pelagic forage community shifts in the California current Large marine ecosystem (1998–2016. Ecol. Indic. 105, 215–228. doi: 10.1016/j.ecolind.2019.05.057
Thompson A. R., Mcclatchie S., Weber E. D., Watson W., Lennert-Cody C. E. (2017b). Correcting for bias in ichthyoplankton abundance estimates associated with the 1977 transition form ring to bongo net sampling. CalCOFI Rep. 58, 113–123.
Thompson A. R., Schroeder I. D., Bograd S. J., Hazen E. L., Jacox M. G., Leising A. L., et al. (2018). State of the California current 2017-18: Still not quite normal in the north and getting interesting in the south. CalCOFI Rep. 59, 1–66.
Thompson A. R., Schroeder I. D., Bograd S. J., Hazen E. L., Jacox M. G., Leising A. L., et al. (2019b). State of the California current 2018-19: a novel anchovy regime and a new marine heatwave? CalCOFI Rep. 60, 1–65.
Warzybok P., Santora J. A., Ainley D. G., Bradley R. W., Field J. C., Capitolo P. J., et al. (2018). Prey switching and consumption by seabirds in the central California current upwelling ecosystem: Implications for forage fish management. J. Mar. Syst. 185, 25–39. doi: 10.1016/j.jmarsys.2018.04.009
Weber E. D., Auth T. D., Baumann-Pickering S., Baumgartner T. R., Bjorkstedt E. P., Bograd S. J., et al. (2021). State of the California current 2019–2020: Back to the future with marine heatwaves? Front. Mar. Sci. 8. doi: 10.3389/fmars.2021.709454
Wells B. K., Schroeder I. D., Bograd S. J., Hazen E.L, Jacox M. G., Leising A., et al. (2017). State of the California current 2016–17: Still anything but “normal” in the north. CalCOFI Rep. 58, 1–55.
Wells B. K., Schroeder I. D., Santora J. A., Hazen E. L., Bograd S. J., Bjorkstedt E. P., et al. (2013). State of the california current 2012-13: No such thing as an “average” year. California Cooperative Oceanic Fisheries Investigations Rep. 54, 37–71.
Keywords: California current ecosystem, La Niña, upwelling, anchovy, ecosystem assessment, white walkers
Citation: Thompson AR, Bjorkstedt EP, Bograd S J, Fisher JL, Hazen EL, Leising A, Santora JA, Satterthwaite EV, Sydeman WJ, Alksne M, Auth TD, Baumann-Pickering S, Bowlin NM, Burke B J, Daly EA, Dewar H, Field J C, Garfield NT, Giddings A, Goericke R, Hildebrand J, Horton CA, Jacobson K C, Jacox MG, Jahncke J, Johns M, Jones J, Kudela RM, Melin S R, Morgan CA, Nickels CF, Orben RA, Porquez JM, Portner EJ, Preti A, Robertson RR, Rudnick DL, Sakuma KM, Schroeder ID, Snodgrass OE, Thompson SA, Trickey JS, Warzybok P, Watson W and Weber ED (2022) State of the California Current Ecosystem in 2021: Winter is coming? Front. Mar. Sci. 9:958727. doi: 10.3389/fmars.2022.958727
Received: 31 May 2022; Accepted: 19 August 2022;
Published: 20 September 2022.
Edited by:
Bernardo Patti, National Research Council (CNR), ItalyReviewed by:
Jaime Färber Lorda, Center for Scientific Research and Higher Education in Ensenada (CICESE), MexicoThomas Bryce Kelly, University of Alaska Fairbanks, United States
Copyright © 2022 Thompson, Bjorkstedt, Bograd, Fisher, Hazen, Leising, Santora, Satterthwaite, Sydeman, Alksne, Auth, Baumann-Pickering, Bowlin, Burke, Daly, Dewar, Field, Garfield, Giddings, Goericke, Hildebrand, Horton, Jacobson, Jacox, Jahncke, Johns, Jones, Kudela, Melin, Morgan, Nickels, Orben, Porquez, Portner, Preti, Robertson, Rudnick, Sakuma, Schroeder, Snodgrass, Thompson, Trickey, Warzybok, Watson and Weber. This is an open-access article distributed under the terms of the Creative Commons Attribution License (CC BY). The use, distribution or reproduction in other forums is permitted, provided the original author(s) and the copyright owner(s) are credited and that the original publication in this journal is cited, in accordance with accepted academic practice. No use, distribution or reproduction is permitted which does not comply with these terms.
*Correspondence: Andrew R. Thompson, YW5kcmV3LnRob21wc29uQG5vYWEuZ292