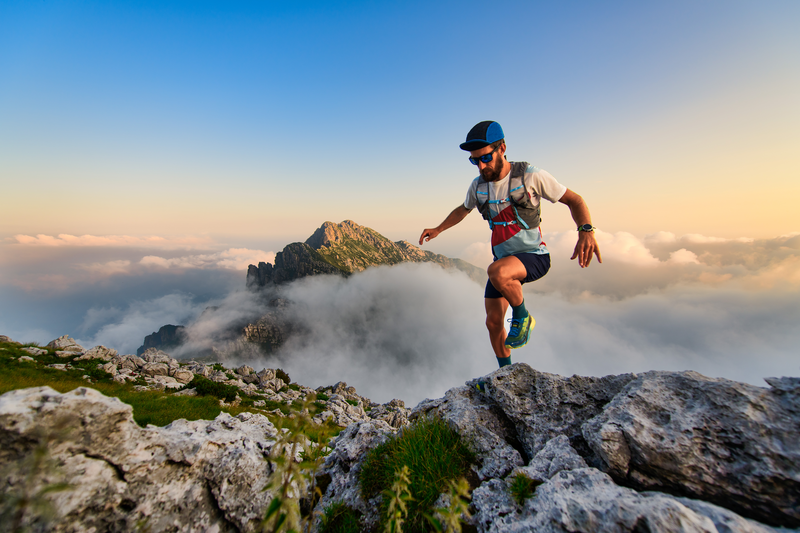
95% of researchers rate our articles as excellent or good
Learn more about the work of our research integrity team to safeguard the quality of each article we publish.
Find out more
ORIGINAL RESEARCH article
Front. Mar. Sci. , 26 September 2022
Sec. Marine Pollution
Volume 9 - 2022 | https://doi.org/10.3389/fmars.2022.953648
This article is part of the Research Topic Coastal Environmental and Ecological Data Analysis View all 30 articles
The geomorphology of coastal lagoons has an important effect on the hydrological balance between fresh water and seawater, which exhibit a wide range of salinity from nearly fresh water to hypersaline water. The present study aimed to characterize seasonal biogeochemical properties of suspended particulate organic matter (POM) in response to environmental variability driven by hydrological dynamics in contrasting coastal lagoon systems. We selected two lagoons distinguished by their geomorphological features, a permanently open artificial lagoon (Gyeongpoho) and an intermittently open natural lagoon (Hyangho), analyzed environmental characteristics, and compared the seasonal differences in the biochemical compositions of POM. Variations in temperature and precipitation in both lagoons showed the characteristics of a typical monsoon climate but variation in salinity differed 6.5−35.0 in Gyeongpoho and 1.1−10.8 in Hyangho. A self-organizing map analysis using the environmental data revealed that the spatiotemporal variations in salinity and nutrient concentrations differed significantly between the two lagoon systems, indicating a difference in hydrologic connectivity between lagoons and marine systems. Furthermore, a canonical correlation analysis highlighted that the POM properties differed according to physicochemical factors. The differing environmental conditions may affect the spatial and temporal variations in organic matter composition, resulting in significant differences in the biochemical composition of POM between two lagoon systems. Overall, this information provides insight into the origin and transport process of POM under changing coastal lagoon environments caused by climatic and human activities.
Coastal lagoons are highly heterogeneous aquatic ecosystems formed as a result of the input of variable amounts of freshwater and seawater as transition zones between terrestrial and marine systems (Kjerfve, 1994). These coastal lagoons are among the most productive ecosystems which promote the biological activities of primary producers, owing to a great input of nutrients and organic matter (Pérez-Ruzafa et al., 2019). However, the estimated primary production in such coastal systems generally has highly variable ranges with spatiotemporal variability in biotic and abiotic factors (Carrasco Navas-Parejo et al., 2020). These drivers are significantly influenced by the geomorphological features of lagoons, which can control the hydrological balance between freshwater and seawater (Kjerfve, 1994). Furthermore, the degree of mixing between freshwater and seawater may lead to dynamic ecological processes causing high biodiversity due to environmental heterogeneity and habitat diversity (Pérez-Ruzafa et al., 2011; Lopes and Dias, 2015). As a result, coastal lagoon ecosystems are strongly influenced by hydrogeomorphological dynamics with high intrinsic variability in physicochemical factors at a regional scale, resulting in significant changes in the entire ecosystem.
The particulate organic matter (POM) in coastal waters is affected by a series of physicochemical and biological processes (Chen et al., 2021; Lu et al., 2021). The origins of POM in coastal lagoons are heterogeneous because of the influence of different sources of organic matter on the dynamics of physical, chemical, and biological factors (Rossi et al., 2003; Cresson et al., 2012; Lønborg et al., 2017; Garcia et al., 2018). Primary production by phytoplankton is a major source of POM in coastal lagoons. In addition, local benthic primary producers, such as macroalgae and marsh plants, and input of terrestrial material by freshwater discharge, also considerably contribute to the POM pool in the lagoons. Temporal changes in the diverse sources related to hydrological variations in lagoon systems may lead to a complex mixture of POM (Garcia et al., 2017; Lønborg et al., 2017). The POM in coastal environments exhibits seasonal variability in both quantitative and qualitative characteristics (Grémare et al., 1997; Rossi et al., 2003). Such seasonality in POM can influence the abundance, biomass, and recruitment of pelagic and benthic animal communities, and are major factors controlling food availability for consumer species (Kleppel and Burkart, 1995; Grémare et al., 1997). However, because of the complexity of the POM pool, it is very difficult to assess its quantity, quality, and relative importance for consumers in coastal lagoon systems.
Carbon and nitrogen stable isotope analysis has been successfully used to describe the source of organic matter in coastal environments, which varies with the spatial and temporal heterogeneity of biotic and abiotic factors (Cresson et al., 2012; Briand et al., 2015; Ke et al., 2017). Because marine and terrestrial primary producers have different photosynthetic pathways, they have distinct isotope ratios (δ13C and δ15N) from each other (Peterson and Fry, 1987). In general, marine-derived organic matter has higher δ13C and δ15N values than terrestrial-derived organic matter. Moreover, major primary producers in temperate coastal systems may have highly variable δ13C values; green macroalgae (−12‰ to −10‰) and microphytobenthos (−20‰ to −10‰) are more 13C-enriched than marine phytoplankton (−24‰ to − 18‰) and marsh plants (−23‰ to − 29‰) (Fry and Sherr, 1984; Kang et al., 2003; Park et al., 2015; Park et al., 2016). The δ15N values of primary producers may also reflect the source of inorganic nutrients and the magnitude of discrimination based on nutrient concentrations (McClelland and Valiela, 1998). The δ15N values have been used as indicators of anthropogenic effects on coastal ecosystems because organic matter derived from anthropogenic sources (e.g., artificial fertilizer and sewage) is usually more 15N-enriched than that from natural sources (Cole et al., 2004; Ke et al., 2017). Accordingly, the δ13C and δ15N values of POM are representative of the relative contribution of each of the diverse sources in complex coastal lagoon systems.
Analysis of the biochemical compositions of POM pools has been used to assess the origin of organic matter and its nutritional value as a basal food source available to primary consumers (Navarro and Thompson, 1995; Danovaro et al., 2000; Cresson et al., 2012). Labile compounds such as proteins, lipids, and carbohydrates of POM are metabolically utilized by consumers as readily bioavailable fractions (Mazzola et al., 2001). In general, labile POM, which is a recently synthesized organic matter, is produced by phytoplankton photosynthesis. The biochemical composition of POM may reflect the physiological status and nutritional value of phytoplankton species in response to diverse environmental conditions, such as light, river discharge, and nutrient availability (Danovaro et al., 2000; Lee et al., 2017; Bhavya et al., 2019). Thus, this approach can provide useful information on the ambient environmental conditions and/or changes related to biogeochemical processes in complex coastal ecosystems.
Gyeongpoho and Hyangho, located adjacent to each other, have different ecological environments due to their hydrological characteristics (Park et al., 2020). However, there is no study on spatiotemporal variations in carbon and nitrogen dynamics of POM that could explain the ecosystem structure and function of the lagoons as a transition zone between ocean and land (Chen et al., 2021; Lu et al., 2022). The present study aimed to characterize the seasonal biogeochemical processes of POM in coastal lagoon systems in response to environmental variability using hydrological dynamics. We hypothesized that the biogeochemical characteristics of POM may be seasonally different depending on lagoon-specific processes, reflecting the hydrological conditions. To test this hypothesis, we compared the seasonal differences in isotopic signatures and biochemical compositions of POM with variations in environmental parameters between two permanently and intermittently open lagoons distinguished by the hydrological features. This information provides insight into the origin and transport process of POM under changing coastal lagoon environments caused by climatic and human activities.
The sampling sites were located in two coastal lagoons [Gyeongpoho (sites Ga–c) and Hyangho (sites Ha–b)] on the eastern coast of the Korean Peninsula (Figure 1). The two lagoons have different environmental characteristics due to presence or absence of artificial channel that cause differences in salinity gradients, although the depth ranges in both lagoons were generally below 1.0 m. Gyeongpoho has an area of 0.90 km2, and has several inlets through which freshwater inflows, and is connected to the sea via single artificial channel (WREO, 2008; Yoon et al., 2008). The artificial channel allows for continuous exchange of freshwater with seawater, which increases the freshwater’s salinity. In contrast, Hyangho has an area of 0.14 km2, and is usually separated from the sea by sand dunes, but is seasonally connected to the sea by a channel generated by heavy rainfall during summer monsoons (Park et al., 2020).
Samplings were conducted monthly from June 2019 to April 2020 at three and two sites in Gyeongpoho and Hyangho, respectively, depending on their distance from the sea. Water samples for nutrient, POC, PON, biochemical composition (PCHO, PPr, and PLip), and Chl-a analyses were collected from the surface of each study site. Monthly precipitation and atmospheric temperature data for the study area were obtained from the Korean Meteorological Administration (https://data.kma.go.kr/data/grnd/selectAsosRltmList.do?pgmNo=36).
The water temperature and salinity were measured using a YSI 30 handheld meter (YSI Inc., Yellow Springs, OH, USA). Samples for Chl-a and biochemical composition analyses were filtered through 47 mm Whatman GF/F filters. Samples for POC analyses were pre-filtration through a 200 µm net for eliminating large debris and any zooplankton and then gently filtered through 25 mm pre-combusted (450°C, 2 h) Whatman GF/F filters. The filtered water samples for dissolved inorganic nutrient analyses were transferred into acid-washed polyethylene bottles and frozen at −20°C, and the filter samples were wrapped in aluminum foil and then kept in a deep freezer (at –80°C) until the analysis.
The filtered samples for POC, PON, and 13C and 15N isotope ratio analyses were fumed with HCl for 12 h to remove carbonate and were subsequently freeze-dried. The dried samples were sealed within a tin disk and analyzed using an elemental analyzer (Vario MICRO Cube; Elementar, Hanau, Germany) coupled to a continuous-flow isotope ratio mass spectrometer (CF-IRMS; Isoprime 100; Cheadle Hulme, UK). The samples were oxidized at high temperature (1150°C) using an elemental analyzer. The resultant CO2 and N2 gases were separated using an adsorption column and then moved into the CF-IRMS using a He carrier gas. The C and N stable isotope ratios are expressed as δ notation relative to Pee Dee Belemnite for C and atmospheric air for N, using the equation δ(‰) = [(Rsample/Rstandard)−1] × 103, where R is the 13C/12C or 15N/14N ratio. International standards of reference materials sucrose [8542 ANU, δ13C = −10.47 ± 0.13‰, National Institute of Standards and Technology (NIST), Gaithersburg, MD, USA; RM 8568 UG, δ15N = −1.8 ± 0.1‰, NIST] were used for calibration after analyzing every 10th unknown sample. The precision based on repeated analyses of acetanilide (Thermo Scientific) as an internal standard was within 0.07‰ for δ13C and 0.11‰ for δ15N.
The filter samples for carbohydrates, proteins, and lipids of POM (PCHO, PPr, and PLip, respectively) were analyzed according to Lowry et al. (1951); Dubois et al. (1956); Bligh and Dyer (1959), and Marsh and Weinstein (1966), respectively.
The samples for PCHO were put into 15 mL PP tubes containing 1 mL of deionized water and triturated using a glass rod. Next, 1 mL of 5% phenol was added for PCHO extraction, and the solutions were stored at room temperature for 40 min. Subsequently, 5 mL sulfuric acid was added to the solution and mixed using a vortex mixer. The mixture was centrifuged at 3500 rpm for 10 min. The PCHO concentration of POM was determined based on the absorbance at 490 nm using a UV spectrophotometer (Labomed Inc., Los Angeles, CA, USA) and quantified as glucose equivalents (Sigma-Aldrich, St. Louis, MO, USA).
The PPr samples were placed in centrifuge tubes containing 1 ml of deionized water. For PPr extraction, the filter was triturated using glass rod and 5 mL of an alkaline copper solution (a mixture of 2% Na2CO3 in 0.1 N NaOH with 0.5% CuSO4·5H2O in 1% sodium tartrate; 50:1, v/v) was added to the tube. The solution was mixed using a vortex mixer and maintained at room temperature for 10 min. Subsequently, 0.5 mL of Folin–Ciocalteu phenol reagent was added to the solution. The solution was then centrifuged at 3000 rpm for 10 min. The PPr concentration of POM was determined based on the absorbance at 750 nm using a UV spectrophotometer and quantified as bovine serum albumin equivalents (Sigma-Aldrich).
For extraction of PLip, the samples were put in 16 mL glass tubes containing 3 mL of chloroform-methan (1:2, v/v), followed by triturating using a glass rod and mixing using a vortex mixer. The solutions were allowed to stand for 1 h in a refrigerator (4°C) to prevent evaporation loss. Subsequently, the solutions were centrifuged at 2000 rpm for 10 min and the supernatants were collected and transferred into new tubes. The extraction procedure was performed immediately and repeated. After completing the extraction, 4 mL of deionized water was added to new tubes containing the extractant. The solution was then homogenized using a vortex mixer and centrifuged at 2000 rpm to separate the solvents into two phases (chloroform for lipids and methanol + water). The methanol/water phase was removed from the solvent using a Pasteur pipette. The tubes containing the chloroform phase were exposed to N2 gas and were placed in a dry oven at 40°C to remove chloroform. After the chloroform phase was completely dried, 2 mL H2SO4 was added to the tubes. The tubes were heated to 200°C for 15 min using a heating block and cooled to room temperature. When the heating procedure was completed, the tubes were placed in a water bath at room temperature, and 3 ml of deionized water was added to the tubes. After all bubbles in the tubes disappeared, the PLip concentrations of POM were analyzed based on the absorbance at a wavelength of 375 nm and quantified as tripalmitin equivalents.
Water samples for dissolved inorganic nutrients were analyzed using a QuAAtro nutrient analyzer (SEAL Analytical GmbH, Norderstedt, Germany) to determine ammonium (NH4), nitrate (NO3), nitrate (NO2), phosphate (PO4), and silicate (SiO4) concentrations, according to the procedures developed for automated chemical analysis, with detection limits for 0.01 µM, 0.1 µM, 0.1 µM, 0.02 µM, and 0.1 µM, respectively (Murphy and Riley, 1962; Helder and De Vries, 1979; Hansen and Koroleff, 1999). Filter samples for Chl-a were extracted with 90% acetone in the dark at 4°C for 20 h and the concentrations of Chl-a were determined fluorometrically using a fluorometer (Turner Designs, Sunnyvale, CA) (Parsons et al., 1984).
Student t tests were performed to test variations in salinity data which were normal distribution. For data not normality distributed, Mann–Whitney U tests were performed to test the differences in C/N ratios and δ13C and δ15N values between the Gyeonghoho and Hyangho samples, and Kruskal–Wallis analysis of variance (ANOVA) was used to compare spatial and temporal variations in nutrients, Chl-a, POC, and PON concentrations, C/N ratios, and biochemical compositions among the study sites.
The environmental characteristics of the study sites were classified using a self-organizing map algorithm (SOM), which is a heuristic model used to visualize linear and nonlinear relationships in high-dimensional datasets (Kohonen, 1982). During the training process of the SOM, the data were assigned to neurons (processing units) in the input layer. After coarse training and fine-tuning steps, an output layer composed of output neurons was generated. In the output layer, a real sample was allocated to the most similar neuron, and samples with similar characteristics were arranged in adjacent neurons, which were placed in a two-dimensional grid. The number of output neurons was chosen according to the heuristic rule 5√n, where n is the number of training samples (Vesanto and Alhoniemi, 2000; Park et al., 2006). Subsequently, the entire procedure was performed several times to determine the final size of the SOM at the minimum levels of the quantization error and topographic error by running the entire procedure several times (Park et al., 2003). Here, 4 5 output neurons were used for clustering of physicochemical data in this study (7 variables, 33 samples from Gyeongpoho and 22 samples from 7 variables in Hyangho). After training the SOM, the cluster boundaries between different SOM units were determined using Ward’s linkage method with Euclidean distance measurements. The SOM training and clustering procedures were performed using version 4.0.2 of the R software (Licen et al., 2021).
Canonical correspondence analysis (CCA) using the CANOCO program was applied to outline the relationships between environmental factors (seven variables) and POM properties (six variables) at each study site (Ter Braak, 1986; Sundbäck and Snoeijs, 1991).
Variations in precipitation and air temperature in Gangneung City during the study period showed the characteristics of a typical monsoon climate (Figure 2). Precipitation increased rapidly from June 2019 with increasing temperature, peaked at 432 mm in October, and then decreased in winter. In both lagoons, temporal variations in water temperature in the study area represented also typical seasonality ranging from 3.6°C to 30.8°C (Figure 3).
Figure 2 Monthly precipitation and average of atmospheric temperature variations from January 2019 to December 2020 in Gangneung city. Green box represents sampling period.
Figure 3 Monthly variations in water temperature and salinity at study sites in Gyeongpoho (A) and Hyangho (B) during sampling period.
The monthly variations in salinity showed different patterns between the two lagoons. In Gyeongpoho, salinity remained low during the summer monsoon period (from July to October 2019) and increased with decreasing precipitation in the fall and winter. Spatial variation in salinity among sites in Gyeongpoho was different, ranging from 6.5−21.2 at St. Ga, 11.6−26.9 at St. Gb, and 14.8−35 at St. Gc (Figure 3A). On the contrary, spatial differences in salinity between St. Ha (ranging 1.1−8.8) and St. Hb (1.1−10.8) were not significant (Student t-test, p = 0.437). At the Hyangho sites, salinity increased in September, three months after the increase in precipitation.
Widespread spatiotemporal variations in nutrient concentrations were observed in the study regions (Figures 4A–E). The maximum concentrations of ammonium (78.1 µM at St. Hb in August), nitrate (39.2 µM at St. Gc in July), nitrate (3.4 µM St. Gc in July), phosphate (4.1 µM at St. Gc in August), and silicate (82.5 µM at St. Ha in October) were recorded during the summer monsoon period. Significant differences in the nutrient concentrations (Kruskal–Wallis test, p < 0.05) among the study sites were detected, except for silicate during the non-monsoon period. These differences were attributed to the relatively high nutrient concentrations at St. Gc.
Figure 4 Box plots of data for summer monsoon and non-monsoon periods showing (A) ammonium, (B) nitrate, (C) phosphate, (D) nitrite, (E) silicate, (F) Chl-a, (G) POC, (H) PON, (I) C/N ratio, (J) PCHO, (K) PPr, and (L) PLip. The blue and yellow boxes represent the sites of Gyeongpoho, and Hyanho, respectively. The median and average values are displayed with the black and red horizontal bar inside the box.
Chl-a concentrations peaked in March at St. Ga (15.0 µg L−1), St. Ha (18.5 µg L−1), and St. Hb (17.9 µg L−1), showing no significant spatiotemporal variations (Kruskal–Wallis test, p > 0.05) among the study sites (Figure 4F). Although the temporal variations in Chl-a concentrations among the study sites were not consistent, the concentrations tended to increase in July and March, except for St. Gc.
POC and PON concentrations at the study sites peaked in March 2020, except for St. Gc. The highest POC and PON concentrations were observed in March at St. Ga (9.20 mg C L−1) and St. Gb (2.02 mg N L−1), respectively. Interestingly, there were no significant differences (Kruskal-Wallis test, p > 0.05) among the study sites in both POC and PON concentrations during the monsoon period, but significant differences (Kruskal-Wallis test, p < 0.05) were observed during the non-monsoon period (Figures 4G, H). The C/N ratios in both lagoons tended to be higher (Mann–Whitney U test, p = 0.01) in the summer monsoon period than in the non-monsoon period (Figure 4I). Spatial variations in the ratios at Gyeongpoho sites (6.7 ± 2.4) were lower (Mann–Whitney U test, p = 0.08) than those at Hyangho sites (9.7 ± 4.2).
PCHO, PPr, and PLip concentrations varied 6.3−96.9 µg L−1, 1.1−53.1 µg L−1, 2.9−6.2 µg L−1, respectively. Large variations in PCHO and PPr concentrations were observed at St. Ga and St. Gb during the non-monsoon period. However, there were no significant differences in these concentrations (Kruskal–Wallis test, p > 0.05) between the study sites during the monsoon period (Figures 4J–L).
The δ13C values for POM tended to decrease gradually during summer and increase in fall and winter in both lagoons (Figure 5). In contrast, δ15N values for POM showed opposite trends to temporal changes in δ13C values. The δ13C and δ15N values were higher (Mann–Whitney U test, p < 0.001) in Gyeongpoho sites (−24.2 ± 2.5‰ and 4.9 ± 1.0‰, respectively) than in Hyanho sites (−26.4 ± 0.6‰ and 3.8± 0.5‰, respectively).
Figure 5 Monthly variations in δ13C and δ15N variations at study sites in Gyeongpoho (A) and Hyangho (B) during sampling period.
SOM training results were condensed onto 4×5 rectangular grid maps based on 33 datasets (three stations over 11 months) and 22 datasets (two stations over 11 months) of seven physicochemical variables in Gyeongpoho and Hyangho, respectively (Figure 6). In both lagoons, the dendrograms of SOM results grouped major 2 cluster coinciding with monsoon and non-monsoon periods, reflecting temperature and salinity variations and minor 2 clusters with high nutrient concentrations.
Figure 6 (A) Grouping of characteristics in study sites according to time and space on the Kohonen self-organizing map algorithm (SOM) trained with environmental factors. Gradient of unit box color (white-red) represents neighbor distance. Dendrogram of the trained SOM groups based on Ward’s linkage method and information table of individual samples (B).
Canonical correspondence analysis (CCA) was applied to investigate the relationships between environmental factors (water temperature, salinity, and nutrients) and biogeochemical properties (POC, PON, Chl-a, PCHO, PPr, and PLip) (Figure 7 and Table 1). The first two ordination axes of the CCA explained 96.5%, 87.5%, and 94.3% of the factors in biogeochemical properties at St. Ga and St. Gb, St. Gc, and St. Ha and St. Hb, respectively, with respect to environmental factors. Samples with each dataset, represented by colored circles, were dispersed on CCA diagrams according to the characteristics of environmental and biochemical factors. The samples were closely distributed and coincided with the clusters classified in SOM analysis. In St. Ga and St. Gb, Chl-a and PLip are located opposite other biogeochemical properties along axis 1 of the diagram reflecting negative correlation, and PPr is highly related to dissolved inorganic nitrogen. At St. Gc, salinity and PPr were negatively correlated with other environmental factors and biogeochemical properties, respectively. At St. Ha and Hb, PCHO was negatively correlated with other biogeochemical properties.
Figure 7 Ordination diagram obtained from canonical correspondence analysis based on Chl-a, POC, PON, PCHO, PPr, and PLip located in St. Ga and Gb (A), St. Gc (B), and St. Ha and St. Hb (C) with respect to environmental factors.
The present study assessed both seasonal and spatial dynamics of the biochemical and isotopic characteristics of suspended POM in the coastal lagoons of Korea by comparing environmental conditions and biological variabilities between permanently and intermittently open lagoons. Our study showed that seasonal and spatial biogeochemical variations in POM are closely associated with changes in freshwater input after the monsoon period and hydrologic connectivity between lagoons and marine systems. The seasonal dynamics of environmental conditions resulting from a response to the variation in the hydrological balance between freshwater and seawater might affect the biogeochemical composition of POM, which is comprised of diverse organic matter sources. In addition, multivariate analysis allowed us to better understand the relationships between environmental factors and POM characteristics in the lagoons. The SOM results confirmed that the variations in environmental factors at the study sites were differentiated by the monsoon and non-monsoon periods. As results of CCA, POM characteristics in the lagoons varied according to the changes of environmental factors and there were no significant correlations between Chl-a and the other biogeochemical properties, reflecting high contribution of anthropogenic sources for POM (Pérez-Ruzafa et al., 2019). Overall, the seasonal difference in the degree of mixing of freshwater and seawater by geomorphological lagoon features may lead to spatial and temporal heterogeneity in POM composition with environmental conditions.
In general, the biogeochemical characteristics of POM in a variety of coastal areas show high spatial and temporal variability (Vizzini and Mazzola, 2003; Bănaru et al., 2007). Environmental factors and hydrodynamic regimes may lead to differences in the origin, distribution, and transfer of POM within coastal areas (Deegan and Garritt, 1997; Vizzini and Mazzola, 2006). POM in coastal lagoons is generally a heterogeneous pool consisting of a mixture of living phytoplankton and detrital material derived from terrestrial and/or marsh organic matter (Berto et al., 2013; Escalas et al., 2015; Garcia et al., 2017). Seasonal and spatial variations in the relative contribution of each organic matter source to POM pools may reflect their isotopic signatures (Fichez et al., 1993; Cresson et al., 2012).
The δ13C and δ15N ranges of POM as a tracer for the source identification have been reported for spatio-temporal variations in different lagoons under various environmental conditions (Table 2). In the present study, the δ13C values for POM during all sampling periods almost showed more positive at the Gyeongpoho sites (−24.2 ± 2.5‰) than at the Hyangho sites (−26.4 ± 0.6‰). These results may be explained by the difference in salinity distribution between the lagoons, showing that Hyangho had a much lower salinity than Gyeongpoho. The Hyangho sites were characterized by oligohaline conditions, whereas the Gyeongpoho sites exhibited a wide range of salinity from nearly freshwater to hypersaline seawater. At the Gyeongpoho sites, the POM composition may reflect the spatial variation in δ13C values with a diverse mixture of organic matter sources along the salinity gradient (Park et al., 2020). In contrast, the 13C-depletion of POM at the Hyangho sites may be explained by freshwater-dominated conditions resulting from the blocking of seawater intrusion by the presence of sand bars during most of the sampling periods. Such lower δ13C values suggested the prevalence of organic matter derived from terrestrial and marsh plants within the intermittently open lagoons. Several studies have reported that the connection or separation of the lagoon from the ocean by geomorphological characteristics can result in changes in the POM composition due to seasonal and spatial variations in the distribution and productivity of primary producers (Hoeinghaus et al., 2011; Escalas et al., 2015; Pelage et al., 2021). Thus, the isotopic difference in POM between the two lagoons is likely due to the differences in the mixing degree of seawater and freshwater by the geomorphology for physical connectivity with the adjacent coast.
Table 2 Previous results of δ13C and δ15N values for particulate organic matter in other lagoon ecosystems.
C/N ratios between 6 and 10 have been usually are characteristic of the predominance of phytoplanktonic cells in POM (Cifuentes et al., 1988; Cresson et al., 2012; Berto et al., 2013; Remeikaitė-Nikienė et al., 2017). Relatively high C/N ratios of more than 30 have been found in marsh vascular plants and terrestrial organic matter (Wainright et al., 2000; Kang et al., 2003). The relatively high C/N values for POM throughout the sampling period at all sites in Hyangho suggested the dominance of non-living detrital materials. In contrast, Gyeongpoho displayed different patterns in C/N values for POM among the within-lagoon sites. Freshwater input with terrestrial organic matter at the upper site of Gyeongpoho (St. Ga) may be responsible for the increase in C/N values for POM, which are similar to those at the Hyangho sites during the monsoon period. The high contribution of phytoplankton to the POM pool at the lower site (St. Gc) suggested that the intrusion of seawater mainly consisted of phytoplanktonic material owing to the permanently open system.
The biochemical composition of POM may be significantly influenced by environmental conditions such as salinity, temperature, light conditions, terrestrial inputs, and species composition of phytoplankton, which can allow us to distinguish phytoplankton production from refractory organic matter (Danovaro et al., 2000; Cresson et al., 2012; Lee et al., 2017). In the present study, the POM biochemical compositions in both lagoons displayed relatively high concentrations of PCHO compared with those of PPr and PLip. The dominance of PCHO is likely due to a major contribution of terrestrial matter to the POM pool, as shown by the lower δ13C values at most sampling sites, which may be linked to freshwater inputs from upstream. As terrestrial and marsh plant-derived organic matter is known to have very low δ13C values, ranging between −23‰ and −30‰ (Fry and Sherr, 1984; Park et al., 2020), the high concentrations of PCHO support this explanation. In addition, the presence of POM in PPr and PLip has been reported to increase the number of phytoplanktonic cells along with phytoplankton blooms (Pusceddu et al., 1996). Similar to the C/N values for POM, however, there were different patterns in the concentrations of biochemical components between the two lagoons. In Gyeongpoho, under the influence of freshwater and seawater, significant δ13C differences for POM among sites were observed, and relatively high concentrations of PCHO and PPr at the upper and lower sites appeared to be driven by the proximity of upstream and marine zones out of the lagoon, respectively. A comparable pattern was observed in the two different lagoon systems, where the existence of consistent connectivity between the freshwater wetland and marine systems was demonstrated to be the major contributor to the spatial differences in the isotopic and biochemical characteristics of POM.
In both lagoons, freshwater input following the monsoon period may have resulted in high nutrient concentrations. Despite the apparent evidence of rainfall effects (e.g., low salinity and high inorganic nutrients) on both lagoon systems, isotopic signatures and biochemical concentrations for POM showed seasonally different patterns between them. The enrichment in δ15N of POM during monsoon period are consistent with the general results for freshwater inputs influenced by anthropogenic nitrogen (McClelland et al., 1997), Seasonal pattern of the δ15N of POM in both systems may be explained by the differences in complex microbial processes by bacterial community and rapid interactions between nutrients and phytoplankton within each lagoon (Cifuentes et al., 1988). Our results suggested that seasonality in POM characteristics was clearer in Gyeongpoho than in Hyangho. At the Gyeongpoho site, the lowest δ13C values of POM were recorded immediately after the monsoon period, which may reflect the input of terrestrial organic matter by heavy rainfall (Riera and Richard, 1996; Berto et al., 2013). The input of terrestrial organic matter is known to increase the trophic contribution to consumer production at the upper site of Gyeongpoho, and macroalgal blooms caused by nutrient enrichment can also appear after the monsoon period (Park et al., 2020). For Gyeongpoho, the highly variable salinity was positively correlated with the δ13C values of POM. However, the lower site of Gyeongpoho is commonly characterized by organic matter transport from neighboring outer marine systems, as supported by the δ13C and C/N values of POM being close to those of typical marine phytoplankton, even during the monsoon period. In contrast, the isotopic variations for POM at the Hyangho sites showed little significance monthly, despite the occurrence of the heavy rainfall event. Although the POM composition may be linked with freshwater inputs during the monsoon period, this was mainly influenced by the presence of terrestrial and marsh plant-derived organic matter. In these environments, organic matter derived from primary producers such as seagrasses and terrestrial and marsh plants are generally known to mainly constitute high molecular weights of carbohydrates, which are difficult to use by consumer species, may be sustained throughout the seasons, and may reflect the isotopic and biochemical signatures of POM (Boudouresque et al., 2006; Cresson et al., 2012). These results can also be explained by the different systems open and semi-closed to the sea by distinguishing geomorphological features. Slightly more 13C-enriched POM values at the Hyangho sites following the monsoon period likely reflect the increased marine-derived organic matter transport via the intrusion of sea water when the lagoon inlet was opened due to a sustained flood pulse from heavy rainfall (Garcia et al., 2017; Park et al., 2020). Accordingly, the increase in terrestrial organic matter and the timing of inlet opening by heavy rainfall may be the main drivers of seasonal variation in POM characteristics in permanently and intermittently open systems, respectively.
This study provides a biogeochemical characterization of the seasonal and spatial dynamics of POM in permanently and intermittently open coastal lagoons of Korea using isotopic and biochemical tracers for the first time. The environmental conditions and POM compositions showed different seasonal and spatial patterns in the two lagoons during the sampling period. Our results estimated the seasonal and spatial differences in the POM composition influenced by the input of allochthonous materials (terrestrial and marine-derived organic matter) with respect to the geomorphological features of each lagoon. The degree of hydrological connectivity between freshwater and seawater may play an important role in determining the different POM pool compositions between permanently and intermittently open lagoon systems. The isotopic and biochemical signatures in the intermittently open lagoon suggested the prevalence of organic matter derived from terrestrial and marsh plants during most periods, while its temporary opening period (following the post-monsoon period) may provide organic matter transport from the sea. In the permanently open lagoon, these tracers provide evidence of the connectivity between lagoon systems and marine systems by the artificially constructed open inlet during most periods, and thereby, the highly transient pool of POM by the mixing degree of freshwater and seawater along the salinity gradient. Thus, these geomorphological characteristics may contribute to the contrasting biogeochemical responses of POM pools in the two lagoon systems to summer monsoon rainfall. Further studies are needed to assess the global climate effects due to the altered frequency and intensity of heavy rainfall events on the differential integration of POM pools within lagoon trophic networks, which may provide an integrated ecosystem-based management of temperate coastal lagoon systems.
The original contributions presented in the study are included in the article/Supplementary Material. Further inquiries can be directed to the corresponding author.
D-YL, JHK, and HJP designed the experiments and drafted the manuscript. D-YL and JHK carried out for field observation and sample collection. THP, HYK, and DK analyzed the biochemical and stable isotope compositions of POM samples. JHK and SH performed the statistical analyses. D-YL, JHK, and HJP wrote the main manuscript text. HJP supervised all processes. All authors contributed to the article and approved the submitted version.
This work was supported by the National Institute of Fisheries Science, Ministry of Oceans and Fisheries, Korea (R2022038) and the National Research Foundation of Korea grant (NRF-2018R1C1B6004501) funded by the Ministry of Science and ICT, Korea.
The authors declare that the research was conducted in the absence of any commercial or financial relationships that could be construed as a potential conflict of interest.
All claims expressed in this article are solely those of the authors and do not necessarily represent those of their affiliated organizations, or those of the publisher, the editors and the reviewers. Any product that may be evaluated in this article, or claim that may be made by its manufacturer, is not guaranteed or endorsed by the publisher.
Bănaru D., Harmelin-Vivien M., Gomoiu M. T., Onciu T. M. (2007). Influence of the Danube river inputs on c and n stable isotope ratios of the Romanian coastal waters and sediment (Black Sea). Mar. Pollut. Bull. 54, 1385–1394. doi: 10.1016/j.marpolbul.2007.05.022
Berto D., Rampazzo F., Noventa S., Cacciatore F., Gabellini M., Aubry F. B., et al. (2013). Stable carbon and nitrogen isotope ratios as tools to evaluate the nature of particulate organic matter in the Venice lagoon. Estuar. Coast. Shelf Sci. 135, 66–76. doi: 10.1016/j.ecss.2013.06.021
Bhavya P. S., Kim B. K., Jo N., Kim K., Kang J. J., Lee J. H., et al. (2019). A review on the macromolecular compositions of phytoplankton and the implications for aquatic biogeochemistry. Ocean Sci. J. 54, 1–14. doi: 10.1007/s12601-018-0061-8
Bligh E. G., Dyer W. J. (1959). A rapid method of total lipid extraction and purification. Can. J. Biochem. Physiol. 37, 911–917. doi: 10.1139/o59-099
Boudouresque C., Mayot N., Pergent G. (2006). The outstanding traits of the functioning of the posidonia oceanica seagrass ecosystem. Biol. Mar. Mediterr. 13, 109–113.
Briand M. J., Bonnet X., Goiran C., Guillou G., Letourneur Y. (2015). Major sources of organic matter in a complex coral reef lagoon: identification from isotopic signatures (δ13C and δ15N). PloS One 10, e0131555. doi: 10.1371/journal.pone.0131555
Carrasco Navas-Parejo J. C., Corzo A., Papaspyrou S. (2020). Seasonal cycles of phytoplankton biomass and primary production in a tropical temporarily open-closed estuarine lagoon–the effect of an extreme climatic event. Sci. Total Environ. 723. doi: 10.1016/j.scitotenv.2020.138014
Chen F., Lu X., Song Z., Huang C., Jin G., Chen C., et al. (2021). Coastal currents regulate the distribution of the particulate organic matter in western guangdong offshore waters as evidenced by carbon and nitrogen isotopes. Mar. Pollut. Bull. 172, 112856. doi: 10.1016/j.marpolbul.2021.112856
Cifuentes L. A., Sharp J. H., Fogel M. L. (1988). Stable carbon and nitrogen isotope biogeochemistry in the Delaware estuary. Limnol. Oceanogr. 33, 1102–1115. doi: 10.4319/lo.1988.33.5.1102
Cole M. L., Valiela I., Kroeger K. D., Tomasky G. L., Cebrian J., Wigand C., et al. (2004). Assessment of a δ15N isotopic method to indicate anthropogenic eutrophication in aquatic ecosystems. J. Environ. Qual. 33, 124–132. doi: 10.2134/jeq2004.1240
Cresson P., Ruitton S., Fontaine M. F., Harmelin-Vivien M. (2012). Spatio-temporal variation of suspended and sedimentary organic matter quality in the bay of Marseilles (NW Mediterranean) assessed by biochemical and isotopic analyses. Mar. Pollut. Bull. 64, 1112–1121. doi: 10.1016/j.marpolbul.2012.04.003
Danovaro R., Dell’Anno A., Pusceddu A., Daniela Marrale D., Della Croce N., Fabiano M., et al. (2000). Biochemical composition of pico-, nano- and micro-particulate organic matter and bacterioplankton biomass in the oligotrophic Cretan Sea (NE Mediterranean). Prog. Oceanogr. 46, 279–310. doi: 10.1016/S0079-6611(00)00023-9
Deegan L. A., Garritt R. H. (1997). Evidence for spatial variability in estuarine food webs. Mar. Ecol. Prog. Ser. 147, 31–47. doi: 10.3354/meps147031
Dubois M., Gilles K. A., Hamilton J. K., Rebers P. A., Smith F. (1956). Colorimetric method for determination of sugars and related substances. Anal. Chem. 28, 350–356. doi: 10.1021/ac60111a017
Escalas A., Ferraton F., Paillon C., Vidy G., Carcaillet F., Salen-Picard C., et al. (2015). Spatial variations in dietary organic matter sources modulate the size and condition of fish juveniles in temperate lagoon nursery sites. Estuar. Coast. Shelf Sci. 152, 78–90. doi: 10.1016/j.ecss.2014.11.021
Fichez R., Dennis P., Fontaine M. F., Jickells T. D. (1993). Isotopic and biochemical composition of particulate organic matter in a shallow water estuary (Great ouse, north Sea, England). Mar. Chem. 43, 263–276. doi: 10.1016/0304-4203(93)90231-C
Fry B., Sherr E. (1984). δ13C measurements as indicators of carbon flow in marine and freshwater ecosystems. Contribution Mar. Sci. 27, 13–47.
Garcia C. A., Baer S. E., Garcia N. S., Rauschenberg S., Twining B. S., Lomas M. W., et al. (2018). Nutrient supply controls particulate elemental concentrations and ratios in the low latitude eastern Indian ocean. Nat. Commun. 9, 1–10. doi: 10.1038/s41467-018-06892-w
Garcia A. M., Winemiller K., Hoeinghaus D., Claudino M., Bastos R., Correa F., et al. (2017). Hydrologic pulsing promotes spatial connectivity and food web subsidies in a subtropical coastal ecosystem. Mar. Ecol. Prog. Ser. 567, 17–28. doi: 10.3354/meps12060
Grémare A., Amouroux J. M., Charles F., Dinet A., Riaux-Gobin C., Baudart J., et al. (1997). Temporal changes in the biochemical composition and nutritional value of the particulate organic matter available to surface deposit-feeders: a two year study. Mar. Ecol. Prog. Ser. 150, 195–206. doi: 10.3354/meps150195
Hansen H. P., Koroleff F. (1999). “Determination of nutrients,” in Methods of seawater analysis. Eds. Grasshoff K., Kremling K., Ehrhardt M. (Weinheim: Wiley-VCH Verlag), 159–228.
Helder W., De Vries R. T. P. (1979). An automatic phenol-hypochlorite method for the determination of ammonia in sea- and brackish waters. Neth. J. Sea Res. 13, 154–160. doi: 10.1016/0077-7579(79)90038-3
Hoeinghaus D. J., Vieira J. P., Costa C. S., Bemvenuti C. E., Winemiller K. O., Garcia A. M. (2011). Estuary hydrogeomorphology affects carbon sources supporting aquatic consumers within and among ecological guilds. Hydrobiologia. 673, 79–92. doi: 10.1007/s10750-011-0751-z
Kang C.-K., Kim J. B., Lee K.-S., Kim J. B., Lee P.-Y., Hong J.-S. (2003). Trophic importance of benthic microalgae to macrozoobenthos in coastal bay systems in Korea: Dual stable c and n isotope analyses. Mar. Ecol. Prog. Ser. 259, 79–92. doi: 10.3354/meps259079
Ke Z., Tan Y., Huang L., Zhao C., Jiang X. (2017). Spatial distributions of δ13C, δ15N and C/N ratios in suspended particulate organic matter of a bay under serious anthropogenic influences: Daya bay, China. Mar. Pollut. Bull. 114, 183–191. doi: 10.1016/j.marpolbul.2016.08.078
Kjerfve B. (1994). “Coastal lagoons,” in Coastal lagoon processes. Ed. Kjerfve B. (Amsterdam: Elsevier), 1–8. doi: 10.1016/s0422-9894(08)70006-0
Kleppel G. S., Burkart C. A. (1995). Egg production and the nutritional environment of acartia tonsa: The role of food quality in copepod nutrition. ICES J. Mar. Sci. 52, 297–304. doi: 10.1016/1054-3139(95)80045-X
Kohonen T. (1982). Self-organized formation of topologically correct feature maps. Biol. Cybern. 43, 59–69. doi: 10.1007/BF00337288
Lønborg C., Doyle J., Furnas M., Menendez P., Benthuysen J. A., Carreira C. (2017). Seasonal organic matter dynamics in the great barrier reef lagoon: contribution of carbohydrates and proteins. Contin. Shelf Res. 138, 95–105. doi: 10.1016/j.csr.2017.01.010
Lee J. H., Lee D., Kang J. J., Joo H. T., Lee J. H., Lee H. W., et al. (2017). The effects of different environmental factors on the biochemical composition of particulate organic matter in gwangyang bay, south Korea. Biogeosciences. 14, 1903–1917. doi: 10.5194/bg-14-1903-2017
Licen S., Franzon M., Rodani T., Barbieri P. (2021). SOMEnv: an r package for mining environmental monitoring datasets by self-organizing map and k-means algorithms with a graphical user interface. Microchem. J. 165, 106181. doi: 10.1016/j.microc.2021.106181
Lopes C. L., Dias J. M. (2015). Assessment of flood hazard during extreme sea levels in a tidally dominated lagoon. Nat. Hazards 77, 1345–1364. doi: 10.1007/s11069-015-1659-0
Lowry O. H., Rosebrough N. J., Farr A. L., Randall R. J. (1951). Protein measurement with the folin phenol reagent. J. Biol. Chem. 193, 265–275. doi: 10.1016/S0021-9258(19)52451-6
Lu X., Huang C., Chen F., Zhang S., Lao Q., Chen C., et al. (2021). Carbon and nitrogen isotopic compositions of particulate organic matter in the upwelling zone off the east coast of hainan island, China. Mar. Pollut. Bull. 167, 112349. doi: 10.1016/j.marpolbul.2021.112349
Lu X., Zhou X., Jin G., Chen F., Zhang S., Li Z., et al. (2022). Biological impact of typhoon wipha in the coastal area of western guangdong: A comparative field observation perspective. J. Geophys. Res.-Biogeosci 127 (2), e2021JG006589. doi: 10.1029/2021JG006589
Machás R., Santos R. (1999). Sources of organic matter in ria Formosa revealed by stable isotope analysis. Acta Oecol. 20, 463–469. doi: 10.1016/S1146-609X(99)00122-8
Marsh J. B., Weinstein D. B. (1966). Simple charring method for determination of lipids. J. Lipid Res. 7, 574–576. doi: 10.1016/S0022-2275(20)39274-9
Mazzola A., Fabiano M., Pusceddu A., Sarà G. (2001). Particulate organic matter composition in a semi-enclosed marine system. Chem. Ecol. 17, 315–334. doi: 10.1080/02757540108035561
McClelland J. W., Valiela I. (1998). Linking nitrogen in estuarine producers to land-derived sources. Limnol. Oceanogr. 43, 577–585. doi: 10.4319/lo.1998.43.4.0577
McClelland J. W., Valiela I., Michener R. H. (1997). Nitrogen stable isotope signatures in estuarine food webs: A record of increasing urbanization in coastal watersheds. Limnol. Oceanogr. 42, 930–937. doi: 10.4319/lo.1997.42.5.0930
Mukherjee R., Muduli P. R., Barik S. K., Kumar S. (2019). Sources and transformations of organic matter in sediments of asia’s largest brackish water lagoon (Chilika, India) and nearby mangrove ecosystem. Environ. Earth Sci. 78, 332. doi: 10.1007/s12665-019-8329-6
Murphy J., Riley J. P. (1962). A modified single solution method for the determination of phosphate in natural waters. Anal. Chim. Acta. 27, 31–36. doi: 10.1016/S0003-2670(00)88444-5
Navarro J., Thompson R. (1995). Seasonal fluctuations in the size spectra, biochemical composition and nutritive value of the seston available to a suspension-feeding bivalve in a subarctic environment. Mar. Ecol. Prog. Ser. 125, 95–106. doi: 10.3354/meps125095
Park Y.-S., Céréghino R., Compin A., Lek S. (2003). Applications of artificial neural networks for patterning and predicting aquatic insect species richness in running waters. Ecol. Modell. 160, 265–280. doi: 10.1016/S0304-3800(02)00258-2
Park H. J., Han E., Lee Y. J., Kang C. K. (2016). Trophic linkage of a temperate intertidal macrobenthic food web under opportunistic macroalgal blooms: A stable isotope approach. Mar. Pollut. Bull. 111, 86–94. doi: 10.1016/j.marpolbul.2016.07.026
Park H. J., Kwak J. H., Kang C.-K. (2015). Trophic consistency of benthic invertebrates among diversified vegetational habitats in a temperate coastal wetland of Korea as determined by stable isotopes. Estuaries Coast. 38, 599–611. doi: 10.1007/s12237-014-9834-1
Park H. J., Park T. H., Kang H. Y., Lee C. I., Kang C. K. (2020). Shifting resource utilization of the lacustrine shrimp palaemon paucidens in temperate coastal lagoons of Korea as revealed by stable isotopes. Mar. Pollut. Bull. 160, 111672. doi: 10.1016/j.marpolbul.2020.111672
Park Y.-S., Tison J., Lek S., Giraudel J.-L., Coste M., Delmas F. (2006). Application of a self-organizing map to select representative species in multivariate analysis: A case study determining diatom distribution patterns across France. Ecol. Inform. 1, 247–257. doi: 10.1016/j.ecoinf.2006.03.005
Parsons T. R., Maita Y., Lalli C. M. (1984). A manual of chemical & biological methods for seawater analysis. (Amsterdam: Pergamon Press).
Pelage L., Gonzalez J. G., Le Loc'h. F., Ferreira V., Munaron J.-M., Lucena-Fredou F., et al. (2021). Importance of estuary morphology for ecological connectivity with their adjacent coast: A case study in Brazilian tropical estuaries. Estuar. Coast. Shelf Sci. 251, 107184. doi: 10.1016/j.ecss.2021.107184
Pérez-Ruzafa A., Marcos C., Pérez-Ruzafa I. M., Pérez-Marcos M. (2011). Coastal lagoons: “Transitional ecosystems” between transitional and coastal waters. J. Coast. Conserv. 15, 369–392. doi: 10.1007/s11852-010-0095-2
Pérez-Ruzafa A., Pérez-Ruzafa I. M., Newton A., Marcos C. (2019). “Coastal lagoons: environmental variability, ecosystem complexity, and goods and services uniformity,” in Coasts and estuaries, the future. Eds. Wolanski E., Day J. W., Elliott M., Ramesh R. (New York: Elsevier), 253–276. doi: 10.1016/b978-0-12-814003-1.00015-0
Peterson B. J., Fry B. (1987). Stable isotopes in ecosystem studies. Annu. Rev. Ecol. Syst. 18, 293–320. doi: 10.1146/annurev.es.18.110187.001453
Pusceddu A., Serra E., Sanna O., Fabiano M. (1996). Seasonal fluctuations in the nutritional value of particulate organic matter in a lagoon. Chem. Ecol. 13, 21–37. doi: 10.1080/02757549608039099
Remeikaitė-Nikienė N., Lujanienė G., Malejevas V., Barisevičiūtė R., Zilius M., Vybernaitė-Lubienė I., et al. (2017). Assessing nature and dynamics of POM in transitional environment (the curonian lagoon, SE Baltic Sea) using a stable isotope approach. Ecol. Indic. 82, 217–226. doi: 10.1016/j.ecolind.2017.06.035
Riera P., Richard P. (1996). Isotopic determination of food sources ofCrassostrea gigasAlong a trophic gradient in the estuarine bay of marennes-ol éron. Estuar. Coast. Shelf Sci. 42, 347–360. doi: 10.1006/ecss.1996.0023
Rossi S., Grémare A., Gili J.-M., Amouroux J.-M., Jordana E., Vétion G. (2003). Biochemical characteristics of settling particulate organic matter at two north-western Mediterranean sites: a seasonal comparison. Estuar. Coast. Shelf Sci. 58, 423–434. doi: 10.1016/S0272-7714(03)00108-2
Sundbäck K., Snoeijs P. (1991). Effects of nutrient enrichment on microalgal community composition in a coastal shallow-water sediment system: An experimental study. Botanica Marina. 34:341–358. doi: 10.1515/botm.1991.34.4.341
Ter Braak C. J. F. (1986). Canonical correspondence analysis: a new eigenvector technique for multivariate direct gradient analysis. Ecology. 67, 1167–1179. doi: 10.2307/1938672
Vesanto J., Alhoniemi E. (2000). Clustering of the self-organizing map. IEEE Trans. Neural Netw. 11, 586–600. doi: 10.1109/72.846731
Vizzini S., Mazzola A. (2003). Seasonal variations in the stable carbon and nitrogen isotope ratios (13C/12C and 15N/14N) of primary producers and consumers in a western Mediterranean coastal lagoon. Mar. Biol. 142, 1009–1018. doi: 10.1007/s00227-003-1027-6
Vizzini S., Mazzola A. (2006). Sources and transfer of organic matter in food webs of a Mediterranean coastal environment: Evidence for spatial variability. Estuar. Coast. Shelf Sci. 66, 459–467. doi: 10.1016/j.ecss.2005.10.004
Vizzini S., Savona B., Chi T. D., Mazzola A. (2005). Spatial variability of stable carbon and nitrogen isotope ratios in a Mediterranean coastal lagoon. Hydrobiologia. 550, 73–82. doi: 10.1007/s10750-005-4364-2
Wainright S. C., Weinstein M. P., Able K. W., Currin C. A. (2000). Relative importance of benthic microalgae, phytoplankton and the detritus of smooth cordgrass spartina alterniflora and the common reed phragmites australis to brackish-marsh food webs. Mar. Ecol. Prog. Ser. 200, 77–91. doi: 10.3354/meps200077
WREO (2008). The study of ecosystem scrutiny and management for ecosystem conservation and restoration of lagoons on the East coast (I). (Wonju: Wonju Regional Environmental Office).
Keywords: Lagoons, suspended particulate organic matter, stable isotopes, biochemical composition, hydrologic connectivity
Citation: Lee D-Y, Kwak JH, Park TH, Kang HY, Kim D, Hwang S and Park HJ (2022) Seasonal variations in biochemical and stable isotope compositions of particulate organic matter in two contrasting temperate coastal lagoons of Korea. Front. Mar. Sci. 9:953648. doi: 10.3389/fmars.2022.953648
Received: 26 May 2022; Accepted: 07 September 2022;
Published: 26 September 2022.
Edited by:
Ana Carolina Ruiz-Fernández, National Autonomous University of Mexico, MexicoReviewed by:
Peng Zhang, Guangdong Ocean University, ChinaCopyright © 2022 Lee, Kwak, Park, Kang, Kim, Hwang and Park. This is an open-access article distributed under the terms of the Creative Commons Attribution License (CC BY). The use, distribution or reproduction in other forums is permitted, provided the original author(s) and the copyright owner(s) are credited and that the original publication in this journal is cited, in accordance with accepted academic practice. No use, distribution or reproduction is permitted which does not comply with these terms.
*Correspondence: Hyun Je Park, cGhqMTM1NzlAZ3dudS5hYy5rcg==
†These authors have contributed equally to this work
Disclaimer: All claims expressed in this article are solely those of the authors and do not necessarily represent those of their affiliated organizations, or those of the publisher, the editors and the reviewers. Any product that may be evaluated in this article or claim that may be made by its manufacturer is not guaranteed or endorsed by the publisher.
Research integrity at Frontiers
Learn more about the work of our research integrity team to safeguard the quality of each article we publish.