- 1College of Life Sciences, Qingdao Agricultural University, Qingdao, China
- 2CAS Key Laboratory of Marine Ecology and Environmental Sciences, Institute of Oceanology, Chinese Academy of Sciences, Qingdao, China
- 3CAS Engineering Laboratory for Marine Ranching, Institute of Oceanology, Chinese Academy of Sciences, Qingdao, China
- 4Laboratory for Marine Ecology and Environmental Science, Qingdao National Laboratory for Marine Science and Technology, Qingdao, China
- 5Center for Ocean Mega-Sciences, Chinese Academy of Sciences, Qingdao, China
- 6Shandong Province Key Laboratory of Experimental Marine Biology, Qingdao, China
- 7Laizhou Municipal Bureau of Marine Development And Fisheries, Yantai, China
The sea cucumber (Apostichopus japonicus) is the main economic species in China and has a significant role in aquaculture. Gut microbiome composition is closely related to external environments. In this study, we identified the effects of seasonal changes on the composition and main metabolites of symbiotic microorganisms in the intestine of A. japonicus. We used 16S rRNA sequencing to identify the composition of symbiotic microorganisms in different seasons. Intestinal metabolites were determined using liquid chromatography with tandem mass spectrometry, which linked symbiotic microorganisms to intestinal metabolites. Analyzing changes in intestinal microbial composition across different seasons. The results showed that seasonal changes of intestinal microorganisms were significant, A. japonicus were infected by Vibrio easily in summer, Stigmasterol and sitosterol could affect the growth of body wall of A. japonicus. It is vital importance for A. japonicus that the results benefit for the growth, immunity and aquaculture.
Introduction
The sea cucumber (Apostichopus japonicus) is highly favored by Asian and Chinese populations due to its unique flavor and taste. The aquaculture of sea cucumbers has significantly increased in recent years, and has become a major aquaculture species in China, generating huge revenues for the aquaculture industry (Ru et al., 2019). Sea cucumbers mainly ingest sediment to extract organic matter and microorganisms (Xu et al., 2015; Mohsen et al., 2018) and are colloquially known as “Ocean scavengers”, therefore, they have crucial roles in marine ecosystems. The diet of sea cucumber is closely related to the variation of environment, so, the intestinal contents of sea cucumbers can reflect ecosystem characteristics and environmental changes.
Sea cucumber activities are closely related to the seasons. As a temperate species, spring and autumn are fast-growing seasons when the water temperature is 10–20°C. Thus, feeding rates increase and are concomitant with increased body weight. Aestivation begins when the water temperature reaches 20.0–24.5°C. During aestivation, sea cucumbers stop eating and intestinal degeneration occurs and activities slow down (Mitsukuri, 1903; Tanaka, 1958a; Tanaka, 1958b; Yang et al., 2015). In autumn, water temperatures are decreased and feeding activity returns to normal. In winter, water temperatures are very low and activity, growth, and metabolism of sea cucumber are decreased.
Intestinal microbiotas are associated with all aspects of host health, including metabolism and digestion, the immune system, and even behavior, while disorders or abnormal intestinal microbiota development are related to different gastrointestinal and metabolic diseases (Spor et al., 2011). The gut houses an enormous variety of microbial communities with important roles in host metabolism and immunity (Egerton et al., 2018). The gut microbiome is essential for host health and physiological functions and has important roles enhancing host digestion, regulating gut endocrine functions and neurological signaling, and maintaining host reproductive capacity (Lynch and Pedersen, 2016; Fan and Pedersen, 2021). In particular, the microbiome performs functions that the host cannot perform, including the production and regulation of metabolites which are used as metabolic substrates and signaling molecules, with major impact on host metabolism and health (Schoeler and Caesar, 2019). Thus, gut microbiome variations may lead to metabolite alterations, such as metabolites are the end products of many cell regulatory processes, significant microbiome alterations can impact responses to the external environment (Fiehn, 2020; Xing et al., 2021). Thus, joint microbiome and metabolome studies are required to evaluate and characterize host-microbiome interactions (Turnbaugh and Gordon, 2008; Visconti et al., 2019).
The gut microbiome is highly plastic and is altered throughout life by environmental factors (Goodrich et al., 2014; Goodrich et al., 2016; Xie et al., 2016; Visconti et al., 2019). Microorganisms in organism have critical roles; however, relatively few studies have focused on intestinal microorganism and metabolite links and their relationship with seasonal changes, therefore, we investigated sea cucumber microbiota and metabolism across different seasons using 16S rRNA and liquid chromatography with tandem mass spectrometry (LC-MS/MS). Additionally, using Kyoto Encyclopedia of Genes and Genomes (KEGG) resources, we analyzed different molecular pathways to explore metabolic differences. Our aims were to; (1) identify seasonal variations of intestinal microorganisms, (2) examine the effects of seasonal variations of sea cucumber microbiota and metabolite on immune of sea cucumbers, (3) explore the effects of seasonal variations of sea cucumber microbiota and metabolite on growth of sea cucumbers.
Materials and method
Experimental animals
Sea cucumbers were collected from Rongcheng Swan Lake (Weihai City, Shandong Province, China, 122°59’E, 37°36’N) in November, 2019 (autumn, the seawater temperature was 16.8°C); January, 2020 (winter, the seawater temperature was 1.6°C); April, 2020 (spring, the seawater temperature was 14.1°C); and June, 2020 (summer, the seawater temperature was 23.2°C). Sea cucumbers were weighed approximately 150–200 g. Sea cucumbers were euthanized by decollation, the whole sampling process was aseptic. The intestinal contents from ten individuals, during each season, (40 individuals in total), were used for sampling, 16S rRNA sequencing, and metabolomic analysis. Animals were frozen in liquid nitrogen and stored at −80°C.
Microbial 16S rRNA sequencing and analysis
Microbial DNA was extracted using HiPure Stool DNA Kits (Magen, Guangzhou, China) according to manufacturer’s protocols. The 16S rDNA hypervariable V3–V4 region was amplified by polymerase chain reaction (PCR) (95°C for 2 min, followed by 35 cycles of 95°C for 30 s, 60°C for 45 s, and 72°C for 90 s, with a final extension of 72°C for 10 min) using primers 341F (5’-CCTACGGGNGGCWGCAG-3’) and 806 R (5’-GGACTACHVGGGTATCTAAT-3’). PCR was performed in 50 μL reaction volumes and contained TransGen High-Fidelity PCR SuperMix (TransGen Biotech, Beijing, China), 0.2 μM forward and reverse primers, and 5 ng template DNA.
Clean tags were clustered into operational taxonomic units (OTUs) at ≥ 97% similarity using the UPARSE pipeline (version 9.2.64). Chimeric tags were removed using the UCHIME algorithm to generate effective tags for further analysis. Tag sequences with the highest abundance were selected. Taxonomic classifications were performed in BLAST (version 2.6.0) (Altschul et al., 1990) and representative OTU sequences were searched against the National Center for Biotechnology Information 16S ribosomal RNA database (http://www.ncbi.nlm.nih.gov) (version 202101) for cluster analysis.
Metabolite profiling
Approximately 50 mg intestinal contents were weighed into a clean microtube. After, 1000 μL extract solvent (acetonitrile-methanol-water in a 2:2:1) was added, the sample was vortexed for 30 s, homogenized at 45 Hz for 4 min, and sonicated for 5 min in an ice-water bath. The homogenate and sonicate circle were repeated three times, followed by incubation for 1 hour at −20°C, and centrifugation at 12000 rpm for 15 min at 4°C. The supernatant was transferred to LC-MS/MS vials and stored at −80°C until MS analysis.
LC-MS/MS analyses were performed using an UHPLC system (1290, Agilent Technologies) on a UPLC HSS T3 column (2.1 mm × 100 mm, 1.8 μm) coupled to a Q Exactive system (Orbitrap MS, Thermo). Mobile phase A comprised 0.1% formic acid in water for positive, and 5 mmol/L ammonium acetate in water for negative. Mobile phase B was acetonitrile. MS raw data (.raw) files were converted to mzML format using ProteoWizard, processed in R package XCMS (version 3.2), and included retention time alignment, peak detection, and peak matching. Data were then filtered using the following criterion: sample numbers contain a metabolite was less than 50% all sample numbers in a group. Normalization to an internal standard (Roberts et al., 2012) for each sample was also performed.
Statistical analysis
Microbiota analyses incorporated abundance, species, α-diversity indices and LEFSe. The abundance statistics of each taxonomy was visualized using Krona (Cheung et al., 2010) (version 2.6). Chao1 and Shannon indices were calculated in QIIME (Caporaso et al., 2010) (version 1.9.1). Species comparison between groups was calculated by welch’s t-test in R project Vegan package (version 2.5.3). Biomarker features in each group were screened by LEFSe software (version 1.0), taking Linear Discriminant Analysis (LDA)>3 as a threshold.
For metabolomic analyses, we performed principal component analysis (PCA), analyzed KEGG pathways. T-tests were used as a univariate analysis for screening differential metabolites. P values from t-tests < 0.05 and VIP ≥ 1 were considered differential metabolites between two groups. Metabolites were mapped to KEGG pathways for pathway and enrichment analysis. The calculated p-value was gone through FDR correction, taking FDR ≤ 0.05 as a threshold. Pathways meeting these conditions were defined as significantly enriched for differential metabolites.
Results
Gut microbial profiles of A. japonicus across different seasons
Gut microbiota composition varied greatly with seasons. The dominant phyla were Planctomycetes and Proteobacteria, as the results shown, these phyla are complementary (Figures 1A, B). It’s worth noting that it is higher than other seasons that the relative abundance of Cyanobacteria, Verrucomicrobia and Bacteroidetes in spring (Figure 1B). According to the PCA which revealed the relationship of intestinal microbes among different seasons, the results showed that the compositions of autumn and winter are similar, however, the compositions among spring, summer and autumn are different significantly (Figure 1C).
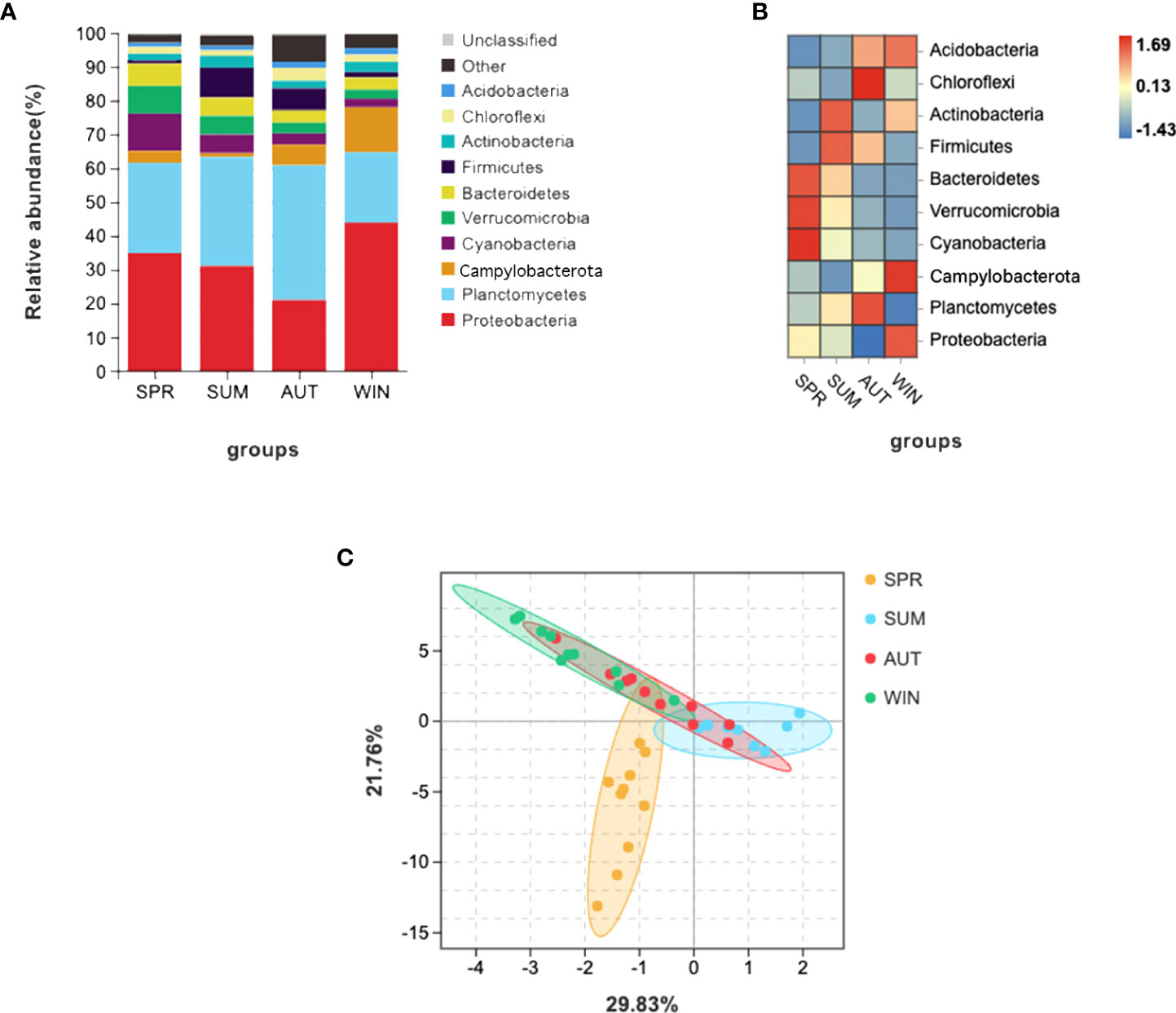
Figure 1 16S RNA summary and principal component analysis (PCA) of gut microbiota in A. japonicus. (A) Relative abundance (%) of the main phyla in the gut microbiota. (B) Heatmap showing the dynamic abundance of gut microbiota from A. japonicus across different seasons. (C) PCA of OTU levels.
The statistics results showed that four phyla of intestinal microbiota were significantly different in spring and summer, and four phyla were significantly different in autumn and summer (Figures 2A, B). Notably, the mean abundance of Proteobacteria was higher in summer than in autumn. Thus, LEFSe analysis further on this phenomenon between summer and autumn. Then, we found that Vibrio populations spike in summer, significantly higher than in autumn (Figure 3).
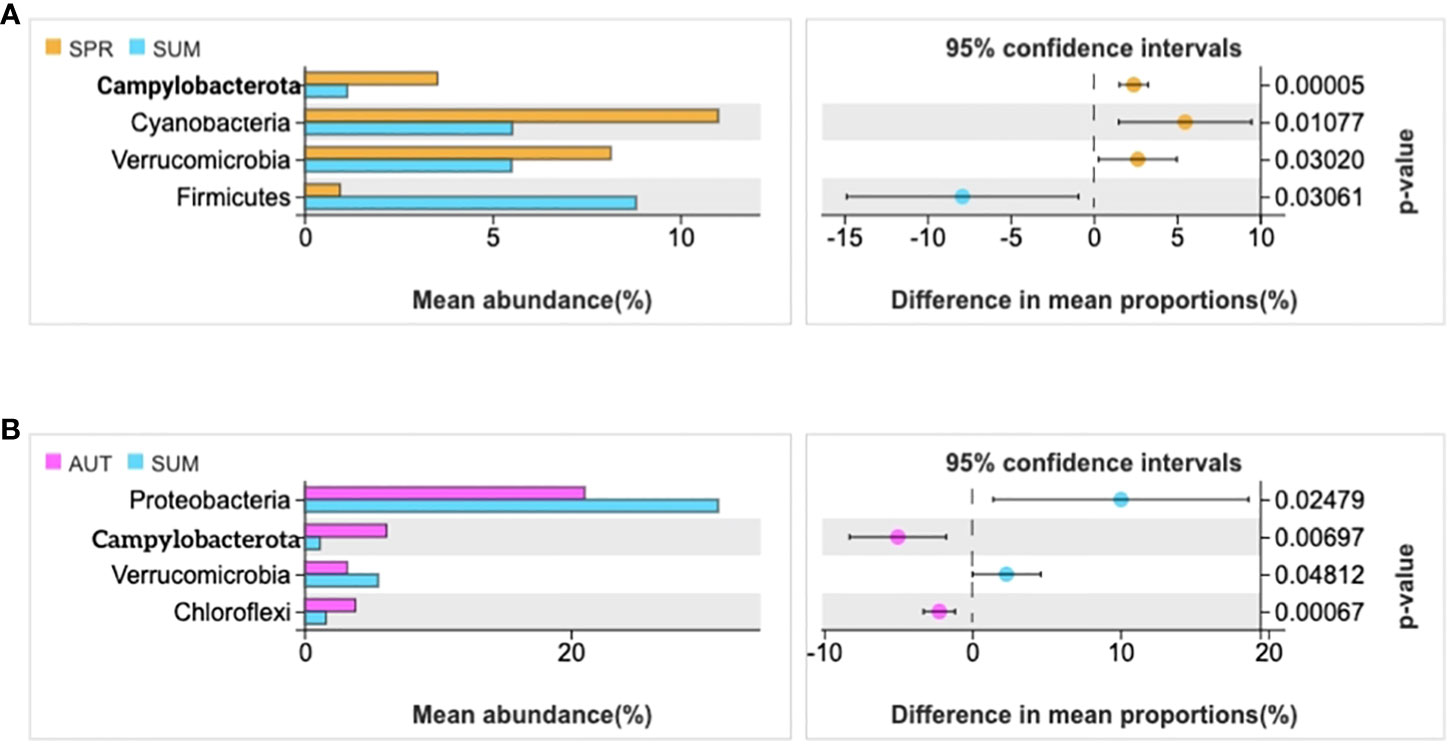
Figure 2 Statistical examination of intestinal microbial diversity of A. japonicus across different seasons (P ≤ 0.05). (A) the statistical examination between spring and summer. (B) the statistical examination between summer and autumn.
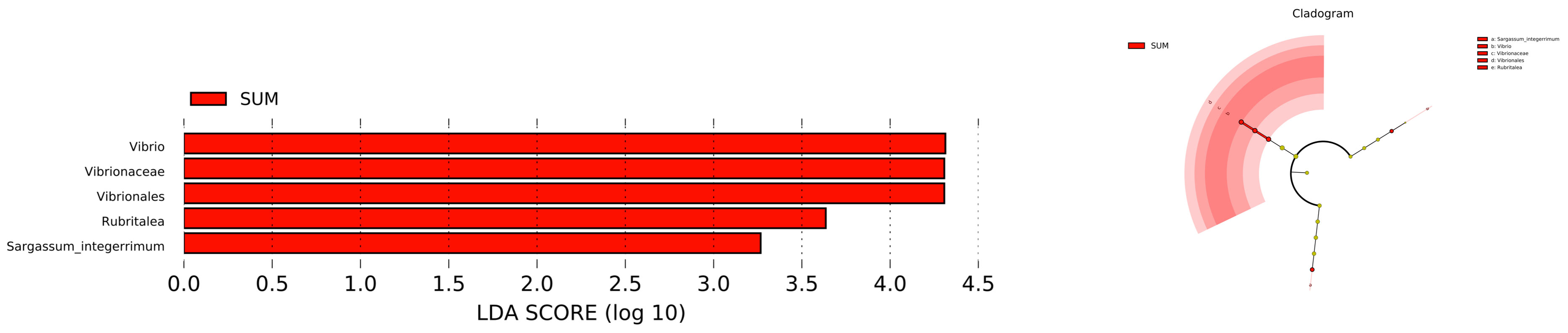
Figure 3 LEFSe analyzed the species of gut microbiota of A. japonicus between summer and autumn (LDA>3).
Gut microbial diversity in different seasons
Alpha-diversity indices reflected the diversity and richness of symbiotic microbiota in each season, incorporated Chao1 and Shannon indices. Chao1 indices reflect species abundance in samples; thus, symbiotic microbiota abundance was poor in summer, (Figure 4A). Simpson indices reflect species richness, we can learn that the highest richness was in autumn, but the richness was relatively low in spring (Figure 4B). The phenomenon of richness has significant seasonal trends. It rises from spring to autumn, and then falls until the following spring.
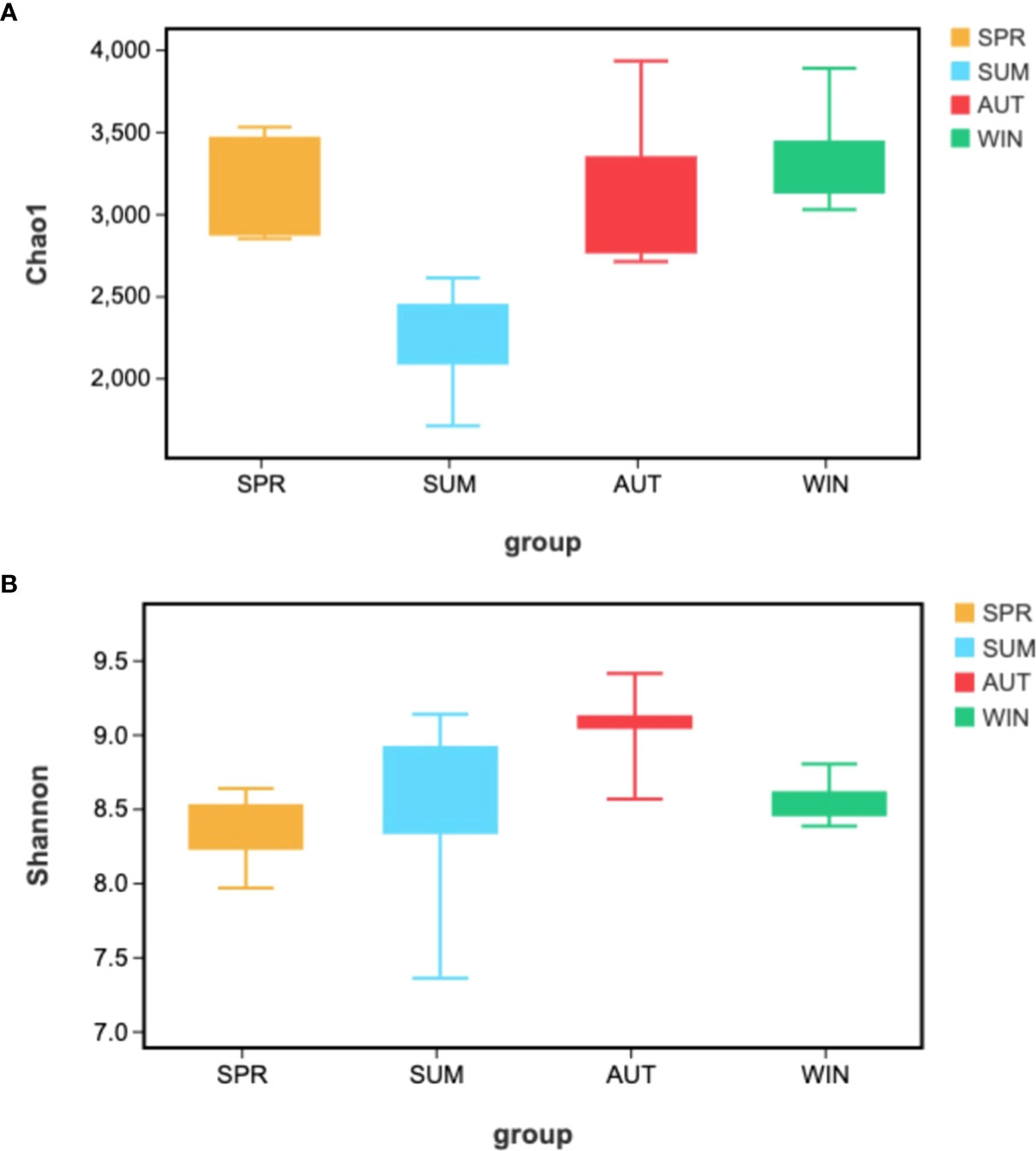
Figure 4 Kruskal-Walli’s rank sum test analyzed Alpha-diversity difference analysis of gut microbiota from A. japonicus across different seasons by (A) Chao1, (B) Shannon.
Metabolite variations across different seasons
To further understand gut microbe metabolic interactions, LC-MS/MS was used for metabolic profiling. Among the 26,136 identified metabolites, 13,116 were tested in positive ion mode (POS) and 13,020 in negative ion mode (NEG). From PCA, we could learn that the relationships among seasons (Figure 5). It is interesting that these PCA results are similar with the PCA analysis of microbiota. The principal component of metabolites of autumn and winter are similar, however, the principal component of metabolites among spring, summer and autumn are different significantly.
Differential metabolites were further analyzed using KEGG annotations (VIP > 1, P < 0.05). We analyzed three secondary metabolic pathways: amino acid, carbohydrate, and lipid metabolism between summer and spring, and these pathway between summer and autumn (Figure 6). Our results showed that protein digestion and absorption exhibited the most significant differences between summer and autumn (Figure 6). Pathways of biosynthesis of unsaturated fat acids, primary bile acid biosynthesis, and mineral absorption were more significant, while sulfur metabolism exhibited the most significant differences between summer and spring (Figure 7). We also analyzed differences in metabolites among summer, spring, and autumn. Metabolites in summer were significantly higher than those in spring and autumn and mainly included benzene and substituted derivatives (phenylacetaldehyde, L-formylkynurenine, and phenylethylamine), steroids and steroid derivatives (cholesterol sulfate), carboxylic acids and derivatives (L-isoleucine), and glycerophospholipids (lysoPC).
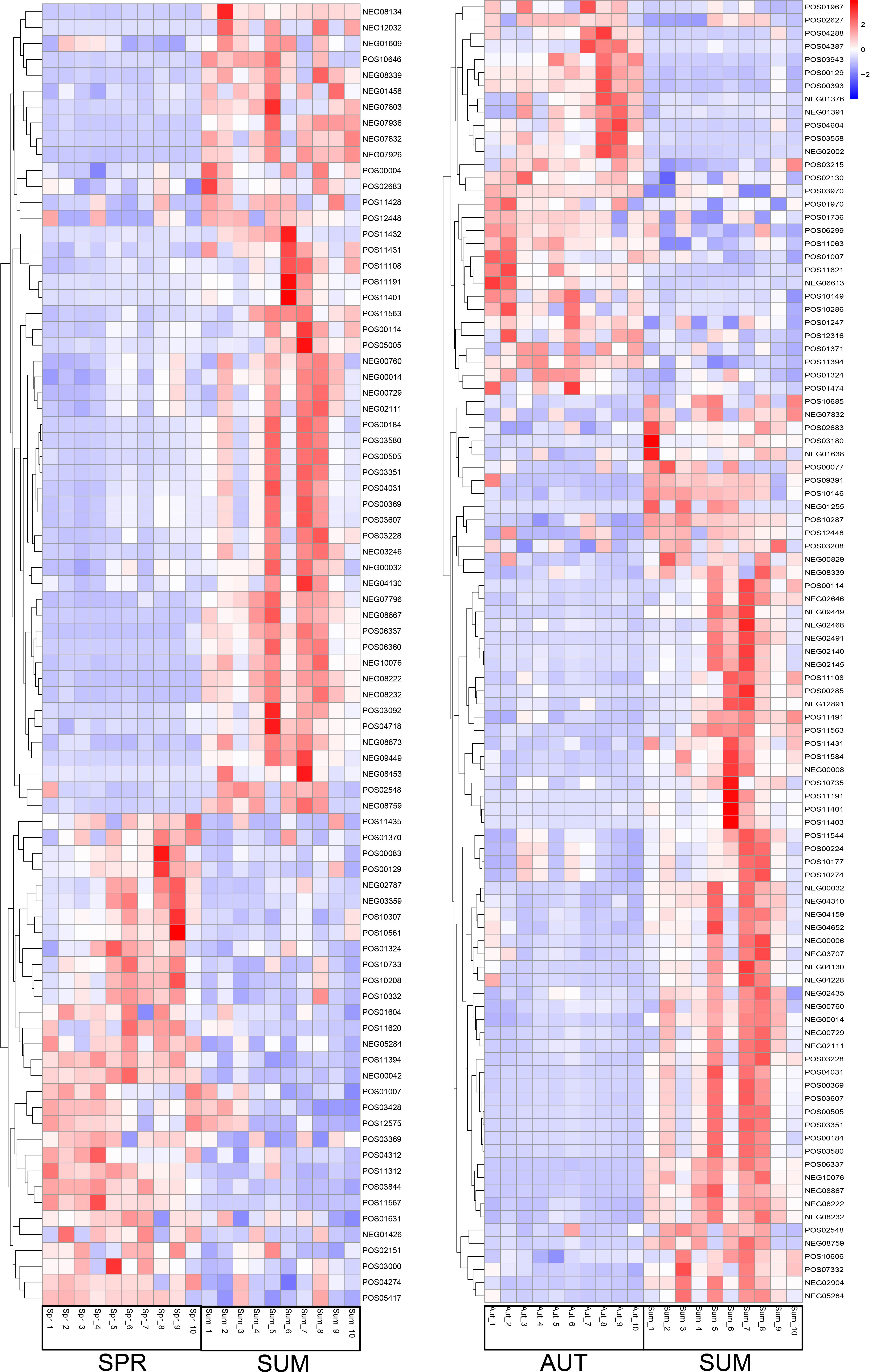
Figure 6 Partial metabolite heatmap of A. japonicus across different seasons between spring and summer (left), between autumn and summer (right).
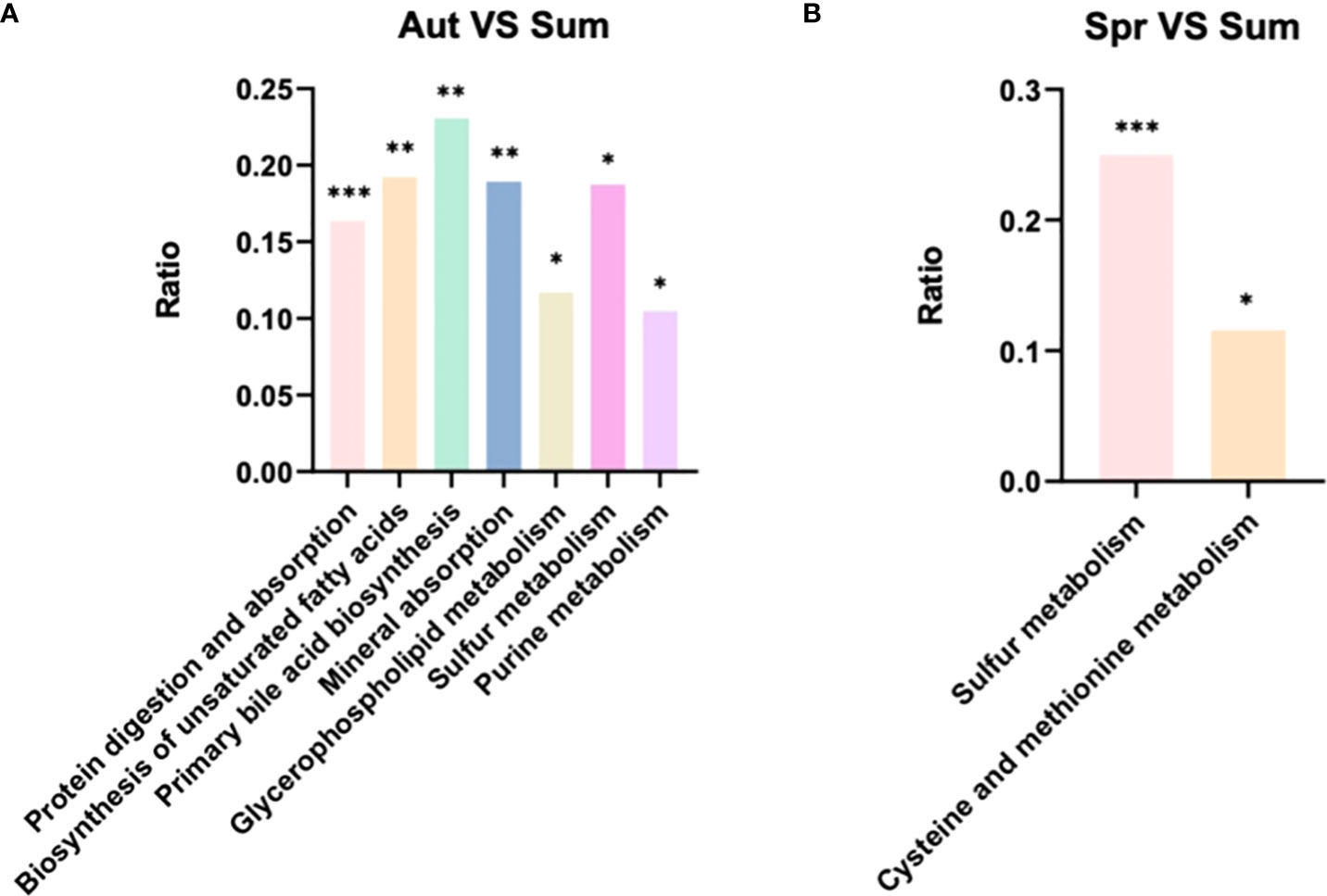
Figure 7 KEGG pathway analysis of level 3 differential metabolic pathways (A) between autumn and summer and (B) spring and summer.
Discussion
Variations in gut microbiota in A. japonicus across different seasons
According to the results, we find that the microbiota has seasonal variations in the gut of A. japonicus, and traits are significant. Variations of spring, summer and autumn are obviously different. According to Chao1 index, the richness in samples of species is lowest in summer and increased gradually from autumn. It is closely that this phenomenon related to the life history of A. japonicus. The reason is that A. japonicus entered aestivation in summer, the intestine degenerated, stop feeding, thus, the microbial contents in intestinal tract decrease sharply (Mitsukuri, 1903; Tanaka, 1958a; Tanaka, 1958b; Yang et al., 2015). In autumn, A. japonicus recovered feeding, thus, the abundance of gut microbiota increased. According to the Shannon index, the seasonal variations of intestinal microbial richness were more obvious, it gradually increased from spring to autumn and began to decrease after reaching its maximum in autumn. The change trend of Shannon index is opposite to Chao1, this is the same as the change trend of microorganisms in the environment. This trend maybe relates to the change of sea water nutrient. According to previous research in Weihai sea area (Shandong Province, China), the content of inorganic nitrogen (DIN) in summer is lower than in spring and autumn, DIN changes were positively correlated with Chao1 changes, the N/P of nutrients in autumn is higher than summer and spring (Fang et al., 2012), which is positively correlated with Shannon index. The change trend of microbe in the intestine of A. japonicus is consistent with the change of nutrient in the environment. Microbial richness in the gut of the sea cucumber was highest in the autumn, due to the high nutrient composition in seawater in autumn, which promoted the growth of a variety of microbiota (Bunse and Pinhassi, 2017).
From our analysis of intestinal microbial diversity across different seasons, we observed Proteobacteria maintained dominant positions. Proteobacteria is the largest bacterial flora population in aquaculture systems, and the most abundant phylum in the trench sediment and water samples (Herlambang et al., 2021). Thus, Proteobacteria of gut of A. japonicus may come from environment. Previous studies have shown that, Proteobacteria is also dominant bacteria in sea urchin (Hakim et al., 2016), and this bacterium has major roles in nitrogen and carbon cycles in aquaculture environments (Wiegand et al., 2018). According to previous research on the rice-fish farming system which investigated the effects of several classes of Proteobacteria in nutrient cycle, such as, Alphaproteobacteria members are involved in the nitrogen cycle (Newton et al., 2011), Gammaproteobacteria help modulate excess nitrate (Fernandes et al., 2014; Herlambang et al., 2021). At the same time, this bacterium is related to sulfur-related processes, from the study of metabolites, it can be found that the sulfur metabolism pathway is indeed subjected to some changes. These phenomena indicate that A. japonicus participate in biogeochemical cycles through microbial changes.
The effects of Vibrio infection on A. japonicus
At the species level, Vibrio ratios were significantly higher in summer than the other seasons, it may have been due to accelerated growth levels of aquatic Vibrio induced by increased temperatures. Importantly, Vibrio infection can lead to skin ulcer syndrome (SUS) in sea cucumbers (Yan et al., 2014). It makes us pay attention to prevent bacterial diseases caused by environmental factors in summer. The immune function of A. japonicus is mainly controlled by the innate immune system, which is divided into two: cellular and humoral immunity (Gross et al., 2000; Eliseikina and Magarlamov, 2002). Coelomic fluid has im portant roles in the immune processes of A.japonicus, the fluid contains immune cells, lysozyme, lectin and other immune active substances, it is an important medium for A. japonicus immune cells and immune factors. Analysis of related metabolites which had increased in summer; L-isoleucine, 3-ketosphinganine, lysoPC ((20:4 (5z, 8z, 11z, 14z)), (22:6 (4z, 7z, 10z, 13z, 16z, 19z)), (22:4 (7z, 10z, 13z, 16z)), and cholesterol sulfate. Alterations in these metabolites may be directly related to vibriosis. Isoleucine is an essential metabolite of the immune system; it provides energy for new molecule and cell biosynthesis (Calder, 2006; Nguyen et al., 2018). These observations suggest a correlation possibly exists between isoleucine and immune responses in A. japonicus. 3-Ketosphinganine is involved in sphingolipid metabolism. Studies have shown that sphingolipids regulate cell-cell interactions, cell adhesion, cell proliferation and migration, cell death, and other processes partly regulated by sphingolipids. It is also an inflammatory and metabolic disease, Lysosomal storage disorders and other key signaling molecules in many pathophysiological processes (Ponnusamy et al., 2012; Orsini et al., 2019; Dai et al., 2020; Quinville et al., 2021).
In previous studies, after A. japonicus infected by Vibrio, isoleucine levels are elevated and energy demands increased (Shao et al., 2013). Also, Vibrio infection causes oxidative stress (Nguyen et al., 2018). In our study, L-isoleucine levels in summer were significantly higher than in autumn, concomitant with increased Vibrio levels in summer. To ensure survival, isoleucine metabolism in A. japonicus may have been increased to resist Vibrio infection. Also, lysoPC-related metabolites increased as oxidative stress was previously shown to increase lysoPC levels (Balboa and Balsinde, 2006; Oresic et al., 2008; Ru et al., 2017; Ding et al., 2020). Immune system activation produces reactive oxygen species which directly increases oxidative damage, potentially affecting reproduction processes (Faivre et al., 2003; Alonso-Alvarez et al., 2004; Grether et al., 2004; Torres and Velando, 2007). Therefore, Vibrio influences in summer may have indirectly impacted A. japonicus reproduction in the autumn.
The effects of diet on A. japonicus body wall growth across different seasons
In spring and autumn, metabolism levels were significantly higher than in winter and summer. In a previous study on Holothuria forskali, stigmasterol and sitosterol extracts are identified in the digestive tract and body wall and were significantly higher than in other body parts (Telahigue et al., 2020). Interestingly, phytosterols are not synthesized by animals, but enter the body via the diet (Özyurt et al., 2013). Due to the high consumption rates of A. japonicus in the spring and autumn, stigmasterol and sitosterol from the environment are consumed. However, from shrimp research, sitosterol composition changed during the year, levels in shrimp muscle in spring and autumn were at their highest (Özyurt et al., 2013). Therefore, sitosterol levels altered with the seasons. Sterols in nature typically come from specific biological sources of stigmasterol and sitosterol are the main components in higher plants. Therefore, these sterols are used as markers of terrestrial organic matter input in near-shore environments (Rielley et al., 1991; Nytoft et al., 2000) which provide terrestrial higher plant debris. For marine sedimentary environments, sterols can also come from marine phytoplankton, such as some diatoms, and also Cyanobacteria and golden algae produce sterols (Volkman, 1986; Barrett et al., 1995).
By analyzing lipid content in the A. japonicus body wall, spring and autumn levels were higher than winter and summer (Gao et al., 2011). This phenomenon may be related to seasonal stigmasterol and sitosterol levels in the body. Also, in fish muscle, stigmasterol was confirmed as a secondary component while sitosterol was a main sterol (Özyurt et al., 2013). Phytosterols also regulate the expression of lipid regulatory genes and new fat formation (Rideout et al., 2010). Therefore, phytosterols may impact body wall growth, and the specific way of nutrition remains to be solved.
Conclusions
We showed that seasonal changes exerted a significant impact on intestinal microorganisms and metabolites in A. japonicus. Our research reveals the relationship between the changes of individual metabolic function of sea cucumbers caused by the changes of microorganisms in the intestine, found A. japonicus participated in the process of carbon, nitrogen and sulfur cycle in the ocean. The increase of Vibrio in summer is a serious threat to the reproduction of A. japonicus potentially, which should be paid more attention in aquaculture. Stigmasterol and sitosterol has a potential impact on the growth of A. japonicus. These materials can increase the growth of the body wall of A. japonicus, which is very important for aquaculture. It provides support for the intestinal immune regulation, self-metabolism, energy absorption, and body growth of sea cucumber in the future.
In this research, we have some deficiency as following, in future, we will (1) subdivide the gut microbiota in different parts of A. japonicus; (2) survey seasonal variations of environmental microbiota in experimental sea area; (3) design an experiment to validate effects of Vibrio; (4) design an experiment to verify effects of stigmasterol and sitosterol on the body wall of A. japonicus.
Data availability statement
The datasets presented in this study can be found in online repositories. The names of the repository/repositories and accession number(s) can be found in the article/Supplementary Material.
Author contributions
BD performed the research and wrote the manuscript. XR conceived and designed the research. TW and CZ acquired the data, WS collected sea cucumbers, SL revised the manuscript for important intellectual content. LZ supervised this study. All authors contributed to the article and approved the submitted version.
Funding
This study was supported by the National Natural Science Foundation of China (41906079), the Scientific Research and Technological Development Program of Fangchenggang (AB20014021), and the International Partners Program of the Chinese Academy of Sciences (133137KYSB20180069).
Conflict of interest
The reviewer CL is currently organizing a Research Topic with the author LZ.
The remaining authors declare that the research was conducted in the absence of any commercial or financial relationships that could be construed as a potential conflict of interest.
Publisher’s note
All claims expressed in this article are solely those of the authors and do not necessarily represent those of their affiliated organizations, or those of the publisher, the editors and the reviewers. Any product that may be evaluated in this article, or claim that may be made by its manufacturer, is not guaranteed or endorsed by the publisher.
References
Alonso-Alvarez C., Bertrand S., Devevey G., Gaillard M., Prost J., Faivre B., et al. (2004). An experimental test of the dose-dependent effect of carotenoids and immune activation on sexual signals and antioxidant activity. Am. Naturalist 164, 651–659. doi: 10.1086/424971
Altschul S., Gish W., Miller W., Myers E., Lipman D. (1990). Basic local alignment search tool. J. Mol. Biol. 15 (3), 403–410. doi: 10.1016/S0022-2836(05)80360-2
Balboa M., Balsinde J. (2006). Oxidative stress and arachidonic acid mobilization. Biochim. Biophys. Acta (BBA)-Molecular Cell Biol. Lipids. 1761, 385–391. doi: 10.1016/j.bbalip.2006.03.014
Barrett S. M., Volkman J. K., Dunstan G. A., LeRoi J. M. (1995). Sterols of 14 species of marine diatoms (bacillariophyta). J. Phycol. 31 (3), 360–369. doi: 10.1111/j.0022-3646.1995.00360.x
Bunse C., Pinhassi J. (2017). Marine bacterioplankton seasonal succession dynamics. Trends Microbiol. 25 (6), 494–505. doi: 10.1016/j.tim.2016.12.013
Calder P. C. (2006). Branched-chain amino acids and immunity. J. Nutr. 136 (1), 288S–293S. doi: 10.1093/jn/136.1.288S
Caporaso J., Kuczynski J., Stombaugh J., Bittinger K., Bushman F. D., Costello E. K., et al. (2010). QIIME allows analysis of high-throughput community sequencing data. Nat. Methods 7, 335–336. doi: 10.1038/nmeth.f.303
Cheung M. K., Au C. H., Chu K. H., Kwan H., Wong C. (2010). Composition and genetic diversity of picoeukaryotes in subtropical coastal waters as revealed by 454 pyrosequencing. ISME J. 4 (8), 1053–1059. doi: 10.1038/ismej.2010.26
Dai Y., Zhang M., Shi X., Wang K., Gao G., Shen L., et al. (2020). Kinetic study of aβ (1-42) amyloidosis in the presence of ganglioside-containing vesicles. Colloids Surfaces B: Biointerfaces. 185, 110615. doi: 10.1016/j.colsurfb.2019.110615
Ding K., Zhang L., Fan X., Guo X., Liu X., Yang H. (2020). The effect of pedal peptide-type neuropeptide on locomotor behavior and muscle physiology in the sea cucumber Apostichopus japonicus. Front. Physiol. 11. doi: 10.3389/fphys.2020.559348
Egerton S., Culloty S., Whooley J., Stanton C., Ross R. P. (2018). The gut microbiota of marine fish. Front. Microbiol. 9. doi: 10.3389/fmicb.2018.00873
Eliseikina M. G., Magarlamov T. Y. (2002). Coelomocyte morphoogy in the holothurians Apostichopus japonicus (Aspidochirota: Stichopodidae) and Cucumaria japonica (Dendrochirota: Cucumariidae). J. Mar. Biol. 28, 197–202. doi: 10.1023/A:1016801521216
Faivre B., Grégoire A., Préault M., Cézilly F., Sorci G. (2003). Immune activation rapidly mirrored in a secondary trait. Science 300, 103. doi: 10.1126/science.1081802
Fang Y., Lv Z., Zhang H., Li F., Xu B. (2012). “Distribution and variation characteristics of nutrients in rongcheng bay,” in Marine limnology bulletin (Shandong, China: Transactions of Oceanology and Limnology), vol. 3. , 9.
Fan Y., Pedersen O. (2021). Gut microbiota in human metabolic health and disease. Nat. Rev. Microbiol. 19, 55–71. doi: 10.1038/s41579-020-0433-9
Fernandes S. O., Kirchman D. L., Michotey V. D., Bonin P. C., Lokabharathi P. A. (2014). Bacterial diversity in relatively pristine and anthropogenically-influenced mangrove ecosystems (Goa, India). Braz. J. Microbiol. 45 (4), 1161–1171. doi: 10.1590/S1517-83822014000400006
Fiehn O. (2020). Metabolomics — the link between genotypes and phenotypes. Plant Mol. Biol. 48 (1), 155–171. doi: 10.1023/A:1013713905833
Gao F., Xu Q., Yang H. (2011). Seasonal biochemical changes in composition of body wall tissues of sea cucumber Apostichopus japonicus. Chin. J. Oceanol. Limnol. 29 (2), 252–260. doi: 10.1007/s00343-011-0041-7
Goodrich J. K., Davenport E. R., Beaumont M., Jackson M. A., Knight R., Ober C., et al. (2016). Genetic determinants of the gut microbiome in UK twins. Cell Host Microbe 19, 731–743. doi: 10.1016/j.chom.2016.04.017
Goodrich J. K., Waters J. L., Poole A. C., Sutter. J. L., Koren O., Blekhman R., et al. (2014). Human genetics shape the gut microbiome. Cell 159, 789–799. doi: 10.1016/j.cell.2014.09.053
Grether G. F., Kasahara S., Kolluru G. R., Cooper E. L. (2004). Sex-specific effects of carotenoid intake on the immunological response to allografts in guppies (Poecilia reticulata). Proc. R. Soc. London Ser. B 271, 45–49. doi: 10.1098/rspb.2003.2526
Gross P. S., Clow L. A., Smith L. C. (2000). SpC3, the complement homologue from the purple sea urchin, strongylocentrotus purpuratus, is expressed in two subpopulations of the phagocytic coelomocytes. Immunogenetics 51, 1034–1044. doi: 10.1007/s002510000234
Hakim J. A., Koo H., Kumar R., Lefkowitz E. J., Morrow C. D., Powell M. L., et al. (2016). The gut microbiome of the sea urchin, lytechinus variegatus, from its natural habitat demonstrates selective attributes of microbial taxa and predictive metabolic profiles. FEMS Microbiol. Ecol. 92, 9. doi: 10.1093/femsec/fiw146
Herlambang A., Murwantoko M., Istiqomah I. (2021). Dynamic change in bacterial communities in the integrated rice–fish farming system in sleman, yogyakarta, Indonesia. Aquaculture Res. 52 (11), 5566–5578. doi: 10.1111/are.15432
Lynch S. V., Pedersen O. (2016). The human intestinal microbiome in health and disease. New Engl. J. Med. 375, 2369–2379. doi: 10.1056/NEJMra1600266
Mitsukuri K. (1903). Notes on the habits and life history of Stichopus japonicus selenka. Annotation Zool. Japan 5, 1–21.
Mohsen M., Wang Q., Zhang L., Sun L., Lin C., Yang H. (2018). Microplastic ingestion by the farmed sea cucumber Apostichopus japonicus in China. Environ. Pollution 245, 1071–1078. doi: 10.1016/j.envpol.2018.11.083
Newton R. J., Jones S. E., Eiler A., Mcmahon K. D., Bertilsson S. A. (2011). Guide to the natural history of freshwater lake bacteria. Microbiol. Mol. Biol. Rev. 75 (1), 14–49. doi: 10.1128/MMBR.00028-10
Nguyen T. V., Alfaro A. C., Young T., Ravi S., Merien F. (2018). Metabolomics study of immune responses of new Zealand greenshell™ mussels (Perna canaliculus) infected with pathogenic vibrio sp. Mar. Biotechnol. 20 (3), 396–409. doi: 10.1007/s10126-018-9804-x
Nytoft H. P., Bojesen-Koefoed J. A., Christiansen F. G. (2000). C26 and C28-C34 28-norhopanes in sediments and petroleum. Organic Geochem. 31 (1), 25–39. doi: 10.1016/S0146-6380(99)00150-3
Oresic M., Simell S., Sysi-Aho M., Nanto-Salonen K., Seppanen-Laakso T., Parikka V., et al. (2008). Dysregulation of lipid and amino acid metabolism precedes islet autoimmunity in children who later progress to type 1 diabetes. J. Exp. Med. 205, 2975–2984. doi: 10.1084/jem.20081800
Orsini M., Chateauvieux S., Rhim J., Gaigneaux A., Cheillan D., Christov C., et al. (2019). Sphingolipid-mediated inflammatory signaling leading to autophagy inhibition converts erythropoiesis to myelopoiesis in human hematopoietic Stem/Progenitor cells. Cell Death Differ. 26, 1796–1812. doi: 10.1038/s41418-018-0245-x
Özyurt G., Kuley E., Etyemez M., Özoğul F. (2013). Comparative seasonal sterol profiles in edible parts of Mediterranean fish and shellfish species. Int. J. Food Sci. Nutr. 64 (4), 476–483. doi: 10.3109/09637486.2012.749836
Ponnusamy S., Selvam S. P., Mehrotra S., Kawamori T., Snider A. J., Obeid L. M., et al. (2012). Communication between host organism and cancer cells is transduced by systemic sphingosine kinase 1/Sphingosine 1-phosphate signalling to regulate tumour metastasis. EMBO Mol. Med. 4, 761–775. doi: 10.1002/emmm.201200244
Quinville B. M., Deschenes N. M., Ryckman A. E., Walia J. S. (2021). A comprehensive review: Sphingolipid metabolism and implications of disruption in sphingolipid homeostasis. Int. J. Mol. Sci. 22 (11), 5793. doi: 10.3390/ijms22115793
Rideout T. C., Harding S. V., Jones P. J. H. (2010). Consumption of plant sterols reduces plasma and hepatic triglycerides and modulates the expression of lipid regulatory genes and de novo lipogenesis in C57BL/6J mice. Mol. Nutr. Food Res. 54, S7–S13. doi: 10.1002/mnfr.201000027
Rielley G., Collier R. J., Jones D. M., Eglinton G. (1991). The biogeochemistry of Ellesmere lake, UK–I: source correlation of leaf wax inputs to the sedimentary lipid record. Organic Geochem. 17 (6), 901–912. doi: 10.1016/0146-6380(91)90031-E
Roberts L. D., Souza A. L., Gerszten R. E., Clish C. E. (2012). Targeted metabolomics. Curr. Protoc. Mol. Biol. 98 (1), 30.2.1–30.2.24. doi: 10.1002/0471142727.mb3002s98
Ru X., Zhang L., Li X., Liu S., Yang H. (2019). Development strategies for the sea cucumber industry in China. J. Oceanol. Limnol. 37, 300–312. doi: 10.1007/s00343-019-7344-5
Ru X., Zhang L., Liu S., Yang H. (2017). Reproduction affects locomotor behaviour and muscle physiology in the sea cucumber, Apostichopus japonicus. Anim. Behaviour 133, 223–228. doi: 10.1016/j.anbehav.2017.09.024
Schoeler M., Caesar R. (2019). Dietary lipids, gut microbiota and lipid metabolism. Rev. Endocrine Metab. Disorder 20 (4), 461–472. doi: 10.1007/s11154-019-09512-0
Shao Y., Li C., Ou C., Zhang P., Liu Y., Su X., et al. (2013). Divergent metabolic responses of Apostichopus japonicus suffered from skin ulceration syndrome and pathogen challenge. J. Agric. Food Chem. 61 (45), 10766–10771. doi: 10.1021/jf4038776
Spor A., Koren O., Ley R. (2011). Unravelling the effects of the environment and host genotype on the gut microbiome. Nat. Rev. Microbiol. 9 (4), 279–2290. doi: 10.1038/nrmicro2540
Tanaka Y. (1958a). Seasonal changes occurring in the gonad of Stichopus japonicus. Bull. Faculty Fisheries 9, 29–36.
Tanaka Y. (1958b). Feeding and digestive processes of Stichopus japonicus. Bull. Faculty Fisheries 9 (1), 14–28.
Telahigue K., Ghali R., Nouiri E., Labidi A., Hajji T. (2020). Antibacterial activities and bioactive compounds of the ethyl acetate extract of the sea cucumber Holothuria forskali from Tunisian coasts. J. Mar. Biol. Assoc. UK. 100 (2), 1–9. doi: 10.1017/S0025315420000016
Torres R., Velando A. (2007). Male Reproductive senescence: the price of immune-induced oxidative damage on sexual attractiveness in the blue-footed booby. J. Anim. Ecol. 76 (6), 1161–1168. doi: 10.1111/j.1365-2656.2007.01282.x
Turnbaugh P. J., Gordon J. I. (2008). An invitation to the marriage of metagenomics and metabolomics. Cell 134, 708–713. doi: 10.1016/j.cell.2008.08.025
Visconti A., Roy C., Rosa F., Rossi F., Martin T. C., Mohney R. P., et al. (2019). Interplay between the human gut microbiome and host metabolism. Nat. Commun. 10, 1. doi: 10.1038/s41467-019-12476-z
Volkman J. K. (1986). A review of sterol markers for marine and terrigenous organic matter. Organic Geochem. 9 (2), 83–99. doi: 10.1016/0146-6380(86)90089-6
Wiegand S., Jogler M., Jogler C. (2018). On the maverick planctomycetes. FEMS Microbiol. Rev. 42, 739–760. doi: 10.1093/femsre/fuy029
Xie H., Guo R., Zhong H., Feng Q., Lan Z., Qin B., et al. (2016). Shotgun metagenomics of 250 adult twins reveals genetic and environmental impacts on the gut microbiome. Cell Systems 3 (6), 572–584. doi: 10.1016/j.cels.2016.10.004
Xing L., Sun L., Liu S., Zhang L., Yang H. (2021). Comparative metabolomic analysis of the body wall from four varieties of the sea cucumber Apostichopus japonicus. Food Chem. 352, 10. doi: 10.1016/j.foodchem.2021.129339
Xu Q., Hamel J. F., Mercier A. (2015). Feeding, digestion, nutritional physiology, and bioenergetics - ScienceDirect. Developments Aquaculture Fisheries Sci. 39, 153–175. doi: 10.1016/B978-0-12-799953-1.00010-6
Yang H., Yuan X., Zhou Y., Mao Y. Z., Zhang T., Liu Y., et al. (2015). Effects of body size and water temperature on food consumption and growth in the sea cucumber Apostichopus japonicus (Selenka) with special reference to aestivation. Aquaculture Res. 36 (11), 1085–1092. doi: 10.1111/j.1365-2109.2005.01325.x
Yan F., Tian X., Dong S., Fang Z., Yang G. (2014). Growth performance, immune response, and disease resistance against vibrio splendidus infection in juvenile sea cucumber Apostichopus japonicus fed a supplementary diet of the potential probiotic paracoccus marcusii DB11. Aquaculture 420, 105–111. doi: 10.1016/j.aquaculture.2013.10.045
Keywords: 16S rRNA, gut microbiome, metabolomics, sea cucumber (Apostichopus japonicus), seasonal variations
Citation: Deng B, Ru X, Wang T, Zhang C, Sun W, Lu S and Zhang L (2022) Seasonal variations in microbial diversity and metabolite profiles of the gut of sea cucumber (Apostichopus japonicus). Front. Mar. Sci. 9:953388. doi: 10.3389/fmars.2022.953388
Received: 26 May 2022; Accepted: 20 October 2022;
Published: 03 November 2022.
Edited by:
Wei Huang, Ministry of Natural Resources, ChinaCopyright © 2022 Deng, Ru, Wang, Zhang, Sun, Lu and Zhang. This is an open-access article distributed under the terms of the Creative Commons Attribution License (CC BY). The use, distribution or reproduction in other forums is permitted, provided the original author(s) and the copyright owner(s) are credited and that the original publication in this journal is cited, in accordance with accepted academic practice. No use, distribution or reproduction is permitted which does not comply with these terms.
*Correspondence: Songchong Lu, luschvip@outlook.com; Libin Zhang, zhanglibin@qdio.ac.cn