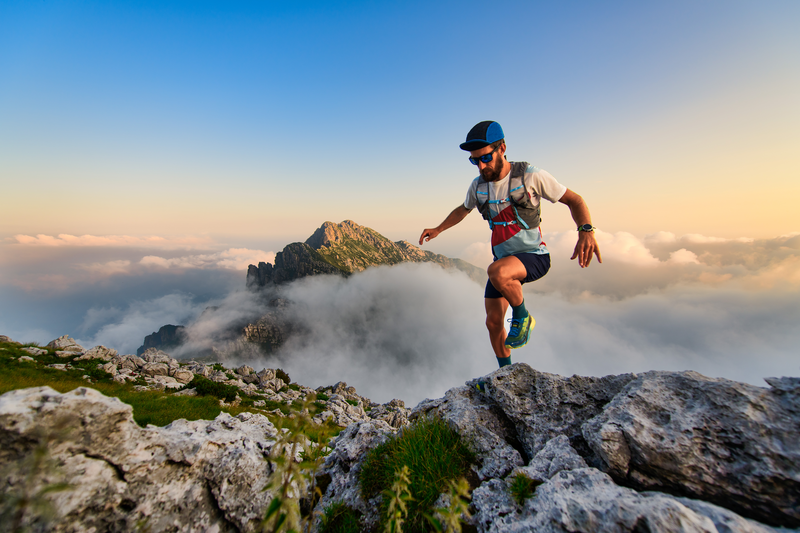
94% of researchers rate our articles as excellent or good
Learn more about the work of our research integrity team to safeguard the quality of each article we publish.
Find out more
ORIGINAL RESEARCH article
Front. Mar. Sci. , 14 September 2022
Sec. Marine Evolutionary Biology, Biogeography and Species Diversity
Volume 9 - 2022 | https://doi.org/10.3389/fmars.2022.948580
The Humboldt Current Marine Ecosystem (HCE) is one of the most productive areas in the global ocean, but current anthropogenic stressors, particularly overfishing, pose a significant threat to marine biodiversity. Moreover, the limited time scale of modern assessments may underestimate the magnitude of human alterations to marine biodiversity. Here we use the rich Quaternary fossil record present along the HCE coast, encompassing the last ca. 500 kyr, to build a baseline to evaluate the impact of human activities on the diversity of mollusk assemblages. We compiled an extensive database of >13,000 occurrences and ca. 370,000 individuals of 164 species of gastropods and bivalves from modern and fossiliferous outcrops from southern Peru to northern Chile (15-30°S). We tested for changes in coverage-based species richness, species dominance, species composition (Chao dissimilarity, unweighted and weighted by abundance), and the relative abundance (i.e., the proportion of individuals) of species exploited by the artisanal fisheries. Comparisons between fossil and modern assemblages were carried out at different scales of spatial aggregation to buffer against inherent differences in spatial and temporal averaging. Species composition shows remarkable stability in fossil assemblages, from Middle Pleistocene to Holocene, at most scales of spatial aggregation. Modern assemblages showed drastic alterations compared to fossil counterparts when analyses considered spatial aggregation scales, i.e., significant changes in species composition, and a 3 to 6-fold reduction in the relative abundance of exploited species, but not changes in species richness and dominance. Results suggest that contemporaneous anthropogenic activities disrupted a long-term stability in the species composition. The diversity of modern mollusk assemblages is unseen in the past 500 kyr and seems deeply perturbated by overfishing. Our synthesis sets the foundations for a conservation paleobiology approach to robustly understand the impacts of anthropogenic stressors at the HCE.
The growing human population will increase the pressure for getting goods and services from the ocean during the next decades, i.e., the blue acceleration (Jouffray et al., 2020) which includes seafood (Costello et al., 2020), and meeting these demands will require far-reaching transformations in the way in which humans relate to the oceans. In fact, fishing stocks show drastic declines and collapses during the last seven decades across the global ocean (Worm et al., 2006; Coll et al., 2008). The subsequent collapse of large-sized species has been compensated by the exploitation of new stocks, typically smaller-sized and lower-trophic level forms inhabiting deeper waters (Pauly et al., 1998; Pauly et al., 2005). Overfishing per se may lead species to the verge of extinction (Pauly et al., 2005; Davies and Baum, 2012; Burgess et al., 2013). For instance, more than one third of shark species are threatened by extinction as a consequence of overfishing (Dulvy et al., 2021). Moreover, the mass removal of fishing stocks may also have strong indirect effects on unfished marine biodiversity, through trophic cascading effects (Scheffer et al., 2005; Myers et al., 2007; Heithaus et al., 2008). Despite the pervasiveness of human impacts across oceans, global maps of these impacts show large variations among geographic regions (Halpern et al., 2008; Halpern et al., 2012). Factors underlying the spatial variability of overfishing, and other indicators provided by the Ocean Health Index (Halpern et al., 2012), are often attributed to socioeconomic, cultural, and ecological variables. However, these assessments document trends occurred only during last decades and may suffer from the ‘shifting baseline’ syndrome, i.e., a baseline placed well after the onset of alterations (Pauly, 1995).
One way of overcoming the limitations of short-scale ecological timeseries to assess the long-term impacts of human alterations on marine biodiversity is using the temporal record imbibed by other sources such as historical accounts, archaeological shell middens, and subfossil and fossil assemblages (Jackson, 2001; Jackson et al., 2001; Kidwell, 2015; Dietl and Flessa, 2017; Tyler and Schneider, 2018). There is growing awareness of the usefulness of paleontological analysis to inform modern conservation biology problems (Rick and Lockwood, 2013; Kiessling et al., 2019), for instance assessing more robustly the magnitude of present-day extinction risk of species (Barnosky et al., 2011; Finnegan et al., 2015; Kosnik and Kowalewski, 2016). These studies also illustrate that the diversity of modern assemblages not only departs from past counterparts at scales of few 100’s to 100 kyr (Kowalewski et al., 2000; Pandolfi et al., 2003; Kidwell, 2007), but that human alterations interrupt the previous stability in the species composition during the Quaternary (Greenstein et al., 1998; Pandolfi and Jackson, 2006) leading to the emergence of novel assemblages unseen in the fossil record (Toth et al., 2019).
The Humboldt Current Marine Ecosystem (HCE) is one of the largest and most productive marine ecosystems in the world (Thiel et al., 2007; Gutiérrez et al., 2016), encompassing a large latitudinal gradient of ca. 50°, harboring > 105 species (Miloslavich et al., 2011), and providing goods and services valued at US$19.5 billion per year (Gutiérrez et al., 2017). Overfishing is considered the most important anthropogenic threat to the HCE (Chatwin, 2007) as many stocks show signs of over-exploitation (Rivadeneira et al., 2010; Gutiérrez et al., 2016), and a very low fraction of stocks are considered as sustainable (Coll et al., 2008). Experimental studies carried out in marine reserves of central and southern Chile show how the exclusion of humans lead to profound changes in the local community structure, via increasing abundances of exploited species producing a cascade of trophic effects (Castilla, 1999; Moreno, 2001). However, it is uncertain whether these experiments carried out in small-size reserves could be scaled up to the entire seascape of the HCE. Previous studies have highlighted the potential of the rich and well-preserved fossil record of mollusk species present in Quaternary terraces of southern Peru and northern Chile (Ortlieb et al., 1995; Paskoff et al., 1995) as sources for the establishment of a baseline to evaluate anthropogenic impacts on modern assemblages (Rivadeneira and Carmona, 2008; Rivadeneira et al., 2010; Martinelli et al., 2017). Here we reappraise this idea, providing the first large-scale evaluation of human impact on marine coastal biodiversity at the HCE using a near-time fossil baseline and marine mollusks as a study model. Our goals are: i) to test the existence of long-term stability in the species composition during the Pleistocene-Holocene, and ii) to evaluate the magnitude and direction of human-driven impacts on different facets of the diversity of assemblages. We put these ideas to a test compiling a large database of fossil and modern species assemblages, devising a scheme to deal with the inherent difference in spatial and temporal averaging of fossil and modern faunas (Kidwell and Bosence, 1991; Behrensmeyer et al., 2000).
We gathered information on the geo-referenced occurrences and individual abundance of mollusk species (i.e., bivalves and gastropods) in ecological (modern), and paleontological (fossil) assemblages along the coast of Peru and northern Chile, from ca. 15.3°S to 30.4°S (Figure 1), encompassing more than 1,600 km of coastline. We only considered shelled species ≥5 mm length, since micromollusks are little studied in both modern and fossil assemblages. The information was obtained from a comprehensive literature analysis, including published and unpublished information (i.e., theses, technical reports, see supplemental information) and new field surveys for ecological and paleontological assemblages.
Figure 1 Map of the study region, in coast of the Humboldt Current Ecosystem, showing the number of georeferenced occurrences for (A) modern and (B) Quaternary fossil assemblages. It is also provided an example of the spatial aggregation scheme, using grids cells of 0.1° (C).
Modern sites include different structural habitats (hard and soft bottoms) from intertidal to subtidal (< 200 m depth). We classified samples according to the bathymetry (intertidal/subtidal) and seabed type (hard/soft/biogenic/mixed) and created categories of habitats based on the combinations of these factors. Present-day assemblages were spread throughout our study area, with few gaps mainly in little studied areas between 24-25°S (Figure 1A). Most modern sites were snapshots or were sampled only few times over a few years, but the overall present dataset encompasses sites sampled from 1948 to 2013. In addition, we have used shells collected from death assemblages on beaches. Our preliminary analyses, based on 14C dating of these death assemblages, suggest ages no older than ca. 500 years BP.
Fossiliferous outcrops are present in late Quaternary terraces along the coast of Peru and northern Chile (Figure 1B), formed due to the intense tectonic uplift of the coast (Victor et al., 2011; Rodríguez et al., 2013). These deposits have been assigned to interglacial marine isotopic ages, using different dating methods (Radtke, 1987; Ortlieb et al., 1995; Paskoff et al., 1995; Marquardt et al., 2004; Regard et al., 2010; Saillard et al., 2012). Most fossiliferous localities have been assigned to MIS 5, and comparatively less to MIS 1, 7, 9, 11, and > 11, and therefore we categorized our sites, as much as possible, in coarser time intervals that considers formal subdivision of the Quaternary (Head, 2019). Hence, we grouped our fossil sites in Middle Pleistocene (Chibanian; MIS >11 to 7; ~500-126 ka), Upper Pleistocene (MIS 7 to 5; ~126-11.7 ka), and Holocene (~11.7-0.5 ka). Taphonomic analyses (Herm, 1969) suggest that these sites represent ‘allochthonous assemblages’ (i.e., mixed habitats and depths) from high energy shallow-water environments. Fossil specimens were collected from bulk samples (20-50 l per site) sieved using a 2-mm mesh size.
Each modern and fossil record was georeferenced. Abundance data (i.e., number of total shells per species) was available only for a subset of collections. All taxonomic entities were checked in MolluscaBase (https://molluscabase.org/, accessed in March 2022), and only valid accepted species were included in further analyses. The final database is composed of 13,368 occurrences, 369,153 individuals, 164 species (96 gastropods and 68 bivalves), in 68 families and 23 orders. The fossil record includes 6,734 occurrences, 35,692 individuals, and 128 species, and the modern record is composed of 6,634 occurrences, 333,461 individuals, and 136 species. The entire dataset is available in the Supplementary Material.
The validity of fossil-modern comparisons in conservation paleobiology analyses may be affected by various taphonomic effects that need to be accounted for, including the completeness, fidelity, and time and space-averaging of assemblages. To evaluate the magnitude of fossil completeness, we categorized each species described in present assemblages according to its fossilization potential. This was carried out evaluating whether the genus to which each species belongs has a described fossil counterpart somewhere in the world (including our study area). We compared our list of genera with a list of all molluscan genera (gastropods and bivalves), accepted or unaccepted, entered to the Paleobiology database (https://paleobiodb.org/, accessed in April 2022). Out of the 136 modern species, 133 belong to a genus with a fossil counterpart (98%). However, only 74% of modern species (100 out of 136) are also present in fossil assemblages in the same study area. Therefore, there is a potential number of rare species that remain to be detected (Rivadeneira, 2010; Rivadeneira and Nielsen, 2017). We carried out further analyses excluding modern species without a fossil counterpart, which is rather a conservative approach which minimizes differences between fossil and present. However, it is unlikely that this approach seriously distorts our interpretations, as species without a fossil record account for only ca. 3% of occurrences and 2% of total individuals. The dataset used in further analyses totaled 13,311 occurrences, 367,553 individuals and 161 species.
Modern-fossil comparisons may be also biased by the fact that fossil counterparts are both spatially and time-averaged (Kidwell and Bosence, 1991; Kidwell and Flessa, 1995; Behrensmeyer et al., 2000). To account for these potential effects, we created spatially and time-averaged present-day assemblages by pooling all the modern sites lying within spatial cell grids of variable scale (0.01°, 0.05°, 0.1°, 0.5°, 1°, 5°, 10°, and 25°), (see example in Figure 1C). The gridding procedure was carried out using the library raster (Hijmans, 2022) in R (R Core Team, 2022). Although this procedure reduces the number of sites available for further analysis, the pooled assemblages allow us to increase the spatial extension of modern assemblages, combining information from different coastal habitats, which is not normally done in modern survey studies (i.e., aimed at only inter and subtidal stands in either soft or hard bottoms). In addition, the pooled assemblages encompass surveys from several decades, thus creating artificially time-averaged assemblages, although of lower magnitude than estimated in fossiliferous assemblages (Scarponi et al., 2013; Kowalewski, 2017). We evaluated the effect of the scale of spatial aggregation on the median number of habitats, habitat diversity, and time average on modern assemblages. For each grid cell, we counted all the habitat types sampled. Habitat diversity was measured as the 1-Simpson diversity index, based on the total number of occurrences in each habitat. Time average was estimated as the difference between the sampling years of the oldest and youngest survey at each grid cell. Fossil occurrences were also pooled to include sites with potentially different depositional conditions. We acknowledge this procedure cannot correct all the problems introduced by the space-time-averaging; the time-average of modern assemblages should still be several orders of magnitude lower than in fossil assemblages (Scarponi et al., 2013; Kowalewski, 2017), but the increasing spatial scale should at least reduce this offset.
We first tested whether the species composition of fossil assemblages varied throughout the Quaternary. Analyses were carried out using the entire dataset based on species occurrences, and a reduced dataset from which we have local counts of individuals. For the abundance dataset, Lower Pleistocene sites were not available and it thus contains samples of Chibanian to Holocene age. Species-site matrices were converted to distance matrices using the Jaccard index, based on presence-absence for the occurrence dataset, and the Chao index, based on the abundance dataset. The Jaccard index does not weigh species abundance, and hence, differences are solely driven by changes in the presence of species. The Chao index weighs for the species abundance and also accounts for missing species in the inventories. We evaluated differences in the species composition among time intervals using a Permutational Analysis of Variance (PERMANOVA) (Anderson, 2001), with 9,999 permutations, at each spatial scale of aggregation separately. Differences in species composition were visualized using a non-metric multidimension scaling (nMDS). All analyses were caried out in the library vegan (Oksanen et al., 2020) in R (R Core Team, 2022).
We considered four main aspects (i.e., species richness, dominance, species composition, and relative abundance of mollusk species) to evaluate possible changes in diversity in present-day assemblages compared to a late Quaternary fossil baseline. To this end, all fossil information was pooled, a decision supported by the lack of significant changes in species composition among time intervals (see Results). We estimated species richness and dominance for each grid cell and time interval using coverage-based estimations of the species richness to account for possible sampling biases (Chao and Jost, 2012). We used abundance-based estimators of the species richness, at a coverage level of 0.95. Dominance was estimated using the Simpson index. For both richness and dominance, only assemblages with a minimum of 50 individuals were used in further analyses. Analyses were carried out using the library iNEXT (Hsieh et al., 2016) in R (R Core Team, 2022). Changes in species composition were evaluated using the same procedure explained previously, but with PERMANOVA comparing modern versus fossil assemblages (with 9,999 permutations). Finally, species were categorized as exploited/non-exploited by the artisanal fisheries fleet of southern Peru or Chile. We evaluated changes in the relative abundance of exploited species by comparing their proportion of total abundance (individuals) in fossil and modern assemblages with ≥50 individuals. To assess temporal changes in species richness, dominance, and relative abundance of exploited species we used a meta-analysis approach, accounting for variability within and among assemblages. Meta-analyses were carried out using the library metafor (Viechtbauer, 2010) in R (R Core Team, 2022). All the R code written to reproduce all the analyses and figures is available in the Supplementary Material.
The scale of spatial aggregation had a marked effect on the total number of habitats, their diversity, and the temporal scale of aggregation (Figure 2). At smaller scales (≤ 10 km), median values across grid cells show a very low number and diversity of habitats sampled, typically during a single year. This trend changes abruptly at scales ≥50 km, and tends to stabilize at scales ≥ 500 km, with a maximum number and diversity of sampled habitats, and a time average of > 60 years.
Figure 2 Effect of the spatial aggregation scale on (A) the median number of benthic habitats, (B) median diversity of benthic habitats (1-Simpson index), and (C) median time-average (years) of modern assemblages.
The species composition of fossil assemblages showed significant differences among time intervals only at a few spatial scales of aggregation (Figure 3). In the case of binary data (Figure 3A), differences in species composition were significant only at ~1 and ~10 km scales. When using quantitative matrices (Figure 3B) differences in species composition were only significant at scales ≤5 km. P-values of the PERMANOVA remained above the 0.05 threshold at scales ≥ 50 km, independently of the use of binary or quantitative matrices. These patterns were also evident in the nMDS which shows a major overlap in the species composition projected onto a two-dimension space among time intervals (Figures 3C, D)
Figure 3 Long-term stability in the species composition of fossil assemblages, showing p-values of the permutational analysis of variance among time intervals based on the Jaccard (A) and Chao indices (B) across spatial scales of aggregation. Also shown are non-metric scaling 2-D representations of the species composition, using as an example the 10 km aggregation scale, for the Jaccard (C) and Chao indices (D).
The coverage-based species richness increases towards higher spatial scales of aggregation (Figure 4A) but it was significantly higher for fossil assemblages only at a scale of ~1 km. Above this scale, differences between fossil and modern assemblages became non-significant. Simpson’s dominance index also increases towards higher spatial scales of aggregation, yet this increase was less pronounced in modern assemblages. Fossil assemblages showed a higher dominance at all scales, but these differences were not significant (p> 0.05) in most cases, except at a scale of ~10 km (Figure 4B). Species composition, measured as the Chao dissimilarity index, shows very high values (β=0.97) at smaller spatial scales, steadily declining towards higher scales, where β ~0.24 at a aggregation scale of ~1,000 km. The PERMANOVA shows significant difference at scales up to 500 km (Figure 4C). The nMDS illustrates striking differences in species composition between fossil and modern assemblages at a ~100 km scale (Figure 4C). Finally, the relative abundance of exploited species was significantly higher in fossil (42-53% of total abundance) compared to modern assemblages (7-16% of the total abundance), a three to six-fold difference across spatial scales of aggregation (Figure 4D).
Figure 4 Changes in the diversity of mollusk assemblages between fossil and modern assemblages across different spatial aggregation scales: (A) coverage-based species richness, (B) species dominance (Simpson index), (C) Median dissimilarity (Chao index), with a nMDS illustrated for ~100 km aggregation scale, and d) relative abundance of exploited species. (A, C, D) based on meta-analysis mean values and 95% CIs.
Our results provide the first large-scale evaluation of the stability of species diversity of the HCE over the last ca. 500 kyr, based on the analysis of ca 370.000 shells, accounting for the biases of taphonomic processes, and hence providing a robust baseline against which it is possible to evaluate the magnitude of human-induced alterations on marine biota.
Fossil assemblages show a strong similarity in terms of species composition among time intervals from the Chibanian (Middle Pleistocene) to the Holocene, a conclusion robust to the use of binary (presence-absence) or quantitative (abundance) matrices of dissimilarities. This trend suggests a long-term stability in the species composition operating at scales of 100’s kyr, as observed in other Quaternary marine benthic assemblages (Pandolfi, 1996; Gardiner, 2001; Pandolfi and Jackson, 2006; Scarponi et al., 2022). The stability in species composition in our fossil dataset is concluded only at intermediate or larger scales (i.e., ≥ 10-50 km), as observed by previous studies in Quaternary corals (Pandolfi, 2002) and Devonian benthic invertebrates (Ivany et al., 2009). A study carried out in the southern region of our study area, at Tongoy Bay, showed a similar species composition over the last 300 kyr (Martinelli et al., 2017). The ultimate mechanisms for stability in species composition of mollusk assemblages may be related to strong species interactions stabilizing community structure, and/or to stable environmental conditions or environmental tracking due to strong niche conservatism in the preference of paleoenvironmental and/or paleoceanographic conditions (Ivany, 1996; Brett et al., 2016; Precht and Aronson, 2016). The stability in species composition may be also tested against the predictions of the Neutral Theory of Biodiversity (Hubbell, 2001), as carried out by McGill et al. (2005) with Quaternary mammals in North America. Although we cannot rule out any of these hypotheses, if species exhibit a strong niche conservatism over long-term timescales, as observed in Atlantic mollusk species (Saupe et al., 2014), and paleoceanographic conditions (e.g., sea surface temperature) among inter-glacial stages at HCE (Ho et al., 2012) were comparatively similar, then this may lead to a stability in species composition driven by an environmental tracking of communities.
We devised a spatial aggregation scheme to deal with the temporal and spatial averaging of fossil assemblages (Kidwell and Bosence, 1991; Behrensmeyer et al., 2000; Kidwell and Holland, 2002), thus improving its comparability with modern assemblages. This needs to be explicitly considered as surveys of modern assemblages are typically aimed at particular habitats and are snapshots or temporally limited in scope. Therefore, modern assemblages need to be aggregated over scales of ca. 50-100 km in order to be comparable to fossil assemblages that, in contrast, are composed of a mixture of habitats/facies, and so, a relatively modest spatial aggregation scale captures a fuller variety of paleoenvironments. Our analyses suggest that the magnitude and direction of changes in species between fossil and modern assemblages depend upon the spatial scale of aggregation, driven, at least in part, by taphonomic processes (Kidwell and Tomasovych, 2013). For instance, live-dead agreement syntheses have shown that species richness tends to be higher in dead assemblages, while dominance is roughly similar (Kidwell, 2002; Olszewski and Kidwell, 2007), as seen in our analyses at the smaller scales of aggregation. Likewise, temporal beta diversity is also expected to be variable across spatial scales, being more pronounced at smaller scales and declining towards larger scales (Pandolfi, 2002; Precht and Aronson, 2016).
Our analyses reveal strong differences in the species diversity of molluscan assemblages between Quaternary and modern assemblages, interrupting a long-term stability in species composition observed for the previous ca. 500 kyr. The main differences are related to: i) a change in species composition, and ii) a reduction in the relative abundance of exploited species. Taken together, these results are evidence for a major role of overharvesting in re-shaping modern assemblages. Alterations are not expressed in a reduction of the species richness or dominance, as coverage-based estimations and Simpson’s dominance were not different at most spatial scales of aggregation. The loss of the relative abundance of exploited species was evident at all spatial scales of aggregation; modern abundance of exploited species is less than a third of that observed in fossil assemblages. This change in the relative abundance of exploited species does not seem linked to a change in dominance, but to the significant changes in species composition, as the used beta diversity index weighs for the species relative abundance. Overfishing has long been considered a major structuring agent shaping rocky nearshore communities along the Chilean coast, as revealed by classic experimental studies in small-sized reserves (Moreno et al., 1984; Castilla, 1999). Meta-analyses reveal that, after humans are excluded from temperate marine reserves, exploited species undergo an up to 3-fold increase in their abundances (Lester et al., 2009), a difference similar to that observed between our modern and fossil assemblages. In addition, previous analyses based on a very limited subset of fossil and modern sites showed similar differences in species composition between modern and fossil assemblages in northern Chile (Rivadeneira and Carmona, 2008; Rivadeneira et al., 2010). Studies comparing fossil vs modern assemblages carried out at particular localities, however, have yielded contrasting results, rather supporting the idea of a strong resilience of the HCE to anthropogenic impacts (Rivadeneira and Carmona, 2008; Martinelli et al., 2017). Hence, it is possible and plausible that overfishing is not evenly spread across the coast or that some areas are more resilient than others (Chevallier et al., 2021). For instance, molluscan assemblages from the Tongoy Bay area, one of the major upwelling centers of the HCE, show strong similarities in composition throughout the last 300 kyr (Martinelli et al., 2017). Thus, while human exploitation of mollusks as a food source significantly alters community abundance compositions, it does not significantly affect species composition through extinction of species. This suggests that, ignoring other factors like climate change or effects on other parts of the ecosystem, these human food resources are still able to recover given responsible management. However, if current trends of switching to ever smaller sizes and species continues, extinction of species at different spatial levels are likely.
Our study adds to a large number of studies from the last two decades reporting strong anthropogenic impacts on marine ecosystems from a conservation paleobiology approach (Jackson, 2001; Jackson et al., 2001; Pandolfi and Jackson, 2001). However, our results are at odds with global scale assessments reporting rather low anthropogenic impacts for the HCE, including overfishing by artisanal fisheries (Halpern et al., 2008; Halpern et al., 2015). In fact, the Ocean Health Index (Halpern et al., 2012) for the HCE shows high scores, comparable to relatively healthy marine ecosystems (Gutiérrez et al., 2016). Another country-level assessment for South America, however, indicates that fisheries pose a very high risk to marine biodiversity in Peru and northern Chile (Chatwin, 2007), yet the overfishing on benthic and pelagic stocks may not be similar. The main cause for these discrepancies may be related to the shifting-baseline effect, i.e., the baseline used to assess present-day anthropogenic alterations was set well after major alterations took place (Pauly, 1995; Knowlton and Jackson, 2008; Pinnegar and Engelhard, 2008). Our preliminary meta-analysis reveals that the relative abundance of exploited species does not show any temporal trend from ca. 1948 to 2013 (Figure 5) but is well below the levels estimated for fossil assemblages. Hence, anthropogenic impacts may have taken place well before that time (Rivadeneira et al., 2010), as is seen in other marine systems (Worm et al., 2006). Overexploitation of local stocks of benthic mollusks is reported as early as 1880 in central and southern Chile (Couyoumdjian, 2009), and the highly destructive dynamite fishing was a common practice among artisanal fishermen up to early 1900’s, with devasting effects on natural stocks (Sougarret Muñoz and Ríos Ther, 2013; Camus Gayán and Arias, 2020). The use of live-dead agreement studies (Kidwell, 2015; Gallmetzer et al., 2017; Kowalewski, 2017), aimed at studying subfossil shells during the Holocene-Anthropocene transition, may help to pinpoint the timing of onset of overfishing effects on marine biodiversity of the HCE.
Figure 5 Meta-analysis testing the existence of temporal changes in the relative abundance (log10) of exploited species during the last ca. 70 years at the HCE, aggregated a scale of 1° (~100 km). The size of circles is proportional to the sampling size. The dotted red line shows the meta-regression fit line between the relative abundance and the sampling year (not significant). For comparison, the mean relative abundance of exploited species obtained for fossil assemblages at a similar spatial aggregation scale are given.
The demands of resources from the ocean, including food will increase even more during the next decades as part of the blue acceleration phenomenon (Costello et al., 2020; Jouffray et al., 2020). This represents a major concern as most marine stocks of the HCE are currently overexploited and are unsustainable in the long-term (Worm et al., 2006; Coll et al., 2008). In addition, the HCE also faces major threats from increasing water temperatures and extreme weather events, intensifying oxygen minimum zones, increasing acidification, changes in the upwelling phenology, and habitat degradation (Gutiérrez et al., 2016; Gutiérrez et al., 2017). A conservation paleobiology approach (Kidwell, 2015; Barnosky et al., 2017; Dietl and Flessa, 2017) maximizing the usefulness of the late Cenozoic mollusk fossil record of the HCE may help providing robust baselines against which the real magnitude of anthropogenic-driven threats to marine biodiversity can be tested.
The original contributions presented in the study are included in the article/Supplementary Material. Further inquiries can be directed to the corresponding author.
MR conceived the study. MR conducted the analyses. The manuscript was written by MR and SN. All authors contributed to the article and approved the submitted version.
This work was funded by several ANID/FONDECYT grants (11070147, 1110582, 1200843), National Geographic Research Grant # 8690-09, ANID- Millennium Science Initiative Program-NCN19_153 (UPWELL), ANID-CENTROS REGIONALES R20F0008 (CLAP), and INACH RG_51-19.
This work represents a synthesis achieved by almost two decades studying fossil mollusks from the northern Chile coast. We are grateful for the assistance provided throughout all these years (prospecting, collecting and processing samples, and digitalizing and analyzing information), by many past, current, and honorific members of the PaleoLab, including Daniela Carreño, Marcela Salinas, Patricio Soto, Alex Alballay, Jaime Villafaña, Albert Neira, Pablo Oyanadel, Julieta Martinelli, Yusse Hernández, Benjamín Araya, Selene Araya, Leandro Ledezma, Héctor Ramos, Yara Bugueño and Rosmary Liz. This paper is dedicated to the memory of Eduardo ‘Moro’ Quiroga, a dear friend, passionate scientist, excellent benthologist, and above all, a marvelous human being. May you be at the Valhalla, where you belong.
The authors declare that the research was conducted in the absence of any commercial or financial relationships that could be construed as a potential conflict of interest.
The reviewer DS declared a past co-authorship with one of the authors MR to the handling Editor.
All claims expressed in this article are solely those of the authors and do not necessarily represent those of their affiliated organizations, or those of the publisher, the editors and the reviewers. Any product that may be evaluated in this article, or claim that may be made by its manufacturer, is not guaranteed or endorsed by the publisher.
The Supplementary Material for this article can be found online at: https://www.frontiersin.org/articles/10.3389/fmars.2022.948580/full#supplementary-material
Anderson M. J. (2001). Permutation tests for univariate or multivariate analysis of variance and regression. Can. J. Fish. Aquat. Sci. 58 (3), 626–639. doi: 10.1139/f01-004
Barnosky A. D., Hadly E. A., Gonzalez P., Head J., Polly P. D., Lawing A. M., et al. (2017). Merging paleobiology with conservation biology to guide the future of terrestrial ecosystems. Science 355 (6325), eaah4787. doi: 10.1126/Science.aah4787
Barnosky A. D., Matzke N., Tomiya S., Wogan G. O. U., Swartz B., Quental T. B., et al. (2011). Has the Earth’s sixth mass extinction already arrived? Nature 471 (7336), 51–57. doi: 10.1038/nature09678
Behrensmeyer A. K., Kidwell S. M., Gastaldo R. A. (2000). Taphonomy and paleobiology. Paleobiology 26 (S4), 103–147. doi: 10.1666/0094-8373(2000)26[103:TAP]2.0.CO;2
Brett C. E., Zaffos A., Miller A. I. (2016). “Niche conservatism, tracking, and ecological stasis,” in Evolutionary theory: A hierarchical perspective. Eds. Eldredge N., Pievani T., Serrelli E., Temkin I., 282–306. Chicago, IL
Burgess M. G., Polasky S., Tilman D. (2013). Predicting overfishing and extinction threats in multispecies fisheries. Proc. Natl. Acad. Sci. 110 (40), 15943–15948. doi: 10.1073/pnas.1314472110
Camus Gayán P., Arias M. (2020). Pescadores artesanales y prácticas pesqueras a inicios del siglo XX. entre el control estatal y la libertad de pesca. Estudios Atacameños 64), 109–125. doi: 10.22199/issn.0718-1043-2020-0006
Castilla J. C. (1999). Coastal marine communities: trends and perspectives from human-exclusion experiments. Trends Ecol. Evol. 14 (7), 280–283. doi: 10.1016/S0169-5347(99)01602-X
Chao A., Jost L. (2012). Coverage-based rarefaction and extrapolation: standardizing samples by completeness rather than size. Ecology 93 (12), 2533–2547. doi: 10.1890/11-1952.1
Chatwin A. (2007). Priorities for coastal and marine conservation in South America (Arlington, Virginia, USA: The Nature Conservancy).
Chevallier A., Broitman B. R., Barahona N., Vicencio-Estay C., Hui F. K., Inchausti P., et al. (2021). Diversity of small-scale fisheries in Chile: Environmental patterns and biogeography can inform fisheries management. Environ. Sci. Policy 124, 33–44. doi: 10.1016/j.envsci.2021.06.002
Coll M., Libralato S., Tudela S., Palomera I., Pranovi F. (2008). Ecosystem overfishing in the ocean. PloS One 3 (12), e3881. doi: 10.1371/journal.pone.0003881
Costello C., Cao L., Gelcich S., Cisneros-Mata M.Á., Free C. M., Froehlich H. E., et al. (2020). The future of food from the sea. Nature 588 (7836), 95–100. doi: 10.1038/s41586-020-2616-y
Couyoumdjian J. R. (2009). El Mar y el paladar: el consumo de pescados y mariscos en Chile desde la independencia hasta 1930. Historia 42 (1), 57–107. doi: 10.4067/S0717-71942009000100002
Davies T. D., Baum J. K. (2012). Extinction risk and overfishing: reconciling conservation and fisheries perspectives on the status of marine fishes. Sci. Rep. 2 (1), 1–9. doi: 10.1038/srep00561
Dietl G. P., Flessa K. W. (2017). “Conservation paleobiology,” in Science and practice (Chicago, IL: University of Chicago Press).
Dulvy N. K., Pacoureau N., Rigby C. L., Pollom R. A., Jabado R. W., Ebert D. A., et al. (2021). Overfishing drives over one-third of all sharks and rays toward a global extinction crisis. Curr. Biol. 31 (21), 4773–4787.e4778. doi: 10.1016/j.cub.2021.08.062
Finnegan S., Anderson S. C., Harnik P. G., Simpson C., Tittensor D. P., Byrnes J. E., et al. (2015). Paleontological baselines for evaluating extinction risk in the modern oceans. Science 348 (6234), 567–570. doi: 10.1126/Science.aaa6635
Gallmetzer I., Haselmair A., Tomašových A., Stachowitsch M., Zuschin M. (2017). Responses of molluscan communities to centuries of human impact in the northern Adriatic Sea. PloS One 12 (7), e0180820. doi: 10.1371/journal.pone.0180820
Gardiner L. (2001). Stability of late Pleistocene reef mollusks from San Salvador island, Bahamas. Palaios 16 (4), 372–386. doi: 10.1669/0883-1351(2001)016<0372:SOLPRM>2.0.CO;2
Greenstein B. J., Pandolfi J. M., Curran A. (1998). “The completeness of the Pleistocene fossil record: implications for stratigraphic adequacy,” in The adequacy of the fossil record. Eds. Donovan S. K., Pau C. R. C. (London: John Wiley and Sons), 75–109.
Gutiérrez D., Akester M., Naranjo L. (2016). Productivity and sustainable management of the Humboldt current Large marine ecosystem under climate change. Environ. Dev. 17 (Supplement 1), 126–144. doi: 10.1016/j.envdev.2015.11.004
Gutiérrez T. M., Castillo P. J., Naranjo B. L., Akester M. J. (2017). Current state of goods, services and governance of the Humboldt current Large marine ecosystem in the context of climate change. Environ. Dev. 22, 175–190. doi: 10.1016/j.envdev.2017.02.006
Halpern B. S., Frazier M., Potapenko J., Casey K. S., Koenig K., Longo C., et al. (2015). Spatial and temporal changes in cumulative human impacts on the world’s ocean. Nat. Commun. 6 (1), 1–7. doi: 10.1038/ncomms8615
Halpern B. S., Longo C., Hardy D., McLeod K. L., Samhouri J. F., Katona S. K., et al. (2012). An index to assess the health and benefits of the global ocean. Nature 488 (7413), 615–620. doi: 10.1038/nature11397
Halpern B. S., Walbridge S., Selkoe K. A., Kappel C. V., Micheli F., D’Agrosa C., et al. (2008). A global map of human impact on marine ecosystems. Science 319 (5865), 948–952. doi: 10.1126/Science.1149345
Head M. J. (2019). Formal subdivision of the Quaternary System/Period: Present status and future directions. Quaternary Int. 500, 32–51. doi: 10.1016/j.quaint.2019.05.018
Heithaus M. R., Frid A., Wirsing A. J., Worm B. (2008). Predicting ecological consequences of marine top predator declines. Trends Ecol. Evol. 23 (4), 202–210. doi: 10.1016/j.tree.2008.01.003
Herm D. (1969). Marines Pliozän und Pleistozän in Nord und Mittel-Chile Unter Besonderer Berücksichtigung der Entwicklung der Mollusken-Faunen. Zitteliana 2, 1–159.
Hijmans R. J. (2022) Raster: Geographic data analysis and modeling. R package version 3. Available at: https://CRAN.R-project.org/package=raster.
Ho S. L., Mollenhauer G., Lamy F., Martínez-Garcia A., Mohtadi M., Gersonde R., et al. (2012). Sea Surface temperature variability in the Pacific sector of the Southern Ocean over the past 700 kyr. Paleoceanography 27 (4), 1–15. doi: 10.1029/2012PA002317
Hsieh T., Ma K., Chao A. (2016). iNEXT: an R package for rarefaction and extrapolation of species diversity (Hill numbers). Methods Ecol. Evol. 7 (12), 1451–1456. doi: 10.1111/2041-210X.12613
Hubbell S. P. (2001). The unified neutral theory of biodiversity and biogeography (Princeton, NJ: Princeton University Press).
Ivany L. C. (1996). Coordinated stasis or coordinated turnover? exploring intrinsic vs. extrinsic controls on pattern. Palaeogeog. Palaeoclimatol. Palaeoecol. 127 (1-4), 239–256. doi: 10.1016/S0031-0182(96)00098-3
Ivany L. C., Brett C. E., Wall H. L., Wall P. D., Handley J. C. (2009). Relative taxonomic and ecologic stability in Devonian marine faunas of New York state: a test of coordinated stasis. Paleobiology 35 (4), 499–524. doi: 10.1666/0094-8373-35.4.499
Jackson J. B. (2001). What was natural in the coastal oceans? Proc. Natl. Acad. Sci. 98 (10), 5411–5418. doi: 10.1073/pnas.091092898
Jackson J. B., Kirby M. X., Berger W. H., Bjorndal K. A., Botsford L. W., Bourque B. J., et al. (2001). Historical overfishing and the recent collapse of coastal ecosystems. Science 293 (5530), 629–637. doi: 10.1126/Science.1059199
Jouffray J.-B., Blasiak R., Norström A. V., Österblom H., Nyström M. (2020). The blue acceleration: the trajectory of human expansion into the ocean. One Earth 2 (1), 43–54. doi: 10.1016/j.oneear.2019.12.016
Kidwell S. M. (2002). Time-averaged molluscan death assemblages: Palimpsests of richness, snapshots of abundance. Geology 30 (9), 803–806. doi: 10.1130/0091-7613(2002)030<0803:TAMDAP>2.0.CO;2
Kidwell S. M. (2007). Discordance between living and death assemblages as evidence for anthropogenic ecological change. Proc. Natl. Acad. Sci. 104 (45), 17701–17706. doi: 10.1073/pnas.0707194104
Kidwell S. M. (2015). Biology in the anthropocene: Challenges and insights from young fossil records. Proc. Natl. Acad. Sci. 112 (16), 4922–4929. doi: 10.1073/pnas.1403660112
Kidwell S. M., Bosence D. W. (1991). “Taphonomy and time-averaging of marine shelly faunas,” in Taphonomy: Releasing the data locked in the fossil record. Eds. Allison P. A., Briggs D. E. (New York: Plenum Press), 115–209.
Kidwell S. M., Flessa K. W. (1995). The quality of the fossil record - populations, species, and communities. Annu. Rev. Ecol. Systemat. 26, 269–299. doi: 10.1146/annurev.es.26.110195.001413
Kidwell S. M., Holland S. M. (2002). The quality of the fossil record: Implications for evolutionary analyses. Annu. Rev. Ecol. Systemat. 33 (1), 561–588. doi: 10.1146/annurev.ecolsys.33.030602.152151
Kidwell S. M., Tomasovych A. (2013). Implications of time-averaged death assemblages for ecology and conservation biology. Annu. Rev. Ecol. Evol. Systemat. 44, 539–563. doi: 10.1146/annurev-ecolsys-110512-135838
Kiessling W., Raja N. B., Roden V. J., Turvey S. T., Saupe E. E. (2019). Addressing priority questions of conservation Science with palaeontological data. Philos. Trans. R. Soc. B 374 (1788), 20190222. doi: 10.1098/rstb.2019.0222
Knowlton N., Jackson J. B. C. (2008). Shifting baselines, local impacts, and global change on coral reefs. PloS Biol. 6 (2), e54. doi: 10.1371/journal.pbio.0060054
Kosnik M. A., Kowalewski M. (2016). Understanding modern extinctions in marine ecosystems: the role of palaeoecological data. Biol. Lett. 12 (4), 20150951. doi: 10.1098/rsbl.2015.0951
Kowalewski M. (2017). “The youngest fossil record and conservation biology: Holocene shells as eco-environmental recorders,” in Conservation paleobiology: Science and practice. Eds. Dietl G. P., Flessa K. W. (Chicago and London: The University of Chicago Press), 7–29.
Kowalewski M., Serrano G. E. A., Flessa K. W., Goodfriend G. A. (2000). Dead delta’s former productivity: two trillion shells at the mouth of the Colorado river. Geology 28 (12), 1059–1062. doi: 10.1130/0091-7613(2000)28<1059:DDFPTT>2.0.CO;2
Lester S. E., Halpern B. S., Grorud-Colvert K., Lubchenco J., Ruttenberg B. I., Gaines S. D., et al. (2009). Biological effects within no-take marine reserves: a global synthesis. Mar. Ecology-Progress Ser. 384, 33–46. doi: 10.3354/meps08029
Marquardt C., Lavenu A., Ortlieb L., Godoy E., Comte D. (2004). Coastal neotectonics in southern central Andes: uplift and deformation of marine terraces in northern Chile (27 degrees s). Tectonophysics 394 (3-4), 193–219. doi: 10.1016/j.tecto.2004.07.059
Martinelli J. C., Soto L. P., González J., Rivadeneira M. M. (2017). Benthic communities under anthropogenic pressure show resilience across the Quaternary. R. Soc. Open Sci. 4 (9), 170796. doi: 10.1098/rsos.170796
McGill B. J., Hadly E. A., Maurer B. A. (2005). Community inertia of Quaternary small mammal assemblages in North America. Proc. Natl. Acad. Sci. United States America 102 (46), 16701–16706. doi: 10.1073/pnas.0504225102
Miloslavich P., Klein E., Díaz J. M., Hernandez C. E., Bigatti G., Campos L., et al. (2011). Marine biodiversity in the Atlantic and Pacific coasts of South America: knowledge and gaps. PloS One 6 (1), e14631. doi: 10.1371/journal.pone.0014631
Moreno C. A. (2001). Community patterns generated by human harvesting on Chilean shores: A review. Aquat. Conservat. Mar. Freshw. Ecosyst. 11 (1), 19–30. doi: 10.1002/aqc.430
Moreno C. A., Sutherland J. P., Jara H. F. (1984). Man as a predator in the intertidal zone of southern Chile. Oikos 42 (2), 155–160. doi: 10.2307/3544787
Myers R. A., Baum J. K., Shepherd T. D., Powers S. P., Peterson C. H. (2007). Cascading effects of the loss of apex predatory sharks from a coastal ocean. Science 315 (5820), 1846–1850. doi: 10.1126/Science.1138657
Oksanen J., Blanchet F. G., Friendly M., Kindt R., Legendre P., McGlinn D., et al. (2020) Vegan: Community ecology package. R package version 2. Available at: http://CRAN.R-project.org/package=vegan.
Olszewski T. D., Kidwell S. M. (2007). The preservational fidelity of evenness in molluscan death assemblages. Paleobiology 33 (1), 1–23. doi: 10.1666/05059.1
Ortlieb L., Goy J. L., Zazo C., Hillaire-Marcel C. I., Vargas G. (1995). “Late Quaternary coastal changes in northern Chile. guidebook for a fieldtrip, 23-25 November 1995,” in II anual meeting of the international geological correlation program (IGCP), project 367 (Antofagasta: ORSTOM).
Pandolfi J. M. (1996). Limited membership in Pleistocene reef coral assemblages from the Huon peninsula, Papua New Guinea: Constancy during global change. Paleobiology 22 (2), 152–176. doi: 10.1017/S0094837300016158
Pandolfi J. (2002). Coral community dynamics at multiple scales. Coral Reefs 21 (1), 13–23. doi: 10.1007/s00338-001-0204-7
Pandolfi J. M., Bradbury R. H., Sala E., Hughes T. P., Bjorndal K. A., Cooke R. G., et al. (2003). Global trajectories of the long-term decline of coral reef ecosystems. Science 301 (5635), 955–958. doi: 10.1126/science.1085706
Pandolfi J. M., Jackson J. B. C. (2001). Community structure of Pleistocene coral reefs of Curacao, Netherlands Antilles. Ecol. Monogr. 71 (1), 49–67. doi: 10.1890/0012-9615(2001)071[0049:CSOPCR]2.0.CO;2
Pandolfi J. M., Jackson J. B. C. (2006). Ecological persistence interrupted in Caribbean coral reefs. Ecol. Lett. 9 (7), 818–826. doi: 10.1111/j.1461-0248.2006.00933.x
Paskoff R., Leonard E. M., Novoa J. E., Ortlieb L., Radtke U., Wehmiller J. (1995). “Field meeting in the la Serena-coquimbo bay area (Chile). guidebook for a fieldtrip, 27-28 November 1995,” in II anual meeting of the international geological correlation program (IGCP), project 367 (Antofagasta: ORSTOM).
Pauly D. (1995). Anecdotes and the shifting baseline syndrome of fisheries. Trends Ecol. Evol. 10, 430. doi: 10.1016/S0169-5347(00)89171-5
Pauly D., Christensen V., Dalsgaard J., Froese R., Torres F. (1998). Fishing down marine food webs. Science 279 (5352), 860–863. doi: 10.1126/Science.279.5352.860
Pauly D., Watson R., Alder J. (2005). Global trends in world fisheries: impacts on marine ecosystems and food security. Philos. Trans. R. Soc. B-Biol. Sci. 360 (1453), 5–12. doi: 10.1098/rstb.2004.1574
Pinnegar J. K., Engelhard G. H. (2008). The ‘shifting baseline’ phenomenon: A global perspective. Rev. Fish Biol. Fish. 18 (1), 1–16. doi: 10.1007/s11160-007-9058-6
Precht W. F., Aronson R. B. (2016). “Stability of reef-coral assemblages in the Quaternary,” in Coral reefs at the crossroads. Eds. Hubbard D. K., Rogers A. D., Lipps J. H., Stanley G. D. (Dordrecht, Netherlands: Springer), 155–173.
Radtke U. (1987). Marine terraces in Chile (22°-32°S) geomorphology, chronostratigraphy, and neotectonics: preliminary results II. Quaternary South America Antarctic Peninsula Rabassa J. (London, UK: CRC Press) 5, 239–256.
R Core Team (2022). “R: A language and environment for statistical computing” in R foundation for statistical computing(Vienna, Austria). Available at: http://www.R-project.org/.
Regard V., Saillard M., Martinod J., Audin L., Carretier S., Pedoja K., et al. (2010). Renewed uplift of the central Andes forearc revealed by coastal evolution during the Quaternary. Earth Planet. Sci. Lett. 297 (1-2), 199–210. doi: 10.1016/j.epsl.2010.06.020
Rick T. C., Lockwood R. (2013). Integrating paleobiology, archeology, and history to inform biological conservation. Conserv. Biol. 27 (1), 45–54. doi: 10.1111/j.1523-1739.2012.01920.x
Rivadeneira M. M. (2010). On the completeness and fidelity of the Quaternary bivalve record from the temperate Pacific coast of South America. Palaios 25 (1-2), 40–45. doi: 10.2110/palo.2009.p09-035r
Rivadeneira M. M., Carmona E. R. (2008). A late Pleistocene macrobenthic assemblage in Caleta Patillos, northern Chile: Paleoecological and paleobiogeographical interpretations. Rev. Geológica Chile 35 (1), 163–173. doi: 10.5027/andgeoV35n1-a08
Rivadeneira M. M., Nielsen S. N. (2017). Diversification dynamics, species sorting, and changes in the functional diversity of marine benthic gastropods during the Pliocene-Quaternary at temperate western South America. PloS One 12 (10), e0187140. doi: 10.1371/journal.pone.0187140
Rivadeneira M. M., Santoro C. M., Marquet P. A. (2010). Reconstructing the history of human impacts on coastal biodiversity in Chile: Constraints and opportunities. Aquat. Conservat. Mar. Freshw. Ecosyst. 20 (1), 74–82. doi: 10.1002/aqc.1051
Rodríguez M. P., Carretier S., Charrier R., Saillard M., Regard V., Hérail G., et al. (2013). Geochronology of pediments and marine terraces in North-central Chile and their implications for Quaternary uplift in the Western Andes. Geomorphology 180, 33–46. doi: 10.1016/j.geomorph.2012.09.003
Saillard M., Riotte J., Regard V., Violette A., Hérail G., Audin L., et al. (2012). Beach ridges U–Th dating in Tongoy bay and tectonic implications for a peninsula–bay system, Chile. J. South Am. Earth Sci. 40, 77–84. doi: 10.1016/j.jsames.2012.09.001
Saupe E., Hendricks J. R., Portell R., Dowsett H., Haywood A., Hunter S., et al. (2014). Macroevolutionary consequences of profound climate change on niche evolution in marine molluscs over the past three million years. Proc. R. Soc. London B: Biol. Sci. 281 (1795), 20141995. doi: 10.1098/rspb.2014.1995
Scarponi D., Kaufman D., Amorosi A., Kowalewski M. (2013). Sequence stratigraphy and the resolution of the fossil record. Geology 41 (2), 239–242. doi: 10.1130/G33849.1
Scarponi D., Nawrot R., Azzarone M., Pellegrini C., Gamberi F., Trincardi F., et al. (2022). Resilient biotic response to long-term climate change in the Adriatic Sea. Global Change Biol 28, 4041–4053. doi: 10.1111/gcb.16168
Scheffer M., Carpenter S., de Young B. (2005). Cascading effects of overfishing marine systems. Trends Ecol. Evol. 20 (11), 579–581. doi: 10.1016/j.tree.2005.08.018
Sougarret Muñoz J., Ríos Ther F. (2013). El Pescador en el imaginario científico durante la etapa de formación de la academia ictiológica chilena, 1829-1909. História Ciências Saúde-Manguinhos 20, 1621–1633. doi: 10.1590/S0104-597020130005000011
Thiel M., Macaya E. C., Acuna E., Arntz W. E., Bastias H., Brokordt K., et al. (2007). "The Humboldt Current System of northern and central Chile: Oceanographic processes, ecological interactions and socioeconomic feedback," in Oceanography and Marine Biology: An Annual Review. eds. R.N. Gibson, R.J.A. Atkinson, J.D.M. Gordon. (Boca Raton, FL: CRC Press), 45, 195–344.
Toth L. T., Stathakopoulos A., Kuffner I. B., Ruzicka R. R., Colella M. A., Shinn E. A. (2019). The unprecedented loss of florida’s reef-building corals and the emergence of a novel coral-reef assemblage. Ecology 100 (9), e02781. doi: 10.1002/ecy.2781
Tyler C. L., Schneider C. L. (2018). Marine conservation paleobiology (Cham, Switzerland: Springer).
Victor P., Sobiesiak M., Glodny J., Nielsen S., Oncken O. (2011). Long-term persistence of subduction earthquake segment boundaries: Evidence from Mejillones peninsula, northern Chile. J. Geophys. Res.: Solid Earth 116, B02402. doi: 10.1029/2010JB007771
Viechtbauer W. (2010). Conducting meta-analyses in R with the metafor package. J. Stat. Soft. 36 (3), 1–48. doi: 10.18637/jss.v036.i03
Keywords: shifting baselines, mollusk, fossil record, Chile, Peru, near-time, community structure, overfishing
Citation: Rivadeneira MM and Nielsen SN (2022) Deep anthropogenic impacts on benthic marine diversity of the Humboldt Current Marine Ecosystem: Insights from a Quaternary fossil baseline. Front. Mar. Sci. 9:948580. doi: 10.3389/fmars.2022.948580
Received: 23 May 2022; Accepted: 19 August 2022;
Published: 14 September 2022.
Edited by:
Fernando P. Lima, Centro de Investigacao em Biodiversidade e Recursos Geneticos (CIBIO-InBIO), PortugalReviewed by:
Oleg Mandic, Natural History Museum Vienna, AustriaCopyright © 2022 Rivadeneira and Nielsen. This is an open-access article distributed under the terms of the Creative Commons Attribution License (CC BY). The use, distribution or reproduction in other forums is permitted, provided the original author(s) and the copyright owner(s) are credited and that the original publication in this journal is cited, in accordance with accepted academic practice. No use, distribution or reproduction is permitted which does not comply with these terms.
*Correspondence: Marcelo M. Rivadeneira, bWFyY2Vsby5yaXZhZGVuZWlyYUBjZWF6YS5jbA==
Disclaimer: All claims expressed in this article are solely those of the authors and do not necessarily represent those of their affiliated organizations, or those of the publisher, the editors and the reviewers. Any product that may be evaluated in this article or claim that may be made by its manufacturer is not guaranteed or endorsed by the publisher.
Research integrity at Frontiers
Learn more about the work of our research integrity team to safeguard the quality of each article we publish.