Corrigendum: Ten lessons on the resilience of the EU common fisheries policy towards climate change and fuel efficiency - A call for adaptive, flexible and well-informed fisheries management
- 1National Institute of Aquatic Resources, Danish Technical University, Lyngby, Denmark
- 2MRAG Ltd., London, United Kingdom
- 3Wagenningen Marine Research, Wageningen University and Research, Ijmuiden, Netherlands
- 4Centre for Environmental Policy, Imperial College London, London, United Kingdom
- 5Thuenen-Institute of Sea Fisheries, Bremerhaven, Germany
- 6Sakana Consultants, Brest, France
- 7Ciencia y tecnología marina y alimentaria (AZTI), Marine Research, Basque Research and Technology Alliance (BRTA), Pasaia, Spain
- 8Department of Aquatic Resources, Swedish University of Agricultural Sciences, Lysekil, Sweden
- 9Natural England, London, United Kingdom
- 10Institute of Biodiversity and Ecosystem Research at the Bulgarian Academy of Sciences (IBER-BAS), Division of Functional Ecology and Bioresources of Marine and Coastal Ecosystems, Sofia, Bulgaria
- 11Flanders Research Institute for Agriculture, Fisheries and Food (ILVO), Fisheries and Aquatic Production, Oostende, Belgium
- 12Wageningen Economic Research, Wageningen University & Research, The Hague, Netherlands
- 13Spanish Institute of Oceanography (IEO-CSIC) (Palma), Ecosystem Oceanography Group (GRECO), Palma de Mallorca, Spain
- 14Department of Marine Sciences, University of the Aegean, Mytilene, Greece
- 15Blue Bio Consulting, Vyronas, Greece
- 16Institute of Marine Biological Resources and Inland Waters, Hellenic Centre for Marine Research, Anavyssos, Greece
To effectively future-proof the management of the European Union fishing fleets we have explored a suite of case studies encompassing the northeast and tropical Atlantic, the Mediterranean, Baltic and Black Seas. This study shows that European Union (EU) fisheries are likely resilient to climate-driven short-term stresses, but may be negatively impacted by long-term trends in climate change. However, fisheries’ long-term stock resilience can be improved (and therefore be more resilient to increasing changes in climate) by adopting robust and adaptive fisheries management, provided such measures are based on sound scientific advice which includes uncertainty. Such management requires regular updates of biological reference points. Such updates will delineate safe biological limits for exploitation, providing both high long-term yields with reduced risk of stock collapse when affected by short-term stresses, and enhanced compliance with advice to avoid higher than intended fishing mortality. However, high resilience of the exploited ecosystem does not necessarily lead to the resilience of the economy of EU fisheries from suffering shocks associated with reduced yields, neither to a reduced carbon footprint if fuel use increases from lower stock abundances. Fuel consumption is impacted by stock development, but also by changes in vessel and gear technologies, as well as fishing techniques. In this respect, energy-efficient fishing technologies already exist within the EU, though implementing them would require improving the uptake of innovations and demonstrating to stakeholders the potential for both reduced fuel costs and increased catch rates. A transition towards reducing fuel consumption and costs would need to be supported by the setup of EU regulatory instruments. Overall, to effectively manage EU fisheries within a changing climate, flexible, adaptive, well-informed and well-enforced management is needed, with incentives provided for innovations and ocean literacy to cope with the changing conditions, while also reducing the dependency of the capture fishing industry on fossil fuels. To support such management, we provide 10 lessons to characterize ‘win-win’ fishing strategies for the European Union, which develop leverages in which fishing effort deployed corresponds to Maximum Sustainable Yield targets and Common Fisheries Policy minimal effects objectives. In these strategies, higher catch is obtained in the long run, less fuel is spent to attain the catch, and the fisheries have a higher resistance and resilience to shock and long-term factors to face climate-induced stresses.
Introduction
Marine ecosystems have inherent variation that interacts and combines with fishing impacts and natural environmental variability to affect fishing opportunities (e.g., Planque et al., 2010; Bahri et al., 2021; Bastardie et al., 2021). Thus, stocks can fluctuate independently of fishing, e.g., due to environmental and internal demographic properties (Hjort, 1914; Bjørnstad and Grenfell, 2001; Kjesbu et al., 2022), with shifts in productivity impacting over 70% of stocks (Vert-pre et al., 2013). Such variability is even more challenging from a management perspective when significant environmental changes can result in the marine ecosystem either departing from historical ranges or trending to new levels (Beaugrand, 2004; Beare et al., 2004, Peck and Pinnegar, 2018, Pinsky et al., 2018; Baudron et al., 2020; O'Leary et al., 2022). Trending toward unknown ecosystem status and adding more unpredictability in stock trajectories and their environmental drivers result in more uncertain advice on suitable catch levels. Such unpredictability may increase when the stocks are impacted by shocks, including climate change and associated extreme events, with little understanding of the short- and long-term implications on their dynamics (but see, Free et al., 2019).
Within the European Union (EU), policymakers intend to follow a precautionary approach for managing fisheries (EU, 2013a, Art. 2), though such management is structured by contrasting socio-ecological system priorities. For example, Northeast Atlantic stocks (managed under the ICES scientific advice framework; ICES, 2020) integrate ecosystem-based management intending to achieve Maximum Sustainable Yield (MSY), while fisheries management with the Mediterranean and Black Sea (under the General Fisheries Commission for the Mediterranean, GFCM) focuses on reversing the generalized overexploitation state of most Mediterranean stocks without quota management, by applying a diversity of measures to reduce fishing effort (FAO, 2020; STECF, 2022b). In the Northeast Atlantic, fisheries involve mainly large boats and therefore are more easily regulated by catch allowances (quotas) that complement technical measures (minimum conservation reference size, closed seasons and areas, area-based and species gear selectivity requirements). In the Mediterranean, the fishing industry is diverse, encapsulating a large number of small boats utilizing a diversity of gears and landing sites and capturing an array of species, to a large polyvalent fleet under effort control and technical measures. As in tropical areas (EU Outermost regions), large seiners fisheries for tuna and swordfish under quota management are also apparent within the Mediterranean. With such a diverse array of different management protocols established throughout the EU, a precautionary approach is required to support the development of flexible and agile fishing strategies to maintain long-term productivity, economic returns and stock resistance within the array of fisheries to increasing climate associated shocks and climate associated threats.
Due to the increasing concerns regarding climate change, there is growing interest in reducing fossil fuel dependency and the contribution of the fishing sector to global emissions of greenhouse gases (GHGs) (FAO, 2015). Despite this, a clear pathway to achieve such reductions is still lacking (but see Ziegler and Hansson, 2003; Schau et al., 2009; Suuronen et al., 2012; Poos et al., 2013; Pelletier et al., 2014; Parker and Tyedmers, 2015, Parker et al., 2018, Fiorella et al., 2021; Bastardie et al., 2022). Within Europe, although there has been a renewed interest in reducing fuel dependency given new environmental targets (EU targets for 2030) and enhanced energy sovereignty, there is still little assessment of the measures needed to achieve such independence. This is despite the EU Common Fisheries Policy (CFP in EU, 2013 Art. 17) stipulating that allocation of catch or effort allowances should also consider respective EU Member State’s fleet fuel use and energy efficiency. However, there is increasing evidence to suggest a correlation between reduced fuel use and stock recovery (Hornborg and Smith, 2020; Byrne et al., 2021; Bastardie et al., 2022). Therefore, seeking a reduction of the fuel use and GHG emissions appears to be interlinked with the capacity of the fisheries and the ability of the stocks they fish upon to resist and recover from climate-induced stresses and shocks. How best to implement fuel use efficiency will also depend on the range of technologies available, but there is still little consensus on the availability of such technologies and their applicability to the fishing industry. Within the EU, there is still little understanding of the range of technologies potentially available to the fishing industry to optimize fuel use and efficiency.
In this study, we first tested the resilience of fisheries and marine ecosystems managed under the current EU CFP to abrupt environmental change and examined how management may mitigate the impacts of climate-induced stresses. In a second part of this work, we evaluated to which extent fishing strategies aimed at rebuilding stocks can help improve energy use and efficiency, decrease the dependency of highly dependent fisheries on fuel use, increase fisheries profitability and ensure stable yields. In the final part, we investigated how reduction of GHG emissions can be achieved by technical means. Our present study recognizes the need to change the fisheries management paradigm from developing scientific capability that helps to “predict and act” to framework evaluations that make it possible to “learn and adapt”. By deploying a suite of simulation studies, we intend to extrapolate stock trajectories to domains where there is some uncertainty about the central parameter values (i.e., future stock productivity, spatial distribution, fuel-saving potentials), and describe possible courses of the system, depending upon a choice of parameter values that are likely to be impacted by the climate change effects. As Harfoot et al. (2014) and Mouquet et al. (2015) describe, these anticipatory predictions are not meant to represent the actual future but mimic changes in an integrated evaluation. The fact that the projections do not match reality does not count against the validity of the underlying hypotheses. Instead, the simulation studies should be regarded as a guide for present action, with a renewed governance that enables learning and adapting when facing shocks and long-term changes induced by climate change on marine species and ecosystems. Using this work, we provide 10 ‘win-win’ fishing strategy lessons to contribute to the foundations for a renewed fishery management in Europe. Win-win strategies would develop leverages in which fishing effort deployed corresponds to MSY targets and CFP minimal effects objectives. In these strategies, higher catch is obtained, less fuel is spent to attain the catch, and the fishery has a higher resistance and resilience to shock factors to face climate-induced stresses.
Methods
A suite of case studies focusing on different EU stocks and undertaking simulation, data investigation and predictive modelling were undertaken. We present the lessons learnt from the synthesis of these works (Table 1) and provide in-depth descriptions of the methods used and the main outcomes of each case study (Annexes 1 to 14 in Supplementary Material). Using a range of case studies across the EU provides a substantial assessment of the potential role of climate in impacting fisheries within this region. Detailed information regarding the extent of data on the environmental drivers per area and the stock specifications we have used in the simulations are detailed within the Annexes.
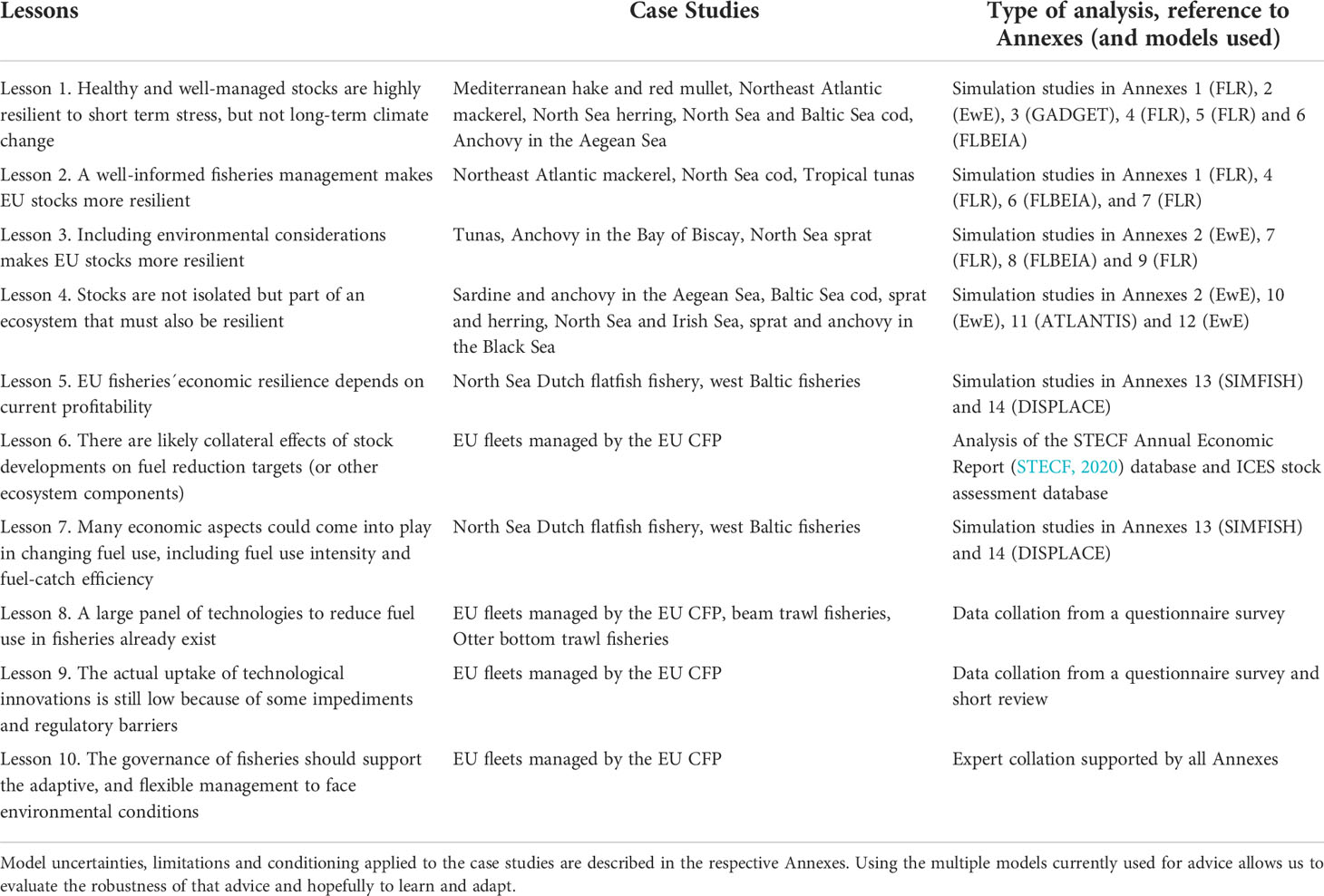
Table 1 Listing 10 lessons learnt from case studies on the resilience of the EU CFP to climate change-induced stresses and long term trends, and references to Annexes and models used among the Fishery library in R (FLR, Kell et al., 2007), the Fishery Library for Bioeconomic Impact Assessment (FLBEIA, Garcia et al., 2017), the Globally Applicable Area Disaggregated General Ecosystem Toolbox (GADGET, Begley, 2017), Ecopath with Ecosim (EwE, Christensen and Pauly, 1992), the ATLANTIS model (Fulton et al., 2011), the SIMFISH model (Bartelings et al., 2015), the DISPLACE model (Bastardie et al., 2014).
Plausible scenarios and simulation studies
The impact of climate change-induced stresses in structuring stock trajectory was investigated using plausible, ecosystem-coherent scenarios over a span of 10 to 30 years. To develop such plausible scenarios, the nature and direction of impact on stocks of a range of environmental factors were categorized using a rigorous literature review (see more details on methods in CINEA, 2022, Annexes). Importantly, this assessment showed that changes in a range of environmental factors (excluding fishing) are impacting different fish stocks’ demographic parameters (e.g., Mackenzie et al., 2007; Brunel and Dickey-Collas, 2010; Hsieh et al., 2010; Hidalgo et al., 2011; Pécuchet et al., 2015; Brosset et al., 2017; Daskalov et al., 2017; Clausen et al., 2018; Rak et al., 2020; Wu et al., 2020; Köster et al., 2020; Wåhlström et al., 2020; Peck et al., 2021; van de Wolfshaar et al., 2022) and spatial distribution (Ciannelli et al., 2013; Druon et al., 2015; Gucu et al., 2017; Erauskin-Extramiana et al., 2019; Baudron et al., 2020). However, most literature focusing on EU stocks has shown that increased water temperature has been the most impactful environmental parameter, with fluctuations in recruitment dynamics the most likely output of such environmental change (e.g., Drinkwater, 2005; Bartolino et al., 2008; Maynou et al., 2014; Zimmermann et al., 2019; Kühn et al., 2021; Kjesbu et al., 2022). Therefore, the simulations studies within this work focused on examine the role of increased water temperature (i.e., climate shock) on recruitment (i.e., demographic parameter). We predominantly explore these two factors (temperature and its impact on recruitment) in these studies, as in most cases the literature did not hold enough material to assume coherent and accurate relationships between other environmental and demographic factors. Despite this, within several case studies we examined other environmental factors (e.g., currents, oxygen, water salinity, primary production) and their effect on stock productivity (recruits, growth, maturity, natural mortality) and delineating the species’ spatial distribution whenever these factors had been identified for such respective stocks within the literature. Overall, we examined a suite of different fish stocks and deployed several models with different assumptions about the resolution (age-structured models, single stock models or food web models, spatial models, or end-to-end models, etc.), accounting for linkages (e.g., the temperature effect on recruitment strength), and feedback loops (e.g., trophodynamics) which were organized in a management strategy evaluation framework (in the sense of Kell et al., 2007, Punt et al., 2016) (discussed in detail in Annexes 1 to 14).
The predicted long-term environmental changes (i.e., future baseline environments for stocks) and short-term climatic anomalies (i.e., climate shocks) used in scenarios were based on past oceanographic data and projections to account for viable alternative ocean environment pathways (defined by the IPCC: RCP 4.5, RCP 8.5). We set the time horizon in the simulation studies to 10 to 30 years to capture the possible long-term effect of path divergence between the two IPCC scenarios (30 years). We used past studies to form scenarios even if there were inherent uncertainties in future climate conditions. For example, for the Baltic Sea, although there may be a clear trend in sea surface temperature, the trend in water salinity is less evident in the south and central part of the basin, due to expected higher rain and river runoff being counteracted by more inflows of saline waters from the North Sea (Saraiva et al., 2019). We used environmental projections (see Annexes) based on physical-biogeochemical models developed by Sein et al. (2015) for the North Sea, Sakalli and Başusta (2018) for the Black Sea, Taylor et al. (2012) for the Aegean Sea and Jungclaus et al. (2013) for the Irish Sea. We identified only a few prior studies demonstrating short-term environmental shocks affecting fish stocks, and they were not necessarily linked to stresses related to climate change (Peck et al., 2021). The stresses ranged from the effect of storms, anomalies in hydrological conditions, invasive species and toxic algal bloom. These stresses mainly had adverse effects, except in severe winters in the North Sea that resulted in high recruitments of flatfish (van der Veer et al., 2000). Hence, we assumed that short-term anomalies would occur more frequently or with more intensity under climate change, affecting biological aspects impacted by environmental factors, with the nature and magnitude of the effects captured in ad hoc relationships described in each case study (see Annexes).
The effect of short-term environmental shocks on selected fish stocks was evaluated against the selected stocks’ resources and ecosystems, as well as the economic resilience of the fishing fleets. Resource resilience was defined as the ability of fish stocks to withstand the adverse impact of a shock and remain above biomass limits (Blim) at which productivity is impaired, and to its ability to rebuild to biomass levels that correspond to management targets (the lower bound of spawning-stock biomass fluctuation when fished at the maximum rate of fishing mortality: MSY Btrigger in the International Council for the Exploration of the Sea (ICES) and BMSY in other Regional Fisheries Management Organisations (RFMOs)).
As exploited stocks do not develop in isolation, we also looked at the resilience of the marine ecosystem. Ecosystem resilience was defined as the capacity of the ecosystem to absorb disturbance and reorganize to retain the same broad function, structure, identity and feedback (i.e., stability and compensatory effects, Figure 1). Finally, the economic resilience of fishing fleets was defined as their ability to withstand short-term economic stresses, which, in addition to the biological component, measure the risk of attaining negative economic returns (Figure 1).
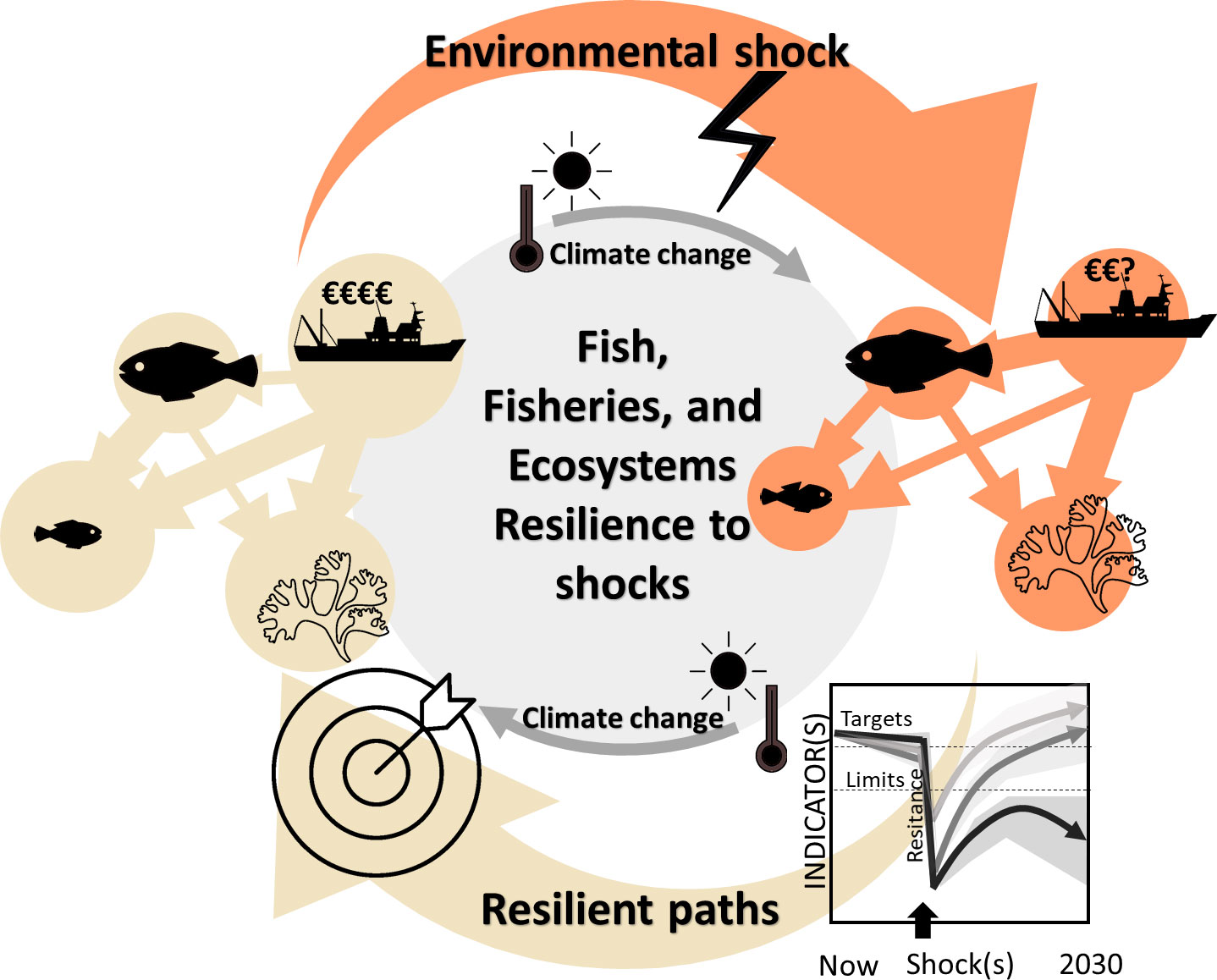
Figure 1 Conceptual figure on potential for resources, ecosystems and economic resilience, showing the transition from an initial state (left) to a degraded state (right) induced by short-term climate-induced stresses and long-term climate change effects, and back to the initial state along with recovery when reaching targets or exceeding precautionary limit levels set by fisheries management strategies. Resource resilience focused on individual stock components, Ecosystem resilience focused on the unaltered interactions between individual components, including the fishing activities themselves, while Economic resilience focused on assessing the resilience of fishing activities individually. Arrows and their size in-between circles represent trophodynamic links and their magnitude between fish stocks, supporting habitats, and fleets, including the fleets’ economy, altered by climate-induced shocks and long-term trends in climate change.
Reconstruction of energy use time series and link to stock status
We examined the state of play of energy use within the EU fishing industry between 2002 and 2021. Such economic data is annually collected across the Member States (STECF, 2020, Annual Economic Report [AER]), being aggregated at the EU fleet level, with yearly costs and earnings (including energy costs) and the total amount of consumed fuel collected. This data source, which is different from the ones used in the simulation studies, gives a standard aggregation per fleet, making a comparison of fleet estimates possible across our case studies. Also, not all case studies informed the fishing fleets or their fuel use.
We analyzed the retrieved data to find correlations between fuel use and stock status of all stocks evaluated within ICES (www.ices.dk) and monitored by the CFP (STECF, 2021a). In this respect, for each stock (http://standardgraphs.ices.dk/stockList.aspx), we created a stock health status indicator ranging from 0 to 1. To maximize the number of stocks for which the indicator could be evaluated, we based our calculations on the annual stock biomass indicator reported by ICES. Depending on the stock, this indicator can be the Spawning Stock Biomass (SSB), an abundance index or any other metrics defined by ICES working groups. We determined the maximum of the stock biomass indicator for each stock, allowing us to normalize the annual stock status indicator to 1. Because most EU fleets fish on an array of species, we combined the stocks’ status by the fleet to obtain a combined targeted stock status for each fleet-segment. This was obtained by multiplying a given species stock biomass indicator by the ratio of the landings of a given fleet derived from a given species over the total landings of that species. In addition, for each EU fleet, we calculated the landings per unit of effort as the ratio between total landings and effort expressed in days at sea (this was the only effort metric available in the retrieved STECF data but is considered a good proxy for fuel use). We have calculated these indicators for all fleets identified in the economic data collection, though we utilize only the fleets with more than 50% of their landings assessed by ICES.
Review of technological innovations for reducing fuel use
To understand the range of energy efficiency technologies which could be applied to EU fishing fleets, we used a rigorous literature review complemented by consultation with scientific and commercial stakeholders. Information in published peer-reviewed scientific studies was accessed through a dedicated search in the Scopus literature database on 23 February 2021. Additionally, non-peer-reviewed or unpublished studies and project reports were identified through in-house expert knowledge. The review highlighted that the factors that affect fisheries energy consumption and efficiency can be classified as human, biological, technological and political, and include the target species, status of the stock, fish quotas, quota allocation policies, harvesting methods, distance to the fishing ground, fleet age, skipper effect, sale system and fuel cost, technical regulations and spatial and temporal limitation of fisheries, structural policies, and the availability of fuel subsidies/taxes (Sala et al., 2012; Jafarzadeh et al., 2016; Villasante et al., 2022). The consultation with stakeholders aimed to collect information regarding the experiences undertaken on board fishing vessels on reducing fuel consumption or GHG emissions and their views on the subject. Two stakeholder groups were targeted: scientists and commercial stakeholders.
Results
Lesson 1. Healthy and well-managed stocks are highly resilient to short term stress, but not long-term climate change
As found in several case studies, when stocks are poorly managed, they show low resilience to short-term stress (i.e., temperature increase resulting in short-term recruitment failure). For example, hake in the Mediterranean, when undergoing a shock, showed a substantial decline in productivity (Annex 1, Mediterranean red mullet and hake). Such demographic impacts of a shock are exacerbated by the current depletion of the stock associated with overfishing. Hake is overexploited in the Mediterranean due to fishing mortality being ten times higher than the optimal target (FAO, 2020), and such decline will continue with or without a shock. Such overexploitation truncates the population size structure by removing the larger animals, further jeopardizing future hake productivity (recruitment and growth) (Hidalgo et al., 2012). In addition to this, the stock identity delineation poses a challenge to fisheries management. Hence, maintaining hake exploitation within safe limits will also depend on management considering only one or, on the contrary, several separated hake stocks in the west Mediterranean. If considering one stock would likely make it easier to avoid redefining biological reference points too frequently, fully considering the complex structure of hake distribution in subpopulations would, on the other hand, steer toward more resilient hake subpopulations at the scale of the western Mediterranean.
To ensure stocks are well managed, fishing pressure should not exceed rates of maximum yield (FMSY). In this respect, within the Mediterranean, red mullet is fished at the target level, and although populations were negatively impacted by shock, these recover and can be termed relatively resilient to such shocks (Annex 1). In line with such management of red mullet, within the Aegean Sea, when sardine biomass is fished at optimum levels, such populations are anticipated to stabilize at much higher levels, making the shocks much less impactful to the structure of populations (Annex 2 Aegean Sea fisheries).
Within the Baltic Sea, the shock directly impacted populations of cod, herring and sprat. However, expected environmental changes in this sea will substantially impact the magnitude of such shocks (Annex 3, Baltic Sea fisheries). In this respect, there were moderate differences in cod levels resulting from alternative climate change scenarios, while sea warming led to enhanced sprat recruitment. For cod, plausible levels of future Baltic Sea eutrophication had a larger impact than climate on the productivity of the stock via an effect on the size of the suitable habitat (so-called “cod reproductive volume”). The impact of a shock was more pronounced on cod than herring and sprat, primarily due to the poor status of cod and low recruitment. In addition, such low productivity and a truncated cod population size structure are likely the cause of a faster drop after the shock than in herring and a longer recovery time. Despite a single shock having a low to moderate impact on populations for both herring and sprat and was unlikely to bring biomass below precautionary reference levels, they had improved response to the perturbation when fishing exploitation was reduced.
Ensuring that management decisions on fishing opportunities align with scientific advice provides the best insurance for stock resilience with increasing changes in climate. For example, although Northeast Atlantic (NEA) mackerel stock holds substantial biomass, this stock is poorly managed, making the stock more sensitive to shock effects (Annex 4, NEA mackerel). Discrepancies between ICES advice and realized catch for North-East Atlantic mackerel may arise from changes in the spatial extent of stocks, especially when such changes overlap with different Member States’ EEZs. Even if there is good knowledge of changes in the stock and fishery, and the stock assessment is considered to be acceptable, such spatial changes in stock structure may induce TAC overshoot compared to ICES catch advice, due to a lack of international agreement between EU and non-EU countries (Annex 4). In comparison, the shock did not substantially impact population dynamics for North Sea herring stock, the TAC being aligned with the scientific advice. Such lack of change is masked by population impacts being driven by long-term climate change (increased temperature leading to low recruitment levels toward a new lower SBB level), with the effect of the shock acting in conjunction (not in addition) to long-term climate change, preventing herring stock from recovering to its initial abundance level (Annex 5, North Sea herring).
Notably, after a stock has suffered from a climate-induced shock, following scientific advice on harvesting and enforcing the catch limits will benefit the stock’s resilience. For example, despite North Sea cod stocks being overfished, rapid recovery of the spawning biomass (SSB) to healthy levels was projected if total allowable catch (TAC) quotas were followed (see Annex 6, North Sea mixed fisheries and cod). Since cod is the main choke species for many fleets, other stocks are also maintained in good stock status under the limited fishing effort. Furthermore, North Sea cod was observed to recover from recruitment shocks within the short-term (Figure 2 left); however, this resilience is lost when combined with long-term climate change trends due to productivity changes in the stock (Figure 2 right). This shift in equilibria toward new levels shows the need for adaptive fisheries management, where biological reference points are regularly revised to changing productivity regimes (see also Lesson 10) (Annex 6).
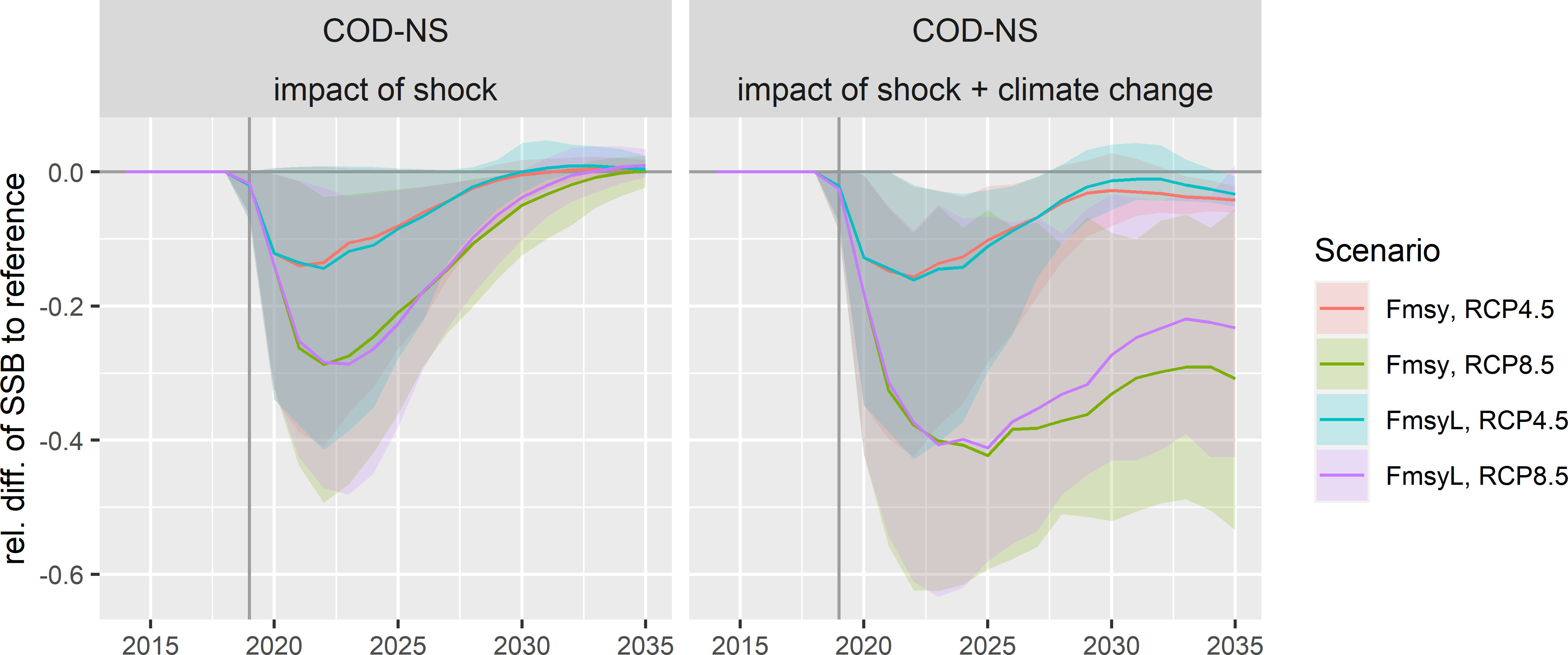
Figure 2 Relative spawning stock biomass (SSB) of North Sea cod (COD-NS) following recruitment shocks as compared to their respective non-shock scenarios. Trajectories are presented by climate change and target F scenarios. The impact of the shocks alone (left) is illustrated by comparing the SSB of the shock scenarios to a non-shock reference that includes the long-term climate changes of the RCP4.5 and RCP8.5 scenarios. The impact of the shocks plus climate change effects (right) compares to a non-shock reference that excludes the long-term climate changes, emphasizing both the shock impact and long-term changes in SSB equilibria. (see Annex, North Sea mixed fisheries and cod for further details).
Lesson 2. A well-informed fisheries management makes EU stocks more resilient
Well-informed fisheries management reduces the mismatch between target F (and quota) and realized F. For example, within Annex 7 (Tropical tunas) bigeye and yellowfin tuna stocks were found to be sensitive to changes in natural mortality (M) and in virgin biomass (i.e. carrying capacity K) (Figure 3 and see Lesson 3). Despite this, current catch levels are set higher than those required to achieve BMSY, and in these circumstances, climate-induced shocks were shown to have significant impacts. Similarly, hake and red mullet stocks in the Mediterranean Sea (Annex 1) showed differences in response to shocks, linked to differences in individual body condition between species. Hence, large changes are seen in recent stock level estimates for the red mullet. This may indicate issues in the scientific assessment, such as missing data or changes in biological parameters, natural mortality, recruitment, stock distribution, or fishery operations. All of which is likely under the effect of climate change (Annex 1). In addition to this, misspecifications about the age structure of future catches can also have large consequences in terms of the fishing effort required to uptake the quota as the same catch allowance in kg represent a different number of individuals of different ages (Annex 6).
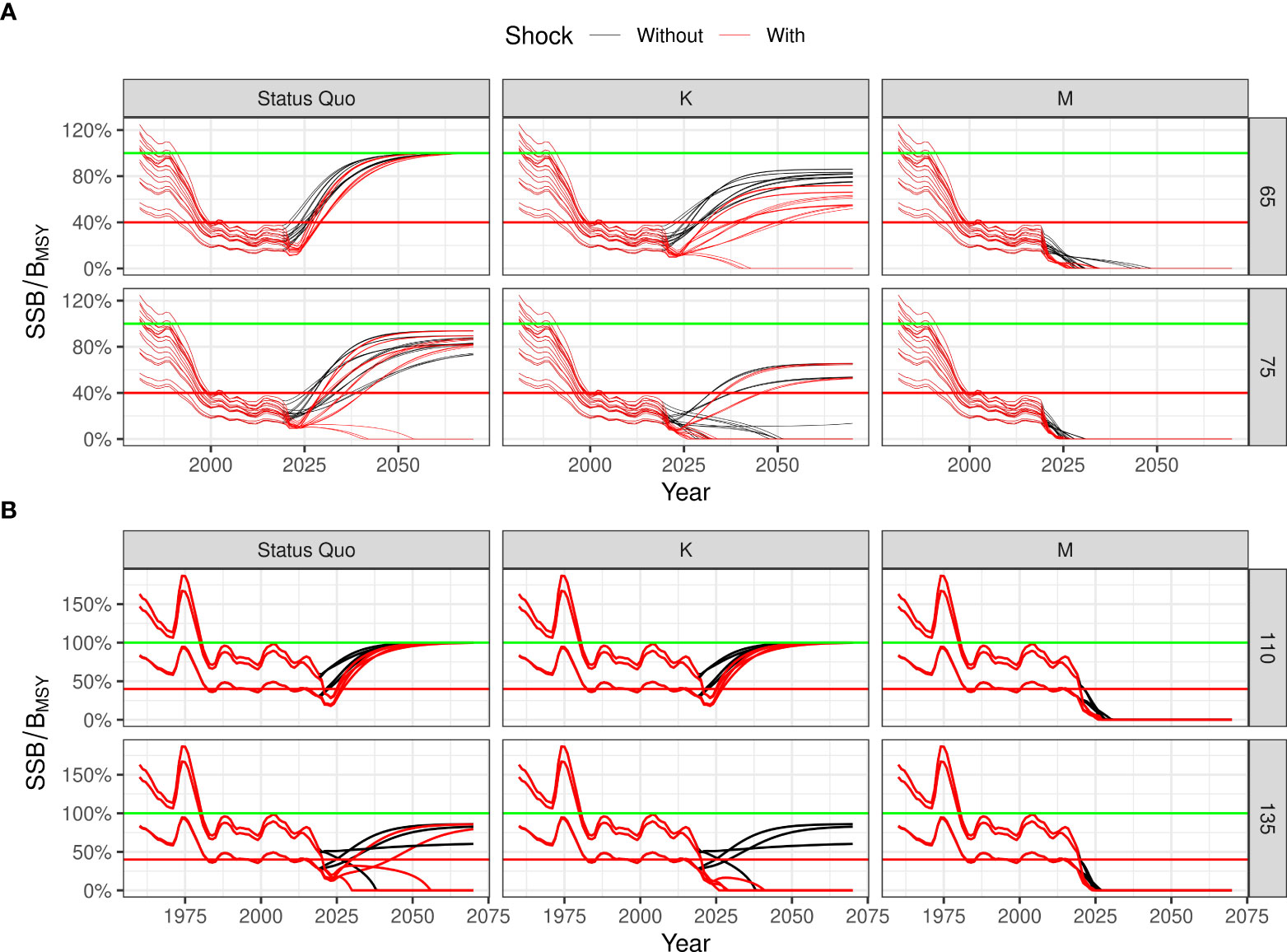
Figure 3 Tropical tunas projections for sub-cohort that spawns in the quarter with and without shock for (A) bigeye and (B) yellowfin, for climate scenarios (column) and catch levels in ´000 tonne (row); the green line is the target level (BMSY) and the red line gives the limit (40%). Projections were run for three scenarios corresponding to i) Status Quo (no change in future parameters), iii) negative trend in Virgin Biomass (K) from 2020 onward, and iii) a positive trend in natural mortality (M) and growth for 2020 onwards. In addition, a shock is applied, i.e., an increase in M of 150% in 2020 in 2 seasons (see Annex 7, Tropical tunas for further details).
Lesson 3. Including environmental considerations makes EU stocks more resilient
Fisheries assessment and management need to consider the range of environmental processes structuring stock dynamics to manage such stocks effectively. Importantly, there is a lack of knowledge on the environmental factors impacting natural mortality (e.g., Annex 7), growth (e.g., Annex 8, anchovy in the Bay of Biscay) or recruitment (Annex 9, North Sea sprat) for a range of EU stocks. Accounting for underlying local or regional environmental conditions and how such conditions impact the biology of different stocks would make EU stocks more resilient. In this respect, an understanding of the relationship between environmental factors, will allow managers to consider the right level of uncertainty when developing management measures, and therefore avoid setting unbalanced catch limits. For tropical tunas, while levels of natural mortality from environmental factors are poorly known, we can expect stocks to crash when impacted by environmental shocks as stocks are being substantially structured by overly high catch limits (Figure 3).
Most stock dynamic determinants (e.g., driving recruitment dynamics) are independent of the stock size, such as for a range of Mediterranean stocks (Annex 2). Those scenarios have predominantly still not been tested in the present study. This calls for a precautionary approach to be used to manage such stocks, including one that accounts for contextual environmental effects on the stocks. In this respect, ICES recently adopted a new ecosystem-based fishing mortality reference point that considers the potential negative impacts of environmental variability on stock production (Feco, described in Bentley et al., 2021; ICES, 2022b). Feco is a dynamic reference point within the FMSY ranges determined by stock assessment models. Using environmental indicators, for which there is evidence that they regulate stock production, Feco can be used to scale fishing mortality down when the ecosystem conditions for the stock are poor, and up when conditions are good.
Lesson 4. Stocks are not isolated but part of an ecosystem that must also be resilient
Despite most stocks examined showing resilience to short term shocks (Annex 10, North Sea ecosystem), there may be other ecosystem impacts associated with changes in species dynamics. For example, shocks on sprat in the Baltic Sea had wide impacts on a range of species due to sprat being central to this ecosystem’s food web, including non-marine species (Annex 11, Baltic Sea ecosystem). Because of cumulative pressures, there may also be tipping points and non-linear responses to increasing shock magnitudes. For example, biomass for Irish Sea whiting increased under simulations of heatwave shocks ranging from 10.9°C to 14.3°C, due to the reduced biomass and predation pressure from cod (Annex 10, the Irish Sea and North Sea ecosystems). However, with heatwave scenarios above this (14.4°C to 15°C), whiting biomass declined as the benefit of reduced predation mortality no longer outweighing the negative impacts of temperature on stock production (Annex 10).
Shocks to lower trophic productivity had the most profound impacts on commercial stock biomass and ecosystem structure and function, highlighting the importance of including changes in productivity in future management considerations. Hence, through trophic interactions, shocks cascade through the food web (predation and competition). Alternatively, compensatory effects may arise within the ecosystems to absorb these cascading effects. For example, we observed benthic prey compensated for a shock on primary production (Figure 4 extracted from Annex 10), leading to a stock recovery in the long run.
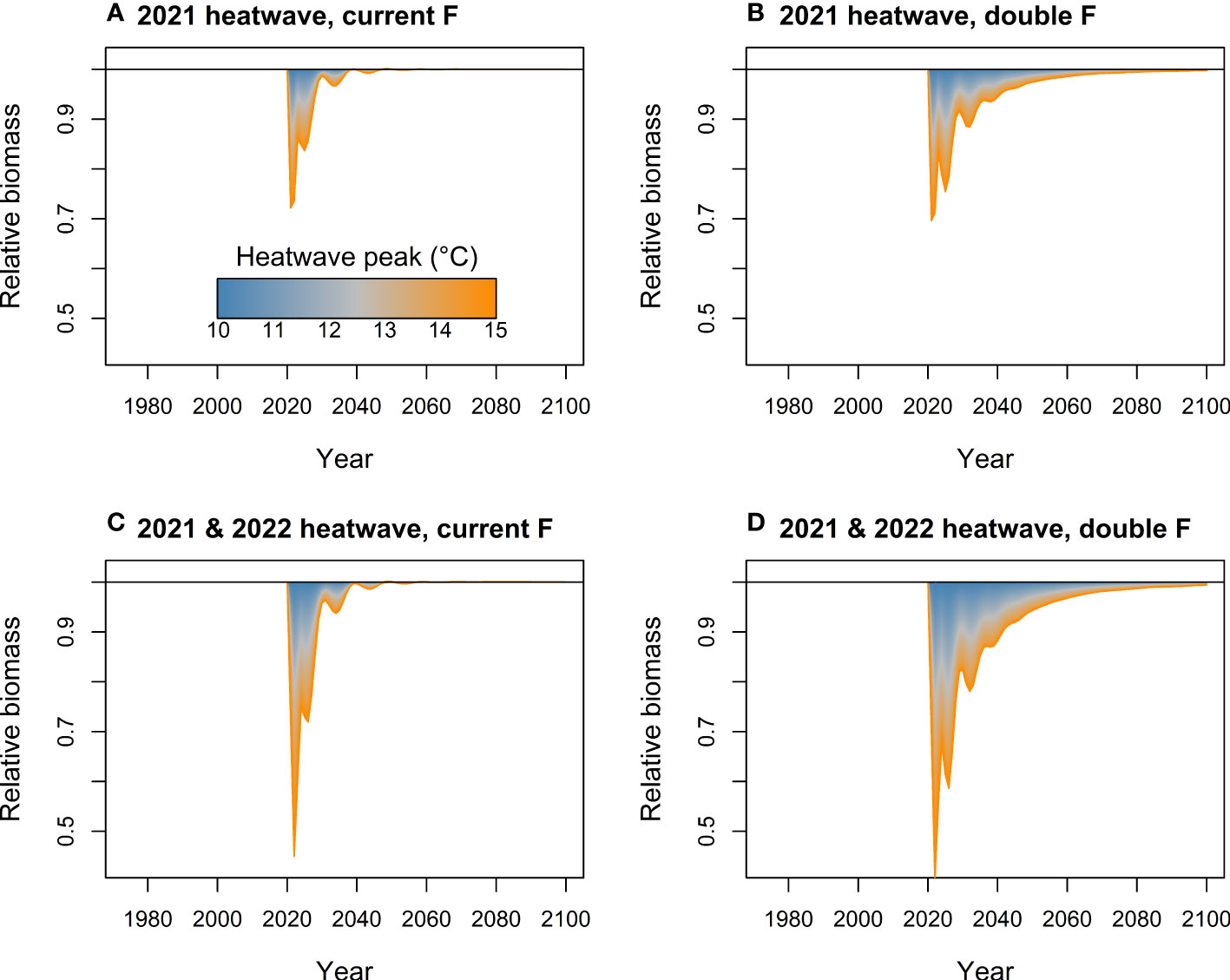
Figure 4 Biomass projections for cod in the Irish Sea ecosystem model in response to shock scenarios, that were (A) a heatwave applied in 2021 with the current fishing mortality, (B) same as A but with twice the fishing mortality applied, (C) two successive heatwaves (one applied in 2021 and the other in 2022) with the current fishing mortality, and (D) same as (C) but with twice the fishing mortality. Fishing mortality was kept constant and doubled for both durations of a heatwave shock. Color scale depicts range of heatwave intensity (see Annex 10 for further details).
Even if biological features are assumed to be static with climate variation, fisheries management must be well-informed due to co-occurring stocks and their varying impact on the broader ecosystem. For example, changes in the biomass of anchovy or sprat in the Black Sea had a substantial impact in the simulations on the structure of top predator populations (Annex 12, Black Sea ecosystem), with increased red mullet populations if anchovy increase in abundance, and reductions in such populations if sprat increase in abundance. Similarly, a shock increasing gelatinous plankton affected the impact of this group on small pelagics with increased predation on early life stages and competition with adults of small pelagic anchovy and sardine, impacting the balance of such species within the Aegean Sea (Annex 2).
Lesson 5. EU fisheries’ economic resilience depends on current profitability
Resource resilience to shock does not equate to the economic resilience of the fishing fleet exploiting those resources, as the effect of climate change on the economic resilience of the fleet is highly dependent on its initial economic situation. Importantly, within fishing fleets with poor or low economic performance, shock effects result in vessels being more vulnerable to future shocks. For example, Dutch beam trawls targeting North Sea flatfish were anticipated to leave the fishery where shocks impact recruitment of the target species of sole and plaice (Annex 13, North Sea flatfish fishery). In addition, for the Dutch beam trawl vessels targeting flatfish, lower profitability is expected from 2022 due to the ban on pulse-trawling, forcing the fleet to switch away from this relatively fuel-efficient fishing method. In the simulations, the transition from pulse to beam trawl reduced the profitability of the fleets and their economic resilience to shocks. If a fleet generates a high profit, a slight increase in costs or decrease in revenue has no impact on short-term economic viability. The reduced fleet could improve profitability by deploying less effort in the long run and therefore be more resilient to future shocks (Annex 13, North Sea flatfish fishery).
Within the Baltic Sea, although following an FMSY strategy increases future fishing opportunities, the effect of climate was to reduce in the simulations these increased opportunities and therefore associated revenues. Therefore, for such fisheries, following a lower F strategy would correct the loss of opportunity induced by the shocks. However, such effects will be fleet-dependent. The redistribution of resources spatially makes such resources accessible to some fleets or gear types and not accessible to others (Annex 14, western Baltic Sea fisheries). For instance, a fleet with polyvalent vessels with more flexibility regarding target species or available fishing opportunities (e.g. easier quota swaps) would allow better adjustments to shocks (Lesson 10 below). For example, during the COVID-19 or the fuel crisis of 2008 many vessels were not able to adjust their tactics to target other species or adapt their fishing patterns to reduce fishing costs. Especially vessels selling fresh catches to restaurants, hotels and catering (i.e., the HORECA sector) suffered the most because of the sanitary lockdowns (EU, 2021a).
Lesson 6. Stock developments are likely to have collateral effects on fuel reduction targets (or other ecosystem components)
The re-constructed estimates of fuel use intensity, fuel efficiency, and catch efficiency for the North Sea beam trawl flatfish fishery showed that fuel use intensity (fuel quantity burned to catch one kilogram of fish) has decreased over the last two decades (Figure 5). However, the fuel efficiency (liter of fuel per unit of effort) has been stable in recent years (Figure 5). We found that such decreases in fuel intensity were predominantly due to better catch efficiency (i.e., kg of fish landed per unit of effort), derived from technological innovations that improved the catch, as well as to technological innovations that reduce fuel used to fish (Figure 5, see also Lesson 8). Moreover, there was a positive relationship between combined targeted stock status indicator and the normalized catch rate (LPUE), indicating that better catch rates may correlate with the better status of the targeted stocks (Figure 6).
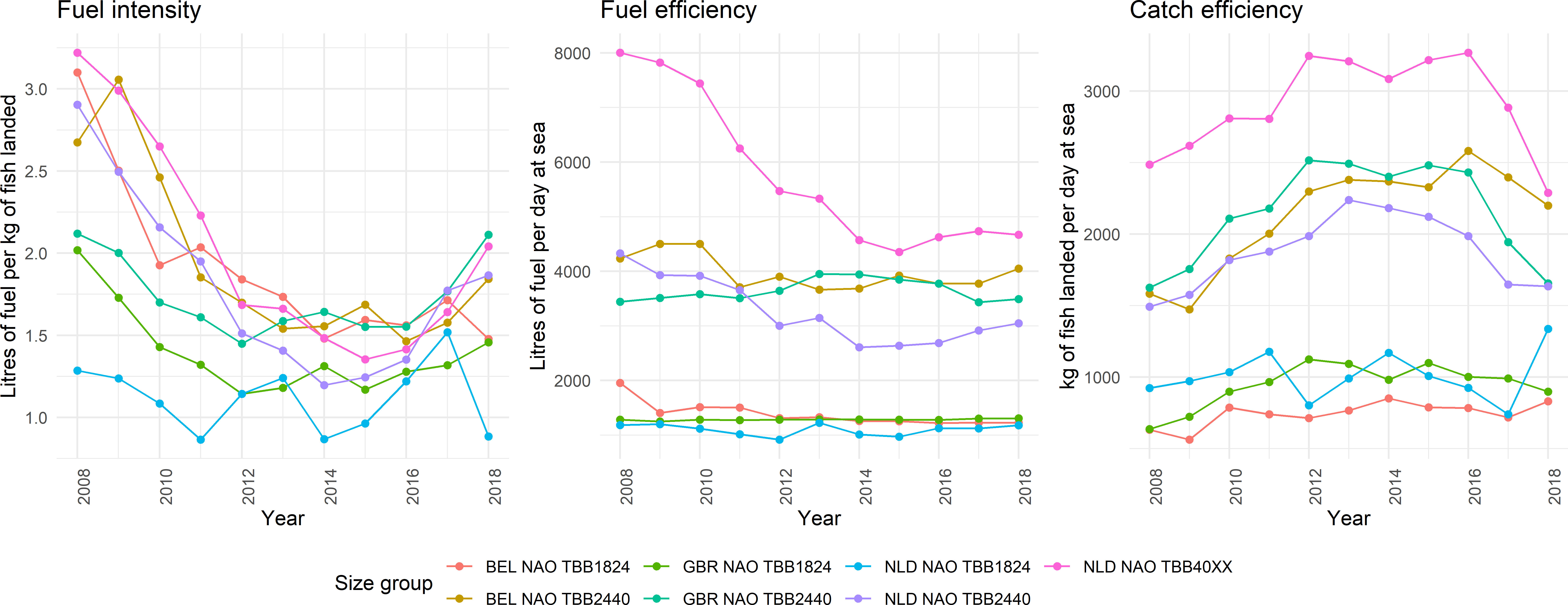
Figure 5 Re-constructed estimates of fuel use intensity, fuel efficiency and catch efficiency for the North Sea beam trawl fisheries.
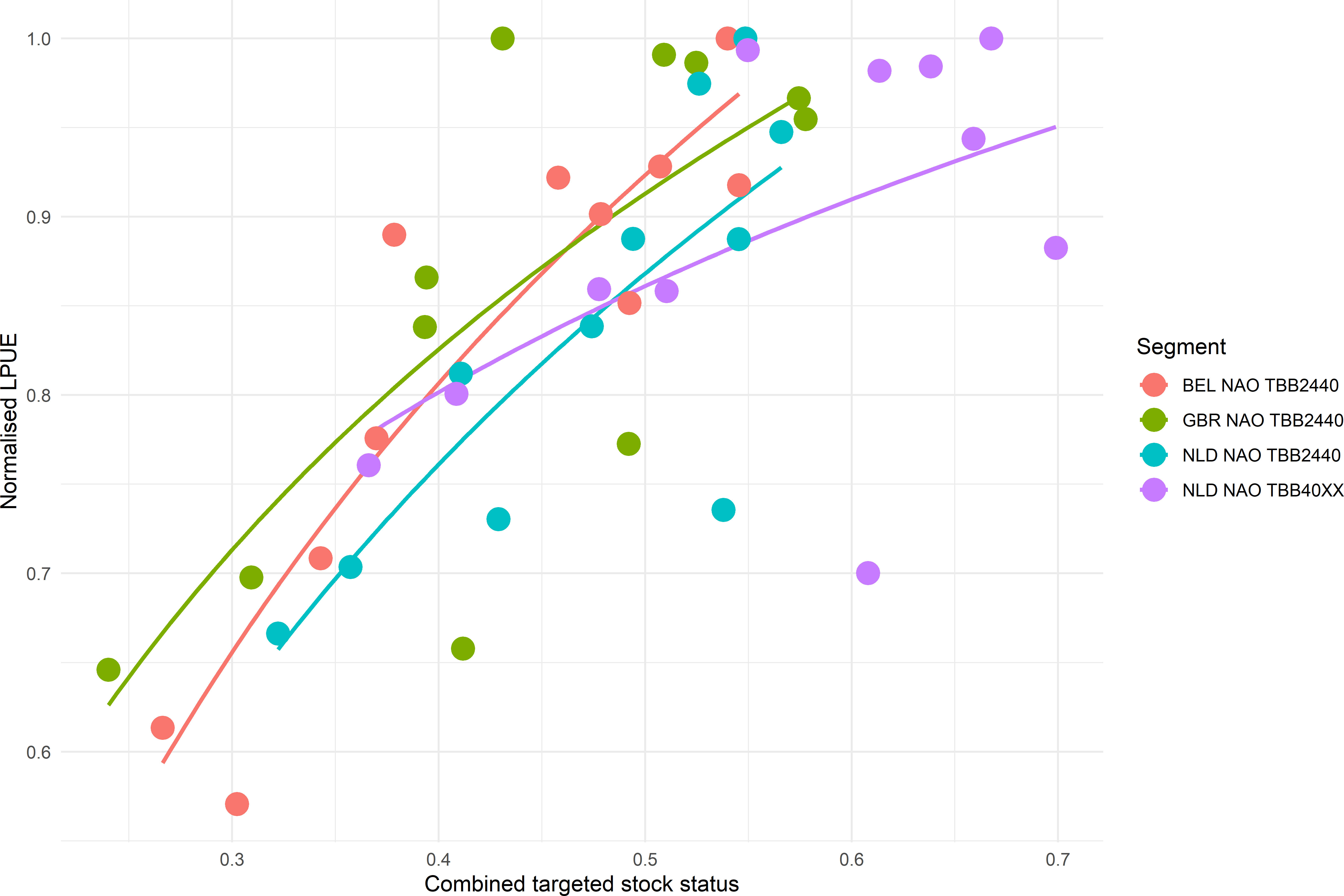
Figure 6 Relationships between the combined targeted stock status indicator and a normalized catch rate (LPUE).
In the absence of obvious technological advances that would decrease the fuel used per unit of effort, several potential factors may explain lowered temporal patterns in fuel use intensity. Lowered fuel use intensity may be associated with better stock status, as less fuel is required to catch one kilogram of fish when stock is well-managed (Bastardie et al., 2022). However, it is also well known that drivers for fuel use depend on the physical characteristics of the vessels and the gear in use, while also fishing techniques (Bastardie et al., 2022) and auditing of the different techniques to collect fuel use data are potentially crucial to understanding the changing levels of fuel use.
Fisheries management within the EU needs to capture and compare fuel use intensity across the fleets at the right level of data resolution. Notably, at present, for other segments than the ones depicted Figure 6, we found that it is not accurate to apply current EU fisheries data collection (EU DCF) and draw conclusions on the fuel use intensity and efficiency of the fleet segments at the fleet level, also given the lousy quality of the fuel use data collected at that level. Finely resolved national fleet-segmented data associated with appropriate bio-economic models will also contribute to assessing how fuel use intensity and efficiency may develop. However, this will need further support and research project-based investigation (STECF, 2017).
Lesson 7. Many economic aspects could come into play in changing fuel use including a change in fuel intensity and fuel-catch efficiency
Further reductions in fuel use intensity within the EU fleets may not automatically decrease fuel use. Firstly, the assumption that fishing vessels will save fuel when towing modified gears (for example, by limiting the hydrodynamic drag required to tow the gear in contact with the seabed, or assuming less fuel-intense gear specifications for midwater pelagic trawls, see Lesson 8) has led to better development of anticipated revenue from fishing (Annex 14, west Baltic Sea fisheries). In addition, more fuel-efficient fishing may have a marked indirect impact on different stocks (e.g., west Baltic cod and plaice, Annex 14) by increasing the part of the available stock abundance subject to fishing and, therefore, impacting the size and species catch composition and subsequent TACs. This change is likely the result of increased spatial effort allocation towards more distant fishing grounds that have become more attractive when fuel is less a limiting factor, which ultimately increases the fuel use.
Within this work, unexpectedly, simulations showed no apparent link between stock development and fuel use, aside from saving on costs (along with savings per unit effort). We can predict that there are likely dominating compensatory/rebound effects that prevent overall savings in fuel consumption from stocks in better shape. This includes a possible indirect effect associated with fishers spending more time fishing (more days at sea and therefore fuel spent at sea) associated with healthier catch levels. In addition, saving fuel costs may also change patterns in fishing, releasing pressure on some stocks but increasing pressure on others when fuel becomes less limiting for the expected profits on some attractive, more distant stocks. For example, in the Dutch flatfish case study (Annex 13, North Sea flatfish fishery), the TACs of target species are underused because costs and limited fishing capacity make it impossible to increase fishing effort. If costs were to decrease, fishers would increase their catch to reach their quota. However, the data resolution we have does not allow us to tag this “rebound effect” to specific parameters in the simulations; counteracting this effect would require fisheries management to follow a precautionary approach. For example, environmental economists (e.g. Freire-González and Puig-Ventosa, 2015) have pointed out that fuel use will decrease only if increased fuel and economic efficiency is coupled with an intervention to keep the fuel costs the same or higher (i.e., a fuel tax), or with an effort reduction.
Lesson 8. A large panel of technologies to reduce fuel use in fisheries already exist
Within the EU fishing fleet, energy-use patterns are highly related to whether the fishery employs passive or active fishing gears; active fisheries that require towing a gear, such as trawlers or Danish seines, tend to consume most of their fuel during the fishing mode. Hence, measures designed to reduce fuel consumption while in fishing operation are the most cost-efficient for such fisheries. There is substantial literature devoted to assessing how best to reduce the drag in trawling fisheries, compared to actions undertaken to improve other measures related to the ‘vessel’ or ‘strategy’ categories. In contrast, for purse seiners and pole and liners targeting migratory pelagic species, most of their fuel consumption is associated with time steaming to the fishing grounds or finding fish. This translates into higher fuel consumption spent during the steaming stage; hence, measures such as route optimization and slow steaming appear the most suitable for this type of fishery.
Despite the extensive list of technological solutions (108 measures were identified), stakeholders’ participation and viewpoints showed that scientific respondents (N=9) tended to respond with solutions that focused on experimental measures. In contrast, commercial stakeholders (N=17) highlighted successful measures. Regarding the measures coming from different métiers, stakeholders from passive fisheries prioritized improvements to the ‘vessel’ category (N=24), such as engine change or hybrid engines, and some strategic measures (N=2), e.g. use of auto-pilot. Stakeholders from pelagic fisheries focused on both the ‘vessel’ (N=8) (e.g. changes in the main engine to hybrid counterparts) and the ‘strategy’ category (N=4) (e.g. route optimization and slow steaming), as did generic measures (N=10) (e.g. behavioral changes of the skipper, more efficient propulsion system, improved maintenance, shift to electric-powered consumers from mechanical-hydraulic consumers, use of LED lights and fuel monitoring devices). Stakeholders from bottom trawl fisheries included measures for all categories. Bottom trawling was the only métier that included ‘gear’ as a category (Nvessel=18, Nstrategy=11, Ngear=31); the most implemented solutions for the gear category are the use of more efficient gear and materials such as the use of dyneema, Sumwing and shift to pulse trawl, visual monitoring of the trawling operation, replacement for lighter gears and components producing less bottom contact (e.g. trawl shoes with wheels, Figure 7).
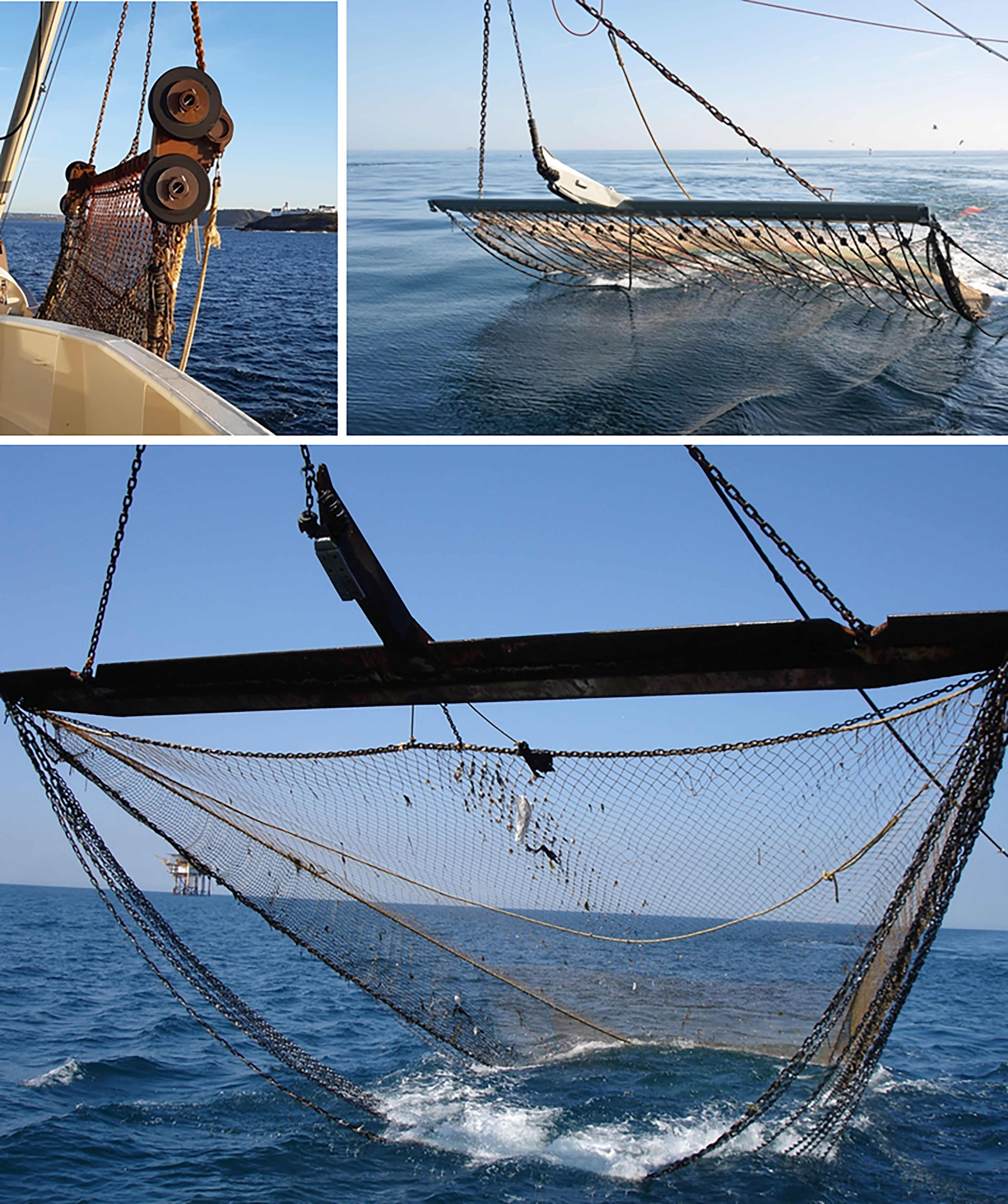
Figure 7 Top left - Rolling wheels assist trawl shoes to reduce drag in chain mat beam trawl fisheries. Top right- A pulse trawl gear, bottom – SumWing with tickler chains (copyright ILVO).
Remarkably, the 2008 fuel crisis stimulated investigations to reduce fuel consumption in beam trawl fisheries. These investigations are an excellent example of fuel savings that arise from gear modifications. Beam trawls with chain mats were equipped with roller wheels to reduce the drag on the seabed, while beam trawls with tickler chains started using the SumWing to reduce seabed contact points (Figure 7). Beam trawlers targeting Common sole additionally switched from mechanical to electric stimulation to further reduce fuel use (Figure 7) until pulse trawls were prohibited in 2021 (Rijnsdorp et al., 2021).
There has been substantial development effort put into reducing fuel use when towing bottom trawls and midwater trawls. Improvements include modifying otter trawls with new netting designs and materials, deployment of twin trawl or multi-rig which consist of two or more juxtaposed smaller trawls rather than one larger (to increase gear spread and catchability without also increasing towing resistance, see Eigaard et al., 2014), and replacement of bottom-contacting trawl doors with innovative, lighter and more hydrodynamic pelagic doors (Figure 8) If more of these modifications can be applied jointly to an otter trawl, substantial energy savings (up to 40% have reported in the stakeholder survey) and/or catch efficiency increases are possible.
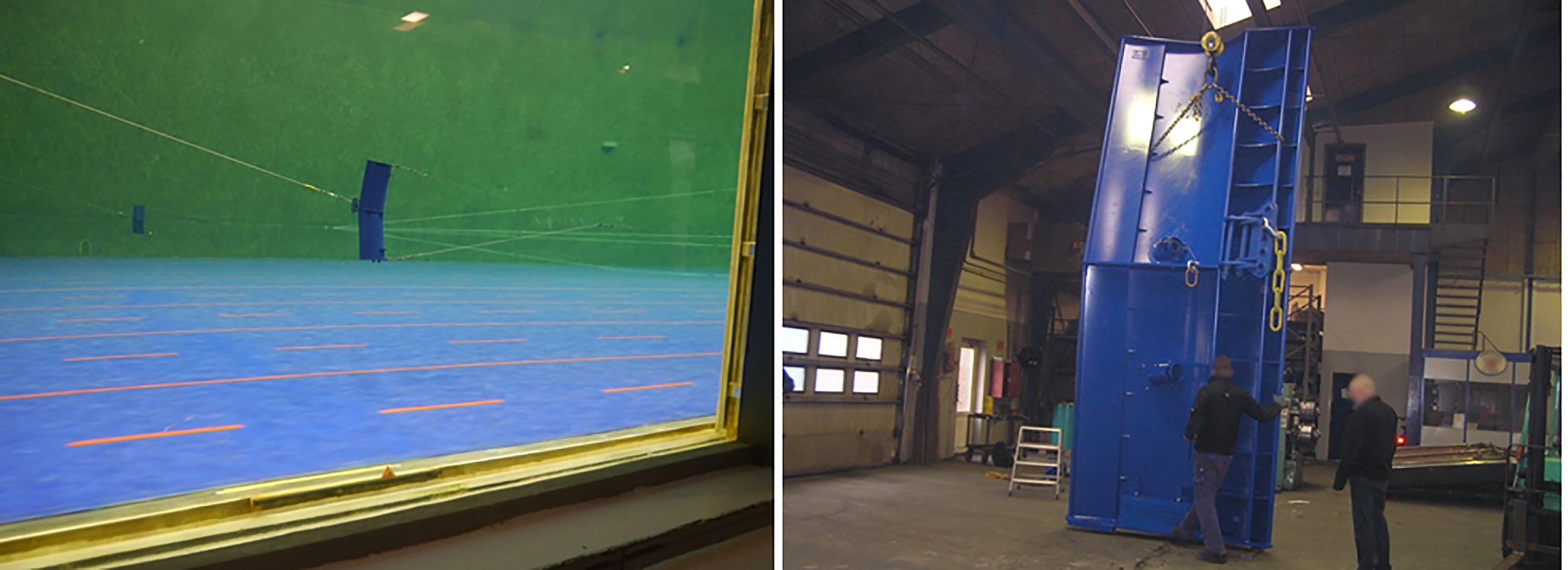
Figure 8 Hydrodynamic testing of down-scaled pelagic trawl doors designed for the Danish sandeel (Ammodytes marinus) fishery with bottom trawls (left) and full-size trawl door produced for the market/fishing fleet by Thyborøn Trawldoor company (right) (copyright Aquamind).
Lesson 9. The actual uptake of technological innovations is still low because of impediments and regulatory barriers
There is a range of energy-efficient technological solutions available, but the implementation of such technologies is low within the EU. We identified likely explanations as structural and economic barriers to innovation uptake, while not all technological solutions are applicable outside the specific fisheries they have been developed for. Often technological innovations utilize species characteristics to improve catch and fuel efficiency of gears (e.g., the schooling behavior of many pelagic species, which has enabled a substantial reduction of hydrodynamic drag by enlarging the meshes in the front of the trawl, see Eigaard et al., 2011) and consequently the innovations cannot be transferred directly to fisheries for other species. The barriers may be related to the lack of collaborative work with and among end-users; limited information on technologies and applicability, which hamper fishers to develop accurate technological knowledge, for which training courses and ocean literacy are needed; the difference in priorities between skippers and vessel owners, where skippers may be less concerned than vessel owners on the need to improve fuel efficiency; investment costs may slow down implementation of new, expensive technologies, mainly in the small-scale fishing that usually has a lower turnover; new technologies can be ineligible for the current scheme of public funding (e.g. fishing capacity capping would limit using technologies such as gas or battery/electric engines that may require a kW increase of the vessels to carry new, heavier and larger engines) or because the capacity for these fisheries is currently misaligned with the fishing opportunities (e.g. STECF, 2021), national regulations may sometimes limit the opportunities for improvements supported by the EU; and finally, fuel tax reductions and exemptions for fishing fleets may limit the incentive for the sector to develop alternative fuel-efficient practices (EP, 2013).
The information exchange between the EU Commission and the International Maritime Organization (IMO) needs to be more fluid, as many regulations set by the IMO affect the fishing sector, and there may be instruments that are being applied in the commercial shipping industry that the fishing industry could benefit from. This effort includes developing instruments that would reduce fuel use also in the fishing sector, such as taxation based on performance in saving fuel, fuel tax, restricting engine power, restricting gear, improving fish stocks, fish quota systems, increased fuel price, eco-certification, reducing effort, improving skippers’ skills, and rewarding skippers for implementing good practice. Funding for the development and uptake of energy-efficient innovations should also be a key instrument in the EU, which developed the European and Maritime Fisheries and Aquaculture Fund (EMFAF 2021-2027). Such funding should be accompanied by market strategies to boost energy efficiency in the fishing sector. If the CFP includes provisions for the EU Member States concerning incentives for energy-efficient vessels, the EMFAF should be one of them providing financial support for onboard investments for energy efficiency.
Any gain in efficiency would need to be backed up with scientific evidence that makes the auditing and monitoring of the fuel use a prerequisite. This reiterates the need for fuel monitoring tools onboard vessels such as those of Basurko et al. (2013) and Sala et al. (2011) to collect adequately resolved and sufficiently representative data, for example with pilot studies, to align research needs with policy ambition, which should also contribute to ocean literacy and promote uptake of innovative solutions. Pilot studies would test with standard scientific protocol the solutions that can reduce fuel use and costs, increase catch rates or reduce bycatch, and therefore decrease fuel intensity.
Lesson 10. The governance of fisheries should support adaptive and flexible management
We identified EU CFP instruments to meet the challenges induced by climate change (Table 2) that would anticipate and respond to the changes (Table 3). The most striking lesson learned is that fisheries management within the EU must be more flexible and adaptive in terms of the quota management system, where there might be more space for swapping quotas between countries (dependent on the availability of quota to swap) or transferring quotas across regions within a country (Hoefnagel et al., 2015), which is permitted since 1983. A synthesis of the landing obligation policy and discard rates has shown that swaps have reduced some of the TAC constraints induced by the risk for a choke species, as a fix for the Member States to avoid losing shares (EU, 2021b).
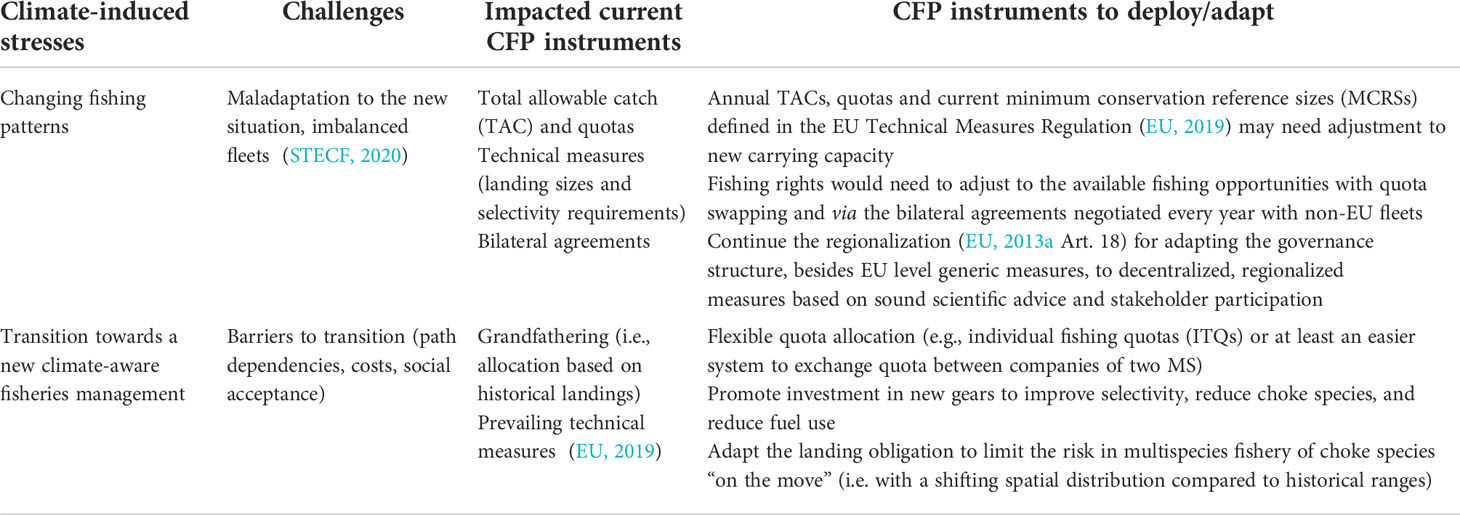
Table 2 Towards renewed or adapted EU CFP (EU, 2013a) instruments identified by the study to fit the new challenges induced by climate change stresses.
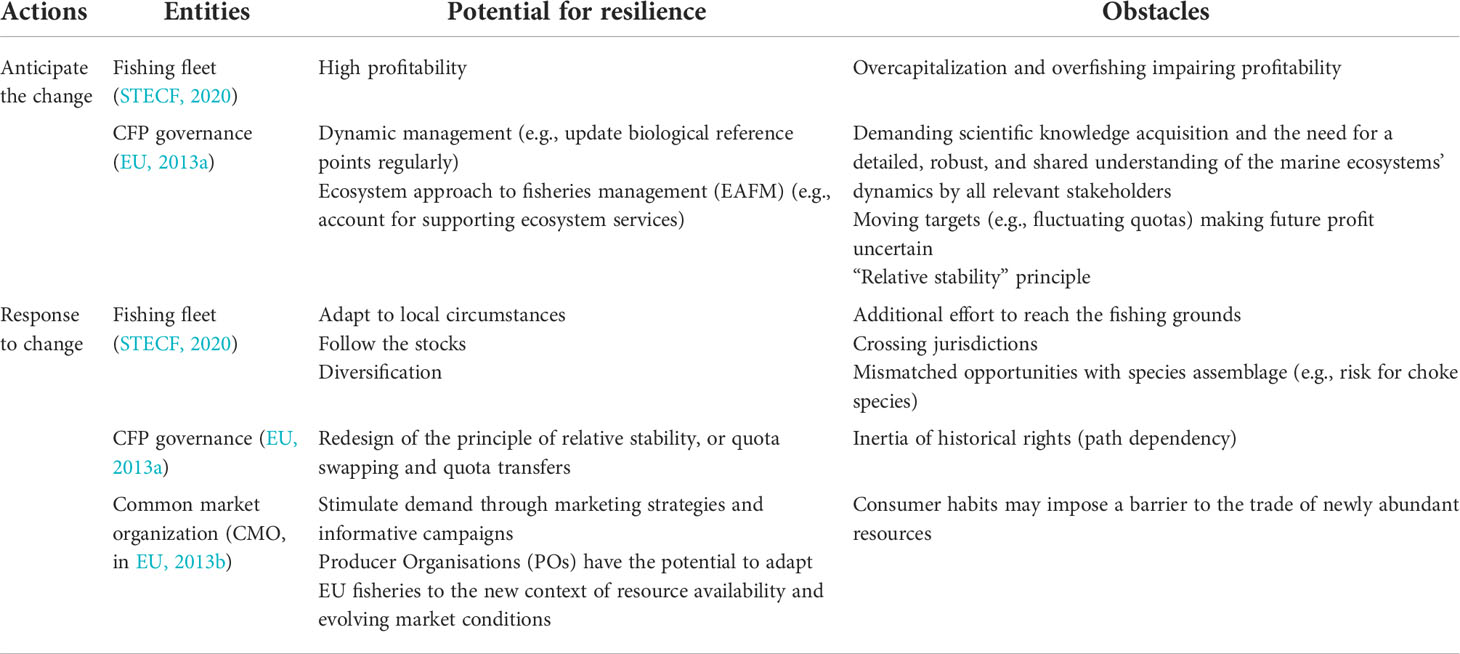
Table 3 Potentials for resilience within the current (EU, 2013a) or a reformed CFP governance.
The possibility for quota swapping or transferring may not be enough to face a change in species spatial distribution and certainly not as a long-term adaptation. Such circumvolution will also be difficult for countries with zero quotas because quotas are usually swapped in exchange for another quota stock, and less frequently for money. Neither discard reduction nor correcting for over-quota catches are easy if there are zero quotas for a significant part of the species. Although this does not pose a problem for some countries, this becomes a complex problem to overcome for some others. Hence, new quota exchange agreements at the scale of the regional advisory councils have been recently deployed for fixing short-term issues with countries with zero quota (EU, 2020). Quotas are fixed by the “principle of relative stability” since 1982 and still in force in the last EU CFP revision. The European Parliament discussed the “relative stability” principle, and some members of the fisheries committee asked for a revision (Fishing daily 18.06.2021, https://thefishingdaily.com/featured-news/pech-committee-told-eu-should-rethink-relative-stability-policy-in-cfp/). However, many EU Member States argue that the relative stability key is the exchange for giving up national resource management in the EEZ for the CFP. It is not possible to simply change the key as exchanges need to be exact equivalents (when all countries need to agree to a change for many stocks, many regional seas, and many fleets). It is, therefore, unlikely that there will be a change in the relative stability key soon.
Discussion
Manage EU fisheries within a climate change future
The goal of managing EU’s living marine resources under the CFP is to ensure that fishing activities are environmentally sustainable in the long term (EU, 2013a). To do so, the impact of fisheries on marine ecosystems needs to be minimized, while also considering how ecosystem dynamics affect fisheries opportunities and profitability. With climate change firmly impacting marine ecosystems, it is now crucial to also manage EU fisheries in a climate change future (Holsman et al., 2019; Howell et al., 2021; Woods et al., 2021; Hidalgo et al., 2022). Facing uncertainties in future climate, we have developed an impact assessment in a scenario-based framework for testing the sensitivity to ecological and economic effects arising from environmental changes induced by climate change under current management measures. In our study, the EU fisheries assessed here were predominantly found to be resilient to the impact of climate-driven short-term stresses, provided management of such fisheries is based on and follows sound scientific advice (Lesson 1). If in the short-term, well-managed stocks (that is, stocks that are not overfished and not suffering from overfishing) are resilient to shocks, building resilience to long-term trends due to climate change will be less challenging. Firstly, it was found that the health of a stock is a prerequisite for stock resilience. Secondly, while changes are unavoidable, adaptive harvest rules, i.e., that respond to available biomass, e.g., by updating target, threshold, and limit reference points as changes in stock productivity are detected, have been shown to provide benefits under both static and changing climates (Gaines et al., 2018). Undesirable stock trajectories show the need for flexible fisheries management, which uses lower F in managing the fisheries to buffer against those risks. Harmful mismatches between reference points and actual productivity levels call for more conservative management targets than currently undertaken. Besides, non-compliance with quotas with higher than intended fishing mortality combined with climate change effects could act to push stocks even further outside levels that safeguard the reproductive capability of the stocks (Lesson 2).
The lack of accurate information on natural phenomena affecting stock productivity on which to base the management of fisheries has substantial consequences on the robustness and the accuracy of the scientific advice for fisheries deployed to support evidence-based management. In this respect, our simulations show that most trajectories returning to initial states might be a consequence of fisheries and ecosystem model designs that, by construction, still assume constant biological features of individual species (e.g. incorporating range changes in biological features, mainly recruitment, but not changing them dynamically, or changing some but not all correlatively). However, regime changes are more likely to happen if biological features are not assumed to be static (as in the present ecosystem simulation studies). The robustness of the reference points and the management system in which they are used also depends on assumptions about the stability of biological processes. However, determining whether biological parameters have changed because of density dependence, the evolutionary effect of fishing, or the environment requires further research. The natural mortality suffered by marine species is a key parameter that cannot be directly observed but instead is derived based on various assumptions and models (Lesson 3). If all biological features remain the same, the risk of the stocks passing a tipping point toward a new regime is unlikely because of compensatory effects. A new regime is unlikely unless thresholds on some oceanographic features might be reached as for the Baltic Sea (on oxygen, salinity determinants of cod spawning, etc.), or the Irish Sea example (sensitivity to affecting the lower trophic level productivity, etc.).
Uncertainties in both science and management call for a precautionary approach. In the absence of accurate knowledge, lower targets for fisheries decrease the impact of (climate-induced) shocks with a buffer. Following the precautionary approach, as adopted in the (EU 2013a, Art. 2.2), and managing fisheries at levels below the theoretical maximum sustainable yield, can result in a minor expected loss of yield and provide resilience to short-term stress and permit the application of an adaptive approach (Earle, 2021). Since impacts are more likely to be detected, allowing an appropriate response to being made, such an approach may then provide a buffer against risks from unexpected environmental changes (Lesson 3). This is even more important since the risk (of overexploiting or under exploiting) facing climate change effects is asymmetric, the risk of losing it all being incommensurately more important than losing a little, which calls for applying a fishing pressure slightly lower than FMSY, therefore not using the MSY as a target but as a limit instead (ICES, 2022a; ICES, 2022b).
Aiming at lower exploitation levels than the FMSY has been suggested several times (e.g. the “pretty good yield”, PGY, see Rindorf et al., 2017 after Hilborn, 2010, or Earle, 2021). PGY is a more resilient fishing strategy by maintaining stocks in a safe space even if the fisheries management face uncertainties and unknowns. Aiming at PGY means fishing at a lower level than the FMSY. For example, in the EU CFP multiannual plans where FMSY ranges are used, F in the lower range is derived from delivering no more than a 5% reduction from maximum sustainable yield obtained by fishing at FMSY in the long term (ICES, 2021). However, anticipating an economic loss from models assuming stable long-term yield from a current, unchanged situation is likely misleading and underestimates the benefit of the precautionary exploitation. An example is given by the North Sea herring, where recovery to MSY Btrigger after the shock is anticipated to be more successful when management is based on FMSY in the lower range, while yields are similar except in the short term. The same effect is anticipated for herring and sprat in the Baltic Sea and the NEA mackerel stock, showing a reduced risk when the management advice is based on the lower bound of the FMSY range, at the cost of lower catches in the short term. Similarly, in the Med, most stocks are managed with a target F of F0.1, which is intended to be a lower estimate of FMSY, while in tuna stocks the F target is commonly set to be less than FMSY as the objective is to keep biomass> BMSY and F<FMSY. If fishing at levels corresponding to the PGY comes with a loss of potential yield in the short term by fishing less than FMSY, such a target provides a buffer against the risk of an unexpected reduction in productivity from external factors to the fishing which justifies recommending target F < FMSY.
Species interactions in the food web also alter MSY (Lesson 4), as some stocks may collapse whenever passing a tipping point in the ecosystem, while “pretty good yield” can also be expanded to a pretty good multispecies yield (PGMY) to accommodate situations where the yield from a stock affects the ecosystem, economic and social benefits, or sustainability (Rindorf et al., 2017). Therefore, the role of species interactions in the ecosystem is an essential predictor of resilience, though we observed that marine populations always return to an initial state in the 10-30y horizon we investigated and not to a new equilibrium if no significant changes affect the biological features of individual species in the food webs. The risk created by the ecosystem to pass some tipping points with abrupt shifts under pressures and cumulative impacts would need further consideration on a longer time horizon and inflate the level of uncertainty in future pathways. In the short term, aligned with the time scale that matters to the fishing, bioeconomic models showed that using flexible management with lower than Maximum Sustainable Yield targets improves stock resilience at the cost of reducing catch in the short term (Lesson 5).
Following a precautionary strategy is more problematic in a mixed fisheries context. In mixed fisheries such as the North Sea fisheries for demersal species, as long as compliance to the EU landing obligation (LO) is met, the simulations anticipate that all stocks will recover from an initial shock by 2030. However, the FMSY in the lower range strategy increases the risk for North Sea cod to choke the fleets by being the limiting quotas, leading to missing fishing opportunities in the short term but likely compensated with gain in the longer term. Choke species can lead to a dramatic decrease in effort and, therefore, favorable stock developments with higher SSB for others (now underexploited) stocks. Such indirect protection assumes the full compliance of the North Sea mixed fisheries with the LO, which has been fully implemented since 2019 in Europe, requiring the fishing to stop as soon as the limiting quota is exhausted. Because of this possible adverse impact on the fleets, the risk of non-compliance to the LO is high (STECF, 2022b) and therefore the direct and indirect gains expected from respecting it are still unlikely in the EU. We then learnt that high resource resilience would not necessarily ensure the resilience of the fleets as long as some fishing capacity must be removed, at least in the short term, when more limiting management is implemented (Lesson 5).
We also learnt that resource resilience would not necessarily come with the use of less fuel, as other economic factors and effects come into play, such as the ones induced by short term economic losses experienced by different fleets (Lesson 6). Fuel consumption in the fishing sector may be affected by the harvested stock development, and healthy fish stocks would reduce the risk of increasing fuel use and affecting fishery economics or other ecosystem components such as the seafloor integrity. Fuel use intensity and efficiency may also change along with investing and implementing new fuel-efficient technologies in operating the fishing, but possibly not reducing the absolute fuel consumption because of the “rebound effect” (Lesson 7). The collection of high-resolution vessel data may show that fuel use depends on fishing techniques. In parallel, energy-efficient technologies already exist (Lesson 8). Implementing them would require improving the uptake of innovations, including demonstrating to stakeholders the potential for increased catch rates and reduced fuel costs. The transition towards reducing fuel consumption would need to be supported by the setup of EU regulatory instruments for promoting the possible synergy in facing climate change, reducing fossil fuel use, and developing innovations and ocean literacy (Lesson 9).
Therefore, the way forward is to ensure governance that will support the continuation of dynamic, adaptive management to cope with the changing conditions induced by climate change in EU Waters (Lesson 10). As a co-benefit, we expect favorable stock development from resilient stocks to climate effects to reduce fuel use as long as there is a relationship between higher catch rates and high stock biomasses.
Ensure the fishing sector meets the sustainability and fuel reduction targets
Lower catch rates and then more effort induced by the climate change effects and shocks would increase fuel use, as shown, for example, in the case of the demersal fisheries in the North Sea or the Dutch flatfish fisheries. On the contrary, there is, therefore, a win-win-win situation as a way forward for fisheries which would consist of ensuring i) the resilience of the stocks and their habitats (with a precautionary approach ensuring fishing at a lower level than the FMSY) and ii) reducing the fuel use, while iii) saving on costs that would increase the economic resilience of the EU fleet. Such management is at the core of the current suite of multiannual management plans (MAPs), especially implementing a continuous effort reduction in the western Mediterranean (EU, 2019), and in the Adriatic Sea (GFCM, 2019). However, it is still currently hard to check this virtuous circle (i.e., Figure 9) against the real world, other than by testing with bio-economic modelling. Fuel measurement data are not sufficiently available, as we learned in this study, and fuel consumption data are estimates or reconstructions based on fishing effort data and not direct measurements collected onboard. There is a further need to understand the amount of energy being used now (the fuel and lubricants used during the fishing operations by the main engine, the auxiliary engines, or in the upstream or downstream businesses onshore, etc.). Also, because we found that implementing existing solutions to reduce energy use in fisheries has been very limited, the signal toward improvement in this virtuous cycle will not be captured in any existing specific datasets. Hence, the main challenge faced in this work was the analysis and development of the narrative surrounding EU Member States’ fuel use, which is collected along with a wide range of fisheries-related data (the EU DCF), but again none of these data results from direct measurement of fuel use. We found that it is not accurate to apply the DCR/DCF data and draw conclusions on the fuel efficiency of the fleet segments (but see Guillen et al., 2016). Overall, knowledge is key, and monitoring programs and pilot studies should be promoted to support the policymakers with scientific shreds of evidence and align research needs with policy ambition. There is, therefore, an identified need for supporting and extending research project-based investigation with bioeconomic models to anticipate the effects of management measures on reducing fuel use in fisheries, especially identifying reduction potentials when switching fishing techniques, and risk for compensatory/rebound effects that may prevent saving fuel from stocks development towards recovery.
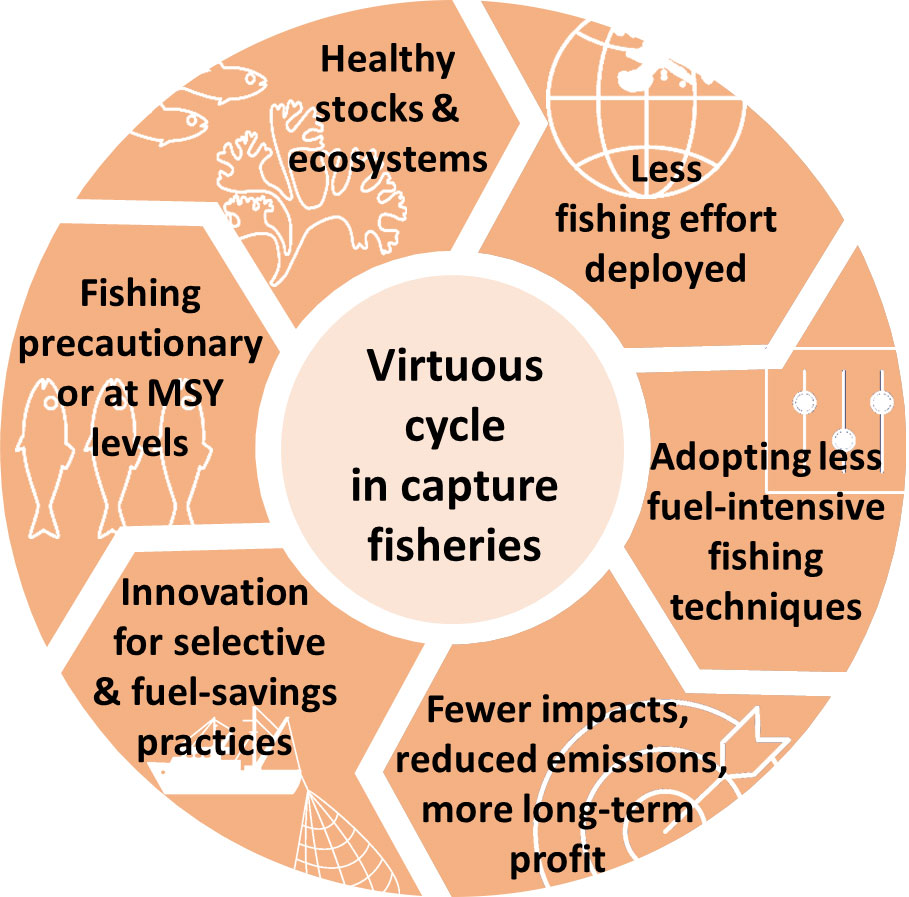
Figure 9 The figure shows (extracted from Bastardie et al., 2022) an idealized virtuous circle showing incremental steps that would provide incentives to fishing vessels deploying selective fishing gears or using fishing techniques with reduced environmental impact toward maintaining healthy and productive marine ecosystems and therefore supporting the fisheries and their future yields.
Propagating the change induced by reductions in fishing impacts to other supportive ecosystem components, the fisheries management (EU CFP) and environmental policies (EU Marine Strategy Framework Directivein EU (2017), and other EU directives, see Boyes and Elliott, 2014) could contribute to securing future fishing opportunities for the fishing fleets in the EU, along with fulfilling the market demand and ensuring coherence in meeting European and national targets. Collection of harmonized, reliable, and accurate data sets is needed along with scientific development to favor this propagation and engage the virtuous cycle (Figure 9), furthering the need for an Ecosystem Approach to Fisheries Management beyond considering standard fisheries science only, including developing of research efforts to:
i. Better describe the link between the physical drivers and the ecological response. For example, recruitment of some fish species is mainly determined by environmental factors and trophodynamics, and only to a less extent by the adult fish or potential spawning stock size (e.g. well-illustrated by the east Baltic cod in Plikshs, 2015, or Svedäng et al., 2022). Therefore, there is a need to enhance stock assessment models to consider climate factors that affect the productivity of the stocks (e.g., including stock abundance, distribution, timing, physiology, recruitment success, reproductive rate, growth, feeding and spawning migrations), as well as biological reference points, management limits, and targets; and
ii. Better describe and capture the link between the ecological response and fisheries’ effects and response to management. A typical example is to study the effect of area-based management. Indeed, the benefits from closed areas may come from holding individuals that have (on average) a larger average body size, which result in higher overall population biomass within the closed area (as large fishes are not impacted by fishing pressure). Such enhanced biomass may then result in adult migration (i.e., spillover) and/or increased number of larvae dispersing from the closed area (but see Caveen et al., 2015). Such dispersal may then replenish adjacent fishing grounds, as long as fishing effort displacement does not cancel out these effects Bastardie et al., 2020. Therefore, there is a need to better describe the different possible fishers´ response to changes in the demographic structure of fish populations, including the decisions, tactics or strategies to cope with environmental stressors (such as the climate change) on stock access. Given different degrees of vulnerability and adaptability, the impact on fishers is likely to depend on the type of fishing employed (e.g., small-scale fishing vs industrial fishing having different profitability levels, different mobility range etc.) and therefore vulnerability to variance in stock structure and change in spatial distribution (as discussed by Adger et al., 2005, Marshall and Marshall, 2007, Holland et al., 2017);
iii. Better describe the possible management actions and viable socio-ecological pathways that could mitigate the effect or adapt to it under the constraints of the social and ecological system. For example, regulatory barriers constraining the flexibility of the fisheries to adapt to novel conditions may be removed, as long as they will not impair future opportunities, and incentives for redirecting the fisheries toward viable pathways may be deployed (e.g. Hamon et al., 2021; Woods et al., 2022). Describing this link also requires understanding the adaptive responses available to fishers, that is, whether there is the capacity to change operations or whether social, financial or regulatory barriers exist, and under what conditions they would implement this adaptive response (i.e. the level of change before an adaptive new path is taken in response to the change).
Conclusion
Along with this study, we advocate for climate-aware fisheries management and a precautionary approach, which will by nature reinforce the resilience of the fishing sector to face a changing climate and its effects. At the same time, the fisheries sector is challenged by higher fuel prices lowering their profitability and the necessity to reduce the greenhouse gas emissions to meet environmental targets. Also in parallel, the growing need for food security may push soon toward finding ways for boosting seafood production. At the time of writing, the EU has developed a package of policies that would possibly reconcile environmental, economic and social sustainability by heading toward reducing the fuel used in the fishing sector to meet the environmental targets while ensuring a consolidated CFP. Regulations that impair the opportunities for improvements supported by the EU would be amended with the “Fit for 55” package initiative from the European Commission, comprising a proposal for energy taxation (Energy Taxation Directive in EU, 2021c) where the Member States may no longer exempt fishing vessels for fuel tax to accelerate a transition toward low-carbon fishing techniques and practices. Proposals for alternative fuels infrastructure will also mandate investments within a transitional period in ports for shore-side electricity and alternative fuels, including sustainable biofuels and biogas, and renewable fuels of non-biological origin (EU, 2021d). The European Green Deal (COM(2019) 640 final) sets out an objective of resource-efficiency, reaching zero emissions by 2050 and protecting, conserving and enhancing the EU’s natural capital, with an intermediate target of 50 to 55% reduction of emissions by 2030 (compared to 1990 levels; a reduction by 23% had already been achieved by 2018). The EU Commission also committed to strengthening climate-proofing, resilience-building, prevention, and preparedness, which should be the basis for a revised or reformed policy to come in 2023.
There might be no immediate need to reform the current CFP to increase the resilience of stocks exploited by the EU fleets, whenever the current management measures are applied and complied with, along with promoting a climate-aware fisheries science, and tailored to local circumstances. Improving fuel efficiency is different. The environmental climate targets and the worldwide fuel price increase after the geopolitical events of start 2022 show the necessity to reduce the dependency on fossil fuels in the fishing sector in the short term. We identified barriers that impair the complete uptake of science and innovative solutions into the advice chain in fisheries management. Hence knowledge of the socio-bio-economic dynamics should be acquired, new trends and innovative solutions explored, and translated from science to policy, which would also require supporting mutual trust among stakeholders (Cvitanovic et al., 2021). Documenting effects as we do should promote the further development of knowledge-based decision-making and confidence for managing living marine resources in EU waters and their exploitation with minimal impact. If the changing climate impacting the seas was not on the political agenda of fisheries policy in Europe so far, this risk has now to be considered for mitigating the uncertainty it implies on the future fishing opportunities, the provision of fish to the European markets, and the interlinked ambition to reach environmental targets including phasing out fossil fuel use. The purpose of this study was then to provide the policymakers with a technical background for the CFP assessment regarding issues related to climate change. Again, the issues are twofold: whether the current CFP caters for the implications on fisheries management from the climate change (adaptation and resilience) and how the CFP can contribute to climate change mitigation by reducing emissions of greenhouse gases from the European fishing fleet. Reaching these objectives includes the provision of climate-resilient socio-ecological pathways for further decarbonization of the EU’s energy system and the continuation of seafood provisioning to the European markets with minimal environmental impact and in a changing climate.
Data availability statement
The raw data supporting the conclusions of this article will be made available by the authors, without undue reservation.
Author contributions
Main authors FB, DF, TB, LK, SM, RD, OE, and OB have led and conducted the study and have written the first draft, and along with all other authors have conducted the simulation studies, investigations, reviewed and agreed on the final text, and provided materials to support the study with the Supplementary Materials. All authors contributed to the article and approved the submitted version.
Funding
The preparation of this paper has been supported by the European Union. The information and views set out in this article were based on scientific data and information collected under the Specific Contracts EASME/EMFF/2020/3.2.6/Lot1/S07 and EASME/EMFF/2020/3.2.6/Lot2/SC08, signed with the CINEA (The European Climate, Infrastructure and Environment Executive Agency) and funded by the European Union. The information and views set out in this publication were those of the authors and do not necessarily reflect the official opinion of CINEA or of the European Commission. Neither CINEA, nor the European Commission can guarantee the accuracy of the scientific data/information collected under the above Specific Contract or the data/information included in this publication. Neither CINEA nor the European Commission or any person acting on their behalf may be held responsible for the use which may be made of the information contained therein.
Conflict of interest
DF was employed by the company MRAG Ltd., SM is the CEO of Sakana Consultants, and GT belongs to Blue Bio Consulting.
The remaining authors declare that the research was conducted in the absence of any commercial or financial relationships that could be construed as a potential conflict of interest.
Publisher’s note
All claims expressed in this article are solely those of the authors and do not necessarily represent those of their affiliated organizations, or those of the publisher, the editors and the reviewers. Any product that may be evaluated in this article, or claim that may be made by its manufacturer, is not guaranteed or endorsed by the publisher.
Supplementary material
The Supplementary Material for this article can be found online at: https://www.frontiersin.org/articles/10.3389/fmars.2022.947150/full#supplementary-material
References
Adger W. N., Hughes T. P., Folke C., Carpenter S. R., Rockström J. (2005). Social-ecological resilience tocoastal disasters Science 309, 1036–1039. doi: 10.1126/science.1112122
Bahri T., Vasconcellos M., Welch D. J., Johnson J., Perry R. I., Ma X., Sharma R. (2021). “Adaptive management of fisheries in response to climate change,” in FAO fisheries and aquaculture technical paper (Rome: FAO).
Bartelings H., Hamon K. G., Berkenhagen J., Buisman F. C. (2015). "Bio-economic modelling for marine spatial planning application in north Sea shrimp and flatfish fisheries.". Environ. Model. Softw. 74, 156–172. doi: 10.1016/j.envsoft.2015.09.013
Bartolino V., Colloca F., Sartor P., Ardizzone G. (2008). Modelling recruitment dynamics of hake, merluccius merluccius, in the central Mediterranean in relation to key environmental variables. Fish. Res. 92, 277–288. doi: 10.1016/j.fishres.2008.01.007
Bastardie F., Brown E. J., Andonegi E., Arthur R., Beukhof E., Depestele J., et al. (2021). A review characterizing 25 ecosystem challenges to be addressed by an ecosystem approach to fisheries management in Europe. Front. Mar. Sci. 7. doi: 10.3389/fmars.2020.629186
Bastardie F., Danto J., Rufener M.-C., van Denderen P. D., Eigaard O. R., Dinesen G. E., et al. (2020). Reducing fisheries impacts on the seafloor: a bio-economic evaluation of policy strategies for improving sustainability in the Baltic Sea. Fish. Res. 230, 105681. doi: 10.1016/j.fishres.2020.105681
Bastardie F., Hornborg S., Ziegler F., Gislason H., Eigaard O. R. (2022). Reducing the fuel use intensity of fisheries: through efficient fishing techniques and recovered fish stocks. Front. Mar. Sci 9, 817335. doi: 10.3389/fmars.2022.817335
Bastardie F., Nielsen J. R., Miethe T. (2014). DISPLACE: a dynamic, individual-based model for spatial fishing planning and effort displacement - integrating underlying fish population models. Can. J. Fish Aquat. Sci. 71 (3), 366–386. doi: 10.1139/cjfas-2013-0126
Basurko O. C., Gabiña G., Uriondo Z. (2013). Energy performance of fishing vessels and potential savings. J. Cleaner Production 54, 30–40. doi: 10.1016/j.jclepro.2013.05.024
Baudron A. R., Brunel T., Blanchet M.-A., Hidalgo M., Chust G., Brown E. J., et al. (2020). "Changing fish distributions challenge the effective management of European fisheries.". Ecography 43, 494–505. doi: 10.1111/ecog.04864
Beare D., Burns F., Greig A., Jones E., Peach K., Kienzle M., et al. (2004). Long-term increases in prevalence of north Sea fishes having southern biogeographic affinities. Mar. Ecol. Prog. Ser. 284, 269–278. doi: 10.3354/meps284269
Beaugrand G. (2004). The north Sea regime shift: evidence, causes, mechanisms and consequences. Prog. Oceanogr. 60, 245–262. doi: 10.1016/j.pocean.2004.02.018
Bentley J. W., Lundy M. G., Howell D., Beggs S. E., Bundy A., De Castro F., et al. (2021). Refining fisheries advice with stock-specific ecosystem information. Front. Mar. Sci. 8, 346. doi: 10.3389/fmars.2021.602072
Bjørnstad O. N., Grenfell B. T. (2001). Noisy clockwork: time series analysis of population fluctuations in animals. Science 293 (5530), 638–643. doi: 10.1126/science.1062226
Boyes S. J., Elliott M. (2014). Marine legislation – the ultimate ‘horrendogram’: International law, European directives & national implementation. Mar. pollut. Bull. 86, 39–47. doi: 10.1016/j.marpolbul.2014.06.055
Brosset P., Fromentin J. M., Van Beveren E., Lloret J., Marques V., Basilone G., et al. (2017). Spatio-temporal patterns and environmental controls of small pelagic fish body condition from contrasted Mediterranean areas. Prog. Oceanogr. 151, 149–162. doi: 10.1016/j.pocean.2016.12.002
Brunel T., Dickey-Collas M. (2010). "Effects of temperature and population density on von bertalanffy growth parameters in Atlantic herring: A macro-ecological analysis.". Mar. Ecol. Prog. Ser. 405, 15–28. doi: 10.3354/meps08491
Byrne C., Agnarsson S., Davidsdottir B. (2021). Fuel intensity in icelandic fisheries and opportunities to reduce emissions. Mar. Policy 127, 104448. doi: 10.1016/j.marpol.2021.104448
Caveen A., Polunin N., Gray T., Stead S. M. (2015). “Critique of the scientific evidence for fisheries benefits of MRs,” in The controversy over marine protected areas (Cham: Springer), 51–80). Available at: http://www.int-res.com/abstracts/meps/v405/p15-28.
Christensen V., Pauly D. (1992). ECOPATH II: A software for balancing steady-state ecosystem models and calculating network characteristics. Ecol. Model. 61, 169–185. doi: 10.1016/0304-3800(92)90016-8
Ciannelli L., Fisher J., Skern-Mauritzen M., Hunsicker M., Hidalgo M., Frank K., et al. (2013). Theory, consequences and evidence of eroding population spatial structure in harvested marine fishes: a review. Mar. Ecol. Prog. Ser. 480, 227–243. doi: 10.3354/meps10067
Clausen L. W., Rindorf A., van Deurs M., Dickey-Collas M., Hintzen N. T. (2018). Shifts in north Sea forage fish productivity and potential fisheries yield. J. Appl. Ecol. 55 (3), 1092–1101. doi: 10.1111/1365-2664.13038
Cvitanovic C., Shellock R. J., Mackay M., van Putten E. I., Karcher D. B., Dickey-Collas M., et al. (2021). Strategies for building and managing 'trust' to enable knowledge exchange at the interface of environmental science and policy. Environ. Sci. Policy 123, 179–189. doi: 10.1016/j.envsci.2021.05.020
Daskalov G. M., Boicenco L., Grishin A. N., Lazar L., Mihneva V., Shlyakhov V. A., et al. (2017). Architecture of collapse: regime shift and recovery in an hierarchically structured marine ecosystem. Glob Change Biol. 23 (4), 1486–1498. doi: 10.1111/gcb.13508
Drinkwater K. F. (2005). The response of Atlantic cod (Gadus morhua) to future climate change. ICES J. Mar. Sci. 62, 1327–1337. doi: 10.1016/j.icesjms.2005.05.015
Druon J.-N., Fiorentino F., Murenu M., Knittweis L., Colloca F., Osio C., et al. (2015). Modelling of European hake nurseries in the Mediterranean Sea: An ecological niche approach. Prog. Oceanogr. 130, 188–204. doi: 10.1016/j.pocean.2014.11.005
Earle M. (2021). Maximum sustainable yield in the EU’s common fisheries policy - a political history. ICES J. Mar. Sci. 78, 2173–2181. doi: 10.1093/icesjms/fsab037
Eigaard O. R., Marchal P., Gislason H., Rijnsdorp A. D. (2014). Technological development in fisheries management. Rev. Fish Sci. 22 (2), 156–174. doi: 10.1080/23308249.2014.899557
Eigaard O. R., Rihan D., Graham N., Sala A., Zachariassen K. (2011). Improving fishing effort descriptors: Modelling engine power and gear-size relations of five European trawl fleets. Fish Res. 110, 39–46. doi: 10.1016/j.fishres.2011.03.010
EP (2013). Fuel subsidies in the EU fisheries sector. EU parliament, policy departement b, structural and cohesion policies, Note authored by Borerello A, Motova, A., and Carvalho, N. doi: 10.2861/33430
Erauskin-Extramiana M., Alvarez P., Arrizabalaga H., Ibaibarriaga L., Uriarte A., Cotano U, et al, et al. (2019). Historical trends and future distribution of anchovy spawning in the bay of Biscay. Deep Sea Res. Part II 159, 169–182. doi: 10.1016/j.dsr2.2018.07.007
EU (2013a). Regulation (EU) no 1380/2013 of the European parliament and of the council of 11 December 2013 on the common fisheries policy, amending council regulations (EC) no 1954/2003 and (EC) no 1224/2009 and repealing council regulations (EC) no 2371/2002 and (EC) no 639/2004 and council decision 2004/585/EC.
EU (2013b). Regulation (EU) no 1379/2013 of the European parliament and of the council of 11 December 2013 on the common organisation of the markets in fishery and aquaculture products, amending council regulations (EC) no 1184/2006 and (EC) no 1224/2009 and repealing council regulation (EC) no 104/2000.
EU (2017). Commission decision (EU) 2017/848 of 17 may 2017 laying down criteria and methodological standards on good environmental status of marine waters and specifications and standardised methods for monitoring and assessment, and repealing decision 2010/477/EU (Text with EEA relevance).
EU (2019). Regulation (EU) 2019/1022 of the European parliament and of the council of 20 June 2019 establishing a multiannual plan for the fisheries exploiting demersal stocks in the western Mediterranean Sea and amending regulation (EU) no 508/2014.
EU (2020). Council regulation (EU) 2020/123 of 27 January 2020 fixing for 2020 the fishing opportunities for certain fish stocks and groups of fish stocks, applicable in union waters and, for union fishing vessels, in certain non-union waters.
EU (2021a). Study on the main effects of the COVID-19 pandemic on the EU fishing and aquaculture sectors. final report. CINEA. doi: 10.2826/634795
EU (2021b). Synthesis of the landing obligation measures and discard rates. final report. CINEA. doi: 10.2826/176808
EU (2021c). COM(2021)563 proposal for a COUNCIL DIRECTIVE restructuring the union framework for the taxation of energy products and electricity (recast).
EU (2021d). Proposal for a regulation of the European parliament and of the council on the deployment of alternative fuels infrastructure, and repealing directive 2014/94/EU of the European parliament and of the council. COM(2021) 559 final.
FAO (2020). “The state of Mediterranean and Black Sea fisheries 2020,” in General fisheries commission for the Mediterranean (Rome:Food and Agriculture Organization).
Fiorella K. J., Bageant E. R., Schwartz N. B., Thilsted S. H., Barrett C. B. (2021). Fishers’ response to temperature change reveals the importance of integrating human behavior in climate change analysis. Sci. Adv. 7 (18), eabc7425. doi: 10.1126/sciadv.abc7425
Free C. M., Thorson J. T., Pinsky M. L., Oken K. L., Wiedenmann J., Jensen O. P. (2019). Impacts of historical warming on marine fisheries production. Science 363, 979–983. doi: 10.1126/science.aau1758
Freire-González J., Puig-Ventosa I. (2015). "Energy efficiency policies and the jevons paradox". Int. J. Energy Economics Policy. 5 (1), 69–79.
Fulton E. A., Link J. S., Kaplan I. C., Savina-Rolland M., Johnson P., Ainsworth C., et al. (2011). Lessons in modelling and management of marine ecosystems: the Atlantis experience. Fish Fish. 12 (2), 171–188. doi: 10.1111/j.1467-2979.2011.00412.x
Gaines S. D., Costello C., Owashi B., Mangin T., Bone J., Molinos J. G., et al. (2018). Improved fisheries management could offset many negative effects of climate change. Sci. Adv. 4 (8), eaao1378. doi: 10.1126/sciadv.aao1378
Garcia D., Sánchez S., Prellezo R., Urtizberea A., Andrés M. (2017). FLBEIA: A simulation model to conduct bio-economic evaluation of fisheries management strategies. SoftwareX, Vol. 6. 141–147.
GFCM (2019). Recommendation GFCM/43/2019/5 on a multiannual management plan for sustainable demersal fisheries in the Adriatic Sea (geographical subareas 17 and 18).
Gucu A. C., Genç Y., Dağtekin M., Sakınan S., Ak O., Ok M, et al. (2017). On black Sea anchovy and its fishery. Rev. Fish. Sci. Aquacult. 25, 230–244. doi: 10.1080/23308249.2016.1276152
Guillen J., Cheilari A., Damalas D., Barbas T. (2016). Oil for fish: An energy return on investment analysis of selected European union fishing fleets. J. Ind. Ecol. 20, 145–153. doi: 10.1111/jiec.12272
Hamon K. G., Kreiss C. M., Pinnegar J. K., Bartelings H., Batsleer J., Catalán I. A., et al. (2021). Future socio-political scenarios for aquatic resources in Europe: An operationalized framework for marine fisheries projections. Front. Mar. Sci. 8, 578516. doi: 10.3389/fmars.2021.578516
Harfoot M. B. J., Newbold T., Tittensor D. P., Emmott S., Hutton J., Lyutsarev V, et al (2014). 'Emergent global patterns of ecosystem structure and function from a mechanistic general ecosystem model. PLoS Biol. 12, e1001841. doi: 10.1371/journal.pbio.1001841
Hidalgo M., Bartolino V., Coll M., Hunsicker M. E., Travers-Trolet M., Browman H. I. (2022). 'Adaptation science' is needed to inform the sustainable management of the world's oceans in the face of climate change. ICES J. Mar. Sci. 79, 457–462. doi: 10.1093/icesjms/fsac014
Hidalgo M., Rouyer T., Bartolino V., Cerviño S., Ciannelli L., Massutí E, et al. (2012). Context-dependent interplays between truncated demographies and climate variation shape the population growth rate of a harvested species. Ecography 35, 637–649. doi: 10.1111/j.1600-0587.2011.07314.x
Hidalgo M., Rouyer T., Molinero J., Massutí E., Moranta J., Guijarro B., et al. (2011). Synergistic effects of fishing-induced demographic changes and climate variation on fish population dynamics. Mar. Ecol. Prog. Ser. 426, 1–12. doi: 10.3354/meps09077
Hilborn R. (2010). Pretty good yield and exploited fishes. Mar. Policy 34, 193–196. doi: 10.1016/j.marpol.2009.04.013
Hjort J. (1914). Fluctuations in the great fisheries of northern Europe viewed in the light of biological research. ICES 20, 1–228.
Hoefnagel E., de Vos B., Buisman E. (2015). Quota swapping, relative stability, and transparency. Mar. Policy 57, 111–119. doi: 10.1016/j.marpol.2015.03.012
Holland D. S., Speir C., Agar J., Crosson S., DePiper G., Kasperski S, et al. (2017). Impact of catch shares on diversification of fishers’ income and risk. Proc. Natl. Acad. Sci 114, 9302–9307. doi: 10.1073/pnas.170238211
Holsman K. K., Hazen E. L., Haynie A., Gourguet S., Hollowed A., Bograd S. J., et al. (2019). Towards climate resiliency in fisheries management. ICES J. Mar. Sci. 76 (5), 1368–1378. doi: 10.1093/icesjms/fsz031
Hornborg S., Smith A. D.M. (2020). Fisheries for the future: greenhouse gas emission consequences of different fishery reference points. ICES J. Mar. Sci. 77, 1666–1671. doi: 10.1093/icesjms/fsaa077
Howell D., Schueller A. M., Bentley J. W., Buchheister A., Chagaris D., Cieri M., et al. (2021). Combining ecosystem and single-species modeling to provide ecosystem-based fisheries management advice within current management systems. Front. Mar. Sci. 7, 1163. doi: 10.3389/fmars.2020.607831
Hsieh C. H., Yamauchi A., Nakazawa T, Wang W.F. (2010). Fishing effects on age and spatial structures undermine population stability of fishes. Aquat. Sci. 72, 165–178. doi: 10.1007/s00027-009-0122-2
ICES (2020). “International Council for the Exploration of the Sea Guide to ICES advisory framework and principles,” in Report of the ICES advisory committee 2020 (Copenhagen, Denmark:ICES Advice). doi: 10.17895/ices.advice.7648
ICES (2021). “International Council for the Exploration of the Sea. Advice on fishing opportunities,” in Report of the ICES advisory committe (Copenhagen, Denmark:ICES Advice). doi: 10.17895/ices.advice.7720
ICES (2022a). “International Council for the Exploration of the Sea Workshop on ICES reference points (WKREF1),” in ICES scientific reports. doi: 10.17895/ices.pub.9822
ICES (2022b). International Council for the Exploration of the Sea “Workshop on ICES reference points (WKREF2)” (Copenhagen: ICES Scientific Reports). doi: 10.17895/ices.pub.9822
Jafarzadeh S., Ellingsen H., Aanondsen S. A. (2016). Energy efficiency of Norwegian fisheries from 2003 to 2012. J. Cleaner Production 112, 3616–3630. doi: 10.1016/j.jclepro.2015.06.114
Jungclaus J. H., Fischer N., Haak H., Lohmann K., Marotzke J., Matei D., et al. (2013). Characteristics of the ocean simulations in the max planck institute ocean model (MPIOM) the ocean component of the MPI-earth system model. J. Adv. Model. Earth Syst. 5, 422–446. doi: 10.1002/jame.20023
Kell L. T., Mosqueira I., Grosjean P., Fromentin J.-M., Garcia D., Hillary R., et al. (2007). FLR: an open-source framework for the evaluation and development of management strategies. ICES J. Mar. Sci. 64, 640–646. doi: 10.1093/icesjms/fsm012
Kjesbu O. S., Sundby S., Sandø A. B., Alix M., Hjøllo S. S., Tiedemann M., et al. (2022). Highly mixed impacts of near-future climate change on stock productivity proxies in the north East Atlantic. Fish Fish 23, 601–615. doi: 10.1111/faf.12635
Köster F. W., Huwer B., Kraus G., Diekmann R., Eero M., Makarchouk A., et al. (2020). Egg production methods applied to Eastern Baltic cod provide indices of spawning stock dynamics. Fish Res. 227, 105553. doi: 10.1016/j.fishres.2020.105553
Kühn B., Taylor M. H., Kempf A. (2021). Using machine learning to link spatiotemporal information to biological processes in the ocean: a case study for north Sea cod recruitment. Mar. Ecol. Prog. Ser. 664, 1–22. doi: 10.3354/meps13689
Mackenzie B. R., Gislason H., Möllmann C., Köster F. W. (2007). Impact of 21st century climate change on the Baltic Sea fish community and fisheries. Global Change Biol. 13, 1348–1367. doi: 10.1111/j.1365-2486.2007.01369.x
Marshall N., Marshall P. (2007). Conceptualizing and operationalizing social resilience within commercial fisheries in northern Australia. Ecol. Soc. 12 (1). doi: 10.5751/ES-01940-120101
Maynou F., Sabatés A., Salat J. (2014). Clues from the recent past to assess recruitment of Mediterranean small pelagic fshes under sea warming scenarios. Clim. Change 126 (1), 175–188. doi: 10.1007/s10584-014-1194-0
Mouquet N., Lagadeuc Y., Devictor V., Doyen L., Duputié A., Eveillard D., et al. (2015). Review: predictive ecology in a changing world. J. Appl. Ecol, 52:1293–310. doi: 10.1111/1365-2664.12482
O'Leary C. A., DeFilippo L. B., Thorson J. T., Kotwicki S., Hoff G. R., Kulik V. V., et al. (2022). Understanding transboundary stocks' availability by combining multiple fisheries-independent surveys and oceanographic conditions in spatiotemporal models. ICES J. Mar. Sci. 79, 1063–74. doi: 10.1093/icesjms/fsac046
Parker R. W., Blanchard J. L., Gardner C., Green B. S., Hartmann K., Tyedmers P. H., et al. (2018). Fuel use and greenhouse gas emissions of world fisheries. Nat. Climate Change 8 (4), 333–337. doi: 10.1038/s41558-018-0117-x
Parker R. W., Vázquez-Rowe I., Tyedmers P. H. (2015). Fuel performance and carbon footprint of the global purse seine tuna fleet. J. Cleaner production 103, 517–524. doi: 10.1016/j.jclepro.2014.05.017
Parker R. W.R., Tyedmers P. H. (2015). Fuel consumption of global fishing fleets: current understanding and knowledge gaps. Fish Fish 16, 684–96. doi: 10.1111/faf.12087
Peck M. A., Alheit J., Bertrand A., Catalan I. A., Garrido S., Moyano M., et al. (2021). Small pelagic fish in the new millennium: A bottom-up view of global research effort. Prog. Oceanogr. 191, 102494. doi: 10.1016/j.pocean.2020.102494
Peck M. A., Pinnegar J. (2018). “"Climate change impacts, vulnerabilities and adaptations: North Atlantic and Atlantic Arctic marine fisheries,",” in Impacts of climate change on fisheries and aquaculture: Synthesis of current knowledge, adaptation and mitigation options. Eds. Barange M., Bahri T., Beveridge M. C. M., Cochrane K. L., Funge-Smith S., Poulain F. (Rome: FAO), 87–112.
Pécuchet L., Nielsen J. R., Christensen A. (2015). Impacts of the local environment on recruitment: a comparative study of north Sea and Baltic Sea fish stocks. ICES J. Mar. Sci. 72, 1323–1335. doi: 10.1093/icesjms/fsu220
Pelletier N., André J., Charef A., Damalas D., Green B., Parker R. (2014). Energy prices and seafood security. Global Environ. Change 24, 30–41. doi: 10.1016/j.gloenvcha.2013.11.014
Pinsky M. L., Reygondeau G., Caddell R., Palacios-Abrantes J., Spijkers J., Cheung W. W. (2018). Preparing ocean governance for species on the move. Science 360 (6394), 1189–1191. doi: 10.7282/T3M61PPC
Planque B., Fromentin J.-M., Cury P., Drinkwater K. F., Jennings S., Perry R. I., et al. (2010). How does fishing alter marine populations and ecosystems sensitivity to climate? J. Mar. Syst. 79, 403–417. doi: 10.1016/j.jmarsys.2008.12.018
Plikshs M. (2015). Reproduction of Baltic cod, gadus morhua (Actinopterygii: Gadiformes: Gadidae), in the gotland basin: Causes of annual variability. Acta Ichthyologica Piscatoria 45, 247–258. doi: 10.3750/AIP2015.45.3.04
Poos J. J., Turenhout M. N., AE van Oostenbrugge H., Rijnsdorp A. D. (2013). Adaptive response of beam trawl fishers to rising fuel cost. Ices J. Mar. Sci. 70 (3), 675–684. doi: 10.1093/icesjms/fss196
Punt A. E., Butterworth D. S., de Moor C. L., De Oliveira J. A. A., Haddon M. (2016). Management strategy evaluation: best practices. Fish Fish 17, 303–334. doi: 10.1111/faf.12104
Rak D., Walczowski W., Dzierzbicka-Głowacka L., Shchuka S. (2020). Dissolved oxygen variability in the southern Baltic Sea in 2013–2018. Oceanologia 62, 525–537. doi: 10.1016/j.oceano.2020.08.005
Rijnsdorp A. D., Depestele J., Eigaard O. R., Hintzen N. T., Ivanovic A., Molenaar P., et al. (2020). Mitigating seafloor disturbance of bottom trawl fisheries for north Sea sole solea solea by replacing mechanical with electrical stimulation. P L o S One 15 (11), e0228528. doi: 10.1371/journal.pone.0228528
Rindorf A., Dichmont C. M., Levin P. S., Mace P., Pascoe S., Prellezo R., et al. (2017). Food for thought: pretty good multispecies yield. ICES J. Mar. Sci. 74, 475–486. doi: 10.1093/icesjms/fsw071
Rijnsdorp A. D., Batsleer J., Molenaar P (2021). The effect of electrical stimulation on the footrope and cod-end selection of a flatfish bottom trawl. Fish. Res 243, 106104. doi: 10.1016/j.fishres.2021.106104
Sakalli A., Başusta N. (2018). Sea Surface temperature change in the black Sea under climate change: A simulation of the sea surface temperature up to 2100. Int. J. Climatol. 38, 4687–4698. doi: 10.1002/joc.5688
Sala A., De Carlo F., Buglioni G., Lucchetti A. (2011). Energy performance evaluation of fishing vessels by fuel mass flow measuring system. Ocean Eng. 38, 804–809. doi: 10.1016/j.oceaneng.2011.02.004
Sala A., Notti E., Martinsohn J., Damalas D. (2012). “Information collection in energy efficiency for fisheries (ICEEF2011). final report,” in JRC Scientific and Policy Reports (European Union, Luxembourg: JRC Joint Research Center), 132.
Saraiva S., Meier H. E. M, Andersson H., Höglund A., Dieterich C., Gröger M, et al. (2019). Uncertainties in projections of the baltic sea ecosystem driven by an ensemble of global climate models. Front. Earth Sci 6, 244. doi: 10.3389/feart.2018.00244
Schau E. M., Ellingsen H., Endal A., Aanondsen S. A. (2009). Energy consumption in the Norwegian fisheries. J. Cleaner production 17 (3), 325–334. doi: 10.1016/j.jclepro.2008.08.015
Sein D. V., Mikolajewicz U., Gröger M., Fast I., Cabos W., Pinto J. G., et al. (2015). Regionally coupled atmosphere-ocean-sea ice-marine biogeochemistry model ROM: 1. Description Valid. J. Adv. Model. Earth Syst. 7 (1), 268–304. doi: 10.1002/2014MS000357
STECF (2017). Scientific, technical and economic committee for fisheries (STECF) – bio-economic methodology (EWG-17-05); (Luxembourg; EUR: Publications Office of the European Union). doi: 10.2760/759034
STECF (2020). Scientific, technical and economic committee for fisheries (STECF) – assessment of balance indicators for key fleet segments and review of national reports on member states efforts to achieve balance between fleet capacity and fishing opportunities (STECF-21-16) (Luxembourg: Publications Office of the European Union)2020.
STECF (2021). Scientific, technical and economic committee for fisheries (STECF) - the 2021 annual economic report on the EU fishing fleet (STECF 21-08) (Luxembourg: Publications Office of the European Union). doi: 10.2760/60996
STECF (2022a). Scientific, technical and economic committee for fisheries (STECF) – monitoring of the performance of the common fisheries policy (STECF-Adhoc-22-01) (Luxembourg: Publications Office of the European Union). doi: 10.2760/566544
STECF (2022b). Scientific, technical and economic committee for fisheries (STECF) – 69th plenary report (PLEN-22-01) (Luxembourg: Publications Office of the European Union). doi: 10.2760/192738
Suuronen P., Chopin F., Glass C., Løkkeborg S., Matsushita Y., Queirolo D., et al. (2012). Low impact and fuel efficient fishing–looking beyond the horizon. Fish Res. 119, 135–146. doi: 10.1016/j.fishres.2011.12.009
Svedäng H., Savchuk O., Villnäs A., Norkko A., Gustafsson B. G., Wikström S. A., et al. (2022). Re-thinking the “ecological envelope” of Eastern Baltic cod (gadus morhua): conditions for productivity, reproduction, and feeding over time. ICES J. Mar. Sci. 79, 689–708. doi: 10.1093/icesjms/fsac017
Taylor K. E., Stouffer R. J., Meehl G. A. (2012). An overview of CMIP5 and the experiment design. Bull. Am. Meteorol. Soc. 93, 485–498. doi: 10.1175/BAMS-D-11-00094.1
van der Veer H. W., Geffen A. J., Witte J. I. J. (2000). Exceptionally strong year classes in plaice pleuronectes platessa: are they generated during the pelagic stage only, or also in the juvenile stage? Mar. Ecol. Prog. Ser. 199, 255–262. doi: 10.3354/meps199255
van de Wolfshaar K. E., Barbut L., Lacroix G. (2022). From spawning to first-year recruitment: the fate of juvenile sole growth and survival under future climate conditions in the north Sea. ICES J. Mar. Sci. 79, 495–505. doi: 10.1093/icesjms/fsab025
Vert-pre K. A., Amoroso R. O., Jensen O. P., Hilborn R. (2013). Frequency and intensity of productivity regime shifts in marine fish stocks. Proc. Natl. Acad. Sci 110, 1779–1784. doi: 10.1073/pnas.1214879110
Villasante S., Sumaila R., Da-Rocha J. M., Carvalho N., Skerritt D. J., Schuhbauer A., et al. (2022). Strengthening European union fisheries by removing harmful subsidies. Mar. Policy 136, 104884. doi: 10.1016/j.marpol.2021.104884
Wåhlström I., Höglund A., Almroth-Rosell E., MacKenzie B. R., Gröger M., Eilola K., et al. (2020). Combined climate change and nutrient load impacts on future habitats and eutrophication indicators in a eutrophic coastal sea. Limnol. Oceanogr. 65, 2170–2187. doi: 10.1002/lno.11446
Woods P. J., Macdonald J. I., Bárðarson H., Bonanomi S., Boonstra W. J., Cornell G., et al. (2022).A review of adaptation options in fisheries management to support resilience and transition under socio-ecological change. ICES J. Mar. Sci. 79, 463–479. doi: 10.1093/icesjms/fsab146
Wu Y.-L., Lan K.-W., Tian Y. (2020). Determining the effect of multiscale climate indices on the global yellowfin tuna (Thunnus albacares) population using a time series analysis. Deep Sea Res. Part II: Topical Stud. Oceanogr. 175, 104808. doi: 10.1016/j.dsr2.2020.104808
Ziegler F., Hansson P.-A. (2003). Emissions from fuel combustion in Swedish cod fishery. J. Cleaner production 11 (3), 303–314. doi: 10.1016/S0959-6526(02)00050-1
Keywords: blue economy, decarbonization, fisheries economics, fisheries management, resilience, climate change, EU green deal
Citation: Bastardie F, Feary DA, Brunel T, Kell LT, Döring R, Metz S, Eigaard OR, Basurko OC, Bartolino V, Bentley J, Berges B, Bossier S, Brooks ME, Caballero A, Citores L, Daskalov G, Depestele J, Gabiña G, Aranda M, Hamon KG, Hidalgo M, Katsanevakis S, Kempf A, Kühn B, Nielsen JR, Püts M, Taylor M, Triantaphyllidis G, Tsagarakis K, Urtizberea A, van Hoof L and van Vlasselaer J (2022) Ten lessons on the resilience of the EU common fisheries policy towards climate change and fuel efficiency - A call for adaptive, flexible and well-informed fisheries management. Front. Mar. Sci. 9:947150. doi: 10.3389/fmars.2022.947150
Received: 18 May 2022; Accepted: 06 July 2022;
Published: 04 August 2022.
Edited by:
Rachel Gjelsvik Tiller, SINTEF, NorwayReviewed by:
Tannaz Alizadeh Ashrafi, UiT The Arctic University of Norway, NorwayHung-Tai Lee, National Taiwan Ocean University, Taiwan
Dimitrios K. Moutopoulos, University of Patras, Greece
Copyright © 2022 Bastardie, Feary, Brunel, Kell, Döring, Metz, Eigaard, Basurko, Bartolino, Bentley, Berges, Bossier, Brooks, Caballero, Citores, Daskalov, Depestele, Gabiña, Aranda, Hamon, Hidalgo, Katsanevakis, Kempf, Kühn, Nielsen, Püts, Taylor, Triantaphyllidis, Tsagarakis, Urtizberea, van Hoof and van Vlasselaer. This is an open-access article distributed under the terms of the Creative Commons Attribution License (CC BY). The use, distribution or reproduction in other forums is permitted, provided the original author(s) and the copyright owner(s) are credited and that the original publication in this journal is cited, in accordance with accepted academic practice. No use, distribution or reproduction is permitted which does not comply with these terms.
*Correspondence: Francois Bastardie, fba@aqua.dtu.dk; David A. Feary, d.feary@mrag.co.uk
†These authors have contributed equally to this work