- 1Centro de Investigación y de Estudios Avanzados, Depto. de Recursos del Mar, Instituto Politécnico Nacional, Mérida, Yucatán, Mexico
- 2Centro Interdisciplinario de Ciencias Marinas, Depto. de Plancton y Ecología Marina, Instituto Politécnico Nacional, La Paz, Baja California Sur, Mexico
- 3Laboratorio de Entomología Molecular, Red de Estudios Moleculares Avanzados, Clúster Científico y Tecnológico BioMimic®, El Instituto de Ecología, Xalapa, Veracruz, Mexico
Global commercial catches of squid have increased greatly in the last few years. However, approximately a quarter of the squid catches are still unidentified. In the southeastern Gulf of Mexico (SGoM), the squid catches are not recorded most of the time and are unidentified. This lack of knowledge limits the evaluation of the populations and prevents the establishment of conservation strategies. In this study, we used morphological and molecular (DNA barcoding– cytochrome c oxidase subunit I gene) identification tools to analyze the species composition of the family Loliginidae based on their paralarvae (PL), their abundance distribution by size class, and their genetic structure on the Yucatan Shelf, SGoM. A total of 134 PL were also collected from three oceanographic cruises held in 2015, 2016, and 2018. A total of 56 adults were collected from three ports of Yucatan. Both adults and PL were identified as Doryteuthis pleii (Blainville, 1823). The highest abundances of PL were detected from the West and the Central zones of the Yucatan Shelf at 50- and 15-m depth isobaths at 163 and 21 km from the coastline, respectively. The abundance was higher (316 PL•1,000 m-3) in the early summer (June 2018), medium (213 PL•1,000 m-3) at the end of summer and early autumn, and very low (24 PL•1,000 m-3) in late autumn. A high haplotype and nucleotide diversity (Hd= 0.77; π= 0.002) with no structured population (Fst < 0) was also found, suggesting a continuous gene flow throughout the Yucatan Shelf. This information establishes the basis for a future comprehensive understanding of their biological cycle and population dynamics.
Introduction
Squids are relevant species for several fisheries around the world (FAO, 2020). Over the past two decades, the commercial catch and trade of squid have remained at the relatively high levels that have marked its almost continuous growth worldwide, while many coastal human populations obtain a significant proportion of their protein intake from locally caught cephalopod species (Arkhipkin et al., 2015; FAO, 2020).
The bulk of the global squid catch comprises species from two families, the Ommastrephidae and Loliginidae (Arkhipkin et al., 2015). However, approximately a quarter of the squid catches are still unidentified and/or are usually reported as common squid “Loliginidae” and/or “various squids” categories (Rodhouse, 2005). This is also true for the southeastern Gulf of Mexico (SGoM), where the loliginid squid fishery is mostly obtained incidentally as a by-catch from the shrimp and sardine fisheries, while catches per unit of effort are not recorded most of the time (Solís-Ramírez et al., 1998; Comisión Nacional de Pesca (CONAPESCA), 2018). The lack of reliable information limits the evaluation of the stocks and impedes the establishment of local management and/or conservation strategies (Ward, 2000; Olmos-Pérez et al., 2018).
Loliginid squids are demersal species, usually occupying coastal marine waters in tropical and temperate regions worldwide (Jereb and Roper, 2010). Like other cephalopods, loliginids have a zooplanktonic larval stage known as paralarvae (PL) (Young and Harmnan, 1988). Most PL are distributed in the first 200 m, so their catch is more efficient than adults and they are good indicators of the species richness of a region (De Silva-Dávila et al., 2018).
The taxonomy and systematics of the adults of the Loliginidae family are complicated due to the lack of taxonomic stability (subfamily to subgenus levels), inconsistent identification diagnosis, the existence of cryptic species, and natural hybridization (Brakoniecki, 1996; Vecchione et al., 2005; Jereb and Roper, 2010; Granados-Amores et al., 2013). Moreover, there is a lack of PL descriptions for tropical species (Sweeney et al., 1992; Vecchione et al., 2005), and there are important morphological differences between PL and juveniles, which do not allow morphological identification through their ontogenetic development (De Silva-Dávila et al., 2013; Kim et al., 2019). These constraints prevent unraveling loliginid life histories, particularly in tropical areas of the western Atlantic region (Rodhouse, 2015), such as the Gulf of Mexico (GoM).
At least five loliginid species inhabit the GoM, that is, Doryteuthis (Amerigo) pealeii (Lesueur, 1821), D. (Doryteuthis) pleii (Blainville, 1823), Lolliguncula (Lolliguncula) brevis (Blainville, 1823), D. (Doryteuthis) roperi (Cohen, 1976), and Sepioteuthis sepioidea (Blainville, 1823) (Judkins et al., 2009; Jereb and Roper, 2010; Judkins et al., 2010; Judkins et al., 2017). Ecological information available relies on adult collections created for taxonomic identification (Barrientos and García-Cubas, 1997; Díaz-Santana-Iturrios et al., 2019; Bravo-Muñoz, 2020), to describe their distribution, abundance, fishing prospects (Judkins et al., 2009; Díaz-Santana-Iturrios et al., 2019), and on fishing reports aimed to analyze the exploitation state of squid populations (Arreguín-Sánchez and Arcos-Huitrón, 2011). Although there are descriptions of loliginid hatchlings, the information on their presence in the GoM is scanty (Sweeney et al., 1992).
Molecular tools have facilitated the identification of cephalopod species at any developmental stage (Allcock et al., 2015; Díaz-Santana-Iturrios et al., 2019; Castillo-Estrada et al., 2020; Santana-Cisneros et al., 2021). This can be done using molecular markers such as the cytochrome c oxidase subunit I (COI) gene, which serves as a universal DNA barcode for organisms (Hebert et al., 2003). This gene can also provide information about many ecological traits, such as genetic diversity, population structure, genetic connectivity, phylogeographic analysis, and gene flow (Cowen and Sponaugle, 2009; Hellberg, 2009; Bucklin et al., 2011). This information is fundamental for the studies of population ecology and fisheries management (Ibáñez and Poulin, 2014; Sales et al., 2017; Roura et al., 2019).
Based on the above, in this study, we identified loliginid PL collected in the SGoM and compared them with the adult species reported in the fishing landings in the Yucatan Peninsula to know if they are the same species/populations. This information will aid in the understanding of the species composition and genetic structure of loliginids in the SGoM useful to support further fishing programs and/or management of this fishery.
Materials and methods
Study area
The SGoM is dominated by a large continental shelf that extends 200 km to the north offshore the Yucatan Peninsula and is 600 km long in an East–West direction. The study area (Figure 1) comprises the complete length and breadth of the Yucatan Shelf (YS), also known as the Campeche Bank, where the main fisheries of the region take place (Botello et al., 2010). In this area, there are three climatic seasons: the rainy season (June–October)—when tropical depressions occur, the dry season (March–May), and the anticyclonic (“Nortes”) season (November–February), with cold fronts in which the water column is mixed, homogenizing environmental conditions (Lara-Lara et al., 2008; Enriquez et al., 2013).
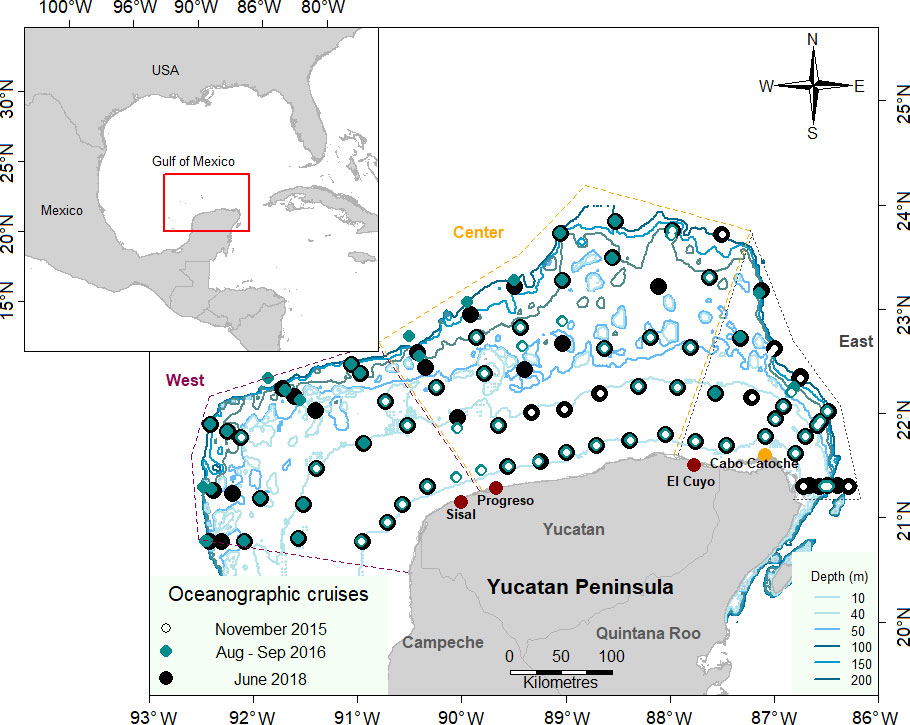
Figure 1 Study area and zooplankton sampling stations in the Yucatan Shelf, Southeastern Gulf of Mexico (SGoM). Red dots represent the ports where adult organisms were sampled. The dotted lines represent the three zones: Western (— purple), Central (—orange), and Eastern (—gray).
Field sample collection
Zooplankton samples were collected during three oceanographic cruises held from 2nd to 20th November 2015 (GOMEX-4, G4), 25th August to 8th September 2016 (GOMEX-5, G5) both onboard of the R/V “Justo Sierra” of the Universidad Nacional Autónoma de México, and from 5th to 18th July 2018 (GOMEX-6, G6) onboard the O/V Alpha Helix of the Centro de Investigación Científica y de Educación Superior de Ensenada. A permit for the mentioned collections (PPF/DGOPA-070/16) was issued by “Comisión Nacional de Acuacultura y Pesca.”
The sampling design consisted of 18 transects perpendicular to the Yucatan coast, separated horizontally from each other by 35 km. Each transect comprised five sampling stations, and these were located to follow five different isobaths: 15, 50, 100, 150, and 200 m. The study area was also divided into three zones according to the oceanographic conditions on the shelf: West (influenced mainly by the Campeche Canyon Current, CCC), Central (as a transition zone and influenced by submarine groundwater discharges near the coast), and East, influenced by the Cabo Catoche upwelling (Enriquez et al., 2013) (Figure 1).
In each sampling station, surface trawls (10-m depth) were carried out at a constant speed of 2.5 knots using a standard Bongo structure (0.6-m diameter, with 333-µm mesh nets), and equipped with mechanically calibrated flowmeters (Sea Gear), used to estimate the volume of filtered water (Sameoto et al., 2000).
The samples of the G4 and G6 cruises were fixed in 96% ethanol with a complete replacement of the fixative after 24 h. The G5 samples were fixed in a 7% buffered formaldehyde solution. The G5 samples were not considered for molecular identification but were deposited at the collection of the Zooplankton Laboratory of the Centro de Investigación y de Estudios Avanzados of the IPN Unit Mérida. In addition, 34% (26 PL) of the G6 samples were included in the biological repository.
Adult loliginid specimens from the Yucatán coast were sampled in September and November 2019 in Sisal, Progreso, and El Cuyo ports where the by-catch fauna of the local artisanal sardine fishery is landed. Moreover, these ports are in the West, Central, and East zones of the studied area, respectively. Adults were sampled in only one vessel per port, and in each case, a tissue sample of ~1 cm3 was dissected from the third left arm, fixed in 96% ethanol for molecular identification, and tagged.
Morphological and molecular identification
Loliginid PL were extracted from the entire zooplankton samples without fractionation. Some of the best-preserved specimens were photographed in dorsal and ventral views to keep a visual record of their main taxonomic characteristics (dorsal head and mantle chromatophore patterns) and were identified to the most precise taxonomic level according to the criteria of Hanlon et al. (1992) and Vecchione et al. (2001). Adults from the landings were identified according to Jereb and Roper (2010) and Young et al. (2019).
The full body of G4 and G6 loliginid PL, and the adult samples (<1 g) were used for genomic DNA (gDNA) extraction using the Quick-DNA™ Universal kit (Zymo Research©) according to the manufacturer’s protocol for total DNA isolation from solid tissues. The DNA concentration and quality were checked in a UV-visible Spectrophotometer QQIAxpert System de Qiagen. The genetic barcode identification was done with the mitochondrial cytochrome c oxidase (COI) gene, amplified using the universal metazoan primers LCO1490 (5’-GGTCAA CAA ATC ATA AAG ATA TTG G-3 ‘) and HCO2198 (5’-TAA ACT TCA GGG TGA CCA AAA AATCA-3’) (Folmer et al., 1994). Polymerase chain reactions (PCRs) were carried out using 0.25 μl of a mix of the two primers (10 μM), 12.5 μl of DreamTaq Green PCR Master Mix (Thermo Scientific©), 1 μl of gDNA (5–300 ng μl−1), and the remainder of nuclease-free water to obtain a final volume of 25 μl. Amplification conditions were: initial denaturation at 95°C for 3 min, followed by 40 cycles of denaturation at 95°C for 30 s, annealing at 50°C for 30 s, and extension at 72°C for 1 min, with a final elongation at 72°C for 5 min in a C1000 Touch Thermal Cycler (BIORAD™). Amplicons were sequenced in both senses using the Sanger et al. (1977) method by Macrogen Inc., Seoul, South Korea. DNA sequences were aligned with MUSCLE (Edgar, 2004) in MEGA7 software (Kumar et al., 2016), and aligned sequences were manually curated. The filtered sequences were compared with the Barcode of Life Data (BOLD) using their Identification System for COI-accepted sequences (https://boldsystems.org).
To corroborate the identity of the DNA sequences, we used published sequences of each loliginid species whose geographical distribution is within the study area (Table S1): D. pealeii, L. brevis, S. sepioidea, and sequences of D. pleii for North America (northern GoM and the northwestern Atlantic Ocean), western GoM—Tamaulipas, and for Brazil. The sequence of Dosidicus gigas was used as an outgroup. These sequences, together with the new sequences obtained from our analysis, were collapsed with CD-HIT-EST (t=0, c=0.98, n=5) to eliminate redundancies (Niu et al., 2010; Fu et al., 2012). The collapsed sequences were aligned with MUSCLE (Edgar, 2004) and formatted to nexus with Seqmagick (https://github.com/fhcrc/seqmagick).
The best evolutionary model was determined using the jModelTest2 (Darriba et al., 2012), using the Bayesian information criterion (BIC) for the Bayesian inference analyses. According to jModelTest2, we selected the GTR + I + G model. The phylogenetic tree was inferred using Mr. Bayes v. 3.2 (Ronquist et al., 2012). The tree was based on MCMC (Markov chain Monte Carlo) sampling, with 3,000,000 generations, a sampling frequency of 1,000, a consensus rule of 50%, and four chains (one cold and three hot), until achieving an average standard deviation of split frequencies >0.01. The 25% of the first trees sampled in the MCMC run were discarded as burn-in, and a consensus tree was obtained with the remainder. The resulting tree was converted to the Newick format with AfterPhylo.pl and was exported to the Interactive Tree of Life (iTol) (Ciccarelli et al., 2006 https://itol.embl.de/) for its visualization.
Size structure and abundance distribution of loliginid paralarvae
The dorsal mantle length (ML ± 0.01 mm) of 123 PL was measured, except for 11 PL that had no mantle and were not considered for this analysis, using the NEO PiWeb reporting plus Basic software, imaging ZEN and AxioVision connected to a digital camera AxioCam ERC Rev.2 under a binocular microscope ZEISS SteREO Discovery V8. To estimate the fresh ML based on the preserved PL (PPL) sizes, a shrinkage correction factor (SCF) of 25.8% was added to the computed values (Villanueva et al., 2016) because the size of fresh PL decreases with fixation and preservation (Goto, 2005).
Since the abundance of PL at the species-specific hatching size is a precise indicator of a recent hatching event (Yatsu et al., 1999; Zeidberg et al., 2012), we used the ML of fresh and of PPL for size-frequency analysis. Considering that the hatching size reported for D. pleii is 1.5-mm ML (McConathy et al., 1980) and that the general hatching size interval of 0.1–2.5 mm is likely for the newly hatched in several cephalopods (Villanueva et al., 2016), those PL from 2.6- to 4.0-mm ML were considered older than recently hatched. The total abundance per sampling station and the abundances by size interval by the sampling station were standardized as the number of PL in 1,000 m3 of filtered seawater (PL•1,000 m-3) (Smith and Richardson, 1977; Diekmann et al., 2006). The abundance distribution of PL at the sampling stations per cruise was plotted using the R programming language (R Core Team, 2019).
Genetic analysis
Genetic diversity indices [haplotype number (h), haplotype diversity (Hd), and nucleotide diversity (π)] (Nei, 1987) were estimated using the DnaSP v5 software (Librado and Rozas, 2009). To test the genetic population differences between zones, we performed a genetic structure analysis (Fst) and the analysis of molecular variance (AMOVA) with 10,000 permutations using Arlequin v. 3.5.2 (Excoffier and Lischer, 2010). Finally, a haplotype network was generated to visualize the relationships among haplotypes using the median-joining method implemented in Network Software V 10.1.0.0 (Bandelt et al., 1999).
Results
During the three oceanographic surveys, we collected a total of 134 loliginid PL, whereas 56 adult specimens were sampled from the fishing landings in the three ports of Yucatán (Table 1).
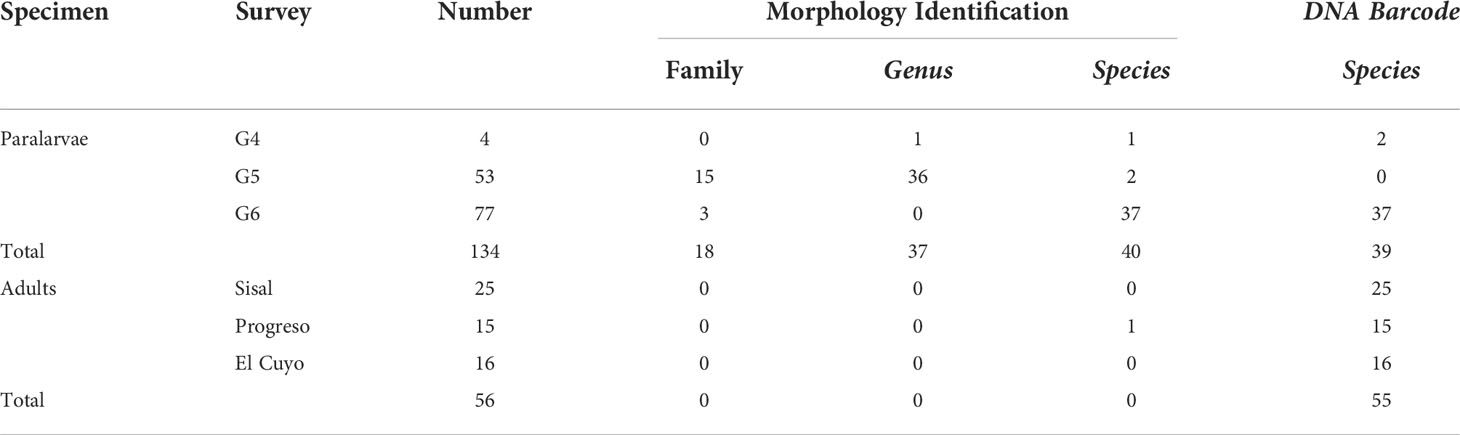
Table 1 Number of paralarvae and adults of loliginids from the southeastern Gulf of Mexico (SGoM) and level of identification by morphological and DNA barcoding approach.
Morphological and molecular identification
Using the morphological identification approach, we identified 70 PL at the species level, 38 at the genus level (Doryteuthis spp.), and 26 at the family level (Loliginidae). All the adults were identified to species level. D. pleii was the only species found for both PL (Figure 2A) and adults (Figure 2B) according to the following morphological traits.
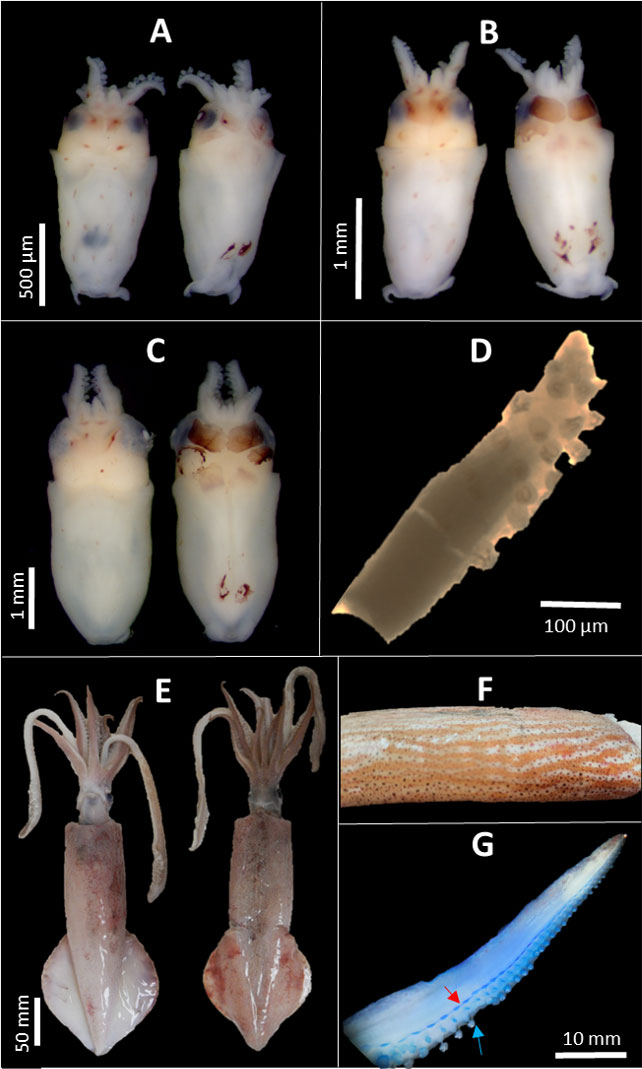
Figure 2 Doryteuthis pleii paralarvae collected in the Yucatan Shelf. Ventral and dorsal views of paralarvae of (A) 0.8 mm (B) 1.4 mm, (C) 3 mm of mantle length. (D) Tentacle club with four rows of suckers on manus of a 3 mm paralarvae. (E) Ventral and dorsal (265 mm ML), (F) hectocotylus (red arrow indicating modified suckers and blue arrow the regular ones) and (G) ventral mantle with the striped pattern along the surface of a male adult from Progreso port of Yucatan.
PL—(Figures 2A–C) Body form as bullet-shaped with well-developed terminal fins; well-developed ventral arms (arms IV > I) and tentacle clubs with four rows of suckers on the manus (Figure 2D). The chromatophore arrangement in the ventral head (in a roughly diamond-shaped quadrangle posterior to each eye) was initially observed but later faded with the fixation. Chromatophore pattern in the dorsal head, in well-preserved specimens, is 2 + 2 + 2, forming a hexagon and two central chromatophores in the lower dorsal mantle (McConathy et al., 1980; Hanlon et al., 1992).
Adult—Body long, fusiform. Mantle long, slender, cylindrical, the posterior end acutely pointed (Figure 2E), mature males present a striped pattern along the ventral surface of the mantle (Figure 2F). Fins are rhomboidal, longer than broad, usually 60% ML, and their sides fairly straight. Edge of vane straight (often slightly curved in females), thick, and ribbed or rod-like (especially in mature males). Suckers on ventral buccal lappets. Eyes not large; diameter of the externally visible eyeball 14%–19% ML, diameter of dissected lens 2%–7% ML. Left ventral arm hectocotylized in mature males by a modification of distal half to a fourth of arm that extends to arm tip; one-half to three-fourths (42–82) of suckers in a dorsal row much smaller than half the size of their ventral counterparts; modified (small) suckers on small, narrow, triangular pedicels (Figure 2G) (Hanlon et al., 1992; Vecchione & Young, 2010; Díaz-Santana-Iturrios et al., 2019; Migkuavacca and Simone, 2020).
We extracted DNA from 45 PL previously identified morphologically at the species level, two identified at the genus level, and eight identified at the family level. All adult samples were used for the molecular identification. DNA sequences were successfully obtained from 39 PL (71% of the DNA extractions: 30 from morphologically identified PL at the species level, 1 at the genus level, and 8 at the family level) and 56 adults (100%). In the remaining 16 PL, we did not obtain DNA samples of good quality, probably due to poor conservation of the samples. Thus, their identification was only based on morphological characteristics, such as the 26 PL of the G6 samples selected for the biological collection and the 53 PL of the G5 samples fixed in formalin (Table 1).
The BLAST analysis revealed that the sequences from the PL (39) and the adults (56) had 99.76%–100% similarity (99%–100% coverage) with the sequences of D. pleii (Table S2). The sequences obtained in this study were phylogenetically clustered with the D. pleii sequences from North America and western GoM but were separated from the Brazil sequences (Figure 3). The number of PL and adults and their final identification, either morphologically or molecularly, are shown in Table 1. Therefore, with both identification approaches, D. pleii was the only loliginid species found for the SGoM during November 2015, August–September 2016, and July 2018 in surface-collected zooplankton samples (Table 1, Figure 2).
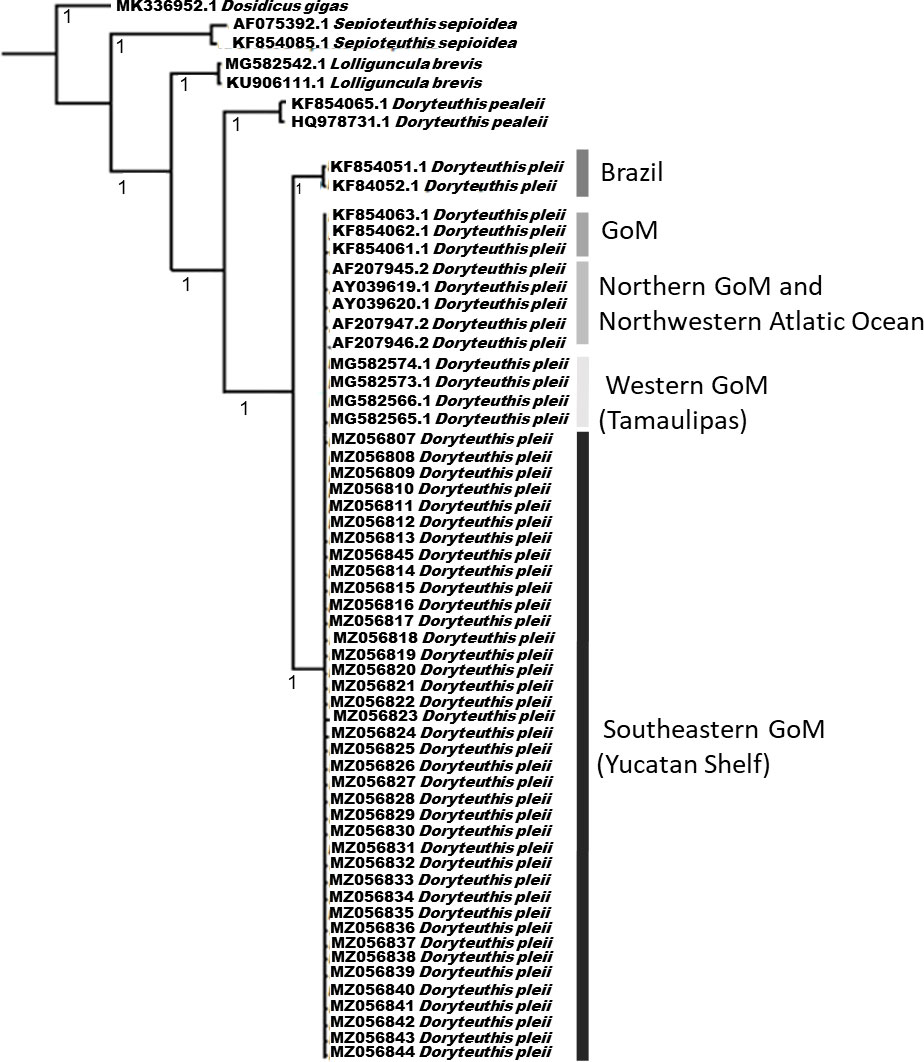
Figure 3 D. pleii phylogenetic tree comparing representative sequences of the species in other geographic regions and the sequences of other three loliginid species that occur in the same distributional area. Dosidicus gigas is used as an outgroup. The posterior probability values are plotted on each resolved branch.
Size structure and abundance distribution of loliginid paralarvae
In G4 (November 2015), the total loliginid PL collected accounted for 24 PL•1,000 m-3, during G5 (August–September 2016), they increased to 428 PL•1,000 m-3, and abundance during G6 (July 2018) reached a maximum of 974 PL•1,000 m-3. However, only those PL measured (in good morphological conditions) and their standardized abundance were included for size structure analysis. The loliginid PL from the three cruises recorded a size range between 0.88 and 4.15 mm ML (2.04 ± 0.74, n= 123) after applying the SCF (Figure 4A). The total abundance of hatchlings (≤2.5-mm ML) (for the entire period of study) was 380 PL•1,000 m-3, representing 75% of total abundance (this percentage increases by 16% if the SCF is not applied).
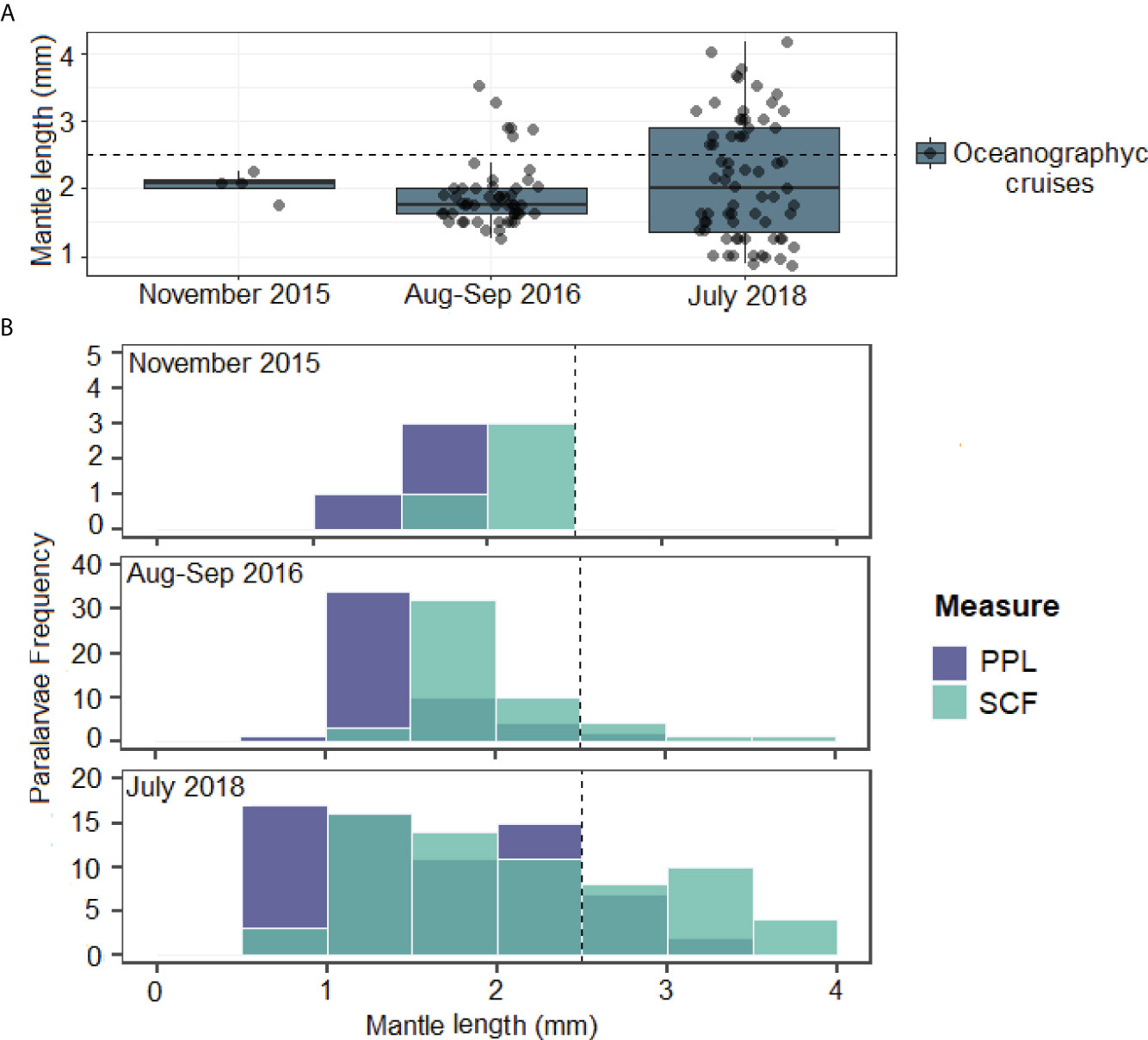
Figure 4 Loliginidae paralarvae. (A) Mantle length (ML) data dispersion by cruise carried out at the southeastern Gulf of Mexico. (B) Size structure of paralarvae during November 2015, August-September 2016, and July 2018. Frequency of preserved paralarvae (PPL) and frequency of fresh specimens were calculated with the shrinkage correction factor (SCF). The percentage of newly hatched organisms (<2.5 mm ML) with the SCF is shown.
The loliginids collected in November 2015 had a range size of 1.76–2.26-mm ML (2.05 ± 0.21, n= 4) and all were hatchlings (Figures 4A, B). In this cruise (November 2015), the lowest PL abundance recorded was 24 PL•1,000 m-3, from which 4 PL•1,000 m-3 were Doryteuthis spp. and 20 PL•1,000 m-3 were identified as D. pleii occurring mainly at the Central zone at 20–50 m deep and between 24 and 120 km from the coastline (Figure 5A, B, 6A, B).
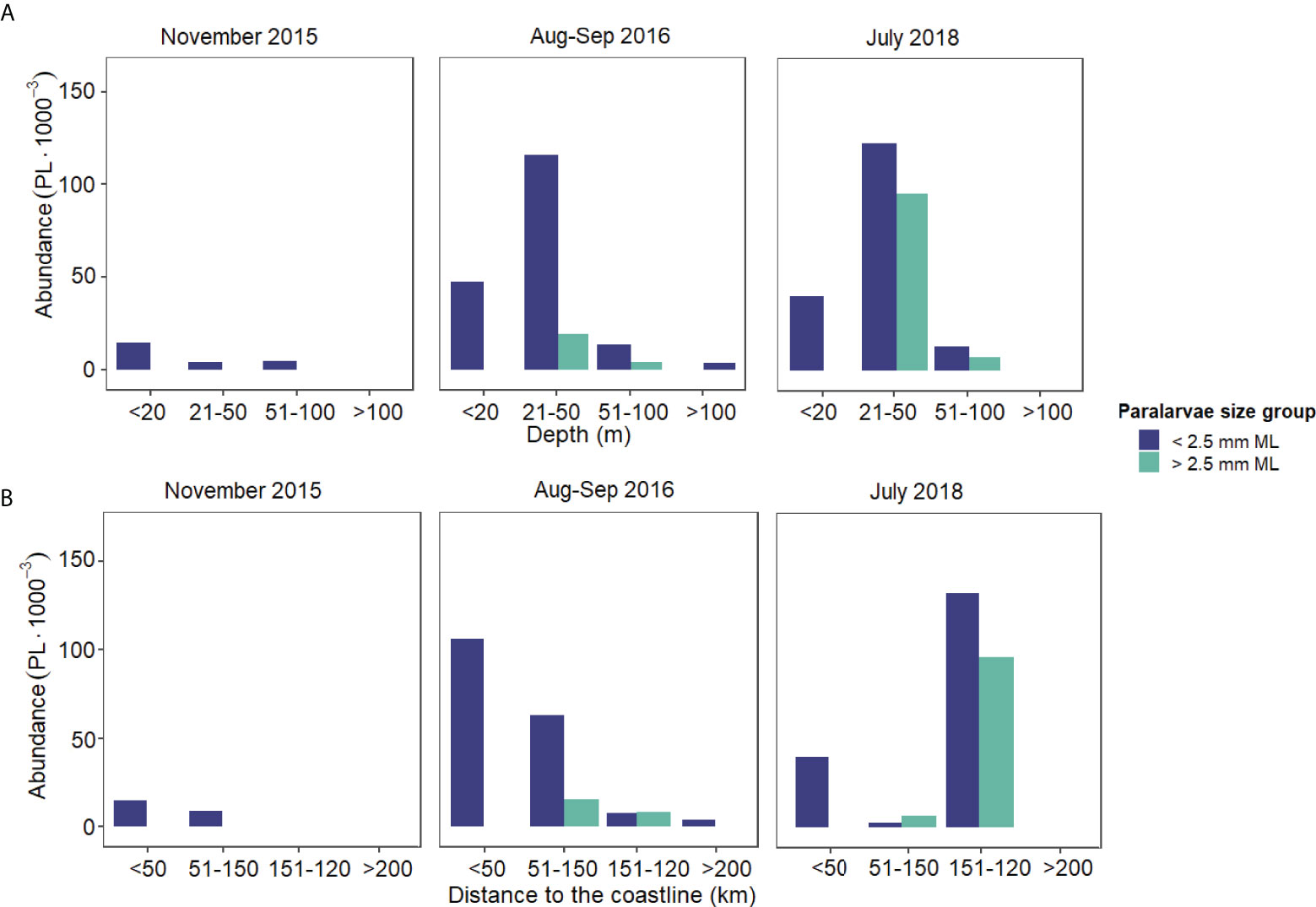
Figure 5 Horizontal distribution of Loliginidae PL abundance by size class (0.1–2.5 mm ML and 2.6–4.15-mm ML), (A) depth range and (B) distance to coastline per survey performed at the Yucatan Shelf, Southeastern Gulf of Mexico.
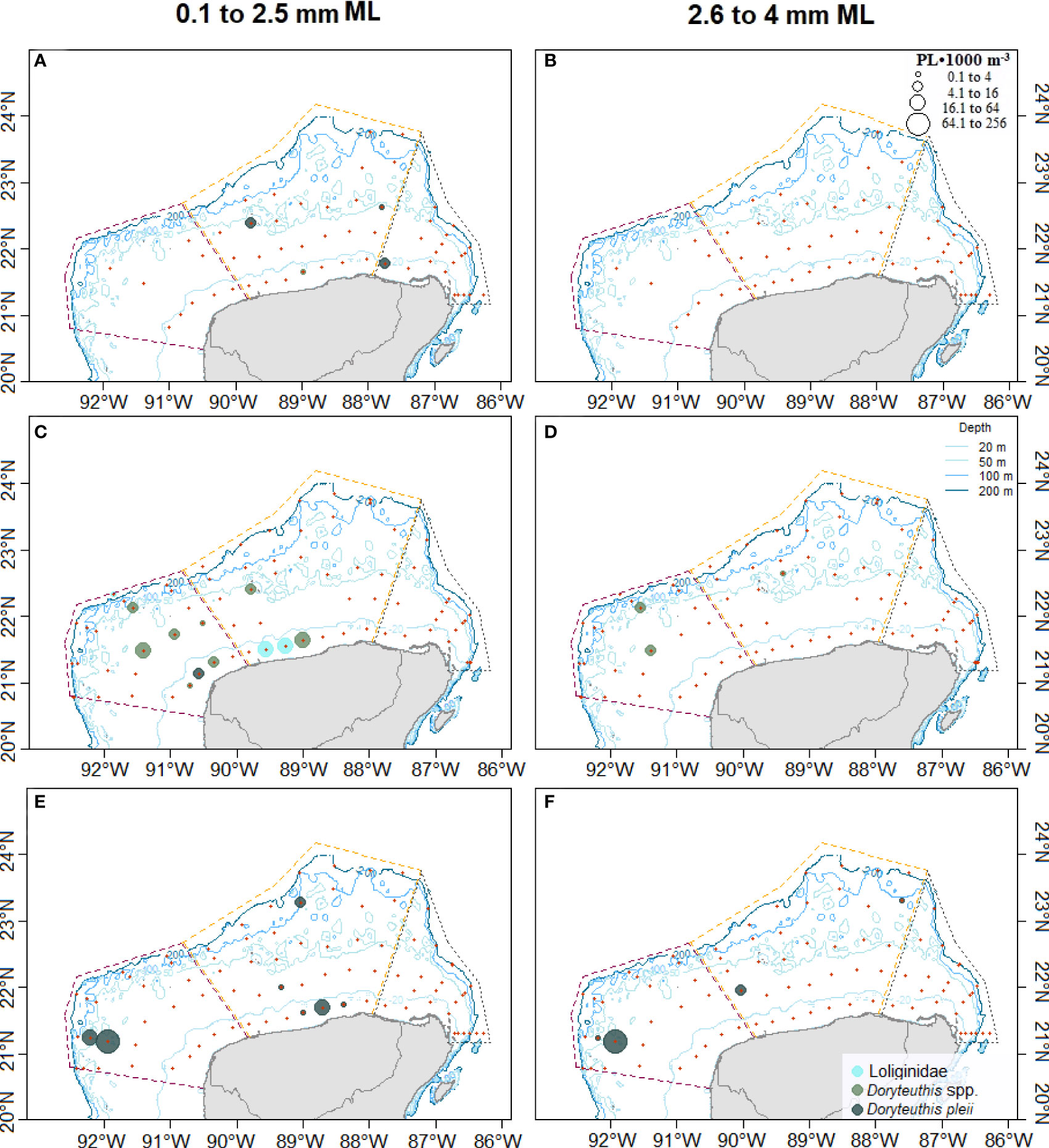
Figure 6 Distribution of the abundance of loliginids by cruise and size group in the Yucatan Shelf, Southeastern Gulf of Mexico (A, B) November 2015, (C, D) August-September 2016, and (E, F) July 2018. The dotted lines represent the three zones: Western (— purple), Central (—orange), and Eastern (—gray). Red crosses represent sampling stations.
In August–September 2016 (G5), the size range was 1.25–3.52 mm ML (1.91 ± 0.48, n= 51) (Figure 4A) of which 88% were hatchlings, but this percentage dropped by 8% in the PPL (Figure 4B). Total PL abundance in this cruise was 213 PL•1,000 m-3, of which 63 PL•1,000 m-3 were identified only at the family level, 141 PL•1,000 m-3 as Doryteuthis spp., and 9 PL•1,000 m-3 as D. pleii (Figures 6C, D). Hatchlings represented 42% of the total abundance occurring more in the West zone, over the isobaths of 20–50 m (Figure 6C). The zones with the highest abundance are in the range of 20–150 km from the coastline (Figure 5B).
In July 2018 (G6), the largest size range for loliginid PL was registered. The PL measured from 0.88- to 4.15-mm ML (2.14 ± 0.9, n= 68) (Figure 4A) of which 65% were hatchlings (Figure 4B). The highest abundance (316 PL•1,000 m-3) was also recorded on this cruise. From these, 13 PL•1,000 m-3 were identified as Loliginidae, and 303 PL•1,000 m-3 as D. pleii (Figures 6E, F). Hatchlings representing 56% of the total abundance occurred with the highest abundance at the West zone between the isobath 20–50 m and 44 m of depth and 162 km away from the coastline (Figure 5, 6E).
D. pleii paralarvae genetic structure
All the 91 COI sequences analyzed (35 PL and 56 adults) corresponded to D. pleii, distributed in 39 haplotypes (Table S2). Based on the values calculated by Goodall-Copestake et al. (2012), there was a high haplotype diversity (Hd= 0.77) and nucleotide diversity (π = 0.002). These values varied slightly among sampling zones, with a general high haplotypic and nucleotide diversity (Table 2). The AMOVA with 1,023 permutations showed no differences among populations (Table 3), the fixation index among the three zones had a negative value (Fst= -0.0035, p= 0.67) which should be taken as zero and indicates that there is no population structuring. This is consistent with the haplotype network results, where we did not find any formed haplogroup, with the presence of a central haplotype has the highest frequency (n= 44), shared by the PL and adults from the three zones (Figure 7).

Table 3 Analysis of molecular variance of the cytochrome c oxidase subunit I region of D. pleii mtDNA, from the Yucatan Shelf, SGoM.
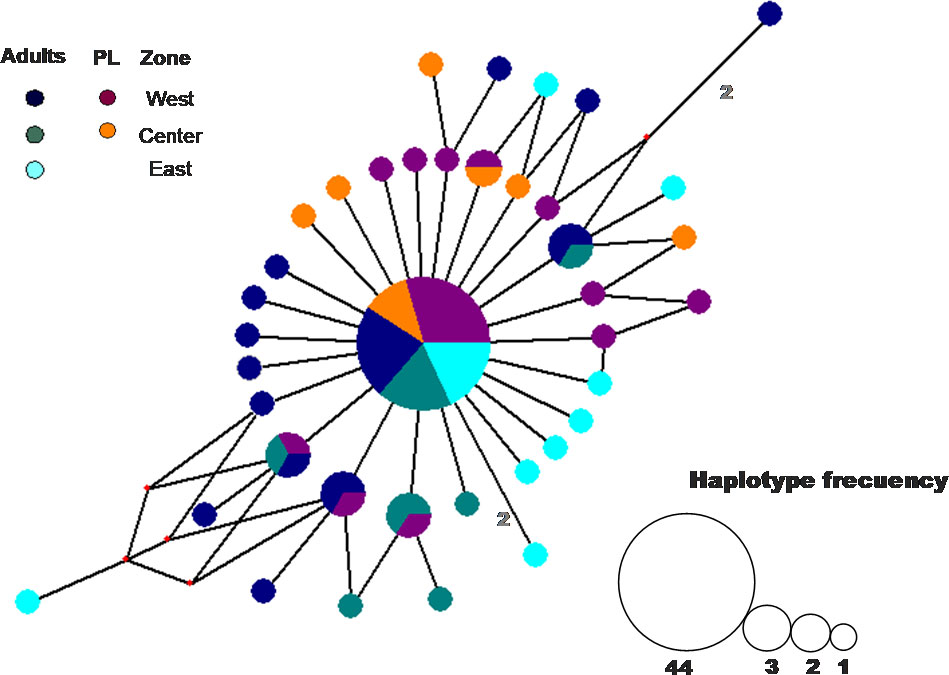
Figure 7 D. pleii haplotype network of PL and adult specimens from Southeastern Gulf of Mexico. Circles represent a unique allele. Red dots are the calculated average vectors.
Discussion
Morphological and DNA barcoding identification
In the GoM, there are five loliginid species reported (D. pealeii, D. pleii, L. brevis, D. roperi, and S. sepioidea) (Judkins et al., 2009, 2010; Jereb and Roper, 2010; Judkins et al., 2017). However, based on the morphological characteristics and molecular analysis of PL and adults reported herein, D. pleii (Blainville, 1823) was the only species of the Loliginidae family recorded during our surface sampling. In the Northwest Atlantic, D. pealeii (the most closely related species to D. pleii) migrate to shallow waters to reproduce in spring (Jereb and Roper, 2010). As this season was not sampled in our study, it is reasonable that we could not register this species. Other factors to consider in explaining the absence of the other loliginid species are the differences in PL distribution and egg size. In L. brevis, PL are most abundant near the bottom in inshore coastal waters with a salinity of approximately 26 UPS and can already tolerate very low oxygen concentrations. Sepioteuthis sepioidea (the Caribbean reef squid) has a distribution mainly limited to coral reefs, and turtle-grass flats (Thalassia testudinum), where they laid small clusters of three-to-four eggs in large, gelatinous capsules under rocks or in conch shells (Jereb and Roper, 2010). Doyteuthis roperi has been described as associated to islands (Vecchione and Young, 2010); nevertheless, Diaz-Santana-Iturrios et al. (2019) considered that this species could be synonymous to D. pleii since they are very similar morphologically and the morphometric differences are the result of intraspecific variability.
Furthermore, in the phylogenetic analysis with the new sequences from the present study, we found evidence supporting Sales et al. (2013; 2017) that D. pleii from the northwestern Atlantic and Gulf of Mexico represents a genetically distinct species from D. pleii in the southwestern Atlantic.
Abundance distribution of loliginid paralarvae according to ML
The hatching size in loliginids varies according to species (Guerra et al., 2001). The hatching size reported for D. pleii was 1.5-mm ML in a range of temperatures between 21°C and 23°C (Mc Conathy et al., 1980), being one of the loliginid species with the smallest reported hatching size. In the YS, we found PL with less than 1-mm ML size at a temperature range of 21°C–29.8°C. The smaller PL with MLs <1.0 mm representing a low proportion of the PL collected probably reflects the effect of the temperature since squid are known to display different growth strategies depending on temperature (Jackson and Forsythe, 2002; Rosa et al., 2012), with warmer temperature resulting in smaller hatchlings (Vidal et al., 2002; Villanueva et al., 2003). In addition, the small size could be affected by the shrinkage that occurred during the fixation and preservation of the PL; the use of the SCF determined by Villanueva et al. (2016) provides a better approximation of the probable size of our collected PL previous to the fixative solution application. When applying the SCF adjustment, the percentage of PL smaller than 2.5-mm ML dropped to 16%. Similarly, Martínez-Soler et al. (2021) analyzed the PL community in the mouth of the Gulf of California, Mexico, and observed an overall decrease (11%) in the percentage of PL recently hatched after applying the same SCF. However, the high proportion of specimens with sizes <2.5-mm ML in our study, suggests that hatching occurs over the north YS mainly in the Central and Western zones. Due to the limited information about the shrinkage percentage that occurred by fixation in loliginids, the SCF we used here must be considered with caution as it was estimated using five cephalopod species of families Octopodidae, Eledonidae, Megaleledonidae, and Sepiolidae (Villanueva et al., 2016), which are very different from our PL from YS.
The PL abundance (with sizes close to their species-specific hatching size) has been used as an indicator of the reproductive activity of adults (Martínez-Soler et al., 2021). In the YS, the PL abundance showed an important variability, with higher abundance in early summer (June 2018), medium at the end of summer and early autumn (August–September 2016), and low in late autumn (November 2015). This PL abundance pattern could be related to the maturity season of the species.
The maturity and lifespan of D. pleii vary by region due to environmental factors (Jackson and Forsythe, 2002; Perez et al., 2006). Mature squids have been found year-round with two seasonal peaks: spring and autumn in the Caribbean Sea (during late spring to early summer and autumn in northeastern Venezuela), and in summer and winter in the southern Brazilian waters (Perez et al., 2002; Jereb and Roper, 2010). The life span of D. pleii in the northwestern GM encompasses 6 months (Jackson and Forsythe, 2002) and an annual cycle in the southern Brazilian waters (Perez et al., 2006). In the SGoM, it has not been determined yet, but considering the temporal abundance of hatchlings of D. pleii, and using the D. pealeii egg size, spatial distribution, and hatching time between 15 and 20 days (Summers, 1983) as the closest species reference, together with the results of this study, we infer that the spawning event for D. pleii may occur during the spring season (March–May) and that the hatching may take place during the summer and early autumn (June–October) were the highest abundance took place in the Central-Western region. The East zone is mainly influenced by the Cabo Catoche upwelling and the Yucatan Current. Thus, the small number of PL (11 PL•1,000 m-3) recorded in this area suggests that hatching could be transported to the Central and West zones or contrastingly advected far from the shallow YS to the northern Gulf of Mexico, favoring the connectivity of the species.
D. pleii genetic structure
Dispersion plays a fundamental role in structuring populations (Weersing and Toonen, 2009). The biological and ecological characteristics of organisms affect their dispersal ability and the gene flow among their populations (Ibáñez and Poulin, 2014). The loliginids PL exhibit a “merobenthic” dispersal (Boletzky, 2003), which can affect their horizontal distribution along the continental shelf (Roura et al., 2016). However, they could travel hundreds of kilometers (Roberts, 2005).
The period that D. pleii remains as a planktonic PL is still unknown, but loliginids remain between 2 and 3 months as part of the plankton (García-Mayoral et al., 2020). In addition, the smaller the hatchling, the broader the latitudinal distributional range of the species (Villanueva et al., 2016). As a result of its high dispersal capacity, loliginids should keep genetic homogeneity through an increased gene flow (Weersing and Toonen, 2009; Ibáñez and Poulin, 2014; Roura et al., 2019). In the present study, we observed that D. pleii had a high haplotype and nucleotide diversity, according to the values proposed by Goodall-Copestake et al. (2012), with no population structuring. The haplotype network presented a star shape also found in this species (Sales et al., 2017) and other loliginid species (Olmos-Pérez et al., 2018; Roura et al., 2019; García-Mayoral et al., 2020) where many unique haplotypes radiate from few common haplotypes. This pattern indicates high gene flow, which suggests that there are no barriers preventing the spread of this species along and across the YS. In contrast, Sales et al. (2017) reported a subpopulation of this species in Campeche (Southern GoM), which has a low haplotypic diversity (Hd= 0.396) and does not share haplotypes with the other populations throughout the distribution of the species (North Northwestern Atlantic, Northern Gulf of Mexico, SGoM–Campeche, Southwestern Atlantic). Our study does not clarify whether the YS population shares haplotypes with the Campeche population or with the rest of the populations around, but it is consistent with the high haplotype diversity of the species and differs from the low haplotypic diversity of Campeche specimens reported for Sales J.B. de et al. (2017).
Based on our results, we encourage further surveys to explore different seasons in which the presence of other species in the area might be revealed and to relate the oceanographic influence on the PL distribution and their dispersal capacity, to explain the genetic structure of D. pleii on the YS. It is also the key to analyze the relation of the greatest upwelling pulse, which occurs mainly in the eastern region of the YS, since it is reported that loliginid PL is positively related to them (Moreno et al., 2009; Roura et al., 2016; Ruvalcaba-Aroche et al., 2018; Roura et al., 2019).
Conclusions
This study reports for the first time the species composition of PL of the family Loliginidae, their abundance distribution by size class, and the genetic structure of D. pleii on the Yucatan Shelf, SGoM. Based on morphological and molecular identification, the only species of loliginid identified was D. pleii. The highest abundance of PL reported here was found in the West and Central zones of the YS. We suggest that the spawning event of D. pleii may occur from May to September. Genetic analysis indicates that there is a high gene flow throughout the Yucatan Shelf, SGoM. This knowledge establishes the basis for a future comprehensive understanding of their biological cycle and population dynamics for the design of strategies for management and conservation.
Data availability statement
The original contributions presented in the study are included in the article/Supplementary Material. Further inquiries can be directed to the corresponding author.
Ethics statement
The approval was not necessary since the organisms sampled were obtained from the plankton samples and the adult organisms came from the commercial catches of the fishermen.
Author contributions
All authors contributed to the study conception and design. Material preparation, data collection, and analysis were performed by PG-N, RS-D, JZ-B, and MA-P. Funding acquisitions, project administration, resources, and software were performed by RR-C, AS, UO, and IV-A. The first draft of the manuscript was written by PG-N, and all authors commented on previous versions of the manuscript. All authors read and approved the final manuscript.
Funding
This research is a contribution of the Gulf of Mexico Research Consortium (CIGoM). We acknowledge PEMEX’s specific request to the Hydrocarbon Fund to address the environmental effects of oil spills in the Gulf of Mexico. The first author is grateful to CINVESTAV and CONACyT for the scholarship funding (CVU: 666902). RS-D is a fellow of the Comisión de Operación y Fomento de Actividades Académicas and Programa de Estı́mulos al Desempeño de los Investigadores (EDI) from IPN. CONACyT projects (grant numbers: 265894 and 319258).
Acknowledgments
This study is the result of a Graduate Studies doctoral degree thesis in the postgraduate program of Marine Sciences of the Centro de Investigación y de Estudios Avanzados del Instituto Politécnico Nacional (IPN)—Unidad Mérida (CINVESTAV–Mérida). All the samples and data used in this study were obtained from the Mexican National Council for Science and Technology—Mexican Ministry of Energy—Hydrocarbon Fund, project 201441”.
Conflict of interest
The authors declare that the research was conducted in the absence of any commercial or financial relationships that could be construed as a potential conflict of interest.
Publisher’s note
All claims expressed in this article are solely those of the authors and do not necessarily represent those of their affiliated organizations, or those of the publisher, the editors and the reviewers. Any product that may be evaluated in this article, or claim that may be made by its manufacturer, is not guaranteed or endorsed by the publisher.
Supplementary material
The Supplementary Material for this article can be found online at: https://www.frontiersin.org/articles/10.3389/fmars.2022.941908/full#supplementary-material
References
Allcock A. L., Lindgren A., Strugnell J. M. (2015). The contribution of molecular data to our understanding of cephalopod evolution and systematics: a review. J. Nat. Hist. 49, 1373–1421. doi: 10.1080/00222933.2013.825342
Arkhipkin A. I., Rodhouse P. G. K., Pierce G. J., Sauer W., Sakai M., Allcock L., et al. (2015). World squid fisheries. Rev. Fish. Sci. Aquac. 23, 92–252. doi: 10.1080/23308249.2015.1026226
Arreguín-Sánchez F., Arcos-Huitrón E. (2011). La pesca en méxico: estado de la explotación y uso de los ecosistemas. Hidrobiológica. 21, 431–462.
Bandelt H.-J., Forster P., Röhl A. (1999). Median-joining networks for inferring intraspecific phylogenies. Mol. Biol. Evol. 16, 37–48. doi: 10.1093/oxfordjournals.molbev.a026036
Barrientos G., García-Cubas A. (1997). Distribución y abundancia de la familia loliginidae (Mollusca: Cephalopoda) en aguas mexicanas del golfo de méxico. Rev. Soc Mex. Hist. Nat. 47, 123–139.
Boletzky S. V. (2003). Biology of early life stages in cephalopod Molluscs. Adv. Mar. Biol. 44, 143–203.
Botello R. M., Villaseñor T. R., Rodríguez M. F. (Eds) (2010). Ordenamiento de Pesquerías por Recursos Estratégicos de México, Tomo 1. Comisión Nacional de Acuacultura y Pesca (CONAPESCA). Secretaría de Agricultura, Ganadería, Desarrollo Rural, Pesca y Alimentación (México: SAGARPA), 300
Brakoniecki T. F. (1996). A revision of the genus pickfordiateuthis vos(Cephalopoda: Myopsida). Bull. Mar. Sci. 58 (1), 9–28.
Bravo-Muñoz R. A. (2020). An annotated catalogue of squids of the colección de peces e invertebrados marinos y estuarinos de la escuela nacional de ciencias biológicas (cpime-encb). Hidrobiológica 30, 281–290. doi: 10.24275/uam/izt/dcbs/hidro/2020v30n3/bravo
Bucklin A., Steinke D., Blanco-Bercial L. (2011). DNA Barcoding of marine metazoa. Ann. Rev. Mar. Sci. 3, 471–508. doi: 10.1146/annurev-marine-120308-080950
Castillo-Estrada G., De Silva-Dávila R., Carrillo L., Vásquez-Yeomans L., Silva-Segundo C. A., Avilés-Diáz L., et al. (2020). Horizontal and vertical distribution of cephalopod paralarvae in the mesoamerican barrier reef system. J. Mar. Biol. Assoc. U. K. 100, 927–937. doi: 10.1017/S0025315420000648
Ciccarelli F. D., Doerks T., von Mering C., Creevey C. J., Snel B., Bork P. (2006). Toward automatic reconstruction of a highly resolved tree of life. Science 311 (5765), 1283–1287. doi: 10.1126/science.1123061
Comisión Nacional de Pesca (CONAPESCA) (2018). Anuario estadístico de acuacultura y pesca (Gobierno de México). Available at: https://www.gob.mx/conapesca/documentos/anuario-estadistico-de-acuacultura-y-pesca.
Core Team R. (2019). R: A language and environment for statistical computing (Vienna, Austria: R Foundation for Statistical Computing). Available at: https://www.R-project.org/.
Cowen R. K., Sponaugle S. (2009). Larval dispersal and marine population connectivity. Ann. Rev. Mar. Sci. 1, 443–466. doi: 10.1146/annurev.marine.010908.163757
Darriba D., Taboada G., Doallo R., Posada D. (2012). jModelTest 2: more models, new heuristics and parallel computing. Nat. Methods 9, 772. doi: 10.1038/nmeth.2109
De Silva-Dávila R., Granados-Amores J., García-Guillén R. M., Avendaño-Ibarra R., Hernández-Rivas J., Pelayo-Martínez G. C., et al. (2018). “Riqueza de paralarvas de cefalópodos en el pacífico mexicano,” in Estudios recientes en el océano pacífico mexicano. Eds. Pérez-Morales A., Álvarez-García M. C. (México: Universidad de Colima, Colima), 249–273.
De Silva-Dávila R., Hochberg F. G., Lindgren A. R., Franco-Gordo M. C. (2013). Paralarval development, abundance, and distribution of Pterygioteuthis hoylei (Cephalopoda: Oegopsida: Pyroteuthidae) in the gulf of California, méxico. Molluscan Res. 33 (1), 50–64. doi: 10.1080/13235818.2012.754148
Díaz-Santana-Iturrios M., Salinas-Zavala C. A., Granados-Amores J., de la Cruz-Agüero J., García-Rodríguez F. J. (2019). Taxonomic considerations of squids of the family loliginidae (Cephalopoda: Myopsida) supported by morphological, morphometric, and molecular data. Mar. Biodivers. 49, 2401–2409. doi: 10.1007/s12526-019-00979-3
Diekmann R., Nellenb W., Piatkowski U. (2006). A multivariate analysis of larval fish and paralarval cephalopod assemblages at great meteor seamount. Deep-Sea Res. I 53, 1635–1657. doi: 10.1016/j.dsr.2006.08.008
Edgar R. C. (2004). MUSCLE: Multiple sequence alignment with high accuracy and high throughput. Nucleic Acids Res. 32, 1792–1797. doi: 10.1093/nar/gkh340
Enriquez C., Mariño-Tapia I., Jeronimo G., Capurro-Filograsso L. (2013). Thermohaline processes in a tropical coastal zone. Cont. Shelf. Res. 69, 101–109. doi: 10.1016/j.csr.2013.08.018
Excoffier L., Lischer H. E. L. (2015). Arlequin suite ver 3.5: A new series of programs to perform population genetics analyses under Linux and Windows. Mol. Ecol. Resour. 10, 564–567. doi: 10.1111/j.1755-0998.2010.02847.x.
Folmer O., Black M., Hoeh W., Lutz R., Vrijenhoek R. (1994). DNA Primers for amplification of mitochondrial cytochrome c oxidase subuit I from diverse metazoan invertebrates. Mol. Mar. Biol. Biotechnol. 3, 294–299. doi: 10.1071/ZO9660275
Fu L., Niu B., Zhu Z., Wu S., Li W. (2012). CD-HIT: Accelerated for clustering the next-generation sequencing data. Bioinformatics 28, 3150–3152. doi: 10.1093/bioinformatics/bts565
García-Mayoral E., Roura Á., Ramilo A., González Á.F. (2020). Spatial distribution and genetic structure of loliginid paralarvae along the Galician coast (NW Spain). Fish. Res. 222, 105406. doi: 10.1016/j.fishres.2019.105406
Goodall-Copestake W. P., Tarling G. A., Murphy E. J. (2012). On the comparison of population-level estimates of haplotype and nucleotide diversity: A case study using the gene cox1 in animals. Heredity 109, 50–56. doi: 10.1038/hdy.2012.12
Goto T. (2005). Examination of different preservatives for Todarodes pacificus paralarvae fixed with borax-buffered formalin-seawater solution. Phuket. Mar. Biol. Cent. Res. Bull. 66, 213–219.
Granados-Amores J., Hochberg F. G., Salinas-Zavala C. A. (2013). New records of Lolliguncula (Lolliguncula) argus brakoniecki & rope(Myopsida: Loliginidae) in northwestern Mexico. Lat. Am. J. Aquat. Res. 41 (3), 595–599. doi: 10.3856/vol41-issue3-fulltext-22
Guerra A., Rocha F., González Á.F. (2001). Embryonic stages of the Patagonian squid Loligo gahi (Mollusca: Cephalopoda). Veliger. 44 (2), 1O9–115.
Hanlon R. T., Boletzky S. V., Okutani T., Pérez-Gandaras G., Sanchez P., Sousa-Reis C., et al. (1992). “Myopsida,” in Larval’ and juvenile cephalopods: A manual for their identification. Eds. Sweeney M. J., Roper C. F. E., Mangold K. M., Clarke M., Boletzky S. V. (Washington, D.C.: Smithsonian Institution Press), 37–55.
Hebert P. D. N., Cywinska A., Ball S. L., deWaard J. R. (2003). Biological identifications through DNA barcodes. Proc. R. Soc Lond. B. 270, 313–322. doi: 10.1098/rspb.2002.2218
Hellberg M. E. (2009). Gene flow and isolation among populations of marine animals. Annu. Rev. Ecol. Evol. Syst. 40, 291–310. doi: 10.1146/annurev.ecolsys.110308.120223
Ibáñez C. M., Poulin E. (2014). Genetic structure and diversity of squids with contrasting life histories in the Humboldt current system. Hidrobiologica 24, 1–10.
Jackson G. D., Forsythe J. W. (2002). Statolith age validation and growth of Loligo plei (Cephalopoda: Loliginidae) in the north-west gulf of Mexico during spring/summer. J. Mar. Biol. Ass. U. K. 82, 677–678. doi: 10.1017/S0025315402006069
Jereb P., Roper C. F. E. (2010). “Cephalopods of the world: An annotated and illustrated catalog of cephalopod species known to date,” in Myopsid and oegopsid squids, vol. Volume 2. (Rome: FAO Species Catalogue for Fishery Purposes, N° 4).
Judkins H., Vecchione M., Cook A., Sutton T. (2017). Diversity of midwater cephalopods in the northern gulf of Mexico: comparison of two collecting methods. Mar. Biodivers. 47, 647–657. doi: 10.1007/s12526-016-0597-8
Judkins H. L., Vecchione M., Roper C. F. E. (2009). “Cephalopoda (Mollusca) of the gulf of Mexico,” in Gulf of Mexico–origins, waters, and biota. biodiversity. Eds. Felder D. L., Camp D. K. (Texas: Texas A&M University Press), 701–709.
Judkins H. L., Vecchione M., Roper C. F. E., Torres J. (2010). Cephalopod species richness in the wider Caribbean region. ICES J. Mar. Sci. 67, 1392–1400. doi: 10.1093/icesjms/fsq092
Kim E.-B., Lee S. R., Lee C. I., Park H., Kim H.-W. (2019). Development of the cephalopod-specific universal primer set and its application for the metabarcoding analysis of planktonic cephalopods in Korean waters. PeerJ 7, e7140. doi: 10.7717/peerj.7140
Kumar S., Stecher G., Tamura K. (2016). MEGA7: Molecular evolutionary genetics analysis version 7.0 for bigger datasets. Mol. Biol. Evol. 33, 1870–1874. doi: 10.1093/molbev/msw054
Lara-Lara J. R., Arreola-Lizárraga J. A., Calderon-Aguilera L. E., Camacho-Ibar V. F., de la Lanza-Espino G., Escofet-Giansone A., et al. (2008). Los Ecosistemas costeros, insulares y epicontinentales. Cap. Nat. México. I, 109–134. doi: 10.4067/S0717-66432002000200006
Librado P., Rozas J. (2009). DnaSP v5: A software for comprehensive analysis of DNA polymorphism data. Bioinformatics 25, 1451–1452. doi: 10.1093/bioinformatics/btp187
Martínez-Soler E., Gómez-Gutiérrez J., De Silva-Dávila R., González-Rodríguez E., Aburto-Operaza O. (2021). Cephalopod paralarval species richness, abundance and size structure during the 2014–2017 anomalous warm period in the southern gulf of California, J. Plankton. Res. 43 (2), 224–243. doi: 10.1093/plankt/fbab010
McConathy D. A., Hanlon R. T., Hixon R. F. (1980). Chromatophore arrangements of hatchling loliginid squids (Cephalopoda, myopsida). Malacologia 19 (2), 279–288.
Migkuavacca P. P., Simone L. R. (2020). Morphological comparison between Doryteuthis pleii and D. sanpaulensis (Cephalopoda, myopsida, loliginidae) from Brazil. Pap. Avulsos. Zool. 60, e20206001. doi: 10.11606/1807-0205/2020.60.01
Moreno A., Dos Santos A., Piatkowski U., Santos A. M. P., Cabral H. (2009). Distribution of cephalopod paralarvae in relation to the regional oceanography of the western Iberia. J. Plankton. Res. 31, 73–91. doi: 10.1093/plankt/fbn103
Niu B., Fu L., Sun S., Li W. (2010). Artificial and natural duplicates in pyrosequencing reads of metagenomic data. BMC Bioinf. 11, 87. doi: 10.1186/1471-2105-11-187
Olmos-Pérez L., Roura Á., Pierce G. J., González Á.F. (2018). Sepiolid paralarval diversity in a regional upwelling area of the NE Atlantic. Hydrobiologia 808, 57–70. doi: 10.1007/s10750-017-3186-3
Perez J. A. A., Cordella de Aguiar D., Cavalcante Oliveira U. (2002). Biology and population dynamics of the long-finned squid Loligo plei (Cephalopoda: Loliginidae) in southern Brazilian waters. Fish. Res. 58, 267–279. doi: 10.1016/S0165-7836(01)00397-6
Perez J. A. A., Cordella de Aguiar D., Teixeira dos Santos J. A. (2006). Gladius and statolith as tools for age and growth studies of the squid Loligo plei (Teuthida: Loliginidae) off southern Brazil. Braz. Arch. Biol. Technol. 49, 747–755. doi: 10.1590/S1516-89132006000600009
Roberts M. J. (2005). Chokka squid (Loligo vulgaris reynaudii) abundance linked to changes in south africa’s agulhas bank ecosystem during spawning and the early life cycle. ICES J. Mar. Sci. 62, 33–55. doi: 10.1016/j.icesjms.2004.10.002
Rodhouse P. (2005). “World squid resources,” in Review of the state of world marine fishery resources, ed. fishery and aquaculture economics and policy division (FAO Fisheries Technical Paper), 175–187.
Ronquist F., Teslenko M., van der Mark P., Ayres D. L., Darling A., Höhna S., et al. (2012). MrBayes 3.2: efficient Bayesian phylogenetic inference and model choice across a large model space. Syst. Biol. 61 (3), 539–542. doi: 10.1093/sysbio/sys029
Rosa R., Gonzales L., Dierssen H. M., Seibel B. A. (2012). Environmental determinants of latitudinal size-trends in cephalopods. Mar. Ecol. Prog. Ser. 464, 153–165. doi: 10.3354/meps09822
Roura Á., Amor M., González Á.F., Guerra Á., Barton E. D., Strugnell J. M. (2019). Oceanographic processes shape genetic signatures of planktonic cephalopod paralarvae in two upwelling regions. Prog. Oceanogr. 170, 11–27. doi: 10.1016/j.pocean.2018.10.005
Roura Á., Antón Álvarez-Salgado X., González Á.F., Gregori M., Rosón G., Otero J., et al. (2016). Life strategies of cephalopod paralarvae in a coastal upwelling system (NW Iberian peninsula): Insights from zooplankton community and spatio-temporal analyses. Fish. Oceanogr. 25 (3), 241–258. doi: 10.1111/fog.12151
Ruvalcaba-Aroche E. D., Sánchez-Velasco L., Beier E., Godínez V. M., Barton E. D., Pacheco M. R. (2018). Effects of mesoscale structures on the distribution of cephalopod paralarvae in the gulf of California and adjacent pacific. Deep. Res. Part I Oceanogr. Res. Pap. 131, 62–74. doi: 10.1016/j.dsr.2017.11.005
Sales J. B. de L., Rodrigues-Filho L. F., da S., Ferreira Y., do S., Carneiro J., et al. (2017). Divergence of cryptic species of Doryteuthis plei blainvill (Loliginidae, Cephalopoda) in the Western Atlantic ocean is associated with the formation of the Caribbean Sea. Mol. Phylogenet. Evol. 106, 44–54. doi: 10.1016/j.ympev.2016.09.014
Sales J. B., de L., Shaw P. W., Haimovici M., Markaida U., Cunha D. B., et al. (2013). New molecular phylogeny of the squids of the family loliginidae with emphasis on the genus Doryteuthis Naef, 1912: Mitochondrial and nuclear sequences indicate the presence of cryptic species in the southern Atlantic ocean. Mol. Phylogenet. Evol. 68, 293–299. doi: 10.1016/j.ympev.2013.03.027
Sameoto D., Wiebe P., Runge J., Postel L., Dunn J., Miller C., et al. (2000). “Collecting zooplankton,” in In ICES zooplankton methodology manual. Eds. Harris R., Wiebe P., Lenz J., Skjoldal H. R., Huntley M. (London: Academic Press), 55–81.
Sanger F., Nicklen S., Coulson R. A. (1977). DNA Sequencing with chain-terminating inhibitors. Proc. Nati. Acad. Sci. U.S.A. 74 (12), 5463–5467. doi: 10.1073/pnas.74.12.5463
Santana-Cisneros M. L., Rodríguez-Canul R., Zamora-Briseño J. A., Améndola-Pimenta M., De Silva-Dávila R., Ordóñez-López U., et al. (2021). Morphological and molecular identification of octopoda (Mollusca: Cephalopoda) paralarvae from the southern gulf of Mexico. Bull. Mar. Sci. 97, 281–304. doi: 10.5343/BMS.2020.0027
Smith P. E., Richardson S. (1977). Standard techniques for pelagic fish egg and larva surveys. FAO Fish. Tech. Pap. 175, 112.
Solís-Ramírez M. J., Garduño M., Espinoza J. C., Ramírez F. G. (1998). ¿Pesquería de calamares en la plataforma continental de yucatán? GCFI 50, 262–276.
Summers W. C. (1983). “Loligo pealeii,” in Cephalopod life cycles. Ed. Boyle P. R. (Michigan: Academic Press), 115–142.
Sweeney M. J., Roper C. F. E., Mangold K. M., Clarke M., Boletzky S. V. (1992). ‘Larval’ and juvenile cephalopods: A manual for their identification (Washington, D.C: Smithsonian Contributions to Zoology).
Vecchione M., Roper C. F. E., Sweeney M. J., Lu C. C. (2001). Distribution, relative abundance and developmental morphology of paralarval cephalopods in the Western north Atlantic Ocean (Seattle, Washington: NOAA Technical Report NMFS), 152.
Vecchione M., Shea E., Bussarawit S., Anderson F., Alexeyev D., Lu C.-C., et al. (2005). Systematics of indo-West pacific loliginids. Phuket. Mar. Biol. Cent. Res. Bull. 66, 23–26.
Vecchione M., Young R. E. (2010) Doryteuthis (Doryteuthis) roperi (Cohen 1976). island inshore squid. version 02. Available at: http://tolweb.org/Cephalopoda/Doryteuthis_%28Doryteuthis%29_roperi/52729/201.
Vidal A. G., DiMarco F. P., Wormuth J. H., Lee P. G. (2002). Influence of temperature and food availability on survival, growth and yolk utilization in hatching squid. Bull. Mar. Sci. 71 (2), 915–931.
Villanueva R., Arkhipkin A., Jereb P., Lefkaditou E., Lipinski M. R., Perales-Raya C., et al. (2003). Embryonic life of the loliginid squid Loligo vulgaris: comparison between statoliths of Atlantic and Mediterranean populations. Mar. Ecol. Prog. Ser. 253, 197–208. doi: 10.3354/meps253197
Villanueva R., Vidal E. A. G., Fernández-Álvarez F.Á., Nabhitabhata J. (2016). Early mode of life and hatchling size in cephalopod molluscs: Influence on the species distributional ranges. PloS One 11 (11), e0165334. doi: 10.1371/journal.pone.0165334
Ward R. D. (2000). Genetics in fisheries management. Hydrobiologia 420, 191–201. doi: 10.1023/A:1003928327503
Weersing K., Toonen R. J. (2009). Population genetics, larval dispersal, and connectivity in marine systems. Mar. Ecol. Prog. Ser. 393, 1–12. doi: 10.3354/meps08287
Yatsu A., Tafur R., Maravi C. (1999). Embryos and rhynchoteuthion paralarvae of the jumbo flying squid Dosidicus gigas (Cephalopoda) obtained through artificial fertilization from Peruvian waters. Fisheries. Sci. 65 (6), 904–908. doi: 10.2331/fishsci.65.904
Young R. E., Harmnan R. F. (1988). “Larva”, “Paralarva ‘‘ and “Subadult ‘‘ in the cephalopod terminology. Malacología 29 (1), 201–207.
Young R. E., Vecchione M., Mangold K. M. (2019) Cephalopoda Cuvier 1797. octopods, squids, nautiluses, etc. Available at: http://tolweb.org/Cephalopoda/19386/2019.03.26http://tolweb.org/.
Keywords: squids, Doryteuthis pleii, hatchlings, DNA barcoding, Yucatan Shelf
Citation: Guarneros-Narváez PV, Rodríguez-Canul R, De Silva-Dávila R, Zamora-Briseño JA, Améndola-Pimenta M, Souza AJ, Ordoñez U and Velázquez-Abunader I (2022) Loliginid paralarvae from the Southeastern Gulf of Mexico: Abundance, distribution, and genetic structure. Front. Mar. Sci. 9:941908. doi: 10.3389/fmars.2022.941908
Received: 12 May 2022; Accepted: 18 July 2022;
Published: 10 August 2022.
Edited by:
Roger Villanueva, Institute of Marine Sciences (CSIC), SpainReviewed by:
Joao Braullio Luna Sales, Federal University of Pará, BrazilRodrigo Silvestre Martins, Federal University of São Paulo, Brazil
Heather Judkins, University of South Florida St. Petersburg, United States
Copyright © 2022 Guarneros-Narváez, Rodríguez-Canul, De Silva-Dávila, Zamora-Briseño, Améndola-Pimenta, Souza, Ordoñez and Velázquez-Abunader. This is an open-access article distributed under the terms of the Creative Commons Attribution License (CC BY). The use, distribution or reproduction in other forums is permitted, provided the original author(s) and the copyright owner(s) are credited and that the original publication in this journal is cited, in accordance with accepted academic practice. No use, distribution or reproduction is permitted which does not comply with these terms.
*Correspondence: Iván Velázquez-Abunader, jvelazquez@cinvestav.mx