- 1State Key Laboratory of Fresh water Ecology and Biotechnology, Institute of Hydrobiology, Chinese Academy of Sciences, Wuhan, China
- 2College of Advanced Agricultural Sciences, University of Chinese Academy of Sciences, Beijing, China
- 3Hubei Engineering Research Center for Aquatic Animal Nutrition and Feed, Wuhan, China
- 4The Innovative Academy of Seed Design, Chinese Academy of Sciences, Wuhan, China
Inosine monophosphate (IMP) improves the flesh quality of livestock and poultry, but studies about its effect on fishes are limited. The aim of the present study was to investigate the effect of exogenous IMP on the liver health and flesh quality of gibel carp (Carassius auratus gibelio). The results showed that IMP supplementation alleviated hepatic lipid deposition by increasing the AMP/ATP ratio and activating the AMPK signaling pathway then decreased inflammatory cytokine expression levels. Additionally, IMP increased the intramuscular fat content. Metabolomic analysis of meat revealed that the IMP, adenosine monophosphate (AMP), guanosine, inosine and purine metabolism pathways were involved in the improvements in fish meat quality, composition and taste that resulted from IMP supplementation. Our study provides a relatively comprehensive assessment of the effect of exogenous IMP on the liver metabolism and flesh quality of farmed fish. The results provide an effective food supplementation strategy for cultured fish.
Introduction
Liver health has become a major health concern in both humans and cultivated animals (Jiang et al., 2020; Huang et al., 2020). Due to the high price and unsustainability of fishmeal, high-energy formulations are commonly fed to farmed fish in order to save costs in aquaculture. However, the long-term consumption of high-energy feed can result in the accumulation of excessive fat deposits in the liver tissues of farmed fish and cause various liver diseases (Yan et al., 2015). Currently, the problem of fat accumulation in the liver during aquaculture has been reported in many fish species, including grass carp (Ctenopharyngodon idella) and Nile tilapia (Oreochromis niloticus) (Wang et al., 2019; Yu et al., 2021). Excessive fat deposition leads to clinical symptoms, such as reduced growth performance and feed utilization, decreased disease and environmental stress resistance and reduced meat quality. These factors have caused substantial losses in the aquaculture industry. Therefore, addressing excessive fat deposition in fish and exploring the underlying regulatory mechanisms have become topics of interest in research.
The synthesis and catabolism of adenosine triphosphate (ATP) are dynamic processes that maintain intracellular homeostasis in organisms. Adenosine monophosphate-activated protein kinase (AMPK) senses changes in energy via changes in the adenosine 5′-monophosphate (AMP)/ATP or adenosine diphosphate (ADP)/ATP ratio and then regulates energy homeostasis (Hardie et al., 2012). When excess free AMP or ADP binds to the γ subunit of AMPK, leading to rearrangement of the allosteric domain, a site on the α subunit of AMPK is phosphorylated, and thus, AMPK is activated (Ke et al., 2018). Exercise, starvation, hypoxia and other factors have long been known to activate AMPK. When AMPK is activated, the sensitivity of tissues to fatty acid oxidation and glucose uptake is enhanced, fatty acid β oxidation and glycolysis are promoted, lipid synthesis and gluconeogenesis are inhibited, and more ATP is released to support cellular functions (Bao et al., 2020). Some natural products have been reported to activate AMPK directly or indirectly. Babaodan, a traditional Chinese medicine, alleviates high-fat diet-induced nonalcoholic fatty liver disease through activation of AMPK signalling (Sheng et al., 2019). Capsiate improves the metabolism of lipids and glucose via activation of the AMPK/SIRT1 signalling pathway and exerts anti-obesity effects in animals and humans (Zang et al., 2018). In this respect, the exploration of AMPK activators in natural products is a sensible strategy for treating metabolic diseases related to excessive fat accumulation.
Inosine monophosphate (IMP) is a purine nucleotide that is extensively involved in cellular composition, energy supply, metabolism and other important biological processes (Naik et al., 2018; Pareek et al., 2020). A previous study confirmed that IMP has flavour characteristics and has been widely used as an umami agent (Sako et al., 2003). Currently, the effects of IMP supplementation in the diets of farmed animals have been widely reported, and these effects include enhanced growth (Hossain et al., 2016), improved innate immunity and increased disease resistance (Lin et al., 2009; Song et al., 2012). Interestingly, previous studies found that the administration of inosine could regulate ATP metabolism and prevent ethionine-induced fatty liver in rats (Farber et al., 1965). Hepatic lipid accumulation is also caused by dietary nucleotide restriction and can be reversed by dietary nucleotide or adenosine monophosphate supplementation (Carver, 1994). IMP is at the centre of purine nucleotide metabolism. However, a comprehensive and integrated evaluation of the potential effects of exogenous IMP on the liver health and muscle quality of farmed animals is lacking.
Gibel carp (Carassius auratus gibelio) is a very popular freshwater fish species that is known for its high yield, fast growth, and strong resistance, and it is widely cultivated in China (Gui and Zhou, 2010). According to recent statistical analyses, the production of gibel carp reached 2 million tons in China in 2020 (Fisheries Bureau, Department of Agriculture of China, 2020). Currently, there are a few researches of IMP on the aquatic animals. Those studies have focused on the impacts of IMP on immunity, gut health and growth performance in farmed species. To the best of our knowledge, this is the first report on the effects of IMP on liver health and muscle quality in farmed fish. Therefore, this study comprehensively evaluates the potential regulatory mechanisms by exogenous IMP affects the hepatic health and flesh quality of gibel carp, to provide theoretical support for improving the flesh quality and liver health of farmed fish.
Materials and methods
All the experimental animal care protocols were approved by the ethics committee of the Institute of Hydrobiology of Chinese Academy of Sciences.
Experimental diets
Three isonitrogenous (41% crude protein), isolipidic (11% crude lipid) and isoenergetic (19 MJ kg-1 gross energy) diets were formulated with three concentrations (0%, 0.2% and 0.4%) of exogenous IMP by Excel (Office 2016, Microsoft Corporation, USA). The formulations and chemical compositions of the diets are shown in Table S1. Casein was used as the main protein source in the diets, corn starch was used as the carbohydrate source, and equal proportions of fish oil and soybean oil were combined and used as the fat source. The exogenous nonessential amino acid glycine was added to normalize and maintain its total amount in the diet at a constant level. The addition of the limiting amino acid arginine was used to maintain the balance of amino acids in the diet. First of all, all ingredients passed through a 375 μm sieve. The crystalline IMP was mixed with equal amounts of corn starch. The mixture was in turn mixed with equal amounts of corn starch in a gradient. After the mixture containing the IMP had been mixed well with other ingredients, the oil was added to the mixture. Mixed the mixture with oil and re-sieved. After sieving, water was added into mixture and mixed well. A laboratory single screw pelleting mill (SLR-150; Fishery Machinery and Instrument Research Institute, Chinese Academy of Fishery Science, Shanghai, China) was used to make the pellets. Firstly, the speed of the screw was set to 25 Hz. The mixture was added slowly and uniformly into feed opening. The screw propels the mixture forward in a spiral. The mixture is pelleted by the mould into long strips with a diameter of 3.6mm, which are cut into pellets by the cutter. The pellets were dried in an oven at 60°C and then stored at 4°C.
Fish and feeding trial
Juvenile gibel carp were obtained from the Guanqiao hatchery of the Institute of Hydrobiology, Chinese Academy of Sciences (Wuhan, Hubei, China). The experimental fish were reared in fibreglass tanks (water volume of 883 L; diameter of 150 cm; and water depth of 50 cm) and allowed to adapt to the laboratory conditions. All the fish were fed commercial feed without exogenous IMP supplementation twice a day for four weeks before the formal feeding trials began. After acclimatization, the fish were fasted for 24 h. Then, fish that were apparently healthy and of similar size (mean initial weight of 8.03 ± 0.05 g) were randomly divided into 9 fibreglass tanks. Each diet was randomly assigned to be fed to the fish in triplicate tanks. During the feeding trial, the fish were fed their corresponding diets to apparent satiation three times per day (8:30, 14:00 and 18:00) for 60 days. During the experiment, the water temperature was recorded daily and maintained at 30.05 ± 1.60°C. The dissolved oxygen content was maintained above 7.5 mg L-1, and the ammonia nitrogen content was lower than 0.5 mg L-1. The photoperiod was 12-h light:12-h dark with a light period from 8:00 to 20:00.
Sample collection
At the start of the trial, triplicate groups of fish were randomly sampled and stored at -20°C for initial body composition analysis. At the end of the feeding trial, all the fish from each tank were weighed after fasting for 24 h. Two fish per tank were randomly selected and stored at -20°C for final body proximate composition analysis. Another two fish were anaesthetized with MS-222 (100 mg L−1 tricaine methane sulfonate, Argent Chemical Laboratories Inc., Redmond, WA, USA). Blood samples were collected via the caudal vein using 2-ml heparinized injectors and then centrifuged at 3500 rpm for 10 min. Plasma samples were stored at -80°C for further analysis. After blood sampling, liver and dorsal muscle samples were harvested from the fish mentioned above. These samples were kept on ice and then stored at -80°C for further analysis.
Biochemical assays
The experimental fish and diet samples were analysed following the method described by the Association of Official Analytical Chemists (AOAC, 2005). The sample moisture content was determined by oven drying at 105°C to a constant weight. The ash content was quantified by incineration in a muffle furnace at 550°C for 12 h. A Kjeltec Analyzer Unit (FOSS Tecator 8400, Haganas, Sweden) was used to investigate the crude protein content. The crude lipid content was measured by a Soxtec system (Soxtec™ 2055, FOSS Tecator, Haganas, Sweden). The total triglyceride (TG), total cholesterol (TC), low-density lipoprotein cholesterol (LDL-C), high-density lipoprotein cholesterol (HDL-C), aspartate aminotransferase (AST) and alanine aminotransferase (ALT) contents were determined by an automatic biochemical analyser (Mindray BS-460, Shenzhen, China) using standard kits according to the manufacturer’s instructions (TG (P/N:105-000449-00), TC (P/N:105-000448-00), LDL-C (P/N:105-000464-00), HDL-C (P/N:105-000463-00), AST (P/N:105-000443-00), and ALT (P/N:105-000442-00)). The glycogen contents in the liver were measured with commercial assay kits (A043-1-1, Nanjing Jiancheng Bioengineering Institute, Nanjing, Jiangsu, China). The total fat contents of the liver and muscle were determined by the trichloromethane-methanol method, and the procedure based on that described by Wang et al. (1993) with minor modifications.
Real-time quantitative polymerase chain reaction analysis
The primers used for qPCR were designed using the National Center for Biotechnology Information (NCBI) primer BLAST service or following the sequences described by Jin et al. (2017) (Table S5). Total RNA was extracted from liver and dorsal muscle samples with TRIzol Reagent (Invitrogen, Carlsbad, CA, USA) according to the manufacturer’s recommendations. The quantity and purity of the total RNA were evaluated by a NanoDrop® ND-2000 UV–Vis Spectrophotometer (NanoDrop Technologies, Wilmington, DE, USA). In addition, a 1% denaturing agarose gel was used to test the integrity of the RNA samples. Then, the isolated RNA was reverse transcribed into cDNA using an M-MLV First-Strand Synthesis Kit (Invitrogen, Shanghai, China) according to the manufacturer’s protocol. Quantitative RT-PCR was performed using a LightCycle 480 II system (Roche, Basel, Switzerland). Three biological replicates were established for each sample (n = 6). β-actin and ef1α were selected as the internal reference genes to assess the transcription levels in the liver and dorsal muscle samples. The relative expression levels of the target genes were determined with the mathematical model described by Vandesompele et al. (2002).
Histological analysis
The liver tissues from the fish subjected to different experimental treatments were examined using oil red staining, and this staining was performed by Servicebio Company (Wuhan, China). Thus, the deposition of fat in the liver tissues was visualized. After observation under a light microscope, the liver fat contents were quantified by calculating the percentage of the area of oil red-stained dots with ImageJ software. To observe the pathological changes in the liver, liver specimens were embedded in paraffin wax and sectioned to a thickness of 4 μm. The sections were stained with haematoxylin-eosin (H&E) to observe the histopathological changes in the liver.
Western blotting analysis
Total hepatic proteins were extracted with protein extraction buffer (Thermo Scientific, Massachusetts, United States of America) according to the manufacturer’s instructions. Equal amounts of protein (20 μg of protein from liver samples) were subjected to SDS–PAGE and then transferred to PVDF membranes. The PVDF membranes were blocked with 5% milk in TBST and then incubated with antibodies against glyceraldehyde-3-phosphate dehydrogenase (GAPDH) (ab70699, Abcam) and phosphorylated AMPK (2535, CST). The antibodies were diluted to 1:1000. Horseradish peroxidase (HRP)-labelled secondary antibodies were used to produce a chemiluminescent signal, which was detected by a CCD camera-based imager (Chemidoc MP Imaging System, Bio–Rad, California, United States of America). GAPDH served as the control. All the processes were repeated three times.
Determination of ATP, AMP and IMP concentrations
The concentrations of nucleotides and related compounds in the diet, liver and muscle samples were determined according to the high-performance liquid chromatography (HPLC, Waters 2695e, USA) procedure described by Cheng et al. (2015) with some modifications. Five grams of minced fish liver was homogenized for 15 min with 10 ml of 0.1 M perchloric acid at 4°C. Then, the homogenate was centrifuged for 10 min with a revolving speed of 4400 rpm at 4°C. Five millilitres of 0.1 M perchloric acid was added to the residues, and the sample was centrifuged again for 5 min. The supernatant was then decanted and neutralized with 1 M potassium hydroxide solution to a pH of approximately 6.4. After incubation at 4°C for 10 min, the precipitated potassium perchlorate was removed by filtration. The filtrate was diluted to 50 ml. Then, part of the solution was passed through a 0.45-μm Millipore syringe filter. A 20-μl aliquot of the filtrate was harvested from the sample extract and injected into the HPLC system. A 5-μm C18 column (CNW Athena C18-WP, 4.6*250 mm, 5 μm) was used to separate ATP, AMP and IMP from the sample. Mobile phase A was 0.01mol L-1 potassium (KH2PO4). The pH was adjusted to 6.10 with 0.1 mol L-1 dipotassium hydrogen phosphate (K2HPO4). Mobile phase B was methanol, and the ratio of mobile phases A and B was 98:2. A flow rate of 1.0 ml min-1 and a constant column temperature of 30°C were used, and the elution time was 20 min. The UV detector wavelength was 254 nm and HPLC eluent compositions and program in Table S3.
Metabolomics analysis of muscle samples
The sample was thawed on ice. Fifty milligrams of one sample were homogenized with 1000 µl of ice-cold methanol/water (70%, v/v). Cold steel balls were added to the mixture, and the sample was homogenized at 30 Hz for 3 min. The mixture was stirred for 1 min and then centrifuged at 12,000 rpm at 4°C for 10 min. The collected supernatant was used for ultrahigh-performance liquid chromatography coupled with high-resolution mass spectrometry (LC-MS/MS) analysis. All data was processed and normalized by MetaboAnalyst 5.0 and then to analyse the differences in the levels of metabolites in the gibel carp dorsal muscles between the control group and the 0.4% IMP group (Pang et al., 2021).
Statistical analysis
SPSS (IBM SPSS Statistics 20.0, IBM, USA) was used to analyse all the data from the groups subjected to different treatments. First, normality of distribution (one-sample Kolmogorov–Smirnov test) and homogeneity of variance (Levene’s test) analyses were conducted to check all the values, and then, the significance of the differences among the treatment groups was analysed with one-way analysis of variance (ANOVA). Meanwhile, linear regression was carried out to analysed the responses of growth performance, whole body composition, metabolite contents and relative genes expression. All the values are presented as the mean ± standard error (n = 6; SE), and differences were considered statistically significant at P < 0.05.
Results
Effects of IMP supplementation on the growth and chemical composition of gibel carp
We conducted a 60-day breeding trial in order to evaluate the effects of exogenous IMP on liver health and flesh quality of gibel carp. There was no significant difference in the weight gain, specific growth rate and feeding rate among the experimental groups (P > 0.05) (Table S2). In terms of body composition, the fish in the 0.2% and 0.4% groups had higher whole-body protein contents than the fish in the control group (Table S2), but the whole-body moisture, lipid contents and ash content of the gibel carp was not significantly different among all the groups (P > 0.05) (Table S2).
Effects of IMP on hepatic lipid deposition and liver health of gibel carp
We observed obvious fat deposition and pathological damage in the livers of gibel carp after the feeding experiment (Figures 1A–C). To examine whether IMP supplementation influenced liver health, a number of haematological indicators that reflect liver function were investigated. Significant decreases in the plasma TG and TC levels were observed in the 0.4% IMP group compared to the 0% IMP group (P < 0.05) (Figures 1D, E). As demonstrated in Figures 1F, G, the serum levels of HDL-C and LDL-C were also significantly decreased in the 0.2% IMP and 0.4% IMP groups (P < 0.05). Additionally, the plasma levels of ALT and AST, important indicators of liver function, were significantly reduced in the 0.4% IMP group (P < 0.05) (Figures 1H, I). IMP supplementation (0.4%) significantly decreased the ratio of liver weight to body weight (P < 0.05) (Figure 1J). We also measured the expression levels of the inflammatory factors TGF-β, IL-6, TNF-α, and IL-1β in the liver, and the results are shown in Figure 1K. Compared with those in the 0% IMP group, the TGF-β, IL-6 and IL-1β expression levels were dramatically decreased in the 0.4 IMP group (P < 0.05). Thus, IMP reduced hepatic lipid droplet deposition and ameliorated hepatocyte quality (Figures 1A–C).
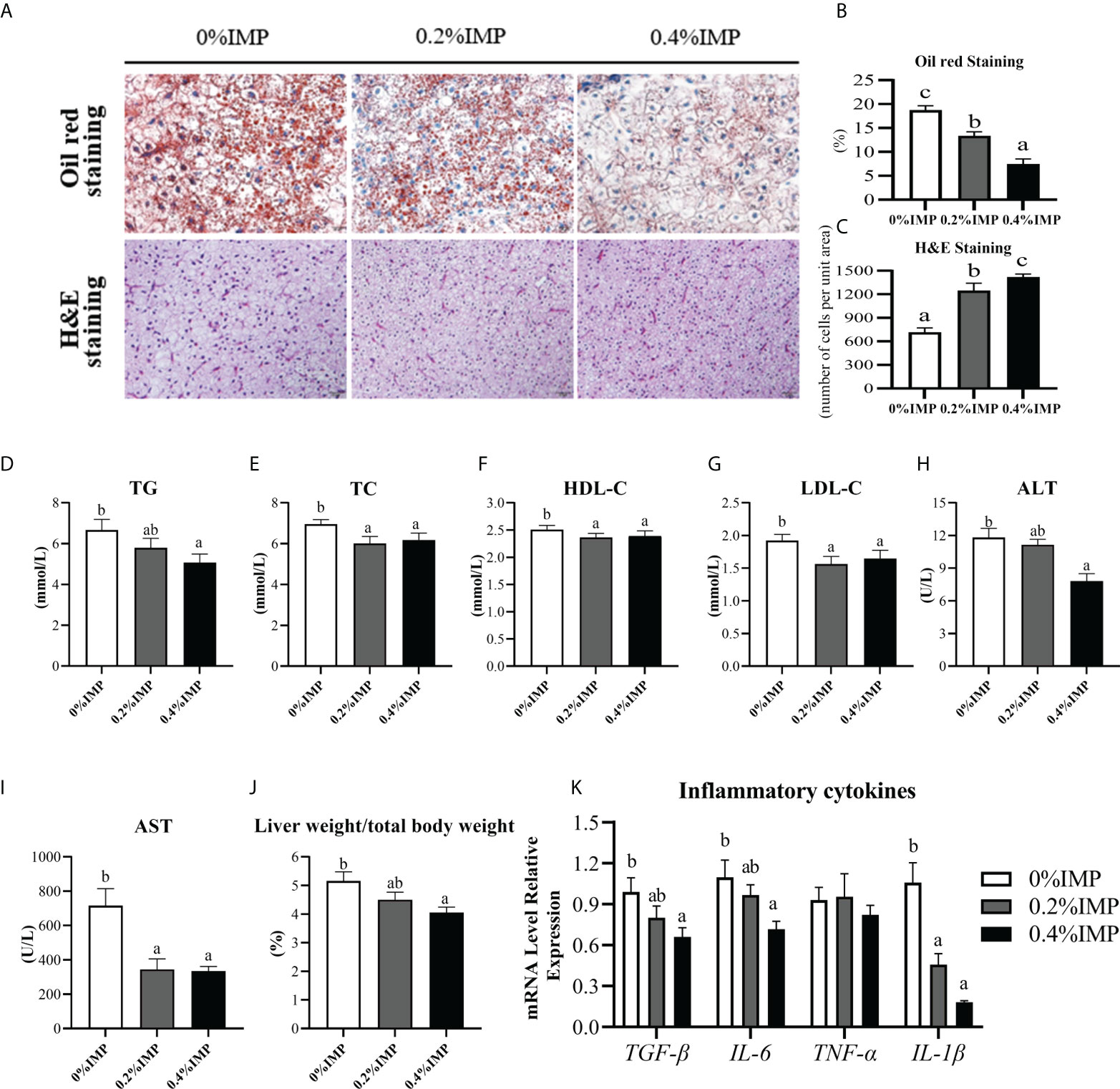
Figure 1 Dietary IMP supplementation could alleviate lipid deposition and improve the liver health of gibel carp. (A) Histological observations of the liver, stained with oil red (magnification 300×) and H&E (magnification 300×). (B) The quantitative graph of oil red staining. (C) The quantitative graph of H&E staining. (D) Total triglyceride (TG) level in serum of different groups. (E) Total cholesterol (TC) level in serum of different groups. (F) The level of high-density lipoprotein cholesterol (HDL-C) in the serum of different groups. (G) The level of low-density lipoprotein cholesterol (LDL-C) in the serum of different groups. (H) The content of alanine aminotransferase (ALT) in the serum of different groups. (I) The content of aspartate aminotransferase (AST) in the serum of different groups. (J) Ration of liver weight to body weight of different groups. (K) The hepatic expression of TGF-β, IL-6, TNF-α, IL-1β. Columns represent the mean ± SEM (n=6). For each index, bars without sharing a common letter indicate significant differences (P <.05).
Effect of IMP supplementation on hepatic glycolipid metabolism in gibel carp
We evaluated lipid and glucose metabolism in the livers of gibel carp. The hepatic fat contents and glycogen contents showed similar trends and were significantly lower in the 0.4% IMP group than in the 0% IMP group (P < 0.05) (Figures 2A, B). Additionally, we examined the expression of key genes involved in lipogenesis (acc, srebp1 and fas), lipolysis (hsl), fatty acid β-oxidation (cpt1α and aco3) and glucose metabolism (pepck, g6pase, gk, glut4, pfk and pk) in the liver. The expression of acc and fas was significantly lower in the 0.4% IMP-treated fish (P < 0.05) (Figure 2C). However, the expression of cpt1α and hsl, key genes involved in lipolysis, was upregulated in the 0.4% IMP group (Figure 2D). The expression of gluconeogenic genes (pepck and g6pase) and glucose transport genes (glut4) was significantly lower in the 0.4% IMP group than in the control group (P < 0.05) (Figure 2E). There was no significant difference in the expression of srebp1, aco3, gk, pfk and pk among all the groups (P > 0.05) (Figure 2E).
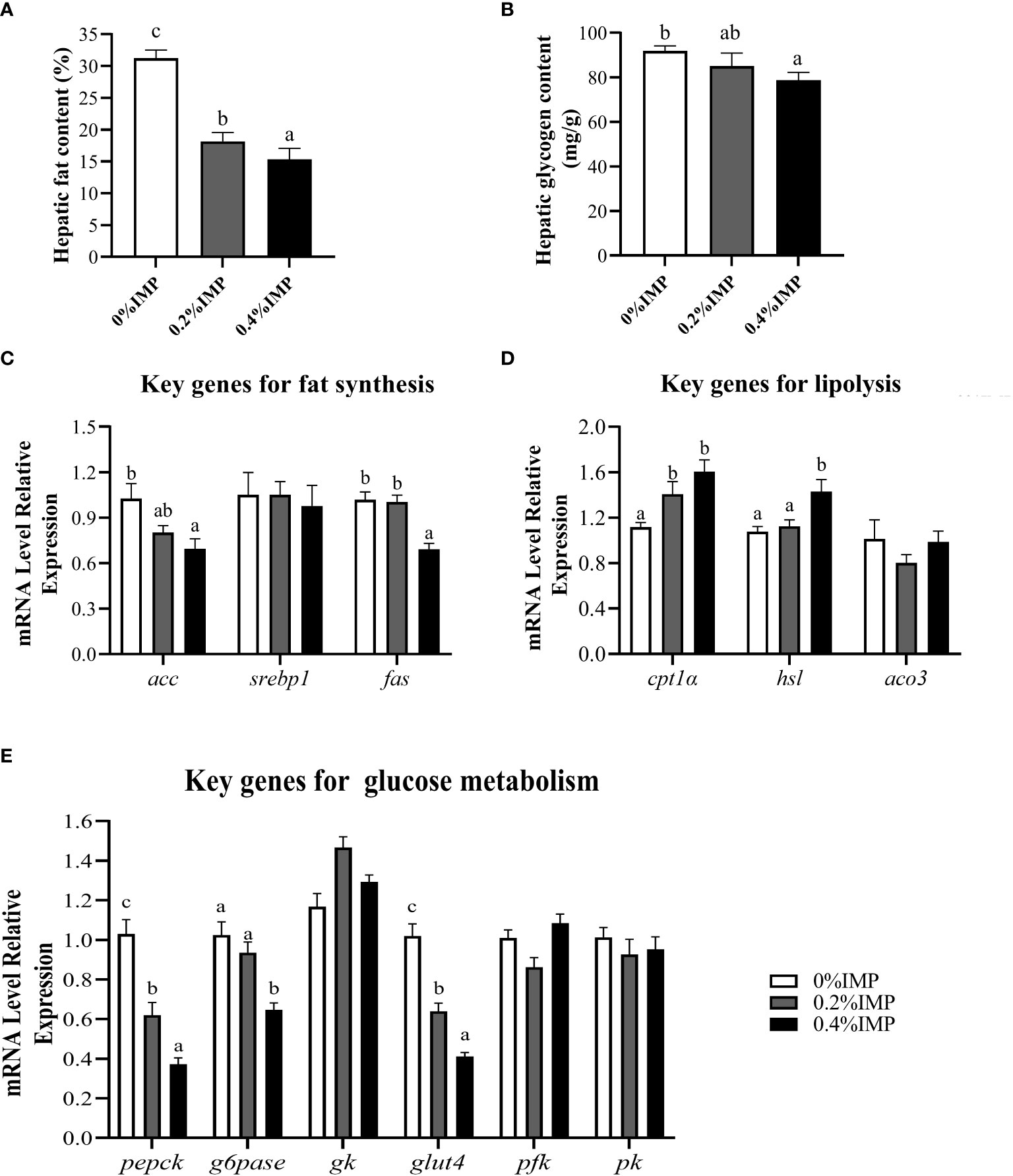
Figure 2 Effect of IMP supplementation on hepatic glycolipid metabolism of gibel carp. (A) The fat content in liver (B) The glycogen content in liver. (C) The expression of fat synthesis genes in liver (acc, srebp1 and fas). (D) The expression of lipolysis genes in liver (cpt1α, hsl, aco3). (E) The expression of glycometabolism genes in liver (pepck, g6pase, gk, glut4, pfk, pk). Columns represent the mean ± SEM (n=6). For each index, bars without sharing a common letter indicate significant differences (P <.05).
Effects of IMP supplementation on AMPK protein phosphorylation and nucleotides (ATP, AMP and IMP) contents in liver
In the present study, we investigated the phosphorylation of AMPK in the liver. Following IMP supplementation, the liver phospho-AMPK protein levels were increased. The 0.4% IMP group showed a significant increase in AMPK phosphorylation compared with the control group (P < 0.05) (Figures 3E, F). We also assessed the contents of IMP, adenosine 5′-triphosphate (ATP) and adenosine 5′-monophosphate (AMP) in the livers of gibel carp. We observed that the contents of IMP and AMP in the 0.4% IMP group were significantly increased compared to those in the control group (P < 0.05) (Figures 3A, C). However, there was no difference in the ATP content among all the groups (P > 0.05) (Figure 3B). The AMP/ATP ratio in the 0.4% IMP group was significantly higher than that in the control group (P < 0.05) (Figure 3D). A previous study showed that AMPK (AMP-activated protein kinase) is a conserved sensor of cellular energy changes and is activated when the AMP/ATP ratio increases (Ke et al., 2018). In this study, IMP supplementation activated AMPK by increasing the AMP/ATP ratio.
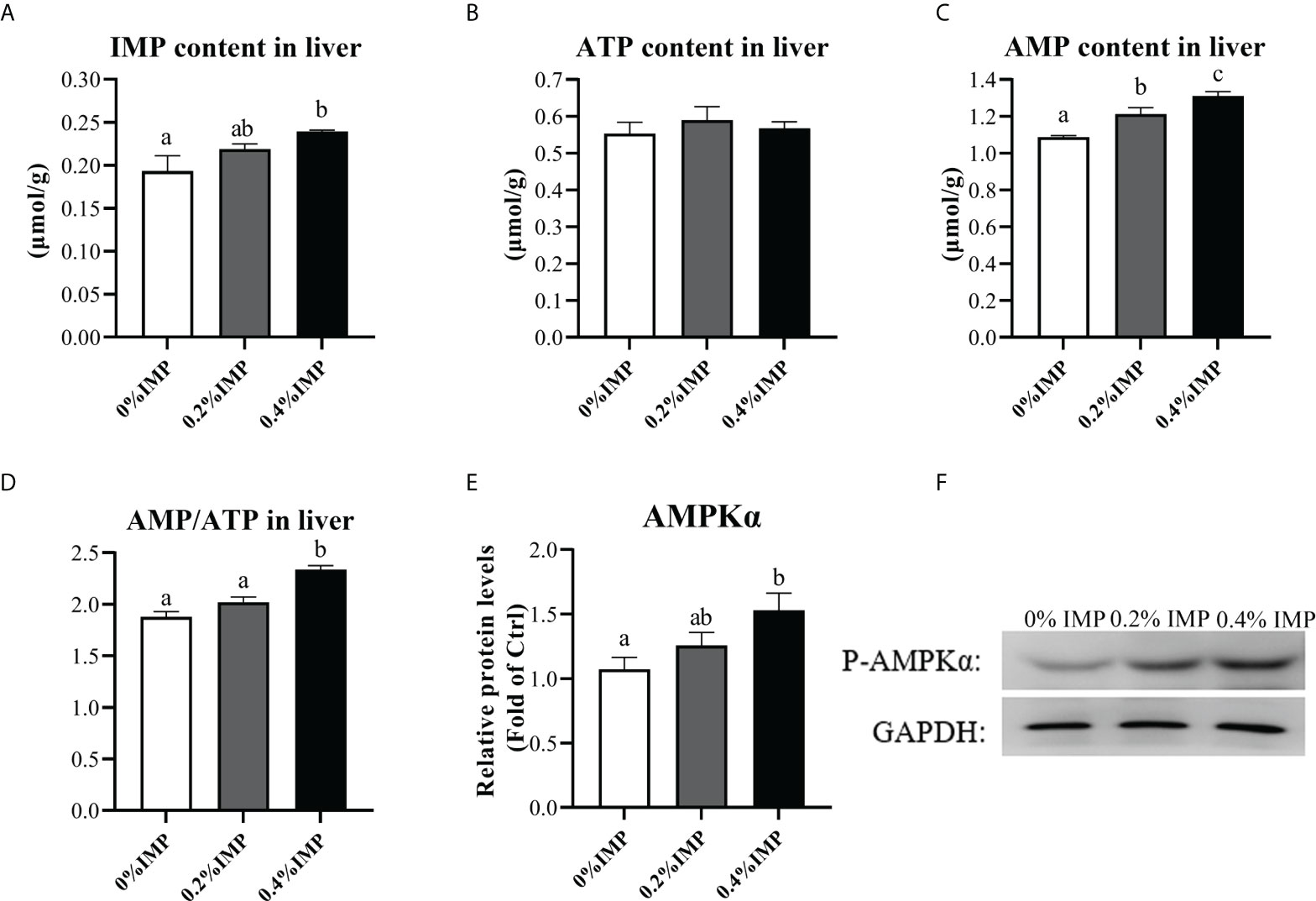
Figure 3 Dietary IMP supplementation activates the AMPK pathway by altering the ratio of AMP/ATP. (A) IMP content in the liver of groups. (B) Adenosine 5′-triphosphate (ATP) content in the liver of groups. (C) Adenosine 5′-monophosphate (AMP) content in the liver of groups. (D) Ration of AMP and ATP in the liver of groups. (E) The image quantization results of AMPK protein phosphorylation. (F) AMPK protein phosphorylation in the liver was measured by western blot. Columns represent the mean ± SEM (n=6). For each index, bars without sharing a common letter indicate significant differences (P <.05).
Effect of IMP supplementation on muscle energy substance level and lipid metabolism
To evaluate the possible beneficial effects of IMP on the muscles of gibel carp, we first measured the contents of fat, glycogen and protein in the dorsal muscles of gibel carp. The muscle fat content in the 0.4% IMP group was significantly higher than that in the control group (P < 0.05) (Figure 4A). However, compared to that in the 0% IMP group, the muscle glycogen content in the 0.4% IMP group was significantly decreased (P < 0.05) (Figure 4B). There was no significant difference between the 0% IMP group and the 0.4% IMP group (P > 0.05) (Figure 4C). Further experiments were performed to analyse the ATP and AMP contents in muscle by high-performance liquid chromatography (HPLC, Waters 2695e, USA). We found that the ATP and AMP contents and the AMP/ATP ratio were not significantly different among all the groups (P > 0.05) (Figures 4D–F). To explore the molecular mechanisms by which IMP regulates fat accumulation in muscle, we assessed the expression of key genes involved in lipogenesis (acc, srebp1 and pparγ), lipolysis (hsl and lpl) and fatty acid β-oxidation (cpt1α). The expression of acc, srebp1 and pparγ was downregulated in the 0.4% IMP group (Figure 4G). The expression of the key genes cpt1α and lpl was significantly reduced in the 0.4% IMP group compared with the control group (P < 0.05) (Figure 4H).
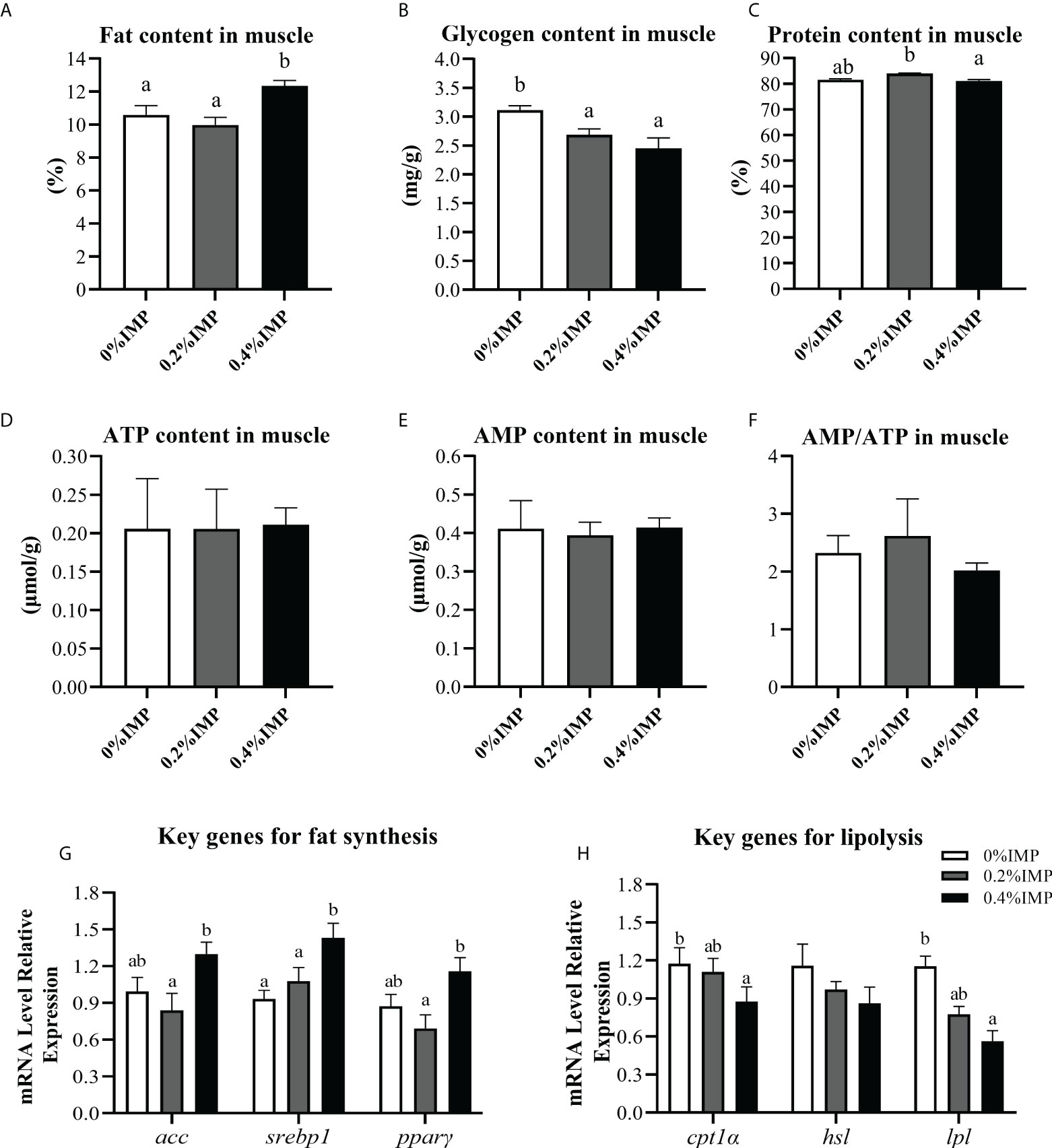
Figure 4 Effect of IMP supplementation on muscle energy substances and lipid metabolism. (A) The fat content in muscle was measured by the method of methanol chloroform. (B) The glycogen content in muscle. (C) The crude protein content in muscle (dry matter). (D) Adenosine 5′-triphosphate (ATP) content in the muscle of groups. (E) Adenosine 5′-monophosphate (AMP) content in the muscle of groups. (F) Ration of AMP and ATP in the liver of groups. (G) The expression of fat synthesis genes in muscle (acc, srebp1 and pparγ). (H) The expression of lipolysis genes in muscle (cpt1α, hsl, lpl). Columns represent the mean ± SEM (n=6). For each index, bars without sharing a common letter indicate significant differences (P < .05).
Metabolomics analysis of the effect of IMP supplementation on the flavour of gibel carp muscle
The contents of IMP were measured by HPLC, and the results showed that 0.4% IMP supplementation could increase the IMP content in dorsal muscles (Figure 5A). In addition, to further reveal the effects of IMP on the dorsal muscles of gibel carp, a metabolomics analysis based on LC-MS/MS was conducted to evaluate the metabolites. A total of 103 metabolites were identified in the muscle samples (Table S4). The heatmap displays the 50 metabolites that were most significantly different between the control group and the 0.4% IMP group (Figure 5B). A partial least squares discriminant analysis (PLS-DA) classification model was constructed to evaluate the metabolic differences of the muscle tissues in the control group and the 0.4% IMP group (Figure 5C). The picture shows that the metabolic profile of the control group was clearly different from that of the 0.4% IMP group. The results shown in the pathway enrichment analysis map and bubble plots (Figure S1 and Figure S2) indicated that the differential metabolites were mainly enriched in purine metabolism; pentose and glucuronate interconversions; pyrimidine metabolism; glyoxylate and dicarboxylate metabolism; tryptophan metabolism; and alanine, aspartate and glutamate metabolism (Table S2). In addition, among all the pathways, the difference in purine metabolism was the most significant (Figure 5D). Differential levels of metabolites that are important for purine metabolism was clearly visualized using boxplots (Figure 5E and Figure S3). The contents of IMP, inosine, hypoxanthine, guanosine, adenylic acid, adenosine and adenine in the 0.4% IMP group were higher than those in the control group.
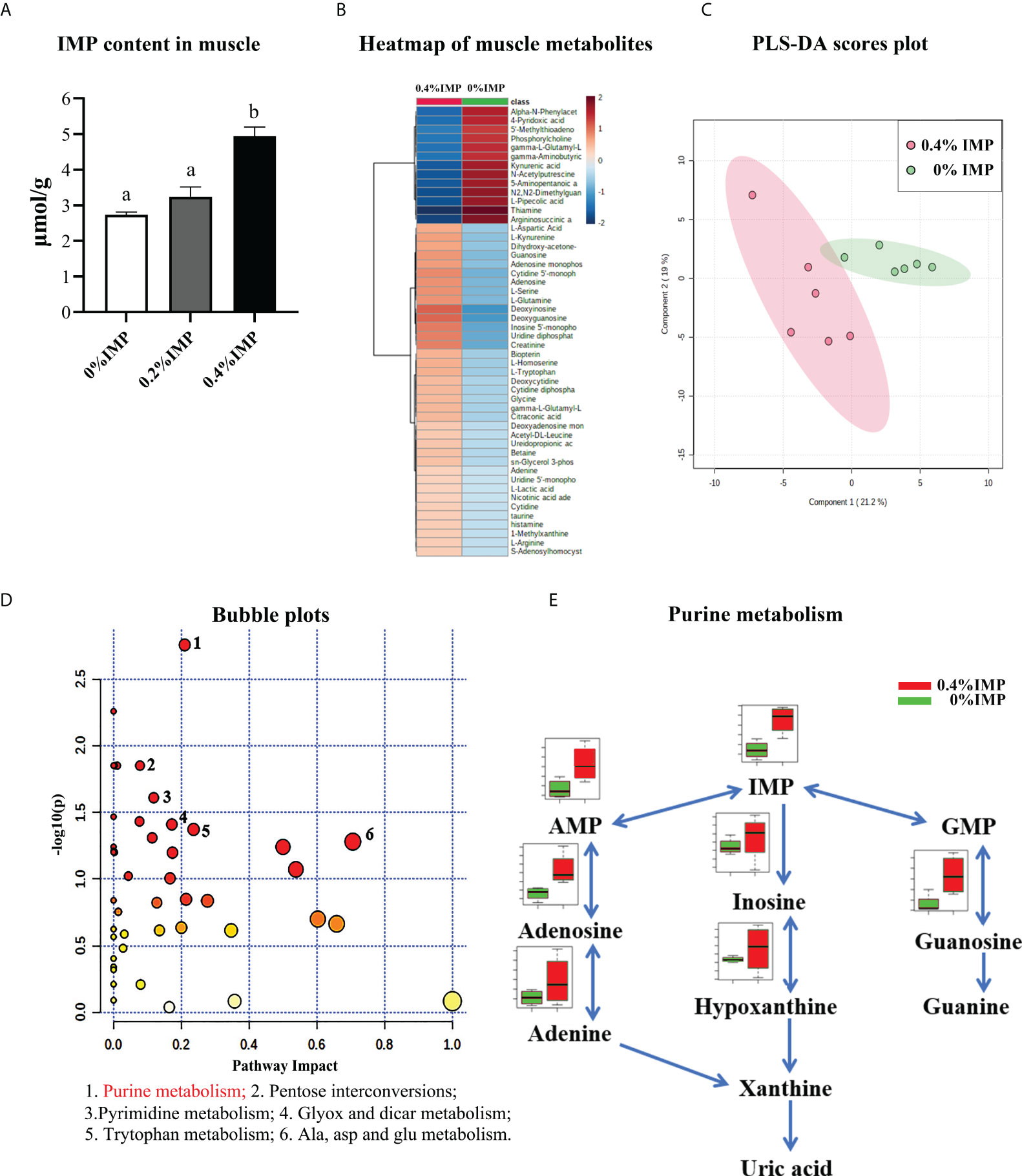
Figure 5 Metabolomics analysis—effect of IMP supplementation on muscle flavour of gibel carp. (A) IMP content in the muscle measured by HPLC. (B) Heat map representing the hierarchical cluster analysis (top 50) in 0%IMP VS 0.4%IMP. Red indicates high abundance, whereas the relatively low-abundance metabolites are shown in blue. (C) The partial least squares discriminant analysis (PLS-DA) score plots for metabolomics of muscle samples. 0.4%IMP group in red; 0%IMP group in green. (D) KEGG pathway enrichment analysis (top 25) of IMP, pathway enrichment in 0%IMP VS 0.4%IMP. The x-axis represents the enrichment ratio, while the y-axis represents the enrichment pathway. (E) integrative map of purine metabolism in muscle tissue. 0%IMP group in red; 0.4%IMP group in green.
Linear regression analysis of the effect of IMP supplementation on the gibel carp
The growth performance, whole body composition, metabolite contents and relative genes expression were analysed using linear regression analysis were showed in Table S6. In the growth performance and whole-body composition, the lines showed the significant linear correlation between the crude protein of whole-body and dietary IMP contents of gibel carp. In the metabolites content, the results were showed the significant linear correlation between the ALT, AST contents and dietary IMP contents. The hepatic fat, glycogen, IMP AMP contents and IMP content in muscle also were observed had a significant linear correlation with dietary IMP contents. In the relative genes expression, the results were showed that the significant linear correlation between the hepatic inflammatory cytokines genes (IL-6 and IL-1β), hepatic glycolipid genes (acc, fas, cpt1α, pepck, g6pase and glut4), lipid metabolism genes (srebp1 and lpl) in muscle and dietary IMP contents.
Discussion
In previous studies, it was revealed that exogenous IMP exerts growth-promoting and immune-enhancing effects (Song et al., 2012). This experiment provided a comprehensive evaluation of the effect of IMP supplementation on the metabolism of glycose and lipids in the liver and on the flavour quality of muscles in gibel carp. We found that dietary IMP supplementation could reduce the contents of fat and glycogen in the liver. These significant effects of IMP were mediated by the AMPK signalling pathway, which was activated by altering the AMP/ATP ratio. Additionally, dietary IMP supplementation increased the fat content in muscle, hence improving the tenderness and palatability of the edible portion. Metabolomics analysis revealed that exogenous IMP accelerated purine metabolism in muscle, resulting in increased content of the metabolite IMP, which enhanced the flavour of gibel carp muscle. The changes in these indicators showed that dietary supplementation with IMP could improve the muscle quality of gibel carp.
Dietary IMP supplementation could alleviate lipid deposition and protect the liver health of gibel carp
This study found that dietary IMP supplementation could reduce fat accumulation and decrease the inflammatory response of the liver. The liver, which plays central role in metabolic homeostasis, is a major metabolic organ for the synthesis, metabolism, storage and redistribution of carbohydrates, proteins and lipids. In the present study, the lipid level in the basal diet was slightly higher than that in commercially produced feed and led to the accumulation of fat in the livers of gibel carp. Excessive dietary fat intake could increase the concentration of free fatty acids in the blood and increase the levels of triglycerides stored in the liver, causing lipid accumulation (Honma et al., 2012). Excess glucose could also be used to synthesize fatty acids in the liver. When the liver does not process and breakdown fat as it normally should, excess fat accumulates. This study demonstrated that dietary IMP supplementation plays a positive role in hepatic lipid metabolism. Compared with the control diet, when the diet was supplemented with 0.4% IMP, oil red-stained sections and actual liver fat values revealed a significant reduction in the fat contents of the liver. In addition, the HSI was significantly decreased. TC and TG are important blood lipid indicators in patients with fatty liver disease (Huang et al., 2018). The TC, TG, LDL-C and HDL-C concentrations in the plasma of the 0.4% IMP treatment group were significantly lower than those in the control group. This result was consistent with observations of Nile tilapia (Yu et al., 2021) and obese db/db mouse (Noratto et al., 2019). These results indicated that IMP is an important regulator of lipid metabolism in the liver. Thus, it is clear that IMP plays a role in regulating hepatic lipid metabolism. Furthermore, we found that dietary IMP supplementation was able to significantly reduce the serum levels of AST and ALT, and this phenomenon is likely the result of improved liver function (De Silva et al., 2019). The excessive intake of nutrients, such as fat, and obesity could lead to the accumulation of immune cells and trigger an inflammatory response in the liver (Gregor and Hotamisligil, 2011; Dagdeviren et al., 2016). The levels of inflammatory cytokines (TGF-β, IL-6, TNF-α and IL-1β) are elevated with the onset of inflammation (Tortelli et al., 2020). We found that IMP supplementation reduced the gene expression levels of these cytokines in the liver, which suggested that dietary IMP supplementation could reduce the inflammatory response in the liver of gibel carp. Based on histological examination, dietary IMP supplementation reduced lipid deposition and decreased vacuolation in hepatocytes. These results led us to consider the possible mechanisms by which IMP supplementation could improve the liver health of gibel carp.
Dietary IMP supplementation reduced hepatic fat and glycogen deposition via regulating glycolipid metabolism in liver
The deposition of fat in the liver is a complex process, and the anabolism and catabolism of fat are two important factors that affect fat deposition (Guo et al., 2021). By measuring liver fat content and assessing liver oil red staining, this study found that the fat content was significantly lower in the IMP supplementation groups than in the control group. To investigate the underlying causes, the expression of genes associated with lipid anabolism and catabolism was analysed. ACC is often considered the rate-limiting enzyme in fatty acid biosynthesis. FAS can catalyse the formation of long-chain fatty acids from acetyl-CoA (Bueno et al., 2019). These proteins play key roles in fat anabolism. In this study, it was observed that dietary IMP supplementation increased the gene expression of acc and fas in gibel carp livers. Similar results of gene expression were also found in tilapia (Li et al., 2021). HSL and CPT1α are two important factors in lipolytic metabolism. HSL is an enzyme that plays a role in the first step of lipolysis (Lampidonis et al., 2011), and CPT1α is the rate-limiting enzyme in fatty acid β-oxidation (Lu et al., 2014). We found that the mRNA levels of acc and fas were significantly decreased in the 0.4% IMP group compared with the control group. Similar gene expression results were observed in a study in which anthocyanins from purple corn ameliorated obesity in mice fed a high-fat diet (Xu et al., 2021). These data suggested that decreased lipid deposition in the liver might be related to the increased expression of key genes in lipolysis and the decreased expression of key genes in lipid anabolism.
Glucose metabolism in the liver was also explored in the present study, as fat metabolism and glucose homeostasis are processes that are highly interconnected. The liver regulates glucose homeostasis mainly by maintaining the balance between gluconeogenesis and glycolysis (Zang et al., 2018). Glycogen is an indicator of glucose metabolism. Excess energy is partly turned into glycogen and then accumulates in the liver. The current study found that the hepatic glycogen level in the 0.4% IMP group was significantly decreased compared to that in the control group. The expression of key genes associated with glucose metabolism was analysed to reveal the underlying mechanisms. PEPCK and G6pase are key catalytic enzymes in the process of gluconeogenesis (Wang and Dong, 2019). In the present study, we observed a significant decrease in the expression of the pepck and g6pase genes in the 0.4% IMP group. However, the expression of genes (pfk and pk) related to glycolysis was not significantly different from that in the control group. Therefore, the data described above indicated that the decreased hepatic glycogen levels in the livers of gibel carp fed IMP-supplemented diets may be related to the downregulation of gluconeogenesis-related gene expression. The related gene expression results were consistent with a study using a combination of metformin and genistein to alleviate nonalcoholic fatty liver disease in high-fat diet-fed mice (Zamani-Garmsiri et al., 2021).
Dietary IMP supplementation activated the AMPK pathway by altering the ratio of AMP/ATP
AMPK is a nutrient and energy sensor that maintains energy homeostasis by regulating the expression of genes related to lipid and glucose metabolism (Ahmadian et al., 2011; Wu et al., 2013). To further explore the role of IMP in hepatic energy metabolism in fish, we investigated the phosphorylation of AMPK. In the present study, as dietary IMP supplementation increased, the phosphorylation of AMPK increased. Previous literature shows that when AMPK is activated, it inhibits the activity of acc and fas, which results in decreased fatty acid synthesis substrate levels and decreased lipid synthesis (Hardie, 2011). This was also confirmed by the relative gene expression data in the current study. Therefore, we hypothesized that dietary IMP supplementation could reduce hepatic fat deposition in gibel carp by activating the AMPK signalling pathway. Previous studies have shown that AMPK is activated by calcium/calmodulin-dependent protein kinase kinase II (CAMKK2), liver kinase B1 (LKB1) or the AMP/ATP ratio (Fogarty et al., 2016; Timm and Tyler, 2020; Dengler, 2020). Studies by Liu found that the AMPK activators metformin and genistein reduced liver fat accumulation in mice by mediating the AMPK signalling pathway (Liu et al., 2017). To explore the mechanism underlying AMPK activation, the contents of ATP, AMP and IMP in the livers of gibel carp were assessed by HPLC. The results showed a significant increase in the IMP and AMP levels and the AMP to ATP ratio in the 0.4% IMP group. Dietary nucleotides could replenish the nucleotide content of the body (Fustin et al., 2012). IMP and AMP are both purine nucleotide metabolites that are closely related to ATP (Xu et al., 2021). The ratio of AMP to ATP was significantly increased and resulted in the phosphorylation of AMPKα, which is a key regulator of energy metabolism. Further studies are needed to explore the details of the mechanism by which IMP activates the AMPK signalling pathway.
Dietary IMP supplementation increased the fat accumulation in muscle by up-regulating lipid synthesis genes
In farmed animals, fat deposition in the muscle is an important factor that influences meat quality (Zhang et al., 2020). In fish, muscle fat content is a key feature that strongly determines the organoleptic qualities and nutritional status of muscle (Medale et al., 2003). Besides, fat is a precursor substance for many volatile substances, which play an important role in the flavour of fish flesh. Therefore, it is necessary to investigate the effect of dietary IMP supplementation on the muscle fat content in gibel carp. This study observed that IMP supplementation significantly increased the deposition of fat in the muscle of gibel carp. Interestingly, this result is opposite that observed for fat deposition in the liver. To determine the underlying mechanism, we also assessed the contents of ATP and AMP in the muscle. However, no significant differences were found in the ATP and AMP contents in the muscle. We hypothesized that hepatic AMPK activation enhanced energy transport from the liver to peripheral tissues, and a similar phenomenon was also observed by Frayn et al. (2006) and Lopez (2018). In skeletal muscle, excess energy is resynthesized into fat for storage (Jensen-Urstad and Semenkovich, 2012). In the current study, we found that the expression of genes associated with lipid synthesis (acc, srebp1 and pparγ) was upregulated and that the expression of genes related to lipolysis (lpl and cpt1α) was downregulated in the 0.4% IMP group compared with the control group. Therefore, we assumed that the effect of IMP on fat deposition in muscle may be due to its direct regulation of the expression of certain genes related to lipid metabolism or to indirect mechanisms. The content of intramuscular fat is highly correlated with the overall flavour of farmed animals (Gandemer, 2002). Dietary IMP supplementation increased intramuscular fat contents, which was beneficial for improving the meat quality of gibel carp.
Metabolomics analysis revealed IMP increased the muscle flavor substance via the purine metabolism
The quality of fish is one of the decisive factors that affects consumer purchase choices. The components of flavour, such as intramuscular fat, free amino acids, free fatty acids and IMP, in muscle are closely associated with meat quality (Zhang et al., 2019). IMP is an important umami substance in animal tissues and can increase the umami taste of foods by 40-fold compared with sodium glutamate (Wang et al., 2020). In fish, IMP greatly contributes to the umami in flesh and has been used in many counties as an indicator of the freshness in fish (Suzuki et al., 1994). Therefore, we examined the IMP content in the dorsal muscle of gibel carp. The results showed that the IMP content in muscle was significantly higher in the 0.4% IMP group than in the control group. This result indicated that dietary IMP supplementation could increase the content of IMP in fish muscle.
IMP is an important factor in purine metabolism and represents a branch point in purine biosynthesis (Tolstikov et al., 2014). To investigate the effect of dietary IMP supplementation on its metabolic mechanism in muscle, we used metabolomics analysis based on LC-MS/MS, and the results demonstrated that dietary IMP supplementation could cause profound changes in the metabolites related to purine metabolism; pentose and glucuronate interconversion; pyrimidine metabolism; glyoxylate and dicarboxylate metabolism; tryptophan metabolism; and alanine, aspartate and glutamate metabolism. The present study showed that IMP supplementation notably changed the levels of many metabolites. Among them, a number of metabolites were enriched by KEGG into the purine metabolic pathway, such as IMP, AMP, adenine, guanosine and inosine, some of them were identified as key biomarkers for meat quality evaluation (Wen et al., 2020). Therefore, the alterations of purine metabolism pathway induced by dietary IMP supplementation may be the cause of the improvements in meat quality and composition. The precise mechanism underlying the effect of dietary IMP supplementation on purine metabolism requires further study.
Conclusion
In conclusion, our study revealed that exogenous IMP could reduce liver fat content and thus maintain liver health in gibel carp. The underlying mechanism may be that dietary IMP supplementation increases the AMP levels in the liver, thereby activating the AMPK signalling pathway. In addition, exogenous IMP can increase the deposition of fat and IMP in dorsal muscle and improve the meat quality of gibel carp (Figure 6). Our study provides a relatively comprehensive assessment of the effect of exogenous IMP on liver metabolism and flesh quality in farmed fish to provide an effective food supplementation strategy for increasing liver health and improving the flesh quality of cultured fish.
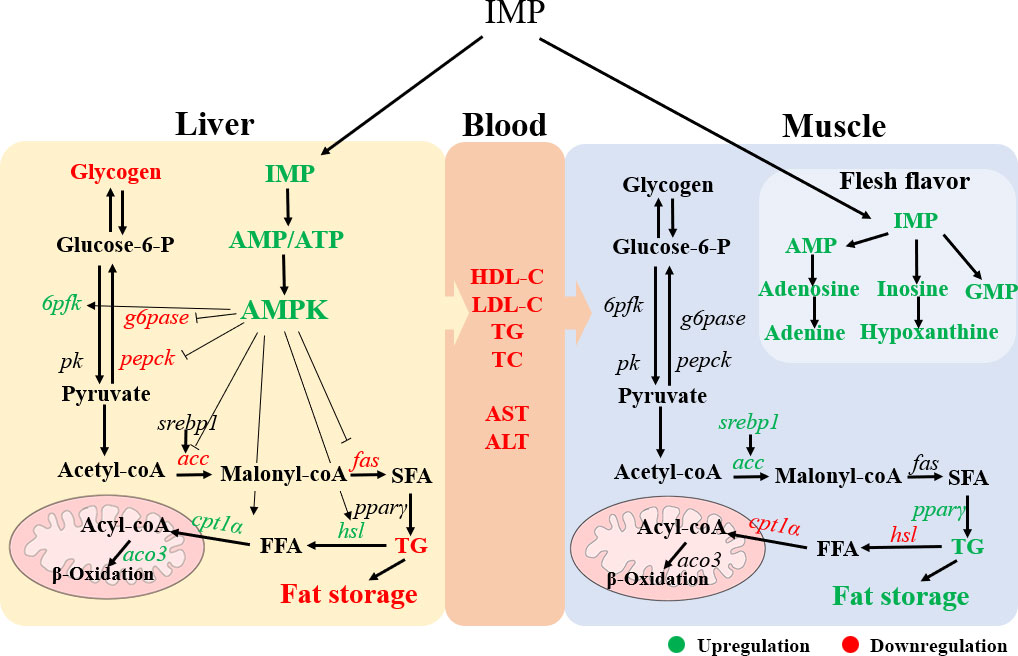
Figure 6 The schematic diagram showing a proposed mechanism for exogenous IMP on physiological metabolism of gibel carp. IMP increased the ration of AMP/ATP in liver, which activated AMPK signal pathway. This activation reduced the content of fat and glycogen in liver by regulating fat and glucose metabolism. In addition, increased fat deposition and the flavor substance inosinic acid in the muscle. Green color indicates upregulation and red color indicates downregulation.
Data availability statement
The original contributions presented in the study are included in the article/Supplementary Material. Further inquiries can be directed to the corresponding author.
Ethics statement
The animal study was reviewed and approved by ethics committee of the Institute of Hydrobiology of Chinese Academy of Sciences.
Author contributions
WC: investigation, formal analysis, data curation, writing- original draft preparation. HL: conceptualization, data curation, project administration, writing - review & editing. LF: investigation. DH: methodology. XZ: methodology. JJ and YY: resources. SX: supervision, funding acquisition. All authors contributed to the article and approved the submitted version.
Funding
This work was financially supported by National Key R&D Program of China (2018YFD0900400 and 2019YFD0900200), China Agriculture Research System (CARS-45), Science and Technology Project of Wuhan (2019020701011459), Poverty Alleviation through Agricultural Projects from the Agricultural Office of Chinese Academy of Sciences (KFJ-FP-201803) and National Natural Science Foundation of China (32071651).
Acknowledgments
The authors thank Ms. Jun Men (the Center for Instrumental Analysis and Metrology, Institute of Hydrobiology, Chinese Academy of Science) for technical assistance in the measurement of IMP, ATP and AMP. The authors also thank Mr. Guanghan Nie for his technical assistance.
Conflict of interest
The authors declare that the research was conducted in the absence of any commercial or financial relationships that could be construed as potential conflicts of interest.
Publisher’s note
All claims expressed in this article are solely those of the authors and do not necessarily represent those of their affiliated organizations, or those of the publisher, the editors and the reviewers. Any product that may be evaluated in this article, or claim that may be made by its manufacturer, is not guaranteed or endorsed by the publisher.
Supplementary material
The Supplementary Material for this article can be found online at: https://www.frontiersin.org/articles/10.3389/fmars.2022.940732/full#supplementary-material
References
Ahmadian M., Abbott M. J., Tang T. Y., Hudak C. S., Kim Y., Bruss M., et al. (2011). Desnutrin/ATGL is regulated by AMPK and is required for a brown adipose phenotype. Cell Metab. 13 (6), 739–748. doi: 10.1016/j.cmet.2011.05.002
AOAC (2005). Official methods of analysis of AOAC international, eighteenth ed (Arlington, Virginia, USA: Association of Official Analytical Chemists).
Bao Y. F., Xiao J. B., Weng Z. B., Lu X. Y., Shen X. C., Wang F. (2020). A phenolic glycoside from moringa oleifera lam. improves the carbohydrate and lipid metabolisms through AMPK in db/db mice. Food Chem. 311, 125948. doi: 10.1016/j.foodchem.2019.125948
Bueno M. J., Jimenez-Renard V., Samino S., Capellades J., Junza A., López-RodrÃguez M. L., et al. (2019). Essentiality of fatty acid synthase in the 2D to anchorage-independent growth transition in transforming cells. Nat. Commun. 10, 5011. doi: 10.1038/s41467-019-13028-1
Carver J. D. (1994). Dietary nucleotides: Cellular immune, intestinal and hepatic system effects. J. Nutr. 124, 144S–148S. doi: 10.1093/jn/124.suppl_1.144S
Cheng J. H., Sun D. W., Pu H. B., Zhu Z. W. (2015). Development of hyperspectral imaging coupled with chemometric analysis to monitor K value for evaluation of chemical spoilage in fish fillets. Food Chem. 185, 245–253. doi: 10.1016/j.foodchem.2015.03.111
Dagdeviren S., Jung D. Y., Lee E., Friedline R. H., Noh H. L., Kim J. H., et al. (2016). Altered interleukin-10 signaling in skeletal muscle regulates obesity-mediated inflammation and insulin resistance. Mol. Cell. Biol. 36 (23), 2956–2966. doi: 10.1128/MCB.00181-16
Dengler F. (2020). Activation of AMPK under hypoxia: Many roads leading to Rome. Int. J. Mol. Sci. 21 (7), 2428. doi: 10.3390/ijms21072428
De Silva N. M. G., Borges M. C., Hingorani A. D., Engmann J., Shah T., Zhang X. S., et al. (2019). Liver function and risk of type 2 diabetes: Bidirectional mendelian randomization study. Diabetes 68 (8), 1681–1691. doi: 10.2337/db18-1048
Farber E., Shull K. H., McConomy J. M., Castillo A. E. (1965). The effects of inosine and aminoimidazole carboxamide upon the ethionine fatty liver. Biochem. Pharmacol. 14, 761–767. doi: 10.1016/0006-2952(65)90094-8
Fisheries Bureau, Department of Agriculture of China (2020). China Fishery statistical yearbook (Beijing: China Agriculture Press), 24.
Fogarty S., Ross F. A., Ciruelos D. V., Gray A., Gowans G. J., Hardie D. G. (2016). AMPK causes cell cycle arrest in LKB1-deficient cells via activation of CAMKK2. Mol. Cancer Res. 14 (8), 683–695. doi: 10.1158/1541-7786.MCR-15-0479
Frayn K. N., Arner P., Yki-Jarvinen H. (2006). Fatty acid metabolism in adipose tissue, muscle and liver in health and disease. Essays Biochem. 42, 89–103. doi: 10.1042/bse0420089
Fustin J. M., Doi M., Yamada H., Komatsu R., Shimba S., Okamura H. (2012). Rhythmic nucleotide synthesis in the liver: Temporal segregation of metabolites. Cell Rep. 1 (4), 341–349. doi: 10.1016/j.celrep.2012.03.001
Gandemer G. (2002). Lipids in muscles and adipose tissues, changes during processing and sensory properties of meat products. Meat Sci. 62 (3), 309–321. doi: 10.1016/S0309-1740(02)00128-6
Gregor M. F., Hotamisligil G. S. (2011). Inflammatory mechanisms in obesity. Annu. Rev. Immunol. 29, 415–445. doi: 10.1146/annurev-immunol-031210-101322
Gui J. F., Zhou L. (2010). Genetic basis and breeding application of clonal diversity and dual reproduction modes in polyploid carassius auratus gibelio. Sci. China Life Sci. 53 (4), 409–415. doi: 10.1007/s11427-010-0092-6
Guo Y. L., Huang D., Chen F. Y., Ma S. L., Zhou W. Y., Zhang W. B., et al. (2021). Lipid deposition in abalone Haliotis discus hannai affected by dietary lipid levels through AMPKα2/PPARα and JNK/mTOR/SREBP-1c pathway. Aquaculture 532, 736040. doi: 10.1016/j.aquaculture.2020.736040
Hardie D. G. (2011). Sensing of energy and nutrients by AMP-activated protein kinase. Am. J. Clin. Nutr. 93 (4), 891s–896s. doi: 10.3945/ajcn.110.001925
Hardie D. G., Ross F. A., Hawley S. A. (2012). AMPK: a nutrient and energy sensor that maintains energy homeostasis. Nat. Rev. Mol. Cell Biol. 13 (4), 251–262. doi: 10.1038/nrm3311
Honma T., Shinohara N., Ito J., Kijima R., Sugawara S., Arai T., et al. (2012). High-fat diet intake accelerates aging, increases expression of Hsd11b1, and promotes lipid accumulation in liver of SAMP10 mouse. Biogerontology 13, 93–103. doi: 10.1007/s10522-011-9363-2
Hossain M. S., Koshio S., Ishikawa M., Yokoyama S., Sony N. M. (2016). Dietary effects of adenosine monophosphate to enhance growth, digestibility, innate immune responses and stress resistance of juvenile red sea bream, pagrus major. Fish Shellfish Immunol. 56, 523–533. doi: 10.1016/j.fsi.2016.08.009
Huang L. D., Cheng Y., Huang K., Zhou Y., Ma Y. Q., Zhang M. C. (2018). Ameliorative effect of Sedum sarmentosum bunge extract on tilapia fatty liver via the PPAR and P53 signaling pathway. Sci. Rep. 8, 8456. doi: 10.1038/s41598-018-26084-2
Huang T. W., Lin C. W., Ding S. T. (2020). Development of a chicken model of nonalcoholic fatty liver disease induced by high cholesterol and low choline diet. FASEB J. 34 (S1), 1–1. doi: 10.1096/fasebj.2020.34.s1.05036
Jensen-Urstad A. P. L., Semenkovich C. F. (2012). Fatty acid synthase and liver triglyceride metabolism: Housekeeper or messenger? Biochim. Biophysica-Mol. Cell Biol. Lipids 1821 (5), 747–753. doi: 10.1016/j.bbalip.2011.09.017
Jiang F., Chen Q., Wang W., Ling Y., Yan Y., Xia P. (2020). Hepatocyte-derived extracellular vesicles promote endothelial inflammation and atherogenesis via microRNA1. J. Hepatol. 72, 156–166. doi: 10.1016/j.jhep.2019.09.014
Jin J. Y., Zhu X. M., Han D., Yang Y. X., Liu H. K., Xie S. Q. (2017). Different regulation of insulin on glucose and lipid metabolism in 2 strains of gibel carp. Gen. Comp. Endocrinol. 246, 363–371. doi: 10.1016/j.ygcen.2017.01.012
Ke R., Xu Q. C., Li C., Luo L. Y., Huang D. Q. (2018). Mechanisms of AMPK in the maintenance of ATP balance during energy metabolism. Cell Biol. Int. 42, 384–392. doi: 10.1002/cbin.10915
Lampidonis A. D., Rogdakis E., Voutsinas G. E., Stravopodis D. J. (2011). The resurgence of hormone-sensitive lipase (HSL) in mammalian lipolysis. Gene 477, 1–11. doi: 10.1016/j.gene.2011.01.007
Lin Y. H., Wang H., Shiau S. Y. (2009). Dietary nucleotide supplementation enhances growth and immune responses of grouper, epinephelus malabaricus. Aquacult. Nutr. 15 (2), 117–122. doi: 10.1111/j.1365-2095.2007.00561.x
Liu H. H., Zhong H. J., Yin Y. M., Jiang Z. Q. (2017). Genistein has beneficial effects on hepatic steatosis in high fat-high sucrose diet-treated rats. BioMed. Pharmacother. 91, 964–969. doi: 10.1016/j.biopha.2017.04.130
Li L. Y., Wang Y., Limbu S. M., Li J. M., Qiao F., Chen L. Q., et al. (2021). Reduced fatty acid β-oxidation improves glucose catabolism and liver health in Nile tilapia (Oreochromis niloticus) juveniles fed a high-starch diet. Aquaculture 535, 736392. doi: 10.1016/j.aquaculture.2021.736392
Lopez M. (2018). Hypothalamic AMPK and energy balance. Eur. J. Clin. Invest. 48 (9), e12996. doi: 10.1111/eci.12996
Lu K. L., Xu W. N., Wang L. N., Zhang D. D., Zhang C. N., Liu W. B. (2014). Hepaticβ-oxidation and regulation of carnitine palmitoyltransferase (CPT) I in blunt snout bream Megalobrama amblycephala fed a high fat diet. PLoS One 588, 9–16. doi: 10.1080/15421406.2013.822298
MeDa≤F.,LefeVre F., Corraze G. (2003). Nutritional and health promoting value of fish: main factors affecting flesh components. Cahiers Nutr. Diététique. 38, 37–44. doi: CND-02-2003-38-1-0007-9960-101019-ART4
Naik H. M., Majewska N. I., Betenbaugh M. J. (2018). Impact of nucleotide sugar metabolism on protein n-glycosylation in Chinese hamster ovary (CHO) cell culture. Curr. Opin. Chem. Engineer. 22, 167–176. doi: 10.1016/j.coche.2018.10.002
Noratto G. D., Murphy K., Chew B. P. (2019). Quinoa intake reduces plasma and liver cholesterol, lessens obesity-associated inflammation, and helps to prevent hepatic steatosis in obese db/db mouse. Food Chem. 287, 107–114. doi: 10.1016/j.foodchem.2019.02.061
Pang Z. Q., Chong J., Zhou G. Y., de Lima Morais D. A., Chang L., Barrette M., et al. (2021). MetaboAnalyst5.0: Narrowing the gap between raw spectra and functional insights. Nucleic Acids Res. 49 (W1), W388–W396. doi: 10.1093/nar/gkab382
Pareek V., Tian H., Winograd N., Benkovic S. J. (2020). Metabolomics and mass spectrometry imaging reveal channeled de novo purine synthesis in cells. Science 368 (6488), 283. doi: 10.1126/science.aaz6465
Sako N., Tokita K., Sugimura T., Yamamoto T. (2003). Synergistic responses of the chorda tympani to mixtures of umami and sweet substances in rats. Chem. Senses 28 (3), 261–266. doi: 10.1093/chemse/28.3.261
Sheng S. S., Zhao S. M., Gao L., Zheng H. F., Liu W. T., Hou J., et al. (2019). BabaoDan attenuates high-fat diet-induced non-alcoholic fatty liver disease via activation of AMPK signaling. Cell Biosci. 9 (1), 77. doi: 10.1186/s13578-019-0339-2
Song J. W., Lim S. J., Lee K. J. (2012). Effects of dietary supplementation of inosine monophosphate on growth performance, innate immunity and disease resistance of olive flounder (Paralichthys olivaceus). Fish Shellfish Immunol. 33 (4), 1050–1054. doi: 10.1016/j.fsi.2012.07.011
Suzuki A., Homma N., Fukuda A., Hirao K., Uryu T., Ikeuchi Y. (1994). Effects of high- pressure treatment on the flavour-related components in meat. Meat Sci. 37 (3), 369–379. doi: 10.1016/0309-1740(94)90053-1
Timm K. N., Tyler D. J. (2020). The role of AMPK activation for cardio protection in doxorubicin-induced cardiotoxicity. Cardiovasc. Ther. 34 (2), 255–269. doi: 10.1007/s10557-020-06941-x
Tolstikov V., Nikolayev A., Dong S., Zhao G., Kuo M. S. (2014). Metabolomics analysis of metabolic effects of nicotinamide phosphoribosyltransferase (NAMPT) inhibition on human cancer cells. PLoS One 9 (12), e114019. doi: 10.1371/journal.pone.0114019
Tortelli R., Zecca C., Piccininni M., Benmahamed S., Dell-Abate M. T., Barulli M. R., et al. (2020). Plasma inflammatory cytokines are elevated in ALS. Front. Neurol. 11. doi: 10.3389/fneur.2020.552295
Vandesompele J., De Preter K., Pattyn F., Poppe B., Van Roy N., De Paepe A., et al. (2002). Accurate normalization of real-time quantitative RT-PCR data by geometric averaging of multiple internal control genes. Genome Biol. 3 (7), research0034. doi: 10.1186/gb-2002-3-7-research0034
Wang S. M., Chen S. L., Cui Y. B. (1993). On the procedures of chloroform-methanol extraction for the determination of lipid content of fish samples. Acta Hydrobiol. Sinica 17 (2), 193. doi: 10.1007/BF02943552
Wang Z., Dong C. (2019). Gluconeogenesis in cancer: Function and regulation of PEPCK, FBPase, and G6Pase. Trends Cancer 5, 30–45. doi: 10.1016/j.trecan.2018.11.003
Wang G. X., Sun J. F., Yao Y., An X. S., Zhang H., Chu G. L., et al. (2020). Detection of inosine monophosphate (IMP) in meat using double-enzyme sensor. Food Analytical Methods 13 (2), 420–432. doi: 10.1007/s12161-019-01652-y
Wang T., Wei X. Z., Chen T. Q., Wang W., Xia X. Z., Miao J. H., et al. (2019). Studies of the mechanism of fatty liver formation in Takifugu fasciatus following copper exposure. Ecotoxicol. Environ. Safety 181, 353–361. doi: 10.1016/j.ecoenv.2019.06.013
Wen D., Liu Y., Yu Q. (2020). Metabolomic approach to measuring quality of chilled chicken meat during storage. Poultry Sci. 99 (5), 2543–2554. doi: 10.1016/j.psj.2019.11.070
Wu N., Zhang B., Shaywitz A., Dagon Y., Tower C., Bellinger G., et al. (2013). AMPK-dependent degradation of TXNIP upon energy stress leads to enhanced glucose uptake via GLUT1. Mol. Cell. 49 (6), 1167–1175. doi: 10.1016/j.molcel.2013.01.035
Xu H. Y., Liu M. H., Liu H. M., Zhao B. L., Zhang M. Z., Liu J. S. (2021). Anthocyanins from purple corn ameliorated obesity in high fat diet-induced obese mice through activating hepatic AMPK. J. Funct. Foods 84, 104582. doi: 10.1016/j.jff.2021.104582
Yan J., Liao K., Wang T. J., Mai K. S., Xu W., Ai Q. H. (2015). Dietary lipid levels influence lipid deposition in the liver of large yellow croaker (Larimichthys crocea) by regulating lipoprotein receptors, fatty acid uptake and triacylglycerol synthesis and catabolism at the transcriptional level. PLoS One 10 (6), e0129937. doi: 10.1371/journal.pone.0129937
Yu K., Huan K., Jiang S. S., Tang X., Huang X. Y., Sun L. L., et al. (2021). Protective function on liver and proteomic analysis of the improvement mechanism of Sedum sarmentosum bunge extract on nonalcoholic fatty liver disease in Nile tilapia (Oreochromis niloticus). Aquaculture 531, 735977. doi: 10.1016/j.aquaculture.2020.735977
Zamani-Garmsiri F., Hashemnia S. M. R., Shabani M., Bagherieh M., Emamgholipour S., Meshkani R. (2021). Combination of metformin and genistein alleviates non-alcoholic fatty liver disease in high -fat diet-fed mice. J. Nutr. Biochem. 87, 108505. doi: 10.1016/j.jnutbio.2020.108505
Zang Y. F., Fan L., Chen J. H., Huang R. X., Hong Q. (2018). Improvement of lipid and glucose metabolism by capsiate in palmitic acid-treated HepG2 cells via activation of AMPK/SIRT1 signal pathway. J. Agric. Food Chem. 66 (26), 6772–6781. doi: 10.1021/acs.jafc.8b01831
Zhang M., Li D. H., Zhai Y. H., Wang Z. Z., Ma X. F., Zhang D. Y., et al. (2020). The landscape of DNA methylation associated with the transcriptomic network of intramuscular adipocytes generates insight into intramuscular fat deposition in chicken. Front. Cell Dev. Biol. 8. doi: 10.3389/fcell.2020.00206
Keywords: inosine monophosphate, AMPK, liver health, flesh quality, purine metabolism
Citation: Cai W, Liu H, Fu L, Han D, Zhu X, Jin J, Yang Y and Xie S (2022) Dietary inosine monophosphate improved liver health and flesh quality of gibel carp (Carassius auratus gibelio) via activating AMPK signalling pathway and enhancing the contents of muscle fat and flavour substance. Front. Mar. Sci. 9:940732. doi: 10.3389/fmars.2022.940732
Received: 16 May 2022; Accepted: 15 July 2022;
Published: 08 August 2022.
Edited by:
Samad Rahimnejad, University of South Bohemia in České Budějovice, CzechiaReviewed by:
Jun Xie, Pearl River Fisheries Research Institute (CAFS), ChinaShiwei Xie, Guangdong Ocean University, China
Omid Safari, Ferdowsi University of Mashhad, Iran
Copyright © 2022 Cai, Liu, Fu, Han, Zhu, Jin, Yang and Xie. This is an open-access article distributed under the terms of the Creative Commons Attribution License (CC BY). The use, distribution or reproduction in other forums is permitted, provided the original author(s) and the copyright owner(s) are credited and that the original publication in this journal is cited, in accordance with accepted academic practice. No use, distribution or reproduction is permitted which does not comply with these terms.
*Correspondence: Haokun Liu, liuhaokun@ihb.ac.cn