- 1Fisheries College, Jimei University, Xiamen, China
- 2Ornamental Aquarium Engineering Research Centre in University of Fujian Province, Xiamen, China
- 3Key Laboratory of Healthy Mariculture for the East China Sea, Ministry of Agriculture and Rural Affairs, Xiamen, China
Bestiolina amoyensis distributes in subtropical inshore waters across the Pacific Ocean, with a relatively long reproductive lifespan and high intrinsic population increase rate compared with other small paracalanid species, which makes it a good candidate to develop culture techniques for hatchery larval rearing. However, the reproductive biology of this subtropical broadcast spawning species is still largely unknown. The present investigation provides the first published data on the embryo development and effects of different light intensities (0, 500, and 1,000 lx), temperatures (16°C, 18°C, 20°C, 22°C, 24°C, 26°C, 28°C, 30°C, 32°C, and 34°C), and salinities (22, 24, 26, 28, 30, 32, and 34 psu) on hatching success rates of B. amoyensis. The same batch of eggs were collected from gravid females to observe their embryonic development and incubated under designed light intensities, temperatures, and salinities. Results showed that the whole embryonic development of B. amoyensis lasted, on average, 6 h and 40 min at 26°C, and egg hatching time of B. amoyensis shortened exponentially with the increasing temperature. The highest egg hatching rate (100%) was recorded from the 0-lx treatment, indicating that the dark condition was favorable for the egg incubation of B. amoyensis. The optimum temperature and salinity range for the hatching success of B. amoyensis was 22°C–30°C (above 94%) and 22–34 psu salinity (above 88%), respectively, indicating that B. amoyensis had wide adaptability to temperature and salinity. Light and too low or high temperature leads to abnormal embryonic development and malformed nauplii. The relatively wide adaptability to temperature and salinity and fast embryo development also suggests that B. amoyensis was a good candidate as live feed for hatchery larval rearing.
1 Introduction
Planktonic copepods are natural and preferable feeds for fish and invertebrate larvae in marine environment and are widely used as live feed in marine larviculture hatcheries (Drillet et al., 2011; Santhanam et al., 2019; Fernández-Ojeda et al., 2021; Pan et al., 2022), especially some species of paracalanid family, such as Parvocalanus carssirostris (Alajmi & Zeng, 2015; Kline & Laidley, 2015; Valencia et al., 2022), Bestiolina similis (McKinnon et al., 2003; Camus et al., 2009; VanderLugt et al., 2009; Camus & Zeng, 2010; Camus et al., 2021), and Bestiolina amoyensis (Wang et al., 2021). Small body size and the herbivorous characteristics make them good candidates as larvae prey for fish larvae with small mouth gape (Wang et al., 2021).
Calanoid copepods are generally well adapted to seasonal fluctuations in temperature and salinity under natural conditions (Miller & Marcus, 1994; Engel and Hirche, 2004; Marcus, 2005). Examples are Eurytemora affinis (Roddie et al., 1984; Nagaraj, 1992; Devreker et al., 2009; Souissi et al., 2016; Karlsson et al., 2018) and a number of Acartia congeners (Gaudy et al, 2000; Castro-Longoria, 2003; Chinnery & Williams, 2004; Milione & Zeng, 2008; Choi et al., 2021; Choi et al., 2022); these estuarine and coastal calanoid copepods are more widely adapted to temperature and salinity than pelagic species, which makes them more suitable for culture as live feed. For most of Paracalanidae species, information about reproduction is still very limited, and the functional response of reproductive success (i.e., egg production and hatching) to salinity and/or temperature only have been studied in a handful species, such as Paracalanus parvus (Jang et al., 2013). In addition, light is one of the most significant ecological factors influencing many biological functions of organisms (Hairston & Kearns, 1995; Chinnery & Williams, 2004; Radhakrishnan et al., 2020), but there are few studies that focus on the functional response of copepods to light intensity and/or periodicity (Peck & Holste, 2006, Peck et al., 2008; Radhakrishnan et al., 2020; Wang et al., 2021). Therefore, it is necessary to further study the effects of environmental factors on the reproductive success of copepods in order to optimize the culture conditions and maintain a relative high population density.
The embryonic development study of Crustaceans can be retracted to the nineteenth century, but it mainly focus on the species of Decapoda. Copepods have always received very little attention (Loose and Scholtz, 2019). Few available copepod embryonic studies were mainly on species with large eggs (>100 μm) (Marshall & Orr, 1954; Marshall and Orr, 1955; Conover, 1967; Gao, 2014; Loose and Scholtz, 2019) or resting eggs (Marshall & Orr, 1954; Marshall and Orr, 1955; Chen, 2014; Nilsson & Hansen, 2018). Small size copepods usually have a tiny egg (50–90 m in diameter) and covering with a very complicated chitin shell, which make the observation became difficult. We still know very little about the embryonic development of copepods nowadays.
Bestiolina amoyensis distributes in subtropical coastal and estuarine waters, which is easily cultured in laboratory (Wang et al., 2021) and hence is recommended as one of the promising live feed candidates. Previous studies have quantified the effects of food concentration and photoperiod on the reproductive performance of B. amoyensis, including egg production and female life expectancy (Wang et al., 2021). Clarifying the embryonic development of B. amoyensis not only could improve the basic understanding about ontogenesis of crustaceans but also could enhance the intensive culture techniques as a live feed. For some fish larvae with a small gape, they only could use Nauplii I copepods as the first feed. Farmers could predict the exact time when Nauplii I copepods could be collected for fish feeding. Therefore, the embryonic development process was investigated at 26°C, and the hatching time at different temperatures was evaluated. In an effort to optimize the incubation conditions for egg hatching and embryonic development of B. amoyensis, three experiments were conducted to quantify egg hatching success: (1) under different light intensities, (2) at different temperatures, and (3) at different salinities.
2 Materials and Methods
2.1 B. amoyensis Stock Culture
Bestiolina amoyensis was obtained from Ornamental Aquarium Engineering Research Centre of Jimei University, Xiamen, China. It was scaled up and kept in several 18-L containers filled with 0.01-μm filtered seawater and with gentle aeration. For stock culture conditions, B. amoyensis was fed daily with microalgae Isochrysis spp. at a concentration of 1 × 105 cells ml−1, and the temperature and salinity were maintained at 26 ± 1°C and 28 ± 1 psu, respectively. Light intensity was 500 lx with a light/dark cycle of 12 h:12 h (Wang et al, 2021).
2.2 Experimental Design and Setup
A series of experiments were carried out to explore the embryonic development of B. amoyensis and assess the effects of temperature, salinity, and light intensity on the hatching of embryos.
2.2.1 Embryogenesis of B. amoyensis
The mature male/female ratio was designed to be 2:1 to make sure the mating success and placed into six-well cell culture plates with 28 ± 1 psu seawater, and the concentration of microalgae Isochrysis spp. was 1 × 105 cells ml−1. Plates with paired adults were placed into an incubator with constant temperature (26 ± 1°C) and a light/dark cycle of 0L:24D. After 20–30 min of dark culture in the incubator, newly spawned fertilized eggs were collected under a microscope using a glass pipette with an arc tip.
Embryo development experiments were performed with 90 replicates (two to three eggs from the same batch per replicate). Eggs were transferred to 35 mm in diameter NEST® glass-bottom cell culture dishes, containing filtered seawater of the same salinity. Eggs were incubated at 26 ± 1°C for embryonic development under dark conditions.The frequency of embryo observation was determined by pre-experiments as a way to calculate the percentage of specific stages within a set time. Microscopic observations were made at 5-min intervals for 40 min after spawning and at 30-min intervals for the next 90 min. Microscopic observations were made at 1-h intervals after 2 h and 10 min of spawning and at 30-min intervals after 5 h and 10 min of spawning. Median development time of a specific stage was defined as the period when 50% of the species had passed into that stage (Landry, 1975).
2.2.2 Hatching Time Experiment
This experiment was designed with five temperatures of 22°C, 24°C, 26°C, 28°C, and 30°C. With 30 replicates (two to three eggs from the same batch per replicate) per treatment, a total of 150 NEST® glass-bottom cell culture dishes were set up. Eggs were incubated at designed temperatures under dark conditions. After 3 h of incubation per treatment, microscopic observations were made every 30 min, recording the incubation time until all eggs had hatched. The relationship between hatching time (D; h) and temperature (T; °C) in small copepods was fitted to Bělehrádek’s function (McLaren et al, 1969; Lee et al, 2003):
where A, B, and α are fitted constants.
2.2.3 Spawning Rhythm Experiment
To study the daily egg spawning rhythm of B. amoyensis, 36 replicates were set up, each with one pair of adults, and the paired adults were placed into six-well cell culture plates, one pair per well, and feed microalgae Isochrysis spp. at a concentration of 1 × 105 cells ml−1; the temperature and salinity were maintained at 26 ± 1°C and 28 ± 1 psu, respectively. Light intensity was 500 lx with a light/dark cycle of 12 h:12 h. Spawning rhythm experiment of B. amoyensis within 24 h was started at 8:00 a.m. The plates were examined microscopically at 2-h intervals, and the eggs were sucked out with an aforementioned arc pipette, the spawning time, and the number of eggs were recorded.
2.2.4 Egg Hatching Rate
Three environmental factors affecting incubation were set up, namely, light intensity, temperature, and salinity. Three light intensity treatments were 0, 500, and 1,000 lx, with a total of 45 replicates for each treatment (two to three eggs from the same batch per replicate), and eggs were collected in the same way as described previously. Salinity and temperature were the same as the stock culture (i.e., 26 ± 1°C, 28 ± 1 psu). Similarly, 10 temperature treatments of 16°C, 18°C, 20°C, 22°C, 24°C, 26°C, 28°C, 30°C, 32°C, and 34°C were set up, each with 45 replicates per treatment (two to three eggs from the same batch per replicate), incubated at the same salinity as the stock culture (28 ± 1 psu). In addition, seven salinity treatments of 22, 24, 26, 28, 30, 32, and 34 psu were set up with a total of 45 replicates, incubated at the same temperature as the stock culture (26 ± 1°C). The effect of temperature and salinity on incubation were conducted in dark conditions. The egg hatching rates (EHRs) of B. amoyensis under different designed regimes are presented in two categories: “Proportion of Hatching Normal Nauplii,” which included normal nauplii, and “Proportion of Hatching Abnormal Nauplii,” which included deformed nauplii (with twisted and distorted appendages and body). All eggs were kept in designed culture conditions for 24 h of observation until hatched. Unhatched eggs were moved into 26 ± 1°C, 28 ± 1 psu, dark condition after 24 h of experiment, and culturing was continued for another 24 h to make sure of the status of the egg.
2.3 Data Collection and Analysis
Data from all experiments were analyzed using one-way ANOVA. All data were tested for normality (Shapiro–Wilk test) and homogeneity of variance (Levene’s test) prior to analysis of ANOVA. When significant differences (p<0.05) were found, Tukey’s multiple comparisons test was performed to determine significant differences among treatments (p<0.05).
3 Results
3.1 Embryo Morphology of B. amoyensis
The whole embryonic development of B. amoyensis lasted, on average, 6 h and 40 min at 26°C. It was classified into seven main stages: fertilized eggs, cleavage stage, grastrulation, no structure visible, limb bud, early nauplii, and hatching. Percentages of embryonic stages during the embryo development of B. amoyensis was shown in Table 1.
3.1.1 Fertilized Eggs
The fertilized egg was about 71.74 μm in diameter, brownish yellow in color, and has two layers of membrane. The eggs, which sank to the bottom of the container after being laid, were long and elliptical in shape, very similar to the long strips shape of fecal pellet. They quickly absorbed water and became ellipsoidal in shape, turning into near-spherical shape a few minutes later (Figure 1A).
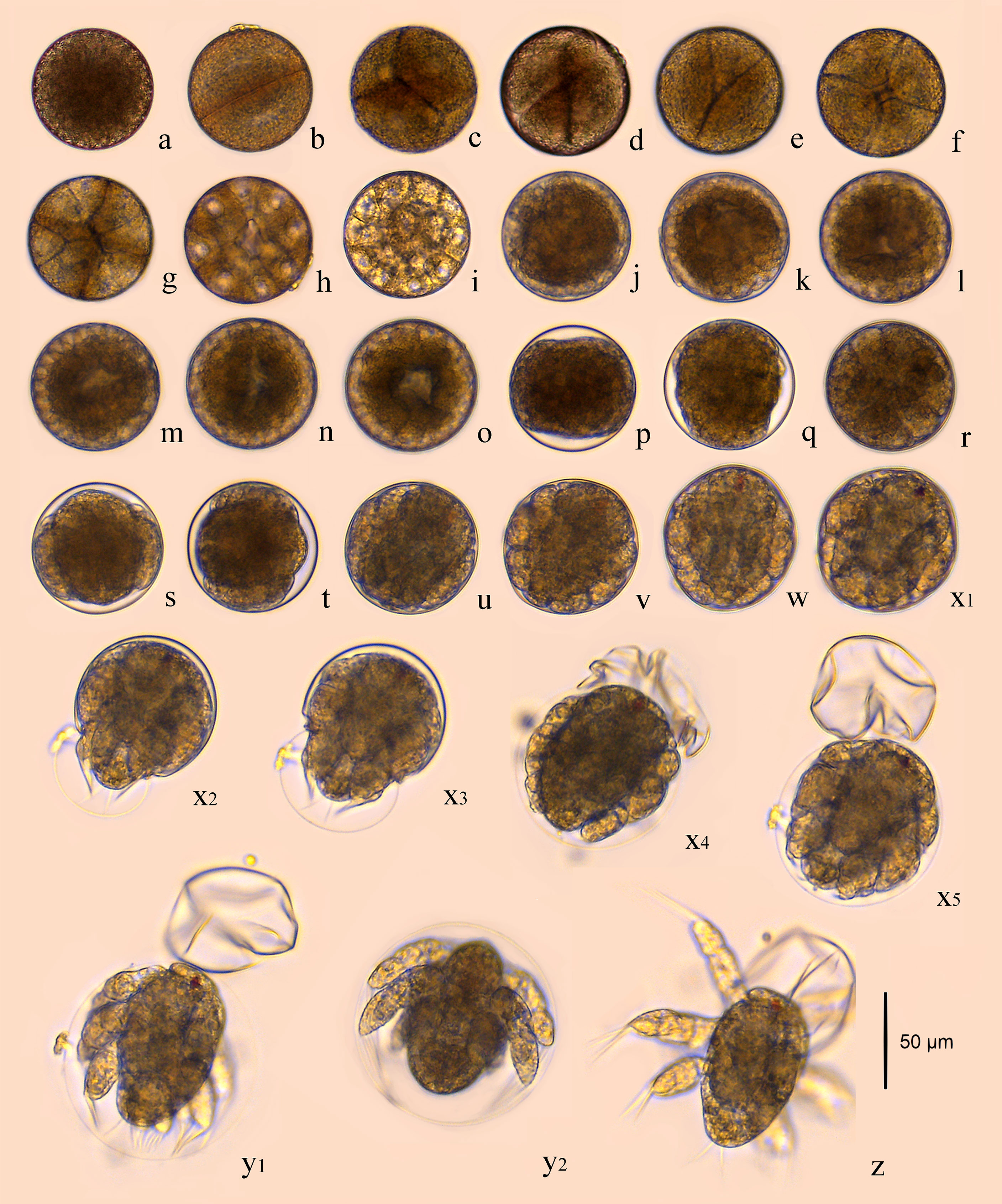
Figure 1 Embryonic development of B. amoyensis. (A), fertilized eggs; (B), 2-cell stage; (C–E), 4-cell stage; (F), 8-cell stage; (G), 16-cell stage; (H–I), multicellular phase; (J–O), grastrula stage; (P–Q), no structure visible; (R–T), limb bud stage; (U–W), the early stage of nauplii; (X1–X5), the rupture stage of outer membrane; (Y1–Y2), the rupture stage of inner membrane; (Z), the first stage of nauplii.
3.1.2 Cleavage Stage
The cleavage of B. amoyensis was total and equal to adequal. After the onset of oogenesis, the fertilized egg cells underwent mitosis in sequence, with the cleavage furrow clearly visible at each stage. The number of cells was increasing, and the nuclei were arranged in columns, showing the typical features of radial cleavage (Figures 1B–I).
The first cleavage occurred about 15 min (majority of 90%, Figure 1B) after the egg was deposited, followed by 4-cell, 8-cell, 16-cell, and multicellular stage at about 20, 25, 35, and 40 min after, respectively (majority of 80%, 55%, 90%, and 55%, Figures 1C–I). The first, second, and fourth cleavage divisions were meridional, while the third cleavage was equatorial and oriented perpendicularly to the previous cleavage. “I-section shaped” (Figure 1C), “X-section shaped” (Figure 1D), and “Herringbone” (Figure 1E) division furrow of second cleavage were obvious from different angles of view, while the division furrow of 8- and 16-cell resembled “cross-shaped” (Figure 1F) and “*” shaped (Figure 1G).
3.1.3 Gastrulation
The gastrulae were formed 1 h and 40 min after oviposition (majority of 55%, Figures 1J–O) when translucent area appeared at the edge of the egg, and the cells were continuously invaginated to form the archenteric cavity.
3.1.4 No Structure Visible
The stage of no structure visible was formed 4 h and 10 min after oviposition (100%, Figures 1P, Q), and the internal structure of the embryo was variable, with numerous transparent areas appearing on both sides (Figures 1P, Q).
3.1.5 Limb Bud Stage
The embryo developed into the early limb bud stage 1 h after of the stage of no structure visible, and the limb primordia was initially distinguishable, outlined with lateral invaginations between the three pairs of limbs in horizontal view (Figure 1R). At the late stage of limb bud, tubular structures were seen at the distal end of the limb (Figures 1S, T).
3.1.6 The Early Stage of Nauplii
The embryo assumed the typical nauplii configuration about 30 min after the limb bud stage. At this stage, a large amount of red pigment gathered toward the anterior end of the embryo, forming a red eyespot, with obvious segmentation of the appendages and a clear endwise furrow between the body and the appendages (Figures 1U–W).
3.1.7 Hatching Stage
This stage was formed 6 h and 10 min after oviposition (majority of 55%) and could be divided into the rupture stage of outer and inner membranes.
3.1.8 The Rupture Stage of Outer Membrane
This stage could be recognized by the intermittent twitch of appendages and the concentrations of muscles. The nauplii twitched on an average frequency of three to four times per minute initially. This twitching process could last for about 20–30 min, and it was not at a consistent speed; sometimes it twitched strongly, sometimes it stopped twitching. The frequency of twitching accelerated to once per 1 or 2 s near the rupture stage of the outer membrane (Figure 1X). The embryo ruptured the outer membrane from the posterior end (Figure 1X2). The transparent outer membrane slid and shrank backward, and the inner membrane, which enclosed the fully developed nauplii, extruded from the outer membrane, which took about 10 s (Figures 1X3–X5).
3.1.9 The Rupture Stage of Inner Membrane
The inner membrane was thinner and more transparent than the outer membrane. The appendages of nauplii stretched out and the inner membrane expanded; subsequently, the nauplii rapidly ruptured membrane from the anterior end of the body 70 s after the rupture of the outer membrane (Figures 1Y1, Y2). The nauplii remained stationary for about 10 s after hatching and then began to do skipping movements (Figure 1Z).
3.2 Effects of Temperature on Egg Hatching Time
The time required for hatching of viable eggs (exceed 50%) at various temperatures are shown in Figure 2. A Bělehrádek’s function was applied to describe the relationship between egg hatching time and the water temperature. By fitting the data between 22°C and 30°C, the following equation was derived:
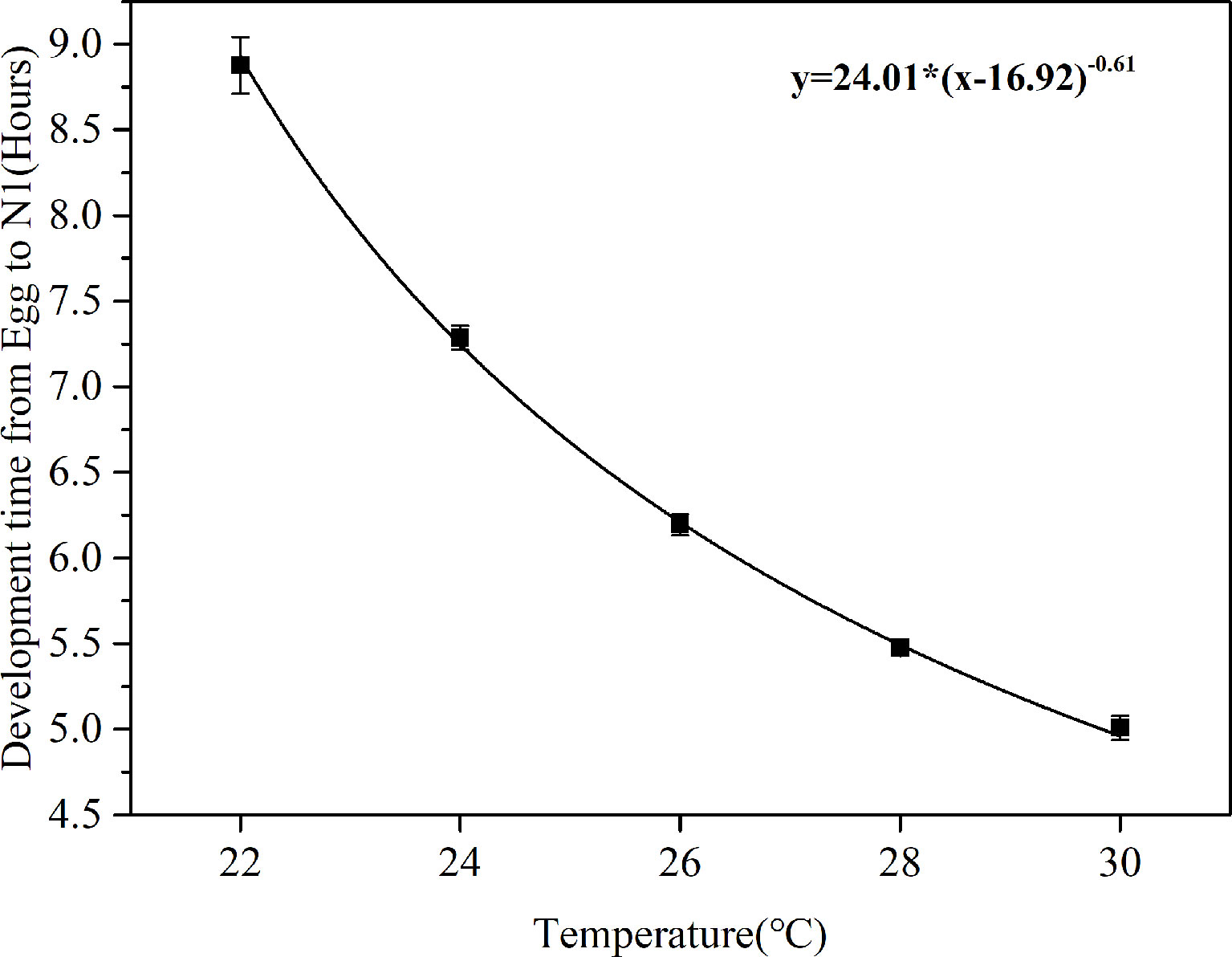
Figure 2 Correlation curves between egg development time (D) and water temperature (T) in B. amoyensis (N=30). Data are presented as mean± standard error (SE).
The physiological relationship between temperature and development time was obvious: water temperature significantly affected egg development time of B. amoyensis (p< 0.001, one-way ANOVA), and egg hatching time of B. amoyensis shortened exponentially with increasing temperature.
3.3 Spawning Rhythm
The fluctuations of egg production of B. amoyensis from 8:00 a.m. to next day 8:00 a.m. are shown in Figure 3. Copepods kept spawning during the 24 h of the whole experiment. Females usually spawn two eggs at a time, sometimes up to four, and the eggs are arranged in pairs. The maximum egg production was 44 eggs female−1 day−1, and the minimum egg production was 24 eggs female−1 day−1, with an average egg production of 33 eggs female−1 day−1. The peak of egg production of B. amoyensis presented after 2 h of dark; more than 54% of eggs spawn during dark period (p<0.001).
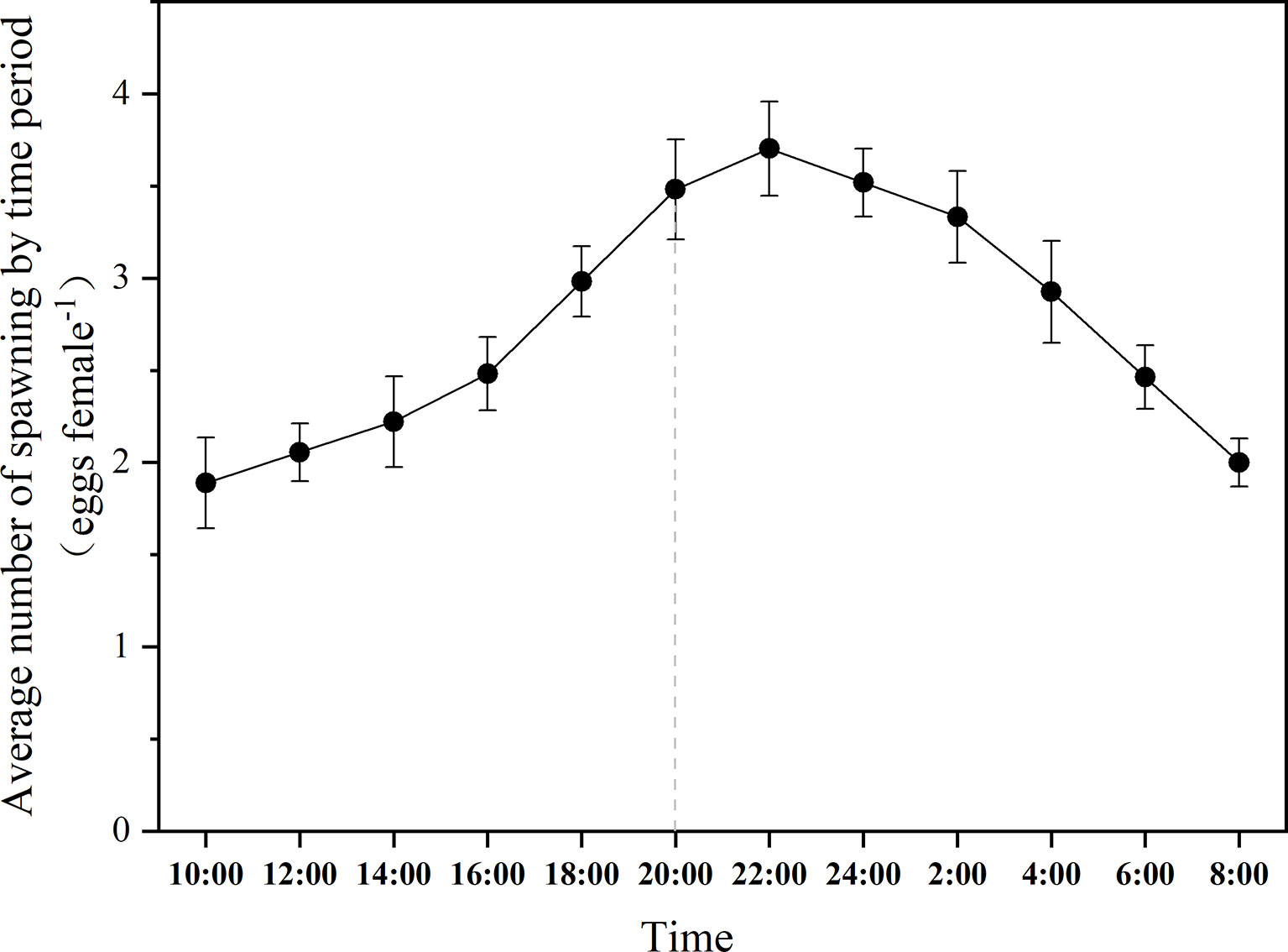
Figure 3 The daily spawning rhythm of B. amoyensis. Data are presented as mean± standard error (SE). The dotted line is the point in time when the dark period is entered.
3.4 Effects of Light Intensity, Temperature, and Salinity on Egg Hatching Rate
3.4.1 Effects of Light Intensity on Egg Hatching Rate
The EHRs of B. amoyensis are presented in Figure 4. Significant differences were detected under different light intensities, and the EHR of B. amoyensis decreased with increasing light intensity. The data were used to show the overall egg hatching rate, the rate of hatching normal nauplii, and deformed nauplii (p< 0.05, one-way ANOVA).
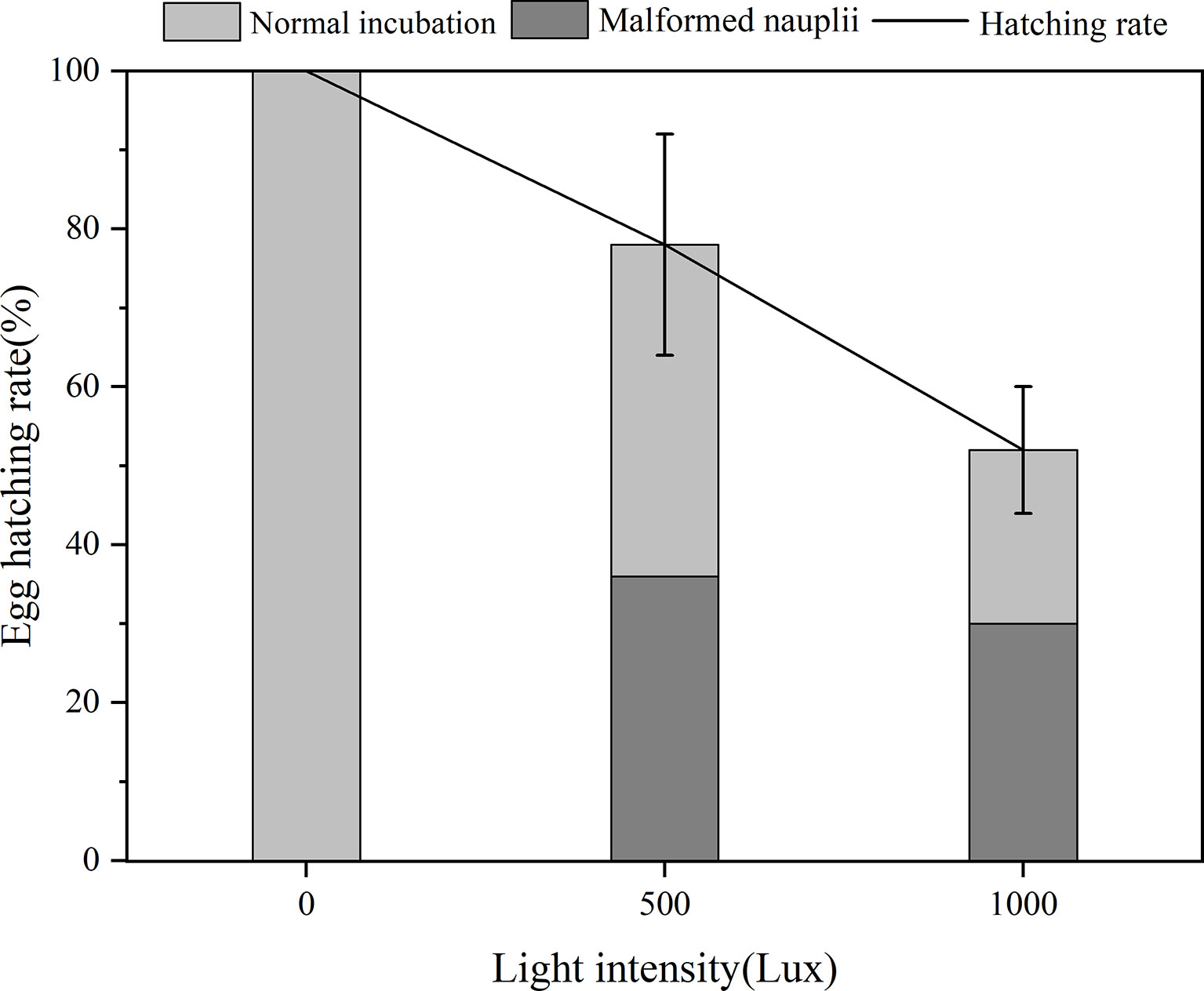
Figure 4 Egg hatching rate of B. amoyensis incubated at three different light intensities (N=45). Data are presented as mean ± standard error (SE).
There was >50% success in EHR across all treatments, and the highest EHR (100%) was recorded for the dark treatment, which was significantly higher (p< 0.01) than that of the 1,000 lx treatment. Malformed nauplii, which usually could not survive, were observed at both two treatments of 500 and 1,000 lx, and the ratio of malformed nauplii was 36% and 30%, respectively, which indicated that these two light intensities were detrimental to egg hatching of B. amoyensis and dark condition was favorable for the egg incubation of B. amoyensis. The percentage of normal nauplii from the 500 lx group was 42%, while in the 1,000 lx group, it was 22%. The unhatched embryos stopped developing and presented an abnormal shape, which were obviously dead.
3.4.2 Effects of Temperature on Egg Hatching Rate
Temperature significantly affected egg incubation (p< 0.01, one-way ANOVA). Hatching success of B. amoyensis eggs incubated at different temperatures was highest at a range of 24–28°C (Figure 5), with 100% of eggs hatched in all replicates, followed by 97% and 96% at 30°C and 22°C, respectively.
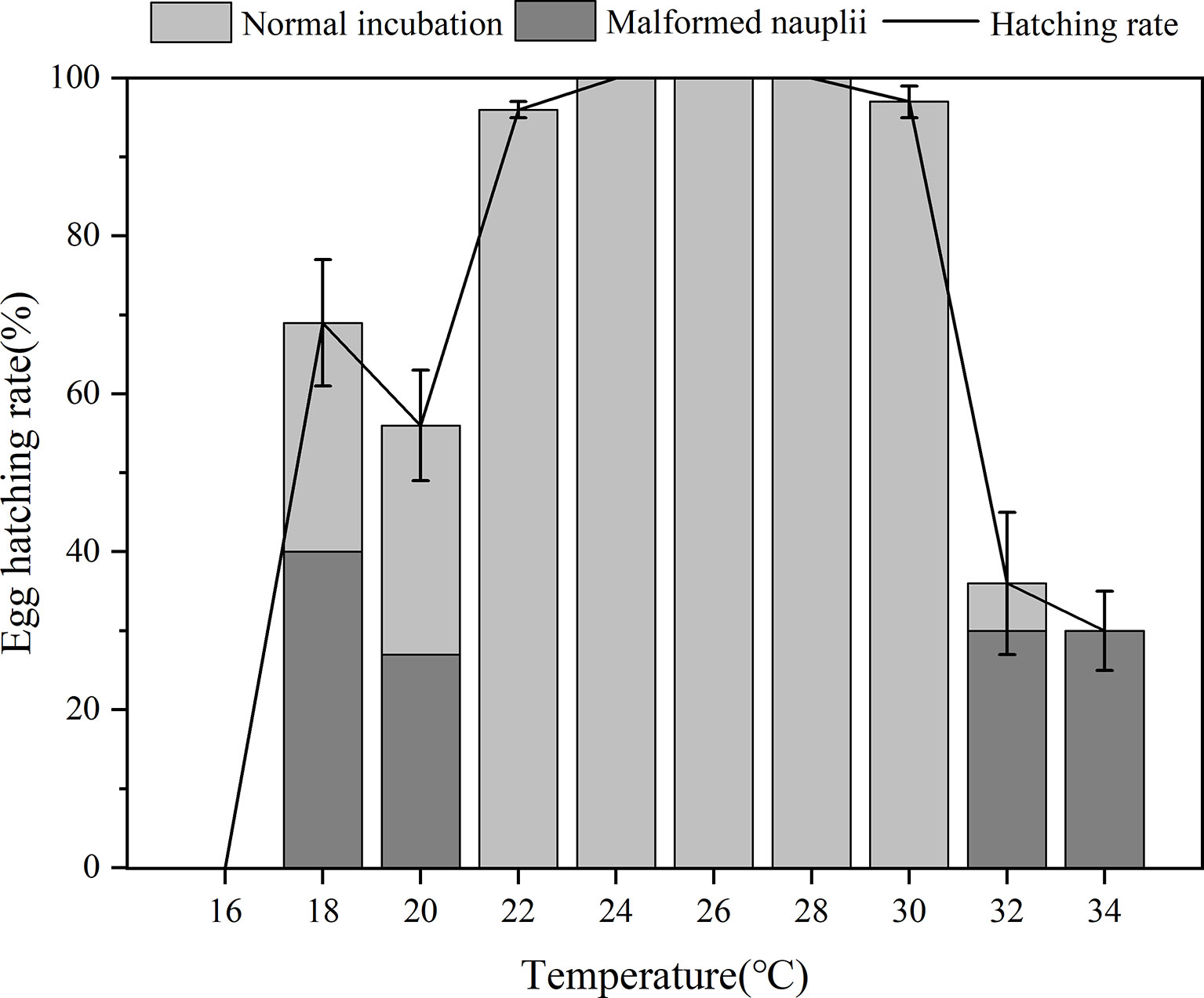
Figure 5 Egg hatching rate of B. amoyensis incubated at different temperatures(N=45). Data are presented as mean± standard error (SE).
Hatching percentages from 32°C (36%) and 34°C (30%) were significantly lower than that of other treatments (p< 0.01). Hatching success also was significantly lower (p< 0.01) from three temperatures lower than 22°C, which was 56%, 69%, and 0% at 20°C, 18°C, and 16°C, respectively (Figure 5). These results suggested that too low and too high temperatures would decrease EHR and lead to malformed nauplii. Hatching success at 22°C–30°C was above 94% for all (Figure 5), and no statistical differences were found among them, which indicated that this temperature range was optimum for the egg incubation of B. amoyensis. The percentage of malformed nauplii from 18°C, 20°C, 32°C, and 34°C was 40%, 20%, 30%, and 30%, respectively, while no malformed nauplii was found in the treatments of 22°C, 24°C, 26°C, and 28°C. The ratio of normal nauplii from 18°C, 20°C, 32°C, and 34°C was 29%, 29%, 6%, and 0%, respectively. Embryo development of the unhatched eggs stopped, and the abnormal shape obviously indicated that they were already dead.
3.4.3 Effects of Salinity on Egg Hatching Rate
Hatching success was not significantly different among the six salinity treatments (p > 0.05, one-way ANOVA), which were all above 88% and the hatching success reached 100% at 26–30 psu salinity treatments (Figure 6). Although a few malformed nauplii were hatched at salinity of 34 psu, the hatching rate from that salinity was still high and that of all replicates was above 96%. These results indicated that B. amoyensis eggs could hatch well within a salinity range of 22–34 psu.
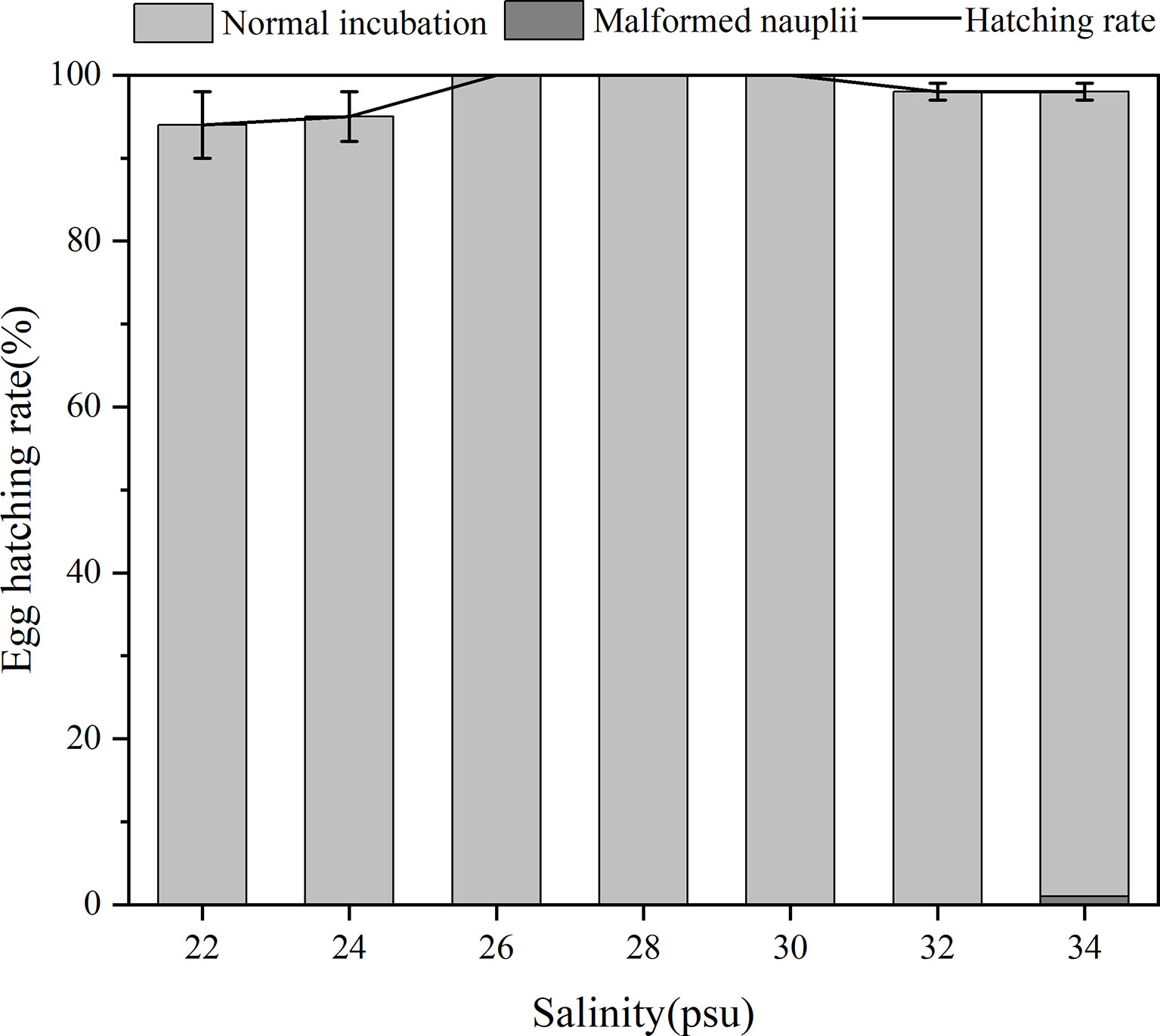
Figure 6 Egg hatching rate of B. amoyensis incubated at seven different salinities (N=45). Data are presented as mean± standard error (SE).
4 Discussion
Bestiolina amoyensis has a small egg (<75 μm) compared to other free-spawning copepods, such as Calanus spp., whose eggs are about 145–340 μm in diameter (Marshall and Orr, 1954; Marshall and Orr, 1955; Conover, 1967); that of Acartia spp. are about 77–78 μm (Marshall & Orr, 1954; Marshall and Orr, 1955; Nilsson & Hansen, 2018). Bestiolina amoyensis also has a demersal egg, which quickly sinks into the water after spawning, and the sinking egg facilitated its collection from the bottom of the culture vessel and the embryonic observation.
The embryonic development of copepods starts from the nuclear division of fertilized egg to the hatching of the first stages of nauplii, which are generally divided into different stages according to the external morphological features of embryos at different development process, such as blastomere division, the appearance of appendages, and the formation of the compound eyes and other organs. Similar to free-spawning calanoid copepods such as Calanus finmarchicus (Marshall & Orr, 1954; Marshall and Orr, 1955), Parvocalanus carssirostris (Yang, 1977), Centropages tenuiremis (Gao, 2014), Sinocalanus tenellus and Acartia spp. (Chen, 2014), and A. tonsa (Nilsson & Hansen, 2018), the embryonic development of B. amoyensis was divided into seven stages: newly laid eggs, cleavage stages (2-cell stage, 4-cell stage, 8-cell stage 16-cell stage, and multicellular stage), grastrulation, no structure visible, limb bud stage, early stage of nauplii, and hatching.
The early cleavage stages of copepods, although with more or less stereotyped patterns of blastomere divisions, have been largely neglected in copepod embryology (Loose and Scholtz, 2019). The cleavage of free-living copepods is total and equal to adequal (Loose and Scholtz, 2019), such as Calanus helgolandicus (Poulet et al., 1995), C. finmarchicus, C. pacificus, C. marshallae, Metridia spp. (Zirbel et al., 2007), A. tonsa (Nilsson and Hansen, 2018), and Skistodiaptomus spp. (Loose and Scholtz, 2019). Yang (1977) showed that the first and second cleavage of P. carssirostris were meridional, and the third cleavage was equatorial. Loose and Scholtz (2019) showed that the first and second cleavages of Skistodiaptomus spp. are both meridional; the third cleavage is equatorial, forming a total of four smaller and four larger blastomeres; and the fourth and fifth cleavage are asynchronous. The cleavage of B. amoyensis is similar to the above-mentioned free-spawning copepod in that the first cleavages are meridional, bisecting the two blastomeres, which are approximately equal in size. Furthermore, the second cleavages are meridional, the third cleavage is equatorial, the fourth cleavages are meridional, and the fifth cleavages are difficult to distinguish under light microscopy due to the small size of the blastomere.
The early cleavage stage of B. amoyensis developed very fast at 26°C. It took 30 min from spawning to the fourth cleavage stage, which was very similar with P. carssirostris, in which it took 28 min from newly laid eggs to the fourth cleavage stage at 29°C–30°C (Yang, 1977). The duration of the 2-, 4-, 8-, and 16-cell stages of B. amoyensis was short and almost isochronous, taking about 5 min each at 26°C. In addition, the two-, four-, and eight-cell stages of early cleavage in C. tenuiremis at 20°C also approximated isochronous development, which was 0.8, 1.0, and 0.9 h, respectively (Gao, 2014). These results indicated that, to some extent, the duration of the early cleavage stage of copepods may be approximately isochronous. The relative long early cleavage duration of C. tenuiremis mainly related to the much lower embryo incubation temperature, which was 20°C for C. tenuiremis, while for B. amoyensis and P. carssirostris, the incubation temperature was 26°C and 29°C–30°C, respectively.Some studies stained embryonic nucleus to observe the cleavage (Poulet et al., 1995; Nilsson & Hansen, 2018; Loose and Scholtz, 2019), while those copepods typically had either large eggs or hard eggshells, facilitating the manipulation of the staining process. We tried hard to stain the embryo of B. amoyensis but failed. The relatively small (<75 µm) size and the thin egg membranes made it very difficult to manipulate and easy to lose during the staining process. In addition, the eggs of Parvocalanus aculeatus and P. parvus not only have a thin egg membrane that is easily ruptured but also are transparent, which make the observation difficult. These might be the reasons why the study of copepod embryology is somewhat neglected.It is noteworthy that the nauplius of almost all species started twitching shortly before hatching (Marshall & Orr, 1954; Marshall and Orr, 1955). Although the eggs of numerous copepods hatched in much the same way (Marshall & Orr, 1954; Marshall and Orr, 1955), studies and specific descriptions of copepod hatching need to be refined, and even further studies are needed to determine whether the hatching patterns are the same. Marshall & Orr (1954) stated that in free-spawning copepods such as Calanus spp., Metridia spp., and Acartia spp., the embryonic inner membrane was completely separated from the outer membrane. Several studies (Marshall & Orr, 1954; Marshall and Orr, 1955; Yang, 1977; Chen, 2014) indicated that copepod eggs had both inner and outer membranes. Separation of the outer membrane from the inner membrane was also observed in B. amoyensis, and the inner membrane was bulged out of the outer membrane. This is similar to the description of the incubation process by Davis (1959), who examined the freshwater copepod Diaptomus asblandi, Diaptomus siciloides, Cyclops bicuspidatus, and Mesocyclops edax, where the outer membrane was ruptured by pressure from the inner membrane, while the inner membrane was expanded by the osmotic entry of water.It had been observed that the nauplii of B. amoyensis, B. similis, and P. carssirostris all broke the outer membrane from the tail end during hatching, which was consistent with the description by Marshall and Orr (1954), who proposed that the nauplius usually extrudes from the head or tail end during the process of rupturing the outer membrane. Marshall and Orr (1954) also found that although some nauplii also could extrude out from lateral side of the limbs occasionally, it usually leads to hatching failure. When nauplii just got rid of inner membranes, they often did not start swimming immediately; they usually remained stationary for 10–20 s and then swam off (Marshall & Orr, 1954; Marshall and Orr, 1955), and this phenomenon was also observed in B. amoyensis.In an environment where food is not a limiting factor, the temperature is the main environmental factor regulating the development time of copepods (Ban, 1994; Anzueto-Sánchez et al., 2014), and temperature changes could increase or decrease the hatching time of eggs (Castro-Longoria, 1998; Chinnery & Williams, 2004). Previous investigations indicated that the development time of copepod decreased with increasing temperature (Ozaki & Ikeda, 1997; Chinnery & Williams, 2004), as does the hatching time of B. amoyensis eggs, which shortened progressively as temperature increased at a range of 22°C–30°C.Light is one of the most important structuring factors for life in aquatic biology (Radhakrishnan et al., 2020). There are some evidence suggesting that subitanous and/or dormant eggs of various invertebrates (e.g., crustaceans, insects, and rotifers) can exhibit photoreception (Hagiwara & Hino, 1989; Itoh & Sumi, 2000; Blackmer et al., 2002). Photic stimulations represent important extrinsic factors controlling aspects of reproduction in many marine invertebrates (Moraitou-Apostolopoulou & Verriopoulos, 1982). A majority of studies reported that light could induce hatching in aquatic invertebrates (Takahashi, 1977; Mitchell, 1990; Hagiwara et al.,1995; Murugan & Dumont, 1995). Conversely, the present results indicated that too much light (500 and 1,000 lx) inhibited the hatching of B. amoyensis eggs. Hagemann et al. (2016) also found a highly negative effect of light on EHR of cold storage eggs of A. tonsa and noted that light hindered the oxygen diffusion across the eggshell, resulting in a high bacterial load that prevented the eggs from hatching. Although the exact cause of the negative effects of light on EHR of B. amoyensis still needs further experiment, the present hindrance to the hatching of B. amoyensis eggs might be related to its nocturnal spawning rhythm. Marine calanoid copepods had a distinct diurnal spawning rhythm, and Calanus spp. (Harding et al., 1951) and P. crassirostris (Sun, 2008) were mostly nocturnal. The daily spawn rhythms of B. amoyensis (500 lx, 12L:12D) were observed. The peak of egg production of B. amoyensis presented after 2 h of dark; more than 54% of eggs spawned during dark conditions (p<0.001). We also cultured B. amoyensis under light intensity of 4,000–5,000 lx and found that over 90% of the eggs produced during dark period. These characteristics indicated that B. amoyensis was a nocturnal spawning species, and high levels of light intensities (500 and 1,000 lux in the present experiment) were detrimental for its embryo development. Unfortunately, there is very little reference involving the effects of light intensity on embryonic development of crustacean. Liang and Chen (2010) reported that Panulirus ornatus eggs should incubated in a light intensity<200 lx; lots of malformation were observed when light intensity was higher than 500 lx. Further study is needed to explore the effect of light on the malformation.Unlike Acartia species, whose eggs are hard and with an opaque egg outer membrane, B. amoyensis has transparent and thin egg membrane. Considering the mode of action of light, it could act directly on germ cells by passing through the integument in transparent or translucent organisms (Moraitou-Apostolopoulou & Verriopoulos, 1982). Most marine copepods including B. amoyensis, B. similis, P. parvus, Calanus spp. (Marshall & Orr, 1954; Marshall and Orr, 1955), and P. carsirostris (Yang, 1977) have transparent egg membranes and nocturnal spawning habits, and thus, the effects of light on their hatching could be direct. If such nocturnally laid eggs are incubated under light conditions, the light might directly affect the embryo through the transparent egg membrane, altering the rhythm of long-adapted dark incubation, which placed the embryo in a state of stress, further leading to abnormal embryonic development and malformed nauplii. This would account why more than 30% malformed B. amoyensis nauplii were recorded under 500 and 1,000 lx of light intensity. Meanwhile, light condition, i.e., photoperiod and light intensity, can be easily manipulated with minimal costs in larviculture (Chinnery & Williams, 2003). For B. amoyensis, farmers could incubate the eggs during the night, which ensures that large numbers of nauplii could be collected the next daytime to timely meet the prey requirements of marine fish larvae.
Temperature and salinity are the two most important environmental parameters affecting the seasonal and spatial distribution of marine copepods in the wild (Miller & Marcus, 1994). Copepods like P. crassirostris, A. clausi, and P. parvus (Li et al., 2001; Yang, 2007; Lian et al., 2018), living in nearshore and warm water areas, are a group of high temperature and low salinity species; surface water temperatures of their natural waters are generally >20°C (Sun et al., 2014), and the distribution salinity range are approximately within 20–31.7 psu (Huang & Zheng, 1984). Bestiolina amoyensis usually lives in coastal and estuarine waters (Wang et al., 2021), where it adapts to a temperature range of 14°C–29.5°C and a salinity range of 16.6–30.7 psu in nature. Larger numbers of B. amoyensis were reported when salinity was higher than 25 psu (Li & Huang, 1984). The hatching success of B. amoyensis at 22°C–30°C, i.e., within the acclimation temperature range of their natural distribution area, was above 94% in the present experiment. Moreover, the hatching success reached 100% at salinities of 26–30 psu, which was consistent with the larger distribution populations at salinity >25 psu (Li & Huang, 1984). All of the above indicate that B. amoyensis is a nearshore and warm-water species.
Temperature and salinity exceeding suitable range caused malformation of nauplii of B. amoyensis. Planktonic copepods maintain large free amino acid pools, such as C. finmarchicus (Cowey & Corner, 1963) and A. tonsa (Alzara, 1968; Farmer & Reeve, 1978), and catabolism of free amino acids is important in the regulation of cellular osmotic pressure exposed to low and high salinity (Farmer & Reeve, 1978). Previous research also indicated that prolonged exposure to salinity and temperature stress enhanced protein absence (Gonzalez & Bradley, 1994; Kimmel & Bradley, 2001). Free amino acid would be catabolized to cope with the temperature and salinity stress and increase the consumption of protein, which might lead to abnormal embryonic development and malformed nauplii.
5 Conclusion
The embryonic development process of B. amoyensis is similar to other calanoid copepods including seven sequential stages: fertilized eggs, cleavage stage, gastrulation, no structure visible stage, limb bud stage, early nauplii, and hatching. The egg hatching time of B. amoyensis shortened exponentially with the increasing temperature. The optimum light intensity, temperature, and salinity ranges for the egg hatching of B. amoyensis was 0 lx, 22°C–30°C, and 22–34 psu, respectively.
Data Availability Statement
The original contributions presented in the study are included in the article/supplementary material. Further inquiries can be directed to the corresponding author.
Author Contributions
SW and LW conceived the original idea of this study and designed the experiments. LW conducted the experiments and collected the data. LW, SW, and YC performed the data analyses. LW and YW wrote the draft of the article and revised it with SW. SW supervised the research. All authors contributed to the article and approved the submitted version.
Funding
This work was contribution to the Regional Demonstration Project of the 13th Five-Year Plan of Marine Economy Innovation & Development in Xiamen (funded by the State Oceanic Administration, People’s Republic of China, grant no. 16PZY002SF18).
Conflict of Interest
The authors declare that the research was conducted in the absence of any commercial or financial relationships that could be construed as a potential conflict of interest. The handling editor CZ declared a past co-authorship with the authors SW, LW, and YW.
Publisher’s Note
All claims expressed in this article are solely those of the authors and do not necessarily represent those of their affiliated organizations, or those of the publisher, the editors and the reviewers. Any product that may be evaluated in this article, or claim that may be made by its manufacturer, is not guaranteed or endorsed by the publisher.
Acknowledgments
We thank researcher Changshou Zhu from Fujian Institute of Oceanography for professionally guiding the identification of B. amoyensis.
References
Alajmi F., Zeng C. (2015). Evaluation of Microalgal Diets for the Intensive Cultivation of the Tropical Calanoid Copepod, Parvocalanus Crassirostris. Aquac. Res. 46, 1025–1038. doi: 10.1111/are.12254
Alzara L. (1968). Survey of Free Amino Acids in Copepod Populations (Kingston: University of Rhode Island), 97 pp.
Anzueto-Sánchez M. A., Barón-Sevilla B., Cordero-Esquivel B., Celaya-Ortega A. (2014). Effects of Food Concentration and Temperature on Development, Growth, Reproduction and Survival of the Copepod Pseudodiaptomus Euryhalinus. Aquac. Int. 22, 1911–1923. doi: 10.1007/s10499-014-9791-5
Ban S. (1994). Effect of Temperature and Food Concentration on Post-Embryonic Development, Egg Production and Adult Body Size of Calanoid Copepod Eurytemora Affinis. J. Plankton Res. 16, 721–735. doi: 10.1093/plankt/16.6.721
Blackmer J. L., Lee L. L., Henneberry T. J. (2002). Factors Affecting Egg Hatch, Development, and Survival of Bemisia Argentifolii (Homoptera: Aleyrodidae) Reared on an Artificial Feeding System. Environ. Entomol. 31, 306–312. doi: 10.1603/0046-225X-31.2.306
Camus T., Rolla L., Jiang J., Zeng C. (2021). Effects of Microalgal Food Quantity on Several Productivity-Related Parameters of the Calanoid Copepod Bestiolina Similis (Calanoida: Paracalanidae). Front. Mar. Sci. 8. doi: 10.3389/fmars.2021.812240
Camus T., Zeng C., & (2010). Roles of Microalgae on Total Egg Production Over Female Lifespan and Egg Incubation Time, Naupliar and Copepodite Survival, Sex Ratio and Female Life Expectancy of the Copepod Bestiolina similis. Aquac. Res. 41, 1717-1726. doi: 10.1111/j.1365-2109.2010.02565.x
Camus T., Zeng C., McKinnon A. D. (2009). Egg Production, Egg Hatching Success and Population Increase of the Tropical Paracalanid Copepod, Bestiolina Similis (Calanoida: Paracalanidae) Fed Different Microalgal Diets. Aquaculture 297, 169–175. doi: 10.1016/j.aquaculture.2009.09.018
Castro-Longoria E. (1998). Seasonal and Spatial Distribution Patterns of the Congeneric Group Acartia in the Solent–Southampton Water Estuarine System, With Special Reference to Aspects of Their Fecundity (Southampton: University of Southampton).
Castro-Longoria E. (2003). Egg Production and Hatching Success of Four Acartia Species Under Different Temperature and Salinity Regimes. J. Crustac. Biol. 23, 289–299. doi: 10.1163/20021975-99990339
Chen L. D. (2014). Preliminary Study on Ecology of Zooplankton Resting Eggs in Typical Waters of Pearl River Estuary and the Eastern Guangdong Coast (Guangzhou: Jinan University).
Chinnery F. E., Williams J. A. (2003). Photoperiod and Temperature Regulation of Diapause Egg Production in Acartia Bifilosa From Southampton Water. Mar. Ecol. Prog. 263, 149–157. doi: 10.3354/meps263149
Chinnery F. E., Williams J. A. (2004). The Influence of Temperature and Salinity on Acartia (Copepoda: Calanoida) Nauplii Survival. Mar. Biol. 145, 733–738. doi: 10.1007/s00227-004-1354-2
Choi S. Y., Jeon S. C., Soh H. Y. (2022). Effects of Cold Storage and Salinity on Acartia Sinjiensis (Copepoda: Calanoida) Egg Hatching. Aquac. Res., 53, 3568–3574. 1–7. doi: 10.1111/are.15861
Choi S. Y., Lee E. H., Soh H. Y., Jang M. C. (2021). Effects of Temperature and Salinity on Egg Production, Hatching, and Mortality Rates in Acartia Ohtsukai (Copepoda, Calanoida). Front. Mar. Sci. 8. doi: 10.3389/fmars.2021.704479
Conover R. J. (1967). Reproductive Cycle, Early Development, and Fecundity in Laboratory Populations of the Copepod Calanus Hyperboreus. Crustaceana 13 (1), 61–72. doi: 10.1163/156854067X00080
Cowey C. B., Corner E. D. S. (1963). Amino Acids and Some Other Nitrogenous Compounds in Calanus Finmarchicus. J. Mar. Biol. Ass. U.K. 43, 485–493. doi: 10.1017/S0025315400000461
Davis C. C. (1959). Osmotic Hatching in the Eggs of Some Fresh-Water Copepods. Biol. Bull. 116, 15–29. doi: 10.2307/1539152
Devreker D., Souissi S., Winkler G., Forget-Leray J., Leboulenger F. (2009). Effects of Salinity, Temperature and Individual Variability on the Reproduction of Eurytemora Affinis (Copepoda; Calanoida) From the Seine Estuary: A Laboratory Study. J. Exp. Mar. Biol. Ecol. 368, 113–123. doi: 10.1016/j.jembe.2008.10.015
Drillet G., Frouël S., Sichlau M. H., Jepsen P. M., Højgaard J. K., Joarder A. K., et al. (2011). Status and Recommendations on Marine Copepod Cultivation for Use as Live Feed. Aquaculture 315, 155–166. doi: 10.1016/j.aquaculture.2011.02.027
Engel M., Hirche H. J. (2004). Seasonal Variability and Inter-Specific Differences in Hatching of Calanoid Copepod Resting Eggs From Sediments of the German Bight (North Sea). J. Plankton Res. 26, 1083–1094. doi: 10.1093/plankt/fbh099
Farmer L., Reeve M. R. (1978). Role of the Free Amino Acid Pool of the Copepod Acartia Tonsa in Adjustment to Salinity Change. Mar. Biol. 48, 311–316. doi: 10.1007/BF00391634
Fernández-Ojeda C., Muniz M. C., Cardoso R. P., dos Anjos R. M., Huaringa E., Nakazaki C., et al. (2021). Plastic Debris and Natural Food in Two Commercially Important Fish Species From the Coast of Peru. Mar. pollut. Bull. 173, 113039. doi: 10.1016/j.marpolbul.2021.113039
Gao Q. J. (2014). Study on Embryonic Development of Centropages Tenuiremis (Xiamen: Xiamen University).
Gaudy R., Cervetto G., Pagano M. (2000). Comparison of the Metabolism of Acartia Clausi and A. Tonsa: Influence of Temperature and Salinity. J. Exp. Mar. Biol. Ecol. 247, 51–65. doi: 10.1016/S0022-0981(00)00139-8
Gonzalez C. R., Bradley B. P. (1994). “Salinity Stress Proteins in Eurytemora Affinis0,” in Ecology and Morphology of Copepods (Dordrecht: Springer), 461–468.
Hagemann A., Øie G., Evjemo J. O., Olsen Y. (2016). Effects of Light and Short-Term Temperature Elevation on the 48-H Hatching Success of Cold-Stored Acartia Tonsa Dana Eggs. Aquac. Int. 24, 57–68. doi: 10.1007/s10499-015-9908-5
Hagiwara A., Hino A. (1989). Effect of Incubation and Preservation on Resting Egg Hatching and Mixis in the Derived Clones of the Rotifer Brachionus Plicatilis. Hydrobiologia 186 (187), 415–421. doi: 10.1007/BF00048940
Hagiwara A., Hoshi N., Kawahara F., Tominaga K., Hirayama K. (1995). Resting Eggs of the Marine Rotifer Brachionus Plicatilis Müller: Development, and Effect of Irradiation on Hatching. Hydrobiologia 313, 223–229. doi: 10.1007/BF00025955
Hairston N. G., Kearns C. M. (1995). The Interaction of Photoperiod and Temperature in Diapause Timing: A Copepod Example. Biol. Bull. 189, 42–48. doi: 10.2307/1542200
Harding J. P., Marshall S. M., Orr A. P. (1951). Time of Egg-Laying in the Planktonic Copepod Calanus. Nature 167, 953–953. doi: 10.1038/167953a0
Huang Q. S., Zheng Z. (1984). The Relation of Copepods to Salinity in the Estuary of Jiulong River. J. Xiamen Univ. (Natural Science) 23, 497–505.
Itoh M. T., Sumi Y. (2000). Circadian Clock Controlling Egg Hatching in the Cricket (Gryllus Bimaculatus). J. Biol. Rhythms. 15, 241–245. doi: 10.1177/074873040001500305
Jang M. C., Shin K., Hyun B., Lee T., Choi K. H. (2013). Temperature-Regulated Egg Production Rate, and Seasonal and Interannual Variations in Paracalanus Parvus. J. Plankton Res. 35 (5), 1035–1045. doi: 10.1093/plankt/fbt050
Karlsson K., Puiac S., Winder M. (2018). Life-History Responses to Changing Temperature and Salinity of the Baltic Sea Copepod Eurytemora Affinis. Mar. Biol. 165, 1–11. doi: 10.1007/s00227-017-3279-6
Kimmel D. G., Bradley B. P. (2001). Specific Protein Responses in the Calanoid Copepod Eurytemora Affinis (Popp) to Salinity and Temperature Variation. J. Exp. Mar. Biol. Ecol. 266, 135–149. doi: 10.1016/S0022-0981(01)00352-5
Kline M. D., Laidley C. W. (2015). Development of Intensive Copepod Culture Technology for Parvocalanus Crassirostris: Optimizing Adult Density. Aquaculture 435, 128–136. doi: 10.1016/j.aquaculture.2014.09.022
Landry M. R. (1975). The Relationship Between Temperature and the Development of Life Stages of the Marine Copepod Acartia Clausi Giesbr. 1. Limnol. Oceanogr. 20, 854–857. doi: 10.4319/lo.1975.20.5.0854
Lee H. W., Ban S., Ikeda T., Matsuishi T. (2003). Effect of Temperature on Development, Growth and Reproduction in the Marine Copepod Pseudocalanus Newmani at Satiating Food Condition. J. Plankton Res. 25, 261–271. doi: 10.1093/plankt/25.3.261
Li S. J., Huang J. Q. (1984). On Two New Species of Planktonic Copepoda From the Estuary of Jiulong River, Fujian, China. J. Xiamen Univ. Nat. Sci. 3, 381–390
Li S. J., Xu Z. Z., Huang J. Q., Chao W. Q., Chen G., Ke C. H., et al. (2001). Studies on Biology of Marine Zooplankton in China. J. xiamen Univ. Natural Sci. 40, 585–590.
Liang H. F., Chen J. F. (2010)Effects of Four Ecological Factors on Embryonic Development of Panulirus Ornatus. Available at: https://xueshu.baidu.com/usercenter/paper/show?paperid=ecac02515a9e7dc7a30541a74a1d062b&site=xueshu_se&hitarticle=1 (Accessed June 3, 2022).
Lian G. S., Wang Y. G., Sun R. X., Hwang J. S. (2018). Species Diversity of Marine Planktonic Copepods in China’s Seas (Beijing: China Ocean Press). doi: 10.3724/SP.J.1003.2011.11130
Loose G., Scholtz G. (2019). The Cleavage Pattern of Calanoid Copepods—a Case Study. Dev. Genes Evol. 229, 103–124. doi: 10.1007/s00427-019-00634-8
Marcus N. (2005). “Calanoid Copepods, Resting Eggs, and Aquaculture,” in Copepods in Aquaculture. Eds. Cheng-Sheng L., O’Bryen P. J., Marcus N. H. (Melbourne: Blackwell), 3–9.
Marshall S. M., Orr A. P. (1954). Hatching in Calanus Finmarchicus and Some Other Copepods. J. Mar. Biolog. Assoc. U.K. 33, 393–401. doi: 10.1017/S0025315400008432
Marshall S. M., Orr A. P. (1955). The Biology of a Marine Copepod: Calanus Finmarchicus (Gunnerus) (London: Oliver and Boyd).
McKinnon A. D., Duggan S., Nichols P. D., Rimmer M. A., Semmens G., Robino B. (2003). The Potential of Tropical Paracalanid Copepods as Live Feeds in Aquaculture. Aquaculture 223, 89–106. doi: 10.1016/S0044-8486(03)00161-3
McLaren I. A., Corkett C. J., Zillioux E. J. (1969). Temperature Adaptations of Copepod Eggs From the Arctic to the Tropics. Biol. Bull. 137, 486–493. doi: 10.2307/1540170
Milione M., Zeng C. (2008). The Effects of Temperature and Salinity on Population Growth and Egg Hatching Success of the Tropical Calanoid Copepod, Acartia Sinjiensis. Aquaculture 275, 116–123. doi: 10.1016/j.aquaculture.2007.12.010
Miller D. D., Marcus N. H. (1994). The Effects of Salinity and Temperature on the Density and Sinking Velocity of Eggs of the Calanoid Copepod Acartia Tonsa Dana. J. Exp. Mar. Biol. Ecol. 179, 235–252. doi: 10.1016/0022-0981(94)90117-1
Mitchell S. A. (1990). Factors Affecting the Hatching of Streptocephalus Macrourus Daday (Crustacea; Eubranchiopoda) Eggs. Hidrobiological 194, 13–22. doi: 10.1007/BF00012108
Moraitou-Apostolopoulou M., Verriopoulos G. (1982). Influence of Light Conditions on the Offspring Production and the Sex Ratio of Ti’sbe Holothuriae, Humes (Copepoda, Harpacticoida). Arch. Hydrobiol. 96, 120–127.
Murugan G., Dumont H. J. (1995). Influence of Light, DMSO and Glycerol on the Hatchability of Thamnocephalus Platyurus Packard Cysts. Hidrobiological 298, 175–178. doi: 10.1007/978-94-011-0291-9_16
Nagaraj M. (1992). Combined Effects of Temperature and Salinity on the Development of the Copepod Eurytemora Affinis. Aquaculture 103, 65–71. doi: 10.1016/0044-8486(92)90279-T
Nilsson B., Hansen B. W. (2018). Timing of Embryonic Quiescence Determines Viability of Embryos From the Calanoid Copepod, Acartia Tonsa (Dana). PLos One 13, e0193727. doi: 10.1371/journal.pone.0193727
Ozaki K., Ikeda T. (1997). The Effect of Temperature on the Development of Eggs and Nauplii of the Mesopelagic Copepod Paraeuchaeta Elongata. Plankton Biol. Ecol. 44, 91–95.
Pan Y. J., Dahms H. U., Hwang J. S., Souissi S. (2022). Recent Trends in Live Feeds for Marine Larviculture: A Mini Review. Front. Mar. Sci. 9. doi: 10.3389/fmars.2022.864165
Peck M. A., Ewest B., Holste L., Kanstinger P., Martin M. (2008). Impacts of Light Regime on Egg Harvests and 48-H Egg Hatching Success of Acartia Tonsa (Copepoda: Calanoida) Within Intensive Culture. Aquaculture 275, 102–107. doi: 10.1016/j.aquaculture.2007.12.008
Peck M. A., Holste L. (2006). Effects of Salinity, Photoperiod and Adult Stocking Density on Egg Production and Egg Hatching Success in Acartia Tonsa (Calanoida: Copepoda): Optimizing Intensive Cultures. Aquaculture 255, 341–350. doi: 10.1016/j.aquaculture.2005.11.055
Poulet S. A., Laabir M., Ianora A., Miralto A. (1995). Reproductive Response of Calanus Helgolandicus. I. Abnormal Embryonic and Naupliar Development. Mar. Ecol. Prog. 129, 85–95. doi: 10.3354/meps129085
Radhakrishnan D. K., AkbarAli I., Sathrajith A. T., Schmidt B. V., Sivanpillai S., Vasunambesan S. T. (2020). Grazing Rates of Freshwater Copepod Thermocyclops Decipiens (Kiefe) on Chlorella Vulgaris Under Different Light Intensities. Aquaculture 525, 735321. doi: 10.1016/j.aquaculture.2020.735321
Roddie B. D., Leakey R. J. G., Berry A. J. (1984). Salinity-Temperature Tolerance and Osmoregulation in Eurytemora Affinis (Poppe)(Copepoda: Calanoida) in Relation to its Distribution in the Zooplankton of the Upper Reaches of the Forth Estuary. J. Exp. Mar. Biol. Ecol. 79, 191–211. doi: 10.1016/0022-0981(84)90219-3
Santhanam P., Jeyaraj N., Jothiraj K., Ananth S., Kumar S. D., Pachiappan P. (2019). “Assessing the Efficacy of Marine Copepods as an Alternative First Feed for Larval Production of Tiger Shrimp Penaeus Monodon,” in Basic and Applied Zooplankton Biology. Eds. Santhanam P., Begum A., Pachiappan P. (Singapore: Springer), 293–303. doi: 10.1007/978-981-10-7953-5_12
Souissi A., Souissi S., Hwang J. S. (2016). Evaluation of the Copepod Eurytemora Affinis Life History Response to Temperature and Salinity Increases. Zool. Stud. 55, e4. doi: 10.6620/ZS.2016.55-04
Sun X. (2008). Population Dynamics of Small Copepod (Qingdao: Institute of oceanology, Chinese Academy of Sciences).
Sun R., Wang Y., Lian G., Lin M. (2014). Distribution and Community Characteristics of Planktonic Copepods in the Northwest Coastal Waters Off Hainan Island. Biodiv. Sci. 22, 320–328. doi: 10.3724/SP.J.1003.2014.13137
Takahashi F. (1977). Pioneer Life of the Tadpole Shrimps, Triops Spp. (Notostraca: Triopsidae). Appl. Entomol. Zool. 12, 104–117.
Valencia G. A. T., Merino G. E., Prieto-Guevara M. J., Portillo J. E. A., Arboleda J. E. L., Chapman F. A. (2022). Spawning Parvocalanus Crassirostris at a High Adult Density: Explaining Low Adult Population Numbers and Means for Improving Their Intensive Culture. Aquaculture 546, 737347. doi: 10.1016/j.aquaculture.2021.737347
VanderLugt K., Cooney M. J., Lechner A., Lenz P. H. (2009). Cultivation of the Paracalanid Copepod, Bestiolina Similis (Calanoida: Crustacea). J. World Aquac. Soc 40, 616–628. doi: 10.1111/J.1749-7345.2009.00282.X
Wang L., Wang S., Zeng C., Wang Y., Zeng C. (2021). Effects of Food Concentration and Photoperiod on Egg Production, Female Life Expectancy and Population Dynamics of the Paracalanid Copepod, Bestiolina Amoyensis. Front. Mar. Sci. 8. doi: 10.3389/fmars.2021.788744
World Health Organization (2018) E. Coli. Available at: https://www.who.int/news-room/fact-sheets/detail/e-coli (Accessed March 15, 2018).
Yang C. M. (1977). The Egg Development of Paracalanus Crassirostris Dahl 1894 (Copepoda, Calanoida). Crustaceana 31, 33–38. doi: 10.1163/156854077X00205
Yang W. D., (2007) Studies of Zooplankton Grazing Impacts on Phytoplankton in Xiamen Harbor. Master’s Thesis. Xiamen zXiamen University
Keywords: hatching time, egg hatching rate, hatching success, live feed, Calanoida
Citation: Wang S, Wang L, Wang Y, Chen Y, Chen J and Chen N (2022) Embryo Development and Effects of Temperature, Salinity, and Light Intensity on Egg Hatching of Calanoid Copepod Bestiolina amoyensis (Calanoida: Paracalanidae). Front. Mar. Sci. 9:940303. doi: 10.3389/fmars.2022.940303
Received: 10 May 2022; Accepted: 09 June 2022;
Published: 15 July 2022.
Edited by:
Chaoshu Zeng, James Cook University, AustraliaReviewed by:
Per Meyer Jepsen, Roskilde University, DenmarkYen-Ju Pan, National Taiwan Ocean University, Taiwan
Copyright © 2022 Wang, Wang, Wang, Chen, Chen and Chen. This is an open-access article distributed under the terms of the Creative Commons Attribution License (CC BY). The use, distribution or reproduction in other forums is permitted, provided the original author(s) and the copyright owner(s) are credited and that the original publication in this journal is cited, in accordance with accepted academic practice. No use, distribution or reproduction is permitted which does not comply with these terms.
*Correspondence: Shuhong Wang, c2h3YW5nQGptdS5lZHUuY24=