- 1Chongqing Key Laboratory of Nonlinear Circuits and Intelligent Information Processing, College of Electronic and Information Engineering, Southwest University, Chongqing, China
- 2Key Laboratory of Microelectronic Devices & Integrated Technology, Institute of Microelectronics Chinese Academy of Sciences, Beijing, China
Introduction
With the continuous progress of science and technology, especially the advent of the era of the Internet of things, the electronic industry has become an emerging science and technology development industry (Qin et al., 2011). Electronic technology has a large number of applications in many application fields, such as aerospace, navigation, vehicle and so on. In the marine environment, many application scenarios such as ship positioning, marine environment monitoring and satellite communication are inseparable from the support of electronic equipment (Dong and Shi, 2017; K. K. Ku et al., 2008). The development of electronic technology is of positive significance to the development of marine electronic devices such as marine floats and underwater sensor network (Figures 1A, B). At present, due to the miniaturization and high integration of integrated circuits, the electronic industry has gradually entered the nano devices category. Integrated circuits use nano semiconductor materials (Nanowires, nanorods, nanopores, nanostar particles, nanostar particle aggregates, etc.) and nano semiconductor fabrication technology leads to nano electronic structures such as metal wires, which causes unknown pollution influence to marine environment. Particularly, in addition to the traditional integrated circuit technology, 3D integrated circuits and transient electronics, as cutting-edge technologies, the impact of their waste nano materials on the marine environment has attracted increasing attention.
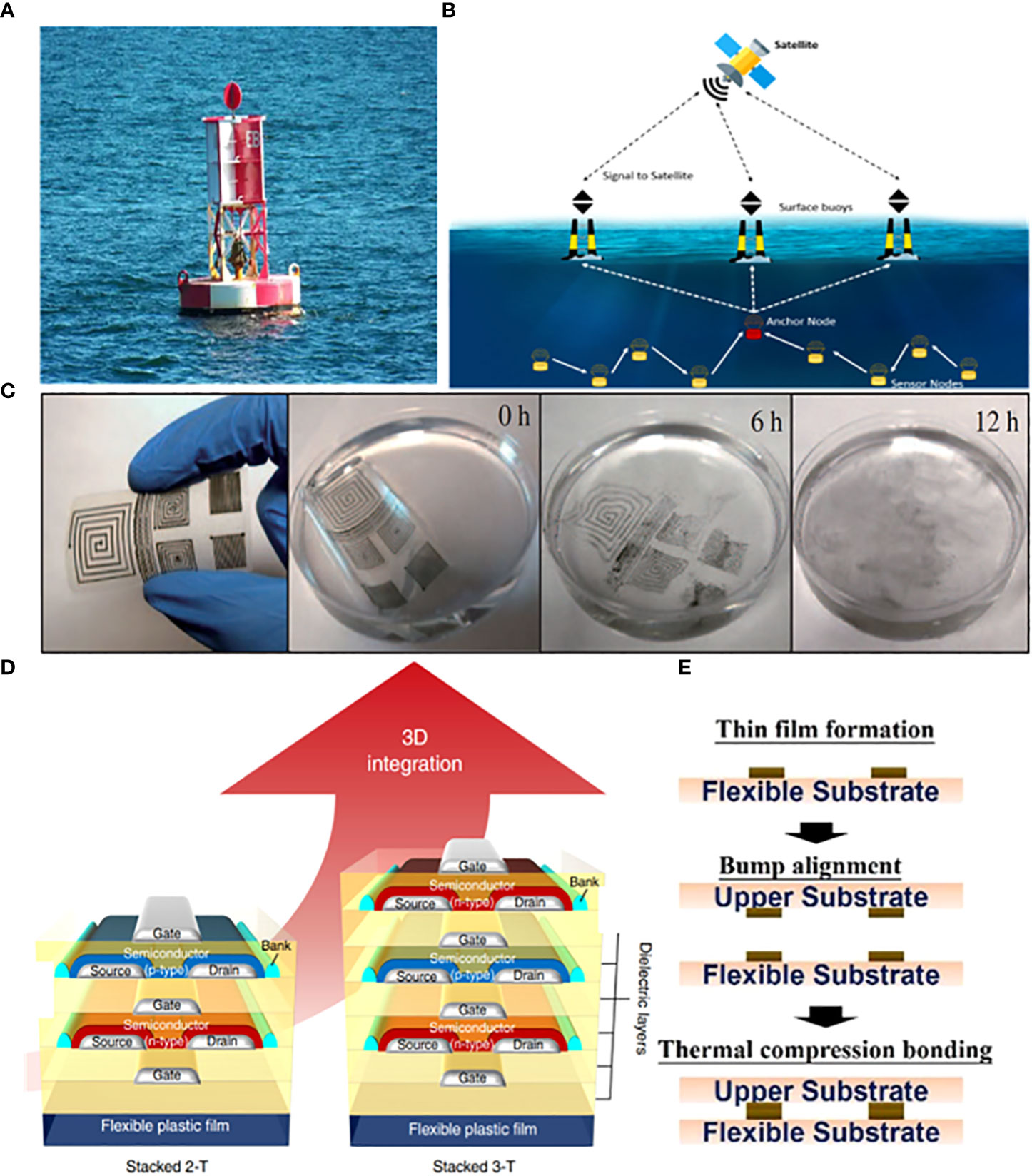
Figure 1 Marine electronic devices and advanced electronic technology (A) ocean buoy (B) Underwater sensor network (Shams et al., 2021) (C) Dissolution process of transient electronic in water (Mahajan et al., 2018) (D) 3D monolithic integration in flexible printed transistors (Kwon et al., 2019) (E) A Novel bonding method based on metal film (Hu and Chen, 2016).
3D integrated circuits mainly use silicon through-hole technology to stack and bond multilayer integrated circuit chips or wafers (The thickness of each layer is about 20 ~ 100 μ m), and realize 3D integration with the help of the third dimension (Topol et al., 2006), which can greatly accelerate the growth of chip scale. Compared with traditional integrated circuits (Lee and Chakrabarty, 2009; Knickerbocker et al., 2008), 3D integrated circuits can shorten the internal interconnection length of the chip (About 30 ~ 100 μ m), reduce power consumption (Zhang et al., 2001; Li and Wu, 2010), and have more I/O resources and stronger computing power (Li and Wu, 2010). In the current research, most researchers are committed to solving its thermal problems, yield, layout and wiring design and testing (Jung et al., 2012; Bazargan et al., 2000), and there are few relevant studies on marine pollution caused by its waste nano materials. Most 3D integrated circuit devices are similar to traditional electronic devices. However, due to the increased number of device integration and wafer stacking, there are more toxic and harmful substances such as lead, cadmium, mercury and PVC plastics per chip. Once these substances enter the marine environment, they will bring a certain degree of marine pollution and eventually endanger human beings, marine animals and plants.
On the other hand, different from the 3D integrated circuit, the main feature of transient electronics is that its electronic structure can be partially or completely degraded into the surrounding environment in a controlled manner within a specified time (Hwang et al., 2012). Transient electronics does not focus on the improvement of device performance, but more considers the actual application requirements. From the perspective of environmental protection, the research of transient electronics mainly focuses on the degradability of electronic products under the condition of meeting the basic functions of the products (Ji et al., 2018; Yu et al., 2018). The degradation of electronic devices can greatly reduce the emergence of harmful substances in e-waste. The image of dissolution process of transient electronic in water is shown in Figure 1C.
This paper focuses on the analysis of the current cutting-edge electronic technology, the pollution of nano materials of 3D integrated circuit devices and transient electronic devices to the marine environment, and the possible impact of a marine atmospheric environment on electronic products. Combined with reality, this paper analyzes the feasible measures to reduce the pollution of marine electronic wastes, and puts forward the possibility of combining 3D integrated technology with transient electronic devices to establish a 3D transient microsystem.
Analysis of influencing factors of electronic devices
3D-integrated circuit
3D-integrated circuits have been the development direction under the background of the nano integrated circuit. It can greatly improve the integration of chip with advantages of high density, low power consumption, large bandwidth, etc. It is noted that nano semiconductor materials such as silicon, germanium, gallium arsenide, silicon carbide and indium phosphide, as well as metal materials such as gold, copper, iron, lead and aluminum and some non-metallic materials such as resin and plastic, are used for construction of 3D integrated circuits. Once the waste nanoparticle materials flow into the marine environment, the pollution problem is characterized by wide sources, long residue time, transfer and enrichment along the food chain, difficult to detect and recover after pollution, and heavy metal materials will harm marine organisms to oxidative stress, cause DNA damage, protein modification, lipid peroxidation, and even biological death (Wu et al., 2016). The plastic shell of electronic equipment flows into the marine environment and will be broken and degraded into small-size plastic with a particle size of less than 5 mm under the action of wind, waves, ocean current and solar radiation (Thompson et al., 2004). This substance will produce biological toxicity to marine organisms and affect the eating preference and reproduction of the organisms (Jeong et al., 2017; Ory et al., 2018). When it enters the deep sea, it will make the marine composition more complex and the biological environment more demanding (Kershaw et al., 2015). While causing physical pollution, it may also cause compound pollution, which will eventually affect the marine biological ecological chain through material circulation.
Transient electronics
The transient electronic device is an electronic device that can degrade itself within a certain time range (Kang et al., 2020). Through relatively mature flexible electronic device processing technology and transfer printing technology, its performance can be comparable to that of ordinary devices. There are many transient electronic devices, such as field effect transistors (Acar et al., 2012), energy collectors (Li et al., 2019), energy storage batteries (Chen et al., 2016), induction antenna (Jamshidi et al., 2015). These transient electronic devices mainly include substrates, electrodes, dielectric layers, active layers (interconnect wires or semiconductors) and packaging layers (Loh et al., 2016). At present, the thickness of each layer of transient electronic chip using xi10 technology is generally between 100 and 900 nm. The substrate and packaging layer play the role of carrier and support in transient electronic devices. Their main materials are natural polymer materials such as silk protein (Tao et al., 2014; Jia et al., 2017; Ji et al., 2018), rice paper (Hosseini and Lee, 2015), synthetic polymer materials such as polyanhydride (Choi et al., 2020), polycaprolactone (Li et al., 2018), Polyoxymethylene (Feig et al., 2018), graphene oxide and so on. Generally, this part of materials can be completely absorbed by the water body without affecting the marine ecological environment. Electrodes, dielectric layers and active layers are the basis for the realization of transient electronic functions. Electrodes generally use metal materials, such as Mg, Ag, Cu, Al, Fe, Au, graphene and indium-tin oxide (ITO). The most common transient dielectric materials are semiconductor materials such as Si and Ge and oxides such as silica, zinc oxide and magnesium oxide (Corbet et al., 2014; Hwang et al., 2015; Kang et al., 2015). Among them, monocrystalline silicon can be dissolved in aqueous medium (Si + 4H2O → Si(OH)4 + 2H2) at near neutral pH, and monocrystalline silicon with 100 nm thickness can be completely dissolved in phosphate buffered saline within 20 days (Choi et al., 2020). Compared with Si, Ge has a similar hydrolysis mechanism (Ge + O2 + H2O → H2GeO3), and the pattern array of Ge squares (3μm×3μm×100nm) can be dissolved in a few hours at pH 10. ZnO films can be dissolved into hydroxide (ZnO + H2O → Zn(OH)2) or zinc ions under different thickness conditions. The interconnection conductor material is mainly soluble metal with good performance. Semiconductor materials are also widely used in active layers, such as monocrystalline silicon (Kang et al., 2018), black phosphorus (BP) (Song et al., 2018).
Contamination of transient electronics is manageable compared to conventional electronics. Although the marine atmospheric environment has a certain corrosive effect on transient electrons, it is generally difficult to achieve the triggering conditions of transient electrons. For example, the memristor device prepared by Liu et al. (Liu et al., 2019) has a high temperature of 180°C for its degradation triggering condition, such a temperature cannot be reached under natural conditions. The triggering methods that are highly sensitive to the marine environment are wet chemical reaction and light responses. But these can be avoided by adding proper encapsulation, or using a well-protected metal or plastic case.
3D transient microsystem
The advantage of the 3D integrated circuit lies in its high integration, low power consumption and high performance, but a very important but unsolved problem is the pollution problem after the devices flow into the marine environment. An attractive method is to combine 3D integration technology with transient electronic devices and use degradable materials to prepare 3D transient microsystem devices, so that the new devices have the advantages of 3D integrated circuits and transient electronic devices at the same time, that is, reduce the impact on the marine ecological environment on the premise of smaller volume and higher performance. However, due to the current problems of transient electronics, such as simple structure, low process integration, and certain requirements for the research and development of new materials, it is difficult to directly apply to three-dimensional integrated circuits. At present, in the research of flexible printed circuits, researchers have adopted the strategy of multi-dimensional nanomaterials and structure design to improve the integration and stability of circuits. Kwon et al. (Kwon et al., 2019) adopt a dual-gate configuration in the 3D transistor-on-transistor structure for the improvement of their electrical characteristics. This method can effectively increase the density of printed transistors. Meanwhile, by interconnecting these 3D integrated dual-gate transistors, the authors propose a 3D universal logic gate and its array as a new facile route to design printed digital circuits that are essential for emerging flexible electronics applications. The image of 3D monolithic integration in flexible printed transistors is shown in Figure 1D. In the work of Hu et al. (Hu and Chen, 2016), Sn/In and Cu are selected as the bond materials due to their low melting point and good ductility. And a novel bonding method using metal thin films is proposed. This bonding approach achieves ultra-fine pitch, less reliability issue factors and a simple process which can be applied in the flexible substrate 3D stacking. The image of the novel bonding method based on metal film is shown in Figure 1E. These works have a certain reference value for 3D transient microsystem.
Discussion
Conventional printed circuit boards of electronic devices are developing towards thin lines, small holes and micro spacing, and are becoming more and more sensitive to corrosion (Ahmad, 2006; Ghosh, 2017). The main influencing factors of the marine environment on electronic products include temperature, humidity, sea salt particles and dust. Temperature is an important factor in improving the corrosion reaction. When the temperature is higher than 40°C, it has a great influence on the corrosion reaction rate of electronic materials. At the same time, due to the high integration density of electronic circuits, they have certain heat dissipation problems. Once the heat accumulation is formed, it is easy to reduce the operation efficiency of the chip, even damage the components. Moist air is conducive to the growth of mold. Mold contains a lot of water, which greatly reduces the insulation performance of electronic equipment. The acidic substances secreted in the metabolic process of mold interact with insulating materials to degrade the insulation performance of equipment. and will affect the reliability of the device. The main components of sea salt particles are 75% sodium chloride and 10% magnesium chloride. The dust contains water-soluble components such as sulfate ion, nitrate ion and hydrochloric acid ion. When marine particles and dust are attached to chips or electronic devices, corrosion reaction will occur more easily under certain temperature and humidity conditions (Xiao et al., 2017; Cengiz and Cansoy, 2015). Once the package is damaged and corrosive gas infiltrates, it is very easy to form an adsorptive thin liquid film (Schneider and Buttry, 1993; Wadsak et al., 2000) in PCB vias, pads and pollutant residues, resulting in electromigration corrosion and galvanic corrosion of pads or plug-in pins (Wang et al, 2017), affecting the service life of devices.
The impact of the marine environment and advanced electronic devices is interactive. Therefore, while considering the marine pollution of advanced electronic products, it is also necessary to consider the corrosivity of the marine environment to the products. Aiming at the specific environment of the ocean, improving the reliability of advanced electronic devices and reducing the output of e-waste have a certain positive significance for the marine environment. Therefore, for the research of advanced electronics, while considering the degree of marine pollution caused by its materials, it is also necessary to improve the reliability and stability of electronic devices, achieve a good forward cycle, and reduce the impact on the marine environment as much as possible while meeting the functional requirements of marine electronics. For the 3D transient microsystem, the design of its degradation mechanism needs to consider not only the corrosion effect of marine complex atmospheric environment on degradable materials, but also the stability of the device itself, which can work stably and reliably at a given time. Although its design and integration are difficult, we look forward to the emergence of related devices in the future.
Author contributions
MP: original draft preparation and editing. ZZ: writing—review and project administration. JG: review and quality oversight. All authors contributed to the article and approved the submitted version.
Funding
This project is supported by State key laboratory of precision measuring technology and instruments (No.pilab2208), and the Opening Project of Key Laboratory of Microelectronic Devices & Integrated Technology, Institute of Microelectronics, Chinese Academy of Sciences.
Conflict of interest
The authors declare that the research was conducted in the absence of any commercial or financial relationships that could be construed as a potential conflict of interest.
Publisher’s note
All claims expressed in this article are solely those of the authors and do not necessarily represent those of their affiliated organizations, or those of the publisher, the editors and the reviewers. Any product that may be evaluated in this article, or claim that may be made by its manufacturer, is not guaranteed or endorsed by the publisher.
References
Acar H., Genc R., Urel M., Erkal T., Dana A., Guler O. M. (2012). Self-assembled peptide nanofiber templated one-dimensional gold nanostructures exhibiting resistive switching. Langmuir 28 (47), 16347–16354. doi: 10.1021/la3035215
Bazargan K., Kastner R., Sarrafzadeh M. (2000). 3-d floorplanning: Simulated annealing and greedy placement methods for reconfigurable computing systems. Proc. Int. Workshop Rapid System Prototyping 5, 329–338. doi: 10.1023/A:1008962420726
Cengiz U., Cansoy C. E. (2015). Applicability of cassie-Baxter equation for superhydrophobic fluoropolymer-silica composite films. Appl. Surface Sci. 335, 99–106. doi: 10.1016/j.apsusc.2015.02.033
Chen Y., Jamshidi R., White K., Çinar S., Gallegos E., Hashemi N., et al. (2016). Physical-chemical hybrid transiency: A fully transient li-ion battery based on insoluble active materials. J. Polymer Sci. Part B: Polymer Phys 54 (20), 2021–2027. doi: 10.1002/polb.24113
Choi Y. S., Koo J., Lee Y. J., Lee G., Avila R., Ying H., et al. (2020). Biodegradable polyanhydrides as encapsulation layers for transient electronics. Adv Funct. Mater. 30 (31), 2000941. doi: 10.1002/adfm.202000941
Choi Y., Koo J., Lee Y. J., Lee G., Avila R., Ying H., et al (2020). Inorganic materials for transient electronics in biomedical applications. Adv. Funct. Mater.30 (31):2000941. doi: 10.1557/mrs.2020.25
Ory N. C., Gallardo C., Lenz M., Thiel M. (2018). Capture, swallowing, and egestion of microplastics by a planktivorous juvenile fish. Environ. pollut. 240, 566–573. doi: 10.1016/j.envpol.2018.04.093
Corbet C. M., Mcclellan C., Kim K., Sonde S., Tutuc E., Banerjee S. K. (2014). Oxidized titanium as a gate dielectric for graphene field effect transistors and its tunneling mechanisms. ACS Nano 8 (10), 10480–10485. doi: 10.1021/nn5038509
Dong S., Shi L. (2017). “The design and realization of ship dynamic positioning controller based on DSP,” in 2017 36th Chinese Control Conference (CCC). IEEE, 2017, 6348–6352. doi: 10.23919/ChiCC.2017.8028363
Feig V. R., Tran H., Bao Z. (2018). Biodegradable polymeric materials in degradable electronic devices. ACS Cent. Sci. 2018, 337. doi: 10.1021/acscentsci.7b00595
Ghosh S. (2017). Three-dimensional microplate formation with evaporating nanoparticle suspensions on superhydrophobic surfaces. Colloids Surfaces A Physicochem Eng. Aspects 529, 901–906. doi: 10.1016/j.colsurfa.2017.07.007
Hosseini N. R., Lee J. (2015). Biocompatible and flexible chitosan-based resistive switching memory with magnesium electrodes. Adv. Funct. Mater. 25 (35), 5586–5592. doi: 10.1002/adfm.201502592
Hu Y., Chen K. (2016). A novel bonding approach and its electrical performance for flexible substrate integration. IEEE J. Electron Devices Soc. 4 (4), 185–188. doi: 10.1109/JEDS.2016.2537359
Hwang S. W., Hu T., Kim D. H., Sonde S., Tutuc E., Banerjee S. K., et al (2012). A physically transient form of silicon electronics. Science 337 (6102), 1640–1644. doi: 10.1126/science.1226325
Hwang S. W., Kang S. K., Huang X., Brenckle M., Omenetto F., John A. R. (2015). Materials for programmed, functional transformation in transient electronic systems. Adv Mater 27 (1), 47–52. doi: 10.1002/adma.201403051
Jamshidi R., Cinar S., Chen Y., Hashemi N., Montazami R. (2015). Transient bioelectronics: Electronic properties of silver microparticle-based circuits on polymeric substrates subjected to mechanical load. J. Polymer Sci. Part B: Polymer Phys. 53 (22), 1603–1610. doi: 10.1002/polb.23804
Jeong C. B., Kang H. M., Lee M. C., Kim D. H., Han J., Hwang D. S., et al. (2017). Adverse effects of microplastics and oxidative stress-induced MAPK/Nrf2 pathway-mediated defense mechanisms in the marine copepod paracyclopina nana. Sci. Rep. 7 (1), 1–11. doi: 10.1038/srep41323
Jia X., Wang C., Ranganathan V., Napier B., Yu C., Chao Y, et al. (2017). A biodegradable thin-film magnesium primary battery using silk fibroin-ionic liquid polymer electrolyte[J]. ACS Energy Letters 2 (4), 831–36. doi: 10.1021/acsenergylett.7b0001
Ji X., Song L., Zhong S., Jiang Y., Lim K.G., Wang C., et al. (2018). Biodegradable and flexible resistive memory for transient electronics. J. Phys. Chem. C 122 (29), 16909–16915. doi: 10.1021/acs.jpcc.8b03075
Jung M., Mitra J., Pan D. Z., Lim S. K. (2012). TSV stress-aware full-chip mechanical reliability analysis and optimization for 3-d IC. IEEE Trans. Computer-Aided Design Integrated Circuits Syst. 31 (8), 1194–1207. doi: 10.1109/TCAD.2012.2188400
Kang S. K., Koo J., Lee Y. K., Rogers J. A. (2018). Advanced materials and devices for bioresorbable electronics. Accounts of Chem. Res. 51 (5), 988–998. doi: 10.1021/acs.accounts.7b00548
Kang S. K., Park G., Kim K., Hwang S. W., Cheng H., Shin J., et al. (2015). Dissolution chemistry and biocompatibility of silicon- and germanium-based semiconductors for transient electronics. ACS Appl. Mater Interfaces 7 (17), 9297. doi: 10.1021/acsami.5b02526
Kang S. K., Yin L., Bettinger C. (2020). The emergence of transient electronic devices. MRS Bull. 45 (2), 87–95. doi: 10.1557/mrs.2020.19
Knickerbocker J. U., Andry P. S., Dang B., Horton R. R., Interrante M. J., Patel C. S, et al. (2008). Three-dimensional silicon integration. IBM J. Res. Dev. 52 (6), 553–569. doi: 10.1147/JRD.2008.5388564
Kershaw P. (2015). International Maritime Organization. Rep. Stud. GESAMP No. 90, 96 p. Available at: http://hdl.handle.net/123456789/735
Ku K., Bradbeer R., Hodgson P., Lam K., Yeung L.. (2008). OCEANS 2008 . MTS/IEEE Kobe Techno-Ocean, IEEE, 1–5. doi: 10.1109/OCEANSKOBE.2008.4530964
Kwon J., Takeda Y., Shiwaku R., Tokito S., Cho K., Jung S. (2019). Three-dimensional monolithic integration in flexible printed organic transistors. Nat. Commun. 10 (54), 1–10. doi: 10.1038/s41467-018-07904-5
Lee H., Chakrabarty K. (2009). Test challenges for 3D integrated circuits. IEEE Design Test Comput. 26 (5), 26–35. doi: 10.1109/MDT.2009.125
Li R., Wang L., Kong D., Yin L. (2018). Recent progress on biodegradable materials and transient electronics. Bioactive Mater 3 (3), 322–333. doi: 10.1016/j.bioactmat.2017.12.001
Liu D., Zhang S., Cheng H., Peng R., Luo Z. (2019). Thermally triggered vanishing bulk polyoxymethylene for transient electronics. Sci. Rep. 9 (1), 1–9. doi: 10.1038/s41598-019-54565-5
Li J. F., Wu C. W. (2010). “Is 3D integration an opportunity or just a hype?,” in 2010 15th Asia and South Pacific Design Automation Conference (ASP-DAC) IEEE, 2010, 541–543. doi: 10.1109/ASPDAC.2010.5419826
Li H., Zhao C., Wang X. X., Meng J. P., Zou Y., Noreen S, et al. (2019). Bioabsorbable capacitors: Fully bioabsorbable capacitor as an energy storage unit for implantable medical electronics. Adv. Sci. 6 (6), 1801625. doi: 10.1002/advs.201801625
Loh X. J., Tan M. J., Owh C., Chee P. L., Kyaw A. K. K., Kai D., et al. (2016). Biodegradable electronics: cornerstone for sustainable electronics and transient applications. J. Mater Chem C Mater Optical Electronic Devices 4 (24), 5531–5558. doi: 10.1039/C6TC00678G
Mahajan B. K., Ludwig B., Shou W., Yu X., Fregene E., Xu H., et al. (2018). Aerosol printing and photonic sintering of bioresorbable zinc nanoparticle ink fortransient electronics manufacturing. Sci. China Inf. Sci 61, 060412. doi: 10.1007/s11432-018-9366-5
Qin W., Qiao W., Xie M. (2011). “Research on the power of industry control in electronic information industry,” in 2011 International Conference on Computer Science and Service System (CSSS) IEEE, 4029–4032. doi: 10.1109/CSSS.2011.5974973
Schneider T. W., Buttry D. A. (1993). Electrochemical quartz crystal microbalance studies of adsorption and desorption of self-assembled monolayers of alkyl thiols on gold. J. Am. Chem. Soc. 155 (26), 12391–12397. doi: 10.1021/ja00079a021
Song M. K., Namgung S. D., Sung T., Cho A. J., Lee J., Ju M., et al. (2018). Physically transient field-effect transistors based on black phosphorus. ACS Appl. Mater Interfaces 10 (49), 42630–42636. doi: 10.1021/acsami.8b15015
Shams R., Otero P., Aamir M, Khan F. H. (2021). Joint Algorithm for Multi-HopLocalization and Time Synchronization in Underwater Sensors Networks UsingSingle Anchor, IEEE Access 9, 27945–27958. doi: 10.1109/ACCESS.2021.3058160
Tao H., Hwang S. W., Marelli B., An B., Moreau J. E., Yang M., et al. (2014). Silk-based resorbable electronic devices for remotely controlled therapy and in vivo infection abatement. Proc. Natl. Acad. Sci 111 (49), 17385–17389. doi: 10.1073/pnas.1407743111
Thompson R., Olsen Y., Mitchell R. P., Davis A., Rowland S. J., John A. W. G., et al. (2004). Lost at Sea: Where is all the plastic? Science 304 (5672), 838. doi: 10.1126/science.1094559
Topol A. W., Tulipe D. C. L., Shi L., Frank D. J., Bernstein K., Steen S. E, et al. (2006). Three dimensional integrated circuits: US, US7091604 B2. IBM Journal of Research and Development 50 (4.5), 491–506. doi: 10.1147/rd.504.0491
Wadsak M., Schreiner M., Aastrup T., Leygraf C. (2000). Combined in-situ investigations of atmospheric corrosion of copper with SFM and IRAS coupled with QCM. Surface Sci. 454, 246–250. doi: 10.1016/S0039-6028(00)00081-9
Wang Y., Zhu Y., Zhang C., Li J., Guan Z. (2017). Transparent, superhydrophobic surface with varied surface tension responsiveness in wettability based on tunable porous silica structure for gauging liquid surface tension. ACS Appl. Mater. Interfaces 9 (4), 4142–4150. doi: 10.1021/acsami.6b12779
Wu X., Cobbina S. J., Mao G., Xu H., Zhang Z., Yang L. (2016). A review of toxicity and mechanisms of individual and mixtures of heavy metals in the environment. Environ. Sci. pollut. Res. 23, 8244–8259. doi: 10.1007/s11356-016-6333-x
Xiao Y., Huang W., Tsui C. P., Wang G., Tang C. Y., Zhong L. (2017). Ultrasonic atomization based fabrication of bio-inspired micro-nano-binary particles for superhydrophobic composite coatings with lotus/petal effect. Composites Part B: Eng. 121, 92–98. doi: 10.1016/j.compositesb.2017.03.029
Yu X., Shou W., Mahajan B. K., Huang X., Pan H. (2018). Materials, processes, and facile manufacturing for bioresorbable electronics: A review. Adv Mater 30 (28), 1707624. doi: 10.1002/adma.201707624
Keywords: 3D integrated circuit, transient electron, marine electronics, nanometer material, marine environmental pollution
Citation: Pu M, Zhu Z and Guo J (2022) Interaction of advanced micro/nano electronics and materials with marine environment. Front. Mar. Sci. 9:940297. doi: 10.3389/fmars.2022.940297
Received: 10 May 2022; Accepted: 18 July 2022;
Published: 11 August 2022.
Edited by:
Roger C. Prince, Stonybrook Apiary, United StatesReviewed by:
Aung Ko Ko Kyaw, Southern University of Science and Technology, ChinaCopyright © 2022 Pu, Zhu and Guo. This is an open-access article distributed under the terms of the Creative Commons Attribution License (CC BY). The use, distribution or reproduction in other forums is permitted, provided the original author(s) and the copyright owner(s) are credited and that the original publication in this journal is cited, in accordance with accepted academic practice. No use, distribution or reproduction is permitted which does not comply with these terms.
*Correspondence: Zhiyuan Zhu, enl1YW56aHVAc3d1LmVkdS5jbg==; Jing Guo, cG9lbTI0QHN3dS5lZHUuY24=