- Key Laboratory of Marine Biotechnology of Zhejiang Province, School of Marine Sciences, Ningbo University, Ningbo, China
Gracilariopsis lemaneiformis, an important commercial red macroalga, is facing significant impacts from global warming, which limits algal growth and yield in China. Polyamines (PAs), spermidine (SPD) and spermine (SPM), are ubiquitous polycations important for growth and environmental stress responses including high temperature (HT) tolerance. Spermidine synthase (SPDS) gene is one of the important genes in higher PA biosynthesis, which plays critical roles in HT stress response. Here, we isolated an SPDS gene from G. lemaneiformis and further analyzed its phylogenetic tree, subcellular localization, and gene expression patterns under stress conditions. Meanwhile, supplemented with SPD and SPM were used to study the effects of PAs on HT tolerance in G. lemaneiformis. It showed exogenous 0.5 mM SPD and SPM, respectively, remarkably improved the algal relative growth rate (RGR) compared to those in the CK treatment groups under HT conditions. In addition, they both significantly elevated the activities of antioxidant enzymes and significantly upregulated the expression of genes encoding antioxidant enzymes, triggered transcription factors (TFs) signaling, and improved the expression of genes encoding small heat shock proteins (sHSP20s) during HT stress. Moreover, exogenous PA also enhanced the expression of genes involved in pyruvate metabolism, ascorbate and aldarate metabolism, and nucleotide excision repair in G. lemaneiformis, which helped to maintain better energy supply, redox homeostasis, and genome integrity under HT stress. Taken together, these data provided valuable information for functional characterization of specific gene in endogenous PA synthesis and uncovered the importance of exogenous PAs in promoting algae adaptation to HT stress.
1 Introduction
Gracilariopsis lemaneiformis, one of the marine red algae from the Rhodophyta, is an important source of nutrients for both abalone and humans (Zou et al., 2004). It has high content and quality of agar and is regarded as the most important commercial species that cultivated in several countries and regions, especially in Fujian and Guangdong provinces in southern China (Fu and Kim, 2010; Zou and Gao, 2014). Additionally, G. lemaneiformis is also effective in decreasing eutrophication, playing pivotal roles in marine ecological restoration (Yang et al., 2015; Luo et al., 2020).
However, climatic changes induced continuing temperature rise in surface oceans exerts a detrimental effect on G. lemaneiformis, particularly in summer time on the southern coast of China. As is known, the wild G. lemaneiformis can growth prolifically under the temperature range of 10°C to 23°C, once beyond the upper limit its production may be reduced or even cease (Lv et al., 2019). Many studies showed high temperature (HT) stress could induce overaccumulation of reactive oxygen species (ROS) in algae, which oxidizes cellular components of lipids, proteins, and nucleic acids, and brings damage to cellmembranes (Zhang et al., 2020; Zhang et al., 2021). Under these circumstances, algae and plants may upregulate the activities of antioxidant enzymes to decrease the malondialdehyde (MDA) content and thereby reduce membrane damage (Mittler et al., 2004; Wang et al., 2018b). Moreover, in response to HT stress, systemic regulation of genes including many transcription factors, such as the basic/helix–loop–helix (bHLH), basic leucine zipper (bZIP), and the v-myb avian myeloblastosis viral oncogene homolog (MYB) family genes (Singh et al., 2002; Wang et al., 2018a), and as well as stress-responsive genes encoding heat shock proteins (HSPs; Kobayashi et al., 2014), play pivotal roles in plant HT stress adaptation.
Using exogenous protectants such as plant hormones, amino acids, vitamins, and signaling molecules are now common in research and is expected to enhance stress tolerance in algae (Xie et al., 2013; Hou et al., 2018; Zhang et al., 2020). As a bioactive substance, polyamines (PAs) mainly putrescine (PUT), spermidine (SPD), and spermine (SPM) can act as “stress-relievers” under different types of abiotic stresses (Wang et al., 2019; Alcazar et al., 2020). Exogenous PAs has been found to enhance abiotic tolerance to salt damage, heavy metal (Cu, Fe, and Ni) stress, dehydration and flooding stress, chilling, and heat stress in various species (Gill and Tuteja, 2010a; Alcazar et al., 2020). Especially in terms of heat stress, many researches have reported that PA treatment can alleviate the heat injury of wheat grain filling and improve heat tolerance by upregulating HSP genes, enhancing antioxidant defense efficiency and chlorophyll metabolism, and modulating endogenous starch and polyamine metabolism in Arabidopsis, rice, and tomato (Sagor et al., 2013; Fu et al., 2019; Jing et al., 2020; Jahan et al., 2022). Currently, compared with higher plants, the roles of exogenous PA in response to HT stress in algae, particularly G. lemaneiformis, are not yet known.
Moreover, we have to mention that the PA biosynthetic genes such as spermidine synthase (SPDS) and spermine synthase (SPMS) genes also play vital roles in abiotic stress by acquiring SPDS/SPMS-transgenic plants. Overexpressing SPDS in the marine cyanobacteria Synechococcus sp. PCC 7942 showed a better growth and photosynthetic efficiency together with the tolerance against osmotic stress (Pothipongsa et al., 2016). As for the synthesis of SPD and SPM, it requires the enzyme SPDS to add an aminopropyl moiety to PUT, hence forming SPD, while the enzyme SPMS adds an aminopropyl group to SPD and convert it to SPM. SPDS was the ancestral enzyme in all three domains of life, and SPMS, evolved from the duplication of SPDS, appear to be limited to the flowering plants (angiosperms; Minguet et al., 2008). However, previous studies showed that all the ancient organisms, such as archea, algae, and moss, contain no SPMS sequences (Pegg and Michael, 2010), so there is no SPMS gene in G. lemaneiformis. Therefore, the SPDS-encoding gene that is responsible for PA synthesis and its response to abiotic stress in G. lemaneiformis are needed to be uncovered.In this study, we characterized a G. lemaneiformis spermidine synthase (SPDS) gene which was strongly induced by high temperature. We speculated it might be responsible for the heat tolerance and extend the potential of genetic engineering using the SPDS gene to obtain algae with higher tolerance to HT stress. Further we investigated the possible involvement of exogenous SPD and SPM in G. lemaneiformis resistance to high temperature stress in G. lemaneiformis. To elucidate its possible mechanisms, we employed RNA-Seq to study the genome-wide expression profiles during HT acclimation for 24 h and characterized of the biological pathways based on the transcriptome profile. It indicated the underlying role of SPD and SPM in alleviating of HT stress in G. lemaneiformis through antioxidant defense, the heat shock response, TF signaling, and DNA repair mechanism, which allowed the algae to adapt to high temperature.
2 Materials and Methods
2.1 Algal Culture and Stress Treatment
Sample of G. lemaneiformis was collected from Ningde, Fujian Province of China. The cleaned algae thalli were domesticated under laboratory conditions at 23°C and 25 salinity (CK or the control group) in a 12:12 light–dark regime in an incubator with 30 μmol photons m−2 s−1 light intensity. For analysis of gene expression, algal thalli were subjected to 33°C conditions (day/night) for high temperature (HT, 33°C) treatment. For other abiotic stress treatment, we designed as follows: low temperature (LT, 13°C), high salinity (HS, 35 salinity) and low salinity (LS, 15 salinity). Samples were collected at indicated time points after treatment. Each treatment with three biological replicates.
2.2 Identification of Spermidine Synthase Gene and Bioinformatics Analyses
Sequence of spermidine synthase gene is searched in the genome database using a local BLAST method. The isoelectric point and molecular weight were predicted with the help of the Compute pI/Mw tool on the ExPASy Proteomics Server (http://expasy.org/). Sequence alignment of protein sequences was performed using the Clustal W program. Phylogenetic trees were constructed using the neighbor-joining (NJ) method of the MEGA6 program with the p-distance and complete deletion option parameters. Bootstrap analysis with 1,000 replicates was also conducted in order to obtain the reliability for the branches.
2.3 Subcellular Localization and Western Blot Detection of GlSPDS
Coding sequence of GlSPDS, amplified using a pair of primers GlSPDS-GFP-F/R (Supplementary Table S1), was inserted into pCAMBIA2300-GFP vector, yielding p pCAMBIA2300-GFP : GlSPDS. The sequence-verified pCAMBIA2300-GlSPDS construct and the empty vector pCAMBIA2300-GFP were introduced into Agrobacterium tumefaciens strain GV3101. Four-week-old Nicotiana benthamiana plants were infiltrated with agrobacteria carrying different constructs and grew at 25°C for 48 h. Microscopic observation was performed using a ZeissLSM-780 microscope (Carl Zeiss, Germany) and representative photographs were taken.
Leaf discs, at 48 h after agroinfiltration, were ground into 200 μl lysis buffer (50 mM Tris–HCl, pH 7.4, 150 mM NaCl, 1 mM EDTA, 1 mM DDT, 0.1% (v/v) Triton X-100, 1 mM PMSF, and 1× protease inhibitor cocktail from Sigma). The samples with loading buffer were boiled for 5 min and subsequently centrifuged at10,000×g for 10 min at 4°C. Proteins (20 μL) were separated on a 12% SDS-PAGE gel and transferred onto PVDF membrane by semi-dry transfer. Detection of GFP was performed using a polyclonal rabbit anti-GFP antibody (1:5,000 dilution; GenScript, Nanjing, China) and a horseradish peroxidase (HRP)-conjugated anti-rabbit antibody (1:10,000 dilution; GenScript, Nanjing, China) according to the manufacturer’s instructions. Proteins on PVDF membranes were detected by SuperSignalWest Pico Chemiluminescent Substrate (Thermo Scientific, Rockford, IL, USA). Tanon automatic gel imaging system (Tianneng Corporation, Shanghai, China) was used for image acquisition.
2.4 Expression of HIS-GlSPDS Fusion Protein in the Escherichia coli Strain Rosetta (DE3)
The coding sequence of GlSPDS was amplified with gene-specific primers and was cloned into pET32a vector, yielding pET32a-GlSPDS, to make the His-GlSPDS fusion protein, then was introduced into the E. coli Rosetta strain (Novagen, San Diego, CA, USA). E. coli strain carrying pET32a-GlSPDS was grown at 37°C with shaking (220 rpm) in Luria–Bertani (LB) liquid medium to OD600 = 0.5 and were induced at 28°C for 4–6 h by the addition of 1 mM isopropyl β-D-1-thiogalactopyranoside (IPTG). Then cell supernatant was collected for ultrasonication to release target protein and further performed with a 12% SDS-PAGE, subsequently followed by Coomassie brilliant blue G-250-based assay for the visualization of His-GlSPDS fusion protein.
2.5 Exogenous PAs Treatments and Relative Growth Rate Analysis
Algal growth media were supplemented with SPD and SPM at the indicated concentrations (0.5 mM), which was chosen based on a preliminary test with a range of concentrations (0, 0.1, 0.5, and 1 mM). Then algal thalli were subjected to 30°C conditions (day/night) with or without SPD and SPM treatment, respectively, for variable time periods. The high temperature (30°C) we chose for the following physiological experiment based on Lv et al. (2019), which is more closer to high temperature stress in natural environment. The experiment had at least three treatments, and each treatment had three replicates.
The effects of SPD and SPM on algal growth at high temperatures were evaluated by measuring the weight of G. lemaneiformis after 7 days of 30°C treatment with or without PAs in the seawater medium. The RGR was calculated according to the formula as follows:RGR (% d-1) = ln (Wt/W0)/t×100 (Wt represents the weight after t days treatment, W0 is the weight of initial treatment, and t refers to the treatment time (d).
2.6 MDA and Antioxidant Enzyme Activity Measurements
The content of MDA was measured as described by Kumari et al. (2015). Briefly, for each sample, algae thalli (0.1 g) were powdered in liquid nitrogen and were homogenized in 2 mL 10% (w/v) trichloroacetic acid (TCA) and centrifuged at 10,000×g for 15 min, after which 1 mL supernatant was mixed with the reagents in an MDA assay kit (Jianchengbio, Nanjing, China), heated at 95°C for 30 min in a water bath and then quickly cooled in an ice bath. After centrifugation at 10,000×g for 10 min, the absorbance was measured at 532 nm using a spectrophotometer (UV-6100, METASH, Shanghai, China).
2.7 RNA Extraction, Sequencing, and Differentially Expressed Gene Analysis
Total RNA was extracted from G. lemaneiformis thalli after a 30°C treatment for 24 h using the RNeasy Plant Mini Kit (Qiagen, Shanghai, China) according to the manufacturer’s instructions. Briefly, the quality and quantification of RNA were separately determined by agarose gel electrophoresis and a NanoDrop 2000 spectrophotometer (Thermo Fisher Scientific, Waltham, MA, USA). More accurate RNA quantification was performed by an Agilent 2100 Bioanalyzer (Agilent Technologies, Santa Clara, CA, USA) for 28S/18S and RNA integrity number (RIN). The data of the RNA quality and quantification are listed in Supplementary Table S2.
For RNA sequencing, nine cDNA libraries from three treatments (0, SPD and SPM) of algal samples for 24 h under 30°C were used to generate 391 million PE reads. Sequencing was carried out on each library to generate 150 bp PE reads for transcriptome sequencing on an MGISEQ-2000 platform (MGI-Shenzhen, China). Clean reads were mapped to the previously assembled reference genes by using Bowtie2 software (Langmead and Salzberg, 2012). The DEGs were identified using the DESeq analysis (Wang et al., 2010). The criteria of adjusted p value ≤0.001 and log2 (fold change) ≥1 were set as the threshold for DEGs. GO and KEGG enrichment analyses were performed using Phyper based on hypergeometric tests.
2.8 Verification of Sequencing Results by Quantitative Real-Time PCR
To verify the reliability of the RNA-seq data, ten genes were selected for validation by qRT-PCR. Total RNA for each sample was prepared as described in 2.4. cDNA was synthesized using the HiScript II Q RT SuperMix for qPCR (Vazyme, Nanjing, China) according to the manufacturer’s instructions. The cDNA was diluted and amplified using an ABI7500 Real-Time PCR System (Applied Biosystems, Foster City, CA, USA) and ChamQ Universal SYBR qPCR Master Mix (Vazyme, Nanjing, China)according to the manufacturer’s instructions. The reaction conditions were 95°C for 30 s, followed by 40cycles of 95°C for 10 s, 60°C for 30 s and 72°C for 10 s. qRT-PCR analysis included three independent technical repeats with three biological replicates. The relative expression level of genes was determined by the 2−ΔΔCT method using 18S rRNA as the respective reference gene. The primers for qPCR are listed in Supplementary Table S2.
2.9 Statistical Analysis
The data are expressed as the mean and standard deviation. The significance of differences between treatments was determined with SPSS (version 22.0, SPSS Institute, Chicago, USA) using Duncan’s multiple range test at the p < 0.05 level of significance.
3 Results
3.1 Spermidine Synthase Gene in G. lemaneiformis
3.1.1 Bioinformatics Analysis of GlSPDS
Firstly, we obtained full-length ORF of SPDS gene based on genome annotation and the full-length transcriptome of G. lemaneiformis. It contains an ORF of 855 bp that encodes a polypeptideof 284 amino acids. The detailed information concerning molecular mass and isoelectric point is also listed in Table 1. The protein domain analysis of SPDS shows that it contains the spermidine synthase tetramerization domain (Spermine_synt_N) and the spermidine synthase domain (Spermidine_synth) based on protein sequence analysis by Pfam matches (Supplementary Figure S1). Blastp search against NCBI showed that the amino acid sequence had 100% identity to the spermidine synthase in Gracilariopsis chorda.(PXF47790.1). Thus, we referred to the protein as GlSPDS that converts put rescine to spermidine.
To analyze the homology of SPDS gene between algal and higher plant, sequence alignment and phylogenetic tree analysis were performed (Figure 1 and Supplementary Figure S1B). In the present study, we chose 19 representative SPDS/SPMS protein sequences from higher plants (7) and algae (12) to assess their sequence similarity. Phylogenetic tree and pairwise distances analysis showed that GlSPDS had the most close homology with SPDS1 in Thalassiosira pseudonana and Phaeodactylum tricornutum, which were clustered into one subgroup (Figure 1 and Supplementary Figure S2A). Alignment of GlSPDS with other SPDS/SPMS proteins showed that the GlSPDS shared conserved domain regions, confirmed the close relationship between plants and algae (Supplementary Figure S2). Since SPDS/SPMS all contained the conserved spermine_synth domain, which are important for catalytic activity.
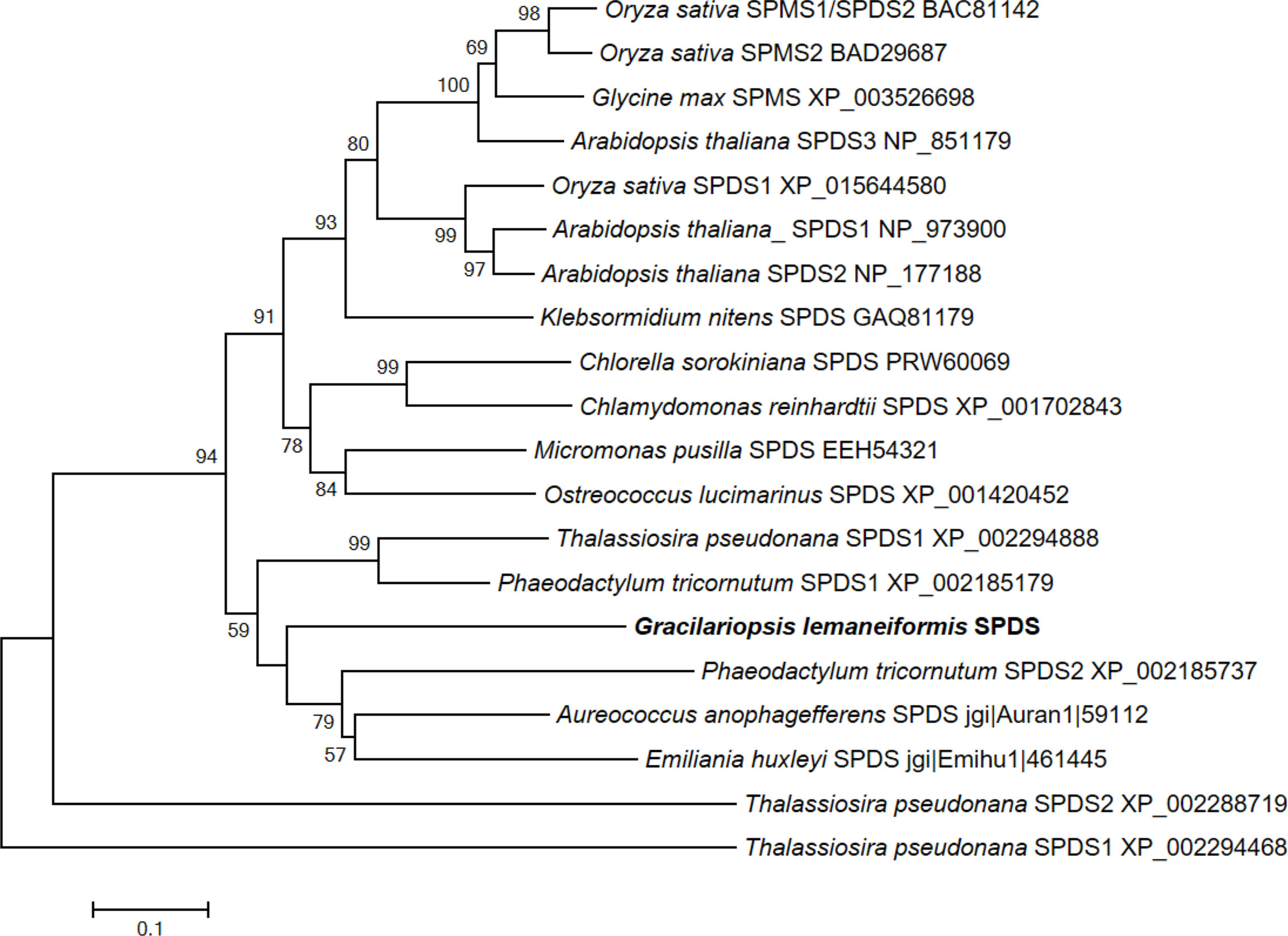
Figure 1 Phylogenetic tree analysis of GlSPDS with other algae and plant SPDSs. Phylogenetic tree was constructed by neighbor-joining method using MEGA6 program. Percentage bootstrap values of 1,000 replications are indicated at each node. Public database accession numbers of SPDS proteins are shown following the protein nomenclature.
3.1.2 Responsiveness of GlSPDS to Stress
The justification on the selection of stress treatments can be referred to Lv et al. (2019). We examined the responsiveness of GlSPDS to high temperature stress. As shown in Figure 2, the expression level of GlSPDS in CK treatment remained almost unchanged during a period of 48 h, whereas the expression level in HT-treated algae increased significantly, leading to an approximately fivefold increase over that in CK-treated algae at 6 h, particularly. Moreover, we also analyzed the expression changes of GlSPDS gene to other abiotic stress such as low temperature (LT), high salinity (HS), and low salinity (LS). The expressions of GlSPDS were induced significantly with peaks at 6 or 12 h, which shared almost similar expression patterns with that under HT stress (Supplementary Figure S3). These results indicate that the GlSPDS gene can respond to a wide array of abiotic stresses.
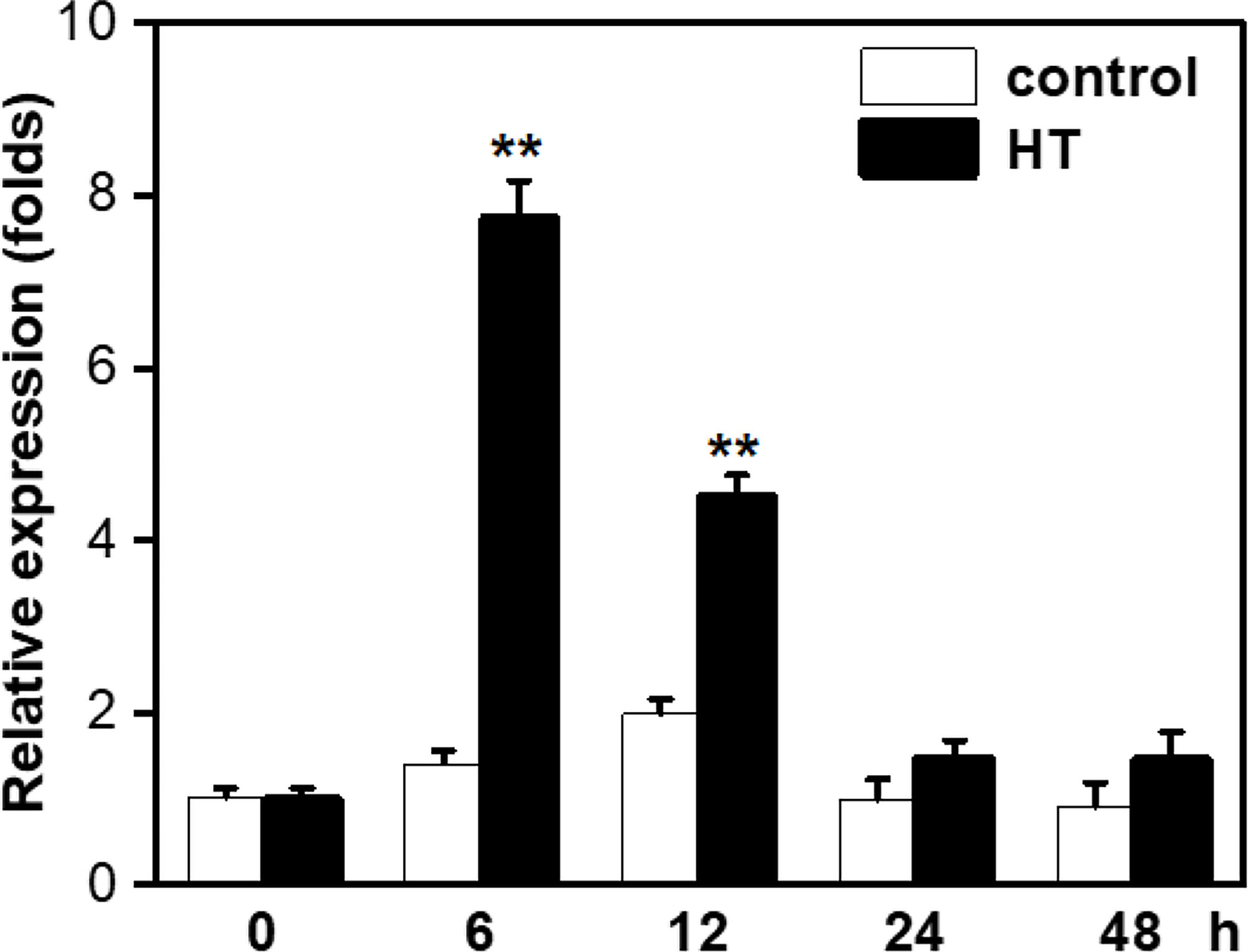
Figure 2 Expression of GlSPDS in response to high temperature (HT) stress. Algal samples with HT treatment and control treatment in an incubator were collected at indicated time points for analysis of gene expression. 18S Actin was used as an internal reference gene and relative expression was shown as folds of the transcript value of the 18S Actin gene. Data presented are the means ± standard errors (SE) from three independent experiments.
3.1.3 Subcellular Localization of GlSPDS
Then we examined the subcellular localization of GlSPDS, the coding sequence of GlSPDS was fused in-frame with GFP in pCambia 2300-GFP vector and transiently expressed in leaves of N. benthamiana harboring a red nuclear marker RFP-H2B protein. Microscopic observations of the agroinfiltrated N. benthamiana leaves collected at 48 h after agroinfiltration revealed that the GFP-tagged GlSPDS fusion was localized to the nucleus and plasma membrane similar to GFP alone (Figure 3A). To verify that the fusion protein was successfully expressed in N. benthamiana and better confirm the predicted molecular weight of GlSPDS, we examined through western blotting the detection of the GFP tags in N. benthamiana leaves at 48 h after infiltration. As shown in Figure 3B, the GFP : GlSPDS fusion was detected as revealed by the band of ~60 kDa, while molecular weight of GFP is approximately 26 kDa. This is consistent with the predicted molecular mass 32 kDa, and prokaryotic expression of HIS : GlSPDS fusion protein (~45 kDa) also showed the reliability of this result (Supplementary Figure S4).
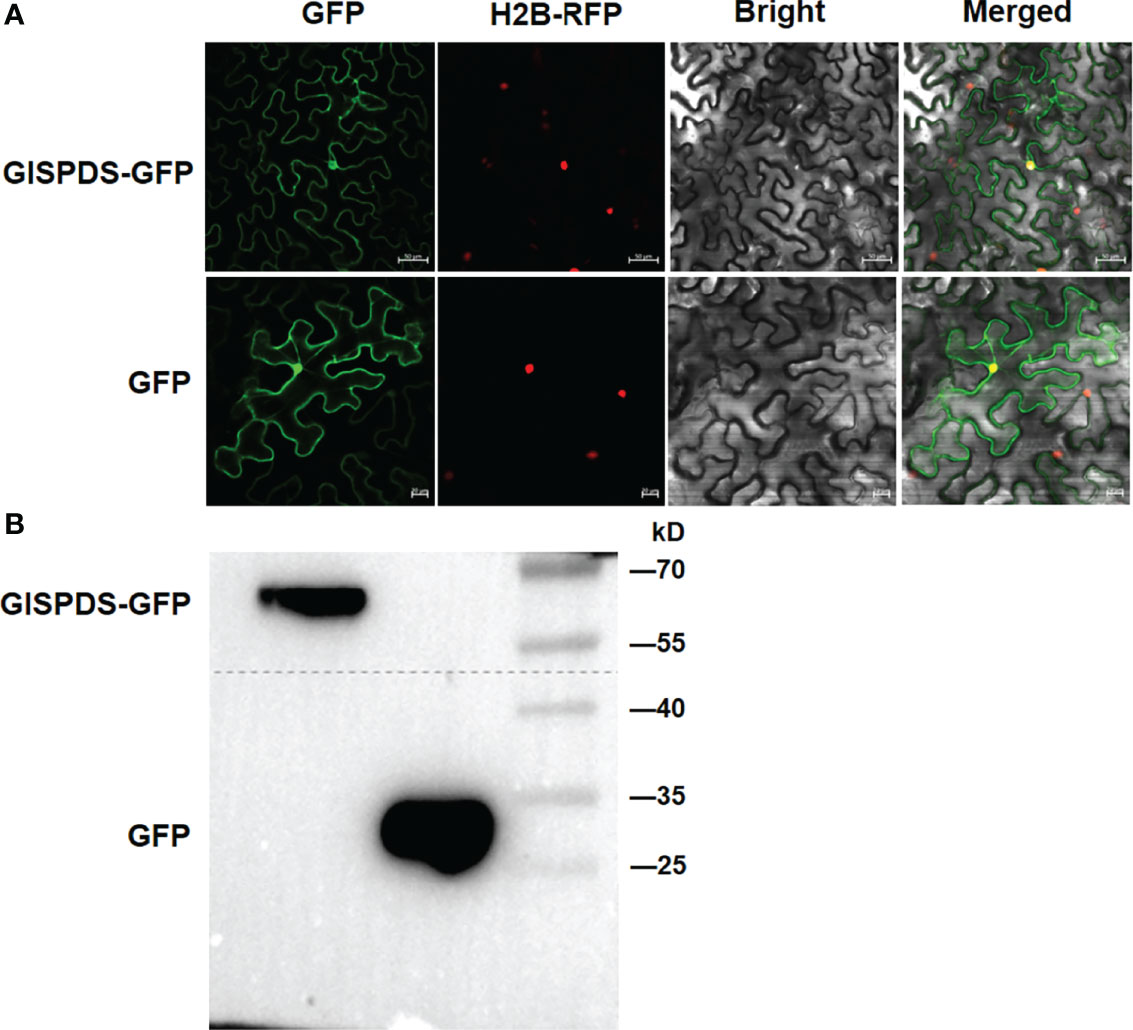
Figure 3 Subcellular localization and western blotting detection of GlSPDS protein. (A) Subcellular localization of GlSPDS protein. Agrobacteria carrying pCambia 2300-GFP-GlSPDS or pCambia 2300-GFP were infiltrated into leaves of N. benthamiana expressing a red nucleus marker RFP-tagged histone H2B (H2B-RFP) and the images were taken at 48 h after infiltration under dark field for green fluorescence (left) and red fluorescence (middle right), white field for cell morphology (middle left) and in combination (right), respectively. (B) Western blotting detection of GlSPDS-GFP fusion protein. Total proteins were resolved by SDS-PAGE and probed with anti-GFP antibody.
3.2 Physiological Analysis of Exogenous PA on G. lemaneiformis Under High Temperature
3.2.1 Relative Growth Rate
Since the PA synthetic gene GlSPDS markedly response to high temperature, we aimed to reveal the role of exogenous PA [spermidine (SPD) and spermine (SPM)] on G. lemaneiformis under high temperature. As our previous study indicated, RGR of G. lemaneiformis at 30/30°C was significantly decreased compared with 23/23°C (Zhang et al., 2021). In the present study, we first examined whether SPD and SPM could alleviate HT-induced growth decline of G. lemaneiformis. Therefore, different concentrations (0, 0.1, 0.5, 1, and 2 mM) SPD and SPM were applied into G. lemaneiformis culture under HT stress, respectively. During our repeated experiments, we found that the algae thalli in the PA treatments (0.1 and 0.5 mM) after 14 days of heat stress exhibited an alleviated yellowing phenotype as compared with no PA treatments (0 mM PAs) in G. lemaneiformis, while 2 mM PA caused algal thalli to turn white due to an excessive concentration (Figure 4A), which might suggest an alleviating effect of relatively low concentration of PA on growth of G. lemaneiformis under HT stress. To further determine the most effective and economical concentration, we measured the RGR of algae thalli at day 7, it indicated that algae in 0.5 mM SPD and 0.5 mM SPM treatments was significantly greater than that of the control culture (0 mM PA), which suggested that the 0.5 mM PA did have the most obviously promoting effect (Figure 4B). Thus, 0.5 mM SPD/SPM was used in the following experiments.
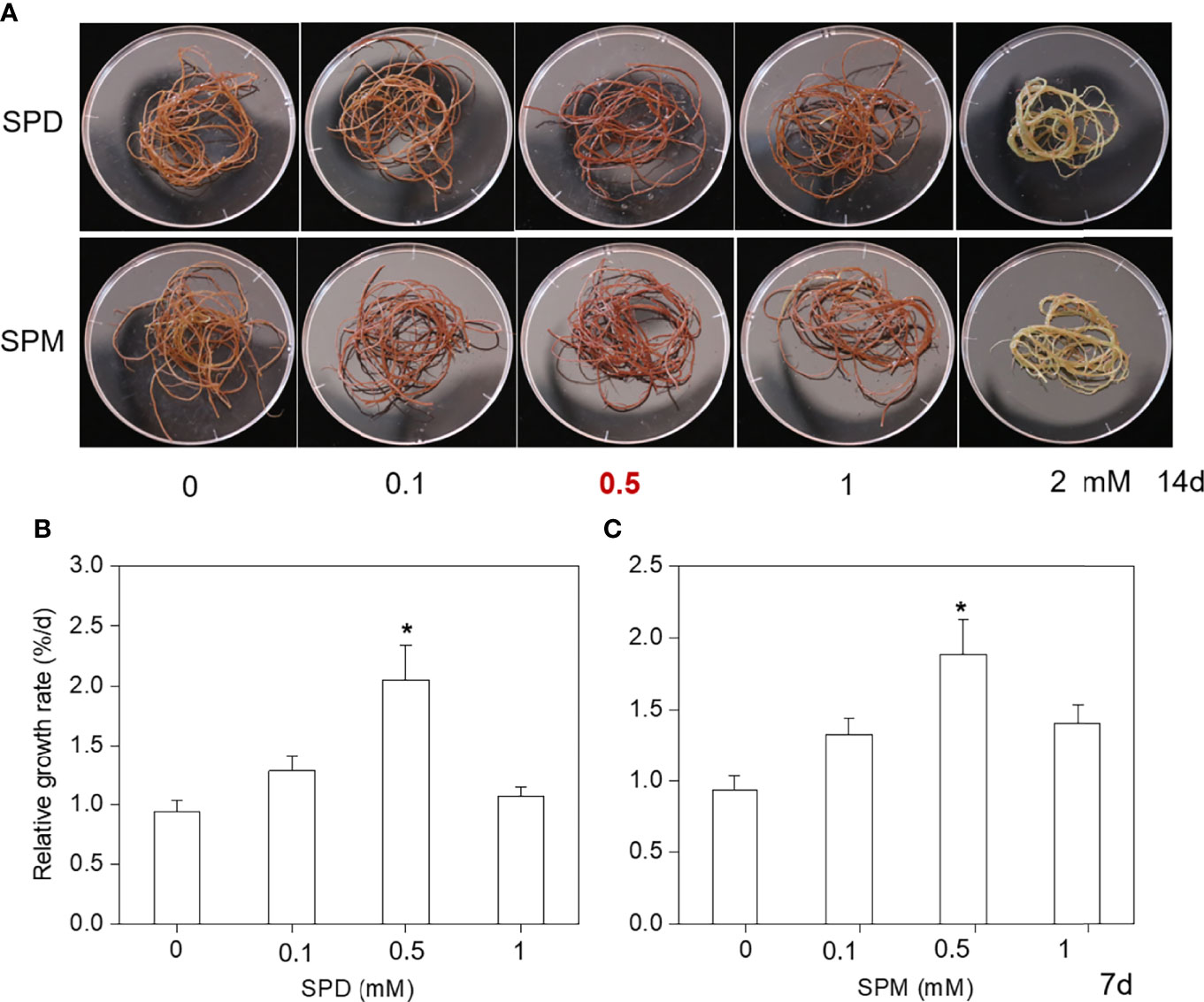
Figure 4 Effect of exogenous PA (SPD and SPM, respectively) at different concentrations on Glemaneiformis under HT stress. (A) Morphological changes of Glemaneiformis during different concentrations (0, 0.1, 0.5, 1, and 2 mM) of SPD and SPM for 14 days high temperature stress treatment. (B) The relative growth rate (RGR) of algae thalli during different concentrations (0, 0.1, 0.5, and 1 mM) of SPD at day 7. (C) The relative growth rate (RGR) of algae thalli during different concentrations (0, 0.1, 0.5, and 1 mM) ofSPM at day 7. Data presented are the means ± standard deviation (SD) from three independent experiments and the asterisk (*) above the columns indicates significant differences at the p < 0.05 level in the algae thalli under HT stress among the different concentrations of PAs treatments and the CK (without PA) treatment.
3.2.2 MDA and Antioxidant Enzyme Activities
To explore the possible role of exogenous application of SPD and SPM on membrane lipid peroxidation under HT stress, respectively, we measured the content of malondialdehyde (MDA) and antioxidant enzyme activities in thalli under HT with or without PA treatments after 1, 2, and 3 days of incubation. We found that heat stress increased MDA content and reduced the activities of superoxide dismutase (SOD) and catalase (CAT) after 3 days of treatment (Figure 5). However, exogenous supplementation of SPD and SPM, respectively, reduced the MDA content compared to CK groups under HT stress. SPD treatment increased the activities of SOD, CAT, and POD at day 1, 2, and 3, while PA-treated algae showed significant increased enzyme activities at day 2, particularly, when compared to control group at each time point under high temperature (Figures 5B, D). These data clearly revealed the positive function of SPD and SPM in enhancing algae thalli tolerance to HT stress by reducing ROS-induced membrane lipid peroxidation.
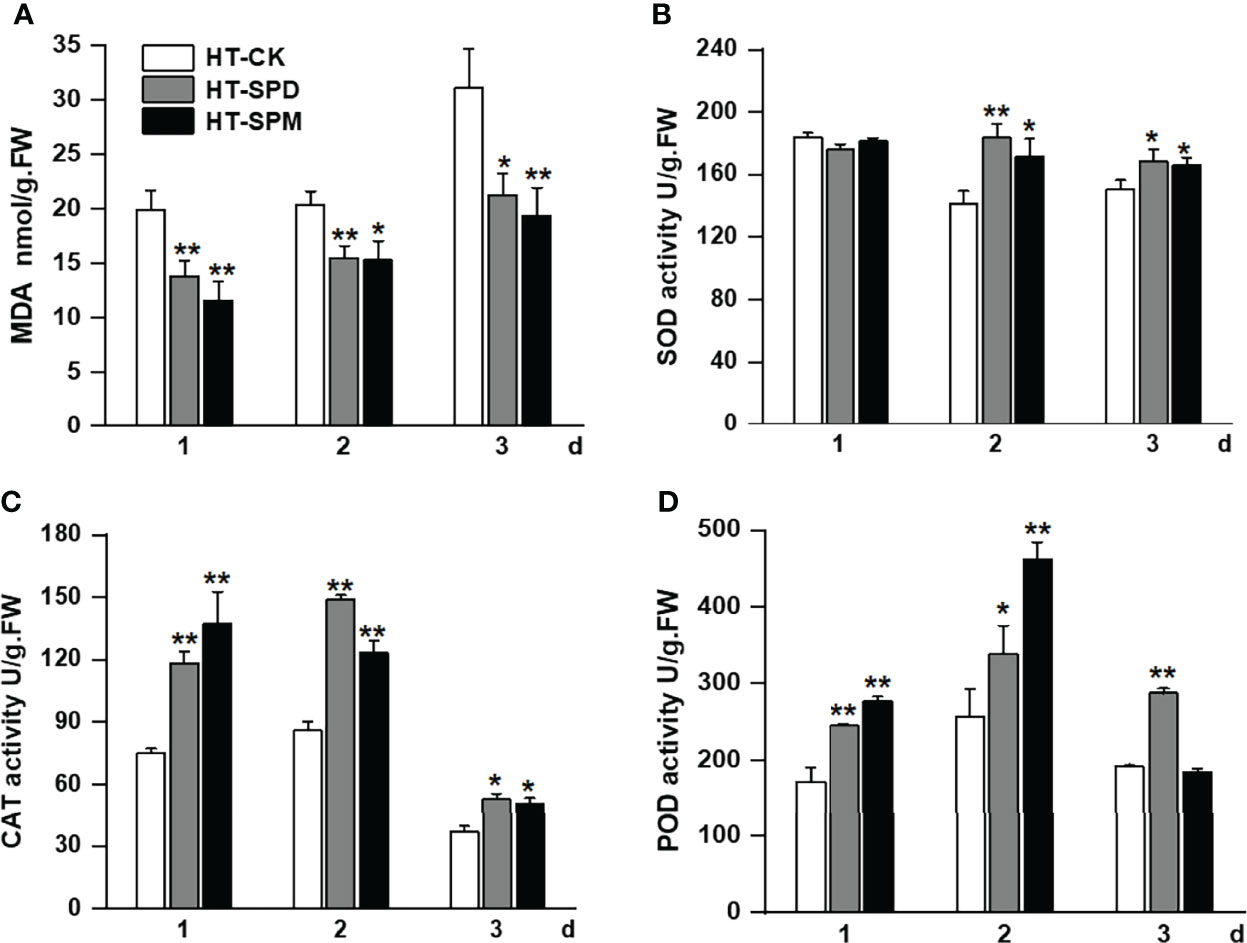
Figure 5 Effect of PA on malondialdehyde (MDA) content and antioxidant activity under HT stress. (A) MDA contents, (B) superoxide dismutase (SOD) activities, (C) catalase (CAT) activities, and (D) peroxidase (POD) activities of Glemaneiformis under HT stress treatment. Data presented are the means ± SD from three independent experiments, and different letters above the columns indicate significant differences at *p < 0.05 and **p < 0.01, respectively.
3.3 Transcriptome Analysis
3.3.1 Sequence Assembly and Unigenes Annotation
To reveal the mechanism of the effect of PA in enhancing G. lemaneiformis tolerance to HT stress, the thalli from both the SPD, SPM, and CK groups after HT treatment for 24 h were subjected to RNA-seq analysis. Sequence analysis of G. lemaneiformis generated 21.57–21.66 M total clean reads from 9 samples and obtained a total of 22,950 unigenes. The detailed information of each sample was listed in Supplementary Table S4. Functional annotation of genes was achieved by mapping genes to different databases (TFs, PRG, KEGG, GO, and NR) using BLAST searches with an e-value cutoff of 1 × 10−5.
3.3.2 Gene Expression Comparison
PCA was performed to describe differences of RNA sequencing gene expression between the SPD, SPM, and CK groups at 24 h. In this study, significant transcriptional changes in G. lemaneiformis were observed under HT stress (Supplementary Figure S5A). Genes (2,524) were differentially expressed between SPD and CK at 24 h (1,153 increased and 1,371 decreased), and 3,927 genes were differentially expressed between SPM and CK at 24 h (2,217 increased and 1,710 decreased) as shown in Supplementary Figure S4B. Criteria of log2|fold change|≥1 and stringent FDR values ≤0.001 were used as thresholds to identify differentially expressed genes (DEGs). To validate the reliability of the RNA-Seq results, 11 unigenes were randomly selected from the DEGs for qRT-PCR analysis. The expression patterns of the 11 unigenes were generally in agreement with the RNA-Seq results (Supplementary Table S3).
3.3.3 GO and KEGG Pathway Analysis
To analyze the functions of the DEGs, GO and KEGG enrichment analyses were performed. GO analysis showed that many terms, including DNA metabolic process, DNA integration, membrane, peroxidase activity, etc., that enriched among the DEGs co-regulated both in SPD and SPM treatment, possibly suggesting the role of PA in response to HT stress at the DNA and cell membrane level (Supplementary Table S5). We then focused on the KEGG analysis, 11 most enriched KEGG pathways were discovered between SPD/SPM and CK at 24 h (Figure 6). Among them, there were 6 shared pathways including ascorbate and aldarate metabolism, glycolysis, starch and sucrose metabolism, pyruvate metabolism, betalain biosynthesis, and biotin metabolism at 24 h. These data indicated that most of the DEGs clustered into antioxidant systems and energy metabolism that helped us to reveal the following possible mechanism for the promoting effect of PA on G. lemaneiformis resistance to HT stress.
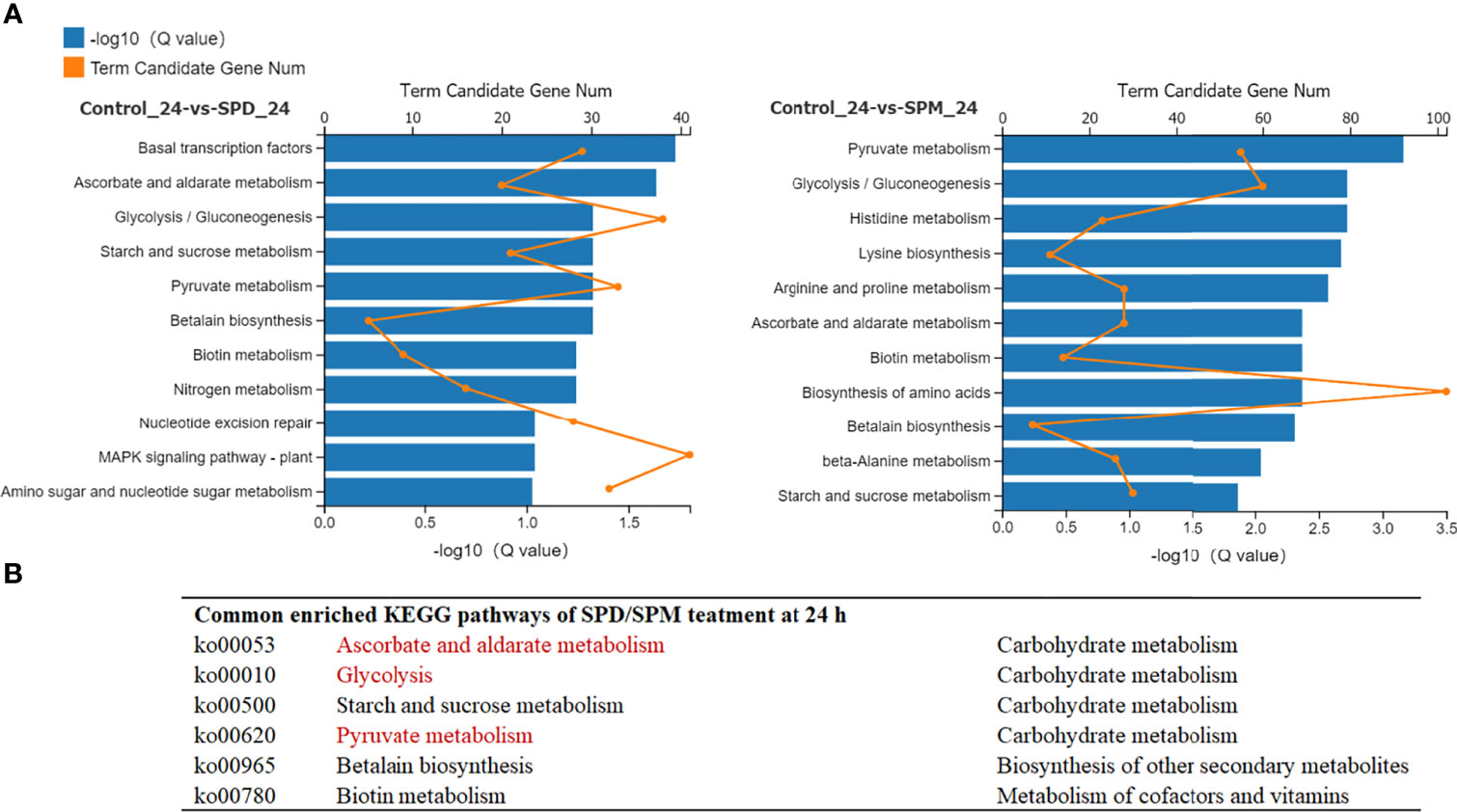
Figure 6 Overview of transcriptome changes of PA-induced differentially expressed genes (DEGs) at 24 h under HT stress. (A) Enriched KEGG pathways of DEGs between the SPD (or SPM)-treated and CK groups at 24 h, respectively. (B) The common enriched KEGG pathways of SPD and SPM at 24 h, respectively. The values shown are the averages from three biological replicates.
3.4 Functional Analysis of DEGs
3.4.1 DEGs Encoding Antioxidant Enzymes
In our study, the expression of unigenes encoding ROS scavenging enzymes was significantly changed in G. lemaneiformis with SPD and SPM treatments under HT stress (Supplementary Table S6). Exogenous PA activated genes encoding ascorbate peroxidase (APX), catalase (CAT), peroxiredoxin (PRXR), and glutaredoxin (GRX) at 24 h under stress. For example, PA upregulated expression of APX (Log2 SPD/CK ≤ 5.21, Log2 SPM/CK ≤ 6.03) in different degrees. Exogenous SPD and SPM also remarkably elevated the expression of CAT gene with a log2 fold change of 5.55 and 7.28, respectively. Additionally, genes encoding peroxidase (POD) exhibited different expression patterns, while most unigenes were upregulated in PA-treated algae as was shown in Supplementary Table S6. In summary, these results indicated that most DEGs encoding ROS scavenging enzymes in G. lemaneiformis could be significantly changed by SPD and SPM under HT stress.
3.4.2 DEGs Involved in Ascorbate and Aldarate Metabolism
Ascorbate and aldarate metabolism is also an antioxidant defense-related pathway. In this process, it involves L-galactose 1-phosphate phosphatase (VTC4), L-galactose dehydrogenase (GalDH), L-galactono-1,4-lactone dehydrogenase (GLDH), and monodehydroascorbate reductase (NADH; Figure 7 and Supplementary Table S7). In our study, all the genes involved in ascorbate and aldarate metabolism were significantly upregulated at SPM treatment, while most of the unigenes encoding for VTC4 and NADH showed no significant changes in SPD-treated algae, when compared to CK group (0 mM PA) under high temperature. For example, SPM upregulated expression of VTC4, GalDH, and GLDH with a log2 fold change of 2.12, 1.09–2.07, and 2.05, respectively, while exogenous SPD slightly elevated those genes (Log2 SPD/CK ≤ 1.25). The detailed information of fold changes was listed in Supplementary Table S7.
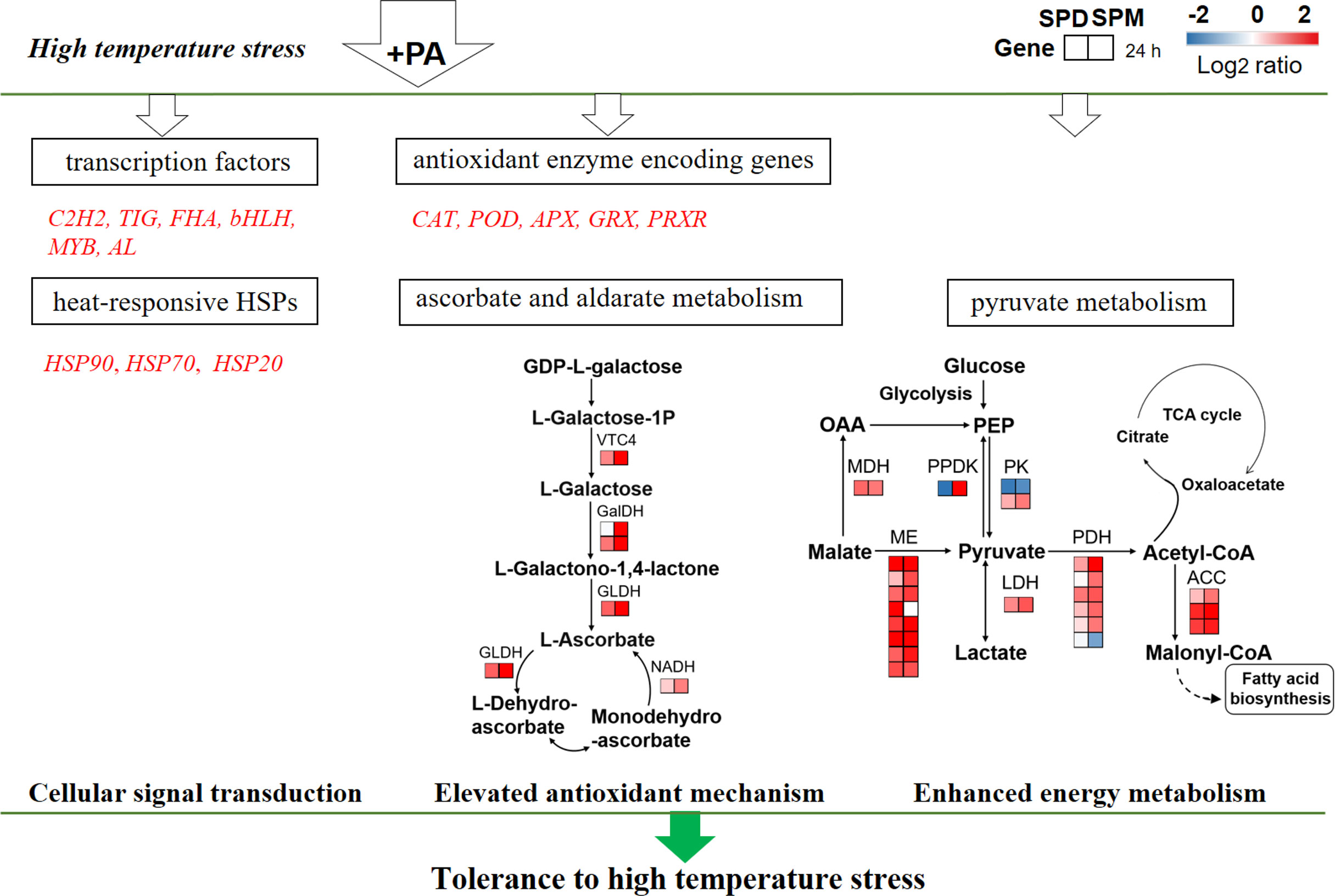
Figure 7 PA-induced transcriptomic changes of DEGs and pathways under HT stress. For DEGs analysis, the red font indicates upregulated genes between the PA-treated and CK groups. Each square represents the log2 FC level of the corresponding DEG at 24 h, respectively. A red square indicates upregulation and a blue square indicates downregulation. The ratios of gene expression levels between the PA-treated and CK groups are listed in Supplementary Table S8. Abbreviations: C2H2, cys2his2-type zinc-finger protein family; TIG, tetanus immune globulin family; FHA, fork head-associated domain (FHA domain) containing protein; bHLH, basic helix–loop–helix protein family; MYB, myeloblastosis family; AL, alfin-like transcription factor family; CAT, catalase; POD, peroxidase; APX, ascorbate peroxidase; GRX, glutaredoxin; PRXR, peroxiredoxins; HSP, heat shock protein.
3.4.3 DEGs Involved in Pyruvate Metabolism
According to the results of transcriptome analysis, many genes involved in pyruvate metabolism were significantly induced by SPD and SPM under heat stress. In the process, it referred to many enzymes such aspyruvate kinase (PK), malate dehydrogenase (MDH), malate dehydrogenase (ME), orthophosphate dikinase (PPDK), pyruvate dehydrogenase complex (PDH), and acetyl-CoA carboxylase (ACC; Figure 7). In the present study, almost all genes excluding PK were significantly upregulated both at SPD and SPM treatments. For example, exogenous PA upregulated expression of MDH with a log2 fold change of 1.2 (Log2 SPD/CK) and 1.06 (Log2 SPM/CK), while the expression of ME showed more varied fold changes (0.52 ≤ Log2 SPD/CK ≤ 5.08; 1.37 ≤ Log2 SPM/CK ≤ 4.86; Supplementary Table S8). Particularly worth mentioning is the PDH compound enzymes [(pyruvate dehydrogenase (PDH), dihydrolipoamide acetyltransferase (DLAT), and dihydrolipoamide dehydrogenase (DLD)] that leading to TCA cycle for energy supply and the genes encoding ACC related to fatty acid (FA) biosynthesis. The expression of those unigenes critical for TCA and FA biosynthesis was significantly induced at SPM treatment under HT stress (Figure 7 and Supplementary Table S8).
3.4.4 DEGs Involved in Nucleotide Excision Repair
As shown in Figure 8, there are two sub-pathways of nucleotide excision repair (NER), global genome repair (GGR), and transcription coupled repair (TCR). The expression of DEGs involved in NER was significantly changed by SPD and SPM treatments under high temperature. In NER, exogenous PA induced the homolog of yeast Rad23 protein (HR23B) and Cockayne syndrome protein (CSB) which act as essential factors to initiate GGR and TCR machinery, respectively. In the following DNA unwinding for transcription by NER, it involved the 7-subunit transcription factor IIH (TFIIH) Core sub-complex, the CDK-activating-kinase CDK7, XPB, and XPD. Then it comes to the replication factor C (RFC) that recruits DNA polymerase clamp (PolD1) to start DNA repair synthesis, and lastly DNA ligase I (Lig1) completes the BER. In the process, almost all genes encoding above enzymes were significantly upregulated at 24 h in SPM treatment compared to CK group under high temperature (Figure 8 and Supplementary Table S9). However, most of the genes involved in RFC, PolD1, and PolE showed no significant changes in SPD treatment, while other genes in NER were markedly induced as was shown in Supplementary Table S9. In summary, exogenous application of PA possibly activate the NER pathway to maintain the genome integrity from the DNA level under high temperature adversity.
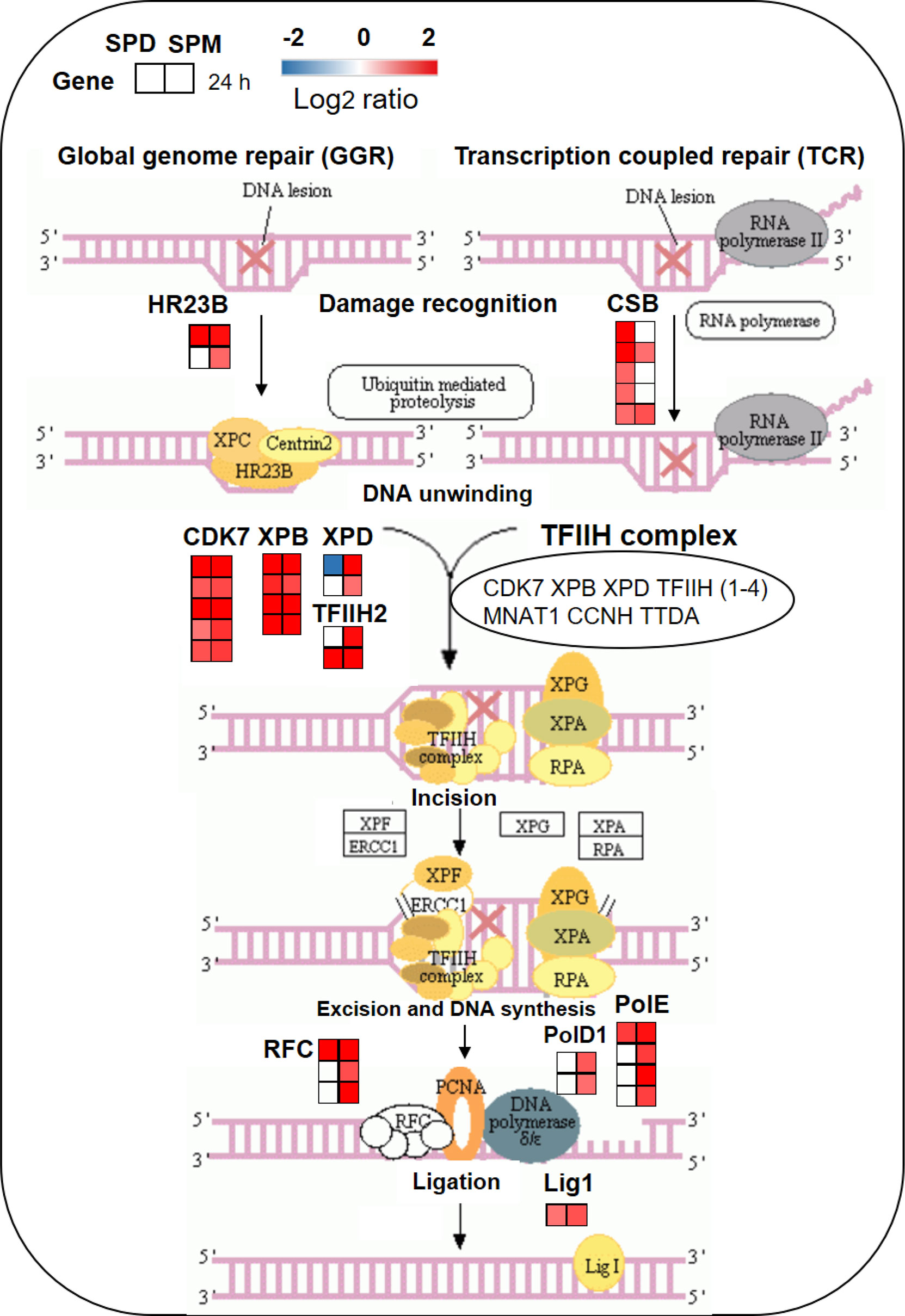
Figure 8 PA-induced transcriptomic changes of DEGs in nucleotide excision repair under HT stress. For pathway analysis, each square represents the log2 FC level the corresponding DEG at 24 h, respectively. A red square indicates upregulation and a blue square indicates downregulation. The ratios of gene expression levels between the PA-treated and CK groups are listed in Supplementary Table S9.
3.4.5 DEGs Encoding HSPs
Transcriptome analysis revealed that genes related to heat shock proteins (HSPs) including HSP90, HSP70, and HSP20 proteins were markedly changed under HT stress with PA (SPD and SPM) treatment at 24 h, respectively. Those HSP encoding genes exhibited different patterns between PA and CK treatment under HT stress, and most of the unigenes were upregulated. It showed 1 upregulated HSP90 (1 transcript) and 5 upregulated HSP20s (8 transcripts) in both SPD and SPM treatment. It was worth mentioning that HSP20 family proteins were most significantly activated by PA as shown in Table 2.
3.4.6 DEGs Encoding Transcription Factors
According to the results shown in Table 3, most unigenes were annotated into 13 transcription factors (TFs) families including Cys2His2 (C2H2)-type zinc-finger protein, the v-myb avian myeloblastosis viral oncogene homolog (MYB) family, tetanus immune globulin (TIG) family, forkhead-associated (FHA) domain containing protein and the basic/helix–loop–helix (bHLH) family, etc. Most of the TFs encoding unigenes (29 transcripts) were markedly activated by SPD/SPM treatments under HT stress. Among these, 14 transcripts (12 up- and 1 downregulated)encoding C2H2 differentially expressed between SPD/SPM and CK at 24 h, while the unigenes in SPM group exhibited relatively high expression ratio compared to the SPD treatment (Table 3). Moreover, the expression of other TFs including MYB, Sigma70-like, and bHLH in SPM group was also more upregulated than SPD treatment in response to high temperature. These data indicated that PA could regulate thermotolerance associated with the activation of most TFs, especially C2H2 and MYB in G. lemaneiformis.
4 Discussion
High temperature (HT) is considered to be one of critical environmental factors inhibiting algal growth and yield. Polyamines (PAs), such as spermidine (SPD) and spermine (SPM), have been described as important plant growth regulators (PGRs), functioning as the regulator of plant growth and senescence, including resistance to heat stress (Savvides et al., 2016; Hussain et al., 2019). Spermidine synthase encoded by spermidine synthase (SPDS) gene is responsible for SPD biosynthesis (Pothipongsa et al., 2016). SPDS can positively regulate tolerance to multiple environmental stresses (cold, heat, and salt) in Arabidopsis (Kasukabe et al., 2004), in Ipomoea batatas (Kasukabe et al., 2006), and in apple (Wen et al., 2008). For example, the apple SPDS1-overexpressing transgenic pear plants were revealed to have high SPD accumulation and showed tolerance to stresses (Wen et al., 2008). In the present study, we characterized a SPDS in G. lemaneiformis, localized in the cytoplasm and the nucleus, and the transcription of GlSPDS was markedly upregulated after high-temperature treatment (Figures 1, 3).As for the bioinformatics analysis of GlSPDS in our study, GlSPDS inG. Lemaneiformis showed great similarity in 30–40 kDa molecular weight to known spermidine synthases in many species (Parvin et al., 2010; Jiang et al., 2019), suggesting that SPDS gene is highly conserved during evolution. SPDS belongs to the putrescine aminopropyltransferase family, which contains conserved motif and amino acid residues that are proposed to be critical for the catalytic activity of this enzyme (Korolev et al., 2002). The alignment of SPDS in the present study revealed that the catalytic sites were also observed in G. lemaneiformis. Protein domain analysis also showed that it contained the N-terminal tetramerization domain and the spermidine synthase domain, which had roles in catalyzing spermidine biosynthesis (Supplementary Figure S1). However, we still need further study to prove it is a functional SPDS with enzymatic activity in the following. But we could speculate that GlSPDS possibly be involved in HT stress response and potentially provided the gene pool for obtaining stress-tolerant algae in future.
As previous study showed, HT could increase the expression levels of SPDS genes in rice, that ultimately increasing the levels of endogenous spermidine (SPD) and spermine (SPM), which mediated many physiological processes, including stress response (Zhou et al., 2020). It further suggested a pivotal role of polyamines in the tolerance to environmental stresses, but direct evidence for the involvement of polyamine in stress tolerance is still lacking in algae.In this study, algae thalli with exogenous SPD or SPM showed higher relative growth rate (RGR) compared to CK group under high temperature (Figure 4), indicating a positive role of PA in algae developmental and physiological processes in G. lemaneiformis under stress conditions. This is similar with the fact that exogenous polyamines such as SPD or SPM could enhance heat tolerance of rice seeds and wheat grain-filling from physiological parameters (Fu et al., 2019; Jing et al., 2020). However, the mechanism(s) underlying the involvement of polyamine in HT stress responses in G. lemaneiformis is not known.
High temperature imposed oxidative stress, however, plants and algae are efficient in enhancing antioxidative defense system through both nonenzymatic and enzymatic antioxidants such as superoxide dismutase (SOD), catalase (CAT), ascorbate peroxidase (APX), glutaredoxin (GRX), and phenolic compounds, which ultimately reduce lipid peroxidation (Ahmad et al., 2010; Gill and Tuteja, 2010b). Effects of PA on improving antioxidant defense have been reported in many plant species under different abiotic stresses. Exogenous application of PA could enhance an antioxidant defense by improving the activity of enzymes mentioned above under metal/metalloid(s) stress (Groppa et al., 2007; Nahar et al., 2016). In response to heat stress, spraying 1 mM SPD also alleviated the oxidative damage to the lettuce seedlings by enhancing the antioxidant system associated with a significant decrease in MDA content (Li et al., 2020). In current study, exogenous PA (SPD and SPM) elevated the activities of SOD, CAT and POD in G. lemaneiformis under HT stress, which also showed a decrease in MDA content (Figure 5). Moreover, DEG analysis showed that PA treatment enhanced the transcript levels of genes encoding many antioxidant enzymes, such as CAT, POD, APX, and GRX, whereas changes in the level of SOD encoding genes in PA treatment group were not significant (Supplementary Table S6).At this point, we verified that PA could actively participate in mitigating oxidative damage in algae via enhancement of antioxidant enzyme activities and protecting membrane lipid peroxidation under HT stress condition.Exogenously applied SPD and SPM but not PUT had a potential to protect Arabidopsis from HT-induced damage, and SPM increases the HT response at transcriptional and translational levels associated with the increased expression of the HT-related transcription factors (TFs) and heat shock proteins (HSPs; Sagor et al., 2013). It has also been shown that overexpression of the SPDS gene could induce expressions of stress-regulated genes and many transcription factors, such as WRKY, B-box zinc finger proteins, and the v-myb avian myeloblastosis viral oncogene homolog (MYB; Kasukabe et al., 2004). At this point, we speculated that SPDS-mediated endogenous PA or exogenous PA might interact with TFs and other signaling molecules in response to plant stresses. TFs, such as basic leucine zipper (bZIP), MYB, and Cys2His2 (C2H2)-type zinc-finger protein, binding to the promoters of small HSPs genes, could confer multiple stress tolerance (Sagor et al., 2013; Wen et al., 2019). In our study, SPD and SPM increased the expression of TFs including C2H2 and MYB in G. lemaneiformis from DEG analysis (Table 3).HSP-family proteins mainly including HSP100, HSP90, HSP70, HSP60, and small HSP (sHSP), all of those families excluding sHSP are highly conserved across plants and algae (Wang et al., 2004; Sathasivam and Ki, 2019). Previous studies have supported beneficial functions of HSP90, HSP70, and sHSP on thermotolerance in plants (Xu et al., 2011; Xu et al., 2012), whereas in the primitive red alga Cyanidioschyzon merolae and green microalga Chlamydomonas reinhardtii and Scenedesmus vacuolatus, algae sense exact temperatures mainly via upregulating expression of sHSP-encoding genes (Kobayashi et al., 2014; Ko et al., 2019). Other study showed that high accumulation of small HSPs not HSP70A in Chlamydomonas acidophila was most related to metal stress (Spijkerman et al., 2007). Consistent with this conclusion, we found that exogenous PA significantly upregulated HSP90, HSP70 expression, in particular the sHSP in G. lemaneiformis under high temperature condition (Figure 7). Algal small HSPs were not members of the land plant sHSPs based on the phylogenetic analyses; they may possess foremost vitally important roles in algal cellular stress response (Kobayashi et al., 2014).PA has been reported to play critical roles in epigenetic modifications of DNA and histones (Pasini et al., 2014), while another level of complexity that has been little explored is the effect of PA in DNA damage repair pathways under HT conditions. Radiation exposure and ROS production are well known to cause DNA damage, while stressful high temperatures also disturb genome integrity by causing DNA damage (Tuteja et al., 2001; Gupta et al., 2020). However, the damaged lesions can be eventually repaired by removal of damaged DNA through base-excision repair or nucleoside excision repair that better adapting to environmental pressure (Sancar and Tang, 1993). Han et al. previously demonstrated that high expression of osmotically reponsive genes 1 (HOS1) induces thermotolerance by activating DNA repair components (Han et al., 2020). Previous study showed that the increased DNA damage was primarily due to the disruption of polyamine metabolism and attenuation of polyamine metabolism may contribute to the decline of DNA damage-repair efficiency (Zahedi et al., 2007; Manna et al., 2013). Nucleotide/base excision repair (BER) is a DNA repair process and that has an important role in preventing mutations associated with damaged bases and DNA single-strand break bases, which may facilitate cells survive in stressful damage (Yague-Capilla et al., 2019). Recent work demonstrates the novel cellular function of polyamines in the DNA damage response and maintenance of genome integrity via homology-directed DNA repair (Lee et al., 2019).Current results showed that SPD and SPM supplementation significantly upregulates the expression of BER pathway corresponding genes, such as HR23B, CSB, CDK7, XPB, XPD, TFIIH2, RFC, PolE, and Lig1, in G. lemaneiformis under HT stress (Figure 8). Therefore, exogenous PA is probably involved in preserving the genome integrity from the DNA level so as to alleviate the damage of high temperature induced oxidative stress in G. lemaneiformis.It is worth mentioning that in the present study, exogenous application of SPD and SPM showed similar effects but in different degrees when it comes to algal growth, enzymes of the antioxidative system, and the expression level of differently expressed genes under HT stress conditions. Previous study shows that they are of different importance although both are polyamines, SPD is thought to be more effective against environment stress than SPM in plant, and it has been reported as the major polyamine found in many species of cyanobacteria, which classified as the “blue-green algae” (Hosoya et al., 2005; Cha-um et al., 2006). However, there has been limited in evidence to decide whether SPD or SPM is more effective in alleviating high temperature stress in G. lemaneiformis. Moreover, the molecular evidence that might explain the differences needs to be further identified in future.
5 Conclusion
G. lemaneiformis often undergo sustained high temperature (HT) in summer and possibly evolved specific adaptations and tolerance mechanisms via regulating stress-related genes and signaling to survive. We characterized a polyamine (PA) synthetic gene (spermidine synthase gene, SPDS) in G. lemaneiformis, encoding a protein of 284 amino acid residues, which responded to multiple abiotic stresses including high temperature. It provided a new candidate gene into the “gene bank” that may be applicable to obtain thermotolerant transgenic algae with novel technologies in the future. Furthermore, we demonstrated that exogenous PA (SPD and SPM, respectively) alleviated algal damage from high temperature with a decline in MDA content. RNA-seq-based DEGs analysis showed that PA modulating HT stress tolerance via upregulated the genes encoding antioxidant enzymes (CAT, POD, APX, PRXR, and GRX); TF signaling and heat shock proteins (HSPs), especially the small HSPs; DNA damage repair pathways; these results revealed the possible mechanism of PA in G. lemaneiformis under HT stress.
Data Availability Statement
The original contributions presented in the study are included in the article/Supplementary Material. Further inquiries can be directed to the corresponding author.
Author Contributions
Conceptualization: SL and NX. Data curation: SL Formal analysis: SL and JZ. Funding acquisition: NX, SL, and XS. Supervision: SL and NX. Validation: XS. Visualization: SL. Writing—original draft: SL. Writing—review & editing: SL and NX. All authors contributed to the article and approved the submitted version.
Funding
This work was supported by the National Key R&D Program of China (2018YFD0901502), the National Natural Science Foundation of China (32001889; 31672674) and the Natural Science Foundation of Zhejiang Province (LY19C190003).
Conflict of Interest
The authors declare that the research was conducted in the absence of any commercial or financial relationships that could be construed as a potential conflict of interest.
Publisher’s Note
All claims expressed in this article are solely those of the authors and do not necessarily represent those of their affiliated organizations, or those of the publisher, the editors and the reviewers. Any product that may be evaluated in this article, or claim that may be made by its manufacturer, is not guaranteed or endorsed by the publisher.
Supplementary Material
The Supplementary Material for this article can be found online at: https://www.frontiersin.org/articles/10.3389/fmars.2022.939888/full#supplementary-material
References
Ahmad P., Jaleel C. A., Salem M. A., Nabi G., Sharma S. (2010). Roles of Enzymatic and Nonenzymatic Antioxidants in Plants During Abiotic Stress. Crit. Rev. Biotechnol. 30, 161–175. doi: 10.3109/07388550903524243
Alcazar R., Bueno M., Tiburcio A. F. (2020). Polyamines: Small Amines With Large Effects on Plant Abiotic Stress Tolerance. Cells 9 2373. doi: 10.3390/cells9112373
Cha-um S., Supaibulwatana K., Kirdmanee C. (2006). Water Relation, Photosynthetic Ability and Growth of Thai Jasmine Rice (Oryza Sativa L. Ssp. Indica Cv. KDML 105) to Salt Stress by Application of Exogenous Glycinebetaine and Choline. J. Agron. Crop Sci. 192, 25–36. doi: 10.1111/j.1439-037X.2006.00186.x
Fu Y., Gu Q., Dong Q., Zhang Z., Lin C., Hu W., et al. (2019). Spermidine Enhances Heat Tolerance of Rice Seeds by Modulating Endogenous Starch and Polyamine Metabolism. Molecules 24, 1395. doi: 10.3390/molecules24071395
Fu X. T., Kim S. M. (2010). Agarase: Review of Major Sources, Categories, Purification Method, Enzyme Characteristics and Applications. Mar. Drugs 8, 200–218. doi: 10.3390/md8010200
Gill S. S., Tuteja N. (2010a). Polyamines and Abiotic Stress Tolerance in Plants. Plant Signal Behav. 5, 26–33. doi: 10.4161/psb.5.1.10291
Gill S. S., Tuteja N. (2010b). Reactive Oxygen Species and Antioxidant Machinery in Abiotic Stress Tolerance in Crop Plants. Plant Physiol. Biochem. 48, 909–930. doi: 10.1016/j.plaphy.2010.08.016
Groppa M. D., Tomaro M. L., Benavides M. P. (2007). Polyamines and Heavy Metal Stress: The Antioxidant Behavior of Spermine in Cadmium- and Copper-Treated Wheat Leaves. Biometals 20, 185–195. doi: 10.1007/s10534-006-9026-y
Gupta S. K., Verma K., Kumar R., Sarkar B., Mantha A. K., Kumar S. (2020). Priming Alleviates High Temperature Induced Oxidative DNA Damage and Repair Using Apurinic/apyrimidinic Endonuclease (Ape1L) Homologue in Wheat (Triticum Aestivum L.). Plant Physiol. Biochem. 156, 304–313. doi: 10.1016/j.plaphy.2020.08.050
Han S. H., Park Y. J., Park C. M. (2020). HOS1 Activates DNA Repair Systems to Enhance Plant Thermotolerance. Nat. Plants 6, 1439–1446. doi: 10.1038/s41477-020-00809-6
Hosoya R., Hamana K., Isobe M., Yokota A. (2005). Polyamine Distribution Profiles Within Cyanobacteria. Microbiol. Cult Coll. 21, 3–8.
Hou S., Lin L., Lv Y., Xu N., Sun X. (2018). Responses of Lipoxygenase, Jasmonic Acid, and Salicylic Acid to Temperature and Exogenous Phytohormone Treatments in Gracilariopsis Lemaneiformis (Rhodophyta). J. Appl. Phycol 30, 3387–3394. doi: 10.1007/s10811-018-1514-2
Hussain A., Nazir F., Fariduddin Q. (2019). Polyamines (Spermidine and Putrescine) Mitigate the Adverse Effects of Manganese Induced Toxicity Through Improved Antioxidant System and Photosynthetic Attributes in Brassica Juncea. Chemosphere 236, 124830. doi: 10.1016/j.chemosphere.2019.124830
Jahan M. S., Hasan M. M., Alotaibi F. S., Alabdallah N. M., Alharbi B. M., Ramadan K. M. A., et al. (2022). Exogenous Putrescine Increases Heat Tolerance in Tomato Seedlings by Regulating Chlorophyll Metabolism and Enhancing Antioxidant Defense Efficiency. Plants (Basel) 11, 1038. doi: 10.3390/plants11081038
Jiang Y. R., Wang T. T., Chen D. B., Xia R. X., Li Q., Wang H., et al. (2019). Characterization of a Highly Conserved Antheraea Pernyi Spermidine Synthase Gene. 3 Biotech. 9, 224. doi: 10.1007/s13205-019-1762-0
Jing J., Guo S., Li Y., Li W. (2020). The Alleviating Effect of Exogenous Polyamines on Heat Stress Susceptibility of Different Heat Resistant Wheat (Triticum Aestivum L.) Varieties. Sci. Rep. 10, 7467. doi: 10.1038/s41598-020-64468-5
Kasukabe Y., He L. X., Nada K., Misawa S., Ihara I., Tachibana S. (2004). Overexpression of Spermidine Synthase Enhances Tolerance to Multiple Environmental Stresses and Up-Regulates the Expression of Various Stress Regulated Genes in Transgenic Arabidopsis Thaliana. Plant Cell Physiol. 45, 712–722. doi: 10.1093/pcp/pch083
Kasukabe Y., He L. X., Watakabe Y., Otani M., Shimada T., Tachibana S. (2006). Improvement of Environmental Stress Tolerance of Sweet Potato by Introduction of Genes for Spermidine Synthase. Plant Biotechnol. 23, 75–83. doi: 10.5511/plantbiotechnology.23.75
Kobayashi Y., Harada N., Nishimura Y., Saito T., Nakamura M., Fujiwara T., et al. (2014). Algae Sense Exact Temperatures: Small Heat Shock Proteins are Expressed at the Survival Threshold Temperature in Cyanidioschyzon Merolae and Chlamydomonas Reinhardtii. Genome Biol. Evol. 6, 2731–2740. doi: 10.1093/gbe/evu216
Ko T. H., Leu K. L., Hsu B. D., Lee T. C. (2019). Protein Expression Analysis in Reversible Photobleached Cells of Scenedesmus Vacuolatus After High Temperature Stress. Int. J. Mol. Sci. 20 3082. doi: 10.3390/ijms20123082
Korolev S., Ikeguchi Y., Skarina T., Beasley S., Arrowsmith C., Edwards A., et al. (2002). The Crystal Structure of Spermidine Synthase With a Multisubstrate Adduct Inhibitor. Nat. Struct. Biol. 9, 27–31. doi: 10.1038/nsb737
Kumari P., Reddy C. R., Jha B. (2015). Methyl Jasmonate-Induced Lipidomic and Biochemical Alterations in the Intertidal Macroalga Gracilaria Dura (Gracilariaceae, Rhodophyta). Plant Cell Physiol. 56, 1877–1889. doi: 10.1093/pcp/pcv115
Langmead B., Salzberg S. L. (2012). Fast Gapped-Read Alignment With Bowtie 2. Nat. Methods 9, 357–359. doi: 10.1038/nmeth.1923
Lee C. Y., Su G. C., Huang W. Y., Ko M. Y., Yeh H. Y., Chang G. D., et al. (2019). Promotion of Homology-Directed DNA Repair by Polyamines. Nat. Commun. 10, 65. doi: 10.1038/s41467-018-08011-1
Li C., Han Y., Hao J., Qin X., Liu C., Fan S. (2020). Effects of Exogenous Spermidine on Antioxidants and Glyoxalase System of Lettuce Seedlings Under High Temperature. Plant Signal Behav. 15, 1824697. doi: 10.1080/15592324.2020.1824697
Luo H., Wang Q., Liu Z., Wang S., Long A., Yang Y. (2020). Potential Bioremediation Effects of Seaweed Gracilaria Lemaneiformis on Heavy Metals in Coastal Sediment From a Typical Mariculture Zone. Chemosphere 245, 125636. doi: 10.1016/j.chemosphere.2019.125636
Lv Y., Sun P., Zhang Y., Xuan W., Xu N., Sun X. (2019). Response of Trehalose, its Degrading Enzyme, Sucrose, and Floridoside/Isofloridoside Under Abiotic Stresses in Gracilariopsis Lemaneiformis (Rhodophyta). J. Appl. Phycol 31, 3861–3869. doi: 10.1007/s10811-019-01869-8
Manna S. K., Krausz K. W., Bonzo J. A., Idle J. R., Gonzalez F. J. (2013). Metabolomics Reveals Aging-Associated Attenuation of Noninvasive Radiation Biomarkers in Mice: Potential Role of Polyamine Catabolism and Incoherent DNA Damage-Repair. J. Proteome Res. 12, 2269–2281. doi: 10.1021/pr400161k
Minguet E. G., Vera-Sirera F., Marina A., Carbonell J., Blazquez M. A. (2008). Evolutionary Diversification in Polyamine Biosynthesis. Mol. Biol. Evol. 25, 2119–2128. doi: 10.1093/molbev/msn161
Mittler R, Vanderauwera S, Gollery M, Van Breusegem F. (2004). Reactive Oxygen Gene Network of Plants.Trends Plant Sci. 9, 490–498. doi: 10.1016/j.tplants.2004.08.009
Nahar K., Hasanuzzaman M., Alam M. M., Rahman A., Suzuki T., Fujita M. (2016). Polyamine and Nitric Oxide Crosstalk: Antagonistic Effects on Cadmium Toxicity in Mung Bean Plants Through Upregulating the Metal Detoxification, Antioxidant Defense and Methylglyoxal Detoxification Systems. Ecotoxicol Environ. Saf. 126, 245–255. doi: 10.1016/j.ecoenv.2015.12.026
Parvin S., Kim Y. J., Pulla R. K., Sathiyamoorthy S., Miah M. G., Kim Y. J., et al. (2010). Identification and Characterization of Spermidine Synthase Gene From Panax Ginseng. Mol. Biol. Rep. 37, 923–932. doi: 10.1007/s11033-009-9725-x
Pasini A., Caldarera C. M., Giordano E. (2014). Chromatin Remodeling by Polyamines and Polyamine Analogs. Amino Acids 46, 595–603. doi: 10.1007/s00726-013-1550-9
Pegg A. E., Michael A. J. (2010). Spermine Synthase. Cell Mol. Life Sci. 67, 113–121. doi: 10.1007/s00018-009-0165-5
Pothipongsa A., Jantaro S., Incharoensakdi A. (2016). Spermidine Synthase is Required for Growth of Synechococcus Sp. PCC 7942 Under Osmotic Stress. Curr. Microbiol. 73, 639–645. doi: 10.1007/s00284-016-1107-8
Sagor G. H. M., Berberich T., Takahashi Y., Niitsu M., Kusano T. (2013). The Polyamine Spermine Protects Arabidopsis From Heat Stress-Induced Damage by Increasing Expression of Heat Shock-Related Genes. Transgenic Res. 22, 595–605. doi: 10.1007/s11248-012-9666-3
Sancar A., Tang M. S. (1993). Nucleotide Excision Repair. Photochem. Photobiol. 57, 905–921. doi: 10.1111/j.1751-1097.1993.tb09233.x
Sathasivam R., Ki J. S. (2019). Heat Shock Protein Genes in the Green Alga Tetraselmis Suecica and Their Role Against Redox and non-Redox Active Metals. Eur. J. Protistol 69, 37–51. doi: 10.1016/j.ejop.2019.01.006
Savvides A., Ali S., Tester M., Fotopoulos V. (2016). Chemical Priming of Plants Against Multiple Abiotic Stresses: Mission Possible? Trends Plant Sci. 21, 329–340. doi: 10.1016/j.tplants.2015.11.003
Singh K., Foley R. C., Onate-Sanchez L. (2002). Transcription Factors in Plant Defense and Stress Responses. Curr. Opin. Plant Biol. 5, 430–436. doi: 10.1016/S1369-5266(02)00289-3
Spijkerman E., Barua D., Gerloff-Elias A., Kern J., Gaedke U., Heckathorn S. A. (2007). Stress Responses and Metal Tolerance of Chlamydomonas Acidophila in Metal-Enriched Lake Water and Artificial Medium. Extremophiles 11, 551–562. doi: 10.1007/s00792-007-0067-0
Tuteja N., Singh M. B., Misra M. K., Bhalla P. L., Tuteja R. (2001). Molecular Mechanisms of DNA Damage and Repair: Progress in Plants. Crit. Rev. Biochem. Mol. Biol. 36, 337–397. doi: 10.1080/20014091074219
Wang L., Feng Z., Wang X., Wang X., Zhang X. (2010). DEGseq: An R Package for Identifying Differentially Expressed Genes From RNA-Seq Data. Bioinformatics 26, 136–138. doi: 10.1093/bioinformatics/btp612
Wang W., Paschalidis K., Feng J. C., Song J., Liu J. H. (2019). Polyamine Catabolism in Plants: A Universal Process With Diverse Functions. Front. Plant Sci. 10. doi: 10.3389/fpls.2019.00561
Wang W., Teng F., Lin Y., Ji D., Xu Y., Chen C., et al. (2018b). Transcriptomic Study to Understand Thermal Adaptation in a High Temperature-Tolerant Strain of Pyropia Haitanensis. PLoS One 13, e0195842. doi: 10.1371/journal.pone.0195842
Wang W., Vinocur B., Shoseyov O., Altman A. (2004). Role of Plant Heat-Shock Proteins and Molecular Chaperones in the Abiotic Stress Response. Trends Plant Sci. 9, 244–252. doi: 10.1016/j.tplants.2004.03.006
Wang R., Zhao P., Kong N., Lu R., Pei Y., Huang C., et al. (2018a). Genome-Wide Identification and Characterization of the Potato bHLH Transcription Factor Family. Genes (Basel) 9, 54. doi: 10.3390/genes9010054
Wen X. P., Pang X. M., Matsuda N., Kita M., Inoue H., Hao Y. J., et al. (2008). Over-Expression of the Apple Spermidine Synthase Gene in Pear Confers Multiple Abiotic Stress Tolerance by Altering Polyamine Titers. Transgenic Res. 17, 251–263. doi: 10.1007/s11248-007-9098-7
Wen X., Wang J., Zhang D., Wang Y. (2019). A Gene Regulatory Network Controlled by BpERF2 and BpMYB102 in Birch Under Drought Conditions. Int. J. Mol. Sci. 20, 3071. doi: 10.3390/ijms20123071
Xie B., Bishop S., Stessman D., Wright D., Spalding M. H., Halverson L. J. (2013). Chlamydomonas Reinhardtii Thermal Tolerance Enhancement Mediated by a Mutualistic Interaction With Vitamin B12-Producing Bacteria. ISME J. 7, 1544–1555. doi: 10.1038/ismej.2013.43
Xu Z. S., Li Z. Y., Chen Y., Chen M., Li L. C., Ma Y. Z. (2012). Heat Shock Protein 90 in Plants: Molecular Mechanisms and Roles in Stress Responses. Int. J. Mol. Sci. 13, 15706–15723. doi: 10.3390/ijms131215706
Xu Y., Zhan C., Huang B. (2011). Heat Shock Proteins in Association With Heat Tolerance in Grasses. Int. J. Proteomics 2011, 529648. doi: 10.1155/2011/529648
Yague-Capilla M., Garcia-Caballero D., Aguilar-Pereyra F., Castillo-Acosta V. M., Ruiz-Perez L. M., Vidal A. E., et al. (2019). Base Excision Repair Plays an Important Role in the Protection Against Nitric Oxide- and In Vivo-Induced DNA Damage in Trypanosoma Brucei. Free Radic. Biol. Med. 131, 59–71. doi: 10.1016/j.freeradbiomed.2018.11.025
Yang Y., Chai Z., Wang Q., Chen W., He Z., Jiang S. (2015). Cultivation of Seaweed Gracilaria in Chinese Coastal Waters and its Contribution to Environmental Improvements. Algal Res. 9, 236–244. doi: 10.1016/j.algal.2015.03.017
Zahedi K., Bissler J. J., Wang Z., Josyula A., Lu L., Diegelman P., et al. (2007). Spermidine/spermine N1-Acetyltransferase Overexpression in Kidney Epithelial Cells Disrupts Polyamine Homeostasis, Leads to DNA Damage, and Causes G2 Arrest. Am. J. Physiol. Cell Physiol. 292, C1204–C1215. doi: 10.1152/ajpcell.00451.2006
Zhang X. Q., Hu C. Y., Sun X., Zang X. N., Zhang X. C., Fang T., et al. (2020). Comparative Transcriptome Analysis Reveals Chitooligosaccharides-Induced Stress Tolerance of Gracilariopsis Lemaneiformis Under High Temperature Stress. Aquaculture 519, 34876. doi: 10.1016/j.aquaculture.2019.734876
Zhang J., Liu S. X., Hu C. Y., Chen X. J., Sun X., Xu N. J. (2021). Physiological and Transcriptome Analysis of Exogenous L-Arginine in Alleviation of High Temperature Stress in Gracilariopsis Lemaneiformis. Front. Mar. Sci. 8, 784586. doi: 10.3389/fmars.2021.784586
Zhou R., Hu Q., Pu Q., Chen M., Zhu X., Gao C., et al. (2020). Spermidine Enhanced Free Polyamine Levels and Expression of Polyamine Biosynthesis Enzyme Gene in Rice Spikelets Under Heat Tolerance Before Heading. Sci. Rep. 10, 8976. doi: 10.1038/s41598-020-64978-2
Zou D., Gao K. (2014). Temperature Response of Photosynthetic Light- and Carbon-Use Characteristics in the Red Seaweed Gracilariopsis Lemaneiformis (Gracilariales, Rhodophyta). J. Phycol, 50366–375. doi: 10.1111/jpy.12171
Keywords: Gracilariopsis lemaneiformis, spermidine synthase (SPDS) gene, spermidine and spermine, high temperature stress, transcriptome
Citation: Liu S, Zhang J, Sun X and Xu N (2022) Characterization of Spermidine Synthase (SPDS) Gene and RNA−Seq Based Identification of Spermidine (SPD) and Spermine (SPM) Involvement in Improving High Temperature Stress Tolerance in Gracilariopsis lemaneiformis (Rhodophyta). Front. Mar. Sci. 9:939888. doi: 10.3389/fmars.2022.939888
Received: 09 May 2022; Accepted: 30 May 2022;
Published: 18 July 2022.
Edited by:
Peng Jin, University of Guangzhou, ChinaCopyright © 2022 Liu, Zhang, Sun and Xu. This is an open-access article distributed under the terms of the Creative Commons Attribution License (CC BY). The use, distribution or reproduction in other forums is permitted, provided the original author(s) and the copyright owner(s) are credited and that the original publication in this journal is cited, in accordance with accepted academic practice. No use, distribution or reproduction is permitted which does not comply with these terms.
*Correspondence: Nianjun Xu, xunianjun@nbu.edu.cn