- 1Key Laboratory of Aquatic Animal Diseases and Immune Technology of Heilongjiang Province, Heilongjiang River Fisheries Research Institute, Chinese Academy of Fishery Sciences, Harbin, China
- 2Key Laboratory of Freshwater Fisheries and Germplasm Resources Utilization, Ministry of Agriculture and Rural Affairs, Freshwater Fisheries Research Center, Chinese Academy of Fishery Sciences, Wuxi, China
- 3State Key Laboratory of Freshwater Ecology and Biotechnology, Institute of Hydrobiology, Chinese Academy of Sciences, Wuhan, China
Costs associated with the use of soybean as a food source in aquaculture have imposed constraints on its use in this industry. Thus, research is now focusing on alternative sources of protein for use in aquaculture. Here, we assessed the effect of total replacement of soybean meal (SBM) by Clostridium autoethanogenum protein (CAP), Tenebrio molitor meal (TMM), cottonseed protein concentrate (CPC), and chlorella powder (CHP) in diets for small specimens (initial body weight: 239.72 ± 10.75 g) and large specimens (initial body weight: 638.32 ± 12.64 g) of grass carp. For that purpose, five isonitrogenous (300 g/kg) and isoaliphatic (58 g/kg) diets were formulated with SBM, CAP, TMM, CPC, and CHP as the only dietary protein source. Triplicate groups of fish were fed the experimental diets for 56 days. Results showed that irrespective of protein sources, large specimens of grass carp obtained the significantly reduced weight gain rate (WGR), specific growth rate, moisture, crude ash contents, drip loss rate, and gene expressions of muscle S6K, MyoD, and Myf-5, and the significantly increased crude protein and crude lipid contents, hardness, cohesiveness, adhesiveness, springiness, chewiness and shear force of muscles, and gene expressions of muscle TOR, 4EBP1, MyoG, MRF4, and MSTN-1. Regardless of size, the CHP group possessed markedly higher WGR, protein efficiency ratio, crude protein contents, hardness, adhesiveness, chewiness values, and gene expression levels of muscle TOR, 4EBP1, Myf-5, and MRF4, and significantly lower feed conversion rate, drip loss rate, and gene expressions of muscle S6K and MSTN-1. For small specimens or for large specimens, the essential amino acid contents of the CHP group were highest, and CHP markedly elevated the delicious amino acid and glycine contents in comparison with the SBM. Moreover, the CPC group also realized the function of promoting the growth and improving the flesh quality to a certain degree. However, total replacement of soybean meal by CAP and TMM in grass carp diets restrained the growth and damaged the flesh quality in varying degrees. Overall, comparatively, total replacement of SBM with CHP not only improved growth performance and feed use, but also enhanced the flavor and texture of the fish by improving the amino acid composition and water-holding capacity of muscle, as well as improving muscle growth by positively impacting the expression of genes associated with the regulation of growth and development. Therefore, CHP could be a potential protein source to replace SBM in diets for use in the aquaculture of grass carp.
Introduction
The insufficient provision and high cost of feedstuff, such as fish meal (FM) and soybean meal (SBM), the primary sources of protein for aquafeeds, has imposed constraints on the sustainability of aquaculture. Despite being an ideal source of protein, SBM has become a limiting factor in the development of feeds for herbivorous fish, such as grass carp (Ctenopharyngodon idellus) (Ye et al., 2013; Zou et al., 2021). Thus, interest has increased in the development of novel, cost-efficient protein sources as substitutes for SBM in aquafeeds. Research demonstrated that applying extra protein sources from terrestrial plant and animal sources instead of SBM could maintain normal growth and amino acid balance in aquaculture fish (Yao and Liu, 2013; Zhang et al., 2013; Zhong et al., 2013; Lu et al., 2020).
However, substitution in the existing studies focused primarily on peanut meal, cottonseed meal, distillers dried grains with soluble (DDGS), etc., which could be the main protein supply source for livestock, agriculture sectors, and even humans, thus increasing competition for such compounds across different industries. Thus, there is an urgent need to achieve the sustainable development of alternative sources of raw protein. Single-cell protein (SCP) has been reported as a good alternative to plant protein sources because of its accessibility (Vermeulen et al., 2012) and rich protein supplement (Ritala et al., 2017). Clostridium autoethanogenum protein (bacterioprotein, CAP) and chlorella powder (microalgae protein, CHP) are novel SCPs that have been preliminarily used as protein sources for largemouth bass (Xi et al., 2022), genetically improved farmed tilapia (GIFT, Oreochromis niloticus) (Maulu et al., 2021), and crucian carp (Carassius auratus) (Shi et al., 2015). Compared with plant protein sources, novel animal protein sources are characterized by a low carbohydrate content and high mineral and vitamin content, independent of seasonal and climatic variations and, thus, have been more studied as protein source substitutions (Liu et al., 2019). For example, Tenebrio molitor meal (TMM) was found to be a suitable source of animal protein for partial substitution of FM for many species of fish, including mandarin fish (Siniperca scherzeri) (Sankian et al., 2018), European perch (Perca fluviatilis) (Tran et al., 2021), and blackspot sea bream (Pagellus bogaraveo) (Iaconisi et al., 2017), without reducing fish growth. For a variety of plant protein sources, there is a need to remove anti-nutrient factors by using technologies such as fermentation and enzymatic hydrolysis to improve the utilization rate of plant protein (Zheng et al., 2021). For example, cottonseed protein concentrate (CPC) is a source of high protein levels following dephenolization and detoxification, as investigated in pompano (Trachinotus ovatus) (Shen et al., 2019) and black sea bass (Centropristis striata) (Zhang et al., 2020). However, it is not clear how these alternative sources of plant protein compare with those of SBM in terms of their effects on fish.
Previous research has investigated the effects of SBM on fish growth (Liu et al., 2021), oxidation resistance (Chen et al., 2019), and immunity function (Zhao et al., 2012). Less work has focused on the effect of fish flesh quality, which is an important consideration in aquaculture, given its crucial role in consumer preference, thereby influencing aquaculture profits. Fish flesh quality is characterized by a set of traits including muscle nutritive composition, amino acid composition, fatty acid profiles, texture, water-holding capacity, and muscle pH, among others (Fuentes et al., 2010). The amino acid composition is one of the most important determinants of flesh flavor (Li et al., 2018). Texture also influences the mechanical processing of fillets and their acceptability by consumers (Cheng et al., 2014), and is closely associated with the nutritive composition of muscle. For example, Andersen et al. (1999) found that the muscle structure and texture of fish with a high lipid content were less resistant to mechanical stress compared with fish with a low lipid content. Fish flesh quality is also impacted by external factors, including breeding conditions (Wang et al., 2021) and feed nutrition (Zhao et al., 2018). Thus, fish flesh quality is another important attribute to consider when investigating the effects of dietary SBM substitution.
Gene effects are known to impact fish flesh quality (Cheng et al., 2014). As candidate genes determining fish flesh quality, those encoding myogenic regulatory factors (MRFs) are particularly important, including primary myogenic regulatory MRFs (MYOD and MYF5) involved in myogenic progenitor proliferation, and secondary MRFs (myogenin and MYF4) involved in myoblast differentiation and fusion to form muscle fibers (Fuentes et al., 2013). In addition, insulin growth factor (IGF) expression impacts positively on fish muscle proliferation and differentiation, whereas myostatin (MSTN) has a negative impact on fish muscle growth (De-Santis and Jerry, 2007; Fuentes et al., 2013). Research on rainbow trout (Alami et al., 2010), Nile tilapia (Nebo et al., 2013), and grass carp (Lin et al., 2015) showed that various feed factors (plant protein sources, lipid sources, feeding strategy, etc.) affect the expression of MRFs and related positive and negative regulatory factors during development and later growth periods. Thus, it is also important to determine the potential relationship between the novel protein sources replacing soybean meal and related molecular mechanisms involved in muscle growth.
As a high-quality animal protein for human consumption, the demand for fish has steadily increased over the past few decades (Food and Agriculture Organization, 2022). For example, the sustained output of grass carp over a 3-year period relied significantly on the rapid growth and superior quality of this fish (Fishery administration et al., 2020). However, SBM has imposed restrictions on the sustainable and ecological development of grass carp aquaculture, especially for small (~250 g) and large specifications (~600 g), which are crucial growth stages in terms of attaining the market size of grass carp (~1,000 g). Taking this into consideration, the study was conducted to investigate the effect of four novel protein sources (CAP, TMM, CPC, and CHP) as total alternatives to soybean meal for two specifications of grass carp (C. idellus) deeds on growth performance, flesh quality, and gene expression regarding muscle growth in order to provide a more abundant theoretical basis for developing suitable protein source of grass carp feed.
Materials and methods
Formulation and preparation of experimental feed
The ingredients and nutritional components of the experimental diets used in the study are detailed in Table 1, whereas their amino acid composition of experimental diets is detailed in Table 2. Briefly, five isonitrogenous (300 g/kg) and isoaliphatic (58 g/kg) diets were formulated. A basal diet containing 691 g/kg SBM (439 g/kg crude protein, 12.4 g/kg crude lipid) was regarded as the control group (named as SBM), whereas 100% of SBM was replaced with CAP (867 g/kg crude protein, 34.3 g/kg crude lipid, obtained from Beijing Shoulang Biological Technology Co., LTD), TMM (659 g/kg crude protein, 50.9 g/kg crude lipid, obtained from Guangdong Zehecheng Biotechnology Co., LTD), CPC (631 g/kg crude protein, 13.8 g/kg crude lipid, obtained from Xinjiang Jinlan Vegetable Protein Co. LTD), and CHP (575 g/kg crude protein, 102 g/kg crude lipid, obtained from Institute of Hydrobiology, Chinese Academy of Sciences) in each of the other groups. Fish oil and soybean oil were used as dietary lipid sources. All the diets were granulated into 1.5-mm pellets using a pelletizer. The resulting pellets were air dried and stored at −20°C until used.
Experimental fish and feeding protocol
Grass carp were supported by Hulan Experimental Station of Heilongjiang Fisheries Research Institute (Harbin, China), and reared in the acclimatization cages (2 m × 2 m × 2 m, length × width × height) for 2 weeks. In detail, two size classes of grass carp (including 375 small specimens with an initial weight of 239.72 ± 10.75 g and 375 large specimens with an initial weight of 638.32 ± 12.64 g) were carefully selected. For each size class, grass carp were allotted into 15 cages (size as above) with 25 grass carp in each cage, and the amount of water used in each cage was 2 m × 2 m × 1.8 m, because the water level was 0.2 m below the cage. Each of five experimental diets was fed to three replicates three times daily (08:00 h, 13:00 h, and 17:30 h) at a rate of 4% of the body weight to apparent satiation for 8 weeks according to Lu et al. (2020). Throughout the rearing period, the water temperature, total ammonia, dissolved oxygen, and salinity were maintained at 28–32°C, 0–0.20 mg/L, 5.8–6.2 mg/L, and 275 mg/L, respectively.
Sample collection
After a 24-h fasting at the ending of the 8-week rearing period, fish in each cage were weighed, measured, and counted to determine the weight gain rate (WGR), specific growth rate (SGR), condition factor (CF), feed conversion ratio (FCR), and protein efficiency ratio (PER). Then, muscle samples of six fish from each treatment were dissected from the left side, immediately frozen in liquid nitrogen, and then stored in a −80°C freezer for late determination of gene expression. Meanwhile, from the right side of the same fish, muscles from fish in each treatment were collected for subsequent instrumental texture analysis and proximate composition measurement.
Proximate composition measurement
The proximate composition of grass carp muscles was measured by reference to the Association of Official Analytical Chemists protocol (AOAC (Association of Official Analytical Chemists), 2006). Moisture was determined by drying the muscles at 105°C to constant weight. Crude protein content was determined by the Kjeldahl method through a fully automated Kjeldahl nitrogen/protein analyzer after acid digestion in digestion furnace. Similarly, crude lipid was determined with ether extraction method with a Soxtec system and crude ash was determined by incinerating the muscles in a muffle furnace at 550°C to constant weight.
Amino acid analysis
The amino acid content of all samples was carried out according to the method of Liu et al. (2015). First, freeze-dried samples were hydrolyzed for 24 h at 110°C in sealed glass tubes filled with 10–15 ml 6 M HCl and nitrogen. After being dried under vacuum conditions at 50°C, the hydrolysate was dissolved in sodium citrate (pH 2.2) and filtered with a 0.45-mm Millipore nylon membrane filter. Amino acids were extracted by a HITACHI L-8900 automatic amino acid analyzer. Amino acid concentration was represented as % dry weight.
Texture and drip loss analysis
Six muscle samples from the same site in each fish were selected from each treatment group. The samples were cut to a uniform size and thickness (2.0 cm × 2.0 cm × 0.5 cm) for texture analysis and another six muscle samples with a uniform size and thickness (3.0 cm × 1.0 cm × 0.5 cm) were obtained for shear force measurement. The muscles were kept at 4°C until analysis (~48 h after death). The texture and shear force of the muscles were determined by an instrumental method texture profile analysis (TPA) and calculated by using a software TPA application associated with an FTC TMS Pro texture analyzer. As one of the most important characteristics in terms of the quality of fish muscle for human consumption, texture is represented by the hardness, adhesiveness, cohesiveness, springiness, gumminess, and chewiness of the fish. The test conditions for shear force measurement involved consecutive cycles of compression with a constant speed of 30 mm/min with a pressing distance of 60% of the original length. The muscle drip loss rate was determined according to the method described by Wei et al. (2020).
qPCR analysis of gene expression in muscle
To determine the level of gene expression in each experimental group, the total RNA in the muscles was isolated using RNAiso Plus according to the manufacturer’s instructions. Spectrophotometric analysis at a A260:280 nm ratio and 1% agarose gel electrophoresis at 1% were used to evaluate the RNA quality and quantity. cDNA was then synthesized through reverse transcription based on 1,000 ng of RNA with a TaKaRa PrimeScript RT Reagent Kit with gDNA Eraser (Perfect Real Time) (Dalian Takara Company, Dalian, China). With an ABI 7500 real-time PCR machine (ABI, Applied Biosystems), targeted gene expression levels in the cDNA templates were detected using TaKaRa SYBR Premix Ex Taq (Tli RNaseH Plus) according to the manufacturer’s instructions. The expression levels of the genes were normalized to the expression levels of a grass carp internal reference gene (β-actin). The primer sequences and optimal annealing temperatures are detailed in Table 3. A 10-fold serial dilution, including six concentrations (in triplicate), was used to generate standard curves for both targeted genes and β-actin, which indicated ~100% amplification efficiencies for all primers. The comparative CT method (2−ΔΔCt) method was used to calculate the relative expression levels of each gene.
Data processing and analysis
Results were subjected to two-way analysis of variance (ANOVA), followed by a Duncan’s test to delineate significance among fish groups using SPSS 22.0 software (SPSS Inc., Chicago, IL, USA). For all analyses, significant levels were set at p< 0.05 unless otherwise stated. All data are represented as means ± SD.
Results
Growth performance and feed conversion
Irrespective of protein sources, the significantly reduced WGR and PER were associated with increasing grass carp size (p< 0.05). There was a trend for increased FCR with increasing grass carp size although this was not significant (p > 0.05).
Protein source had significant effects on growth performance and feed conversion of grass carp. Despite grass carp size, the markedly higher WGR and PER and lower FCR were observed in the CHP group (p< 0.05), although there was no significant difference compared to the SBM and CPC groups (p > 0.05) (Table 4).
Proximate composition of grass carp muscles
The proximate composition of grass carp muscles from fish in the different groups is detailed in Table 5. The moisture and crude ash contents significantly declined with increasing grass carp size (p< 0.05), whereas crude protein and crude lipid contents significantly increased with increasing grass carp size (p< 0.05).
Regardless of grass carp size, fish in the CHP group had significantly higher crude protein contents in their muscles compared with fish from the TMM group at the end of the experiment (p< 0.05). By contrast, fish in the CAP group had significantly higher crude lipid contents of muscle compared with those in all other groups (p< 0.05).
Muscle texture, shear force, and drip loss
According to the muscle texture analysis results (Table 6), there was significant impact on muscle springiness determined by the interaction between fish size and protein source (p< 0.05).
Regardless of protein source, hardness, cohesiveness, adhesiveness, springiness, chewiness, and shear force of muscles were significantly increased with increasing grass carp size, while drip loss rate was significantly decreased with increasing grass carp size (p< 0.05). Irrespective of grass carp size, the CHP group obtained a markedly higher hardness, adhesiveness, and chewiness values of grass carp muscles compared with the CAP and TMM groups, and a lower drip loss rate compared with the CAP group (p< 0.05). The SBM group obtained the markedly higher springiness value of grass carp compared with the CAP and TMM groups (p< 0.05).
Amino acid composition of grass carp muscle
As shown in Table 7, muscles from small specimens in the CHP and CPC groups had significantly higher ΣDAA and ΣTAA compared with those of the other groups, with the CHP group having significantly higher ΣEAA compared with the CAP group, and significantly lower ΣNEAA than either the TMM or CPC groups (p< 0.05). In terms of essential amino acids (EAAs), the CHP group had a significantly higher lysine content than those in the SBM, CAP, and TMM groups, significantly higher phenylalanine content than those in the other groups, significantly higher leucine contents than that of the CAP group, and a significantly higher isoleucine contents than that of the TMM group (p< 0.05). Regarding the delicious amino acid (DAA), the CHP group had significantly higher contents of alanine and glycine than those of the CAP and TMM groups, and significantly higher glutamine and aspartic acid contents than those in the SBM, CAP, and TMM groups (p< 0.05).
In Table 8, muscles from larger specimens in the CHP group had significantly higher ΣEAA compared with those of the SBM, CAP, and TMM groups; significantly higher ΣDAA compared with those of the SBM and CAP groups; and significantly lower ΣNEAA compared with that of the CAP group (p< 0.05).
In terms of EAAs, the CHP group had significantly higher lysine, phenylalanine, isoleucine, leucine, and valine contents than those of the SBM, CAP, and TMM groups; a significantly higher threonine content than that of the CAP and TMM groups; and a significantly higher methionine content than that of the CAP group (p< 0.05). In terms of DAAs, the CHP group had a significantly higher alanine and glycine content compared with the SBM, CAP, and TMM groups, and a significantly higher glycine content compared with all other groups (p< 0.05).
Gene expression levels of protein synthesis in the muscles
Gene expression analysis of TOR and 4EBP1 revealed a significant interaction between size and protein source (p< 0.05). Ignoring the effect of protein source, the larger fish showed higher gene expression levels of TOR and 4EBP1 and lower gene expression levels of S6K (p< 0.05). Without the effect of grass carp size, fish in the CHP group showed higher gene expression levels of TOR, 4EBP1, and S6K compared with all other groups (p< 0.05) (Table 9).
Related gene expression levels of growth and development in the muscles
The interaction between size and protein source significantly impacted the gene expression levels of MyoG, MyoD, MRF4, MSTN1, and IGF1 in the muscles (Table 10). Irrespective of protein source, the larger fish showed higher gene expression levels of MyoG, MRF4, and MSTN1 in the muscles, and the scarcer gene expression levels of MyoD and MYF5 and in the muscles compared with the small specimens (p< 0.05). Regardless of size, fish in the CHP group had a higher MYF5 expression level in muscle compared with those in the CAP and TMM groups; a higher MRF4 expression level compared with those in the SBM, CAP, and TMM groups; and a higher IGF1 expression level compared with those in all other groups (p< 0.05). By contrast, the expression level of MSTN1 in muscle from fish in the CHP group was significantly downregulated compared with those in the CAP and TMM groups.
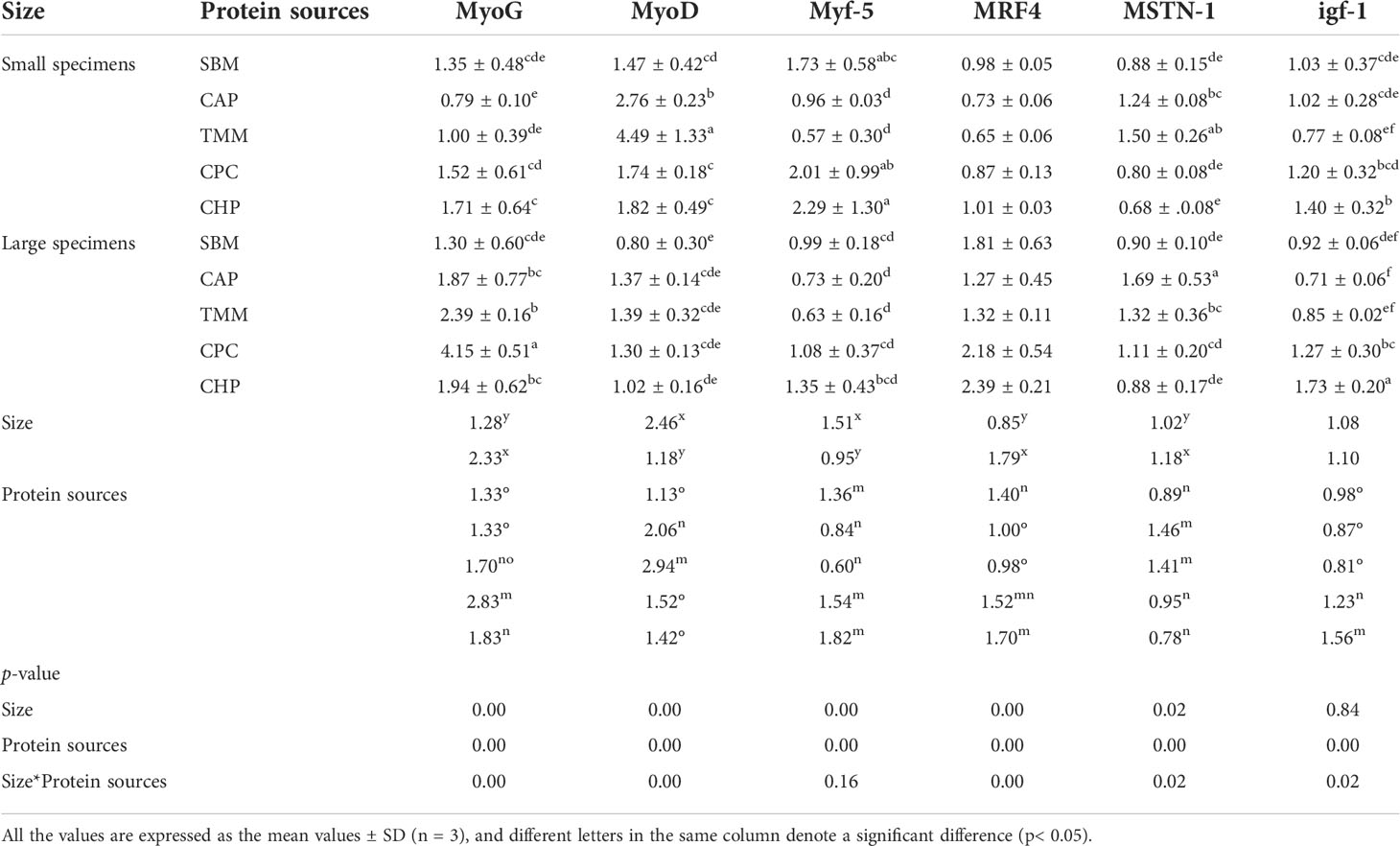
Table 10 Related gene expression levels of growth and development in the muscles in different treatments.
Discussion
Previous research on grass carp reported that replacement of SBM with more than 50% peanut meal significantly negatively affected the weight gain of grass carp (Yao and Liu, 2013). However, in the current study, significant effects on growth and feed utilization occurred in response to replacing SBM with different protein sources. For both small specimens and large specimens, entire replacement of SBM with CAP and TMM retrained the increase of growth and feed utilization and so the hypothesis was proved. However, unexpectedly, entire replacement of SBM with CPC and CHP did not hinder growth and feed utilization. which implied that CPC and CHP might have more potential as a replacement for SBM in feed for grass carp than the other two novel protein sources in grass carp feeds. This outcome might be the result of the method used in this study. To evaluate the potential application value of CAP, TMM, CPC, and CHP in feed for grass carp of different specifications, the SBM was completely replaced in this study by an alternative protein source. When SBM is used as the only feed protein source, the anti-nutritional factors in SBM destroy the microbial environment of the intestine, reducing the activities of digestive enzymes and causing intestinal dysfunction, thereby weakening the absorption capacity for nutrients and inhibiting fish growth (Maytorena-Verdugo and Cordova-Murueta, 2017). By contrast, CPC is dephenolized to reduce the anti-nutritional factor content (Xue, 2021), whereas CHP provides balanced nutrition and no anti-nutritional factors (Luo et al., 2016). Therefore, the difference in anti-nutrient factors of each protein source could be one of the main reasons for difference in growth across the experimental groups. In addition, the substitution efficiency of different protein sources is closely related to feeding habits. In general, plant proteins as an alternative protein source are better for herbivorous carnivorous fish than for omnivorous fish (Liu et al., 2014). Thus, given that grass carp are herbivorous, our results suggest that herbivory contributes to the improved substitution efficiency of CPC and CHP in grass carp feed compared with CAP and TMM.
The efficient utilization of certain protein sources in fish feeds hinges on appropriate dietary amino acid (especially EAA) supplementation. Deficiencies in methionine and lysine, which are limiting amino acids in SBM, can inhibit protein synthesis efficiency of fish, restraining their growth (Maytorena-Verdugo and Cordova-Murueta, 2017; Guo et al., 2021). Yin (2019) reported that when 45% FM was replaced by SBM in the diet of pearl gentian grouper, dietary methionine and lysine contents decreased by 39.55% and 39.61%, respectively, which was one of the reasons limiting the growth performance of these fish. In the current study, in terms of the amino acid composition of the different feeds, the EAA compositions of fish in the TMM, CPC, and CHP groups were superior to those of the SBM group. For example, the lysine content of the CAP, TMM, and CHP groups increased by 166.06%, 43.12%, and 58.41%, respectively, compared with the SBM group, whereas the methionine content of the CAP, TMM, CPC, and CHP groups increased by 288.14%, 169.49%, 52.54%, and 98.31% compared with the SBM group. Moreover, for smaller grass carp, the EAA content of muscle in the CHP group was higher than those in the SBM group, particularly the lysine content. Lysine regulates the expression of TOR and 4EBP1 to improve protein synthesis in fish and, thus, their protein deposition efficiency and growth performance (Fan and Wang, 2021). For large specimens of grass carp, the essential amino acid contents of the CHP group were also highest, and hereof the contents of leucine, isoleucine, and valine, which belong to branched-chain amino acid, were markedly elevated compared to the other groups. The result indicated that the difference in content and composition of branched-chain amino acids was the main reason that the growth performance of carp in the SBM group was weaker than that in the CHP group. Branched-chain amino acids can participate in protein metabolism, among which the main branched-chain amino acid regulating protein synthesis is leucine (Wang et al., 2015). Leucine can improve the protein synthesis efficiency of fish by participating in the regulation of target rapamycin (TOR) signaling pathway, thus promoting body growth (Ren et al., 2015; Zou et al., 2018). Furthermore, regardless of fish size, the changes in muscle amino acid content induced by dietary protein sources resulted in corresponding changes in the growth indices, muscle protein content, and expression of genes involved in the TOR pathway. These results suggest that CHP enhances the activity of the mammalian TOR (mTOR) signaling pathway by increasing the amino acid level (e.g., lysine and branched-chain amino acids), promoting protein deposition, which could explain the improved growth-promoting effect of CHP compared with that of SBM, although the specific regulatory mechanisms involved require further investigation.
The composition of DAAs, including glycine, alanine, glutamic acid, and aspartic acid, is the main factor determining the flavor of fish meat. Of these, glutamic acid has the most important role, followed by glycine (Lv et al., 2022). In Jiang’s study on Jian carp, the entire replacement of FM with SBM significantly reduced the content of glutamic acid and glycine and thus destroyed the flavor of fish muscle (Jiang et al., 2015), and our experimental results were consistent with Jiang’s research. Meanwhile, our study also revealed that, regardless of fish size, CHP used as the only protein source in grass carp diets increased the DAA and glycine contents compared with SBM, which would enhance the sweetish taste of the fish. Likewise, the effect of CPC on the DAA and glycine contents showed a similar effect to that of CHP. By contrast, CAP and TMM caused a significant reduction in the DAA content compared with SBM. Combined with the above results, it was revealed that the complete replacement of SBM by CHP and CPC could have efficacy to improve the amino acid nutritional value and umami taste of fillet of grass carp.
Texture parameters and shear force are two important parameters that describe the characteristics of muscle tissue, and are usually affected by many factors, such as fish species, genotype, feed, and culture environment (Wang et al., 2022). Texture parameters are used to simulate the chewing of human teeth to represent the taste of meat, including its hardness, cohesion, elasticity, and chewiness, whereas shear force is used to simulate the cutting of muscle fibers by human teeth to represent the meat tenderness (Cheng et al., 2014). In the current study, the hardness and elasticity of the muscles increased with the size of the grass carp, rendering the muscle harder and more tasty, which was in agreement with the positive growth-promoting effects on texture parameters in a previous study on grass carp (Hu et al., 2011). Meanwhile, significant alterations of texture parameters were induced by the replacement of SBM. Diets with CPC and CHP increased the hardness, cohesion, elasticity, chewiness, and shear force of the muscles compared with SBM. By contrast, decreased texture parameters were observed in the CAP and TMM groups. This showed that, compared with CAP and TMM, CPC and CHP not only increase muscle hardness by enhancing muscle shear force, thus improving muscle taste, but also strengthen the binding force between muscle cells by elevating the cohesion between muscle cells and improving the ability of fish to resist damage, rendering them more delicate with an improved flavor (Zhang et al., 2013). Previous research reported that the texture characteristics of fish muscle were related to the moisture and lipid content of muscle. Dunajski (1979) reported that a high moisture and lipid content reduced the mechanical strength of fish. In agreement, the current results revealed that the lower moisture and lipid content in the CHP group resulted in increased muscle hardness compared with the CAP and TMM groups, which suggested that replacement of SBM with CHP would have a positive impact on muscle texture. Water-holding capacity is also an important indicator of flesh quality, which is usually represented by drip loss rate, with a positive beneficial correlation between drip loss rate and water retention (Zhong et al., 2018). In the current study, both size and protein source had a significant effect on the drip loss rate. Moreover, a significantly lower drop loss rate was observed in the large grass carp compared with small fish. Regardless of size, grass carp in the SBM, CPC, and especially the CHP group, had lower drop loss rates compared with the CAP group. These results support the hypothesis that replacing SBM with CHP could improve the flesh quality of grass carp.
Muscle growth, development, and quality regulation are regulated by various endogenous molecular mechanisms (Salem et al., 2013). In the current study, genes involved in muscle growth and development (MyoD, MyoG, MYF5, and MRF4) and their related positive and negative regulatory factors (IGFs and MSTNs) were analyzed. Both size and protein source had a notable impact on the expression of MyoD, MyoG, MYF5, MRF4, and MSTN1. In terms of size, large grass carp showed significantly upregulated expression of MyoG, MRF4, and MSTN1, and significantly downregulated expression of MyoD and MYF5 compared with small fish. These findings could be because MyoD and MYF5 have a role as myogenic determinants, whereas MyoG and MRF4 have a crucial role in late differentiation of muscle cells (Yun and Wold, 1996; Kassar et al., 2004). In terms of protein source, grass carp in the CHP group showed the highest expression levels of MYF5, MRF4, and IGF1, and the lowest gene expression levels of MSTN1. The results suggested that CHP inhibited the negative regulation of MSTN1 to promote muscle growth and development (Zhao et al., 2018). Thus, CHP could prompt the production of more muscle cells to overcome damage resulting from anti-nutritional factors in SBM by downregulating the expression of MSTN1, thereby exerting a growth-promoting effect (Abouel et al., 2021).
Conclusion
Overall, based on the results of the present study, this study indicated that total replacement of soybean meal by CHP in diets for small specimens and large specimens of grass carp could not only improve growth performance and feed utilization, but also enhance the meat flavor by improving the amino acid composition of muscle, meat taste by improving the texture parameters and water-holding capacity, and muscle growth by positively impacting the expression of genes involved in the growth and development. Similar results were also seen with CPC. However, total replacement of SBM with CAP and TMM suppressed fish growth and reduced meat quality to varying degrees. Thus, in terms of its positive effects on fish growth and flesh quality, CHP could be a potential protein source to replace SBM in diets for both small and large grass carp.
Data availability statement
The original contributions presented in the study are included in the article/supplementary material. Further inquiries can be directed to the corresponding authors.
Ethics statement
All Animal procedures in this study were conducted according to the guidelines for the care and use of laboratory animals of Heilongjiang River Fisheries Research Institute (CAFS). The studies in animals were reviewed and approved by the Committee for the Welfare and Ethics of Laboratory Animals of Heilongjiang River Fisheries Research Institute.
Author contributions
SX and LW: Conceptualization, investigation, writing-review, and editing. ZF: Writing the original draft preparation, formal analysis, and resources. DW and LM: Formal analysis and investigation. JL and CL: Formal analysis. DC: Experiment management. All authors contributed to the article and approved the submitted version.
Funding
This research was financially supported by the National Key R & D Program of China (2019YFD0900200), China Agriculture Research System of MOF and MARA, Central Public-Interest Scientific Institution Basal Research Fund, and CAFS (2022XT0402). The authors also thank International Science Editing (http://www.internationalscienceediting.com) for editing this manuscript.
Conflict of interest
The authors declare that the research was conducted in the absence of any commercial or financial relationships that could be construed as a potential conflict of interest.
Publisher’s note
All claims expressed in this article are solely those of the authors and do not necessarily represent those of their affiliated organizations, or those of the publisher, the editors and the reviewers. Any product that may be evaluated in this article, or claim that may be made by its manufacturer, is not guaranteed or endorsed by the publisher.
References
Abouel A. F. R., Kong F. S., Tan Q. S., Zhu Y. H., Yu H. J., Yao J. P., et al. (2021). Effects of replacement of dietary rapeseed meal by distiller's dried grains with solubles (DDGS) on growth performance, muscle texture, health and expression of muscle-related genes in grass carp (Ctenopharyngodon idellus). Aquaculture 533, 736169. doi: 10.1016/j.aquaculture.2020.736169
Alami D. H., Wrutniak C. C., Kaushik S. J., Médale F. (2010). Skeletal muscle cellularity and expression of myogenic regulatory factors and myosin heavy chains in rainbow trout (Oncorhynchus mykiss): Effects of changes in dietary plant protein sources and amino acid profiles. Comp. Biochem. Physiol. A 156, 561–568. doi: 10.1016/j.cbpa.2010.04.015
Andersen U. B., Thomassen M. S., Røra A. M. B. (1999). Texture properties of farmed rainbow trout (Oncorhynchus mykiss): Effects of diet, muscle fat content and time of storage on ice. J. Sci. Food Agric. 74 (3), 347–353. doi: 10.1002/(SICI)1097-0010(199707)74:33.0.CO;2-F
AOAC (Association of Official Analytical Chemists) (2006). Official methods of analysis of the association of official analytical chemists international. 16th edition (Arlington, Virginia, USA: AOAC).
Chen X. B., Chen R., Shao J. J., Jiang X. J., Zhang M. C., Wan J. J. (2019). Effects of soya repalcing soybean meal on growth, digestive enzyme activities and antioxidant capacity of Chinese mitten crab (Eriocheir sinensis). J. Aquac. 40 (10), 12–15. doi: 10.3969/j.issn.1004-2091.2019.10.003
Cheng J. H., Sun D. W., Han Z., Zeng X. A. (2014). Texture and structure measurements and analyses for evaluation of fish and fillet freshness quality: A review. Compr. Rev. Food Sci. Food Saf. 13, 52–61. doi: 10.1111/1541-4337.12043
De-Santis C., Jerry D. R. (2007). Candidate growth genes in finfish-where should we be looking? Aquaculture 272, 22–38. doi: 10.1016/j.aquaculture.2007.08.036
Dunajski E. (1979). Texture of fish muscle. J. Texture Stud. 10 (4), 301–318. doi: 10.1111/j.1745-4603.1980.tb00862.x
Fan Z., Wang L. S. (2021). Advances in fish lysine nutrition research. China Feed 13), 66–72. doi: 10.15906/j.cnki.cn11-2975/s.20211314
Fishery administration, Ministry of Agriculture and Rural Affairs, Nationalaquatic technology extension station, China Society of Fisheries (2020). China Fishery statistical yearbook (Beijing: China Agriculture Press).
Food and Agriculture Organization (2022). The state of food and agriculture 2022. Towards Blue Transformation (Rome: FAO). doi: 10.4060/cc0461en
Fuentes A., Fernández S. I., Serra J. A., Barat J. M. (2010). Comparison of wild and cultured Sea bass (Dicentrarchus labrax) quality. Food Chem. 119 (4), 1514–1518. doi: 10.1016/j.foodchem.2009.09.036
Fuentes E. N., Valdés J. A., Molina A., Björnsson B. T. (2013). Regulation of skeletal muscle growth in fish by the growth hormone-Insulin-Like growth factor system. Gen. Comp. Endocrinol. 192, 136–148. doi: 10.1016/j.ygcen.2013.06.009
Guo X., Dai X. L., Hua X. M., Yu Z. L., Gao X. Z., Wei X., et al. (2021). Optimum lysine requirement of juvenile pacific white shrimp, litopenaeus vannamei when fermented soybean meal partially replacing fish meal in the feed. J. Fish. China 45 (6), 910–919. doi: 10.11964/jfc.20201112508
Hu F., Li X. D., Xiong S. B., Fu N., Wang H. M., Yang X. B., et al. (2011). Texture properties of freshwater fish and their correlation with nutritional components. Food Sci. 32 (11), 69–73.
Iaconisi V., Marono S., Parisi G., Gasco L., Genovese L., Maricchiolo G., et al. (2017). Dietary inclusion of tenebrio molitor larvae meal: Effects on growth performance and final quality treats of blackspot Sea bream (Pagellus bogaraveo). Aquaculture 476, 49–58. doi: 10.1016/j.aquaculture.2017.04.007
Jiang J., Hu Y., Zhou X. Q., Yin L., Feng L., Jiang W. D., et al. (2015). Effects of replacement ratio of fish meal by soybean meal in extruded diets on muscle quality of jian carp (Cyprinus carpio var. jian). Chin. J. Anim. Nutr. 27 (2), 623–630. doi: 10.3969/j.issn.1006-267x.2015.02.035
Kassar D. L., Gayraud M. B., Gomès D., Rocancourt D., Buckingham M., Shinin V., et al. (2004). Mrf4 determines skeletal muscle identity in Myf5: MyoD double-mutant mice. Nature 431, 466–471. doi: 10.1038/nature02876
Li J. P., Chen D. H., Fang Z. S. (2018). The mechanism of amino acid metabolism on promoting growth of fish. Agric. Jilin. 14), 72–73. doi: 10.14025/j.cnki.jlny.2018.14.044
Lin Y., Zhou J., Li R., Zhao Y., Zheng Y. (2015). Cloning and expression patterns of MRFs and effect of replacing dietary fish oil with vegetable oils on MRFs expression in grass carp (Ctenopharyngodon idellus). Turkish J. Fish. Aquat. Sci. 15, 257–266. doi: 10.4194/1303-2712-v15_2_07
Liu C., Chen S., Zhuang Z., Yan J., Liu C., Cui H. (2015). Potential of utilizing jellyfish as food in culturing pampus argenteus juveniles. Hydrobiologia 754 (1), 189–200. doi: 10.1007/s10750-014-1869-6
Liu Y. Z., He G., Zhou H. H. (2014). Substitution system of animal and plant protein source in aquatic feed and its relationship with feeding habits of cultured fish. Hebei Fish. 8), 54–57. doi: 10.3969/j.issn.1004-6755.2014.08.021
Liu M., Zhang H. T., Sun G. W. (2019). Research progress on substitution of fish meal by animal protein source in aquatic feed. Feed Ind. 40 (22), 48–54. doi: 10.13302/j.cnki.fi.2019.22.009
Liu Y., Zhou X. W., Gao R. F., Ding H. T., Cheng H. L., Chen X. N., et al. (2021). Effect of soybean meal replacement with peanut meal on growth performance, muscle tissue composition and physiological and biochemical indexes of cyprinus carpio jian II. Feed Res. 44 (10), 40–44. doi: 10.13557/j.cnki.issn1002-2813.2021.10.010
Lu R. H., Chen Y. N., Yu W. P., Lin M. J., Yang G. K., Qin C. B., et al. (2020). Defatted black soldier fly (Hermetia illucens) larvae meal can replace soybean meal in juvenile grass carp (Ctenopharyngodon idellus) diets. Aquac. Rep. 18, 100520. doi: 10.1016/j.aqrep.2020.100520
Luo L. Y., Li J. Y., Chen Z., Liu H., Ming C. B., Liu R., et al. (2016). Research progress in the application of aquatic animal feed of chlorella. Cereal Feed Ind. 6): 55, 7+61. doi: 10.7633/j.issn.1003-6202.2016.06.013
Lv M., Gan H., Chen T. C., Lu X. H., Ruan Z. D., Zhu J. J., et al. (2022). Comparison of growth performance and muscle quality of yellow catfish (Pseudobagrus vachelli) cultured in rice fields and ponds. Chin. J. Fish. 35 (1), 75–81. 1005-3832(2022)01-0075-07
Maulu S., Liang H. L., Ke J., Ren M. C., Ge X. P., Huang D., et al. (2021). Dietary clostridium autoethanogenum protein modulates intestinal absorption, antioxidant status, and immune response in GIFT (Oreochromis niloticus) juveniles. Aquac. Res. 52, 5787–5799. doi: 10.1111/are.15454
Maytorena-Verdugo C. I., Cordova-Murueta J. H. (2017). Peptidase compensation in the digestive system of whiteleg shrimp penaeus vannamei against dietary kunitz-type soybean trypsin inhibitor. Aquac. Nutr. 23 (5), 1095–1103. doi: 10.1111/anu.12477
Nebo C., Portella M. C., Carani F. R., Almeida F. L., Padovani C. R., Carvalho R. F., et al. (2013). Short periods of fasting followed by refeeding change the expression of muscle growth-related genes in juvenile Nile tilapia (Oreochromis niloticus). Comp. Biochem. Physiol. B: Biochem. Mol. Biol. 164, 268–274. doi: 10.1016/j.cbpb.2013.02.003
Ren M. C., Habte-Tsion H. M., Liu B., Miao L. H., Ge X. P., Xie J., et al. (2015). Dietary leucine level affects growth performance, whole body composition, plasma parameters and relative expression of TOR and TNF-alpha in juvenile blunt snout bream, megalobrama amblycephala. Aquaculture 448, 162–168. doi: 10.1016/j.aquaculture.2015.06.008
Ritala A., Häkkinen S. T., Toivari M., Wiebe M. G. (2017). Single cell protein-State-of-Theart, industrial landscape and patents 2001-2016. Front. Microbiol. 8. doi: 10.3389/fmicb.2017.02009
Salem M., Manor M. L., Aussanasuwannakul A., Kenney P. B., Weber G. M., Yao J. B. (2013). Effect of sexual maturation on muscle gene expression of rainbow trout: RNA-seq approach. Physiol. Rep. 1, 100–120. doi: 10.1002/phy2.120
Sankian Z., Khosravi K., Kim Y. O., Lee S. M. (2018). Effects of dietary inclusion of yellow mealworm (Tenebrio molitor) meal on growth performance, feed utilization, body composition, plasma biochemical indices, selected immune parameters and antioxidant enzyme activities of mandarin fish (Siniperca scherzeri) juveniles. Aquaculture 496, 79–87. doi: 10.1016/j.aquaculture.2018.07.012
Shen J. F., Chen M. C., Liu H. Y., Tan B. P., Dong X. H., Yang Q. H., et al. (2019). Effects of fish meal replacement by concentrated cottonseed protein on growth performance, serum biochemical indices, liver antioxidant indices and gastrointestinal tract protease activities of juvenile trachinotus ovatus. Chin. J. Anim. Nutr. 31 (2), 746–756. doi: 10.3969/j.issn.1006-267x.2019.02.031
Shi X., Luo Z., Huang C., Zhu X. M., Liu X. (2015). Effect of substituting chilorella SP. for regular fishmeal on growth, body composition, heoatic lipid metabolism, and histology in crucian carp carassius auratus. Acta Hydrobiol. Sin. 39 (3), 498–506. doi: 10.7541/2015.66
Tran H. Q., Prokešová M., Zare M., Matoušek J., Ferrocino I., Gasco L., et al. (2021). Production performance, nutrient digestibility, serum biochemistry, fillet composition, intestinal microbiota and environmental impacts of European perch (Perca fluviatilis) fed defatted mealworm (Tenebrio molitor). Aquaculture 547, 737499. doi: 10.1016/j.aquaculture.2021.737499
Vermeulen S. J., Campbell B. M., Ingram J. S. I. (2012). Climate change and food systems. Annu. Rev. Environ. Resour. 37, 195–222. doi: 10.1146/annurevenviron-020411-130608
Wang L. P., Chen F., Han Y. Z., Sun M. L., Pu N., Si B., et al. (2015). Research progress of branch chain amino acids (BCAA) and its requirement for aquatic animals. Feed Ind. 36 (14), 35–40. doi: 10.13302/j.cnki.fi.2015.14.008
Wang X. Y., Liu G. Q., Xie S. Q., Pan L., Tan Q. S. (2022). Growth and meat quality of grass carp (Ctenopharyngodon idellus) responded to dietary protein (Soybean meal) level through the muscle metabolism and gene expression of myosin heavy chains. Front. Nutr. 9. doi: 10.3389/fnut.2022.833924
Wang C. L., Wang Z. Y., Song C. W., Luo S., Yuan X. Y., Huang Y. Y., et al. (2021). A comparative study on growth, muscle cellularity and flesh quality of farmed and imitative ecological farming loach, misgurnus anguillicaudatus. Aquaculture 543, 736933. doi: 10.1016/j.aquaculture.2021.736933
Wei L. J., Ye C. X., Gan B. J., Teng Z. Z., Mo F. L., Zhang S. (2020). Analysis of growth characters and muscle texture characteristics of pangasius sutchi with different specifications. J. Aquac. 41 (8), 22–25. doi: 10.3969/j.issn.1004-2091.2020.08.005
Xi L., Lu Q., Liu Y., Su J., Chen W., Gong Y., et al. (2022). Effects of fish meal replacement with chlorella meal on growth performance, pigmentation, and liver health of largemouth bass (Micropterus salmoides). Anim. Nutr. 10, 26–40. doi: 10.1016/j.aninu.2022.03.003
Xue M. (2021). Processing technology of cottonseed protein concentrate and its nutritional value in aquatic feed. Feed Ind. 42 (12), 1–5. doi: 10.13302/j.cnki.fi.2021.12.001
Yao D. L., Liu Y. (2013). Effects of replacing soybean meal with peanut meal on growth performance of grass carp (Ctenopharyngodon idellus). China Feed. 11), 33–35. doi: 10.15906/j.cnki.cn11-2975/s.2013.11.012
Ye J. Y., Shao X. P., Ma H. J., Wu C. L., Ming J. H., Yang X., et al. (2013). Research progress on nutritional requirements and compound feed technology of grass garp (II). Sci. Fish. Farming 9), 79–81.
Yin B. (2019). Effects of plant protein sources replacing fishmeal on growth performance, intestinal microflora and immunity of juvenile hybrid grouper (Epinephelus Fuscoguttatus♀×E. lanceolatu♂) (Zhanjiang: Guangdong Ocean University).
Yun K., Wold B. (1996). Skeletal muscle determination and differentiation: Story of a core regulatory network and its context. Curr. Opin. Cell Biol. 8 (6), 877–889. doi: 10.1016/S0955-0674(96)80091-3
Zhang J. Y., Ren X., Li W. Y., Liu M. H., Wang L., Wang Y. (2020). Potential of replacing fish meal with cottonseed protein concentrate in black Sea bass (Centropristis striata) diet. J. Fish. China 44 (11), 1873–1882. doi: 10.11964/jfc.20191112048
Zhang T., Wu Y. Y., Li L. H., Wang Y. N., Ren Z. Y. (2013). Correlation analysis of sensory with instrumental texture measurement of salted fish. J. Fish. China 37 (2), 303–310. doi: 10.3724/SP.J.1231.2013.38218
Zhao F., Wu Z. X., Chen X. X., Jiang L. (2012). Effects of soybean meal replacement by rapeseed meal on the nonspecific immune function of gibel carp, carassius auratus gibelio. J. Huazhong Agric. Univ. 31 (2), 237–242. doi: 10.13300/j.cnki.hnlkxb.2012.02.022
Zhao H. H., Xia J. G., Zhang X., He X. G., Li L., Tang R., et al. (2018). Diet affects flesh quality and growth traits of grass garp (Ctenopharyngodon idellus): A comparison between grass and artificial feed. Front. Physiol. 9. doi: 10.3389/fphys.2018.00283
Zheng Y., Sun T., Ye Q. Q., Chen J., Bin S. Y. (2021). Application of plant protein in aquatic animal feed. Feed Res. 44 (1), 151–153. doi: 10.13557/j.cnki.issn1002-2813.2021.01.041
Zhong X. Q., Li X. F., Cai W. C., Xu C., Li Q. S., Huang J., et al. (2018). Effects of fermented feed on growth performance, digestive enzyme activity, fillet quality and immunity of juvenile carp (Cypriuus carpio). J. Nanjing Agric. Univ. 41 (1), 154–162. doi: 10.7685/jnau.201703024
Zhong G. X., Wang X. Q., Li J. B., Luo H., Deng Y. S. (2013). Effects of imported DDGS replacing soybean meal on growth performance of grass carp. Sci. Fish. Farming 2), 72–74.
Zou T., Cao S. P., Xu W. J., Han D., Liu H. K., Yang Y. X., et al. (2018). Effects of dietary leucine levels on growth, tissue protein content and relative expression of genes related to protein synthesis in juvenile gibel carp (Carassius auratus gibelio var. CAS III). Aquac. Res. 49 (6), 2240–2248. doi: 10.1111/are.13682
Keywords: complete replacement, chlorella powder, growth performance, flesh quality, muscle growth genes
Citation: Fan Z, Li C, Wu D, Li J, Wang L, Cao D, Miao L and Xie S (2022) Evaluation of four novel protein sources as alternatives to soybean meal for two specifications of cage-farmed grass carp (Ctenopharyngodon idellus) deeds: Effect on growth performance, flesh quality, and expressions of muscle-related genes. Front. Mar. Sci. 9:935651. doi: 10.3389/fmars.2022.935651
Received: 05 May 2022; Accepted: 07 July 2022;
Published: 02 August 2022.
Edited by:
Samad Rahimnejad, University of South Bohemia in České Budějovice, CzechiaReviewed by:
Shuang Zhang, Guangdong Ocean University, ChinaQihui Yang, Guangdong Ocean University, China
Copyright © 2022 Fan, Li, Wu, Li, Wang, Cao, Miao and Xie. This is an open-access article distributed under the terms of the Creative Commons Attribution License (CC BY). The use, distribution or reproduction in other forums is permitted, provided the original author(s) and the copyright owner(s) are credited and that the original publication in this journal is cited, in accordance with accepted academic practice. No use, distribution or reproduction is permitted which does not comply with these terms.
*Correspondence: Liansheng Wang, wangliansheng@hrfri.ac.cn; Shouqi Xie, sqxie@ihb.ac.cn