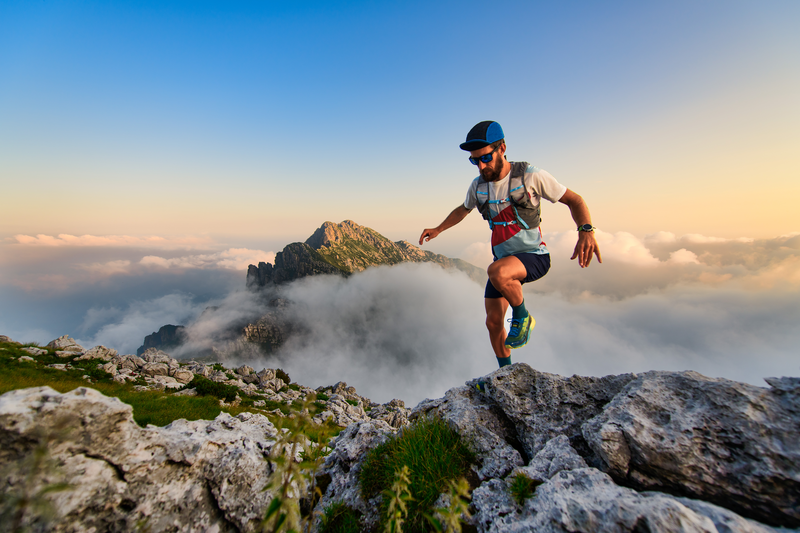
95% of researchers rate our articles as excellent or good
Learn more about the work of our research integrity team to safeguard the quality of each article we publish.
Find out more
ORIGINAL RESEARCH article
Front. Mar. Sci. , 23 November 2022
Sec. Coastal Ocean Processes
Volume 9 - 2022 | https://doi.org/10.3389/fmars.2022.933994
This article is part of the Research Topic Climate Change impacts on Mediterranean Coastal and Transitional Areas: Assessment, Projection, and Adaptation View all 10 articles
This paper discusses how the beaches of Marbella, on the southwestern Mediterranean coast of Spain, may no longer stay in their natural resilience envelope due to a critically delicate natural sediment transport balance and a degree of artificialization that has entered the whole sedimentary system into a new resilience state. The combination of vigorous terrain and millenary human action and disruptions across and alongshore on the coastline have increased stress on sediment availability. Although sediment circulation in the coastal cell has often been studied, the investigation of the connection between soil loss and river sediment transport and retention at a major dam remains a challenge. In this article, a first-order sediment yield prediction was established by using a GIS-based model applied to the area’s main river basin, and validation of model results is provided by empirical measurements of sedimentation in the main reservoir lake of La Concepción using Differential Global Positioning System (D_GPS)/Echo-sounder combination and measurements from remotely piloted aircraft compared with preconstruction blueprint topography documenting spot heights where sediments accumulated or eroded over 50 years. The marine circulation is interpreted from previous research by the authors that established a source-sink pattern similar to the Atlantic platform-fed marine system that originated the significant Cabopino dunes. The significant erodibility that we have estimated seems matched by potentially high sediment accumulation rates along selected profiles and spot heights across the bottom of the reservoir lake, and, in combination with the marine circulation model, our results identify that sediment budget key elements in Mediterranean settings, such as soil loss, sediment entrapment in reservoirs, and the coastal marine circulation, are in a state of deficit that suggests that the resilience envelope is surpassed and the system as a whole is entering a new resilience state in which the engineering factor is key. Some ecosystem services, such as the protection offered by the natural resilience of the beach and dune system of Cabopino, are no longer recoverable in the current artificialization state of the system as a whole.
In the Bay of Marbella, in the southwestern reaches of Mediterranean Spain, we investigate the complex issue of sediment circulation from terrigenous sources and its influence on chronic beach erosion that appears to be occurring in the littoral cell in the last decades. In natural conditions, the setting, at the confluence of the Atlantic and Mediterranean ecosystem transitional environments, provides the basis for vegetation patterns that cover with great efficiency the river basin’s slopes, affecting significantly the sediment production as the river basins’ soils appear to be fully sealed from rainfall with high vegetation interception rates. Thus, beach systems are adjusted to sporadic sediment input to which morphodynamic marine processes respond with narrow beach faces and surf zone where low-energy waves initiate littoral drift and, consequently, redistribute sediments on narrow steep beaches. This is the natural circulation model on most Mediterranean coasts, where beaches develop due to the connection between terrigenous and marine sedimentary systems (Malvárez et al., 2021). A peculiarity of the environment in the Bay of Marbella (Figure 1), as in other Mar de Alboran coastal stretches, is that the continental shelf stores large deposits in some places that serve as a source when connected to the beach-dune system, generating large strand-like settings. Most Mediterranean coastal dunes fronted by steep continental shelves are not associated with glacial deposits but rather with those of river mouths and/or coastal lagoons (Pye, 1983). Sometimes, when conditions are favorable and sediment budgets are sufficient, Mediterranean dunes can grow to extents and volumes comparable to European Atlantic dunes, even under low-energy settings from both wave and wind forcings, as well as intermittent and limited sediment supply. This is the case of the Cabopino dunes, at the eastern end of the Bay of Marbella (Figure 2), with a vegetation that is more complex and diffuse than that of Atlantic or other open-ocean-type dunes (Sanjaume and Gracia, 2011). This vegetation assembly is also more sensitive to Mediterranean/Atlantic exchange and sediment feeding (Malvarez et al., 2019); thus, sediment transport stability from source to sink is key to the natural resilience of the beach–dune system. However, interruption of sediment supply from marine sources to the coastal system, as well as sediment cell nearshore circulation patterns, can disconnect, if not eliminate completely, the nearshore–beach–dune feeding pathway (Willis and Griggs, 2003). The continental shelf fronting Marbella is steep, and the input area of the coastal system is reduced to fluvial yields to the west. The circulation to the east provided sufficient sourcing to beaches in Marbella and even to the Cabopino dune complex, but sequential elimination of input has led the transport to bypass the system and now head directly to deep sinks like canyons and sand bodies beyond closure depth for beach interaction (see Figure 2).
Figure 1 (A) Map of the study area shows the location of the embayment. The river basin of Rio Verde is drawn to indicate the scale of the basin, which has been modeled for sediment production and is interrupted by the La Concepción dam. (B) Wave rises in winter from Alboran Sea wave buoys illustrate the intense bi-directionality of wind waves.
Figure 2 (A) Continental shelf of Marbella Bay. (B) Bathymetric changes from the 1888 seabed map and 2010 bathymetry (from Guisado-Pintado et al., 2015).
Human-induced changes to the natural sediment transport balance have pushed most of the natural coastal systems into a situation of fragile equilibrium. Severe modifications of natural processes are not solely constrained to direct destruction from urbanization or infrastructure on the coastal strip but, more importantly, affect the sediment feeding system by introducing changes in the original settings that generated coastal features such as beaches and dunes (Anthony et al., 2014). Additionally, human action has influenced vegetation cover in river basins and, thus, soil loss has been a long-term issue that has even provided, during most of the Holocene, substantial filling to the lower reaches of the drainage network that, although were estuaries during the Late Pleistocene, form deltas nowadays (Senciales and Malvárez, 2003). However, valley infilling and prograding shorelines have stopped as a result of the intensification of human action, and erosional processes are not only evident along the coastal strip itself but also in the terrestrial hinterland and nearshore environments that control the natural sediment nourishment of beaches (Del Río et al., 2020).
An extraordinary positive balance in sediment yield is caused when the degradation of the upper and mid-reaches of the river basins, brought about by land use change, induces increased yields and sedimentary surplus in the coastal cell (Anthony et al., 2014). Abundant material for the marine environment generates significant deposits on the upper shelf. Volume growth of coastal deposits and littoral drift dominated these phases, generating wide beaches and, then, dune systems. Often, the existence of a long well-fed beach system facilitated economic activity associated with tourism in its many aspects (Navas, 1996; Giordano et al., 2015). However, infrastructure and activities that are deployed and maintained for the recreational industry are at risk from (and pose a risk to) coastal morphological changes (Cooper and McKenna, 2008; Navas et al., 2017) and, consequently, management initiatives are implemented systematically to maintain the position of the shoreline and tourism-related facilities. These facilities are traditionally viewed as human interventions in a natural system, but they have evolved to such an extent that some authors maintain that they now form an integral part of a coupled human-natural coastal system (CHANS), that is characteristic of (and response to) conditions prevailing in the Anthropocene (Polhill and Gotts, 2009; Lazarus, 2017; Quinn and Wood, 2017). In natural beaches, changes in morphology are driven by dynamic forces (waves, currents), operating on a particular volume of sediment, within the boundaries of the surrounding geological framework and position of sea level (e.g., Cooper et al., 2004; Pilkey and Cooper, 2004). How coastal systems operate in the coupled human- and natural-driven conditions is becoming of great interest, with the increased urbanization (Navas et al., 2012) and the intensification of economic activities in coastal areas that involve built infrastructure and often associated sea defenses, whether hard or soft.
In Marbella, during the late nineteenth century and mostly through the twentieth century, the increasing concern in the management of water and beaches led to the construction of dams in the hinterland and groins and many other infrastructures along the shorelines (Malvarez, 2012). Dams were first constructed to generate water flows for iron production and irrigation and then, mostly during the late twentieth century, to satisfy the increasing demand for water as a result of the massive urban development along the coast of Marbella (Figure 1) (Del Río et al., 2020). This has promoted the rapid reversal of the morphodynamic processes that affect the sedimentary units (e.g., beaches, dunes, and submerged sand bars) of the coastal system, resulting in severe erosion of beaches and the interruption of dune building trends.
Socioeconomic activities and related land use had a severe impact on soil erodibility during the last four centuries. In the eighteenth century, the economy revolved around the primary sector, mainly sugar cane cultivation. Later, at the beginning of the nineteenth century, the most powerful iron smelting industry of Spain settled in the largest river basin of the Marbella’s hinterland, the river Verde. Two minerals melting infrastructures, that used wood as fuel and water for cooling the smelting furnaces, were constructed along the river bank and led to the vast deforestation of the surrounding forests in barely 17 years (Madoz, 1848; García-Montoro, 1983). The high-water demand associated with sugar cane cultivation led to the construction, between 1882 and 1903, of 7 dams and reservoirs of some 106 m3 in the lower reaches of the Guadalmina and Guadaiza rivers. During the first decade of the twentieth century, the iron melting and sugar cane manufacturing industries disappeared, and the tourism industry began to progressively increase in importance, reaching its peak during the second half of the twentieth century. Within this period, the number of households increased from 2,000 to 35,000, and the population increased from 10,000 to 100,000 inhabitants. According to the Institute of Statistics and Cartography of Andalusia, the number of inhabitants living in Marbella in 2021 was 147,958, which represents an acute growth of a steady 200% per decade during the last four decades. In response to population increase, the La Concepción Dam was built in 1971 in the lower course of the river Verde, which has the greatest water course in terms of drainage area and length (Figure 1). Besides ensuring a fresh water supply, the La Concepción Dam is also used for flood control, given the seasonality of the precipitation regime in the area. To keep constant water storage during the dry season, a complex water transport system connecting the Guadalmansa, Guadalmina, and Guadaiza rivers, generating a one-way freshwater flux towards La Concepción, was built in 1995. This reservoir lake also receives water from a desalination plant constructed in 1992. Artificial lakes created by the dams tend to silt up due to unresolved land degradation on the steep and bare slopes.
Since shoreline retreat and beach dynamics have been documented in previous research and reports (e.g., Malvarez et al., 2019), in this paper we focus on the linked processes that have generated stress on the natural resilience of the coast of Marbella. This is carried out by documenting calculations of soil loss from the river basin and reservoir lake siltation caused by the construction of the dam at La Concepción. Then, a qualitative assessment addresses natural resilience in the current scenario, where sediment input is restricted to the coastal cell. The nature and efficiency of beach management arrangements are coupled with natural processes as a vital component of coastal resilience. However, as many natural systems are massively distorted by human action, natural and built environments coexist in a blurred and complicated definition of resilience (Masselink and Lazarus, 2019). The concept of a sediment budget then implies an accounting view on the feeding and circulating mechanisms of the coastal cell, whose longevity and potential adaptability are subjected to alternating fluctuations in input and output within the system feeding and sinking sediments (Del Río et al., 2020). Thus, in this study, we take this view and incorporate inherent complex interactions of coastal environments in their natural state as natural resilience. The concept of the resilience of a given natural (eco)system can be regarded as the ability of a system to persist by maintaining the key structures and processes that characterize that domain (Francis, 2013). This implies an adaptive capacity of the system to reorganize in the face of disturbance while maintaining key structures and processes. In a coastal setting, features can shift in position (e.g., by roll-over, retreat/advance) and retain all the key structures and functioning. This contrasts with the definition of engineering resilience, sometimes also called recovery, where the system and functions need to be restored in the same position (Adger et al., 2005; Francis, 2013; Flood and Schechtman, 2014).
Given the multiple components present when a complex natural and human-intervened coastal site is to be studied, in this paper, we present (i) an approach to quantify sediment production and yield from the main river basin in the coastal system, then (ii) an estimation of reservoir lake infilling over the years of the dam’s operation.
The climate regime of the study area is semi-arid, with mild air temperatures in winter that range from 15°C to 24°C. The precipitation regime is seasonal, sharply concentrated in autumn and winter. Heaviest rainfall events, reaching thresholds that occasionally range from 160 to 300 millimeters (mm) in 24 h, are consistent with the arrival of cold fronts coming from the east, originated by the warming of the Mediterranean Sea, causing serious flooding.
The arid climate, the occurrence of episodic, heavy rainfall events discharging large volumes of water with high-energy on steep, impervious soils sparsely vegetated in some cases, would contribute to producing overland flows triggering severe soil erosion and, consequently, potentially high sediment yield rates at the basin scale. The majority of the river basins reveal the presence of alluvial fans, though the largest formation in terms of volume of lithogenous sediments is located on the foothills of the mountain range surrounding the municipality of Marbella to the north, whose origin dates from the Late Pleistocene according to Lario et al. (1993).
The river basins in the western section of the Marbella’s hinterland, the Guadalmina and Guadaiza rivers, have a narrow and elongated morphology, while central watersheds (Verde and Real rivers) show a wider typical leaf shape, as shown in Figure 3 (Rio Verde). According to CMA (1999); CMA (2005), and following the soil classification system of the World Reference Base for Soil Resources (IUSS-FAO, 2014), the prevailing soil types of the river basins are lithosols, cambisols, luvisols, regosols, and fluvisols, whose textures range from sandy-loam (calcareous fluvisols) to silty-loam (chromic luvisols, Rendsina soils, and cambisols rich in calcium) and clay-loam (eutric regosols and eutric cambisols). Following the Hydrologic Soil Group defined by NRCS (1986), such textures are associated with soils having low permeability (Figure 4A).
Figure 3 Topography and land cover classes of the river Verde basin. (A) Topography has been calculated by using the digital elevation model (DEM) at a 25-m spatial resolution taken from the National Geographic Survey of Spain; (B) land cover classes have been represented according to the official Corine Land Cover dataset collected in 2006.
Figure 4 Soil categories and midslope of the river Verde basin. (A) Soil types shown following the nomenclature stated by the Food and Agriculture Organization (FAO). (B) Midslope has been calculated in degrees by using the digital elevation model (DEM) at a 25-m spatial resolution provided by the National Geographic Survey of Spain.
In the marine environment, beach profiles are usually steep and far from dissipative, modally adjusted to short-period waves generated by the local winds of the Alboran Sea, generating a steep and narrow surf zone (Malvárez, 1999). In terms of wave climate, sea waves are common and only rarely does swell occur given the limited effective fetch with an average distance of 500 km (Figure 1B). The wave climate in the region is characterized by long intervals of calms of over 77%/year, in which the wave heights are less than 1 m. Mean heights are around 0.8 m in summer and 1.3 m in winter with wave periods of 4–5 s corresponding to short-crested waves forced by local winds. Wave and wind approaches are characterized by the alternation of almost 50% of westerly and easterly winds, both in summer and winter seasons (see Figure 1B). The tidal range is microtidal (<0.2 m average astronomical tidal range) and semidiurnal and, thus, the main forcing on the coast is wave action.
In terms of high-energy events, decadal records show that storms are characterized by a low variability of wave approach; the majority are easterly events (80° to 135°) with deep water wave heights greater than 5 m, although closure depths are estimated in the Bay to be extraordinarily shallow at some 3.5 m at some 200 m from the shoreline (MTERD, 2022).
The approach provided in this paper is based on the combination of modeled predictions of potential sediment yield at basin scale using a GIS-based model, associated with measurements obtained with D_GPS and Echo-sounder surveys conducted to establish the bottom topography of the reservoir lake formed by the dam of La Concepción. The measured bathymetry was then overlaid over a topographic surface reconstructed from blueprint documentation obtained prior to dam construction. The relationship between sediment yield and retention by the dam is estimated by a number of spot elevations comparing the results of a 2019 survey and 1966 digitized data at La Concepción. This comparison is aimed at quantifying and qualifying the overall accumulation and its implications in sediment starvation in the coastal area. Finally, a resilience conceptual model is described in order to consider sediment budget resilience.
In general, soil erosion and sediment yield models fall into three categories: (i) empirically, (ii) conceptually, and (iii) physically based models. The latter, such as the Water Erosion Prediction Project (WEPP) or Areal Nonpoint Source Watershed Environment Response Simulation (ANSWERS) models, among others, offer the most accurate predictions. They do, however, involve a large number of physical parameters integrated by equations that have been developed to work at small scale (small basins) and under very specific physical conditions (Merrit et al., 2003). This implies that a huge amount of onsite input data are required to run these models with sufficient accuracy. It is important to state at this stage that some data that are needed to run these models (e.g., grain size distribution in the hinterland, land management practices, or hydrological data with temporal and spatial variability) were not available in the literature nor were they collected by the Public Administration. The predictions provided in this work constitute the first attempt to estimate the sediment yield potential in the study area. In such cases, where input data required by more complex modeling tools are either lacking or too limited, the use of empirical models becomes the single feasible solution (Merrit et al., 2003; Rajabi et al., 2022) to predict the sediment behavior at medium-sized river basins. Several empirical models have been developed since the mid-twentieth century (e.g., AGricultural NonPoint Source (AGNPS) or SEdiment Delivery Distributed models (SEDD)), but the Modified Universal Soil Loss Equation (M-USLE; Wischmeier and Smith, 1960; Williams, 1975; Eq. 1) was used to obtain a first-order prediction of sediment yield of the river Verde basin during a single rainfall event.
Where Y is the sediment yield prediction in metric tons, Q is the runoff volume in cubic meters, qp is the peak flow rate in cubic meters per second, K is the USLE soil erodibility factor, LS is the USLE slope length and gradient factor, C is the USLE cover management factor, and P is the USLE erosion control practice factor.
Leaving aside the above, the choice of using the M-USLE against other sediment yield models is sustained by three additional criteria:
1. Data requirements: The input data required by the M-USLE model are described in greater depth within Section 2.2.1.2. Nevertheless, reliable data on topography, soil types, precipitation, and land cover have been made available in the literature and by the Regional Government of Andalusia and were consulted accordingly.
2. Validity of the model: The USLE family of models has been globally used to predict soil erosion and sediment yield at the basin scale since the mid-twentieth century and has been the subject of recurrent validations over time. In fact, the USLE was used by the Regional Government of Andalusia to estimate those soil erodibility and cover management factors that were used for the calculations provided in this work, and the M-USLE is being used recently to predict potential sediment yield in other Mediterranean watersheds (Gwapedza et al., 2020; Berteni et al., 2021; Yousuf et al., 2022; among others).
Software requirements: The use of a Geographic Information System (GIS) for water erosion and sediment yield modeling provides valuable advantages, such as the facilitation of multiple-source data processing in a timely and cost-effective manner (Pandey et al., 2016). Following this premise, a raster-based spatial analysis model called Arc-MUSLE, developed as a redistributable ArcGIS® extension integrating the M-USLE empirical equation by Zhang et al. (2009), was used for sediment yield predictions. The application of the Soil and Water Assessment Tool (SWAT) model was also explored but, as a physically based model, it required (i) hydrological data, such as flow direction, length, and accumulation through the hydrographic network, (ii) spatial variability of the rainfall stations distribution, and (iii) single classification and spatial variability of soils along the watershed that were not available nor were they inviable to be collected in the study area. Due to these reasons, the use of SWAT was ruled out, and the M-USLE was selected as the most appropriate model that matched the scope of this work.
Assumption 1: Sediment yield against soil erosion.
The M-USLE was developed by Williams (1975) as a watershed-based model to estimate the sediment yield, defined by the United States Geological Survey as the amount of terrigenous sediment per unit area removed from a watershed by flowing water during a single storm event. Conversely, the original USLE and RUSLE models were developed to predict the long-term average annual rate of soil erosion from agricultural fields under specific cropping and management practices. Considering the above, the use of the M-USLE is more in line with (i) the nature of the process studied in this work, the prediction of sediment yield rather than soil erosion, (ii) the spatial scale, at the watershed level instead of at experimental field plot scale, and (iii) the temporal framework, at an event-based scale against long-term annual predictions.
Assumption 2: The runoff energy factor as the main driver of sediment yield.
The original USLE and RUSLE models consider the rainfall erosivity factor (R), a multi-annual average index that measures the rainfall kinetic energy and intensity to assess the effect of rainfall on sheet and rill erosion. Instead, the M-USLE considers the runoff energy factor, defined within Eq. 1 as 11.8*(Q*qp)0.56, as the main driver of sediment yield. In fact, the runoff was demonstrated to be better correlated to sediment yield than the R factor, and it was deemed as the best single indicator for sediment yield estimations (Williams, 1975). ArcMUSLE calculates the runoff volume (Q in Eq. 1) following the Curve Number method, developed by the Natural Resources Conservation Service of the United States in 1964 and subjected to subsequent revisions so far. In those areas where no runoff data are available, as it occurs in the study area, this method may estimate the depth of direct runoff from the rainfall depth. The qp is obtained by applying the graphical peak discharge method, developed by the same United States Organization in 1986 as a function of the drainage area, the runoff depth, the time of travel for sheet and shallow flows, and Manning’s roughness coefficient. This last is a methodology commonly used in civil engineering studies in the United States to calculate the resistance of flood flows in channels and flood plains.
Assumption 3: Input data.
Data used to run the model followed Zhang et al. (2009), and thus, layers for topography, land cover classes, soil types, and a 2-year, 24-h rainfall event required were developed from various data sources as input data for the sediment yield assessment at the basin scale.
1. Topography: Watershed topography of the river Verde basin was integrated in ArcMUSLE as a raster layer on the basis of the 25-m resolution DEM, developed by the Spanish National Geographic Institute following the European Terrestrial Reference System 1989 (ETRS89).
2. Land cover data: Land use categories of the river Verde basin (Figure 3B) were extracted from the 2006 CORINE Land Cover (CLC) database, developed by the EU Copernicus. Three attributes were bound to each land cover class; (i) land cover codes, according to the classification system stated by the United States Geological Survey (USGS), (ii) the cover management factor (USLE C factor), and (iii) the Manning’s roughness coefficients. Land cover codes used by the CLC 2006 were converted into the USGS Land Cover classification according to Anderson et al. (1976). Given the lack of recent USLE C factor repositories in Andalusia, the cover management factor was defined according to those empirical values published by the Spanish Institute for Nature Conservation (ICONA) and following Moreira (1991) and Panagos et al. (2015a). Manning’s roughness coefficients were determined according to Engman (1986) and Vieux (2004).
Soil types: Soil types of the river Verde basin were extracted from the Soil Map of Andalusia (Figure 4A; CMA (2005), where soils are spatially classified as categories rather than as single soil types. Three attributes were linked to each soil category; the midslope (Figure 4B), the soil erodibility factor (USLE K factor), and the Hydrologic Soil Groups (HSGs). These HSGs consist of four qualitative groups (A–D) through which soils are categorized according to their runoff potential, which is logically a function of their texture and soil layer depth (A group means loamy sand, sandy loam, and silt loam soils having low runoff potential and D means soils having high runoff potential and having greater than 40% clay). The midslope was calculated on the basis of the DEM. Considering that soil categories comprised more than one soil type and, consequently, a wide range of soil textures interbedded, the definition of representative USLE K Factors and HSG required the prior establishment of the prevailing texture of each soil category on the basis of the World Reference Base for Soil Resources (IUSS-FAO, 2014). The USLE K factor was then determined following the empirical values published by Stewart et al. (1975); Schwab et al. (1981); Parveen and Kumar (2012), and Panagos et al. (2014), and the HSGs were fixed according to the Natural Resources Conservation Services of the United States Department of Agriculture (NRCS, 1986).
Precipitation data: According to Zhang et al. (2009), ArcMUSLE considers the most intense 24-h rainfall event registered in two consecutive years to define the storm event triggering sediment yield. The 2 years assessed were selected according to the same period as both the Soil Map of Andalusia and the CORINE Land Cover database were developed (2005 and 2006, respectively). The daily rainfall volume recorded from the 1 January 2005 until the 31 December 2006 by the weather station installed by the State Meteorological Agency of the Spanish Government in the Marbella harbor was then analyzed. The heaviest rainfall event occurred on 7 November 2006 and registered 91.8 mm in 24 h. Thus, it is reasonable that the two-year rainfall event considered represents conveniently the nature of the storm events that activate the sediment yield processes in the river Verde watershed.
Other input data: Sediment yield assessment is completed by providing (i) the Antecedent Moisture Condition (AMC), (ii) the volume of precipitation (in inches), and (iii) the Conservation Practice Factor (USLE P Factor). First, and following Singh (1982), the AMC of the watershed was determined by analyzing the volume of precipitation registered during the five days prior to the rainfall event considered for the analysis. Therefore, 14.5 mm was registered in Marbella from 2 to 6 November 2006, consistent with average moisture conditions’ according to the Curve Number Method (NRCS, 1986). Secondly, the volume of precipitation was introduced in the modeling interface. Finally, given that the USLE P Factor is not estimated by the Regional Government of Andalusia for soil erosion risk modeling, a value of p = 1, equivalent to “no conservation practices conducted,” was assumed for the sediment yield prediction according to Panagos et al. (2015b), which likewise matches with the default value proposed by Zhang et al. (2009) in those areas where the USLE P Factor value is unknown.
To calculate the approximate position of the bottom topography in the reservoir lake of La Concepción before and after the construction of the dam, we had to determine the initial topography of the valley before the start of sediment retention (1966) and the current topography of the valley after 50 years of sedimentation. First, to obtain the topography of the valley prior to dam construction, secondary sources were searched. Large format plans of the original blueprint documents from the engineering works of 1966 (Water Management Authority; from Demarcación Hidrográfica de las Cuencas Mediterráneas Andaluzas) were first scanned to obtain the topography of the valley prior to dam construction. This surface is called here the 1966 topography (Figures 5, 6). A field visit was carried out to occupy with a Differential GPS the original 1960s bench marks and other topographic triangulation posts. These, and numerous other landmark coordinates obtained from the Orthophoto PNOA 2018 published by the Instituto Cartográfico de Andalucía, were used to carefully georeference the scanned plans using QuantumGIS (QGIS) in order to digitize contour lines from the original scanned plan. After georeferencing the scanned image, vector layers of points were generated. Vector point layers marked the 121.5, 96.5, 71.5, 61.5, 56.5, 51.5, and 46.5 m.
Figure 5 (A) Location of original digitized and surveyed elevations from 1966 to 2019 and spot heights used for comparison. Color in labels indicates the sedimentation/erosion observed in the comparison between 1966 and 2019 in meters. The letter inside the circle symbol refers to the letter mentioned in the text. (B) Benchmarks and positions with XYZ coordinate provided by the blueprint documents of dam construction from 1966. Points and marked ways were reoccupied in 2019 with D_GPS to establish the link between topographic surveys.
Figure 6 Cross sections of the reservoir lake of La Concepción. Labels are referred to Figure 5 locations. (A) Cross section from the east shore of spot elevation B to the west of spot elevation L. (B) From east of point A to directly east.
To obtain the current elevation values for the same surface, now under some 50 m of water in some sections of the La Concepción lake, a vessel was used equipped with a chirp (Garmin Striker Plus 9SV CHIRP Sounder) and integrated GPS for navigation and the 800-kHz transducer for depths with a vertical resolution of 0.1 m in measuring the vertical distance between the water level and the bottom surface. In addition, measurements were made with a GPS Leica Zeno 20 in differential mode using corrections and increased precision by 3G on the shore of the reservoir, thus obtaining the coordinates and height around the water body as a baseline for later calculations. For correct elevation data of the water mark at the time of the survey in 2019, topographic data was generated using a DJI Inspire 1 Remotely Piloted Aircraft (RPA) equipped with a 4K ZENMUSE X3 camera for photogrammetry. Up to 120 images were combined using Pix4D software to develop a Digital Elevation Model with a theoretical precision of 4 cm in three dimensions. Overlap was established at 70%–80% side and front, respectively. Comparison with known bench marks allowed precise estimation of shoreline elevation. Data from the drone survey were used to support shoreline position elevation detection, as well as to document sedimentation bodies that were emerging at the time of the survey at the higher end of the reservoir lake.
In terms of uncertainty levels, the individual methods (i.e., Echo-sounder, GPS, and RPA) may be in the centimeter range of error, but the accumulated error is hard to estimate given the pitch and roll and other effects of the boat in motion. The network of control points occupied by the GPS was used to minimize through global corrections, the potential deviations of the Echo-sounder and RPA. Data acquired with various techniques introduce an overall uncertainty level that could only be tested in the combined files introduced in the GIS for spatial analysis. Thus, once these measurements were obtained, points were exported to QGIS software, and a vector layer of points was created as 2019 elevation points (Figure 5). After corrections, depth values were transformed to a height above mean sea level using lake shoreline elevation from the drone survey, corrected by benchmarks reoccupied at 1966 points with the D_GPS taken to tie elevations. Likewise, numerous features visible in the 1966 plans were visited to use them as ground control points.
In order to assess resilience in this paper, the principle of resilience is defined as “the capacity of a system to absorb disturbance and reorganize while undergoing change so as to still retain essentially the same functions, structure, identity, and feedbacks” (Scheffer and Carpenter, 2003; Folke, 2006; Flood and Schechtman, 2014; Kombiadou et al., 2020). Specifically, the concept used here for assessing resilience follows Kombiadou et al. (2020). A fundamental principle is that the state of resilience can be represented in a Basin of Attraction (BoA) model where three dimensions are used: a, b and c. These are related to the position of the system within the shape of the BoA (Gunderson et al., 2012; Kombiadou et al., 2020). Figure 7A illustrates resilience concepts used in this article expressed in relation to the usual description for wave geometry, (a) Latitude is the wave length of the BoA and indicates how much the system is able to change irreversibly; (b) Resistance is the wave height of BoA and depicts the amplitude of changing range prior to stepping to another trough; (c) Precariousness is the position of the system state in relation to a threshold, a point of no return as the system enters a new BoA. The measure of restoration of the system by natural or assisted “engineering” depicts its ability to recover and stay in the same state and/or position. It is understood that the natural state and position of the system should be the long-term state and position and that any significant change in beach profile and, more specifically, a shift in position landwards of the entire system implies natural resilience “moving” into a new BoA.
Figure 7 (A) Key concepts in the Basin of Attraction model. Once the sediment budget is artificialized, resistance and latitude are greatly diminished, thus compromising sustainability and/or adaptability. (B) The system in its natural state moves well within the threshold for centuries. (C) As the threshold is surpassed frequently due to stress from the input, the system enters a different BoA. (D) Once the system feeding is eliminated, there is no return to the broad natural BoA, and the engineered state remains in a low-resistance BoA.
The potential sediment yield predicted in the river Verde basin during the 2-year, 24-h rainfall event considered was 294,000 t (Figure 8A). Potential sediment production of the rest of the river basins feeding the Bay of Marbella during the same precipitation event was also estimated, and results are shown in Table 1.
Figure 8 Spatial distribution of sediment yield and runoff rate in the river Verde basin. (A) Most erosive areas were predicted in the river Verde basin using the M-USLE. Deforested areas during the nineteenth century were outlined through a dashed line in black according to [18]. (B) Areas of the river Verde basin where the highest runoff rates (darker areas) would be registered according to the predictions obtained by using the M-USLE.
Estimated values presented in Table 1 suggest that potential sediment yield and the drainage area of the river basins seem to be fairly well correlated, except in the case of river Real, where the occurrence of a forest fire in 2005, which was also assumed within the calculations according to the original data source, is probably underestimating the potential sediment yield modeled by ArcMUSLE. The spatial distribution of potential sediment yield and surface runoff rate estimated in the river basin is shown in Figure 8B, where darker areas mark the maximum rate of surface runoff. Steep-slope agricultural lands (midslope ranging from 26.6° to 34.7°; Figure 4B) located on the eastern hillside of the reservoir would also register a high runoff rate and sediment yield. Such results would be consistent (i) with their low Manning’s roughness coefficient (n = 0.035) when compared to the coniferous forest or sport and leisure facilities’ roughness values (n = 0.6 and 0.45, respectively) and (ii) with their moderately high cover management factor (USLE C factor = 0.1457) in comparison with the C factor registered by dense and scattered forested areas (C = 0.0015 and 0.0296, respectively.
The erosive trend obtained in the Discontinuous Urban Fabric (CLC-06) might be interpreted as urban soil erosion rather than sediment yield, so it has been kept out of the calculations of our study.
The minimum sediment yield registered in the limestone rock formations of Sierra Blanca and Sierra de las Nieves, in the lower and upper reaches of the river basin to the east and north, respectively (highlighted in Figure 8A), seems to be consistent considering the soil types of mountain chains. Lithosols, calcic cambisols, and chromic luvisols, mainly composed of limestones with slates, greywackes, marbles, and quartzites interbedded with moderately low runoff potential (Hydrologic Soil Group B), would result in a prevailing water infiltration over the formation of surface runoff and soil erosion.
The elevation was digitized using the original topography of the valley prior to dam construction in 1966. Elevations are related to the mean sea level (MSL of Spanish altimetric datum). Depths, obtained by Echo-sounder and GPS boat survey in 2019, were calculated relative to known locations corrected from local bench marks established in 1966 when the dam was constructed, thus tying the datum to the original 1966 data digitized. The data obtained from the boat survey are shown in Figure 5.
Although volume comparison would have been easier by overlapping two modeled raster surfaces, the comparison of the interpreted surfaces was complex due to the many issues in overlapping the 1966 and 2019 topographies. Data were concentrated in the case of the 1966 digitized lines and also along Echo-sounder lines. Gaps between lines forced interpolation, resulting in inaccurate raster models, and although surfaces were generated by overlapping the data digitized from 1966 and the depths surveyed in 2019, the results were deemed inaccurate. Instead, comparing directly overlapping point data from the two vector layers yielded much more accurate results. The comparison of selected spot elevations is used to help clarify the outcomes of the analysis. It was decided to establish 11 spot elevations in the lake to be representative of bottom topography change at areas where sedimentation or erosion was expected. The average sedimentation at the spot elevations is 2.95 m and is distributed following the center-left-hand side of the lake in what appears to be delta formations of successive sediment transport events. Points D, B, H, and E differ by an average of 5.6 m. There are, however, some erosion spots cut into the bottom sediment pack. Points G and K registered a deepening of 1.9 and 2.9 m, respectively, over the years, and both are located at the right margin of the lake. Thus, the overall increase in the elevation of the bottom surface indicates sedimentation. As an example of the evolution of lake bed bathymetry, two cross-section profiles were drawn, and the bottom topography was represented for the two dates under consideration. Corresponding to the cross sections (Figure 6A, B) that extend from shores east of spot elevation B to the west of L in Figure 6A, two localized accumulations at the banks indicate possible deltas from the right and left banks due to the contribution of small streams in the proximity. However, their sediment supply seems to be substantial as the morphology interpreted along the x-axis of the figure between 200 and 300 m averages an accumulation of 5 to 6 m. The wedge observed from 500 m to the actual shoreline appears to describe a different morphology of gradual accumulation rather than deltaic deposition due to the calcareous nature of the slopes on the left bank of the river and, thus, the lake. Taking an average value over the entire lake of 2.0 m, considering cut and fill, and given the approximate area of 2 × 106 m2, accumulation of 4 × 106 m3 can be calculated which yields a rate of 75.5 × 103 m3 year−1.
The circulation of sediments in the input/output approach offered by the traditional sediment budget model requires free flow from source to sinks and sustainability of fresh input when sinks are permanent, as they appear to be in the deep canyons offshore of Marbella. However, the main management action since the 1970s has been to rebuild the occupation at the frontline, reconstructing roads, parks, and bars/restaurants. This artificially induced engineering resilience, where the system position is preserved, does not intend to maintain the link between source and sink and eliminates the ecosystem services naturally offered as the protection function of the beach. The total volume in front of rigid occupied areas will be reduced and the system will be less and less effective in its inland protection, thus tending to a smaller degree of resilience with time. Higher storm impacts, or even the loss of protection functions such as total erosion of the berm and dune, could be expected in the current climate change trends, with rising sea levels and more frequent and intense storminess in the Mediterranean.
Figure 7 shows, as a conceptual characterization, the beach system of Marbella and its sediment budget resilience (Figure 7A). The natural resilience depicts a narrow, shallow BoA where the conditions of resistance are easily exceeded given that (i) wave direction is highly bimodal and (ii) storm waves can activate a very active surf zone, inducing powerful littoral drift in both eastern and western directions. The sediment input in the system could be translated in the latitude of the BoA, which is critically reduced given the significant decrease in sediment yield due to dam retention. The conceptual assessment would induce the system to move from a scenario of natural resilience (Figure 7C) to one of engineered resilience (Figure 7D). As sediment volumes from the beach system are in circulation and away, the natural resilience moves beyond the threshold of the BoA into a new natural/ecological scenario. Since no new sediment is input in the quantities registered in the original BoA, it moves to an engineering resilience BoA (Figure 7D), typically constrained into a set of boundary conditions. The current situation is hardly a sustainable competition against the system’s attempt to move away from a natural BoA. The new BoA, where the system would develop, would typically be of less resistance and latitude given the limited availability of resources (economic, technological, and beach fill material). The results suggest that an adaptation is not achievable in the long term if sediment yields are not activated by local drainage systems.
The Bay of Marbella is limited to the east by the Cabopino dune system, where some formations of very significant infralittoral prisms suggest the classic geometry of spits but lie submerged (Malvarez et al., 2019). These formations document a previous tendency for sediment accumulation with the structure of a drift-aligned coastline. The morphology corresponds with those in which the feeding system must have been generated by large deposits or injections at a recent time. Likewise, the “source” zone is likely updrift within the Bay of Marbella and fed directly by fluvial input as the coastal cell does not seem connected to other sources (fluvial or marine) further afield to the west from the Guadalmina River (see Figure 1 for reference).
The recent evolution of the shoreline in the Bay of Marbella has shown a consistent retreat since 1956. Though the shoreline has been overall transgressive, its position has been heavily influenced by engineering structures, such as the groin fields of 1973, which produced a net advance of the shoreline but introduced localized hotspots of erosion, causing a sequence of narrow/steep sections at intervals (McDowell et al., 1993). The deterioration of back beach resources for storm protection caused various initiatives to be put in place since the 1970s to date, resulting in significant beach fills over the years (Malvarez, 2012). The sediment volume, which is estimated for the most recent replenishment to allow the creation of a 40-m average width beach over some 6-km stretch (20 m narrower than the beach resulting after the beach nourishment intervention of 1990) is 4.5 × 105 m3 (MTERD, 2022). This amount would generate a profile that aims at remaining for the tourist season rather than increasing the system’s resilience. Given that the estimated sediment transport in the cell is of some 1.45 × 106 m3 year−1 as calculated using the CERC formula, the beach nourishment project only sustains resilience at a mere emergency level, following trends of engineering resilience dependency (Malvarez et al., 2021).
As a direct discussion of the results obtained from the calculation of the model for sediment production and yield, we find that the M-USLE version, made extensive to the river basin, was necessary and satisfactory for the scale and scope of this research. Working scale makes it inviable to collect soil erosion data from the watershed by adding plot size experiments or calibrations. The GIS-based model taking M-USLE inputs and calculations is, actually, the only viable source of information that may be assumed for the study area. Moreover, the intended goal of characterizing a first-order approach to sediment production without intentions to precisely quantify a total load into the marine environment follows the literature for similar studies that, almost inevitably, result in uncalibrated outcomes for real sediment yield, an almost impossible task given the complexity of the processes involved (Zhang et al., 2009; Parveen and Kumar, 2012). The present study focused on illustrating cause and effect rather than a precise quantification of the process. The accumulations found in the reservoir lake respond to the complete interruption of the sediment yield of the main river course. Although predictable, such a direct link had not been established in any of the local basins and represents a significant contribution in demonstrating empirically what many authors are arguing from a theoretical standpoint when attempting to link beach erosion to river basin sediment yield restrictions brought about by human action (Podolak and Doyle, 2015). This topic, of fundamental importance and not yet studied with the necessary detail, highlights the retention of sediments by dams, which has been the subject of studies, but not with satisfactory rigor and application of innovative methods. Access to secondary information sources that require extensive search and time-consuming networking, such as original civil engineering blueprints for works of some 60 years in the past, shows the importance of the intended rigor of data acquisition.
The relationship between land use and sociodemographic changes provides a necessary context to understand the motivations for the events. Many beach nourishment projects include as the main cause for the need to replenish the cut-off of riverine input, failing to document or even attempting to quantify this cause-effect issue (Malvárez et al., 2003; MTERD, 2022). The high complexity brought about when coastal areas become coupled with human interventions and natural processes, where both elements interact in equal measure at times (Masselink and Lazarus, 2019), has led much research to date to focus on addressing whether socioeconomic development affects shoreline stability. Most researchers agree that the impact of infrastructure development has to be negative on the overall resilience of the natural system to maintain its dynamic equilibrium if the sediment balance is altered artificially. However, the assessment of coastal resilience continues to be the future objective of new research, as proxies such as sediment starvation cannot be categorically assumed to have a quantifiable effect on beach erosion. The main sediment source to Mediterranean littoral systems comes from the hydrographic network (McDowell et al., 1993; Liquete et al., 2004; Syvitski et al., 2005; among others), and considering the behavior of reservoir lakes and dams as lithogenous sediment traps (Willis and Griggs, 2003; Randle et al., 2006; among others), their impact on the terrestrial sediment dynamics would be as expected. Damming the main water course of a river produces a huge reduction in the sediment volume transported downstream and, ultimately, leads to severe coastal erosion. The results obtained in this study demonstrate that over the 2.14 km2 of the reservoir La Concepción, a large volume of sediment has been trapped with positive sedimentation at the various spot heights above 5.6 m. The potential sediment accumulation scenario in the reservoir presented in Figure 5 seems like encouraging results that allow the interpretation that a bypass of sediments could be a management option. Taking the average value to be representative of a distributed sedimentation, a total volume of 11.98 × 106 m3 is estimated to be accumulated over the 53 years that elapsed between 1966 and 2019. This represents a rate of 2.26 × 106 m3 year−1, which is directly missing from the coastal cell. However, given that some sediments would have fed the lower reaches of the river basin, the volume estimated is to be taken as less than that missing in the related coastal cell. The calculated sediment transport, as stated above, is 1.45 × 106 m3 year−1 (MTERD, 2022). The embayment of Marbella, like other similar cells of Costa del Sol, is a unidirectional cell that sources from the west and sinks in the east (Senciales and Malvárez, 2003), which implies that the circulation, when interrupted, induces beach erosion in a constant rate that the natural system cannot replenish unassisted.
The excellence of beaches in terms of width, sea water quality, and available services are the main criteria used by decision-makers for tourist appeal, which became the central activity in the economy of Costa del Sol since the 1950s. However, water management, as well as beach conservation, has been one of the main focuses for managers as key resources for the tourism industry. Although many shoreline stabilization projects have been implemented over the years, decision-makers never succeeded in containing the erosive processes from the 1970s of the twentieth century thus far (Anthony et al., 2014). Attempts toward shoreline stabilization have followed the usual sequence of technology and financial-based investment under pressure from local citizens and the tourism industry (Malvárez, 2012). However, the apparent connection highlighted in research and technical reports has not yielded any attempts to even envisage a sediment management strategy of any sort taking into account river basin dynamics in their artificialized functioning, including reservoir lakes (e.g., full pumped sediment bypass, dredging, flushing, sluicing, or venting).
The exploitation of terrigenous sedimentary deposits retained in reservoir lakes in water management could have been of interest, but, although the Costa del Sol was the object of intense beach nourishment for the stabilization of the coast during the 1990s, the use of river dredging or any sediment redirecting from reservoirs has not been considered. The European Sediment Network (SedNet) has been stating, for nearly two decades since its creation by the European Commission in 2002, the extreme importance of introducing the sediment management paradigm within the river basin management plans of Member States (Brils, 2020). To achieve such a goal, at first instance, it is required that decision-makers perceive the dredged sediments from reservoir lakes as a natural resource susceptible to being reused rather than as waste. However, this approach does not seem to have received the necessary support at the government level thus far. The cause is unknown, although it could be based on economic and environmental issues. SedNet has documented a wide range of successful experiences in Belgium (River Scheldt; Creation of a controlled flood area), the United Kingdom (Wallasea; A wetland creation project), and Sweden (Port of Gavle; Creation of a new port area) concerning the extraction of dredged fluvial and marine sediments for their subsequent reuse as raw materials for construction purposes (Brils, 2020). Other experiences documented in Puerto Rico and China have revealed that these types of actions are commonly very expensive and largely dependent on the lithology and composition of terrigenous sediments (Morris and Fan, 1998). This is especially sensitive in this case study, given the presence of a significant outcrop of peridotites within the studied river basin of the Verde River, partially located on an erosive section of the basin (Figure 7), with high slopes and poor vegetation coverage. Peridotites are very abundant in olivines and pyroxenes, and though not heavy pollutants if naturally transported to the sea, they might eventually host heavy metals that can determine higher natural backgrounds that can be taken as contamination when these materials have to be dredged. This particularity might have inferred the sediments retained in La Concepción had a problematic chemical composition for the subsequent reuse. On the other hand, some research initiatives financed by the European Commission, such as the AGRIPORT Project, succeeded in demonstrating the economic and environmental viability of reusing polluted sediments previously dredged from ports in agriculture (Iannelli et al., 2010; Renella, 2021). SedNet has also demonstrated the successful reuse of polluted sediments in some port engineering operations in the Baltic Sea Region. Spain already has experience in this type of procedure; such is the case of the decontamination of the Flix reservoir in Tarragona in Cataluña, northern Spain, carried out by the AcuaMed company, in cooperation with the Administration General of the State (AcuaMed, 2007).
The Flix reservoir case could be used as a conceptual model but also as a quantitative one since the investment of 190 million euros in decontamination enabled the recovery of a total volume of 1 × 106 m3 of toxic sludge. If we consider that the approximate volume intended for nourishing the beaches of Marbella for 10 years (2006:2016) is 8.4 × 104 m3, it may seem like the plausible solution of bypassing the sediments trapped in La Concepción placing the sediments directly onto the beach or nearshore environments as an economically viable scenario.
Despite the high public investment and environmental limitations, it would be interesting to pursue viability studies that provide greater insight into this approach. It may also be of high interest to assess how the reutilization of terrigenous sediments would fit within the Green Deal and Blue Bioeconomy strategies of the European Commission for conducting shoreline stabilization projects. This is now the reference framework for medium-term environmental policies in Europe (Del Río et al., 2022). Despite this, the instruments for managing the water resources at national and regional scales generally overlook this conceptual paradigm, opting instead for the application of simpler and more direct measures, such as the abandonment of hydraulic infrastructures once they undergo filling (e.g., the Robledo de Chavela reservoir, Madrid in 2014, among others).
The adaptation that is expected from a coastal system in natural conditions under current climate change will not be achieved as sea level rise and increasing erosion due to marine-induced flooding are expected along the coastal strip (Malvárez et al., 2003). There seems to be a critical need to fix the system’s position. However, the protection function of the beach is not preserved and, even if the percentage of recovery is high due to frequent beach restoration projects in the area, the total volume in front of rigid occupied areas will experience a consistent reduction.
A comprehensive framework in which natural conditions, managerial decisions, and resilience assessment can be combined should be the main focus of sediment transport approaches following an integrated method. Coastal water resources of both marine and land-based segments of coastal areas could continue to be utilized in a sustainable way by integrating these concepts.
This study explores the natural and managed settings of Mediterranean coastal systems that may no longer stay in the resilience envelope without engineered support in the form of direct sediment feeding to the marine system. Modeled sediment production and measured sediment deposits in a reservoir lake affecting the main basin in Marbella Bay are used in an attempt to establish the link between sediment starvation and chronic erosion of the Bay’s beaches. The behavior of coastal systems to be expected during the Anthropocene requires that hinterland and marine sediment bodies be studied in conjunction and that strategies to manage and monitor their reduced resilience include a broad and deep analysis of sediment production on slopes, sediment flow within basins, as well as its marine distribution due to coastal currents once the materials have reached the beach environments. None of these seem to have been considered in the scenario analyzed in this study, where ad hoc management structures have dealt with reactive measures to control and distribute water for socioeconomic short-term development and to fix shoreline positions to safeguard coastal structures and promote economic growth. The resilience of the natural system is not fully understood by authorities and, thus, short-term solutions will not accommodate the long-term adaptation required. Even though this study has been conducted at a local scale, it may be assumed that the impact of the outcomes obtained may be representative of a general condition or situation at national or even international scales. This argument is underpinned on the basis that the main drivers of coastal erosion which have been tackled here, namely (i) major land-use changes in the middle and lower reaches of river basins, (ii) dam constructions, and (iii) the widespread development of sea defense infrastructures, are recurrent responses faced by decision-makers to deal with the socioeconomic development of the majority of coastal regions in Spain and many countries worldwide.
The datasets presented in this article are not readily available because Data formats and size not easy to share except if requested. Requests to access the datasets should be directed to Z2NtYWxnYXJAdXBvLmVz.
Sediment circulation in the coastal cell has often been studied, but investigations linking soil loss and river sediment transport and retention at a dam remains a challenge. In this article, sediment yield predictions and quantitative validation of model results is provided for the main reservoir lake of the highly developed Marbella. Sediments accumulated or eroded over 50 years are then linked to the issue of marine circulation as a source-sink pattern from the fluvial to the marine environment. The resilience of the sedimentary continuum river-beach-dune system in the Mediterranean is affected by urban sprawl and water management leading the coastal environment into this situation of artificial resilience. The significant erodibility that we have estimated seems matched by potentially high sediment accumulation rates along selected profiles and spot heights across the bottom of the reservoir lake and, in combination to the marine circulation model, our results identifies that sediment budget key elements in Mediterranean settings such as soil loss, sediment entrapment in reservoirs and the coastal marine circulation is in a state of deficit that suggest the natural resilience envelope is surpassed and the system as a whole is entering a new resilience state in which engineering assistance is key. All authors contributed to the article and approved the submitted version.
This research was funded by the Coastal Environments Research Group (RNM 911 of the Plan Andaluz de Investigación y Desarrollo e Innovación) of the Andalusia Regional Government.
The authors are grateful to the Director of La Concepción Dam, Mr. Agustín Merchán, as well as to the employees of the Regional Ministry of Agriculture, Livestock, Fisheries and Sustainable Development, for their willingness and support during the sampling campaign.
The authors declare that the research was conducted in the absence of any commercial or financial relationships that could be construed as a potential conflict of interest.
All claims expressed in this article are solely those of the authors and do not necessarily represent those of their affiliated organizations, or those of the publisher, the editors and the reviewers. Any product that may be evaluated in this article, or claim that may be made by its manufacturer, is not guaranteed or endorsed by the publisher.
AcuaMed (2007). “Informe de viabilidad de la actuación 4.d,” in Eliminación de la contaminación química del embalse del flix (Tarragona, Spain: Aguas de las Cuencas Mediterráneas S.A)
Adger W. N., Hughes T. P., Folke C., Carpenter S. R., Rockstrom J. (2005). Social-ecological resilience to coastal disasters. Science 309 (5737), 1036–1039. doi: 10.1126/science.1112122
Anderson J. R., Hardy E. E., Roach J. T., Witmer R. E. (1976). A land use and land cover classification system for use with remote sensor data. 2nd ed (Washington D.C: Department of the Interior, Geological Survey). doi: 10.3133/pp964
Anthony E. J., Marriner N., Morhange C. (2014). Human influence and the changing geomorphology of Mediterranean deltas and coasts over the last 6000 years: From progradation to destruction phase? Earth-Sci. Rev. 139, 336–361. doi: 10.1016/j.earscirev.2014.10.003
Berteni F., Dada A., Grossi G. (2021). Application of the MUSLE model and potential effects of climate change in a small alpine catchment in northern Italy. Water 13, 2679. doi: 10.3390/w13192679
Brils J. (2020). Including sediment in European river basin management plans: Twenty years of work by SedNet. J. Soils Sediments 20 (12), 4229–4237. doi: 10.1007/s11368-020-02782-1
CMA (1999). Los Criterios y estándares para declarar un suelo contaminado en andalucía y la metodología y técnicas de toma de muestras y análisis para su investigación (Andalusia, Spain: Junta de Andalucía).
CMA (2005). Mapa de suelos de andalucía a escala 1:400.000 (Andalusia, Spain: Red de Información Ambiental de Andalucía (REDIAM).
Cooper J. A. G., McKenna J. (2008). Working with natural processes: The challenge for coastal protection strategies. Geogr. J. 174 (4), 315–331. doi: 10.1111/j.1475-4959.2008.00302.x
Cooper J.A.G., Jackson D.W.T., Navas F., McKenna J., Malvárez G. (2004). Identifying storm impacts on an embayed, high-energy coastline: examples from western Ireland. Marine Geology 210 (1-4), 261–280. doi: 10.1016/j.margeo.2004.05.012
Del Río J. L., Malvárez G., Navas F. (2020). Reservoir lake effects on eroded littoral systems: The case of the bay of marbella, southern Spain. J. Coast. Res. 95 (SI), 443–447. doi: 10.2112/SI95-086.1
Del Río J. L., Malvárez G., Navas F. (2022). “Impact of legal framework and scientific research in reservoir lake sediment management on coastal erosion and shoreline management in spain's south Mediterranean basins,” in Actas de las XI jornadas de geomorfología litoral Galicia 2022. Eds. Blanco R., Costa M., Gómez A., Cajade D., Fontán A., González R., Bernabéu A., López L. (Galicia, Spain: Servicio de Publicaciones e Intercambio Científico de la Universidad de Santiago de Compostela), 383–387.
Engman E. (1986). Roughness coefficients for routing surface runoff. J. Irrigation Drainage Eng. 112 (1), 39–53. doi: 10.1061/(ASCE)0733-9437(1986)112:1(39
Flood S., Schechtman J. (2014). The rise of resilience: Evolution of a new concept in coastal planning in Ireland and the US. Ocean Coast. Manage. 102, 19–31. doi: 10.1016/j.ocecoaman.2014.08.015
Folke C. (2006). Resilience: The emergence of a perspective for social–ecological systems analyses. Global Environ. Change 16 (3), 253–267. doi: 10.1016/j.gloenvcha.2006.04.002
Francis R. (2013) Analysis of resilience in manmade and natural systems (Report D.1.1 of the Resilience2050.eu FP7 EU project). Available at: https://s3.eu-central-1.amazonaws.com/innaxis-comm/RESILIENCE2050/D1.1+Resilience2050.pdf.
García-Montoro C. (1983). La siderurgia de río Verde y la deforestación de los montes de marbella. Cilniana 5, 10–17.
Giordano A., Navas F., Malvárez G. (2015). Herramienta para medir el cambio en el medio físico aplicado a la ensenada de marbella. Geotemas 15, 181–184.
Guisado-Pintado E., Malvarez G., Ojeda J. (2015). Procesos y dinámica costera en ensenada de marbella: evolución morfosedimentaria reciente. Geotemas 15, 29–32.
Gunderson L. H., Allen C. R., Holling C. S (2012). Foundations of ecological resilience. Island Press.
Gwapedza D., Nyamela N., Hughes D. A., Slaughter A. R., Mantel S. K., van der Waal B. (2020). Prediction of sediment yield of the inxu river catchment (South Africa) using the MUSLE. Int. Soil Water Conserv. Res. 9, 37–48. doi: 10.1016/j.iswcr.2020.10.003
Iannelli R., Bianchi V., Masciandaro G., Ceccanti B., Pretner A. (2010). “Agricultural reuse of polluted dredged sediments: the AGRIPORT European project,” in 12th IWA international conference on wetland systems for water pollution control (Venice: International Water Association).
IUSS-FAO (2014). International soil classification system for naming soils and creating legends for soil maps Vol. 106 (Rome: World Soil Resources Report no).
Kombiadou K., Matias A., Costas S., Carrasco A. R., Plomaritis T. A., Ferreira O. (2020). Barrier island resilience assessment: Applying the ecological principles to geomorphological data. Catena 194, 104755. doi: 10.1016/j.catena.2020.104755
Lario J., Zazo C., Somoza L., Goy J. L., Hoyos M., Silva P. G., et al. (1993). Los Episodios marinos cuaternarios de la costa de málaga (España). Rev. la Sociedad Geol. Española 6 (3-4), 41–46.
Lazarus E. (2017). Toward a global classification of coastal anthromes. Land 6 (1), 1–27. doi: 10.3390/land6010013
Liquete C., Canals M., Arnau P., Urgeles R., Durrieu de Madron X. (2004). The impact of humans on strata formation along Mediterranean margins. Oceanography 17 (4), 70–79. doi: 10.5670/oceanog.2004.05
Madoz P. (1848). Diccionario geográfico-estadístico-histórico de españa y sus posesiones de ultramar (Madrid, Spain: Tomo XI).
Malvárez G. (2012). “The history of shoreline stabilization on the Spanish Costa del sol,” in Pitfalls of shoreline stabilisation: Selected case studies. Eds. Cooper A., Pilkey O. H. (Netherlands: Springer), 235–249. doi: 10.1007/978-94-007-4123-2_14
Malvárez G., Ferreira O., Navas F., Cooper J. A. G., Gracia-Prieto F. J., Talavera L. (2021). Storm impacts on a coupled human-natural coastal system: Resilience of developed coasts. Sci. Total Environ. 768, 144987. doi: 10.1016/j.scitotenv.2021.144987
Malvárez G., Navas F., Guisado-Pintado E., Jackson D. W. (2019). Morphodynamic interactions of continental shelf, beach and dunes: The cabopino dune system in southern Mediterranean Spain. Earth Surface Processes Landforms 44 (8), 1647–1658. doi: 10.1002/esp.4600
Malvárez G., Pollard H. J., Dominguez R. (2003). The planning and practice of coastal zone management in southern Spain. J. Sustain. Tourism 11 (2-3), 204–223. doi: 10.1080/09669580308667203
Malvárez G. (1999). Procesos morfodinámicos litorales en la Costa del Sol. In: Elementos de los paisajes de la provincia de Málaga. Eds Ferre E., Senciales J. M. (Servicio de Publicaciones de la Universidad de Málaga), 171–230.
Masselink G., Lazarus E. D. (2019). Defining coastal resilience. Water 11 (12), 2587. doi: 10.3390/w11122587
McDowell A. J., Carter R. W. G., Pollard H. J. (1993). “The impact of man on the shoreline environment of the Costa del sol, southern Spain,” in Tourism vs. environment: The case for coastal areas. Ed. Wong P. P. (Netherlands: Kluwer Academic Publishers), 189–209. doi: 10.1007/978-94-011-2068-5_13
Merrit W. S., Letcher R. A., Jakeman A. J. (2003). A review of erosion and sediment transport models. Environ. Model. Software 18, 761–799. doi: 10.1016/S1364-8152(03)00078-1
Moreira J. M. (1991). “Capacidad de uso y erosión de suelos,” in Una aproximación a la evaluación de tierras en andalucía (Andalusia, Spain: Agencia de Medio Ambiente).
Morris G. L., Fan J. (1998). “Reservoir sedimentation handbook,” in Design and management of dams, reservoirs and watersheds for sustainable use (New York: McGraw-Hill Book Company).
MTERD (2022). “Proyecto constructivo para la regeneración de playa entre la playa de Venus y punta El ancón, en el término municipal de marbella (Málaga),” in Estudio de impacto ambiental (Andalusia, Spain: Referencia), 29–0404.
Navas F. (1996). “La ocupación urbana de la franja litoral de Newcastle (Irlanda del norte) y sus efectos medioambientales,” in II jornadas de geografía Urbana. Eds. Ramos A., Ponce G., Dávila J. M.(Alicante: Instituto Universitario de Geografía UA), 449–456.
Navas F., Carrero R., Cáceres F. (2012). “A new approach to future scenarios for the EU COASTANCE project,” in A coastal governance experience in Andalusia (Andalusia, Spain: Consejería de Medio Ambiente).
Navas F., Malvarez G. C., Moré G. (2017). Coastal hazard prevention: A methodology for cost-benefit analysis (Seville, Spain: The EcosHAZ project. Universidad Pablo de Olavide, Sevilla).
NRCS (1986). “Urban hydrology for small watersheds,” in Technical report release no. 55 (Washington D.C: United States Department of Agriculture).
Panagos P., Borrelli P., Meusburger K., Alewell C., Lugato E., Montanarella L. (2015a). Estimating the soil erosion cover-management factor at the European scale. Land Use Policy 48, 38–50. doi: 10.1016/j.landusepol.2015.05.021
Panagos P., Borrelli P., Meusburger K., van der Zanden E. H., Poesen J., Alewell C. (2015b). Modelling the effect of support practices (P-factor) on the reduction of soil erosion by water at European scale. Environ. Sci. Policy 51, 23–34. doi: 10.1016/j.envsci.2015.03.012
Panagos P., Meusburger K., Ballabio C., Borrelli P., Alewell C. (2014). Soil erodibility in Europe: A high-resolution dataset based on LUCAS. Sci. Total Environ. 479-480, 189–200. doi: 10.1016/j.scitotenv.2014.02.010
Pandey A., Himanshu S. K., Mishra S. K., Singh V. P. (2016). Physically based soil erosion and sediment yield models revisited. Catena 147, 595–620. doi: 10.1016/j.catena.2016.08.002
Parveen R., Kumar U. (2012). Integrated approach of universal soil loss equation (USLE) and geographical information system (SIG) for soil loss risk assessment in upper south koel basin, jharkhand. J. Geogr. Inf. System 4 (6), 588–596. doi: 10.4236/jgis.2012.46061
Pilkey O. H., Cooper J. A. G. (2004). Society and Sea Level Rise. Science 303 (5665), 1781–82. doi: 10.1126/science.1093515
Podolak C. J., Doyle M. W. (2015). Reservoir sedimentation and storage capacity in the united states: Management needs for the 21st century. J. Hydraulic Eng. 141 (4), 02515001. doi: 10.1061/(ASCE)HY.1943-7900.0000999
Polhill J. G., Gotts N. M. (2009). Ontologies for transparent integrated human-natural system modelling. Landscape Ecol. 24 (9), 1255–1267. doi: 10.1007/s10980-009-9381-5
Quinn J. E., Wood J. M. (2017). Application of a coupled human natural system framework to organize and frame challenges and opportunities for biodiversity conservation on private lands. Ecol. Soc. 22 (1), 39. doi: 10.5751/ES-09132-220139
Rajabi A. M., Yavari A., Cheshomi A. (2022). Sediment yield and soil erosion assessment by using empirical models for shazand watershed, a semi-arid area in center of Iran. Natural Hazards 112, 1685–1704. doi: 10.1007/s11069-022-05244-8
Randle T. J., Yang C. T., Daraio J. (2006). “Erosion and reservoir sedimentation,” in Managing water in the west. erosion and sedimentation manual (Washington DC: Technical Service Center), 2.1–2.94.
Renella G. (2021). Recycling and reuse of sediments in agriculture: Where is the problem? Sustainability 13 (4), 1648. doi: 10.3390/su13041648
Scheffer M., Carpenter S. R. (2003). Catastrophic regime shifts in ecosystems: Linking theory to observation. Trends Ecol. Evol. 18 (12), 648–656. doi: 10.1016/j.tree.2003.09.002
Schwab G. O., Frevert R. K., Edminster T. W., Barnes K. K. (1981). Soil water conservation engineering (New York: John Wiley & Sons).
Senciales J. M., Malvárez G. (2003). La desembocadura del río vélez (provincia de málaga, españa). evolución reciente de un delta de comportamiento mediterráneo. Rev. C. G 17 (1-2), 47–61.
Singh K. P. (1982). “Runoff conditions for converting storm rainfall to runoff with S.C.S curve numbers,” in State water survey contract report no. 288. Illinois (Illinois, USA: Department of Energy and Natural Resources).
Stewart B. A., Woolhiser D. A., Wischmeier W. H., Caro J. H., Frere M. H. (1975). “Control of water pollution from cropland. volume I,” in A manual for guideline development (Washington D.C: Agricultural Research Centre, United States Department of Agriculture).
Syvitski J. P. M., Vorosmarty C. J., Kettner A. J., Green P. (2005). Impact of humans on the flux of terrestrial sediment to the global coastal ocean. Science 308 (5720), 376–380. doi: 10.1126/science.1109454
Vieux B. E. (2004). Distributed hydrologic modeling using GIS (Netherlands: Springer Netherlands). doi: 10.1007/978-94-024-0930-7
Williams J. R. (1975). “Sediment-yield predictions with universal equation using runoff energy factor,” in Present and prospective technology for predicting sediment yields and sources (New Orleans: Agricultural Research Service), 244–252.
Willis C. M., Griggs G. B. (2003). Reductions in fluvial sediment discharge by coastal dams in California and implications for beach sustainability. J. Geol. 111 (2), 167–182. doi: 10.1086/345922
Wischmeier W. H., Smith D. D. (1960). “A universal soil-loss equation to guide conservation farm planning,” in Transactions of the 7th international congress of soil science (Wisconsin, USA: Elsevier Publishing for International Society of Soil Science), 418–425.
Yousuf A., Bhardwaj A., Yousuf F. (2022). Development and evaluation of GIS-based runoff and sediment yield watershed scale model. Arabian J. Geosci. 15, 1570. doi: 10.1007/s12517-022-10863-3
Keywords: sediment retention in reservoirs, beach sediment balance, Marbella beach, sediment management, water management, Costa del Sol (Spain)
Citation: Malvarez G, Navas F and del Rio JL (2022) Assessment and projections of sediment budget resilience in Marbella, Spain. Front. Mar. Sci. 9:933994. doi: 10.3389/fmars.2022.933994
Received: 01 May 2022; Accepted: 24 October 2022;
Published: 23 November 2022.
Edited by:
Achilleas G. Samaras, Democritus University of Thrace, GreeceReviewed by:
Carlos Da Silva, Universidade NOVA de Lisboa, PortugalCopyright © 2022 Malvarez, Navas and del Rio. This is an open-access article distributed under the terms of the Creative Commons Attribution License (CC BY). The use, distribution or reproduction in other forums is permitted, provided the original author(s) and the copyright owner(s) are credited and that the original publication in this journal is cited, in accordance with accepted academic practice. No use, distribution or reproduction is permitted which does not comply with these terms.
*Correspondence: Gonzalo Malvarez, Z2NtYWxnYXJAdXBvLmVz
†These authors have contributed equally to this work and share first authorship
Disclaimer: All claims expressed in this article are solely those of the authors and do not necessarily represent those of their affiliated organizations, or those of the publisher, the editors and the reviewers. Any product that may be evaluated in this article or claim that may be made by its manufacturer is not guaranteed or endorsed by the publisher.
Research integrity at Frontiers
Learn more about the work of our research integrity team to safeguard the quality of each article we publish.