- 1Instituto de Investigaciones Oceanológicas, Universidad Autónoma de Baja California, Ensenada, Mexico
- 2Department of Biological Oceanography, CICESE, Centro de Investigación Científica y de Educación Superior de Ensenada, Ensenada, Baja California, Mexico
- 3Department of Ecology & Evolutionary Biology, University of Connecticut, Stamford, CT, United States
Eisenia arborea (sensu Ecklonia arborea) is the kelp with the greatest latitudinal distribution on the Northeastern Pacific Coast. It is harvested in Mexico in small amounts for abalone farm fodder and occasionally exported to Asia for human consumption. Because the high-energy environment where it naturally grows limits its sustainable harvest, we explored the domestication and cultivation of this kelp on the west coast of Baja California, Mexico. The life history of E. arborea was completed in the laboratory following traditional methods for kelp cultivation. Gametophytes became reproductive approximately 50 days after spore release and sporophytes were visible to the naked eye after 80 days. When sporophytes reached 2–3 cm, they were transplanted to two sites on long-line systems: in an open bay (Todos Santos Bay) and in a shallow coastal lagoon (San Quintín Bay). At both sites, full differentiation occurred 6 to 8 months after deployment, with fertile blades occurring within a year. Once individuals reached maximum size, blades were harvested by pruning. Monthly, regrowth of pruned blades and new blade addition were measured. Pruned individuals reached the same weight as controls in three months. On the basis of these results, a seasonal seeding program was implemented at a third site where the presence of natural Eisenia is rare due to the lack of hard substrate but was hypothesized to provide appropriate conditions for kelp cultivation (Santa María Bay). Cultures were deployed at three different depths and growth was correlated with season, depth, and temperature. Only cultures seeded in winter developed successfully at this site. This study shows that the culture of E. arborea in a variety of ocean conditions is possible; however, site selection and seasonality are important considerations. Because E. arborea is perennial, it can be harvested periodically during the year thereby increasing yield, and because it is the warmest tolerant kelp species, it is an ideal target for ocean farming and commercial cultivation, considering climate change.
Introduction
The production of macroalgae through aquaculture has increased 7.2% in the last decade. In 2019, seaweed aquaculture production reached 34.7 million tons with a value of US $14.8 billion compared to 1.1 million tons of harvest from natural beds (FAO, 2021). The interest in macroalgae, traditionally focused on human consumption and the production of phycocolloids, has diversified to a wide variety of potential markets; from the production of pharmaceuticals and nutraceuticals (Hafting et al., 2015) to their potential use to produce extracts for agriculture (Craigie et al., 2008), bioplastics (Zanchetta et al., 2021), biofuels (Rocca et al., 2015), and carbon capture (Chung et al., 2011). The exponential growth of abalone cultivation in Asia in the last two decades has also increased the demand for cultivated macroalgae (Huang et al., 2022). In addition, although the cultivation of macroalgae is a well-established commercial activity, it is generally limited to Asian countries and to a very small number of species.
On the other hand, the farming of marine species is becoming increasingly vulnerable to the effects of climate change, with urgent calls for research on the diversification of species that can survive under new climate regimes. On the eastern temperate Pacific coast of North America, kelp forests, dominated by the giant kelp Macrocystis pyrifera, have been severely affected by climate change and tropicalization (Pfister et al., 2017). The southern distribution of M. pyrifera has suffered a reduction of approximately 500 km in the last four decades (Ladah et al., 1999). However, Eisenia arborea, a kelp that along with M. pyrifera and Pterygophora californica, makes up the kelp forests of the temperate coast of North America; has maintained its distribution from Vancouver, Canada, to Magdalena Bay, Mexico; and has prevailed in warmer areas and periods where M. pyrifera has disappeared (Hernández-Carmona et al., 2000; Gossard and Stellar, 2022), motivating the present study exploring its domestication and farming.
Eisenia arborea J. E. (Areschoug, 1876) is a commonly occurring kelp of the Eastern Pacific Coast of North America, ranging from Vancouver Island in British Columbia, Canada, to Magdalena Bay in the southern region of the Baja California Peninsula, Mexico (Abbott and Hollenberg, 1976; Gossard and Stellar, 2022). It shows a greater depth and latitudinal range than other understory kelps such as Pterygophora and Laminaria. In southern California, E. arborea can be found from the intertidal to 40 m deep (Dayton et al., 1999; Spalding et al., 2003) as part of the kelp forest community in open waters exposed to relatively high wave energy. It has a life history typical of the Laminariales group, with two alternating heteromorphic phases, a perennial diploid macroscopic and microscopic sporophyte, and a microscopic haploid gametophytic phase, originally described by Shults and Herbst (1938) and Hollenberg (1939) from samples collected in Southern California, USA. It is a stipitate kelp that attaches to the benthos by a branched haptera, from which an elongating rigid stipe extends vertically, terminating in a dichotomous bifurcation producing numerous blades. Meiosis occurs in the blades, where sori appear often visible to the naked eye (Abbott and Hollenberg, 1976).
Matson and Edwards (2007) reported that Eisenia arborea can reproduce and produce sporophytes under relatively warm temperatures, potentially making this species less vulnerable to extreme climatic events such as El Niño. Eisenia extends its southern distribution in the northern hemisphere beyond other kelps such as Pterygophora californica and Macrocystis pyrifera. Studies by Sánchez-Barredo et al. (2011) showed that E. arborea can survive longer than other kelps under low nutrient conditions and relatively high temperatures, potentially permitting E. arborea to persist at more southern latitudes in the northern hemisphere than other temperate kelps such as M. pyrifera, Pterygophora californica, and Laminaria spp (Ladah et al., 1999; Hernández-Carmona et al., 2000; Hernández-Carmona et al., 2001; Gossard and Stellar, 2022).
The chemical composition of Eisenia arborea has been shown to vary seasonally near its southern limit in Mexico, with higher carbohydrate and protein content in blades compared to stipes, but with higher ash in stipes (Landa-Cansigno et al., 2017; Serviere-Zaragoza et al., 2002), with carbohydrates being higher in September, whereas higher content of fucoidan coincides with the period of maximum reproduction (July to November). In a study exploring pharmaceutical properties to control type 2 diabetes, E. arborea from Mexico was among the seaweeds with the highest antioxidant activity, with bioactive compounds including phlorotannins and sulfated polysaccharides (fucoidan, laminarin, and alginate) (Múzquiz de la Garza et al., 2019). A similar kelp, E. bicyclis Kjellm, commonly known as “Arame”, is harvested and sold for human consumption in Asia where it is also recognized for its alginate, mannitol, iodine, and fucoidan content (Notoya and Aruga, 1990). It also shows seasonal variability in its chemical composition as well as among different parts of the individual (Arvizu et al., 2007).
In Mexico, the commercial harvest of Eisenia for its numerous uses is limited by the exposed conditions where the populations occur naturally. Harvesting is generally restricted to the collection of drift and beach cast due to high wave action or strong currents. At the same time, the increase in cultivation of red abalone (Haliotis rufescenes) in California and along the Baja California peninsula, which traditionally relied on the availability of currently dwindling Macrocystis pyrifera populations (Smale, 2020) for food, has sparked interest in E. arborea as studies conducted in Mexico show that it is also an adecuate food source (Zertuche-González et al., 2013). In addition, because of the general hardiness of Eisenia, kelp forest restoration of another similar species, Eisenia bicyclis, has been successfully performed in Japan (Terawaki et al., 2000), suggesting that domestication and farming of Eisenia may be of commercial interest and be plausible in Mexico as well.
One of the few wave-protected sites where Eisenia arborea occurs in Mexico is in San Quintín Bay (SQB), which is a shallow coastal lagoon off the Pacific coast of Baja California (Aguilar-Rosas et al., 2005). SQB is a highly productive lagoon due to the influence of coastal upwelling (Álvarez Borrego, 2004), where most of the commercial oyster cultivation in the region occurs (Aguirre-Muñoz et al., 2001). Small patches of E. arborea occur not only on the limited hard natural substrate of the lagoon but also attached to oyster culture racks. This observation provides the opportunity to consider the potential cultivation of this species in wave-protected areas such as coastal lagoons or protected bays, with high-nutrient input but limited hard substrate.
The purpose of this study was to define the laboratory and field conditions for the cultivation of Eisenia arborea from the spores generated in the laboratory to adult sporophyte development in different marine environments. The study explored the appropriate conditions for the production of microscopic sporophytes in the laboratory and for their deployment at three different field sites on the temperate coast of the Baja California peninsula. Growth and harvesting effects from pruning were also evaluated. To our knowledge, this is the first study to explore the domestication of E. arborea.
Materials and methods
This study was conducted in three phases. The first phase defined an effective protocol to produce seedlings in the laboratory. The second phase evaluated the growth and development of Eisenia arborea at two contrasting sites along the coast of Baja California. The third phase explored seasonal cultivation seeding trials in a productive bay with very little natural kelp presence due to its sandy bottom, which might provide a valuable area for mariculture development.
Site description
Three sites were selected for the deployment of Eisenia arborea cultures: Todos Santos Bay (TSB), SQB, and Santa María Bay (SMB) (Supplementary Figure 1) in Northern Baja California, with all sites along this shore showing strong current flows. TSB is a relatively protected bay, located 100 km south of the US–Mexico border on the Pacific coast, with oceanic and upwelling influence, with E. arborea commonly found attached to hard substrate. SQB is a shallow coastal lagoon, situated 200 km south of TSB bay with strong tidal currents, relatively high nutrients due to upwelling influence, with fine sand and mud bottom and limited hard substrate (Aguirre-Muñoz et al., 2001; Álvarez Borrego, 2004). E. arborea grows in shallow areas where hard substrate occurs and is attached to oyster racks. SMB, just south of SQB, is an open bay exposed to south swells with strong upwelling influence with a mostly sand bottom and no conspicuous presence of E. arborea due to lack of hard substrate; however, it shows high potential for mariculture activities due to its oceanographic conditions such as the influence of upwelled waters, no industrial or urban discharges, and greater depth ranges than BSQ. In the first two sites, field culture and pruning methods were initially developed and tested, and in the third site, the seasonality of culture initiation was tested to develop an effective domestication calendar.
Temperature and PAR irradiance were measured at each site by submersible sensors (Onset-Hobo, Pendant temp/light UA-002-08); these sensors were previously calibrated against a LI-COR (LI-190R) quantum sensor for the conversion of lux units to µmol photon m−2 s−1 following Long et al. (2012). Light data were used to select equivalent depths for cultivation at different sites.
Life history, culture rope seeding, and harvesting by pruning
The life history of E. arborea was completed in the laboratory following traditional methods for kelp cultivation (Tseng, 1987; Merrill and Gillingham, 1991; Kawashima, 1993; Flavin et al., 2013; Redmond et al., 2014). Zoospores were obtained from mature fertile sporophylls (with sori) collected from individuals naturally growing in SQB, where reproductive individuals are available all year long, providing a reliable spore source. Reproductive blades with sori were wrapped in a paper towel and transported to the laboratory in a cooler. That same day (5 to 8 h post collection), sori were cut from the blade, brushed, and washed several times with filtered (1 µm) and UV-irradiated seawater. Sori from approximately seven blades were placed into 1-L Erlenmeyer flasks of filtered and UV-irradiated seawater and then placed in the dark at room temperature (around 20°C). After 1 h, spore release was confirmed under the microscope, and the number of spores was enumerated with a hematocytometer.
Nylon string (1 mm diameter) was previously wrapped around 10-cm-long pieces of PVC pipe (1.5 inch diameter), with 5 m of string on each pipe piece. Twenty-five pieces of pipe were placed in plastic sinks with 30 L of filtered and UV-irradiated seawater, and the spore solution was added at a density of 1,000 spores ml−1. Cultures were maintained in the dark at 16°C with no aeration. After 24 h, moderated aeration was added, and photoperiod was set to 12:12 light:dark (55 ± 5 μE m−2 s−1). One-week later, culture medium was replaced by enriched seawater with modified Provasoli media and replaced weekly. After 30 days under culture, light was increased (100 ± 5 μE m−2 s−1) to stimulate growth (Figure 1).
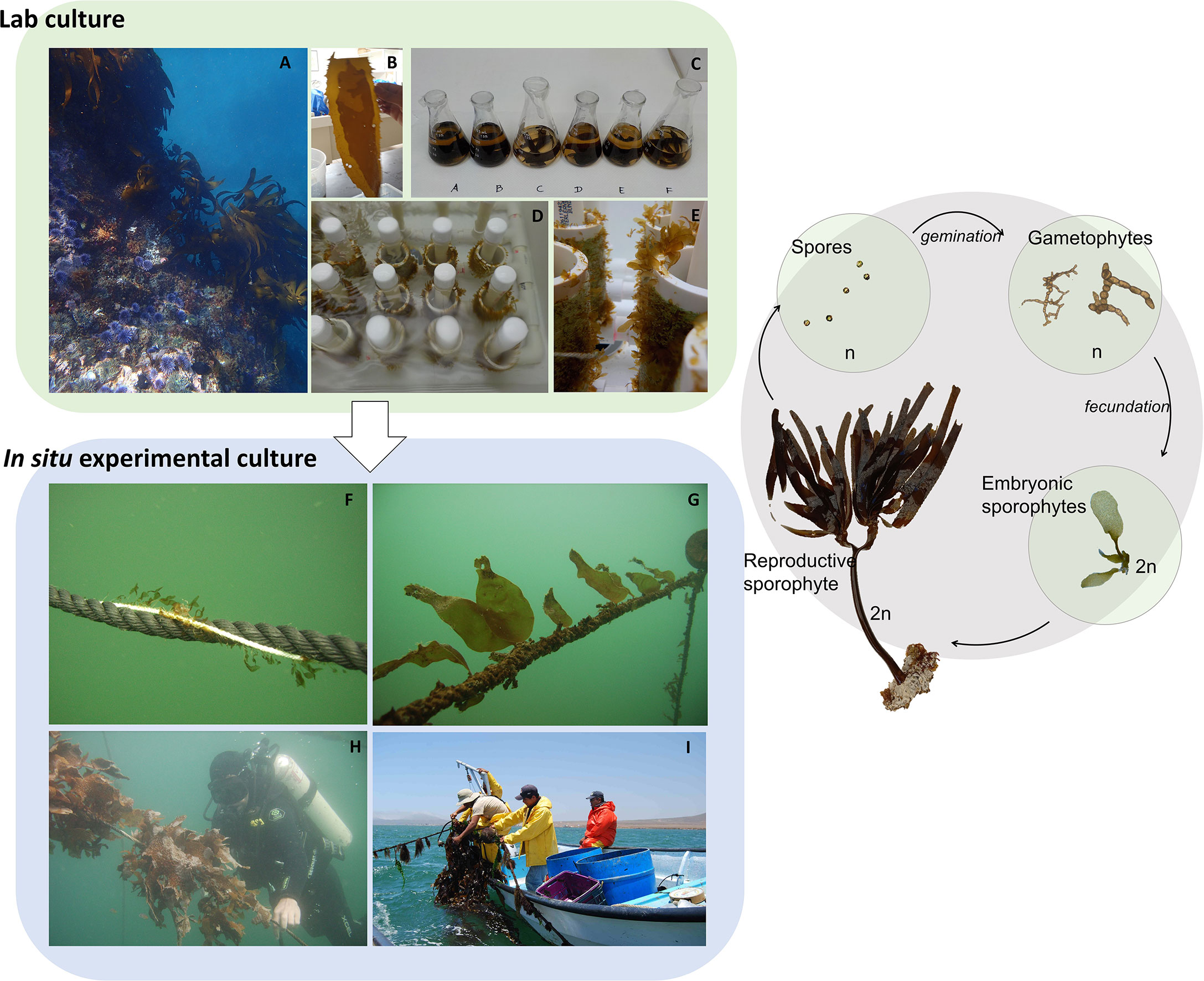
Figure 1 On the left: Pictures showing the different stages of Eisenia arborea culture. In natural populations of E. arborea (A), reproductive blades with sori (B), were collected from mature sporophytes. In the laboratory, sori were induced for spore release (C). Spores were germinated on nylon strings wrapped around PVC tubes placed in tanks filled with seawater. Embryonic sporophytes growing on nylon strings (D, E) were transferred to in situ cultures, wrapping them around polypropylene ropes. Juvenile sporophytes (G) grew over these polypropylene ropes until maturity (H). Harvesting and sampling of plants performed by scuba diving (I). On the right: Schematic representation of the life history of Eisenia arborea.
Eisenia growth at Todos Santos Bay and San Quintín Bay
Field growth experiments were initially tested in TSB and BSQ using spores obtained from the latter (see above). From these experiments, seasonal growth and pruning effects were recorded.
Once sporophytes reached 1–2 cm in length, cultures were moved to outdoor 1 m3 tanks under natural light conditions. Tanks were shaded to 30% of incident light (to match similar field conditions), and temperature was controlled to 16°C–18°C. When individuals reached 6–10 cm in length and a holdfast with haptera had clearly developed, the strings were removed from the PVC pipe pieces and were wrapped around 1-cm polypropylene ropes that were then moved to TSB, attached to a long line installed at 7 m depth, 1 m above the bottom.
After 22 weeks, ropes were densely covered with plants 15 to 60 cm long with development scores of “a” and “b” according to the Arnold, 1980 classification. Eighty plants of approximately 40 cm long were selected and manually attached to 8-mm-thick polypropylene rope. Forty plants remained at TSB, and the rest were moved to SQB, deployed on a long line near the surface (1 m depth), under similar irradiance conditions, to compare growth at two sites with different oceanographic conditions.
Plant wet weight, number of blades, stipe length, and maximum blade length were measured monthly. Specific daily growth rate was calculated monthly using the equation SPG = (Ln Bf − Ln Bo)/t, where Bf is the final biomass, Bo is the initial biomass, and t is the time in days (note that the negative growth rates can occur as mean tissue loss rather than gain over time period, t).
Harvesting by pruning
To evaluate regeneration capacity after harvesting, after 8 months of deployment (when individuals reached maximum biomass), adults from each site were tagged and weighed, and then half were pruned by cutting the blades 5 cm above the base. Pruned biomass was weighed, and biomass recovery was evaluated monthly.
Culture density
To define optimum density for growth on the culture rope at BSQ, individuals from an F2 culture, with similar length (47 cm average) and weight (80 g average), were collected and re-seeded on ropes at 10-, 20-, and 30-cm distance between each individual (equivalent to 3.3, 5, and 10 sporophytes per meter). Two 5-m ropes for each density treatment were tested, and 30 sporophytes of similar size and weight were tagged and re-attached on the original rope as well.
Validation of a semi-open oceanic site, Santa María Bay
Once the basic protocols for the domestication of Eisenia arborea in this region were defined, and a reference for growth in coastal protected areas was established, a protocol for a potentially commercial site in semi-exposed waters was tested. Four seeding initiation exercises (one for every season of the year) were conducted at three different depths. Growth was measured and correlated with in situ temperature and light.
The seeding initiation experiment for the experimental farm was developed in SMB (30° 22′ 43.62″ N, 115° 56′ 01.61″ W; south of SQB), located on the Pacific coast of Baja California, Mexico (see Supplementary Figure 1). Depth at the experimental site was 16 m with a substrate of primarily coarse to fine sands. Because of its geographic location, the culture site was protected from northwestern swells associated with winter storms but exposed to summer southern swells.
Eisenia arborea was cultivated on a long-line system in different seasons at three different depth ranges (2–4, 7–9, and 12–14 m). The design consisted of vertical and horizontal culture ropes (each rope composed by three strands of 6-mm-thick polypropylene rope) with buoys, anchored to the sandy substrate by stainless-steel tubes. The vertical cultures consisted of six primary ropes (84 m) with 10 m available for effective cultivation. A total of 342 m of culture rope with juvenile sporophytes was seeded at densities ranging from 856 to 2,400 juveniles per m.
At each depth, temperature was continuously monitored by submersible sensors (Onset HOBO TidbiT v2 Water Temperature Data Logger). Water column profiles of irradiance were taken using a spherical (4π) quantum sensor LICOR LI-193, whereas salinity was measured with a multiparameter probe (YSI Professional).
Sampling of biological variables
Sporophyte size (i.e., full length from the haptera/holdfast to the tip of the longest blade) of 30 juveniles from two culture ropes per depth was measured in situ by scuba diving every one to two months.
Destructive sampling was conducted in July and November 2016, consisting of recovering two culture ropes, each rope containing sporophytes growing at the three different depth ranges. For each rope, total sporophytes were counted every meter to obtain culture density (sporophytes m−1). Culture ropes were also weighed by using a digital balance (Mustad, ± 10 g) every meter to calculate culture biomass (kg FW m−1), with the weights of the culture rope and epibionts subtracted. Epibiont fresh weight (expressed as the percentage of the total sporophyte weight) was measured by gently scraping ropes and sporophytes with sponges and weighing removed material. In addition, for each culture rope, the length of randomly selected sporophytes was measured and weighed to the nearest 0.1 g (Ohaus SP2001 AM, ± 0.1 g). The ontogenetic stage of these sporophytes was scored following the criteria of Arnold (1980), i.e., (A) main frond with pointed apical termination; (B) degradation and loss of the apical part (truncate), growth of the stipe, and change in appearance in the basal part from smooth to serrated edge; (C) growth of secondary fronds in the form of lobe on the edges of the main frond; (D) evident degradation of main frond and development of secondary fronds; and (E) maximum degree of development, total loss of the main frond, bifurcation, and development of secondary fronds, as well as the stipe.
Statistical analyses
One-way ANOVAs were applied to explore statistical differences in fresh weight and specific growth rates of harvested and not harvested sporophytes during their cultivation in two sites (SQB and TSB) (Figures 2, 3; Supplementary Tables 1, 2) as well as to test for the effect of depth on the biological descriptors of cultivated E. arborea (Figure 5) at each destructive sampling time (July and November 2016), after testing for normality and homoscedasticity (see Supplementary Table 3). Post hoc mean comparisons for the ANOVAs (Student–Newman–Keuls test) were performed to identify specific treatment level(s) causing significant effects. Statistical analyses were performed using STATISTICA software (StatSoft Inc.) with a minimum significance level established at P < 0.05.
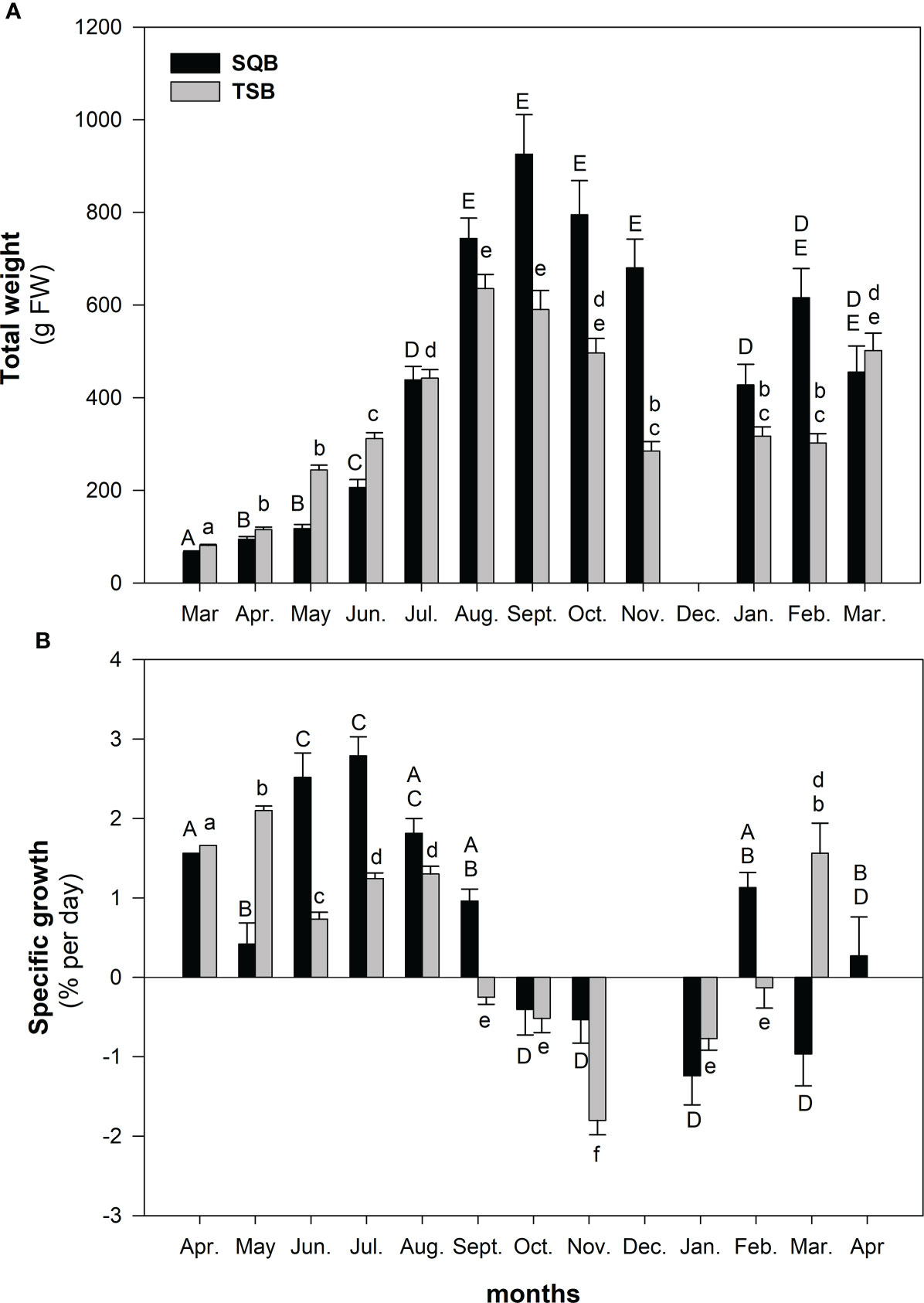
Figure 2 Changes in (A) weight (g FW) and (B) specific growth rate (% per day) of Eisenia arborea sporophytes cultured in San Quintı́n Bay (SQB) and Todos Santos Bay (TSB). Different uppercase and lowercase letters indicate statistically significant differences (one-way ANOVA, post hoc Student Newman Keuls test) obtained at different sampling times during cultivation. Values are means and standard errors.
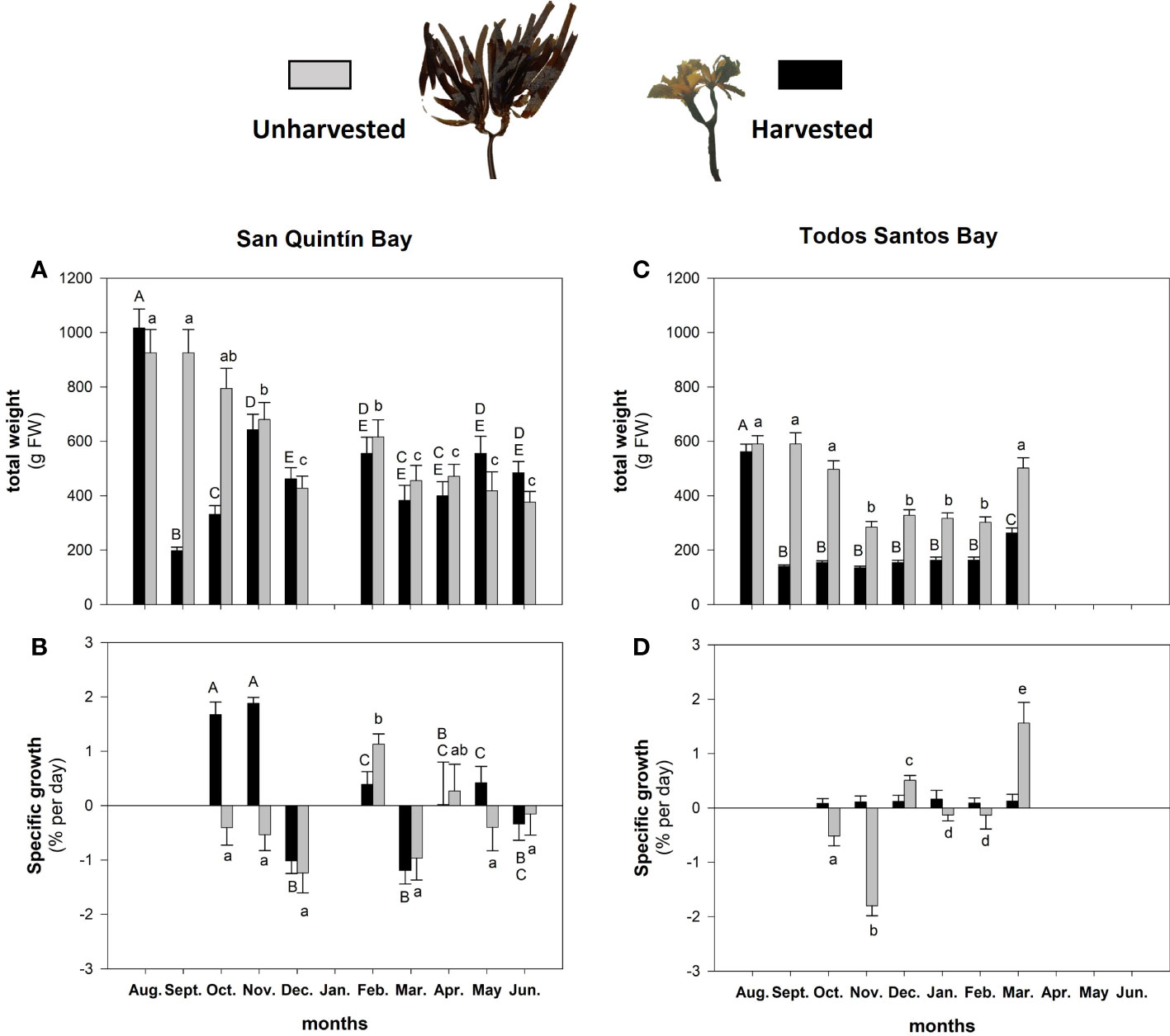
Figure 3 (A, C) Changes in weight (g FW), and (B, D) specific growth rate (% per day), of sporophytes that were harvested by pruning versus those not harvested of Eisenia arborea cultured in San Quintin Bay (SQB) and Todos Santos Bay (TSB). Different uppercase and lowercase letters indicate statistically significant differences (one-way ANOVA, post hoc Student Newman Keuls test) obtained at different sampling times during cultivation. Values are means and standard errors.
Results
The daily light dose (expressed as mol photon m−2 s−1) was similar between culture sites (SQB at 1–1.5 m depth and TSB, 4–6 m depth). Lower values were measured in winter (December to February), being 3.4 ± 0.6 and 3.9 ± 0.4 for SQB and TSB, respectively. In late spring/summer (July to August), maximum values of 18.3 ± 1.3 and 20.2 ± 0.2 were observed for SQB and TSB. Temperature, on the other hand, was notably different between sites in summer, with mean values of 23.2 ± 0.1°C and 17.3 ± 0.8°C measured in SQB and TSB, respectively. Average winter temperatures, however, were similar at 15.4 ± 0.1°C in SQB and 15.1 ± 2°C in TSB.
Life history and culture rope seeding and harvesting
It was common to see biflagellate spores in motion as soon as 1 h after placing the sori in culture media. However, sometimes it would take the sori several hours, at times overnight, to release spores. Once the spores were released, the first cell division was observed, with the differences between thicker female cells and thinner male cells typically evident by day seven. Gametophytes became reproductive approximately 50 days after spore release. Sporophytes were visible after 80 days when plants reached 2–3 cm. At both sites, plants fully differentiated in about 6 to 8 months after deployment and became reproductive within a year.
Eisenia growth at Todos Santos Bay and San Quintín Bay
Deployed cultures grew and developed to adult stages at both sites and reached maximum biomass in summer (Figure 2A). TSB cultures showed net positive growth from April to August with maximum growth rates in May (2.1% day−1). SQB cultures showed net positive growth from April to September (when maximum biomass was reached) with maximum growth rates in July (2.8% day−1) (Figure 2B, P < 0.001, Supplementary Table 1). On average, the SQB biomass yield was 45% higher than for TSB.
The number of blades per individual increased at both sites from early spring to summer. In late spring and early summer, the number of blades was higher in TSB. However, in summer, SQB individuals added blades faster than those in TSB, reaching a maximum average of 81 blades per individual in September. In contrast, the number of blades in TSB decreased to an average of 49 blades per individual at the same time (Table 1). Blade length, however, was larger in TSB during the period of net positive growth (March to September). In early fall (October), the blade length at TSB decreased sharply due to blade erosion, whereas blades in SQB were better preserved. Stipe length at SQB did not change during the study period; however, stipe length increased from April to July in TSB and did not change significantly thereafter.
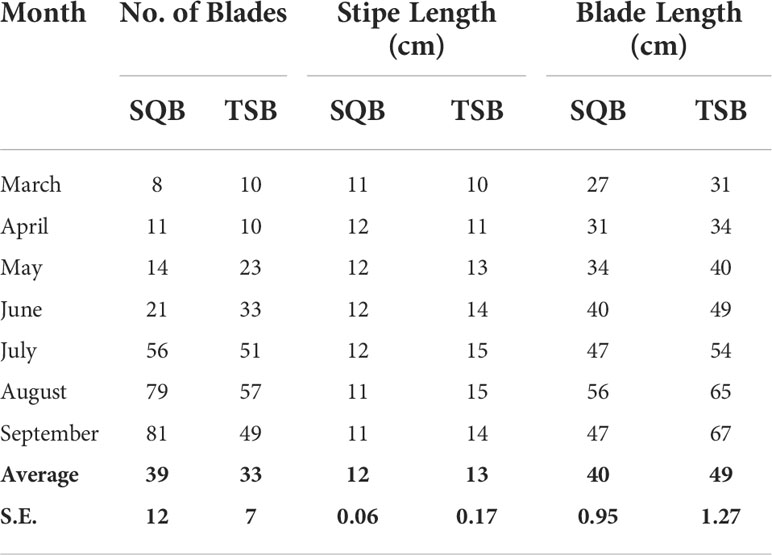
Table 1 Number of blades, stipe length (cm), and blade length (cm) during the growing phase (March to September) of Eisenia arborea cultured from Todos Santos Bay and San Quintín Bay.
Effect of harvesting by pruning
In SQB, the total weight of individuals decreased gradually from August to January (Figure 3A; F = 19.81, P < 0.001; Supplementary Table 2). After harvestings, individuals increased their weight (Figure 3A; F = 28.36, P < 0.001; Supplementary Table 2), and they reached the same weight as those that were not harvested after three months at SQB. At this time, the average harvested biomass was 830 g FW per individual, which was almost 50% more than for TSB individuals (421 g FW, Figure 3C). Growth occurred by the generation of new blades rather than by regrowing pruned blades. From December to May, both treatments maintained similar biomass (Figures 3A, B). Harvested individuals from TSB (Figures 3C, D) never reached the weight of unharvested plants (Figure 3C; F = 178.7, P < 0.001; Supplementary Table 2). In TSB, unharvested sporophytes showed a biomass reduction pattern similar to those cultivated in SQB (Figure 3C; F = 85.31, P < 0.001; Supplementary Table 2).
Culture density effects
No significant effect of culture density on weight, total length, stipe length, number of blades or on the degree of development was detected (Table 2). However, there was a significant difference in reproductive condition. For the lower density culture (3.3 individuals per m), 47% of the individuals were reproductive, whereas at higher densities (5 and 10 individuals per m), only 23% and 9% were reproductive, respectively.
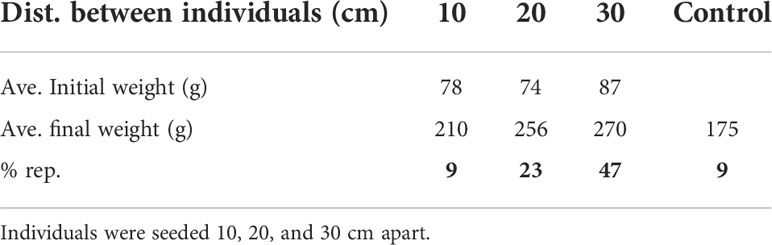
Table 2 Increase in weight of Eisenia arborea individuals cultured from September 2013 to April 2014 in San Quintín Bay.
Validation of a semi-open oceanic site
The highest temperatures (~24°C) were recorded at the end of summer/beginning of autumn in 2015 (Figure 4A). From August to September 2015 through winter 2016, average temperature decreased gradually from ~22°C to ~14°C. Maximum daily variability of temperature (± 3°C) was observed between June and September 2016. The highest temperatures during 2016 (~22.5°C) were recorded between July and August.
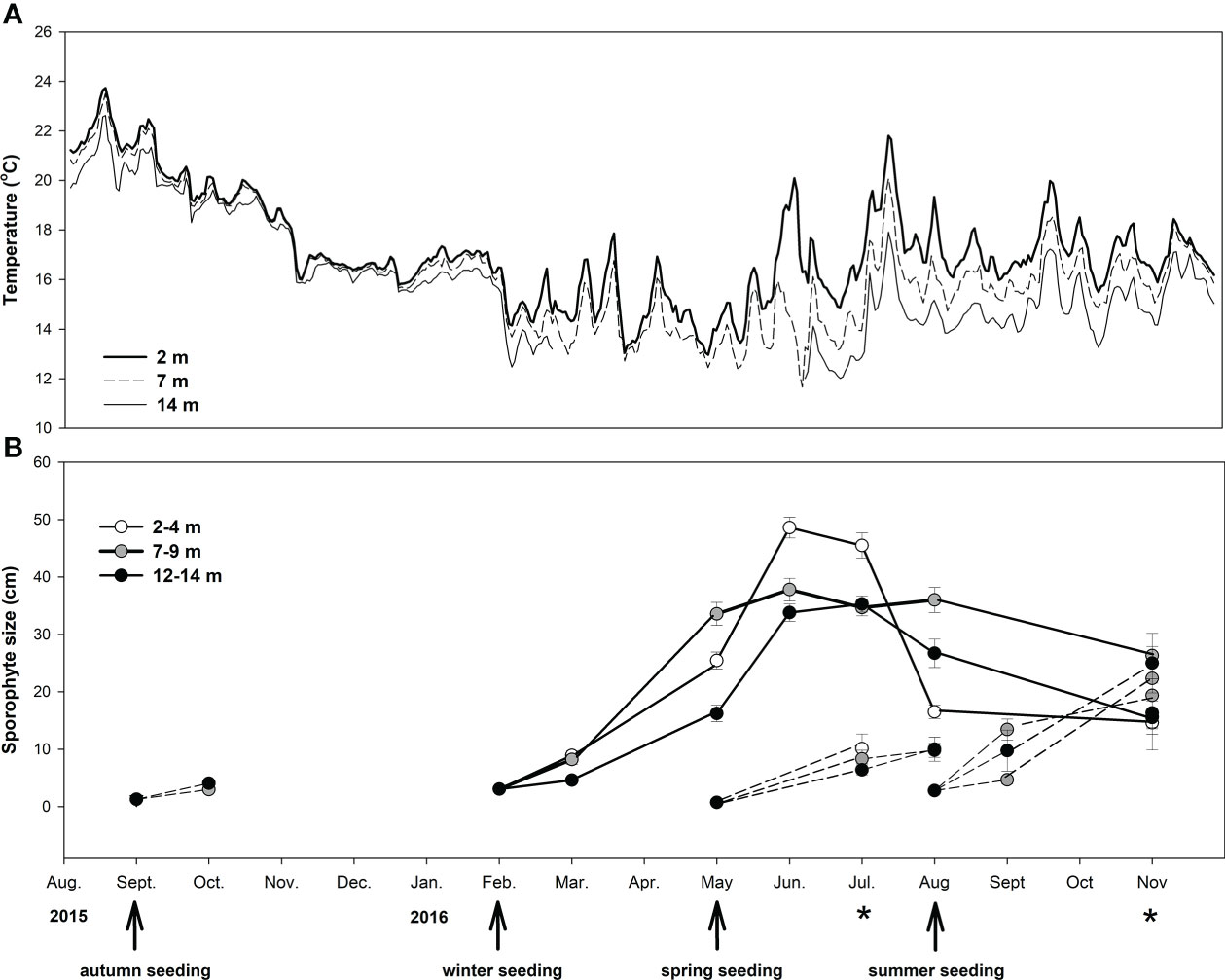
Figure 4 (A) Seawater temperature and (B) change in sporophyte size (i.e., total length, cm) measured during non-destructive sampling throughout the different experimental culture periods. Eisenia arborea was cultivated at different depths (2–4, 7–9, and 12–14 m). Arrows on the x-axis indicate the initial seeding during different seasons, whereas asterisks indicate the dates of destructive sampling for the successful culture initiated in winter. Values of sporophyte size are means and standard errors.
Cultures corresponding to seeding in autumn, spring, and summer failed after 2–3 months, and maximum sporophyte size occurred in summer (Figure 4B). Sporophyte size in winter cultures reached maximum values in June to July, and higher values (~50 cm) were found at the shallow depth treatment (2–4 m) compared to those in deeper waters (35–40 cm). In August, sporophyte size decayed abruptly in shallow sporophytes, from 50 to 15 cm, whereas the size of those growing deeper remained more stable (25–35 cm).
In July, the lowest biomass values (Figure 5A) were found in the deeper treatment (12–14 m) with a near significant trend, whereas sporophytes growing at the shallower treatments (2–4 and 7–9 m depth) showed similar biomass (F = 3.14, P = 0.051; Supplementary Table 3). In November, biomass was reduced (by 67%–80%) compared to that measured in July, and higher values corresponded to sporophytes at the 7- to 9-m-depth treatment (F = 3.55, P = 0.035; Supplementary Table 3). Density (Figure 5B) showed a similar pattern to biomass and was notably reduced in November compared to July, when maximum values were found at 7–9 m depth (F = 5.352, P = 0.007; Supplementary Table 3). Percentage weight of epibionts (Figure 5C) was similar (20%–30%) for both sampling times and depths, except for a significant increase at mid-depth in July (~60%, F = 23.425, P < 0.001; Supplementary Table 3). In July, sporophyte weight (Figure 5D) gradually reduced with depth, from 60 to 20 cm (F = 12.283, P < 0.001; Supplementary Table 3), whereas in November, values notably dropped (from 60 to 15 cm) at shallow depths and the highest values corresponded to the 7- to 9-m treatment. Blade length (Figure 5E) showed a similar pattern to weight. In July, stipe length (Figure 5F) was higher at shallow depths (F = 12.806, P < 0.001; Supplementary Table 3), whereas the lowest values were found in the deepest treatment in November (F = 4.586, P < 0.013; Supplementary Table 3).
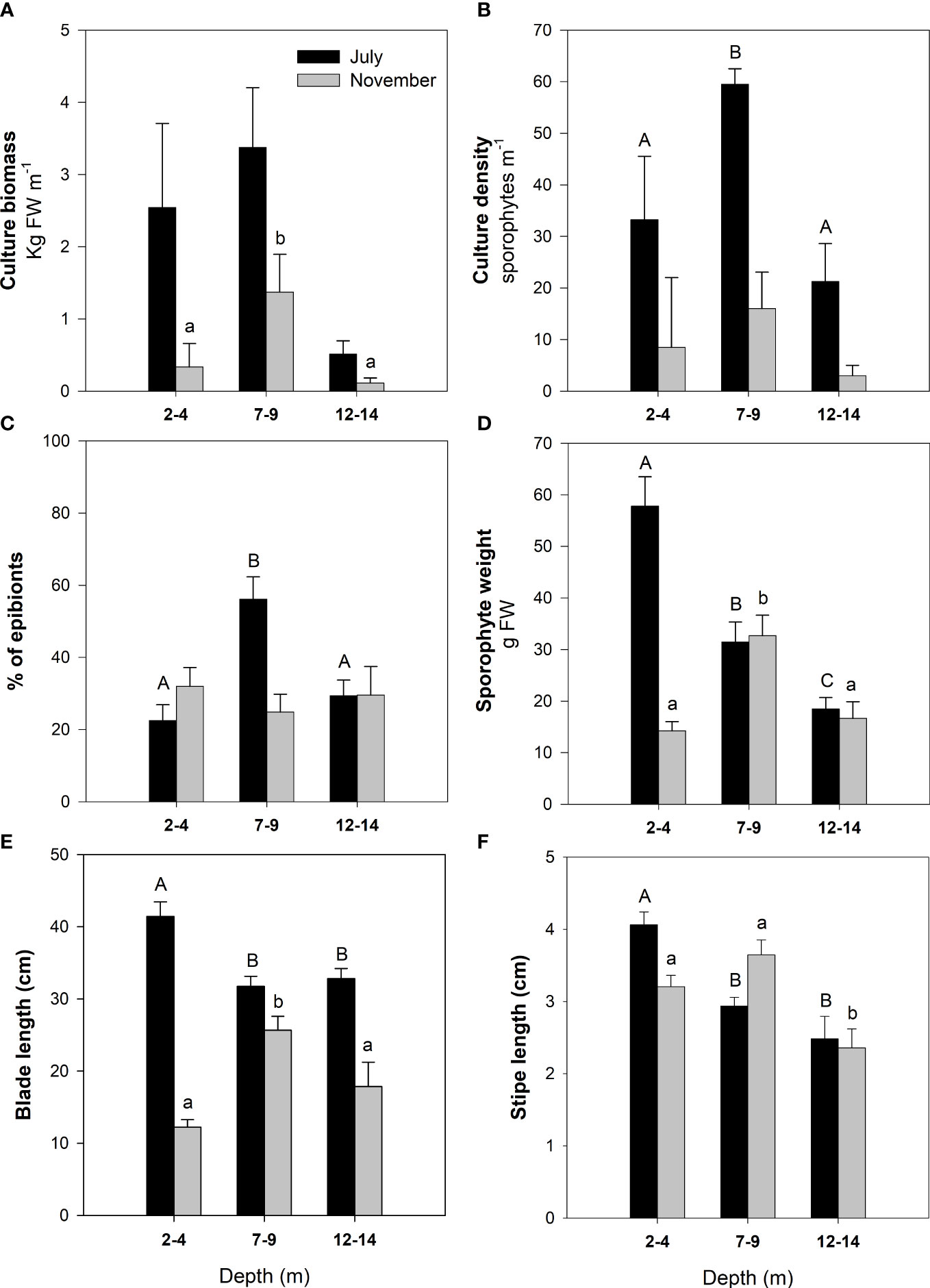
Figure 5 Biological descriptors (means and standard errors) measured at two sampling times (July and November 2016) in Eisenia arborea cultivated at three different depth ranges (2–4, 7–9, and 12–14 m). (A) Culture biomass, (B) culture density, (C) fresh weight of epiboints expressed as the percent of the total fresh weight of sporophytes, (D) sporophyte weight, (E) blade length, and (F) stipe length. Data correspond to the winter culture (i.e., seeding in February 2016). Different uppercase and lowercase letters indicate statistically significant differences (one-way ANOVA, post hoc Student Newman Keuls) among variables obtained at different depths in July and November, respectively.
With regard to the ontogenetic stage of sporophytes (Figure 6), in July, almost all the individuals grew to stage B and C, whereas a small number (< 5%) corresponded to stage A individuals, mostly between 7–9 and 12–14 m depth. The percentage of stage C individuals decreased from 70% to 40% with depth, whereas the opposite pattern was detected for stage B individuals. In November, predominant sporophytes were C and D stages; D stage individuals were only found between 2–4 and 7–9 m depth and comprised near 20% and 50%, respectively.
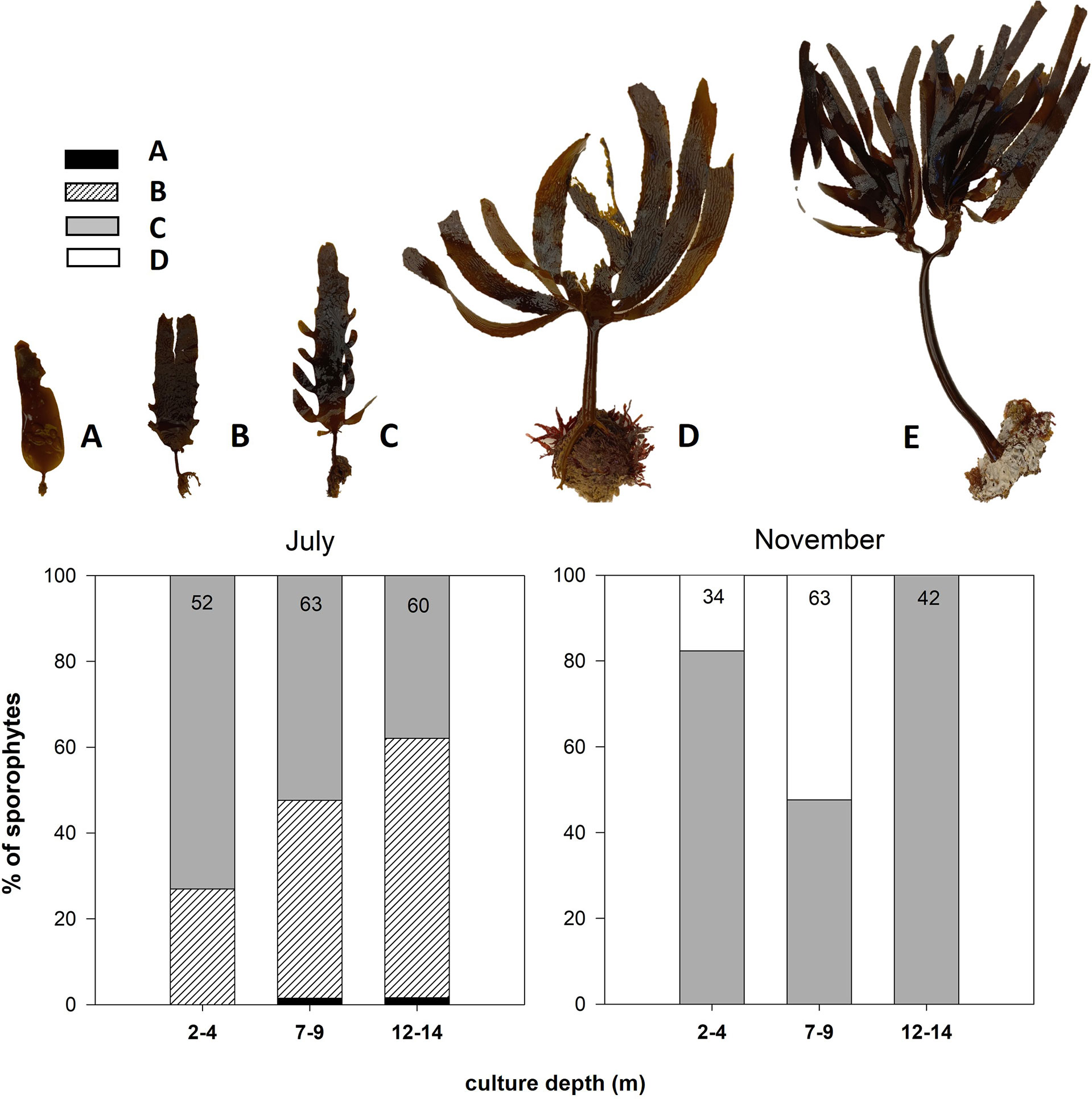
Figure 6 Percent of Eisenia arborea sporophytes of different ontogenetic development measured at two sampling times (July and November 2016) and at three different culture depth ranges (2–4, 7–9, and 12–14 m). Numbers inside the bars indicate the number of sporophytes analyzed per depth. Upper pictures have been modified from Arnold (1980) and show the different ontogenetic stages of E. arborea sporophytes.
Discussion
Eisenia arborea is the kelp with the greatest latitudinal distribution on the Eastern Pacific coast, ranging from Vancouver, Canada, to Magdalena Bay, Mexico. The ability of this species to survive and thrive in the warm low-nutrient waters at its southern limit in the Northern Hemisphere has been attributed to its capacity for rapid nutrient uptake, without evident saturation, during short fertilization events (Sánchez-Barredo et al., 2011). Although this species does not exhibit the fast growth rates observed for Macrocystis pyrifera (Camus and Buschmann, 2017 and Celis-Plá and Alveal, 2012), its resilience under a wide variety of environments across its range makes it an ideal kelp for cultivation under a warming climate change regime.
Previous studies of Eisenia arborea based on stipe or blade length report relatively low growth rates in California (Dayton et al., 1999). However, the results reported in the present study show that growth of E. arborea can be much higher than previously reported. For example, individuals cultured at the SQB site, which is a nutrient-rich environment, showed rapid growth. Increase in biomass was mainly due to new blade generation and not pruned blade regrowth. The successful growth rates obtained using the method of attaching the holdfast/haptera on a braided propylene rope, similar to the technique used for other commercial kelps (Laminaria and Undaria), show that this method can be successfully applied to this kelp as well and used for large-scale E. arborea cultivation.
In the kelp beds along the California and Baja California Pacific coast, Eisenia arborea, Pterygophora, and Laminaria farlowii are part of the understory, growing under the canopy shade of Macrocystis pyrifera (Schiel and Foster, 2006; Schiel and Foster, 2015). In California, Eisenia is reported as fertile in the late summer and fall, as the most tolerant kelp to physical stress, and shows the highest percentage of recruitment survival with an estimated survivorship of up to 12 years. Although no in situ growth rates have been reported for California, clearing field experiments do show growth limitation by light (Dayton et al., 1984; Schiel and Foster, 2015). In the present study in Baja California, fertile sori were surprisingly found throughout the year, particularly in shallower individuals of BSQ. This is an important consideration for any farming or cultivation endeavor as having a reliable spore source is critical for success because the availability of mature sori is a common bottleneck for successful widespread kelp cultivation. The consistent availability of fertile sori identified in this study in BSQ, along with the successful growth rates and fecundity acquired through controlling density on long lines, provides the basis for successful management of domestication and farming activities to increase growth and fecundity in the Baja California region. Although the growth rate and productivity per unit area of Eisenia cultivation was significantly lower than that of M. pyrifera (Camus et al., 2018; Camus et al., 2019), and although it can take around 18 months to reach a fully differentiated individual, the resilience to stress, longer survivorship, and possibility of year-round harvest might compensate for that suggesting Eisenia as an excellent option for commercial cultivation, particularly as the oceans continue to warm under climate change scenarios.
Several manuals exist in the literature outlining basic steps for kelp cultivation (Tseng, 1987; Merrill and Gillingham, 1991; Kawashima, 1993; Flavin et al., 2013; Redmond et al., 2014). Nevertheless, every species can be different, and variability in success rates should be expected for different populations, locations, and seasons (Augyte et al., 2017; Augyte et al., 2018; Mao et al., 2020; Huang et al., 2022). For example, our results showed that fully developed individuals growing in shallow waters with greater light exposure released spores more rapidly after desiccation. In SQB, for instance, fertile sori were found all year around. In our density experiment, although no differences in growth were observed, the lower density cultures exposed to less self-shading did show a higher number of reproductive individuals, further supporting our observations in the natural populations. These results can be applied to increasing farm yields, reproduction, and productivity for optimal results.
Culture outcomes in the three sites tested (TSB, SQB, and SMB) differed significantly. This study showed that Eisenia arborea culture is feasible in a variety of environments, especially in strong upwelling areas such as the SQB and SMB areas, even where it does not commonly occur. However, seasonality was an important factor affecting success, with winter showing the highest seeding success, and would be particularly important to consider going forward. It is well known that both temperature and nutrients can impact seaweed survivorship, mostly varying at seasonal scales with colder nutrient-rich water available in winter and spring, and more stratified conditions in summer and fall; however, both factors are highly variable at smaller spatial and temporal scales and are inversely correlated, making their synergistic impacts difficult to study and interpret (Ladah et al., 2012; Ladah, 2003). Because the seasonal environmental conditions at the time of seeding were a critical factor for success, we recommend a seasonal approach to domestication, negating the need for details on temperature and nutrient variability and rather focusing on a practical seasonal guide going forward for industry applications. Salinity, on the other hand, is not an issue as the region is principally arid and has very little river outflow. Because reproductive sori were also available all year around (in SQB), and some growth was observed during all seeding events, farming efforts can take advantage of well-known seasonal patterns for specific sites to modulate growth or reproduction on long lines.
Similar to the ability of Macrocystis pyrifera to regenerate after harvesting, Eisenia arborea showed the capacity to regenerate when its blades were harvested by pruning at 5 cm above the base of the blade to maintain the meristematic region. This capacity, however, was observed only at one of the sites tested. Further studies are needed to explore the precise protocols for successful re-growth of blades, as this was not the objective of the study. An important observation was when the blades were not harvested, they would eventually decay and were lost. During this process, negative growth rates were observed because the generation of new blades was slower than the decay of older blades (Figures 2, 3). New blades were always observed, but their rapid growth only occurred in the spring and early summer.
Recruitment of Eisenia appears to be a rare event in natural conditions; however, established juveniles generally show high survivorship, with growth and reproduction reduced with depth (Dayton, 1985). Similar to Laminaria farlowii, Eisenia seems especially sensitive to competition for light and space at almost all life history stages. Recruitment is strongly inhibited by canopies of other kelps, as are growth and reproduction. It also seems to be the slowest kelp to reach sexual maturity. In southern Baja California, however, dense beds occur in the intertidal zone, particularly in areas where warmer temperatures and concurrent lower nutrients appear to permit Eisenia to outcompete Macrocystis pyrifera (Matson and Edwards, 2007). Through mariculture activities where depth and light exposure can be controlled, Eisenia can provide important kelp biomass for the rising commercial needs in the region, particularly as an alternative to other kelps in a warming ocean climate, employing appropriate attention to both site selection and seasonality.
This study demonstrates that Eisenia arborea spores generated in the laboratory can be cultured with significant increase in biomass under a variety of oceanic conditions. Furthermore, blades from fully developed individuals were shown to regenerate after harvesting by pruning, providing the opportunity to reutilize the attached base for continued culture of this perennial seaweed, thereby avoiding further manipulation. Environmental conditions, however, were also shown to have a significant influence on initial seeding and deployment success in the growth rate and biomass yield and must be considered. In conclusion, Eisenia commercial culture can become an important ecological and economic endeavor in the Pacific Baja California region with appropriate attention to site selection and seasonality. Future studies, however, are required to assure development of the cultivation of this species, particularly those related to successful production of viable spores as well as the selection of improved cultivars. As in any other mariculture development, a significant effort needs to be dedicated to site selection. As observed in this study, different sites provide different productivity results. Our study, however, shows that Eisenia arborea may be suitable for a variety of environments present along the Pacific coast of Baja California.
Data availability statement
The raw data supporting the conclusions of this article will be made available by the authors, without undue reservation.
Author contributions
JZ-G designed the studies, acquired funding, led the experiments, participated in the field work and writing of the manuscript. JS-G processed the data for the site validation and contributed in the writing of the manuscript. MS-B, ZA-G and JS-G and LR-M participated in the field experiments, data collection and processing. LL participated in the experimental design and writing of the manuscript. RC-L participated in the data interpretation and writing. CY collaborated in the proposal writing and advising for the experimental design. All authors contributed to the article and approved the submitted version.
Funding
This research was supported by Fundación Produce-SAGARPA and Secretaría de Pesca y Acuacultura del estado de Baja California as well as by the UABC (Convocatoria interna CA-16) to the first author and a scholarship from CONACYT to ZA-G.
Acknowledgments
We would like to acknowledge the support of the late Dr. Craig Barilotti for his guidance in kelp cultivation through many years. Moreover, Mr. Vicente Guerrero, Carlos Guerrero and the staff of Ostricola Nautilus SA de CV de RL provided their field support for the San Quintín and Santa María work. In addition, special thanks to our colleagues Guadalupe Paniagua, Guadalupe García, and Guillermo Ballesteros for their support in both field and laboratory work.
Conflict of interest
The authors declare that the research was conducted in the absence of any commercial or financial relationships that could be construed as a potential conflict of interest.
Publisher’s note
All claims expressed in this article are solely those of the authors and do not necessarily represent those of their affiliated organizations, or those of the publisher, the editors and the reviewers. Any product that may be evaluated in this article, or claim that may be made by its manufacturer, is not guaranteed or endorsed by the publisher.
Supplementary material
The Supplementary Material for this article can be found online at: https://www.frontiersin.org/articles/10.3389/fmars.2022.932534/full#supplementary-material
References
Abbott I. A., Hollenberg G. J. (1976). Marine algae of California (Stanford, California, USA:Stanford University Press), 827.
Aguilar-Rosas R., López-Carrillo M., Aguilar-Rosas L. E. (2005). Macroalgas marinas de la bahía de San quintín, Baja California, méxico. Polibotanica 19, 19–38.
Aguirre-Muñoz A., Budemeier R. W., Camacho-Ibar V., Carriquiry J. D., Ibarra-Obando S. E., Massey B. W., et al. (2001). Sustainability of coastal resource use in San quintin bay, Mexico. Ambio 30, 42–149. doi: 10.1579/0044-7447-30.3.142
Álvarez Borrego S. (2004). Nutrients and phytoplankton dynamics in a coastal lagoon. Cienc. Mar. 30, 1–19. doi: 10.7773/cm.v30i1A.2510
Areschoug J. E. (1876). De tribus Laminarieis (Egregia Aresch., Eisenia Aresch., Nereocystis) et de Stephanocystide osmundacea (Turn.). Trevis. observationes praecursorias offert. Botaniska Notiser 1876, 65–73.
Arnold K. E. (1980). Aspects of the production ecology of marine macrophytes. a dissertation for the degree in Ph.D (Irvine: University of California), 149 pp.
Arvizu D. L., Rodríguez Y. E., Hernández G., Murillo J. I. (2007). Chemical constituents of Eisenia arborea areschoug from Baja California sur, méxico. Invest. Mar. Valparaíso. 35 (2), 63–69. doi: 10.4067/S0717-71782007000200007
Augyte S., Lewis L., Lin S., Neefus C. D., Yarish C. (2018). Speciation in the exposed intertidal: the case of Saccharina angustissima comb. nov. & stat. nov. (Laminariales, phaeophyceae). Phycologia 57 (1), 100–112. doi: 10.2216/17-40.1
Augyte S., Yarish C., Redmond S., Kim J. K. (2017). Cultivation of a morphologically distinct strain of the sugar kelp, Saccharina latissima forma angustissima, from coastal Maine, USA, with implications for ecosystem services J appl. Phycol 29 (4), 1967–1976. doi: 10.1007/s10811-017-1102-x
Camus C., Buschmann A. H. (2017). Macrocystis pyrifera aquafarming: production optimization of rope-seeded juvenile sporophytes. Aquaculture 468, 107–114. doi: 10.1016/j.aquaculture.2016.10.010
Camus C., Infante J., Bushmann A. (2018). Overview of 3 years precommercial seafarmin of Macrocystis pyrifera along the Chilean coast. Rev. Aquacult. 10, 543–559. doi: 10.1111/raq.12185
Camus C., Infante J., Bushmann A. (2019). Revisiting the economic profitability of giant kelp Macrocystis pyrifera (Ochrophyta) cultivation in Chile. Aquaculture 502, 80–86. doi: 10.1016/j.aquaculture.2018.12.030
Celis-Plá P., Alveal K. (2012). Development of Macrocystis pyrifera from spores and gametes on artificial substrate. algal production in a surface culture. Latin Amer. J. @ Aquat. Res. 40, 292–299. doi: 10.3856/vol40-issue2-fulltext-5
Chung I. K., Beardall J., Mehta S., Sahoo D., Stojkovic S. (2011). Using marine macroalgae for carbon sequestration: a critical appraisal. J. Appl. Phycol. 23, 877–886. doi: 10.1007/s10811-’10-9604-9
Craigie J. S., MacKinnon S. L., Walter J. A. (2008). Liquid seaweed extracts identified using 1H NMR profiles. J. Appl. Phycol. 20, 665–671. doi: 10.1007/s10811-007-9232-1
Dayton P. K. (1985). Ecology of kelp communities. Annu. Rev. Ecol. Syst. 16, 215–245. doi: 10.1146/annurev.es.16.110185.001243
Dayton P. K., Currie V., Gerrodette T., Keller B. D., Rosenthal R., Ven Tresca D. V. (1984). Patch dinamics and stability of some California kelp communities. Ecol. Monographs 54 (3), 253–289. doi: 10.2307/1942498
Dayton P. K., Tegner M. J., Edwards P. B., Riser K. L. (1999). Temporal and spatial scales of kelp demography: the role of oceanographic climate. Ecol. Monographs 69, 219–250. doi: 10.1890/0012-9615(1999)069[0219:TASSOK]2.0.CO;2
Edwards M. S., Hernández-Carmona G. (2005). Delayed recovery of giant kelp near its southern range limit in the north pacific following El niño. Mar. Biol. 147, 273–279. doi: 10.1007/s00227-004-1548-7
FAO (2021). FAO yearbook. fishery and aquaculture statistics 2019/FAO annuaire. statistiques des pêches et de l’aquaculture 2019/FAO anuario, Estadísticas de pesca y acuicultura 2019. Rome/Roma. FAO:Rome, Italy
Flavin K., Flavin K., Flahive B. (2013). Kelp farming manual. a guide to the processes, techniques, and equipment for farming kelp in new England waters. Ocean Approved, 130.
Foster M. S., Scheil D. R. (2010). Loss of predatos and the collapse of southern caifornia kelp forest (?): alternatives, explanations and generalizations. J. @ Exp. Mar. Biol. Ecol. 393, 59–70. doi: 10.1016/j.jembe.2010.07.002
Gossard D. J., Stellar D. L. (2022). Population persistence of the perennial kelp Eisenia arborea varies across local spatial scales. Algae 37 (1), 63–74. doi: 10.4490/algae.2022.37.2.19
Hafting J. T., Craigie J. S., Stengel D. B., Loureiro R. R., Buschmann A. H., Yarish C., et al. (2015). Prospects and callenges for industrial production of seaweed bioactives. J. Phycol. 51, 821–837. doi: 10.1111/jph.12326
Hernández-Carmona G., García O. D., Robledo D., Foster M. (2000). Restoration techniques for Macrocystis pyrifera (phaeophyceae) populations at the southern limit of their distribution in méxico. Bot. Mar. 43, 273–284. doi: 10.1515/BOT.2000.029
Hernández-Carmona G., Robledo D., Serviere-Zaragoza E. (2001). Effect of nutrient availability on Macrocystis pyrifera recruitment and survival near its southern limit off Baja California. Bot. Mar. 44, 221–229. doi: 10.1515/BOT.2001.029
Hollenberg G. J. (1939). Culture studies of marine algae. i. Eisenia arborea. Ame J. @ Bot. 26, 34–41. doi: 10.1002/j.1537-2197.1939.tb12863.x
Huang M., Robbins K. R., Li Y., Umanzor S., Marty-Rivera M., Bailey D., et al. (2022). Simulation of sugar kelp (Saccharina latissima) breeding guided by practices to accelerate genetic gains. G3: Genes| Genomes| Genet. V12 (3), jkac003. doi: 10.1093/g3journal/jkac003
Kawashima S. (1993). “Cultivation of the Brown Alga, Laminaria “Kombu”. In “Seaweed cultivation and Marine Ranching (Ohno M., Critchley A.T.). First Edition. KIFTC-JICA. Nagai, Yokosuka, Japan,
Ladah L. B., Zertuche-González J. A., Hernández-Carmona G. (1999). Giant kelp (Macrocystis pyriferI, phaeophyceae) recruitment near its southern limit in Baja California after mass disappearance during ENSO 1997-1998. J. Phycol. 35, 1106–1112. doi: 10.1046/j.1529-8817.1999.3561106.x
Ladah L. (2003). The shoaling of nutrient-enriched subsurface waters as a mechanism to sustain primary productivity off Central Baja California during El Niño winters. J Marine Systems 42, 145–152. doi: 10.1016/S0924-7963(03)00072-1
Ladah L., Filonov A., Lavín M., Leichter J., Zertuche-González J., Pérez-Mayorga D. (2012). Cross-shore transport of sub-thermocline nitrate by the internal tide and rapid (3-6 h) incorporation by an inshore macroalga. Continental Shelf Research 42, 10–19. doi: 10.1016/j.csr.2012.03.010
Landa-Cansigno C., Hernández-Carmona G., Aarvizu-Higuera D. L., Muñoz-Ochoa M., Hernández-Guerrero C. J. (2017) Bimonthly variation in the chemical composition and biological activity of the brown seaweed Eisenia arborea (Laminariales: Ochrophyta) from bahía Magdalena, Baja California sur, Mexico. J. Appl. Phycol. V (29), 2605–2615. doi: 10.1007/s10811-017-1195-2
Long J. H., Rheuban J. E., Berg P., Zieman J. C. (2012). A comparison and correction of light intensity loggers to photosynthetically active radiation sensors. Limnol. Oceanogr.: Methods 10 (6), 416–424. doi: 10.4319/lom.2012.10.416
Mao X., Augite S., Huang M., Hare M. P., Bailey D., Umanzor S., et al. (2020). Population genetics of sugar kelp in the Northwest Atlantic region using genome-wide markers. Front. Mar. Sci. 7, 694. doi: 10.3389/2020.00694
Matson P. G., Edwards M. S. (2006). Latitudinal variation in stipe hollowing in Eisenia arborea (Phaeophyceae, laminariales). Phycologia 45 (3), 343–348. doi: 10.2216/05-41.1
Matson P. G., Edwards M. S. (2007). Effects of ocean temperature on the southern range limits of two understory kelps, Pterygophora californica and Eisenia arborea, at multiple life-stages. Mar. Biol. 45, 297–309. doi: 10.1007/s00227-007-0630-3
Merrill J. E., Gillingham D. M. (1991). Bull kelp cultivation handbook Vol. 91 (Portland, Oregon, USA: National Coastal Research and Development Institute). NCRI Publication No. NCRI-T-91-01.
Múzquiz de la Garza A. R., Tapia-Salazar M., Maldonado-Muíz M., de la Rosa J., Gutiérrez-Uribe J. A., Santos-Zea L., et al. (2019). Nutraceutical potential of five Mexican brown seaweeds. BioMed. Res. Int. 19, ID 3795160. doi: 10.1155/2019/3795160
Notoya M., Aruga Y. (1990). Tissue culture from the explant of stipe of Eisenia bicyclis (Kjellman) setchell (Laminariales, phaeophyta). Jpn. J. Phycol. 38 (4), 387–390.
Pfister C. A., Berry H. D., Mumford T. (2017). The dynamics of kelp forests in the northeast pacific ocean and the relationship with environmental drivers. J. Ecol. 106, 1520–1533. doi: 10.1111/1365-2745.12908
Redmond S., Green L., Yarish C., Kim J. K., Neefus C. (2014). “New England seaweed culture handbook,” in Seaweed cultivation. Available at: https://opencommons.uconn.edu/seagrant_weedcult/1/. Paper 1. University of Connecticut Sea Grant: Groton, CT
Rocca S., Agostini A., Giuntoli J., Marelli L. (2015). Biofuels from algae: technology options, energy balance and GHG emissions; insights from a literature review, EUR 27582 EU publications. doi: 10.2790/125847
Sánchez-Barredo M., Ladah L. B., Zertuche-González J. A. (2011). Nitrate uptake and duration of internal nitrogen reserves in the kelp Eisenia arborea. Bot. Mar. 54, 441–446. doi: 10.1515/BOT.2011.058
Schiel D. R., Foster M. S. (2006). The population biology of Large brown seaweeds: Ecological consequences of multiphase life histories in dynamic coastal environments. Ann. Rev. Eco. Evol. Syst. 37 (1), 343. doi: 10.1146/annurev.ecolsys.37.091305.110251
Schiel D. R., Foster M. S. (2015). The biology and ecology of giant kelp forests (Oakland, California, USA:University of California Press), 395 pp.
Serviere-Zaragoza E., Gómez-López D., Ponce-Díaz G. (2002). Gross chemical composition of three common macroalgae and a sea grass on the pacific coast of Baja California, Mexico. Hidrobiológica 12 (2), 113–118.
Shults T. C., Herbst C. C. (1938). The life history of Eisenia arborea. Am. J. Bot. 25, 494–498. doi: 10.1002/j.1537-2197.1938.tb09249.x
Smale D. (2020). Impacts of ocean warming on kelp forest ecosystems. New Phytol. 225, 1447–1454. doi: 10.1111/nph.16107
Spalding H., Foster M. S., Hiene J. N. (2003). Composition, distribution and abundance of deep-water (>30 m) macroalgae in central California. J. Phycol. 39, 273–284. doi: 10.1046/j.1529-8817.2003.02010.x
Terawaki T., Hasegawa H., Shogo A., Ohno M. (2001). Management-free techniques for restoration of Eisenia and Ecklonia beds along the central pacific coast of Japan. J. Appl. Phycol. 13, 13–17. doi: 10.1023/A:1008135515037
Tseng C. K. (1987). “Laminaria mariculture in China,” in Case studies of seven commercial seaweed resources. Eds. Doty M. S., Caddy J. F., Santelices B., 239–264. FAO Fisheries Technical paper 281: Rome, Italy.
Zanchetta E., Damergi E., Patel B., Borgmeyer T., Pick H., Pulgarin A., et al. (2021). Algal cellulose, production and potential use in plastics: Challenges and opportunities. Algal. Res. 56. doi: 10.1016/J.algal.2021.102288
Keywords: Eisenia, Ecklonia, kelp cultivation, seaweed domestication, Baja California
Citation: Zertuche-González JA, Sandoval-Gil JM, Sánchez-Barredo M, Altamirano-Gómez Z, Guzmán-Calderón JM, Rangel-Mendoza LK, Ladah LB, Cruz-López R and Yarish C (2022) Eisenia arborea (Areschoung) domestication and mariculture development on the Pacific coast of Baja California, México. Front. Mar. Sci. 9:932534. doi: 10.3389/fmars.2022.932534
Received: 29 April 2022; Accepted: 25 July 2022;
Published: 24 August 2022.
Edited by:
Cecilia Biancacci, Deakin University, AustraliaCopyright © 2022 Zertuche-González, Sandoval-Gil, Sánchez-Barredo, Altamirano-Gómez, Guzmán-Calderón, Rangel-Mendoza, Ladah, Cruz-López and Yarish. This is an open-access article distributed under the terms of the Creative Commons Attribution License (CC BY). The use, distribution or reproduction in other forums is permitted, provided the original author(s) and the copyright owner(s) are credited and that the original publication in this journal is cited, in accordance with accepted academic practice. No use, distribution or reproduction is permitted which does not comply with these terms.
*Correspondence: José A. Zertuche-González, WmVydHVjaGVAdWFiYy5lZHUubXg=