- 1Department of Sea and Marine Resources, Portuguese Institute for Sea and Atmosphere (IPMA, I.P), Olhão, Portugal
- 2Centre of Marine Sciences (CCMAR), University of Algarve, Faro, Portugal
- 3Interdisciplinary Centre of Marine Environmental Research (CIIMAR), University of Porto, Matosinhos, Portugal
European clam (Ruditapes decussatus) is one of the most relevant emergent bivalve species from the aquaculture sector in Europe with high economic value. Climate changes represent a potential limiting factor to this activity, directly interfering with the survival and behavior of bivalves. Severe fluctuations in temperature along with periods of heavy rainfall or periods of drought that significantly change the salinity can promote physiological stress in bivalves, resulting in changes in physiological and behavioral responses and, in extreme cases, leading to high mortalities. This study aimed to evaluate the combined effect of temperature and salinity on mortality and feeding behavior of R. decussatus. Juveniles and adults were exposed to combined ranges of temperature (5°C–35°C) and salinity (0–40). Mortality and feeding behavior were registered every 24 h of each 120-h trial. A control temperature range was set between 15°C and 23°C, where mortality and feeding behavior were considered as the normal scenario. Our data suggested salinity 15 as a “turning point,” a point from which occurred distinct patterns in mortality and feeding behavior. The results evidently indicate that abrupt reductions in salinity and sharp increases in temperature will lead to high mortality of R. decussatus. Juveniles were revealed to be more sensitive to the increase of temperature in a less saline environment, to suffer greater and faster mortalities, and to be more resistant to extremely high temperatures under more saline conditions. The high temperatures and sporadic heavy rainfall that are predicted to occur in the south of Europe due to climate changes will contribute to compromise the recruitment of European clam, thus threatening the production of this species and consequently impacting the economic sector.
Introduction
Climate changes are of great concern, being the focus of scientists and policymakers (Verdelhos et al., 2015a). In the next 100 years, changes in climate are predicted to occur, at a global scale, through increases in sea and air temperature, water acidification, and changes in seawater salinity, sea level, and precipitation (Poulin and Mouritsen, 2006; Matozzo and Marin, 2011; IPCC, 2021).
According to the Intergovernmental Panel on Climate Change (IPCC) (2021), until the end of the century, it is hypothesized that, in the lower emissions scenario, the global temperature will rise by 1.5°C with increasing intensity and frequency of extreme rainfalls and heatwaves. In the worst possible scenario, global temperature may reach an increment of 4.4°C, with the potential to achieve 5.7°C, causing large-scale coastal inundation and extremely destructive weather with parts of the planet being uninhabitable during heat waves (IPCC, 2021).
Global climate changes also interfere with hydrological cycles, namely, through the occurrence and intensity of extreme events such as precipitation and/or drought (Fenoglio et al., 2010). In turn, these events may have a direct impact on salinity, mainly in coastal and estuarine areas. For instance, in coastal areas, warmer temperatures can cause an increase in seawater evaporation and a reduction in rainfall, thus increasing salinity or causing heavy tropical rainfall that may be formed by warmer temperatures, consequently decreasing salinity (Matozzo and Marin, 2011).
Even though species have dealt with climate changes throughout their evolutionary history, the fast rate at which changes in climate are occurring (Philippart et al., 2003) may comprise the capacity of organisms to adapt to these variations, therefore affecting their physiology, growth, behavior, and survival (Otero et al., 2013; Velez et al., 2016).
Temperature and salinity are two of the most important physical factors affecting intertidal organisms, such as bivalves, playing an important role on their abundance and distribution (Gosling, 2004; Booij, 2005; Gharbi et al., 2016). While temperature is considered to affect energy balance and level of activity (Sobral and Widdows, 1997), salinity has an influence on physiological processes (Navarro and Gonzalez, 1998; Carregosa et al., 2014).
Besides the spatial distribution of bivalves, several aspects of their biology, such as growth, reproduction, feeding, behavior, and respiration (among others), are also affected by changes in climate (Gosling, 2004). During emersion periods, bivalves also have to deal with hypoxia and the interruption of feeding, since they are filter feeders (Sobral and Widdows, 1997; Gharbi et al., 2016). When exposed to stressful conditions, such as severe variations in temperature and salinity, bivalves usually close the valves—as a mechanism of protection against osmotic stress (Carregosa et al., 2014)—reduce feeding activity, and show slower growth and respiration rates and, consequently, alterations in oxygen consumption (Navarro and Gonzalez, 1998; Sarà et al., 2008; Carregosa et al., 2014).
European clam (Ruditapes decussatus) is a commercially and ecologically important species, broadly distributed along the European, Mediterranean, and North African coastal and estuarine waters (de Sousa et al., 2011; Matias et al., 2011; Cravo et al., 2012). In Portugal, this species is crucial to the aquaculture’s revenue, being extensively produced and harvested in Ria Formosa, representing 90% of the national production (Cravo et al., 2012; Matias et al., 2013; De Marchi et al., 2020). Ria Formosa is a highly productive mesotidal lagoon system located in the south coast of Portugal and, as an intertidal environment, is severely affected by temperature and salinity. The Ria Formosa lagoon is vital for local communities not only because of tourism and salt extraction but also due to bivalve harvesting and aquaculture (Matias et al., 2013).
Up to now, majority of the studies focused mainly on the variation of only one factor of climate change, even though, in the natural environment, organisms are affected by the variation of several factors combined. This way, hypothesizing the impact of changes in climate on lagoon areas, such as Ria Formosa, and considering the economic and ecological importance of R. decussatus, the current study was designed to evaluate the combined effects of different salinities (0–40) and temperatures (5°C–35°C) on the mortality and feeding behavior of R. decussatus. To better understand the effects of changes in climate in the life cycle of organisms and in their production, we chose to expose juveniles and adults to these combinations.
Materials and Methods
Experimental Design
Juveniles and adults of clams R. decussatus (3 ± 0.9 mm and 31 ± 3.1 mm shell length, respectively) were captured in the Ria Formosa lagoon (36°59′31.46″N, 7°55′21.90″W) and exposed to different combinations of water temperatures and salinities.
The Ria Formosa lagoon is characterized by subtidal channels, tidal flats, and salt marshes that are protected by a system of barrier islands and interact with oceanic waters via several inlets (Ribeiro et al., 2008; Cravo et al., 2012). This lagoon does not have a significant freshwater input, except for the contribution of the small river Gilão (Cravo et al., 2012).
The annual water temperature variation in this coastal area over the last years (2016–2020) was between 14.14 ± 0.86°C and 22.20 ± 1.14°C (data obtained from Tunipex, S.A., Olhão, Portugal), and salinity was usually around 35–37 (Cravo et al., 2012). Considering these factors, a control temperature range between 15°C and 23°C, inclusive, and salinity 35 were established. The temperatures tested were 5°C, 15°C, 20°C, 23°C, 26°C, 29°C, 32°C, and 35°C, while salinity ranged from 0 to 40 at intervals of 5. Extreme temperatures and salinities regarding control were chosen in order to simulate the predicted scenarios by the IPCC.
A total of 360 individuals of each size class were equally distributed into the experimental treatments: five individuals per treatment, in a total of 72 combinations of temperature and salinity. Each treatment tank (50 l), corresponding to a different temperature, was filled with water that was cooled down to 5°C and 15°C by a refrigerator or heated up to 35°C by a resistance provided with a thermostat. In each tank, nine beakers (V = 0.5 l) were placed and each one was filled with 1 µm of filtered seawater and adjusted to each desired salinity (0–40) (Figure 1). Each salinity was prepared by dissolving salt from the Ria Formosa lagoon in dechlorinated tap water. Tanks and beakers were continuously aerated to ensure oxygenation and to maintain constant temperature and salinity. Temperatures were verified twice a day throughout the experiment and adjusted if needed. The water of beakers was changed daily, and each one was refilled with water with the respective treatment of temperature and salinity.
Individuals were fed daily with 10 ml of the microalgae Tisochrysis lutea (approximately 60 cell/µl). The amount of food was calculated in such a way that it would not become a stress factor and to avoid salinity variations. Three and two trials of 120 h (Verdelhos et al., 2015a; Verdelhos et al., 2015b) were performed for adults and juveniles, respectively.
Mortality and Feeding Behavior
Mortality and feeding behavior were evaluated and registered every 24 h. Individuals were considered dead every time they were unable to close their valves after mechanical stimulus (Gosling, 2004), being then removed from the beakers.
Feeding behavior was evaluated through absence or presence of feces, scored as 0 or 1, respectively (Gosling, 2004).
Data Analysis
One-way ANOVA was applied to test for differences between trials. Since no statistical differences were detected between trials (ANOVA, p > 0.05), the results were analyzed considering the average of the different trials.
A preliminary analysis of mortality results suggested the existence of a “turning point” at salinity 15. Thus, to determine the median lethal temperature required for 50% of mortality (LC50) to occur after 120 h of exposure and the 95% confidence intervals (95% CI), mortality results were divided into two groups: salinities equal or below 15 (S ≤15) and salinities above 15 (S >15). Then, these grouped results were fitted to a logistic equation:
(where y = mortality; x= temperature; min, max, and Hillslope are estimated parameters).
Median lethal time (Lt50), which refers to the exposure time necessary for a given combination of temperature and salinity to cause 50% of mortality, was calculated through linear regressions.
The mean percentage of mortality after 120 h of exposure as well as Lt50 results of both size classes were plotted into contour graphs.
Feeding behavior was analyzed as: Feeding behavior= feces (24h + 48h + 72h + 96h + 120h)/5 ranging from 0 to 1.
Principal component analysis (PCA) was performed to determine the degree of association between factors (temperature, salinity, mortality, and feeding behavior).
All statistical analyses were performed using SigmaPlot 12.5 statistical package and XLSTAT 2022.1.2 statistical software for PCA analysis.
Results
Mortality
Low salinities and high temperatures caused higher mortality rates, in both juveniles and adults, after 120 h of exposure (Figure 2).
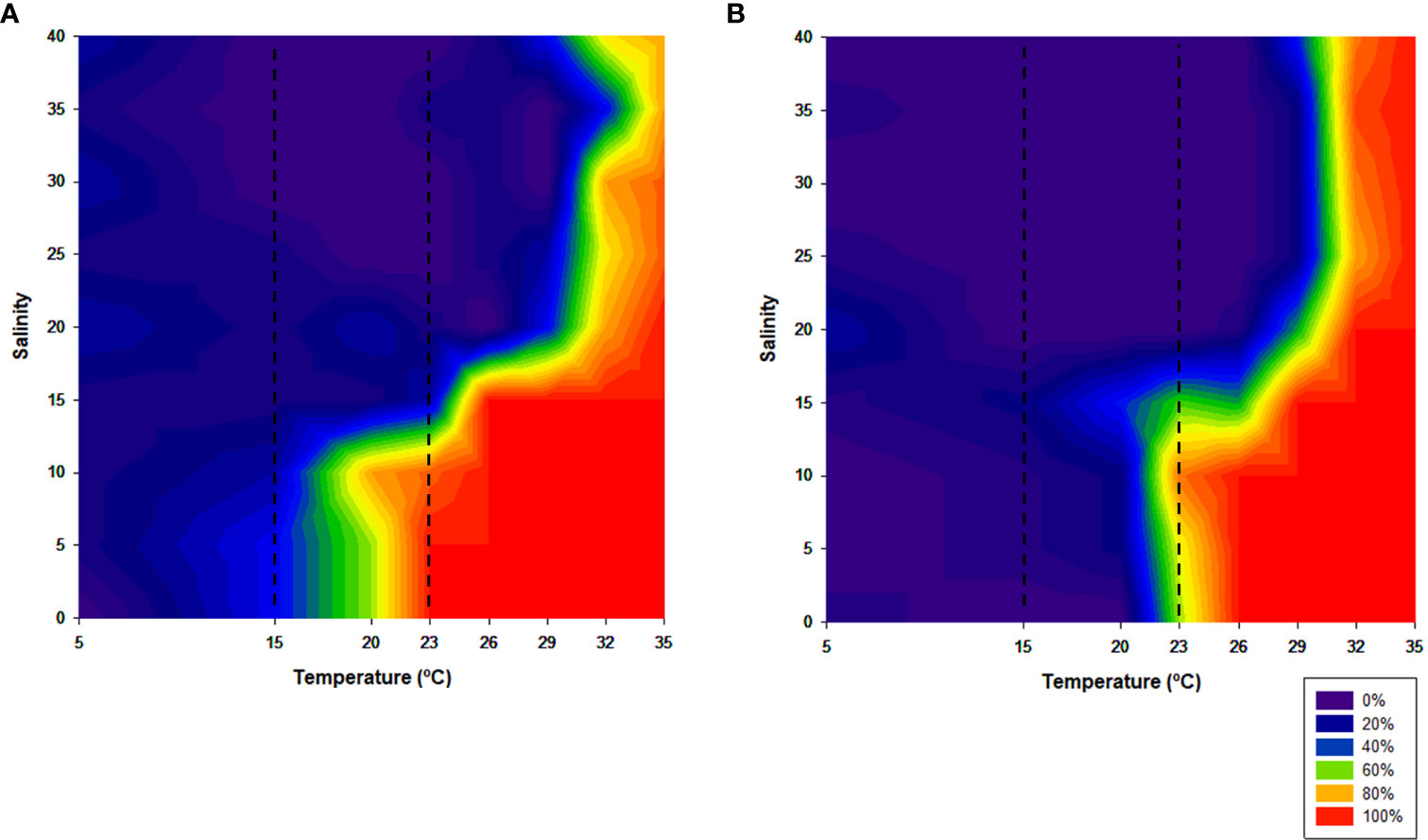
Figure 2 Percentage of mortality after 120 h of exposure along the temperature and salinity ranges. (A) juveniles; (B) adults. Dotted lines represent the established control temperature range (15°C–23°C).
In the control temperature range (15°C–23°C), juveniles were revealed to be more sensitive to the salinity variations, reaching 100% of mortality, mainly at the upper limit of the range (23°C) and at salinities lower than 15. As salinity increased, juveniles’ mortality decreased, varying between 0% and 20%. In adults, the mortality rate reached 60% of the individuals at salinities lower than 15. Above this salinity and at this temperature range, there was no mortality.
Below 15°C, the observed mortality was not significant, being very close to zero, in nearly all of the salinities tested, for both juveniles and adults.
Above the upper limit of the control temperature range (23°C) and salinities inferior to 15, the observed mortality was almost always extremely high, for both size classes. In more saline waters, juveniles seemed to be more resistant than adults, mainly at extreme temperatures (32°C and 35°C), where 100% of mortality of the adult population occurred.
Since our results showed that salinity 15 seemed to be a “turning point” for individuals’ survival, thus LC50 at 120 h of exposure was calculated considering the group of salinities equal and inferior to 15 (S ≤15: 0, 5, 10, 15) and the group of salinities above (S >15: 20, 25, 30, 35, 40).
For both juveniles and adults, mortality results fitted into a logistic distribution within the tested temperature. LC50 at 120 h was estimated according to following equations:
For S ≤15, LC50 after 120 h of exposure was lower than that for S >15, for both juveniles (20.28°C; 95% CI: 18.36–22.19°C and 31.08°C; 95% CI: 30.36–31.80°C, respectively) and adults (22.29°C; 95% CI: 20.54–23.04°C and 30.08°C; 95% CI: 29.68–30.49°C, respectively).
In general, the time needed for 50% mortality (Lt50) of both juveniles and adults to occur is higher than 120 h, mainly until 23°C, with exception of juveniles that, for this temperature and salinities below or equal to 10, it would only take between 65 and 70 h (Figure 3).
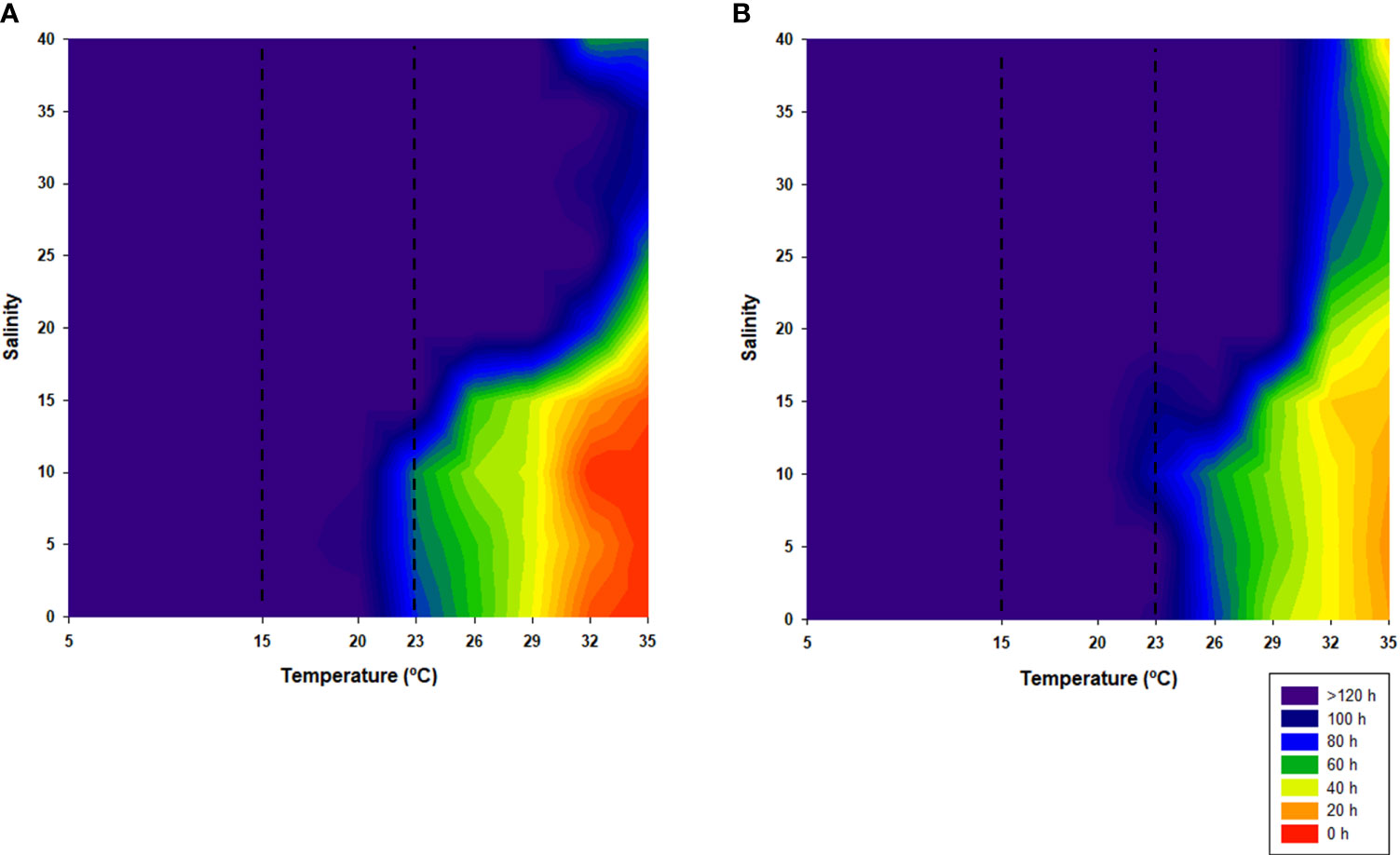
Figure 3 Median lethal time (Lt50) for juveniles (A) and adults (B). Dotted lines represent the established control temperature range (15°C–23°C).
In temperatures higher than 23°C and salinities less than or equal to 15, Lt50 substantially decreased as temperature increased, being that juveniles would only survive less than 20 h at extreme temperatures (32°C and 35°C). Adults appeared to be more resistant, surviving longer at this temperature and salinity combination. On the other hand, at higher salinities, the opposite occurred, i.e., juveniles seemed to be more tolerant than adults; however, the mortality of 50% of the adult population would never be expected to occur below 30 h of exposure.
Feeding Behavior
Feeding behavior seemed to be highly influenced by salinity, regardless of the temperature, for both juveniles and adults. In both size classes, at salinities lower than 15, it appeared that individuals did not feed, since the presence of feces was not observed. From this “turning point” and at the control temperature range, the feeding behavior of juveniles and adults was maximum, with juveniles exhibiting an index of 1 while that for adults was 0.93 (Figure 4).
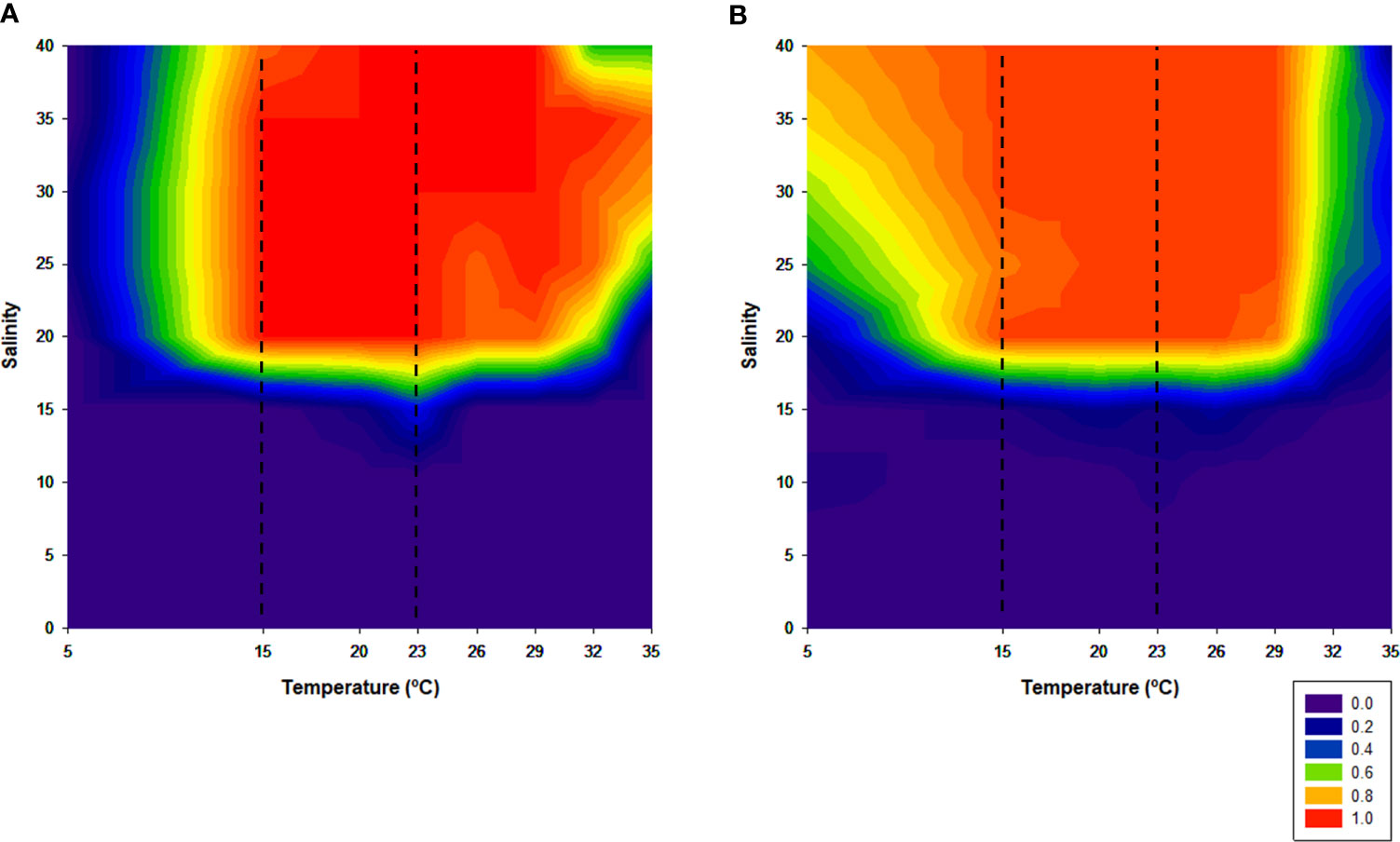
Figure 4 Feeding behavior of juveniles (A) and adults (B). Dotted lines represent the established control temperature range (15°C–23°C).
Out of this range, juveniles were less active at lower temperatures and more active at extreme temperatures. On the other hand, adults showed the opposite pattern. For instance, at lower temperatures, the feeding behavior index of juveniles was 0.1, while for adults this index was about 0.7, namely, at salinities higher than 25. As temperature increased to extreme values, the juveniles’ feeding behavior index remained high, except for the higher salinity (40). Comparatively, at extreme temperatures (32°C and 35°C), adults apparently stop feeding.
Principal Component Analysis
The PCA analysis resulted in two principal components (PC1 and PC2) that together described 90.61% and 93.05% of the overall variability of the data (Figure 5), for juveniles and adults, respectively. For both juveniles and adults, the PCA biplot displays that mortality was positively correlated with temperature (r juveniles = 0.597; r adults = 0.745; p< 0.05) while negatively with salinity (r juveniles = -0.552; r adults = -0.383; p< 0.05) and feeding behavior (r juveniles = -0.667; r adults = -0.603; p< 0.05). Salinity had a strong positive influence on feeding behavior (r juveniles = 0.753; r adults =0.835; p< 0.05).
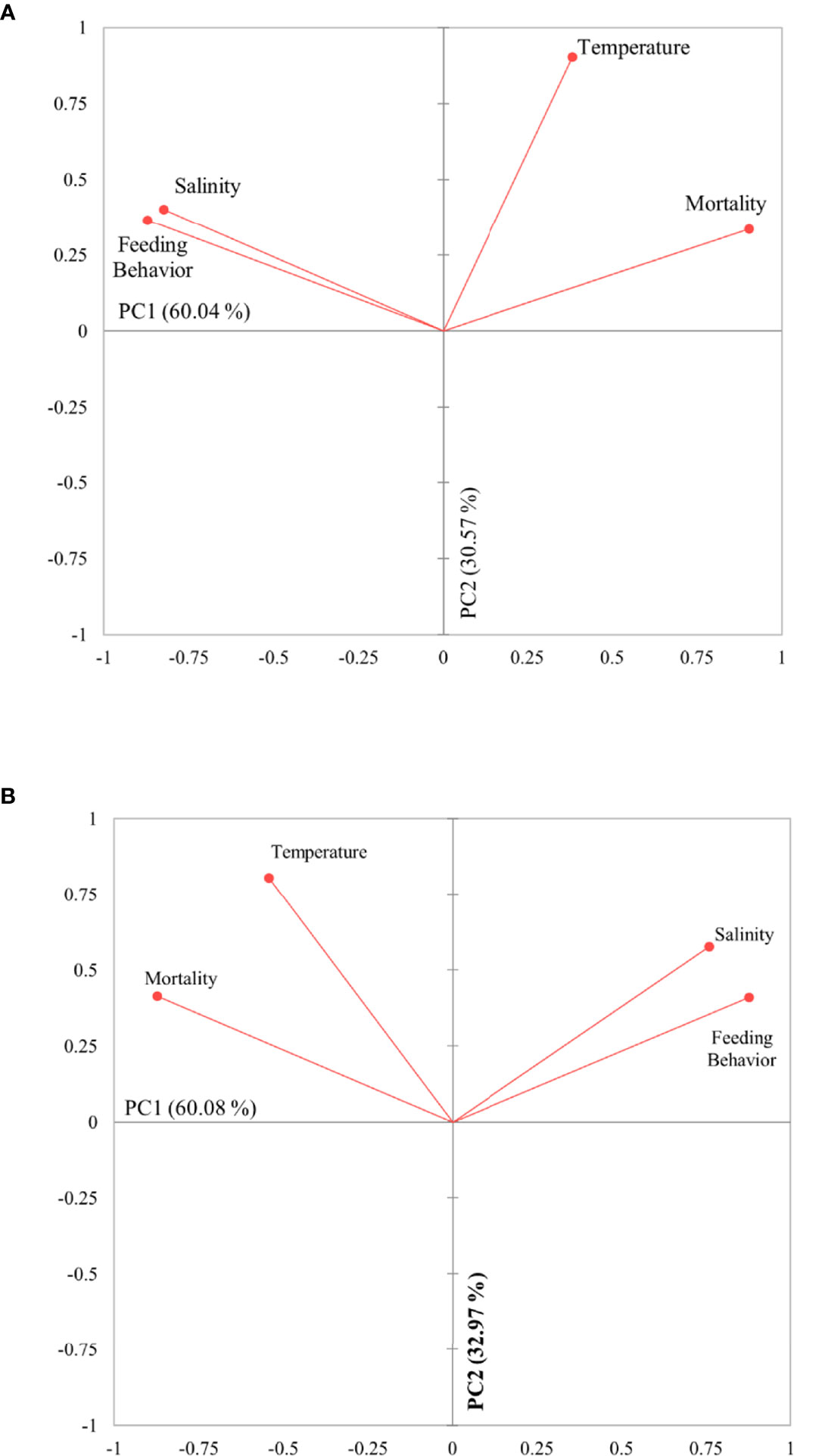
Figure 5 Principal component analysis (PCA) on the parameters used to evaluate the effect of different combinations of temperature and salinity on mortality and feeding behavior. (A) juveniles; (B) adults.
Discussion
Besides global warming and the consequent increase in seawater temperature, the IPCC (2021) has predicted higher intensity and frequency of extreme climatic events, such as heavy rainfalls, heatwaves, and droughts. Variations in temperature and salinity caused by these events will have a great impact on the survival and behavior of organisms, mainly those inhabiting intertidal areas, like bivalves. The stress induced by temperature and salinity fluctuations are distinct from each other (Woodin et al., 2020), and each species responds differently. Indeed, this work revealed distinct effects on the mortality and feeding behavior of R. decussatus juveniles and adults for varied combinations of temperature and salinity. As expected for euryhaline species, clams revealed a higher tolerance to salinity fluctuations. However, the temperature had a great impact on clams’ survival and behavior and, when combined with low salinities, revealed to be lethal.
In the current study, we have assumed a control range of temperatures (15°–23°C) and salinities (35–37), considering the annual variation in the Ria Formosa lagoon. It is important to underline that in this “normal” scenario, the mortality was residual, and the feeding behavior of both juveniles and adults was maximum.
Salinity 15 represents a “turning point,” a point from which occurred a change in mortality and feeding behavior. Woodin et al. (2020) also proposed the existence of a “breaking point” between salinities 15 and 20, below which there was a significant reduction in the behavioral activity of three venerid species (Venerupis corrugata, R. decussatus, and R. philippinarum). Likewise, Domínguez et al. (2020) suggested salinity 15 as a threshold below which was observed a sharp reduction in activity (burrowing and pumping) and scope for growth, and a high frequency of valve closure of Cerastoderma edule, R. decussatus, R. philippinarum, and V. corrugata. Besides the effect on activity behavior, our data suggested that salinities below 15 also had an impact on mortality when combined with high temperatures.
Below the salinity “turning point,” neither juvenile nor adult populations exhibited feeding behavior, therefore suggesting that salinity has a major impact on overall activity. Juveniles’ feeding behavior differed from that of adults at extreme temperatures: juveniles were more active at high temperatures while adults were at lower ones. Sarà et al. (2008) also found that low temperature and salinity caused a reduction in the clearance rate of the bivalve species Brachidontes pharaonis. The reduction in feeding behavior has severe consequences for growth, survival, and reproduction (Woodin et al., 2020).
The temperature had a great impact on clams’ survival, exhibiting a strong positive correlation with mortality. Regardless of the salinity, low temperatures (<15°C) did not cause any mortality. As the temperature increased and below the salinity “turning point,” there was a significant increase in clams’ mortality, with juveniles more susceptible even within the control temperature range.
High temperatures and low salinities proved to be quite lethal to clams. Above the salinity “turning point,” juveniles appeared to be more resistant than adults, mainly at extremely high temperatures (32° and 35°C).
These data are also supported by the median lethal temperature (LC50). The temperature needed to cause death to 50% of juveniles, at salinities ≤15, was inferior (20.28°C) than that of adults (22.29°C). At salinities higher than 15, both adults and juveniles can endure higher temperatures, with juveniles being more resilient, exhibiting 50% of mortality at 31.08°C while 50% of adults died at 30.08°C.
The effect of temperature and salinity on clams’ mortality not only depends on the variations of the parameters themself but also on the time of individuals’ exposure to it (Parada et al., 2012). The higher the exposure time to these variations, namely, to high temperatures, the greater the mortality. At low temperatures (≤23°C) and low salinities (≤15), it would take more than 120 h to cause 50% of mortality, maybe due to the clams’ capacity to remain with the valves closed under these stressful conditions. The situation became more critical in warmer (>23°C) and less saline waters (≤15), especially for juveniles, where only 20 h of exposure to these conditions was needed for 50% of mortality in the juvenile population to occur. In more saline waters (>15), only extremely high temperatures (32°C–35°C) were lethal, with adults more sensitive than juveniles, taking about 30 h to cause 50% mortality. Under these thermal and saline stresses, valve closure seems to be the most important bivalve response and defined the median lethal time (Lt50) of R. decussatus. This mechanism protects the soft tissues by isolating the bivalve from the surrounding environment and, therefore, limiting exposure to osmotic stress (Woodin et al., 2020). Bivalves are able to tolerate valve closure for short and cyclic periods, like between tides (Nossier, 1986; Domínguez et al., 2020), tolerating hypoxia and reducing the necessity for energy adjustments due to osmotic stress (Shumway, 1977). However, prolonged periods of valve closure have severe implications on bivalves’ performance since filtration activity ceases, reducing energy uptake and accumulating excretion products, which results in an increase in oxidative stress and therefore causing significant changes in their biochemical mechanisms (Domínguez et al., 2020).
In summary, clams closed their valves when exposed to low temperatures (<15°C) and salinities (≤15), resulting in a high survival rate and absence of feeding behavior in both juveniles and adults. At salinities below the “turning point,” as temperature increased to high values, clams were forced to open the valves, thus exposing the soft tissues to low salinities, and therefore to osmotic stress. The exposure to low salinities, which caused an osmotic shock, associated with high temperatures and consequent reduction in dissolved oxygen (Deutsch et al., 2015), led to severe mortalities in both size classes, with juveniles exhibiting a narrower thermal limit. Above the salinity “turning point,” mortality decreased and feeding behavior increased, except for extremely high temperatures (32° and 35°C), in which feeding behavior was reduced and mortality occurred probably due to low dissolved oxygen. In fact, the oxygen consumption of intertidal species exhibits circatidal rhythms, which may be modified when individuals are exposed to abrupt changes in salinity (Reyes-Martínez et al., 2020).
Although the shell acts as a boundary between the bivalve and the environment, valve closure does not protect the soft tissues of the bivalves from thermal stress caused by elevated temperatures. This is due to the high thermal diffusion of aragonite shells (Gómez-Martinez et al., 2002; Woodin et al., 2020), the main form of calcium carbonate (CaCO3) of Ruditapes species (Mu et al., 2018).
In this context, it is important to highlight the importance of the substrate. The substrate acts as a thermal buffer (Macho et al., 2016) since the temperature at deeper sediment layers is cooler than at the surface (Befus et al., 2013). When temperatures are high, R. decussatus can be buried in the sediment, therefore escaping the impacts of elevated temperatures. In our study, to avoid the introduction of another variable, we excluded substrate. According to Macho et al. (2016), at a surface temperature of 40°C, R. decussatus buried at 6 or 8 cm will experience a temperature of 30°C–31°C. The authors demonstrated that temperatures of 32°C, in the presence of substrate, did not cause any mortality in the venerid species R. decussatus and R. philippinarum (Macho et al., 2016). In the current study, we observed mortalities at 32°C. Moreover, according to Matias et al. (2013), in the Ria Formosa lagoon the severe clam mortalities occurred during summer, probably due to the junction of high temperatures with a weak physiological condition of individuals after spawning. Vázquez et al. (2021) demonstrated that short-term episodes of low salinities in winter and spring, followed by heatwave in summer, could greatly compromise reproduction in bivalves. Low salinities (<15) during sexual resting and the beginning of gametogenesis caused a delay in the gametogenic cycle and changes in oocytes’ morphology, while higher sediment temperatures (>32°C) caused an acute increase in hemocytes and gonadal reabsorption (Vázquez et al., 2021).
Moreover, warming and hyposalinity caused by changes in climate will promote the emergence, growth, and distribution of pathogenic microorganisms (e.g., viruses, bacteria, protozoans) and toxic microalgae (Zgouridou et al., 2022), therefore negatively impacting the immune and metabolic systems of bivalves (Matozzo and Marin, 2011; Turner et al., 2016), leading to severe mortalities. In fact, Reverter et al. (2020) reported that most of the infected marine organisms showed higher mortalities at warmer temperatures. It has been suggested that temperature and salinity play a critical role on disease outbreaks in marine organisms, by increasing the incidence and infection intensity by pathogens (Villalba et al., 2005; Macho et al., 2016), therefore leading to high clam mortality. In the case of Perkinsus olseni, a parasite of marine mollusks (Villalba et al., 2004), in vitro studies suggested that sporulation optimal temperature ranges between 24°C and 28°C and salinity between 25 and 35 (Auzoux-Bordenave et al., 1995), while it is inhibited below 15°C and slows at salinities between 10 and 15 (Kyoung and Ki, 2001). In conclusion, salinity fluctuations have a direct impact on feeding behavior, while temperature has a major impact on the survival of clams. Low salinities and high temperatures are lethal to R. decussatus. Juveniles are less resistant to the increase in temperature under less saline environments but are more resistant than adults to extremely high temperatures under more saline conditions. It is noteworthy that, in nature, these fluctuations in temperature and salinity may occur gradually, allowing clams to adapt. Likewise, the daily variation of temperature will also oscillate according to the tidal cycles and, therefore, clams will not be continuously exposed to extreme conditions (Sobral and Widdows, 1997). Nevertheless, considering the predicted climate change scenarios, juveniles will die faster when exposed to low salinities and high temperatures, consequences of heavy rainfall and heatwaves, never reaching the adult stage, thus compromising reproduction and consequently bivalve recruitment. On the other hand, these climate events may be coincident with the spawning season, between April and June (Matias et al., 2013), which may cause larval mortality and once more compromising the recruitment. The failure in bivalve recruitment will compromise the exploitation of the species, negatively impacting the economic sector.
Data Availability Statement
The raw data supporting the conclusions of this article will be made available by the authors, without undue reservation.
Author Contributions
AM acquired the funding. SJ and DM designed the experimental work. AR, AMM, and CR performed the experimental work. AR, SJ, and AMM analyzed the data and wrote the manuscript draft. All the authors revised and edited the manuscript. All authors contributed to the article and approved the submitted version.
Funding
This work was supported by the projects CERES – Climate change and European aquatic RESources, funded by European Union’s Horizon 2020 research and innovation program (grant agreement no. 678193), AIM (MAR – 02.01.01 – FEAMP – 0060), and SNMB-Sul-IV (MAR – 02.01.02 – FEAMP – 0221), funded by PO MAR2020. Ph.D. grant (SFRH/BD/147215/2019) was granted to AR by the Portuguese Foundation for Science and Technology (FCT).
Conflict of Interest
The authors declare that the research was conducted in the absence of any commercial or financial relationships that could be construed as a potential conflict of interest.
Publisher’s Note
All claims expressed in this article are solely those of the authors and do not necessarily represent those of their affiliated organizations, or those of the publisher, the editors and the reviewers. Any product that may be evaluated in this article, or claim that may be made by its manufacturer, is not guaranteed or endorsed by the publisher.
Acknowledgments
The authors are grateful to Ana Bela Dias and João Maurício Teixeira for all the support during the experimental trials. The authors are also very thankful to the reviewers for their constructive comments, which helped to improve the manuscript.
References
Auzoux-Bordenave S., Vigario A. M., Ruano F., Domart-Coulon I., Doumenc D. (1995). In Vitro Sporulation of the Clam Pathogen Perkinsus atlanticus (Apicomplexa, Perkinsea) Under Various Environmental Condition. J. Shellfish Res. 14, 469–475.
Befus K. M., Cardenas M. B., Erler D. V., Santos I. R., Eyre B. D. (2013). Heat Transport Dynamics at a Sandy Intertidal Zone. Water Resour. Res. 49, 3770–3786. doi: 10.1002/wrcr.20325
Booij M. J. (2005). Impact of Climate Change on River Flooding Assessed With Different Spatial Model Resolutions. J. Hydrol. 303, 176–198. doi: 10.1016/j.jhydrol.2004.07.013
Carregosa V., Velez C., Soares A. M. V. M., Figueira E., Freitas R. (2014). Physiological and Biochemical Responses of Three Veneridae Clams Exposed to Salinity Changes. Comp. Biochem. Physiol. Part - B Biochem. Mol. Biol. 177–178, 1–9. doi: 10.1016/j.cbpb.2014.08.001
Cravo A., Pereira C., Gomes T., Cardoso C., Serafim A., Almeida C., et al. (2012). A Multibiomarker Approach in the Clam Ruditapes decussatus to Assess the Impact of Pollution in the Ria Formosa Lagoon, South Coast of Portugal. Mar. Environ. Res. 75, 23–34. doi: 10.1016/j.marenvres.2011.09.012
De Marchi L., Rocha R. J. M., Rodrigues A. C. M., Soares A. M. V. M., Pretti C., Chiellini F., et al. (2020). Environmental Fate of Multistressors on Carpet Shell Clam Ruditapes decussatus: Carbon Nanoparticles and Temperature Variation. Sustain. 12, 1–15. doi: 10.3390/SU12124939
de Sousa J. T., Matias D., Joaquim S., Ben-Hamadou R., Leitão A. (2011). Growth Variation in Bivalves: New Insights Into Growth, Physiology and Somatic Aneuploidy in the Carpet Shell Ruditapes decussatus. J. Exp. Mar. Bio. Ecol. 406, 46–53. doi: 10.1016/j.jembe.2011.06.001
Deutsch C., Ferrel A., Seibel B., Pörtner H. O., Huey R. B. (2015). Climate Change Tightens a Metabolic Constraint on Marine Habitats. Science 348:1132–1135. doi: 10.1126/science.aaa1605
Domínguez R., Vázquez E., Woodin S. A., Wethey D. S., Peteiro L. G., Macho G., et al. (2020). Sublethal Responses of Four Commercially Important Bivalves to Low Salinity. Ecol. Indic. 111, 106031. doi: 10.1016/j.ecolind.2019.106031
Fenoglio S., Bo T., Cucco M., Mercalli L., Malacarne G. (2010). Effects of Global Climate Change on Freshwater Biota: A Review With Special Emphasis on the Italian Situation. Ital. J. Zool. 77, 374–383. doi: 10.1080/11250000903176497
Gharbi A., Farcy E., Van Wormhoudt A., Denis F. (2016). Response of the Carpet Shell Clam (Ruditapes decussatus) and the Manila Clam (Ruditapes philippinarum) to Salinity Stress. Biol. (Bratisl). 71, 551–562. doi: 10.1515/biolog-2016-0072
Gómez-Martinez O., Quintana P., Pichardo J. L., Alvarado-Gil J. J. (2002). Photoacoustic Determination of the Thermal Properties of Bivalve Mollusk Shells. Mar. Biol. 141, 911–914. doi: 10.1007/s00227-002-0880-z
IPCC (2021). “Summary for Policymakers,” in Climate Change 2021: The Physical Science Basis. Contribution of Working Group I to the Sixth Assessment Report of the Intergovernmental Panel on Climate Change. Eds. R. Y, Masson-Delmotte B. Z., Zhai V. ,. P., Pirani A., Connors S. L., Péan C., Berger S., Caud N., Chen Y., Goldfarb L., Gomis M. I., Huang M., Leitzell K., Lonnoy E., Matthews J. B. R., Maycock T. K., Waterfield T., Yelekçi O., Yu R., Zhou B., , (eds.) ( Cambridge University Press).
Kyoung J. A., Ki H. K. (2001). Effect of Temperature and Salinity on In Vitro Zoosporulation of Perkinsus Sp. In Manila Clams Ruditapes philippinarum. Dis. Aquat. Organ. 48, 43–46. doi: 10.3354/dao048043
Macho G., Woodin S. A., Wethey D. S., Vázquez E. (2016). Impacts of Sublethal and Lethal High Temperatures on Clams Exploited in European. Fisheries. 35, 405–419. doi: 10.2983/035.035.0215
Matias D., Joaquim S., Matias A. M., Moura P., de Sousa J. T., Sobral P., et al. (2013). The Reproductive Cycle of the European Clam Ruditapes decussatus(L. 1758) in Two Portuguese Populations: Implications for Management and Aquaculture Programs. Aquaculture 406–407, 52–61. doi: 10.1016/j.aquaculture.2013.04.030
Matias D., Joaquim S., Ramos M., Sobral P., Leitão A. (2011). Biochemical Compounds’ Dynamics During Larval Development of the Carpet-Shell Clam Ruditapes decussatus (Linnaeus 1758): Effects of Mono-Specific Diets and Starvation. Helgol. Mar. Res. 65, 369–379. doi: 10.1007/s10152-010-0230-3
Matozzo V., Marin M. G. (2011). Bivalve Immune Responses and Climate Changes : Is There a Relationship ;? ISJ 8, 70–77.
Mu G., Duan F., Zhang G., Li X., Ding X., Zhang L. (2018). Microstructure and Mechanical Property of Ruditapes philippinarum Shell. J. Mech. Behav. Biomed. Mater. 85, 209–217. doi: 10.1016/j.jmbbm.2018.06.012
Navarro J. M., Gonzalez C. M. (1998). Physiological Responses of the Chilean Scallop Argopecten Purpuratus to Decreasing Salinities. Aquaculture 167, 315–327. doi: 10.1016/S0044-8486(98)00310-X
Nossier M. A. (1986). Ecophysiological Responses of Cerastoderma Edule (L.) and C. Glaucum (Bruguière) to Different Salinity Regimes and Exposure to Air. J. Molluscan Stud. 52, 110–119. doi: 10.1093/mollus/52.2.110
Otero M., Cebrian E., Francour P., Galil B., Savini D. (2013). Monitoring Marine Invasive Species in Mediterranean Marine Portected Areas (MPAs): A Strategy and Practical Guide for Managers (Malaga, Spain: IUCN).
Parada J. M., Molares J., Otero X. (2012). Multispecies Mortality Patterns of Commercial Bivalves in Relation to Estuarine Salinity Fluctuation. Estuaries and Coasts, 35: 132–142. doi: 10.1007/s12237-011-9426-2
Philippart C. J. M., Van Aken H. M., Beukema J. J., Bos O. G., Cadée G. C., Dekker R. (2003). Climate-Related Changes in Recruitment of the Bivalve Macoma Balthica. Limnol. Oceanogr. 48, 2171–2185. doi: 10.4319/lo.2003.48.6.2171
Poulin R., Mouritsen K. N. (2006). Climate Change, Parasitism and the Structure of Intertidal Ecosystems. J. Helminthol. 80, 183–191. doi: 10.1079/joh2006341
Reverter M., Sarter S., Caruso D., Avarre J. C., Combe M., Pepey E., et al. (2020). Aquaculture at the Crossroads of Global Warming and Antimicrobial Resistance. Nat. Commun. 11, 1–8. doi: 10.1038/s41467-020-15735-6
Reyes-Martínez M. J., Martínez-Pita I., Soler-Navarro D., García-García F. J. (2020). The Impact of Salinity Changes Associated with Size on the Wedge Clam Donax trunculus Linnaeus, 1758 (Mollusca: Bivalvia): A laboratory assay. Estuar. Coast. Shelf Sci. 241, 106838. doi: 10.1016/j.ecss.2020.106838. 8:42 PM.
Ribeiro J., Monteiro C. C., Monteiro P., Bentes L., Coelho R., Gonçalves J. M. S., et al. (2008). Long-Term Changes in Fish Communities of the Ria Formosa Coastal Lagoon (Southern Portugal) Based on Two Studies Made 20 Years Apart. Estuar. Coast. Shelf Sci. 76, 57–68. doi: 10.1016/j.ecss.2007.06.001
Sarà G., Romano C., Widdows J., Staff F. J. (2008). Effect of Salinity and Temperature on Feeding Physiology and Scope for Growth of an Invasive Species (Brachidontes Pharaonis - MOLLUSCA: BIVALVIA) Within the Mediterranean Sea. J. Exp. Mar. Bio. Ecol. 363, 130–136. doi: 10.1016/j.jembe.2008.06.030
Shumway S. E. (1977). Effect of Salinity Fluctuation on the Osmotic Pressure and Na+, Ca2+ and Mg2+ Ion Concentrations in the Hemolymph of Bivalve Molluscs. Mar. Biol. 41, 153–177. doi: 10.1007/BF00394023
Sobral P., Widdows J. (1997). Effects of Copper Exposure on the Scope for Growth of the Clam Ruditapes decussatus From Southern Portugal. Mar. Pollut. Bull. 34, 992–1000. doi: 10.1016/S0025-326X(97)00116-1
Tunipex S. A. (2022). Available at: http://www.tunipex.eu/pt/information_oceanic.php (Accessed January 28, 2022). Olhão, Portugal.
Turner L. M., Alsterberg C., Turner A. D., Girisha S. K., Rai A., Havenhand J. N., et al. (2016). Pathogenic Marine Microbes Influence the Effects of Climate Change on a Commercially Important Tropical Bivalve. Sci. Rep. 6, 1–10. doi: 10.1038/srep32413
Vázquez E., Woodin S. A., Wethey D. S., Peteiro L. G., Olabarria C. (2021). Reproduction Under Stress: Acute Effect of Low Salinities and Heat Waves on Reproductive Cycle of Four Ecologically and Commercially Important Bivalves. Front. Mar. Sci. 8. doi: 10.3389/fmars.2021.685282
Velez C., Figueira E., Soares A. M. V. M., Freitas R. (2016). Native and Introduced Clams Biochemical Responses to Salinity and pH Changes. Sci. Total Environ. 566–567, 260–268. doi: 10.1016/j.scitotenv.2016.05.019
Verdelhos T., Marques J. C., Anastácio P. (2015a). Behavioral and Mortality Responses of the Bivalves Scrobicularia Plana and Cerastoderma Edule to Temperature, as Indicator of Climate Change’s Potential Impacts. Ecol. Indic. 58, 95–103. doi: 10.1016/j.ecolind.2015.05.042
Verdelhos T., Marques J. C., Anastácio P. (2015b). The Impact of Estuarine Salinity Changes on the Bivalves Scrobicularia Plana and Cerastoderma Edule, Illustrated by Behavioral and Mortality Responses on a Laboratory Assay. Ecol. Indic. 52, 96–104. doi: 10.1016/j.ecolind.2014.11.022
Villalba A., Casas S. M., López C., Carballal M. J. (2005). Study of Perkinsosis in the Carpet Shell Clam Tapes Decussatus in Galicia (NW Spain). II. Temporal Pattern of Disease Dynamics and Association With Clam Mortality. Dis. Aquat. Organ. 65, 257–267. doi: 10.3354/dao065257
Villalba A., Reece K. S., Ordás M. C., Casas S. M., Figueras A. (2004). Perkinsosis in Molluscs: A Review. Aquat. Living Resour. 17, 411–432. doi: 10.1051/alr:2004050
Woodin S. A., Wethey D. S., Olabarria C., Vázquez E., Domínguez R., Macho G., et al. (2020). Behavioral Responses of Three Venerid Bivalves to Fluctuating Salinity Stress. J. Exp. Mar. Bio. Ecol. 522, 151256. doi: 10.1016/j.jembe.2019.151256
Zgouridou A., Tripidaki E., Giantsis I. A., Theodorou J. A., Kalaitzidou M., Raitsos D. E., et al. (2022). The Current Situation and Potential Effects of Climate Change on the Microbial Load of Marine Bivalves of the Greek Coastlines: An Integrative Review. Environ. Microbiol. 24, 1012–1034. doi: 10.1111/1462-2920.15765
Keywords: European clam, heavy rainfalls, heatwaves, mortality, feeding behavior
Citation: Rato A, Joaquim S, Matias AM, Roque C, Marques A and Matias D (2022) The Impact of Climate Change on Bivalve Farming: Combined Effect of Temperature and Salinity on Survival and Feeding Behavior of Clams Ruditapes decussatus. Front. Mar. Sci. 9:932310. doi: 10.3389/fmars.2022.932310
Received: 29 April 2022; Accepted: 21 June 2022;
Published: 22 July 2022.
Edited by:
Sílvia Lourenço, Instituto Politécnico de Leiria, PortugalReviewed by:
Bin Xia, Qingdao Agricultural University, ChinaRula Dominguez, Centro Tecnológico del Mar (CETMAR), Spain
Copyright © 2022 Rato, Joaquim, Matias, Roque, Marques and Matias. This is an open-access article distributed under the terms of the Creative Commons Attribution License (CC BY). The use, distribution or reproduction in other forums is permitted, provided the original author(s) and the copyright owner(s) are credited and that the original publication in this journal is cited, in accordance with accepted academic practice. No use, distribution or reproduction is permitted which does not comply with these terms.
*Correspondence: Ana Rato, ana.rato@ipma.pt