- 1Research Center for Harmful Algae and Marine Biology, Jinan University, Guangzhou, China
- 2Key Laboratory of Eutrophication and Red Tide Prevention of Guangdong Higher Education Institutes, Jinan University, Guangzhou, China
A harmful benthic Prorocentrum concavum bloom was recorded in August 2018 in Xincun Bay, China, which is the location of a national seagrass nature reserve. Annual ecological surveys have been conducted to study the population dynamics of P. concavum in the benthic community and water column. Seasonal variations in benthic P. concavum abundance were found and the abundances on seagrass and macroalgae in the wet season were 2.5 and 2.82 times higher, respectively, than those in the dry season, although the differences were not statistically significant. The abundance of P. concavum in the water column differed significantly between seasons. The maximum abundances of benthic and planktonic P. concavum were (1.7 ± 0.59) × 106 cells (100 cm2)−1 on Thalassia hemperichii in July and 2.0 × 104 ± 4.7 × 103 cells L−1 in June, respectively. High spatial heterogeneity in P. concavum abundance was observed among five sampling sites. Abundances were significantly higher in seagrass beds than those in macroalgae beds, mangroves, and coral reefs. The abundance of P. concavum at site A (in a seagrass bed and close to a cage-culture area) was 5.6 times higher than that at site D (seagrass bed and distant from the cage-culture area). Planktonic P. concavum showed a similar spatial distribution and presented a maximum density at site A. Moreover, the abundance of benthic P. concavum also showed heterogeneity on host substrates, and the abundance on T. hemperichii was significantly higher than that on sediment. Based on a Spearman’s test, temperature, dissolved organic phosphorus, and dissolved organic nitrogen were the three important factors driving the spatiotemporal distribution of benthic P. concavum in Xincun Bay. Planktonic P. concavum were derived from cells on the substrates and were influenced by concentrations of dissolved oxygen. In conclusion, seagrass beds may be a reservoir of harmful benthic algal blooms in Xincun Bay and the dense cage-culture area provides sufficient organic nutrients for the growth and reproduction of benthic dinoflagellates.
1 Introduction
Benthic Prorocentrum is a harmful benthic dinoflagellate group, which is widely distributed from tropical to temperate zones (Hoppenrath et al., 2013; Luo et al., 2017; Chomérat et al., 2019; Zou et al., 2021). To date, nine species of epibenthic Prorocentrum have been identified that produce okadaic acid (OA), and/or its analogs (Dickey et al., 1990; Morton et al., 1998; Ten-Hage et al., 2002; An et al., 2010; Rodríguez et al., 2018; Nishimura et al., 2020). This toxin causes diarrhea, vomiting, abdominal pain, and nausea in humans that ingest shellfish contaminated by OA (Toyofuku, 2006), which called as diarrhetic shellfish poisoning (DSP). In extreme cases, gastric cancer can result from chronic exposure to OA (Aune and Yndestad, 1993). Also, harmful benthic Prorocentrum blooms have been increasingly reported in recent decade (Koike, 2013; Turkoglu, 2016; Cicily et al., 2020), which represents a concerning issue for marine benthic ecosystems and public health (Zou et al., 2020).
Considering the threat of toxic benthic Prorocentrum to marine benthos and humans, ecological studies related to blooms, including the spatial-temporal dynamics of their distribution and relationships with environmental parameters, should be clarified. Significant spatial and temporal distributions of benthic Prorocentrum have been observed, and these distributions are often related to environmental factors including temperature, nutrients, and depth (Glibert et al., 2012; Hachani et al., 2018; Gharbia et al., 2019). Distinct seasonal distribution characteristics are also observed in benthic Prorocentrum (mainly P. concavum and P. lima), with a high abundance in the wet season (Tindall and Morton, 1998). The results of a two-year study in the Mediterranean Sea showed a high abundance of P. lima in the summer (July to October) when high temperatures were observed (27–30°C) (Aissaoui et al., 2014). An 18-month survey at two tropical islands in the Caribbean Sea showed significant spatial heterogeneity between the islands and among different sites within islands (Boisnoir et al., 2019). In the Gulf of Tunis, Mediterranean Sea, the abundance of benthic Prorocentrum, which was related to nutrients, decreased with depth and the maximal abundance was observed at a depth of 0.5–1.5 m (Hachani et al., 2018). However, an investigation explored the vertical distribution of benthic dinoflagellates in the Caribbean Sea in the dry season and then showed the highest density of benthic Prorocentrum at 1.5 m depth, which differed significantly from the abundance at 3 m depth (Boisnoir et al., 2018). A previous study showed that the highest abundances of Prorocentrum occurred at 7–8 m depth and no significant differences were observed between different depths at 20 m in the wet season (Boisnoir et al., 2018). Overall, the distribution of benthic Prorocentrum is a complicated ecological process and is correlated with different environmental parameters.
As mentioned above, the development of benthic Prorocentrum is a complicated phenomenon related to environmental parameters, including both physical and chemical factors. Previous ecological studies discovered that hydrological parameters can affect the growth and toxin production of benthic Prorocentrum (Aissaoui et al., 2014; Accoroni et al., 2018; Aquino-Cruz et al., 2018). For example, the proliferation of P. lima was positively related to temperature, salinity, and dissolved oxygen in a two-year survey in the coastal waters of the Gulf of Tunis (Aissaoui et al., 2014). Similarly, a field survey of the epiphytic abundance of P. lima found that density increased on most macrophytes from April to August (summer) in a lagoon in the UK (Foden et al., 2005). Climate change may expand the geographic distribution of benthic dinoflagellates (Tester et al., 2010). Surveys of the dynamics of benthic Prorocentrum in relation to temperature, to some extent reflect the influences of global warming on the benthic ecosystem. Chemical factors (nutrients) also play an essential role in the proliferation of benthic Prorocentrum (Glibert et al., 2012; Aissaoui et al., 2014). Numerous studies about the physiology of benthic Prorocentrum in the laboratory have found that this dinoflagellate shows preferences for certain forms and ratios of nutrients (Glibert et al., 2012). P. lima from Mahone Bay, Canada, preferentially consumed ammonium over nitrate and nitrite (Pan et al., 1999). Similarly, in situ studies revealed that benthic Prorocentrum had nutrient preferences (Aissaoui et al., 2014), and the occurrence of P. lima blooms was strongly correlated with nutrients in the Gulf of Tunis (Hachani et al., 2018). However, except for P. lima, little is known about the interactions of noxious benthic Prorocentrum with variations in environmental factors.Benthic dinoflagellates can live and/or attach to the surface of seagrass, macroalgae, and sediment based on their flagellates or mucus (García-Portela et al., 2016), and they can also swim in the water column (Gharbia et al., 2019; Zou et al., 2020). Many previous studies have revealed substrate preferences of benthic Prorocentrum (Boisnoir et al., 2019; Gharbia et al., 2019). However, a number of reports found that the three-dimensional architecture of hosts can affect the attachment of benthic dinoflagellates, and those substrates with flexible and complex structures are most suitable (Accoroni and Totti, 2016). Therefore, differences in abundance should not be compared based on a universal unit (i.e., cells g−1 fresh or dry weight macrophytes); the sampling of benthic dinoflagellates needs to be standardized and eliminate the influence of host architecture (Berdalet et al., 2017). Tester et al. (2014) firstly applied a scientific method of artificial screening to investigate the abundance of benthic dinoflagellates. However, this approach is not suitable for some studies, such as substrate preferences. Cells per square 100 centimeters [cells (100 cm2)−1] is a more reliable unit and fully considers the substrate structure (Tester et al., 2014; Berdalet et al., 2017).
Prorocentrum concavum, a tychoplanktonic dinoflagellate, was first described on a coral reef in French Polynesia by Fukuyo (1981). Among nine toxic epibenthic Prorocentrum, results on the production of OA by P. concavum are often contradictory. Some studies report that this dinoflagellate can produce OA (Dickey et al., 1990; Hu et al., 1992; Juranovic et al., 1997), while other recent studies demonstrated no detectable OA using liquid chromatography-tandem mass spectrometry (Luo et al., 2017; Verma et al., 2019). Despite the debate regarding whether P. concavum produces OA or not, this species is certainly a toxic dinoflagellate and can be lethal to marine invertebrates (Zou et al., 2020). As mentioned by Morton et al. (2002), a red tide of P. arabianum [synonymized with P. concavum by Mohammad-Noor et al. (2007)] was collected from plankton samples but there were no data on the abundance in either the plankton or benthos. In August 2018, a toxic and tychoplanktonic P. concavum bloom was detected in a seagrass bed in Xincun Bay, Hainan Island, South China Sea, with high abundances observed on seagrasses and macroalgae, and in the water column (Zou et al., 2020). This bloom presented a suitable model to analyze the population dynamics of toxic benthic Prorocentrum. The present study aims to determine the spatiotemporal distribution of P. concavum and its relationship with environmental factors in a coastal tropical lagoon, to improve our knowledge of the ecology of harmful benthic Prorocentrum blooms.
2 Materials and Methods
2.1 Study Area
The study area, Xincun Bay (18°24′–18°27′N, 109°58′–110°58′E), is a tropical coastal lagoon situated in the southeast of Hainan Island, China (Figure 1). The lagoon has a high annual temperature and abundant rainfall. The bay (~22.6 km2) has a narrow canal connection to the open sea (Yang et al., 2017), hence, the water flow is driven by daily tidal movements. The largest fish aquaculture area (~0.05 km2) on Hainan Island is located in the bay, with annual production of 1105 tons. A mixed seagrass meadow (2 km2) is situated in the shallow waters of southeast Xincun Bay, based on observation in 2002 (Huang et al., 2006). Enhalus acoroides, Thalassia hemprichii, and Cymodocea rotundata are the main species in the meadow, and the former two dominate (Figure 2). Sampling was carried out at stations A, B, C, and D in Xincun lagoon and an outside station (E; Figure 1). Stations A and D were situated in the southwest of the lagoon where seagrasses grow; station A was close to an aquaculture area and station D was located some distance away (Figure 1). Stations B and C were located in the northeast and northwest of the lagoon, respectively. Macroalgae, but no seagrasses, were present at station B during the study period, and the other site (C) was in a mangrove area with no seagrasses or macroalgae. Station E (reference site) was on a coral reef located outside the Xincun lagoon. This station was relatively devoid of human activities.
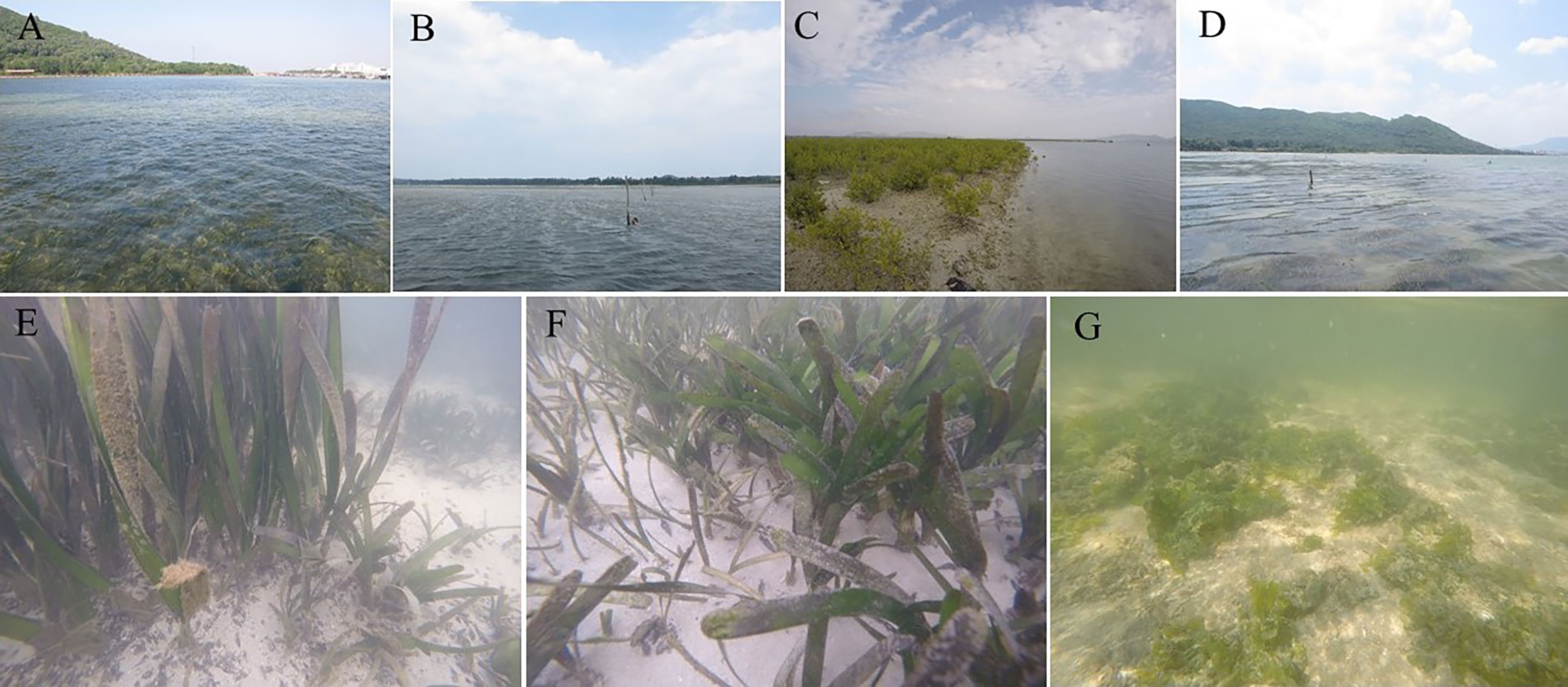
Figure 2 Sampling stations in Xincun Bay. (A–D) represented sampled stations (A) (seagrass bed), (B) (macroalgae meadow), (C) (mangrove) and (D) (seagrass bed), respectively. E–G represented Enhalus acoroides, Thalassia hemperichii and Cymodocea rotundata, Ulva lactuca, respectively.
2.2 Sample Collection
Sampling was conducted bimonthly from December 2018 to December 2019 in the Xincun lagoon and once a month in the summer of 2019 as a result of the P. concavum bloom present at station A in August 2018 (Zou et al., 2020). The wet season ranged from May to October and the other months were the dry season in the tropical Xincun Bay. At stations A and D, each type of seagrass, macroalga (Table 1), and sediment was collected in triplicate. Macroalgal and sediment samples (triplicate) were collected at station B, but only sediment was sampled (in triplicate) at stations C and E. In addition, samples from the water column were also collected in triplicate at each station. At each station, well-developed floating leaves on which benthic dinoflagellates attach were slightly cut and then placed in sealed bags containing a small amount of the surrounding seawater. The cells of benthic dinoflagellate on the sediment were sampled based on the method of Xie et al. (2022). Briefly, the surface sediments (~300 cm-2) were collected in the bags with 1 L surrounding seawaters. A 1.5 L water column (triplicate samples) was sampled using a 1 L white plastic bottle. The samples were collected between 0.2 and 1.5 m depth within the lagoon and between 2 and 3 m depth in the open sea (Table 1).
2.3 Environmental Parameters
Surface water temperature (T), pH, salinity (Sal), and dissolved oxygen (DO) were measured using a YSI meter (YSI-professional plus, YSI Inc., USA) at each station during the sampling period. Each 0.1 L water column sample collected from each station was filtered with GF/F filters (Whatman, USA). The concentrations of nitrate (NO3), nitrite (NO2), ammonium (NH4), silicate (SiO3), phosphate (PO4), total dissolved nitrogen (TDN), and total dissolved phosphorus (TDP) were measured using a flow injection analyzer (LACHAT QC8500, USA) (Murphy and Riley, 1962; Solrzano, 1969; Strickland and Parsons, 1972; Jeffries et al., 1979). In addition, dissolved organic nitrogen (DON) and dissolved organic phosphorus (DOP) were calculated by subtracting the concentrations of dissolved inorganic nitrogen and PO4 from TDN and TDP, respectively.
2.4 Sample Processing
The seagrass, macroalgal, and sediment samples were shaken vigorously and washed three times using filtered water to ensure microalgal cells were separated from the substrate. Benthic dinoflagellates were concentrated using 10 μm nylon filters and then saved in 15 mL centrifuge tubes containing acidic Lugol’s solution (final concentration, 1.5%). The biological substrates (seagrasses and macroalgae) were removed and then weighed. Similarly, the 1 L water column samples were concentrated and reserved in 15 mL centrifuge tubes containing a suitable concentration of acidic Lugol’s solution.
In addition to weighing, the biological substrates were photographed with a DSLR camera and the surface area was measured using Image Pro Plus V. 7.0. In the present study, at least ten weights and surface areas of every substrate were recorded to establish standard curves for the surface area and weight.
2.5 Benthic Dinoflagellate Counts
Samples were quickly transferred to the laboratory and counted using a light microscope (Olympus CX31, Tokyo, Japan) at a magnification of 100×. The morphology of the bloom-forming species, P. concavum, was obvious and easy to identify. Other epibenthic dinoflagellate species were extremely similar and were recorded as genera (Prorocentrum, Coolia, Amphidinium, Gambierdiscus, and Ostreopsis). The abundances of benthic dinoflagellates were expressed as cells (100 cm2)-1 and cells L−1 on substrate and in the water column, respectively. In addition, we defined more than 1×105 cells (100 cm2)-1 as benthic P. concavum bloom based on the results described by Zou et al., 2020.
2.6 Statistical Analysis
All abundances of P. concavum were displayed as means ± standard error. Analysis of seasonal variations in P. concavum in Xincun Bay was restricted to data collected from the seagrass meadow (stations A and D) to avoid errors resulting from the null data collected from the other sites. One-way ANOVA was used to assess the distributions of environmental factors and assess P. concavum abundances among five stations and between two seasons. Non-parametric tests were carried out when the variances were not homogenous. Spearman correlation tests were used to assess the relationships between planktonic and benthic P. concavum and the effects of environmental factors on the development of the P. concavum population. Aall analyses were performed using SPSS Statistics 25 (IBM Corp., USA).
3 Results
3.1 Environmental Factors
Figure 3 showed the spatiotemporal distributions of the environmental factors. Temperature fluctuated from 21.3°C in December 2019 at station B to 32.8°C in July at station B (Figure 3A). The average temperatures of the dry and wet seasons were 26.36°C and 29.32°C, respectively. Salinity ranged from 29.08 to 32.59, with an average value of 31.61 (Figure 3B). pH and concentrations of DO ranged from 7.8 to 8.85 and 3.48 mg L−1 (50.2% of saturation level) to 12.62 mg L−1 (182% of saturation level), respectively (Figures 3C, D). No significant differences were discovered among the five sampling stations for these physical factors (p = 0.814, p = 0.478, p = 0.969, and p = 0.283 for temperature, salinity, pH, and DO, respectively). However, the temperature (Mann-Whitney U test, p = 0.002) and salinity (Mann-Whitney U test, p < 0.01) fluctuated significantly between dry and wet seasons. There were no seasonal differences in pH (Mann-Whitney U test, p = 0.909) or DO concentration (Mann-Whitney U test, p = 0.192).
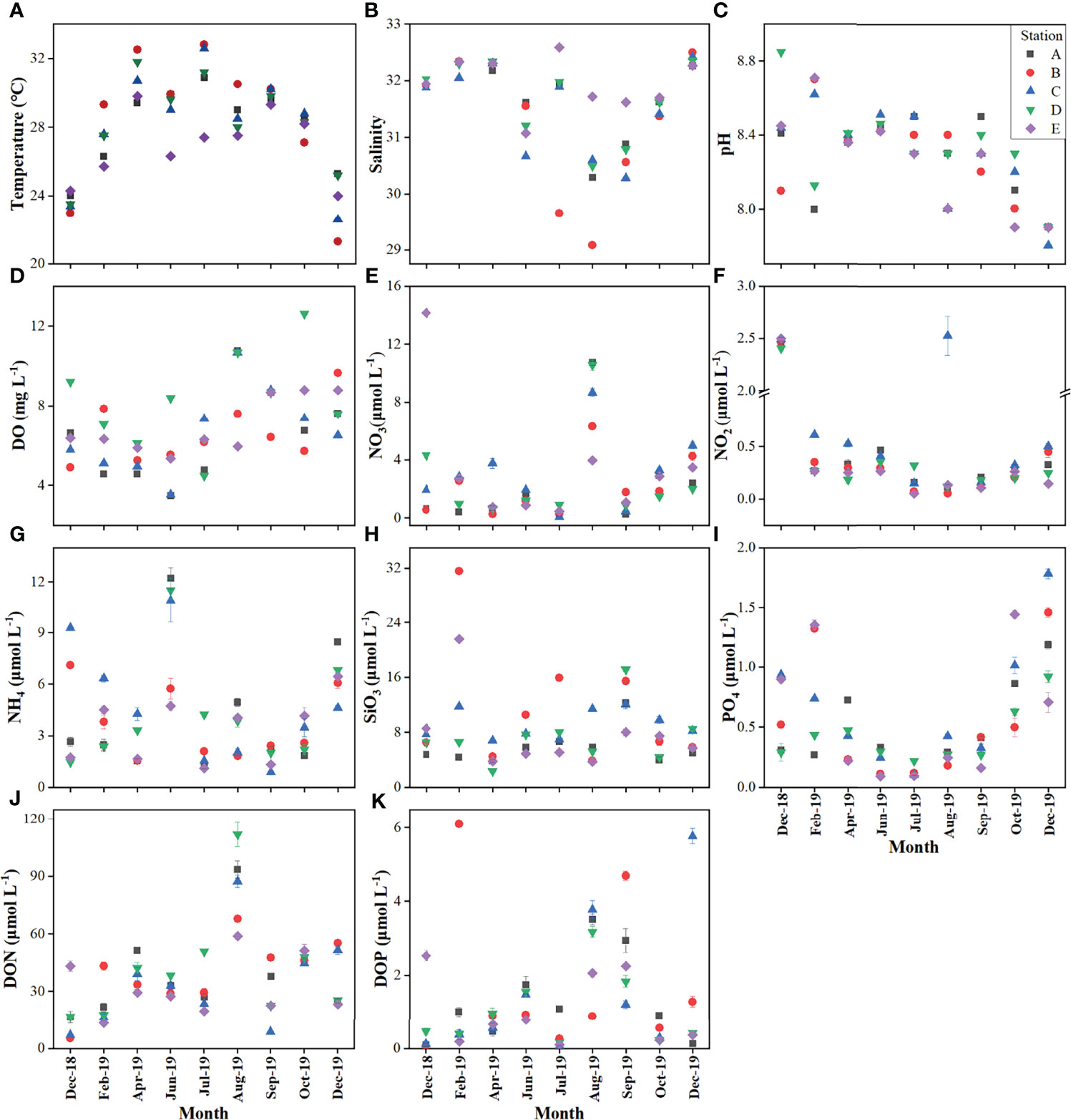
Figure 3 Spatial-temporal variations of environmental parameters during the sampling period. (A) Temperature; (B) Salinity; (C) pH; (D) dissolved oxygen; (E) Nitrate; (F) Nitrite; (G) Ammonium; (H) Silicate; (I) phosphate; (J) dissolved organic nitrogen; (K) dissolved organic phosphorus.
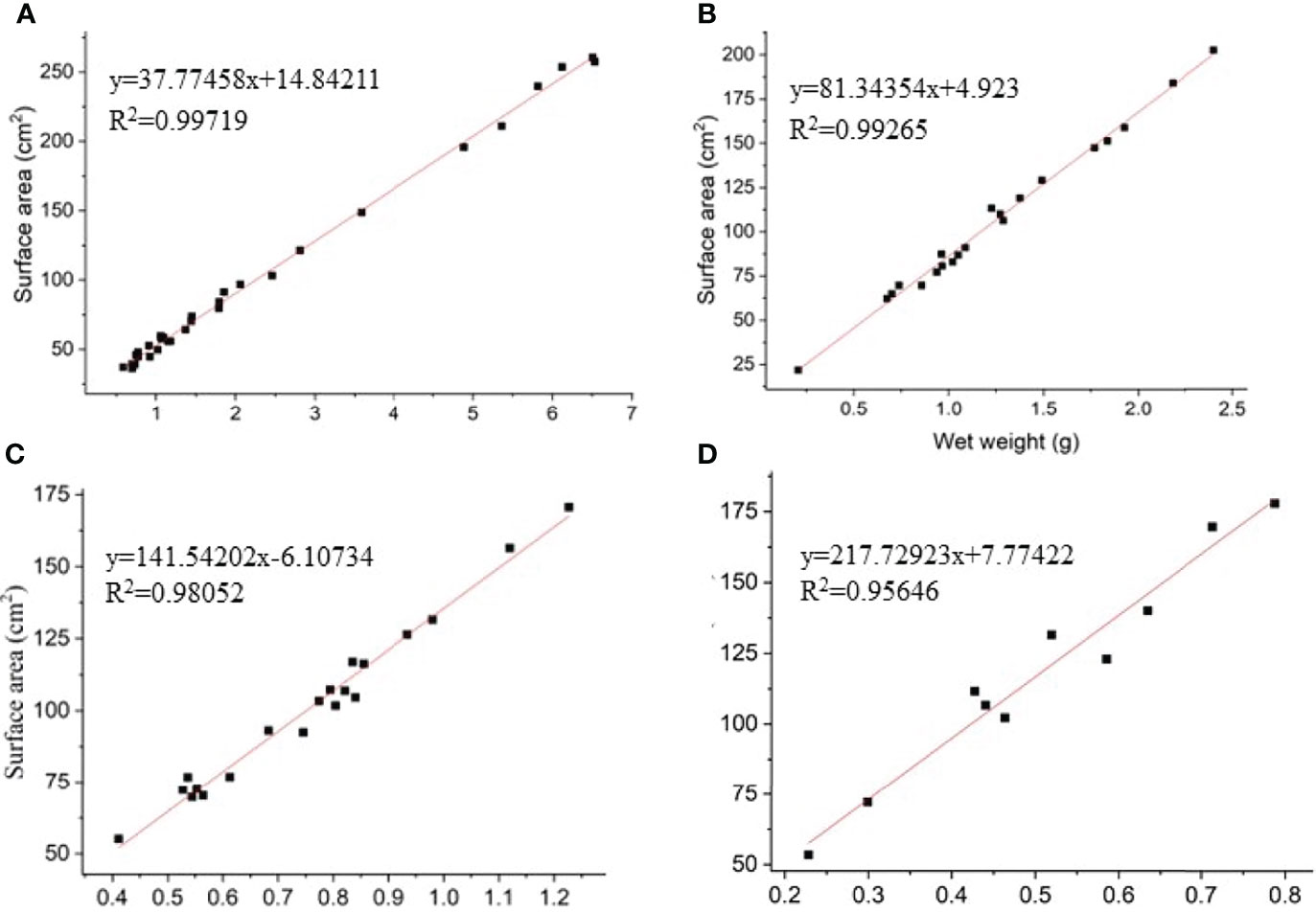
Figure 4 The linear curves and equations between the surface area and weight of Enhalus acoroides(A), Thalassia hemperichii(B), Cymodocea rotundata (C) and Ulva lactuca(D).
In terms of the nutrient levels in Xincun Bay, concentrations of nitrate ranged from 0.06 to 14.16 μmol L−1, with an average of 2.66 ± 3.07 μmol L−1 (Figure 3E). The concentrations of nitrite and ammonium ranged from 0.05 at station B to 2.53 μmol L−1 at station C, and from 0.89 at station C to 12.21 μmol L−1 at station A, respectively (Figures 3F, G). The mean concentrations of silicate and phosphate were 8.24 ± 5.32 and 0.54 ± 0.43 μmol L−1 (Figures 3H, I). DON and DOP showed a wide range of concentrations, from 5.37 to 111.82 μmol L−1 (means of 28.85 ± 15.21 and 44.26 ± 24.45 μmol L−1 in the dry and wet seasons, respectively) and from 0.06 to 6.09 μmol L−1 (means of 1.17 ± 1.73 and 1.47 ± 1.29 μmol L−1 in the dry and wet seasons, respectively), respectively. Each nutrient showed a similar range of values among the five sampling stations, except silicate concentrations, which were significantly higher at stations B and C than those at stations A and E (Kruskal-Wallis test, p < 0.01). Concerning the seasonal variations, significant differences were only observed for concentrations of nitrite, phosphate, and DON (Mann-Whitney U test, p < 0.01).
3.2 Population Dynamics of P. concavum
3.2.1 Linear Curve Between Surface Area and Weight of Macrophytes
The surfaces of four biotic substrates (three seagrasses, E. acoroides, T. hemperichii, C. rotundata; one macroalga, Ulva lactuca) were smooth and correctly measured in the Xincun lagoon. The linear curves and equations between surface area and weight were presented in Figure 4.
3.2.2 Seasonal Variations in P. concavum
The seasonal distribution of P. concavum was shown based on the data from seagrasses, macroalgae, sediments, and the water column in the seagrass bed (stations A and D, Figure 5). P. concavum was found on seagrasses throughout the sampling period in the Xincun Bay, with abundances ranging from 468 ± 134 on E. acoroides in December 2018, to (1.7 ± 0.59) × 106 cells (100 cm2)-1 on T. hemperichii in July (Figure 5). Moreover, the epibenthic abundances on U. lactuca and sediments varied from 230 ± 109 in December 2019, to (2.1 ± 1.2) × 105 cells (100 cm2)-1 in August, and from (7.3 ± 4.5) × 103 in February to (6.7 ± 1.4) × 105 cells (100 cm2)-1 in September, respectively (Figure 5). The first P. concavum bloom occurred in February on T. hemperichii at station A, with up to (3.4 ± 0.27) × 105 cells (100 cm2)-1 at a temperature of 26.3°C (Figures 3A, 5). Although no significant differences were found between the wet and dry seasons (ANOVA, p = 0.383, p = 0.252, and p = 0.864 for P. concavum on seagrasses, macroalgae, and sediments, respectively), the abundances of benthic P. concavum on seagrasses and macroalgae in the wet season were 2.52 and 2.8 times, respectively, greater than those in the dry season. Similarly, the presence of P. concavum was observed in the water column throughout the sampling period. The abundances of P. concavum ranged from 208 ± 295 in December to (2.0 ± 0.47) × 104 cells L−1 in June 2019 in the seagrass bed (Figure 5). At station A, the planktonic abundance of P. concavum increased from February to June (maximum abundance) and then declined continuously. At station D, the abundances were lower than those at Station A and the maximum abundance was observed in August (Figure 5). The average abundance of planktonic P. concavum based on the nine-month survey revealed marked differences between the two seasons (Mann Whitney U test, p = 0.02; abundance 2.3 times higher in the wet season than that in the dry season).
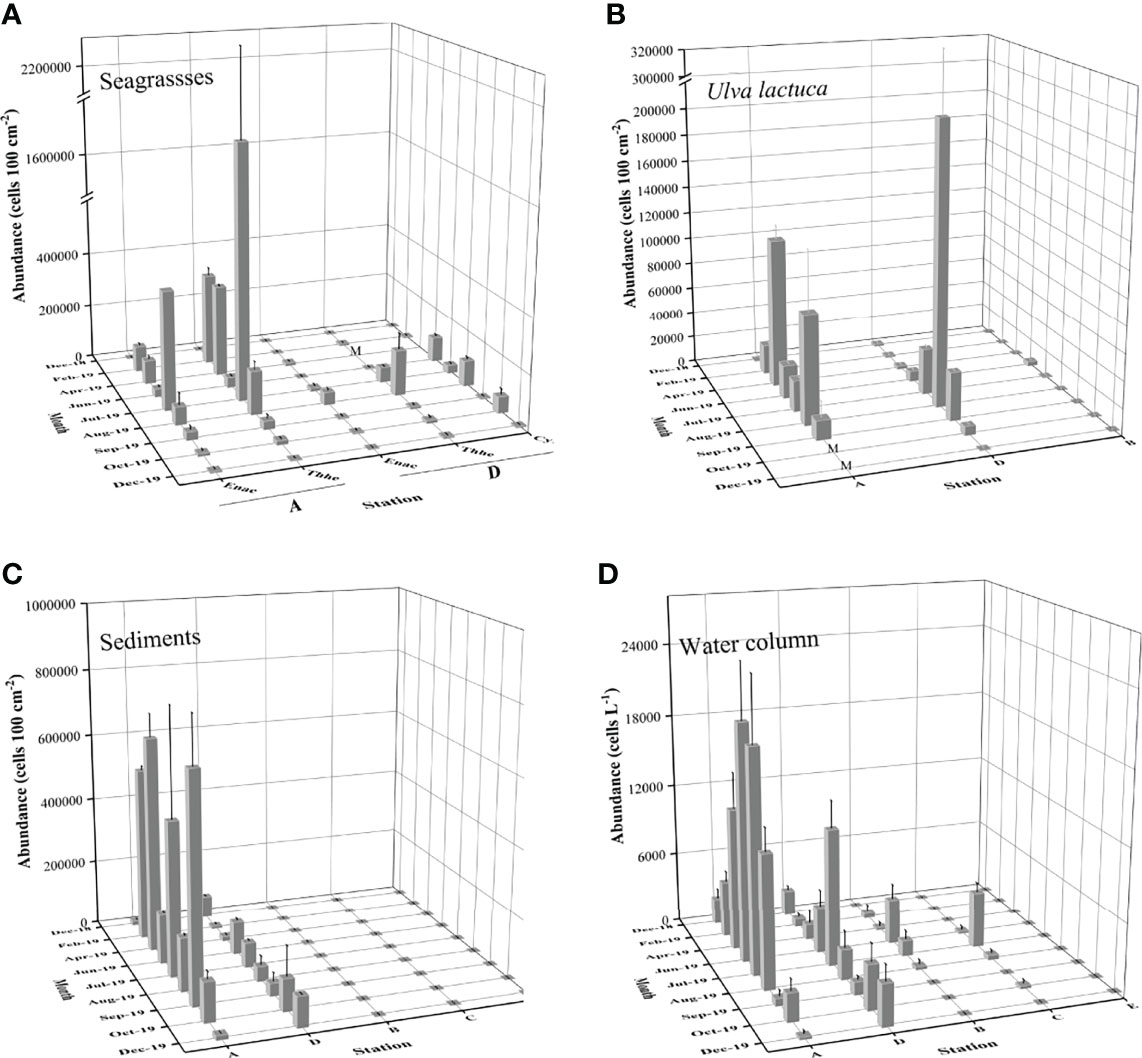
Figure 5 Spatial-temporal variations of mean P. concavum abundance with standard bar on seagrasses, macroalgae, sediments and in the water column in the Xincun Bay. M represents the missing data Spatial-temporal variations of P. concavum abundance with standard bar on seagrasses (A), macroalgae (B), sediments (C) and in the water column (D) in the Xincun Bay. M represents the missing data.
3.2.3 Spatial Distribution
High spatial heterogeneity was found among the four habitats (seagrass, macroalgae, mangrove, and coral reef) in Xincun Bay (Figures 5, 6). The mean abundances of benthic P. concavum based on the nine-month survey showed significant differences among the five sampling stations (Kruskal-Wallis test, p < 0.01). The densities in the seagrass meadow (stations A and D) were markedly higher than those in the macroalgal bed (station B), mangrove (station C), and reference site (coral reef, station E) (Figure 6A; Kruskal-Wallis test, p < 0.01).
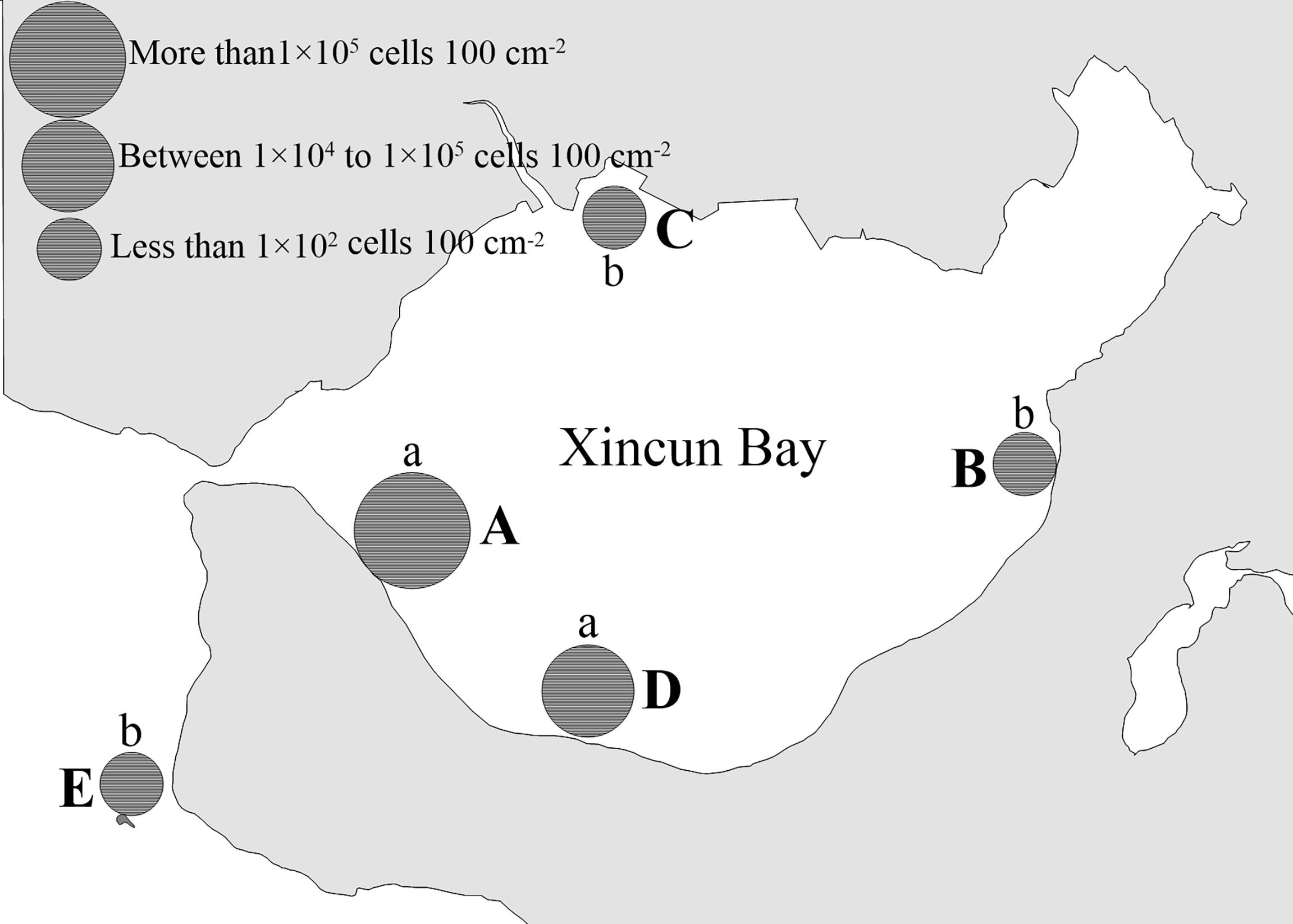
Figure 6 Total mean density of benthic P. concavum presented in Xincun Bay from December 2018 to December 2019. Small letters (a and b) indicated statistically differences according to the non-parameters test (p<0.01). A, B, C, D and E represent sampling Site A,Site B, Site C, Site D and Site E, respectively.
Although there was no statistically significant difference (Kruskal-Wallis test, p = 0.591), approximately 5.6 times more P. concavum was found at station A than at station D (Figure 6). Also, we identified other benthic dinoflagellates, including species of Prorocentrum, Coolia, Ostreopsis, Gambierdiscus, and Amphidinium in Xincun Bay during the sampling period. The ecological dominance of these five genera in the benthic dinoflagellate community could be seen in Table 2. Benthic P. concavum was the most abundant species in the seagrass meadow, with proportions ranging from 42.56% to 59.88%; at stations B and C, the proportions were only 7.82% and 0.25%, respectively, of the benthic dinoflagellate community. P. concavum also dominated in the detached epibenthic dinoflagellates in the water column, with ecological dominance fluctuating from 15.25% to 68.75% (Table 2). Statistically significant differences in planktonic P. concavum abundances were found among the five sites (Figure 6; Kruskal-Wallis test, p < 0.01). Similar to the benthic distribution pattern, proportions of planktonic P. concavum in waters of the seagrass bed were higher than those in the waters of the macroalgal bed (station B), mangrove area (station C), and coral reef (station E). No significant differences in planktonic cells were found between the two seagrass sites, but abundances at site A were 2.4 times greater than those at site D (Figure 6).
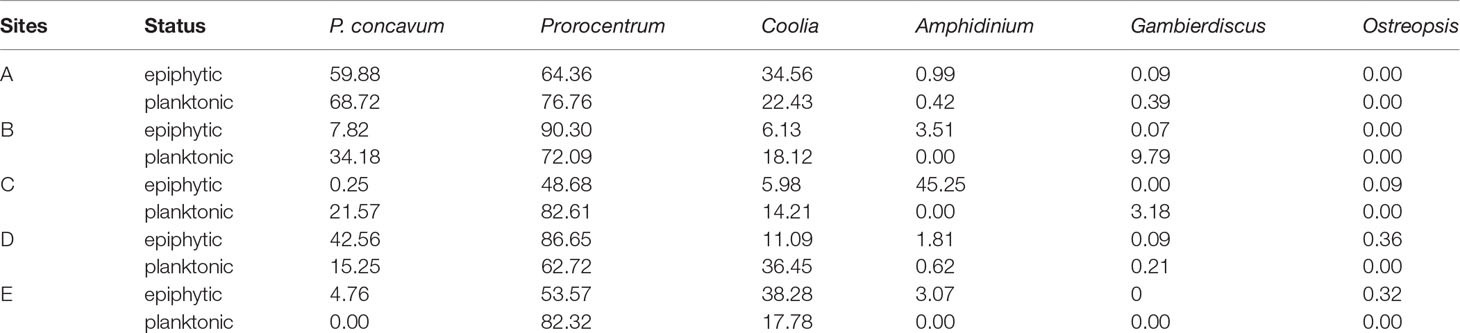
Table 2 Ecological dominance (%) of benthic dinoflagellates attached on the substrate and in the water column in Xincun Bay during sampling period.
In addition to the differences among stations, high heterogeneity in P. concavum abundance on different substrates was also observed, as shown by the high standard errors calculated from the replicates (Figures 5, 7). Mean abundances of P. concavum on seagrasses (E. acoroides, T. hemperichii and C. rotundata), macroalgae (U. lactuca), and sediments were (4.6 ± 2.4) × 104, (1.7 ± 1.1) × 105, (3.6 ± 0.14) × 105, (2.4 ± 0.96) × 104, and (7.3 ± 2.5) cells (100 cm2)-1, respectively (Figure 7). Moreover, the differences in P. concavum on each substrate were compared based on the data collected for the seagrass bed. Mean abundances of P. concavum on these substrates showed significant variations (Kruskal-Wallis test, p = 0.014), and pairwise comparisons showed that the abundance of epiphytic P. concavum (on Thalassia hemperichii) was significantly higher than that of epipelic P. concavum (p = 0.029).
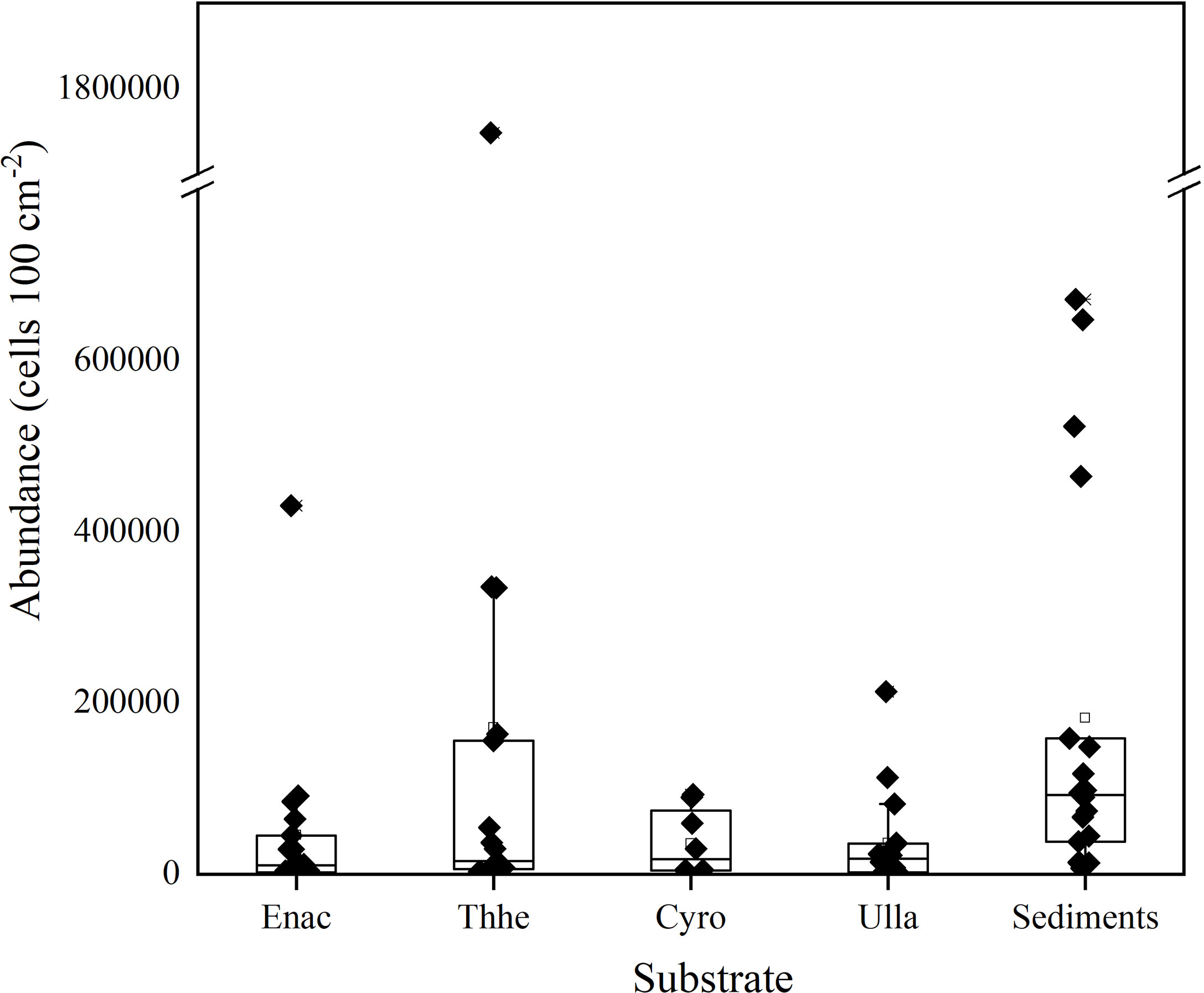
Figure 7 The boxplot showing the epiphytic and epipelic abundances of P. concavum. Enhalus acoroides (Enac); Thalassia hemperichii (Thhe); Cymodocea rotundata (Cyro); Ulva lactuca (Ulla).
3.3 Relationship Between Benthic and Planktonic P. Concavum
P. concavum on seagrasses (E. acoroides, T. hemperichii, and C. rotundata), macroalgae (U. lactuca), and sediments were all positively correlated with the abundance of planktonic cells (Table 3) and the cells on T. hemperichii, U. lactuca, and sediments were clearly related to floating P. concavum (p < 0.01).
3.4 Relationships Between Environmental Factors and P. Concavum Abundance
Over the sampling period, benthic and planktonic abundances of P. concavum were positively correlated with temperature, except the cells on C. rotundata and sediments (Table 3). The maximal abundances of P. concavum on C. rotundata and sediment occurred in August and April, with temperatures of 29.6°C and 29°C, respectively (Figures 3A, 5) but no significant relationships were found. In addition, the Spearman test revealed that concentrations of DO were weakly negatively associated with planktonic P. concavum, whereas no significant relationship was found between DO and epiphytic cells (Table 3). Considering nutrients, the Spearman test showed that densities of P. concavum on E. acoroides, T. hemperichii, C. rotundata and U. lactuca were clearly positively related to DON. The abundances on E. acoroides, U. lactuca and sediment were positively correlated with DOP. Moreover, the abundances of P. concavum on E. acoroides and T. hemperichii were negatively correlated with nitrite and ammonium, and the densities of P. concavum on U. lactuca were negatively associated with nitrite and phosphate (Table 3). Finally, temperature was positively correlated with pH and DON, but negatively associated with salinity, nitrate, ammonium, and phosphate (Table 3).
4 Discussions
4.1 Quantitative Methodology for Benthic Dinoflagellates
Compared with planktonic microalgae, standard methods for the sampling and quantification of benthic dinoflagellates that provide researchers with comparable data among studies are lacking (Berdalet et al., 2017). To date, the universal unit expressing the abundance of benthic dinoflagellates is based on the wet or dry weight of substrates (macrophytes). However, this expressive method of abundance not only neglects the three-dimensional structure of host species but is also unsuitable for sampling stations with a lack of biotic substrates. Benthic dinoflagellates attach on the surface of biotic substrates based on the flagellum and/or produced mucus (Heil, 1993; Reynolds, 2007). The different biotic substrates have obviously various three dimensional structures, which provide different surface area for the attachments of benthic dinoflagellate (Berdalet et al., 2017). For example, surface area per gram were 53 and 225 cm2 in E. acoroides and U. lactuca in Xincun Bay (Figure 4). In the present study, the area unit, cells (100 cm2)-1, was employed and standard curves between the surface area of hosts and fresh weight were established. This method provides a convenient way to calculate a host’s surface area and for comparison among studies. Tester et al. (2014) first used artificial substrates (fiberglass screen) to investigate the abundance of benthic dinoflagellates, which attaches great importance to the use of cells (100 cm2)-1 in filed studies. However, this method is not applicable to some studies that explore the relationships (e.g., substrate preferences) between benthic cells and hosts (Boisnoir et al., 2019; Gharbia et al., 2019). In the present study, a field method was established to quantify the densities of epipelic dinoflagellates. This method provides researchers with a way to compare epiphytic and epipelic abundances. While this method has certain limitations, it provides new insight into surveys of benthic dinoflagellates.
4.2 Spatiotemporal Distribution of P. concavum
P. concavum, a harmful dinoflagellate, was first described by Fukuyo (1981) in French Polynesia, New Caledonia, and the Ryukyu Islands. To date, many studies have demonstrated that P. concavum is a tychoplanktonic dinoflagellate. This species has been reported in the Arabian Sea (Morton et al., 2002), Knight Key, Gulf of Mexico, northwestern Australia (Verma et al., 2019), northern Hainan Island (Luo et al., 2017), and the Xincun Bay of China (present study). Moreover, Morton et al. (2002) described a new species, P. arabianum [synonym of P. concavum; Mohammad-Noor et al. (2007)], and reported a planktonic P. arabianum bloom in the Gulf of Oman, Arabian Sea in May 1995. In spite of a lack of cell abundance data for this bloom, there was evidence that P. concavum was a bloom-causative species, which may be harmful to marine ecosystems and public health. A bloom of P. concavum in Xincun Bay presented high abundances on seagrasses, macroalgae (U. lactuca), and in the water column [3.9 × 105, 1.4 × 104 cells (100 cm2)-1 and 1.7 × 104 cells L−1, respectively]. The population showed extremely high dominance (more than 90% of the benthic dinoflagellate community) in August 2018, in Xincun Bay (Zou et al., 2020). In the present study, the maximal abundances of P. concavum [(1.7 ± 0.59) × 106 cells (100 cm2)-1 on T. hemperichii, Figure 5] and high population dominance were observed in the same seagrass bed in July 2019 (Table 2). These findings suggest that P. concavum is a dominant species in benthic microalgal assemblages and periodically causes blooms in the summer in Xincun Bay, especially in shallow seagrass beds.
While no statistically significant differences between the wet and dry seasons were found (ANOVA, p>0.05), we identified that the abundances of P. concavum on seagrasses and macroalgae were 2.5 and 2.82 times higher in the wet season than those in the dry season. The maximal density was recorded at site A in July with a high temperature of 30.9°C (Figures 3A). Moreover, a significant difference was found in the abundance of planktonic P. concavum between the two seasons. In general, the abundance of P. concavum showed seasonal variation in the tropical Xincun Bay. A harmful benthic bloom of P. concavum occurred in August 2018, which provided further evidence for seasonality (Zou et al., 2020). In another tropical ecosystem, there was also apparent seasonality in benthic Prorocentrum (mainly P. concavum and P. lima), with the lowest abundances of these species recorded between January and May (dry season) (Tindall and Morton, 1998). Similarly, a recent survey investigated the spatial-temporal distributions of benthic dinoflagellate in the Caribbean Sea and demonstrated that the lowest abundances of benthic Prorocentrum appeared from October to January, corresponding with lower seawater temperatures (Boisnoir et al., 2019). Nishimura et al. (2019) reported that the density of benthic Prorocentrum, including P. concavum, was notably higher in subtropical areas than that in temperate areas in Japan. In addition, some studies in the temperate Mediterranean Sea showed a similar phenomenon, that benthic Prorocentrum had a maximal density from July to October (Aissaoui et al., 2014). Hence, we conclude that P. concavum, like other benthic Prorocentrum species, shows high abundance, even blooms, in the summer, associated with higher seawater temperatures.
The abundances of both benthic and planktonic P. concavum were significantly higher in the seagrass bed than those in the macroalgal bed (station B), mangrove (station C), or coral reef (station E). Station A was close to the cage fish-culture area and showed maximal benthic P. concavum abundances 5.6 times higher than those at station D. P. concavum showed high spatial heterogeneity in Xincun Bay, which is consistent with the results of a number of previous field studies. For example, Boisnoir et al. (2019) suggested that the distributions of benthic dinoflagellates, including Prorocentrum, significantly differed between sampling sites and between islands (Guadeloupe and Martinique, Caribbean Sea). A survey of epiphytic dinoflagellates in the Gulf of Tunis, Mediterranean Sea, revealed that P. lima showed significant spatial patterns and higher abundance in seagrass beds (Hachani et al., 2018). Moreover, the spatial heterogeneity of P. concavum among sites in Xincun Bay can be, to some extent, explained by the differences in habitats and substrates. At station A, in addition to the relatively stable hydrometric conditions, the dense fish cages provide sufficient nutrients and suitable substrate for the proliferation of macroalgae. As mentioned by Zhang et al. (2014), the nutrient concentrations are high in cage cultures in Xincun Bay, which results in high macroalgal biomass in this area (Liu et al., 2019). Macroalgae and dense mature seagrasses (Huang et al., 2006) offer suitable environments for the growth of P. concavum (Glibert et al., 2012). Yong et al. (2018) found that microhabitat can be a key factor determining the abundance of benthic dinoflagellates, and Prorocentrum preferred microhabitats covered with high turf algae. In addition, many surveys have suggested that substrate preference, which is also reflected in the spatial heterogeneity, is common in benthic species of Prorocentrum (Boisnoir et al., 2019; Gharbia et al., 2019). In the present study, we found that benthic P. concavum showed maximal abundance on T. hemperichii and abundances were significantly higher on T. hemperichii than on other substrates. This finding suggests that benthic P. concavum has a preference for Thalassia, which is consistent with a previous description by (Delgado et al., 2006). Consequently, we conclude that P. concavum shows a clear spatial distribution pattern in ecosystems and on substrates in Xincun Bay. The differences between ecosystems can be explained by the fact that the abundant and dominant seagrasses T. hemperichii and E. acoroides (also showed high P. concavum density) act as a trap for harmful benthic P. concavum blooms (Huang et al., 2006; Zou et al., 2020). Moreover, the highest abundances of P. concavum at station A were associated with higher nutrient provision from dense caged-fish cultures.
4.3 Environmental Factors Related to the Distribution of P. concavum
4.3.1 Temperature
Seawater temperature was positively correlated with P. concavum on seagrasses, macroalgae, and in the water column, but not on sediments (Table 3). In addition, the significant correlations between temperature and other environmental factors (salinity, pH, nitrate, nitrite, phosphate, and DON) indicated that temperature might be the most important factor driving the spatiotemporal distribution of P. concavum (Table 3). The preference of P. concavum for higher temperatures is consistent with other benthic Prorocentrum, which have been identified as thermophilic in previous studies. Glibert et al. (2012) summarized previous studies and demonstrated that benthic Prorocentrum showed higher densities in tropical/subtropical areas than in temperate zones. Moreover, a two-year survey showed that the maximal abundance of P. lima occurred between July and October, and P. lima (positively correlated with temperature) was discovered when temperature ranged from 18 to 28.5°C, with a preference for 27–30°C (Aissaoui et al., 2014). Results from laboratory studies also demonstrated that higher temperatures are suitable for benthic Prorocentrum. For example, Accoroni et al. (2018) showed that P. hoffmannianum grew rapidly and had a larger maximum quantum yield of PSII at 27°C than at 21°C. We assume that the occurrence of a higher biomass of benthic P. concavum in the Xincun Bay was mainly induced by temperatures between 28 and 30°C.
4.3.2 Salinity, pH, and Dissolved Oxygen
Previous studies showed that salinity can influence the growth of P. concavum and this species had a maximum growth rate at a salinity of 30 (Morton et al., 1992). In the present study, salinity was not significantly related to either benthic or planktonic P. concavum abundances (Table 3). The insignificant effect of salinity on P. concavum in Xincun Bay may be a result of the small salinity range during the sampling period (from 29.08 to 32.59, Figure 3B). In addition, pH was not associated with the abundance of P. concavum in Xincun Bay. While no obvious correlations between benthic cells and dissolved oxygen were found, planktonic P. concavum was negatively associated with this factor (Table 3). Aissaoui et al. (2014) suggested that planktonic P. lima and P. emarginatum in the Mediterranean were also negatively associated with dissolved oxygen.
4.3.3 Nutrients
The abundance of benthic P. concavum was negatively associated with concentrations of nitrite, ammonium, and phosphate, but no correlation was found with nitrate. More importantly, P. concavum densities were positively correlated with concentrations of DOP and DON (Table 3). To date, available data on nutrient utilization by benthic Prorocentrum species is limited and mostly concentrated on P. lima (Glibert et al., 2012). Nitrate is always a primary source of nitrogen, but is rarely a limiting factor for microalgae (Cohu et al., 2013). Aissaoui et al. (2014) demonstrated that P. lima abundance in the Mediterranean was negatively correlated with ammonium, which is consistent with our findings. However, a laboratory study found that P. lima showed a preference for ammonium uptake rather than nitrate or nitrite (Pan et al., 1999). These contradictions could be explained by the descriptions of Aissaoui et al. (2014) that ammonium was taken up rapidly and showed low concentrations at the maximal abundances of P. concavum (Aissaoui et al., 2014). Pan et al. (1999) suggested that the uptake of nitrite by P. lima occurred only when other nitrogen sources were exhausted. Finally, negative correlations between phosphate and P. concavum, coupled with the positive correlation between DOP and this dinoflagellate, indicate that DOP is an important factor driving spatiotemporal variation in P. concavum abundance. Also, P. concavum abundance was positively associated with concentrations of DON (Table 3 and Figure 8). Ou et al. (2022) investigated the activities of extracellular enzymes, including leucine aminopeptidase (LAP) and alkaline phosphatase (AP) which hydrolyzed the DON and DOP, respectively, in the Xincun Bay from December 2018 to December 2019. The results showed that the activities of LAP and AP in the Xincun Bay were greater than other coasts of Chinese waters, even in a bloom period (Ou et al., 2018; Ou et al., 2022). These findings and high concentrations of DON and DOP increased the risk of harmful dinoflagellate blooms in the Xincun Bay. Therefore, concentrations of DON and DOP from aquaculture were the important factors in the occurrences of P. concavum bloom in the Xincun Bay. These findings also explain why the highest abundances were seen at station A (near the cage-culture area).
The spatiotemporal distribution of P. concavum was demonstrated over a 9-month period in Xincun Bay. Both benthic and planktonic P. concavum showed seasonal variation patterns, with higher abundances in the wet season and lower abundances in the dry season, although the benthic abundances were not significantly different. High spatial heterogeneity among different ecosystems and substrates was observed. The seagrass bed had a higher abundance of P. concavum than macroalgal beds, mangroves, or coral reefs. The abundance of P. concavum on the seagrass T. hemperichii was significantly higher than that on sediments. Temperature, DOP, and DON were the three important environmental factors driving the spatiotemporal variation in benthic P. concavum in Xincun Bay. Abundance of planktonic P. concavum was positively associated with benthic cells and negatively associated with dissolved oxygen, indicating that the abundance of P. concavum in the water column is primarily influenced by epiphytic cells and the concentration of dissolved oxygen. Overall, we found that the dense cage-fish culture in the Xincun Bay provided sufficient organic nutrients for the growth and reproduction of P. concavum and the seagrass bed in Xincun Bay may become a reservoir for harmful benthic dinoflagellates.
5 Conclusions
The spatiotemporal distribution of P. concavum was demonstrated over a 9-month period in Xincun Bay. Both benthic and planktonic P. concavum showed seasonal variation patterns, with higher abundances in the wet season and lower abundances in the dry season, although the benthic abundances were not significantly different. High spatial heterogeneity among different ecosystems and substrates was observed. The seagrass bed had a higher abundance of P. concavum than macroalgal beds, mangroves, or coral reefs. The abundance of P. concavum on the seagrass T. hemperichii was significantly higher than that on sediments. Temperature, DOP, and DON were the three important environmental factors driving the spatiotemporal variation in benthic P. concavum in Xincun Bay. Abundance of planktonic P. concavum was positively associated with benthic cells and negatively associated with dissolved oxygen, indicating that the abundance of P. concavum in the water column is primarily influenced by epiphytic cells and the concentration of dissolved oxygen. Overall, we found that the dense cage-fish culture in the Xincun Bay provided sufficient organic nutrients for the growth and reproduction of P. concavum and the seagrass bed in Xincun Bay may become a reservoir for harmful benthic dinoflagellates.
Data Availability Statement
The original contributions presented in the study are included in the article/supplementary material. Further inquiries can be directed to the corresponding author.
Author Contributions
SL and JZ designed the present study. HX and CZ carried out the sampling and experiments. JZ carried out sampling, conducted experiments, analyzed the data and wrote this manuscript. SL revised this manuscript and funded this study. All authors contributed to the article and approved the submitted version.
Conflict of Interest
The authors declare that the research was conducted in the absence of any commercial or financial relationships that could be construed as a potential conflict of interest.
Publisher’s Note
All claims expressed in this article are solely those of the authors and do not necessarily represent those of their affiliated organizations, or those of the publisher, the editors and the reviewers. Any product that may be evaluated in this article, or claim that may be made by its manufacturer, is not guaranteed or endorsed by the publisher.
Acknowledgments
This work was supported by Special Foundation for National Science and Technology Basic Research Program of China (2018FY100200) and National Natural Science Foundation of China (42076144).
References
Accoroni S., Ceci M., Tartaglione L., Romagnoli T., Campanelli A., Marini M., et al. (2018). Role of Temperature and Nutrients on the Growth and Toxin Production of Prorocentrum Hoffmannianum (Dinophyceae) From the Florida Keys. Harmful. Algae. 80, 140–148. doi: 10.1016/j.hal.2018.11.005
Accoroni S., Totti C. (2016). The Toxic Benthic Dinoflagellates of the Genus Ostreopsis in Temperate Areas: A Review. Adv. Oceanogr. Limnol. 7 (1), 1–15. doi: 10.4081/aiol.2016.5591
Aissaoui A., Armi Z., Akrout F., Ben Hassine O. K. (2014). Environmental Factors and Seasonal Dynamics of Prorocentrum Lima Population in Coastal Waters of the Gulf of Tunis, South Mediterranean. Water Environ. Res. 86 (12), 2256–2270. doi: 10.2175/106143014X13975035526266
An T., Winshell J., Scorzetti G., Fell J. W., Rein K. S. (2010). Identification of Okadaic Acid Production in the Marine Dinoflagellate Prorocentrum Rhathymum From Florida Bay. Toxicon 55 (2-3), 653–657. doi: 10.1016/j.toxicon.2009.08.018
Aquino-Cruz A., Purdie D. A., Morris S. (2018). Effect of Increasing Sea Water Temperature on the Growth and Toxin Production of the Benthic Dinoflagellate Prorocentrum Lima. Hydrobiologia 813 (1), 103–122. doi: 10.1007/s10750-018-3512-4
Berdalet E., Tester P. A., Chinain M., Fraga S., Lemée R., Litaker W., et al. (2017). Harmful Algal Blooms in Benthic Systems: Recent Progress and Future Research. Oceanography 30 (1), 36–45. doi: 10.5670/oceanog.2017.108
Boisnoir A., Pascal P.-Y., Cordonnier S., Lemée R. (2018). Depth Distribution of Benthic Dinoflagellates in the Caribbean Sea. J. Sea. Res. 135, 74–83. doi: 10.1016/j.seares.2018.02.001
Boisnoir A., Pascal P.-Y., Cordonnier S., Lemée R. (2019). Spatio-Temporal Dynamics and Biotic Substrate Preferences of Benthic Dinoflagellates in the Lesser Antilles, Caribbean Sea. Harmful. Algae. 81, 18–29. doi: 10.1016/j.hal.2018.11.012
Chomérat N., Bilien G., Zentz F. (2019). A Taxonomical Study of Benthic Prorocentrum Species (Prorocentrales, Dinophyceae) From Anse Dufour (Martinique Island, Eastern Caribbean Sea). Mar. Biodivers. 49 (3), 1299–1319. doi: 10.1007/s12526-018-0913-6
Cicily L., Nandan S. B., Padmakumar K. B. (2020). First Report on an Unusual Bloom of the Potentially Toxic Epibenthic Dinoflagellate Prorocentrum Rhathymum From Bangaram Lagoon of the Lakshadweep Archipelago: Arabian Sea. Reg. Stud. Mar. Sci. 41, 101549. doi: 10.1016/j.rsma.2020.101549
Cohu S., Mangialajo L., Thibaut T., Blanfuné A., Marro S., Lemée R. (2013). Proliferation of the Toxic Dinoflagellate Ostreopsis Cf. Ovata in Relation to Depth, Biotic Substrate and Environmental Factors in the North West Mediterranean Sea. Harmful. Algae. 24, 32–44. doi: 10.1016/j.hal.2013.01.002
Delgado G., Lechuga-Devéze C. H., Popowski G., Troccoli L., Salinas C. A. (2006). Epiphytic Dinoflagellates Associated With Ciguatera in the Northwestern Coast of Cuba. Rev. Biol. Trop. 54 (2), 299–310.
Dickey R. W., Bobzin S. C., Faulkner D. J., Bencsath F. A., Andrzejewski D. (1990). Identification of Okadaic Acid From a Caribbean Dinoflagellate, Prorocentrum Concavum. Toxicon 28 (4), 371–377. doi: 10.1016/0041-0101(90)90074-H
Foden J., Purdie D. A., Morris S., Nascimento S. (2005). Epiphytic Abundance and Toxicity of Prorocentrum Lima Populations in the Fleet Lagoon, UK. Harmful. Algae. 4 (6), 1063–1074. doi: 10.1016/j.hal.2005.03.004
Fukuyo Y. (1981). Taxonomical Study on Benthic Dinoflagellates Collected in Coral Reefs. Bull. Jpn. Soc Sci. Fish 47, 967–978. doi: 10.2331/suisan.47.967
García-Portela M., Riobó P., Franco J. M., Bañuelos R. M., Rodríguez F. (2016). Genetic and Toxinological Characterization of North Atlantic Strains of the Dinoflagellate Ostreopsis and Allelopathic Interactions With Toxic and non-Toxic Species From the Genera Prorocentrum, Coolia and Gambierdiscus. Harmful. Algae. 60, 57–69. doi: 10.1016/j.hal.2016.10.007
Gharbia H. B., Laabir M., Mhamed A. B., Gueroun S. K. M., Yahia M. N. D., Nouri H., et al. (2019). Occurrence of Epibenthic Dinoflagellates in Relation to Biotic Substrates and to Environmental Factors in Southern Mediterranean (Bizerte Bay and Lagoon, Tunisia): An Emphasis on the Harmful Ostreopsis Spp., Prorocentrum Lima and Coolia Monotis. Harmful. algae. 90, 101704. doi: 10.1016/j.hal.2019.101704
Glibert P. M., Burkholder J. A. M., Kana T. M. (2012). Recent Insights About Relationships Between Nutrient Availability, Forms, and Stoichiometry, and the Distribution, Ecophysiology, and Food Web Effects of Pelagic and Benthic Prorocentrum Species. Harmful. Algae. 14, 231–259. doi: 10.1016/j.hal.2011.10.023
Hachani M. A., Dhib A., Fathalli A., Ziadi B., Turki S., Aleya L. (2018). Harmful Epiphytic Dinoflagellate Assemblages on Macrophytes in the Gulf of Tunis. Harmful. Algae. 77, 29–42. doi: 10.1016/j.hal.2018.06.006
Heil C. A. (1993). Mucus-Associated Dinoflagellates: Large Scale Culturing and Estimation of Growth Rate. Toxic. Phytoplankton. Blooms. Sea.
Hoppenrath M., Chomérat N., Horiguchi T., Schweikert M., Nagahama Y., Murray S. (2013). Taxonomy and Phylogeny of the Benthic Prorocentrum Species (Dinophyceae)—A Proposal and Review. Harmful. Algae. 27, 1–28. doi: 10.1016/j.hal.2013.03.006
Huang X., Huang L., Li Y., Xu Z., Fong C. W., Huang D., et al. (2006). Main Seagrass Beds and Threats to Their Habitats in the Coastal Sea of South China. Chin. Sci. Bull. 51, 136–142. doi: 10.1007/s11434-006-9136-5
Hu T., Marr J., de Freitas A. S., Quilliam M. A., Walter J. A., Wright J. L., et al. (1992). New Diol Esters Isolated From Cultures of the Dinoflagellates Prorocentrum Lima and Prorocentrum Concavum. J. Nat.l Prod. 55 (11), 1631–1637.
Jeffries D. S., Dieken F. P., Jones D. E. (1979). Performance of the Autoclave Digestion Method for Total Phosphorus Analysis. Water Res. 13 (3), 275–279.
Juranovic L. R., Park D. L., Fremy J.-M., Nielsen C. A., Ah S. S., Ayala C. E. (1997). Isolation and Separation of Toxins Produced by Gambierdiscus Toxicus and Prorocentrum Concavum. J. Aquat. Food Prod. T. 6 (4), 5–25. doi: 10.1300/J030v06n04_02
Koike K. (2013). A Red Tide Off the Myanmar Coast: Morphological and Genetic Identification of the Dinoflagellate Composition. Harmful. Algae. 27, 149–158. doi: 10.1016/j.hal.2013.05.010
Liu S., Jiang Z., Wu Y., Deng Y., Chen Q., Zhao C., et al. (2019). Macroalgae Bloom Decay Decreases the Sediment Organic Carbon Sequestration Potential in Tropical Seagrass Meadows of the South China Sea. Mar. pollut. Bull. 138, 598–603. doi: 10.1016/j.marpolbul.2018.12.009
Luo Z., Zhang H., Krock B., Lu S., Yang W., Gu H. (2017). Morphology, Molecular Phylogeny and Okadaic Acid Production of Epibenthic Prorocentrum (Dinophyceae) Species From the Northern South China Sea. Algal. Res. 22, 14–30. doi: 10.1016/j.algal.2016.11.020
Mohammad-Noor N., Moestrup Ø., Daugbjerg N. (2007). Light, Electron Microscopy and DNA Sequences of the Dinoflagellate Prorocentrum Concavum (Syn. P. Arabianum) With Special Emphasis on the Periflagellar Area. Phycologia 46 (5), 549–564. doi: 10.2216/06-94.1
Morton S. L., Faust M. A., Fairey E. A., Moeller P. D. (2002). Morphology and Toxicology of Prorocentrum Arabianum Sp. Nov., (Dinophyceae) a Toxic Planktonic Dinoflagellate From the Gulf of Oman, Arabian Sea. Harmful. Algae. 1 (4), 393–400. doi: 10.1016/S1568-9883(02)00047-1
Morton S. L., Moeller P. D., Young K., Lanoue B. (1998). Okadaic Acid Production From the Marine Dinoflagellate Prorocentrum Belizeanum Faust Isolated From the Belizean Coral Reef Ecosystem. Toxicon 36 (1), 201–206. doi: 10.1016/s0041-0101(97)00054-8
Morton S. L., Norris D. R., Bomber J. W. (1992). Effect of Temperature, Salinity and Light Intensity on the Growth and Seasonality of Toxic Dinoflagellates Associated With Ciguatera. J. Exp. Mar. Biol. Ecol. 157 (1), 79–90. doi: 10.1016/0022-0981(92)90076-M
Murphy J., Riley J. P. (1962). A Modified Single Solution Method for the Determination of Phosphate in Natural Waters. Anal. Chim. Acta 27 (C), 678–681.
Nishimura T., Uchida H., Noguchi R., Oikawa H., Suzuki T., Funaki H., et al. (2019). Abundance of the Benthic Dinoflagellate Prorocentrum and the Diversity, Distribution, and Diarrhetic Shellfish Toxin Production of Prorocentrum Lima Complex and P. Caipirignum in Japan. Harmful. Algae.96, 101687. doi: 10.1016/j.hal.2019.101687
Nishimura T., Uchida H., Suzuki T., Tawong W., Abe S., Arimitsu S., et al. (2020). First Report on Okadaic Acid Production of a Benthic Dinoflagellate Prorocentrum Cf. Fukuyoi From Japan. Phycol. Res. 68 (1), 30–40. doi: 10.1111/pre.12401
Ou L., Liu X., Li J., Qin X., Cui L., Lu S. (2018). Significant Activities of Extracellular Enzymes From a Brown Tide in the Coastal Waters of Qinhuangdao, China. Harmful. Algae. 74, 1–9. doi: 10.1016/j.hal.2018.03.005
Ou L., Li J., Zhang X., Wang Z., Zou J., Lu S. (2022). Dissolved Organic Matter and Activities of Extracellular Enzymes in Two Lagoons (South China Sea) Affected by Aquaculture. Front. Mar. Sci. 9. doi: 10.3389/fmars.2022.864579
Pan Y., Cembella A., Quilliam M. (1999). Cell Cycle and Toxin Production in the Benthic Dinoflagellate Prorocentrum Lima. Mar. Biol. 134 (3), 541–549. doi: 10.1016/S1568-9883(02)00047-1
Reynolds C. S. (2007). Variability in the Provision and Function of Mucilage in Phytoplankton: Facultative Responses to the Environment. Hydrobiologia 578 (1), 37–45.
Rodríguez F., Riobó P., Crespín G. D., Daranas A. H., de Vera C. R., Norte M., et al. (2018). The Toxic Benthic Dinoflagellate Prorocentrum Maculosum Faust is a Synonym of Prorocentrum Hoffmannianum Faust. Harmful. Algae. 78, 1–8. doi: 10.1016/j.hal.2018.06.009
Solrzano L. (1969). Determination of Ammonia in Natural Waters by the Phenol-Hypochlorite Method. Limnol. Oceanogr. 14 (5), 799–801.
Strickland J. D. H., Parsons, (1972). A Practical Handbook of Weawater Analysis. 2nd ed Vol. 167 (Ottawa; Canada: Bull Fish Res Bd Can), 310, 1–310310. Supply Serv., Canada.
Ten-Hage L.c., Robillot C., Turquet J., Le Gall F., Le Caer J.-P., Bultel V., et al. (2002). Effects of Toxic Extracts and Purified Borbotoxins From Prorocentrum Borbonicum (Dinophyceae) on Vertebrate Neuromuscular Junctions. Toxicon 40 (2), 137–148. doi: 10.1016/S0041-0101(01)00200-8
Tester P. A., Feldman R. L., Nau A. W., Kibler S. R., Litaker R. W. (2010). Ciguatera Fish Poisoning and Sea Surface Temperatures in the Caribbean Sea and the West Indies. Toxicon 56 (5), 698–710. doi: 10.1016/j.toxicon.2010.02.026
Tester P. A., Kibler S. R., Holland W. C., Usup G., Vandersea M. W., Leaw C. P., et al. (2014). Sampling Harmful Benthic Dinoflagellates: Comparison of Artificial and Natural Substrate Methods. Harmful. Algae. 39, 8–25. doi: 10.1016/j.hal.2014.06.009
Tindall D. R., Morton S. L. (1998). Community Dynamics and Physiology of Epiphytic/Benthic Dinoflagellates Associated With Ciguatera. Physiol. Ecol. Harmful. Algal. Blooms. 41, 293–314.
Toyofuku H. (2006). Joint FAO/WHO/IOC Activities to Provide Scientific Advice on Marine Biotoxins (Research Report). Mar. Pollut. Bull. 52 (12), 1735–1745. doi: 10.1016/j.marpolbul.2006.07.007
Turkoglu M. (2016). First Harmful Algal Bloom Record of Tycoplanktonic Dinoflagellate Prorocentrum Lima (Ehrenberg) F. Stein 1878 in the Dardanelles (Turkish Straits System, Turkey). J. Coast. Life Med. 4 (10), 765–774. doi: 10.12980/jclm.4.2016J6-184
Verma A., Kazandjian A., Sarowar C., Harwood D. T., Murray J. S., Pargmann I., et al. (2019). Morphology and Phylogenetics of Benthic Prorocentrum Species (Dinophyceae) From Tropical Northwestern Australia. Toxins 11 (10), 571. doi: 10.3390/toxins11100571
Xie H., Zou J., Zheng C., Qu Y., Huang K., Lu S. (2022). Biodiversity and Distribution of Benthic Dinoflagellates in Zhongsha, a Tropical Islands in China. J. Oceanol. Limnol. doi: 10.1007/s00343-022-1322-z
Yang Y., Gao S., Zhou L., Wang Y., Li G., Wang Y., et al. (2017). Classifying the Sedimentary Environments of the Xincun Lagoon, Hainan Island, by System Cluster and Principal Component Analyses. Acta Oceanol. Sin. 36 (4), 64–71. doi: 10.1007/s13131-016-0939-1
Yong H. L., Mustapa N. I., Lee L. K., Lim Z. F., Tan T. H., Usup G., et al. (2018). Habitat Complexity Affects Benthic Harmful Dinoflagellate Assemblages in the Fringing Reef of Rawa Island, Malaysia. Harmful. Algae. 78, 56–68. doi: 10.1016/j.hal.2018.07.009
Zhang J., Huang X., Jiang Z. (2014). Physiological Responses of the Seagrass Thalassia Hemprichii (Ehrenb.) Aschers as Indicators of Nutrient Loading. Mar. pollut. Bull. 83 (2), 508–515. doi: 10.1016/j.marpolbul.2013.12.056
Zou J., Li Q., Liu H., Liu Y., Huang L., Wu H., et al. (2021). Taxonomy and Toxin Profile of Harmful Benthic Prorocentrum (Dinophyceae) Species From the Xisha Islands, South China Sea. J. Oceanol. Limnol. 40, 1171–1190. doi: 10.1007/s00343-021-1045-6
Keywords: benthic dinoflagellate, Prorocentrum concavum, Xincun Bay, lagoon, dissolved organic nutrient, seagrass bed
Citation: Zou J, Xie H, Zheng C and Lu S (2022) Spatial-Temporal Distribution of Prorocentrum concavum Population in Relation to Environmental Factors in Xincun Bay, a Tropical Coastal Lagoon in China. Front. Mar. Sci. 9:931533. doi: 10.3389/fmars.2022.931533
Received: 29 April 2022; Accepted: 20 June 2022;
Published: 18 July 2022.
Edited by:
Nathan Jack Robinson, Fundación Oceanográfica, SpainReviewed by:
Zhangxi Hu, Guangdong Ocean University, ChinaXinqing Zheng, State Oceanic Administration, China
Ruoyu Guo, Ministry of Natural Resources, China
Copyright © 2022 Zou, Xie, Zheng and Lu. This is an open-access article distributed under the terms of the Creative Commons Attribution License (CC BY). The use, distribution or reproduction in other forums is permitted, provided the original author(s) and the copyright owner(s) are credited and that the original publication in this journal is cited, in accordance with accepted academic practice. No use, distribution or reproduction is permitted which does not comply with these terms.
*Correspondence: Songhui Lu, bHVzb25naHVpMTk2M0AxNjMuY29t