- 1Dipartimento di Scienze Chimiche, Fisiche, Matematiche e Naturali, Università degli Studi di Sassari, Sassari, Italy
- 2Dipartimento di Scienze dell’Ambiente e della Terra, Università degli Studi di Milano–Bicocca, Milano, Italy
- 3Consorzio Nazionale Interuniversitario per le Scienze del Mare (CoNISMa), Roma, Italy
- 4Dipartimento di Ecologia Marina Integrata, Ischia Marine Centre, Stazione Zoologica Anton Dohrn, Ischia, Italy
- 5Dipartimento di Architettura Design e Urbanistica, Università degli Studi di Sassari, Sassari, Italy
- 6Dipartimento di Scienze della Vita e dell’Ambiente Università Politecnica delle Marche, Ancona, Italy
Understanding how coralline algae may acclimatize to ocean warming is important to understand their survival over the coming century. Taking advantage of natural differences in temperature conditions between coastal areas in Sardinia (Italy) and between depths, the responses in terms of biological traits to warming of the crustose coralline alga Lithophyllum stictiforme, a key bioconstructor of coralligenous reefs in the Mediterranean, were evaluated in the field by two innovative transplant experiments where translocated specimens were used as controls. Results of the first experiment (algae cross transplanted between a cold and a warm site at two depths, 23 and 34 m) showed that the marginal growth of the alga and production of conceptacles were higher in the cold site, regardless of the treatment (transplant and translocation) and depth. However, growth in thickness in algae transferred from the cold to the warm site was higher at 34 m of depth, where they had a better performance than the local (translocated) algae. Results of the second experiment (algae transplanted from 34 m to 15 m of depth under different light irradiance manipulations) evidenced that the increase in temperature of +4°C was tolerated by thalli transplanted at 15 m, but that thallus growth and conceptacles production was negatively affected by the higher light irradiance. These results suggest an overall good adaptability of L. stictiforme under warmer conditions, even those due to thermocline deepening. Overall, these results encourage consideration of the use of transplants of this bioconstructor in future restoration actions of coralligenous habitats.
Introduction
Climate variability is expected to increase as anthropogenic global warming continues, and climate models predict that temperature peaks such as marine heat waves (MHWs) will become more extreme, more frequent and more prolonged in time in many regions (Oliver et al., 2018; Smale et al., 2019; IPCC, 2021). Temperature affects organisms in several ways from the individual level (ecophysiology) to the population and species levels (changes in geographic distribution) (Poloczanska et al., 2013; Bruno et al., 2015; Scheffers et al., 2016; Gauzens et al., 2020). Ocean warming (OW) is altering important physical and structural features of many ecosystems (Hoegh-Guldberg and Poloczanska, 2017; Bruno et al., 2018; Smale et al., 2019) and is perceived as a major threat to key foundation species such as corals (Carpenter et al., 2008; Hughes et al., 2018; Kubicek et al., 2019), kelps (Assis et al., 2016; Wernberg et al., 2016), seagrasses (Marbà and Duarte, 2010; Thomson et al., 2015), and coralline algae (Ragazzola et al., 2012; Ordoñez et al., 2014; McCoy and Kamenos, 2015; Kim et al., 2020). The climate impacts are expressed at various levels of biological organization and often are due to changes in local or regional environmental conditions affecting biological processes such as reproduction and survival (Ådal et al., 2006). Species may respond differently to climate change because of a variety of factors, such as evolutionary history, species interactions, physiology, genetic structure, behavior, and habitat-mediated environmental effects on individuals (Etterson and Shaw, 2001; McCoy and Ragazzola, 2014; Hurd, 2015; Louthan and Morris, 2021). Local habitat conditions can mediate the effects of climate, so populations living in different habitats may exhibit different responses to global changes (Crozier et al., 2008).
Coralline algae (CA) are calcareous red algae, belonging to the orders Corallinales, Corallinapetrales, Hapalidiales and Sporolithales, widespread in the photic zone of rocky shores in both hemispheres (Steneck, 1986; Rindi et al., 2019; Schubert et al., 2020). Their morphological habits include encrusting forms (crustose coralline algae, CCA), free-living thalli (rhodolith-maerl) and geniculate articulated thalli; species with these morphologies occur both in intertidal and subtidal habitats and some act as important ecosystem engineers (Steneck, 1986; Nelson, 2009; Fragkopoulou et al., 2021). Some CCA, in particular, are important habitat builders, as their calcified thalli create and stabilize reefs and greatly increase benthic diversity by providing hard substrate for other organisms to settle on (Foster, 2001; Teichert, 2014). Bioconstructor CA are slow-growing and long-lived organisms and occur from the intertidal zone down to 270 m in the tropics, occurring commonly at all latitudes on modern carbonate shelves worldwide (Bosence, 1983; Littler and Littler, 1984; Nelson, 2009; Teichert et al., 2012; Foster et al., 2013). Many CA species are experiencing a loss of vitality (Blanfuné et al., 2016) and are considered highly vulnerable to long-term climate change (Cornwall et al., 2019; Rindi et al., 2019; Peña et al., 2021). Mineralogical changes have also been evidenced, as the Mg content in CA varies as a function of the sea water temperature (Halfar et al., 2008; Kamenos et al., 2008; Hetzinger et al., 2009; Ragazzola et al., 2020) which is believed to exert primary control by determining incorporation of Mg into the CA cell walls (Kamenos et al., 2008; Caragnano et al., 2014; Diaz-Pulido et al., 2014; Ragazzola et al., 2020).
CCA have occurred in the Mediterranean Sea for ∼140 My and are well-represented in the subsequent fossil record (Chatalov et al., 2015). Among the living taxa, species of the genus Lithophyllum (Corallinales) are key bioconstructors, greatly contributing to the formation of coralligenous concretions (Garrabou and Ballesteros, 2000; Ballesteros, 2006). Coralligenous reefs are the most important deep subtidal habitat in the Mediterranean and provide important ecosystem services, acting as hot spots of marine biodiversity (Ådal et al., 2006; Ballesteros, 2006; Piazzi and Ceccherelli, 2020), carbon sinks (Martin et al., 2014), and providing habitat for economically valuable species (Canals and Ballesteros, 1997; Ballesteros, 2006; Rindi et al., 2019). In coralligenous reefs, CCA constitute a secondary substrate which hosts highly diverse assemblages (Ballesteros, 2006). Spatial analyses of assemblages associated to CCA have evidenced biogeographical patterns (Piazzi et al., 2021), supporting the hypothesis that temperature is a key driver affecting the horizontal and vertical distribution of these communities (Ceccherelli et al., 2020; Pinna et al., 2021). On the contrary, little is known about the influence of temperature on CCA presence and distribution in the Mediterranean Sea, although several diseases referable to thermal effects have been reported (Hereu and Kersting, 2016) and a time-integrated thermogeographic model has been proposed by Adey and Steneck (2001) to demonstrate conditions under which CCA assemblages evolve biogeographic patterns in their distribution and abundance.
At present, only laboratory studies about vulnerability of CCA at increased greenhouse gas conditions have been conducted (Anthony et al., 2008; Martin and Gattuso, 2009; Rodríguez-Prieto, 2016; Vásquez-Elizondo and Enríquez, 2016; Martin and Hall-Spencer, 2017; Kim et al., 2020). Experiments performed on Lithophyllum species have investigated how warming affects growth, respiration, photosynthesis, calcification, and mineralogical responses (Martin and Gattuso, 2009; Martin et al., 2013a; Martin et al., 2013b; Nash et al., 2016; Rodríguez-Prieto, 2016). Thus, despite some knowledge on survival, growth rates and geochemistry of Lithophyllum, it is not yet clear how environmental changes translate into the distribution of Mediterranean populations. The need for further insights is also driven by the results of recent molecular studies (De Jode et al., 2019; Pezzolesi et al., 2019). Lithophyllum stictiforme (Areschoug) Hauck and Lithophyllum cabiochiae (Boudouresque & Verlaque) Athanasiadis, the two main species of the genus in coralligenous concretions, were recently shown to represent a striking case of cryptic diversity: although in the past most authors separated these species, recent treatments (Cormaci et al., 2017; Pezzolesi et al., 2019; Guiry and Guiry, 2022) consider L. cabiochiae and L. stictiforme conspecific. Both Pezzolesi et al. (2019) and De Jode et al. (2019) concluded that these entities belong to a complex of cryptic species, several of which have a geographic distribution seemingly restricted to a particular area or site (Rindi et al., 2019). Therefore, the need of a better knowledge of the biology of the L. stictiforme complex in relation to future scenarios is therefore more important and urgent than ever. Indeed, a better understanding of CCA responses to warming is critical to predict how CCA-based communities may change in response to global environmental changes.
Nowadays, there is a growing need of field experiments which may serve as a useful bridge between laboratory results and natural environmental processes (Chave, 2013). Taking advantage of natural differences in temperature conditions between coastal areas in Sardinia (Italy, Western Mediterranean, Pansini et al., 2021) and along depth gradients (Ceccherelli et al., 2020), we performed crossed transplants of L. stictiforme to examine the temperature effects in terms of mortality, growth, and reproductive structures for the first time in the field. Two experiments were conducted: in the first (between sites experiment), to evaluate the adaptability of the alga to a different thermal regime, we transplanted algae from a cold to a warmer site at two depths; in the second (between depths experiment), transplants were done within the same site from a deeper to a shallower depth under different light irradiance manipulations to evaluate the influence of the thermocline, which could be increasingly deeper and persistent due to global climate change effects (Cerrano et al., 2000; Collins et al., 2010). The main goal was to understand if L. stictiforme is adaptable to changes in thermal conditions and to distinguish the effects of light irradiance from temperature conditions on the algal performance. This species was chosen because it is the most common CCA in the coralligenous concretions considered and occurs throughout the Mediterranean region. The results provide pivotal information collected in the field on the biology of the alga, allowing us to forecast whether future changes in temperature will affect its performance and to propose hypotheses about performances at the population level for the future. Finally, these data may also contribute to draw up future guidelines for a restoration of coralligenous habitats by transplantation of this foundation species.
Materials and methods
The two manipulative experiments, between site experiment (BSE) and between depth experiment (BDE), were both conducted in Sardinia. The BSE was carried out from November 2020 to October 2021 on coralligenous reefs of two sites, Costa Paradiso (CP, 41°04’N, 08°57’E) and Capo Carbonara Marine Protected Area (CC, 39°05’N, 09°31’E, Figure 1), belonging to two different biogeographic regions (Coll et al., 2010; Piazzi et al., 2021). The BDE was done from June to November 2021 on the coralligenous reefs at the CP site.
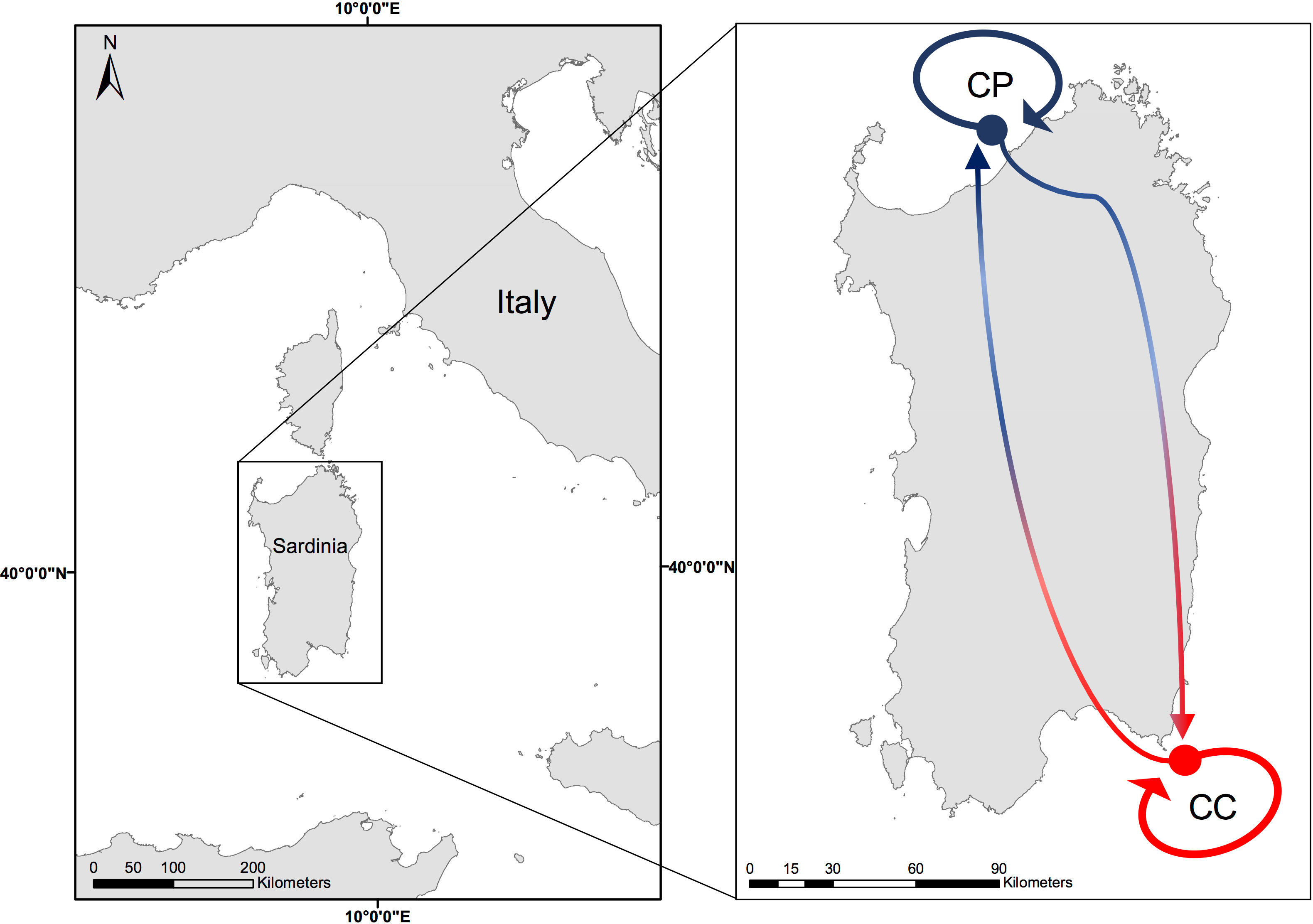
Figure 1 Map of the study sites: Costa Paradiso (CP) in blue = cold site and Capo Carbonara (CC) in red = warm site. Arrows indicate the translocated and transplanted treatments in the BSE.
Both sites are far from any anthropogenic sources of disturbance and are characterized by a high oligotrophy (Barisiello et al., 2002; Regione Autonoma della Sardegna, 2016). However, large differences in sea water temperature were evidenced between sites in the Sea Surface Temperature (SST) climatology, water stratification during the hot season in terms of duration, temperature intensity and variability, and MHWs occurrence (Ceccherelli et al., 2020). Probably due also to such differences, the coralligenous assemblages of the two sites have different geomorphological structures (Piazzi et al., 2021): in particular, CP, which is on average colder than CC, is characterized by massive bioconstructions from 15 m of depth, while in CC the same type of constructions is found only deeper than 30 m (Pinna et al., 2021).
Morphology and identification of the material used for the experiments
Due to the complex taxonomic nature of the L. stictiforme complex (De Jode et al., 2019; Pezzolesi et al., 2019), all possible care was taken to ensure that all specimens used in the experiments belonged to the same species. The specimens used in this study were identified as L. stictiforme based on the circumscription of this species provided by Pezzolesi et al. (2019). They consisted of lobed lamellae, often superimposed, up to 1.5 mm thick, with a smooth to undulate surface, violet to dark pink in colour (Figure S1). Using DNA sequence data, Pezzolesi et al. (2019) showed that specimens exhibiting this habit represent the genuine L. stictiforme and demonstrated that this species is widespread in the central Mediterranean. For this study it was not possible to sequence all specimens used in the experiments; however, DNA sequence data (partial psbA sequences) were obtained for a few representative specimens from CP, confirming the assignment to L. stictiforme (Caragnano and Rindi, unpublished data, Figure S2). These specimens have been kept and will be deposited in the phycological section of the Herbarium Anconitanum, Polytechninc University of Marche (ANC).
Sampling designs and experiment set up
In the BSE a cross-transplant experiment of L. stictiforme was performed between CP and CC maintaining the origin depth (i.e. 23 and 34 m). Based on temperature variations measured by Ceccherelli et al. (2020), CC has considerably warmer water than CP, and the rationale of the BSE relies on the fact that, at the same depth, the algae of the two sites can experience different temperatures for several months, at least from May to November (Ceccherelli et al., 2020). At each combination of site and depth, eight L. stictiforme thalli were fixed on an exposed horizontal substratum next to the coralligenous cliff: four transplanted from the other site (same depth) and four translocated (same site and same depth, sensu Chapman, 1986). The experimental design consisted of three factors (Figure 2): 1) Site, with two levels (cold=CP and warm=CC); 2) Depth, with two levels (23 and 34 m); 3) Treatment, with two levels (transplanted and translocated).
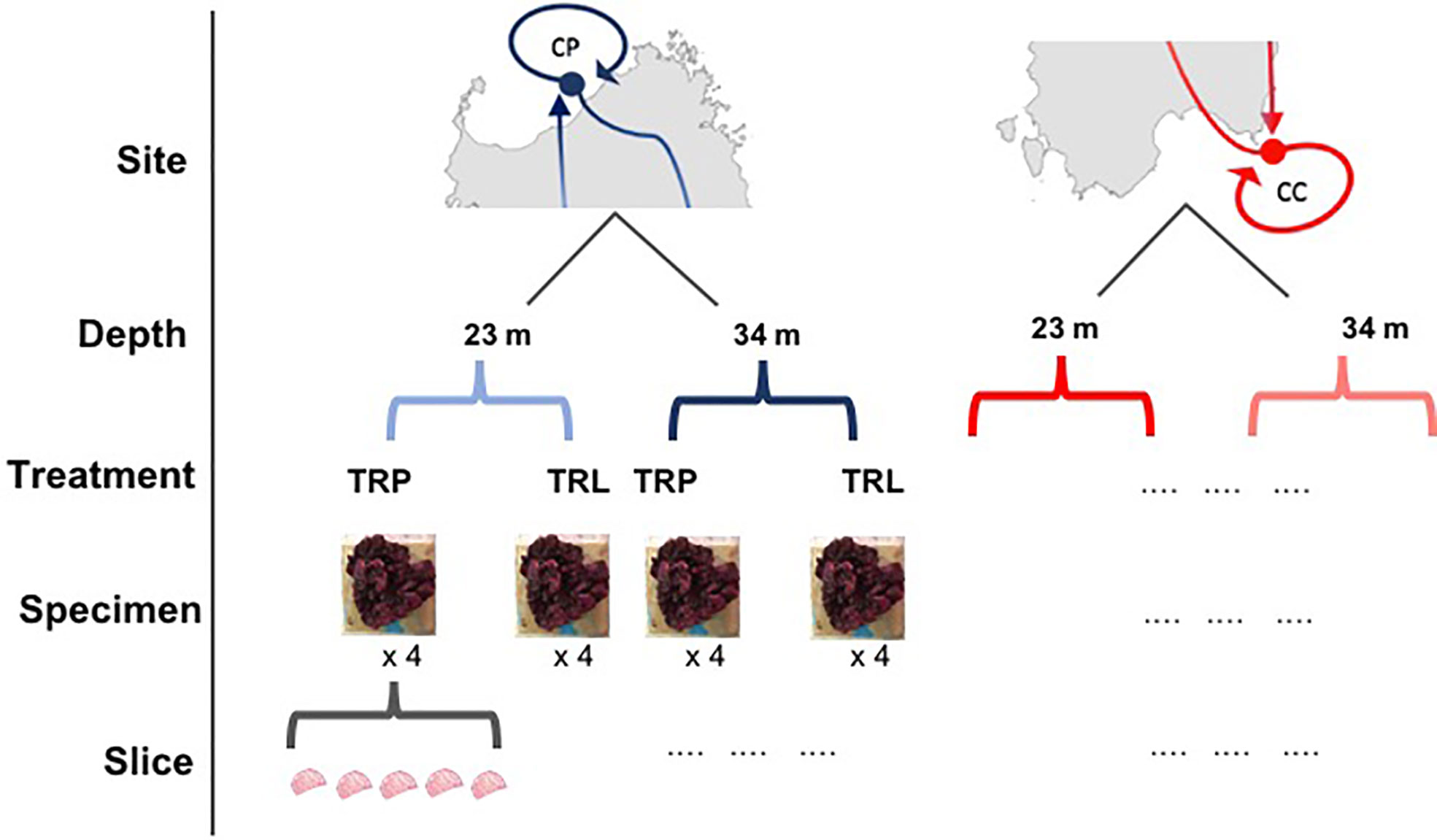
Figure 2 Sampling design of the BSE. Site: CP, cold site; CC, warm site; Depth, 23 and 34 m; Treatment, TRP=transplant; TRL, translocation.
In the BDE, to evaluate the influence of warm water on 34 m depth algae simulating the effects of the near future deeper thermocline, and to disentangle the effects of irradiance from those of the temperature, four treatments were used by transplanting or translocating L. stictiforme thalli (4 replicated treatments) between two depths at CP site: 1) L. stictiforme was transplanted from 34 m to 15 m depth and placed in a natural cavity within the bioconstruction (L TRP=transplanted at low irradiance), so that the algae could experience the 34 m light irradiance but water temperature above the thermocline; 2) L. stictiforme was translocated at the same depth of origin (34 m, L TRL=translocated at low irradiance); 3) L. stictiforme was transplanted from 34 m to 15 m on a exposed substratum (H TRP=transplanted at high irradiance); 4) L. stictiforme was translocated at the same depth of origin (15 m, H TRL=translocated at high irradiance). The L TRP and L TRL treatments simulate the temperature and irradiance conditions that occur at 34 m of depth in the future and current scenario, respectively; the comparison between L TRP and H TRP algae allows disentangling the effect of temperature from light irradiance, while the comparison between the H TRL and H TRP treatments allows to assess the adaptability of algae from different depths to high temperature and high irradiance conditions.
For the setup of both experiments, healthy L. stictiforme (about 8 cm in diameter) with no signs of damage or bleaching were carefully collected (detaching their basal parts using hammer and chisel) by SCUBA diving (Figure 3A) and transported in insulated aquaria to the laboratory within one hour, where they were cleaned from epiphytes and rinsed with new seawater. Afterwards, they were placed in aquaria with oxygenators, kept at the temperature of their origin environment and stained using Alizarine-Red stain (Sigma-Aldrich, Steinheim, Germany) 0.25 g·l-1 for 24 h (Blake and Maggs, 2003; Rivera et al., 2004). The stained L. stictiforme thalli were transported to the receiving sites and glued with a two-component epoxy underwater filler (Sub Coat Veneziani) on granite tiles which were attached on horizontal substrates in the field (Figure 3A). Both CP and CC host extensive populations of healthy L. stictiforme occupying a relatively wide bathymetric range and the collection of the specimens used for the experiments did not compromise the vitality of the populations.
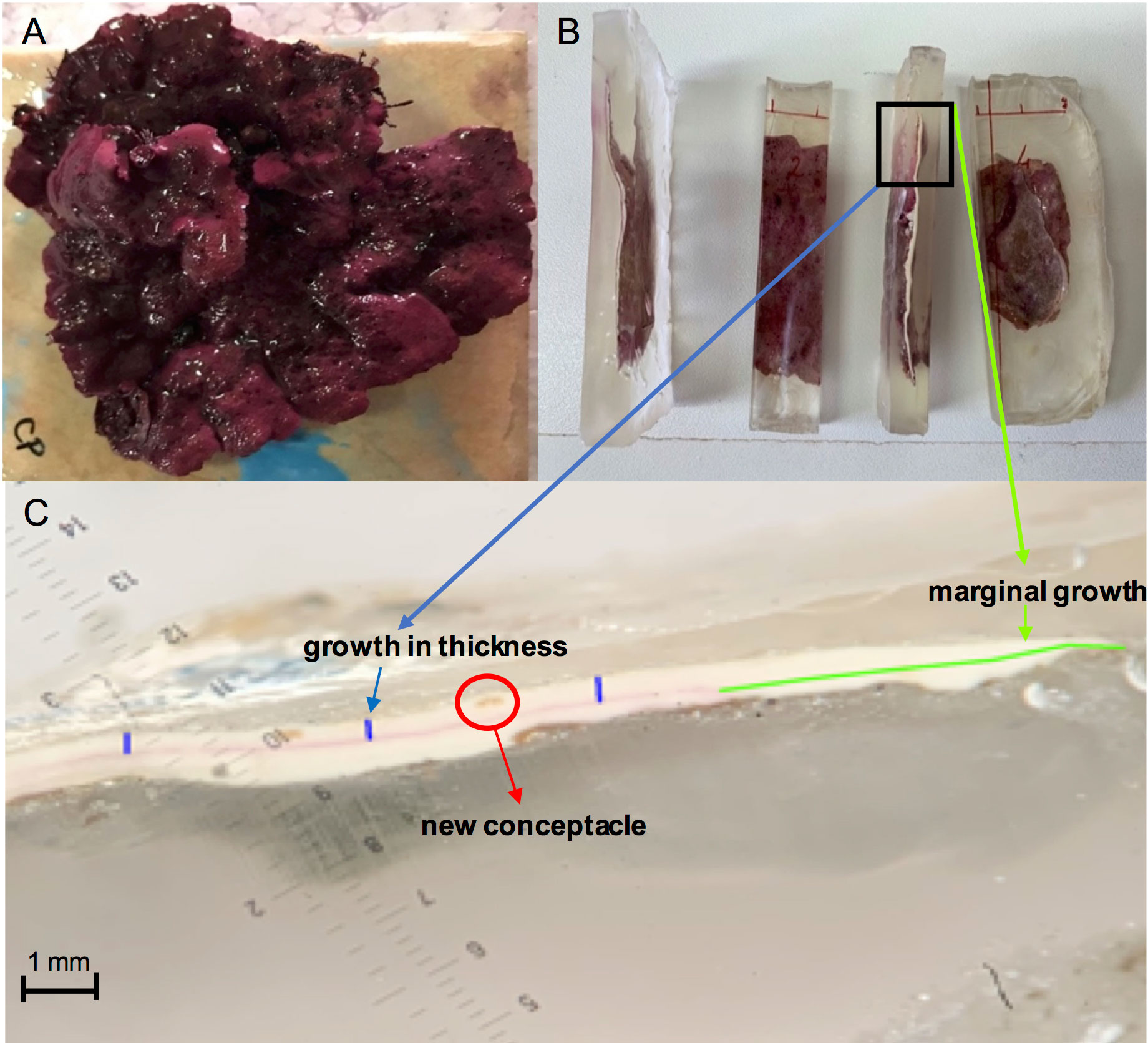
Figure 3 Lithophyllum stictiforme: (A) thallus on tile; (B) embedded into epoxy resin mold; (C) thallus slice under the binocular (in green the marginal growth, in blue the growth in thickness and red a new conceptacle).
For both experiments, two data loggers (HOBO Pendant Temp/Light MX2202, Onset Computer Corporation, USA) per treatment were fixed to record water temperature and light irradiance (only for the BDE) during the whole experimental time. The loggers were checked and cleaned from epiphytes every two months.
At the end of the experimental period (12 months for BSE and 6 months for BDE), each thallus was recollected from the field and transported to the laboratory, where mortality was evaluated by visually inspecting the colour of the thallus surface. Thalli were considered dead when a total bleaching of the surface occurred, since the colour change does not indicate thallus deterioration, as the concentration of phycobilin can vary in relation to the light exposure. Then algae were rinsed with fresh water, air dried and individually embedded into epoxy resin mold (Kit EpoFix Struers; Figure 3B).
Data collection
From each thallus five slices (about 1 cm wide) were obtained using a circular rock saw, and each slice was polished using a machine with abrasive papers of different grain size (i.e. from 30 to 15 μm) on the longitudinal axes and photographed under the binocular. Then thallus marginal growth and growth in thickness were estimated on the images through ImageJ software (https://imageJ.nih.gov, Figure 3C) by measuring the thallus new portions from the Alizarine stain. A total of five measurements at equal distances (approximately 3 mm) were taken along the cross-section of each slice and averaged to obtain the growth in thickness measurements in each slice. Furthermore, in the portion of the alga grown during the experiment, the abundance of conceptacles was checked, and the number of conceptacles was calculated for each slice. Then, for both experiments, the marginal growth, growth in thickness and number of conceptacles of each slice were measured and averaged for each thallus. Each thallus was considered a replicate (Figure 3B).
Data analysis
In order to assess thermal differences between sites (CC and CP), two approaches were used: i) the daily temperature recorded by loggers was analyzed by a three-way permutational analysis of variance (PERMANOVA, Anderson, 2001) based on Euclidean distance using an orthogonal design where site (two levels), depth (two levels) and season (four levels) were fixed factors (n = 28); ii) SST data were used to describe MHWs in both sites according with Hobday et al. (2018); Hobday et al. (2016), using heatwaveR packages in R (Schlegel and Smit, 2018). The levels of the season factor (Autumn, Winter, Spring and Summer) were considered as meteorological seasons.
L. stictiforme thallus marginal growth and growth in thickness in the BSE were analysed by three-way univariate PERMANOVAs (a semiparametric test Anderson, 2001) based on Euclidean distance where site (CC and CP), depth (23 and 34 m), and treatment (TRL and TRP) were fixed orthogonal factors. In the BDE, the effects of treatments on the marginal growth were assessed by one-way PERMANOVA based on Euclidean distance where treatment (L TRL, L TRP, H TRP, and H TRL) was a fixed factor. PERMDISP (Anderson, 2006) was used to test the robustness of PERMANOVA analysis with respect to sample dispersion (Anderson et al., 2008) and a posteriori comparisons of means were done using Pair-Wise comparisons after PERMANOVAs (Anderson, 2001). PERMANOVAs and Pair-Wise tests were performed with PRIMER v7 software, while MHWs detection was performed in R software.
Results
Between sites experiment
Temperature data collected from loggers estimated the temperature differences between CP and CC at the two depths during the study period (Figure 4). Differences between sites in terms of annual average temperature were overall larger at 23 m (1.30°C) than at 34 m (0.68°C) of depth (Table 1). Significant differences in temperature were found between sites, depths, and seasons highlighting that CC site was significantly warmer than CP for half of the study period, even if the dispersion was not homogeneous (Table 2 and Figure 4).
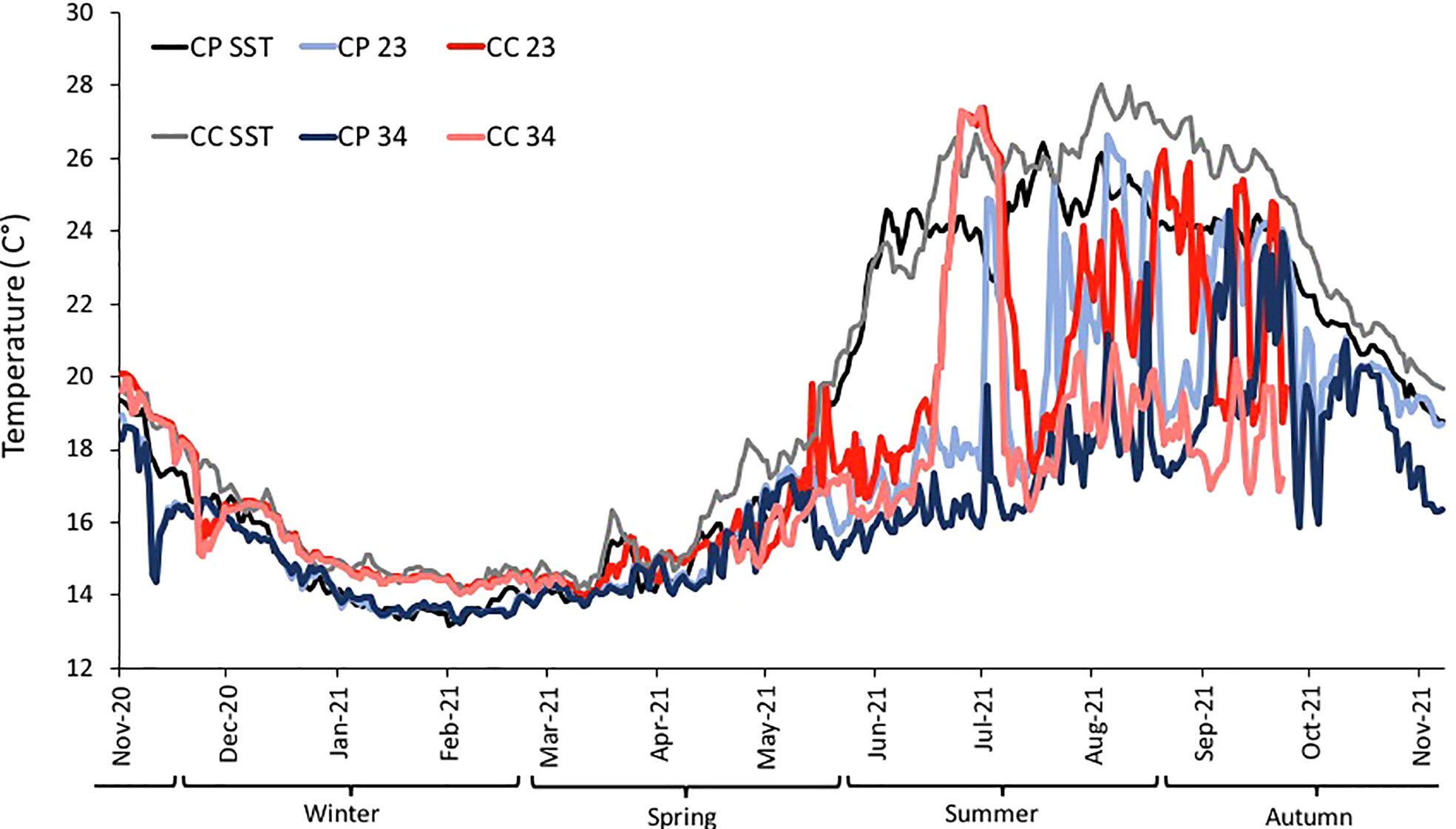
Figure 4 BSE: Daily temperature from November 2020 to October 2021 at the two sites (CP and CC), each depth (23 and 34 m from loggers). In black and grey SST (for CP and CC, respectively) from satellites. Winter, Spring, Summer and Autumn are considered as meteorological Seasons.
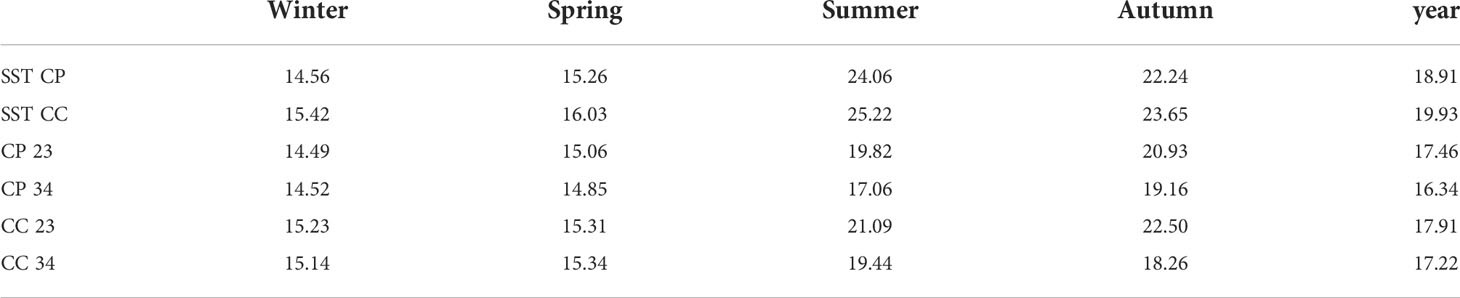
Table 1 BSE: season and year (1 November 2020 - 31 October 2021) average water temperature of sea surface temperature (SST from satellites) and in situ (23 and 34 m of depth from loggers) at Costa Paradiso=CP and Capo Carbonara=CC.
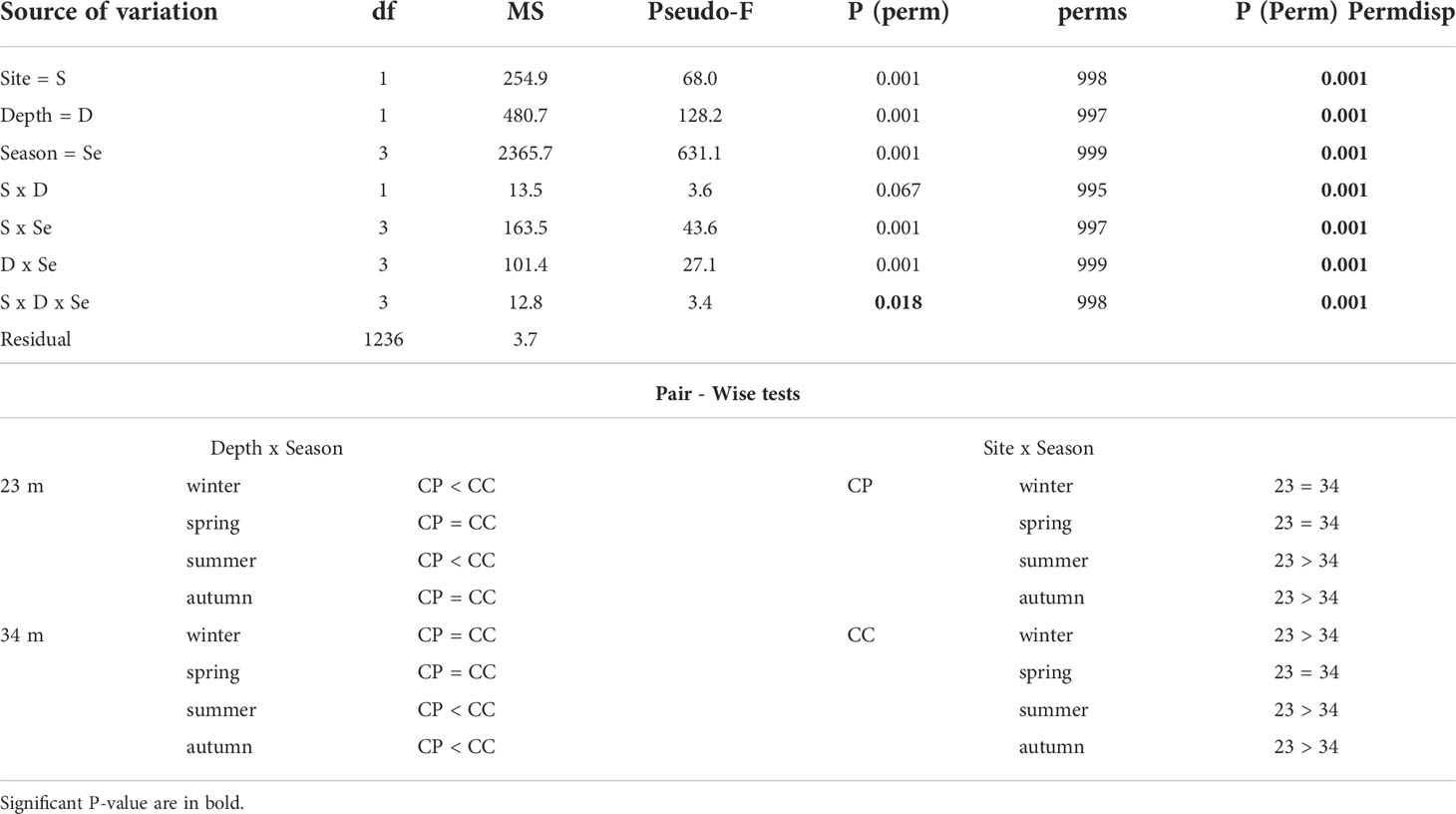
Table 2 BSE - temperature: results of PERMANOVA testing the effect of Site (CP and CC); Depth (23 and 34 m) and Season (Winter, Spring, Summer, and Autumn) and Pair-Wise tests on the interaction Depth x Site and Site x Season.
In addition, during the study period eight MHWs occurred at each of the sites, but not simultaneously: in CP two MHWs during the cooler period (Nov 2020-Apr 2021) and six during the warmer (May-Oct 2021), while in CC four MHWs in each of the periods. Overall, the highest MHW intensity category was recorded at CC during October 2021 (Figure S3).
All algae were found healthy at the end of the study and no mortality was recorded. The marginal growth of L. stictiforme was significantly higher in CP than in CC site, independently on the depth and treatment (Table 3 and Figure 5A). Growth in thickness of L. stictiforme thalli was influenced by the interaction of all conditions (site, depth, and treatment, Table 4): in particular, in the warm site (CC) thalli transplanted from the cold site (CP) had a better performance than the local (translocated) algae and had higher growth in thickness at 34 m of depth than at 23 m (Figure 5B). Moreover, differences between translocated thalli were also found at 34 m of depth (CP>CC) and in CC between depths (23>34 m, Figure 5B). The number of conceptacles was significantly higher in CP than in CC site, independently on the combination of the other factors (depth and treatment, Table 5 and Figure 5C).
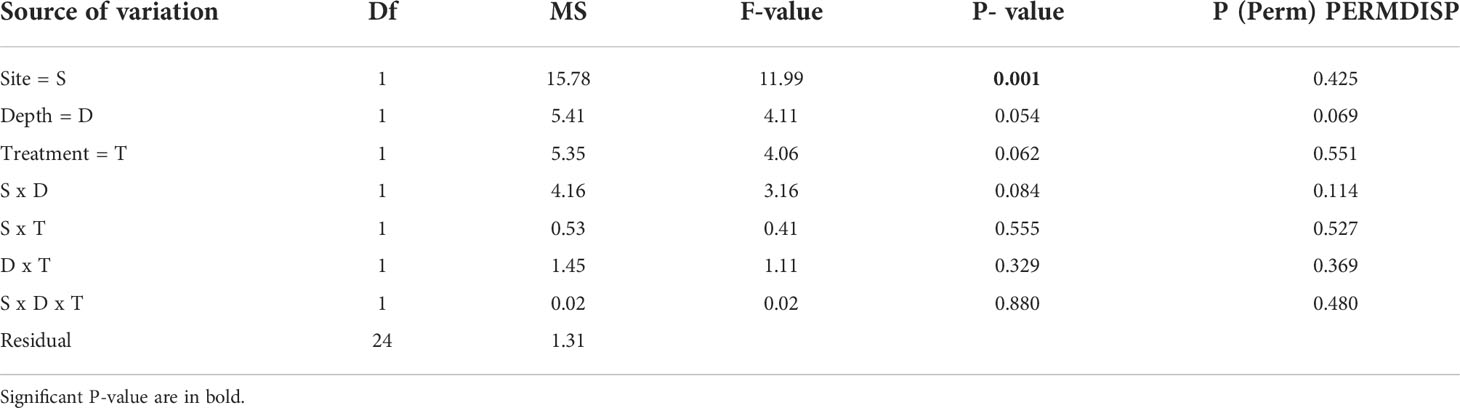
Table 3 BSE - L. stictiforme thallus marginal growth: results of PERMANOVA testing the effect of Site (CP vs CC), Depth (23 vs 34 m) and Treatment (transplant=TRP vs translocated=TRL).
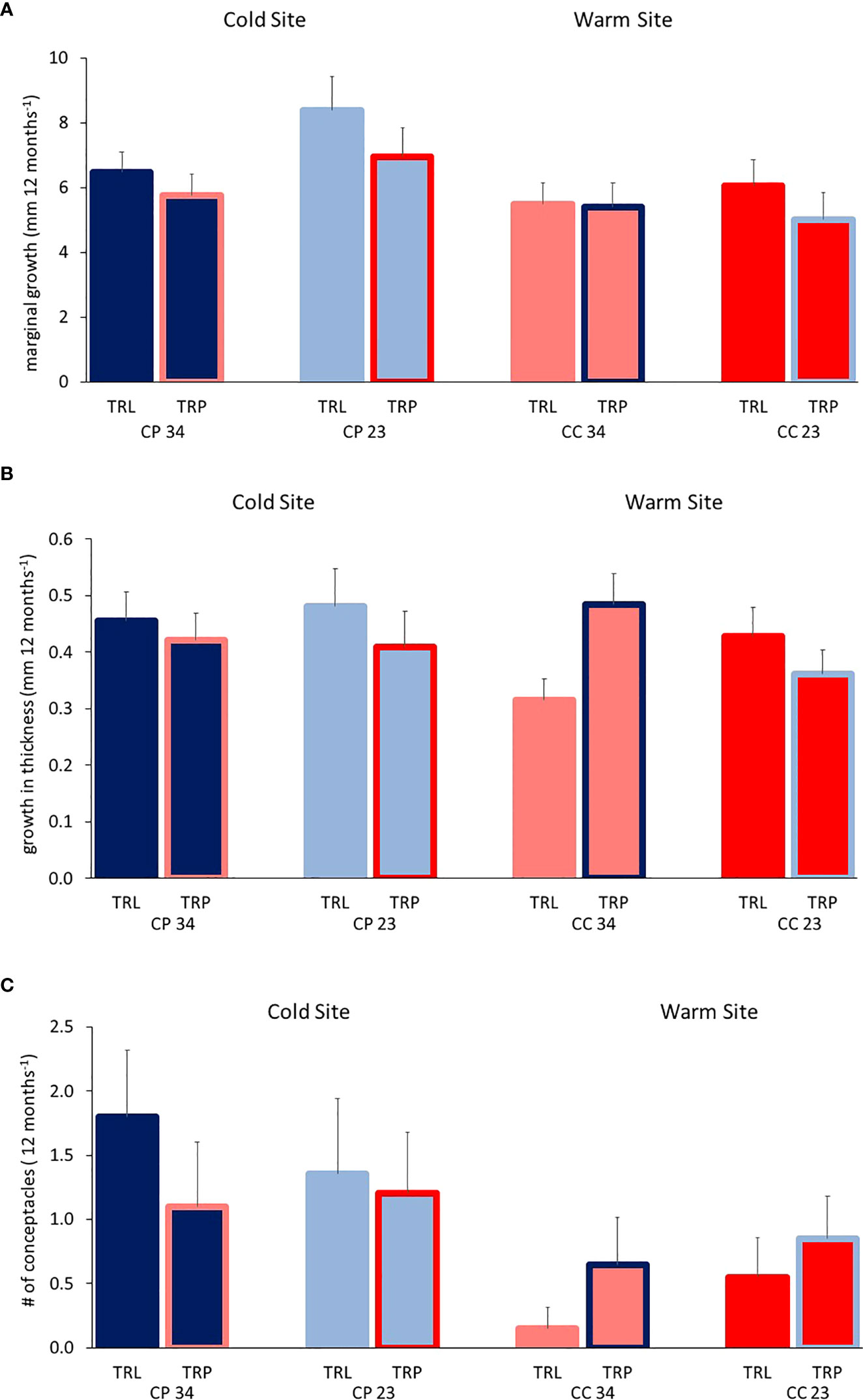
Figure 5 BSE: Average (+SE) L. stictiforme (A) marginal growth, (B) growth in thickness and (C) number of new conceptacles at the two sites (CP and CC), two depths (23 and 34 m), and between treatments (TRL, translocated and TRP, transplanted).
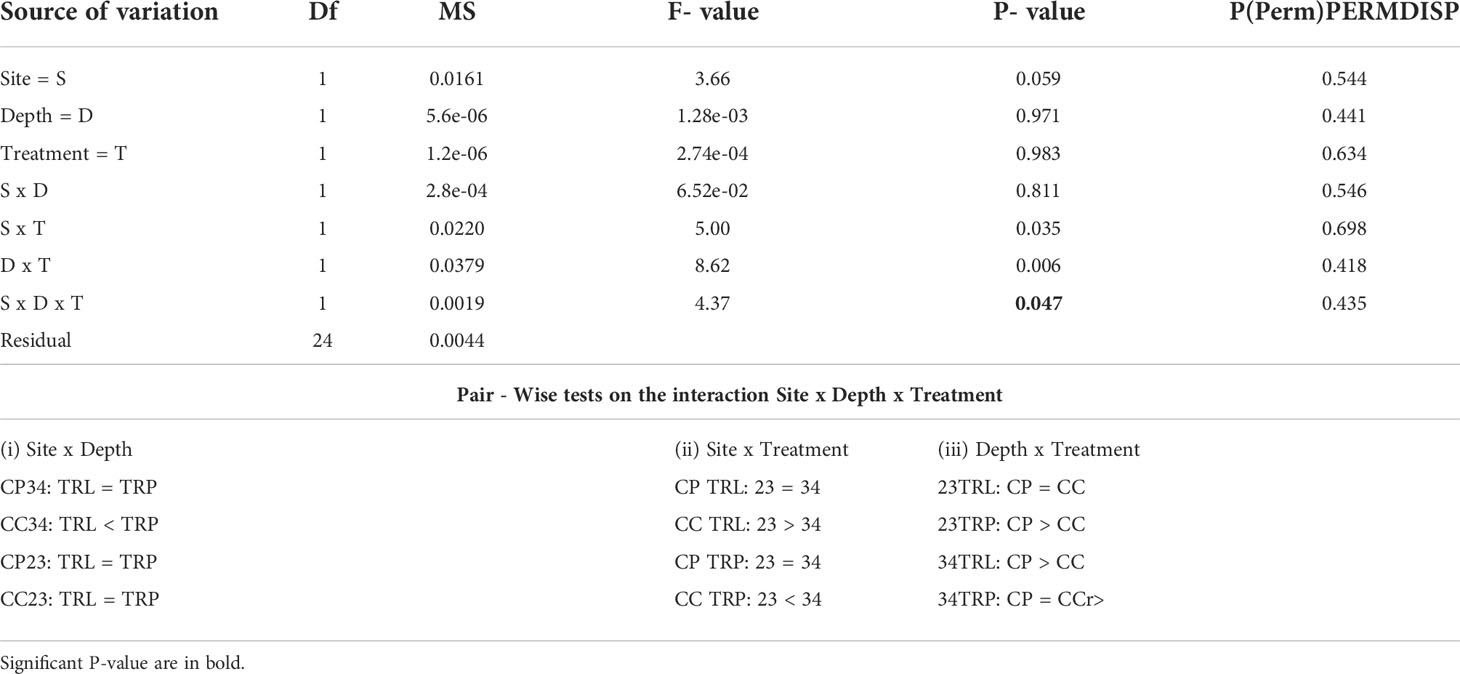
Table 4 BSE - L. stictiforme growth in thickness: results of PERMANOVA testing the effect of Site (CP vs CC), Depth (23 vs 34 m) and Treatment (transplant=TRP vs translocated=TRL) and Pair – Wise tests on the interaction Site x Depth x Treatment.
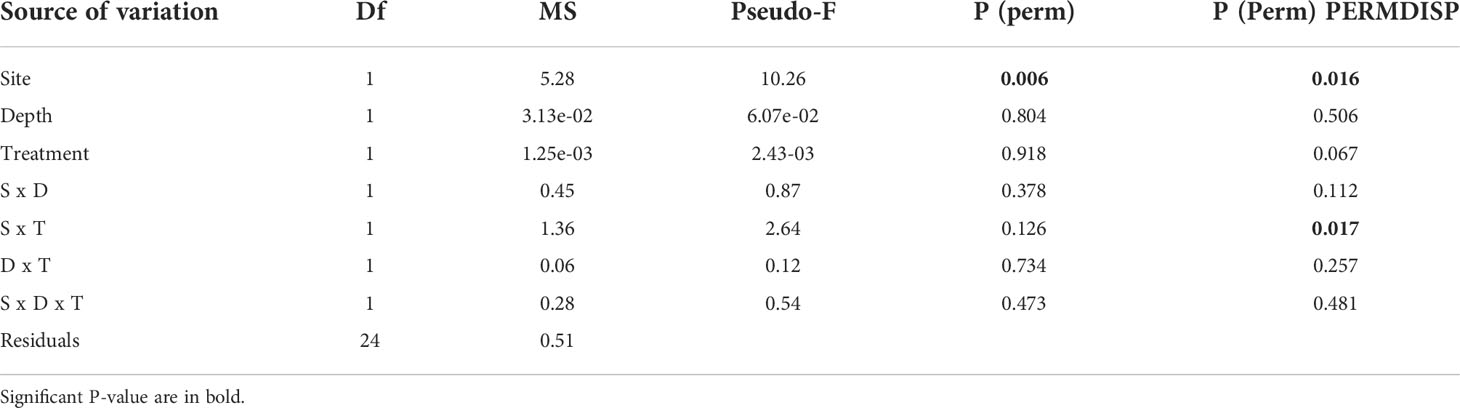
Table 5 BSE - L. stictiforme number of conceptacles: results of PERMANOVA testing the effect of Site (CP vs CC), Depth (23 vs 33 m) and Treatment (transplant=TRP vs translocated=TRL).
Between depths experiment
The temperature experienced by L. stictiforme at 15 m (L TRP, H TRP and H TRL) was warmer than at 34 m (L TRL) in terms of both maximum and average temperature, the difference being 2.08°C and 4.23°C, respectively (Figure 6A). Light irradiance between the L TRL and L TRP was similar, indicating that the natural cavity used for the experiment was adequate to reproduce the 34 m irradiance (the highest intensities recorded were 36.67 and 36.36 μmol m−2 s−1, for the two treatments, respectively), while irradiance levels at the exposed 15 m treatments (H TRL and H TRP) were much higher (highest intensity 160.49 μmol m−2 s−1, Figure 6B).
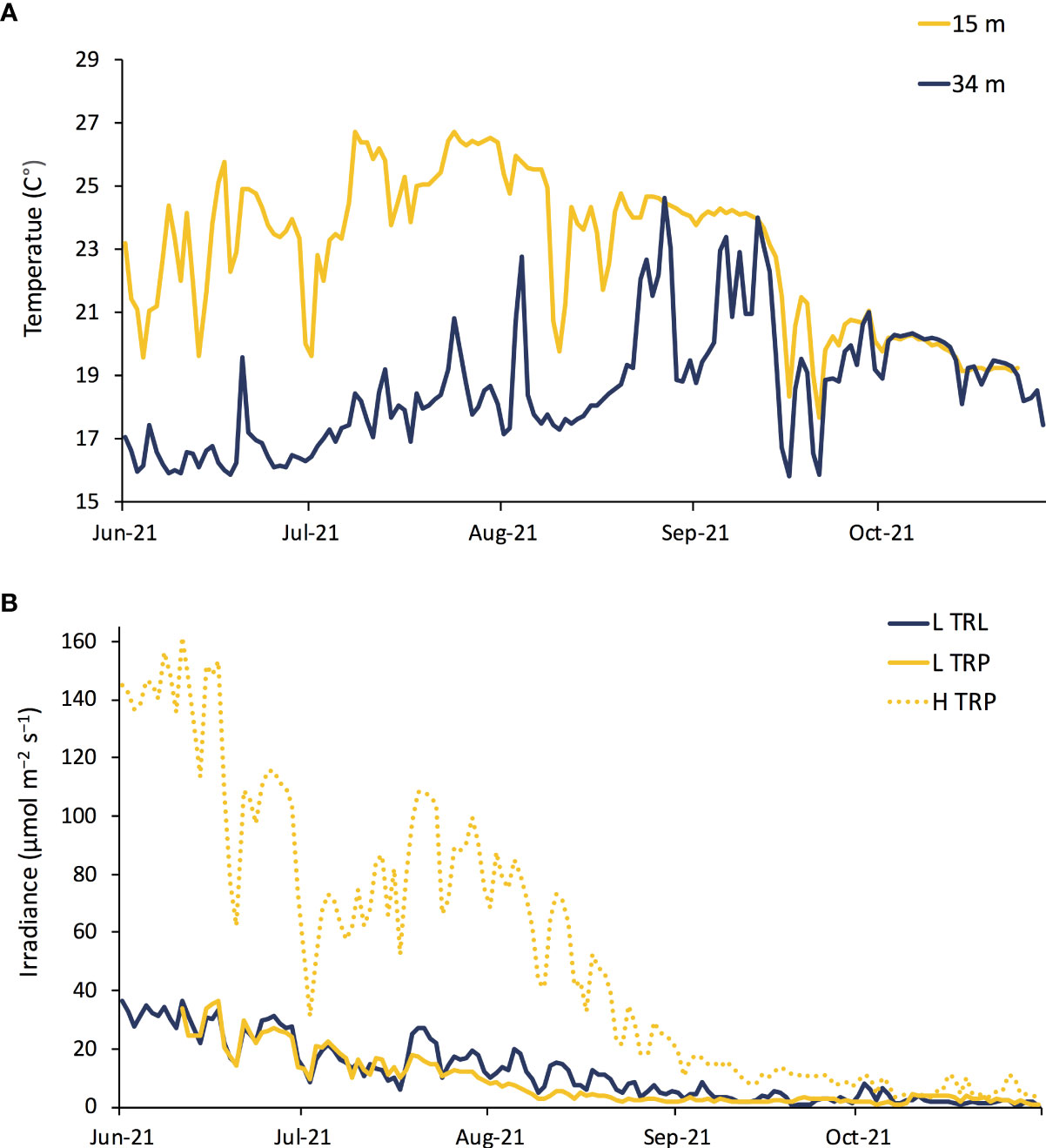
Figure 6 BDE: Daily (A) seawater temperature and (B) irradiance from June to November 2021: conditions at 34 m of depth L TRL in blue, at 15 m L TRP in solid yellow and H TRL and H TRP in dotted yellow.
All algae appeared healthy for the whole study period of the BDE. A higher marginal growth and growth in thickness in L. stictiforme thalli was found at low light conditions (L TRL and L TRP) compared to the high irradiance treatments (H TRL and H TRP, Table 6 and Figures 7A, B), regardless the thallus origin (H TRP = H TRL, Figure 7B). Algae produced conceptacles only where irradiance condition was low, although the higher production occurred in the transplanted algae rather than the translocated (L TRP >L TRL, one-way PERMANOVA, F3,12 = 2.04, P= 0.148).
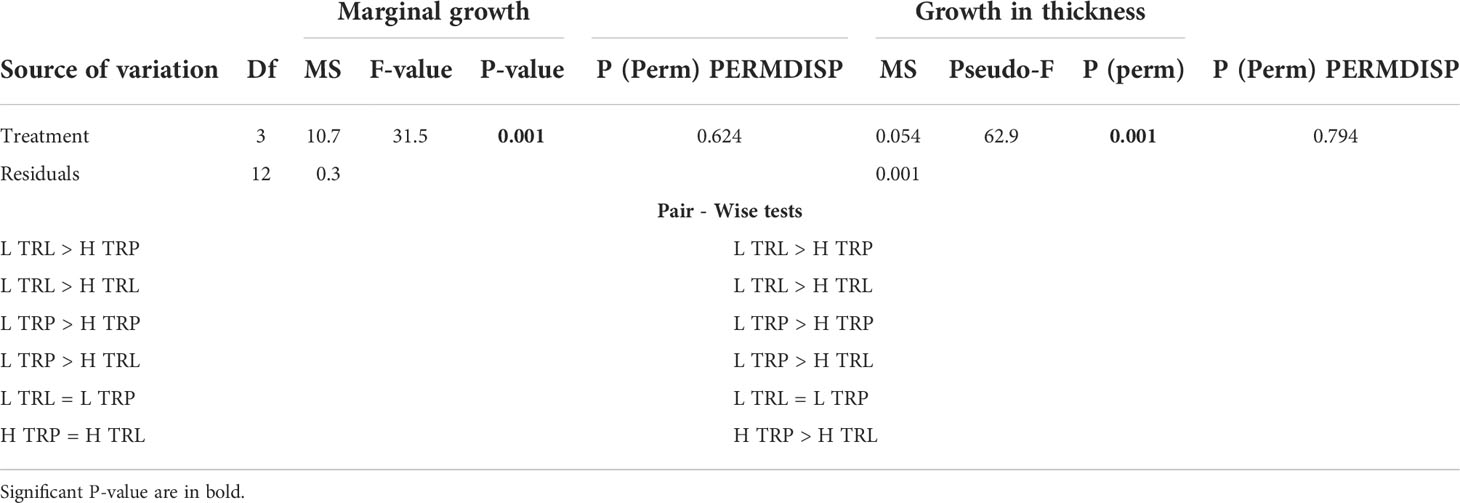
Table 6 BDE - L. stictiforme marginal growth and growth in thickness: results of PERMANOVA testing the effect of treatment (L TRL, L TRP, H TRP, and H TRL).
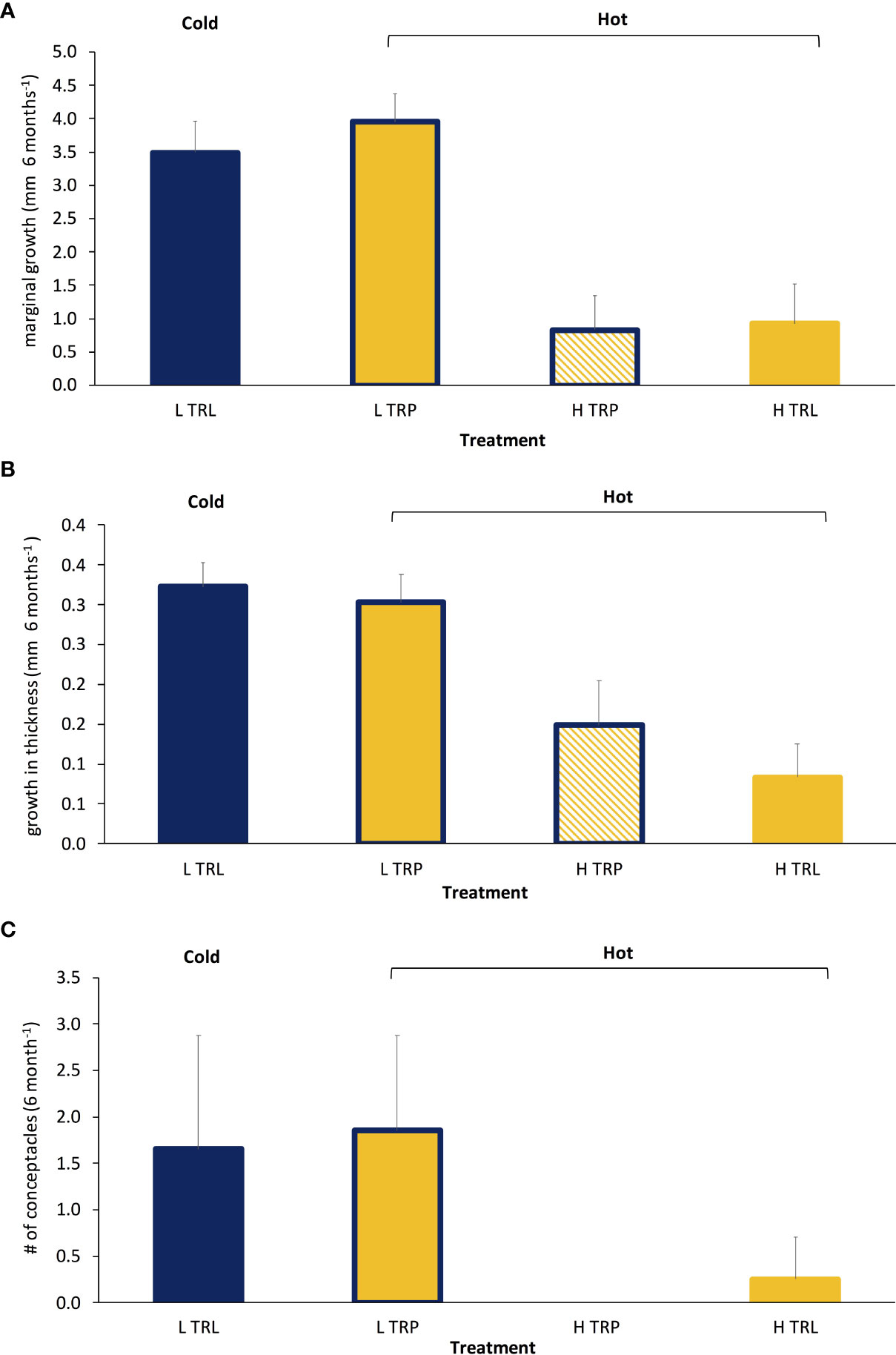
Figure 7 BDE: Average (+SE) L. stictiforme (A) marginal growth, (B) growth in thickness, and (C) number of conceptacles at the four treatments (L TRL, translocated at low irradiance; L TRP, transplanted at low irradiance; H TRP, transplanted at high irradiance and H TRL, translocated at high irradiance). Blue refers to cold and yellow to warm conditions.
Discussion
This is the first study that provides in situ data on the performance of an important bioconstructor of Mediterranean coralligenous reefs by examining the responses of the red crustose alga L. stictiforme to different temperature and light conditions. First, it was shown that L. stictiforme mainly exhibits marginal growth rather than increase in thickness, confirming that this species invests in lateral expansion of thalli, although important differences both in growth and in conceptacles production between sites were evidenced in the BSE. Both thallus growth and number of conceptacles of L. stictiforme had a better performance at the cold site (CP). Differences between treatments were only found at CC 34 m of depth where thalli of CP origin had a higher growth in thickness than the local thalli. At the warmer CC site, a higher thickening was also recorded at 23 m in translocated thalli and at 34 m of depth in the transplanted thalli. Overall, the growth values found in the present study for L. stictiforme exceed those reported in the literature for specimens of CCA, probably referable to the same species, although direct comparison should not be done as the units of measurement and methods differ between the experiments. However, Garrabou and Ballesteros (2000) found a maximum growth of 1.96 mm2 and 0.87 mm2 month-1for Mesophyllum alternans and Lithophyllum frondosum, respectively, while concerning the growth in thickness for both species, a thickening in the order of μm was observed. Similarly, Basso and Rodondi (2007) also found growth values in the same order of measurement (i.e. μm).
The treatments provided several interesting insights. In BSE, the marginal growth and the production of new conceptacles of L. stictiforme were significantly higher in the colder site than in the warmer one, independently on the depth and treatment. The influence of the site factor showed that although there were no significant differences between treatments, the marginal growth and number of conceptacles of the transplanted thalli were consistent with the thalli of the transplant sites, suggesting that the performance of the transplanted thalli may be comparable to those of the controls in both directions. Conversely, a higher increase in thickness was observed in the deeper CP algae (34 m) compared to the controls. Therefore, the conditions experienced at the warm site were not only tolerated, but the performance indicates good adaptability of the CP specimens to the warmer environment. This is particularly relevant in the context of ocean warming and the sensitivity of L. stictiforme to this stressor. The fact that a higher thickness of CP algae seemed promoted by the warmer conditions only at the deeper CC areas opens several scenarios. First, at the CC site the high light irradiance at 23 m could disadvantage the growth promoted by the warm temperature and algae at 34 m of depth could benefit from a darker environment: in fact, evidence gained by a laboratory experiment on this species supported the interacting effect of temperature and irradiance, providing information about the importance of levels for each of the two factors to make accurate predictions on their effects (Rodríguez-Prieto, 2016). However, comparing the conditions that the algae experienced in the field during the BSE with those set in the laboratory experiments can be misleading because the real natural variability cannot be reproduced in aquaria and because the specimens used in Rodríguez-Prieto’s study were collected in Catalonia (eastern Spain). Although probably belonging to the same species, they may be adapted to different local conditions compared to the CP specimens. On the other hand, differences between TRP and TRL at the two depths in CC could be attributed to different adaptations of the CP algae (23 m vs 34 m) and therefore to different performance thresholds. In order to interpret whether the different performance (growth in thickness) of the CP algae in the BSE depended on temperature and irradiance conditions, the data collected should be integrated by testing the two factors on the algal performance across gradients likely using respiration chambers with different sensors such as dissolved oxygen and temperature, to measure metabolic rates (e.g. Anton et al., 2020): much of the experimental evidence gained in laboratory on L. stictiforme (or L. cabiochae) is not based on gradients, but only on increases of 3°C over the ambient temperature (Martin and Gattuso, 2009; Diaz-Pulido et al., 2012; Martin et al., 2013a; Martin et al., 2013b; Nash et al., 2016), because they tested hypotheses derived from modeling predictions for the end of the century (IPCC, 2019).
Results obtained from the BDE have provided solid evidence that the 15 m temperature during summer and autumn (warm) was tolerated by the 34 m algae at both irradiance conditions (L TRP compared to L TRL). The comparison between H TRP and L TRP allowed disentangling the temperature from the irradiance effect, as the 15 m irradiance had a negative effect on the L. stictiforme performance, regardless of the origin (H TRP = H TRL) of the algae. In particular, the most affected trait was the formation of reproductive structures since no new conceptacles were detected at the end of experiment in the light exposed thalli (H TRP and H TRL). Accordingly, Rodríguez-Prieto (2016) highlighted that irradiance was the most limiting factor when compared with temperature. Furthermore, it is noteworthy that no trade-off has been found on the growth and formation of conceptacles. In fact, no significant differences between L TRL and L TRP were found in all variables considered. A possible explanation is that all the considered response variables depend on the same calcification dynamics and L TRP conditions were too harsh for L. stictiforme tolerance thresholds. By fact, such insights suggest the hypothesis that future deepening of the thermocline (Hoegh-Guldberg and Bruno, 2010) can possibly have no effect on the marginal growth of the algae and the conceptacle formation, even though growth in thickness will not be affected. This fact would disagree with the theory that marginal growth and growth in thickness are the result of a trade-off in CCA (Dethier and Steneck, 2001), probably as a result of energetic constraints and biotic interactions effects that incur different maintenance costs (McCoy and Ragazzola, 2014; Legrand et al., 2019). Our results also provide evidence that L. stictiforme may be more resistant to the effects of OW than widely believed. Nevertheless, as thick CCA such as L. stictiforme are foundation species, their adaptability to warming could also favor the future establishment of other species of the coralligenous reefs due to an increase in substrate availability. In this context the fact that conceptacles of L. stictiforme were produced in the warmer conditions (L TRP) supports the expectation that even sexual reproduction will be valuable in the future warming, even if at the moment it is unknown whether abundance of conceptacles reflects both their reproductive strategy (spores or gametes) and their effectiveness.
Interestingly, comparing the performance of algae across the two experiments for the deepest bathymetry in the CP site (the marginal growth was 6.31 mm ± 0.8 in 12 months, i.e. 0.53 mm per month-1 in the BSE, and 3.42 ± 0.19 in 6 months, i.e. 0.57 mm month-1 in the BDE), no seasonal growth pattern is suggested. This result would not be in accordance with Martin et al. (2013a), who obtained a higher growth rate in the hot seasonal period (in L TRL in the BDE). Therefore, further information is needed to estimate the seasonal performance of this species, even because some contrasting evidence was provided by the BSE and BDE for the growth in thickness (0.42 mm and 0.31 mm on average in the 12 months and in the hot 6 months, respectively), supporting the theory that the performance of L. stictiforme depends on complex mechanisms.
The healthy status and survival of the manipulated specimens in the BSE and BDE are overall quite unexpected and encouraging in the warming scenario: studies focusing on temperate (Martin and Gattuso, 2009) and tropical CCA (Anthony et al., 2008; Diaz-Pulido et al., 2012) highlighted that 3°C over the ambient temperature have a negative effect, especially for the tropical ones, as they live close to their thermal limit (Pörtner, 2010), while in the present study all algae were found alive and healthy, including those that were exposed for 6 months to 4°C above the control condition (BDE). This wide tolerance of temperature variation, especially to the combination + 4°C plus MHWs is particularly noteworthy for a deep subtidal species, especially for specimens distributed under the thermocline depth (such as the 34 m L. stictiforme), as it would rather be expected for macroalgae living in much more variable conditions, such as in intertidal habitats (Pakker et al., 1995). Overall, although evidence will need to be corroborated with other field experiments, the adaptability of L. stictiforme here highlighted between sites and depths is encouraging even in relation to the possible use of transplants of this bioconstructor for future restoration actions.
Finally, as general point concerning all ecological and physiological studies on Mediterranean corallines, we stress the critical importance of a correct identification of the material used in the experiments. The identification should be confirmed using DNA sequence data, particularly when dealing with taxa for which the existence of cryptic species is known (Twist et al., 2020). The use of specimens belonging to different cryptic species might lead to ambiguous results that may potentially result into wrong conclusions and interpretations. In the case of this study, budget limitations allowed us to sequence only two specimens. However, their psbA sequences confirmed the morphology-based identification (Figure S2). Given the general morphological uniformity of the specimens used in our experiments, we believe that they all belong to Lithophyllum stictiforme and that the possibility of the occurrence of additional cryptic species is very remote. We acknowledge, however, that we cannot exclude this possibility with absolute certainty. In theory, one or more of the cryptic species discovered by De Jode et al. (2019) might have been present. In consideration of this, we recommend that all future studies on the physiology and responses to climate change of Mediterranean Lithophyllum should include DNA sequence data, at least for a part of the specimens used in the experiments.
Data availability statement
The raw data supporting the conclusions of this article will be made available by the authors, without undue reservation.
Author contributions
FP, AC, LP, FRa, FRi and GC conceived and design the experiment; FP, LP and PS carried out the field survey; FP and LP analyzed the data; FP and PS carried out graphs and tables; FP and GC led the writing of the manuscript. All authors contributed to the article and approved the submitted version.
Funding
The study has been funded by Italian Ministry of Education and Research: PRIN 2017 (MHHWBN) “Marine Habitats restoration in a climate change-impaired Mediterranean Sea (MAHRES)”. It was also supported by “Fondo di Ateneo per la Ricerca 2019” by the University of Sassari.
Acknowledgments
We are grateful to Capo Carbonara Marine Protected Area and Costa Paradiso Diving Center for providing permissions, field, and logistical assistance. We are also grateful to Leonardo Casini for laboratory assistance and to Maria Francesca Cinti, Mario De Luca and Arianna Pansini for their field survey support.
Conflict of interest
The authors declare that the research was conducted in the absence of any commercial or financial relationships that could be construed as a potential conflict of interest.
Publisher’s note
All claims expressed in this article are solely those of the authors and do not necessarily represent those of their affiliated organizations, or those of the publisher, the editors and the reviewers. Any product that may be evaluated in this article, or claim that may be made by its manufacturer, is not guaranteed or endorsed by the publisher.
Supplementary material
The Supplementary Material for this article can be found online at: https://www.frontiersin.org/articles/10.3389/fmars.2022.930750/full#supplementary-material
References
Ådal E., Lundberg P., Jonzèn N. (2006). From climate change to population change: The need to consider annual life cycles. Glob. Change Biol. 12, 1627–1633. doi: 10.1111/j.1365-2486.2006.01196.x
Adey W. E., Steneck R. S. (2001). Thermogeography over time creates biogeographic regions: A temperature/space/time-integrated model and an abundance-weighted test for benthic marine algae. J. Phycol. 37, 677–698. doi: 10.1046/j.1529-8817.2001.00176.x
Anderson M. J. (2001). A new method for non-parametric multivariate analysis of variance. Austral Ecol. (Devon, UK: PRIMER -E Ltd)26, 32–46. doi: 10.1046/j.1442-9993.2001.01070.x
Anderson M. J. (2006). Distance-based test for homogeneity of multivariate dispersions. Biometrics 62, 245–253. doi: 10.1111/j.1541-0420.2005.00440.x
Anderson M. J., Gorley R. N., Clarke K. R. (2008). “PERMANOVA+ for PRIMER,” in Guide to software and statistical methods.
Anthony K. R. N., Kline D. I., Diaz-Pulido G., Dove S., Hoegh-Guldberg O. (2008). Ocean acidification causes bleaching and productivity loss in coral reef builders. Proc. Natl. Acad. Sci. U.S.A. 105 (45), 17442–17446. doi: 10.1073/pnas.0804478105
Anton A., Randle J. L., Garcia F. C., Rossbach S., Ellis J. I., Weinzierl M., et al. (2020). Differential thermal tolerance between algae and corals may trigger the proliferation of algae in coral reefs. Glob. Change Biol. 26 (8), 4316–4327. doi: 10.1111/gcb.15141
Assis J., Lucas A. V., Bárbara I., Serrão E. Á. (2016). Future climate change is predicted to shift long-term persistence zones in the cold-temperate kelp Laminaria hyperborea. Mar. Environ. Res. 113, 174–182. doi: 10.1016/j.marenvres.2015.11.005
Ballesteros E. (2006). Mediterranean Coralligenous assemblages: A synthesis of present knowledge. Oceanogr. Mar. Biol. 44 (An Annual Review), 123–195. doi: 10.1016/j.jenvman.2012.07.024
Barisiello O., Bernabei S., Bucci M., Casazza G., Cavalieri S., Comperini W., et al. (2002). Qualità delle acque marino costiere. annuario dei dati ambientali (Rome, Italy: Agenzia Nazionale per la Protezione dell’Ambiente), 256–274.
Basso D., Rodondi G. (2007). Valutazione del tasso di crescita in coltura delle alghe calcaree Lithophyllum stictaeforme e Mesophyllum lichenoides: primi risultati. Biol. Mar. Mediterr. 14 (3), 172–173.
Blake C., Maggs C. A. (2003). Comparative growth rates and internal banding periodicity of maerl species (Corallinales, rhodophyta) from northern Europe. Phycologia 42 (6), 606–612. doi: 10.2216/i0031-8884-42-6-606.1
Blanfuné A., François Boudouresque C., Thibaut T., Verlaque M. (2016). “Sub-Chapter 2.2.3. the sea level rise and the collapse of a Mediterranean ecosystem, the lithophyllum byssoides algal rim,” in The Mediterranean region under climate change: A scientific update. IRD Éditions (Marseille: IRD Éditions) EdsMoatti J., Thiébault S. doi: 10.4000/books.irdeditions.23472
Bosence D. W. J. (1983). Description and classification of Rhodoliths (Rhodoids, Rhodolites). In: Coated Grains. Perry T.M. Ed., pp. 217–224. Berlin: Springer Verlag.
Bruno J. F., Bates A. E., Cacciapaglia C., Pike E. P., Amstrup S. C., Van Hooidonk R., et al. (2018). Climate change threatens the world’s marine protected areas. Nat. Clim. Change 8 (6), 499–503. doi: 10.1038/s41558-018-0149-2
Bruno J. F., Carr L. A., O’Connor M. I. (2015). Exploring the role of temperature in the ocean through metabolic scaling. Ecology 96 (12), 3126–3140. doi: 10.1890/14-1954.1
Canals M., Ballesteros E. (1997). Production of carbonate particles by phytobenthic communities on the mallorca-menorca shelf, northwestern Mediterranean sea. deep-Sea research part II. Trop. Stud. Oceanogr. 44 (3–4), 611–629. doi: 10.1016/S0967-0645(96)00095-1
Caragnano A., Basso D., Jacob D. E., Storz D., Rodondi G., Benzoni F., et al. (2014). The coralline red alga Lithophyllum kotschyanum f. affine as proxy of climate variability in the Yemen coast, gulf of Aden (NW Indian ocean). Geochim. Cosmochim. Acta 124, 1–17. doi: 10.1016/j.gca.2013.09.021
Carpenter K. E., Abrar M., Aeby G., Aronson R. B., Banks S., Bruckner A., et al. (2008). One-third of reef-building corals face elevated extinction risk from climate change and local impacts. Science 321 (5888), 560–563. doi: 10.1126/science.1159196
Ceccherelli G., Pinna F., Pansini A., Piazzi L., La Manna G. (2020). The constraint of ignoring the subtidal water climatology in evaluating the changes of coralligenous reefs due to heating events. Sci. Rep. 10 (1), 1–13. doi: 10.1038/s41598-020-74249-9
Cerrano C., Bavestrello G., Bianchi C. N., Cattaneo-Vietti R., Bava S., Morganti C., et al. (2000). A catastrophic mass-mortality episode of gorgonians and other organisms in the ligurian Sea (North-western Mediterranean). Ecol. Lett. 3 (4), 284–293. doi: 10.1046/j.1461-0248.2000.00152.x
Chapman M. G. (1986). Assessment of some controls in experimental transplants of intertidal gastropods. J. Exp. Mar. Biol. Ecol. 103 (1–3), 181–201. doi: 10.1016/0022-0981(86)90140-1
Chatalov A., Bonev N., Ivanova D. (2015). Depositional characteristics and constraints on the mid-valanginian demise of a carbonate platform in the intra-tethyan domain, circum-rhodope belt, northern Greece. Cretac. Res. 55, 84–115. doi: 10.1016/j.cretres.2015.02.001
Chave J. (2013). The problem of pattern and scale in ecology: What have we learned in 20 years? Ecol. Lett. 16, 4–16. doi: 10.1111/ele.12048
Collins M., An S., Cai W., Ganachaud A., Guilyardi E., Jin F. F., et al. (2010). The impact of global warming on the tropical pacific ocean and El nĩno. Nat. Geosci. 3 (6), 391–397. doi: 10.1038/ngeo868
Coll M., Piroddi C., Steenbeek J., Kaschner K., Lasram F. B. R., Aguzzi J., et al. (2010). The biodiversity of the Mediterranean Sea: Estimates, patterns, and threats. PloS One 97(3), 549–560. doi: 10.1371/journal.pone.0011842
Cormaci M., Furnari G., Alongi G. (2017). Flora marina bentonica del mediterraneo: Rhodophyta (Rhodymeniophycidae escluse). Bull. Acc. Gioenia Sci. Nat. Catania. 50 (380), 1–391. doi: 10.35352/gioenia.v53i383.87
Cornwall C. E., Diaz-Pulido G., Comeau S. (2019). Impacts of ocean warming on coralline algae: Knowledge gaps and key recommendations for future research. Front. Mar. Sci. 6 (186). doi: 10.3389/fmars.2019.00186
Crozier L. G. W., Zabel R., Hamlet A. F. (2008). Predicting differential effects of climate change at the population level with life-cycle models of spring Chinook salmon. Glob. Change Biol. 14, 236–249. doi: 10.1111/j.1365-2486.2007.01497.x
De Jode A., David R., Haguenauer A., Cahill A. E., Erga Z., Guillemain D., et al. (2019). From seascape ecology to population genomics and back. Spatial and ecological differentiation among cryptic species of the red algae Lithophyllum stictiforme/L. cabiochiae, main bioconstructors of coralligenous habitats. Mol. Phylogenet. Evol. 137, 104–113. doi: 10.1016/j.ympev.2019.04.005
Dethier M. N., Steneck R. S. (2001). Growth and persistence of diverse intertidal crusts: Survival of the slow in a fast-paced world. Mar. Ecol. Prog. Ser. 223, 89–100. doi: 10.3354/meps223089
Diaz-Pulido G., Anthony K. R. N., Kline D. I., Dove S., Hoegh-Guldberg O. (2012). Interactions between ocean acidification and warming on the mortality and dissolution of coralline algae. J. Phycol. 48 (1), 32–39. doi: 10.1111/j.1529-8817.2011.01084.x
Diaz-Pulido G., Nash M. C., Anthony K. R. N., Bender D., Opdyke B. N., Reyes-Nivia C., et al. (2014). Greenhouse conditions induce mineralogical changes and dolomite accumulation in coralline algae on tropical reefs. Nat. Commun. 5, 3310. doi: 10.1038/ncomms4310
Etterson J. R., Shaw R. G. (2001). Constraint to adaptive evolution in response to global warming. Science 294 (5540), 151–154. doi: 10.1126/science.1063656
Foster M. S. (2001). Rhodoliths: Between rocks and soft places. J. Phycol. 37 (5), 659–667. doi: 10.1046/j.1529-8817.2001.00195.x
Foster M. S., Amado Filho G. M., Kamenos N. A., Risomena-Rodríguez R., Steller D. L. (2013). Rhodoliths and rhodolith beds. Smithsonian Contribution to Mar. Sci. 39, 143–55.
Fragkopoulou E., Serrão E. A., Horta P. A., Koerich G., Assis J. (2021). Bottom trawling threatens future climate refugia of rhodoliths globally. Front. Mar. Sci. 7. doi: 10.3389/fmars.2020.594537
Garrabou J., Ballesteros E. (2000). Growth of Mesophyllum alternans and Lithophyllum frondosum (Corallinales, rhodophyta) in the northwestern Mediterranean. Eur. J. Phycol. 35(1), 1–10 doi: 10.1080/09670260010001735571
Gauzens B., Rall B. C., Mendonça V., Vinagre C., Brose U. (2020). Biodiversity of intertidal food webs in response to warming across latitudes. Nat. Clim. Change 10 (3), 264–269. doi: 10.1038/s41558-020-0698-z
Guiry M. D., Guiry G. M. (2022). AlgaeBase (Galway: World-wide electronic publication, National University of Ireland). Available at: https://www.algaebase.org.
Halfar J., Steneck R. S., Joachimski M., Kronz A., Wanamaker A. D. (2008). Coralline red algae as high-resolution climate recorders. Geology 36 (6), 463–466. doi: 10.1130/G24635A.1
Hereu B., Kersting D. K. (2016). Diseases of coralline algae in the Mediterranean Sea. Coral Reefs 35, 713. doi: 10.1007/s00338-016-1428-x
Hetzinger S., Halfar J., Kronz A., Steneck R. S., Adey W., Lebednik P. A., et al. (2009). High-resolution Mg/Ca ratios in a coralline red alga as a proxy for Bering Sea temperature variations from 1902 to 1967. Palaios 24 (6), 406–412. doi: 10.2110/palo.2008.p08-116r
Hobday A. J., Alexander L. V., Perkins S. E., Smale D. A., Straub S. C., Oliver E. C., et al. (2016). A hierarchical approach to defining marine heatwaves. Prog. Oceanogr. 141, 227–238. doi: 10.1016/j.pocean.2015.12.014
Hobday A. J., Oliver E. C. J., Sen Gupta A., Benthuysen J. A., Burrows M. T., Donat M. G., et al. (2018). Categorizing and naming marine heatwaves. Oceanography 31 (2), 162–173. doi: 10.5670/oceanog.2018.205
Hoegh-Guldberg O., Bruno J. F. (2010). The impact of climate change on the world’s marine ecosystems. Science 328 (5985), 1523–1528. doi: 10.1126/science.1189930
Hoegh-Guldberg O., Poloczanska E. S. (2017). Editorial: The effect of climate change across ocean regions. Front. Mar. Sci. 4. doi: 10.3389/fmars.2017.00361
Hughes T. P., Kerry J. T., Baird A. H., Connolly S. R., Dietzel A., Eakin C. M., et al. (2018). Global warming transforms coral reef assemblages. Nature 556 (7702), 492–496. doi: 10.1038/s41586-018-0041-2
Hurd C. L. (2015). Slow-flow habitats as refugia for coastal calcifiers from ocean acidification. J. Phycol. 51, 599–605. doi: 10.1111/jpy.12307
IPCC (2019). Special report on the ocean and cryosphere in a changing climate. Eds. Poörtner H.-O., Roberts D. C., Masson-Delmotte V., Zhai P., Tignor M., Poloczanska E. K., Mintenbeck K., Alegría A., Okem A., Petzold J., Rama B., Wever M. N., In Press.
IPCC (2021). “Climate change 2021: The physical science basis,” in Contribution of working group I to the sixth assessment report of the intergovernmental panel on climate change. Eds. Masson-Delmotte V., Zhai P., Pirani A., Connors S. L., Péan C., Berger S., Caud N., Chen Y., Goldfarb L., Gomis M. I., Huang M., Leitzell K., Lonnoy E., Matthews J. B. R., Maycock T. K., Waterfield T., Yelekçi O., Yu R., Zhou B. (Cambridge University Press). In Press.
Kamenos N. A., Cusack M., Moore P. G. (2008). Coralline algae are global palaeothermometers with bi-weekly resolution. Geochim. Cosmochim. Acta 72 (3), 771–779. doi: 10.1016/j.gca.2007.11.019
Kim J. H., Kim N., Moon H., Lee S., Jeong S. Y., Diaz-Pulido G., et al. (2020). Global warming offsets the ecophysiological stress of ocean acidification on temperate crustose coralline algae. Mar. pollut. Bull. 157, 111324. doi: 10.1016/j.marpolbul.2020.111324
Kubicek A., Breckling B., Hoegh-Guldberg O., Reuter H. (2019). Climate change drives trait-shifts in coral reef communities. Sci. Rep. 9 (1), 1–10. doi: 10.1038/s41598-019-38962-4
Legrand E., Riera P., Lutier M., Coudret J., Grall J., Martin S. (2019). Grazers increase the sensitivity of coralline algae to ocean acidification and warming. J. Sea Res., 148–149. doi: 10.1016/j.seares.2019.03.001
Littler M. M., Littler D. S. (1984). Relationships between macroalgal functional form groups and substrata stability in a subtropical rocky-intertidal system. J. Exp. Mar. Biol. Ecol. 74, 13–34. doi: 10.1016/0022-0981(84)90035-2
Louthan A. M., Morris W. (2021). Climate change impacts on population growth across a species’ range differ due to nonlinear responses of populations to climate and variation in rates of climate change. PloS One 16, 1–20. doi: 10.1371/journal.pone.0247290
Marbà N., Duarte C. M. (2010). Mediterranean Warming triggers seagrass (Posidonia oceanica) shoot mortality. Glob. Change Biol. 16 (8), 2366–2375. doi: 10.1111/j.1365-2486.2009.02130.x
Martin S., Charnoz A., Gattuso J. P. (2013a). Photosynthesis, respiration and calcification in the Mediterranean crustose coralline alga Lithophyllum cabiochae (Corallinales, rhodophyta). Eur. J. Phycol. 48 (2), 163–172. doi: 10.1080/09670262.2013.786790
Martin S., Cohu S., Vignot C., Zimmerman G., Gattuso J. P. (2013b). One-year experiment on the physiological response of the Mediterranean crustose coralline alga, lithophyllum cabiochae, to elevated pCO2 and temperature. Ecol. Evol. 3 (3), 676–693. doi: 10.1002/ece3.475
Martin S., Gattuso J. P. (2009). Response of Mediterranean coralline algae to ocean acidification and elevated temperature. Glob. Change Biol. 15 (8), 2089–2100. doi: 10.1111/j.1365-2486.2009.01874.x
Martin S., Giannoulaki M., De Leo F., Scardi M., Salomidi M., Knitweiss L., et al. (2014). Coralligenous and maërl habitats: Predictive modelling to identify their spatial distributions across the mediterranean sea. Sci. Rep. 4, 5073. doi: 10.1038/srep05073
Martin S., Hall-Spencer J. M. (2017). “Effects of ocean warming and acidification on rhodolith/maërl beds,” in Coastal research library (Cham; Switzerland: Springer International Publishing), vol. Vol. 15. . doi: 10.1007/978-3-319-29315-8_3
McCoy S. J., Kamenos N. A. (2015). Coralline algae (Rhodophyta) in a changing world: Integrating ecological, physiological, and geochemical responses to global change. J. Phycol. 51 (1), 6–24. doi: 10.1111/jpy.12262
McCoy S. J., Ragazzola F. (2014). Skeletal trade-offs in coralline algae in response to ocean acidification. Nat. Clim. Change 4 (8), 719–723. doi: 10.1038/nclimate2273
Nash M. C., Martin S., Gattuso J. P. (2016). Mineralogical response of the Mediterranean crustose coralline alga Lithophyllum cabiochae to near-future ocean acidification and warming. Biogeosciences 13 (21), 5937–5945. doi: 10.5194/bg-13-5937-2016
Nelson W. A. (2009). Calcified macroalgae critical to coastal ecosystems and vulnerable to change: A review. Mar. Freshw. Res. 60 (8), 787–801. doi: 10.1071/MF08335
Oliver E. C. J., Donat M. G., Burrows M. T., Moore P. J., Smale D. A., Alexander L. V., et al. (2018). Longer and more frequent marine heatwaves over the past century. Nat. Commun. 9 (1), 1–12. doi: 10.1038/s41467-018-03732-9
Ordoñez A., Doropoulos C., Diaz-Pulido G. (2014). Effects of ocean acidification on population dynamics and community structure of crustose coralline algae. Biol. Bull. 226 (3), 255–268. doi: 10.1086/BBLv226n3p255
Pakker H., Breeman A. M., Prud’homme van Reine W. F., van den Hock C. (1995). A comparative study of temperature responses of Caribbean seaweeds from different biogeographic groups. J. Phycol. 31 (4), 499–507. doi: 10.1111/j.1529-8817.1995.tb02543.x
Pansini A., La Manna G., Pinna F., Stipcich P., Ceccherelli G. (2021). Trait gradients inform predictions of seagrass meadows changes to future warming. Sci. Rep. 11 (1), 1–12. doi: 10.1038/s41598-021-97611-x
Peña V., Harvey B. P., Agostini S., Porzio L., Milazzo M., Horta P., et al. (2021). Major loss of coralline algal diversity in response to ocean acidification. Glob. Ch. Biol. 27, 4785–4798. doi: 10.1111/gcb.15757
Pezzolesi L., Peña V., Le Gall L., Gabrielson P. W., Kaleb S., Hughey J. R., et al. (2019). Mediterranean Lithophyllum stictiforme (Corallinales, rhodophyta) is a genetically diverse species complex: Implications for species circumscription, biogeography and conservation of coralligenous habitats. J. Phycol. 55 (2), 473–492. doi: 10.1111/jpy.12837
Piazzi L., Ceccherelli G. (2020). Alpha and beta diversity in Mediterranean macroalgal assemblages: relevancy and type of effect of anthropogenic stressors vs natural variability. Mar. Biol. 167 (3), 32. doi: 10.1007/s00227-019-3631-0
Piazzi L., Cinti M. F., Guala I., Grech D., La Manna G., Pansini A., et al. (2021). Variations in coralligenous assemblages from local to biogeographic spatial scale. Mar. Environ. Res. 169, 105375. doi: 10.1016/j.marenvres.2021.105375
Pinna F., Piazzi L., Cinti M. F., Pansini A., Stipcich P., Ceccherelli G. (2021). Vertical variation of coralligenous cliff assemblages in marine biogeographic areas. Estuar. Coast. Shelf Sci. 261, 107554. doi: 10.1016/j.ecss.2021.107554
Poloczanska E. S., Brown C. J., Sydeman W. J., Kiessling W., Schoeman D. S., Moore P. J., et al. (2013). Global imprint of climate change on marine life. Nat. Clim. Change 3 (10), 919–925. doi: 10.1038/nclimate1958
Pörtner H.-O. (2010). Oxygen- and capacity-limitation of thermal tolerance: a matrix for integrating climate-related stressor effects in marine ecosystems. J. Exp. Biol. 213 (6), 881–893. doi: 10.1242/jeb.037523
Ragazzola F., Caragnano A., Basso D., Schmidt Daniela N., Fietzke J. (2020). Enstablishing temperate crustose early Holocene coralline algae as archives for paleoenvironmental reconstrunctions of the shallow water habitats of the Mediterranean Sea. Palaeontology 63, 155–170. doi: 10.1111/pala.12447
Ragazzola F., Foster L. C., Form A., Anderson P. S. L., Hansteen T. H., Fietzke J. (2012). Ocean acidification weakens the structural integrity of coralline algae. Glob. Change Biol. 18 (9), 2804–2812. doi: 10.1111/j.1365-2486.2012.02756.x
Regione Autonoma della Sardegna (2016). Riesame e aggiornamentodel piano di gestione del distretto idrografico della sardegna. Monitoraggio e classificazione delle acque marino-costiere. Monitoraggio e classificazione delle acque superficiali, 121–1148.
Rindi F., Braga J. C., Martin S., Peña V., Le Gall L., Caragnano A., et al. (2019). Coralline algae in a changing Mediterranean Sea: How can we predict their future, if we do not know their present? Front. Mar. Sci. 6. doi: 10.3389/fmars.2019.00723
Rivera M. G., Riosmena-Rodrìfguez R., Foster M. S. (2004). Age and growth of Lithothamnion muelleri (Corallinales, rhodophyta) in the southwestern gulf of California, Mexico. Cienc 30(1B), 235–249. doi: 10.7773/cm.v30i12.104
Rodríguez-Prieto C. (2016). Light and temperature requirements for survival, growth and reproduction of the crustose coralline Lithophyllum stictaeforme from the Mediterranean Sea. Botanica Marina 59 (2–3), 95–104. doi: 10.1515/bot-2015-0070
Scheffers B. R., De Meester L., Bridge T. C. L., Hoffmann A. A., Pandolfi J. M., Corlett R. T., et al. (2016). The broad footprint of climate change from genes to biomes to people. Science 354 (6313), aaf7671. doi: 10.1126/science.aaf7671
Schlegel W. R., Smit J. A. (2018). heatwaveR: A central algorithm for the detection of heatwaves and cold-spells. J. Open Source Software 3 (27), 821. doi: 10.21105/joss.00821
Schubert N., Schoenrock K. M., Aguirre J., Kamenos N. A., Silva J., Horta P. A., et al. (2020). Editorial: Coralline algae: Globally distributed ecosystem engineers. Front. Mar. Sci. 7. doi: 10.3389/fmars.2020.00352
Smale D. A., Wernberg T., Oliver E. C. J. J., Thomsen M., Harvey B. P., Straub S. C., et al. (2019). Marine heatwaves threaten global biodiversity and the provision of ecosystem services. Nat. Clim. Change 9 (4), 306–312. doi: 10.1038/s41558-019-0412-1
Steneck R. S. (1986). The ecology of coralline algal crusts: convergent patterns and adaptative strategies. Annu. Rev. Ecol. Systematics Vol. 17 18, 273–303. doi: 10.1146/annurev.ecolsys.17.1.273
Teichert S. (2014). Hollow rhodoliths increase svalbard’s shelf biodiversity. Sci. Rep. 4, 1–5. doi: 10.1038/srep06972
Teichert S., Woelkerling W., Rüggeberg A., Wisshak M., Piepenburg D., Meyerhöfer M., et al. (2012). Rhodolith beds (Corallinales, rhodophyta) and their physical and biological environment at 80°31′N in nordkappbukta (Nordaustlandet, Svalbard archipelago, Norway). Phycologia 51 (4), 371–390. doi: 10.2216/11-76.1
Thomson J. A., Burkholder D. A., Heithaus M. R., Fourqurean J. W., Fraser M. W., Statton J., et al. (2015). Extreme temperatures, foundation species, and abrupt ecosystem change: An example from an iconic seagrass ecosystem. Glob.Change Biol. 21 (4), 1463–1474. doi: 10.1111/gcb.12694
Twist B. A., Cornwall C. E., McCoy S. J., Gabrielson P. W., Martone P. T., Nelson W. A. (2020). The need to employ reliable and reproducible species identifications in coralline algal research. Mar. Ecol. Prog. Ser. 654, 225–231. doi: 10.3354/meps13506
Vásquez-Elizondo R. M., Enríquez S. (2016). Coralline algal physiology is more adversely affected by elevated temperature than reduced pH. Sci. Rep. 6, 1–14. doi: 10.1038/srep19030
Keywords: adaptability, crustose coralline algae, field experiment, foundation species, irradiance, MHWs, ocean warming
Citation: Pinna F, Caragnano A, Piazzi L, Ragazzola F, Stipcich P, Rindi F and Ceccherelli G (2022) The Mediterranean bioconstructor Lithophyllum stictiforme shows adaptability to future warming. Front. Mar. Sci. 9:930750. doi: 10.3389/fmars.2022.930750
Received: 28 April 2022; Accepted: 27 September 2022;
Published: 13 October 2022.
Edited by:
Anne Chenuil, Centre National de la Recherche Scientifique (CNRS), FranceReviewed by:
Valentina Asnaghi, University of Genoa, ItalyMaggy Nugues, Université de Sciences Lettres de Paris, France
Tessa M. Page, University of Southampton, United Kingdom
Copyright © 2022 Pinna, Caragnano, Piazzi, Ragazzola, Stipcich, Rindi and Ceccherelli. This is an open-access article distributed under the terms of the Creative Commons Attribution License (CC BY). The use, distribution or reproduction in other forums is permitted, provided the original author(s) and the copyright owner(s) are credited and that the original publication in this journal is cited, in accordance with accepted academic practice. No use, distribution or reproduction is permitted which does not comply with these terms.
*Correspondence: Federico Pinna, ZmVkZXJpY29waW5uYTI3QGdtYWlsLmNvbQ==