- East China Sea Fisheries Research Institute, Chinese Academy of Fishery Sciences, Shanghai, China
Larval ecology of gobiid fishes in Xiangshan Bay of the east coast of China was examined from ichthyoplanktonic data collected during 10 weekly cruises in spring–early summer of 2015. Particular attention was given to their environmental preferences and spatiotemporal habitat partitioning. A total of 87, 007 gobiid larval individuals, distributed in 9 species, were collected. The thermal and salinity preferences of 6 of the 9 gobiid larvae have been determined using a quotient-rule analysis. This work filled some gaps in larval ecology of these poorly studied gobiids. Temporal succession in gobiid larvae composition was strongly evident. The bay ecosystem was mainly occupied by Asian freshwater goby Acanthogobius ommaturus, branded goby Chaeturichthys stigmatias and tank goby Glossogobius giuris, with preferences for temperature bellow 19°C during April to early May, while striped sand goby Amoya pflaumi and burrowing goby Trypauchen vagina, which preferred water temperature above 22°C, predominated the larval assemblage in early June. With respect to spatial distribution, relatively little variation was detected among species. Most of gobiid larvae exhibited little obvious habitat preference except pinkgray goby Amblychaeturichthys hexanema and A. ommaturus, which distributed preferably in the inner zone and mouth of the bay ecosystem, respectively. Water temperature was the major structuring factor of gobiid larvae assemblage structure in the bay. The gobiid larvae exhibited to be particularly susceptible to the rapid rising temperature shocks. Sharp decline in the total abundance of gobiid larvae was detected with each rapid heating process. In addition, significant shift in larval fish composition occurred following the process. The results suggest that pattern of temperature increase in spring and early summer plays a vital role in recruitment success of the gobiid larvae.
Introduction
The spatial distribution, abundance, and composition of fish larvae are key ecological features for the conservation of biodiversity and sustainability of fisheries (Miller and Kendall, 2009). In recent decades, a large number of studies have investigated fish larvae assemblage structure and dynamics in relation to the physical and biological processes in various marine habitats and regions (e.g. Auth et al., 2018; Marshall et al., 2019). Early life history ecology, mainly of commercially important species, has also received considerable attention (e.g. Ibaibarriaga et al., 2007; Doyle and Mier, 2016; Siddon et al., 2019). On the other hand, relatively little work has been done on larval phase of non-commercial fish species, such as gobiid fishes, although they are ecologically important to sustaining marine ecosystem structure and function.
Gobiids (Actinopterygii: Gobiidae) are predominantly small benthic fishes forming one of the largest vertebrate families (Nelson, 2006). They are distributed worldwide and abundant components of the ichthyofauna of tropical and subtropical marine and estuarine waters (Zhang et al., 2020; Doll et al., 2021). In Xiangshan Bay (XSB hereafter, a subtropical, semi-enclosed embayment connected to the East China Sea), gobiid fishes are fundamental elements both in adult and larvae stages. Adult gobiids comprise large biomasses in nekton community and occupy medium to top trophic positions in food webs. The larval gobiids were found to be the dominant prey organisms for larvae and juvenile of some economically important fishes (e.g. Japanese Spanish mackerel Scomberomorus niphonius, an important fishery resource for China, Korea and Japan) (Lin et al., 2017). Therefore, they are suggested to have the potential to exert trophodynamic control on recruitment of the early life stages of economically important fishes. In spite of the ecological importance of this group, early life history ecology of gobiid fishes is rarely considered. There have been no specific studies addressing the distribution and ecology of gobiid larvae in XSB, although one study of larval fish assemblage referred to the presence of gobiid larvae. Ecology of gobiid larvae, including larval duration, spatiotemporal distribution and their relationship to environmental variables need to be studied in detail.
In addition, spatially co-occurring fish species are supposed to reduce interspecific competition through resource partitioning, by habitat segregation, temporal succession or food resource partitioning (Sala and Ballesteros, 1997; Munsch et al., 2016; Sbragaglia et al., 2019). Resource partitioning in adult fishes has been intensively studied, however few studies were carried out dealing with larval fishes. The pelagic larvae of gobiid fishes in XSB represent an opportunity to study spatiotemporal habitat partitioning among larval fish of taxonomically closely related species.
The present study described the distribution pattern and environmental preferences of gobiid larvae in XSB during the main spawning period. Particular attention was given to the spatiotemporal habitat partitioning among species. The possible links between larval patterns and physical and biological environment were also discussed. The findings reported here could provide baseline data on the use of a semi-enclosed coastal ecosystem in the East China Sea by early life stages of gobiids.
Materials and Methods
Study Area
XSB is one of the largest semi-enclosed coastal basins along the Chinese coast. It is located on the west coast of the East China Sea with total area of 560 km2 and average depth of ~10 m. It is typical of subtropical region, where the annual range of temperature is 11–28°C. The water mass circulating inside the basin originates chiefly from the coastal water, which is affected by the Changjiang diluted water and Zhe-Min coastal current. The annual range of salinity is between 21 and 28. The freshwater input usually dilutes the salinity of the inner water by 1–3, compared with the mouth water (Fan and Jin, 1989). XSB is excellent for abundant and diverse fishery resources because of its high primary and secondary productivity and relatively stable environment. It serves as spawning, nursery, and rearing area for many commercially important species including S. niphonius, white croaker Pennahia argentata and bombay duck Harpadon nehereus, and plays an important role in maintaining sustainable fish stocks in the offshore East China Sea (Jiang et al., 2013; Wang et al., 2017).
Sampling Procedure
Samples were taken on 10 cruises between April 3 and June 9, 2015 with an almost weekly coverage. A grid of 14 fixed stations, with depths ranging from 5m to 18m, was sampled on each cruise (Figure 1). Larval fishes were collected using an ichthyoplankton trawl net. The trawl net was fixed frame, with a 1.5m×1m rectangular mouth opening and 1-mm mesh size. It had a polystyrene floater attached to the upper rim, so that only the subsurface water stratum was sampled. Subsurface circular tows were performed at a velocity of ca. 1 m s-1 for 10 min and always at daytime (from 9:00 to 16:00). The volume filtered by the net was calculated to cubic meters based on the readings of a flowmeter (Hydro-Bios 438115) fixed at the mouth of the net frame. Samples were fixed in 5% borax-buffered formalin immediately after capture. In the laboratory, all fish larvae were sorted from the preserved samples. The gobiid larvae were identified to the best possible taxonomic resolution using relevant literature (Leis and Carson-Ewart, 2000; Okiyama, 2014). Larval fish catches at each station were standardized to ind. 100 m−3.
At each sampling station, the vertical profiles of the basic hydrographic parameters (temperature, salinity and depth) were obtained with a SeaBird 37 CTD. As zooplankton were suggested to important food sources for larval fishes (Hunter, 1981; Pepin and Penney, 1997), zooplankton samples were collected using a 160 μm mesh net equipped with a flowmeter in vertical hauls. Net samples were preserved immediately after collection with 5% neutral formalin seawater solution. Zooplankton were sorted, counted, and identified to the lowest possible taxa under a stereoscopic microscope. The total zooplankton density in each station was expressed as ind. m−3.
Data Analyses
A quotient-rule analysis was applied to assess the preferred ranges of environmental variables for the different species of gobiid larvae (Van der Lingen et al., 2001; Raya and Sabatés, 2015). The environmental variables included in the analysis were surface water temperature and salinity. Each environmental variable was assigned a number of environmental categories (c), to ensure that the maximum occurrence per category did not exceed 35% of all measurements. Their percentage frequency of occurrence (% environmental variable) and the percentage of total larval abundances (% fish larvae) within each category were calculated. Quotient values (Q) were then calculated for each variable category as follows:
Q(c) = % fish larvae(c) ⁄ % environmental variable(c)
Quotient values >1 were considered positively chosen categories. Only non-rare taxa, defined as those with a frequency of occurrence higher than 5%, were included in the analysis. In addition, the relationship between zooplankton abundance and larval fish concentration was tested using Pearson’s correlation coefficient in SPSS 16.0 (Rodriguez et al., 2013). We log transformed each variable to comply with normality assumptions (Dettmers et al., 2003).
In order to identify spatial distribution of gobiid larvae assemblage, a cluster analysis of the sampling stations was applied using the statistical package Primer. Cluster analysis were performed on Bray–Curtis similarity matrix, generated from the loge (x+1) transformed concentrations of the studied taxa after pooling by sampling stations (Álvarez et al., 2012).
A canonical correspondence analysis (CCA) was used to examine habitat partitioning in time and space by visualizing the spatiotemporal distribution patterns of the gobiid larvae assemblage and to elucidate multivariate interactions between the patterns and environmental characteristics (Franco and dos Santos, 2018; Heupel et al., 2019). The explanatory variables were water temperature, salinity and zooplankton density. Larval densities were loge (x+1) transformed before analysis, and rare species were down-weighted. Statistical significance was determined by Monte-Carlo tests using 499 permutations. CANOCO 5 computer program was used to run CCA (Šmilauer and Lepš, 2014).
Results
Hydrography and Biological Variables
During the sampling period, a typical hydrographic situation in XSB was encountered, being characterized by distinct temporal evolution of water temperature and weak spatial gradient of salinity. Seasonal evolution of water temperature in XSB showed typical conditions of subtropical East China Sea with a progressive increase from spring to summer (Figure 2A). During the sampling period, water temperature increased progressively with time, ranging from 14.3°C to 22.9°C. Notably, water temperature was not increasing homogeneously. It has risen at a mean rate of 0.95 ± 0.28°C per week. Particularly rapid increases were observed in two periods (April 19–May 3 and May 19–26), at rates varying between ~1.5 and ~1.8°C per week. Salinity ranged from 23.8 to 26.2 during the sampling period (Figure 2B). It exhibited a temporally stable spatial gradient along the length of XSB, decreasing from the seaward to the inner zone of the bay with a difference of almost 1.5 in all the cruises. Zooplankton density presented mean values of each cruise between 35 and 755 ind. m-3, with an average of 274 ind. m-3. In general, it was highly variable and showed higher mean values as summer progressed (Figure 2C).
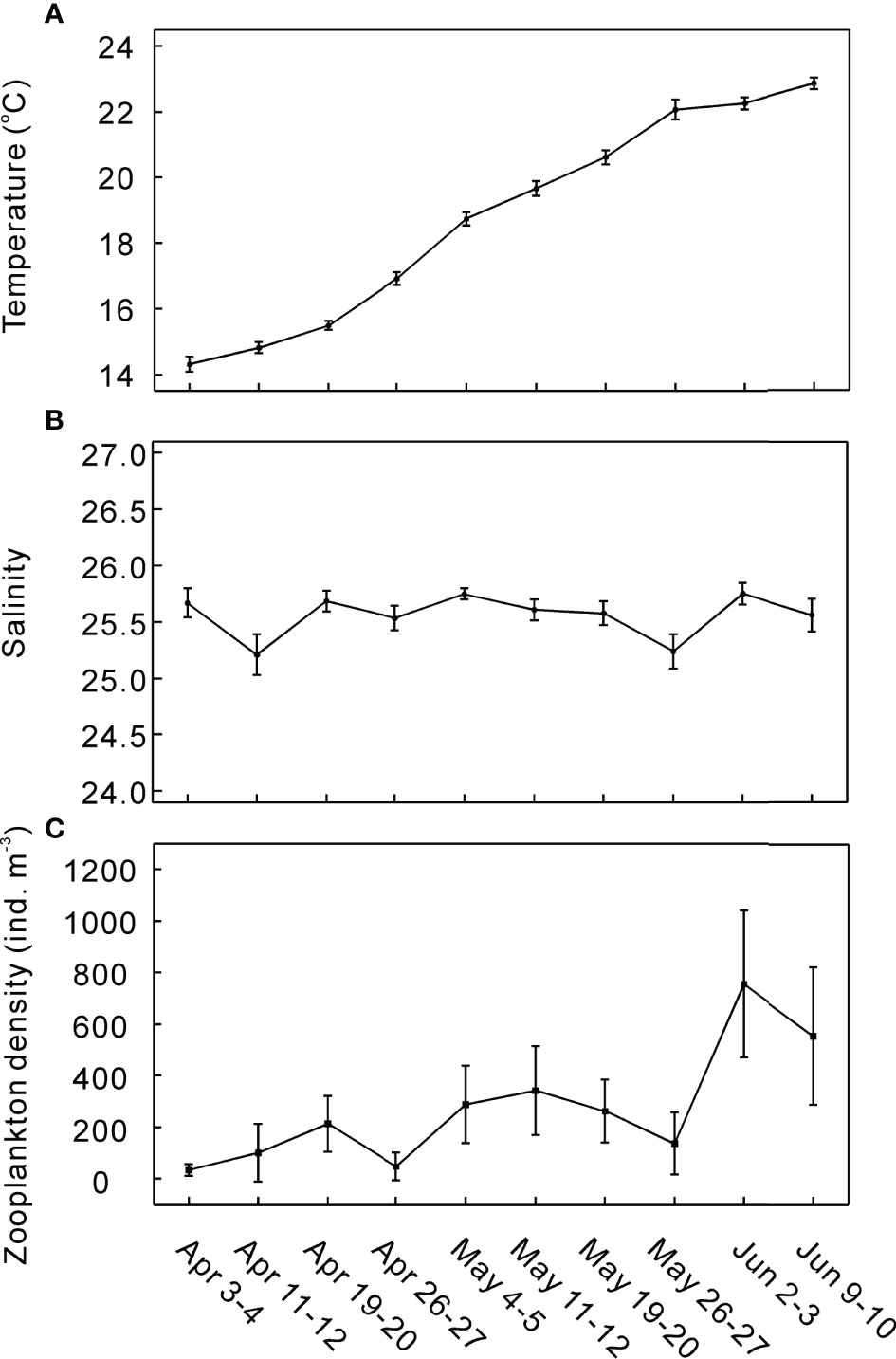
Figure 2 Temporal variation of water temperature (A), salinity (B) and zooplankton density (C) in XSB. Error bars represent standard deviation.
Larval Fish Composition and Distribution Patterns
A total of 87, 007 gobiid larval individuals, distributed in nine species and nine genera, were collected, which represented 48.3% of the total fish larvae during the sampling process. Numerically, branded goby Chaeturichthys stigmatias accounted for 51.7% of gobiid larvae, followed by Asian freshwater goby Acanthogobius ommaturus (23.0%) and striped sand goby Amoya pflaumi (14.5%). The other species pinkgray goby Amblychaeturichthys hexanema, tank goby Glossogobius giuris, red eel goby Odontamblyopus rubicundus, taileyed goby Parachaeturichthys polynema, dusky tripletooth goby Tridentiger obscurus and burrowing goby Trypauchen vagina contributed only 10.8% to the numerical total. Gobiid larvae abundance was highly variable, with maximum mean value of 487.44 ind. 100m-3 recorded during April 19–20 and lowest values of 0.56 ind. 100 m-3 during May 26–27.
Table 1 showed the temporal pattern of the gobiid larvae densities and composition. A. ommaturus, C. stigmatias and G. giuris mainly occurred from early April and early May. All of their abundance peaked in mid-April. P. polynema were present from early May through early June. T. obscurus were only found in early to mid-May. The other four species were exclusively captured in the early June. According to temporal succession in the dominant species, the gobiid larvae samplings could be classified into three temporal groups. The first group included samplings conducted from early April to early May. This group was dominated by C. stigmatias. The second one included samplings collected from mid- to late May. P. polynema dominated the larval assemblage in the period. The third group comprised the rest samplings in early June. A. pflaumi served as the dominate species in the group.
Cluster analysis represented that gobiid larvae assemblage exhibited relatively high similarity among sampling stations (Figure 3). Two sampling groups were identified at a similarity of 75%. These groups delimited two-well defined areas, according to how larval gobiids were represented throughout the study period. Zone A comprised the five stations close to the mouth of XSB. Zone B included the rest of the stations, mainly located in the inner area of the bay. The spatial patterns of gobiid larvae varied among species. The distribution of A. hexanema was restricted to the stations near the mouth of the bay (A). A. ommaturus, on the contrary, mainly concentrated in the inner area of the bay (B). The results of t-test revealed there were no significant differences in larvae density between Zone A and B for all of the other 7 species, indicating that larvae of these species exhibited no obvious preference among the two microhabitats.
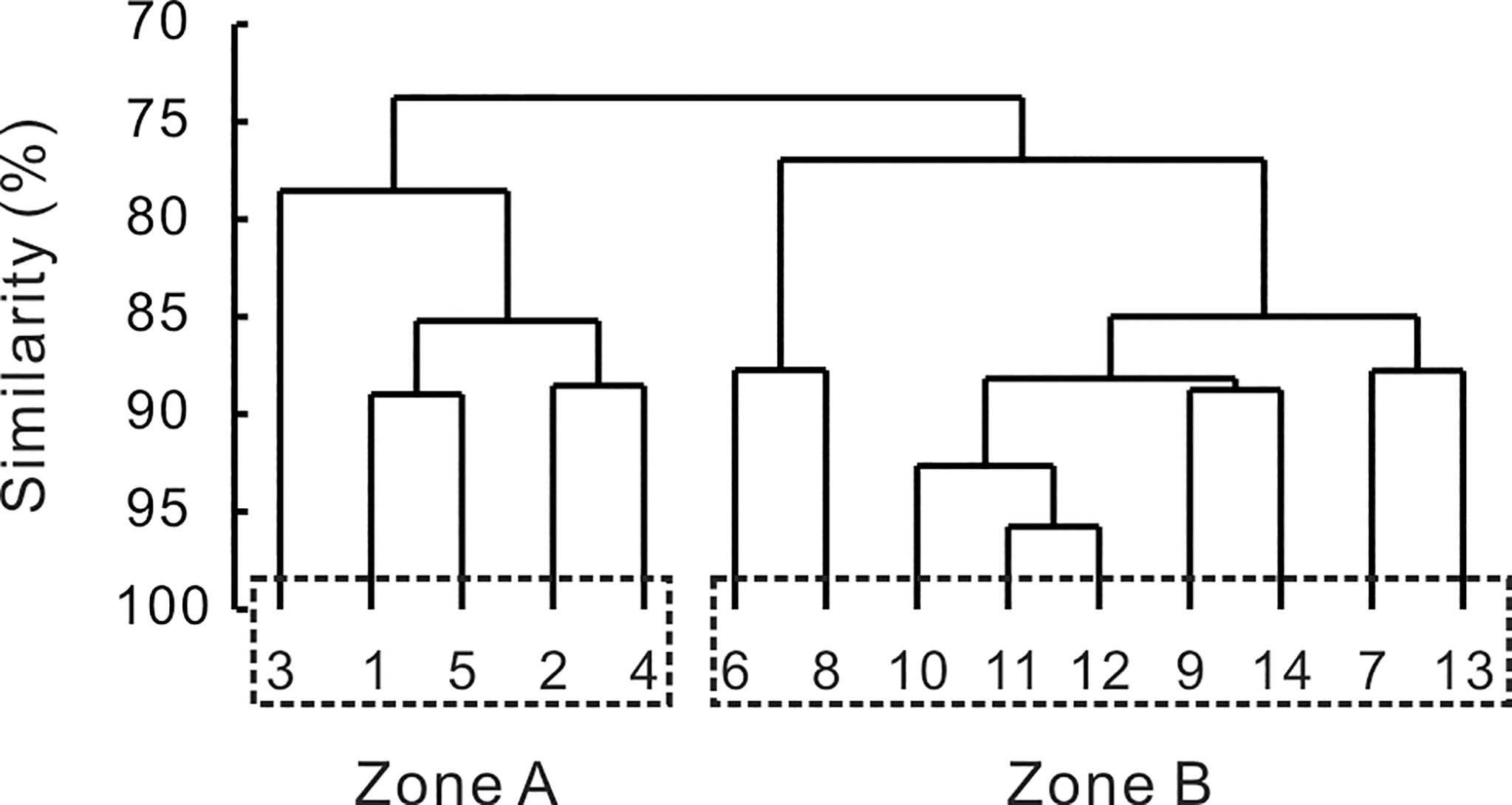
Figure 3 Dendrograms generated by cluster analysis with Bray-Curtis distance representing the spatial distribution pattern of gobiid larvae assemblage in XSB.
Environmental Preference
Six species with frequency of occurrence higher than 5% were included in the analysis. Quotient analysis showed that all of the 6 species preferred relatively narrow temperature intervals (<5°C). The ranking of species based on preference temperature appeared to be: A. ommaturus (14–15°C), C. stigmatias (15–18°C), G. giuris (15–16°C and 19°C), A. pflaumi (22–22.5°C), P. polynema (22 and 24°C) and T. vagina (22.5–23.5°C). With respect to salinity, no clear preference for P. polynema and T. vagina was observed in XSB. C. stigmatias, G. giuris and A. pflaumi exhibited similar preference for salinity range between 25.3 and 25.9, while A. ommaturus showed a comparatively lower and narrower range (25.3–25.5) of salinity (Figure 4).
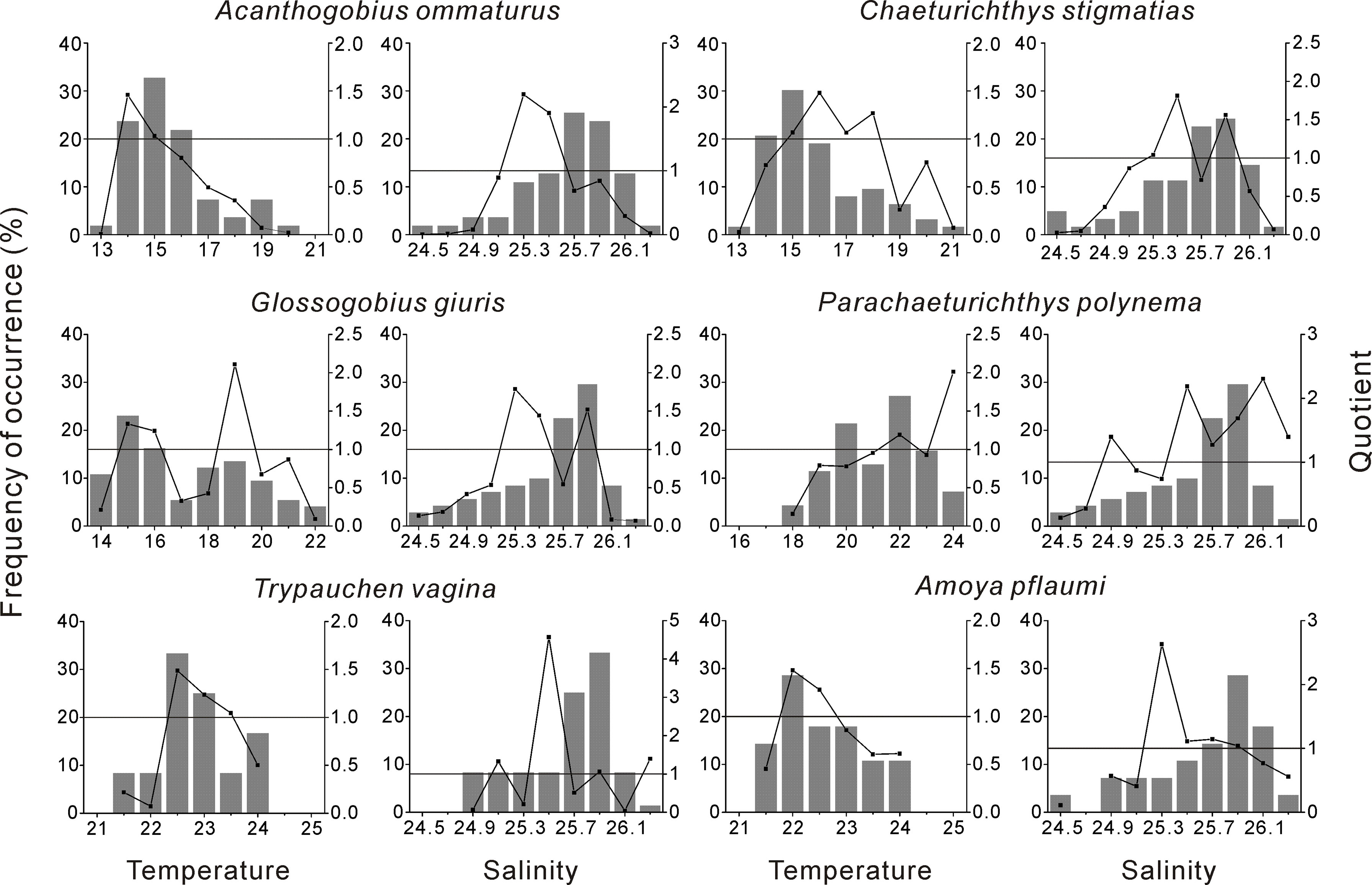
Figure 4 Quotient rule analysis showing the frequency of occurrence of environmental variables (water temperature and salinity) and larval abundance/environmental variable quotient curves for each species. Horizontal solid lines indicate a unit quotient value; quotient values above this indicate positive selection (preference).
Zooplankton density showed significantly positive relationships with the density of A. ommaturus (r = 0.419, p < 0.05), C. stigmatias (r = 0.417, p < 0.05), P. polynema (r = 0.383, p < 0.05) and A. pflaumi (r = 0.718, p < 0.05). For T. vagina and G. giuris, no clear relationships were observed with zooplankton density (p = 0.167 and p = 0.053, respectively). Overall, the total density of gobiid larvae was positively correlated with zooplankton density (r = 0.375, p < 0.05).
Habitat Partitioning and Environmental Drivers
The species-environmental biplot (Figure 5) summarized the results of the CCA of nine species and three environmental variables. The eigenvalues for axis 1 and 2 were 0.881 and 0.045. The axes explained 45.2% and 2.3% of the variability of the larval fish assemblage matrix, respectively. Water temperature, salinity and zooplankton density served as the potential impact factors affecting gobiid larvae assemblage structure, explaining 48.0% of the total variance. Remarkably, water temperature was the most major structuring factor of gobiid larvae compositions, explaining 93.1% of the explanatory variations. This finding suggested that the spatiotemporal distribution patterns of gobiid larvae were largely driven by water temperature.
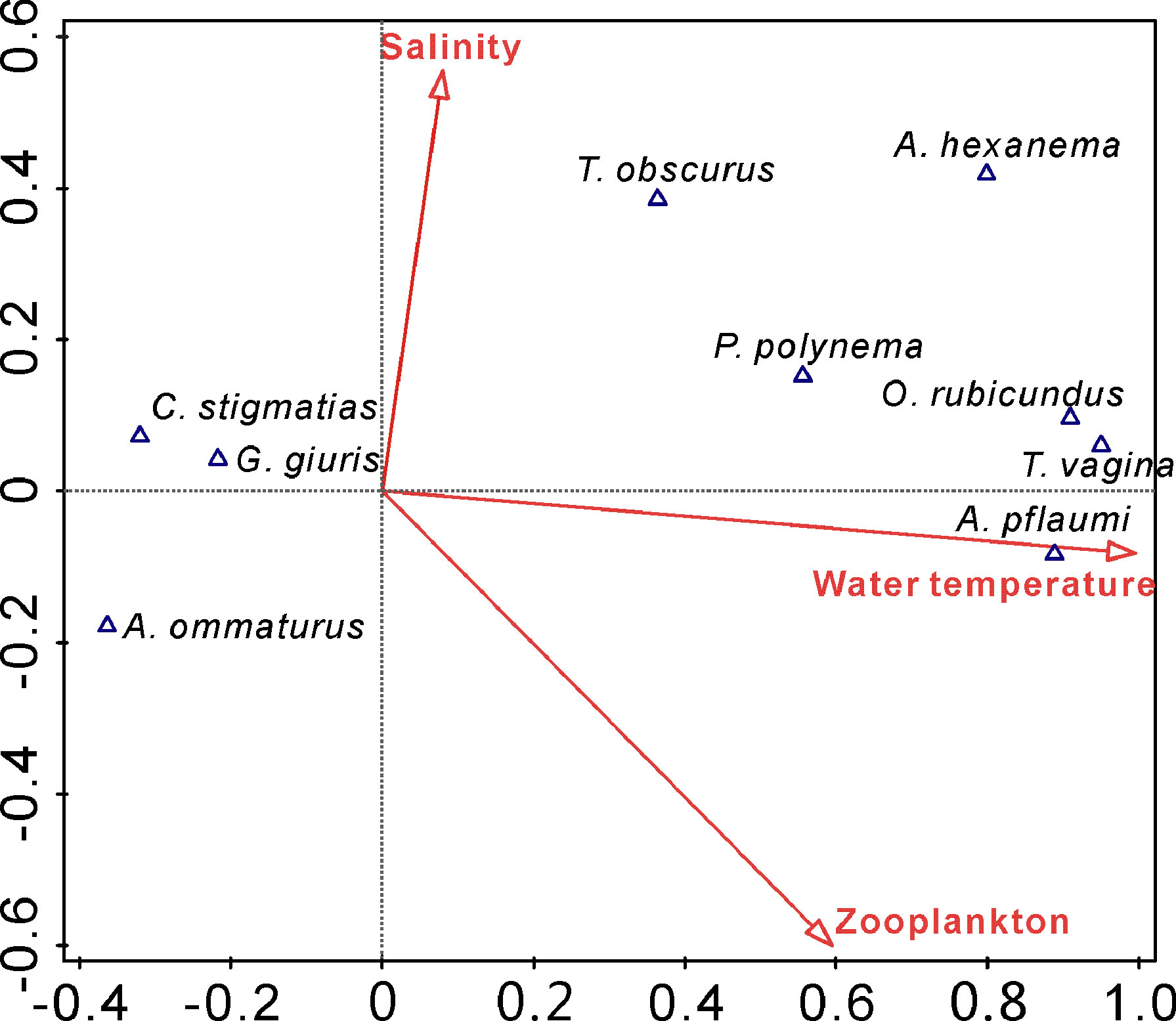
Figure 5 Ordination diagrams of canonical correspondence analysis showing the association of gobiid larvae assemblage with the environmental variables on the two canonical axes.
Species responses along the canonical axes showed some separation of habitat use in time and space. CCA axis 1, correlating best with water temperature, represented a temporal gradient along which taxa were ordered according to sampling date. Temporally early occurring species (A. ommaturus, C. stigmatias and G. giuris) characterized by the preference of relatively low temperature clustered on the negative side of the axis, while the remaining 6 species mainly located in the right half of Axis 1 representative of that relatively warm period. Furthermore, A. ommaturus was separated from C. stigmatias and G. giuris by axis 2, which was mainly correlated to salinity. Compared with the two latter species, A. ommaturus preferably distributed in the zone with lower salinity. This suggested that salinity was minor environmental factor affecting gobiid larvae assemblage under similar temperature conditions.
Discussion
We used high spatiotemporal resolution ichthyoplankton survey data to examine the larval distribution patterns of gobiids (the most diverse group of marine teleosts) and their relationships to the environmental conditions in a typical bay of the East China Sea. Our major findings were documentation of (1) characterization of spatiotemporal distribution patterns and environmental preference for the studied taxa, (2) habitat partitioning in time and space among larval gobiids, and (3) water temperature as the most major structuring factor of gobiid larvae compositions. These findings filled some gaps in our understanding of larval fish ecology of gobiids. They also provided empirical evidence for habitat partitioning among fish larvae of closely related and spatially co-occurring species.
Our results evidenced that XSB sustained diverse and abundant gobiid larvae assemblages during spring and early summer. The larval assemblage consisting of 9 gobiid fishes showed a clear spatiotemporal pattern. Firstly, temporal succession in species composition was strongly evident. The bay ecosystem was mainly occupied by A. ommaturus, C. stigmatias and G. giuris, with preferences for temperature bellow 19°C during April to early May, while A. pflaumi and T. vagina, which preferred relatively warm waters (above 22°C), predominated the larval assemblage in early June. Since the data used in this study, came from cruises directed to other target species, they seemed to provide incomplete temporal coverages for larval duration of some gobiid fishes (e.g. A. pflaumi, T. vagina). The early life stage of these species might last until the warmer summer months. Hence, the temperature preferences in these larval gobiids might be underestimated in our study, and further studies were needed to improve the evaluation of larval duration and temperature preferences for these larval gobiids. With respect to spatial distribution, relatively little variation was detected among species. Most of the study taxa exhibited little obvious habitat preferences except A. hexanema and A. ommaturus, which distributed preferably in the inner zone and mouth of the bay ecosystem, respectively.
Larval distributions generally reflect the spawning strategies of adult fishes, which is the result of an adaptive evolutionary process leading to the population sustaining itself in the long term (Forrest and Miller-Rushing, 2010). This study confirmed that the 9 gobiid fishes were spring-summer spawners, as indicated by previous works. The concentration of fish spawners in spring and summer might be related to seasonal patterns of their planktonic food supply. According to the match/mismatch hypothesis, the spawning of most fishes was synchronized with the annual peaks in their planktonic food supply. In XSB, zooplankton abundance (a proxy of the food availability for fish larvae) reached the annual peak in the spring and early summer (Wang et al., 2003). The temporal coincidence of fish larval stages with their prey was expected to favor the survival and development of larvae and juveniles. Our study also showed a positive relationship between the total density of gobiid larvae and zooplankton, indicating gobiid larvae distributed preferably in the waters with high zooplankton abundance. These facts supported the view of a high spatiotemporal coupling between early life stages of fish and their planktonic food supply (Cushing, 1990).
Closely related species with common life-history characteristics (often belonging to the same genus or family) were expected to exhibit successive and non-overlapping spawning competition among their offspring for food and space (Sabatés et al., 2007; Tsikliras et al., 2010). Our study suggested that gobiid larvae in XSB mainly avoid interspecific competition through temporal segregation. An apparent temporal progression of larval fish taxa was observed in XSB. Generally, the bay ecosystem was mainly occupied by A. ommaturus, C. stigmatias and G. giuris in April to early May, while A. pflaumi, A. hexanema, O. rubicundus and T. vagina was exclusively observed in the early June. Intuitively, we expected separate larval fish habitats when temporally coexisting. However, the results of this study did not support this expectation. Larval fish taxa with overlapping temporal niche (e.g. C. stigmatias and G. giuris) showed no substantial habitat segregation. Future studies are required to be conducted to demonstrate whether or not they have well separated trophic niches to avoid intense food competition.
Relationships between larval fish dynamics and environmental variability are complex, and involve multiple environmental factors and pathways (Hsieh et al., 2005; Auth et al., 2011). Temperature is known to have a great effect on the onset of spawning, egg hatching, survival and growth of early life stages prior to nursery recruitment (Green and Fisher, 2004; Laurel et al., 2004; Warren et al., 2012). Our analysis highlighted that water temperature played a crucial role in shaping the distribution pattern of the larval gobiids in XSB. It exhibited to be a determinant factor driving the temporal succession of gobiid larvae in XSB. During our sampling period, Water temperature increased progressively with time at a non-uniform rate. Particularly rapid rises of water temperature were observed in late April and late May. It is noteworthy that the gobiid larvae appeared to be particularly susceptible to the rapid rising temperature shocks. Sharp decline in the total abundance of gobiid larvae was detected with each rapid heating process. In addition, significant shift in larval fish composition occurred following the process.
Larvae are one of the most thermally sensitive life stages in fishes and display relatively narrow ranges in tolerable temperatures (Pörtner and Farrell, 2008; Dahlke et al., 2020). Any changes in thermal regime were assumed to have major consequences for duration and survival of larvae (Pankhurst and Munday, 2011). The influence mechanisms of rapid rising temperature on gobiid larvae might mainly include the following three aspects. Firstly, the pelagic larval duration is generally negatively correlated with water temperature (O’Connor et al., 2007; Munday et al., 2009). Rapid rising temperature is expected to reduce the larval duration of existing larvae, and promote them to withdraw from gobiid larvae assemblage. Secondly, rapid rising temperature would reduce the suitability of existing larvae. When the temperature reached the high end of thermal tolerance, larval fishes would suffer from extremely physiological stress. Additionally, larvae metabolic rates would be elevated in warmer water and therefore required more food consumption to grow (Jonsson and Jonsson, 2009). Consequently, in addition to stress associated with survival at the edge of one’s physiological capability, food limitation may be exacerbated at the upper end of the fish’s thermal range. Under these conditions, larval mortality would increase, which decreased the larval abundance. Lastly, increasing spring temperature is required to cue maturation in spring and early summer spawners (Shimizu, 2004). Rapid rising temperature could advance the spawning period of some fish species preferring to spawn in the relative high temperature preference, and then triggered the shift in larval fish composition.
With the instruction noted above, the implication is that pattern of temperature increase in spring and early summer plays a vital role in recruitment success of the studied gobiid larvae. In the context of global climate change, extremely hot days are becoming more common and the onset of the warm season is significantly advancing earlier in the year in the coastal waters (Schwartz et al., 2006; Lima and Wethey, 2012). This scenario of climate change implies more uncertainty on the recruitment of gobiid fishes, which in turn increases unpredictability of the pupation dynamics and diversity patterns of gobiid fishes in the bay ecosystem.
Data Availability Statement
The raw data supporting the conclusions of this article will be made available by the authors, without undue reservation.
Ethics Statement
Ethical review and approval was not required for the animal study because The present experimental procedures were carried out in strict accordance with the recommendations in the ethical guidelines of EU Directive 2010/63/EU for animal experiments.
Author Contributions
YJ conceived and designed the study. HZ and XY performed the statistical analysis and wrote the first draft of the manuscript. JL organized the database and prepared the figures. All authors contributed to manuscript revision, read, and approved the submitted version.
Funding
This research was supported by National Key Research and Development Project (2020YFD0900804) and National Special Research Fund for Non-Profit Sector (Agriculture, 201303047).
Conflict of Interest
The authors declare that the research was conducted in the absence of any commercial or financial relationships that could be construed as a potential conflict of interest.
Publisher’s Note
All claims expressed in this article are solely those of the authors and do not necessarily represent those of their affiliated organizations, or those of the publisher, the editors and the reviewers. Any product that may be evaluated in this article, or claim that may be made by its manufacturer, is not guaranteed or endorsed by the publisher.
Acknowledgments
We thank Nan Lin and Yutan Wang for help with field collections. For their contributions to the discussion and scope of this manuscript, the authors acknowledge Qian Gao.
References
Álvarez I., Catalán I. A., Jordi A., Palmer M., Sabatés A., Basterretxea G. (2012). Drivers of Larval Fish Assemblage Shift During the Spring-Summer Transition in the Coastal Mediterranean. Estuar. Coast. Shelf Sci. 97, 127–135. doi: 10.1016/j.ecss.2011.11.029
Auth T. D., Brodeur R. D., Soulen H. L., Ciannelli L., and Peterson W. T.(2011). The Response of Fish Larvae to Decadal Changes in Environmental Forcing Factors off the Oregon Coast. Fish. Oceanogr. Fish. Oceanogr. 20, 314–328. doi: 10.1111/j.1365-2419.2011.00586.x.x
Auth T. D., Brodeur R. D., Soulen H. L., Ciannelli L., Peterson W. T. (2018). Phenological and Distributional Shifts in Ichthyoplankton Associated With Recent Warming in the Northeast Pacific Ocean. Glob. Change Biol. 24, 259–272. doi: 10.1111/gcb.13872
Cushing D. H. (1990). Plankton Production and Year-Class Strength in Fish Populations: An Update of the Match/Mismatch Hypothesis. Adv. Mar. Biol. 26, 249–293. doi: 10.1016/S0065-2881(08)60202-3
Dahlke F. T., Wohlrab S., Butzin M., Pörtner H. O. (2020). Thermal Bottlenecks in the Life Cycle Define Climate Vulnerability of Fish. Science 369, 65–70. doi: 10.1126/science.aaz3658
Dettmers J. M., Raffenberg M. J., Weis A. K. (2003). Exploring Zooplankton Changes in Southern Lake Michigan: Implications for Yellow Perch Recruitment. J. Great Lakes Res. 29, 355–364. doi: 10.1016/S0380-1330(03)70439-0
Doll P. C., Munday P. L., Bonin M. C., Jones G. P. (2021). Habitat Specialisation and Overlap in Coral Reef Gobies of the Genus Eviota (Teleostei: Gobiidae). Mar. Ecol. Prog. Ser. 677, 81–94. doi: 10.3354/meps13863
Doyle M. J., Mier K. L. (2016). Early Life History Pelagic Exposure Profiles of Selected Commercially Important Fish Species in the Gulf of Alaska. Deep-Sea Res. Pt. II 132, 162–193. doi: 10.1016/j.dsr2.2015.06.019
Fan A., Jin X. (1989). Tidal Effect on Nutrient Exchange in Xiangshan Bay, China. Mar. Chem. 27, 259–281. doi: 10.1016/0304-4203(89)90051-0
Forrest J., Miller-Rushing A. J. (2010). Toward a synthetic understanding of the role of phenology in ecology and evolution. Philos. Trans. R. Soc B. 365, 3101–3112. doi: 10.1098/rstb.2010.0145
Franco A. C. S., dos Santos L. N. (2018). Habitat-Dependent Responses of Tropical Fish Assemblages to Environmental Variables in a Marine-Estuarine Transitional System. Estuar. Coast. Shelf Sci. 211, 110–117. doi: 10.1016/j.ecss.2018.02.003
Green B. S., Fisher R. (2004). Temperature Influences Swimming Speed, Growth and Larval Duration in Coral Reef Fish Larvae. J. Exp. Mar. Biol. Ecol. 299, 115–132. doi: 10.1016/j.jembe.2003.09.001
Heupel M. R., Munroe S. E. M., Lédée E. J. I., Chin A., Simpfendorfer C. A. (2019). Interspecific Interactions, Movement Patterns and Habitat Use in a Diverse Coastal Shark Assemblage. Mar. Biol. 166, 1225–1233. doi: 10.1007/s00227-019-3511-7
Hsieh C., Reiss C., Watson W., Allen M. J., Hunter J. R., Lea R. N., et al(2005). A Comparison of Long-term Trends and Variability in Populations of Larvae of Exploited and Unexploited Fishes in the Southern California Region: A Community Approach. Prog. Oceanogr. 67, 160–185. doi: 10.1016/j.pocean.2005.05.002.x
Hunter J. R. (1981). “Feeding Ecology and Predation of Marine Fish Larvae,” in Marine Fish Larvae: Morphology, Ecology and Relation to Fisheries. Ed. Lasker R. (Seattle: Washington Sea Grant), 33–77.
Ibaibarriaga L., Irigoien X., Santos M., Fives J. M., Franco C., De Lanzós A. L., et al. (2007). Egg and Larval Distributions of Seven Fish Species in North-East Atlantic Waters. Prog. Oceanogr. 16, 284–293. doi: 10.1111/j.1365-2419.2007.00430.x
Jiang Y., Lin N., Yuan X., Jiao H., Ling J., Li S. (2013). Community Structure and Species Diversity of Nektons in Xiangshan Bay of East China. Chin. J. Ecol. 32, 920–926. doi: 10.13292/j.1000-4890.2013.0167
Jonsson B., Jonsson N. (2009). A Review of the Likely Effects of Climate Change on Anadromous Atlantic Salmon Salmo Trutta, With Particular Reference to Water Temperature and Flow. J. Fish Biol. 75, 2381–2447. doi: 10.1111/j.1095-8649.2009.02380.x
Laurel B. J., Hurst T. P., Copeman L. A., Davis M. W. (2004). The Role of Temperature on the Growth and Survival of Early and Late Hatching Pacific Cod Larvae (Gadus Macrocephalus). J. Plankton Res. 30, 1051–1060. doi: 10.1093/plankt/fbn057
Leis J. M., Carson-Ewart B. M. (2000). The Larvae of Indo-Pacific Coastal Fishes: An Identification Guide to Marine Fish Larvae (Leiden: Brill).
Lima F. P., Wethey D. S. (2012). Three Decades of High-Resolution Coastal Sea Surface Temperatures Reveal More Than Warming. Nat. Commun. 3, 704. doi: 10.1038/ncomms1713
Lin N., Wang Y., Chen Y., Jiang Y., Yuan X., Li S., et al. (2017). Feeding Habits of Japanese Spanish Mackerel (Scomberomorus Niphonius) Larvae and Juveniles in Xiangshan Bay. Chin. J. Ecol. 36, 2811. doi: 10.13292/j.1000-4890.201710.011
Marshall K. N., Duffy-Anderson J. T., Ward E. J., Anderson S. C., Hunsicker M. E., Williams B. C. (2019). Long-Term Trends in Ichthyoplankton Assemblage Structure, Biodiversity, and Synchrony in the Gulf of Alaska and Their Relationships to Climate. Prog. Oceanogr. 170, 134–145. doi: 10.1016/j.pocean.2018.11.002
Miller B. S., Kendall A. W. (2009). Early Life History of Marine Fishes (Berkeley: University of California Press).
Munday P. L., Leis J. M., Lough J. M., Paris C. B., Kingsford M. J., Berumen M. L., et al. (2009). Climate Change and Coral Reef Connectivity. Coral Reefs 28, 379–395. doi: 10.1007/s00338-008-0461-9
Munsch S. H., Cordell J. R., Toft J. D. (2016). Fine-Scale Habitat Use and Behavior of a Nearshore Fish Community: Nursery Functions, Predation Avoidance, and Spatiotemporal Habitat Partitioning. Mar. Ecol. Prog. Ser. 557, 1–15. doi: 10.3354/meps11862
O’Connor M. I., Bruno J. F., Gaines S. D., Halpern B. S., Lester S. E., Kinlan B. P., et al. (2007). Temperature Control of Larval Dispersal and the Implications for Marine Ecology, Evolution, and Conservation. Proc. Natl. Acad. Sci. U.S.A. 104, 1266–1271. doi: 10.1073/pnas.0603422104
Okiyama M. (2014). An Atlas of Early Stage Fishes in Japan. 2nd ed. (Tokyo: Tokai University Press).
Pankhurst N. W., Munday P. L. (2011). Effects of Climate Change on Fish Reproduction and Early Life History Stages. Mar. Freshw. Res. 62, 1015–1026. doi: 10.1071/MF10269
Pepin P., Penney R. W. (1997). Patterns of Prey Size and Taxonomic Composition in Larval Fish: Are There General Size-Dependent Models? J. Fish Biol. 51 (Supplement A), 84–100. doi: 10.1111/j.1095-8649.1997.tb06094.x
Pörtner H. O., Farrell A. P. (2008). Ecology, Physiology and Climate Change. Science 322, 690–692. doi: 10.1126/science.1163156
Raya V., Sabatés A. (2015). Diversity and Distribution of Early Life Stages of Carangid Fishes in the Northwestern Mediterranean: Responses to Environmental Drivers. Fish Oceanogr. 24, 118–134. doi: 10.1111/fog.12097
Rodriguez J. M., Alvarez I., Lopez-Jurado J. L., Garcia A., Balbin R., Alvarez-Berastegui D., et al. (2013). Environmental Forcing and the Larval Fish Community Associated to the Atlantic Bluefin Tuna Spawning Habitat of the Balearic Region (Western Mediterranean), in Early Summer 2005. Deep-Sea Res. Pt. I 77, 11–22. doi: 10.1016/j.dsr.2013.03.002
Sabatés A., Olivar M. P., Salat J., Palomera I., Alemany F. (2007). Physical and Biological Processes Controlling the Distribution of Fish Larvae in the NW Mediterranean. Prog. Oceanogr. 74, 355–376. doi: 10.1016/j.pocean.2007.04.017
Sala E., Ballesteros E. (1997). Partitioning of Space and Food Resources by Three Fish of the Genus Diplodus (Sparidae) in a Mediterranean Rocky Infralittoral Ecosystem. Mar. Ecol. Prog. Ser. 152, 273–283. doi: 10.3354/meps152273
Sbragaglia V., Nuñez J. D., Dominoni D., Coco S., Fanelli E., Azzurro E., et al. (2019). Annual Rhythms of Temporal Niche Partitioning in the Sparidae Family are Correlated to Different Environmental Variables. Sci. Rep. 9, 1708. doi: 10.1038/s41598-018-37954-0
Schwartz M. D., Ahas R., Aasa A. (2006). Onset of Spring Starting Earlier Across the Northern Hemisphere. Glob. Change Biol. 12, 343–351. doi: 10.1111/j.1365-2486.2005.01097.x
Shimizu A. (2004). Effect of Photoperiod and Temperature on Gonadal Activity and Plasma Steroid Levels in a Reared Strain of the Mummichog (Fundulus Heteroclitus) During Different Phases of Its Annual Reproductive Cycle. Gen. Comp. Endocrinol. 131, 310–324. doi: 10.1016/S0016-6480(03)00026-1
Siddon E. C., De Forest L. G., Blood D. M., Doyle M. J., Matarese A. C. (2019). Early Life History Ecology for Five Commercially and Ecologically Important Fish Species in the Eastern and Western Gulf of Alaska. Deep-Sea Res. Pt. II 165, 7–25. doi: 10.1016/j.dsr2.2016.06.022
Šmilauer P., Lepš J. (2014). Multivariate Analysis of Ecological Data Using CANOCO 5 (Cambridge: Cambridge University Press).
Tsikliras A. C., Antonopoulou E., Stergiou K. I. (2010). Spawning Period of Mediterranean Marine Fishes. Rev. Fish Biol. Fish 20, 499–538. doi: 10.1007/s11160-010-9158-6
Van der Lingen C. D., Hutchings L., Merkle D., van der Westhuizen J. J., Nelson J. (2001). “Comparative Spawning Habitats of Anchovy (Engraulis Capensis) and Sardine (Sardinops Sagax) in the Southern Benguela Upwelling Ecosystem,” in Spatial Processes and Management of Marine Populations. Eds. Kruse G. H., Bez N., Booth A., Dorn M. W., Hill S., Lipcious R. N., Pelletier D., Roy C., Smith S. J., Witherell D. (Fairbanks: University of Alaska Sea Grant), 185–209.
Wang C., Liu Z., He D. (2003). Seasonal Dynamics of Zooplankton Biomass and Abundance in Xiangshan Bay. J. Fish China 27, 595–599. doi: 10.3321/j.issn:1000-0615.2003.06.015
Wang Y., Li S., Yang L., Yuan X., Jiang Y., Lin N. (2017). Species Composition of Larval and Juvenile Fish in the Xiangshan Bay in Spring and Summer. Mar. Fish 39, 286–296. doi: 10.13233/j.cnki.mar.fish.2017.03.006
Warren D. R., Robinson J. M., Josephson D. C., Sheldon D. R., Kraft C. E. (2012). Elevated Summer Temperatures Delay Spawning and Reduce Red Construction for Resident Brook (Salvelinus Fontinalis). Glob. Change Biol. 18, 1804–1811. doi: 10.1111/j.1365-2486.2012.02670.x
Keywords: larvae, spatial distribution, temporal succession, temperature, xiangshan bay
Citation: Zhang H, Yuan X, Ling J and Jiang Y (2022) Larval Ecology of Gobiid Fishes in a Subtropical Embayment: Environmental Preferences and Spatiotemporal Habitat Partitioning. Front. Mar. Sci. 9:929919. doi: 10.3389/fmars.2022.929919
Received: 27 April 2022; Accepted: 30 May 2022;
Published: 29 June 2022.
Edited by:
Wei Huang, Second Institute of Oceanography, Ministry of Natural Resources, ChinaReviewed by:
Yuan Li, Third Institute of Oceanography, Ministry of Natural Resources, ChinaKui Zhang, South China Sea Fisheries Research Institute, Chinese Academy of Fishery Sciences (CAFS), China
Liang Cao, Institute of Oceanology, Chinese Academy of Sciences (CAS), China
Copyright © 2022 Zhang, Yuan, Ling and Jiang. This is an open-access article distributed under the terms of the Creative Commons Attribution License (CC BY). The use, distribution or reproduction in other forums is permitted, provided the original author(s) and the copyright owner(s) are credited and that the original publication in this journal is cited, in accordance with accepted academic practice. No use, distribution or reproduction is permitted which does not comply with these terms.
*Correspondence: Yazhou Jiang, eWF6aG91amlhbmdAMTYzLmNvbQ==
†These authors have contributed equally to this work and share first authorship