- 1Natural Resource Institute Finland Luonnonvarakeskus (LUKE), Turku, Finland
- 2Tvärminne Zoological Station, Helsinki University, Hangö, Finland
Understanding the influence of biogenic habitats on species assemblage structure and ecosystem productivity is crucial for successful conservation of natural systems. Brown algae, Fucus vesiculosus, and blue mussels, Mytilus trossulus, coexist on sheltered and moderately wave exposed shallow rocky reefs of the northern Baltic Proper. Here, they function as important biogenic structures for an abundant associated macroinvertebrate fauna. Despite their dominance and space sympatry, there is little understanding of how they differ in their role as provisioners of biodiversity in this system. While Fucus has been recognized as an important habitat provider for decades, the similar role of blue mussels has been seriously understudied in the northern Baltic Proper, leading to pressing knowledge gaps and an underestimation of their role for overall biodiversity. In this study, we compared macroinvertebrate species assemblages within 40 rocky reefs where Fucus and Mytilus co-occur in either intermixed or adjacent assemblages. We show that both habitats represent a species rich and abundant community that are comparable regarding diversity. However, abundance and biomass of the associated community is much higher in the Mytilus habitat in relation to the Fucus habitat, implying a far higher secondary production in the former habitat. Recognizing key habitats and understanding how they differ in their ability to support biodiversity and ecosystem productivity is necessary for predicting community responses to human pressures, including an altered climate, and for implementing efficient mitigation actions to minimize loss of biodiversity.
Introduction
Defining the composition of communities and disentangling the processes that shape their diversity in biologically generated habitats are crucial for ecology and conservation. Still, much uncertainty exists about how the population-level traits of habitat forming species contribute to species diversity and ecosystem processes (Gribben et al., 2017), and how co-occurring habitat-formers together affect the species pool (Yakovis et al., 2008; Bishop et al., 2013; Passarelli et al., 2014; Khalaman et al., 2021; Yakovis and Artemieva, 2021). Multiple habitat providers - that may differ in structure, function, and density - organize most ecosystems across the globe (Bruno and Bertness, 2001). While there has been a significant improvement in our understanding of how habitat-forming species organize the associated community, research has historically focused mainly on the community effects of habitat providers in isolation. Such singular approaches simplify our understanding of how adjacent communities are organized and how habitat-forming species synergistically determine biodiversity (Angelini et al., 2011).
Seaweeds and mussels in the Baltic Sea are important habitat-formers that increase the complexity on rocky shores and provide shelter and resources that facilitates the occupation of the bedrock by many other species. On the rocky reefs of the Baltic Sea, where the distribution of Mytilus and Fucus overlap, there is a dynamic balance in the distribution of perennial Fucus vesiculosus and Mytilus trossulus largely determined by wave exposure and depth (Kangas et al., 1982; Westerbom et al., 2019a). Near the shore, Fucus dominates in biomass at the more sheltered shallow rocky locations, whereas Mytilus have their strongholds under more wave-exposed conditions and in deeper waters. On shallow moderately exposed shores, mosaics of Fucus stands and Mytilus beds are found together in belts or patches that vary in size and coverage. Here, they co-occur in either intermixed or adjacent assemblages. As seaweeds and mussels show different traits, we would expect changes in the associated community with dominance shifts from one facilitator to the other (Koivisto & Westerbom, 2010). Surprisingly, this difference has not been rigorously quantified in the Baltic Sea. As the distribution of both habitats is threatened by large-scale human pressures such as eutrophication and the ongoing climate change (Westerbom et al., 2019b; Sahla et al., 2020), changes may lead to shifting dominance structures or even total replacements by a different group of species (Kraufvelin & Salovius, 2004; Airoldi et al., 2008). Such changes have inevitable consequences for the associated community and may cause changes in trophic food webs (Gribben et al., 2017).
The two investigated habitat providers, Fucus vesiculosus and Mytilus trossulus, have both evident similarities and differences in their roles as foundation species. F. vesiculosus is a canopy-forming macroalga that plays a decisive role in the northern Baltic Proper, as it contributes to nearshore productivity (Rodil et al., 2020). As a perennial and large structure, Fucus represents the highest level of seaweed complexity in this system contributing with a unique three-dimensional structure for a rich associated community of both algae and macroinvertebrates. In addition, Fucus provides many ecosystem services, such as CO2 sequestration, oxygen production, and nutrient cycling (Attard et al., 2019). Mytilus aggregates into dense beds and host diverse macroinvertebrate communities (Koivisto & Westerbom, 2010). They are also understory organisms in Fucus belts, where they increase the complexity and diversity of the associated community (Koivisto and Westerbom, 2010). Mussel shell interstices create heterogeneous habitats that provide shelter from water movement and predators while trapping organic sediments and facilitating microalgal and bacterial growth (Tsuchiya and Nishihira, 1986). They also affect water circulation by their filtration activity (Kautsky and Wallentinus, 1980), act as temporary storage of carbon and other nutrients (Kautsky and Wallentinus, 1980) and constitute the main prey item for many species of fish (Westerbom et al., 2018) and sea birds (Öst and Kilpi, 1998).
We examined the structure and diversity of invertebrate assemblages associated with both Fucus and Mytilus at the entrance of the Gulf of Finland, northern Baltic Proper. We build on previous studies carried out across the same region (Koivisto and Westerbom, 2012; Westerbom et al., 2019a), but approach the raised questions differently with new data enabling a more precise comparison between the two long-lived habitats. While comparative studies across different habitats have been done in the northern Baltic Proper (e.g. Koivisto and Westerbom, 2010; Henseler et al., 2019; Rodil et al., 2020), previous studies mostly lack adequate replication in space, limiting the understanding of how biodiversity-habitat relationships may change across space (see also Bracken et al., 2017). Adequate spatial replication is important to test predictions, as the effect of space on ecological pattern may be highly context-dependent (Hewitt et al., 2017). Biogenic habitats may affect species diversity and composition very differently as a function of the environmental setting within which they occur and interact (Watt and Scrosati, 2013; Westerbom et al., 2019a) or as a function of the inherent structure or trait of the habitat itself (Tsuchiya and Nishihira, 1986). This means that changes in the abiotic environment can affect the traits of facilitating species (e.g. their size or density), which may have a strong influence on how they affect the associated community (Koivisto and Westerbom, 2010). When space has been included in the analyses, studies from the Baltic Sea have mainly focused on how single biogenic structures provide living space for an associated community. Alternatively, studies have contrasted the community in the biogenic habitat with either bare surfaces or annual ephemeral structures (Kraufvelin and Salovius, 2004; Wikström and Kautsky, 2007) or sampled communities at different depths (Westerbom et al., 2019a) complicating comparisons as depth per se has a strong influence on species composition. Therefore, there is currently little comparative data on how different engineering species under similar conditions affect the composition and structure of the associated community (see also Khalaman et al., 2021) and how they together enlarge space occupation for that community.
In this study, we were interested in comparing the macroinvertebrate diversity and composition in sympatric Fucus and Mytilus beds as a first step towards understanding the relative role of these biogenic habitats for the macroinvertebrates that inhabit these shallow rocky areas. We confine this comparison to these two foundation species, as: (1) they are both long-lived and abundant and cover large areas, (2) they occur side by side on shallow rocky shores. Additionally, (3) expanding the work to other benthic habitats would involve a set of uncontrollable variables (e.g. substratum, depth, predator pressure, wave exposure, temperature) that would make comparisons challenging (Khalaman et al., 2021). Understanding the structure and functioning of biologically generated habitats as biodiversity upholding structures is particularly important as they are increasingly being impacted by large-scale changes, prompted by natural and anthropogenic drivers. With global decline in biodiversity (Barnosky et al., 2011), it is of utmost importance that we understand the structure and drivers for all components of diversity in our major ecosystems and habitats, especially among those that are long-lived species, facilitate biodiversity and those that may be particularly sensitive to change.
The main objective of this study was to improve the understanding of how seaweeds and mussels contribute to species diversity and community structure on rocky reefs of the northern Baltic Sea. More specifically, we wanted to characterize both communities under identical environmental conditions to evaluate their contribution to diversity, abundance, richness and community structure in a controlled manner. Since Fucus has been considered the most important biome in the Baltic Sea (Kangas et al., 1982) hosting the most species rich community (Kautsky et al., 1992), we hypothesized that samples including Fucus would outperform those including only Mytilus regarding diversity and richness.
Methods
Sample regions
To compare faunal composition in Fucus stands and Mytilus beds, we sampled 40 sites (19 in the west and 21 in the east) from two different sampling regions in late summer 2009 (Figure 1). The area is characterized by a mosaic of rocky islands, islets and reefs and shows a high topographic complexity where individual sites are separated by deeper water. Sites were dispersed over ca 70 km-2 within regions and occurred only in the inner part of the outer archipelago where wave exposure is low (Baardseth index describing the degree of wave exposure < 4, see Westerbom and Jattu, 2006). The region Hanko East (59°50’N, 23°25’E) is part of the Gulf of Finland whereas the region Hanko West (59°51’N, 22°50’E) is part of the Archipelago Sea, Baltic Sea.
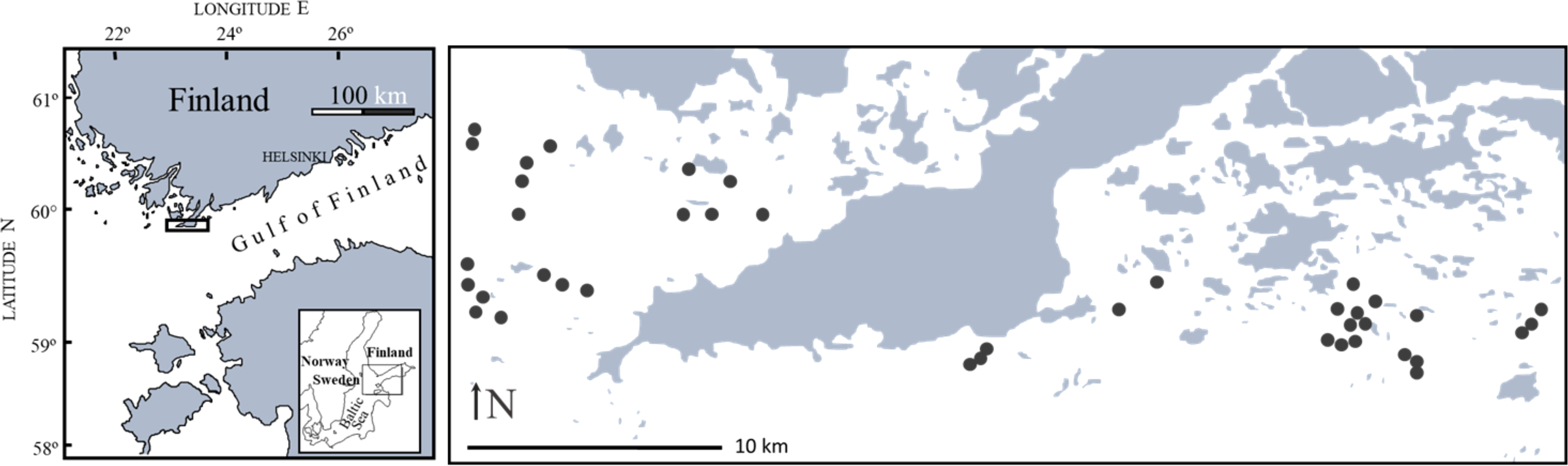
Figure 1 Map of the study area showing the distribution of the 40 sites dispersed over two regions west and east of Hanko Peninsula.
Sampling and processing of samples
Using SCUBA, random samples of the macroinvertebrate fauna were taken from patches of Fucus belts and Mytilus beds with a 20 x 20 cm quadrat frame at 2 m depth. Parallel samples were taken at each site, providing us with two different samples (later called treatment), one including Fucus and its understory organisms and one from patches of Mytilus without Fucus. Distance between the two samples was kept > 10 meters to keep samples independent (Díaz et al., 2015). Samples were collected from locations with a small inclination. We collected samples by placing the frame over Fucus stands and Mytilus beds and scraping the entire frame content into an attached fabric net-bag. Each individual Fucus was detached from the substrate with a sharp spatula and gently enclosed in the bag underwater along with the understory content. Fucus samples, therefore, include the understory community. Being a three-dimensional habitat, algal stands affect the community structure below the algal fronds in the same manner as terrestrial forest canopies affect the understory communities (e.g. Bué et al., 2020). The sample area and technique is standard in phytobenthic sampling programs and research in the northern Baltic Proper (e.g. Råberg and Kautsky, 2007; Wikström and Kautsky, 2007; Koivisto and Westerbom, 2010). Mytilus samples were placed in jars and covered in 70% ethanol. For Fucus, we separated Fucus from the remaining content in the Fucus samples. The understory community was then placed in jars and covered in 70% ethanol, whereas Fucus was put in separate bags, it was brought to laboratory, carefully washed to further remove remaining invertebrates, which were added to the part of the sample including the understory community. Fucus was then dried at 60°C for 5 d and weighed. In the laboratory, the macroinvertebrate fauna from both sample types was then sorted by size through a series of successively finer sieves (mesh sizes 9.5, 4, 2, 1 and 0.5 mm), after which the fauna was identified and counted to the lowest taxonomic level possible. The smallest fraction in all samples was analyzed using a preparation microscope (Leica S6E). Biomasses for Mytilus were estimated according to Westerbom et al. (2008). To estimate the weight of different invertebrates in the community (community biomass), we took additional samples that were kept fresh. They were fractioned by size using the five mesh and then dried at 60°C for 5 d and finally weighed.
Data analysis
Species accumulation plots were used to ascertain that the sample size was sufficient to adequately describe the diversity in the study area. A species accumulation plot reaches an asymptote when an increase in sample size does not increase the power of interpretations. Observed Species Richness (Sobs) for Fucus showed that 91% and 94% of the species were observed in the first half of the samples for both regions, whereas for Mytilus the equivalent value was 94% for both regions. For both treatments and regions, an asymptote was reached well before the total sample size, showing that the used sample size reliably described the communities over the two regions and within the two treatments.
To test for differences in species richness, total invertebrate abundance, and community biomass between the two treatments and regions, we used a nested permutational multivariate analysis of variance (PERMANOVA) with treatment (Fucus or Mytilus) and region as fixed factors and sites as a random factor nested within region. All tests were based on type III sums of squares. PERMANOVA calculates Pseudo-F from a distance/dissimilarity matrix and discriminates group differences. Prior to testing, data were square root transformed and checked for group homogeneity (PERMDISP). Shannon-Wiener diversity index (with log base e) was calculated for all samples and tested with the ANOSIM test for differences between treatment and region. All univariate tests were done on matrices based on Euclidean distance and with 9999 permutations.
Differences in faunal assemblage structure between regions and treatments was tested with the Analysis of Similarity test (ANOSIM). Matrices were based on Bray Curtis dissimilarities using log+1 transformed data and 9999 permutations. Analysis of Similarity test (ANOSIM) was selected over PERMANOVA as PERMDISP revealed multivariate heterogeneity in group dispersions. ANOSIM examines how differences within regions or treatments compare to differences between regions or treatments. ANOSIM computes an R-value that lies between 0 and 1, with 0 indicating sample similarity and 1 indicating dissimilarity. Differences among treatments and regions were then visualized by a non-metric multidimensional scaling ordination (nMDS) to picture the distinctiveness among the groups in multivariate space. Here, the distance between samples on the ordination indicates relative dissimilarity among groups. Similarity percentages procedure (SIMPER) based on the Bray-Curtis measure of dissimilarity was used to identify species that are responsible for differences between treatments and regions.
Finally, a distance-based linear model permutation test (DistLM) on log transformed data was performed to identify how well Fucus and/or Mytilus biomass function as predictor for species richness, macroinvertebrate abundance and overall community structure. DistLM analyses are based on a resemblance matrix that shows the level of similarity in abundance among sampled stations (Anderson et al., 2008). As the independent variables were only two, we used the Adjusted R2 criteria option throughout this study. All statistical analyses in this study were carried out in the PRIMER 7.0 package with the PERMANOVA+ add-on and excluded Mytilus among the response variables when testing for macroinvertebrate community differences.
Results
Differences in biomass of the facilitating species between regions and treatments
The two regions showed a homogeneous biomass of both Fucus (p = 0.16, Table 1 in Appendix 1) and Mytilus (p = 0.9665, Table S2). Mytilus biomass was higher in the Mytilus treatment than in the Fucus treatment (p = 0.0016, Table S2). There was no interaction between region and treatment for Mytilus (p = 0.29, Table S2). Fucus biomass (DW) was on average ( ± SE) 22 ± 6 g/sample (corresponding to 550 g/m2). The corresponding value for Mytilus (DW with shells) was 15 ± 1.4 g/sample (corresponding to 380 g/m2).
Differences in diversity and abundance variables – univariate tests
In total, 37 faunal species or genera were found in the Fucus treatment and 34 in the Mytilus treatment with a sample maximum of 25 and 24 species in the respective treatments. Mytilus had a higher species richness than Fucus (p = 0.028, Figure 2A, Table S3), but there was no difference between the regions (p = 0.27, Table S3), nor were there any interactions between region and treatment (p = 0.08, Table S3). Shannon-Wiener diversity index was equal between treatments (ANOSIM, Global R = 0.03, p = 0.125, Figure 2B). The eastern region showed a small but significantly higher diversity than the western region (ANOSIM, Global R = 0.29, p < 0.001).
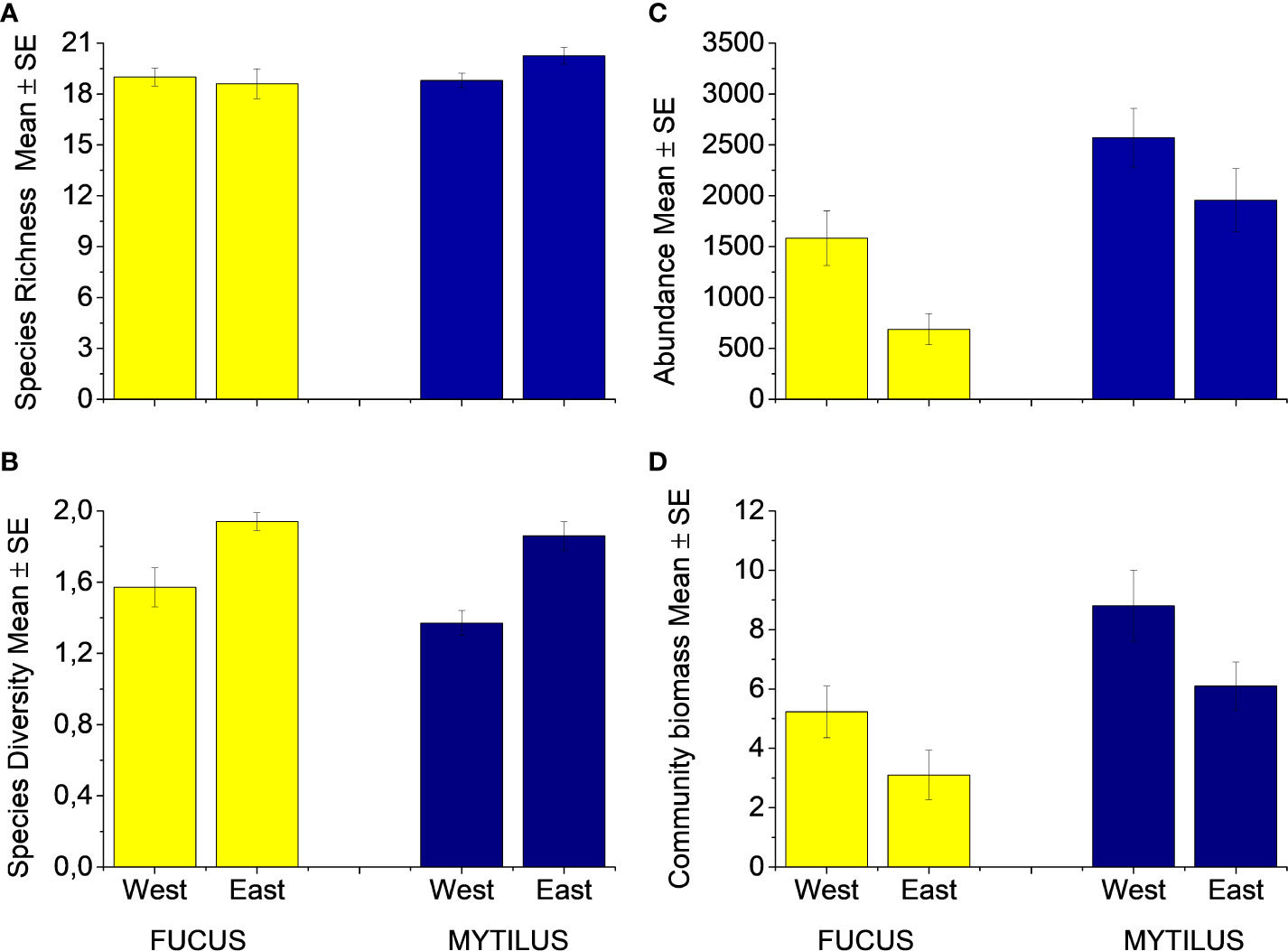
Figure 2 (A) Species Richness, (B) Species Diversity (H´loge), (C) Individual Abundance and (D) Community biomass of fauna inhabiting the Fucus and Mytilus plots (excluding Mytilus as response variable). Abundance and biomass (g per plot) data are counts and weighs per sampled plot (0.04m2). Species richness is the number of species in each sample. West and East refer to the two regions. Data are means ± SE.
The macroinvertebrate abundance was significantly higher in the Mytilus treatment compared to the Fucus treatment (p < 0.001, Figure 2C, Table S4) with Mytilus containing significantly more individuals among the ten most common taxa (see Appendix 2). An exception was formed by the isopods I. baltica and I. granulosa that were significantly more abundant in the Fucus treatment. The western region supported a much higher abundance of macroinvertebrates than the eastern region (p = 0.0025, Table S4). There were no interactions between treatment and region (p = 0.83, Table S4). Sequential tests in DistLM identified both Mytilus biomass (pseudo-F = 44.92, p < 0.001, % explained = 54.2) and Fucus biomass (pseudo-F = 5.73, p = 0.089, % explained = 6.14) as significant predictors for macroinvertebrate abundance in the Fucus treatment (model Adj R2 = 0.58). For species richness, Mytilus biomass explained more (pseudo-F = 19.21, p < 0.001, % explained = 33.57) than Fucus biomass (pseudo-F = 12.94, p < 0.001, % explained = 17.21, model Adj R2 = 0.51). That is, understory Mytilus biomass had a stronger association on both macroinvertebrate abundance and macroinvertebrate richness than Fucus within the Fucus treatment. Surprisingly, the relationship for Fucus was negative, i.e. higher Fucus biomass had a negative effect on macroinvertebrate species richness and total individual abundance (Figures 3A, C). In the Mytilus treatment, Mytilus biomass had a significant, but low impact on macroinvertebrate species richness (pseudo-F =4.6028, p = 0.037, % explained = 8.4, but it had a moderate effect on macroinvertebrate abundance (pseudo-F =18.332, p < 0.001, % explained = 30.7) (Figures 3B, D).
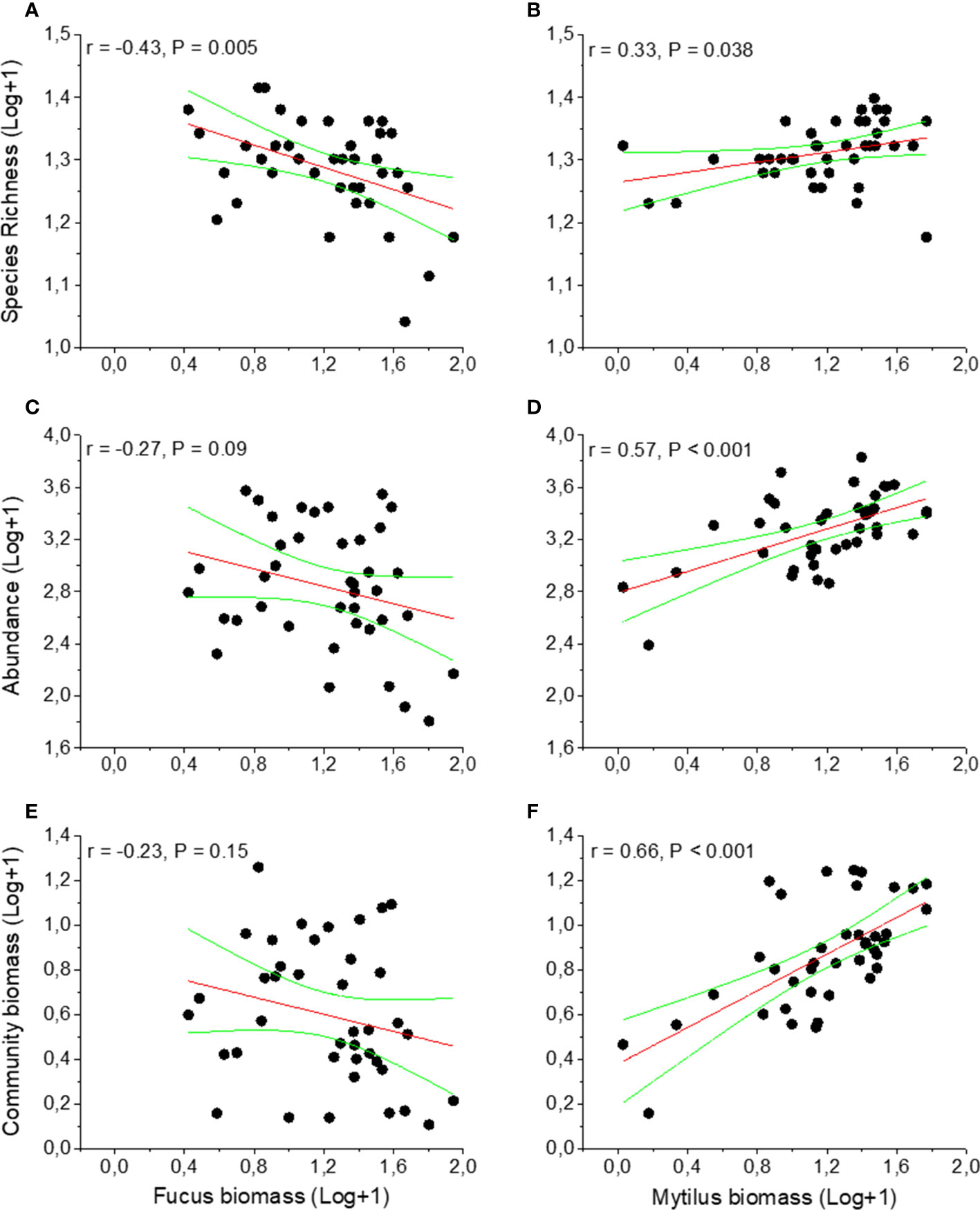
Figure 3 Effects of Fucus and Mytilus biomass on species richness (A, B), abundance (C, D) and biomass (E, F) on invertebrate fauna inhabiting Fucus and Mytilus. Data are per sampled plot (0.04m2) showing trendlines and 95% confidence bands. Data are means with N = 40 for Fucus and 40 for Mytilus. Data exclude Mytilus as response variable.
Differences in faunal composition – multivariate tests
The ANOSIM analysis showed significant, but small differences between faunal composition in the studied treatments (Global R = 0.194, p < 0.001). Also, regional differences were small but significant (Global R = 0.223, p < 0.001). Differences were clearly visualized in the nMDS plot where Mytilus samples clustered to the left, whereas Fucus samples were spread over a wider multivariate space (Figure 4). Results were corroborated by PERMDISP showing a significant degree of group heterogeneity between treatments (p < 0.001) and regions (p < 0.01). Mytilus biomass (shown with numbers in Figure 4) was a relatively good predictor for the faunal composition in the Fucus treatment (as also seen in the DistLM above). As revealed by the SIMPER procedure (analysis excludes Mytilus), the dominating taxa in Mytilus treatments were deposit feeding gastropods (mainly Hydrobidae), crustaceans (Jaera and Gammarus spp.), clams (Macoma baltica and Cardiidae), and flatworms (Turbellaria spp.), whereas Fucus treatments were dominated by grazing crustaceans (Jaera and Gammarus spp.), gastropods (Hydrobidae and Theodoxus fluviatilis) and Idotea balthica. In both treatments, Mytilus was the dominating species when it was included (Figure 5). The average similarity within treatments and regions was relatively high, with 64% and 76% for Fucus and Mytilus, and 67% and 73% for eastern and western regions accordingly. The average dissimilarity was 34% between treatments and 33% between regions. The DistLM identified both Mytilus biomass (pseudo-F = 11.2, p < 0.001, % explained = 22.77) and Fucus biomass (pseudo-F = 3.516, p < 0.001, % explained = 6.7) as significant predictors for overall species composition in the Fucus treatment, although explaining only 25.7% of the total variation in community structure. For the Mytilus treatment, Mytilus biomass explained only 10.6% of the overall species composition (pseudo-F = 5.63, p < 0.001).
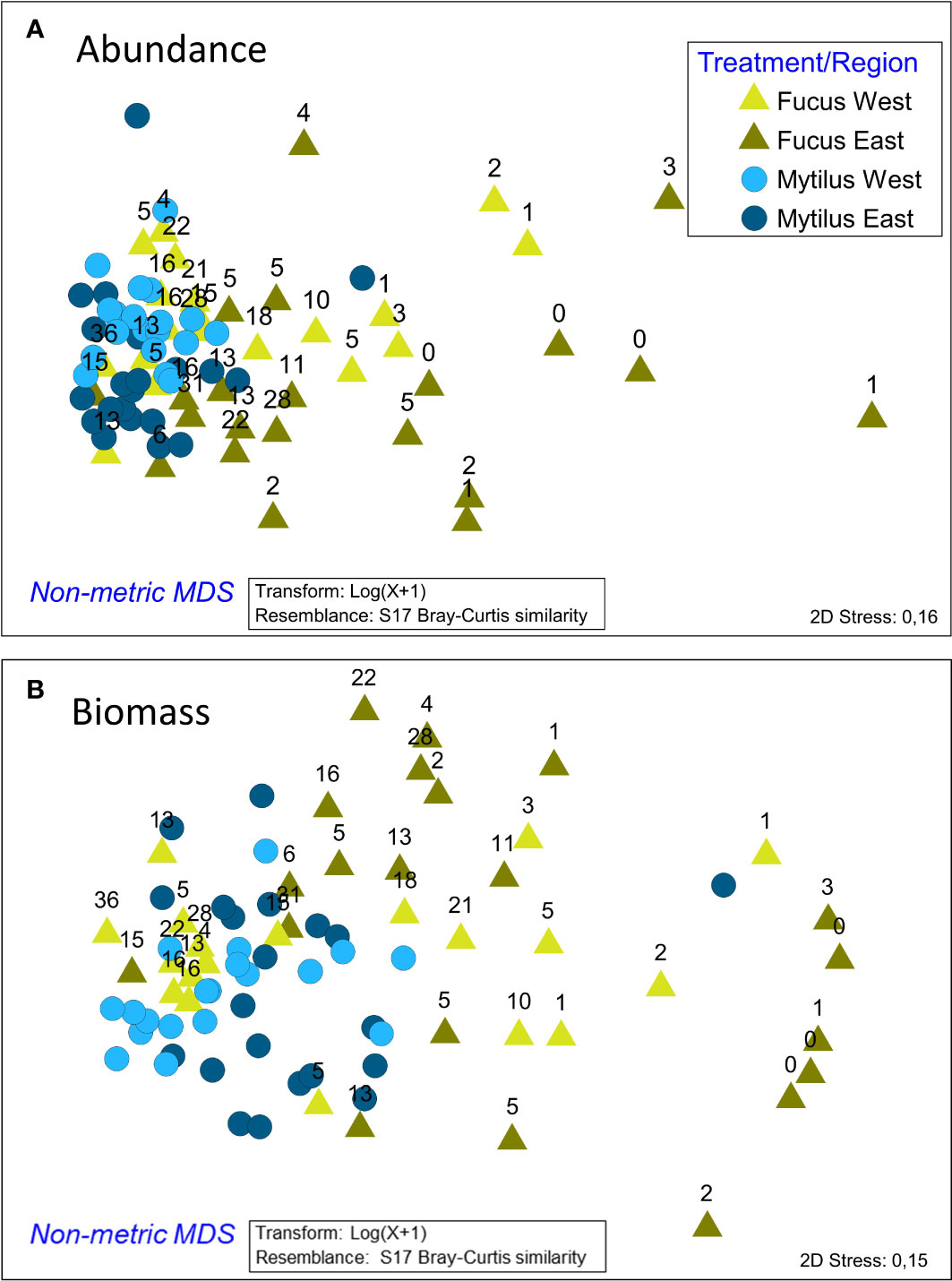
Figure 4 nMDS ordination of the Fucus and Mytilus samples according to their macrobenthic faunal composition for both abundance (A) and biomass (B). The numbers above Fucus samples refer to the biomass (g/sample) of Mytilus in each Fucus sample. As shown, Fucus samples to the right have generally a lower Mytilus biomass than samples to the left where the Mytilus samples are mainly clustered. The ordination is based on the associated fauna, excluding Mytilus as a dependent variable.
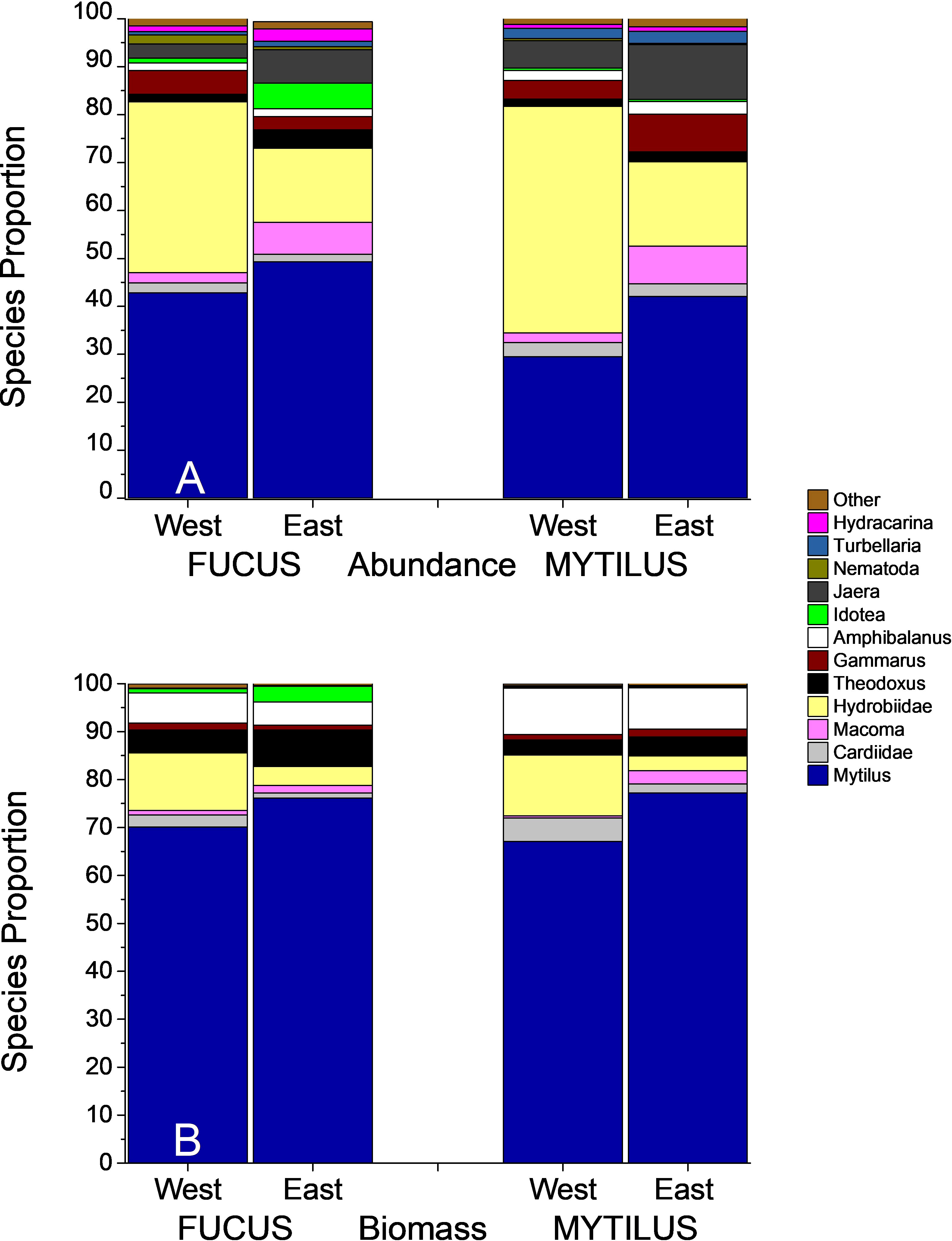
Figure 5 Species proportion for Fucus and Mytilus samples over the two regions. Mytilus is included in this Figure. The upper panel (A) shows abundances, the lower panel (B) shows biomasses of the macroinvertebrate fauna.
Community biomass patterns
The total biomass of the associated macroinvertebrate fauna was significantly higher in the Mytilus treatment compared to the Fucus treatment (p < 0.001, Figure 2D, Table S5). There were also significant effects of region (p = 0.02) but no interaction between treatment and region (p = 0.89). Fucus biomass had a non-significant effect on the total biomass of the associated fauna, whereas Mytilus biomass affected positively (Figures 3E, 3F).
ANOSIM showed significant but small differences in faunal composition based on biomass in the studied treatments (Global R = 0.182, p < 0.001). Also, the faunal composition between regions differed, but differences were small (Global R = 0.18 p < 0.001). Differences were clearly visualized in the nMDS plot where patterns resembled those of the abundance data (Figure 4). Mytilus biomass (shown with numbers above symbols in the Figure) was once again a relatively good predictor for the biomass composition of the Fucus fauna. The average dissimilarity between treatments was higher than when comparing abundances (56% vs 34%). Amphibalanus improvisus, Hydrobidae, Theodoxus fluviatilis and Cardiidae spp. explained most of the dissimilarity. As revealed by the SIMPER procedure, the taxa in the Mytilus treatments that best characterized the treatment in terms of biomass were Amphibalanus improvisus, deposit feeding gastropods (Hydrobidae) and the grazing gastropod Theodoxus fluviatilis. In the Fucus treatment, the corresponding species were Theodoxus fluviatilis, Amphibalanus improvisus, Hydrobidae and Gammarus spp. (Figure 5). The average similarity within treatments was lower than for the abundance data, 41% for Fucus and 60% for Mytilus.
Discussion
We were interested in testing how two sympatric perennial habitat-forming structures maintain macroinvertebrate diversity, individual abundance, and community structure on sublittoral rocky reefs in the northern Baltic Proper. Based on previous research and common beliefs, we hypothesized that samples containing Fucus would outperform those where only Mytilus is present. We show that the macroinvertebrate communities in Mytilus and Fucus treatments differ in their assemblage structure despite generally hosting the same species. The most notable difference is seen in the abundance of invertebrates where Mytilus beds support a much greater abundance and biomass of the associated fauna. In the discussion below, we focus on differences between the two treatments, but pass over aspects on regional differences as these have been thoroughly reported in previous papers (e.g. Koivisto and Westerbom, 2012; Westerbom et al., 2019a).
The decadal long decline of Fucus vesiculosus (e.g. Sahla et al., 2020) along the coasts of the entire northern Baltic Proper has gained much attention and inspired research on the possible ecological outcomes of this loss. Fueled by the notion that the Fucus habitat is the most species rich habitat in the northern Baltic Sea (Kautsky et al., 1992 with references, Kautsky and Svensson, 2003) - even suggested to be the most important littoral biome (Kangas et al., 1982) - there has been a concern that a reduced coverage of Fucus and its replacement by ephemeral structures would reverberate on diversity patterns in the Baltic Sea. This notion has been deeply rooted in science and education, but as pointed out by Kraufvelin and Salovius (2004), it has seldom been critically tested or quantified. Kraufvelin and Salovius (2004) challenged the view and showed that ephemeral macroalgal structures may support a macroinvertebrate species assemblage that regarding diversity is comparable to the one supported by Fucus. The study by Kraufvelin and Salovius (2004) spurred a number of studies, which showed very inconsistent effects of Fucus compared to other habitats (e.g. Råberg and Kautsky, 2007; Wikström and Kautsky, 2007; Saarinen et al., 2018). While previous studies have compared Fucus with ephemeral rocky shore structures (but see Saarinen et al., 2018), here we compare diversity patterns in two long-lived structures. Our sites were well replicated across the regional space and both sample types were always taken in pairs, assuring an identical abiotic and biotic environment for both groups.
The average species richness in both Mytilus and Fucus samples is remarkably similar to what has been reported previously in the northern Baltic Proper (see also Haage and Jansson, 1970; Wikström and Kautsky, 2007; Norling & Kautsky 2008). While some degree of habitat fidelity was evident for some taxa, our results show that most of the macroinvertebrate species are habitat generalists occurring abundantly in both habitats. This finding is coherent with Kraufvelin & Salovius (2004); Wikström & Kautsky (2007) and Saarinen et al. (2018) who showed no clear habitat relationship between Fucus and non-Fucus structures.
Regarding macroinvertebrate diversity and abundance patterns, results and conclusions have been controversial, suggesting that the biodiversity effects of Fucus are strongly context-dependent (i.e. they vary with region or sites, Appendix 3). Such context-dependency could originate from differences in the way studies have been conducted or originate from inadequate spatial replication (e.g. Bracken et al., 2017). Beermann et al. (2013) showed that seaweed canopies of the fucoid algae Ascophyllum nodosum can have both positive and negative effects on understory organisms in the same environmental context. Råberg & Kautsky (2007) showed that wave exposure affected the facilitating function of Fucus in the Baltic Sea, where the positive effects of Fucus were strongest on wave protected sites. They also concluded that differences in sampling depth among sites could explain some of the divergent results in their study.
For Mytilus, the diversity and abundance patterns have been mostly consistent, showing positive effects of Mytilus on community density and species richness (Norling and Kautsky 2008; Koivisto and Westerbom, 2010; Koivisto and Westerbom, 2012). Prior research has also shown that the traits of the mussel bed affect its facilitating function, with generally older or denser mussel beds hosting the highest species richness (Suchanek, 1980; Tsuchiya and Nishihira, 1986) or higher density of the community (Koivisto and Westerbom, 2010). When looking at patterns over wave exposure gradients, Westerbom et al. (2019a) showed that the facilitating function of Mytilus is positive and increases towards increasing wave exposure, whereas the effect of Fucus was less clear. To conclude, we agree with Norling & Kautsky (2008), who stated that the presence of Mytilus likely is as important for species diversity in the Baltic Sea as is the presence of Fucus vesiculosus. Bearing in mind that Mytilus habitat dominates the seascapes on rocky reefs down to > 25 – 30 meters depth in this system (Rinne et al., 2021) where they practically are the sole biogenic habitat, their positive effects stretch deep in the reef ecosystem and must be considered substantial. This pattern contrasts shallow areas, where canopy-forming algae occur side by side with many other biogenic structures that also facilitate species richness and density, likely lessening the importance of a single habitat provider (Kraufvelin & Salovius, 2004; Saarinen et al., 2018).
Biodiversity, productivity and stability are three critical features of ecosystems (Worm & Duffy, 2003). Ecosystem research often emphasizes quality elements such as species richness and diversity. Of equal importance to ecosystem function are the quantity elements, i.e. the amount of materials the habitats produce, which may be especially important for the trophic capacity of habitats (Dolbeth et al., 2005). We have so far discussed our results from a quality perspective, and we have shown that the Mytilus and the Fucus habitats are very similar in terms of their biodiversity effects. However, the most distinct difference in this study was seen in the quantity elements, i.e. in the abundance and biomass patterns of the associated fauna. Here, Mytilus clearly outperformed Fucus indicating that the Mytilus habitat is also associated with a high food web support to higher trophic levels. Rodil et al. (2020) compared secondary production among five different coastal habitats in this very same coastal system. They found that the Mytilus habitat had the greatest macroinvertebrate abundance and biomass and showed the highest secondary production among all studied habitats. Similar patterns have been shown for oyster reefs in other coastal systems (Wong et al., 2011), suggesting that bivalve habitats are absolutely and relatively hot spots areas for productivity and biodiversity. Naturally, net primary production is much higher in the Fucus than in Mytilus habitat, but gross primary production is surprisingly high also in the mussel habitat (Attard et al., 2020).
What are the possible processes and mechanisms behind our results? As a correlative study, we cannot explain the mechanisms behind observed patterns, but we believe there are two likely explanations. While a low number of species characterizes the northern Baltic Sea, the density of these species can be extremely high. Most of the species in the Baltic Sea are motile moving freely among different habitats, which results in an omnipresent distribution of species. However, abundance and biomass patterns of the species differ due to the structural characteristics of different habitats. While Mytilus reefs e.g. trap a considerable amount of sediments and organic products within the mussel matrix, enlarging the niche space for infauna and detrivores (Tsuchiya & Nishihira, 1986; Kochmann et al., 2008), similar sediment accumulation does not occur in the Fucus habitat. Perhaps even more important is the thallus - or whiplash - movement by Fucus that causes mechanical abrasion on bottoms within the reach of the thallus (Figure 6). Thallus movement causes a reduction in sediment loads, it reduces the coverage of filamentous algae (Kiirikki, 1996), but more importantly in the context of this study, likely reduces epifaunal recruitment (Leonard, 1999) and/or increases dislodgement of already established understory organisms (Dayton, 1975). While the whiplash effect has been shown to control the establishment of filamentous algae around Fucus (Kiirikki, 1996), and while its negative effects on the understory community are well described (e.g. Grant, 1977), it has not been used to explain the variation in the macroinvertebrate assemblage structure in Fucus habitats in the Baltic Sea (see however Kersen et al., 2011). Based on previous findings, one must ask whether the recorded positive community effects of Fucus are generic in this system or whether they mainly are valid for the most sheltered sites (see also Watt & Scrosati, 2013) where studies generally have been conducted. The aim of this study was to compare the invertebrate assemblage at sites where both Mytilus and Fucus are common, which also narrowed our spatial scope. The findings could have been different towards even lower wave exposure levels, but here Mytilus is no longer bed forming and loses its habitat forming characteristics (Westerbom & Jattu, 2006), thus preventing a controlled comparison in very sheltered locations.
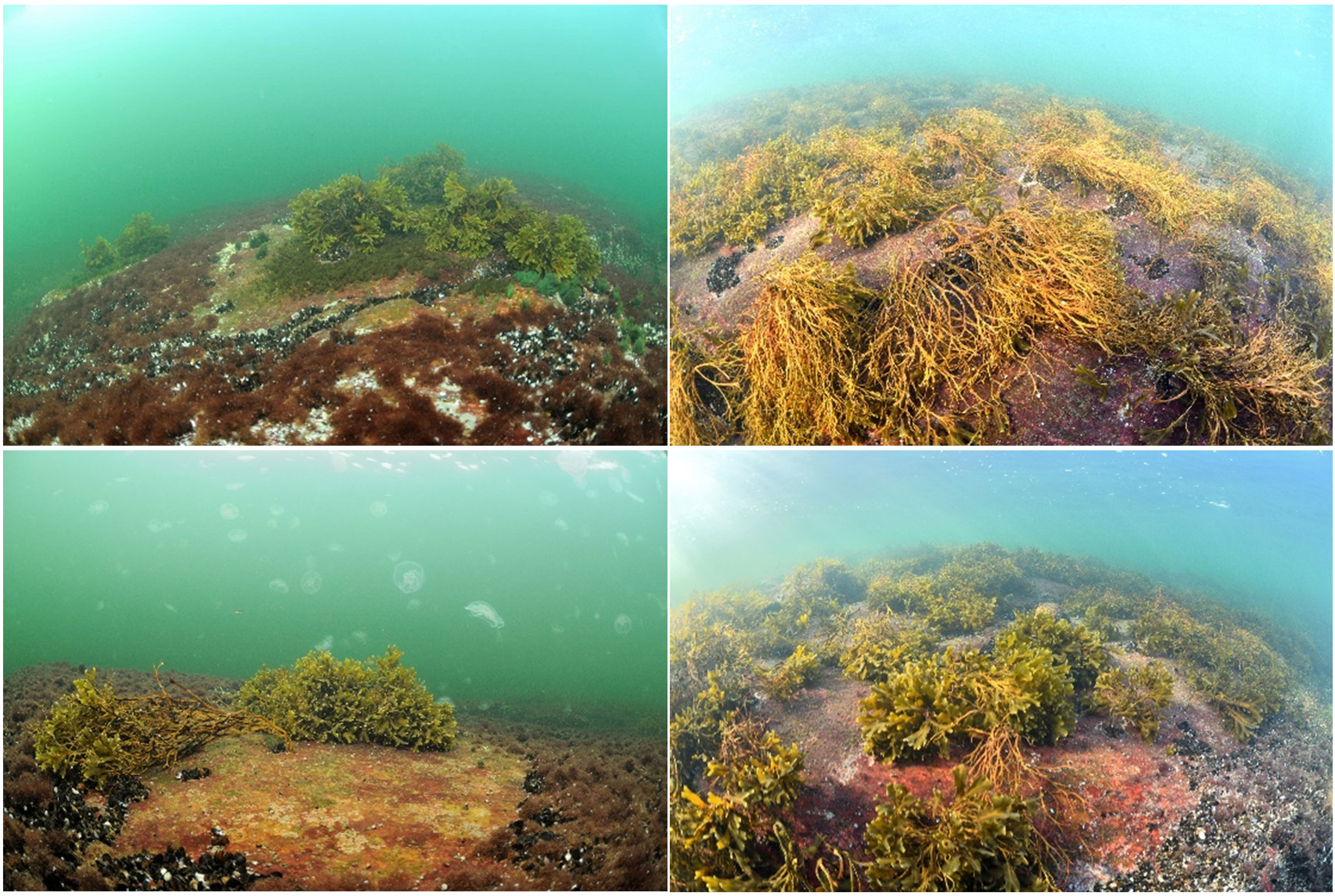
Figure 6 Stands of Fucus showing typical whiplash effects in different sized Fucus patches. The biggest mussels in the pictures are ca 2 cm in length and Fucus varies in size from ca 25 – 40 cm.
One question of interest remains. Why has the Fucus habitat in historic publications been considered critically important for macroinvertebrate biodiversity in the Baltic Sea when contemporary studies one after the other fail in finding any generic and conclusive patterns? Answering the question goes beyond this study, but we will offer one plausible explanation. From historic records, we know that there has been a substantial loss in Fucus coverage and an increased fragmentation of the habitat (see Sahla et al., 2020 with references). Large-scale losses and fragmentation of habitats are often followed by a reduction in community structure and increase in community similarity, leading to homogenization and impoverishment of biodiversity (Olden et al., 2004). The winners in this biotic homogenization process are the generalist species, while the losers are the specialists (e.g. Devictor et al., 2008). Whether habitat fragmentation and homogenization of the species pool is the underlying explanation for the findings in contemporary studies and why present studies often differ in their findings from historic remains unsolved. Nonetheless, together they call for a re-assessment of our perception and understanding of how contemporary biogenic habitats function and differ and how biodiversity at large is structured in a changing Baltic Sea. At current time, several specific habitats are insufficiently included in monitoring activities or classification systems of the northern Baltic Sea and we lack an understanding of how these specific habitats contribute to biodiversity and productivity (Hansen & Snickars, 2014; HELCOM, 2015; HELCOM, 2018; Rinne et al., 2021). In Finland, for example - except for Fucus monitoring - the availability of long-term habitat data fulfilling monitoring requirements from rocky reefs is sub-standard and threat assessments for the rocky reef system are not based on long-term benthic monitoring data.
Ecology is not a static discipline but evolves and changes over time. New pieces of knowledge or the critical re-analysis of existing assumptions generate improved and upgraded hypotheses; encourage the replacement of hypotheses or even formulation of new ones (González Del Solar and Marone, 2001). However, existing views often steer our way of thinking and dictate the very way research is being conducted through academic tradition, also affecting management practices. Without critical examination of the validity of assumptions, perceptions remain and may even lead to “immobile truths” that are passed within academic generations (González Del Solar and Marone, 2001). As science steers management, a key question is whether the current approaches and tools for management are the best ones available, or whether existing principles should be reassessed or even challenged? To guarantee an appropriate assessment of the status of biodiversity, new monitoring efforts alongside with suitable indicators, reflecting the status and trends of different components of the ecosystem, need to be developed and put into action (Bojārs, 2012; HELCOM, 2015; HELCOM, 2018). Our results show a motivated call for widening the focus of benthic conservation efforts, monitoring and the overall research scope in the northern Baltic Sea, and in Finland particularly. It is time to look at the rocky shore system in a much more diverse way and it is time to better assess how an altered sea leads to changes in biogenic structures and how these changes manifest themselves in biodiversity but also in productivity. By comparing the structure and diversity in parallel samples from Mytilus and Fucus, we have shown here that the Mytilus habitat in productivity and diversity is well comparable to that of Fucus and the Mytilus habitat would warrant a much greater attention than what it currently receives.
Data availability statement
The raw data supporting the conclusions of this article will be made available by the authors, without undue reservation.
Author contributions
Both authors contributed with an equal share to the paper. MW led the writing of the final draft. All authors contributed to the article and approved the submitted version.
Funding
The project was funded through Svenska kulturfonden, Walter and Andrée de Nottbeck Foundation and Onni Talaan Säätiö.
Acknowledgments
We are highly thankful to Dr. Patrik Kraufvelin for comments on a draft version of this manuscript. We thank the staff at Tvaürminne Zoological Station for providing excellent working facilities both indoors and outdoors.
Conflict of interest
The authors declare that the research was conducted in the absence of any commercial or financial relationships that could be construed as a potential conflict of interest.
Publisher’s note
All claims expressed in this article are solely those of the authors and do not necessarily represent those of their affiliated organizations, or those of the publisher, the editors and the reviewers. Any product that may be evaluated in this article, or claim that may be made by its manufacturer, is not guaranteed or endorsed by the publisher.
Supplementary material
The Supplementary Material for this article can be found online at: https://www.frontiersin.org/articles/10.3389/fmars.2022.929899/full#supplementary-material
References
Airoldi L., Balata D., Beck M. W. (2008). The Gray zone: Relationships between habitat loss and marine diversity and their applications in conservation. J. Exp. Mar. Biol. Ecol. 366, 8–15. doi: 10.1016/j.jembe.2008.07.034
Anderson M. J., Gorley R. N., Clarke K. R. (2008). PERMANOVA+for PRIMER: Guide to software and statistical methods (Plymouth, UK: PRIMER-E), 214.
Angelini C., Altieri A. H., Silliman S. R., Bertness M. D. (2011). Interactions among foundation species and their consequences for community organization, biodiversity, and conservation. BioScience 61, 782–789. doi: 10.1525/bio.2011.61.10.8
Attard K. M., Rodil I. F., Berg P., Mogg A., Westerbom M., Norkko A., et al. (2020). Metabolism of a subtidal rocky mussel reef in a high-temperate setting: pathways of organic c flow. Mar. Ecol. Prog. Ser. 645, 51–54. doi: 10.3354/meps13372
Attard K. M., Rodil I-, Glud R. N., Berg P., Norkko J., Norkko A. (2019). Seasonal ecosystem metabolism across shallow benthic habitats measured by aquatic eddy covariance. Limnol Oceanogr 4, 79–86. doi: 10.1002/lol2.10107
Barnosky A. D., Matzke N., Tomiya S., Wogan G. O., Swartz B., Quental T. B., et al. (2011). Has the earth's sixth mass extinction already arrived? Nature 471 (7336), 51–57. doi: 10.1038/nature09678
Beermann A. J., Ellrich J. A., Molis M., Ricardo A., Scrosati R. A. (2013). Effects of seaweed canopies and adult barnacles on barnacle recruitment: The interplay of positive and negative influences. J. Exp. Mar. Biol. Ecol. 448, 162–170. doi: 10.1016/j.jembe.2013.07.001
Bishop M. J., Fraser J., Gribben P. E. (2013). Morphological traits and density of foundation species modulate a facilitation cascade in Australian mangroves. Ecology 94, 1927–1936. doi: 10.1890/12-1847.1
Bojārs E. (2012) Availability of marine biodiversity data in Estonia, Latvia, Finland and Sweden for the marmoni project needs. Available at: http://marmoni.balticseaportal.net/wp/wp-content/uploads/2011/03/Report-on-availability-of-marine-biodiversity-data.pdf.
Bracken M. E. S., Douglass J. G., Perini V., Trussell G. C. (2017). Spatial scale mediates the effects of biodiversity on marine primary producers. Ecology 98, 1434–1443. doi: 10.1002/ecy.1812
Bruno J. F., Bertness M. D. (2001). “Habitat modification and facilitation in benthic marine communities,” in Marine community ecology. Eds. Bertness M. D., Gaines S. D., Hay M. E. (Massachusetts, USA: Sinauer Associates Inc), 201–218.
Bué M., Smale D. A., Natanni G., Marshall H., Moore P. J. (2020). Multiple-scale interactions structure macroinvertebrate assemblages associated with kelp understory algae. Diversity Distribution 26, 1551–1565. doi: 10.1111/ddi.13140
Dayton P. K. (1975). Experimental evaluation of ecological dominance in a rocky intertidal algal community. Ecol. Monogr. 45, 137–159. doi: 10.2307/1942404
Devictor V., Julliard R., Clavel J., Jiguet F., Lee A., Couvet D. (2008). Functional biotic homogenization of bird communities in disturbed landscapes. Global Ecol. Biogeogr 17, 252–261. doi: 10.1111/j.1466-8238.2007.00364.x
Díaz E. R., Erlandsson J., Westerbom M., Kraufvelin P. (2015). Depth-related spatial patterns of sublittoral blue mussel beds and their associated macrofauna diversity revealed by geostatistical analyses. Mar. Ecol. Prog. Ser. 540, 121–134. doi: 10.3354/meps11461
Dolbeth M., Lillebø A. I., Cardoso P. G., Ferreira S. M., Pardal M. A. (2005). Annual production of estuarine fauna in different environmental conditions: an evaluation of the estimation methods. J. Exp. Mar. Biol. Ecol. 326, 115–127. doi: 10.1016/j.jembe.2005.05.010
González Del Solar R., Marone L. (2001). The “Freezing” of science: Consequences of the dogmatic teaching of ecology. BioScience 51, 683–686. doi: 10.1641/0006-3568(2001)051[0683:TFOSCO]2.0.CO;2
Grant W. S. (1977). High intertidal community organization on a rocky headland in Maine, USA. Mar. Biol. 44, 15–25. doi: 10.1007/BF00386900
Gribben P. E., Kimbro D. L., Vergés A., Gouhier T. C., Burrell S., Garthwin R. G., et al. (2017). Positive and negative interactions control a facilitation cascade. Ecosphere 8, e02065. doi: 10.1002/ecs2.2065
Haage P., Jansson B. O. (1970). Quantitative investigations of the Baltic Fucus belt macrofauna. Ophelia 8, 187–195. doi: 10.1080/00785326.1970.10429558
Hansen J. P., Snickars M. (2014). Applying macrophyte community indicators to assess anthropogenic pressures on shallow soft bottoms. Hydrobiologia 738, 171–189. doi: 10.1007/s10750-014-1928-z
HELCOM (2015) BALSAM project 2013-2015: Recommendations and guidelines for benthic habitat monitoring with method descriptions for two methods for monitoring of biotope and habitat extent. Available at: https://helcom.fi/media/publications/Recommendations-and-guidelines-for-benthic-habitat-monitoring-in-the-Baltic-Sea.pdf.
HELCOM (2018) Background document to the 2018 HELCOM ministerial meeting implementation of the Baltic Sea action plan 2018: Three years left to reach good environmental status. Available at: https://helcom.fi/wp-content/uploads/2019/08/Implementation-of-the-BSAP-2018.pdf.
Henseler C., Nordström M. C., Törnroos A., Snickars M., Pecuchet L., Lindegren M., et al. (2019). Coastal habitats and their importance for the diversity of benthic communities: a species- and trait-based approach. Estuar Coast. Shelf Sci. 226, 106272. doi: 10.1016/j.ecss.2019.106272
Hewitt J. E., Thrush S. F., Lundquist C. (2017). Scale-dependence in ecological systems. in eLS (Chichester, UK: John Wiley and Sons). doi: 10.1002/9780470015902.a0021903
Kangas P., Autio H., Hällfors G., Luther H., Niemi Å., Salemaa H. (1982). A general model of the decline of fucus vesiculosus at tvärminne, south coast of Finland in 1977–81. Acta Bot. Fenn. 118, 1–27.
Kautsky H., Kautsky L., Kautsky N., Kautsky U., Lindblad C. (1992). Studies on the fucus vesiculosus community in the Baltic Sea. Acta Phytogeogr Suecica 78, 33–48.
Kautsky L., Svensson S. (2003). “Life in the Baltic Sea,” in Environmental science: understanding, protecting and managing the environment in the Baltic Sea region, vol. 1 . Eds. Rydén L., Migula P., Andersson M. (Uppsala: Baltic University Press), 148–181.
Kautsky N., Wallentinus I. (1980). Nutrient release from a Baltic Mytilus-red algal community and its role in benthic and pelagic productivity. Ophelia Suppl1, 17–30.
Kersen P., Kotta J., Bučas M., Kolesova N., Dekere Z. (2011). Epiphytes and associated fauna on the brown alga Fucus vesiculosus in the Baltic and the north seas in relation to different abiotic and biotic variables. Mar. Ecol. 32, 87–95. doi: 10.1111/j.1439-0485.2010.00418.x
Khalaman V., Komendantov A., Golubovskaya N., Manoylina P. (2021). Comparative efficiency of Mytilus edulis as engineering species for shallow-water fouling communities on artificial structures in the white Sea. J. Mar. Biol. Assoc. United Kingdom 101, 511–525. doi: 10.1017/S0025315421000424
Kiirikki M. (1996). Experimental evidence that Fucus vesiculosus (Phaeophyta) controls filamentous algae by means of the whiplash effect. Eur. J. Phycol 31, 61–66. doi: 10.1080/09670269600651201
Kochmann J., Buschbaum C., Volkenborn N., Reise K. (2008). Shift from native mussels to alien oysters: Differential effects of ecosystem engineers. J. Exp. Mar. Biol. Ecol. 364 (1), 1—10. doi: 10.1016/j.jembe.2008.05.015
Koivisto M., Westerbom M. (2010). Habitat structure and complexity as determinants of biodiversity in blue mussel beds on sublittoral rocky shores. Mar. Biol. 157, 1463–1474. doi: 10.1007/s00227-010-1421-9
Koivisto M., Westerbom M. (2012). Invertebrate communities associated with blue mussel beds in a patchy environment: a landscape ecology approach. Mar. Ecol. Prog. Ser. 471, 101–110. doi: 10.3354/meps10010
Kraufvelin P., Salovius S. (2004). Animal biodiversity in Baltic rocky shore macroalgae: Can Cladophora glomerata compensate for lost Fucus vesiculosus? Estuar Coast. Shelf Sci. 61, 369–378. doi: 10.1016/j.ecss.2004.06.006
Leonard G. H. (1999). Positive and negative effects of intertidal algal canopies on recruitment and survival of barnacles. Mar. Ecol. Prog. Ser. 178, 241–249. doi: 10.3354/meps178241
Norling P., Kautsky N. (2008). Patches of the mussel Mytilus sp. are islands of high biodiversity in subtidal sediment habitats in the Baltic Sea. Aquatic Biology 4, 75–87. doi: 10.3354/ab00096
Olden J. D., Poff N. L., Douglas M. B., Douglas M., Fausch K. D. (2004). Ecological and evolutionary consequences of biotic homogenization. Trends Ecol. Evol. 19, 18–24. doi: 10.1016/j.tree.2003.09.010
Öst M., Kilpi M. (1998). Blue mussels Mytilus edulis in the Baltic: Good news for foraging eiders Somateria mollissima. Wildlife Biol. 4, 81–89. doi: 10.2981/wlb.1998.004
Passarelli C., Olivier F., Paterson D. M., Meziane T., Hubas C. (2014). Organisms as cooperative ecosystem engineers in intertidal flats. J. Sea Res. 92, 92–101. doi: 10.1016/j.seares.2013.07.010
Råberg S., Kautsky L. (2007). A comparative biodiversity study of the associated fauna of perennial fucoids and filamentous algae. Estuar Coast. Shelf Sci. 73, 249–258. doi: 10.1016/j.ecss.2007.01.005
Rinne H., Boström M., Björklund C., Sahla M. (2021). Functionality of HELCOM HUB classification in describing variation in rocky shore communities of the northern Baltic Sea. Estuar Coast. Shelf Sci. 249, 107044. doi: 10.1016/j.ecss.2020.107044
Rodil I. F., Attard K. M., Norkko J., Glud R. N., Norkko A. (2020). Estimating respiration rates and secondary production of macrobenthic communities across coastal habitats with contrasting structural biodiversity. Ecosystems 23, 630–647. doi: 10.1007/s10021-019-00427-0
Saarinen A., Salovius-Lauren S., Mattila J. (2018). Epifaunal community composition in five macroalgal species - what are the consequences if some algal species are lost? Estuar Coast. Shelf Sci. 207, 402–413. doi: 10.1016/j.ecss.2017.08.009
Sahla M., Tolvanen H., Ruuskanen A., Kurvinen L. (2020). Assessing long term change of Fucus spp. communities in the northern Baltic Sea using monitoring data and spatial modelling. Estuar Coast. Shelf Sci. 245, 107023. doi: 10.1016/j.ecss.2020.107023
Suchanek T. H. (1980). Diversity in natural and artificial mussel bed communities of Mytilus californianus. Am. Zool 20, 807.
Tsuchiya M., Nishihira M. (1986). Islands of Mytilus edulis as a habitat for small intertidal animals: effect of Mytilus age structure on the species composition of the associated fauna and community organization. Mar. Ecol. Prog. Ser. 31, 171–178. doi: 10.3354/meps031171
Watt C. A., Scrosati R. A. (2013). Regional consistency of intertidal elevation as a mediator of seaweed canopy effects on benthic species richness, diversity, and composition. Mar. Ecol. Prog. Ser. 491, 91–99. doi: 10.3354/meps10521
Westerbom M., Jattu S. (2006). Effects of wave exposure on the sublittoral distribution of blue mussels Mytilus edulis in a heterogeneous archipelago. Mar. Ecol. Prog. Ser. 306, 191–200. doi: 10.3354/meps306191
Westerbom M., Kraufvelin P., Erlandsson J., Korpinen S., Mustonen O., Díaz E. (2019a). Wave stress and biotic facilitation drive community composition in a marginal hard-bottom ecosystem. Ecosphere 10, e02883. doi: 10.1002/ecs2.2883
Westerbom M., Lappalainen A., Mustonen O., Norkko A. (2018). Trophic overlap between expanding and contracting fish predators in a range margin undergoing change. Sci. Rep. 8 7895 . doi: 10.1038/s41598-018-25745-6
Westerbom M., Mustonen O., Jaatinen K., Kilpi M., Norkko A. (2019b). Population dynamics at the range margin: implications of climate change on sublittoral blue mussels (Mytilus trossulus). Front. Mar. Sci. 6. doi: 10.3389/fmars.2019.00292
Westerbom M., Mustonen O., Kilpi M. (2008). Distribution of a marginal population of Mytilus edulis: responses to biotic and abiotic processes at different spatial scales. Mar. Biol. 153, 1153–1164. doi: 10.1007/s00227-007-0886-7
Wikström S. A., Kautsky L. (2007). Structure and diversity of invertebrate communities in the presence and absence of canopy-forming Fucus vesiculosus in the Baltic Sea. Estuar Coast. Shelf Sci. 72, 168–176. doi: 10.1016/j.ecss.2006.10.009
Wong M. C., Peterson C. H., Piehler M. G. (2011). Evaluating estuarine habitats using secondary production as a proxy for food web support. Mar. Ecol. Prog. Ser. 440, 11–25. doi: 10.3354/meps09323
Worm B., Duffy J. E. (2003). Biodiversity, productivity and stability in real food webs. Trends Ecol. Evol. 18, 628–632. doi: 10.1016/j.tree.2003.09.003
Yakovis E., Artemieva A. (2021). Effects of a trophic cascade on a multi-level facilitation cascade. J. Anim. Ecol. 90, 2462–2470. doi: 10.1111/1365-2656.13558
Keywords: biodiversity, facilitation, foundation species, community composition, conservation, Fucus, Mytilus, Baltic Sea
Citation: Westerbom M and Koivisto M (2022) Mussels and canopy-forming algae as ecosystem engineers: their contribution to community organization in the rocky sublittoral. Front. Mar. Sci. 9:929899. doi: 10.3389/fmars.2022.929899
Received: 27 April 2022; Accepted: 06 July 2022;
Published: 04 August 2022.
Edited by:
Francesca Porri, South African Institute for Aquatic Biodiversity, South AfricaReviewed by:
Edson A. Vieira, Federal University of Rio Grande do Norte, BrazilCamilla Bertolini, Ca’ Foscari University of Venice, Italy
Mayya Gogina, Leibniz Institute for Baltic Sea Research (LG), Germany
Copyright © 2022 Westerbom and Koivisto. This is an open-access article distributed under the terms of the Creative Commons Attribution License (CC BY). The use, distribution or reproduction in other forums is permitted, provided the original author(s) and the copyright owner(s) are credited and that the original publication in this journal is cited, in accordance with accepted academic practice. No use, distribution or reproduction is permitted which does not comply with these terms.
*Correspondence: Mats Westerbom, mwesterbom@gmail.com