- 1Coastal and Marine Systems group, Van Hall Larenstein University of Applied Sciences, Leeuwarden, Netherlands
- 2Marine Animal Ecology group, Wageningen University, Wageningen University & Research, Wageningen, Netherlands
- 3Wageningen Marine Research, Wageningen University & Research, Den Helder, Netherlands
The massive die-off of the sea urchin Diadema antillarum in 1983–1984 is one the main reasons for low coral recruitment and little coral recovery in the Caribbean. As the natural recovery of D. antillarum is slow to non-existent, multiple restoration studies have been attempted. There are currently three different approaches to obtain individuals for restocking: the translocation of wild-collected juveniles or adults, lab-reared juveniles cultured from wild-collected settlers, or lab-reared juveniles cultured from gametes. All three methods are costly and can only be applied on a relatively small scale. We here propose a fourth, new, approach, which we term assisted natural recovery (ANR) of D. antillarum populations. ANR, a concept already applied in terrestrial restoration to restore forests and grasslands, can accelerate succession by removing barriers to natural recovery. In this study, performed on the Dutch Caribbean island of Saba, suitable settlement substrate was provided in the form of bio ball streamers that were attached to the reef shortly before the settlement season. At the end of the experiment, reefs with streamers had significantly higher D. antillarum recruit densities than control reefs without additional settlement substrate, indicating that the lack of settlement substrate is an important factor constraining natural recovery. However, D. antillarum recruit abundance was low compared to the measured settlement rates, possibly due to low post-settlement survival. The size distribution of recruits showed that recruits almost never became larger than 20 mm, which is likely due to predation. We conclude that, next to low settlement availability, low post-settlement survival and high predation on recruits also constrain the natural recovery of D. antillarum populations on Saba. To improve the survival of settlers till adults, we propose to 1) reduce predation on settlers by using bio balls or other substrates that can provide shelter to larger individuals and 2) optimize the reef habitat by removing macroalgae, either manually or by facilitating other herbivores. To improve the survival of recruits, we suggest to 1) choose sites with a known lower predation density or 2) protect recruits with a corral around the reef underneath the streamers. The combination of these measures could improve prospects for ANR, and we expect this new approach can contribute to the recovery of D. antillarum populations in the future.
Introduction
The accelerated degradation of Caribbean coral reefs started in the 1970s, when diseases decimated the most important reef-building corals (Gladfelter, 1982; Aronson & Precht, 2001). The decennia that followed were characterized by sequential losses in coral cover by hurricanes, global warming, and additional diseases (Jackson et al., 2014). The degradation of Caribbean coral reefs proceeded quicker than in the Indo-Pacific region, largely because of exceptionally low coral recruitment rates (Precht et al., 2020). This was mainly a consequence of overfishing of herbivorous fish (Jackson et al., 2001; Pandolfi et al., 2003), followed by a massive die-off of the sea urchin Diadema antillarum in 1983 and 1984 (Lessios et al., 1984). At the time of the die-off, D. antillarum was the most abundant remaining herbivore on Caribbean reefs. After 98% of the population had succumbed (Lessios, 2016), the cover of macroalgae increased within days (Carpenter, 1988) and macroalgae have remained dominant on many Caribbean reefs since then (Jackson et al., 2014). Macroalgae are principal competitors of adult corals (Jompa & McCook, 2002; Box & Mumby, 2007) and limit coral recruitment (McCook et al., 2001, Box & Mumby, 2007; Arnold et al., 2010), thereby reducing the ability of the coral reef to recover from disturbances.
With a few exceptions (Carpenter & Edmunds, 2006; Debrot & Nagelkerken, 2006; Myhre & Acevedo-Gutiérrez, 2007), mostly limited to shallow and sheltered waters, the recovery of D. antillarum populations has been very slow to non-existing (Lessios, 2016). The underlying factors constraining the recovery of D. antillarum are not exactly known and will very likely differ per location (Lessios, 2016). For instance, studies of D. antillarum settlement rates show that at some locations, for example, around Puerto Rico (Williams et al., 2011), the Florida Keys (Miller et al., 2009), and Mexico (Maldonado-Sánchez et al., 2019), settlement rates can be very low throughout the year. At these locations, low fertilization success (Lessios, 1988; Lessios, 2005; Feehan et al., 2016) and the lack of an upstream larval source-population (Roberts, 1997) possibly constrain recovery. At other locations, for example, around Curaçao (Vermeij et al., 2010), Puerto Rico (Williams et al., 2010; Williams et al., 2011), St. Eustatius (Hylkema et al., 2022), and Saba (Klokman & Hylkema, in prep), peak settlement rates are in the same order of magnitude as measured before the die-off around Curaçao (Bak, 1985). Hence, at these locations, other factors seem to constrain the natural recovery of D. antillarum.
At the few places where D. antillarum densities on the reef actually recovered, this was correlated with a decrease in macroalgae and an increase in coral recruitment (Edmunds & Carpenter, 2001; Carpenter & Edmunds, 2006; Myhre & Acevedo-Gutiérrez, 2007; Idjadi et al., 2010). These results highlight the importance of the recovery of D. antillarum for increased resilience of Caribbean coral reefs (Lessios, 2016). However, since it could take decades before D. antillarum populations recover naturally throughout the Caribbean (Chiappone et al., 2013) and a new die-off is again reducing population densities in parts of the Caribbean in 2022 (AGRRA, 2022), active intervention might help accelerate D. antillarum recovery (Adam et al., 2015). Three different methods have so far been attempted to help restore D. antillarum populations, and all rely on the restocking of juvenile or adult individuals. These are either obtained by translocating wild D. antillarum from recovered populations (Maciá et al., 2007; Burdick, 2008; Dame, 2008), by collecting settlers and headstarting them till the juvenile stage in a land-based nursery (Williams, 2017; Williams, 2022) or by culturing juveniles in captivity through their larval stages starting from gametes (Pilnick et al., 2021; Wijers et al. in prep).
When using wild D. antillarum for restocking, a local, stable population is needed with thousands of individuals that can likely be transplanted without too many consequences for the donor location itself. This is seldom the case. Collecting juvenile D. antillarum with settlement collectors and raising them in a land-based facility appears more feasible and has already been successfully achieved on Puerto Rico (Williams, 2017; Williams, 2021). D. antillarum cultivation from gametes is an alternative method to produce juveniles year-round. Despite the sensitive nature of the larvae and the relatively long larval phase (Eckert, 1998; Bielmyer et al., 2005), several lab-based culture runs have recently produced over 100 settlers (Pilnick et al., 2021, Wijers et al. in prep). In contrast to using wild-caught individuals, headstarting collected settlers and culturing juveniles in the laboratory from gametes appear scalable, but the costs per juvenile remain relatively high, limiting the large-scale restoration potential of these methods. To circumvent the limitations of current restoration techniques, we here propose assisted natural recovery (ANR) as a new, scalable approach for restoring D. antillarum populations.
ANR is practiced in terrestrial ecosystems to aid the recovery of grasslands (Coiffait-Gombault et al., 2011) and forests (Hardwick et al., 2004; Shono et al., 2007; Mackenzie & Naeth, 2010). ANR aims to accelerate succession by reducing barriers to natural ecosystem recovery. It is an alternative to replanting practices and can be applied on a large scale at a relatively low cost. As the success of ANR is dependent on natural recovery processes, it only functions if some form of natural succession is already in progress (Hardwick et al., 2004; Shono et al., 2007). For forest regeneration, it often means the introduction of seed banks, the removal of competitors, and the minimization of disturbances (Hardwick et al., 2004; Shono et al., 2007). There are some analogies between forest regeneration and D. antillarum restoration. Just like the fact that trees can be replanted, restocking D. antillarum is possible. However, it is often resource consuming and therefore remains relatively limited in scale. At the same time, the essential conditions for natural recovery, indicated by high D. antillarum settlement rates on artificial substrates (Vermeij et al., 2010; Williams et al., 2010; Williams et al., 2011; Hylkema et al., 2022), appear to be present at certain locations. However, high settlement rates on artificial substrates do not always result in natural recovery of D. antillarum populations. This discrepancy can most likely be explained by two barriers to natural recovery, either low natural settlement substrate availability (Rogers and Lorenzen, 2008) or low postsettlement survival, for example, through high predation pressure or low shelter availability (Bechtel et al., 2006; Vermeij et al., 2010; Williams et al., 2011; Hylkema et al., 2022).
ANR of D. antillarum might be possible if suitable settlement substrate is provided and/or if post-settlement mortality is reduced. Hylkema et al. (2022) compared five different types of D. antillarum settlement collectors. It was concluded that strings of plastic bio balls (Figure 2; hereinafter termed “bio ball streamers”) deployed mid-water were the most effective and reproducible method to monitor D. antillarum settlement. This study investigated if bio ball streamers attached to the reef shortly before the settlement season in an area with high potential settlement rates can enhance actual settlement and will result in an increased recruitment of D. antillarum. As such, this study aimed at providing insight whether the availability of suitable settlement substrate is the major barrier in the recovery of D. antillarum.
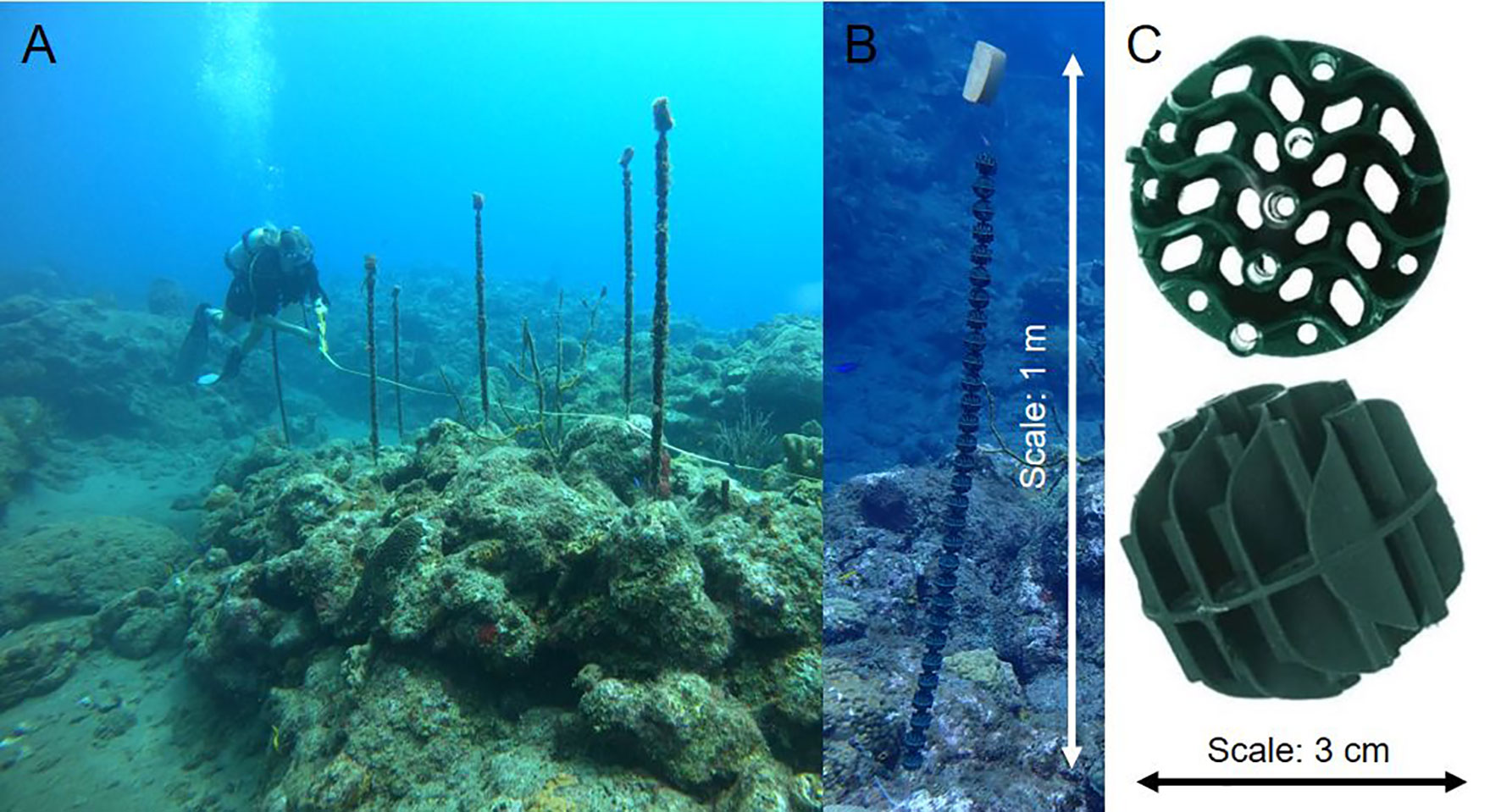
Figure 2 (A) Patch reef (no. 4) with streamers on which the transect tape is removed after monitoring. (B) Streamer with buoy and (C) Close-up of bio balls.
Materials and Methods
In April 2021, six patch reefs were selected at the Southwestern corner of Saba, Caribbean Netherlands, at the dive site locally known as Ladder Labyrinth. Coral reefs at this location developed on hard substrate of volcanic origin (Polunin & Roberts, 1993). The patch reefs were selected to be similar in terms of high shelter availability, a longest dimension of 6–12 m, and at a depth of approximately 15 m. This resulted in the selection of 2 smaller reefs and 4 larger reefs at a depth of 12–18 m (Table 1). To assign experimental treatments, each reef was paired to the reef with the most similar largest dimension. For each pair, treatment and control were randomly assigned, resulting in three treatment and three control reefs (Figure 1). Permanent markers were attached to both sides of the widest cross section of each reef to mark the monitoring area.
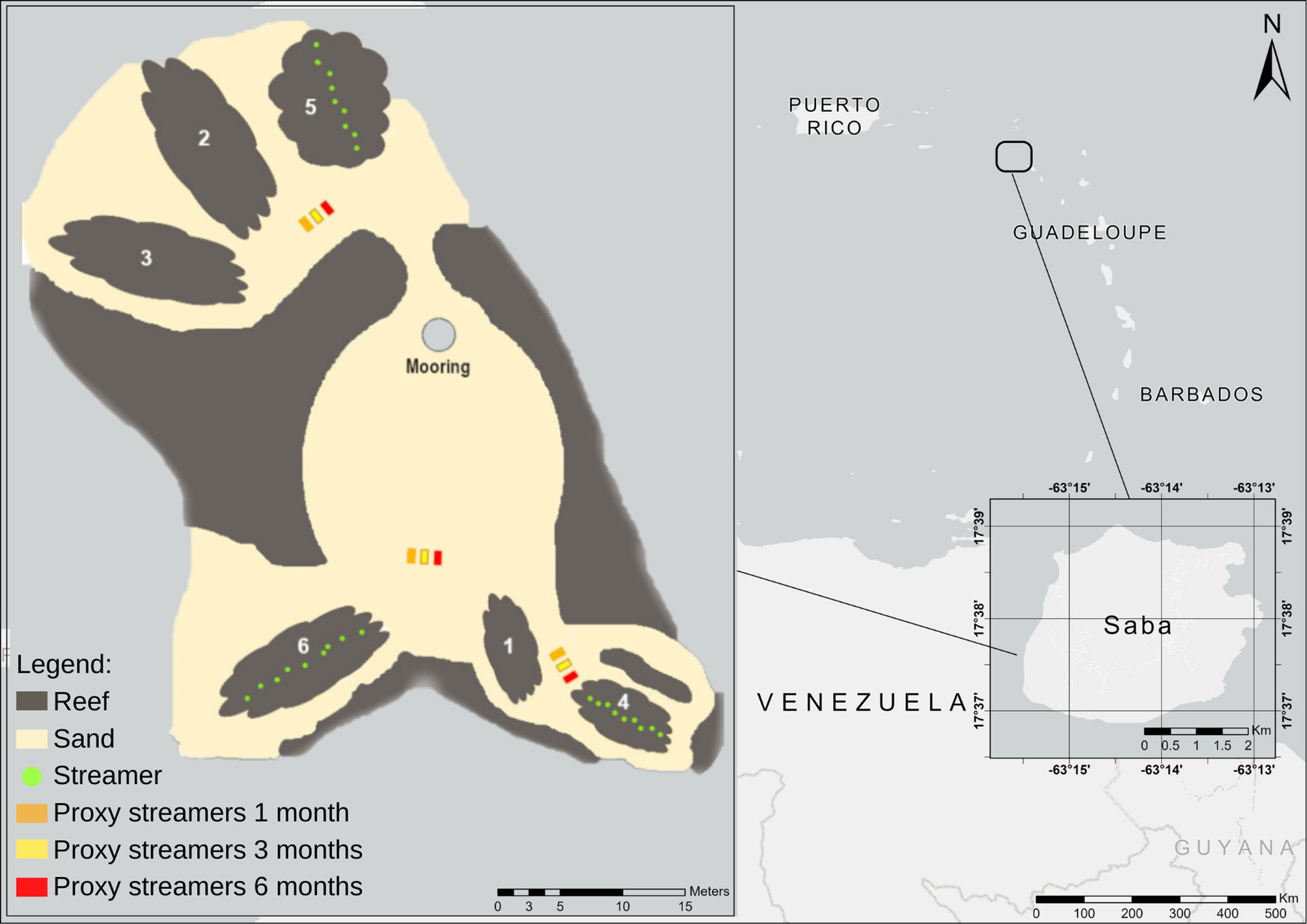
Figure 1 Location of Saba, Caribbean Netherlands in the Caribbean region and location Ladder labyrinth, showing the positions of the experimental reefs and proxy streamers.
To enhance D. antillarum settlement, nine streamers, consisting of 30 bio balls stringed on a fishing line (Figure 2), were attached to each treatment reef right before the start of the settlement season (Hylkema et al., 2022) in May 2021. The bio balls were 3 cm in diameter and made from polypropylene (PP). The streamers were attached to stainless steel rings, which were epoxied into the reef matrix and kept upright using a small buoy on top. Streamers were approximately 1 m long and had a planar surface of 0.04 m2. The streamers were placed close to the transect line that was deployed between the two permanent markers. The experimental area was a band of reef 1 m to each side of the transect line. The control reefs were left undisturbed.
To get an indication of settlement on the streamers attached to the reefs, three extra sets of three streamers were attached to separate cinder blocks. These streamers functioned as a proxy for the streamers on the reef and were replaced monthly to get an indication of settlement on the streamers attached to the reef. Each cinder block was placed at a 2-m distance from a treatment reef on sand. Extra fishing line was used to position each set of proxy streamers at the same depth as the streamers attached to the reef. To determine settlement on the proxy streamers, divers removed the streamers from the cinder block, stored them in a ziplock bag, and immediately attached new streamers. Once on the boat, the ziplock bags were stored in a cooler and transported to land. Each proxy streamer was shaken vigorously in five different white trays, and the number of D. antillarum settlers in each tray was precisely determined (Hylkema et al., 2022). Used streamers were rinsed in fresh water for 24 h and sun-dried before they were redeployed at the next monitoring. To provide insight in the period that D. antillarum settlers remained on the streamers and the suitability of streamers with a longer soaking time as a settlement substrate, six additional sets of three proxy streamers were deployed at the start of the experiment using the above-described methods. Three of these sets were retrieved and analyzed after 3 months and three after 6 months, at the end of the experiment.
The abundance of D. antillarum recruits on all six experimental reefs was determined every month, starting at the beginning of the experiment (May 2021) and ending 6 months later (November 2021) for a total of seven monitoring events. Monitoring was conducted during the day. A transect tape was positioned between the two permanent markers, and the area from 1m left to 1m right of the transect was thoroughly searched by trained observers for D. antillarum recruits using underwater flashlights. The test size of all recruits was estimated to the nearest millimeter using long-jawed calipers. The search time for recruits was standardized to 10 min per reef, excluding the time needed to record and measure the observed D. antillarum. After the monitoring, all D. antillarum were left undisturbed on the reefs.
To determine in which month D. antillarum recruits on the reefs could have settled, we used published settlement sizes and growth rates. Cultivation experiments in the laboratory show that the size of D. antillarum settlers is 0.5 mm (Eckert, 1998) and growth rates are linear for the first year (Idrisi et al., 2003). The initial growth in the laboratory was on average 3 mm per month (Idrisi et al., 2003), while observations in the field show that small juveniles in the size range of 4–11 mm can grow up to 6.7 mm per month (Randall et al., 1964). The predicted size ranges per monitoring and settlement month based on settlement and growth parameters from the literature are given in Table 2.

Table 2 Estimated size range (mm), based on growth rates fromIdrisi et al. (2003) and Randall et al. (1964), per settlement and monitoring month.
Statistical Analysis
A one-way ANOVA was used to determine if the settlement on the proxy streamers differed by month. Initial model validation, using untransformed data, indicated non-normality of the residuals and heterogeneity of the variance, which was corrected for using a square root transformation of the D. antillarum counts per streamer. Tukey post-hoc tests were performed to identify the months with significantly different settlement rates.
A Kruskal–Wallis test was conducted to determine if soaking time of the streamers had an effect on the number of D. antillarum per streamer. Streamers with a soaking time of 3 and 6 months (respectively collected in August and November 2021) were compared with streamers with a soaking time of only 1 month (collected in June 2021). A post-hoc Dunn’s test was performed to detect differences in settlement densities for different soaking times. To assess the effect of streamers with a longer soaking time on new settlement, Mann–Whitney U tests were conducted to compare settlement on streamers with a soaking time of 3 and 6 months with streamers that had a soaking time of only 1 month, all of which were collected on the same day.
Linear mixed models were used for the statistical inference of the monthly D. antillarum abundance and recruit size on the patch reefs. Treatment, monitoring month, monitoring area, and the interaction between treatment and monitoring month were considered as fixed factors. To correct for the monthly repeated counts at the same reefs, reef ID was included as a random factor. Models were run using the lmer function in the R package “lme4” (Bates et al., 2015), and model selection and validation were performed according to Zuur et al. (2009). The Akaike Information Criterion (AIC) was used to select the best-fitting model. For recruit density, this was the model including treatment, monitoring month, and their interaction. For the recruit size, this was the model including only monitoring month. Plotting the residuals versus the fitted values revealed heterogeneity of variance, while a qqplot showed that the residuals were not normally distributed. Both issues were resolved when the density and size were square-root transformed. To test in which months treatment significantly affected D. antillarum density, treatment was contrasted within month using the package “emmeans” (Lenth and Herve, 2019).
All statistical analyses were performed with R (R Core Team, 2021) using R studio version 1.2.5001. All graphs were made using the R package “ggplot2.” P-values <0.05 were considered statistically significant and reported values are means ± standard deviation, except for the graphs, in which the standard error was used.
Results
Settlement on the Proxy Streamers
After 1 month (in June 2021), the first D. antillarum settlers were observed on the streamers (Figure 3). The average number of D. antillarum settlers on the proxy streamers (Figure 4) differed significantly per month (P<0.001, Table 3). Tukey post-hoc tests revealed that May (P < 0.004 for all comparisons) and June (P < 0.001 for all comparisons) differed significantly from all other months but not from each other (P = 0.239). The average number of D. antillarum settlers was 8.6 ± 3.2 streamer-1 in May, 14.9 ± 10.8 streamer-1 in June, and decreased to approximately 2–3 settlers per streamer for the remainder of the study. No significant differences were found between the other months.
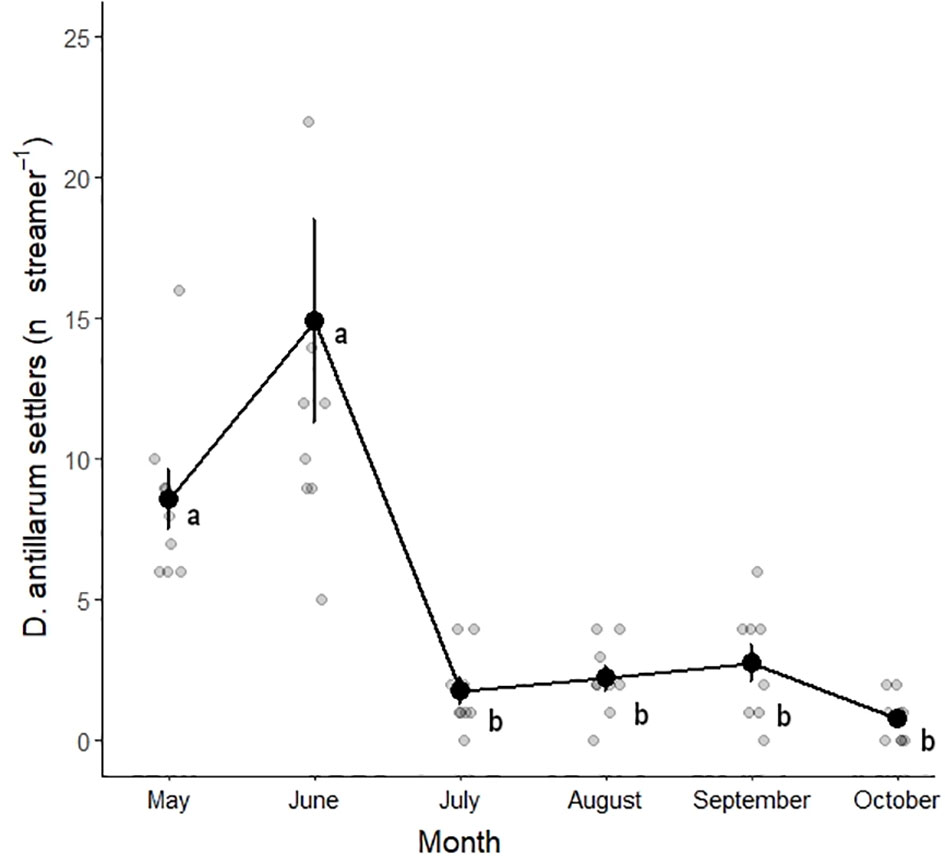
Figure 4 D. antillarum settlers per streamer per month. The line connects averages (± SE) and gray points represent the replicate streamers. Averages sharing the same letter were not significantly different. A single result of 42 D. antillarum documented from a streamer in June falls outside the plotted y-axis limits but was used to calculate average (± SE).
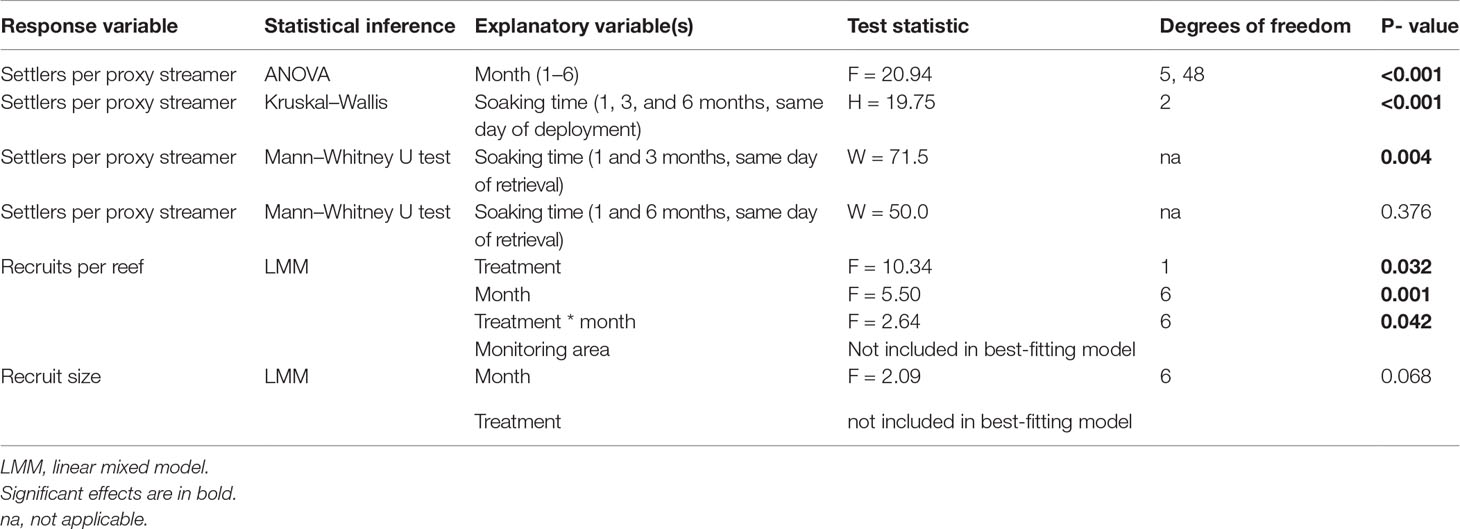
Table 3 Statistical inference, explanatory variables, test statistics, degrees of freedom, and P-values per response variable.
Apart from the proxy streamers that were replaced monthly, 18 additional streamers were deployed at the start of the experiment, of which nine were retrieved after 3 months and nine were retrieved after 6 months. Soaking time had a significant effect on the number of settlers (P < 0.001, Table 3). Streamers with a soaking time of 1 month, retrieved in June 2021, had 8.6 ± 3.2 D. antillarum. This decreased significantly to 0.2 ± 0.4 after 3 months, collected in August 2021, and to 0.4 ± 0.7 after 6 months, collected at the end of October 2021 (P < 0.001 for both comparisons). Streamers retrieved after 3 and 6 months did not differ significantly in their number of D. antillarum settlers.
Settlement on streamers with a soaking time of 3 and 6 months was also compared with streamers that were collected on the same day but had a soaking time of only 1 month. In July 2021, streamers with a soaking time of 1 month had 1.8 ± 1.4 D. antillarum settlers per streamer, which was significantly higher than the 0.2 ± 0.4 per streamer with a soaking time of 3 months (P = 0.004, Table 3). In October 2021, streamers with a soaking time of 1 month had 0.8 ± 0.8 D. antillarum settlers per streamer, which was not significantly different to streamers with a soaking time of 6 months, which had 0.4 ± 0.7 D. antillarum settlers per streamer.
Recruitment on the Patch Reefs
D. antillarum recruit abundance on the patch reefs (Figure 5) was significantly affected by treatment (streamers vs. control, P = 0.032, Table 3), month (P = 0.001, Table 3), and their interactive effect (P = 0.042, Table 3). Monitoring area had no significant effect on the D. antillarum recruit abundance as was not included in the best-fitting model. At the start of the experiment, reefs with and without streamers both had zero D. antillarum. The average D. antillarum recruit abundance increased on all six patch reefs, independent of treatment, and remained similar till month four, when reefs with streamers had an average of 4.3 ± 3.2 D. antillarum per reef and control reefs had an average of 2.7 ± 2.1 D. antillarum per reef. In month five, the average D. antillarum density at the treatment reefs was, for the first time, significantly higher than at the control reefs (P = 0.007). One month later, at the end of the experiment, this effect had strengthened: reefs with streamers had on average 6.3 ± 5.9 D. antillarum recruits, which was more than 20 times higher than control reefs without streamers, which had an average of 0.3 ± 0.6 D. antillarum recruits per reef. This difference was highly significant (P = 0.001).
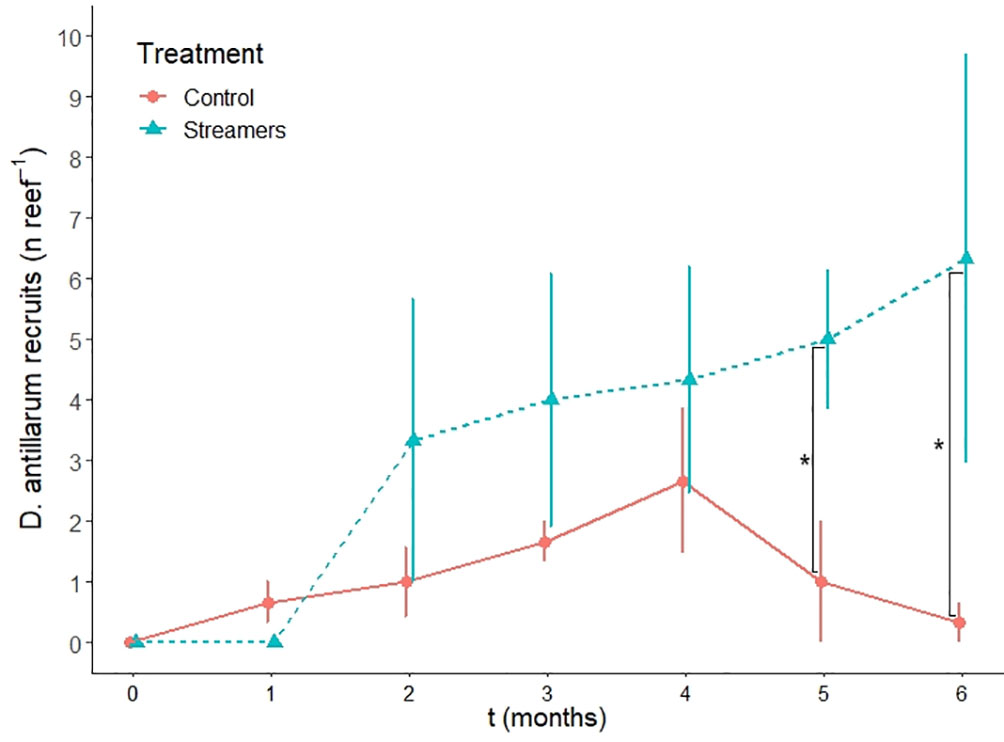
Figure 5 D. antillarum density on treatment (with streamers) and control (without streamers) reefs as a function of time. Lines connect averages ± SE and * indicates a significant difference in treatments for that month.
Size Distribution on the Patch Reefs
Figure 6 shows the D. antillarum recruit size distribution per monitoring month (July–October). The average D. antillarum recruit size was not affected by the monitoring month (P = 0.068, Table 3) or treatment (not included in the best-fitting model). In July, the average D. antillarum recruit size was 10.3 ± 3.5 mm, which corresponded with settlement in April. In August, recruits had a similar average size (11.8 ± 4.0 mm) and their size distribution corresponded most closely with settlement in May. In September, the average recruit size was 13.4 ± 5.1 mm. Half of the recruits were approximately 20 mm; these where likely the remaining recruits from the cohort settling in May or possibly April. The other half of the recruits were smaller and had probably settled in June or July. In October, the average recruit size was 10.7 ± 4.5 mm and most of the recruits corresponded in size most closely to the predicted size range for settlement in July or even August.
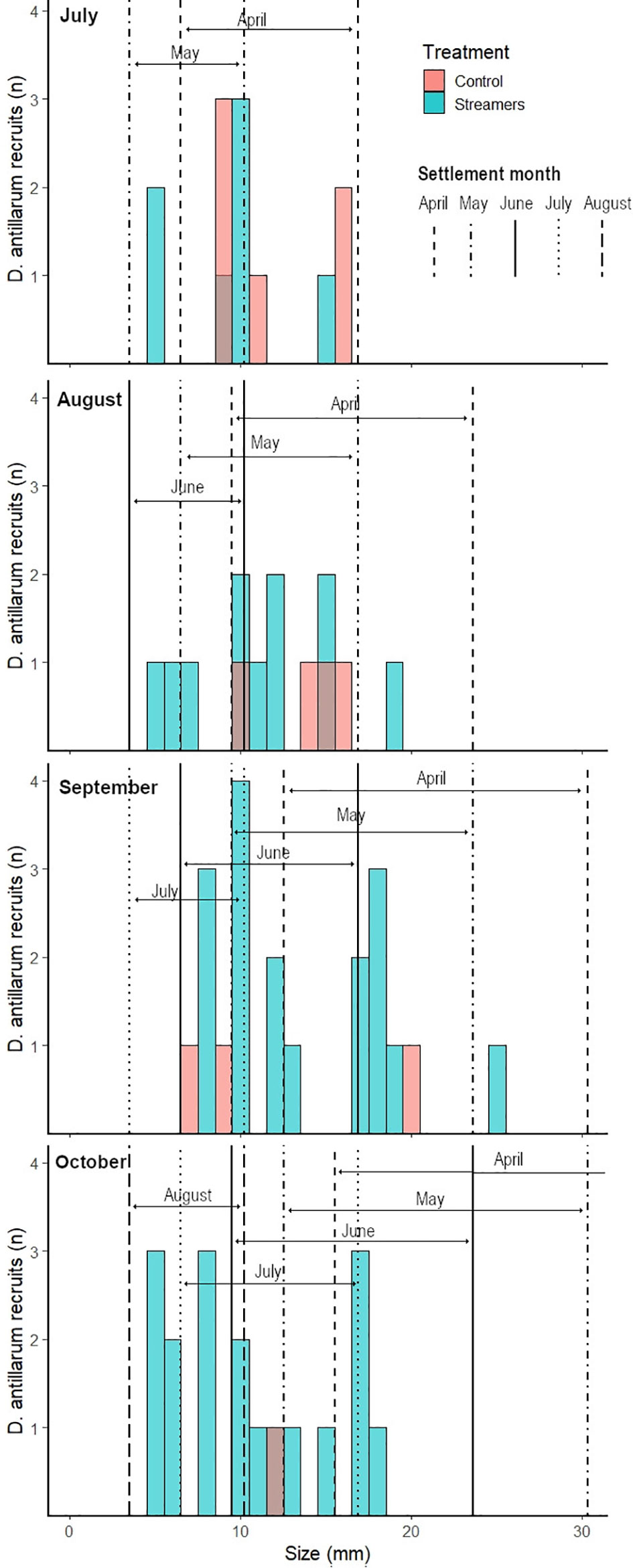
Figure 6 D. antillarum recruit size distribution on control and treatment reefs per monitoring month. The area between vertical lines of the same type (dashed, solid, dotted, etc.) indicate the estimated size range per settlement cohort, based on growth rates from Idrisi et al. (2003) and Randall et al (1964).
Discussion
Settlement on the proxy streamers revealed that the experiment was conducted at the right time of the year and very likely had been started right before the yearly settlement peak. Settlement on the proxy streamers was highest in May and June and was significantly lower during subsequent months. Settlement on the neighboring island of St. Eustatius in 2019 was also highest in May and June (Hylkema et al., 2022). In La Parguera, Puerto tRico, D. antillarum settlement was highest in July in 2006 (Williams et al., 2010) and in September in 2008 (Williams et al., 2011). The highest average D. antillarum settlement in this study was 14.9 ± 10.8 D. antillarum streamer-1 month-1. The planar surface area of a streamer with 30 bio balls was 0.04 m2, resulting in a settlement density of 372 ± 270 D. antillarum m-2 month-1. This density is in the same order of magnitude as the settlement on collectors documented for most of the locations studied around St. Eustatius in 2019 (Hylkema et al., 2022), as well as compared to the location with highest settlement rates near Puerto Rico (Williams et al., 2010; Williams et al., 2011). These results indicate that the bio ball streamers were able to provide a suitable settlement substrate for D. antillarum larvae and thereby likely able to help circumvent one of the key potential barriers to natural recovery (Rogers and Lorenzen, 2008).
The proxy streamers with a soaking time of 3 and 6 months (collected in August and November) contained significantly less settlers compared to proxy streamers with a soaking time of 1 month (collected in June), indicating that D. antillarum settlers remained on the streamers for less than 3 months. D. antillarum settlers have a test diameter of ~0.5 mm (Eckert, 1998) and grow 3–7 mm month-1 (Randall et al., 1964; Idrisi et al., 2003). The space in between the bio ball layers is 5 mm, meaning that settlers outgrew their shelter and probably moved to the outside of the bio balls 1–2 months after settlement. They likely migrated to the reef on their own accord or may have been flushed off the bio balls by currents or were eaten by predators. Proxy streamers with a soaking time of 3 months had significantly less settlers compared to streamers with a soaking time of 1 month that were collected on the same day. Bak (1985) observed that D. antillarum settlers appear to have a preference for relatively clean surfaces with a fresh biofilm. For other sea urchins, the biofilm age has also been found to be important in determining settlement (Pearce & Scheibling, 1991; Rahim et al., 2004). We observed abundant turf and macroalgae growth on the 3- and 6-month old bio balls, which probably made the bio balls less suitable for settlement. In practice, this means that streamers older than 2 months attract less new D. antillarum settlers.
Monthly monitoring revealed an increase of D. antillarum recruits on both control and streamer reefs during the course of the experiment. However, this increase was largest at the treatment reefs, where recruit densities continued to increase till the end of the experiment. In contrast, recruit densities on the control reefs started to decrease halfway through the experiment, after 3 months. This resulted in a significantly higher D. antillarum recruit abundance on reefs with streamers as opposed to reefs without streamers. The highest number of D. antillarum recruits counted on the monitored surface of a single treatment reef was 13 individuals. This is an order of magnitude lower than what would have been expected based on the total settlement on the nine streamers with each ~15 D. antillarum settlers in the month with the highest settlement. The latter would have resulted in 135 settlers in a single month if all settlers had remained on the reef. During monitoring, we sometimes observed substantial currents at the research location, which might have washed juveniles off the streamers, off the monitored area, and possibly even away from the experimental reefs. As control and treatment reefs were located close to each other, strong currents could even be responsible for depositing some of D. antillarum juveniles on the control reefs. However, the reefs just outside the transects were inspected at the end of the experiment and only few D. antillarum recruits were observed there. It is therefore likely that, next to dislocation by currents, other processes reduced the survival of settlers that outgrew the bio balls and colonized the reefs. Macroalgae, which covered large areas of the reefs during the experiment, are known to harbor large numbers of micropredators such as crabs, shrimps, and worms (Bechtel et al., 2006), which could be responsible for high post-settlement mortality. In addition, the dense turf and macroalgae cover of the patch reefs might be a suboptimal habitat for settlers in terms of food (Vermeij et al., 2010), while it might also reduce shelter availability by filling small crevices (Spadaro & Butler, 2021).
Although new recruits were observed on the treatment reefs every month, the total number of recruits barely increased, indicating a low long-term retention of the recruits. This is confirmed by the average D. antillarum test size, which did not increase during the course of the experiment. Size distributions showed that very few recruits made it past a test size of 20 mm and every month from the period of July to October, most recruits were derived from a new cohort of settlers. The low retention of D. antillarum recruits could be attributed to migration to other parts of the reef or to predation. Monitored recruits mostly showed a high shelter fidelity and were often seen in the same crevice during multiple monitoring events. They were increasing in size, appeared healthy, were not yet outgrowing their shelter space and then suddenly disappeared, suggesting that predation rather than migration was the most likely factor explaining the limited long-term increase in D. antillarum recruit density. Among many potential predators, queen triggerfish (Balistes vetula), Spanish hogfish (Bodianus rufus), pudding wife (Halichoeres radiatus), black margate (Anisotremus surinamensis), Spanish grunt (Haemulon macrostomum), and jolthead porgy (Calamus bajonado) are especially known to prey upon D. antillarum (Randall et al., 1964). All these species, with the exception of H. macrostomum, were frequently observed on the experimental site and Harborne et al. (2009) suggested that even a relatively low predation pressure can be sufficient to prevent D. antillarum recovery. It could therefore well be that the predation pressure at the experimental site was too high or the shelter availability too low (Bodmer et al., 2021) to support D. antillarum population recovery, notwithstanding increased settlement due to the streamers.
Figure 7 summarizes the life cycle of D. antillarum and the possible factors constraining its natural recovery. Hylkema et al. (2022) demonstrated high peak settlement rates on Saba at certain times of the year, indicating that limited fertilization of gametes (Feehan et al., 2016) and the lack of upstream adult populations were not the main factors constraining natural recovery. The addition of bio ball streamers in the current study resulted in increased D. antillarum recruitment, confirming that the availability of suitable settlement substrate was limited on the natural reef (Rogers and Lorenzen, 2008). This can be explained by the high turf and macroalgae cover, limiting the amount of fresh biofilm on the patch reefs. The algae-dominated habitat might also have reduced post-settlement survival, as the algae fill potential shelter spaces (Spadaro & Butler, 2021), do not provide the right food for D. antillarum settlers (Vermeij et al., 2010), and harbor high densities of micropredators (Bechtel et al., 2006). Finally, the high predation of larger juveniles might have reduced post-recruitment survival and prevented D. antillarum population recovery.
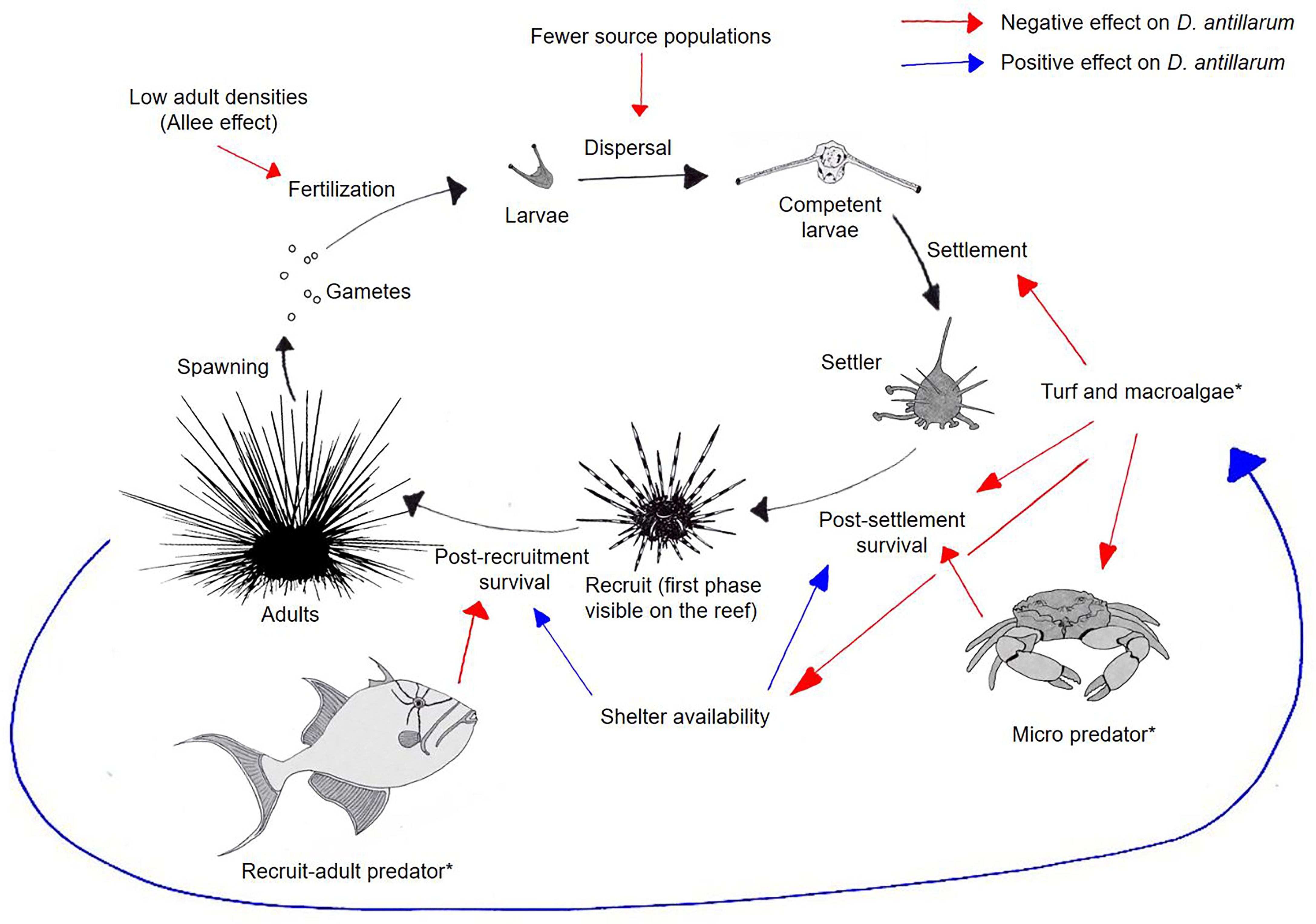
Figure 7 Life cycle of D. antillarum (black arrows) and factors constraining (red arrows) or enhancing (blue arrows) D. antillarum population development. Factors with an asterisk (*) likely affected D. antillarum recruitment in the present study.
We conclude that our new approach of assisted natural recovery (ANR) successfully provided a suitable settlement substrate and significantly increased D. antillarum recruit densities on patch reefs. However, before ANR can be implemented on a large scale, post-settlement and post-recruitment survival should be improved. To optimize prospects for ANR, we propose to: 1) employ bio balls or other substrates that can provide shelter suitable to larger juveniles, 2) optimize the habitat by removing macroalgae, 3) choose sites with a lower predation pressure, and 4) place corrals around the streamers. Larger bio balls that, in addition to the current 5-mm shelters, also provide larger shelters might be able to maintain D. antillarum juveniles safe in mid-water during the intermediate phase between settlement and their ultimate descent to the larger shelter spaces of the reef, thereby improving post-settlement survival. By removing macroalgae from the reefs at the start of the settlement season, the habitat might become more suitable for settlers and less suitable for micropredators, also increasing post-settlement survival. Macroalgae could be removed manually or by restocking adult D. antillarum or other herbivores such as reef urchins (Echinometra viridis) (Bechtel et al., 2006) or the Caribbean king crab (Maguimithrax spinosissimus) (Spadaro & Butler, 2021). To select restoration sites with a low predation pressure, it might, paradoxically, be recommended to start ANR outside marine reserves where fishing pressure can reduce predator densities (Edmunds & Carpenter, 2001; Harborne et al., 2009). Alternatively, shallower reefs (Carpenter & Edmunds, 2006; Debrot & Nagelkerken, 2006; Myhre & Acevedo-Gutiérrez, 2007) or reefs with a high shelter availability (Bodmer et al., 2021) might increase post-recruitment survival and increase the chance of successful D. antillarum recovery. Finally, the post-recruitment survival of D. antillarum on locations with a high predation pressure might be enhanced using corrals placed around the streamers, which can protect the juveniles during their first months on the reef (Williams, 2017; Williams, 2022). The combination of these measures could result in a comprehensive ANR approach. If D. antillarum settlement densities are not significantly reduced by the D. antillarum die-off taking place in parts of the Caribbean in 2022 (AGRRA, 2022), we expect ANR to be able to contribute substantially to D. antillarum population recovery in the near future.
Data Availability Statement
The raw data supporting the conclusions of this article will be made available by the authors, without undue reservation.
Author Contributions
AH: Conceptualization, Methodology, Writing original draft, Formal analysis, Writing - Review & Editing, Supervision AD: Conceptualization, Writing - Review & Editing EP: Investigation RO: Writing - Review & Editing AM: Writing - Review & Editing. All authors contributed to the article and approved the submitted version.
Funding
We declare that all sources of funding received are submitted. This research was conducted in the context of the RAAK PRO Diadema project (project# RAAK.PRO03.005), partly funded by SIA, part of the Dutch Research Council (NWO). The contributions by A.O. (Dolfi) Debrot were funded by Wageningen Marine Research through project 4311500013 R&D Wetenschapsplan.
Conflict of Interest
The authors declare that the research was conducted in the absence of any commercial or financial relationships that could be construed as a potential conflict of interest.
Publisher’s Note
All claims expressed in this article are solely those of the authors and do not necessarily represent those of their affiliated organizations, or those of the publisher, the editors and the reviewers. Any product that may be evaluated in this article, or claim that may be made by its manufacturer, is not guaranteed or endorsed by the publisher.
Acknowledgments
We thank Alex van der Last, Tom Wijers, Michelle Mulken, Fedor den Elzen, Djan Mattijssen, Jan-Luca Mack, and Jasper Bleijenberg for assisting with the recruit counts and the analysis of the proxy streamers. We also thank the SCF, SBMU, and Sea Saba staff, in particular Walter Hynds, Marijn van der Laan, and Tom Brokke, for providing fieldwork assistance and Callum Reid for making the map. We want to thank the two reviewers for their feedback and suggestions on an earlier version of this manuscript.
References
Adam T. C., Burkepile D. E., Ruttenberg B. I., Paddack M. J. (2015). Herbivory and the Resilience of Caribbean Coral Reefs: Knowledge Gaps and Implications for Management. Mar. Ecol. Prog. Ser. 520, 1–20. doi: 10.3354/meps11170
AGRRA (2022). Diadema Response Network – Map of Diadema and Other Sea Urchins in the Caribbean (ArcGIS Online), 31–5-2022. Available at: www.agrra.org.
Arnold S. N., Steneck R. S., Mumby P. J. (2010). Running the Gauntlet: Inhibitory Effects of Algal Turfs on the Processes of Coral Recruitment. Mar. Ecol. Prog. Ser. 414, 91–105. doi: 10.3354/meps08724
Aronson R. B, Precht W. F. (2001). White-Band Disease and the Changing Face of Caribbean Coral Reefs. Ecol. etiology newly emerging Mar. Dis., 25–38. doi: 10.1007/978-94-017-3284-0_2
Bak R. P. M. (1985). “Recruitment Patterns and Mass Mortalities in the Sea Urchin Diadema Antillarum,” in Proc. 5th Int. Coral Reef Congress, Vol. 5. 267–272.
Bates D., Mächler M., Bolker B., Walker S.2015 Fitting Linear Mixed-Effects Models Using lme4. Journal of Statistical Software, 67(1), 1–48. doi: 10.18637/jss.v067.i01
Bechtel J. D., Gayle P., Kaufman L. (2006). “The Return of Diadema Antillarum to Discovery Bay: Patterns of Distribution and Abundance,” in Proceedings of 10th International Coral Reef Symposium. 367–375.
Bielmyer G. K., Brix K. V., Capo T. R., Grosell M. (2005). The Effects of Metals on Embryo-Larval and Adult Life Stages of the Sea Urchin, Diadema Antillarum. Aquat. Toxicol. 74 (3), 254–263. doi: 10.1016/j.aquatox.2005.05.016
Bodmer M. D. V., Wheeler P. M., Anand P., Cameron S. E., Hintikka S., Cai W., et al. (2021). The Ecological Importance of Habitat Complexity to the Caribbean Coral Reef Herbivore Diadema Antillarum: Three Lines of Evidence. Sci. Rep. 11 (1), 1–13. doi: 10.1038/s41598-021-87232-9
Box S. J., Mumby P. J. (2007). Effect of Macroalgal Competition on Growth and Survival of Juvenile Caribbean Corals. Mar. Ecol. Prog. Ser. 342, 139–149. doi: 10.3354/meps342139
Burdick D. R. (2008). “The Effectiveness of Macroalgal Reduction and Diadema Antillarum Addition in Limiting Macroalgal Growth and Facilitating Coral Recovery,” in Proceedings of the 11th International Coral Reef Symposium, Florida: Ft Lauderdale, Vol. Vol. 24. 1204–1208.
Carpenter R. C. (1988). Mass Mortality of a Caribbean Sea Urchin: Immediate Effects on Community Metabolism and Other Herbivores. Proc. Natl. Acad. Sci. 85 (2), 511–514. doi: 10.1073/pnas.85.2.511
Carpenter R. C., Edmunds P. J. (2006). Local and Regional Scale Recovery of Diadema Promotes Recruitment of Scleractinian Corals. Ecol. Lett. 9 (3), 271–280. doi: 10.1111/j.1461-0248.2005.00866.x
Chiappone M., Rutten L. M., Miller S. L., Swanson D. W. (2013). Recent Trends, (1999–2011) in Population Density and Size of the Echinoid Diadema Antillarum in the Florida Keys. Florida Scientist, 76, 23–35.
Coiffait-Gombault C., Buisson E., Dutoit T. (2011). Hay Transfer Promotes Establishment of Mediterranean Steppe Vegetation on Soil Disturbed by Pipeline Construction. Restor. Ecol. 19 (201), 214–222. doi: 10.1111/j.1526-100X.2010.00706.x
Dame E. A. (2008). Assessing the Effect of Artificial Habitat Structure on Translocation of the Long-Spined Sea Urchin, Diadema Antillarum, in Curacao (Netherlands Antilles). Bull. Mar. Sci. 82 (2), 247–254.
Debrot A. O., Nagelkerken I. (2006). Recovery of the Long-Spined Sea Urchin Diadema Antillarum in Curacao (Netherlands Antilles) Linked to Lagoonal and Wave Sheltered Shallow Rocky Habitats. Bull. Mar. Sci. 79, 415–424.
Eckert G. L. (1998). Larval Development, Growth and Morphology of the Sea Urchin Diadema Antillarum. Bull. Mar. Sci. 63 (2), 443–451.
Edmunds P. J., Carpenter R. C. (2001). Recovery of Diadema Antillarum Reduces Macroalgal Cover and Increases Abundance of Juvenile Corals on a Caribbean Reef. Proc. Natl. Acad. Sci. 98 (9), 5067–5071. doi: 10.1073/pnas.071524598
Feehan C. J., Brown M. S., Sharp W. C., Lauzon-Guay J. S., Adams D. K. (2016). Fertilization Limitation of Diadema Antillarum on Coral Reefs in the Florida Keys. Ecology 97 (8), 1897–1904. doi: 10.1002/ecy.1461
Gladfelter W. B. (1982). White-Band Disease in Acropora Palmata: Implications for the Structure and Growth of Shallow Reefs. Bull. Mar. Sci. 32 (2), 639–643.
Harborne A. R., Renaud P. G., Tyler E. H. M., Mumby P. J. (2009). Reduced Density of the Herbivorous Urchin Diadema Antillarum Inside a Caribbean Marine Reserve Linked to Increased Predation Pressure by Fishes. Coral Reefs 28 (3), 783–791. doi: 10.1007/s00338-009-0516-6
Hardwick K., Healey J. R., Elliott S., Blakesley D. (2004). Research Needs for Restoring Seasonal Tropical Forests in Thailand: Accelerated Natural Regeneration. New Forests 27 (3), 285–302. doi: 10.1023/B:NEFO.0000022228.08887.d2
Hylkema A., Debrot A. O., Pistor M., Postma E., Williams S. M., Kitson-Walters K. (2022). High Peak Settlement of Diadema Antillarum on Different Artificial Collectors in the Eastern Caribbean. Journal of Experimental Marine Biology and Ecology 549, 151693. doi: 10.1016/j.jembe.2022.151693
Idjadi J. A., Haring R. N., Precht W. F. (2010). Recovery of the Sea Urchin Diadema Antillarum Promotes Scleractinian Coral Growth and Survivorship on Shallow Jamaican Reefs. Mar. Ecol. Prog. Ser. 403, 91–100. doi: 10.3354/meps08463
Idrisi N., Capo T. R., Serafy J. E. (2003). Postmetamorphic Growth and Metabolism of Long-Spined Black Sea Urchin (Diadema Antillarum) Reared in the Laboratory. Mar. Freshw. Behav. Physiol. 36 (2), 87–95. doi: 10.1080/1023624031000140003
Jackson J. B. C., Donovan M. K., Cramer K. L., Lam V. V. (2014). “Status and Trends of Caribbean Coral Reefs,” in Global Coral Reef Monitoring Network (Gland, Switzerland: IUCN), 1970–2012. doi: 10.1126/science.1059199
Jackson J. B., Kirby M. X., Berger W. H., Bjorndal K. A., Botsford L. W., Bourque B. J., et al. (2001). Historical Overfishing and the Recent Collapse of Coastal Ecosystems. science 293 (5530), 629–637.
Jompa J., McCook L. J. (2002). The Effects of Nutrients and Herbivory on Competition Between a Hard Coral (Porites Cylindrica) and a Brown Alga (Lobophora Variegata). Limnology Oceanography 47 (2), 527–534. doi: 10.4319/lo.2002.47.2.0527
Klokman O., Hylkema A. Settlement densities of D. antillarum around Saba, Dutch Caribbean. Manuscript in preparation.
Lenth R., Herve M. (2019). “Emmeans: Estimated Marginal Means, Aka Least-Square Means,” in R Package Version 1.1, vol. 2. .
Lessios H. A. (1988). Population Dynamics of Diadema Antillarum (Echinodermata: Echinoidea) Following Mass Mortality in Panama. Mar. Biol. 99 (4), 515–526.
Lessios H. A. (2005). Diadema Antillarum Populations in Panama Twenty Years Following Mass Mortality. Coral Reefs 24 (1), 125–127. doi: 10.1007/s00338-004-0443-5
Lessios H. A. (2016). The Great Diadema Antillarum Die-Off: 30 Years Later. Annu. Rev. Mar. Sci. 8, 267–283. doi: 10.1146/annurev-marine-122414-033857
Lessios H. A., Robertson D. R., Cubit J. D. (1984). Spread of Diadema Mass Mortality Through the Caribbean. Science 226 (4672), 335–337. doi: 10.1126/science.226.4672.335
Maciá S., Robinson M. P., Nalevanko A. (2007). Experimental Dispersal of Recovering Diadema Antillarum Increases Grazing Intensity and Reduces Macroalgal Abundance on a Coral Reef. Mar. Ecol. Prog. Ser. 348, 173–182. doi: 10.3354/meps06962
Mackenzie D. D., Naeth M. A. (2010). The Role of the Forest Soil Propagule Bank in Assisted Natural Recovery After Oil Sands Mining. Restor. Ecol. 18 (4), 418–427. doi: 10.1111/j.1526-100X.2008.00500.x
Maldonado-Sánchez J., Mariño-Tapia I., Teresa Herrera-Dorantes M., Ardisson P. L. (2019). Hydrodynamic Conditions That Favor the Settlement of Diadema Antillarum to a Western Caribbean Coral Reef. Bull. Mar. Sci. 95 (2), 251–264. doi: 10.5343/bms.2018.0001
McCook L., Jompa J., Diaz-Pulido G. (2001). Competition Between Corals and Algae on Coral Reefs: A Review of Evidence and Mechanisms. Coral reefs 19 (4), 400–417. doi: 10.1007/s003380000129
Miller M. W., Valdivia A., Kramer K. L., Mason B., Williams D. E., Johnston L. (2009). Alternate Benthic Assemblages on Reef Restoration Structures and Cascading Effects on Coral Settlement. Marine Ecology Progress Series 387, 147–156. doi: 10.3354/meps08097
Myhre S., Acevedo-Gutiérrez A. (2007). Recovery of Sea Urchin Diadema Antillarum Populations is Correlated to Increased Coral and Reduced Macroalgal Cover. Mar. Ecol. Prog. Ser. 329, 205–210. doi: 10.3354/meps329205
Pandolfi J. M., Bradbury R. H., Sala E., Hughes T. P., Bjorndal K. A., Cooke R. G., et al. (2003). Global Trajectories of the Long-Term Decline of Coral Reef Ecosystems. Science 301 (5635), 955–958. doi: 10.1126/science.1085706
Pearce C. M., Scheibling R. E. (1991). Effect of Macroalgae, Microbial Films, and Conspecifics on the Induction of Metamorphosis of the Green Sea Urchin Strongylocentrotus Droebachiensis (Mu¨ Ller). J. Exp. Mar. Biol. Ecol. 147 (2), 147–162. doi: 10.1016/0022-0981(91)90179-Z
Pilnick A. R., O’Neil K. L., Moe M., Patterson J. T. (2021). A Novel System for Intensive Diadema Antillarum Propagation as a Step Towards Population Enhancement. Sci. Rep. 11 (1), 1–13. doi: 10.1038/s41598-021-90564-1
Polunin N. V. C., Roberts C. M. (1993). Greater Biomass and Value of Target Coral-Reef Fishes in Two Small Caribbean Marine Reserves. Mar. Ecology-Progress Ser. 100, 167–167. doi: 10.3354/meps100167
Precht W. F., Aronson R. B., Gardner T. A., Gill J. A., Hawkins J. P., Hernández-Delgado E. A., et al. (2020). The Timing and Causality of Ecological Shifts on Caribbean Reefs. Adv. Mar. Biol. 87 (1), 331–360. doi: 10.1016/bs.amb.2020.08.008
Rahim S. A. K. A., Li J. Y., Kitamura H. (2004). Larval Metamorphosis of the Sea Urchins, Pseudocentrotus Depressus and Anthocidaris Crassispina in Response to Microbial Films. Mar. Biol. 144 (1), 71–78. doi: 10.1007/s00227-003-1171-z
Randall J. E., Schroeder R. E., Starck W. A. (1964). Notes on the Biology of the Echinoid Diadema Antillarum. Caribbean J. Sci. 4 (2-3), 421–433.
R Core Team (2021). R: A Language and Environment for Statistical Computing (Vienna, Austria: R Foundation for Statistical Computing). Available at: https://www.R-project.org/.
Roberts C. M. (1997). Connectivity and Management of Caribbean Coral Reefs. Science 278 (5342), 1454–1457. doi: 10.1126/science.278.5342.1454
Rogers A., Lorenzen K. (2008). “Recovery of Diadema Antillarum and the Potential for Active Rebuilding Measures: Modeling Population Dynamics,” in Proc. 11st Int. Coral Reef Symposium, Ft. Lauderdale, Florida. 956–960.
Shono K., Cadaweng E. A., Durst P. B. (2007). Application of Assisted Natural Regeneration to Restore Degraded Tropical Forestlands. Restor. Ecol. 15 (4), 620–626. doi: 10.1111/j.1526-100X.2007.00274.x
Spadaro A. J., Butler M. J. IV (2021). Herbivorous Crabs Reverse the Seaweed Dilemma on Coral Reefs. Curr. Biol. 31 (4), 853–859. doi: 10.1016/j.cub.2020.10.097
Vermeij M. J., Debrot A. O., van der Hal N., Bakker J., Bak R. P. (2010). Increased Recruitment Rates Indicate Recovering Populations of the Sea Urchin Diadema Antillarum on Curaçao. Bull. Mar. Sci. 86 (3), 719–725.
Wijers T., Hylkema A., Pilnick A. R., Murk A. J., Patterson J. T. Feeding density, larval density and temperature effect on Diadema antillarum larvae survival and growth. Manuscript in preparation.
Williams S. M. (2017). A Novel Approach to the Restoration of Diadema Antillarum on Coral Reefs in the Caribbean. Reef Encounters 31, 48–50.
Williams S. M. (2022). The Reduction of Harmful Algae on Caribbean Coral Reefs Through the Reintroduction of a Keystone Herbivore, the Long Spined Sea Urchin, Diadema Antillarum. Restor. Ecol., 30, e13475. doi: 10.1111/rec.13475
Williams S. M., García-Sais J. R., Yoshioka P. M. (2011). Spatial Variation of Diadema Antillarum Settlement in La Parguera, Puerto Rico. Bull. Mar. Sci. 87 (3), 531–540. doi: 10.5343/bms.2010.1041
Williams S. M., Yoshioka P. M., Sais J. G. (2010). Recruitment Pattern of Diadema Antillarum in La Parguera, Puerto Rico. Coral Reefs 29 (3), 809–812. doi: 10.1007/s00338-010-0633-2
Keywords: accelerated natural recovery, Saba, coral reef, sea urchin, Caribbean
Citation: Hylkema A, Debrot AO, van de Pas EE, Osinga R and Murk AJ (2022) Assisted Natural Recovery: A Novel Approach to Enhance Diadema antillarum Recruitment . Front. Mar. Sci. 9:929355. doi: 10.3389/fmars.2022.929355
Received: 26 April 2022; Accepted: 07 June 2022;
Published: 15 July 2022.
Edited by:
Raúl A. González-Pech, The Pennsylvania State University (PSU), United StatesReviewed by:
Edson A. Vieira, Federal University of Rio Grande do Norte, BrazilKelly Gómez-Campo, The Pennsylvania State University (PSU), United States
Copyright © 2022 Hylkema, Debrot, van de Pas, Osinga and Murk. This is an open-access article distributed under the terms of the Creative Commons Attribution License (CC BY). The use, distribution or reproduction in other forums is permitted, provided the original author(s) and the copyright owner(s) are credited and that the original publication in this journal is cited, in accordance with accepted academic practice. No use, distribution or reproduction is permitted which does not comply with these terms.
*Correspondence: Alwin Hylkema, alwin.hylkema@hvhl.nl