- 1Key Laboratory of Aquacultural Biotechnology Ministry of Education, Ningbo University, Ningbo, China
- 2Collaborative Innovation Center for Zhejiang Marine High-Efficiency and Healthy Aquaculture, Ningbo University, Ningbo, China
- 3Institute of Semiconductors, Chinese Academy of Sciences, Beijing, China
- 4Marine Economic Research Center, Dong Hai Strategic Research Institute, Ningbo University, Ningbo University, Ningbo, China
An eight-week experiment was conducted to evaluate the effects of eight light intensities (0, 0.83, 1.61, 8.17, 15.89, 31.38, 63.69, and 124.31 μmol m-2 s-1) on the survival, growth, antioxidant, molting-related and apoptosis-related gene expression of juvenile swimming crab Portunus trituberculatus. The results showed that the survival rate of crabs was the highest under the light intensities of 8.17 and 15.89 μmol m-2 s-1. Under this light intensity, the crabs had the lowest hemolymph glucose (GLU) but the highest triglyceride (TG) content. The crabs in these treatments also had the highest total antioxidant capacity (T-AOC), superoxide dismutase (SOD), catalase (CAT) activities, and the lowest malondialdehyde (MDA) content. The growth performance (including weight gain and specific growth rate) and molting frequency of crabs increased with increasing light intensity. The improved growth performance and molting of 8.17 and 15.89 μmol m-2 s-1 groups were also accompanied by up-regulated of the retinoid-X receptor (rxr), ecdysone receptor (ecr), nuclear receptor E75 (e75) gene expression, and down-regulated molt-inhibiting hormone (mih) gene expression. In addition, suboptimal light intensity (0, 0.83, 63.69, and 124.31 μmol m-2 s-1) significantly up-regulated the expression of apoptosis-related genes including c-Jun N-terminal kinase (jnk), tumor suppressor p53 (p53), and B-cell lymphoma-2 (bcl-2). Taken together, the suitable light intensity for the juvenile P. trituberculatus was estimated to be 9.96 μmol m-2 s-1.
Introduction
The swimming crab Portunus trituberculatus is an essential economic species naturally distributed from Southeast Asia to the Indian Ocean (Dai et al., 1986). In 2019, the global captured P. trituberculatus reached 460,580 tons, making it the highest fishing yield of crustaceans in the world (FAO, 2021). China contributes more than 95% of the capture yield of P. trituberculatus (Liu et al., 2013), and this proportion is increasing year by year. However, with the decline of fishery resources and strict supervision of the government departments, the captured output of P. trituberculatus has dropped from 542,337 tons in 2015 to 424,630 tons in 2020 (Fisheries Bureau of Agriculture Ministry of China, 2016-2021). However, the decline in capture did not promote the aquaculture production of P. trituberculatus for the seed of swimming crab is mainly produced in earth-pond (Wang et al., 2019), of which the yield is low because of the uncontrollable environment, creating a gap between seed supplies and farmer’s demand. As a result, indoor seedling production has been tried in recent years.
Researchers have shown that indoor nurseries could improve the survival, growth, and reproduction of the crabs by manipulating various environmental factors, including light (Li et al., 2020a; Li et al., 2020b; Chen et al., 2021; Dou et al., 2021), tank color (Shi et al., 2019; Wang et al., 2019), tank bottom area (Yu et al., 2022), water temperature (Yuan et al., 2017; Syafaat et al., 2020), and salinity (Nurdiani and Zeng, 2007; Shentu et al., 2015). Light is a compound environmental factor including three characteristics: light spectrum, light intensity, and photoperiod (Boeuf and Le Bail, 1999). As an important abiotic factor, the light intensity has been reported to affect crustacean survival (Coyle et al., 2011; Dou et al., 2021), molting (Chen et al., 2021), growth (Moss et al., 1999; Hoang et al., 2003; Li et al., 2011; Li et al., 2020a), behavior (Gardner and Maguire, 1998; Haché et al., 2015) and reproduction (Hoang et al., 2002a; Hoang et al., 2002b). Aquatic animals are sensitive to changes in light intensity, and the optimal light intensity varies significantly among species and developmental stages (Villamizar et al., 2011; Ruchin, 2021). In fish, a recent review by Ruchin (2021) summarized the effect of light intensities from 0 - 27300 lx on fish development, growth, physiological and biochemical processes. There are only a few studies regarding the impact of light intensities on crabs (Li et al., 2011; Li et al., 2020a; Li et al., 2020b; Li et al., 2020c; Chen et al., 2021; Dou et al., 2021; Li et al., 2021). For the mud crab Scylla paramamosain, the suitable light intensity for juvenile crabs ranged from 11.36-18.27 W m-2 (ca. 60.55-97.38 μmol m-2 s-1) (The conversion method is based on Villamizar et al., 2011) (Chen et al., 2021). For adults, it was only 1.43 μmol m-2 s-1 (Li et al., 2020a). As to larval P. trituberculatus, the suitable light intensity was 124.31 - 195.31 μmol m-2 s-1 (Dou et al., 2021), but the light requirement for juvenile swimming crabs is still unknown.
Many studies have found that suboptimal light intensity adversely affects crustaceans, from larval development to broodstock reproduction. For the larvae and juveniles, suboptimal light intensity resulted in high mortality, poor growth, and delayed development (Gardner and Maguire, 1998; Li et al., 2011; Dou et al., 2021; Chen et al., 2021). In adults, the suboptimal light intensity could lead to premature maturation, reducing spawning and fecundity (Hoang et al., 2002a; Li et al., 2011; Li et al., 2020b). Some scholars attribute the adverse effects partly to the stress caused by suboptimal light intensity (Chen et al., 2021; Dou et al., 2021; Ruchin, 2021). Under stressful conditions, organisms will produce large amounts of reactive oxygen species (ROS), such as superoxide radical (O2•-), hydroxyl radical (•OH), and hydrogen(H2O2) (Suzuki and Mittler, 2006; Paital and Chainy, 2012). Excessive accumulation of ROS could cause oxidative damage to cells and tissues of crabs (Lin et al., 2017; Zhang et al., 2019). In response to ROS, crabs developed an efficient antioxidant system, including antioxidant enzymes represented by superoxide dismutase (SOD) and catalase (CAT) to remove excess ROS (Dong et al., 2009; Chen et al., 2021). In addition, when cells are damaged by oxidative stress, the organism will clear the damaged cells by apoptosis to maintain homeostasis (Xian et al., 2013; Chen et al., 2021). Apoptosis is a complex process regulated by multiple signals, and the process remains obscure in arthropods (including crustaceans) (Menze et al., 2010). However, some apoptosis-related proteins such as c-Jun N-terminal kinase (JNK), B-cell lymphoma-2 (Bcl-2), and tumor suppressor p53 (p53) were found to regulate apoptosis in crustaceans (Menze et al., 2010; Chen et al., 2021).
In the present study, the Light Emitting Diodes (LED) were used to create eight different light intensities to investigate the effects of light intensities on growth, molting, antioxidant capacity, and apoptosis-related gene expression in the swimming crab P. trituberculatus. This work aims to identify optimal light conditions for P. trituberculatus produced in land-based hatcheries and nurseries to boost juvenile outputs.
Material And Method
Experimental Crabs
The P. trituberculatus were purchased from commercial nurseries (Ningbo, China). First, the megalopa was accommodated in several tanks lined with woolen mesh (as a shelter to reduce cannibalism). The tanks were continuously oxygenated, and the water was changed by 50% daily. Megalopa was fed with Artemia tibetiana until they metamorphosed to 1 st crablet instar (Crablet 1, C1). The Crablets were immediately taken out and kept in a transparent square tank (17 cm × 11.5 cm × 6.8 cm) until they developed into C2. The healthy and lively juvenile crabs (initial body weight of 11.20 mg) were randomly selected for the experiment.
Experimental Design and Management
This experiment was conducted in June and July 2020 at the Intelligent Aquaculture Laboratory, School of Marine Sciences, Ningbo University (Ningbo, China). Full-spectrum LED lights (Shenzhen Yamingjie intelligent technology Co. Ltd., Shenzhen, China) were used as the light source. According to Dou et al. (2021) and Chen et al. (2021), eight light intensities, i.e., 0, 0.83, 1.61, 8.17, 15.89, 31.38, 63.69, and 124.31 μmol m-2 s-1 were used for the experiment. Light intensities were achieved by adjusting the dimmer and the distance between the LEDs and the water surface. Each light intensity treatment had four replicates, with ten crablets cultured for each replicate. Every crablet was kept individually in a transparent square tank as described in 2.1. The photoperiod during the experiment was 12L:12D (light on at 6:00 am). All the tanks were placed on the shelf with shading cloth to avoid external light pollution.
Crablets were fed with Artemia tibetiana at 8:00 am and 5:00 pm daily, with an extra meal [fed a commercial diet (Ningbo Tech-Bank Feed Co. Ltd., Ningbo, China, Crude protein ≥ 40.0, Crude lipid ≥ 6.0)] at 12:00 pm. During the experiment, the molting and dead crabs were counted every day, and the water quality parameters were: the temperature at 26 ± 1°C, salinity at 24 ± 1 psu, pH at 8.5, dissolved oxygen > 6.0 mg L-1, NH3-N < 0.06 mg L-1, NO2-N < 0.1 mg L-1. The experimental protocol of the present study was reviewed and approved by the Animal Care and Use Committee of Ningbo University.
Sample Collection
After eight weeks, all surviving crabs were starved for 24 hours, then anesthetized with ice and weighed. The hemolymph was collected from the heart with a disposable needle, and the eyestalk and hepatopancreas were separated. All samples were frozen in liquid nitrogen and stored at -80°C until analysis.
Hemolymph Index Analysis
The hemolymph glucose (Glu, A154-2-1) was determined by the hexokinase method (Deeg et al., 1980), triglyceride (TG, A110-1-1), and total cholesterol (TCHO, A111-1-1) were determined by the GPO-PAP method (Henkel and Stoltz, 1982). All the hemolymph indexes were determined by commercial kits (Nanjing Jiancheng Bioengineering Institute, Nanjing, China).
Antioxidant Index Analysis
Antioxidative capacity in the hepatopancreas was also measured using commercial kits (Nanjing Jiancheng Bioengineering Institute, Nanjing, China). Briefly, the hepatopancreas was homogenized in pre-cooled normal saline (0.9% NaCl, 4°C) at a ratio of 1:9 (W: V), then centrifuged at 2500 rpm for 10 minutes at 4°C, and the supernatant was taken for antioxidant capacity determination. Total protein concentration (TP, A045-2) of the supernatant was determined by the Coomassie Brilliant Blue method (Bradford, 1976), the total antioxidant capacity (T-AOC, A015-3-1) was determined by the FRAP method (Benzie and Strain, 1996), the concentration of malondialdehyde (MDA, A003-2-2) was measured by thiobarbituric acid reaction (Ohkawa et al., 1979), the activity of superoxide dismutase (SOD, A001-1-2) was measured by the xanthine oxidase method (Peskin and Winterbourn, 2000), catalase (CAT, A007-1-1) was tested using the hydrogen peroxide decomposition method (Góth, 1991).
Gene Expression Analysis
Total RNA in hepatopancreas and eyestalk was extracted using a commercial kit (R401-01, Vazyme, Nanjing, China) by the TRIzol method. RNA quality was checked by ultramicro-spectrophotometer (A260:A280 nm) and 1% gel electrophoresis. Then, RNA was reverse transcribed to cDNA using a commercial kit (CW2569M, CWBIO, Beijing, China).
The qPCR system (LightCycler480, Roche, USA) was used to analyze the relative expression level of target genes. A total 20 μl reaction system (2 × MagicSYBR Mixture,10 μl; cDNA,1 μl; each primer (10 μM), 0.4 μl; ddH2O, 8.2 μl) was prepared using a commercial kit (CW3008H, CWBIO, Beijing, China). The specific primers used in this study are shown in Table 1. The reaction program was set as follows: 95°C for 30 s; 40 cycles at 95°C for 5 s, 60°C for 30 s; and 95°C for 15 s. The relative expression level of the target gene was normalized by β-actin and calculated by the equation of 2 -△△CT (Livak and Schmittgen, 2001).
Calculations
The calculation formula used in this study is as follows:
Survival (%) =100 × (final number of crabs)/(initial number of crabs),
Weight gain (WG, %) = 100 × (final body weight-initial body weight)/initial body weight,
Special growth rate (SGR, % day-1) = 100 × (ln final weight – ln initial weight)/56 days,
Molting frequency (MF)= Σ ((the stage of crab - 1) × the number of molting stages)/the total number of survival crabs.
Statistical Analysis
First, the survival rate data was converted into a continuous probability distribution (arcsine square-root transformation). Then, all data were checked for homogeneity (Levene’s test) and normality (Kolmogorov-Smirnov test). If the data was homogeneous and normally distributed, One-way ANOVA was used to test for significant treatment effects followed by Duncan’s multiple comparison post hoc test; otherwise, a nonparametric test (Kruskal-Wallis tests) was applied. P < 0.05 was considered statistically different between treatments. All statistical processing was performed in SPSS 26.0.
Result
Survival and Growth
The survival of juvenile P. trituberculatus was significantly affected by light intensity (Figure 1). As the light intensity increased from 0 to 15.89 μmol m-2 s-1, the survival rate of crabs increased from 52.50% to 75.00%; then as the light intensity further increased to 124.31 μmol m-2 s-1, the survival rate decreased to 57.50%.
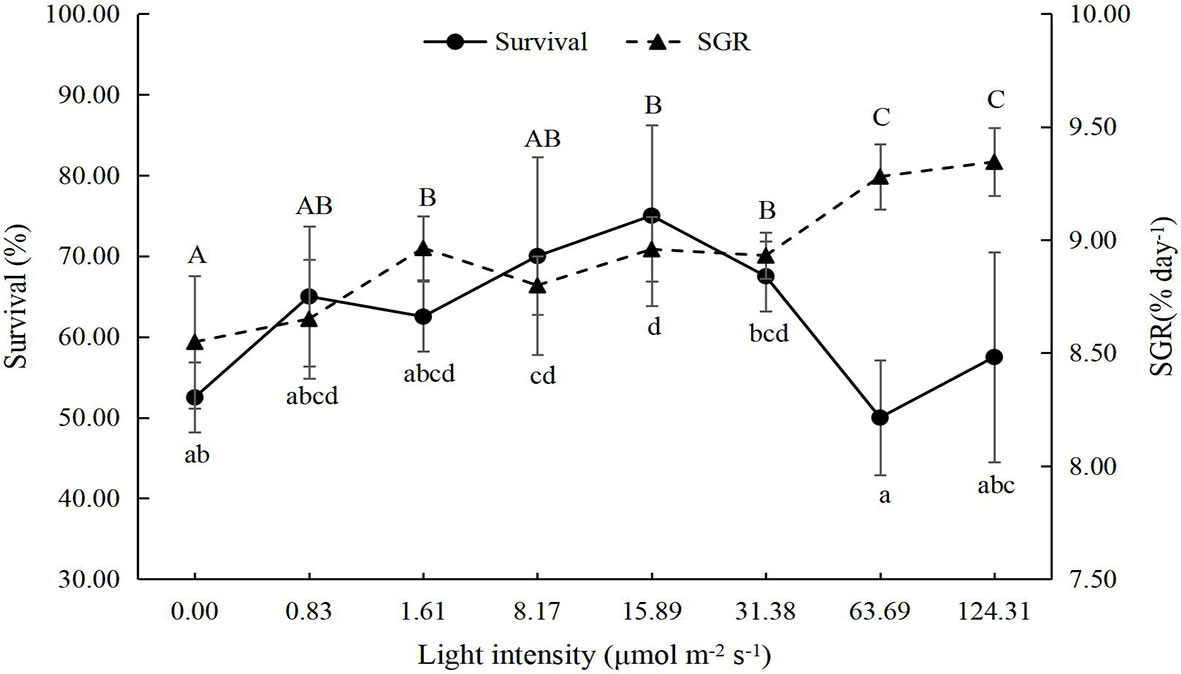
Figure 1 Effect of different light intensities on the survival and special growth rate (SGR) of juvenile Portunus trituberculatus (mean ± SD, n = 4). Different lowercase and capital letters indicate significant differences (P < 0.05) in survival and SGR, respectively.
The growth performance of juvenile P. trituberculatus was significantly improved by light intensity (Figures 1, 2). With the increase in light intensity, the WG, SGR, and MF of crabs increased significantly. Based on the linear fit of the survival, the suitable light intensity for the juvenile P. trituberculatus was 9.96 μmol m-2 s-1 (Figure 3).
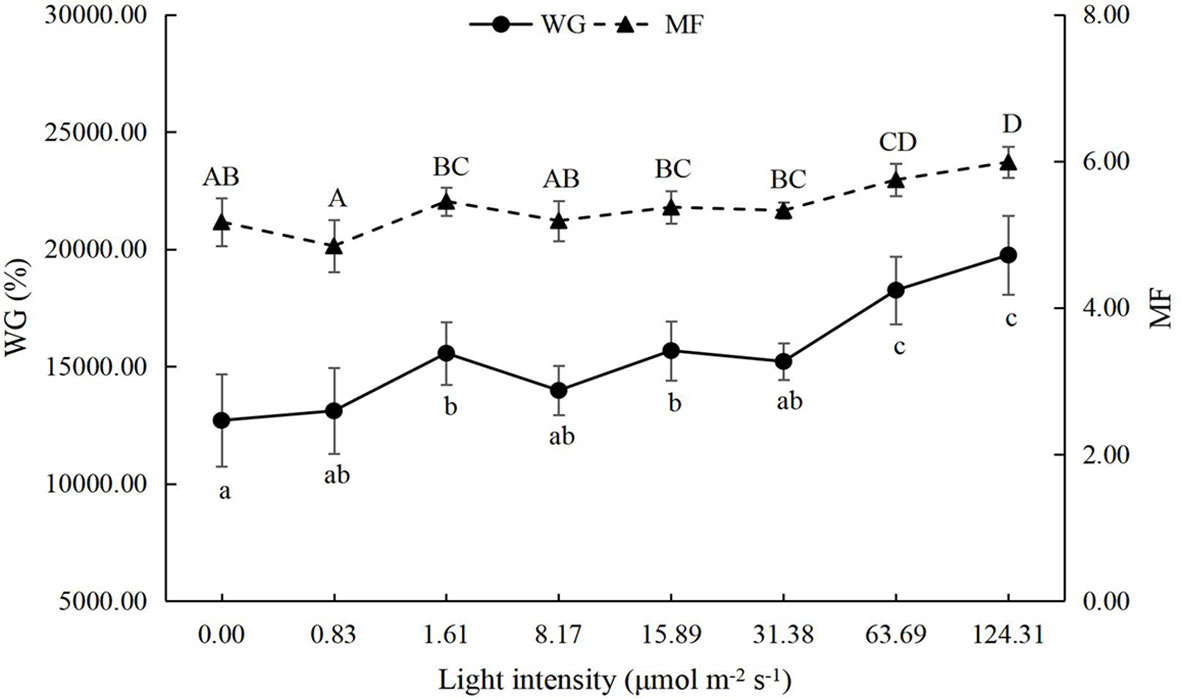
Figure 2 Effect of different light intensities on the weight gain (WG) and molting frequency (MF) of juvenile Portunus trituberculatus (mean ± SD, n = 4). Different lowercase and capital letters indicate significant differences (P < 0.05) in WG and MF, respectively.
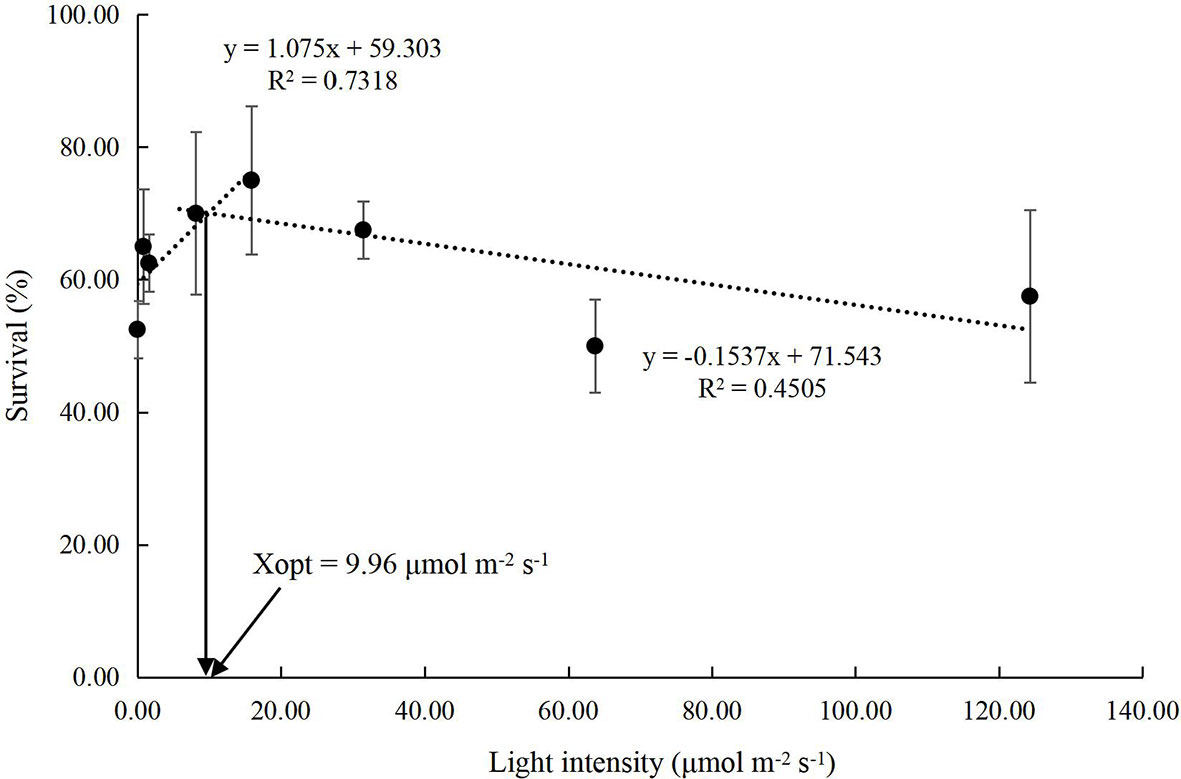
Figure 3 Relationship between light intensity and survival in juvenile Portunus trituberculatus (mean ± SD, n = 4) based on linear models, where Xopt represents the optimal light intensity for the maximum survival.
Hemolymph Indicators
The GLU levels in the hemolymph of the juvenile P. trituberculatus showed a U shape trend with increasing light intensity. Significantly low levels of GLU were observed at 1.61 μmol m-2 s-1, 8.17 μmol m-2 s-1, and 15.89 μmol m-2 s-1 light intensity groups (Figure 4A).
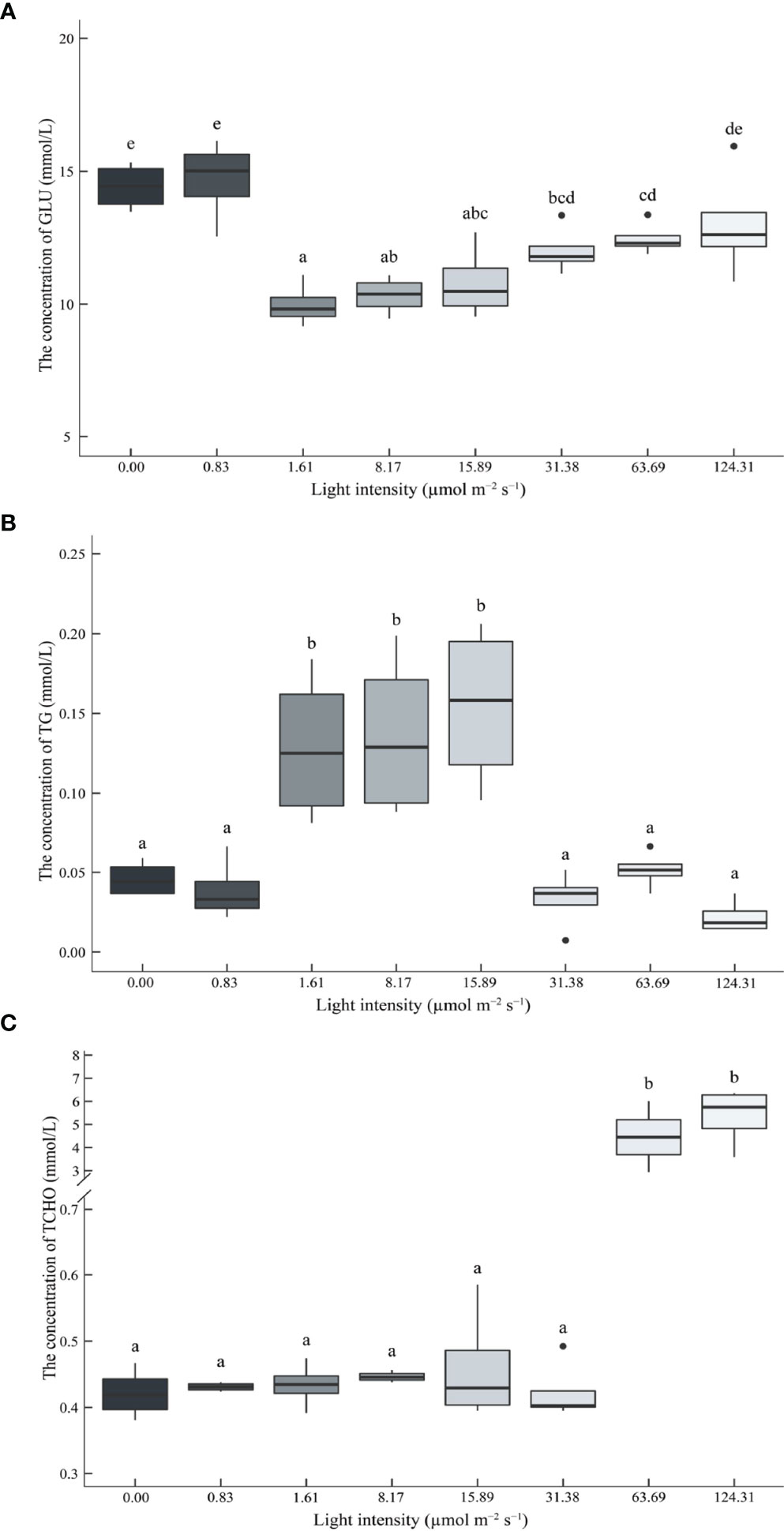
Figure 4 Effect of different light intensities on the glucose (GLU) (A), triglyceride (TG) (B), and total cholesterol (TCHO) (C) contents in the hemolymph of juvenile Portunus trituberculatus. Data (n = 4) with different letters significantly differ (P < 0.05) among treatments.
The TG levels in the hemolymph of the juvenile P. trituberculatus was parabolic. Significantly high levels of TG were also observed at 1.61 μmol m-2 s-1, 8.17 μmol m-2 s-1, and 15.89 μmol m-2 s-1 light intensity groups (Figure 4B).
High light intensity significantly increased TCHO levels in the hemolymph of juvenile P. trituberculatus, and significantly high levels of TCHO were observed at 63.69 μmol m-2 s-1 and 124.31 μmol m-2 s-1 light intensity groups (Figure 4C).
Antioxidant Capacity
In this study, the antioxidant capacity of juvenile P. trituberculatus was significantly affected by light intensity (Figure 5). The content of MDA in the hepatopancreas decreased with the increasing light intensity. The lowest value was observed in the 15.89 μmol m-2 s-1 group (Figure 5A). The T-AOC, SOD, and CAT activity in hepatopancreas showed opposite trends compared to MDA content, and high levels of T-AOC and SOD, CAT activities were observed in 8.17 μmol m-2 s-1 and 15.89 μmol m-2 s-1groups (Figures 5B–D).
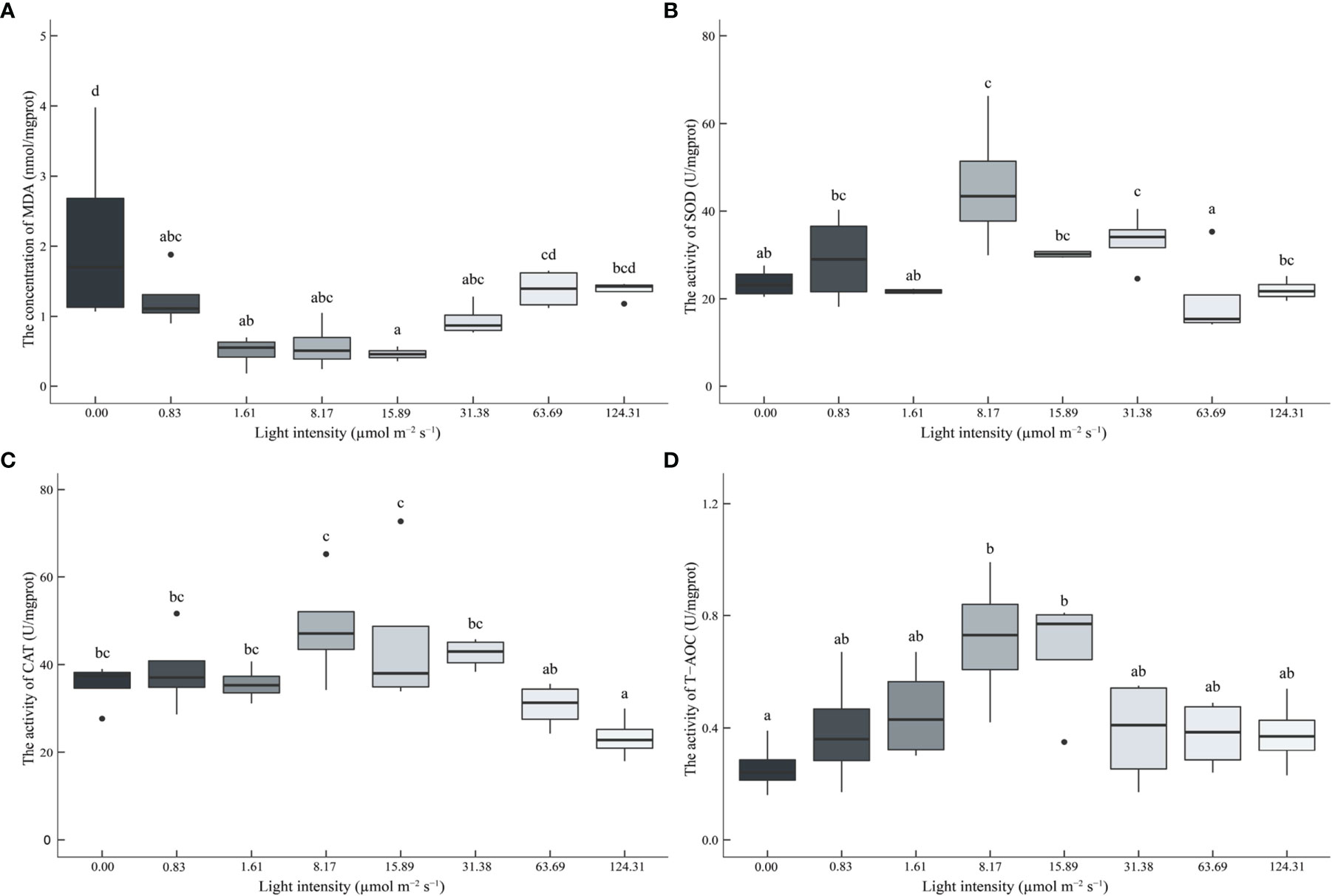
Figure 5 Effect of different light intensities on the malondialdehyde (MDA) (A) contents, superoxide dismutase (SOD) (B), catalase (CAT) (C), and total antioxidant capacity (T-AOC) (D) in the hepatopancreas of juvenile Portunus trituberculatus. Data (n=4) with different letters significantly differ (P < 0.05) among treatments.
Gene Expression
Light intensity significantly affected apoptosis-related genes expression (Figure 6). High light intensity (63.69 μmol m-2 s-1 and 124.31 μmol m-2 s-1) significantly up-regulated the expression of the jnk gene (Figure 6A). The expression levels of p53 and bcl-2 genes showed a U shape trend (63.69 μmol m-2 s-1 and 124.31 μmol m-2 s-1) (Figures 6B, C).
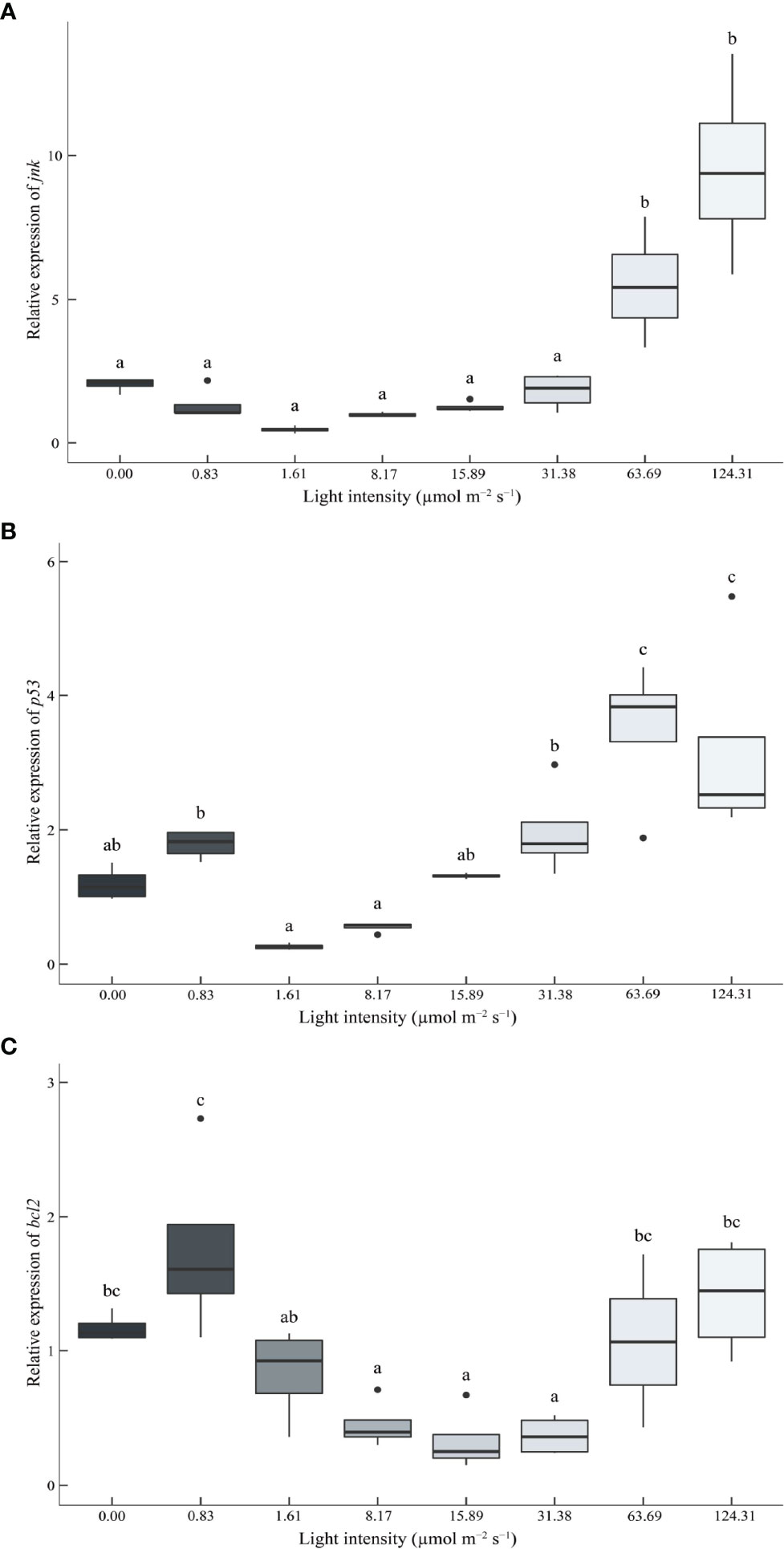
Figure 6 Effect of different light intensities on the c-Jun N-terminal kinase (jnk) (A), tumor suppressor p53 (p53) (B), and B-cell lymphoma-2 (bcl-2) (C) genes in the hepatopancreas of juvenile Portunus trituberculatus. Data (n = 4) with different letters significantly differ (P < 0.05) among treatments.
Molting-related genes were also significantly affected by light intensity (Figure 7). With the increasing light intensity, the gene expression of ecdysone receptor (ecr), retinoid-X receptor (rxr), and nuclear receptor E75 (e75) was significantly up-regulated (Figures 7A–C). On the contrary, the molt-inhibiting hormone (mih) gene decreased with increasing light intensity (except for the 0 μmol m-2 s-1 group) (Figure 7D).
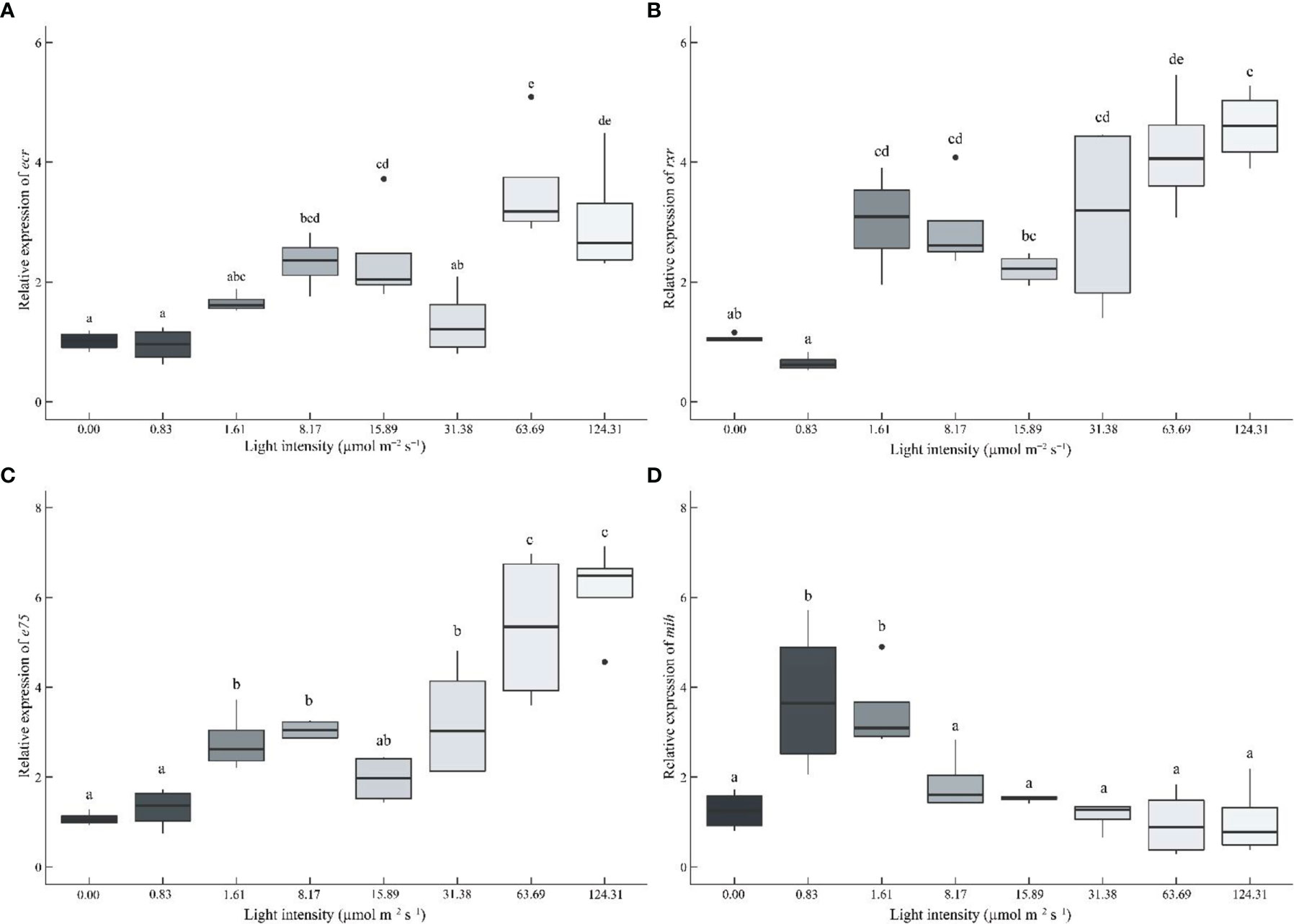
Figure 7 Effect of different light intensities on the ecdysone receptor (ecr) (A), retinoid X receptor (rxr) (B), nuclear receptor E75 (e75) (C), and molt-inhibiting hormone (mih) (D) genes in the eyestalk of juvenile Portunus trituberculatus. Data (n = 4) with different letters significantly differ (P < 0.05) among treatments.
Discussion
Light intensity manipulation is widely used in aquaculture to obtain greater productivity (Villamizar et al., 2011; Ruchin, 2021). In this study, the survival rate of juvenile P. trituberculatus was parabolic with the increasing light intensity. A linear fit based on survival showed that the suitable light intensity for the survival of the juvenile P. trituberculatus was 9.96 μmol m-2 s-1. The value is considerably lower compared with the optimal light intensity for P. trituberculatus larvae, for a previous study suggested that the highest survival rates were detected for Zoea IV larvae and megalopae at 195.31 and 124.31 μmol m−2 s-1 (Dou et al., 2021). The substantial decline in the light requirement of the juvenile P. trituberculatus could be attributed to their adaption to the ecological shift from pelagic to benthic habitats. However, the light intensity did not affect the survival of the juvenile S. paramamosain (Li et al., 2020a; Li et al., 2020b; Chen et al., 2021), probably because S. paramamosain are intertidal benthos (Walton et al., 2006; Yu et al., 2022) and may have broader adaptations to light intensity.
Darkness or low light intensities have been identified as a stressor (Wang et al., 2013). Low light intensities (including darkness) were found to cause oxidative damage in juvenile S. paramamosain (Chen et al., 2021) and gibel carp Carassius auratus gibelio (Wei et al., 2019). Wagner and Congleton (2004) divided blood indicators into four categories: (i) total protein, cholesterol, calcium, and alkaline phosphatase were considered as nutritional indicators; (ii) alanine aminotransferase, aspartate aminotransferase, and creatine kinase were considered as tissue damage indicators; (iii) triacylglycerol lipase and triglycerides were considered as lipid metabolism indicators; (iv) cortisol, glucose, Na+, and Cl- were considered as stress indicators. Blood GLU is widely used as a stress indicator to describe the stress state in aquatic animals (Leclercq et al., 2014; Qi et al., 2016). In this study, significantly higher hemolymph GLU levels were found in the 0 μmol m-2 s-1 and 0.83 μmol m-2 s-1 groups. In addition to GLU, previous studies have found that the blood TG and TCHO levels are also raised in response to increased energy demand due to stressful situations (Montero et al., 1999; Qi et al., 2016). However, high hemolymph TG and TCHO levels were not observed in the dark and low light intensity groups, suggesting lower levels of lipid metabolism in these treatments. Further, the photoperiod (light-dark cycle) acts as a primary environmental cue (zeitgeber) to guide the oscillation of the endogenous biological clock to maintain the normal metabolism and growth of the organism (Ashton et al., 2022). In this study, higher mortality in the dark group may also be attributed to circadian rhythm disruption (Ishibashi et al., 2013; Castejon et al., 2018; Pereira-Davison and Callan, 2018).
On the other hand, as the light intensity increased to 31.38 μmol m-2 s-1, the survival of crabs started to decrease, and significantly lower survival rates were observed in the 63.69 μmol m-2 s-1 and 124.31 μmol m-2 s-1 groups. High light intensity has been reported as a stressor in crabs (Coyle et al., 2011; Chen et al., 2021). Also, in this study, although there was no statistical difference, high hemolymph GLU levels were observed in the high light intensity groups. Moreover, significantly lower TG levels were also observed in the high light intensity group, which may be attributed to the impaired lipid metabolism caused by stress (Roychowdhury et al., 2020). In contrast to the low light intensity group, high light intensity significantly increased hemolymph TCHO levels. Although there is no standard for a normal hemolymph TCHO range of P. trituberculatus, hemolymph TCHO levels < 1 mmol/L were commonly observed in most studies (including different experimental treatments and different growth stages of the crabs) (Han et al., 2015; Wang et al., 2016; Ding et al., 2017; Sun et al., 2017; Han et al., 2018). Thus, the significantly high TCHO levels (> 4 mmol/L) in the 63.69 μmol m-2 s-1 and 124.31 μmol m-2 s-1 groups may suggest a disorder in cholesterol metabolism.
In this study, the growth performance of P. trituberculatus was also significantly affected by light intensity. In general, the growth of aquatic animals will decrease under stress (Wei et al., 2019; Yu et al., 2022). Unexpectedly, in this study, the growth of crabs significantly increased with the increase of light intensity. A possible reason is that stress elevates hemolymph cortisol levels, increases food intake and energy metabolism, and produces anabolic effects that ultimately promote growth (Mommsen et al., 1999; Elverson and Wilson, 2005; Kang and Kim, 2013; Chen et al., 2021). Similarly, Gardner and Maguire (1998) attributed the shortened intermolt duration of Australian giant crab Pseudocarcinus gigas at brighter light (500 lx vs. 2 lx) to increased feeding. However, in Chinese mitten crabs Eriocheir sinensis, different light intensities did not affect digestive enzyme activity under overfeeding conditions (Li et al., 2011). Therefore, future study is needed to determine whether high light intensity promotes growth performance by increasing feeding. In addition, our previous study found that suitable light intensity significantly increased the survival of the larval P. trituberculatus but did not affect development (Dou et al., 2021), implying that the effect of light intensity on crabs’ survival and growth varies according to the growth stage.
Crustacean weight gain is phased and closely related to molting (Devaraj and Natarajan, 2006; Chang et al., 2012). Previous studies observed a positive correlation between crab body weight and MF (Chen et al., 2021; Liu et al., 2021), and the same phenomenon was also observed in this study. The molting behavior of crabs is regulated by a pair of antagonistic hormones, ecdysteroids (Mykles, 2011) and molt-inhibiting hormone (MIH) (Huang et al., 2015). The ecdysteroid binds to EcR and RXR to form a heterodimer, activates the expression of the downstream gene e75, and finally regulates the molting process (Mykles, 2011; Song et al., 2017). On the other hand, MIH inhibits crustaceans’ molting by inhibiting the synthesis of ecdysteroids (Chang and Mykles, 2011). In the present study, the expression of ecr, rxr, and e75 genes was up-regulated with increasing light intensity. In contrast, mih gene expression levels were significantly down-regulated (except for the dark group), matching the MF. In addition, some researchers attributed the high MF under high light intensity to the activation of molt-stimulating hormone (MSH) and crustacean hyperglycemic hormones (CHH) synthesis (Hoang et al., 2003; Li et al., 2011).
The adverse stimulation would induce the excessive production and accumulation of ROS in crustaceans (Paital and Chainy, 2012; Duan et al., 2016). In the present study, SOD and CAT activities and T-AOC in the hepatopancreas of P. trituberculatus were parabolic with increasing light intensity. However, the level of MDA, an indicator of oxidative damage (Draper and Hadley, 1990; Rio et al., 2005), showed an opposite trend, indicating P. trituberculatus has a robust antioxidant capacity under the light intensity range of 8.17-15.89 μmol m-2 s-1.
To maintain homeostasis, organisms clear the damaged cells through programmed cell death, a process known as apoptosis (Xian et al., 2013; Chen et al., 2021). This process is regulated by a series of signals and genes in crustaceans (Menze et al., 2010). JNK, Bcl-2, and p53 are essential protein and transcription factors in the apoptosis signaling pathway that regulates the process of apoptosis (Cadet et al., 2000; Fridman and Lowe, 2003; Liu and Lin, 2005; Menze et al., 2010). In this study, suboptimal light intensity significantly increased the expression levels of these genes, suggesting cellular damage (Cheng et al., 2020; Fei et al., 2020). Similarly, suboptimal light intensity also up-regulated p53 gene expression in S. paramamosain (Chen et al., 2021).
Conclusion
The present study explored the effect of light intensity on the survival, growth, antioxidant, molting-related and apoptosis-related gene expression of juvenile swimming crab P. trituberculatus. Results revealed that low and high light intensities might act as stressors, reflected by the lower survival rate, higher GLU and MDA levels, and upregulation of apoptotic genes expression (jnk, p53, and bcl-2). On the other hand, high light intensity stimulated the expression of molting-related genes (ecr, rxr, e75) while suppressing mih gene expression, consequently boosting molting and growth performance. However, compared with growth, survival could be a more significant economic indicator for the young crabs in aquaculture. Therefore, the optimal light intensity for juvenile P. trituberculatus was estimated to be 9.96 μmol m-2 s-1 in terms of survival rate.
Data Availability Statement
The raw data supporting the conclusions of this article will be made available by the authors, without undue reservation.
Author Contributions
HX and JD performed the experiment, analyzed the data, and draft the MS. QW and CSh designed the experiment and review and edited the MS. All authors participated in the revision of this MS by providing comments. All authors contributed to the article and approved the submitted version.
Funding
The work was supported by the National Natural Science Foundation of China (Grant Nos. 31972783 and 32172994), The Province Key Research and Development Program of Zhejiang (2021C02047), Key Scientific and Technological Grant of Zhejiang for Breeding New Agricultural Varieties (2021C02069-6), Collaborative Promotion Program of Zhejiang Provincial Agricultural Technology of China (No. 2020XTTGSC03), SanNongLiuFang Zhejiang Agricultural Science and Technology Cooperation Project (2021SNLF029), 2025 Technological Innovation for Ningbo (No. 2019B10010), China Agriculture Research System of MOF and MARA (No. CARS48), the Special research funding from the Marine Biotechnology and Marine Engineering Discipline Group in Ningbo University (No. 422004582), and K. C. Wong Magna Fund in Ningbo University.
Conflict of Interest
The authors declare that the research was conducted in the absence of any commercial or financial relationships that could be construed as a potential conflict of interest.
Publisher’s Note
All claims expressed in this article are solely those of the authors and do not necessarily represent those of their affiliated organizations, or those of the publisher, the editors and the reviewers. Any product that may be evaluated in this article, or claim that may be made by its manufacturer, is not guaranteed or endorsed by the publisher.
References
Ashton A., Foster R. G., Jagannath A. (2022). Photic Entrainment of the Circadian System. Int. J. Mol. Sci. 23 (2), 729. doi: 10.3390/ijms23020729
Benzie I. F. F., Strain J. J. (1996). The Ferric Reducing Ability of Plasma (Frap) as a Measure of “Antioxidant Power”: The Frap Assay. Anal Biochem. 1996 (1), 70–76. doi: 10.1006/abio.1996.0292
Boeuf G., Le Bail P. Y. (1999). Does Light Have an Influence on Fish Growth? Aquaculture 177 (1-4), 129–152. doi: 10.1016/S0044-8486(99)00074-5
Bradford M. M. (1976). A Rapid and Sensitive Method for the Quantitation of Microgram Quantities of Protein Utilizing the Principle of Protein-Dye Binding. Anal. Biochem. 72 (1-2), 248–254. doi: 10.1016/0003-2697(76)90527-3
Cadet J. L., Harrington B., Ordonez S. (2000). Bcl-2 Overexpression Attenuates Dopamine-Induced Apoptosis in an Immortalized Neural Cell Line by Suppressing the Production of Reactive Oxygen Species. Synapse 35, 228–233. doi: 10.1002/(SICI)1098-2396(20000301)35:3<228::AID-YN8>3.0.CO;2-#
Castejon D., Rotllant G., Gimenez L., Torres G., Guerao G. (2018). Influence of Temperature and Light Regime on the Larval Development of the Common Spider Crab Maja Brachydactyla Balss 1922 (Brachyura: Majidae). Aquacult. Res. 49 (11), 3548–3558. doi: 10.1111/are.13820
Chang E. S., Mykles D. L. (2011). Regulation of Crustacean Molting: A Review and Our Perspectives. Gen. Comp. Endocrinol. 172, 323–330. doi: 10.1016/j.ygcen.2011.04.003
Chang Y. J., Sun C. L., Chen Y., Yeh S. Z. (2012). Modelling the Growth of Crustacean Species. Rev. Fish Biol. Fish 22 (1), 157–187. doi: 10.1007/s11160-011-9228-4
Cheng C. H., Su Y. L., Ma H. L., Deng Y. Q., Feng J., Chen X. L., et al. (2020). Effect of Nitrite Exposure on Oxidative Stress, DNA Damage and Apoptosis in Mud Crab (Scylla Paramamosain). Chemosphere 239, 124668. doi: 10.1016/j.chemosphere.2019.124668
Chen S., Migaud H., Shi C., Song C., Wang C., Ye Y., et al. (2021). Light Intensity Impacts on Growth, Molting and Oxidative Stress of Juvenile Mud Crab Scylla Paramamosain. Aquaculture 545, 737159. doi: 10.1016/j.aquaculture.2021.737159
Coyle S. D., Bright L. A., Wood D. R., Neal R. S., Tidwell J. H. (2011). Performance of Pacific White Shrimp, Litopenaeus Vannamei, Reared in Zero-Exchange Tank Systems Exposed to Different Light Sources and Intensities. J. World Aquacult. Soc. 42 (5), 687–695. doi: 10.1111/j.1749-7345.2011.00512.x
Dai A. Y., Yang S. L., Song Y. Z. (1986). Marine Crabs in China Sea (Beijing: Marine Publishing Company), 194–196.
Deeg R., Kraemer W., Ziegenhorn J. (1980). Kinetic Determination of Serum Glucose by Use of the Hexokinase/Glucose-6-Phosphate Dehydrogenase Method. Clin. Chem. Lab. Med. 18, 49–52. doi: 10.1515/cclm.1980.18.1.49
Devaraj H., Natarajan A. (2006). Molecular Mechanisms Regulating Molting in a Crustacean. FEBS J. 273 (4), ,819–,846. doi: 10.1111/j.1742-4658.2006.05117.x
Ding L. Y., Fu H. Y., Hou Y. M., Jin M., Sun P., Zhou Q. C. (2017). Effects of Starvation and Feeding on Blood Chemistry, Fatty Acid Composition and Expression of Vitellogenin and Fatty Acid-Binding Protein Genes in Female Swimming Crab Portunus Trituberculatus Broodstock. Fish Sci. 83, 455–464. doi: 10.1007/s12562-017-1075-3
Dong C. H., Zhao J. M., Song L. S., Wang L. L., Qiu L. M., Zheng P. L., et al. (2009). The Immune Responses in Chinese Mitten Crab Eriocheir Sinensis Challenged With Double-Stranded RNA. Fish Shellfish Immunol. 26 (3), 438–442. doi: 10.1016/j.fsi.2009.01.005
Dou J., Zhang G. L., Shi C., Song C. B., Mu C. K., Ye Y. F., et al. (2021). High-Intensity Light of Full-Spectrum LED Promotes Survival Rate But Not Development of the Larval Swimming Crab Portunus Trituberculatus. Aquacult. Eng. 93, 102158. doi: 10.1016/j.aquaeng.2021.102158
Draper H. H., Hadley M. (1990). Malondialdehyde Determination as Index of Lipid Peroxidation. Methods Enzymol 186, 421–431. doi: 10.1016/0076-6879(90)86135-I
Duan Y. F., Zhang Y., Dong H. B., Zhang J. S. (2016). Effect of Desiccation on Oxidative Stress and Antioxidant Response of the Black Tiger Shrimp Penaeus Monodon. Fish Shellfish Immunol. 58, 10–17. doi: 10.1016/j.fsi.2016.09.004
Elverson C. A., Wilson M. E. (2005). Cortisol: Circadian Rhythm and Response to a Stressor. N Newborn Infant Nurs. Rev. 5 (4), 159–169. doi: 10.1053/j.nainr.2005.09.002
FAO. (2021). FAO Yearbook. Fishery and Aquaculture Statistics 2019 (Rome: Food and Agriculture Organization of the United Nations). doi: 10.4060/cb1213t
Fei F., Liu B., Gao X., Wang X., Liu Y., Bin H. (2020). Effects of Supplemental Ultraviolet Light on Growth, Oxidative Stress Responses, and Apoptosis-Related Gene Expression of the Shrimp Litopenaeus Vannamei. Aquaculture 520, 735013. doi: 10.1016/j.aquaculture.2020.735013
Fisheries Bureau of Agriculture Ministry of China. (2016-2021). 2016-2021 China Fishery Statistical Yearbook (Beijing, China: China Agriculture Press), 22, 38.
Fridman J. S., Lowe S. W. (2003). Control of Apoptosis by P53. Oncogene 22, 9030–9040. doi: 10.1038/sj.onc.1207116
Gardner C., Maguire G. B. (1998). Effect of Photoperiod and Light Intensity on Survival, Development and Cannibalism of Larvae of the Australian Giant Crab Pseudocarcinus Gigas (Lamarck). Aquaculture 165 (1-2), 51–63. doi: 10.1016/S0044-8486(98)00245-2
Góth L. (1991). A Simple Method for Determination of Serum Catalase Activity and Revision of Reference Range. Clin. Chimica Acta 196, 143–151. doi: 10.1016/0009-8981(91)90067-M
Haché R., Mallet M. D., Dumas A. (2015). Effects of Light Regime on Larvae Survival, Growth and Settling Behavior of Hatchery Reared American Lobster (Homarus Americanus). Aquaculture 443, 31–39. doi: 10.1016/j.aquaculture.2015.02.028
Han T., Wang J. T., Li X. Y., Yang Y. X., Wang J. X., Hu S. X., et al. (2015). Effects of Dietary Cholesterol Levels on the Growth, Molt Performance, and Immunity of Juvenile Swimming Crab, Portunus Trituberculatus. Israeli J. Aquacult-Bamidgeh 67, 1191. doi: 10.46989/001c.20696
Han T., Wang J. T., Li X. Y., Yang Y. X., Yang M., Tian H. L., et al. (2018). Effects of Dietary Phospholipid and Cholesterol Levels on Growth and Fatty Acid Composition of Juvenile Swimming Crab, Portunus Trituberculatus. Aquacult. Nutr. 24 (1), 164–172. doi: 10.1111/anu.12544
Henkel E., Stoltz M. (1982). A Newly Drafted Colour Test for the Determination of Triglycerides Convenient for Manual and Mechanized Analysis (Glycerolphosphate-Oxidase-PAP Method). Fresenius’ Z. für Analytische Chemie 311, 451–452. doi: 10.1007/BF00481817
Hoang T., Barchiesis M., Lee S. Y., Keenan C. P., Marsden G. E. (2003). Influences of Light Intensity and Photoperiod on Moulting and Growth of Penaeus Merguiensis Cultured Under Laboratory Conditions. Aquaculture 216, 343–354. doi: 10.1016/S0044-8486(02)00460-X
Hoang T., Lee S. Y., Keenan C. P., Marsden G. E. (2002a). Ovarian Maturation of the Banana Prawn, Penaeus Merguiensis De Man Under Different Light Intensities. Aquaculture 208 (1-2), 159–168. doi: 10.1016/S0044-8486(01)00713-X
Hoang T., Lee S. Y., Keenan C. P., Marsden G. E. (2002b). Effect of Light Intensity on Maturation and Spawning of Ablated Female Penaeus Merguiensis. Aquaculture 209 (1–4), 347–358. doi: 10.1016/S0044-8486(01)00814-6
Huang H., Fu C., Chen X., Gong J., Huang X., Ye H. (2015). Molt-Inhibiting Hormone (MIH) Gene From the Green Mud Crab Scylla Paramamosain and its Expression During the Molting and Ovarian Cycle. Aquacult. Res. 46 (11), 2665–2675. doi: 10.1111/are.12421
Ishibashi Y., Honryo T., Miyashita S., Oda S. (2013). Suitable Photoperiod and Light Intensity of Laboratory-Reared Juvenile Pacific Bluefin Tuna Thunnus Orientalis. Aquacult. Sci. 61 (4), 399–402. doi: 10.11233/aquaculturesci.61.399
Kang D. Y., Kim H. C. (2013). Influence of Density and Background Color to Stress Response, Appetite, Growth, and Blind-Side Hypermelanosis of Flounder, Paralichthys Olivaceus. Fish Physiol. Biochem. 39, 221–232. doi: 10.1007/s10695-012-9693-2
Leclercq E., Davie A., Migaud H. (2014). The Physiological Response of Farmed Ballan Wrasse (Labrus Bergylta) Exposed to an Acute Stressor. Aquaculture 434, 1–4. doi: 10.1016/j.aquaculture.2014.07.017
Li X. W., Li Z. J., Liu J. S., Zhang T. L., Zhang C. W. (2011). Effects of Light Intensity on Molting, Growth, Precocity, Digestive Enzyme Activity, and Chemical Composition of Juvenile Chinese Mitten Crab Eriocheir Sinensis. Aquacult. Int. 19 (2), 301–311. doi: 10.1007/s10499-010-9414-8
Lin Y., Huang J. J., Dahms H. U., Zhen J. J., Ying X. P. (2017). Cell Damage and Apoptosis in the Hepatopancreas of Eriocheir Sinensis Induced by Cadmium. Aquat. Toxicol. 190, 190–198. doi: 10.1016/j.aquatox.2017.07.008
Liu J., Lin A. N. (2005). Role of JNK Activation in Apoptosis: A Double-Edged Sword. Cell Res. 15 (1), 36–42. doi: 10.1038/sj.cr.7290262
Liu S., Sun J. S., Hurtado L. A. (2013). Genetic Differentiation of Portunus Trituberculatus, the World’s Largest Crab Fishery, Among its Three Main Fishing Areas. Fish Res. 148, 38–46. doi: 10.1016/j.fishres.2013.08.003
Liu S., Wang X., Bu X., Zhang C., Qiao F., Qin C., et al. (2021). Influences of Dietary Vitamin D3 on Growth, Antioxidant Capacity, Immunity and Molting of Chinese Mitten Crab (Eriocheir Sinensis) Larvae. J. Steroid Biochem. Mol. Biol. 210, 105862. doi: 10.1016/j.jsbmb.2021.105862
Livak K. J., Schmittgen T. D. (2001). Analysis of Relative Gene Expression Data Using Real-Time Quantitative PCR and the 2-△△CT Method. Methods 25 (4), 402–408. doi: 10.1006/meth.2001.1262
Li N., Zhou J. M., He C. Y., Fu Y. Y., Wang C. L., Liu L., et al. (2021). GC-MS-Based Metabolomics Reveal That Light Intensity During Indoor Overwintering Affects the Metabolism of Scylla Paramamosain. Aquacult. Res. 52, 1013–1025. doi: 10.1111/are.14956
Li N., Zhou J. M., Wang H., Mu C. K., Shi C., Liu L., et al. (2020c). Transcriptome Analysis of Genes and Pathways Associated With Metabolism in Scylla Paramamosain Under Different Light Intensities During Indoor Overwintering. BMC Genomics 21, 775. doi: 10.1186/s12864-020-07190-w
Li N., Zhou J., Wang H., Wang C., Mu C., Shi C., et al. (2020a). Effects of Light Intensity on Growth Performance, Biochemical Composition, Fatty Acid Composition and Energy Metabolism of Scylla Paramamosain During Indoor Overwintering. Aquacult. Rep. 18, 100443. doi: 10.1016/j.aqrep.2020.100443
Li N., Zhou J., Wang H., Wang C., Mu C., Shi C., et al. (2020b). Effect of Light Intensity on Digestion and Immune Responses, Plasma Cortisol and Amino Acid Composition of Scylla Paramamosain During Indoor Overwintering. Aquacult. Res. 51, 5005–5014. doi: 10.1111/are.14836
Menze M. A., Fortner G., Nag S., Hand S. C. (2010). Mechanisms of Apoptosis in Crustacea: What Conditions Induce Versus Suppress Cell Death? Apoptosis 15 (3), 293–312. doi: 10.1007/s10495-009-0443-6
Mommsen T. P., Vijayan M. M., Moon T. W. (1999). Cortisol in Teleosts: Dynamics, Mechanisms of Action, and Metabolic Regulation. Rev. Fish Biol. Fish 9, 211–268. doi: 10.1023/A:1008924418720
Montero D., Izquierdo M., Tort L., Robaina L. E., Vergara J. M. (1999). High Stocking Density Produces Crowding Stress Altering Some Physiological and Biochemical Parameters in Gilthead Seabream, Sparus Aurata, Juveniles. Fish Physiol. Biochem. 20, 53–60. doi: 10.1023/A:1007719928905
Moss G. A., Tong L. J., Illingworth J. (1999). Effects of Light Intensity and Food Density on the Growth and Survival of Early-Stage Phyllosoma Larvae of the Rock Lobster Jasus Edwardsii. Mar. Freshwater Res. 50, 129–134. doi: 10.1071/MF98112
Mykles D. L. (2011). Ecdysteroid Metabolism in Crustaceans. J. Steroid Biochem. Mol. Biol. 127, 196–203. doi: 10.1016/j.jsbmb.2010.09.001
Nurdiani R., Zeng C. S. (2007). Effects of Temperature and Salinity on the Survival and Development of Mud Crab, Scylla Serrata (Forsskal), Larvae. Aquacult. Res. 38 (14), 1529–1538. doi: 10.1111/j.1365-2109.2007.01810.x
Ohkawa H., Ohishi N., Yagi K. (1979). Assay for Lipid Peroxides in Animal Tissues by Thiobarbituric Acid Reaction. Anal. Biochem. 95 (2), 351–358. doi: 10.1016/0003-2697(79)90738-3
Paital B., Chainy G. B. N. (2012). Effects of Salinity on O-2 Consumption, ROS Generation and Oxidative Stress Status of Gill Mitochondria of the Mud Crab Scylla Serrata. Comp. Biochem. Physiol. C-Toxicol. Pharmacol. 155 (2), 228–237. doi: 10.1016/j.cbpc.2011.08.009
Pereira-Davison E., Callan C. K. (2018). Effects of Photoperiod, Light Intensity, Turbidity and Prey Density on Feed Incidence and Survival in First Feeding Yellow Tang (Zebrasoma Flavescens) (Bennett). Aquacult. Res. 49 (2), 890–899. doi: 10.1111/are.13535
Peskin A. V., Winterbourn C. C. (2000). A Microtiter Plate Assay for Superoxide Dismutase Using a Water-Soluble Tetrazolium Salt (WST-1). Clin. Chim. Acta 293 (1-2), 157–166. doi: 10.1016/S0009-8981(99)00246-6
Qi C. L., Xie C. X., Tang R., Qin X., Wang D. Y., Li D. P. (2016). Effect of Stocking Density on Growth, Physiological Responses, and Body Composition of Juvenile Blunt Snout Bream, Megalobrama Amblycephala. J. World Aquacult. Soc. 47 (3), 358–368. doi: 10.1111/jwas.12278
Rio D. D., Stewart A. J., Pellegrini N. (2005). A Review of Recent Studies on Malondialdehyde as Toxic Molecule and Biological Marker of Oxidative Stress. Nutr. Metab. Cardiovasc. Dis. 15, 316–328. doi: 10.1016/j.numecd.2005.05.003
Roychowdhury P., Aftabuddin M., Pati M. K. (2020). Thermal Stress Altered Growth Performance and Metabolism and Induced Anaemia and Liver Disorder in Labeo Rohita. Aquacult. Res. 51 (4), 1406–1414. doi: 10.1111/are.14486
Ruchin A. B. (2021). Effect of Illumination on Fish and Amphibian: Development, Growth, Physiological and Biochemical Processes. Rev. Aquacult. 13 (1), 567–600. doi: 10.1111/raq.12487
Shentu J. K., Xu Y. J., Ding Z. N. (2015). Effects of Salinity on Survival, Feeding Behavior and Growth of the Juvenile Swimming Crab, Portunus Trituberculatus (Miers 1876). Chin. J. Oceanol. Limnol. 33 (3), 679–684. doi: 10.1007/s00343-015-4218-3
Shi C., Wang J., Peng K., Mu C., Ye Y., Wang C. (2019). The Effect of Tank Colour on Background Preference, Survival and Development of Larval Swimming Crab Portunus Trituberculatus. Aquaculture 504, 454– 461. doi: 10.1016/j.aquaculture.2019.01.032
Song Y., Villeneuve D. L., Toyota K., Lguchi T., Tollefsen K. E. (2017). Ecdysone Receptor Agonism Leading to Lethal Molting Disruption in Arthropods: Review and Adverse Outcome Pathway Development. Environ. Sci. Technol. 51 (8), 4142–4157. doi: 10.1021/acs.est.7b00480
Sun P., Jin M., Ding L. Y., Lu Y., Yuan Y., Ma H. N., et al. (2017). Effect of Dietary Soybean Lecithin and Cholesterol on Growth, Antioxidant Status and Fatty Acid Composition of Juvenile Swimming Crab, Portunus Trituberculatus. Israeli J. Aquacult-Bamidgeh 69, 1421. doi: 10.46989/001c.20856
Suzuki N., Mittler R. (2006). Reactive Oxygen Species and Temperature Stresses: A Delicate Balance Between Signaling and Destruction. Physiol. Plantarum 126 (1), 45–51. doi: 10.1111/j.0031-9317.2005.00582.x
Syafaat M. N., Mohammad S., Azra M. N., Ma H. Y., Abol-Munafi A. B., Ikhwanuddin M. (2020). Effect of Water Temperature on Survival, Growth and Molting Cycle During Early Crablet Instar of Mud Crab, Scylla Paramamosain (Estampado). Thalassas 36 (2), 543–551. doi: 10.1007/s41208-020-00233-9
Villamizar N., Blanco-Vives B., Migaud H., Davie A., Carboni S., Sanchez-Vazquez F. J. (2011). Effects of Light During Early Larval Development of Some Aquacultured Teleosts: A Review. Aquaculture 315 (1-2), 86–94. doi: 10.1016/j.aquaculture.2010.10.036
Wagner T., Congleton J. L. (2004). Blood Chemistry Correlates of Nutritional Condition, Tissue Damage, and Stress in Migrating Juvenile Chinook Salmon (Oncorhynchus Tshawytscha). Can. J. Fish Aquat. Sci. 61 (7), 1066–1074. doi: 10.1139/F04-050
Walton M. E., Le Vay L., Truong L. M., Ut V. N. (2006). Significance of Mangrove–Mudflat Boundaries as Nursery Grounds for the Mud Crab, Scylla Paramamosain. Mar. Biol. 149, 1199–1207. doi: 10.1007/s00227-006-0267-7
Wang T., Cheng Y. Z., Liu Z. P., Yan S. H., Long X. H. (2013). Effects of Light Intensity on Growth, Immune Response, Plasma Cortisol and Fatty Acid Composition of Juvenile Epinephelus Coioides Reared in Artificial Seawater. Aquaculture 414, 135–139. doi: 10.1016/j.aquaculture.2013.08.004
Wang J. T., Han T., Li X. Y., Hu S. X., Jiang Y. D., Wang C. L. (2016). Effects of Dietary Phosphatidylcholine (PC) Levels on the Growth, Molt Performance and Fatty Acid Composition of Juvenile Swimming Crab, Portunus Trituberculatus. Anim. Feed Sci. Technol. 216, 225–233. doi: 10.1016/j.anifeedsci.2016.03.023
Wang J., Peng K., Lu H., Li R., Song W., Liu L., et al. (2019). The Effect of Tank Colour on Growth Performance, Stress Response and Carapace Colour of Juvenile Swimming Crab Portunus Trituberculatus. Aquacult. Res. 50, 2735–2742. doi: 10.1111/are.14224
Wei H., Li H. D., Xia Y., Liu H. K., Han D., Zhu X. M., et al. (2019). Effects of Light Intensity on Phototaxis, Growth, Antioxidant and Stress of Juvenile Gibel Carp (Carassius Auratus Gibelio). Aquaculture 501, 39–47. doi: 10.1016/j.aquaculture.2021.737159
Xian J. A., Miao Y. T., Li B., Guo H., Wang A. L. (2013). Apoptosis of Tiger Shrimp (Penaeus Monodon) Haemocytes Induced by Escherichia Coli Lipopolysaccharide. Comp. Biochem. Phys. A 164, 301–306. doi: 10.1016/j.cbpa.2012.10.008
Yuan Q., Wang Q. D., Zhang T. L., Li Z. J., Liu J. S. (2017). Effects of Water Temperature on Growth, Feeding and Molting of Juvenile Chinese Mitten Crab Eriocheir Sinensis. Aquaculture 468, 169–174. doi: 10.1016/j.aquaculture.2016.10.007
Yu K. J., Shi C., Liu X. Z., Ye Y. F., Wang C. L., Mu C. K., et al. (2022). Tank Bottom Area Influences the Growth, Molting, Stress Response, and Antioxidant Capacity of Juvenile Mud Crab Scylla Paramamosain. Aquaculture 548, 737705. doi: 10.1016/j.aquaculture.2021.737705
Zhang C., Zhang Q., Pang Y. Y., Song X. Z., Zhou N., Wang J., et al. (2019). The Protective Effects of Melatonin on Oxidative Damage and the Immune System of the Chinese Mitten Crab (Eriocheir Sinensis) Exposed to Deltamethrin. Sci. Total Environ. 653, 1426–1434. doi: 10.1016/j.scitotenv.2018.11.063
Keywords: LED, molting, antioxidant, apoptosis, swimming crab
Citation: Xu H, Dou J, Wu Q, Ye Y, Song C, Mu C, Wang C, Ren Z and Shi C (2022) Investigation of the Light Intensity Effect on Growth, Molting, Hemolymph Lipid, and Antioxidant Capacity of Juvenile Swimming Crab Portunus trituberculatus. Front. Mar. Sci. 9:922021. doi: 10.3389/fmars.2022.922021
Received: 17 April 2022; Accepted: 03 May 2022;
Published: 27 May 2022.
Edited by:
Samad Rahimnejad, University of South Bohemia in České Budějovice, CzechiaCopyright © 2022 Xu, Dou, Wu, Ye, Song, Mu, Wang, Ren and Shi. This is an open-access article distributed under the terms of the Creative Commons Attribution License (CC BY). The use, distribution or reproduction in other forums is permitted, provided the original author(s) and the copyright owner(s) are credited and that the original publication in this journal is cited, in accordance with accepted academic practice. No use, distribution or reproduction is permitted which does not comply with these terms.
*Correspondence: Qingyang Wu, d3VxaW5neWFuZ0BuYnUuZWR1LmNu; Ce Shi, c2hpY2UzMjEwQDEyNi5jb20=