- 1National Research Council, Institute of Marine Sciences, CNR-ISMAR, Venice, Italy
- 2National Research Council, Institute of Marine Sciences, CNR-ISMAR, Milan, Italy
- 3National Institute of Oceanography and Applied Geophysics-OGS, Trieste, Italy
- 4National Research Council, Institute for Electromagnetic Sensing of the Environment, CNR-IREA, Milan, Italy
- 5Blue World Institute of Marine Research and Conservation, Veli Lošinj, Croatia
Implementing effective marine monitoring to detect and track ecosystem shifts, biodiversity alteration, and habitat loss is one of the most crucial challenges to meet the objectives set out by the Post-2020 Biodiversity Framework and by the United Nations Sustainable Development Goals. The lack of coordinated and harmonized monitoring frameworks at different spatial scales and their weakness in accounting for ecological processes, due to incomplete sets of monitoring variables, strongly hinder the achievement of conservation objectives. Here, we propose an approach to build a coherent ecosystem-based system of monitoring variables for target marine species and habitats. The approach is designed to integrate the existing monitoring frameworks set up by the Water and the Marine Strategy Framework directives, and the Essential Ocean and Biodiversity Variables, with the aim to contribute to their harmonization and implementation. Furthermore, by embracing a holistic vision, it aims to incorporate ecological processes and socio-ecological aspects, considering the benefits of public engagement through citizen science, and of the ecosystem services approach for policies’ implementation. The study stems from the Ecological Observing System of the Adriatic Sea (ECOAdS), which was developed in the framework of the Interreg Italy-Croatia project ECOSS, using as exemplary monitoring test cases two relevant conservation targets for Natura 2000 sites of the Adriatic Sea, the common bottlenose dolphin and seagrass meadows. We test the potential of this approach in guiding the prioritization of monitoring variables under ecosystem-based criteria, and provide insights into the benefits delivered by an integrated system of observatories’ networks and monitoring frameworks to support marine conservation at both local and regional scales. The proposed approach can be transferred to other contexts and scales to help build a common knowledge and monitoring framework for conservation and management strategies, saving costs by relying on available resources and on consolidated and long-lasting approaches that might converge towards global initiatives.
Tursiops truncatus urn:lsid:zoobank.org:act:1B8DC3EB-C5D4-42CE-AD8B-EB3A1E500E81
Cymodocea nodosa urn:lsid:catalogueoflife.org:taxon:2634e832-60a7-102d-be47-00304854f810:col20110201
Zostera marina urn:lsid:catalogueoflife.org:taxon:2637f144-60a7-102d-be47-00304854f810:col20110201
Zostera noltii urn:lsid:catalogueoflife.org:taxon:d2c6e5d4-2dc5-11e0-98c6-2ce70255a436:col20110201
Posidonia oceanica urn:lsid:catalogueoflife.org:taxon:f0c509a2-29c1-102b-9a4a-00304854f820:col20110201
Introduction
The marine environment is impacted by a multitude of anthropogenic stressors that, with an unprecedented speed, are impairing its status (Pinsky et al., 2013; Bryndum-Buchholz et al., 2019; Maxwell et al., 2020; Nicholson et al., 2021). Several initiatives are in place struggling to find a way to revert this deterioration trajectory and to ensure the conservation and restoration of nature—among the many, the Convention on Biological Diversity, the European Biodiversity Strategy 2030, and the Global Sustainable Development Goals (SDGs), especially SDG14 “Life Below Water” (Rees et al., 2018; Reimer et al., 2021; Gissi et al., 2022; Hermoso et al., 2022). However, both local and climate-induced pressures acting on marine ecosystems lead to unpredictable changes in their structure and functioning, and forecasting the effects of such changes on their resilience and capacity to provide ecosystem services is challenging (García Molinos et al., 2016; Lotze et al., 2019; Gissi et al., 2021). The most reasonable strategy to put in place for marine management and conservation to be effective is to embrace an adaptive and ecosystem-based approach, able to deal with fast-changing conditions (Magris et al., 2014; Frazão Santos et al., 2016; Rilov et al., 2019).
To operationalize such an approach, a baseline of knowledge and the continuous acquisition of up-to-date data are essential (Lombard et al., 2019). Thus, the monitoring of the marine environment is key and must be systematic and long-lasting to be able to provide the information needed to effectively feed evidence-based management (Addison et al., 2018; McQuatters-Gollop et al., 2019). Monitoring strategies should be coordinated transnationally to favor the collection and sharing of data beyond the geopolitical boundaries to capture large-scale and interconnected ecological processes. Indeed, beyond characterizing species and habitat structure and their distribution within ecosystems, monitoring should depict the key processes that allow species to feed, move, reproduce, and interact among them and with the surrounding environment, thus supporting ecosystem functioning (Williams et al., 2018; UNEP, 2019). Such an approach would enable the advancement of our understanding of the driving forces that affect species, populations, and habitats and the identification of monitoring variables that might better inform anticipatory management strategies, such as describing early-warning signals (Scheffer et al., 2012; Pinsky et al., 2013; Clements et al., 2019). Additionally, to adequately interpret the observed ecosystems’ conditions, due to the mutable and complex nature of the marine environment (Maxwell et al., 2015), the monitoring has to be performed by combining information coming from multiple variables to describe ecosystem shifts (Kroeker et al., 2020) and favor anticipatory conservation actions and management (Van Hoey et al., 2010). However, the vast array of variables that can be potentially monitored in marine environments (Turnhout et al., 2007), along with the complexity and amount of derived data, complicates the selection of the most relevant and useful information to effectively depict ecological processes and to inform management (Fischer and Grimes, 2012; Hayes et al., 2015); therefore, the identification of clear criteria for monitoring variable selection would be of great benefit.
Diverse initiatives that can satisfy these needs are in place, dictated by both legally binding European (EU) frameworks for monitoring (e.g., the Water and the Marine Strategy Framework directives, WFD and MSFD, respectively; EC, 2000; EC, 2008), and global initiatives, such as the Essential Ocean and Biodiversity Variables frameworks (EOVs and EBVs) under the Global Ocean Observing System (GOOS) and the Group on Earth Observations Biodiversity Observation Network (GEO BON), respectively (Pereira et al., 2013; Miloslavich et al., 2018). The synergistic combination of these frameworks represents an exceptional opportunity to boost marine biodiversity and ecological processes monitoring, directly funneling data and observations within decision-making processes and thoroughly depicting the status of the aquatic environment at multiple spatial scales (Zilioli et al., 2021; Satterthwaite et al., 2021). However, the advancement and implementation of these frameworks, despite urgent, are still ongoing with some weaknesses and delays (Borja et al., 2010; Rouillard et al., 2018; Manea et al., 2021). If EU directives do not provide Member States (MSs) with clear guidelines, for instance, on baseline and threshold values against which to assess the status of the environment (Dodds et al., 2010; Fraschetti et al., 2022), leaving them free to detail how to implement their recommendations, MSs fail to establish integrated monitoring strategies transnationally to achieve directives’ goals (Cavallo et al., 2019). A detailed analysis of the lists of monitoring variables indicated by these legal instruments has also highlighted their shortcomings in accounting for ecological processes and a general mismatch between them that hinders data comparison and sharing and the building up of an integrated approach to monitoring (Manea et al., 2020a). As for the EOV and EBV frameworks, these are now under development (Schmeller et al., 2017; Estes Jr et al., 2021), with some monitoring variables (i.e., the emerging ones) still to be defined. Again, these essential variables differ from those adopted by EU legislations, a fact that further limits monitoring frameworks’ integration (Cavallo et al., 2019).
Despite these limitations, all these initiatives and their harmonization are strongly promising and must be supported. Being conceived for acting at different spatial scales, from regional/national (WFD and MSFD) to global (EOVs and EBVs), monitoring frameworks can provide mutual benefits to each other and support conservation at the transboundary level. EOVs and EBVs need to be built on data collected systematically and comparable at multiple temporal and spatial scales (e.g., national, regional, and global) using current technology and existing efforts (Kissling et al., 2015; Brummit et al., 2017; Bax et al., 2018; Satterthwaite et al., 2021). Any research or observing program, initiative, and infrastructure can contribute to EOV and EBV development, independently by the scale on which it acts (Kissling et al., 2018). For this reason, monitoring initiatives under EU legislation are precious to feed these global strategies. Simultaneously, EOVs and EBVs, by embedding regional/national monitoring data, can directly support the achievement of EU directives’ environmental goals at the regional level.
The integration of the above-mentioned frameworks could be a concrete action to enhance marine conservation at multiple spatial scales, also in the context of protected areas. This is the case of the Natura 2000 (N2K) site network—the main conservation instrument at the EU level—that aims at providing protection to target species and habitats at both local (i.e., site level) and regional (i.e., network level) scales. The network is still under implementation (Action Plan, EC 2017) with the need to enhance its monitoring for both gaining improved knowledge on the status of its protection targets and informing conservation measures. Indeed, many coastal and marine N2K sites are still unmanaged and unmonitored, mainly due to the absence of management plans and financial shortcomings (Claudet et al., 2020). The integration of the existing monitoring initiatives, local, national, and regional, and the transnational data sharing have been underlined as effective to fill these gaps and help N2K network enhancement at the basin scale (Orlikowska et al., 2016; Manea et al., 2020a).
This study provides an ecosystem-based approach for the selection of monitoring variables with multiple aims: (i) the incorporation of ecological processes and their combination with variables of monitoring programs in place; (ii) the advancement of the alignment and harmonization of variables included in existing monitoring frameworks—i.e., WFD, MSFD, EOV, and EBV—through a terminology matching exercise, to support their integration; and (iii) the development of guidelines for the prioritization of variables in order to facilitate the overcoming of monitoring shortcomings and simultaneously inform marine policies. Indeed, the approach is built also to incorporate socio-ecological aspects [i.e., including ecosystem services (ES)], as well as considerations on the benefits of public engagement through citizen science for policies’ implementation. Finally, we present a concrete application of the proposed approach in the context of N2K sites for delivering practical insights on the advantages provided by an integrated and multiscale monitoring framework, and for guiding effective monitoring of specific N2K conservation targets, despite the existing limitations. To this purpose, we selected N2K sites in the Adriatic Sea, where several sites are unmanaged and unmonitored, but where, nonetheless, the identification and expansion of the N2K network is underway (de Francesco et al., 2020). In order to help managers and scientists set and accomplish ad hoc monitoring programs, we focused on two practical case studies, both exemplary for their relevance in the Adriatic N2K sites: a target species—the common bottlenose dolphin, Tursiops truncatus, and a target habitat—seagrass meadows.
The approach and case studies have been developed contextually to the design of the marine ecological observatory ECOAdS (Ecological Observing System of the Adriatic Sea), in the framework of the Interreg Italy-Croatia project ECOSS (ECological Observing System in the Adriatic Sea: oceanographic observations for biodiversity; https://www.italy-croatia.eu/web/ecoss).
Materials and methods
Ecosystem-based approach applied to monitoring variable prioritization
In this study, we use the term monitoring variables interchangeably with monitoring indicators, to refer to the measurements carried out for assessing environmental status and related changes (Hayes et al., 2015). This terminology (“indicator”) is used often in ecology and environmental planning, with many different meanings, definitions, and purposes, so that there is no one-fits-all definition. We refer to one of the broadest and all-encompassing definitions of indicator (hereafter refer to as variable), which is “a component or a measure of environmentally relevant phenomena used to depict or evaluate environmental conditions or changes or to set environmental goals” (Heink and Kowarik, 2010). As such, monitoring variables should also be considered as instruments to be used at the science–policy interface to communicate scientific information to policymakers and non-experts, and to assess progress towards conservation goals (Heink and Kowarik, 2010; McQuatters-Gollop et al., 2019).
To drive variables’ selection and prioritization in the context of conservation management, we relied on the tenets of Ecosystem-Based Management (EBM) as defined in UNEP (2011). EBM is a dominant paradigm in ocean management, being flagged as the most appropriate approach to ensure sustainability in the use of marine resources and in conservation planning (Levin et al., 2009; Long et al., 2015). The strength of EBM derives from the application of an integrated approach that stems from recognizing the intrinsic value of ecosystems, passes through its translation into fundamental benefits for people, and finally returns to nature by considering humans as part of ecosystems, leveraging on a balance between conservation and socio-economic interests. Monitoring implementation has always been recognized as compulsory to allow effective EBM for the support of adaptive and fit-for-purpose management (Arkema et al., 2006; Leslie and McLeod, 2007), and to communicate scientific findings to policymakers (Harvey et al., 2020). We advanced the classical approach to monitoring by incorporating socio-ecological aspects and considering the benefits of public engagement and the relevance of feeding an ES approach. The engagement of citizens in marine monitoring practices has long been explored as an approach able to provide multiple benefits by allowing the collection of extensive and reliable environmental data and favoring citizens’ sensitization towards environmental issues (Bramanti et al., 2011; Hidalgo-Ruz and Thiel, 2013; Hyder et al., 2015; Freiwald et al., 2018; Pocock et al., 2018). The ES approach has been conceived as the main means to translate the scientific knowledge on ecosystem characteristics to policymakers, thus allowing its embedding in decision-making processes (Wong et al., 2015).
We structured our approach in three steps (Figure 1), as described below.
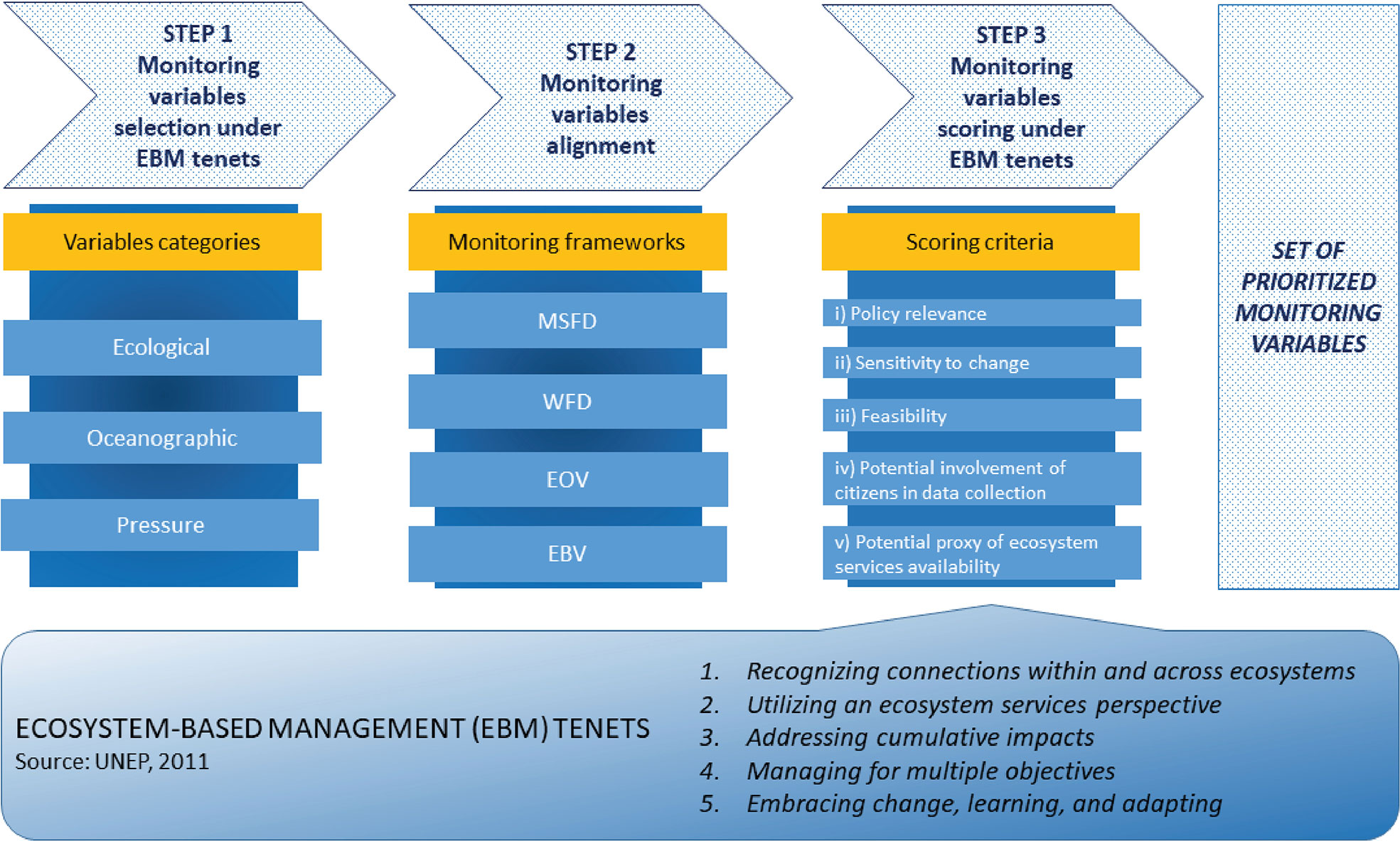
Figure 1 Schematic representation of the Ecosystem-Based Management (EBM) approach proposed to prioritize and distill the variables to monitor the status of defined conservation targets. The monitoring variables are divided into three categories: ecological, oceanographic, and pressure. Their alignment is against the variables listed in four monitoring frameworks: Marine Strategy Framework Directive (MSFD), Water Framework Directive (WFD), Essential Ocean Variables (EOVs), and Essential Biodiversity Variables (EBVs). Their prioritization is based on a scoring approach under five scoring criteria, adapted from Schmeller et al. (2017).
Selection of monitoring variables
The first step foresees the establishment and selection of monitoring variables, which were divided into three categories. The first one is that of ecological variables, i.e., those specifically addressing ecological processes and that can be defined as “measurable characteristics of the structure, composition, or function of ecological systems” (NRC, 2000; Niemi and McDonald, 2004). Indeed, to promote effective conservation of habitats and species, the monitoring and conservation of ecological processes is crucially important (EPA-US, 1999; Williams et al., 2018). Monitoring practices should go beyond the mere description of species and habitat structure, and the protection of ecological processes should be systematically translated into explicit conservation actions (e.g., Klein et al., 2009; Chamberlain et al., 2022). The second category concerns oceanographic variables, which depict physical oceanographic processes that regulate trend, transport, and flux of water, substances, and organisms in the marine system (such as current patterns, tidal and waves dynamics, temperature, light, and turbidity; Carr et al., 2011). The third category is represented by pressure variables, linked to any anthropogenic pressure defined as biological, chemical, or physical agent produced and/or exerted by one or more human activities that elicit an effect and impair the environmental conditions (Stelzenmüller et al., 2018). These are necessary to inform the effectiveness of existing management measures and the setting up of future ones. Indeed, monitoring variables also enable factors to individuate possible pressures acting on conservation targets and lead to any variations from their original status. In risk-based assessment procedures for management, monitoring variables should allow differentiation between natural disturbance and human-induced pressures (Stelzenmüller et al., 2018). Additionally, monitoring anthropogenic impacts has been underlined as essential to assess conservation performances also in marine protected areas (Dunham et al., 2020).
In this step, the variables must be selected to answer the need of considering ecological connectivity and the interconnections among species and between species and their environment in monitoring and conservation practices (Magris et al., 2014), thus relying on EBM principle 1 “Recognizing connections within and across ecosystems”. To guide the selection, we focused on seven key ecological processes described by Bennet et al. (2009) (Table 1): (i) climate, (ii) hydrology, (iii) interactions between organisms, (iv) movement of organisms, (v) spatial/temporal variation in primary productivity, (vi) natural disturbance regime, and (vii) formation of biophysical habitats. The selection of pressure variables must address the sources of pressure that might affect conservation targets to support EBM principles 3 “Addressing cumulative impacts” and 4 “Managing for multiple objectives”, the latter referring to trade-offs between human uses and conservation objectives.
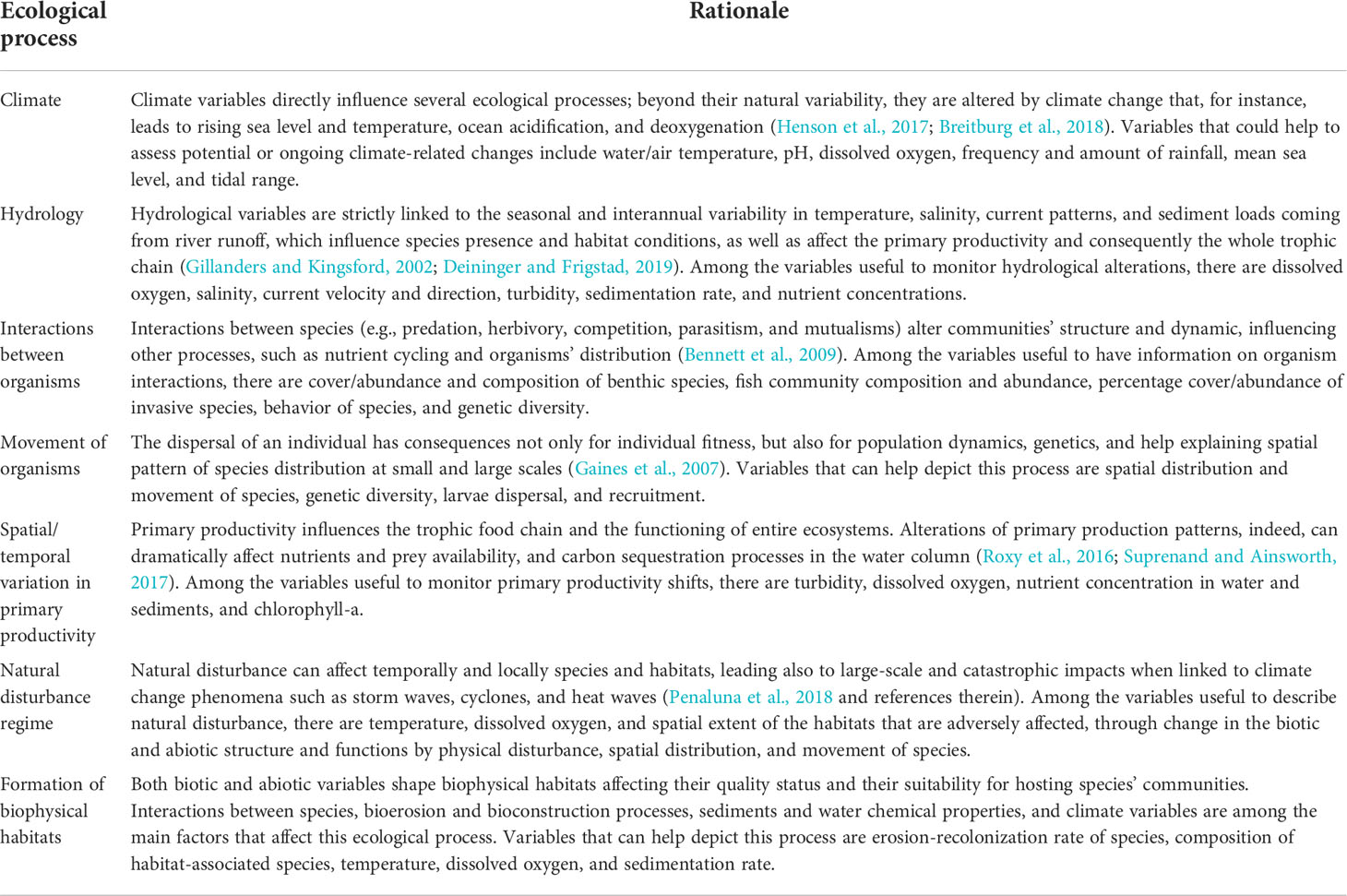
Table 1 Ecological processes to guide monitoring variables’ selection, modified after Bennet et al. (2009).
Alignment of variables with WFD, MSFD, EOV, and EBV frameworks
The second step foresees the alignment of the variables selected in Step 1 with those listed in the WFD, MSFD, EOV, and EBV frameworks through a terminology matching exercise, to allow the definition of possible correlations among them. For both directives and EOVs, the alignment consisted in a careful match among the different frameworks’ monitoring variables. In order to respect the concept driving EBVs’ foundation, which are conceived as collectors that integrate diverse raw data (Brummit et al., 2017; Kissling et al., 2018; Zilioli et al., 2021), the alignment with EBV has been accomplished by considering the potential of a specific variable to contribute to the EBV framework, accounting for the different levels of biodiversity organization (e.g., genetic, species, community, and ecosystem).
Scoring of the selected monitoring variables
The third step consists in a variable scoring exercise based on a set of criteria, adapted by those proposed by Schmeller et al. (2017) for EBV definition, and recalling EBM principles 2 “Utilizing an ecosystem service perspective” and 5 “Embracing change, learning and adapting”. The five prioritization criteria are as follows:
i) Policy Relevance (PR) = variable that falls within the list of indicators of the WFD and MSFD directives and/or contributes to the EOV and/or EBV frameworks.
Scoring modality: 0 none of these frameworks; + at least one of the frameworks; ++ at least one directive plus EOVs and/or EBVs, or both directives; +++ both directives and EOVs and/or EBVs.
ii) Sensitivity to Change (STC) = variable able to communicate changes in time.
Scoring modality: 0 no or hardly changes can be detected; + variable that takes 10 or more years to show changes; ++ variable that reveals changes within 9 years; +++ variable detecting changes in less than 2 years.
iii) Feasibility (F) = it depends on the presence of established monitoring methods and its cost-effectiveness, i.e., the effort of time to collect samples or data and the extension of the area that can be covered by the monitoring in relation to the costs to support the activity. This criterion must be considered in the context and for the purpose of the monitoring.
Scoring modality: 0 not feasible; + there is a scientifically proven method, but it requires great effort and it is not cost-effective; ++ there is a scientifically proven method, no great effort is required, but it is not cost-effective, or the contrary; +++ there is a scientifically proven method, no great effort is required, and normal resource use (human and financial) is sufficient.
iv) Potential for Citizens Involvement in data collection (PCI) = considered as an approach able to support the collection of extensive and reliable environmental data and to enhance citizens’ engagement in monitoring and conservation issues.
Scoring modality: 0 NO; 1 YES.
v) Potential Proxy of Ecosystem Services availability (PES). This criterion was adopted only for ecological and oceanographic variables, as the pressure variables are not directly linked to ecosystem components, and therefore unsuitable to represent proxies of ES provisioning.
Scoring modality: 0 NO; 1 YES.
Application of the ecosystem-based system of variables in the Adriatic N2K sites
In this study, as exemplary cases, we considered six N2K sites of the northern Adriatic (Figure 2), which were selected within the Italy-Croatia Project ECOSS (Markov and ECOSS Partnership, 2019; Ciriaco et al., 2019; Goleć and ECOSS Partnership, 2020; Miočić-Stošić et al., 2020; https://ecoads.eu/sites/natura2000/). These sites can be considered fairly well representative of the northern Adriatic N2K network and are under the management responsibility of the project partners, who could therefore provide first-hand information. We focused on two conservation targets as exemplary case studies for the application of the variable system: (i) the common bottlenose dolphin (Tursiops truncatus), a species under protection in Cres-Lošinj, Viški akvatorij, San Pietro e Bardelli, and Tegnùe di Chioggia, and (ii) seagrass meadows, under protection in Malostonski zaljev and in the two Delta del Po sites. In the Delta del Po, seagrasses used to thrive, but their presence has not been observed in recent years. However, reintroduction activities are planned and seagrass monitoring will hopefully be needed again in the near future. The selection of these two case studies, as examples of monitoring targets, was primarily driven by the fact that they can be considered emblematic of transboundary contexts, such as that of the Adriatic Sea, and for the availability of local expertise and scientific knowledge, which allowed applying pragmatically the proposed variables’ prioritization approach. The common bottlenose dolphin is the only cetacean species regularly inhabiting the entire Adriatic Sea. Basin-wide aerial surveys revealed the highest relative density of this species in the offshore part of the northern Adriatic (Fortuna et al., 2018), whereas boat-based studies describe their regular presence in coastal regions (Genov et al., 2008; Holcer, 2012; Pleslić et al., 2015; Rako-Gospić et al., 2017; Bonizzoni et al., 2021; Pleslić et al., 2021). Even though spatially distinct subpopulations with various levels of site fidelity have been observed, these are not isolated as some individuals were found to migrate between distant areas (Pleslić et al., 2019). The protection of this highly mobile species that moves freely across the whole northern Adriatic, regardless of geopolitical borders, has to be addressed regionally (Fortuna et al., 2018). Therefore, the monitoring strategy and the data collection to assess its conservation status should be established and shared transnationally. The same is true for seagrass meadows, which in the area are mainly represented by Cymodocea nodosa, Zostera marina, Zostera noltii, and Posidonia oceanica, with the latter being distributed in the eastern Adriatic (Giannoulaki et al., 2013). This habitat is recognized as a key conservation target for its ecological role in supporting high biodiversity and for the many ES it provides (e.g., food, carbon sequestration; Duarte and Krause-Jensen, 2017; Unsworth et al., 2019). Its conservation should be implemented at the transboundary level to avoid a patchy distribution that would lead to an important loss of its ecological role. Additionally, connectivity among seagrass populations through fruit dispersal might be a potential reproductive strategy to favor meadows replenishment and seagrass genetic diversity (Mari et al., 2020).
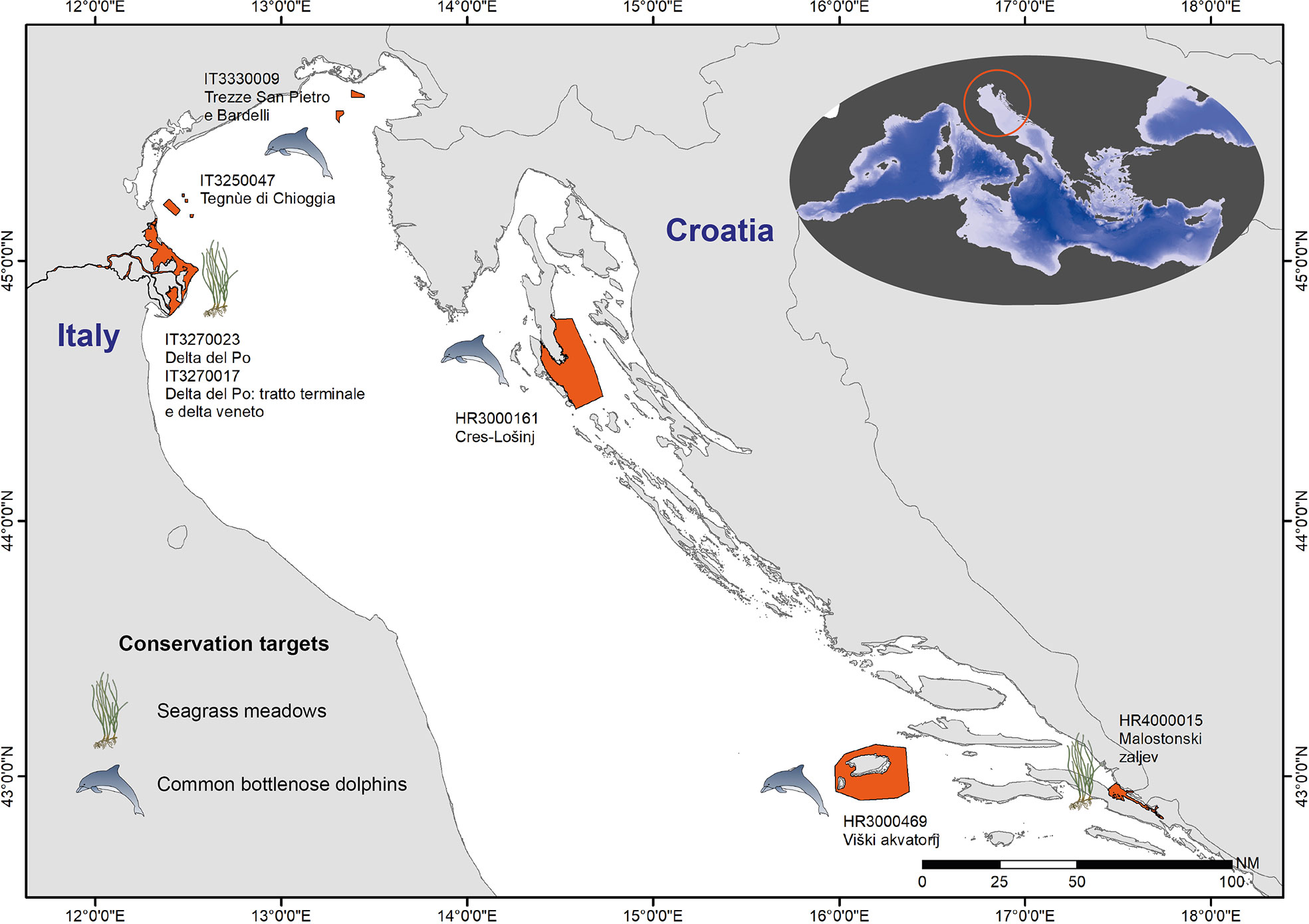
Figure 2 The six selected N2K sites (in orange) and the conservation targets (common bottlenose dolphin and seagrass meadows).
Selection of monitoring variables for the Adriatic N2K conservation targets
In the case of N2K sites, conservation targets are declared in the form of species and habitats, and management levels extend over multiple spatial scales, from local (i.e., at the level of single site), to national, up to regional (i.e., at the level of the entire network). Thus, the variables’ selection process started from the explicit conservation objectives defined by the considered N2K sites. In our case studies, the selection of ecological and oceanographic variables was based on the environmental characteristics of the northern Adriatic N2K sites and reflected the ecology of the two conservation targets, the common bottlenose dolphin and seagrass meadows. Additionally, we integrated the monitoring of anthropogenic impacts (Table 2). The pressure variables’ selection was based on the specific management context of each N2K site depending on the present human activities and related pressures. The all selection step passed through a participative process with N2K sites’ managers and experts involved in monitoring activities, and was further fed by an ad hoc literature review. Indeed, when available, studies focused on the two specific conservation targets in the area were used.
Alignment of variables with the WFD, MSFD, EOV, and EBV frameworks
The selected variables were aligned with those listed in official documents and technical reports of the different monitoring frameworks (WFD Common Implementation Strategy, 2003-2004; EC, 2019; EOVs list within the available technical reports at https://www.goosocean.org/index.php?option=com_content&view=article&id=170&Itemid=114; EBVs list at https://geobon.org/ebvs/what-are-ebvs/). For the WFD and MSFD, monitoring variables were aligned starting from the harmonization carried out in Manea et al. (2021) as a first contribution of ECOAdS to an integrated and transboundary monitoring framework.
Scoring of monitoring variables
The scoring exercise was carried out based on the available information on N2K sites’ monitoring activity collected through the participative process with N2K sites’ managers and experts, and on an ad hoc literature review of studies reporting empirical results coming from both experimental and monitoring activities, and eventually from descriptions of monitoring approaches and data collection. To complete the whole approach, we finally verified which of the variables that should be specifically monitored by N2K managers, according to each site-specific conservation objectives, are actually monitored.
Results
The resulting lists of the selected ecological, oceanographic, and pressure variables for the common bottlenose dolphins and seagrass meadows in the northern Adriatic N2K sites are reported in Tables 3 and Table 4. The complete lists of references that support the variables’ selection are reported in the Supplementary Material.
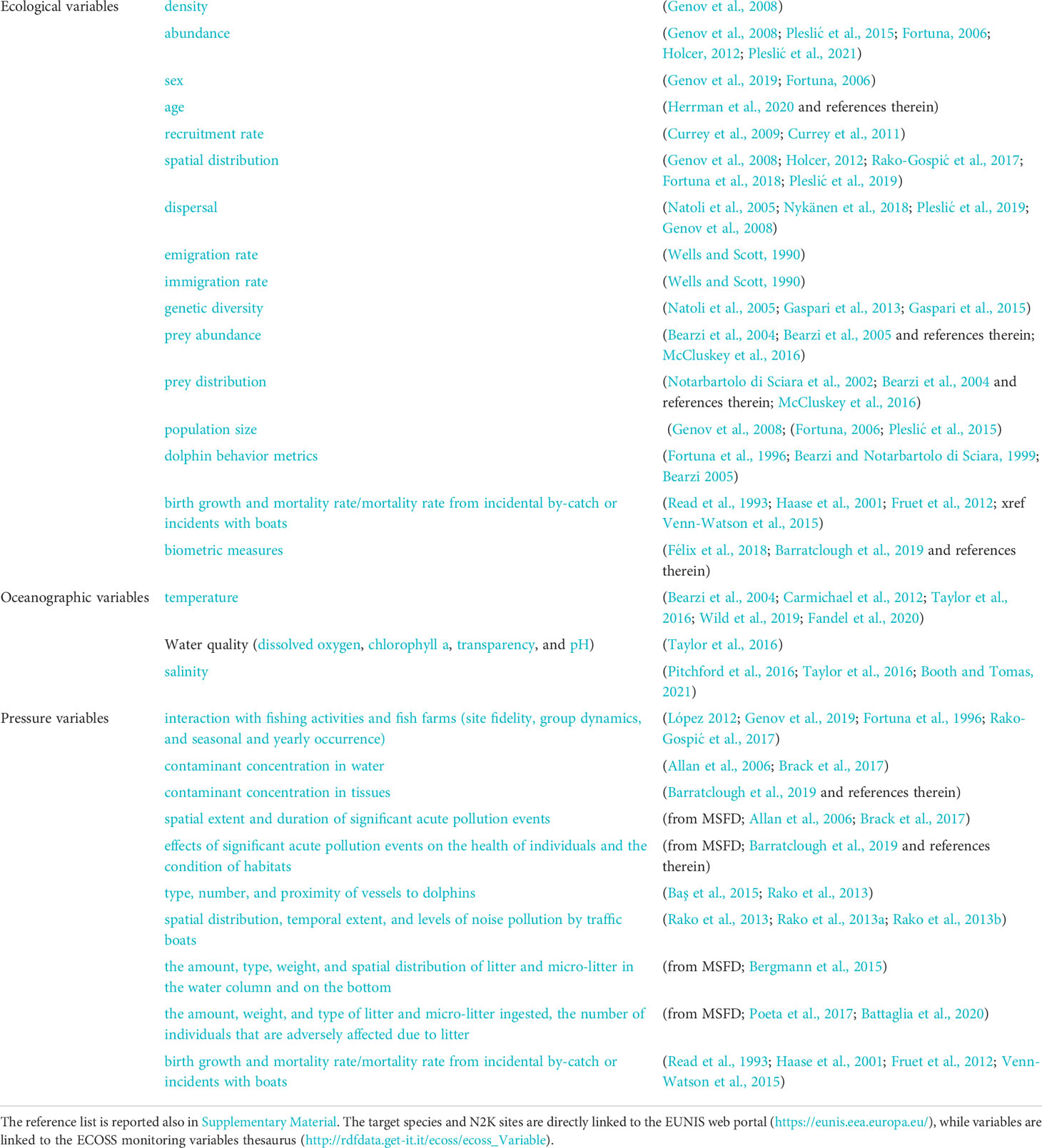
Table 3 List of monitoring variables for common bottlenose dolphin—Tursiops truncatus (Montagu, 1821), species under protection in Cres-Lošinj, Viški akvatorij, Trezze San Pietro e Bardelli, and Tegnùe di Chioggia N2K sites.
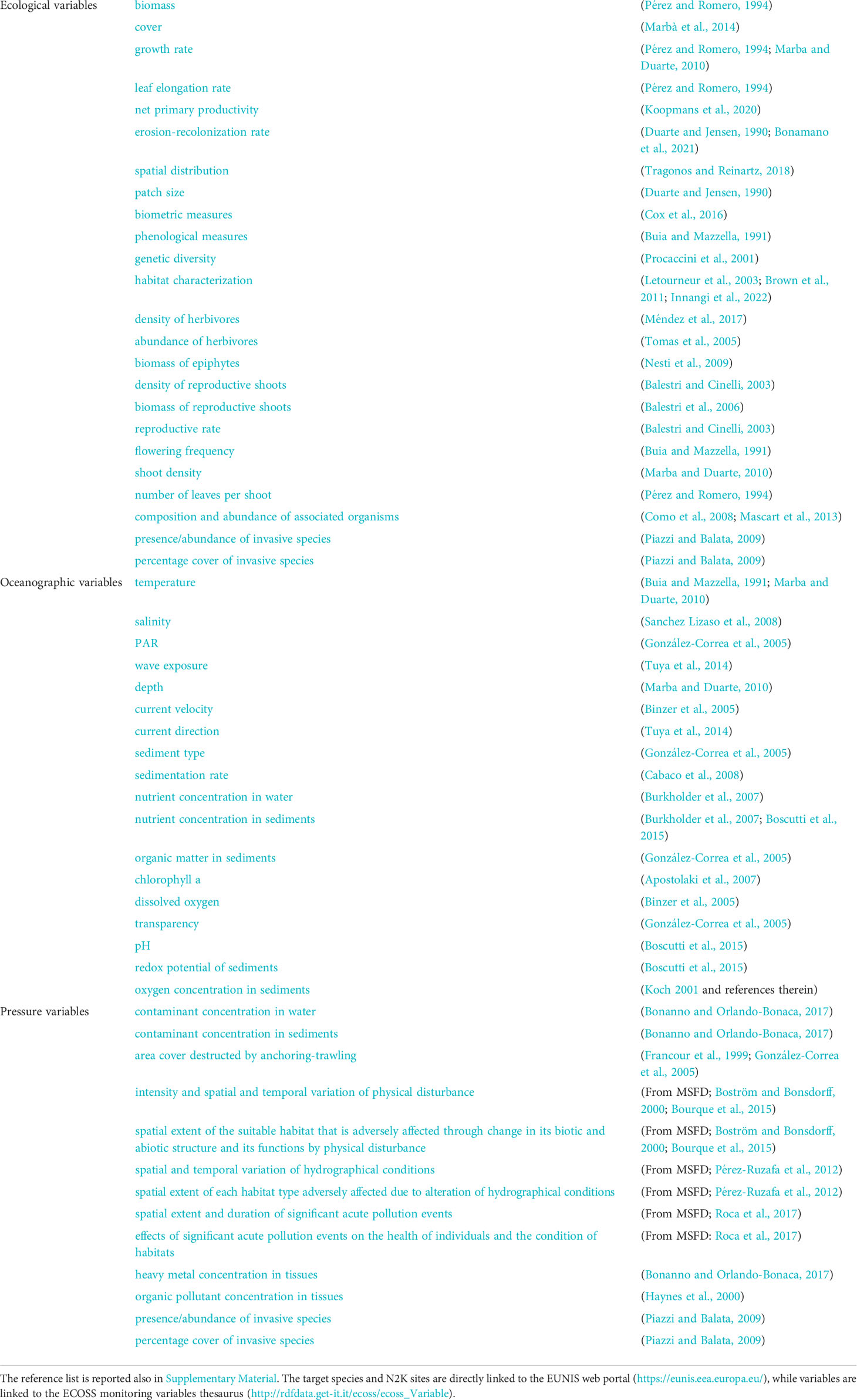
Table 4 List of monitoring variables for seagrass meadows, in particular of Cymodocea nodosa (Ucria) Asch., Posidonia oceanica (L.) Delile, Zostera noltii Hornem., Zostera marina L., habitat under protection in Malostonski zaljev and Delta del Po: tratto terminale e delta Veneto sites.
Monitoring variables’ alignment and scoring
Monitoring variables’ alignment among MSFD, WFD, and EVs for both common bottlenose dolphin and seagrass meadows, and the scoring results are reported in Figures 3–5, respectively. The complete terms of the aligned variables are reported in Tables S1 and S2, related to Figures 3 and Figure 4, respectively. The complete lists of references used to support variables’ selection and scoring are reported in the Supplementary Material. Tables S3 and S4 report the information on which variables are actually monitored in the selected N2K sites, where the target species and habitat are present.
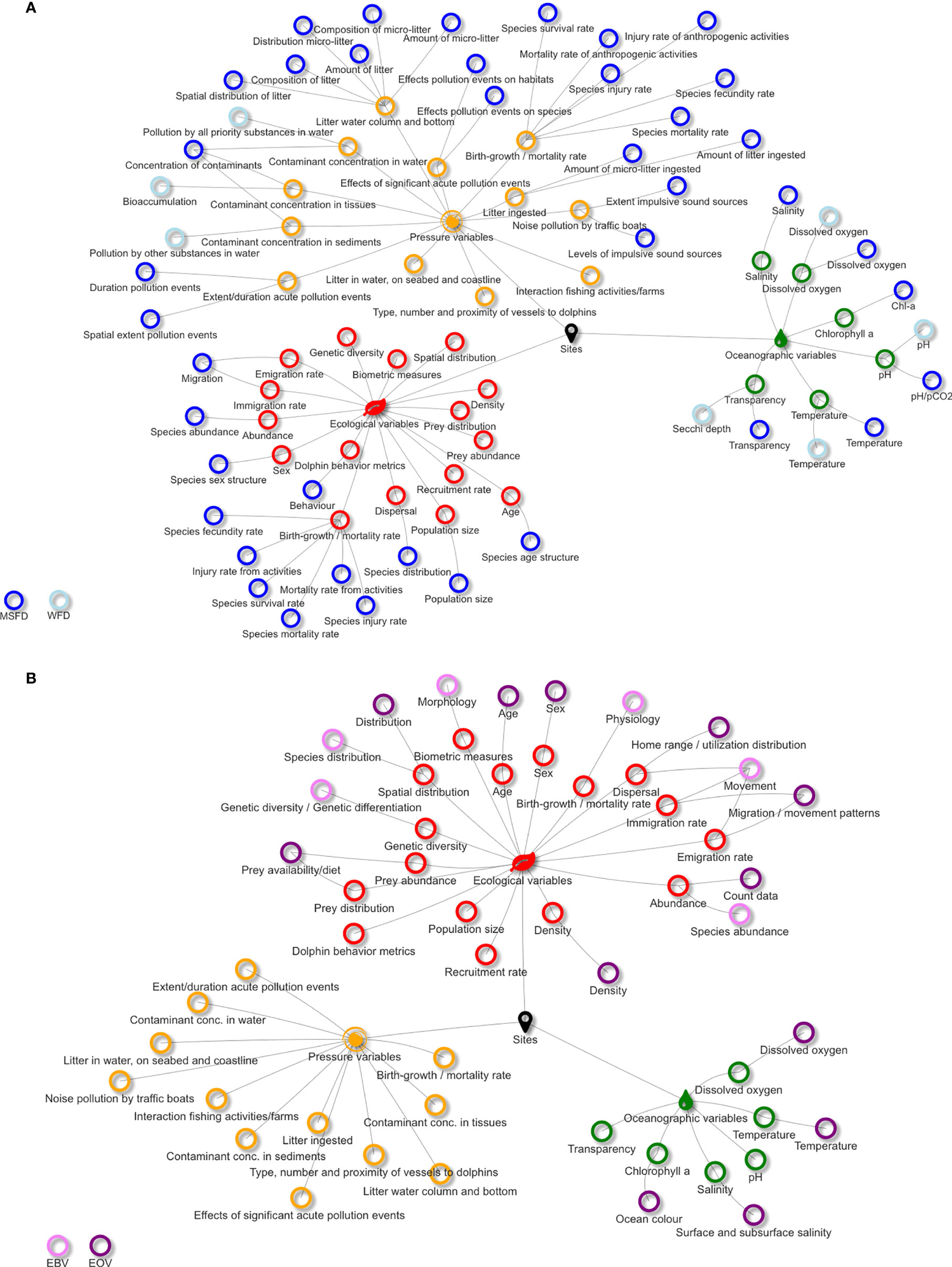
Figure 3 Alignment between ecological, oceanographic, and pressure variables to monitor the common bottlenose dolphin with Water Framework Directive (WFD) and Marine Strategy Framework Directive (MSFD) variables (A), and Essential Ocean Variables (EOVs) and Essential Biodiversity Variables (EBVs) (B).
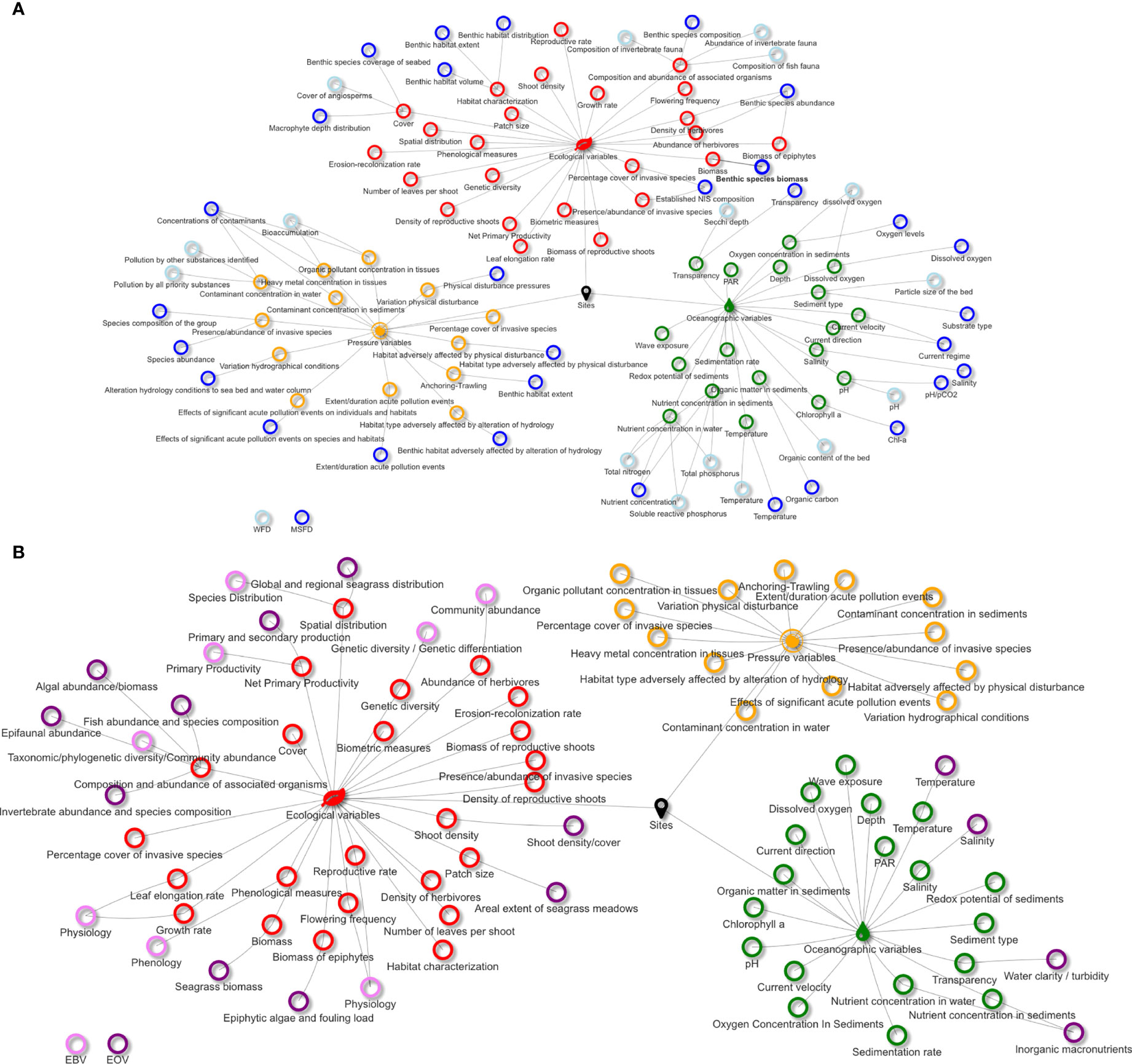
Figure 4 Alignment between ecological, oceanographic, and pressure variables to monitor seagrass meadows with Water Framework Directive (WFD) and Marine Strategy Framework Directive (MSFD) variables (A), and Essential Ocean Variables (EOVs) and Essential Biodiversity Variables (EBVs) (B).
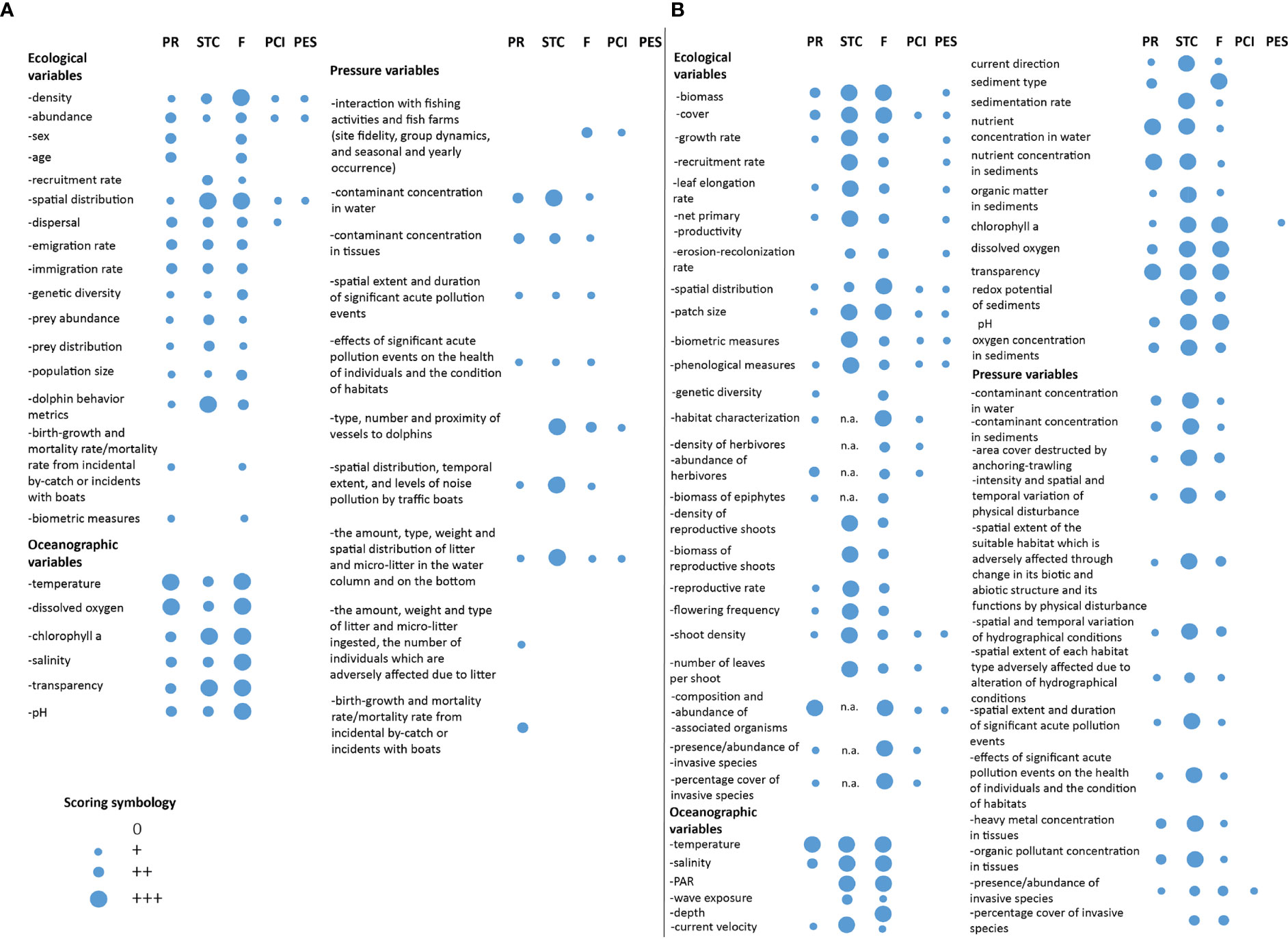
Figure 5 Scores assigned to the ecological, oceanographic, and pressure variables to monitor the health and conservation status of (A) the common bottlenose dolphin, Tursiops truncatus, in Cres-Lošinj, Viški akvatorij, San Pietro e Bardelli, and Tegnue di Chioggia N2K sites, and (B) seagrass meadows in Malostonski zaljev and Delta del Po N2K sites. PR = Policy Relevance, STC = Sensitivity to Change, F = Feasibility, PCI = Potential for Citizens Involvement, PES = Proxy for Ecosystem Services assessment, n.a. = information not available/sufficient.
Common bottlenose dolphin
Around 56% of ecological, 40% of oceanographic, and 70% of pressure variables were not shared between EU directives and EV frameworks, and only three variables did not match any monitoring framework. Among the ecological variables, only “abundance”, “dispersal”, “emigration rate”, and “immigration rate” matched all monitoring frameworks with the exception of the WFD that does not focus on pelagic species living offshore, i.e., outside WFD range of action. Regarding the oceanographic variables, four out of the six listed matched one or both directives and EOVs (exceptions were “pH” and “transparency”, which were shared only between MSFD and WFD). EBVs do not consider oceanographic variables, as the framework is strictly focused on the biological ones. Almost none of the pressure variables matched the WFD and EV frameworks, while good matching was found between most of them and the MSFD.
For what concerns the ranking, among the ecological variables, “density”, “abundance”, and “spatial distribution” were those with the highest scores. Their feasibility score was based on the monitoring approaches adopted in the area, i.e., aerial and boat-based surveys (Fortuna et al., 2018; Bearzi et al., 2021). Furthermore, these variables might be monitored also by citizens (Embling et al., 2015; Giovos et al., 2016), as already happens in certain Mediterranean areas (Ricci et al., 2018; Alessi et al., 2019). “Spatial distribution” was also found to be one of the most sensitive variables to changes, together with “dolphin behavior metrics”, potentially representing appropriate early-warning signals of the status of the species. Indeed, common bottlenose dolphins behave differently depending on the characteristics and geography of the area, the presence of human activities, the trophic niche occupied, and the season, being highly adaptable and opportunistic (Bearzi et al., 2009). Although “sex” and “age” fall in the list of both the MSFD and the EV framework and are monitored in Croatian N2K sites, their scores were low due to the difficulty in detecting changes with time, and because they cannot be easily tracked by citizens and are poorly suitable to ES assessment analyses. For the same reasons, “genetic diversity” has a low score, being also considered only by EBVs. Also, “prey abundance” and “prey distribution” obtained low scores; they are absent from both directives and their monitoring is not advantageous in terms of cost–benefit ratio, although better information on the bottlenose dolphins’ diet is necessary (Ricci et al., 2021). Furthermore, both these variables cannot be monitored by citizens, or used for ES assessment. All these low score variables are not monitored in Croatian N2K sites.
The oceanographic variables all scored high because of the matching among monitoring frameworks, the high sensitivity to changes, and the monitoring feasibility, mainly thanks to the real-time data collection from the fixed observing systems present in the area of study (Ravaioli et al., 2016; Manea et al., 2020a) and to satellite observations (Groom et al., 2019; Medina-Lopez and Ureña-Fuentes, 2019).
The pressure variables found a good match only with the MSFD. Contaminants’ concentration and marine litter amount in environmental matrices were the ones with the highest scores as these can change even in a short time and are feasible to monitor through agreed and widespread methodologies and protocols (EC report, 2013). Marine litter together with dolphin proximity to vessels are variables that can be monitored also by engaging citizens. On the contrary, the monitoring of the concentrations of plastic and contaminants in tissues and organs is difficult because it can only be carried out on stranded or captured animals; thus, these analyses cannot be planned in advance or carried out systematically. Although the ecological and pressure variable “birth growth and mortality rate/mortality rate from incidental by-catch or incidents with boats” matches the MSFD, it had the lowest scores mainly because carcasses usually sink and are not visible and by-catch is difficult to estimate from dead and stranded individuals (Bearzi et al., 2009). The variables “Interaction with fishing activities and fish farms” and “proximity to vessels” are monitored in Cres-Lošinj and Tegnùe di Chioggia and could be monitored with the help of citizens, for instance, fishermen and fish farmers, as well as recreational boaters. Noise pollution and its effect on common bottlenose dolphins emerged as one of the most sensitive variables, since it is directly linked to the level of vessel traffic. As far as we know, this variable is monitored only in the N2K site Cres-Lošinj.
Seagrass meadows
We found that ca. 21% and 22% of selected seagrass ecological and oceanographic variables, respectively, did not match any of the considered monitoring frameworks. Among the ecological and oceanographic variables, ca. 67% and 44%, respectively, did not contemporarily match directives and EVs. Only one pressure variable did not match that of the MSFD, while almost all did not match that of the WFD and EVs.
The ecological variables aligned with the directives were “biomass”, “cover”, “abundance of herbivores”, and “composition and abundance of associated organisms”. The first two were scored high for their high sensitivity to changes, monitoring feasibility, and suitability for ES studies. According to Boudouresque et al. (2009) and Nordlund et al. (2018), different seagrass species present diverse morphological and physiological traits and can answer differently to the same stressor; nonetheless, we found that more than half of the ecological variables can be uniformly considered potential early-warning signals. For instance, a 3-month experiment of temperature variations to test for warming effects on P. oceanica and C. nodosa was enough to appreciate changes in growth rates, leaf formation, and elongation rates and biomass (Olsen et al., 2012), and 6 weeks were enough to induce P. oceanica flowering (Ruiz et al., 2018). Variation in seagrass recruitment rates can be detected within 18 months, which include the period that falls between seed germination and successful seedling establishment (Pereda-Briones et al., 2020). Spatial distribution and especially patches’ size, depending on their extent, can vary appreciably even yearly. Shoot density was found to be a generalized variable for monitoring a wide range of stressors (Roca et al., 2016). However, among these variables, only “cover” is regularly monitored in Malostonski zaljev. Epiphyte biomass is regulated by a multiplicity of factors—their diversity, interactions with the environment, larvae and propagule availability, and the lifetime of the colonized seagrass leaf (Borowitzka et al., 2007). For this reason, estimating the sensitivity of this monitoring variable is difficult. This is also valid for the species associated with seagrass meadows, whether they are herbivores, invasive, or others.
Measurement of seagrass ecological variables at the scale of the N2K site is mainly carried out through SCUBA dive surveys and underwater videos, which are the most effective approaches for their accuracy, even if they can hardly be applied on a large scale. The monitoring feasibility of most of the variables was scored high because we considered the potential of adopting remote sensing techniques, which are reliable approaches to collect information on seagrass meadows (e.g., biomass, cover, spatial distribution, patch size, and habitat characterization), and complementary with in situ data collection (Paul et al., 2011; Duffy et al., 2019).
We found more than half of ecological variables being useful for seagrass ES assessment (e.g., biomass, cover, and shoot density; Ruiz-Frau et al., 2017; Hossain and Hashim, 2019; de los Santos et al., 2020), and also potentially measurable by citizens (Jones et al., 2018; Mannino and Balistreri, 2018; Smale et al., 2019). An example is the Community Seagrass Initiative (CSI), a citizen science project carried out by the National Marine Aquarium in Plymouth (UK), during which citizens were involved in data collection to assess the distribution and status of Z. marina (Smale et al., 2019). In relation to invasive species monitoring, an important citizen science contribution has been reported in the marine protected area of Egadi Islands (Mannino and Balistreri, 2018).
Oceanographic variables, as previously reported for the common bottlenose dolphin, were scored high also for seagrass because they matched diverse monitoring frameworks, and presented high sensitivity to changes and monitoring feasibility. The monitoring feasibility of hydrographic conditions scored high thanks to the presence of fixed buoys or platforms in the area for routine studies and real-time collection of oceanographic parameters. These systems largely contribute to detect any hydrographical variations at large scale that can affect N2K sites.
Pressure variables showed quite similar scores. Contaminant and pollutant concentrations in seagrass tissues and sediments were the only ones matching both the MSFD and the WFD variables. In Malostonski zaljev, only biological and physical/mechanical disturbances are monitored, while pollutants and contamination events are not.
Discussion
Our ecosystem-based system of monitoring variables has been conceived as a tool that can favor the setting of integrated and harmonized local strategic monitoring plans, track the status of habitats and species under protection, by considering driving ecological processes, and contribute effectively to large-scale monitoring. We explored the potential of an integrated approach, which combines diverse monitoring frameworks (i.e., MSFD, WFD, EOV, and EBV) for selected variables and can be able to deliver information needed to boost marine conservation at multiple spatial scales. This could help to define appropriate, focused, and feasible monitoring efforts by converging on a limited number of variables and containing the financial costs, without hindering the achievement of monitoring and conservation objectives. Additionally, it could favor the harmonization and integration of monitoring practices, remedying the current fragmentation of existing programs (Zampoukas et al., 2013).
We tested this approach to support N2K sites and their networking, one of the main European missions to contribute to global conservation needs (EC, 2017). We focused on the northern Adriatic Sea, specifically in the area under the jurisdiction of Italy and Croatia, this work being one of the outcomes of the Interreg-Italy-Croatia project “ECOSS” (https://www.italy-croatia.eu/web/ecoss), and on two significant conservation targets, the species T. truncatus (the common bottlenose dolphin), and the habitat of seagrass meadows (mainly dominated by C. nodosa, Z. noltii, and P. oceanica) as exemplary case studies of selected Adriatic N2K sites. Although our study is based on test conservation targets and selected north Adriatic N2K sites (therefore cannot be considered exhaustive for the whole Adriatic basin), the proposed integrated and multi-scale approach might potentially help to set up frameworks of variable selection in the wider Adriatic macroregion, and in other marine areas of the Mediterranean Sea and beyond. Actually, the approach can be tested and applied to guide the monitoring of other conservation tools, both legally binding and not, such as Large Scale Marine Protected Areas (LSMPAs), Specially Protected Areas of Mediterranean Importance (SPAMI), Ecologically and Biologically Significant Marine Areas (EBSAs), Important Marine Mammal Areas (IMMAs), and Cetacean Critical Habitats (CCHs) specifically for cetaceans (Carlucci et al., 2021). Indeed, it can be of particular relevance for these conservation tools with large spatial footprints, with no clear national commitments if they fall in international waters or are shared between different countries, thus making their monitoring really challenging (O’Leary et al., 2018; Costello and Molina, 2021).
In the proposed three-step approach, we defined three categories of variables, i.e., ecological, oceanographic, and pressure, selecting those that might better depict the complexity of ecological processes (Bennet et al., 2009) and track local pressures and climate change when combined to guide management decisions. Interdisciplinarity in monitoring has been highlighted as crucial to ensure comprehensive observations and understanding of environmental patterns and trends at multiple spatial scales (Benedetti-Cecchi et al., 2018). We support that combining information on biotic, abiotic, and physical elements with those on pressures and their sources helps identify ecosystems’ emerging properties and their possible shifts. Thus, the first step of our approach can be applied to orient the establishment of a first set of variables, among the many, that account for a multiplicity of factors that influence the status of species and habitats.
This preliminary selection might as well inspire an update of the lists of monitoring variables considered in the existing monitoring frameworks. Indeed, the alignment of the selected variables with those required and indicated by the MSFD, the WFD, and the EV frameworks delivered, first of all, insights into the divergences among monitoring frameworks. Main divergences were observed between EU directives and EV frameworks when looking at ecological and pressure variables. In particular, some ecological variables related to the trophic ecology of the common bottlenose dolphin and some related to the physiology and phenology of seagrasses (e.g., growth and leaf elongation rates, and reproduction) matched with EVs, but were neglected by both directives despite being critical for assessing the conservation status of these two targets. The distribution and abundance of common bottlenose dolphins’ prey might not be seen as relevant to affect the distribution and health of this species, which is considered a non-selective feeder, i.e., capable of shifting prey depending on their availability (Holcer, 2012; Gaspari et al., 2015). Instead, monitoring the prey is crucial, since general prey depletion has been recently recognized as a main threat for these animals (Carlucci et al., 2021), especially in the area of study, where an excessive fishing pressure is causing it (Bonizzoni et al., 2021). As for the seagrasses, these have been used for decades as indicators of good environmental status (Lopez y Royo et al., 2010), and decadal data of cover and biomass across the entire Mediterranean recently allowed the assessment of their status and trend at the basin scale (de los Santos et al., 2019); therefore, great merit must be given to the EU legislation that has imposed this kind of monitoring. Nonetheless, in the current context of biodiversity loss, due to rapid climate change and unpredictable effects coming from multi-stressor combinations (Henson et al., 2017; Gissi et al., 2021), anticipatory signals, such as those related to the physiological and phenological status of seagrasses, mostly neglected by the directives, should be prioritized in monitoring practices, to anticipate possible negative shifts of habitats’ conditions. Looking at the pressure variables, they matched almost only the MSFD ones. Monitoring pressures is fundamental for effective conservation planning and management (Dunham et al., 2020), particularly in N2K sites, whose management plans entail socio-economic objectives together with those of conservation (Kati et al., 2015). In general, ecological variables best inform about pressure effects on species and habitats, but they can often be measured once the impact has already occurred. Thus, only waiting for related signals might be insufficient on the temporal scale for taking decisions on the application of mitigation or adaptation measures. Instead, the adoption of a set of composite indicators, as the one we proposed, which considers oceanographic and pressure variables together with the ecological ones, would be most effective to correlate diverse measurements (Schmeller et al., 2017), enabling one to detect impacts earlier and inform anticipatory conservation actions.
Despite these drawbacks, we evidenced that the majority of the variables matched at least one monitoring framework among directives and EVs, bringing out the potential of their integration. This implies that part of them is currently or might be monitored through EU mandatory programs at the local and national level, as well as in N2K sites. This will be fully achievable if coordinated efforts are put in place to arrange and activate joint monitoring programs that will also include N2K sites, currently limited in terms of conservation planning and dedicated resources (Claudet et al., 2020). Simultaneously, the variables shared among directives and EVs could converge to feed the global strategies, while those not yet monitored under national law could be integrated, both to make them most effective and to further support the EVs.
The prioritization exercise allowed us to reduce the number of variables by distilling the ones that emerged as priority to be able to better respond to the ecosystem-based criteria on which our approach is based. These criteria respond to the need to both describe ecological processes and communicate the information acquired through monitoring toward management policies that are directly linked to the directives and regional strategies, or that are appropriate for ES assessments. Furthermore, the application of an EBM approach for the prioritization of the variables helped to define possible advantages and disadvantages in their monitoring.
The concrete application of the proposed approach through case studies allowed us to provide insights and suggestions to guide variable selection based on the context and monitoring objectives of the selected N2K sites, and on the knowledge provided by the local experts. The latter was an indispensable component in the implementation and success of our exercise, and we emphasize the importance of engaging the local experts in each monitoring and management plan. Their contribution allowed us to create a narrower variables’ set of potential priority to facilitate overcoming difficulties and limitations in their choice (Hayes et al., 2015) and data collection. For instance, we found the ecological “abundance and distribution” metrics to be the ones with the highest priority for monitoring the common bottlenose dolphin. Related data have been collected for some decades in the whole study area and at the level of N2K sites to predict critical zones for the conservation of the species (Pleslić et al., 2015; Fortuna et al., 2018; Pleslić et al., 2019; Farella et al., 2020; Bonizzoni et al., 2021; Pleslić et al., 2021) and to inform the recent designation of a new N2K site (IT3270025 “Adriatico Settentrionale Veneto-Delta del Po”). These monitoring efforts are carried out by local associations and institutions and, for their fundamental value, should be long-lasting and supported by constant and appropriate funding mechanisms, which at the moment are lacking. Despite being identified as more difficult to monitor, we recommend adding to the future monitoring plans some variables that could give important indications about ecological connectivity, i.e., dispersal, migration patterns, and genetic diversity. Indeed, distinct subpopulations of common bottlenose dolphin have been detected to inhabit the Adriatic Sea, and this is relevant to guide management actions aimed at avoiding the genetic erosion of this species (Gaspari et al., 2015). Furthermore, the monitoring of these variables would feed the EV frameworks.
Regarding seagrass meadows, we found that in situ data collection of ecological variables is the most reliable strategy, but it is time-consuming and with limited potential to be upscaled also at the level of the entire N2K site. However, new technologies and approaches are advancing, becoming powerful tools to extend the monitoring of biological and ecological metrics, which is a necessity for effective ocean observation (Benedetti-Cecchi et al., 2018). Opportunities to rely on remote data collection with good resolution combined with field data to monitor seagrasses have been described along with useful recommendations to harmonize approaches at large scales (Duffy et al., 2019). These might be considered at the scale of the N2K network and of the whole Adriatic Sea, to extend the monitoring range and avoid further loss of this habitat.
Among the pressure variables, we recommend the monitoring of pollution and contamination events, since habitat deterioration due to urbanization and agricultural activities is one of the main causes of biodiversity loss in coastal areas (Todd et al., 2019). However, land-based sources of pollution potentially able to impair the status of marine species and habitats under protection are many and can act both locally and at a distance from the conserved area, impacting even offshore and remote zones (Kidd et al., 2019; Manea et al., 2020b). In such a context, monitoring should be used as an instrument of support for preventive and integrated coastal management, considering the land–sea continuum (Eger et al., 2021). Finally, we assessed the monitoring of oceanographic variables to be highly feasible. Indeed, the considered sites fall within a marine area that is constellated by multiple fixed-point observing systems that provide real-time data of diverse oceanographic parameters in both coastal and off-shore areas, which should be shared and integrated also at the transnational level (Ravaioli et al., 2016; Manea et al., 2020a). Additionally, the area is covered by monitoring initiatives beyond the ones mandated by law, such as the Italian Long-Term Ecological Research network (LTER-Italy), which works as a collector of long-time series of a variety of ecological data (Pugnetti et al., 2013; Zilioli et al., 2019; Capotondi et al., 2021) that are not provided by N2K monitoring. The integration of these extensive datasets, in both time and space, with those collected by the local agencies under national mandate, and their consideration by N2K sites managers, is advisable and represents an incredible opportunity to benefit from already consolidated monitoring systems able to provide information on both past and present environmental status. Contemporarily, the data already collected in N2K sites should be conveyed together with these datasets to feed the EV frameworks. The concrete application of our approach for the setting up of an ecosystem-based system of variables in the context of N2K sites, therefore, revealed the mutual benefits that can be provided if multilevel monitoring frameworks would be integrated and exploited synergistically by N2K managers. An integrated system would become an available source for N2K site monitoring and contextually local monitoring projects would provide updated data to large-scale monitoring frameworks.
In addition, our framework included the citizen science approach as a possible way to extend data collection of many ecological and some pressure variables in N2K sites. Although a main concern of citizen science studies is the level of reliability of data collection, which requires clear protocols, training of volunteers, and a quality check by professional scientists, this approach has been confirmed as useful in past experiences (Hidalgo-Ruz and Thiel, 2013; Matear et al., 2019). Actually, citizen science programs have contributed to collect information on population dynamics, health and distribution of marine organisms, and marine litter, and have also supported long-term monitoring programs of MPAs (Garcia-Soto et al., 2021 and reference therein). We believe that it can be beneficial to engage citizens, sharing visions and research questions with them in a reciprocal knowledge exchange, and involving them in the observations as a further support for large-scale and cost-effective monitoring initiatives (Couvet et al., 2008; Jones et al., 2018). We finally indicated as priority those variables that can feed ES assessment as well. Recently, the Essential Ecosystem Service Variables (EESVs) have been proposed, delivering a first set of variable classes (Balvanera et al., 2022). This new framework aims precisely at orienting and organizing the collection of harmonized and interoperable data suitable for such assessment, which is necessary to trail progress towards sustainability and conservation goals. Therefore, monitoring certain ecological parameters even with this intent in mind is highly recommended, because it will support an approach that could better address accounting for the benefits that nature provides to humans within decision-making processes.
This three-step EBM approach to monitoring variable prioritization stems from the broad and holistic framework of ECOAdS, the Ecological Observing System of the Adriatic Sea (Pugnetti et al., 2022; https://ecoads.eu/). ECOAdS represents a first step towards the upscaling of coordinated monitoring efforts through shared approach and vision at both N2K sites (the local) and N2K network (the regional) scales in the Adriatic. The EBM approach to monitoring proposed here can be transferred to other contexts and scales to help build a common knowledge and monitoring framework. This is fully in line with the holistic ECOAdS vision, which aims at a common goal of nature conservation by combining multiple disciplines, capitalizing and integrating existing monitoring frameworks through a tiered approach, and recognizing the power of engaging the multitude of stakeholders who benefit from nature (Pugnetti et al., 2020; Boemare et al., 2022).
With this study and our tested approach, we aimed at underlining the need to harmonize and integrate the existing monitoring frameworks in the broadest marine context and at multiple spatial scales. Adopting an EBM and integrated approach to monitoring would deliver concrete benefits to conservation and management strategies, saving costs by relying on available resources and on consolidated and long-lasting approaches that might converge towards global initiatives for the common goal of conserving our marine environment.
Data availability statement
The original contributions presented in the study are included in the article/Supplementary Material. Further inquiries can be directed to the corresponding author.
Author contributions
EM, CB, AP, LB, and AO conceptualized and proposed the idea. FG carried out the literature review on monitoring variables. EM, CB, and AO built the alignment scheme of the variables with the different frameworks (directives and EVs). EM carried out the variable scoring exercise and wrote the first draft of the paper. GP provided knowledge support on the ecology and monitoring of the bottlenose dolphin. All authors contributed to the article and approved the submitted version.
Funding
This research has been funded within the framework of the ECOSS project (Observing System in the Adriatic Sea: oceanographic observations for biodiversity), an EU 2014–2020 Interreg V-A Italy-Croatia CBC programme with ID number 10042301.
Acknowledgments
We acknowledge the ECOSS project partners, who managed and worked on the N2K sites considered in this paper, for their helpful assistance in the definition of the variables and for their monitoring at the sites.
Conflict of interest
The authors declare that the research was conducted in the absence of any commercial or financial relationships that could be construed as a potential conflict of interest.
Publisher’s note
All claims expressed in this article are solely those of the authors and do not necessarily represent those of their affiliated organizations, or those of the publisher, the editors and the reviewers. Any product that may be evaluated in this article, or claim that may be made by its manufacturer, is not guaranteed or endorsed by the publisher.
Supplementary material
The Supplementary Material for this article can be found online at: https://www.frontiersin.org/articles/10.3389/fmars.2022.920366/full#supplementary-material
References
Addison P. F. E., Collins D. J., Trebilco R., Howe S., Bax N., Hedge P., et al. (2018). A new wave of marine evidence-based management: emerging challenges and solutions to transform monitoring, evaluating, and reporting. ICES J. Mar. Sci. 75 (3), 941–952. doi: 10.1093/icesjms/fsx216
Allan I. J., Mills G. A., Vrana B., Knutsson J., Holmberg A., Guigues N., et al. (2006). Strategic monitoring for the European water framework directive. Trends Anal. Chem. 25, 704–715.
Alessi J., Bruccoleri F., Cafaro V. (2019). How citizens can encourage scientific research: The case study of bottlenose dolphins monitoring. Ocean Coast. Manage. 167, 9–19. doi: 10.1016/j.ocecoaman.2018.09.018
Arkema K. K., Abramson S. C., Dewsbury B. M. (2006). Marine ecosystem-based management: from characterization to implementation. Front. Ecol. Environ. 4 (10), 525–532. doi: 10.1890/1540-9295(2006)4[525:MEMFCT]2.0.CO;2
Apostolaki E. T., Tsagaraki T., Tsapakis M., Karakassis I. (2007). Fish farming impact on sediments and macrofauna associated with seagrass meadows in the Mediterranean. Estuar. Coast. Shelf Sci. 75 (3), 408–416.
Balestri E., Cinelli F. (2003). Sexual reproductive success in Posidonia oceanica. Aquat. Bot. 75 (1), 21–32.
Balestri E., Vallerini F., Lardicci C. (2006). A qualitative and quantitative assessment of the reproductive litter from Posidonia oceanica accumulated on a sand beach following a storm. Estuar. Coast. Shelf Sci. 66 (1-2), 30–34.
Balvanera P., Brauman K. A., Cord A. F., Drakou E. G., Geijzendorffer I. R., Karp D. S., et al. (2022). Essential ecosystem service variables for monitoring progress towards sustainability. Curr. Opin. Environ. Sustain. 54, 101152. doi: 10.1016/j.cosust.2022.101152
Binzer T., Borum J., Pedersen O. (2005). Flow velocity affects internal oxygen conditions in the seagrass Cymodocea nodosa. Aquat. Bot. 83 (3), 239–247.
Barratclough A., Wells R. S., Schwacke L. H., Rowles T. K., Gomez F. M., Fauquier D. A., et al. (2019). Health assessments of common bottlenose dolphins (Tursiops truncatus): Past, present, and potential conservation applications. Front. Vet. Sci. 6, 444.
Baş A. A., Amaha Öztürk A., Öztürk B. (2015). Selection of critical habitats for bottlenose dolphins (Tursiops truncatus) based on behavioral data, in relation to marine traffic in the Istanbul Strait, Turkey. Mar. Mammal Sci. 31 (3), 979–997.
Battaglia F. M., Beckingham B. A., McFee W. E. (2020). First report from North America of microplastics in the gastrointestinal tract of stranded bottlenose dolphins (Tursiops truncatus). Mar. Pollut. Bull. 160, 111677.
Bax N. J., Appeltans W., Brainard R., Emmett Duffy J., Dunstan P., Hanich Q., et al. (2018). Linking capacity development to GOOS monitoring networks to achieve sustained ocean observation. Front. Mar. Sci. 5. doi: 10.3389/fmars.2018.00346
Bearzi G., Bonizzoni S., Riley M. A., Santostasi N. L. (2021). Bottlenose dolphins in the north-western Adriatic Sea: Abundance and management implications. Aquat. Conserv.: Mar. Freshw. Ecosyst. 31 (3), 651–664. doi: 10.1002/aqc.3450
Bearzi G., Fortuna C., Reeves R. (2009). Ecology and conservation of common bottlenose dolphins tursiops truncatus in the Mediterranean Sea. Mammal Rev. 39 (2), 92. doi: 10.1111/j.1365-2907.2008.00133.x
Bearzi M. (2005). Aspects of the ecology and behaviour of bottlenose dolphins (Tursiops truncatus) in Santa Monica Bay, California.
Bearzi G., Holcer D., Notarbartolo di Sciara G. (2004). The role of historical dolphin takes and habitat degradation in shaping the present status of northern Adriatic cetaceans. Aquat. Conserv.: Mar. Freshw. Ecosyst. 14 (4), 363–379.
Bearzi G., Politi E., Agazzi S., Bruno S., Costa M., Bonizzoni S. (2005). Occurrence and present status of coastal dolphins (Delphinus delphis and Tursiops truncatus) in the eastern Ionian Sea. Aquat. Conserv.: Mar. Freshw. Ecosyst. 15 (3), 243–257.
Bearzi G., Politi E., Notarbartolo di Sciara G. (1999). Diurnal behavior of free-ranging bottlenose dolphins in the kvarnerić (northern adriatic sea). Mar. Mammal Sci. 15 (4), 1065–1097.
Benedetti-Cecchi L., Crowe T., Boehme L., Boero F., Christensen A., Grémare A., et al. (2018). “Strengthening europe’s capability in biological ocean observations,” in Future science brief 3 of the European marine board. Eds. Muñiz Piniella Á., Kellett P., Larkin K., Heymans J. J.(Ostend, Belgium) 76 pp.
Bennett A. F., Haslem A., Cheal D. C., Clarke M. F., Jones R. N., Koehn J. D., et al. (2009). Ecological processes: A key element in strategies for nature conservation. Ecol. Manage. Restor. 10 (3), 192–199. doi: 10.1111/j.1442-8903.2009.00489.x
Bergmann M., Gutow L., Klages M. (2015). Marine anthropogenic litter Vol. p (Springer Nature), 447).
Boemare C., Mosseri E., Agin G., Bramanti L., Certain R., Claudet J., et al. (2022). Hybridizing research and decision-making: a path toward sustainability in marine spaces (Preprint from Research Square). doi: 10.21203/rs.3.rs-1374903/v1
Bonamano S., Piazzolla D., Scanu S., Mancini E., Madonia A., Piermattei V., et al. (2021). Modelling approach for the evaluation of burial and erosion processes on Posidonia oceanica meadows. Estuar. Coast. Shelf Sci. 254, 107321.
Bonanno G., Orlando-Bonaca M. (2017). Trace elements in Mediterranean seagrasses: accumulation, tolerance and biomonitoring. A review. Mar. Pollut. Bull. 125 (1-2), 8–18.
Bonizzoni S., Furey N. B., Bearzi G. (2021). Bottlenose dolphins (Tursiops truncatus) in the north-western Adriatic Sea: Spatial distribution and effects of trawling. Aquat. Conserv.: Mar. Freshw. Ecosyst. 31 (3), 635–650. doi: 10.1002/aqc.3433
Booth C., Thomas L. (2021). An expert elicitation of the effects of low salinity water exposure on bottlenose dolphins. Oceans 21pp, 179–192).
Borja Á., Elliott M., Carstensen J., Heiskanen A. S., van de Bund W. (2010). Marine management–towards an integrated implementation of the European marine strategy framework and the water framework directives. Mar. pollut. Bull. 60, 2175–2186. doi: 10.1016/j.marpolbul.2010.09.026
Borowitzka M. A., Lavery P. S., van Keulen M. (2007). “). epiphytes of seagrasses,” in Seagrasses: Biology, ecology and conservation (Dordrecht: Springer), 441–461.
Boscutti F., Marcorin I., Sigura M., Bressan E., Tamberlich F., Vianello A., et al. (2015). Distribution modeling of seagrasses in brackish waters of Grado-Marano lagoon (Northern Adriatic Sea). Estuar. Coast. Shelf Sci. 164, 183–193.
Boström C., Bonsdorff E. (2000). Zoobenthic community establishment and habitat complexity the importance of seagrass shoot-density, morphology and physical disturbance for faunal recruitment. Mar. Ecol. Prog. Ser. 205, 123–138.
Bourque A. S., Kenworthy W. J., Fourqurean J. W. (2015). Impacts of physical disturbance on ecosystem structure in subtropical seagrass meadows. Mar. Ecol. Prog. Ser. 540, 27–41.
Brown C. J., Smith S. J., Lawton P., Anderson J. T. (2011). Benthic habitat mapping: A review of progress towards improved understanding of the spatial ecology of the seafloor using acoustic techniques. Estuar. Coast. Shelf Sci. 92 (3), 502–520.
Boudouresque C. F., Bernard G., Pergent G., Shili A., Verlaque M. (2009). Regression of Mediterranean seagrasses caused by natural processes and anthropogenic disturbances and stress: a critical review. Botanica marina 52 (5), 395–418. doi: 10.1515/BOT.2009.057
Bramanti L., Vielmini I., Rossi S., Stolfa S., Santangelo G. (2011). Involvement of recreational scuba divers in emblematic species monitoring: the case of Mediterranean red coral (Corallium rubrum). J. Nat. Conserv. 19 (5), 312–318. doi: 10.1016/j.jnc.2011.05.004
Brack W., Dulio V., Ågerstrand M., et al. (2017). Towards the review of the European Union Water Framework Directive: Recommendations for more efficient assessment and management of chemical contamination in European surface water resources. Sci. Total Environ. 576, 720–737.
Breitburg D., Levin L. A., Oschlies A., Grégoire M., Chavez F. P., Conley D. J., et al. (2018). Declining oxygen in the global ocean and coastal waters. Science 359 (6371). doi: 10.1126/science.aam7240
Brummitt N., Regan E. C., Weatherdon L. V., Martin C. S., Geijzendorffer I. R., Rocchini D., et al. (2017). Taking stock of nature: Essential biodiversity variables explained. Biol. Conserv. 213, 252–255. doi: 10.1016/j.biocon.2016.09.006
Bryndum-Buchholz A., Tittensor D. P., Blanchard J. L., Cheung W. W., Coll M., Galbraith E. D., et al. (2019). Twenty-first-century climate change impacts on marine animal biomass and ecosystem structure across ocean basins. Glob. Change Boil. 25 (2), 459–472. doi: 10.1111/gcb.14512
Buia M. C., Mazzella L. (1991). Reproductive phenology of the Mediterranean seagrasses Posidonia oceanica (L.) Delile, Cymodocea nodosa (Ucria) Aschers., and Zostera noltii Hornem. Aquat. Bot. 40 (4), 343–362.
Burkholder J. M., Tomasko D. A., Touchette B. W. (2007). Seagrasses and eutrophication. J. Exp. Mar. Biol. Ecol. 350 (1-2), 46–72.
Cabaço S., Santos R., Duarte C. M. (2008). The impact of sediment burial and erosion on seagrasses: a review. Estuar. Coast. Shelf Sci. 79 (3), 354–366.
Capotondi L., Ravaioli M., Acosta A., Chiarini F., Lami A., Stanisci A., et al. (2021). © CNR edizion 806. doi: 10.5281/zenodo.5570272
Carlucci R., Manea E., Ricci P., Cipriano G., Fanizza C., Maglietta R., et al. (2021). Managing multiple pressures for cetaceans’ conservation with an ecosystem-based marine spatial planning approach. J. Environ. Manage. 287, 112240. doi: 10.1016/j.jenvman.2021.112240
Carmichael R. H., Graham W. M., Aven A., Worthy G., Howden S. (2012). Were multiple stressors a ‘perfect storm’for northern Gulf of Mexico bottlenose dolphins (Tursiops truncatus) in 2011? PloS One 7 (7), e41155.
Carr M. H., Woodson C. B., Cheriton O. M., Malone D., McManus M. A., Raimondi P. T. (2011). Knowledge through partnerships: integrating marine protected area monitoring and ocean observing systems. Front. Ecol. Environ. 9 (6), 342–350. doi: 10.1890/090096
Cavallo M., Borja Á., Elliott M., Quintino V., Touza J. (2019). Impediments to achieving integrated marine management across borders: the case of the EU marine strategy framework directive. Mar. Policy 103, 68–73. doi: 10.1016/j.marpol.2019.02.033
Chamberlain D. A., Possingham H. P., Phinn S. R. (2022). Decision-making with ecological process for coastal and marine planning: current literature and future directions. Aquat. Ecol. 56 (1), 1–19. doi: 10.1007/s10452-021-09896-9
Ciriaco S., Menon S., Franzosini C., ECOSS Partnership (2019). D4.3.1 review of the knowledge of the ecological processes in the selected natura 2000 sites. Zenodo 69. doi: 10.5281/zenodo.6200186
Claudet J., Loiseau C., Sostres M., Zupan M. (2020). Underprotected marine protected areas in a global biodiversity hotspot. One Earth 2, 380–384. doi: 10.1016/j.oneear.2020.03.008
Clements C. F., McCarthy M. A., Blanchard J. L. (2019). Early warning signals of recovery in complex systems. Nat. Commun. 10 (1), 1–9. doi: 10.1038/s41467-019-09684-y
Como S., Magni P., Baroli M., Casu D., De Falco G., Floris A. (2008). Comparative analysis of macrofaunal species richness and composition in Posidonia oceanica, Cymodocea nodosa and leaf litter beds. Mar. Biol. 153 (6), 1087–1101.
Cox T. E., Gazeau F., Alliouane S., Hendriks I. E., Mahacek P., Le Fur A., et al. (2016). Effects of in situ CO 2 enrichment on structural characteristics, photosynthesis, and growth of the Mediterranean seagrass Posidonia oceanica. Biogeosciences 13 (7), 2179–2194.
Costello C., Molina R. (2021). Transboundary marine protected areas. Resour. Energy Econ 65, 101239. doi: 10.1016/j.reseneeco.2021.101239
Couvet D., Jiguet F., Julliard R., Levrel H., Teyssedre A. (2008). Enhancing citizen contributions to biodiversity science and public policy. Interdiscip. Sci. Rev. 33 (1), 95–103. doi: 10.1179/030801808X260031
Currey R. J. C., Dawson S. M., Schneider K., Lusseau D., Boisseau O., Haase P., et al. (2011). Inferring causal factors for a declining population of bottlenose dolphins via temporal symmetry capture-recapture modeling. Mar. Mammal Sci. 29, 554–566.
Currey R. J. C., Dawson S. M., Slooten E., et al. (2009). Survival rates for a declining population of bottlenose dolphins in Doubtful Sound, New Zealand: An information theoretic approach to assessing the role of human impacts. Aquat. Conserv.: Mar. Freshw. Ecosyst. 19, 658–670.
de Francesco M. C., Chiuchiarelli I., Frate L., Carranza M. L., Pagliani T., Stanisci A. (2020). Towards new marine-coastal natura 2000 sites in the central Adriatic Sea. (Italy: Firenze University Press Livorno) 529–539, CC BY 4.0 International. doi: 10.36253/978-88-5518-147-1.53
Deininger A., Frigstad H. (2019). Reevaluating the role of organic matter sources for coastal eutrophication, oligotrophication, and ecosystem health. Front. Mar. Sci. 6. doi: 10.3389/fmars.2019.00210
de los Santos C. B., Krause-Jensen D., Alcoverro T., Marbà N., Duarte C. M., Van Katwijk M. M., et al. (2019). Recent trend reversal for declining European seagrass meadows. Nat. Commun. 10 (1), 1–8. doi: 10.1038/s41467-019-11340-4
de los Santos C. B., Scott A., Arias-Ortiz A., Jones B., Kennedy H., Mazarrasa I., et al. (2020). Seagrass ecosystem services: assessment and scale of benefits, in Out of the blue: The value of seagrasses to the environment and to people. Eds. Potouroglou M., Grimsditch G., Weatherdon L., Lutz S. [Eds.] (United Nations Environment) 19–21
Dodds W. K., Clements W. H., Gido K., Hilderbrand R. H., King R. S. (2010). Thresholds, breakpoints, and nonlinearity in freshwaters as related to management. J. N. Am. Benthol. Soc 29, 988–997. doi: 10.1899/09-148.1
Duarte C. M., Krause-Jensen D. (2017). Export from seagrass meadows contributes to marine carbon sequestration. Front. Mar. Sci. 4. doi: 10.3389/fmars.2017.00013
Duarte C. M., Sand-Jensen K. (1990). Seagrass colonization: patch formation and patch growth in Cymodocea nodosa. Mar. Ecol. Prog. Ser., 193–200.
Duffy J. E., Benedetti-Cecchi L., Trinanes J., Muller-Karger F. E., Ambo-Rappe R., Boström C., et al. (2019). Toward a coordinated global observing system for seagrasses and marine macroalgae. Front. Mar. Sci. 6. doi: 10.3389/fmars.2019.00317
Dunham A., Dunham J. S., Rubidge E., Iacarella J. C., Metaxas A. (2020). Contextualizing ecological performance: Rethinking monitoring in marine protected areas. Aquat. Conserv.: Mar. Freshw. Ecosyst. 30 (10), 2004–2011. doi: 10.1002/aqc.3381
Eger S. L., de Loë R. C., Pittman J., Epstein G., Courtenay S. C. (2021). A systematic review of integrated coastal and marine management progress reveals core governance characteristics for successful implementation. Mar. Policy 132, 104688. doi: 10.1016/j.marpol.2021.104688
Embling C. B., Walters A. E. M., Dolman S. J. (2015). How much effort is enough? the power of citizen science to monitor trends in coastal cetacean species. Glob. Ecol. Conserv. 3, 867–877. doi: 10.1016/j.gecco.2015.04.003
EPA-US Environmental Protection Agency, Office of Federal Activities (1999) Considering ecological processes in environmental impact assessments. Available at: https://www.epa.gov/sites/production/files/2014-08/documents/ecological-processes-eia-pg.pdf.
Estes M. Jr., Anderson C., Appeltans W., Bax N., Bednaršek N., Canonico G., et al. (2021). Enhanced monitoring of life in the sea is a critical component of conservation management and sustainable economic growth. Mar. Policy 132, 104699. doi: 10.1016/j.marpol.2021.104699
European Commission (2000). Directive 2000/60/EC of the European parliament and of the council of 23 October 2000 establishing a framework for community action in the field of water policy (Water framework directive). Off. J. Eur. Union 73.
European Commission (2008). Directive 2008/56/EC of the European parliament of the council of 17 June 2008 establishing a framework for community action in the field of marine environmental policy (Marine strategy framework directive). Off. J. Eur. Union 27
European Commission (2017). Communication from the commission to the european parliament, the council, the european economic and social committee and the committee of the regions an action plan for nature, people and the economy Brussels, COM/2017/0198 final.
European Commission (2019). Reporting on the 2020 update of article 11 for the marine strategy framework directive (version 3.0, draft) (Brussels) 42.
European Commission Report (2013). Guidance on monitoring of marine litter in European seas (Luxembourg: Publications Office of the European Union) 10, 99475.
Fandel A. D., Garrod A., Hoover A. L., Wingfield J. E., Lyubchich V., Secor D. H., et al. (2020). Effects of intense storm events on dolphin occurrence and foraging behavior. Sci. Rep. 10, 19247.
Farella G., Menegon S., Fadini A., Depellegrin D., Manea E., Perini L., et al. (2020). Incorporating ecosystem services conservation into a scenario-based MSP framework: An Adriatic case study. Ocean Coast. Manage. 193, 105230. doi: 10.1016/j.ocecoaman.2020.105230
Félix F., Van Waerebeek K., Sanino G. P., Castro C., Van Bressem M. F., Santillán L. (2018). Variation in dorsal fin morphology in common bottlenose dolphin Tursiops truncatus (Cetacea: Delphinidae) populations from the Southeast Pacific Ocean. Pacific Sci. 72 (3), 307–320.
Fischer A., Grimes S. (2012). Definition of ecosystem essential ocean variables. technical report, GEOWOW-WP6-IOU-I6.1.1, GEOSS Interoperability for Weather, Ocean and Water, IOC-UNESCO 24.
Fortuna C. M., Cañadas A., Holcer D., Brecciaroli B., Donovan G. P., Lazar B., et al. (2018). The coherence of the European union marine natura 2000 network for wide-ranging charismatic species: A Mediterranean case study. Front. Mar. Sci. 5. doi: 10.3389/fmars.2018.00356
Fortuna C. M. (2006). Ecology and conservation of bottlenose dolphins (Tursiops truncatus) in the north-eastern Adriatic Sea. PhD Thesis (University of St AndrewsScotland).
Fortuna C., Bearzi G., Delfino G. (1996). Surfacing pattern of bottlenose dolphins following bottom trawlers in the Kvarneric (northern Adriatic Sea). Eur. Res. Cetaceans 10, 244.
Francour P., Ganteaume A., Poulain M. (1999). Effects of boat anchoring in Posidonia oceanica seagrass beds in the Port-Cros National Park (north-western Mediterranean Sea). Aquat. Conserv.: Mar. Freshw. Ecosyst. 9 (4), 391–400.
Fraschetti S., Fabbrizzi E., Tamburello L., Uyarra M. C., Micheli F., Sala E., et al. (2022). An integrated assessment of the good environmental status of Mediterranean marine protected areas. J. Environ. Manage. 305, 114370. doi: 10.1016/j.jenvman.2021.114370
Frazão Santos C., Agardy T., Andrade F., Barange M., Crowder L. B., Ehler C. N., et al. (2016). Ocean planning in a changing climate. Nat. Geosci. 9 (10), 730–730. doi: 10.1038/ngeo2821
Freiwald J., Meyer R., Caselle J. E., Blanchette C. A., Hovel K., Neilson D., et al. (2018). Citizen science monitoring of marine protected areas: case studies and recommendations for integration into monitoring programs. Mar. Ecol. 39, e12470. doi: 10.1111/maec.12470
Fruet P. F., Kinas P. G., da Silva K. G., Di Tullio J. C., Monteiro D. S., Dalla Rosa L., et al. (2012). Temporal trends in mortality and effects of by-catch on common bottlenose dolphins, Tursiops truncatus, in southern Brazil. J. Mar. Biol. Assoc. U.K. 92 (8), 1865–1876.
Gaines S. D., Gaylor B., Gerber L. R., Hastings A., Kinlan B. P. (2007). Connecting places: the ecological consequences of dispersal in the sea. Oceanography 20 (3), 90–99.
García Molinos J., Halpern B. S., Schoeman D. S., Brown C. J., Kiessling W., Moore P. J., et al. (2016). Climate velocity and the future global redistribution of marine biodiversity. Nat. Clim. Change. 6 (1), 83–88. doi: 10.1038/nclimate2769
Garcia-Soto C., Seys J. J., Zielinski O., Busch J. A., Luna S. I., Baez J. C., et al. (2021). Marine citizen science: current state in Europe and new technological developments. Front. Mar. Sci. 8. doi: 10.3389/fmars.2021.621472
Gaspari S., Holcer D., Mackelworth P., Fortuna C., Frantzis A., Genov T., et al. (2015). Population genetic structure of common bottlenose dolphins (Tursiops truncatus) in the Adriatic Sea and contiguous regions: implications for international conservation. Aquat. Conserv.: Mar. Freshw. Ecosyst. 25 (2), 212–222. doi: 10.1002/aqc.2415
Gaspari S., Holcer D., Mackelworth P., Fortuna C., Frantzis A., Genov T., et al. (2013). Population genetic structure of common bottlenose dolphins (Tursiops truncatus) in the Adriatic Sea and contiguous regions: implications for international conservation. Aquat. Conserv.: Mar. Freshw. Ecosyst. 25 (2), 212–222.
Gaspari S., Scheinin A., Holcer D., Fortuna C., Natali C., Genov T., et al. (2015). Drivers of population structure of the bottlenose dolphin (Tursiops truncatus) in the Eastern Mediterranean Sea. Evol. Biol. 42, 177–190.
Genov T., Kotnjek P., Lesjak J., Hace A., Fortuna C. M. (2008). Bottlenose dolphins (Tursiops truncatus) in Slovenian and adjacent waters (northern Adriatic Sea). Ann. Ser. Hist. Nat. 18 (2), 227–244.
Genov T., Centrih T., Kotnjek P., Hace A. (2019). Behavioural and temporal partitioning of dolphin social groups in the northern Adriatic Sea. Mar. Biol. 166 (1), 1–14.
Genov T. W., Fortuna C. M. (2009). Towards identification of the bottlenose dolphin (Tursiops truncatus) population structure in the north-eastern Adriatic Sea: preliminary results. Varstvo narave 22, 73–80.
Genov T., Kotnjek P., Lesjak J., Hace A., Fortuna C. M. (2008). Bottlenose dolphins (Tursiops truncatus) in Slovenian and adjacent waters (northern Adriatic Sea). Annales Ser. Hist. Naturalis (Vol. 18 No. 2 pp, 227–244).
Giannoulaki M., Belluscio A., Colloca F., Fraschetti S., Scardi M., Smith C., et al. (2013). Mediterranean Sensitive habitats (mediseh), final project report. DG MARE Specific Contract SI2 600 (741), 557.
Gillanders B. M., Kingsford M. J. (2002). “Impact of changes in flow of freshwater on estuarine and open coastal habitats and the associated organisms,” in Oceanography and Marine Biology an Annual Review. Eds. Gibson R. N., Barnes M., Atkinson &, R. J. A. (Taylor & Francis) 40, 233.
Giovos I., Ganias K., Garagouni M., Gonzalvo J. (2016). Social media in the service of conservation: A case study of dolphins in the Hellenic seas. Aquat. Mamm 42 (1), 12–20.
González-Correa J. M., Bayle J. T., Sánchez-Lizaso J. L., Valle C., Sánchez-Jerez P., Ruiz J. M. (2005). Recovery of deep Posidonia oceanica meadows degraded by trawling. J. Exp. Mar. Biol. Ecol. 320 (1), 65–76.
Gissi E., Maes F., Kyriazi Z., Ruiz-Frau A., Santos C. F., Neumann B., et al. (2022). Contributions of marine area-based management tools to the UN sustainable development goals. J. Clean. Prod. 330, 129910. doi: 10.1016/j.jclepro.2021.129910
Gissi E., Manea E., Mazaris A. D., Fraschetti S., Almpanidou V., Bevilacqua S., et al. (2021). A review of the combined effects of climate change and other local human stressors on the marine environment. Sci. Total Environ. 755, 142564. doi: 10.1016/j.scitotenv.2020.142564
Golec I., ECOSS Partnership (2020). D3.2.1. report on the ecological monitoring, conservation strategies and management questions of natura 2000 marine sites. Zenodo 71. doi: 10.5281/zenodo.6135905
Groom S., Sathyendranath S., Ban Y., Bernard S., Brewin R., Brotas V., et al. (2019). Satellite ocean colour: current status and future perspective. Front. Mar. Sci. 6. doi: 10.3389/fmars.2019.00485
Harvey C. J., Fisher J. L., Samhouri J. F., Williams G. D., Francis T. B., Jacobson K. C., et al. (2020). The importance of long-term ecological time series for integrated ecosystem assessment and ecosystem-based management. Prog. Oceanogr. 188, 102418. doi: 10.1016/j.pocean.2020.102418
Haase P. A., Schneider K. (2001). Birth demographics of bottlenose dolphins, Tursiops truncatus,” (New Zealand: Doubtful Sound, Fiordland), 675–680.
Hayes K. R., Dambacher J. M., Hosack G. R., Bax N. J., Dunstan P. K., Fulton E. A., et al. (2015). Identifying indicators and essential variables for marine ecosystems. Ecol. Indic. 57, 409–419. doi: 10.1016/j.ecolind.2015.05.006
Haynes D., Müller J., Carter S. (2000). Pesticide and herbicide residues in sediments and seagrasses from the Great Barrier Reef World Heritage Area and Queensland coast. Mar. Pollut. Bull. 41 (7-12), 279–287.
Heink U., Ingo Kowarik I. (2010). What are indicators? on the definition of indicators in ecology and environmental planning. Ecol. Indic. 10, 584–593. doi: 10.1016/j.ecolind.2009.09.009
Henson S. A., Beaulieu C., Ilyina T., John J. G., Long M., Séférian R., et al. (2017). Rapid emergence of climate change in environmental drivers of marine ecosystems. Nat. Commun. 8 (1), 1–9. doi: 10.1038/ncomms14682
Herrman J. M., Morey J. S., Takeshita R., Guise S. D., Wells R. S., McFee W., et al. (2020). Age determination of common bottlenose dolphins (Tursiops truncatus) using dental radiography pulp:tooth area ratio measurements. PloS One 15 (11), e0242273.
Hermoso V., Carvalho S. B., Giakoumi S., Goldsborough D., Katsanevakis S., Leontiou S., et al. (2022). The EU biodiversity strategy for 2030: Opportunities and challenges on the path towards biodiversity recovery. Environ. Sci. Policy 127, 263–271. doi: 10.1016/j.envsci.2021.10.028
Hidalgo-Ruz V., Thiel M. (2013). Distribution and abundance of small plastic debris on beaches in the SE pacific (Chile): a study supported by a citizen science project. Mar. Environ. Res. 87, 12–18. doi: 10.1016/j.marenvres.2013.02.015
Holcer D. (2012). Ecology of the common bottlenose dolphin, Tursiops truncatus (Montagu, 1821) in the Central Adriatic sea (Ekologija običnog dobrog dupina, Tursiops truncatus (Montagu, 1821) u području srednjeg Jadrana). PhD thesis, University of Zagreb, Faculty of Science, Department of Biology Zagreb
Holcer D. (2012). Ecology of the common bottlenose dolphin, Tursiops truncatus (Montag) in the Central Adriatic sea (Ekologija običnog dobrog dupina, Tursiops truncatus (Montag) u području srednjeg Jadrana) (Faculty of Science, Department of Biology Zagreb: PhD thesis, University of Zagreb).
Hossain M. S., Hashim M. (2019). Potential of earth observation (EO) technologies for seagrass ecosystem service assessments. Int. J. Appl. Earth Obs. Geoinf. 77, 15–29. doi: 10.1016/j.jag.2018.12.009
Hyder K., Townhill B., Anderson L. G., Delany J., Pinnegar J. K. (2015). Can citizen science contribute to the evidence-base that underpins marine policy? Mar. Policy 59, 112–120. doi: 10.1016/j.marpol.2015.04.022
Innangi S., Innangi M., Di Febbraro M., Di Martino G., Sacchi M., Tonielli R. (2022). Continuous, High-Resolution Mapping of Coastal Seafloor Sediment Distribution. Remote Sens. 14 (5), 1268.
Jones B. L., Unsworth R. K., McKenzie L. J., Yoshida R. L., Cullen-Unsworth L. C. (2018). Crowdsourcing conservation: The role of citizen science in securing a future for seagrass. Mar. pollut. Bull. 134, 210–215. doi: 10.1016/j.marpolbul.2017.11.005
Kati V., Hovardas T., Dieterich M., Ibisch P. L., Mihok B., Selva N. (2015). The challenge of implementing the European network of protected areas natura 2000. Conserv. Biol. 29 (1), 260–270. doi: 10.1111/cobi.12366
Kidd S., Jones H., Jay S. (2019). “Taking account of land-sea interactions in marine spatial planning,” in Maritime spatial planning. Eds. Zaucha J., Gee K. (Switzerland: Palgrave Macmillan Cham, Cham), 245–270. doi: 10.1007/978-3-319-98696-8
Kissling W. D., Ahumada J. A., Bowser A., Fernandez M., Fernández N., García E. A., et al. (2018). Building essential biodiversity variables (EBV s) of species distribution and abundance at a global scale. Biol. Rev. 93 (1), 600–625. doi: 10.1111/brv.12359
Kissling W. D., Hardisty A., García E. A., Santamaria M., De Leo F., Pesole G., et al. (2015). Towards global interoperability for supporting biodiversity research on essential biodiversity variables (EBVs). Biodiversity 16 (2-3), 99–107. doi: 10.1080/14888386.2015.1068709
Klein C., Wilson K., Watts M., Stein J., Berry S., Carwardine J., et al. (2009). Incorporating ecological and evolutionary processes into continental-scale conservation planning. Ecol. Appl. 19 (1), 206–217. doi: 10.1890/07-1684.1
Koch E. W. (2001). Beyond light: physical, geological, and geochemical parameters as possible submersed aquatic vegetation habitat requirements. Estuaries 24 (1), 1–17.
Koopmans D., Holtappels M., Chennu A., Weber M., De Beer D. (2020). High net primary production of Mediterranean seagrass (Posidonia oceanica) meadows determined with aquatic eddy covariance. Front. Mar. Sci. 7, 118.
Kroeker K. J., Bell L. E., Donham E. M., Hoshijima U., Lummis S., Toy J. A., et al. (2020). Ecological change in dynamic environments: Accounting for temporal environmental variability in studies of ocean change biology. Glob. Change Biol. 26 (1), 54–67. doi: 10.1111/gcb.14868
Letourneur Y., Ruitton S., Sartoretto S. (2003). Environmental and benthic habitat factors structuring the spatial distribution of a summer infralittoral fish assemblage in the north-western Mediterranean Sea. J. Mar. Biol. Assoc. U.K. 83 (1), 193–204.
Leslie H. M., McLeod K. L. (2007). Confronting the challenges of implementing marine ecosystem-based management. Front. Ecol. Environ. 5 (10), 540–548. doi: 10.1890/060093
Levin P. S., Fogarty M. J., Murawski S. A., Fluharty D. (2009). Integrated ecosystem assessments: developing the scientific basis for ecosystem-based management of the ocean. PloS Biol. 7 (1), e1000014. doi: 10.1371/journal.pbio.1000014
Lombard A. T., Dorrington R. A., Reed J. R., Ortega-Cisneros K., Penry G. S., Pichegru L., et al. (2019). Key challenges in advancing an ecosystem-based approach to marine spatial planning under economic growth imperatives. Front. Mar. Sci. 6. doi: 10.3389/fmars.2019.00146
Long R. D., Charles A., Stephenson R. L. (2015). Key principles of marine ecosystem-based management. Mar. Policy 57, 53–60. doi: 10.1016/j.marpol.2015.01.013
Lopez y Royo C., Pergent G., Pergent-Martini C., Casazza G. (2010). Seagrass (Posidonia oceanica) monitoring in western Mediterranean: implications for management and conservation. Environ. Monit. Assess. 171 (1), 365–380. doi: 10.1007/s10661-009-1284-z
López B. D. (2012). Bottlenose dolphins and aquaculture: interaction and site fidelity on the north-eastern coast of Sardinia (Italy). Mar. Biol. 159 (10), 2161–2172.
Lotze H. K., Tittensor D. P., Bryndum-Buchholz A., Eddy T. D., Cheung W. W., Galbraith E. D., et al. (2019). Global ensemble projections reveal trophic amplification of ocean biomass declines with climate change. Proc. Natl. Acad. Sci. U.S.A 116 (26), 12907–12912. doi: 10.1073/pnas.1900194116
Magris R. A., Pressey R. L., Weeks R., Ban N. C. (2014). Integrating connectivity and climate change into marine conservation planning. Biol. Conserv. 170, 207–221. doi: 10.1016/j.biocon.2013.12.032
Manea E., Bergami C., Bongiorni L., Capotondi L., De Maio E., Oggioni A., et al. (2021). A transnational marine ecological observatory in the Adriatic Sea to harmonize a fragmented approach to monitoring and conservation. Adv. Oceanogr. Limnol. 12 (1). doi: 10.4081/aiol.2021.9811
Manea E., Bianchelli S., Fanelli E., Danovaro R., Gissi E. (2020b). Towards an ecosystem-based marine spatial planning in the deep Mediterranean Sea. Sci. Total Environ. 715, 136884. doi: 10.1016/j.scitotenv.2020.136884
Manea E., Bongiorni L., Bergami C., Pugnetti A. (2020a). Challenges for marine ecological observatories to promote effective GMS of Natura 2000 network. The case study of ECOAdS in the Adriatic Sea. in Governing future challenges in protected areas. Eds. Alfaré L., Ruoss E. (CNR Edizioni, Roma, Italy) 23–39.
Mannino A. M., Balistreri P. (2018). Citizen science: a successful tool for monitoring invasive alien species (IAS) in marine protected areas. the case study of the egadi islands MPA (Tyrrhenian Sea, Italy). Biodiversity 19 (1-2), 42–48. doi: 10.1080/14888386.2018.1468280
Marbà N., Díaz-Almela E., Duarte C. M. (2014). Mediterranean seagrass (Posidonia oceanica) loss between 1842 and 2009. Biol. Conserv. 176, 183–190.
Marbà N., Duarte C. M. (2010). Mediterranean warming triggers seagrass (Posidonia oceanica) shoot mortality. Glob. Change Biol. 16 (8), 2366–2375.
Marco-Méndez C., Ferrero-Vicente L. M., Prado P., Sánchez-Lizaso J. L. (2017). Epiphytes and nutrient contents influence Sarpa salpa herbivory on Caulerpa spp vs. seagrass species in Mediterranean meadows. Estuar. Coast. Shelf Sci. 184, 54–66.
McCluskey S. M., Bejder L., Loneragan N. R. (2016). Dolphin prey availability and calorific value in an estuarine and coastal environment. Front. Mar. Sci. 3, 30.
Mari L., Melià P., Fraschetti S., Gatto M., Casagrandi R. (2020). Spatial patterns and temporal variability of seagrass connectivity in the Mediterranean Sea. Divers. Distrib. 26 (2), 169–182. doi: 10.1111/ddi.12998
Markov M., ECOSS Partnership (2019). D4.1.1 report on the characterization of the selected natura 2000 sites. Zenodo 86. doi: 10.5281/zenodo.6136088
Mascart T., Lepoint G., De Troch M. (2013). Meiofauna and harpacticoid copepods in different habitats of a Mediterranean seagrass meadow. J. Mar. Biol. Assoc. U.K. 93 (6), 1557–1566.
Matear L., Robbins J. R., Hale M., Potts J. (2019). Cetacean biodiversity in the bay of Biscay: suggestions for environmental protection derived from citizen science data. Mar. Policy 109, 103672. doi: 10.1016/j.marpol.2019.103672
Maxwell S. L., Cazalis V., Dudley N., Hoffmann M., Rodrigues A. S., Stolton S., et al. (2020). Area-based conservation in the twenty-first century. Nature 586 (7828), 217–227. doi: 10.1038/s41586-020-2773-z
Maxwell S. M., Hazen E. L., Lewison R. L., Dunn D. C., Bailey H., Bograd S. J., et al. (2015). Dynamic ocean management: Defining and conceptualizing real-time management of the ocean. Mar. Policy 58, 42–50. doi: 10.1016/j.marpol.2015.03.014
McQuatters-Gollop A., Mitchell I., Vina-Herbon C., Bedford J., Addison P. F., Lynam C. P., et al. (2019). From science to evidence–how biodiversity indicators can be used for effective marine conservation policy and management. Front. Mar. Sci. 6. doi: 10.3389/fmars.2019.00109
Medina-Lopez E., Ureña-Fuentes L. (2019). High-resolution sea surface temperature and salinity in coastal areas worldwide from raw satellite data. Remote Sens. 11 (19), 2191. doi: 10.3390/rs11192191
Miloslavich P., Bax N. J., Simmons S. E., Klein E., Appeltans W., Aburto-Oropeza O., et al. (2018). Essential ocean variables for global sustained observations of biodiversity and ecosystem changes. Glob. Change Biol. 24 (6), 2416–2433. doi: 10.1111/gcb.14108
Miočić-Stošić J., Pleslić G., ECOSS Partnership (2020). D4.2.1 review of the knowledge of the target species at the selected natura 2000 sites. Zenodo 91. doi: 10.5281/zenodo.6199909
Natoli A., Birkun A., Aguilar A., Lopez A., Hoelzel A. R. (2005). Habitat structure and the dispersal of male and female bottlenose dolphins (Tursiops truncatus). Proc. R. Soc B: Biol. Sci. 272 (1569), 1217–1226.
Nesti U., Piazzi L., Balata D. (2009). Variability in the structure of epiphytic assemblages of the Mediterranean seagrass Posidonia oceanica in relation to depth. Mar. Ecol. 30 (3), 276–287.
Nicholson E., Watermeyer K. E., Rowland J. A., Sato C. F., Stevenson S. L., Andrade A., et al. (2021). Scientific foundations for an ecosystem goal, milestones and indicators for the post-2020 global biodiversity framework. Nat. Ecol. Evol. 5 (10), 1338–1349. doi: 10.1038/s41559-021-01538-5
Niemi G. J., McDonald M. E. (2004). Application of ecological indicators. Annu. Rev. Ecol. Evol. Syst. 35, 89–111. doi: 10.1146/annurev.ecolsys.35.112202.130132
Nordlund L. M., Jackson E. L., Nakaoka M., Samper-Villarreal J., Beca-Carretero P., Creed J. C. (2018). Seagrass ecosystem services–what’s next? Mar. pollut. Bull. 134, 145–151. doi: 10.1016/j.marpolbul.2017.09.014
NRC, National Research Council (2000). Ecological indicators for the nation. Natl. Acad. Washington, DC: The National Academies Press. pp 198 doi: 10.17226/9720
Notarbartolo di Sciara G., Aguilar A., Bearzi G., Birkun. A., Frantzis A. (2002). Overview of known or presumed impacts on the different species of cetaceans in the Mediterranean and Black Seas. Cetaceans Mediterranean Black Seas: State Knowledge Conserv. Strategies.
Nykänen M., Dillane E., Englund A., et al. (2018). Quantifying dispersal between marine protected areas by a highly mobile species, the bottlenose dolphin, Tursiops truncatus. Ecol. Evol. 8 (18), 9241–9258.
O’Leary B. C., Ban N. C., Fernandez M., Friedlander A. M., García-Borboroglu P., Golbuu Y., et al. (2018). Addressing criticisms of large-scale marine protected areas. Bioscience 68 (5), 359–370. doi: 10.1093/biosci/biy021
Olsen Y. S., Sánchez-Camacho M., Marbà N., Duarte C. M. (2012). Mediterranean Seagrass growth and demography responses to experimental warming. Estuaries Coasts 35 (5), 1205–1213. doi: 10.1007/s12237-012-9521-z
Orlikowska E. H., Roberge J.-M., Blicharska M., Mikusiński G. (2016). Gaps in ecological research on the world’s largest internationally coordinated network of protected areas: a review of natura 2000. Biol. Conserv. 200, 216–227. doi: 10.1016/j.biocon.2016.06.015
Paul M., Lefebvre A., Manca E., Amos C. L. (2011). An acoustic method for the remote measurement of seagrass metrics. Estuar. Coast. Shelf Sci. 93 (1), 68–79. doi: 10.1016/j.ecss.2011.04.006
Penaluna B. E., Reeves G. H., Barnett Z. C., Bisson P. A., Buffington J. M., Dolloff C. A., et al. (2018). Using natural disturbance and portfolio concepts to guide aquatic–riparian ecosystem management. Fisheries 43 (9), 406–422. doi: 10.1002/fsh.10097
Pereda-Briones L., Terrados J., Agulles M., Tomas F. (2020). Influence of biotic and abiotic factors of seagrass posidonia oceanica recruitment: Identifying suitable microsites. Mar. Environ. Res. 162, 105076. doi: 10.1016/j.marenvres.2020.105076
Pereira H. M., Ferrier S., Walters M., Geller G. N., Jongman R. H. G., Scholes R. J., et al. (2013). Essential biodiversity variables. Science 339 (6117), 277–278. doi: 10.1126/science.1229931
Pérez M., Romero J. (1994). Growth dynamics, production, and nutrient status of the seagrass Cymodocea nodosa in a Mediterranean semi-estuarine environment. Mar. Ecol. 15 (1), 51–64.
Pérez-Ruzafa A., Marcos C., Bernal C. M., Quintino V., Freitas R., Rodrigues A. M., et al. (2012). Cymodocea nodosa vs. Caulerpa prolifera: Causes and consequences of a long term history of interaction in macrophyte meadows in the Mar Menor coastal lagoon (Spain, southwestern Mediterranean). Estuar. Coast. Shelf Sci. 110, 101–115.
Piazzi L., Balata D. (2009). Invasion of alien macroalgae in different Mediterranean habitats. Biol. Invasions 11 (2), 193–204.
Pinsky M. L., Worm B., Fogarty M. J., Sarmiento J. L., Levin S. A. (2013). Marine taxa track local climate velocities. Science 341 (6151), 1239–1242. doi: 10.1126/science.1239352
Pitchford J. L., Howard V. A., Shelley J. K., Serafin B. J., Coleman A. T., Solangi M. (2016). Predictive spatial modelling of seasonal bottlenose dolphin (Tursiops truncatus) distributions in the Mississippi Sound. Aquat. Conserv.: Mar. Freshw. Ecosyst. 26 (2), 289–306.
Pleslić G., Rako-Gospić N., Holcer D. (2021). Bottlenose dolphins (Tursiops truncatus) in north dalmatia, Croatia: Occurrence and demographic parameters. Mar. Mammal Sci. 37 (1), 142–161. doi: 10.1111/mms.12735
Procaccini G., Orsini L., Ruggiero M. V., Scardi M. (2001). Spatial patterns of genetic diversity in Posidonia oceanica, an endemic Mediterranean seagrass. Mol. Ecol. 10 (6), 1413–1421.
Pleslić G., Rako Gospić N., Mackelworth P., Wiemann A., Holcer D., Fortuna C. (2015). The abundance of common bottlenose dolphins (Tursiops truncatus) in the former special marine reserve of the cres-lošinj archipelago, Croatia. Aquat. Conserv.: Mar. Freshw. Ecosyst. 25 (1), 125–137. doi: 10.1002/aqc.2416
Pleslić G., Rako-Gospić N., Miočić-Stošić J., Blazinić Vučur T., Radulović M., Mackelworth P., et al. (2019). Social structure and spatial distribution of bottlenose dolphins (Tursiops truncatus) along the Croatian Adriatic coast. Aquat. Conserv.: Mar. Freshw. Ecosyst. 29 (12), 2116–2132. doi: 10.1002/aqc.3213
Pleslić G., Rako-Gospić N., Holcer D. (2021). Bottlenose dolphins (Tursiops truncatus) in North Dalmatia, Croatia: Occurrence and demographic parameters. Mar. Mammal Sci. 37 (1), 142–161.
Pleslić G., Rako-Gospić N., Mackelworth P., Wiemann A., Holcer D., Fortuna C. (2015). The abundance of common bottlenose dolphins (Tursiops truncatus) in the former special marine reserve of the Cres-Lošinj Archipelago, Croatia. Aquat. Conserv.: Mar. Freshw. Ecosyst. 25 (1), 125–137.
Pleslić G., Rako-Gospić N., Miočić-Stošić J., Blazinić Vučur T., Radulović M., Mackelworth P., et al. (2019). Social structure and spatial distribution of bottlenose dolphins (Tursiops truncatus) along the Croatian Adriatic coast. Aquat. Conserv.: Mar. Freshw. Ecosyst. 29 (12), 2116–2132.
Pocock M. J., Chandler M., Bonney R., Thornhill I., Albin A., August T., et al. (2018). A vision for global biodiversity monitoring with citizen science. Adv. Ecol. Res. 59, 169–223. doi: 10.1016/bs.aecr.2018.06.003
Poeta G., Staffieri E., Acosta A. T., Battisti C. (2017). Ecological effects of anthropogenic litter on marine mammals: A global review with a “black-list”. impacted taxa. Hystrix 28 (2), 253.
Pugnetti A. (2020). Voices from the water: experience, knowledge, and emotions in long-term ecological research (LTER Italy). Adv. Oceanogr. Limnol. 11 (2). doi: 10.4081/aiol.2020.9508
Pugnetti A., Acri F., Bernardi Aubry F., Camatti E., Cecere E., Facca C., et al. (2013). The Italian long-term ecosystem research (LTER-Italy) network: results, opportunities, and challenges for coastal transitional ecosystems. Transit. Water Bull. 7 (1), 43–63. doi: 10.1285/i1825229Xv7n1p43
Pugnetti A., Manea E., Vilibić I., Sarretta A., Capotondi L., Cataletto B., et al. (2022). The ecological observing system of the Adriatic Sea (ECOAdS): structure and perspectives within the main European biodiversity and environmental strategies. Res. Ideas Outcomes 8, e82597. doi: 10.3897/rio.8.e82597
Rako-Gospić N., Radulović M., Vučur T., Pleslić G., Holcer D., Mackelworth P. (2017). Factor associated variations in the home range of a resident Adriatic common bottlenose dolphin population. Mar. pollut. Bull. 124 (1), 234–244. doi: 10.1016/j.marpolbul.2017.07.040
Rako N., Fortuna C. M., Holcer D., Mackelworth P., Nimak-Wood M., Pleslić G., et al. (2013a). Leisure boating noise as a trigger for the displacement of the bottlenose dolphins of the Cres–Lošinj archipelago (northern Adriatic Sea, Croatia). Mar. Pollut. Bull. 68, 77–84.
Rako-Gospić N., Radulović M., Vučur T., Pleslić G., Holcer D., Mackelworth P. (2017). Factor associated variations in the home range of a resident Adriatic common bottlenose dolphin population. Mar. Pollut. Bull. 124 (1), 234–244.
Rako N., Picciulin M., Vilibić I., Fortuna C. M. (2013b). Spatial and temporal variability of sea ambient noise as an anthropogenic pressure index: the case of the Cres-Lošinj archipelago, Croatia. J. Mar. Biol. Assoc. U.K. 93 (1), 27–36.
Ravaioli M., Bergami C., Riminucci F., Langone L., Cardin V., Di Sarra A., et al. (2016). The RITMARE Italian fixed-point observatory network (IFON) for marine environmental monitoring: A case study. J. Oper. Oceanogr. 9 (sup1), s202–s214. doi: 10.1080/1755876X.2015.1114806
Read A. J., Wells R. S., Hohn A. A., Scott M. D. (1993). Patterns of growth in wild bottlenose dolphins, Tursiops truncatus. J. Zool. 231 (1), 107–123.
Rees S. E., Foster N. L., Langmead O., Pittman S., Johnson D. E. (2018). Defining the qualitative elements of aichi biodiversity target 11 with regard to the marine and coastal environment in order to strengthen global efforts for marine biodiversity conservation outlined in the united nations sustainable development goal 14. Mar. Policy 93, 241–250. doi: 10.1016/j.marpol.2017.05.016
Reimer J. M., Devillers R., Claudet J. (2021). Benefits and gaps in area-based management tools for the ocean sustainable development goal. Nat. Sustain. 4 (4), 349–357. doi: 10.1038/s41893-020-00659-2
Ricci P., Cipriano G., Pollazzon V., Fanizza C., Maglietta R., Sion L., et al. (2018). “I cetacei di taranto: elementi ecologici e culturali investigati attraverso la citizen science,” in Ricerca in vetrina 2018: Ricerca è democrazia. Eds. Lai L., Mastinu M., Mura E. ,. V. (Franco Angeli, Milano)
Ricci P., Manea E., Cipriano G., Cascione D., D’Onghia G., Ingrosso M., et al. (2021). Addressing cetacean–fishery interactions to inform a deep-Sea ecosystem-based management in the gulf of taranto (Northern Ionian Sea, central Mediterranean Sea). J. Mar. Sci. Eng. 9 (8), 872. doi: 10.3390/jmse9080872
Rilov G., Mazaris A. D., Stelzenmüller V., Helmuth B., Wahl M., Guy-Haim T., et al. (2019). Adaptive marine conservation planning in the face of climate change: What can we learn from physiological, ecological and genetic studies? Glob. Ecol. Conserv. 17, e00566. doi: 10.1016/j.gecco.2019.e00566
Roca G., Alcoverro T., Krause-Jensen D., Balsby T. J., Van Katwijk M. M., Marbà N., et al. (2016). Response of seagrass indicators to shifts in environmental stressors: a global review and management synthesis. Ecol. Indic. 63, 310–323. doi: 10.1016/j.ecolind.2015.12.007
Roca G., Romero J., Farina S., Martínez-Crego B., Alcoverro T. (2017). Using seagrasses to identify local and large-scale trends of metals in the Mediterranean Sea. Mar. Pollut. Bull. 123 (1-2), 83–91.
Rouillard J., Lago M., Abhold K., Röschel L., Kafyeke T., Mattheiß V., et al. (2018). Protecting aquatic biodiversity in Europe: How much do EU environmental policies support ecosystem-based management? Ambio 47, 15–24. doi: 10.1007/s13280-017-0928-4
Roxy M. K., Modi A., Murtugudde R., Valsala V., Panickal S., Prasanna Kumar S., et al. (2016). A reduction in marine primary productivity driven by rapid warming over the tropical Indian ocean. Geophys. Res. Lett. 43 (2), 826–833. doi: 10.1002/2015GL066979
Ruiz-Frau A., Gelcich S., Hendriks I. E., Duarte C. M., Marbà N. (2017). Current state of seagrass ecosystem services: research and policy integration. Ocean Coast. Manage. 149, 107–115. doi: 10.1016/j.ocecoaman.2017.10.004
Ruiz J. M., Marín-Guirao L., García-Muñoz R., Ramos-Segura A., Bernardeau-Esteller J., Pérez M., et al. (2018). Experimental evidence of warming-induced flowering in the Mediterranean seagrass posidonia oceanica. Mar. pollut. Bull. 134, 49–54. doi: 10.1016/j.marpolbul.2017.10.037
Sanchez-Lizaso J. L., Romero J., Ruiz J., Gacia E., Buceta J. L., Invers O., et al. (2008). Salinity tolerance of the Mediterranean seagrass Posidonia oceanica: recommendations to minimize the impact of brine discharges from desalination plants. Desalination 221 (1-3), 602–607.
Satterthwaite E. V., Bax N. J., Miloslavich P., Ratnarajah L., Canonico G., Dunn D., et al. (2021). Establishing the foundation for the global observing system for marine life. Front. Mar. Sci. 8. doi: 10.3389/fmars.2021.737416
Scheffer M., Carpenter S. R., Lenton T. M., Bascompte J., Brock W., Dakos V., et al. (2012). Anticipating critical transitions. Science 338, 344–348. doi: 10.1126/science.1225244
Schmeller D. S., Weatherdon L. V., Loyau A., Bondeau A., Brotons L., Brummit N., et al. (2017). A suite of essential biodiversity variables for detecting critical biodiversity change. Biol. Rev. 93 (1), 55–71. doi: 10.1111/brv.12332
Smale D. A., Epstein G., Parry M., Attrill M. J. (2019). Spatiotemporal variability in the structure of seagrass meadows and associated macrofaunal assemblages in southwest England (UK): Using citizen science to benchmark ecological pattern. Ecol. Evol. 9 (7), 3958–3972. doi: 10.1002/ece3.5025
Stelzenmüller V., Coll M., Mazaris A. D., Giakoumi S., Katsanevakis S., Portman M. E., et al. (2018). A risk-based approach to cumulative effect assessments for marine management. Sci. Total Environ. 612, 1132–1140. doi: 10.1016/j.scitotenv.2017.08.289
Suprenand P. M., Ainsworth C. H. (2017). Trophodynamic effects of climate change-induced alterations to primary production along the western Antarctic peninsula. Mar. Ecol. Prog. Ser. 569, 37–54. doi: 10.3354/meps12100
Taylor A. R., Schacke J. H., Speakman T. R., Castleberry S. B., Chandler R. B. (2016). Factors related to common bottlenose dolphin (Tursiops truncatus) seasonal migration along South Carolina and Georgia coasts. U.S.A. Anim. Migr. 3 (1), 14–26.
Todd P. A., Heery E. C., Loke L. H., Thurstan R. H., Kotze D. J., Swan C. (2019). Towards an urban marine ecology: characterizing the drivers, patterns and processes of marine ecosystems in coastal cities. Oikos 128 (9), 1215–1242. doi: 10.1111/oik.05946
Tomas F., Turon X., Romero J. (2005). Seasonal and small-scale spatial variability of herbivory pressure on the temperate seagrass Posidonia oceanica. Mar. Ecol. Prog. Ser. 301, 95–107.
Turnhout E., Hisschemoeller M., Eijsackers H. (2007). Ecological indicators: between the two fires of science and policy. Ecol. Indic. 7, 215–228. doi: 10.1016/j.ecolind.2005.12.003
Traganos D., Reinartz P. (2018). Mapping Mediterranean seagrasses with Sentinel-2 imagery. Mar. Pollut. Bull. 134, 197–209.
Tuya F., Ribeiro-Leite L., Arto-Cuesta N., Coca J., Haroun R., Espino F. (2014). Decadal changes in the structure of Cymodocea nodosa seagrass meadows: Natural vs. human influences. Estuar. Coast. Shelf Sci. 137, 41–49.
United Nations Environment Programme (UNEP) (2011) Taking steps toward marine and coastal ecosystem-based management - an introductory guide. Available at: https://www.unep.org/resources/report/taking-steps-toward-marine-and-coastal-ecosystem-based-management-introductory.
United Nations Environment Programme (UNEP) (2019) Frontiers 2018/19: Emerging issues of environmental concern. Available at: https://www.unep.org/resources/frontiers-201819-emerging-issues-environmental-concern.
Unsworth R. K., Nordlund L. M., Cullen-Unsworth L. C. (2019). Seagrass meadows support global fisheries production. Conserv. Lett. 12 (1), e12566. doi: 10.1111/conl.12566
Van Hoey G., Borja A., Birchenough S., Buhl-Mortensen L., Degraer S., Fleischer D., et al. (2010). The use of benthic indicators in Europe: from the water framework directive to the marine strategy framework directive. Mar. pollut. Bull. 60 (12), 2187–2196. doi: 10.1016/j.marpolbul.2010.09.015
Venn-Watson S. K., Jensen E. D., Smith C. R., Xitco M., Ridgway S. H. (2015). Evaluation of annual survival and mortality rates and longevity of bottlenose dolphins (Tursiops truncatus) at the United States Navy Marine Mammal Program from 2004 through 2013. J. Am. Vet. Med. Assoc. 246 (8), 893–898.
WFD Common Implementation Strategy (2003-2004). Common implementation strategy for the water framework directiv/60/ec) guidance document no 7 monitoring under the water framework directive produced by working group 2.7 – monitoring. (Luxembourg: Office for Official Publications of the European Communities)
Williams P. J., Hooten M. B., Womble J. N., Esslinger G. G., Bower M. R. (2018). Monitoring dynamic spatio-temporal ecological processes optimally. Ecology 99 (3), 524–535. doi: 10.1002/ecy.2120
Wells R. S., Scott M. D. (1990). Estimating bottlenose dolphin population parameters from individual identification and capture-release techniques. Rep. Int. Whaling Commission 12, 407–415.
Wild S., Krützen M., Rankin R. W., Hoppitt W. J., Gerber L., Allen S. J. (2019). Long-term decline in survival and reproduction of dolphins following a marine heatwave. Curr. Biol. 29 (7), R239–R240.
Wong C. P., Jiang B., Kinzig A. P., Lee K. N., Ouyang Z. (2015). Linking ecosystem characteristics to final ecosystem services for public policy. Ecol. Lett. 18 (1), 108–118. doi: 10.1111/ele.12389
Zampoukas N., Piha H., Bigagli E., Hoepffner N., Hanke G., Cardoso A. C. (2013). Marine monitoring in the European union: how to fulfill the requirements for the marine strategy framework directive in an efficient and integrated way. Mar. Policy 39, 349–351. doi: 10.1016/j.marpol.2012.12.004
Zilioli M., Bergami C., Carrara P., Fugazza C., Oggioni A., Pugnetti A., et al. (2021). Enabling the reuse of long-term marine biological observations in essential variables frameworks through a practical approach. Front. Mar. Sci. 8, 645997. doi: 10.3389/fmars.2021.645997
Keywords: essential variables, MSFD, WFD, natura 2000, marine ecological observatory, transboundary conservation
Citation: Manea E, Bergami C, Pugnetti A, Gianni F, Oggioni A, Bandelj V, Cataletto B, Pleslić G and Bongiorni L (2022) An ecosystem-based system of variables to enhance marine species and habitat monitoring and conservation: The Adriatic Natura 2000 case study. Front. Mar. Sci. 9:920366. doi: 10.3389/fmars.2022.920366
Received: 14 April 2022; Accepted: 07 September 2022;
Published: 07 October 2022.
Edited by:
Mariana Mayer Pinto, University of New South Wales, AustraliaReviewed by:
Sara Pensieri, National Research Council (CNR), ItalyAngela Banaduc, Lucian Blaga University of Sibiu, Romania
Copyright © 2022 Manea, Bergami, Pugnetti, Gianni, Oggioni, Bandelj, Cataletto, Pleslić and Bongiorni. This is an open-access article distributed under the terms of the Creative Commons Attribution License (CC BY). The use, distribution or reproduction in other forums is permitted, provided the original author(s) and the copyright owner(s) are credited and that the original publication in this journal is cited, in accordance with accepted academic practice. No use, distribution or reproduction is permitted which does not comply with these terms.
*Correspondence: Caterina Bergami, caterina.bergami@ismar.cnr.it