- 1Institute for Marine and Antarctic Studies, University of Tasmania, Hobart, TAS, Australia
- 2Centre for Marine Socioecology, University of Tasmania, Hobart, TAS, Australia
- 3State Key Laboratory of Marine Pollution and Department of Chemistry, City University of Hong Kong, Kowloon, Hong Kong SAR, China
- 4Department of Climate and Environment, Stiftelsen for industriell og teknisk forskning (SINTEF) Ocean, Trondheim, Norway
- 5Southern Marine Science and Engineering Guangdong Laboratory (Zhuhai), Zhuhai, China
- 6Chioggia Hydrobiological Station “Umberto D’Ancona”, Department of Biology, University of Padova, UO CoNISMa), Chioggia, Italy
- 7Department for the Cultural Heritage, University of Bologna, Ravenna, Italy
- 8School of BioSciences, University of Melbourne, Parkville, VIC, Australia
Editorial on the Research Topic
Marine Pollution - Emerging Issues and Challenges
Introduction: Classic and Emerging Research Trends in Marine Pollution
With the rapid development of human society, there is an increasing diversity and geographic spread of substances being released into the marine environment. Above threshold values these substances can have negative effects on the biological component of these systems and are therefore classified as pollutants (Cabral et al., 2019). Pollutants can be introduced to marine environments directly through human activities, indirectly through runoff such as discharges of untreated or partially treated wastewater, and or by exchange with the atmosphere (Noone et al., 2013). The relative contribution of different pollutants from these pathways varies substantially between substances and also spatially and temporally (Bierman et al., 2011). In this editorial we conducted a review of current and emerging trends in marine pollution research based on a keyword search of the literature in Web of Science. Our aim was to provide context to the articles published in the special issue.
Research on marine pollution is an important component of marine science, with the number of studies on this topic rapidly increasing through time (Figure 1A). Most of these studies have been conducted in shallow nearshore environments of sheltered estuaries and bays where human activities are concentrated (Halpern et al., 2008) whereas only very few studies have been conducted in open oceans and deep seas (Van Cauwenberghe et al., 2013; Cózar et al., 2014; Tournadre, 2014). The research effort is not evenly distributed across the globe, with much of the published literature being produced in China, followed closely by the USA and various countries in Europe (Figure 1B). This reflects the substantial impacts of these nations on the marine environment (Halpern et al., 2019; Bhuyan et al., 2021) and their leading role in producing scientific outputs (Marginson, 2021). Alarmingly, recent research on pollution in many developing regions such as Africa, Asia, and South America (Figure 1B), has demonstrated high levels of pharmaceuticals (Wilkinson et al., 2022). Hence, more research on marine pollution in these little-studied regions is necessary to gain a greater understanding of the spatial footprint of anthropogenic activities.
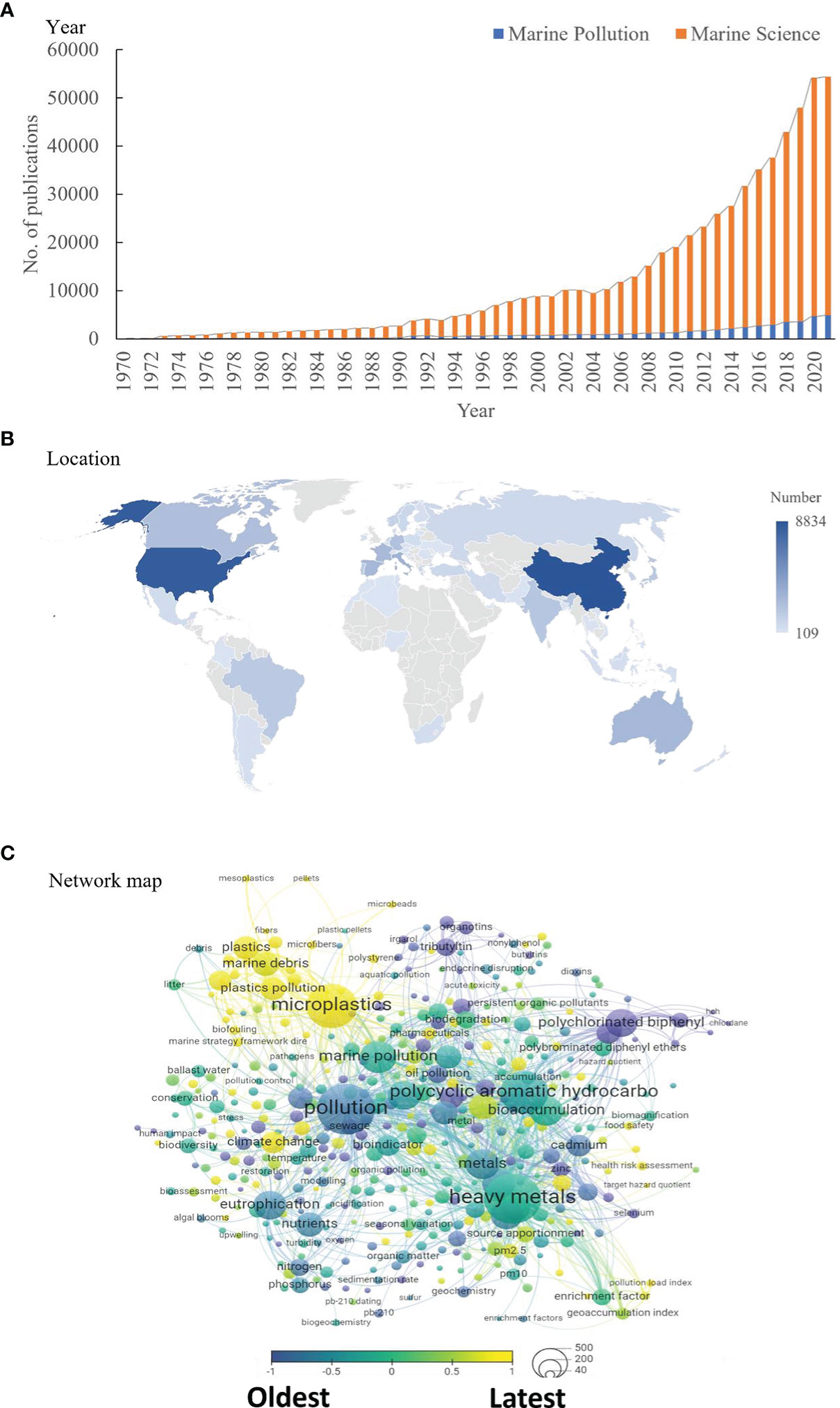
Figure 1 The total number of studies (A) on marine pollution and marine science by year and (B) by country (with more than 100 results) and (C) a network map (online version at https://bit.ly/31Z88uo) showing the most research topics in marine pollution during 1970 to 2021 based on keyword searches in the search engine Web of Science. The search for studies on “marine pollution” and “marine science” was conducted on 23rd August 2021. A total of 39,550 research articles on “marine pollution” and 568,755 on “marine science” were identified.
Traditionally, research on marine pollution has focused on studying how the release of hazardous or waste substances in particular heavy metals and nutrients affects individual organisms (O’Brien and Keough, 2014). More recently, however, research on marine pollution has highlighted how the input and spread of other substances including debris, microplastics, antibiotics and persistent organic pollutants (POPs) Shamskhany et al., antibiotics (Dahms, 2014) can influence populations, assemblages and ecosystems (Figure 1C). The range of topics in this special issue suggests the definition for marine pollution should be expanded to include the individual and interactive impacts of these substances with other forms of pollution from energy transfer (e.g., heat, noise and light) and artificial structures (e.g., sea cages, oil rigs, coastal infrastructure) or from the construction of marine artificial structures (e.g., sea cages, oil rigs, coastal infrastructure).
Melbourne-Thomas et al.; Georgiades et al.. We also highlight that the high uncertainty about effects of multiple substances entering the marine environment has important repercussions for developing effective monitoring, management, and mitigation solutions.
Effects of Pollutants and Their Impacts
Nutrient Enrichment
High nitrogen and phosphorus concentrations are common sources of pollution in the marine environment, with inputs derived from various point and diffuse sources. Resultant eutrophication has many undesirable ecological effects, for example phytoplankton blooms and/or shifts toward noxious cyanobacteria (Wurtsbaugh et al., 2019), replacement of ecosystem engineers including kelps and scleractinian corals with structurally less complex foliose or turf-algae (D’Angelo and Wiedenmann, 2014; Strain et al., 2014) and increases in the occurrence and severity of marine diseases. Most devastatingly, nutrient pollution has been linked to low levels of dissolved oxygen in marine environments, or hypoxia conditions which create large areas devoid of macrofauna, due to emigration or mortality, leading to the creation of dead zones (Altieri and Diaz, 2019). Understanding the impacts of nutrients, through time and across multiple locations and marine habitats remains an ongoing challenge in marine science.
Heavy Metals
Heavy metals (the group of metals and metalloids of relatively high atomic mass, that can cause toxicity problems) are primarily brought into marine environments through industrial, urban runoff and shipping activities, where they disperse into the water column or are incorporated into sediments (Birch, 2017). Some metals such as Fe, Zn and Cu play vital roles in marine organism’s metabolism and only become toxic at high concentrations. Other metals, including Hg, Pb, Cd and Cr are detrimental to marine organisms even at low concentrations. As pollutants, heavy metals have known effects on the physiological and individual performance of seaweeds (Baumann et al., 2009; Costa et al., 2020), invertebrates (Fowles et al., 2018) and invertebrates with knock on effects on fishes Tamburini et al. In sheltered locations, heavy metals are particularly hazardous pollutants in the marine environment because they do not degrade and can bioaccumulate through the food chain with potential detrimental effects to human health via consumption of contaminated seafood (Lavoie et al., 2018). Rare earth elements (e.g. lanthanides and + yttrium) and nanoparticles of Ag, ZnO and TiO have also been increasingly detected in the marine environment (Gwenzi et al., 2018). However, the extent and impact of these rare elements and nanoparticles on marine organisms and ecosystems have yet to be determined (Piarulli et al., 2021).
Persistent Organic Pollutants
POPs are a broad range of organic chemicals that are persistent, bioaccumulative and toxic substances in the environment and include some polycyclic aromatic hydrocarbons (PAHs). They are a relatively diverse group of pollutants derived from multiple sources. For example, PAHs are mainly derived from activities related to the petrochemical industry, combustion, and oil spills (Ghosal et al., 2016). These chemicals have an extremely long half-life in the marine environment and have been found from coastal beaches to the deepest ocean trenches (Jamieson et al., 2017). POPs accumulate in the tissues of marine flora and fauna, where they may cause damage, with effects exacerbated via bioaccumulation and biomagnification in marine food webs (Andrady, 2011; Matthies et al., 2016). There is increasing scientific interest in the presence of newly identified POPs in the marine environment such as perfluoroalkyl and polyfluoroalkyl substances, polychlorinated naphthalenes, flame retardants and paraffins (e.g. Lee et al.). Often the use of these emerging compounds has not regulated and their effects on the surrounding marine environment are still being investigated.
Plastic Debris
Inefficient land-based waste management and human negligence have led anthropogenic litter entering and accumulating in the marine environment. Plastic litter is omnipresent across polar regions to the equator and persistent in the marine environment both in the water column and sediments (Barnes et al., 2009; Rosevelt et al., 2013). More than 200 species, including marine megafauna such as seabirds, cetaceans, pinnipeds, and sea turtles, are negatively affected by large plastic debris through entanglement or ingestion (Derraik, 2002; Gregory, 2009). The smaller particle size may pose greater risk, as the particles can be ingested by a wider range of invertebrate and vertebrate species, enter the food chain and transfer across different trophic levels (de Souza Machado et al., 2018). Recent research has demonstrated that microplastics (plastic particles between 1 µm and 5 mm) can have physical, chemical, and biological impacts on organisms either directly or indirectly through associated additives (plasticisers) and adsorbed chemical contaminants. Adverse effects include physical damages such as obstructions, abrasions and inflammation and physiological alterations such as decreased food consumption, weight loss, decreased growth rate and fecundity and energy depletion (Zhang et al., 2019). Despite growing research interest, the broader ecological effects of macro-, micro- and nano plastic debris in different marine compartments and ecosystem remain largely unexplored. Also, the role of different biological processes in affecting the dynamics and fate of these particles necessitates further study.
Artificial Structures
Urbanisation of coastal areas (Small and Nicholls, 2003; Strain et al., 2019), and growth in the blue economy have resulted in the proliferation of artificial structures (e.g. breakwaters, reefs, sewage/storm water outflows and offshore platforms etc) in the marine environment (Bugnot et al., 2021). Artificial structures are not traditionally considered a pollutant, but they are included in the broader definition of pollution established by this special issue (see definition above). Artificial structures include contamination associated with the discharge of toxins and nutrients and increased noise and light pollution (Heery et al., 2017; Komyakova et al., 2022). These structures frequently destroy and fragment natural habitats but also provide novel surfaces for colonising marine organisms (Firth et al., 2016). Artificial structures can facilitate the establishment and spread of non-native species (Airoldi et al., 2015, https://research-topic-management-app.frontiersin.org/manage/17385/manuscript) and in some cases form ecological traps which reduce organism fitness (Swearer et al., 2021) and reduce ecological connectivity by acting as physical barriers to the movement of organisms within and among habitats (Bishop et al., 2017). Globally it was estimated that in 2018 the footprint of marine artificial structures 1.0–3.4 × 106 km2 was greater than the extent of some natural vegetated habitats and predicted to increase by at least 23% over the next twenty years (Bugnot et al., 2021).
Effects of Multiple Pollutants and Secondary Feedback Loops
Given the broad connectivity of the marine environment, there is the general assumption that various forms of marine pollution will be in some way interactive (Crain et al., 2008; Black et al., 2015). As the diversity of pollutants in the marine environment increases, so does the complexity of interactions. Thus, our ability to interpret and manage such complex and interacting impacts should be enhanced as well. Complex interactions occur between mixtures of chemicals (Belden et al., 2007) but also between artificial infrastructure, nutrients, and other sources of pollution (e.g. Rivero et al., 2013). Although studies on the effect of multiple pollutants and secondary or indirect pathways are complex, their outcomes are more realistic and aligned with management actions (Adams, 2005)
The current challenge is to establish a framework to assess the effects of multiple pollutants that is realistic and can be used to guide management decisions. This is partly constrained by the lack of research on multiple pollutants in urban marine and estuarine environments (Van den Brink et al., 2019). On a global scale, research has focussed over the last 10 years on understanding the effects of individual sources of pollutants (O’Brien et al., 2019), occasionally two pollutants (Black et al., 2015) but rarely more (e.g. Martin et al., 2021). Climate change, although not a source of pollution, is also stressor that can influence the effects of pollution (e.g. Wang et al., 2019) and should also be considered a component in the context of multiple stressors and marine pollution research (Cabral et al., 2019, https://research-topic-management-app.frontiersin.org/manage/17385/manuscript).
The effects of multiple pollutants are commonly categorised as additive, antagonistic or synergistic (Piggott et al., 2015). Additive effects are those where the combined effect is equal to the sum of their individual effects, antagonistic effects occur when the interactions between stressors result in a lesser combined effect and synergistic effects result in an amplification of effects (Orr et al., 2020). The effects of marine pollutants can accumulate over time (Jackson et al., 2001) or occur at different frequencies and intensities making the overall impacts difficult to predict (e.g. Blasco et al., 2016). A recent review identified synergistic or antagonistic interactions for specific chemical combinations were not consistent (Martin et al., 2021) and often did not exceed the magnitude of the effect predicted by an additive model (e.g. Boobis et al., 2011).
Another option for understanding the effects of multiple pollutants is to consider the severity of the impact based on the magnitude of the interactive effects. The magnitude of the effect could be measured by the extent of the deviation from the expected additive model (Martin et al., 2021) or other null models depending on the stressor mode of action or species sensitivities (Schäfer and Piggott, 2018). For example, an antagonistic interaction is predicted for nutrients and metal contamination whereby the effects of the individual pollutants are greater than the interactive effects. This type of interactive effect has been shown to occur in coastal marine microbial communities. However, the direction and magnitude of the antagonistic interactive effect can change at higher levels of biological organisation. This approach is useful as it can identify different magnitudes of the additive, antagonistic or synergistic interactive effects, and potentially more flexible to multiple sources and definitions of marine pollution.
Approaches to Monitoring Marine Pollution
The development of novel techniques and methods is critical for monitoring the impacts of marine pollutants. Studies on the effects of emerging and multiple pollutants often require innovative models, equipment, or methods to detect impacts. Here we briefly provide an overview of some of the emerging approaches and techniques that are currently being used or proposed for monitoring marine pollutants.
Environmental Risk Assessment
Conventional quantitative environmental risk assessment (ERA) focuses on characterising the exposure and effect of a specific chemical substances in the environment, with their ratio used to estimate risk (Environmental Risk Assessment, 1998). In the marine environment, however, organisms are simultaneously exposed to a cocktail of various anthropogenic chemicals. This discrepancy between the real environment and ERA presents a challenge for monitoring and management (Backhaus and Faust, 2012; Kortenkamp and Faust, 2018). Recent studies have developed more complicated ERA frameworks such as (1) summing up the toxicity thresholds derived from the species sensitivity distribution based on either the concentration addition or independent action models and (2) deriving the mixture toxicity for each species separately based on the mode of action before fitting species sensitivity distribution and deriving the overall toxicity threshold. However, none of methods consider the potential non-additive interactions among the environmental pollutants (Warne and Hawker, 1995; Belden et al., 2007). Therefore, future ERAs probably require a case-by-case assessments of the interactions between chemicals and other sources of pollution by trained specialists, instead of using standard protocols for risk assessments (Heys et al., 2016).
Molecular Approaches and eDNA
Metabolomics, proteomics, and transcriptomics are, high-throughput technologies that are used for environmental genomics and eDNA monitoring (Leung, 2018, https://research-topic-management-app.frontiersin.org/manage/17385/manuscript). These approaches can be used to detect individual-level responses (e.g. metabolomics; Jeppe et al., 2017; Sinclair et al., 2019), populations changes (e.g. eDNA; Smart et al., 2015), and occasionally community-level effects of pollution (e.g. metabarcoding; Chariton et al., 2010). More recently, novel applications that combine multiple molecular approaches to detect the effects of pollution on ecosystem functioning have emerged. For example, using metabolomics combined with metabarcoding to detect the effects of pollution on microbial community function (Morris et al., 2018). Despite the broad knowledge and potential use of molecular approaches to detect impacts that align with management goals, barriers adopting them in routine monitoring and pollution assessments remain (Cordier et al., 2021).
Unmanned Platforms
Mooring, satellites, and vehicles have great potential to measure the effects of marine pollutants over a wide range of spatial and temporal scales, and in difficult to assess environments including open oceans and deeper waters (Verfuss et al., 2019; Salgado-Hernanz et al., 2021). However, much of the research on developing and testing sensors has been targeted to specific pollutant types and terrestrial settings (e.g. detection of litter along beaches) (Salgado-Hernanz et al., 2021). In water, the application of these newly developing technologies is often limited to specific locations and depths, and subject to biofouling and maritime growth, which can influence the measurement outcomes (Verfuss et al., 2019; Salgado-Hernanz et al., 2021, Part et al.). Further development of sensor technologies and subsequent reductions in costs, will allow these platforms to become increasingly important monitoring tools in marine environments.
Artificial Intelligence/Machine Learning
Machine learning, a subset of artificial intelligence, refers to the ability of machines to learn and understand relationships between inputs and outputs from a full set of representative training samples (He et al., 2021). Monitoring approaches that use machine learning to monitor marine pollution are still relatively conceptual, although it is a field of research where progress is being made very quickly. There have been successful applications in the context of oil spill detection (Al-Ruzouq et al., 2020), benthic monitoring (Mohamed et al., 2018) and monitoring of fish populations (Ditria et al., 2021) while machine learning has been incorporated widely into bioinformatics associated with molecular approaches (Cordier et al., 2017; Fruhe et al., 2021). There is clear application where monitoring techniques generate thousands of images or video, or where large data sets are produced. With ongoing improvement and development in computing and technology, there is clear potential for progress in this space.
Solutions for Marine Pollutions
Reducing marine pollution is a global challenge that needs to be addressed for the health of the oceans and the maintenance of its ecosystem goods and services. Solutions to reduce marine pollution at local scales can be applied singularly or in combination and include 1) detection and prevention; 2) sustainable management; 3) habitat restoration and reconciliation. We suggest a combined approach may be more appropriate given the broadening definition of marine pollution and the complexity of interactions. Here we explore the application of these strategies in the context of nutrients enrichment, plastic litter, and artificial structures. More research in this area is needed to address the complex interactions between marine pollutants and the global nature of impacts.
Case Study 1: Nutrient Pollution
Chesapeake Bay is an example of where the combined approach of scientific monitoring, sustainable management, and targeted habitat restoration has successfully been implemented to restore estuarine health (Lefcheck et al., 2018). Concerns about the loss of large areas of submerged aquatic vegetation (SAV) within the estuary during the 1970s and 80’s, resulted in development of a key goals and comprehensive scientific monitoring program for monitoring changes in water quality through time (Orth et al., 2017). Local management measures which included reduction of land-based nonpoint sources, improved design of watersheds, technological implementation of sewage treatment plants and restoration of the SAV area in the Bay were applied by multiple agencies to reduce the amount of nutrients and sediments entering and concentrating in the Bay (Orth et al., 2017; Lefcheck et al., 2018) A scientific monitoring program developed and reported on in an “Annual Ecological Report Card” (AERC) that provides quantitative measurements of (1) water quality through chlorophyll-a, dissolved oxygen, and Secchi depth assessments (2) biological measures of phytoplankton diversity and abundance and (3) quantifies the area of SAV which are combined to determine performance-driven numeric grades of the ecosystem health (Bay Health Index) (Williams et al., 2009). The AERC is important for communicating the results of the monitoring to decision-makers and stakeholders (Williams et al., 2009). Through time, the AERC has been used to document substantial improvements in water quality and recovery of tens of thousands of hectares of SAV in the estuarine with flow on benefits for biodiversity and other ecosystems services (Lefcheck et al., 2018).
Case Study 2: Plastic Debris
Globally marine plastic pollution has become a significant environmental concern for multiple stakeholder groups (Seltenrich, 2015). Effective solutions to reduce marine plastic debris are still under development at international level, but focus on combing detection, prevention and sustainable management using four main categories (Chen, 2015):
1. Source prevention based on the 3R rule (i.e. reuse, reduce and recycle) and accompanied by land-based management actions to prevent plastic debris to enter the marine environment;
2. Removal based on the environmental monitoring of the marine debris followed by local initiatives at both at institutional and citizenship for plastic clean-up;
3. Regulative and sustainable management frameworks developing and implementing regulations for production of single use plastics, litter disposal, reuse and recycling;
4. Educational which covers campaigns to raise societal awareness and economic/incentive approaches.
The beaches of Cijin, Kaohsiung (Taiwan) have demonstrated that the reduction, reuse and recycling of plastics in terrestrial environments can abated the transfer of these pollutants into marine environments (Liu et al., 2013). In 2001, plastic constituted the 21.1% of household waste (Liu et al., 2013). In 2002, TEPA developed the Plastic Restriction Policy under the Waste Disposal Act which prohibited the use of plastic shopping bags and disposable plastic tableware in all government agencies and public facilities. Through time, the quantity of shoppers using recyclable shopping bags increased considerably, resulting in a substantial reduction of plastic waste. In 2002 and 2005, Taiwan implemented the Resource Recycling Act (RRA) and the Compulsory Trash-sorting Policy (CTP) which required the use of recycling bins in all public places and encouraged users to sort their waste. An economic penalty of 1200–6000 NT was applied for unsorted trash. These policies reduced the waste disposal rate from 2001 to 2010, by 50% (from 0.9 to 0.48 kg per capita) (Liu et al., 2013). Over ten years, the development and implementation of a stricter waste-management programs and associated policies has successfully reduced the amount of plastic debris on the surrounding beaches by approximately 20% (Liu et al., 2013).
Case Study 3: Artificial Structures
Coastal artificial structures are typically built to protect infrastructure and assets but can have negative impacts on natural intertidal habitats (Morris et al., 2019). The artificial structures in Caress Bush Park, NSW, Australia represent a key example of where sustainable management or habitat restoration solutions have been applied during construction to allow natural processes and tidal inundation to occur (Heath, 2017). The development contains a mixture of habitats, rock pools, crevices, mudflats for colonising mangroves and planted saltmarshes. This simultaneously protects public land from flooding and erosion and improves native biodiversity (Heath and Moody, 2013; Strain et al., 2018b) and habitat connectivity (Strain et al., 2018a). This approach to habitat restoration also has the potential to restore other ecosystem services (e.g. nutrient cycling and carbon sequestration), while developing relationships with the community through educational and recreational engagement (Heath, 2017).
Conclusion
The study of marine pollution has traditionally focused on understanding the detrimental effects of human wastes and hazardous substances on living resources, human health, and activities, at various spatial and temporal scales. More recently, studies have also considered the input of energy including heat, light, and noise, and changing environmental conditions (e.g. acidification), as sources of pollution. Apart from direct discharges from untreated and partially treated sewage, inputs of pollutants can also be transferred into the marine environment indirectly through surface runoff, freshwater inputs, and atmospheric processes. However, research on marine pollution is rapidly expanding, this special issue highlights, new and emerging types of marine pollution, the complexity of their interactions, and approaches for monitoring with a specific focus on scientific papers published over the last five years. We provide three key examples of solutions to address the hot or emerging topics in marine pollution, focusing on nutrients, plastics, and artificial structures. A combination of scientific monitoring, sustainable management and restoration solutions will be fundamental to addressing the UN sustainable development goal 14.1. ”preventing and significantly reduce marine pollution of all kinds” and the UN Decade of Ocean Science for Sustainable Development (2021-2030) (https://www.oceandecade.org/) that supports all international efforts to reverse the cycle of decline in ocean health, making the ocean cleaner and safer for all.
Author Contributions
ES, CW and RL and AO’B conceived of the presented ideas. All authors discussed the concepts and contributed to the final manuscript.
Funding
Support for LA was from project MUR EMME - PRIN 2017-2018.
Conflict of Interest
The authors declare that the research was conducted in the absence of any commercial or financial relationships that could be construed as a potential conflict of interest.
Publisher’s Note
All claims expressed in this article are solely those of the authors and do not necessarily represent those of their affiliated organizations, or those of the publisher, the editors and the reviewers. Any product that may be evaluated in this article, or claim that may be made by its manufacturer, is not guaranteed or endorsed by the publisher.
References
Adams S. M. (2005). Assessing Cause and Effect of Multiple Stressors on Marine Systems. Marine Pollution Bull. 51, 649–657. doi: 10.1016/j.marpolbul.2004.11.040
Airoldi L., Turon X., Perkol-Finkel S., Rius M. (2015). Corridors for Aliens But Not for Natives: Effects of Marine Urban Sprawl at a Regional Scale. Diversity Distributions 21, 755–768. doi: 10.1111/ddi.12301
Altieri A. H., Diaz R. J. (2019). “Dead Zones: Oxygen Depletion in Coastal Ecosystems,” in World Seas: An Environmental Evaluation (Oxford, UK: Elsevier), Pages 453–473.
Al-Ruzouq R., Gibril M. B. A, Shanableh A., Kais A., Hamed O., Al-Mansoori S., et al. (2020). Sensors, Features and Machine Learning for Oil Spill Detection and Monitoring: A Review. Remote Sensing 12 (20), 3338. doi: 10.3390/rs12203338
Andrady A. L. (2011). Microplastics in the Marine Environment. Marine Pollution Bull. 62, 1596–1605. doi: 10.1016/j.marpolbul.2011.05.030
Backhaus T., Faust M. (2012). Predictive Environmental Risk Assessment of Chemical Mixtures: A Conceptual Framework. Environ. Sci. Technol. 46, 2564–2573. doi: 10.1021/es2034125
Barnes D. K., Galgani F., Thompson R. C., Barlaz M. (2009). Accumulation and Fragmentation of Plastic Debris in Global Environments. Philos. Trans. R. Soc. B: Biol. Sci. 364, 1985–1998. doi: 10.1098/rstb.2008.0205
Baumann H. A., Morrison L., Stengel D. B. (2009). Metal Accumulation and Toxicity Measured by PAM—Chlorophyll Fluorescence in Seven Species of Marine Macroalgae. Ecotoxicol. Environ. Saf. 72, 1063–1075. doi: 10.1016/j.ecoenv.2008.10.010
Belden J. B., Gilliom R. J., Lydy M. J. (2007). How Well can We Predict the Toxicity of Pesticide Mixtures to Aquatic Life? Integrated Environ. Assess. Management: Int. J. 3, 364–372. doi: 10.1002/ieam.5630030307
Bhuyan M. S., Venkatramanan S., Selvam S., Szabo S., Hossain M. M., Rashed-Un-Nabi M., et al. (2021). Plastics in Marine Ecosystem: A Review of Their Sources and Pollution Conduits. Regional Stud. Marine Sci. 41, 101539. doi: 10.1016/j.rsma.2020.101539
Bierman P., Lewis M., Ostendorf B., Tanner J. (2011). A Review of Methods for Analysing Spatial and Temporal Patterns in Coastal Water Quality. Ecol. Indic. 11, 103–114. doi: 10.1016/j.ecolind.2009.11.001
Birch G. (2017). Determination of Sediment Metal Background Concentrations and Enrichment in Marine Environments–a Critical Review. Sci. Total Environ. 580, 813–831. doi: 10.1016/j.scitotenv.2016.12.028
Bishop M. J., Mayer-Pinto M., Airoldi L., Firth L. B., Morris R. L., Loke L. H., et al. (2017). Effects of Ocean Sprawl on Ecological Connectivity: Impacts and Solutions. J. Exp. Marine Biol. Ecol. 492, 7–30. doi: 10.1016/j.jembe.2017.01.021
Black J. G., Reichelt-Brushett A. J., Clark M. W. (2015). The Effect of Copper and Temperature on Juveniles of the Eurybathic Brittle Star Amphipholis Squamata–Exploring Responses Related to Motility and the Water Vascular System. Chemosphere 124, 32–39. doi: 10.1016/j.chemosphere.2014.10.063
Blasco J., Chapman P. M., Campana O., Hampel M. (2016). Marine Ecotoxicology: Current Knowledge and Future Issues (Amsterdam, Netherlands: Elsvier).
Boobis A., Budinsky R., Collie S., Crofton K., Embry M., Felter S., et al. (2011). Critical Analysis of Literature on Low-Dose Synergy for Use in Screening Chemical Mixtures for Risk Assessment. Crit. Rev. Toxicol. 41, 369–383. doi: 10.3109/10408444.2010.543655
Bugnot A., Mayer-Pinto M., Airoldi L., Heery E., Johnston E., Critchley L., et al. (2021). Current and Projected Global Extent of Marine Built Structures. Nat. Sustainability 4, 33–41. doi: 10.1038/s41893-020-00595-1
Cabral H., Fonseca V., Sousa T., Costa Leal M. (2019). Synergistic Effects of Climate Change and Marine Pollution: An Overlooked Interaction in Coastal and Estuarine Areas. Int. J. Environ. Res. Public Health 16, 2737. doi: 10.3390/ijerph16152737
Chariton A. A., Court L. N., Hartley D. M., Colloff M. J., Hardy C. M. (2010). Ecological Assessment of Estuarine Sediments by Pyrosequencing Eukaryotic Ribosomal DNA. Front. Ecol. Environ. 8, 233–238. doi: 10.1890/090115
Chen C. L. (2015). “Regulation and Management of Marine Litter,” in Marine Anthropogenic Litter (Cham: Springer), Pages 395–428.
Costa G. B., Koerich G., d. Ramos B., Ramlov F., Martínez-Crego B., Costa M. M., et al. (2020). A Review of Common Parameters and Descriptors Used in Studies of the Impacts of Heavy Metal Pollution on Marine Macroalgae: Identification of Knowledge Gaps and Future Needs. Acta Botanica Brasilica 34, 460–477. doi: 10.1590/0102-33062020abb0072
Cózar A., Echevarría F., González-Gordillo J. I., Irigoien X., Úbeda B., Hernández-León S., et al. (2014). Plastic Debris in the Open Ocean. Proc. Natl. Acad. Sci. 111, 10239–10244. doi: 10.1073/pnas.1314705111
Crain C. M., Kroeker K., Halpern B. S. (2008). Interactive and Cumulative Effects of Multiple Human Stressors in Marine Systems. Ecol. Lett. 11, 1304–1315. doi: 10.1111/j.1461-0248.2008.01253.x
Cordier T., Alonso-Sáez L., Apothéloz-Perret-Gentil L., Aylagas E., Bohan D. A., Bouchez A, et al. (2021). Ecosystems Monitoring Powered by Environmental Genomics: A Review of Current Strategies With an Implementation Roadmap. Mol. Eco. 30(13), 2937–2958
Cordier T., Esling P., Lejzerowicz F., Visco J., Ouadahi A., Martins C., et al. (2017). Predicting The Ecology quality Status of Marine Environments From eDNA Metabarcoding Data Using Supervised Machine Learning. Enviror. Sci. Tech. 51(16), 9118–26. doi: 10.1021/acs.est.7b01518
Dahms H. U. (2014). The Grand Challenges in Marine Pollution Research. Front. Marine Sci. 1, 9. doi: 10.3389/fmars.2014.00009
D’Angelo C., Wiedenmann J. (2014). Impacts of Nutrient Enrichment on Coral Reefs: New Perspectives and Implications for Coastal Management and Reef Survival. Curr. Opin. Environ. Sustainability 7, 82–93. doi: 10.1016/j.cosust.2013.11.029
Derraik J. G. (2002). The Pollution of the Marine Environment by Plastic Debris: A Review. Marine Pollution Bull. 44, 842–852. doi: 10.1016/S0025-326X(02)00220-5
de Souza Machado A. A., Kloas W., Zarfl C., Hempel S., Rillig M. C. (2018). Microplastics as an Emerging Threat to Terrestrial Ecosystems. Global Change Biol. 24, 1405–1416. doi: 10.1111/gcb.14020
Ditria E. M, Connolly R. M, Jinks E. L, Lopez-Marcano S.(2021). Annotated Video Footage for Automated Identification and Counting of Fish in Unconstrained Seagrass Habitats. Front. Mar. Sci. 54. doi: 10.3389/fmars.2021.629485
Environmental Risk Assessment. (1998). Guidelines for Ecological Risk Assessment. (Washington, DC, United States: Environmental Protection Agency).
Firth L. B., Knights A. M., Bridger D., Evans A., Mieskowska N., Moore P. J., et al. (2016). Ocean Sprawl: Challenges and Opportunities for Biodiversity Management in a Changing World. Oceanography Marine Biol.: an Annu. Rev. 54, 189–191.
Fowles A. E., Stuart-Smith R. D., Stuart-Smith J. F., Hill N. A., Kirkpatrick J. B., Edgar G. J. (2018). Effects of Urbanisation on Macroalgae and Sessile Invertebrates in Southeast Australian Estuaries. Estuarine Coastal Shelf Sci. 205, 30–39. doi: 10.1016/j.ecss.2018.02.010
Fruhe L., Cordier T., Dully V., Breiner H., Lentendu G., Pawlowski J., et al. (2020). Supervised Machine Learning is Superior to Indicator Value Inference in Monitoring the Environmental Impacts of Salmon Aquaculture Using eDNA Metabarcode. Mol. Eco. doi: 10.1111/mec.15434
Ghosal D., Ghosh S., Dutta T. K., Ahn Y. (2016). Current State of Knowledge in Microbial Degradation of Polycyclic Aromatic Hydrocarbons (Pahs): A Review. Front. Microbiol., 1369. doi: 10.3389/fmicb.2016.01369
Gregory M. R. (2009). Environmental Implications of Plastic Debris in Marine Settings—Entanglement, Ingestion, Smothering, Hangers-on, Hitch-Hiking and Alien Invasions. Philos. Trans. R. Soc. B: Biol. Sci. 364, 2013–2025. doi: 10.1098/rstb.2008.0265
Gwenzi W., Lynda M., Concilia D., Nhamo C., Nothando D., Edmond S. (2018). Sources, Behaviour, and Environmental and Human Health Risks of High-Technology Rare Earth Elements as Emerging Contaminants. Sci Environ 636, 299–313.
Halpern B. S., Frazier M., Afflerbach J., Lowndes J. S., Micheli F., O’Hara C., et al. (2019). Recent Pace of Change in Human Impact on the World’s Ocean. Sci. Rep. 9, 1–8. doi: 10.1038/s41598-019-47201-9
Halpern B. S., Walbridge S., Selkoe K. A., Kappel C. V., Micheli F., D’Agrosa C., et al. (2008). A Global Map of Human Impact on Marine Ecosystems. Science 319, 948–952. doi: 10.1126/science.1149345
Heath T. (2017). Carss Bush Park Environmentally Friendly Seawall–Stage 1 (Sydney, Australia: Fish Friendly Marine Infrastructure).
Heath T., Moody G. (2013). “Habitat Development Along a Highly Urbanised Foreshore”, in Proceedings of New South Wales Coastal Conference 2003. Sydney, Australia.
He L., Bai L., Dionysiou D. D., Wei Z., Spinney R., Chu C., et al. (2021). Applications of Computational Chemistry, Artificial Intelligence, and Machine Learning in Aquatic Chemistry Research. Chem. Eng. J. 426, 131810. doi: 10.1016/j.cej.2021.131810
Heery E. C., Bishop M. J., Critchley L. P., Bugnot A. B., Airoldi L., Mayer-Pinto M., et al. (2017). Identifying the Consequences of Ocean Sprawl for Sedimentary Habitats. J. Exp. Marine Biol. Ecol. 492, 31–48. doi: 10.1016/j.jembe.2017.01.020
Heys K. A., Shore R. F., Pereira M. G., Jones K. C., Martin F. L. (2016). Risk assessment of environmental mixture effects. RSC advances 6, 47488–57. doi: 10.1039/C6RA05406D
Jackson J. B., Kirby M. X., Berger W. H., Bjorndal K. A., Botsford L. W., Bourque B. J., et al. (2001). Historical Overfishing and the Recent Collapse of Coastal Ecosystems. Science 293, 629–637. doi: 10.1126/science.1059199
Jamieson A. J., Malkocs T., Piertney S. B., Fujii T., Zhang Z. (2017). Bioaccumulation of Persistent Organic Pollutants in the Deepest Ocean Fauna. Nat. Ecol. Evol. 1, 1–4. doi: 10.1038/s41559-016-0051
Jeppe K. J., Yang J., Long S. M., Carew M. E., Zhang X., Pettigrove V., et al. (2017). Detecting Copper Toxicity in Sediments: From the Subindividual Level to the Population Level. J. Appl. Ecol. 54, 1331–1342. doi: 10.1111/1365-2664.12840
Komyakova V., Jaffrés J. B., Strain E. M., Cullen-Knox C., Fudge M., Langhamer O., et al. (2022). Conceptualisation of Multiple Impacts Interacting in the Marine Environment Using Marine Infrastructure as an Example. Sci. Total Environ., 154748. doi: 10.1016/j.scitotenv.2022.154748
Kortenkamp A., Faust M. (2018). Regulate to Reduce Chemical Mixture Risk. Science 361, 224–226. doi: 10.1126/science.aat9219
Lavoie R. A., Bouffard A., Maranger R., Amyot M. (2018). Mercury Transport and Human Exposure From Global Marine Fisheries. Sci. Rep. 8, 1–9. doi: 10.1038/s41598-018-24938-3
Lefcheck J. S., Orth R. J., Dennison W. C., Wilcox D. J., Murphy R. R., Keisman J., et al. (2018). Long-Term Nutrient Reductions Lead to the Unprecedented Recovery of a Temperate Coastal Region. Proc. Natl. Acad. Sci. 115, 3658–3662. doi: 10.1073/pnas.1715798115
Leung K. M. (2018). Joining the Dots Between Omics and Environmental Management. Integrated Environ. Assess. Manage. 14, 169–173. doi: 10.1002/ieam.2007
Liu T. K., Wang M. W., Chen P. (2013). Influence of Waste Management Policy on the Characteristics of Beach Litter in Kaohsiung, Taiwan. Marine Pollution Bull. 72, 99–106. doi: 10.1016/j.marpolbul.2013.04.015
Marginson S. (2021). ‘All Things are in Flux’: China in Global Science. Higher Educ. 83, 1–30. doi: 10.1007/s10734-021-00712-9
Martin O., Scholze M., Ermler S., McPhie J., Bopp S. K., Kienzler A., et al. (2021). Ten Years of Research on Synergisms and Antagonisms in Chemical Mixtures: A Systematic Review and Quantitative Reappraisal of Mixture Studies. Environ. Int. 146, 106206. doi: 10.1016/j.envint.2020.106206
Matthies M., Solomon K., Vighi M., Gilman A., Tarazona J. V. (2016). The Origin and Evolution of Assessment Criteria for Persistent, Bioaccumulative and Toxic (PBT) Chemicals and Persistent Organic Pollutants (Pops). Environ. Sci.: Processes Impacts 18, 1114–1128. doi: 10.1039/C6EM00311G
Mohamed H., Nadaoka K., Nakamura T. (2018). Assessment of Machine Learning Algorithms for Automatic Benthic Cover Monitoring and Mapping Using Towed Underwater Video Camera and High-Resolution Satellite Images. Remote Sensing 10 (5), 773. doi: 10.3390/rs10050773
Morris R. L., Heery E. C., Loke L. H., Lau E., Strain E., Airoldi L., et al. (2019). Design Options, Implementation Issues and Evaluating Success of Ecologically Engineered Shorelines. Oceanography Marine Biol.: an Annu. Rev. 57, 169–228.
Morris L., O’brien A., Natera S. H., Lutz A., Roessner U., Long S. M. (2018). Structural and Functional Measures of Marine Microbial Communities: An Experiment to Assess Implications for Oil Spill Management. Marine Pollution Bull. 131, 525–529. doi: 10.1016/j.marpolbul.2018.04.054
Noone K. J., Sumaila U. R., Diaz R. J. (2013). Managing Ocean Environments in a Changing Climate: Sustainability and Economic Perspectives (St Louis: Newnes).
O’Brien A. L., Keough M. J. (2014). Ecological response to contamination: A meta-analysis of experimental marine studies. Environmental Pollution 195, 185–191.
O’Brien A., Dafforn K., Chariton A., Johnston E., Mayer-Pinto M. (2019). After Decades of Stressor Research in Urban Estuarine Ecosystems the Focus is Still on Single Stressors: A Systematic Literature Review and Meta-Analysis. Sci. Total Environ. 684, 753–764. doi: 10.1016/j.scitotenv.2019.02.131
Orr J. A., Vinebrooke R. D., Jackson M. C., Kroeker K. J., Kordas R. L., Mantyka-Pringle C., et al. (2020). Towards a Unified Study of Multiple Stressors: Divisions and Common Goals Across Research Disciplines. Proc. R. Soc. B 287, 20200421. doi: 10.1098/rspb.2020.0421
Orth R. J., Wilcox D. J., Whiting J. R., Nagey L. S., Kenne A. K., Smith E. R. (2017). 2016 Distribution of Submerged Aquatic Vegetation in Chesapeake Bay and Coastal Bays (Virginia, USA: Virginia Institute of Marine Science, College of William and Mary).
Piarulli S., Hansen B. H., Ciesielski T., Zocher A. L., Malzahn A., Olsvik P. A., et al. (2021). Sources, Distribution and Effects of Rare Earth Elements in the Marine Environment: Current Knowledge and Research Gaps. Environ. Pollution 291, 118230. doi: 10.1016/j.envpol.2021.118230
Piggott J. J., Townsend C. R., Matthaei C. D. (2015). Reconceptualizing Synergism and Antagonism Among Multiple Stressors. Ecol. Evol. 5, 1538–1547. doi: 10.1002/ece3.1465
Rivero N. K., Dafforn K. A., Coleman M. A., Johnston E. L. (2013). Environmental and Ecological Changes Associated With a Marina. Biofouling 29, 803–815. doi: 10.1080/08927014.2013.805751
Rosevelt C., Los Huertos M., Garza C., Nevins H. (2013). Marine Debris in Central California: Quantifying Type and Abundance of Beach Litter in Monterey Bay, CA. Marine Pollution Bull. 71, 299–306. doi: 10.1016/j.marpolbul.2013.01.015
Salgado-Hernanz P. M., Bauzà J., Alomar C., Compa M., Romero L., Deudero S. (2021). Assessment of Marine Litter Through Remote Sensing: Recent Approaches and Future Goals. Marine Pollution Bull. 168, 112347. doi: 10.1016/j.marpolbul.2021.112347
Schäfer R. B., Piggott J. J. (2018). Advancing Understanding and Prediction in Multiple Stressor Research Through a Mechanistic Basis for Null Models. Global Change Biol. 24, 1817–1826. doi: 10.1111/gcb.14073
Seltenrich N. (2015). New Link in the Food Chain? Marine Plastic Pollution and Seafood Safety (USA: NLM-Export).
Sinclair G. M., O’Brien A. L., Keough M., De Souza D. P., Dayalan S., Kanojia K., et al. (2019). Using Metabolomics to Assess the Sub-Lethal Effects of Zinc and Boscalid on an Estuarine Polychaete Worm Over Time. Metabolomics 15, 1–13. doi: 10.1007/s11306-019-1570-x
Small C., Nicholls R. J. (2003). A Global Analysis of Human Settlement in Coastal Zones. Journal of Coastal Research, 584–99.
Smart A. S., Tingley R., Weeks A. R., Van Rooyen A. R., McCarthy M. A. (2015). Environmental DNA Sampling is More Sensitive Than a Traditional Survey Technique for Detecting an Aquatic Invader. Ecol. Appl. 25, 1944–1952. doi: 10.1890/14-1751.1
Strain E., Alexander K., Kienker S., Morris R., Jarvis R., Coleman R., et al. (2019). Urban Blue: A Global Analysis of the Factors Shaping People’s Perceptions of the Marine Environment and Ecological Engineering in Harbours. Sci. Total Environ. 658, 1293–1305. doi: 10.1016/j.scitotenv.2018.12.285
Strain E., Heath T., Steinberg P., Bishop M. (2018a). Eco-Engineering of Modified Shorelines Recovers Wrack Subsidies. Ecol. Eng. 112, 26–33. doi: 10.1016/j.ecoleng.2017.12.009
Strain E. M., Olabarria C., Mayer-Pinto M., Cumbo V., Morris R. L., Bugnot A. B., et al. (2018b). Eco-Engineering Urban Infrastructure for Marine and Coastal Biodiversity: Which Interventions Have the Greatest Ecological Benefit? J. Appl. Ecol. 55, 426–441. doi: 10.1111/1365-2664.12961
Strain E. M., Thomson R. J., Micheli F., Mancuso F. P., Airoldi L. (2014). Identifying the Interacting Roles of Stressors in Driving the Global Loss of Canopy-Forming to Mat-Forming Algae in Marine Ecosystems. Global Change Biol. 20, 3300–3312. doi: 10.1111/gcb.12619
Swearer S. E., Morris R. L., Barrett L. T., Sievers M., Dempster T., Hale R. (2021). An Overview of Ecological Traps in Marine Ecosystems. Front. Ecol. Environ. 19, 234–242. doi: 10.1002/fee.2322
Tournadre J. (2014). Anthropogenic Pressure on the Open Ocean: The Growth of Ship Traffic Revealed by Altimeter Data Analysis. Geophysical Res. Lett. 41, 7924–7932. doi: 10.1002/2014GL061786
Van Cauwenberghe L., Vanreusel A., Mees J., Janssen C. R. (2013). Microplastic Pollution in Deep-Sea Sediments. Environ. Pollution 182, 495–499. doi: 10.1016/j.envpol.2013.08.013
Van den Brink P. J., Bracewell S. A., Bush A., Chariton A., Choung C. B., Compson Z. G., et al. (2019). Towards a General Framework for the Assessment of Interactive Effects of Multiple Stressors on Aquatic Ecosystems: Results From the Making Aquatic Ecosystems Great Again (MAEGA) Workshop. Sci. Total Environ. 684, 722–726. doi: 10.1016/j.scitotenv.2019.02.455
Verfuss U. K., Aniceto A. S., Harris D. V., Gillespie D., Fielding S., Jiménez G., et al. (2019). A Review of Unmanned Vehicles for the Detection and Monitoring of Marine Fauna. Marine Pollution Bull. 140, 17–29. doi: 10.1016/j.marpolbul.2019.01.009
Wang W., Gao H., Jin S., Li R., Na G. (2019). The Ecotoxicological Effects of Microplastics on Aquatic Food Web, From Primary Producer to Human: A Review. Ecotoxicol. Environ. Saf. 173, 110–117. doi: 10.1016/j.ecoenv.2019.01.113
Warne M. S. J., Hawker D. W. (1995). The Number of Components in a Mixture Determines Whether Synergistic and Antagonistic or Additive Toxicity Predominate: The Funnel Hypothesis. Ecotoxicol. Environ. Saf. 31, 23–28. doi: 10.1006/eesa.1995.1039
Wilkinson J. L., Boxall A. B., Kolpin D. W., Leung K. M., Lai R. W., Galbán-Malagón C., et al. (2022). Pharmaceutical Pollution of the World’s Rivers. Proc. Natl. Acad. Sci. 119, e2113947119. doi: 10.1073/pnas.2113947119
Williams M., Longstaff B., Buchanan C., Llansó R., Dennison W. (2009). Development and Evaluation of a Spatially-Explicit Index of Chesapeake Bay Health. Marine Pollution Bull. 59, 14–25. doi: 10.1016/j.marpolbul.2008.11.018
Wurtsbaugh W. A., Paerl H. W., Dodds W. K. (2019). Nutrients, Eutrophication and Harmful Algal Blooms Along the Freshwater to Marine Continuum. Water 6, e1373. doi: 10.1002/wat2.1373
Keywords: nutrient enrichment, heavy metals, persistent organic pollutants, plastic debris, artificial structures, multiple stressors, management options
Citation: Strain EMA, Lai RWS, White CA, Piarulli S, Leung KMY, Airoldi L and O’Brien A (2022) Editorial: Marine Pollution - Emerging Issues and Challenges. Front. Mar. Sci. 9:918984. doi: 10.3389/fmars.2022.918984
Received: 13 April 2022; Accepted: 16 May 2022;
Published: 08 June 2022.
Edited by:
Ilaria Corsi, University of Siena, ItalyReviewed by:
Julian Alberto Gallego Urrea, Horiba Europe, SwedenJulian Blasco, Spanish National Research Council (CSIC), Spain
Copyright © 2022 Strain, Lai, White, Piarulli, Leung, Airoldi and O’Brien. This is an open-access article distributed under the terms of the Creative Commons Attribution License (CC BY). The use, distribution or reproduction in other forums is permitted, provided the original author(s) and the copyright owner(s) are credited and that the original publication in this journal is cited, in accordance with accepted academic practice. No use, distribution or reproduction is permitted which does not comply with these terms.
*Correspondence: Elisabeth Marijke Anne Strain, ZWxpc2FiZXRoLnN0cmFpbkB1dGFzLmVkdS5hdQ==