- 1Marine Ecology Research Centre, Southern Cross University, Lismore, NSW, Australia
- 2Faculty of Science and Engineering, Southern Cross University, Lismore, NSW, Australia
- 3The National Sea Simulator, Australian Institute of Marine Science, Townsville, QLD, Australia
Intensifying anthropogenic stressors have contributed to declines in reef-building corals in many regions. These disturbances result in reduced live coral cover, impacting key population-level processes such as coral larval settlement and recruitment that are essential for reef recovery. Reef restoration efforts that rely on enhanced larval supply provide a pathway for the recovery of degraded reefs. However, corals at very early life stages experience high post-settlement mortality bottlenecks, which impede stock-recruitment processes. Overcoming these bottlenecks is a high priority goal in coral restoration. Some coral larvae are known to be capable of gaining exogenous nutrients. Therefore, we hypothesised that the capacity to access exogenous nutrients may confer advantages to larval survival, settlement and post-settlement success. The present study aimed to quantify the effect of larval feeding on coral larvae settlement and early post-settlement survival. We completed an ex-situ experiment using aposymbiotic larvae of two broadcast spawning reef-building coral species - Acropora tenuis and Acropora millepora. Larvae were randomly assigned to either fed or unfed treatment groups for each species. Fed larvae received homogenised Artemia once a day, for three days. Results show that for both species, feeding significantly increased larval settlement. Feeding A. millepora larvae more than doubled mean settlement (13.0 ± 1.17 SE vs 31.4 ± 2.88 SE; p <0.001). Similarly, feeding A. tenuis larvae increased mean settlement from 18.2 ( ± 1.85 SE) to 29.9 ( ± 2.22 SE; p <0.001). Larval feeding had an immediate positive effect on spat survival, such that A. millepora and A. tenuis spat from fed treatments had increased survival three days post-settlement (89.5% ± 3.75 SE vs 70.6% ± 2.59 SE, p <0.001; 88.8% ± 2.21 SE vs 71.4% ± 3.80 SE, p <0.001, respectively). Therefore, enhancing settlement and early post-settlement survival by feeding larvae homogenised Artemia has the potential to improve the effectiveness of larval rearing protocols and coral restoration efforts.
Introduction
Coral reefs are vulnerable ecosystems impacted by a range of environmental disturbances across small and large spatial scales (Bellwood et al., 2004) and from natural and anthropogenic sources (Hughes et al., 2003; Burke et al., 2011). Intensifying anthropogenic impacts, including climate change, have contributed to recent declines in reef-building corals (Hoegh-Guldberg et al., 2007; De’Ath et al., 2012; Hughes et al., 2018). Declines in live coral cover can impact key population-level processes, including reproductive capacity and concomitant replenishment of corals via coral larval recruitment, a primary driver of recovery on degraded reefs (Harrison and Wallace, 1990; Mumby and Harborne, 2010; Gouezo et al., 2019). These early life stages often represent a bottleneck in the successful reproduction and recruitment process for marine invertebrates (Pineda, 2000). Therefore, negatively impacted stock-recruitment processes reduce the overall ability for coral communities and reefs to naturally recover (Hughes et al., 2019).
Reef restoration is a pathway to recover degraded reefs and is increasingly included in natural resource management (Edwards, 2010; Omori, 2019; Boström-Einarsson et al., 2020). Coral restoration has routinely relied on using asexually produced coral fragments to restore corals (Rinkevich, 2005), but the use of sexually derived coral larvae for coral and reef restoration is rapidly increasing (dela Cruz and Harrison, 2017; Doropoulos et al., 2019; dela Cruz and Harrison, 2020; Harrison et al., 2021). Using coral larvae for restoration relies on the high fecundity of broadcast spawning coral colonies (Harrison and Wallace, 1990), allowing collection of millions of gametes on reefs and in laboratory settings. Collected gametes enable the mass culture of larvae which allows restoration efforts to supply sufficiently high numbers of larvae to degraded reefs. Equally critical is ensuring settlement of coral larvae and metamorphosis into settled polyps, and survival and growth into juvenile corals. These early life stages are an essential step in recruitment to adult populations (Harrison and Wallace, 1990; Richmond, 1997) and dictate the effectiveness of restoration efforts (Boström-Einarsson et al., 2020).
Most wild-spawned coral larvae die or are lost from reef systems during their planktonic larval phase or in the first few weeks and months post-settlement (Harrison and Wallace, 1990; Wilson and Harrison, 2005; Doropoulos et al., 2016; dela Cruz and Harrison, 2017; Harrison et al., 2021), and there remain many questions around the biological determinants of settlement and recruitment processes for corals. For example, endogenous energy stores in non-feeding larvae are a limiting factor that dictate settlement outcomes (Strathmann, 1985; Marshall and Keough, 2003; Botello and Krug, 2006; Graham et al., 2013a). Egg provisioning impacts larval competency and the initial stages of settlement and post-settlement survival. Prolonged larval phases are thought to decrease settlement rates as energy stores are depleted, thereby compromising the potential to complete metamorphosis with increasing age (Wilson and Harrison, 1998; Graham et al., 2008). Therefore, the dynamic interplay between larval endogenous energy stores, settlement success and the latent effects on recruitment success highlight the importance of understanding the link between larval energetics and restoration efforts (Harrison and Wallace, 1990; Graham et al., 2013b).
While coral larvae are considered lecithotrophic, provisioned with sufficient energy needed during their larval phase, competency periods for broadcast spawning coral larvae are often longer than energetic models predict (Tranter et al., 1982; Wilson and Harrison, 1998; Nozawa and Harrison, 2002). As a result, some researchers suggest that certain species of coral larvae gain exogenous nutrients to bridge these gaps (Fadlallah, 1983; Arai et al., 1993; Zaslow and Benayahu, 1996; Graham et al., 2013a; Rivest et al., 2017). One important source of nutrients for coral larvae appears to be dissolved organic material (DOM) (Sorokin, 1973; Fadlallah, 1983; Graham et al., 2013a). Moreover, there is evidence that free amino acids contribute up to 11% of the metabolic needs of coral larvae (Zaslow and Benayahu, 2000) and may play a role in inducing settlement (Baird and Morse, 2004). Other nutrients may also be physiologically important, for example, Pocillopora damicornis larvae have been found to lack sufficient quantities of nitrogen and phosphorous necessary for metamorphosis (Titlyanov et al., 1998). Similarly, when deprived of all sources of exogenous nutrients, Porites porites larvae cannot settle successfully (Fadlallah, 1983).
In addition to the assimilation of DOM, there is evidence that some coral larvae actively feed on particulate matter via ciliary currents and mucus strings (Tranter et al., 1982; Harii et al., 2009). The use of ciliary currents to move particulate matter to the oral pore has been observed in the larvae of Stylophora pistillata (Rinkevich and Loya, 1979), Cyphastrea ocellina (Wright, 1986), Pocillopora damicornis (Richmond, 1985) and Fungia scutaria (Krupp, 1983), and is common in other single-banded ciliated invertebrates (Strathmann et al., 1972; Paulay et al., 1985). In conjunction with ciliary currents, larvae have been observed using mucus strings to capture food particles (Tranter et al., 1982; Schwarz et al., 1999; Harii et al., 2009) in a process known as mucociliary transport (Young, 1971; Brown and Bythell, 2005). In this process, nutritive particulate matter is captured in mucus and transported to the oral pore, where it is then ingested (Tranter et al., 1982; Schwarz et al., 1999; Harii et al., 2009). With evidence that certain species of coral larvae are capable of gaining exogenous nutrients, the capacity to access exogenous nutrients may confer advantages to larval survival, settlement and post-settlement success.
Therefore, a strict dichotomy of binary nutritional modes - feeding or non-feeding - does not suit the wide biological variation seen among coral larvae. Alternatively, researchers have proposed an intermediate nutritional mode - facultative planktotrophy - to account for similar variation in other non-coral marine invertebrates (Allen and Pernet, 2007; Collin, 2012; Pernet, 2018). This nutritional mode is characterised by larvae capable of feeding but which are not required to do so to complete metamorphosis (Boidron-Métairon, 1995). Furthermore, when fed, facultative planktotrophic larvae often metamorphose into larger juveniles and exhibit increased post-metamorphic survivorship (Boidron-Métairon, 1995; McEdward, 2000; Allen and Pernet, 2007). However, to date, there are limited data that examine the relationships between the supply of exogenous nutrients and settlement in coral larvae. Therefore, these critical aspects of coral larval biology and ecology warrant more detailed investigation. Hence, the present study aims to quantify the effect of feeding on coral larval settlement and early post-settlement survival. Specifically, we hypothesise that rearing larvae with access to exogenous nutrients will enhance settlement and potentially improve post-settlement survival.
Materials and Methods
This experiment was conducted on Bindal country at the Australian Institute of Marine Science’s (AIMS) National Sea Simulator (SeaSim) in Townsville, Australia, to assess the effect of supplying nutrients to coral larvae on their settlement and early post-settlement survival. Aposymbiotic larvae of two broadcast spawning corals were used - Acropora tenuis and Acropora millepora. Both species are widespread in the Indo-Pacific region and common on a range of reef sites (Wallace, 1999). Acropora tenuis has been previously used in ex-situ larval restoration studies (dela Cruz and Harrison, 2017; Cameron and Harrison, 2020; Harrison et al., 2021). Furthermore, both species have been successfully spawned and reared in aquaria with well-established culturing and settlement techniques (Heyward and Negri, 1999; Humanes et al., 2016; Conlan et al., 2017; Pollock et al., 2017).
Coral Collection
Gravid colonies of each species were collected from near-shore and mid-shelf reefs on Wulgurukaba and Bindal Sea Country on the central Great Barrier Reef in the days leading up to the November 2020 full moon and transported to SeaSim (GBRMPA Permit G12/35236.1).
Larval Culture
On arrival at SeaSim, colonies were transferred to outdoor flow-through holding tanks and maintained at 27°CC ( ± 0.5°CC) under 30% shaded natural sunlight until spawning occurred. Once spawning occurred, gamete bundles were skimmed from the surface, and a 60µm mesh sieve was used to separate eggs and sperm. Eggs were then transferred to a separate 60L tank filled with 1µm filtered seawater. Sperm were then added at a concentration of 1 x 106 sperm/ml (Willis et al., 1997), and after two hours, when embryos were observed to be cleaving, they were rinsed and transferred to a 500L culture tank. Light aeration was added after 24hrs.
Experimental Design
Rearing
Thirty-six hours after spawning, larvae were transferred to individual rearing tanks, which consisted of 14L aerated cone-shaped plastic flow-through tanks. All tanks had a 12L per hour flow rate using 1µm filtered seawater, equating to a complete water turnover every 70 mins. Fluorescent lights over the tanks were set on a 12:12 hr timer with the first light at 6 am and the last light at 6 pm. The average light level over the rearing tanks was 40 µmoles m-2 s-1. Water temperature in the tanks remained at 27°CC (± 0.5°CC) for the duration of the experiment. Acropora tenuis larvae were added at one larva per 5 ml, resulting in approximately 2800 larvae per tank. Acropora millepora larvae were added at a concentration of 0.16 larvae per ml, with about 2250 larvae per tank.
All tanks with larvae were randomly assigned to one of two treatments, either unfed or fed for each species. Both species had the same number of unfed replicates (n = 4). However, due to larval supply differences, there was an uneven number of fed replicates for A. millepora larvae (n = 3) and A. tenuis larvae (n = 5). In the unfed treatment, larvae were reared without exogenous food supplied in ultrafiltered seawater (<0.05 µm). In the fed treatment, larvae received 50ml of homogenised Artemia at 4,185 nauplii per ml daily for three days. Food was provided on days six, seven, and eight after the spawning period and the onset of embryo and larval development. The water supply remained on when the food was administered to maintain water quality and avoid a build-up of organic matter that could lead to diseases. Therefore, the food was cleared approximately 70 mins after being provided.
Settlement
Nine days after spawning, larvae were transferred from rearing tanks to settlement tanks. Replicates were kept consistent between the rearing and settlement phases. Each settlement tank consisted of a 5L rectangular flow-through plastic container that housed custom made PVC settlement trays (300mm x 150mm x 20mm) with a grid of 50 holes (dia. 20mm) drilled into the top surface so that aragonite settlement plugs (dia. 20mm) could be inserted flush with the surface of the tray. Each settlement tray had twenty-five settlement plugs that had been biologically conditioned in a flow-through seawater system for six weeks prior to experiments to develop biofilms and crustose coralline algae (CCA) which are known settlement inducers for A. tenuis and A. millepora larvae. The remaining 25 plugs were not conditioned prior to experiments and therefore contained no CCA. Unconditioned plugs were rinsed in freshwater before being added to the settlement tray to remove any loose aragonite. The unconditioned plugs were used to fill the remaining plug holes to create a level surface across the settlement tray. Coral larvae settle preferentially on conditioned plugs. Light over each settlement tray was provided by Aqua Illumination Sol LED lights, simulating daylight and day length cycles. The mean light intensity over the settlement containers was 40 µmoles m-2 s-1. The first light occurred at 6 am with a 5-hour ramp up time. The light remained at maximum intensity for 2 hours from 11 am until 1 pm, at which point light intensity decreased until the last light at 6 pm. Water temperature remained consistent at 27°CC ( ± 0.5°CC).
Data Collection and Statistical Analysis
At days three and seven, after introducing larvae into the settlement tanks, coral settlement plugs within each tank were photographed using a high-resolution Nikon camera system attached to a pre-programmed robotic mechanism. The first timepoint approximated the ecological time frame for settlement to occur, including searching for a settlement surface and metamorphosing into a coral spat. The second time point was arbitrarily chosen to aid in logistical planning allowing for the capture of early mortality. Each photo was analysed by counting the coral spat present on each conditioned settlement plug. Coral spat were identified as larvae that had fully attached to the settlement plug and metamorphosed into a single polyp with a mouth and skeletal structure (Figure 1). Spat were included in plug counts if they were attached to the conditioned plug or in the crevice between the plug and tray if it was clearly attached to the settlement plug. Spat attached to the settlement tray, or an unconditioned plug, were counted separately. Additionally, any spat that had lost colour and appeared white and displayed tissue deterioration were presumed to be dead (Figure 1). Three days post-settlement, for each conditioned plug, all coral spat were counted and identified as either alive or dead. At seven days post settlement, only live coral spat were counted.
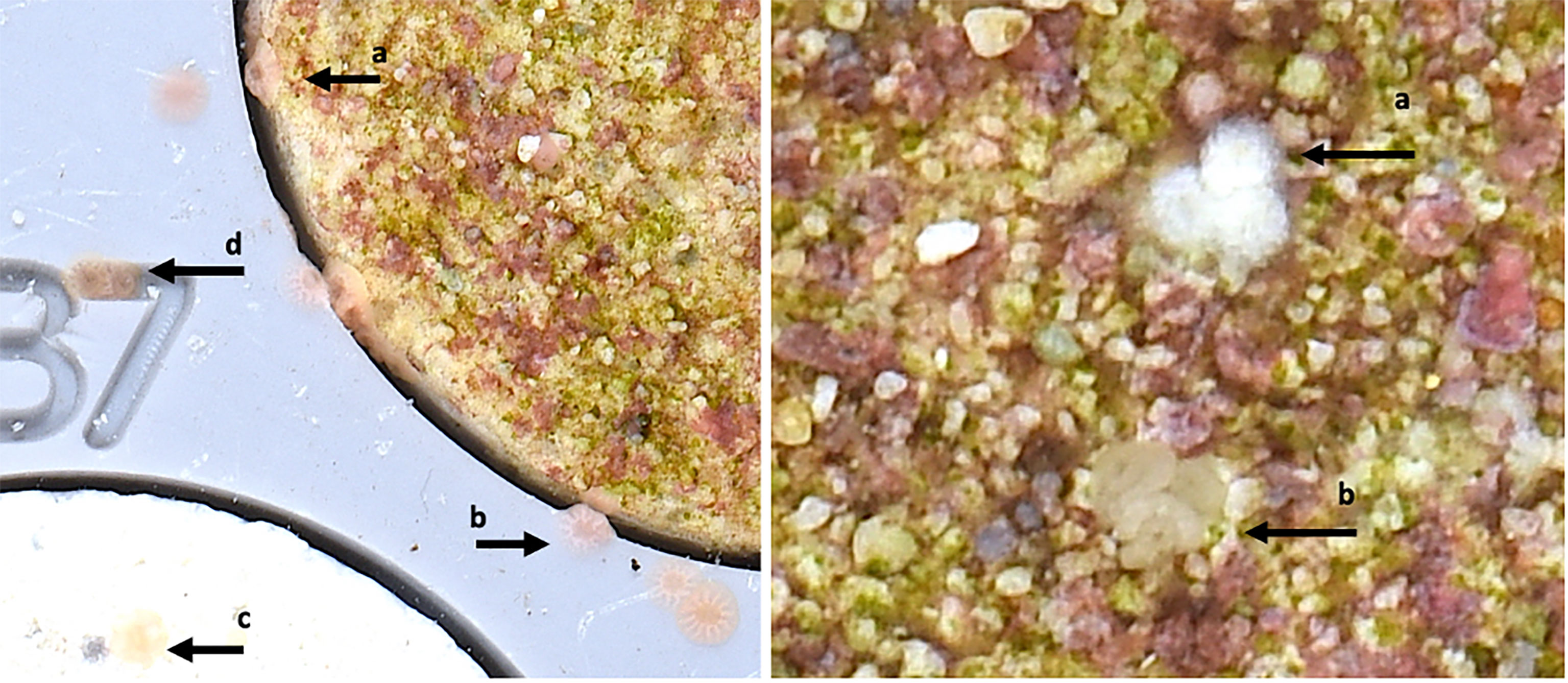
Figure 1 (Left) Acropora tenuis spat showing various settlement locations, (a) spat on a conditioned settlement plug (b) spat on the PVC settlement tray, (c) spat on an unconditioned settlement plug, (d) spat in the crevice of the plug identification number. (Right) Examples of a cluster of opaque white dead A. millepora spat (a), and a cluster of alive A. millepora spat (b) on a conditioned settlement plug.
Spat counts of each species were calculated for each treatment. The three-day settlement counts resulted in a mean number of live, dead, and total spat per conditioned plug for each fed and unfed treatment. Together, spat on the tray, unconditioned plugs, and conditioned plugs were combined yielding total settlement for each replicate. The number of dead recruits at and the difference between the number of spat at three days post settlement was used to calculate a three-day survival rate. At seven days post-settlement, the spat counts yielded the mean number of surviving recruits per plug for each treatment. The difference in numbers of spat present between each plug at three days compared to seven days was used to calculate the percentage of surviving spat. As with mean settlement, the percentage of surviving spat was calculated for each treatment, yielding the mean survival within treatment groups.
The mean settlement of each category of spat between the unfed and fed treatment groups was analysed using a paired sample t-test. To meet the variance assumption of the test, variances were tested using an F-test for variance. The variance was found to vary, and therefore, the paired-sample t-test assuming unequal variances was used. T-tests were run on the mean settlement at three days post-settlement, and seven days post-settlement for each species. The mean survival between the unfed and fed treatment groups was also measured using a paired sample t-test assuming unequal variances for each species.
Results
Larval Settlement
Total larval settlement was first censused three days after exposure to settlement cues. For both A. millepora and A. tenuis larval settlement was significantly higher in fed treatments compared to unfed treatments (Table 1; Figure 2). At the first census period, the total mean settlement, including both alive and dead spat, was significantly higher in fed A. millepora larvae than unfed larvae (31.4 ± 2.88 SE vs 13.0 ± 1.17 SE, respectively t(65) = 5.91, p <0.001) (Figure 2A). The mean number of alive spat was also significantly different between the fed and unfed treatments for A. millepora (Figure 3) whereby the mean number of alive A. millepora spat was higher in the fed treatments than in the unfed treatments (29.2 ± 3.05 SE vs 10.6 ± 1.22 SE, t(65) = 5.65, p <0.001) (Figure 3A).
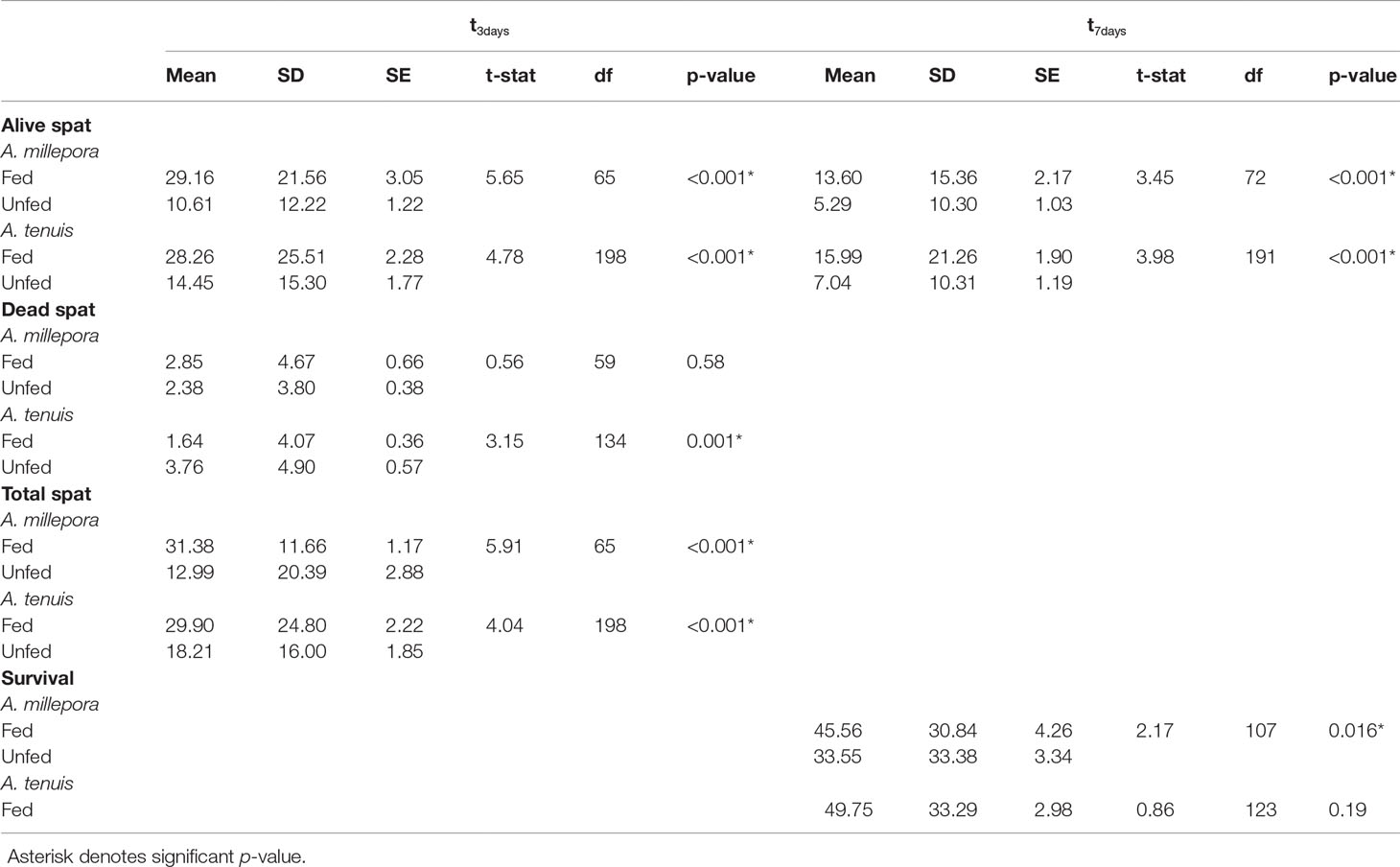
Table 1 Observed mean, standard deviation (SD), standard error (SE), t-statistic, degrees of freedom (df), and p values for each t-test performed at either three (t3days) or seven days (t7days) after the introduction of settlement cues.
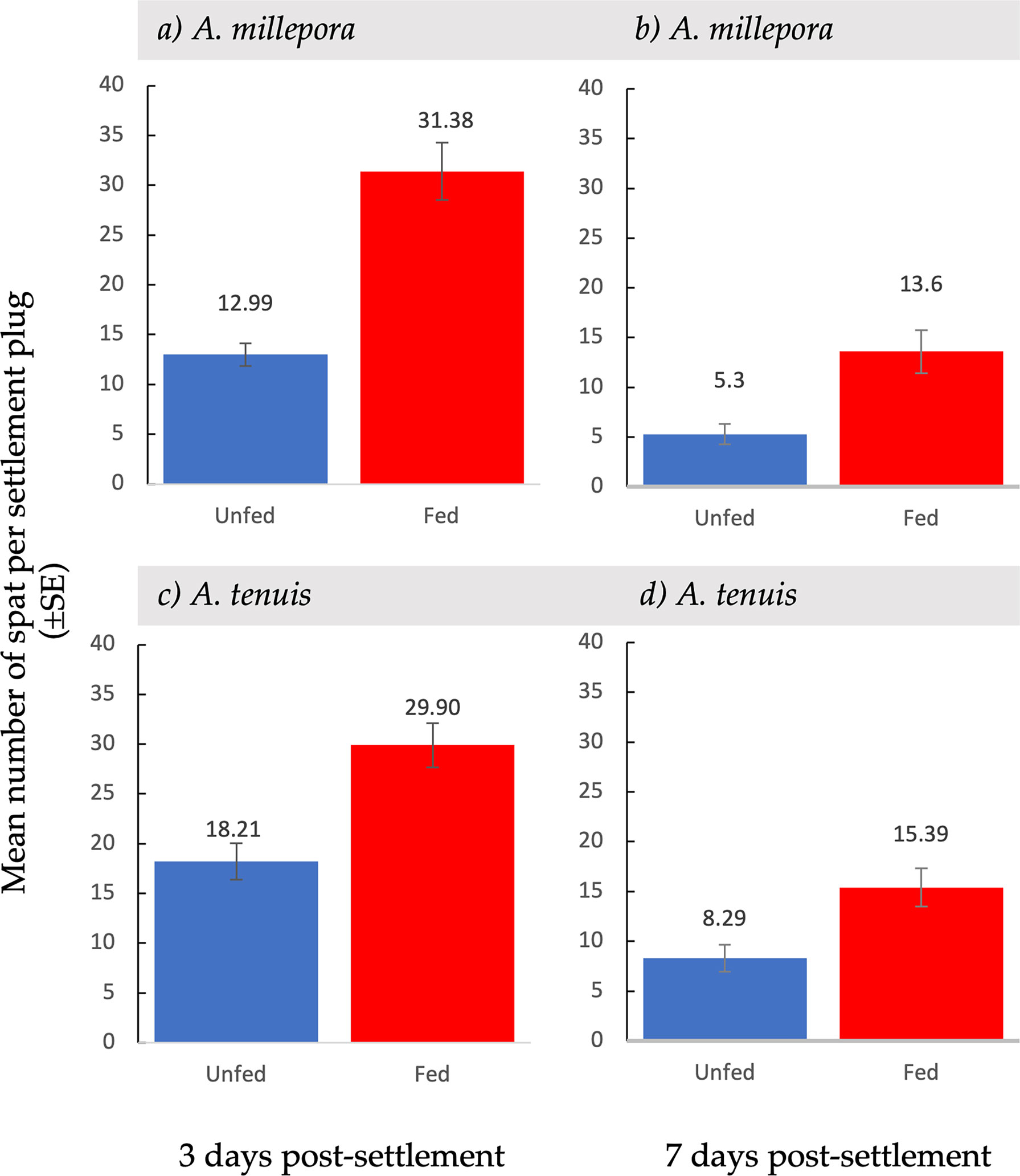
Figure 2 Mean settlement ( ± SE) of unfed and fed Acropora millepora (A, B) and Acropora tenuis larvae (C, D), after three days (A, C) and after seven days (B, D) after larvae were provided with settlement surfaces.
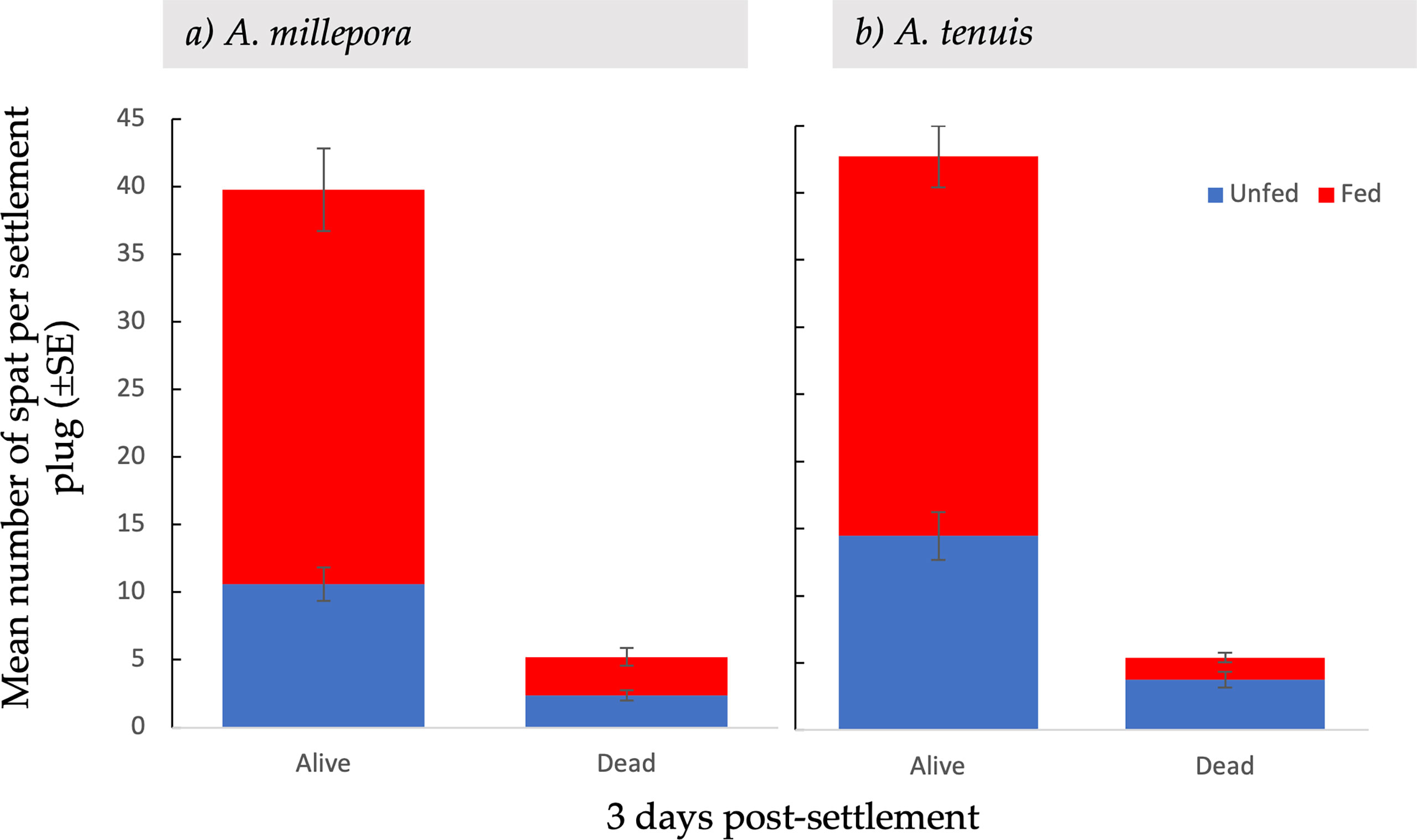
Figure 3 Mean settlement ( ± SE) of alive and dead Acropora millepora (A) and Acropora tenuis spat (B), from unfed and fed treatments, three days after the introduction of settlement cues.
Similarly, three days after larvae were exposed to settlement cues, the total mean settlement of fed A. tenuis larvae that settled was significantly higher than unfed larvae (29.9 ± 2.22 SE vs 18.2 ± 1.85 SE, t(198) = 4.04, p<0.01) (Figure 2C). The mean number of settled A. tenuis larvae alive at the three-day census period was significantly higher in the fed treatments than in the unfed treatments (28.3 ± 2.28 SE vs 14.5 ± 1.77 SE, t(198) = 4.78, p <0.001) (Figure 3B). This trend remained consistent over the first-week post-settlement.
Overcoming high post-settlement mortality bottlenecks is a high priorityIn A. millepora larvae, the trend of higher settlement in fed treatments was maintained when all spat were counted. The average number of settled larvae, including those on unconditioned surfaces in the unfed treatment, was more than half that of the fed larvae (436.3 ± 74.53 SE vs 1000.5 ± 34.50 SE, respectively, t(2) = 5.37 p = 0.017). Of the total number of A. millepora spat across all fed treatments, 78.4% settled on conditioned settlement surfaces (1569 vs 432 spat). Meanwhile, A. millepora larvae across all unfed treatments settled on an unconditioned settlement surface 74.4% of the time (1299 vs 446 spat). The mean total spat per settlement tray was significantly higher in A. millepora larvae (p = 0.016). Similarly, the mean totalsettlement per settlement tray in A. tenuis larvae was twice as high in the fed treatments (1146.2 ± 279.00 SE) than those in the unfed treatments (619.3 ± 244.06 SE). However, the difference in the mean total settlement per settlement tray was not statistically significant between the fed and unfed treatments (t(6) = 1.42, p = 0.21). As with the A. millepora larvae, A. tenuis larvae from fed treatments settled on conditioned settlement surfaces 65.2% of the time (3737 vs 1994 spat) while 73.5% of larvae from unfed treatments settled on conditioned settlement surfaces (1366 vs 492).
Post-Settlement Survival
Three Days After Exposure to Settlement Cues
At the first census period, survival and mortality were recorded. The mean number of dead spat per conditioned plug varied between A. millepora and A. tenuis (Figure 3). Specifically, the mean number of dead A. tenuis spat was significantly higher in the unfed treatments than in the fed treatments (t(134) = 3.15, p = 0.001) (Figure 3B). The mean number of dead A. millepora spat was consistent between fed and unfed treatments (2.9 ± 0.66 SE vs 2.4 ± 0.38 SE, t(59) = 0.56, p = 0.58) (Figure 3B).
Spat survival three days post-settlement varied between fed and unfed treatments (Figure 4). Mean survival of A. millepora spat three days post-settlement was significantly higher in fed treatments than in unfed treatments (t(146) = 4.11, p <0.001) (Figure 4A). Spat from fed treatments exhibited an 89.5% ( ± 2.59 SE) survival rate while spat from unfed treatments had a 70.6% ( ± 3.75 SE) survival rate. Three days post settlement, A. tenuis spat from fed treatments, also had a significantly higher survival rate than spat from unfed treatments (t(120) = 3.91, p <0.001) (Figure 4C). Spat from fed treatment had a survival rate of 88.8% ( ± 2.21 SE) while spat from unfed treatments had a 71.4% ( ± 3.80 SE) survival rate.
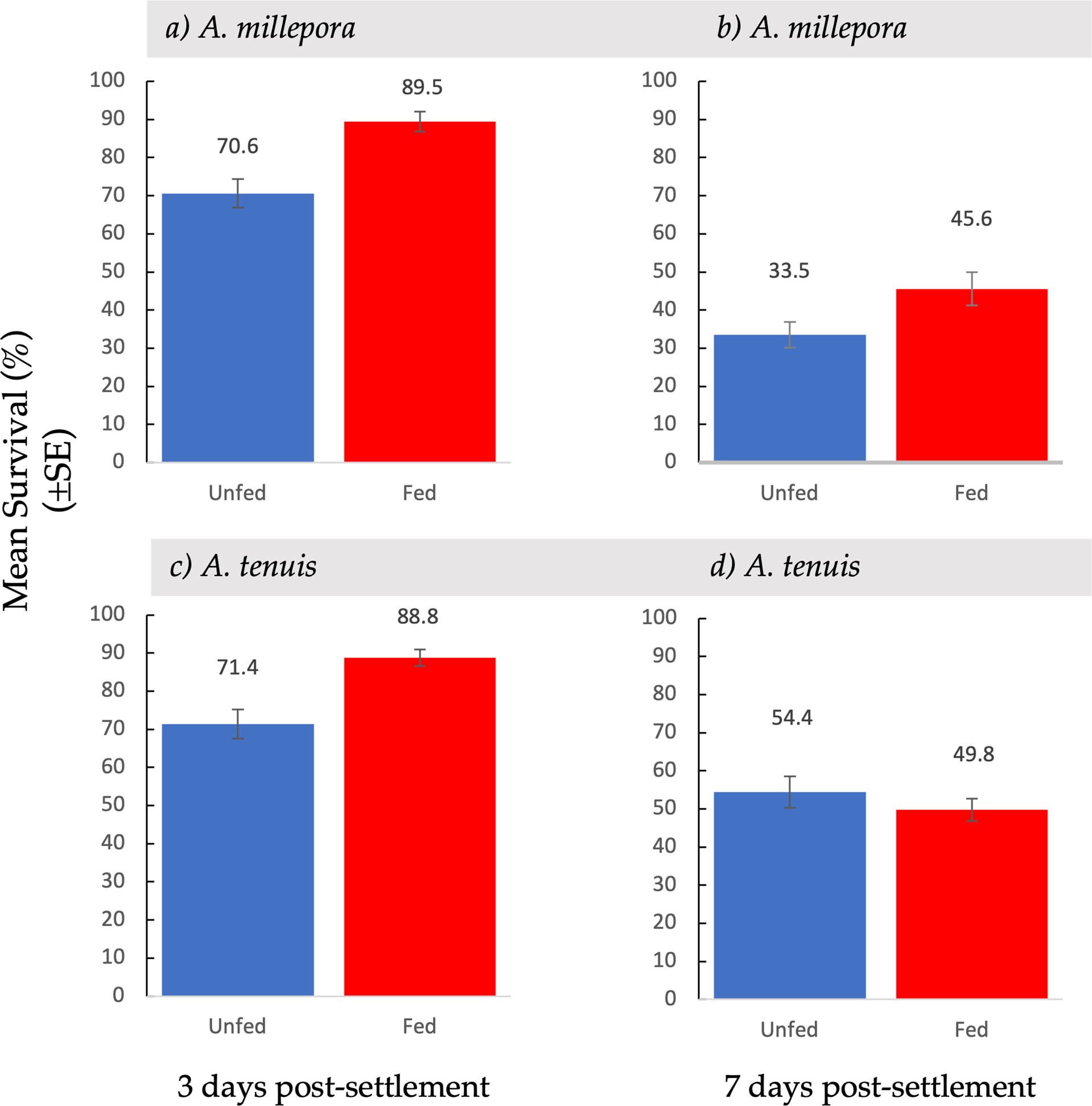
Figure 4 Mean survival ( ± SE) of Acropora millepora (A, B) and Acropora tenuis spat (C, D) from unfed and fed treatments, three days (A, C) and seven days (B, D) after larvae were provided with settlement surfaces.
Seven Days After the Exposure to Settlement Cues
At the second census period, seven days after the introduction of settlement cues, survival was recorded again. Feeding treatments had a variable effect on spat survival seven days post-settlement (Figure 4). Acropora millepora spat from fed treatments had a significantly higher survival rate than spat from unfed treatments (t(107) = 2.17, p <0.05) (Figure 4B). Spat from fed treatments showed a 45.6% ( ± 4.36 SE) survival rate, while spat from unfed treatments had a 33.5% ( ± 3.34 SE) survival rate. There was a 30% increase in survival of fed A. millepora larvae compared to unfed larvae. An increased early post-settlement survival rate, combined with a higher mean settlement in fed larvae, resulted in a larger difference in mean settlement over time (Figure 2B). Consequently, mean settlement of living A. millepora larvae remained significantly higher in the fed treatments than in the unfed treatments at seven days (13.6 ± 2.17 SE vs 5.30 ± 1.03 SE, respectively p <0.001) (Figure 2B). In contrast, there was no significant difference in seven-day survival between treatments in A. tenuis spat (t(123) = 0.86, p = 0.19) (Figure 4D). However, due to the similar survival rates in both the fed and unfed larval treatments but overall higher settlement in the fed treatments, the mean settlement of alive spat in fed A. tenuis larvae remained higher than settlement in unfed larvae seven days post-settlement (15.4 ± 1.92 SE vs 8.3 ± 1.35 SE, respectively t(191) = p <0.001) (Figure 2D).
Discussion
The results of this study demonstrate that rearing larvae with homogenised Artemia as a source of nutritive particulate matter doubled settlement rates for Acropora tenuis larvae and were 2.5 times higher for Acropora millepora larvae. This result suggests that endogenous energy content may be a limiting factor affecting larval settlement patterns. It has been demonstrated that Acropora tenuis larvae can typically lose between 30% and 75% of their energy stores within the first week of development (Harii et al., 2007; Figueiredo et al., 2012; Graham et al., 2013a), a result which is reflected in lipid depletion across other Acropora species (Harii et al., 2007; Okubo et al., 2008; Harii et al., 2010; Figueiredo et al., 2012; Graham et al., 2013a). Previous studies have shown that some coral larvae have the ability to feed (Tranter et al., 1982; Titlyanov et al., 1998; Schwarz et al., 1999; Harii et al., 2009). Therefore, by providing access to exogenous nutrients, larvae could supplement their endogenous energy stores, extending their potential to settle successfully and survive. This advantage is clearly seen in corals that produce larvae with photo-symbionts, which use photosynthetically derived nutrition to enhance survival and settlement (Harii et al., 2010). Photosymbiotic larvae reared under light were more likely to survive until settlement than larvae reared in the dark, as light-reared larvae could supplement up to 40% of their metabolic demands with exogenous nutrients (Harii et al., 2010). The results of the present study are consistent with the results of previous studies examining enhanced settlement associated with increased nutritional potential (Richmond, 1985; Schwarz et al., 1999; Harii et al., 2010).
In addition to increased settlement of fed larvae, another key finding from the present study was the increased post-settlement survival in A. millepora and A. tenuis spat. Mortality in the first year after settlement can be extremely high (Babcock and Heyward, 1986; Babcock and Mundy, 1996; Wilson and Harrison, 2005; Davies et al., 2013; dela Cruz and Harrison, 2017; Suzuki et al., 2018) and is highest immediately following settlement, declining markedly as coral spat increase in size (Martinez and Abelson, 2013; Doropoulos et al., 2016; Harrison et al., 2021). High early mortality may be due to the energy investment required for larvae to complete metamorphosis, as this process represents a significant energy investment for corals (Harrison and Wallace, 1990; Graham et al., 2013a). Therefore, coral larvae with lower energy stores may deplete these energy stores during metamorphosis and die shortly thereafter. However, for larvae provisioned with ample energy stores, metamorphosis into a coral spat should allow sufficient energy reserves to meet metabolic demands until they acquire photosymbiotic Symbiodiniaceae and gain photosynthetically derived nutrition (Harii et al., 2007; Harii et al., 2009; Harrison et al., 2021). Once the coral spat gain symbionts and develop tentacles, they can begin to feed in the same manner as adults, namely through autotrophy and planktotrophy, respectively. The high early mortality of coral spat from the unfed treatments in this study supports the hypothesis that some larvae run out of energy shortly after metamorphosis. Furthermore, the increased early post-settlement survival of both A. millepora and A. tenuis spat derived from larvae that were supplied with homogenised Artemia in this study, highlights the potential for supplying exogenous food to supplement energy stores to enhance post-settlement survival.
Although there was an initial difference in survival between A. tenuis spat from fed and unfed treatments, by seven days post-settlement, survival was similar between treatments. Fed A. tenuis larvae settled in much higher numbers than unfed larvae, even with consistent settlement surface availability, resulting in a higher density of spat in the fed treatments than the unfed treatments. Therefore, similar survival rates among recruits from both the fed and unfed A. tenuis larvae (Figure 4) may have been influenced by post-settlement competition for space rather than metabolic demands. Juvenile survival after settlement can be affected by various ecological processes such as predation, sedimentation, and density-dependent competition (Ritson-Williams et al., 2009; Ricardo et al., 2017; Cameron and Harrison, 2020; Randall et al., 2021) While accidental grazing and predation are large sources of mortality in in-situ environments (Baria et al., 2010; Gallagher and Doropoulos, 2017), those pressures were not present in the current laboratory experiment and therefore, unlikely to influence the observed survival rates. Alternatively, density-dependent processes are known to impact survival as spat compete for space (Martinez and Abelson, 2013; Cameron and Harrison, 2020; Randall et al., 2020). With overall higher settlement in the fed A. tenuis larval treatment compared with the unfed larvae (Figure 2), mortality due to space competition could have been higher in the fed than in the unfed treatment, resulting in less spat from fed treatments surviving overall. Moderate densities reduce space competition among newly settled spat (Cameron and Harrison, 2020). Therefore, more research is needed to optimise settlement density to maximise recruitment success.
While A. tenuis and A. millepora larvae benefit from being reared with access to exogenous nutrients, the exact feeding mechanism could not be determined during this study. Whether larvae assimilate nutrients via osmotrophy or ingest particulate matter through direct planktotrophy is yet to be resolved. However, due to their ability to utilise nutrients, A. tenuis and A. millepora are likely facultative planktotrophic rather than strictly lecithotrophic. Regardless of the feeding mechanism, the ecological relevance of enhanced settlement is clear- as is the importance of this approach for developing new larval rearing protocols for mass culture of coral larvae in future. Species-specific differences in larval quality impact settlement success in marine invertebrates (Marshall and Keough, 2004) with egg size and lipid composition influencing post-metamorphic performance (Pechenik, 2006; Pechenik, 2018). Furthermore, egg size, lipid composition, and lipid depletion are known to vary across acroporid coral larvae (Richmond, 1987; Zaslow and Benayahu, 2000; Graham et al., 2013a). For example, A. tenuis has been shown to deplete energetic lipids at a faster rate than other Acropora coral larvae examined (Graham et al., 2013a). These differences in egg quality may have resulted in a differential benefit of exogenous nutrients between A. tenuis and A. millepora. Therefore, differential utilisation of exogenous nutrients may be contributing to the differences in the mean settlement between A. tenuis and A. millepora. However, direct uptake of nutrients between species was not measured in the present experiment.
Overcoming high post-settlement mortality bottlenecks is a high priority goal in coral restoration (Boström-Einarsson et al., 2020; Randall et al., 2020). Current larval restoration techniques supply large numbers (often millions) of cultured larvae to degraded reefs (dela Cruz and Harrison, 2017; dela Cruz and Harrison, 2020; Harrison et al., 2021). Once on the reef, cultured larvae face the same barriers to settlement, survival and recruitment as wild-spawned larvae. Therefore, rearing cultured larvae with access to exogenous nutrients could produce energetically enhanced larvae with an increased capacity for settlement and post-settlement survival, thereby significantly increasing the efficiency of larval restoration efforts.
Data Availability Statement
The raw data supporting the conclusions of this article will be made available by the authors, without undue reservation.
Author Contributions
PH provided the overall rationale for the study. CR, SW, and PH conceived and designed the experiment. CR and CH conducted the experiments. CR analysed the data and wrote the draft manuscript. PH obtained the primary research funding. All authors edited and approved the final draft.
Funding
Funding was provided by a Paul G. Allen Family Foundation grant to PH, with additional funding and support from the Australian Institute of Marine Science, and an SCU postgraduate research grant to CR.
Conflict of Interest
The authors declare that the research was conducted in the absence of any commercial or financial relationships that could be construed as a potential conflict of interest.
Publisher’s Note
All claims expressed in this article are solely those of the authors and do not necessarily represent those of their affiliated organizations, or those of the publisher, the editors and the reviewers. Any product that may be evaluated in this article, or claim that may be made by its manufacturer, is not guaranteed or endorsed by the publisher.
Acknowledgments
We acknowledge the Bindal and Wulgurukaba People as the traditional owners of the Sea Country from which the corals were collected, and the land on which this research took place. We pay our respects to their elders, past, present, and emerging and acknowledge their continuing spiritual connection to their Sea Country. We thank K. Kaposi, L. Myers, C. Langley, and D. dela Cruz for their assistance in conducting the experiment. We thank the staff of the National Sea Simulator for assistance during spawning and larval rearing.
References
Allen J. D., Pernet B. (2007). Intermediate Modes of Larval Development: Bridging the Gap Between Planktotrophy and Lecithotrophy. Evol. Dev. 9 (6), 643–653. doi: 10.1111/j.1525-142X.2007.00202.x
Arai T., Kato M., Heyward A. J., Ikeda Y., Iizuka T., Maruyama T. (1993). Lipid Composition of Postitively Buoyant Eggs of Reef Building Corals. Coral. Reefs. 12, 71–75. doi: 10.1007/BF00302104
Babcock R. C., Heyward A. J. (1986). Larval Development of Certain Gamete-Spawning Scleractinian Corals. Coral. Reefs. 5 (3), 111–116. doi: 10.1007/BF00298178
Babcock R. C., Mundy C. N. (1996). Coral Recruitment: Consequences of Settlement Choice for Early Growth and Survivorship in Two Scleractinians. J. Exp. Mar. Biol. Ecol. 206 (1-2), 179–201. doi: 10.1016/S0022-0981(96)02622-6
Baird A. H., Morse A. N. C. (2004). Induction of Metamorphosis in Larvae of the Brooding Corals Acropora Palifera and Stylophora Pistillata. Mar. Freshw. Res. 55 (5), 469–472. doi: 10.1071/MF03121
Baria M. V. B., Guest J. R., Edwards A. J., Aliño P. M., Heyward A. J., Gomez E. D. (2010). Caging Enhances Post-Settlement Survival of Juveniles of the Scleractinian Coral Acropora Tenuis. J. Exp. Mar. Biol. Ecol. 394 (1), 149–153. doi: 10.1016/j.jembe.2010.08.003
Bellwood D. R., Hughes T. P., Folke C., Nyström M. (2004). Confronting the Coral Reef Crisis. Nature 429, 827–833. doi: 10.1038/nature02691
Boidron-Métairon I. F. (1995). Larval Nutrition in Ecology of Marine Invertebrate Larvae. Ed. McEdward L. R. (United States of America: CRC Press), 223–248.
Boström-Einarsson L., Babcock R. C., Bayraktarov E., Ceccarelli D. M., Cook N., Ferse S. C. A., et al. (2020). Coral Restoration – A Systematic Review of Current Methods, Successes, Failures and Future Directions. PLos One 15 (1). 1−24 doi: 10.1371/journal.pone.0226631
Botello G., Krug P. J. (2006). ‘Desperate Larvae’ Revisited: Age, Energy and Experience Affect Sensitivity to Settlement Cues in Larvae of the Gastropod Alderia Sp. Mar. Ecol. Prog. Ser. 312, 149–159. doi: 10.3354/meps312149
Brown B. E., Bythell J. C. (2005). Perspectives on Mucus Secretion in Reef Corals. Mar. Ecol. Prog. Ser. 296, 291–309. doi: 10.3354/meps296291
Burke L., Reytar K., Spalding M., Perry A. (2011). Reefs at Risk: Revisted. World Resources Institute, Washington, D.C.
Cameron K. A., Harrison P. L. (2020). Density of Coral Larvae can Influence Settlement, Post-Settlement Colony Abundance and Coral Cover in Larval Restoration. Sci. Rep. 10 (1), 5488–5488. doi: 10.1038/s41598-020-62366-4
Collin R. (2012). Nontraditional Life-History Choices: What can “Intermediates” Tell Us About Evolutionary Transitions Between Modes of Invertebrate Development? Integr. Comp. Biol. 52 (1), 128–137. doi: 10.1093/icb/ics065
Conlan J. A., Humphrey C. A., Severati A., Francis D. S. (2017). Influence of Different Feeding Regimes on the Survival, Growth, and Biochemical Composition of Acropora Coral Recruits. PLos One 12 (11), 1–20. doi: 10.1371/journal.pone.0188568
Davies S. W., Matz M. V., Vize P. D. (2013). Ecological Complexity of Coral Recruitment Processes: Effects of Invertebrate Herbivores on Coral Recruitment and Growth Depends Upon Substratum Properties and Coral Species. PLos One 8 (9). 1−10 doi: 10.1371/journal.pone.0072830
De’Ath G., Fabricius K. E., Sweatman H., Puotinen M. (2012). The 27-Year Decline of Coral Cover on the Great Barrier Reef and its Causes. Proc. Natl. Acad. Sci. United. States America 109 (44), 17995–17999. doi: 10.1073/pnas.1208909109
dela Cruz D. W., Harrison P. L. (2017). Enhanced Larval Supply and Recruitment can Replenish Reef Corals on Degraded Reefs. Sci. Rep. 7 (1). 1–13 doi: 10.1038/s41598-017-14546-y
dela Cruz D. W., Harrison P. L. (2020). Enhancing Coral Recruitment Through Assisted Mass Settlement of Cultured Coral Larvae. PLos One 15, 1–21. doi: 10.1371/journal.pone.0242847
Doropoulos C., Roff G., Bozec Y.-M., Zupan M., Werminghausen J., Mumby P. J. (2016). Characterizing the Ecological Trade-Offs Throughout the Early Ontogeny of Coral Recruitment. Ecol. Monogr. 86 (1), 20–44. doi: 10.1890/15-0668.1
Doropoulos C., Vons F., Elzinga J., ter Hofstede R., Salee K., van Koningsveld M., et al. (2019). Testing Industrial-Scale Coral Restoration Techniques: Harvesting and Culturing Wild Coral-Spawn Slicks. Front. Mar. Sci. 6. doi: 10.3389/fmars.2019.00658
Edwards A. J. (2010). Reef Rehabilitation Manual (St Lucia, Australia: Coral Reef Targeted Research & Capacity Building for Management Program).
Fadlallah Y. H. (1983). Sexual Reproduction, Development and Larval Biology in Scleractinian Corals. Coral. Reefs. 2, 129–150. doi: 10.1007/BF00336720
Figueiredo J., Baird A. H., Cohen M. F., Flot J. F., Kamiki T., Meziane T., et al. (2012). Ontogenetic Change in the Lipid and Fatty Acid Composition of Scleractinian Coral Larvae. Coral. Reefs. 31 (2), 613–619. doi: 10.1007/s00338-012-0874-3
Gallagher C., Doropoulos C. (2017). Spatial Refugia Mediate Juvenile Coral Survival During Coral–Predator Interactions. Coral. Reefs. 36 (1), 51–61. doi: 10.1007/s00338-016-1518-9
Gouezo M., Golbuu Y., Fabricius K. E., Olsudong D., Mereb G., Nestor V., et al. (2019). Drivers of Recovery and Reassembly of Coral Reef Communities. Proc. R. Soc. B: Biol. Sci. 286 (1897). 1–10 doi: 10.1098/rspb.2018.2908
Graham E. M., Baird A. H., Connolly S. R. (2008). Survival Dynamics of Scleractinian Coral Larvae and Implications for Dispersal. Coral. Reefs. 27 (3), 529–539. doi: 10.1007/s00338-008-0361-z
Graham E. M., Baird A. H., Connolly S. R., Sewell M. A., Willis B. L. (2013a). Rapid Declines in Metabolism Explain Extended Coral Larval Longevity. Coral. Reefs. 32 (2), 539–549. doi: 10.1007/s00338-012-0999-4
Graham N. A. J., Bellwood D. R., Dinner J. E., Hughes T. P., Norström A. V., Nyström M. (2013b). Managing Resilience to Reverse Phase Shifts in Coral Reefs. Front. Ecol. Environ. 11 (10), 541–548. doi: 10.1890/120305
Harii S., Nadaoka K., Yamamoto M., Iwao K. (2007). Temporal Changes in Settlement, Lipid Content and Lipid Composition of Larvae of the Spawning Hermatypic Coral Acropora Tenuis. Mar. Ecol. Prog. Ser. 346, 89–96. doi: 10.3354/meps07114
Harii S., Yamamoto M., Hoegh-Guldberg O. (2010). The Relative Contribution of Dinoflagellate Photosynthesis and Stored Lipids to the Survivorship of Symbiotic Larvae of the Reef-Building Corals. Mar. Biol. 157 (6), 1215–1224. doi: 10.1007/s00227-010-1401-0
Harii S., Yasuda N., Rodriguez-Lanetty M., Irie T., Hidaka M. (2009). Onset of Symbiosis and Distribution Patterns of Symbiotic Dinoflagellates in the Larvae of Scleractinian Corals. Mar. Biol. 156 (6), 1203–1212. doi: 10.1007/s00227-009-1162-9
Harrison P. L., dela Cruz D. W., Cameron K. A., Cabaitan P. C. (2021). Increased Coral Larval Supply Enhances Recruitment for Coral and Fish Habitat Restoration. Front. Mar. Sci. 8. doi: 10.3389/fmars.2021.750210
Harrison P. L., Wallace C. C. (1990). ““Reproduction, Dispersal and Recruitment of Scleractinian Corals,”,” in Ecosystems of the World Coral Reefs, vol. 25 . Ed. Dubinsky Z. (Amsterdam: Elsevier Science Publisher), 133–207.
Heyward A. J., Negri A. P. (1999). Natural Inducers for Coral Larval Metamorphosis. Coral. Reefs. 18, 273–279. doi: 10.1007/s003380050193
Hoegh-Guldberg O., Mumby P. J., Hooten A. J., Steneck R. S., Greenfield P., Gomez E. D., et al. (2007). Coral Reefs Under Rapid Climate Change and Ocean Acidification. Science 318, 1737–1742. doi: 10.1126/science.1152509
Hughes T. P., Baird A. H., Bellwood D. R., Card M., Connolly S. R., Folke C., et al. (2003). Climate Change, Human Impacts, and the Resilience of Coral Reefs. Science 301 (301), 929–933. doi: 10.1126/science.1085046
Hughes T. P, Kerry J. T, Baird A. H, Connolly S. R, Chase T. J, Dietzel A, et al. (2019). Global Warming Impairs Stock–Recruitment Dynamics of Corals. Nature568(7752), 387–390-
Hughes T. P., Kerry J. T., Baird A. H., Connolly S. R., Dietzel A., Eakin C. M., et al. (2018). Global Warming Transforms Coral Reef Assemblages. Nature 556 (7702), 492–496. doi: 10.1038/s41586-018-0041-2
Humanes A., Noonan S. H. C., Willis B. L., Fabricius K. E., Negri A. P. (2016). Cumulative Effects of Nutrient Enrichment and Elevated Temperature Compromise the Early Life History Stages of the Coral Acropora Tenuis. PLos One 11 (8), 1–23 doi: 10.1371/journal.pone.0161616
Krupp D. A. (1983). Sexual Reproduction and Early Development of the Solitary Coral Fungia Scutaria (Anthozoa: Scleractinia). Coral. Reefs. 2 (3), 159–164. doi: 10.1007/BF00336722
Marshall D. J., Keough M. J. (2003). Variation in the Dispersal Potential of non-Feeding Invertebrate Larvae: The Desperate Larva Hypothesis and Larval Size. Mar. Ecol. Prog. Ser. 255, 145–153. doi: 10.3354/meps255145
Marshall D. J., Keough M. J. (2004). Variable Effects of Larval Size on Post-Metamorphic Performance in the Field. Mar. Ecol. Prog. Ser. 279, 73–80. doi: 10.3354/meps279073
Martinez S., Abelson A. (2013). Coral Recruitment: The Critical Role of Early Post-Settlement Survival. ICES. J. Mar. Sci. 70 (7), 1294–1298. doi: 10.1093/icesjms/fst035
McEdward L. R. (2000). Adaptive Evolution of Larvae and Life Cycles. Semin. Cell Dev. Biol. 11 (6), 403–409. doi: 10.1006/scdb.2000.0193
Mumby P. J., Harborne A. R. (2010). Marine Reserves Enhance the Recovery of Corals on Caribbean Reefs. PLos One 5 (1), 509–519 doi: 10.1371/journal.pone.0008657
Nozawa Y., Harrison P. L. (2002).Larval Settlement Patterns, Dispersal Potential, and the Effect of Temperature on Settlement of Larvae of the Reef Coral, Platygyra daedalea, from the Great Barrier ReefProceedings of the 9th international coral reef symposium1409–416
Okubo N., Yamamoto H. H., Nakaya F., Okaji K. (2008). Oxygen Consumption of a Single Embryo/Planula in the Reef-Building Coral Acropora Intermedia. Mar. Ecol. Prog. Ser. 366, 305–309. doi: 10.3354/meps07562
Omori M. (2019). Coral Restoration Research and Technical Developments: What We Have Learned So Far. Mar. Biol. Res. 15, 7, 377–409 doi: 10.1080/17451000.2019.1662050
Paulay G., Boring L., Strathmann R. R. (1985). Food Limited Growth and Development of Larvae: Experiments With Natural Sea Water. J. Exp. Mar. Biol. Ecol. 93 (1-2), 1–10. doi: 10.1016/0022-0981(85)90145-5
Pechenik J. A. (2006). Larval Experience and Latent Effects - Metamorphosis is Not a New Beginning. Integr. Comp. Biol. 46 (3), 323–333. doi: 10.1093/icb/icj028
Pechenik J. A. (2018). “Latent Effects: Surprising Consequences of Embryonic and Larval Experience on Life After Metamorphosis,” in Evolutionary Ecology of Marine Invertebrate Larvae (Croydon, UK: Oxford University Press), 208–225.
Pernet B. (2018). “Larval Feeding: Mechanisms, Rates, and Performance in Nature,” in Evolutionary Ecology of Marine Invertebrate Larvae. Eds. Carrier T. J., Reitzel A. M., Heyland A. (Croydon, UK: Oxford University Press), 87–102.
Pineda J. (2000). Linking Larval Settlement to Larval Transport: Assumptions, Potentials, and Pitfalls. Oceanography. Eastern. Pacific. 1, 84–105.
Pollock J. F., Katz S. M., van de Water J. A. J. M., Davies S. W., Hein M. Y., Torda G., et al. (2017). Coral Larvae for Restoration and Research: A Large-Scale Method for Rearing Acropora Millepora Larvae, Inducing Settlement, and Establishing Symbiosis. Peer J 5, e3732. doi: 10.7717/peerj.3732
Randall C. J., Giuliano C., Heyward A. J., Negri A. P. (2021). Enhancing Coral Survival on Deployment Devices With Microrefugia. Front. Mar. Sci. 8. doi: 10.3389/fmars.2021.662263
Randall C. J., Negri A. P., Quigley K. M., Foster T., Ricardo G. F., Webster N. S., et al. (2020). Sexual Production of Corals for Reef Restoration in the Anthropocene. Mar. Ecol. Prog. Ser. 635, 203–232. doi: 10.3354/meps13206
Ricardo G. F., Jones R. J., Nordborg M., Negri A. P. (2017). Settlement Patterns of the Coral Acropora Millepora on Sediment-Laden Surfaces. Sci. Total. Environ. 609, 277–288. doi: 10.1016/j.scitotenv.2017.07.153
Richmond R. H. (1985). Reversible Metamorphosis in Coral Planula Larvae. Mar. Ecol. Prog. Ser. 22, 181–185. doi: 10.3354/meps022181
Richmond R. H. (1987). Energetics, Competency, and Long-Distnace Dispersal of Planula Larvae of the Coral Pocillopora Damicornis. Nature 164 (4178), 914–914. doi: 10.1038/164914a0
Richmond R. H. (1997). "Reproduction and Recruitment in Corals: Critical Links in the Persistence of Reefs," in Life and Death of Coral Reefs (US: Springer), 175–197.
Rinkevich B. (2005). Conservation of Coral Reefs Through Active Restoration Measures: Recent Approaches and Last Decade Progress. Environ. Sci. Technol.39(12)4333–4342 doi: 10.1021/es0482583
Rinkevich B., Loya Y. (1979). The Reproduction of the Red Sea Coral Stylophora Pistillata. I. Gonads and Planulae. Mar. Ecol. Prog. Ser. 1, 133–144. doi: 10.3354/meps001133
Ritson-Williams R., Arnold S. N., Fogarty N. D., Steneck R. S., Vermeij M. J. A., Paul V. J. (2009). New Perspectives on Ecological Mechanisms Affecting Coral Recruitment on Reefs. Smithosnian. Contributions. to Mar. Sci. 38, 437–457 doi: 10.5479/si.01960768.38.437
Rivest E. B., Chen C. S., Fan T. Y., Li H. H., Hofmann G. E. (2017). Lipid Consumption in Coral Larvae Differs Among Sites: A Consideration of Environmental History in a Global Ocean Change Scenario. Proc. R. Soc. B: Biol. Sci. 284 (1853). 1–9 doi: 10.1098/rspb.2016.2825
Schwarz J. A., Krupp D. A., Weis V. M. (1999). Late Larval Development and Onset of Symbiosis in the Scleractinian Coral Fungia Scutaria. Biol. Bull. 196 (1), 70–79. doi: 10.2307/1543169
Sorokin Y. I. (1973). On the Feeding of Some Scleractinian Corals With Bacteria and Dissolved Organic Matter. Limnology. Oceanography. 18 (3). 380–385.doi: 10.4319/lo.1973.18.3.0380
Strathmann R. R. (1985). Life-History Evolution in Marine Invertebrates. Ann. Rev. Ecol. Syst. 16 (c), 339–361. doi: 10.1146/annurev.es.16.110185.002011
Strathmann R. R., Jahn L., Fonseca J. R. C. (1972). Suspension Feeding by Marine Invertebrate Larvae: Clearance of Particules by Ciliated Badnds of a Rotifer, Pluteus, and Trochopore. Biol. Bull. 142, 505–519. doi: 10.2307/1540326
Suzuki G., Kai S., Fujikura Y., Yamashita H. (2018). Post-Settlement Survivorship of Artificially Supplied Acropora Coral Larvae in the Sekisei Lagoon. Mar. Ecol. Prog. Ser. 603, 105–115. doi: 10.3354/meps12698
Titlyanov E. A., Titlyanova T. V., Loya Y., Yamazato K. (1998). Degradation and Proliferation of Zooxanthellae in Planulae of the Hermatypic Coral Stylophora Pistillata. Mar. Biol. 130 (3), 471–477. doi: 10.1007/s002270050267
Tranter P. R. G., Nicholson D. N., Kinchington D. (1982). A Description of Spawning and Post-Gastrula Development of the Cool Temperate Coral, Caryophyllia Smithi. J. Mar. Biol. Assoc. United. Kingdom. 62 (4), 845–854. doi: 10.1017/S0025315400070387
Wallace CC., (1999). Staghorn Corals of the World: A Revision of the Genus AcroporaCollingwood, Australia: CSIRO Publishing
Willis B. L., Babcock R. C., Harrison P. L., Wallace C. C. (1997). Experimental Hybridization and Breeding Incompatibilities Within the Mating Systems of Mass Spawning Reef Corals. Coral. Reefs. 16 (SUPPL. 1), 53–65. doi: 10.1007/s003380050242
Wilson J. R., Harrison P. L. (1998). Settlement-Competency Periods of Larvae of Three Species of Scleractinian Corals. Mar. Biol. 131 (2), 339–345. doi: 10.1007/s002270050327
Wilson J., Harrison P. L. (2005). Post-Settlement Mortality and Growth of Newly Settled Reef Corals in a Subtropical Environment. Coral. Reefs. 24 (3), 418–421. doi: 10.1007/s00338-005-0033-1
Wright N. (1986). “Aspects of Reproduction and Planula Development in the Reef Coral Cyphastrea Ocellina” in Coral Reef Population Biology. Eds. Jokiel P. L., Richmond R. H., Rogers R. A. (Hawaii, USA: Hawaii Inst. Mar. Biol. Tech), 179–192.
Young S. D. (1971). Organic Material From Scleractinian Coral Skeletons-I. Variation in Composition Between Several Species. Comp. Biochem. Physiol. – Part B: Biochem. 40 (1), 113–120. doi: 10.1016/0305-0491(71)90067-8
Zaslow R. B. D., Benayahu Y. (1996). Longevity, Competence and Energetic Content in Planulae of the Soft Coral Heteroxenia Fuscescens. J. Exp. Mar. Biol. Ecol. 206 (1-2), 55–68. doi: 10.1016/S0022-0981(96)02618-4
Keywords: recruitment, post-settlement survival, coral restoration, reef restoration, facultative planktotrophy, larval energetics, aquaculture
Citation: Rodd C, Whalan S, Humphrey C and Harrison PL (2022) Enhancing Coral Settlement Through a Novel Larval Feeding Protocol. Front. Mar. Sci. 9:918232. doi: 10.3389/fmars.2022.918232
Received: 12 April 2022; Accepted: 20 June 2022;
Published: 20 July 2022.
Edited by:
Thierry Work, United States Geological Survey (USGS), United StatesReviewed by:
Luis Eduardo Calderon Aguilera, Center for Scientific Research and Higher Education in Ensenada (CICESE), MexicoPatricia Briones-Fourzan, National Autonomous University of Mexico, Mexico
Copyright © 2022 Rodd, Whalan, Humphrey and Harrison. This is an open-access article distributed under the terms of the Creative Commons Attribution License (CC BY). The use, distribution or reproduction in other forums is permitted, provided the original author(s) and the copyright owner(s) are credited and that the original publication in this journal is cited, in accordance with accepted academic practice. No use, distribution or reproduction is permitted which does not comply with these terms.
*Correspondence: Colleen Rodd, colleen.rodd@scu.edu.au