- 1First Institute of Oceanography, and Key Laboratory of Marine Science and Numerical Modeling, Ministry of Natural Resources, Qingdao, China
- 2Laboratory for Regional Oceanography and Numerical Modeling, Pilot National Laboratory for Marine Science and Technology, Qingdao, China
- 3Shandong Key Laboratory of Marine Science and Numerical Modeling, Qingdao, China
- 4Jiangsu Key Laboratory for Biodiversity and Biotechnology, Nanjing Normal University, Nanjing, China
Pulsed signal trains comprising clicks, buzzes, and burst-pulses play important roles in the life activities of odontocetes, but they have not been distinguished in Indo-Pacific humpback dolphins. Underwater vocalizations of this species were recorded from 27 September to 2 October 2019 in the Beibu Gulf, South China Sea. Pulsed signal trains were detected with variations in the pulsed signal number (range of 6–76), mean inter-pulse interval (IPIs_m: 0.1–315 ms), and mean duration (D ranged from tens to thousands of milliseconds). Principal component analysis and hierarchical cluster analysis based on six acoustic parameters in the pulsed signal trains identified three categories of trains designated as clicks, burst-pulses, and buzzes. Buzzes and burst-pulses (different from those described in previous research) were detected for the first time in Indo-Pacific humpback dolphins in China. The results indicated that the IPIs_m was longest for clicks but shortest for buzzes, and the D values were longer for both clicks and burst-pulses than buzzes. The three train types could be identified based on the IPIs_m, with threshold values of 4.9 and 15.5 ms. The significant variations in the three vocalization types were related to surface behaviors, and buzzes could have a special function in foraging by this species, thereby requiring further research. These findings may facilitate future quantitative evaluations of the echolocation performance in wild Indo-Pacific humpback dolphins and provide important guidance regarding acoustic observations and the identification of this species.
Introduction
During their long-term adaptation to marine environments with limited visibility, odontocetes can acquire information about the underwater environment through sound (Allen et al., 2018; Jiang et al., 2020; Cusano et al., 2022). They generally produce pulsed signals that form trains to allow the fine-grained discrimination of targets (Au, 1993). Studies have demonstrated that many odontocetes use three main types of pulsed signal trains, which are commonly referred to as clicks, buzzes, and burst-pulses, and all are high frequency with short repetition intervals and high pulse repetition rates (Lammers et al., 2004; Madsen and Wahlberg, 2007).. Clicks comprise a series of higher-frequency pulses, which have functions in echolocation, and they are mainly used for navigation and localization. Buzzes involve a rapid increase in the pulse repetition rate, and their characteristics generally vary with the distance from prey and prey size (Sarnocińska et al., 2020). In recent years, the function of buzzes has extended into the social realm, not just during foragings, such as courtship activity and mother/calf discipline (Herzing and dos Santos, 2004; Herzing, 2004; Martin et al., 2019). Burst-pulses are generally regarded as functional signals in intraspecific communication (Lammers et al., 2004; Yoshida et al., 2014; Martin et al., 2018; Sørensen et al., 2018).
Martin et al. (2018) showed that three pulsed signal trains produced by Hector’s dolphin could be distinguished according to their inter-pulse intervals (IPIs). The IPIs of clicks were more than 10 ms, and they could be further divided into narrowband and broadband pulses (Morisaka et al., 2011). The IPIs of buzzes were less than 10 ms, where they started below 10 ms and ended above 10 ms (Griffin et al., 1960). The IPIs of burst-pulses usually started, followed, and ended at less than 10 ms (Lammers et al., 2004; Martin et al., 2018). Au (1993) concluded that the clicks and burst-pulses of odontocetes mainly differ in terms of their IPIs.
Indo-Pacific humpback dolphins (Sousa chinensis) are medium-sized delphinids (adult body length of 2.0–2.5 m), which are mainly distributed in the Western Pacific and Indian Oceans (Folkens and Reeves, 2002; Jefferson, 2000; Shirihai et al., 2006; Parra et al., 2004; John and Yang, 2009; Zhang et al., 2011). In China, there are several distinct currently recognized geographic populations, including Xiamen Bay, the Shantou waters, the eastern Taiwan Strait, the Pearl River Delta region, Sanniang Bay, Leizhou Bay, the Beibu Gulf, and the western Hainan coastal waters (Wang et al., 2014; Li et al., 2015). Indo-Pacific humpback dolphins were recently assessed for The IUCN Red List of Threatened Species in 2015 and listed as Vulnerable (Jefferson et al., 2017).
Pulsed signals play the same important roles for Indo-Pacific humpback dolphins, but few studies have investigated the pulsed signals produced by this species (Li et al., 2013; Soto et al., 2014; Li et al., 2015; Jayathilaka and Arulananthan, 2019) and none have classified their signal trains using a quantitative approach. The pulsed signal trains that are collectively referred to as clicks last 0.21–15.3 s (Soto et al., 2014), and the duration of each pulse is tens of microseconds (Niu et al., 2012). The IPIs of clicks are 3.3–349.2 ms (Wang et al., 2014). The Indo-Pacific humpback dolphins continue to increase the pulse repetition rate as the distance to the target decreases (Goold and Jefferson, 2004; Sims et al., 2012). Burst-pulses by Indo-Pacific humpback dolphins were recorded in Morton Bay, Southeast Queensland, Australia (Van Parijs and Corkeron, 2001; Soto et al., 2014), and they were divided into two types comprising barks and quacks (Van Parijs and Corkeron, 2001). However, according to phylogenetic analyses of mitochondrial and nuclear DNA, the population of Australian humpback dolphins (named Sousa sahulensis) is genetically distinct and possibly a different species compared with those found in China and Indonesia (Frere et al., 2011; Mendez et al., 2013).
In the present study, pulsed signals by Indo-Pacific humpback dolphins were recorded in Beibu Gulf of the South China Sea. The parameters were determined for the pulsed signal trains, and the different types were identified. We then selected a criterion for classifying the pulsed signal trains and assessed the function of each type.
Materials and methods
Sound collection
Underwater acoustic recordings of Indo-Pacific humpback dolphins were made during the daytime from 27 September to 2 October 2019, in Beibu Gulf, South China Sea. The observation point is indicated by a red five-pointed star (21°14′N, 109°29′E) in Figure 1. The acoustic recordings were acquired at water depths of 7–17 m. During the observation period, the water temperature was 23°CC–30°CC and the sea state was 0–1.
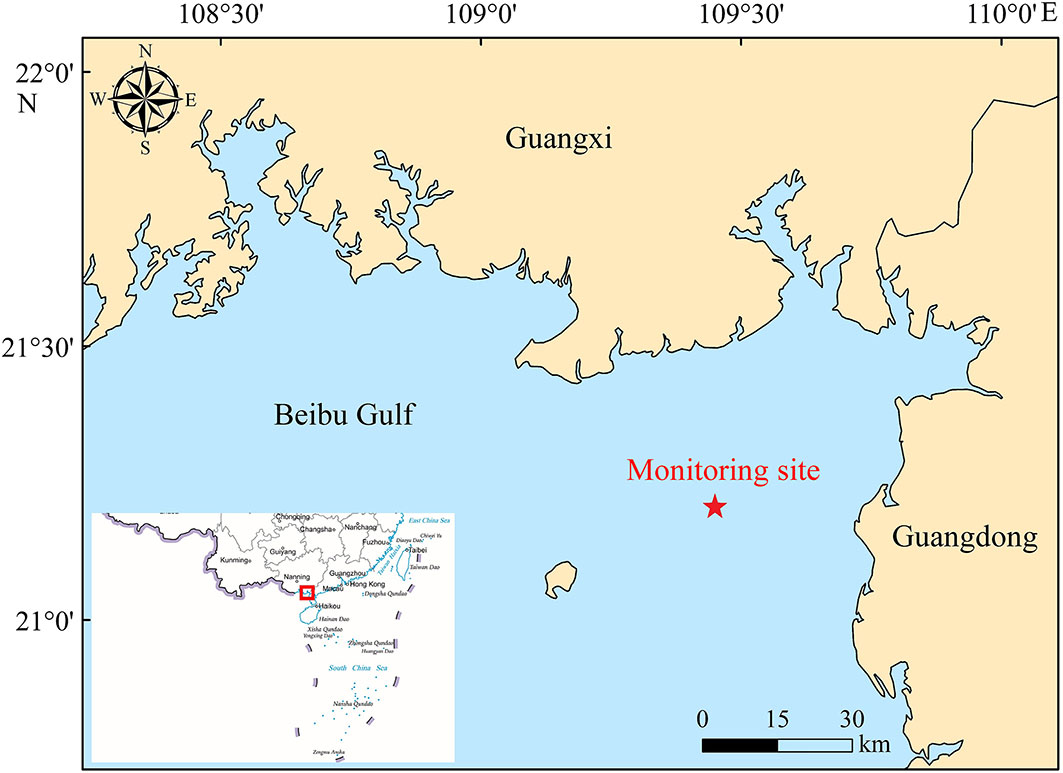
Figure 1 Map of the study area. The red five-pointed star represents the location where sound recordings were made of Indo-Pacific humpback dolphins in the Beibu Gulf, South China Sea (outlined in the red box on the left-hand side of the map).
Underwater sounds were recorded using a high-frequency intelligent hydrophone icListen (Integrated System Ltd.) (flat frequency response: 10 Hz to 200 kHz; sensitivity: (170 ± 6) dB (0 dB ≅ 1 V/μPa); sampling rate: 512 kHz; and resolution: 24 bit). The survey vessel is a 12-m fishing boat. During the observations, two experienced observers searched for targets at the bow of the boat. When targets were found, the boat approached in order to identify the species, estimate the school size, conduct behavioral observations, and make acoustic recordings. The boat stopped within 100 m of the dolphins, before turning off the engine and placing the hydrophone down the bow. The hydrophone was placed in the water at a depth of 1–4 m to make the recordings. Visual observations of behaviors (Henderson et al., 2012) and group size information were collected at the same time as the sound recordings. A group was defined as either (1) a single animal or (2) any collection of dolphins where a member was within 15 m of others, and more than half of the animals exhibited the same behavior (Van Parijs et al., 2002; Hartman et al., 2008). Surface behaviors were recorded within each group focal followed by a single observer at as consistent an interval period as possible (e.g., 1 min, or upon the next surfacing if the group was underwater), and classified as milling, foraging, traveling, socializing, or trawlers. During the entire observation period, more than 10 Indo-Pacific humpback dolphins presented at the surface within 20 m of the recording site, and no other species were observed in the study area. Distances were estimated manually and recorded when the dolphins were observed. However, the sexes and exact locations of the calling dolphins were unknown.
Sound analysis
In total, 665 audio files (50 GB) were acquired from the observations. Only files with a recording distance less than 50 m were selected for analysis. Data processing was conducted in the following four steps.
(1) Detection of pulsed signals
Pulsed signals were visually detected using Adobe Audition CC 2020 (Adobe Systems Inc., San Jose, CA, USA). The pulsed signal trains were intercepted and saved as separate files according to the following criteria: signals with clear contours in the spectrogram, i.e., a high signal-to-noise ratio (> 10 dB), and non-overlapping signal trains.
(2) Extraction of acoustic parameters
The parameters of each pulsed signal train were extracted with a custom-designed program in MATLAB version 2020b (The MathWorks Inc., Natick, MA, USA), specifically the mean peak frequency (Fp_m), mean of −3-dB bandwidth (BW−3 dB_m), mean −10-dB bandwidth (BW−10 dB_m), mean IPI (IPIs_m), pulsed signal number (NumP), and duration (D) (Table 1 summarizes the acoustic parameters of the pulsed signals and trains). It should be noted that all of the acoustic parameters were derived from the received signals.
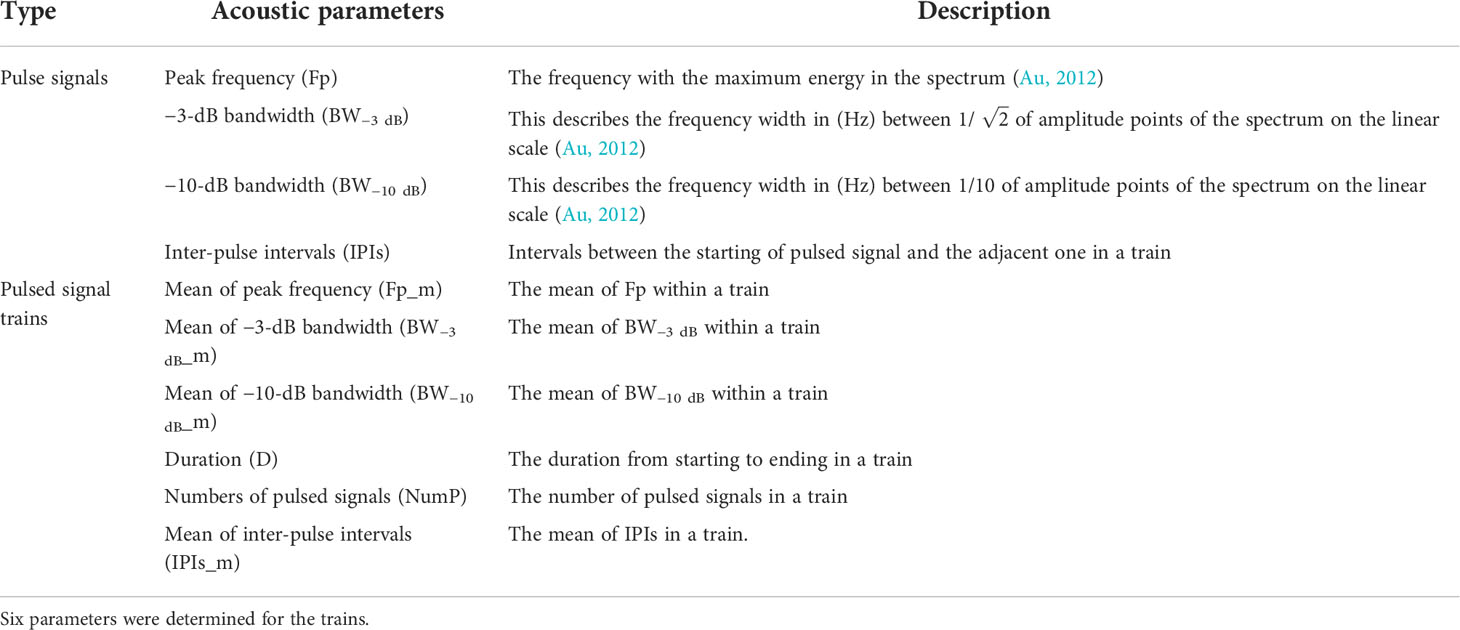
Table 1 Descriptions of acoustic parameters of pulsed signals and pulsed signal trains produced by Sousa chinensis in Beibu Gulf, South China Sea.
(3) Classification of pulsed signal trains
Principal component analysis (PCA) and hierarchical cluster analysis (HCA) were conducted to identify the train types using MINITAB 17.0 (The Minitab LLC Inc., PA, USA). PCA was used to reduce the number of train parameters, and HCA was performed based on the PCA results with eigenvalues greater than 1. The two main inspection criteria for HCA comprised belonging to a category with more than 80% similarity and the number in each category exceeding 10. All train types were then checked again by visual inspection of the spectrograms to assess the validity of the train type classifications generated by HCA.
(4) Collection of statistics for each train type and corresponding behavioral information
A specific classification standard was proposed to distinguish the train types.
Results
In total, 850 pulsed signal trains produced by Indo-Pacific humpback dolphins were obtained, which contained 27,000 pulsed signals. All of the trains were high frequency (maximum of Fp_m > 180 kHz). The D values of the trains had a wide dynamic range from tens to thousands of milliseconds. The variations in the NumP and IPIs_m were significant within the trains (NumP ranged from 6 to 76 and IPIs_m from 0.1 to 315 ms).
PCA was performed based on the six acoustic parameters obtained for the trains, and three principal components (PCs) were determined, which together explained 99.5% of the variance in the results (see Table 2). PC1 explained 40.06% of the variance, and it was mainly related to two parameters (IPIs_m and D). PC2 explained 35.91% of the variance, and it was strongly correlated with three frequency parameters (Fp_m, BW−3 dB_m, and BW−10 dB_m). PC3 explained 23.62% of the variance, and it was most strongly correlated with the NumP. Remarkably, there were no correlations between the three PCs.
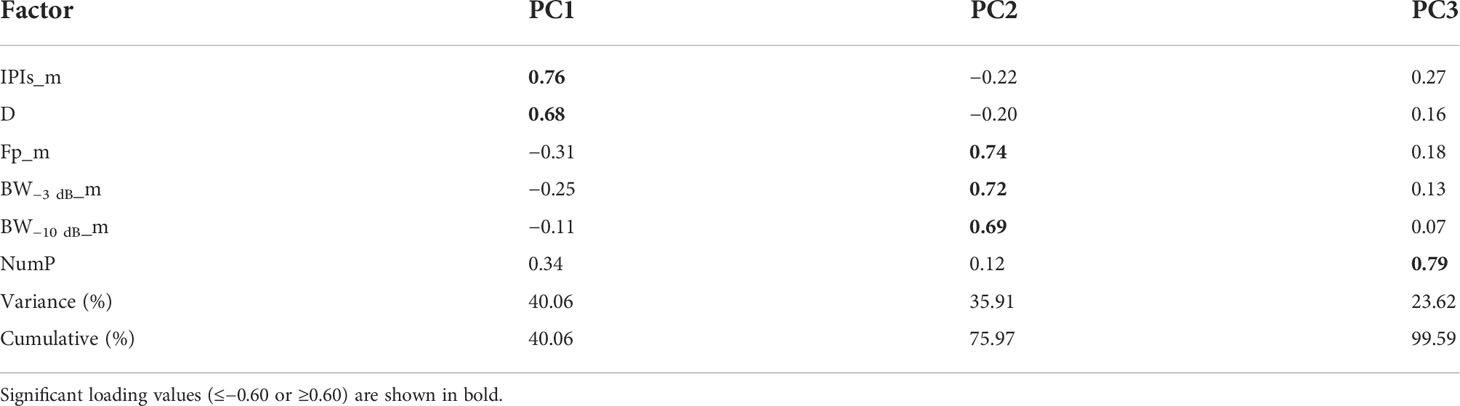
Table 2 Loadings by factor according to principal component analysis for the six acoustic parameters (pulsed signal trains) determined in underwater vocalizations by wild Sousa chinensis in Beibu Gulf, South China Sea.
HCA was used to cluster the three extracted PCs and a dendrogram (Figure 2) was obtained from the HCA results. Three main clusters were determined all with similarity exceeding 95%.
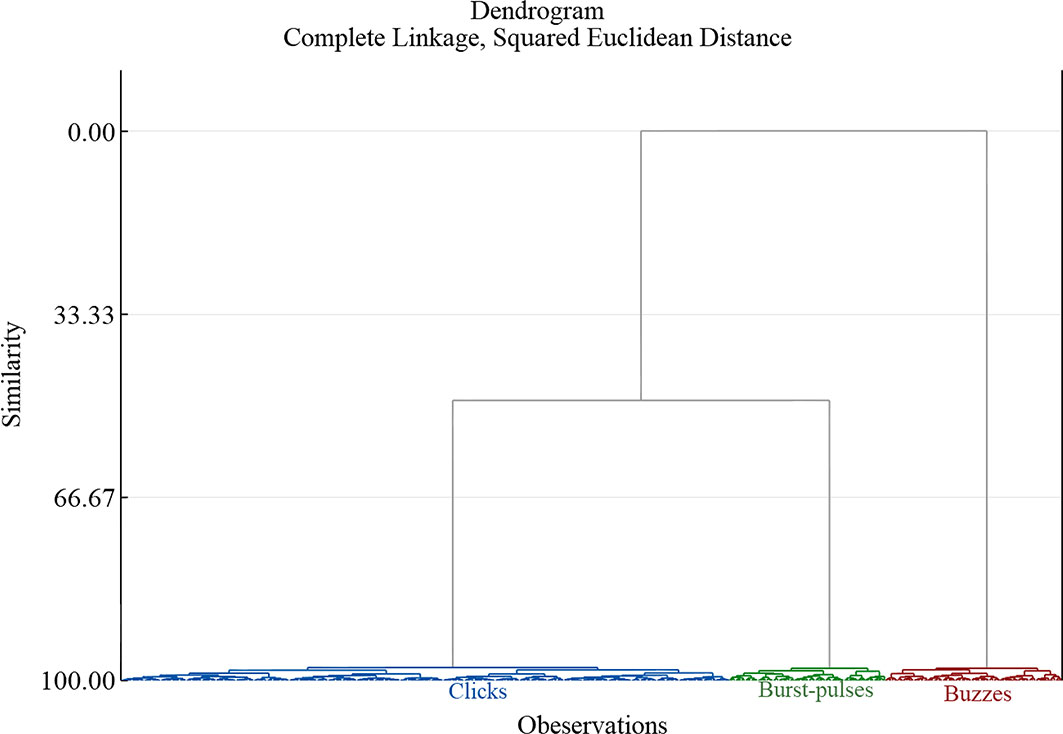
Figure 2 Dendrogram obtained for the pulsed signal trains recorded from Indo-Pacific humpback dolphins based on hierarchical cluster analysis. Different main clusters (similarity over 95%) are shown in different colors.
Based on the HCA results and further visual examination, the pulsed signal trains of Indo-Pacific humpback dolphins in Beibu Gulf were identified as belonging to three train types: clicks, burst-pulses, and buzzes. The designations of these train types are consistent with those used in previous studies of vocalizations by odontocetes (Yoshida et al., 2014; Martin et al., 2018; Sorensen et al., 2018). Examples of each type of pulsed signal train are shown in Figure 3.
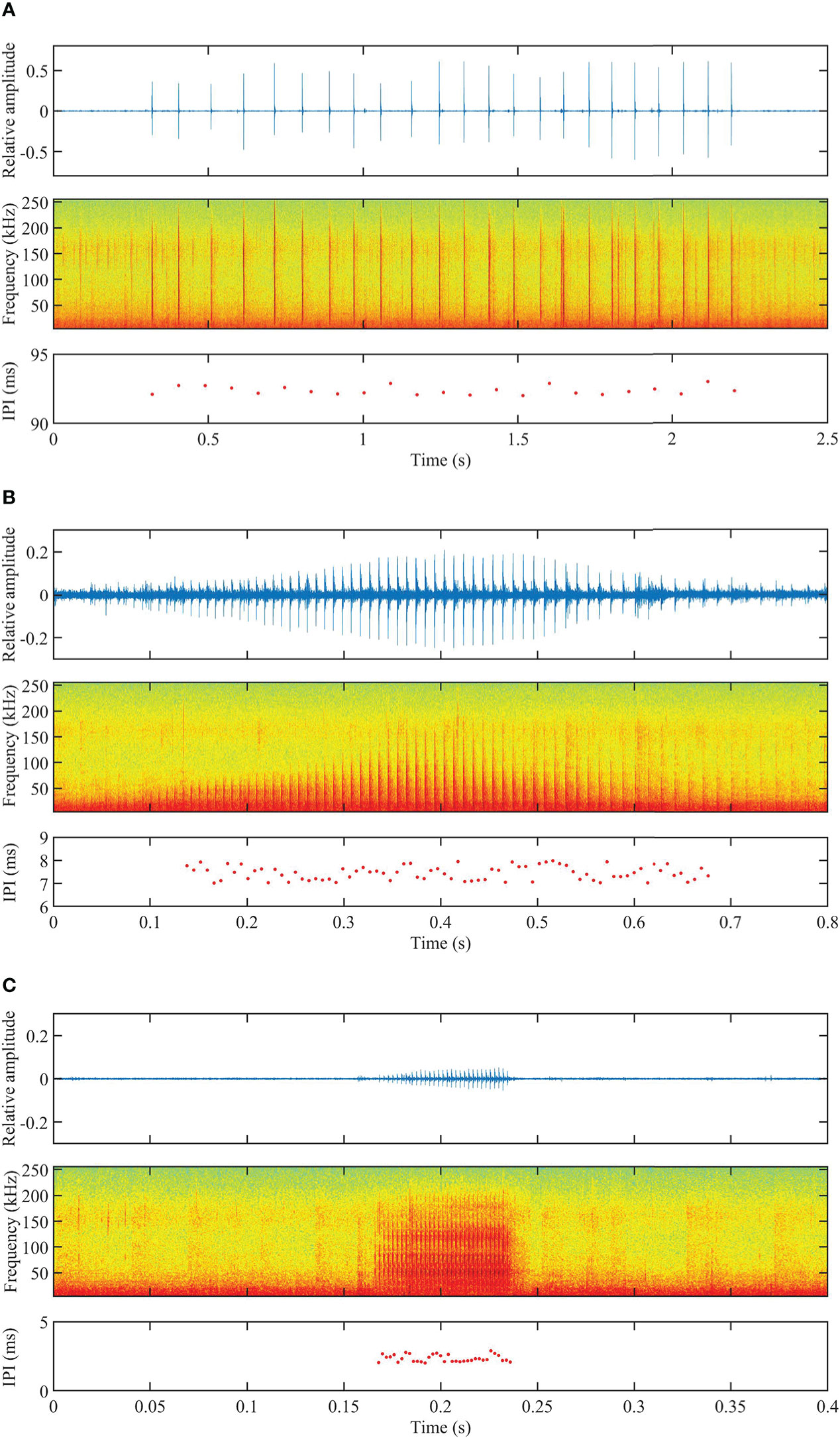
Figure 3 (Color online) Examples of waveforms (upper panels), spectrograms (middle panels), and IPIs throughout the pulsed signal train (bottom panels) showing a (A) click, (B) burst-pulse, and (C) buzz, representatively, produced by Indo-Pacific humpback dolphins. Spectrogram settings: high pass filter, filter-out noise under 4 kHz, Hamming window, FFT size = 512 points, frequency overlap = 50%. (Please note the difference in time scales (x-axis) on the three panels.).
Descriptive statistics for the acoustic parameters for each train type are presented in Table 3. Clicks had the widest ranges in terms of several parameters (Fp_m, BW−3 dB_m, BW−10 dB_m, and NumP), which covered those of the other types. The D values of clicks had the widest range and highest mean, which exceeded the upper limits of those for the other train types, and the minimum D value was higher than those for the other types. The IPIs_m for clicks had the highest range of 16 to 315.4 ms. The Fp_m values of burst-pulses had the lowest mean value and narrowest range. The minimum value of D exceeded the maximum D for buzzes. The NumP and IPIs_m values for burst-pulses were both larger than those for buzzes. Buzzes had the smallest D and IPIs_m values, and the lowest NumP among the three train types. Buzzes usually occurred (60 of 160, 37.5%) after clicks in our recordings (Figure 4).
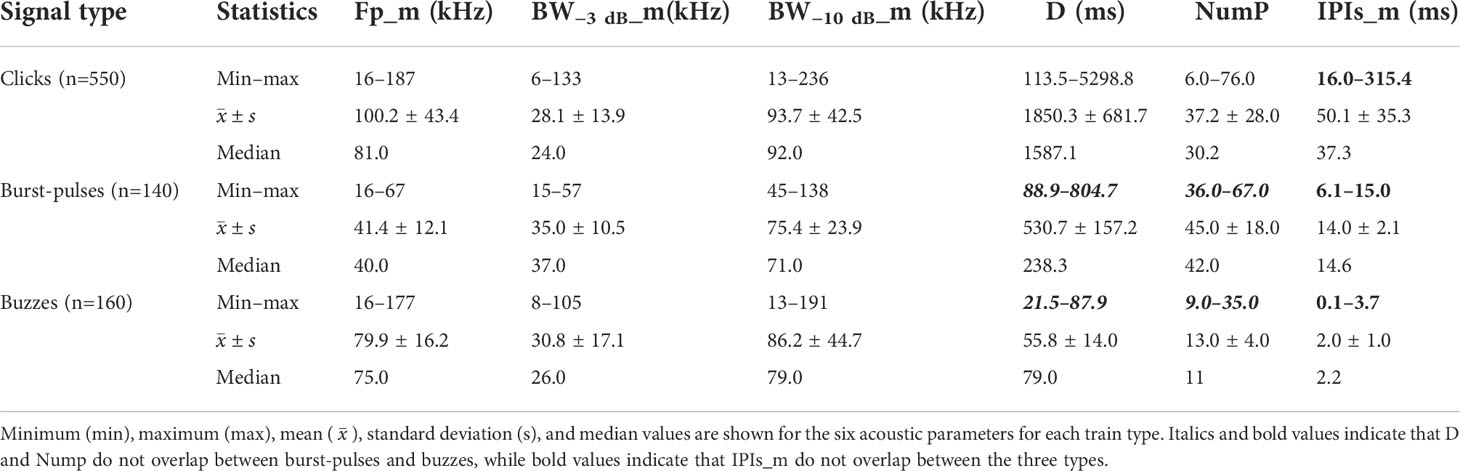
Table 3 Descriptive statistics for acoustic characteristics of train types produced by wild Indo-Pacific humpback dolphins in Beibu Gulf, South China Sea.
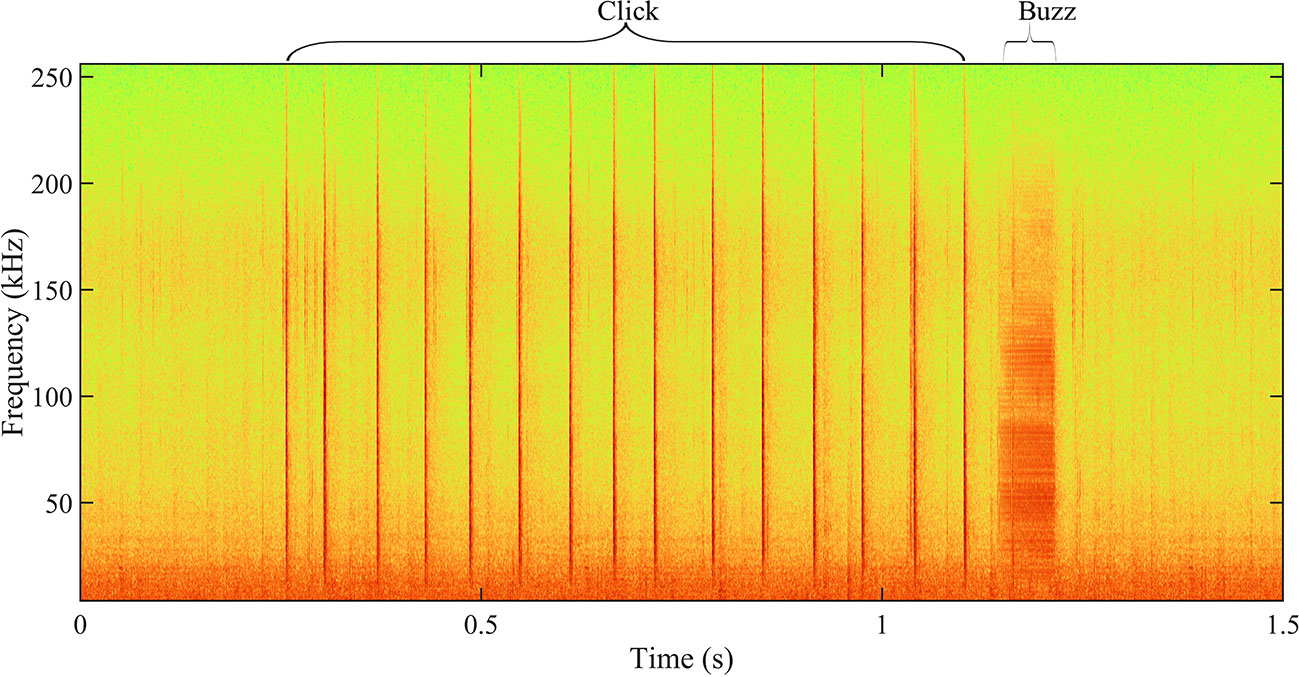
Figure 4 Example of sequential appearance in spectrograms showing representative click and buzz signal produced by Indo-Pacific humpback dolphins. Spectrogram settings: high pass filter, filter-out noise under 4 kHz, Hamming window, FFT size = 512 points, frequency overlap = 50%.
The ranges of NumP and D were non-overlapping between burst-pulses and buzzes, and both were covered by those of clicks (bold and italic in Table 3). Burst-pulses had a higher NumP and longer D. The ranges of the IPIs_m did not overlap among the three train types (bold in Table 3). The IPIs_m values were longest for clicks, followed by burst-pulses, and shortest for buzzes.
In conclusion, the train types could be classified according to the IPIs_m, where IPIs were greater than 15.5 ms for clicks, in the range of 4.9–15.5 ms for burst-pulses, and less than 4.9 ms for buzzes. These classification criteria could be of great significance for future research by allowing the rapid and effective identification of the three train types.
All of the recorded trains were identified (consisting of overlapping and non-overlapping ones) and matched with the behavioral information. In total, 1,080 trains were associated with behavioral observations, as shown in Table 4. According to Table 4 and Figure 5, this species emitted many clicks during almost every behavior and the highest percentage of clicks occurred during traveling. Buzzes were rarely used except during foraging. Burst-pulses accounted for a large proportion of the train types associated with socializing.
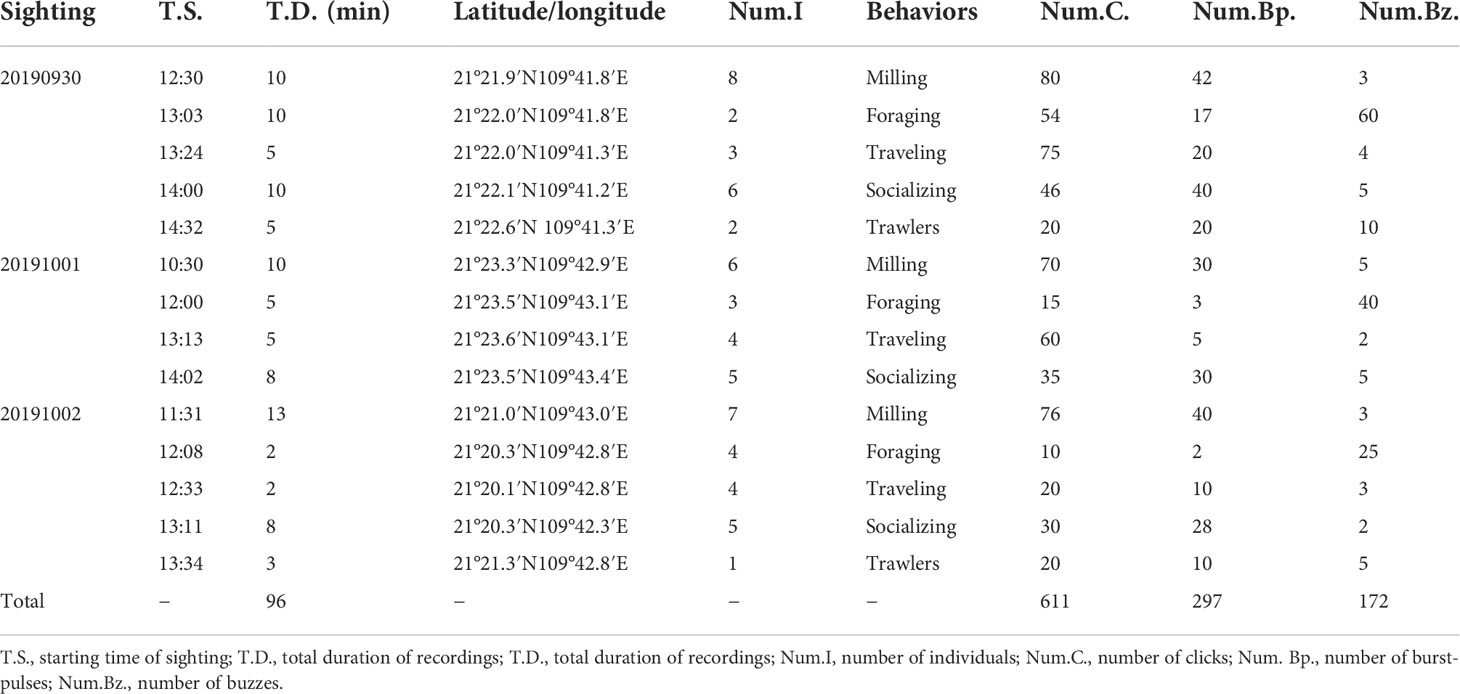
Table 4 Summary information and train types collected for Indo-Pacific humpback dolphins in Beibu Gulf, South China Sea.
Discussion
In this study, for the first time, we showed in an objective method that Indo-Pacific humpback dolphins emitted three distinct pulsed signal train forms comprising clicks, burst-pulses, and buzzes, based on the recordings in Beibu Gulf. All three types comprised a series of pulsed signals, but they differed in terms of their frequency, IPIs, and D. Clicks had higher frequencies and longer IPIs_m. Burst-pulses were relatively lower in frequency and had narrower bandwidths. Buzzes were characterized by a rapid reduction in the IPIs_m with the shortest D, and they usually occurred after clicks (Figure 4), which might be related to the final stage of prey capture. These features are similar to the three train types produced by other odontocetes (Griffin et al., 1960; Martin et al., 2018; Yang et al., 2021). Therefore, it was reasonable to divide the pulsed signal trains produced by Indo-Pacific humpback dolphins into three categories and designate them accordingly.
The IPI characteristics of clicks produced by Indo-Pacific humpback dolphins were mentioned in previous studies in the same manner as the buzzes and burst-pulses found in our results, but no further classification of clicks was performed (Van Parijs and Corkeron, 2001; Soto et al., 2014). The burst-pulses produced by this species in previous studies were all emitted by Indo-Pacific humpback dolphins in Australia, which differ in terms of their DNA compared with the Chinese species (Frere et al., 2011; Mendez et al., 2013).
The buzzes and burst-pulses identified in this species were just vaguely described or collectively referred to as one type in previous studies. Buzzes were called one of the two categories of the pulsed signals of Indo-Pacific humpback dolphins, referring to sounds with minimum inter-pulse intervals shorter than 10 ms reported in Wang et al. (2015). Caruso et al. (2020) noted a quick overview of the other type (called rapid click-series of pulsed sounds including buzzes and burst pulses) in other species. The general characteristics of buzzes and burst-pulses in our recordings are similar to those of the feeding buzzes (Thomas and Turl, 1990; Morozov et al., 1972; Au et al., 1974; Au and Snyder, 1980; Au, 1993) and burst-pulses (Martin et al., 2018; Yang et al., 2021) emitted by other odontocetes. Bottlenose dolphins emit feeding buzzes with a quickening pulse as they feed and terminal buzzes in courtship and play with objects (Pirotta et al., 2015; Herzing, 1996; Herzing, 2015; Herzing and dos Santos, 2004; Herzing, 2004) and they produce burst-pulses during social behaviors (Overstrom et al., 1983; Branstetter et al., 2012; Marian et al., 2021; Ridgway and Sam, 2011). Given that they are close relatives (John and Yang, 2009), it is reasonable to speculate that Indo-Pacific humpback dolphins could produce feeding buzzes and burst-pulses that sound similar to those of bottlenose dolphins in addition to clicks.
The pulsed signals of odontocetes are characterized by their directivity. A shallow water environment and the employment of a single hydrophone will lead to differences in the acoustic parameters of the received and transmitted signals. The differences in the frequency and energy are most significant for high-frequency signals (Martin et al., 2018), but the difference is very small in D and almost none in IPIs. It is feasible to classify the train types according to the parameters of the received signals (Martin et al., 2018; Yang et al., 2021; Arranz, 2016; Martin et al., 2018; Fregosi et al., 2020). In the present study, IPIs_m and D were identified as very important for classification according to PC1 (Table 2). The statistical results showed that the classifications of the three categories depended on IPIs (Table 3). Therefore, classification is possible based on a single hydrophone.
We conducted HCA and artificially stipulated that the similarity must be greater than 80% and the category number must be greater than 10. According to the results, the similarity was greater than 95% in every cluster and the number in each category was greater than 40 (Figure 2 and Table 3). Finally, a manual check of the clustering results showed that the specified thresholds were reasonable, and the classification results were reliable.
The three pulsed signal train types produced by Indo-Pacific humpback dolphins in Beibu Gulf could be distinguished by IPIs_m, with thresholds of 4.9 and 15.5 (Table 3). The threshold values could be different for this dolphin in other locations. Clicks had the longest IPIs_m, and the IPIs_m values for burst-pulses were between those for clicks and buzzes. The IPIs of buzzes could be used as a two-way travel time for targets to roughly estimate the distance to prey (Au et al., 1974; Au and Snyder, 1980; Turl and Penner, 1989; Thomas and Turl, 1990; Au, 1993). Shorter IPIs are associated with a shorter distance (Kalko, 1995). The IPIs of buzzes could be used to estimate the distance of the prey from Indo-Pacific humpback dolphins in a prey chase.
The variations in the three pulsed signal train types produced by Indo-Pacific humpback dolphins may allow more information to be encoded (Martin et al., 2018; Madsen et al., 2004a; Moore and Pawloski, 1990; Au, 1993; Li et al., 2013). Variations in sounds play key roles in social behavior, and studies have shown that changes in the acoustic parameters of clicks and burst-pulses emitted by striped dolphins are associated with the active environment and objective (Jarvis et al., 2013; Papale et al., 2020). Pulsed signal trains with longer IPIs and large D values can help dolphins to search, detect, and select targets (Madsen et al., 2004b). Shorter IPIs can allow the identification and capture of smaller targets. Ultrasonic pulsed signals have high directionality, and they decay rapidly as the distance increases (Niu et al., 2012). Therefore, reducing the frequency to an appropriate level is conducive to the further propagation of the signal and increasing the detection range (Martin et al., 2018). In this study, among the three types recorded, D and the IPIs_m were large for clicks, which are mainly used for navigation and positioning. The IPIs_m values were shortest for buzzes, which are conducive to the foraging activities of dolphins. The Fp_m values were lower for burst-pulses, which are mainly used for intraspecific communication (Table 3 and Table 4). Therefore, the changes in the three types of pulsed signal trains recorded in our observations were consistent with their functions, and it is reasonable to speculate that the changes in pulsed signal trains emitted by Indo-Pacific humpback dolphins were related to different behaviors.
Sound signals are very important for regulating the individual and group activities of dolphins (Fichtel and Manser, 2010). The complexity of individual and group behavior is considered to be the driving force responsible for the development of the dolphin communication system (Blumstein and Armitage, 1997; Freeberg et al., 2012a; Van Cise et al., 2018; Gustison et al., 2019). The three train types were produced by Indo-Pacific humpback dolphins in variable proportions during different behaviors (Table 4 and Figure 5) due to their different social functions.
Clicks are usually related to navigation, detection, and foraging (Au, 1993). In our recordings, the clicks were used in all behaviors and present at the highest proportion of three types in almost all states except foraging, most prevalent during traveling and milling (Table 4 and Figure 5), which is consistent with previous research (Au, 1993).
Studies have shown that burst-pulses are related to communication in the background environment, and they are considered intraspecific communicative signals (Lammers et al., 2004). Burst-pulses usually occur during courtship, aggression, and other states of relative excitement (collectively referred to as social activities) (Dawson, 1991; Jones and Sayigh, 2002; Blomqvist and Amundin, 2004; Simard et al., 2008; Gridley et al., 2016). Burst-pulses were mostly recorded in the present study during milling and socializing activities and occupied the highest proportion in socializing than other behavioral states (Figure 5). The number of burst-pulses increased when the group was large (≥3) or when trawlers passed by (Table 4). Dolphins emitted burst-pulses besides buzzes when they approached kayak in an investigatory manner (Martin et al., 2019). Captured Commerson’s dolphins emit burst-pulses when a new object enters their tank (Yoshida et al., 2014). Thus, burst-pulses might have an intraspecific communicative function in the Indo-Pacific humpback dolphin.
Buzzes are generally a sign of food capture during foraging to make other members of the population aware (Kellogg, 1959; Janik, 2000; Miller et al., 2004; Johnson et al., 2004; Madsen et al., 2005; Zimmer et al., 2005; Stimpert et al., 2007; Barlow et al., 2013), and they were used more frequently during foraging in the present study (Figure 5). Indo-Pacific humpback dolphins usually gather in small groups of three to five, or alone, and they may preferentially travel with trawlers to acquire food (Jefferson, 2000; Parra et al., 2006; Parra et al., 2011). Buzzes were observed when a dolphin was either play-chasing a prey or even a floating object like sargassum (Herzing and Santos, 2014; Herzing, 2014). In our recordings, buzzes occurred frequently in foraging activities, which was a general state but not in detail. Buzzes were also recorded when a trawler was observed passing (Table 4 and Figure 5). Dolphins may be present near trawlers because they are attracted to trawlers out of curiosity or for hunting purposes (Clay et al., 2018). When dolphins interact with kayaks, buzzes usually occur simultaneously (Martin et al., 2019). Furthermore, buzzes can be seen in other behaviors (for example, a mating activity and female calf discipline), with more social functions (Martin et al., 2019). It was similar to our recordings that buzzes were also detected in other behaviors (Table 4 and Figure 5). Thus, more social functions of buzzes in the Indo-Pacific humpback dolphin need to be explored with detailed behavior studies.
In conclusion, this study is the first to report the recording of buzzes and burst-pulses emitted by Indo-Pacific humpback dolphins in Beibu Gulf, South China Sea. Three typical types of pulsed train signals were identified using multivariate statistical analyses, and the detailed acoustic characteristics of each train type were described. Criteria were defined based on IPIs_m to identify the train types produced by these dolphins. Age, sex, individual variation, behavior, and other parameters may influence the threshold values. The functions of the three signals were further validated based on behavioral observations and identification. Conducting detailed behavioral studies and using an acoustic recording array would allow more information to be obtained in order to help interpret the functions of the different train types.
Data availability statement
The raw data supporting the conclusions of this article will be made available by the authors, without undue reservation.
Ethics statement
The animal study was reviewed and approved by Guangxi Hepu Dugong National Nature Reserve.
Author contributions
L-gL: review, editing, and approval of final manuscript. YJ: funding acquisition, data collection, editing, and approval of final manuscript. Z-wL, C-mY, and B-yC: data collection. X-yW: data analysis and writing—first draft and revision. All authors contributed to the article and approved the submitted version.
Funding
This work was supported by the National Natural Science Foundation of China (No. 41906170 and No. 31970497).
Acknowledgments
The authors thank the crew of Guangxi Hepu Dugong National Nature Reserve for support with data collection. The authors thank the Associate Editor Lance Garrison and two anonymous reviewers for their constructive comments on a previous version of this script.
Conflict of interest
The authors declare that the research was conducted in the absence of any commercial or financial relationships that could be construed as a potential conflict of interest.
Publisher’s note
All claims expressed in this article are solely those of the authors and do not necessarily represent those of their affiliated organizations, or those of the publisher, the editors and the reviewers. Any product that may be evaluated in this article, or claim that may be made by its manufacturer, is not guaranteed or endorsed by the publisher.
References
Allen J. A., Garland E. C., Dunlop R. A., Noad M. J. (2018). Cultural revolutions reduce complexity in the songs of humpback whales. P. R. Soc B-Biol. Sci. 285, 20182088. doi: 10.1098/rspb.2018.2088
Arranz P., DeRuiter S. L., Stimpert A. K., Neves S., Friedlaender A. S., Goldbogen J. A., et al (2016). Discrimination of fast click-series produced by tagged Risso's dolphins (Grampus griseus) for echolocation or communication. J. Exp. Bio. 219 (18), 2898–2907. doi: 10.1242/jeb.144295
Au W. W. L., Floyd R. W., Penner R. H., Murchinson A. E. (1974). Measurement of echolocation signals of the Atlantic bottlenose dolphin, Tursiops truncatus montagu, in open waters. J. Acoust. Soc Am. 56, 1280–1290. doi: 10.1121/1.1903419
Au W. W. L., Snyder K. J. (1980). Long-range target detection in open waters by an echolocating Atlantic bottlenose dolphin (Tursiops truncatus). J. Acoust. Soc Am. 68, 1077–1084. doi: 10.1121/1.384993
Barlow J., Tyack P. L., Johnson M. P., Baird R. W., Schorr G. S., Andrews R. D. (2013). Trackline and point detection probabilities for acoustic surveys of cuvier’s and blainville’s beaked whales. J. Acoust. Soc Am. 134, 2486–2496. doi: 10.1121/1.4816573
Blomqvist C., Amundin M. (2004). High-frequency burst-pulse sounds in agonistic/aggressive interactions in bottlenose dolphins, tursiops truncatus (Chicago, IL: The University of Chicago Press).
Blumstein D. T., Armitage K. B. (1997). Does sociality drive the evolution of communicative complexity? a comparative test with ground-dwelling sciurid alarm calls. Am. Nat. 150, 179–200. doi: 10.1086/286062
Branstetter B. K., Moore P. W., Finneran J. J., Tormey M. N., Aihara H. (2012). Directional properties of bottlenose dolphin (Tursiops truncatus) clicks, burst-pulse, and whistle sounds. J. Acoust. Soc Am. 131, 1613–1621. doi: 10.1121/1.3676694
Caruso F., Dong L., Lin M., Liu M., Xu S., Li S. (2020). Influence of acoustic habitat variation on Indo-Pacific humpback dolphin (Sousa chinensis) in shallow waters of Hainan Island, China. J. Acoust. Soc Am. 147 (6), 3871–3882. doi: 10.1121/10.0001384
Clay T. A., Mangel J. C., Alfaro-Shigueto J., Hodgson D. J., Godley B. J. (2018). Distribution and habitat use of a cryptic small cetacean, the burmeister’s porpoise, monitored from a small-scale fishery platform. Front. Mar. Sci. 5. doi: 10.3389/fmars.2018.00220
Cusano D. A., Paton D., Noad M. J., Dunlop R. A. (2022). Socially complex breeding interactions in humpback whales are mediated using a complex acoustic repertoire. Front. Mar. Sci. 8. doi: 10.3389/fmars.2021.665186
Dawson S. M. (1991). Clicks and communication: The behavioral and social contexts of hector’s dolphin vocalizations. Ethology 88, 265–276. doi: 10.1111/j.1439-0310.1991.tb00281.x
Fenton M. B. (2013). Questions, ideas and tools: Lessons from bat echolocation. Anim. Behav. 85, 869–879. doi: 10.1016/j.anbehav.2013.02.024
Fichtel C., Manser M. (2010). Vocal communication in social groups. In: Kappeler P. (eds) Animal Behaviour: Evolution and Mechanisms Berlin, Heidelberg: Springer, 29–54. doi: 10.1007/978-3-642-02624-9_2
Folkens P., Reeves R. (2002). Guide to marine mammals of the world. Ed. Alfred A. (New York: Knopf, Inc).
Freeberg T. M., Dunbar R. I., Ord T. J. (2012a). Social complexity as a proximate and ultimate factor in communicative complexity (London: The Royal Society).
Fregosi S., Harris D. V., Matsumoto H., Mellinger D. K., Barlow J., Baumann-Pickering S., et al. (2020). Detections of whale vocalizations by simultaneously deployed bottom-moored and deep-water mobile autonomous hydrophones. Front. Mar. Sci. 7. doi: 10.3389/fmars.2020.00721
Frere C., Seddon J., Palmer C., Porter L. J., Parra G. J. (2011). Multiple lines of evidence for an Australasian geographic boundary in the indo-pacific humpback dolphin (Sousa chinensis): Population or species divergence? Conserv. Genet. 1, 1–6. doi: 10.1007/s10592-011-0242-9
Goold J. C., Jefferson T. A. (2004). A note on clicks recorded from free-ranging indo-pacific humpback dolphins, Sousa chinensis. Aquat. Mamm. 30, 175–178. doi: 10.1578/AM.30.1.2004.175
Gridley T., Elwen S. H., Rashley G., Badenas Krakauer A., Heiler J. (2016). Bottlenose dolphins change their whistling characteristics in relation to vessel presence, surface behavior and group composition. J. Acoust. Soc Am. 27 (1), 010030. doi: 10.1121/2.0000312
Griffin D. R., Webster F. A., Michael C. R. (1960). The echolocation of flying insects by bats. Anim. Behav. 8, 141–154. doi: 10.1016/0003-3472(60)90022-1
Gustison M. L., Tinsley Johnson E., Beehner J. C., Bergman T. J. (2019). The social functions of complex vocal sequences in wild geladas. Behav. Ecol. Sociobiol. 73, 1–12. doi: 10.1007/s00265-018-2612-5
Hartman K. L., Visser F., Hendriks A. J. E. (2008). Social structure of risso's dolphins (Grampus griseus) at the Azores: A stratified community based on highly associated social units. Can. J. Zool. 86, 294–306. doi: 10.1139/Z07-138
Henderson E. E., Hildebrand J. A., Smith M. H., Falcone E. A. (2012). The behavioral context of common dolphin (Delphinus sp.) vocalizations. Mar. Mammal Sci. 28, 439–460. doi: 10.1111/j.1748-7692.2011.00498.x
Herzing D. L. (1996). Vocalizations and associated underwater behavior of free-ranging Atlantic spotted dolphins, stenella frontalis and bottlenose dolphins, Tursiops truncatus. Aquat. Mamm. 22, 61–80. doi: 10.1007/978-0-387-30425-0_20
Herzing D. L. (2004). “Social and non-social uses of echolocation in free-ranging stenella frontalis and tursiops truncatus,” in Advances in the study of echolocation in bats and dolphins (Springer-Verlag Press), 404–410.
Herzing D. L. (2015). Synchronous and rhythmic vocalizations and correlated underwater behavior of free-ranging Atlantic spotted dolphins (Stenella frontalis) and bottlenose dolphins (Tursiops truncatus) in the Bahamas. Anim. Behav. Cogn. 2, 14–29. doi: 10.12966/abc.02.02.2015
Herzing D. L., dos Santos M. E. (2004). “Functional aspects of echolocation in dolphins,” in Advances in the study of echolocation in bats and dolphins (Springer-Verlag Press), 386–393.
Janik V. M. (2000). Source levels and the estimated active space of bottlenose dolphin (Tursiops truncatus) whistles in the Moray firth, Scotland. J. Comp. Psych. 186, 673–680. doi: 10.1007/s003590000120
Jarvis J., Jackson W., Smotherman M. (2013). Groups of bats improve sonar efficiency through mutual suppression of pulse emissions. Front. Physiol. 4. doi: 10.3389/fphys.2013.00140
Jayathilaka R. M., Arulananthan K. (2019). Vocalization patterns of indo-pacific humpback dolphins (Sousa plumbea) in puttalam lagoon, Sri Lanka. J. Natl. Aquat. Res. Res. Dev. Agency. 45, 38–51.
Jefferson T. (2000). Population biology of the indo-pacific hump-backed dolphin in Hong Kong waters. Wildlife Monogr. 144, 1–65.
Jefferson T. A., Smith B. D., Braulik G. T., Perrin W. (2016). Re-assessment of the conservation status of the Indo-Pacific humpback dolphin (Sousa chinensis) using the IUCN Red List criteria Adv. Mar. Biol. 73, 1–26. doi: 10.1016/bs.amb.2015.04.002
Jiang Y., Liu Z. W., Yang C. M., Lü L. G., Muda A. M. (2020). High-frequency whistles of irrawaddy dolphins (Orcaella brevirostris) recorded in Brunei bay. Mar. Mammal Sci. 36 (3), 846–857. doi: 10.1111/mms.12681
Johnson M., Madsen P. T., Zimmer W. M. X., Soto N. A., Tyack P. L. (2004). Beaked whales echolocate on prey. P. R. Soc B-Biol Sci. 271, 383–386. doi: 10.2307/4143015
John Y. W., Yang S. C. (2009). “Indo-pacific bottlenose dolphin: Tursiops aduncus,” in Encyclopedia of marine mammals, 2nd ed. (Academic Press), 602–608. doi: 10.1016/B978-0-12-373553-9.00141-3
Jones G. J., Sayigh L. S. (2002). Geographic variation in rates of vocal production of free-ranging bottlenose dolphins. Mar. Mammal Sci. 18, 374–393. doi: 10.1111/j.1748-7692.2002.tb01044.x
Kalko E. K. V. (1995). Insect pursuit, prey capture and echolocation in pipestirelle bats (Microchiroptera). Anim. Behav. 50, 861–880. doi: 10.1016/0003-3472(95)80090-5
Kellogg W. N. (1959). Auditory perception of submerged objects by porpoise. J. Acoust. Soc Am. 31, 1. doi: 10.1121/1.1907606
Lammers M. O., Au W. W. L., Aubauer R., Nachtigall P. E. (2004). Echolocation bats dolphins (Chicago: University Chicago Press).
Li S. H., Shi W. J., Fang L., Wang K. X. (2015). Echolocation signals of free-ranging indo-pacific humpback dolphins (Sousa chinensis) in sanniang bay, China. J. Acoust. Soc Am. 138, 1346–1352. doi: 10.1121/1.4929492
Li S., Wang D., Wang K., Hoffmann-Kuhnt M., Fernando N., Taylor E. A. (2013). Possible age-related hearing loss (presbycusis) and corresponding change in echolocation parameters in a stranded indo-pacific humpback dolphin. J. Exp. Biol. 216, 4144–4153. doi: 10.1242/jeb.091504
Madsen P. T., Johnson M., Aguilar De Soto N., Zimmer W. M. X., Tyack P. (2005). Biosonar performance of foraging beaked whales (Mesoplodon densirostris). J. Exp. Biol. 208, 181–194. doi: 10.1242/jeb.01327
Madsen P. T., Kerr I., Payne R. (2004a). Source parameter estimates of echolocation clicks from wild pygmy killer whales (Feresa attenuata) J. Acoust. Soc Am. 116, 1909–1912. doi: 10.1121/1.1788726
Madsen P. T., Kerr I., Payne R. (2004b). Echolocation clicks of two free-ranging, oceanic delphinids with different food preferences: false killer whales Pseudorca crassidens and risso's dolphins Grampus griseus. J. Exp. Biol. 207, 1811–1823. doi: 10.1242/jeb.00966
Madsen P. T., Wahlberg M. (2007). Recording and quantification of ultrasonic echolocation clicks from free-ranging toothed whales. Deep. Res. Part I Oceanogr. Res. Pap. 54, 1421–1444. doi: 10.1016/j.dsr.2007.04.020
Marian A. D., Monczak A., Balmer B. C., Hart L. B., Montie E. W. (2021). Long-term passive acoustics to assess spatial and temporal vocalization patterns of Atlantic common bottlenose dolphins (Tursiops truncatus) in the may river estuary, south Carolina. Mar. Mammal Sci., 1–25. doi: 10.1111/mms.12800
Martin M. J., Elwen S. H., Kassanjee R., Gridley T. (2019). To buzz or burst-pulse? the functional role of heaviside's dolphin, Cephalorhynchus heavisidii, rapidly pulsed signals. Anim. Behav. 150, 273–284. doi: 10.1016/j.anbehav.2019.01.007
Martin M. J., Gridley T., Elwen S. H., Jensen F. H. (2018). Heavisides dolphins (Cephalorhynchus heavisidii) relax acoustic crypsis to increase communication range. Proc. R. Soc B Biol. Sci. 285, 20181178. doi: 10.1098/rspb.2018.1178
Mendez M., Jefferson T. A., Kolokotronis S. O., Kr€utzen M., Parra G. J., Collins T., et al. (2013). Integrating multiple lines of evidence to better understand the evolutionary divergence of humpback dolphins along their entire range: A new dolphin species in Australian waters? Mol. Ecol. 22, 5936–5948. doi: 10.1111/mec.12535
Miller P. J., Johnson M. P., Tyack P. L. (2004). Sperm whale behaviour indicates the use of echolocation click buzzes ‘creaks’ in prey capture. P. R. Soc B-Biol. Sci. 271, 2239–2247. doi: 10.1098/rspb.2004.2863
Moore P., Pawloski D. A. (1990). “Investigations on the control of echolcation pulses in the dolphin (Tursiops truncatus),” in Sensory abilities of cetaceans. Eds. Thomas J. A., Kastelein R. A. (Boston, MA: Springer), 305–316. doi: 10.1007/978-1-4899-0858-2_19
Morisaka T., Karczmarski L., Akamatsu T., Sakai M., Dawson S., Thornton M. (2011). Echolocation signals of heaviside’s dolphins (Cephalorhynchus heavisidii). J. Acoust. Soc Am. 129, 449–457. doi: 10.1121/1.3519401
Morozov A. I., Esipchuk Y. V., Kapulkin A. M., Nevrovskii V. A., Smirnov V. A. (1972). Effect of the magnetic field on a closed-electron-drift accelerator. Sov. Phys. Tech. Phys. (Engl. Transl.) 17 (3), 482–87.
Niu F. Q., Yang Y. M., Xu X. M., Wen H. T., Liu Z. W. (2012). Study on the characteristics of echolocation clicks from indo-pacific humpback dolphins (Sousa chinensis) in xiamen (in Chinese). J. XiaMen Univ. 51, 951–954. doi: 10.1007/s11783-011-0280-z
Overstrom N. A. (1983). Association between burst-pulse sounds and aggressive behavior in captive Atlantic bottlenosed dolphins (Tursiops truncatus). Zoo Biol. 2, 93–103. doi: 10.1002/zoo.1430020203
Papale E., Fanizza C., Buscaino G., Ceraulo M., Cipriano G., Crugliano R., et al. (2020). The social role of vocal complexity in striped dolphins. Front. Mar. Sci. 7. doi: 10.3389/fmars.2020.584301
Parra G. J., Corkeron P. J., Arnold P. W. (2011). Grouping and fission-fusion dynamics in Australia snubfin and indo-pacific humpback dolphins. Anim. Behav. 82, 1423–1433. doi: 10.1016/j.anbehav.2011.09.027
Parra G. J., Corkeron P. J., Marsh H. (2004). The indo-pacific humpback dolphin, Sousa chinensis (Osbeck 1765), in Australian waters: A summary of current knowledge. Aquat. Mamm. 30, 197–206. doi: 10.1578/AM.30.1.2004.197
Parra G. J., Corkeron P. J., Marsh H. (2006). Population sizes, site fidelity and residence patterns of Australian snubfin and indo-pacific humpback dolphins: Implications for conservation. Biol. Conserv. 129, 167–180. doi: 10.1016/j.biocon.2005.10.031
Pirotta E., Merchant N. D., Thompson P. M., Barton T. R., Lusseau D. (2015). Quantifying the effect of boat disturbance on bottlenose dolphin foraging activity. Biol. Conserv. 181, 82–89. doi: 10.1016/j.biocon.2014.11.003
Ridgway S. H. (2011). Neural time and movement time in choice of whistle or pulse burst responses to different auditory stimuli by dolphins. J. Acoust. Soc Am. 129, 1073. doi: 10.1121/1.3523431
Sarnocińska J., Teilmann J., Balle J. D., Beest F. M. V., and Tougaard J. (2020). Harbor porpoise (Phocoena phocoena) reaction to a 3d seismic airgun survey in the north sea. Front. Mar. Sci. 6, 824. doi: 10.3389/fmars.2019.00824
Sørensen P. M., Wisniewska D. M., Jensen F. H., Johnson M., Teilmann J., Madsen P. T. (2018). Click communication in wild harbour porpoises (Phocoena phocoena). Sci. Rep. 8, 1–11. doi: 10.1038/s41598-018-28022-8
Shirihai H., Jarrett B., Kirwan G. M. (2006). Whales, dolphins, and other marine mammals of the world (Princeton and Oxford: Princeton University Press).
Simard P., Mann D. A., Gowans S. (2008). Burst-pulse sounds recorded from white-beaked dolphins (Lagenorhynchus albirostris). Aquat. Mamm. 34, 464–470. doi: 10.1578/am.34.4.2008.464
Sims P. Q., Vaughn R., Hung S. K., Würsig B. (2012). Sounds of indo-pacific humpback dolphins (Sousa chinensis) in West Hong Kong: A preliminary description. J. Acoust. Soc Am. 131, 48–53. doi: 10.1121/1.3663281
Soto A. B., Marsh H., Everingham Y., Smith J. N., Parra G. J., Noad M. (2014). Discriminating between the vocalizations of indo-pacific humpback and Australian snubfin dolphins in Queensland, Australia. J. Acoust. Soc Am. 136, 930–938. doi: 10.1121/1.4884772
Stimpert A. K., Wiley D. N., Au W. W. L., Johnson M. P., Arsenault R. (2007). “Megapclicks”: acoustic click trains and buzzes produced during night-time foraging of humpback whales (Megaptera novaeangliae). Biol. Lett. 3, 467–470. doi: 10.1098/rsbl.2007.0281
Thomas J. A., Turl W. (1990). “Echolocation characteristics and range detection threshold of a false killer whale (Pseudorca crassidens),” in Sensory abilities of cetaceans. Eds. Thomas J. A., Kastelein R. A. (Boston, MA: Springer), 321–334.
Turl C. W., Penner R. H. (1989). Differences in echolocation click patterns of the beluga (Delphinapterus leucas) and the bottlenose dolphin (Tursiops truncatus). J. Acoust. Soc Am. 86, 497–502. doi: 10.1121/1.398229
Van Cise A. M., Mahaffy S. D., Baird R. W., Mooney T. A., Barlow J. (2018). Song of my people: dialect differences among sympatric social groups of short-finned pilot whales in hawai’i. Behav. Ecol. Sociobiol. 72, 1–13. doi: 10.1007/s00265-018-2596-1
Van Parijs S. M., Corkeron P. J. (2001). Vocalizations and behaviour of pacific humpback dolphins Sousa chinensis. Ethology 107, 701–716. doi: 10.1046/j.1439-0310.2001.00714.x
Van Parijs S. M., Smith J., Corkeron P. J. (2002). Using calls to estimate the abundance of inshore dolphins: A case study with pacific humpback dolphins Sousa chinensis. J. Appl. Ecol. 39, 853–864. doi: 10.1046/j.13652664.2002.00756.x
Wang Q. M., Xu X. M., Su G. L., Su H. T. (2014). The analysis and comparison of the clicks of Sousa chinensis in xiamen Sea and leizhou bay (in Chinese). J. XiaMen Univ. 53, 104–109. doi: 10.6043/j.issn.0438-0479.2014.01.021
Wang Z. T., Nachtigall P. E., Akamatsu T., Wang K. X., Wu Y. P., Liu J. C., et al (2015). Passive Acoustic Monitoring the Diel, Lunar, Seasonal and Tidal Patterns in the Biosonar Activity of the Indo-Pacific Humpback Dolphins (Sousa chinensis) in the Pearl River Estuary, China. PLOS ONE 10 (11), e0141807. doi: 10.1371/journal.pone.0141807
Yang L. L., Sharpe M., Temple A. J., Berggren P. (2021). Characterization and comparison of echolocation clicks of white-beaked dolphins (Lagenorhynchus albirostris) off the Northumberland coast, UK. J. Acoust. Soc Am. 149, 1498–1506. doi: 10.1121/10.0003560
Yoshida Y. M., Morisaka T., Sakai M., Iwasaki M., Wakabayashi I., Seko A. (2014). Sound variation and function in captive commerson’s dolphins (Cephalorhynchus commersonii). Behav. Processes. 108, 11–19. doi: 10.1016/j.beproc.2014.08.017
Zhang L. H., Xu X. M., Wei C. (2011). Comparison between indo-pacific bottlenose dolphin (Tursiops aduncus) and indo-pacific humpback (Sousa chinensis) whistle (in Chinese). Tech. Acoust. 30, 41–44.
Keywords: burst-pulse, buzz, classification, click, foraging, Indo-Pacific humpback dolphin, pulsed signal train, social function
Citation: Wang X-y, Jiang Y, Liu Z-w, Yang C-m, Chen B-y and Lü L-g (2022) Three types of pulsed signal trains emitted by Indo-Pacific humpback dolphins (Sousa chinensis) in Beibu Gulf, South China Sea. Front. Mar. Sci. 9:915668. doi: 10.3389/fmars.2022.915668
Received: 08 April 2022; Accepted: 07 July 2022;
Published: 05 August 2022.
Edited by:
Lance Garrison, National Oceanic and Atmospheric Administration (NOAA), United StatesReviewed by:
Denise Lore Herzing, Florida Atlantic University, United StatesYvonne M. Barkley, University of Hawaii at Mānoa, United States
Copyright © 2022 Wang, Jiang, Liu, Yang, Chen and Lü. This is an open-access article distributed under the terms of the Creative Commons Attribution License (CC BY). The use, distribution or reproduction in other forums is permitted, provided the original author(s) and the copyright owner(s) are credited and that the original publication in this journal is cited, in accordance with accepted academic practice. No use, distribution or reproduction is permitted which does not comply with these terms.
*Correspondence: Lian-gang Lü, bHZsZ0BmaW8ub3JnLmNu