- 1Ocean and Earth Science, University of Southampton, National Oceanography Centre Southampton, European Way, Southampton, United Kingdom
- 2British Antarctic Survey, Cambridge, United Kingdom
- 3World Wide Fund for Nature, Australia (WWF-Australia), Melbourne, VC, Australia
- 4Curtin University, Centre for Marine Science & Technology, Perth, WA, Australia
- 5Marine Mammal Institute, Oregon State University, Newport, OR, United States
- 6Ocean Sciences & Institute for Marine Sciences, University of California Santa Cruz, Santa Cruz, CA, United States
In Antarctica, abundant consumers rely on Antarctic krill for food, but krill are also the subject of a commercial fishery. The fishery overlaps in time and space with the foraging areas of these consumers, thus potential competition between krill fisheries and krill consumers is a major management concern. The fishery is managed by the Commission for the Conservation of Antarctic Marine Living Resources with an ecosystem approach, according to which fishing should not interfere with either the population growth of krill, or krill-dependent consumers. Krill catches have become increasingly spatially concentrated in a small number of hotspots, raising concerns about how local depletion of krill impacts consumers. Such concentrated fishing demonstrates that there is a mismatch between the spatial and temporal scale at which krill fisheries are currently managed, and that at which fisheries operate and consumers forage. Information on the seasonal dynamics of predator abundance and their foraging behaviour is fundamental to future precautionary management of the krill fishery. We analysed the spatiotemporal distribution of two major krill consumers – humpback and minke whales – and that of krill fishing, off the Western Antarctic Peninsula. We used whale tracking data (58 humpback whale tracks and 19 minke whale tracks) to develop spatial random forest models predicting the monthly distribution of whale foraging areas from January-July. Using these predictions, we calculated spatiotemporally-explicit geographic overlap between whales and fisheries, the latter represented by krill fishing effort and catch data. Over the krill fishing season, fishing effort and catch hotspots shifted to the southwest, into the Bransfield Strait where effort and catch was highest. Predicted humpback whale foraging areas increased in the Bransfield Strait over the same period, while predicted minke whale foraging areas showed an opposite trend. For both we predicted a whale-fishing interaction hotspot in the Bransfield Strait, strongest in April and May. Our results illustrate the fine spatial scale of likely interactions between baleen whales and the krill fishery, and their concentration over the season, underlining the need for fishery management more closely aligned to the spatiotemporal scale of likely predator-fishery interactions.
Introduction
Large marine vertebrates, like marine mammals, frequently occupy high trophic levels and often rely on prey that are also subject to human fishing. Thus, spatial overlap between large marine vertebrates and fishing is apparently widespread (e.g., Queiroz et al., 2019; Hindell et al., 2020), but can be more acute when and where prey are aggregated (e.g., Scales et al., 2018). Fisheries impact large marine vertebrates through direct interactions (targeted or incidental capture, e.g., Lewison et al., 2014) and indirect interactions (provisioning, resource competition, e.g., Grémillet et al., 2018) and potential interactions are consequently a conservation concern for marine top predators as well as for fishery management.
The Antarctic Krill Fishery and Krill Consumers
In Antarctica, abundant consumers rely on Antarctic krill (Euphausia superba, hereafter ‘krill’) for food, and the top-down and bottom-up interactions between krill and its consumers play a key role in structuring Antarctic marine ecosystems (Trathan & Hill, 2016). Krill are also the subject of a commercial fishery (Nicol et al., 2012) that overlaps in time and space with foraging of consumers and the potential competition between krill fisheries and krill consumers is thus a major management concern (e.g., Trivelpiece et al., 2011; Forcada et al., 2012; Trathan & Hill, 2016; Weinstein et al., 2017; Warwick-Evans et al., 2018; Watters et al., 2020; Bamford et al., 2021; Krüger et al., 2021).
The krill fishery is managed by the Commission for the Conservation of Antarctic Marine Living Resources (CCAMLR) with an ecosystem approach, according to which fishing should not interfere with the population growth of krill, or krill-dependent consumers, including those that are recovering from historical exploitation (Constable et al., 2000). Almost all krill catches are from the southwest Atlantic sector of the Southern Ocean, FAO Area 48, where the krill population is concentrated (Atkinson et al., 2017). In some years small amounts have also been taken from the Indian Ocean sector of Antarctica. The current (annual) total allowable catch (precautionary catch limit) for the southwest Atlantic, based on an acoustic estimate of stock biomass undertaken in 2000 (Hewitt et al., 2004; SC-CCAMLR, 2010), is currently 5.61 million tonnes. Over recent years, catches have been increasing, reaching a maximum of 450,813 tonnes in 2020, with a mean catch of 267,386 tonnes over the period between 2010 and 2020 (CCAMLR, 2021). As such, krill is “one of the world’s largest known underexploited marine stocks” (Nicol et al., 2012) and could account for about 10% of future marine landings (Trathan et al., 2022).
Now, with the potential expansion of the krill fishery, there is increasing pressure on this shared resource, since populations of many krill consumers are changing (Bestley et al., 2020; Strycker et al., 2020). In line with their precautionary, ecosystem approach to management, CCAMLR has set an interim catch limit (or ‘trigger level’) of 620,000 tonnes for the southwest Atlantic krill fishery (Hill et al., 2016, Trathan et al., 2022). This trigger level has been set until such a time that CCAMLR has agreed a way in which to spatially (and temporally) subdivide the much larger precautionary catch limit. Although catches are considered to be low compared to overall krill abundance (the interim catch limit is ~1% of the total estimated biomass across the southwest Atlantic [SC-CCAMLR, 2019]), catches are expected to keep increasing into the future with the development of new processing and fishing technologies. Further, due to interannual variability in total krill abundance and spatial distribution, local catch limits based on a single year assessment or even average assessments across multiple years, may exceed local krill abundance in some years. In recent years, krill catches have become increasingly spatially concentrated in a small number of hotspots (Weinstein et al., 2017; Santa Cruz et al., 2018; Krüger, 2019; Santa Cruz et al., 2022; Trathan et al., 2022), raising concerns about how local depletion of krill impacts its consumers (e.g., Weinstein et al., 2017; Watters et al., 2020; Krüger et al., 2021; Santa Cruz et al., 2022).
CCAMLR has recognized that this increasingly concentrated krill fishing necessitates a smaller-scale management approach that better reflects the scale of krill-predator interactions and the current behaviour of the fishing fleet (i.e., at the scale of tens of kms) (SC-CCAMLR, 2016, paragraph 3.62 and 3.106). However, catches are still managed at the Subarea scale (658,730 km2 - 1,033,248 km2 for Subareas 48.1-48.4), even though ‘Small Scale Management Units’ (16,000 km2 - 440,000 km2 (Figure 1) have been designated. In the southwest Atlantic (Subareas 48.1, 48.2, 48.3 and 48.4) harvesting is limited to 25%, 45%, 45% and 15%, respectively, of the trigger level. These interim Subarea limits sum to 130% of the overall trigger level to allow spatial flexibility for the fishery, but the overall catch across these four Subareas is still limited by the overall trigger level (Trathan et al., 2022).
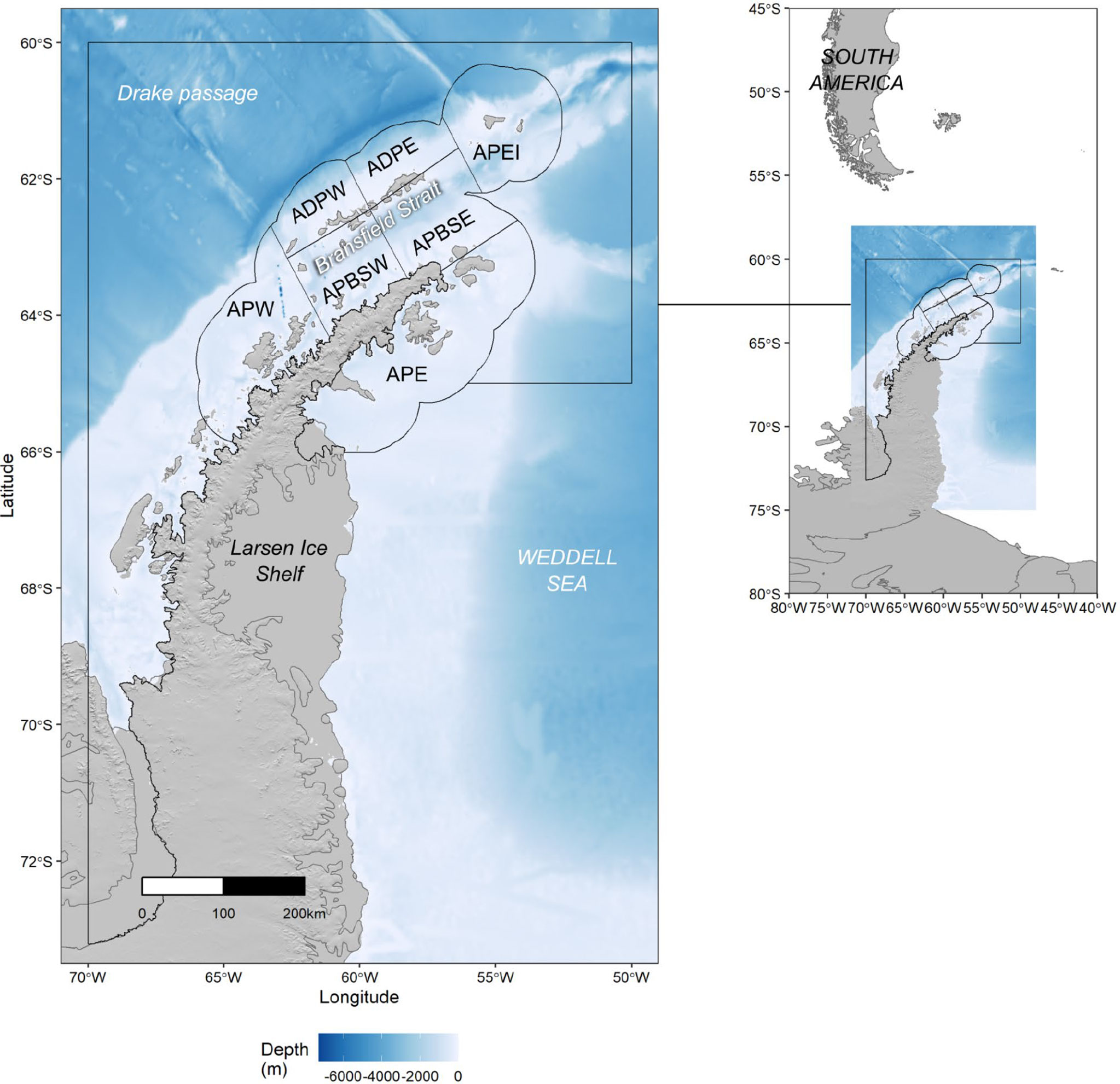
Figure 1 Map of the Antarctic Peninsula, Western Antarctica, showing Subarea 48.1 (black bounding box). Within Subarea 48.1, seven Small Scale Management Units (SSMUs) are indicated; APW, Antarctic Peninsula West; APDPW, Antarctic Peninsula Drake Passage West: APDPE, Antarctic Peninsula Drake Passage East; APEI, Antarctic Peninsula Elephant Island; APBSW, Antarctic Peninsula Bransfield Strait West; APBSE, Antarctic Peninsula Bransfield Strait East: APE, Antarctic Peninsula East.
Competing with the commercial krill fishery are numerous natural krill consumers including fishes, squid, seabirds and marine mammals, not to mention the numerous invertebrate species that feed on early life-history stages of krill (Trathan and Hill, 2016). The air-breathing consumers adjust their foraging areas in response to prey availability and accessibility, but are spatially restricted due to their intrinsic ecology, such as migratory patterns in baleen whales or the central place foraging constraints associated with breeding on land for penguins and seals. This means that consumers usually shift their foraging areas (e.g., Trathan et al., 2018) over an approximately annual timescale and over spatial scales of 10s – 100s or 1000s of kms. Accompanied by these changes in the areas foraged by consumers are changes in their energy requirements. Together this produces a dynamic pattern of space-use and energy requirement that must be incorporated into the risk assessment framework for managing the Antarctic krill fishery (e.g., Hinke et al., 2017a; Weinstein et al., 2017). Integral to the finer scale (10s of km and hours to weeks) space-time structuring of these patterns is the way in which predators find and exploit patchily distributed prey. Predators initially search for prey using faster, more linear movements but when they encounter prey they slow down and turn more often to remain in the prey patch. Predators forage in these patches until prey reaches some ‘giving up’ density below which foraging is no longer profitable. It is at these spatiotemporal scales that we might expect to detect predator-prey overlap, as well as overlap with fisheries, since similar dynamics seem to operate in the krill fishing fleet. Vessels must locate large, dense krill swarms that they exploit before moving to search for a new krill swarm (Santa Cruz et al., 2018). Such dynamics may also be intensified if fishing vessels also use cues similar to those used by dependent predators, for example the presence of other foraging predators such as baleen whales. Krill fishing vessels exploit krill hotspots for about 3-17 days, whereafter they move on due to declining catches indicating local krill depletion (Santa Cruz et al., 2018). However, in some hotspots close to shore off the Western Antarctic Peninsula, concentrated fishing can last longer, but still with evident declines in catches towards the end of the event, which differed for different vessels within the fishing hotspot (Trathan et al., 2016).
Such concentrated fishing demonstrates a mismatch between the spatial and temporal scale at which krill fisheries are currently managed, and that at which fisheries operate and consumers forage. Information on the seasonal dynamics of predator abundance and their foraging behaviour is fundamental to future precautionary management of the krill fishery. Improved analyses on both spatial and functional overlap of consumers with the fishery and how they both interact with krill will be extremely informative, especially for key predator groups about which foraging information is limited. In particular, baleen whales are not included in CCAMLR’s management approach even though they are known to be major krill consumers (e.g., Savoca et al., 2021). Importantly, baleen whales are also not included in the CCAMLR Ecosystem Monitoring Program which monitors other krill consumers (Agnew, 1997).
Thus, there is a clear and urgent need to better understand the spatiotemporal characteristics of potential interactions between krill consumers and the krill fishery. This is perhaps most urgent for those species that have not previously been considered in detail and which are known to be recovering rapidly from previous exploitation. Krill-eating baleen whales are therefore probably the most important taxonomic group for which CCAMLR needs information (Trathan et al., 2022). Moreover, recent by-catch of whales in the krill fishery demonstrate that fishing vessels and baleen whales do not always easily coexist (CCAMLR Secretariat, 2021; Delegations of Norway and the United Kingdom, 2021). Understanding the spatiotemporal distribution of both whales and fishing vessels is now urgent. Fortunately, animal biologging and vessel tracking provides data that can be used to address this need.
Humpback and Minke Whales
One of the most important consumers of krill in the Southern Ocean is the humpback whale (Megaptera novaeangliae), a medium sized (~15 m) baleen whale which, migrates annually from low latitude overwintering grounds to mid and high latitude summer feeding grounds (Clapham, 2018). Southern hemisphere humpback whale populations were severely depleted by catches of ~215,000 animals during industrial whaling from 1900-1979 (Rocha et al., 2015). Circumpolar surveys suggest a total Southern Ocean abundance >55,000 in 2011 (Branch, 2011). Most populations are increasing (albeit at different rates) and some are estimated to be near their pre-exploitation abundance (International Whaling Commission, 2016). The humpback whale population that uses waters off the Western Antarctic Peninsula is increasing rapidly (Pallin et al., 2018) and unprecedentedly dense ‘super-aggregations’ have been observed in this region (Nowacek et al., 2011). Spatial abundance models estimate around 19,000 individuals in this region in early summer (Johannessen et al., 2022; see also Warwick-Evans et al., 2022), a 5.1% per annum increase from the estimated 7,000 individuals in the same region in 2000 (Hedley et al., 2001; Johannessen et al., 2022). Their global IUCN Red List classification is Least Concern (Cooke, 2018).
Another major Southern Ocean krill consumer is the smaller (~8m) but much more abundant Antarctic minke whale (Balaenoptera bonaerensis) (Friedlaender et al., 2014). Their Southern Ocean population has been estimated to be around 500,000 individuals, based on circumpolar surveys from 1993-2004 (International Whaling Commission, 2013). This represents a 13% decline from the 1986-1991 survey estimate of around 700,000 animals, although both estimates are imprecise [due largely to minke whales’ affinity for sea ice (Williams et al., 2014; Herr et al., 2019)] and the trend is thus not significant (International Whaling Commission, 2013). Nonetheless, the apparent decline is one of the reasons for their current IUCN Red List classification as Near-Threatened (Cooke et al., 2018).
Habitat modelling of humpback and minke whale sighting data in the Western Antarctic Peninsula showed that the distribution of these whales was best explained by krill distribution (Friedlaender et al., 2006; Friedlaender et al., 2011). Using tracking data from five humpback whales, Curtice et al. (2015) showed that their distribution changed over their feeding season from January to June. Individuals moved inshore and their movements became more concentrated, likely a response to the inshore movement and aggregation of krill over the same period (Curtice et al., 2015), given krill are thought also to follow a seasonal migration (Siegel, 1988).
Weinstein et al. (2017) used tracking data from 33 humpback whales in the Western Antarctic Peninsula (Subarea 48.1) to map the month-by-month foraging areas of whales in relation to krill catches. Individuals used some of the Small-Scale Management Units significantly more than expected, suggesting that a uniform krill catch limit for all SSMUs in Subarea 48.1 is overly broad and ignores important variation in humpback whale distribution and critical foraging areas. The authors recommended that two Small Scale Management Units particularly – Antarctic Peninsula West and Bransfield Strait West – deserve special attention if fine scale management is to be implemented (Weinstein et al., 2017). However, this work did not account for the dynamic behaviour of the fishery on a predator-relevant timescale. Friedlaender et al. (2021) showed that humpback whales range throughout continental shelf waters and nearshore bays along the Western Antarctic Peninsula, while minke whales prefer sheltered bays and areas where sea ice is present. Minke whales thus occupy areas within the areas used by humpback whales (Friedlaender et al., 2021).
Aims
This simultaneous concentration of krill, whales and fisheries inshore late in the fishing season (late austral summer into autumn) heightens the possibility of local depletion and disruption of whale populations, but new information is required to address this issue in light of CCAMLR’s Risk Assessment Framework for krill fishery management. Here, we address this information gap by investigating the spatiotemporal distribution of humpback whale and minke whale foraging behaviour in the Western Antarctic Peninsula as well as that of krill fishing in the same region. We do this using recent whale tracking data and fishing catch and effort data. We develop spatial models to predict monthly whale distribution from January-July, thereby calculating spatiotemporally explicit geographic overlap between whales and fisheries.
Methods
Computation
All analyses were performed in the R environment (R Core Team, 2022). Scripts used to conduct the analyses can be found in the Github repository https://github.com/ryanreisinger/whaleKrillOverlap.
Whale Movement Behaviour
We collated published and unpublished satellite tag tracking data for humpback and minke whales. For humpback whales, we used a circumpolar dataset of tracks (Reisinger et al., 2021) totalling 367 tracks and 214,865 locations. Individuals were tagged on Antarctic foraging grounds as well as lower latitude breeding grounds (Reisinger et al., 2021). For minke whales, we used published tracks from tags deployed off the Western Antarctic Peninsula (Friedlaender et al., 2021), as well as unpublished tracks from tags deployed in the Weddell Sea and off the Western Antarctic Peninsula. Together these totalled 20 tracks and 10,286 locations. The tags were all Argos-linked satellite tags in various configurations (see Curtice et al. (2015); Weinstein et al. (2017); Weinstein & Friedlaender (2017) and Friedlaender et al. (2021) for specific details). For both species, we restricted the dataset to tracks from 1 January 2012 onwards, with locations within CCAMLR statistical Subarea 48.1: 58 humpback whale tracks (2012-2018) and 19 minke whale tracks (2013-2017) (Supplementary Table S1).
Argos-linked tags provide geographic location estimates at irregular intervals due to the dive behaviour of whales and the availability of overhead satellites. Argos location estimates have some geographic uncertainty, categorised by system Argos into four classes with error radiuses from <250 m (class 3) to >1,500 m (class 0) (CLS, 2016). To estimate locations at regular intervals, while accounting for location uncertainty, we fitted state-space models to the tracking data. Specifically, we chose a behaviour-switching state-space model (Jonsen et al., 2005), which also estimates a behavioural parameter (b) for each location. The behavioural parameter b is estimated using the turning angles and persistence in speed and direction from the fitted tracks (Jonsen et al., 2007). Low b values are associated with faster, straighter movements indicative of ‘transit’ behaviour, while high b values indicate slower, more tortuous movements, assumed to be associated with ‘Area-Restricted Search’ behaviour (Jonsen et al., 2007). The rationale is that, when prey is heterogeneously distributed, consumers initially search for prey using faster, more linear movements; when they encounter prey they then slow down and turn more often to remain in the prey patch (e.g., Kareiva & Odell, 1987; Benhamou, 1992; Fauchald et al., 2000; Fauchald and Tveraa, 2003). Thus, locations with high b values – hereafter ‘restricted’ behavioural state – are assumed to represent areas where whales have encountered prey.
Before fitting the state-space models, we split tracks with temporal gaps greater than three days into track segments and treated these separately to avoid excessive interpolation. We also filtered the data using a speed filter (retaining positions with apparent speeds less than 5 m s-1). Behavioural-switching state space models were fitted using the bsam R package (Jonsen et al., 2005; Jonsen, 2016). We estimated locations 3-hourly. Locations with b values 1.0 ≤ b ≥ 1.4 were classified as transit, 1.6 ≤ b ≥ 2.0 as restricted, and 1.4 < b > 1.6 as uncertain (a slightly relaxed threshold cf.Jonsen et al., 2007).
Since the satellite tracking data depict only the behaviour of the tracked individuals, we fitted spatial random forest models (Hengl et al., 2018) to predict the behaviour of whales over the entire study area in each month where there was sufficient tracking data. These models relate the behavioural state in the tracks to a set of environmental covariates as well as a set of covariates capturing the spatial relationships among the tracking locations. Hengl et al. (2018) show that spatial random forests perform at least as well as state-of-the-art Kriging methods, but with greater flexibility.
Following Hengl et al. (2018), our spatial random forest is represented as:
The response variable Y at point s is the behavioural state b, either ‘transit’ or ‘restricted’; Xg are predictors/covariates accounting for geographic proximity among points, in this case the distance (km) from the given point s to all other points, calculated using the gdistance R package (Van Etten, 2017), Xe are environmental predictors/covariates at the given points, in this case: ocean depth, distance to shelf, sea surface temperature (mean and coefficient of variation), sea ice concentration (mean and coefficient of variation) and distance to the sea ice edge (mean and coefficient of variation) (Table 1). These are among the covariates that have previously been linked to the occurrence of minke (Friedlaender et al., 2006; Ainley et al., 2012; Friedlaender et al., 2014; Williams et al., 2014; Ainley et al., 2017; Herr et al., 2019; Ainley et al., 2020; Friedlaender et al., 2021) and humpback (Andrews-Goff et al., 2018; Bestley et al., 2019; Riekkola et al., 2019; Friedlaender et al., 2021; Reisinger et al., 2021) whales around Antarctica. The primary aim of our modelling was spatial prediction, and in addition to the spatial covariates we therefore included only some environmental covariates from a variety of possible ones (e.g., Reisinger et al., 2021). For dynamic covariates (sea surface temperature, sea ice concentration, distance to sea ice edge), monthly mean and coefficient of variation were calculated using daily data from the respective months over the period December 2015 - November 2020. Additionally, we included one- and two-month lags of these covariates, since lagged sea ice covariates have been linked to humpback whale movements previously (Andrews-Goff et al., 2018; Riekkola et al., 2019). Environmental data were extracted from a data collection maintained by the Australian Antarctic Division, using the raadtools (Sumner, 2021) and raster (Hijmans, 2020) R packages. The latter package was used for most raster manipulations. Random forests were fitted using the ranger package (Wright & Ziegler, 2017). The number of trees was set to 500 and prediction error was assessed with the Brier score. Covariate importance was assessed with the Gini index. We assessed the temporal trend in predictions by calculating Kendall’s tau for each grid cell over months, using the spatialEco package (Evans & Murphy, 2021).
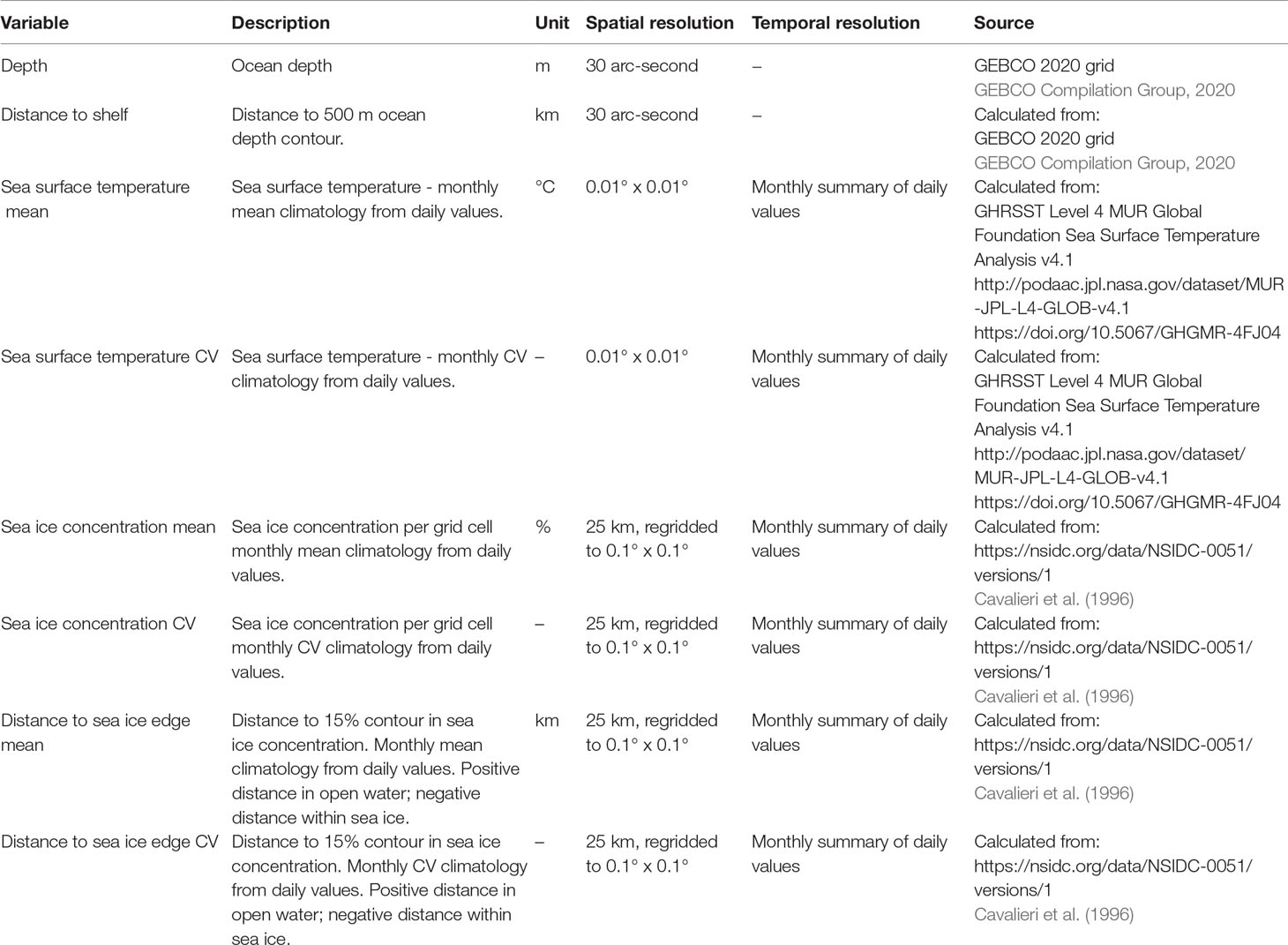
Table 1 Environmental covariates used as predictors of whale behavioural state in spatial random forest models.
Krill Fishing
We compiled data on Antarctic krill fishing in statistical Subarea 48.1 from two sources: CCAMLR fine-scale catch and effort data for trawl fisheries (“C1 data”) obtained from (https://www.ccamlr.org/en/node/74767) and Global Fishing Watch global fishing effort data version 2.0 (https://globalfishingwatch.org/data-download/datasets/public-fishing-effort) (Kroodsma et al., 2018). The C1 data are reported to CCAMLR on a haul-by-haul basis. We used data from December 2015 - May 2020 (2016-2020 fishing seasons), summing the krill catch (tonnes) per haul onto an aggregated 0.1° x 0.1° (approximately 11.1 km x 5.6 km at 60°S) spatial grid by month, using the haul set end locations. The Global Fishing Watch effort dataset is based on AIS-detections that are identified as fishing using a suite of algorithms, including a neural network classifier; these detections are binned onto a spatial grid and effort is calculated per grid as the time between the current and previous position (Kroodsma et al., 2018). We filtered these effort data for the same period as the catch data, summing fishing effort (hours) on the same spatial grid. We calculated temporal trends in krill fishing catch and effort as for the whale behaviour predictions.
Overlap Between Important Whale Areas and Fishing
We calculated an overlap index between whales and fishing by multiplying the predicted probabilities for whales to be in a restricted behavioural state in a given grid cell, by the monthly krill catch.
Results
Whale Movement Behaviour
After limiting the 367 humpback whale and 20 minke whale tracks to our study area, splitting tracks with time gaps > 3 days into track segments, and fitting behaviour-switching state-space models to regularise the locations in time accounting for the errors inherent to satellite tracking data, we retained 52 humpback whale track segments totalling 18,236 locations (Figure 2A) and 25 minke whale track segments totalling 4,188 locations (Figure 2B). The distribution of b values for each species is shown in Supplementary Figure S1.
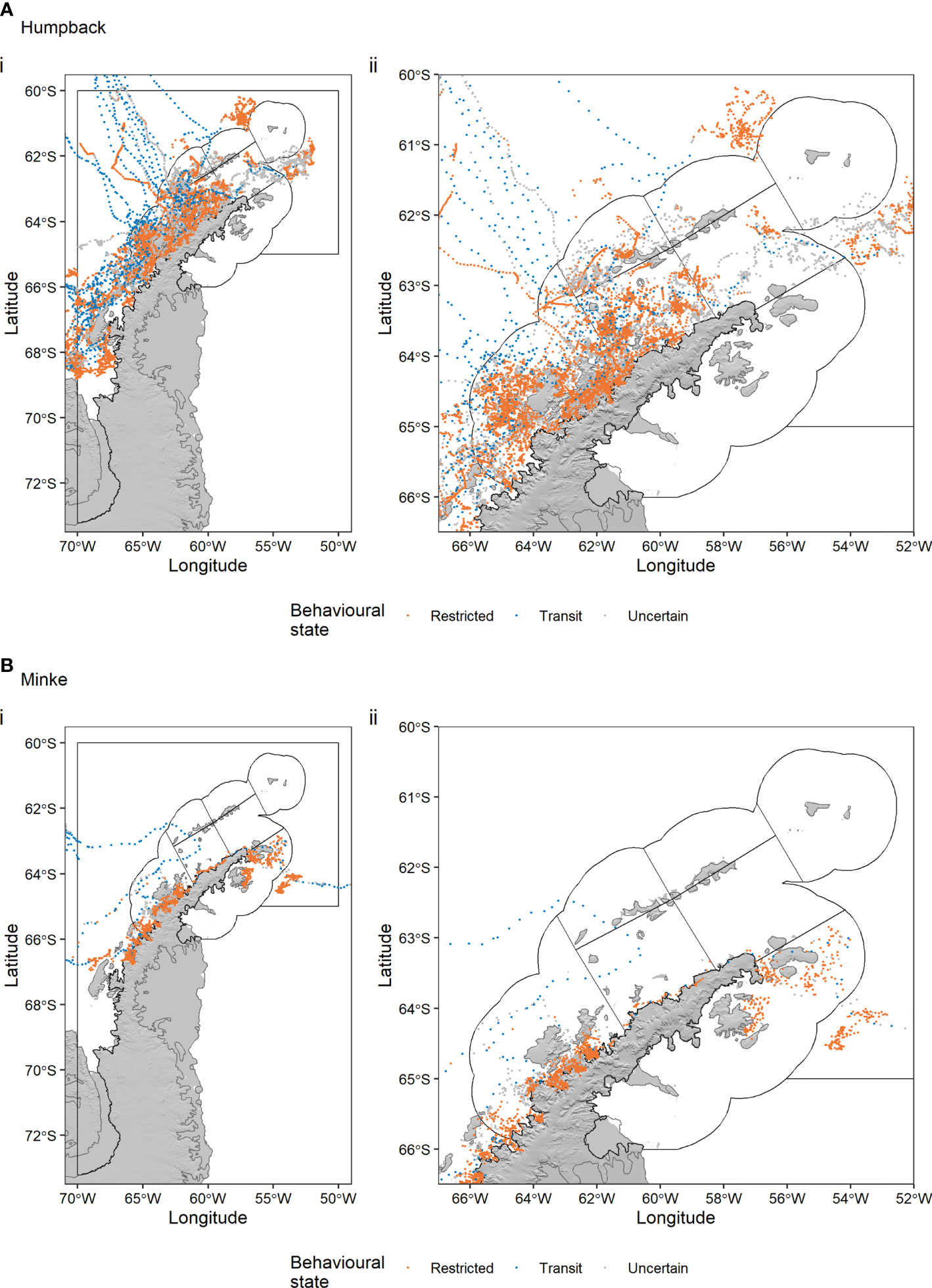
Figure 2 Humpback whale (A) and minke whale movement (B) tracks off the Antarctic Peninsula. A behaviour switching state-space model was used to regularise the locations in time (6-hour timestep), to account for errors associated with satellite tracking data, and estimate the behavioural state of whales. Black polygons indicate the seven CCAMLR Small Scale Management Units (SSMUs) within Subarea 48.1, which is delineated by the outer black polygon.
Movements of humpback whales were more extensive than those of minke whales, with humpback locations distributed throughout Subarea 48.1 (Figure 2A). Minke whale locations, in contrast, were mainly close to the Antarctic Peninsula coast, often in bays (Figure 2B). Humpback whales typically switched from transit to restricted behaviour upon reaching the South Shetland Islands or Bransfield and Gerlache Straits, where there were high concentrations of restricted behaviour locations (Figure 2A). Most whale locations were within the seven CCAMLR SSMUs, although both humpbacks and minkes recorded locations further south along the Peninsula.
Prediction error (Brier score, which can range from 0 [all cases correctly predicted] to 1 [no cases correctly predicted] of the random forest models ranged (best-worst) from 0.02-0.06 for humpback whales and from 0.03-0.08 for minke whales, indicating very low error when predicting the datasets used for model fitting.
The trend in random forest predictions over the season was very different for humpback and minke whales. For humpback whales (Figure 3A) there is an increasing trend in the Bransfield Strait West, Antarctic Peninsula West and Antarctic Peninsula Pelagic SSMUs over the season, with a decreasing trend elsewhere. For minke whales (Figure 3B) there is a decrease in the Bransfield Strait East SSMUs, strong decrease in the Antarctic Peninsula West SSMU nearshore and Antarctic Peninsula East SSMU (around James Ross Island), with a strong increase in the pelagic SSMU, off Adelaide Island.
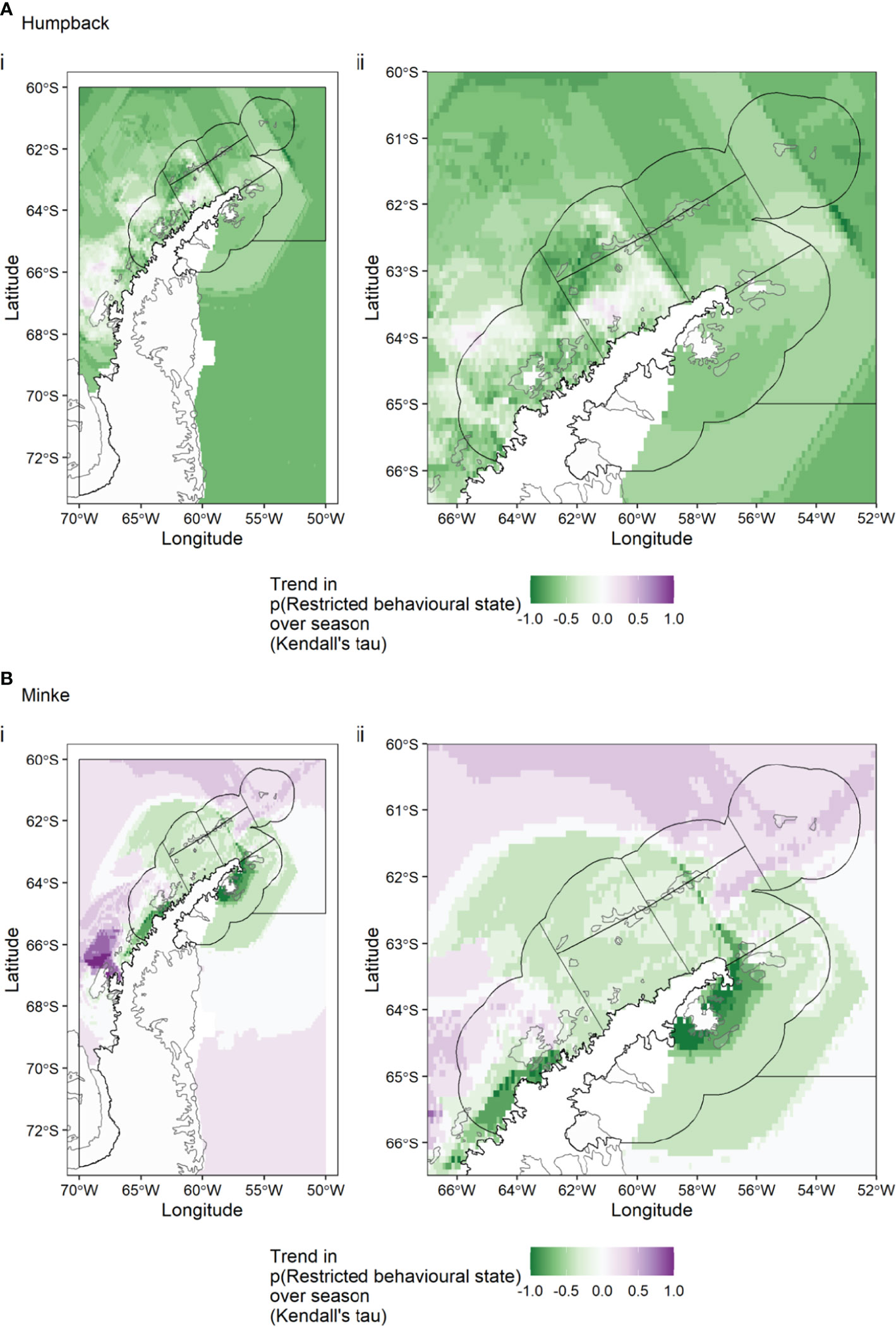
Figure 3 For humpback whales (A) and minke whales (B), the trend in behavioural state predictions over the season. A negative trend (green colours) means that, in a given grid cell, whales were decreasingly likely to be in a restricted behavioural state as the season progressed, while a positive trend indicates the opposite.
Among the environmental covariates, 1- and 2-month lagged mean sea surface temperature followed by 1-month lagged mean sea ice concentration had the highest to third-highest mean variable importance for humpback whales (Figure 4). For minke whales, 1-month lagged sea surface temperature mean, followed by 2-month lagged mean sea ice concentration had the highest mean importance (Figure 4).
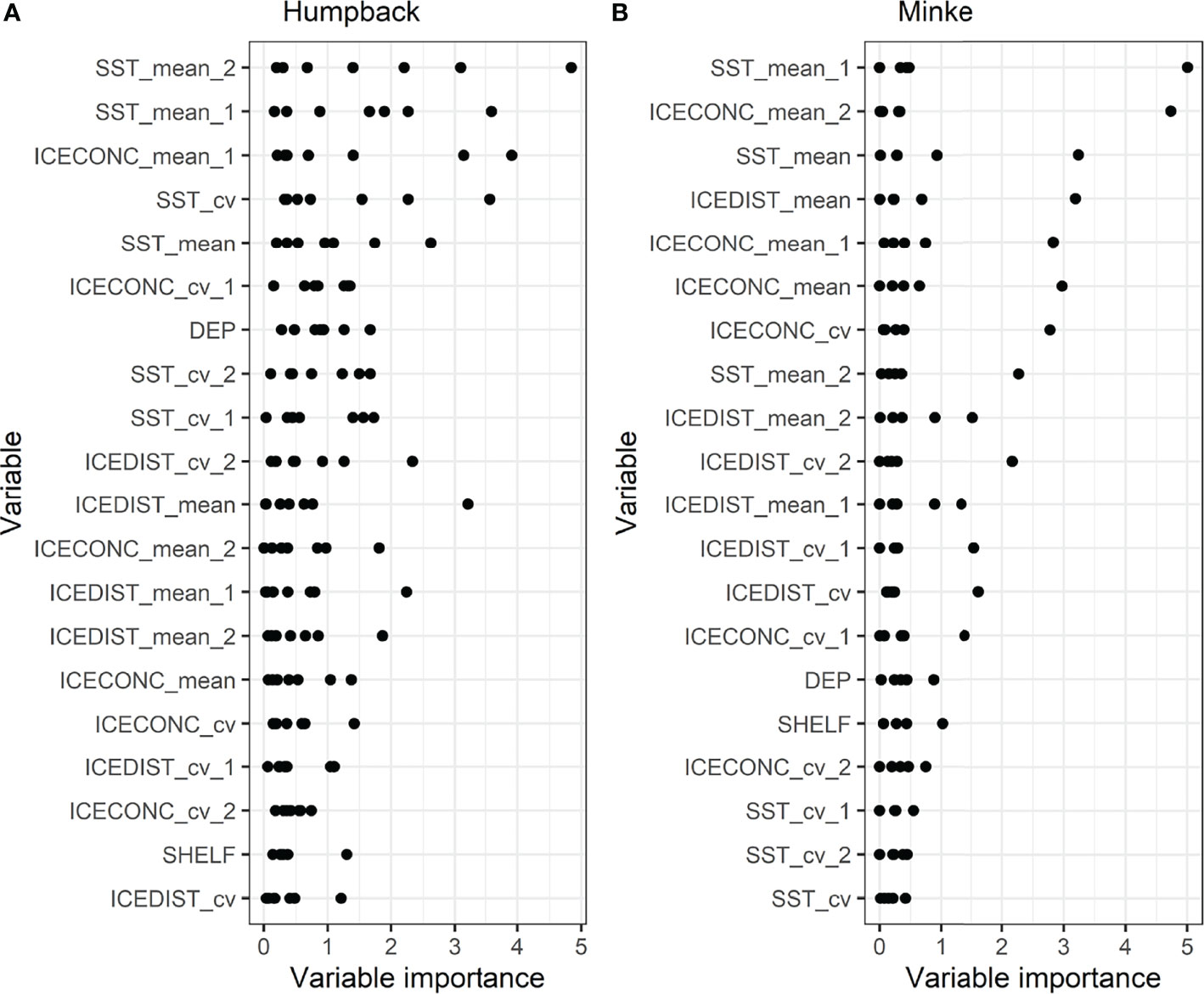
Figure 4 Importance of environmental covariates in random forest models predicting the behavioural state of (A) humpback and (B) minke whales. Covariates are ordered from lowest (bottom) to highest (top) mean importance, with each point representing a monthly random forest model (seven models for humpback whales and five models for minke whales). SST_mean, mean sea surface temperature; SST_cv, coefficient of variation of sea surface temperature; ICECONC_cv, coefficient of variation of sea ice concentration; ICEDIST_mean, mean of distance to sea ice edge (15% sea ice concentration contour); ICECONC_mean, mean of sea ice concentration; DEP - ocean depth; SHELF, distance to shelf (500 m bathymetry contour); ICEDIST_cv, coefficient of variation of distance to sea ice edge (15% sea ice concentration contour). Suffixes _1 and _2 indicate 1- and 2-month time lags.
Fishing
Fishing effort and catch took place in the Drake Passage near the South Shetland Islands (SSMUs ADPW and ADPE), near Elephant Island (SSMU APEI) in the Bransfield Strait (SSMUs APBSW and APBSW) and in the Gerlache Strait (SSMU APW) (Figure 5). The greatest effort (up to 34 hours in a grid cell) and greatest catch (up to 12,908 tonnes in a grid cell) was located in a cluster of grid cells around the shelf edge of the Antarctic Peninsula within the Bransfield Strait (near Lafond Trough), straddling SSMUs APBSW and APBSE with a size in the region of 30 x 60 km.
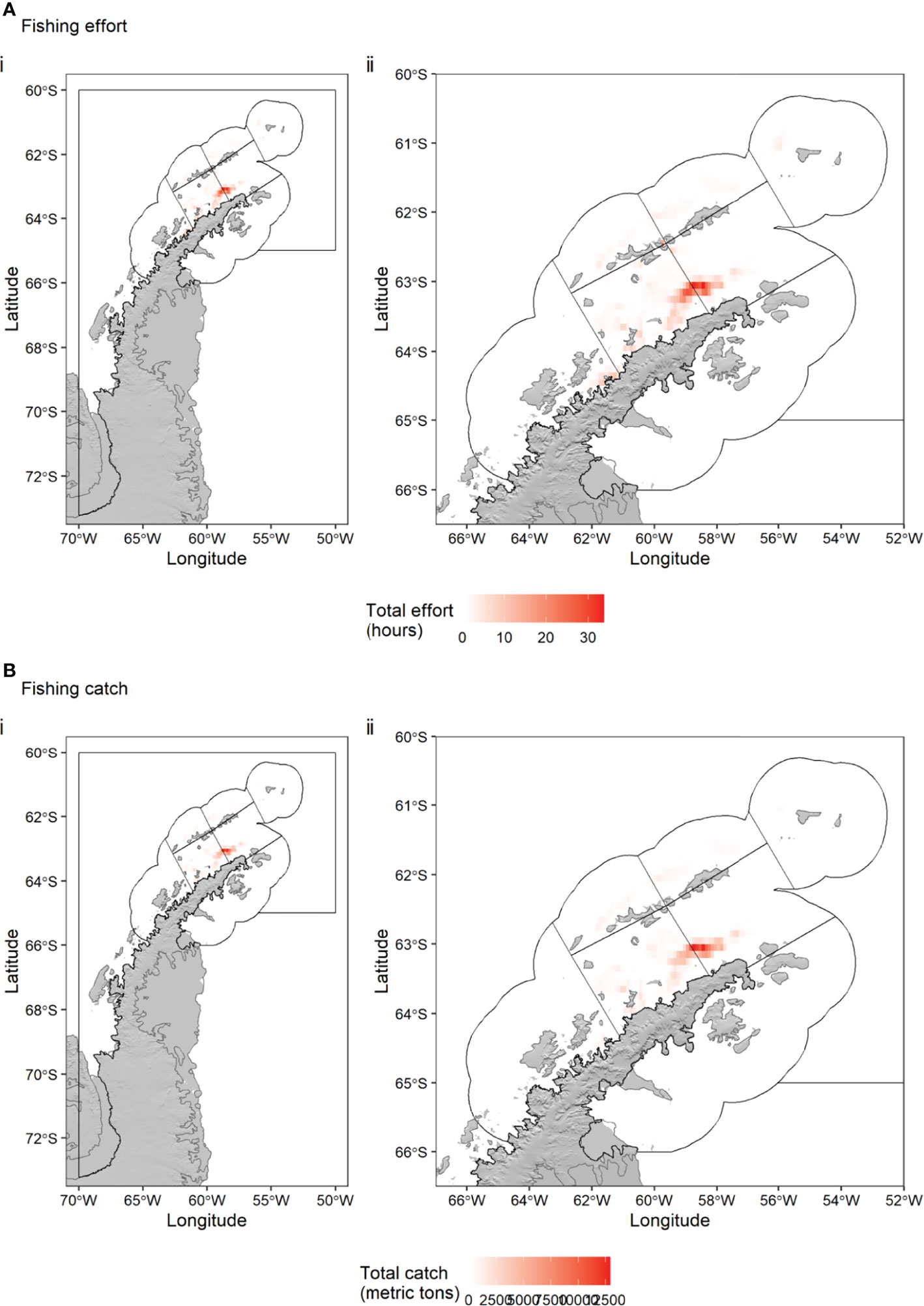
Figure 5 Total Antarctic krill fishing effort (hours) (A) and catch (tonnes) (B) per 0.1° x 0.1° grid cell in statistical subarea 48.1 over the period December 2015 – May 2020. Black polygons indicate the seven Small Scale Management Units (SSMUs) within the Subarea, named in Figure 1. Catch data: CCAMLR. Effort data: Global Fishing Watch.
Each Antarctic krill fishing season lasts from 1 December to 30 November, but Subarea 48.1 is usually closed early, because the local catch limit is reached, usually in May, June or July of a given year. We calculated the spatial trend (Kendall’s tau) in krill catch (Figure 5) and fishing effort (Figure 6) over the season (for a given calendar month, summing the data over all years). Figures 5, 6 show the clear seasonal progression from areas in the Drake Passage off Elephant Island (APEI SSMU) and King George Island (APDPE SSMU) to more south-westerly areas in the Drake Passage (APDPW SSMU) and areas in the Bransfield Strait (APBSW and APBSE SSMUs). Monthly, interannual variance in fishing effort was highest in the Bransfield Strait in April and May (Supplementary Figure S2).
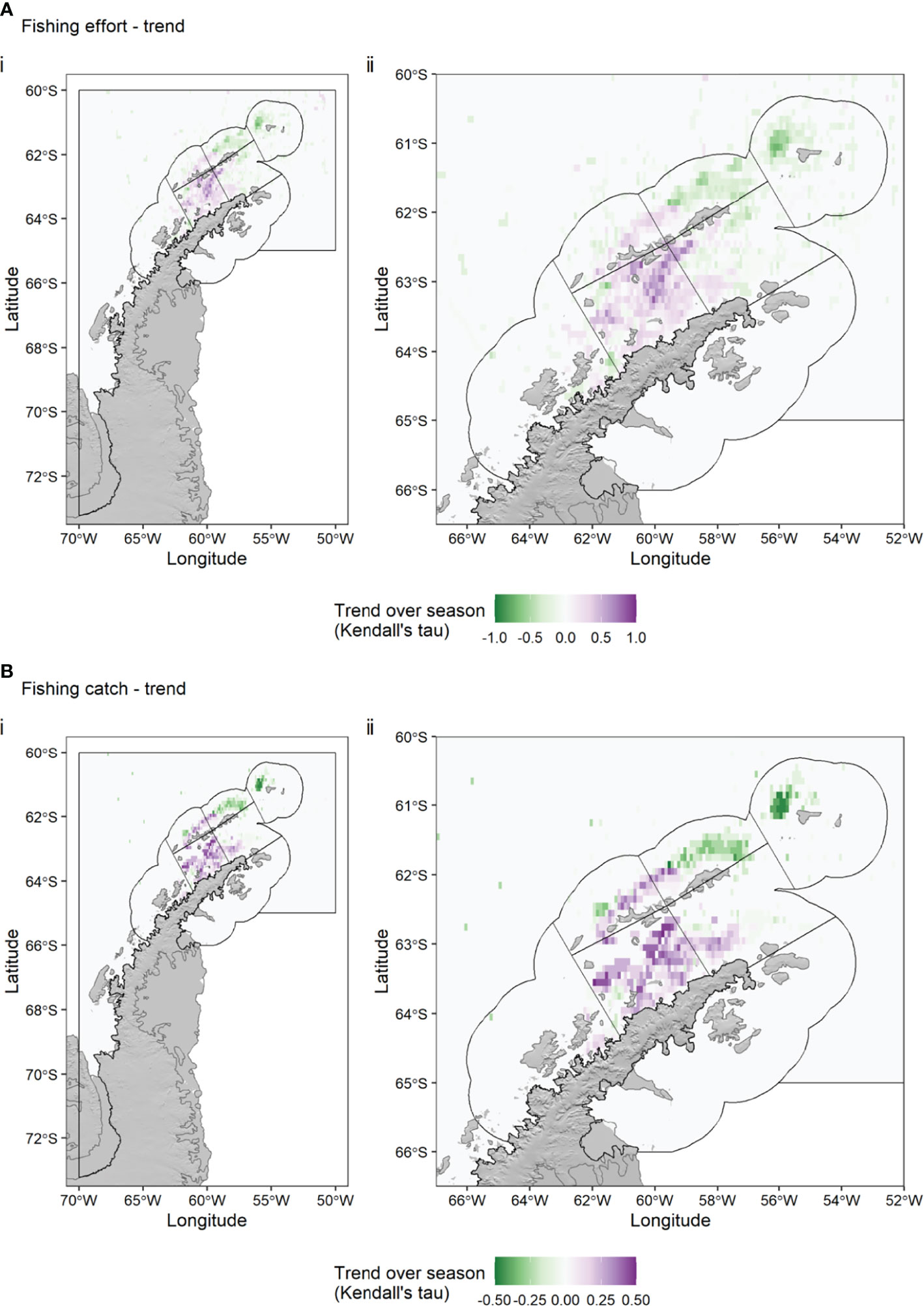
Figure 6 Trend (Kendall’s tau) in Antarctic krill fishing effort (A) and catch (B) over all fishing seasons (December – July), 2015-2020, depicting the average movement of the fishing effort (top) and catch (bottom) within seasons. Green colours indicate a decrease in effort (top) or catch (bottom) in a given 0.1° x 0.1° grid cell over the season, while purple colours indicate an increase. Black polygons indicate the seven Small Scale Management Units (SSMUs) within the Subarea. Effort data: Global Fishing Watch. Catch data: CCAMLR.
Overlap
Overlap index values reveal a clear seasonal progression in potential interactions between predicted whale foraging and krill fisheries. For humpback whales, overlap early in the season, in January, is located in the Elephant Island (APEI) and Drake Passage (APDPW and APDPE) SSMUs (Figures 7A, 8), while from February, overlap for humpback whales develops in the Bransfield Strait. For minke whales, overlap is highest in the Bransfield Strait (APBSE and APBSW), firstly, and off the South Shetland Islands (APDPE and APDWE), secondly (Figures 7B,8). For both humpback (Figure 7A) and minke (Figure 7B) whales, the highest overlap occurs in April and May, concentrated in the Bransfield Strait (Figure 8), driven by high krill catch in this area (see Figure 5). An approximate comparative phenology of whales – using the tracking data and International Whaling Commission whaling catch dataset (Allison, 2016) south of 60° S – and fisheries – using the effort data – is shown in Figure 9.
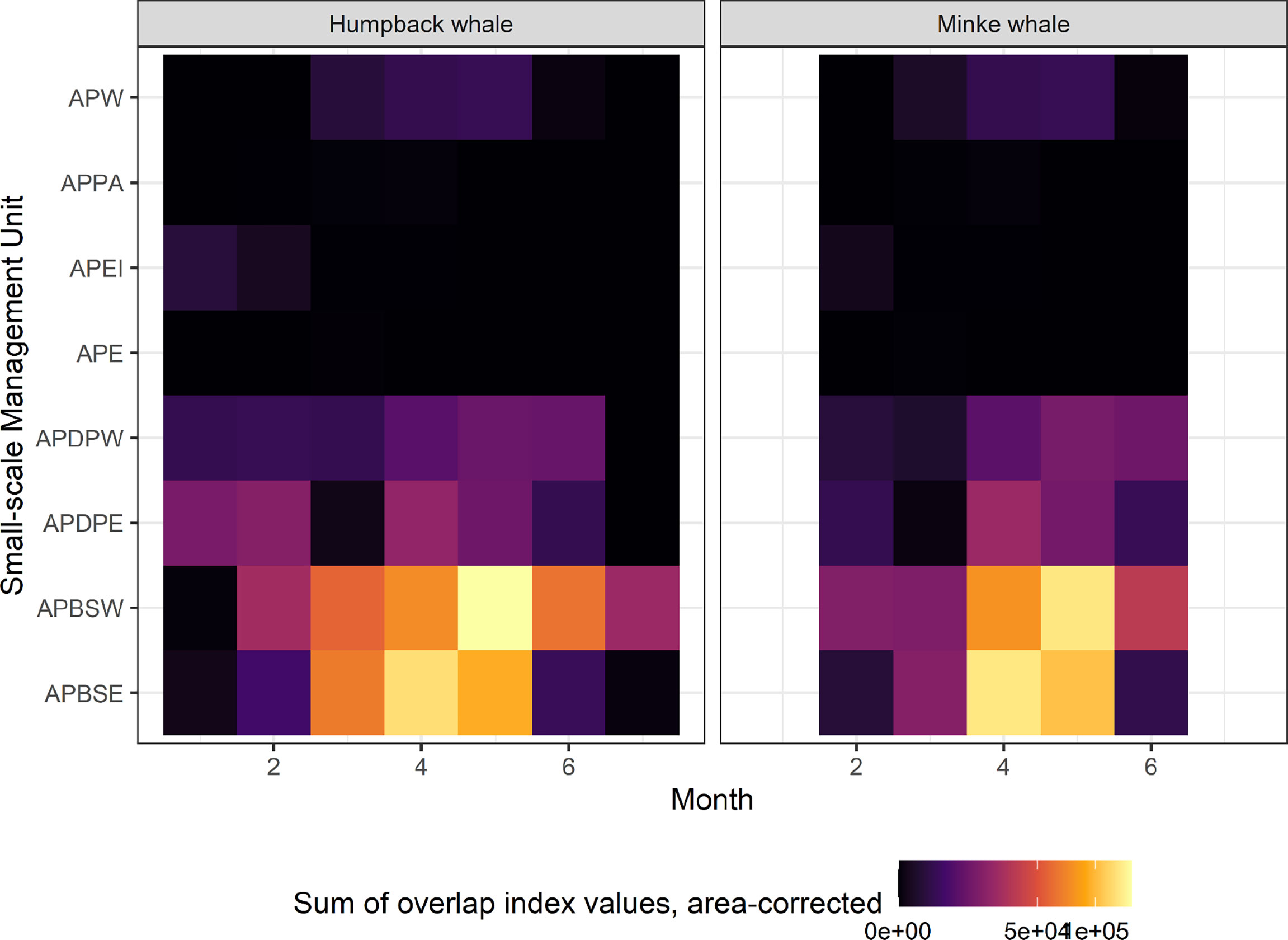
Figure 8 Heatmap of the summed fishing-whale overlap index values (mapped in Figure 7) in each Small-Scale Management Unit (vertical axes), by month (horizontal axes). The summed values were divided by the number of grid cells in each Small-Scale Management Unit, to correct for size differences. APW, Antarctic Peninsula West; APPA, Antarctic Peninsula Pelagic Area; APEI, Antarctic Peninsula Elephant Island, APE, Antarctic Peninsula East; APDPW, Antarctic Peninsula Drake Passage West; APDPE, Antarctic Peninsula Drake Passage East; APBSW, Antarctic Peninsula Bransfield Strait West; APBSE, Antarctic Peninsula Bransfield Strait East.
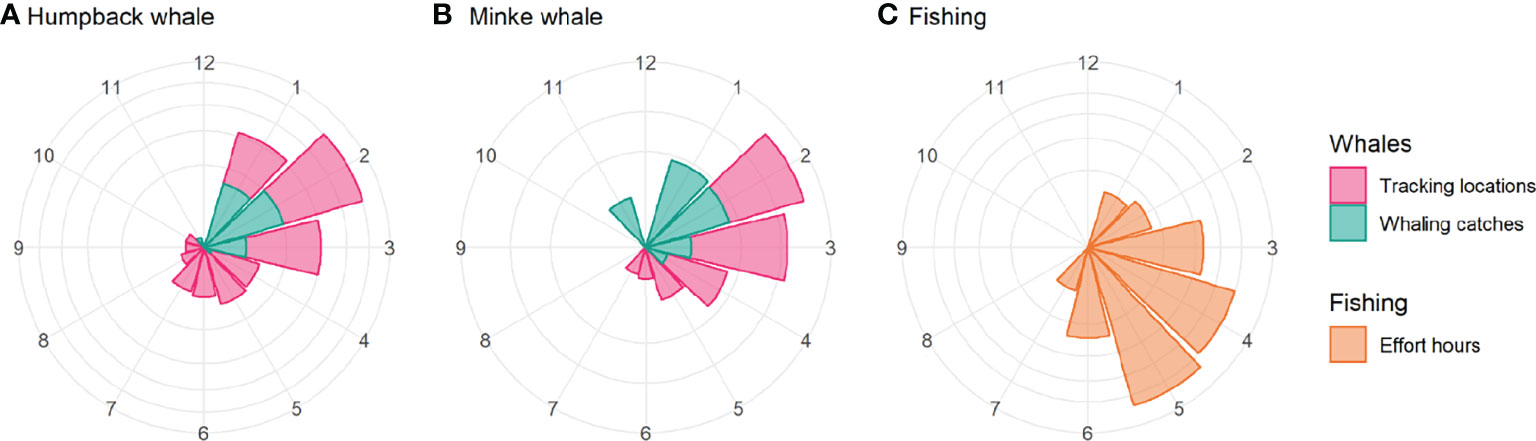
Figure 9 Figure 9 |Approximate phenology of humpback whales (A), minke whales (B) and fisheries (C) in the Western Antarctic Peninsula by month. The figure shows an approximate comparative phenology for whales – using the monthly number of satellite tracking location estimates in the study area (magenta) and the monthly number of whaling catches south of 60° S from 1900-2015 (Allison, 2016) (cyan) – and fisheries – using the monthly hours of effort (orange). Values were normalised for presentation, and the y-axis is square root-transformed. The whale phenology will be biased towards tagging activity during the summer and autumn, and whaling catches will be biased against catches in winter and spring. Minke whales (Antarctic and common) were only caught in large numbers from around 1960 onwards, around which time humpback whale catches declined (Rocha et al., 2015).
Discussion
Using humpback whale and minke whale tracking data, we fitted spatial random forest models to predict the monthly distribution of whale behavioural state over the summer foraging period in the Western Antarctic Peninsula region. For humpback whales, we predicted a shift towards a concentrated foraging area in the Bransfield Strait. For minke whales, there was a more subtle trend suggesting diffusion from their early season foraging area in the Gerlache Strait. We used fine-scale data on krill fishing effort and catch to examine the monthly spatial distribution of the krill fishery in the same region. These data show that fishing effort and catch is highly concentrated in relatively small areas, as shown by Santa Cruz et al. (2018) (see also Weinstein et al., 2017 and Krüger, 2019). Krill fishing is particularly concentrated in two SSMUs in the Bransfield Strait: Bransfield Strait West (APBSW) and Bransfield Strait East (APBSW) (see also Weinstein et al., 2017; Santa Cruz et al., 2018).
Several studies (e.g., Trivelpiece et al., 2011; Forcada et al., 2012; Trathan & Hill, 2016; Warwick-Evans et al., 2018; Watters et al., 2020; Bamford et al., 2021; Krüger et al., 2021) highlight overlap between krill consumers and krill fisheries as a serious management concern. However, most work has so far focussed on penguins and seals while baleen whales, despite being major krill consumers (Savoca et al., 2021), have received comparatively little attention (cf.Weinstein et al., 2017; Johannessen et al., 2022; Trathan et al., 2022). Indeed, baleen whales are not explicitly included in CCAMLR’s ecosystem approach to fishery management or in the CCAMLR Ecosystem Monitoring Program that monitors other krill consumers (Agnew, 1997). Indeed, by the time that the CAMLR Convention was negotiated, populations of baleen whales had already been exploited to such levels that management concerns principally focused upon land-based krill predators. However, negotiation of the CAMLR Convention did allow for the restoration of previously depleted populations, which clearly must now include baleen whales.
Our results indicate the likely overlap between humpback and minke whales and the krill fishery at fine spatial scale on the order of tens of km. Further, the temporal changes in humpback whale foraging distribution and krill fishing distribution are likely to result in intensified potential competition as both krill consumers and krill fisheries track the movement of krill in coastal regions as the summer progresses. This underlines the importance of including information on baleen whales in managing the krill fishery. Moreover, our results further emphasise that management of the krill fishery should move towards understanding actual impacts on krill consumers at fine temporal and spatial scales, as well as being adaptive by, for example, using spatial models similar to those we present to predict overlap between krill consumers and the krill fishery. This is now increasingly urgent as the ecosystem changes with changing predator populations and rapid environmental change in this region, but also in the context of an expanding krill fishing (Trathan et al., 2022).
Having recognized the increasing spatial concentration of krill fishing and the typical spatial scale of krill-predator interactions, CCAMLR designated SSMUs ranging in size from 16,000 km2 - 440,000 km2 (Figure 1). However, krill catches are still managed by Subarea, for Subarea 48.1 an area of 658,739 km2. Even if catch were to be managed by SSMU, our analyses show that potential interactions are concentrated in hotspots in only a few SSMUs. Weinstein et al. (2017) showed that humpback whales used the Antarctic Peninsula West (APW), Bransfield Strait West (APBSW), and Drake (APDP) Passage West SSMUs more often than expected, spending more time in a restricted behavioural state in these SSMUs. Similarly, density modelling of humpback whale sightings data likewise identifies the Bransfield Strait and northern Gerlache Strait as areas of high humpback whale abundance, at least during December (Johannessen et al., 2022). In the last decade, krill fishing has shifted spatially into the Bransfield Strait (Santa Cruz et al., 2018), representing the primary krill fishing hotspot in our analysis (Figure 5). Thus, these areas represent hotspots of likely high overlap between humpback whales and the krill fishery (Figures 7, 8). Minke whales had a more restricted distribution, with most of their restricted-behaviour locations in the nearshore waters of the Gerlache Strait (Danco Coast) and Grenadier Channel (Graham Coast and Loubet Coast). There was some fishing effort in the northern Gerlache Strait, but little further south, and consequently overlap between predicted minke whale foraging areas and the krill fishery was much lower than for humpback whales. A caveat is that fewer tracking data for whales in some months – mainly April but also May for minke whales, and March for humpback whales – resulted in less informative models with somewhat uniform predictions in those months. The trend in predictions across months is likely to be robust despite this, but, for minke whales, extending the seasonal data window in this region should be a priority in light of projected environmental changes in the Western Antarctic Peninsula (see below). Further, tagging may have been biased towards smaller individuals more likely to feed in protected bays, and our study did not include information on Antarctic blue (Balaenoptera musculus intermedia) and fin (Balaenoptera physalus) whales, two important krill consumers likely to overlap with the krill fishery in more open waters (Santora et al., 2010; Santora et al., 2014; Herr et al., 2016). Lastly, behavioural differences between humpback and minke whales could influence the definition of ‘restricted’ and ‘transit’ locations estimated from the state-space models, with minke whales likely switching behavioural states in a manner that is more difficult to detect with a state-space switching model. Further, directed work, where satellite tracking is augmented with accelerometery to detect foraging more directly, is required to investigate this fully.
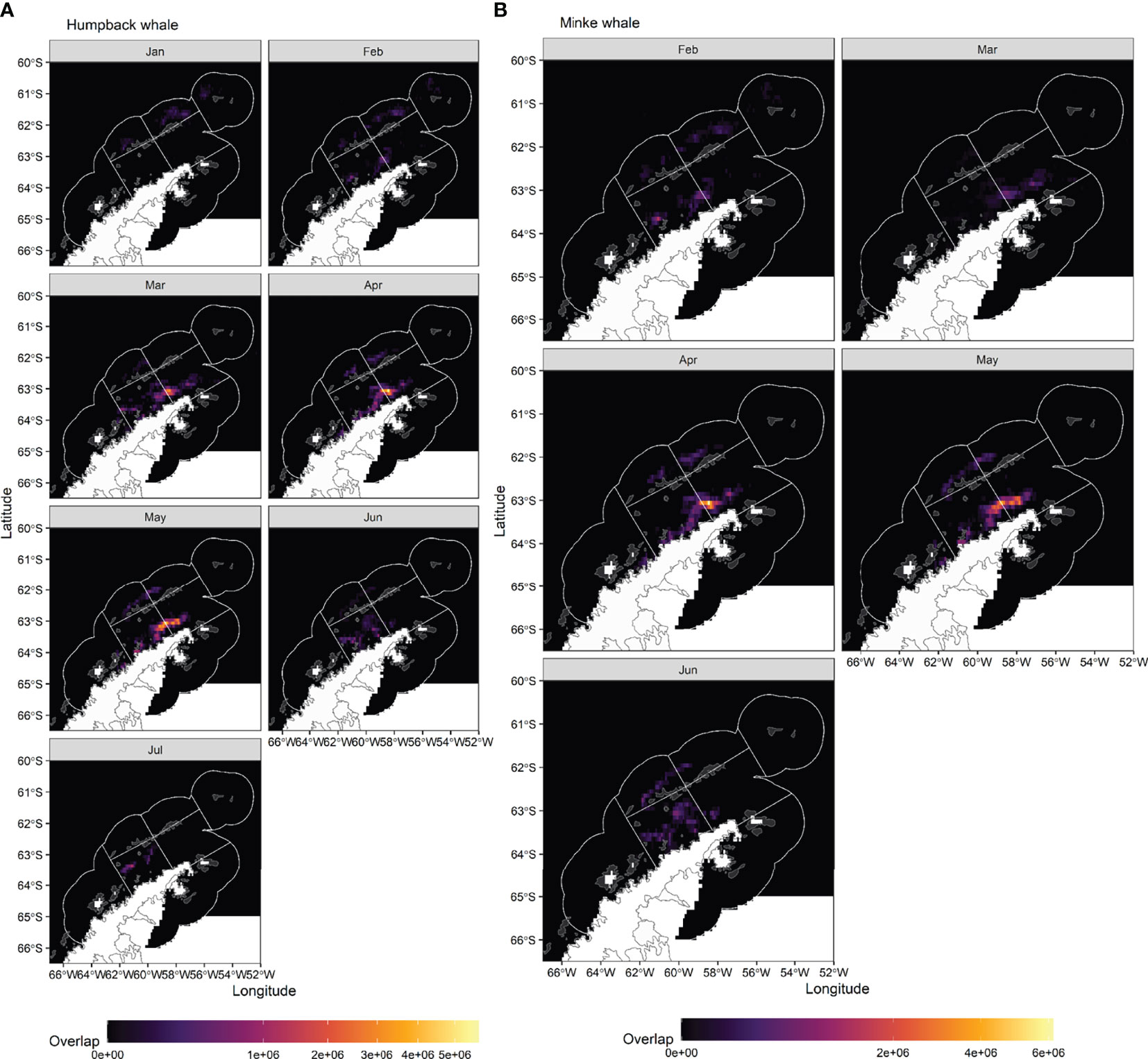
Figure 7 Monthly overlap between (A) humpback whales and krill fishing and (B) minke whales and krill fishing, estimated as predicted probability of whales being in a restricted behavioural state in a given grid cell in a given month, multiplied by monthly fishing catch.
Predator foraging behaviour is highly variable, including seasonally, depending upon animal status (e.g., for different phases of the penguin breeding season, Warwick-Evans et al., 2018). As such, the scale and intensity of habitat used by a predator can change. This has important consequences for management of the krill fishery (e.g., see Trathan et al., 2022, for a broader discussion of scale in the management of the krill fishery). Briefly, the dynamic mosaic of predator demand across time and space and across species presents a number of challenges to CCAMLR, particularly as habitat use preferences are unknown for many species, or are only partially known for others. For most species, there is a lack of understanding about how predators use their habitat in winter. How predators interact with the available krill in an area now requires deeper understanding, particularly where carryover effects may be important (Trathan et al., 2021).
Our analysis shows that as the season progresses, fishing shifts south and inshore, similar to the predicted seasonal movement of humpback whale foraging areas. Tracking data from five humpback whales analysed by Curtice et al. (2015) showed that whales used smaller more concentrated areas and moved nearer to the coast over the foraging season. Since both fishing vessels and humpback whales are targeting krill, a similar progression of their hotspots should be expected if krill distribution shifts through the year, and especially if krill behaviour or ecological drivers of krill habitat use have a seasonal component. Indeed, Atkinson et al. (2008) note that krill density peaks in the middle of summer (January) and declines thereafter, reflecting the pulse of recruitment from larvae during spring–summer and subsequent mortality. However, adult krill are also thought to migrate from shelf waters to deeper oceanic waters at the edge of the shelf to spawn in summer, returning at the end of the summer to winter in shelf or coastal waters (Siegel, 1988; Trathan et al., 1993; Siegel, 2005). Apparently, krill overwinter in coastal waters, regardless of sea ice cover (Reiss et al., 2017). In this context, Lascara et al. (1999) have also reported that at the Western Antarctic Peninsula, there is a strong decrease in krill biomass, with altered aggregation characteristics between spring (November) and winter (August-September). As such, in summer (January-February), there are widespread, dense aggregations of smaller size, which are located in the upper water column. Whereas, in autumn (March-May), there are larger, less dense aggregations that are located deeper in the water column. Such a change in krill aggregation state could, at least partially, be associated with predator foraging behaviour (Tarling and Fielding, 2016), particularly if krill show differential responses to different feeding behaviours, by for example, baleen whales or penguins, and these predators remain seasonally in the region for different periods of time. Krill behaviour will also be affected by complex dispersal and retention of krill due to local currents (Trathan et al., 2022). Krill may concentrate near to local bathymetric features, entrained by local oceanographic flows (Capella et al., 1992; Piñones et al., 2013b). In late autumn/winter, massive, extremely dense aggregations of krill have been documented in the nearshore bays on the western side of the Antarctic Peninsula (Nowacek et al., 2011) coincident with where the krill fishery is known to operate at these times (Weinstein and Friedlaender, 2017). Baleen whale concentrations (Johnston et al., 2012) mirror these supper aggregations of krill and whales consistently distribute in these places and forage in a manner that optimises energy gain (Friedlaender et al., 2013; Friedlaender et al., 2016; Tyson et al., 2016)
Johannessen et al. (2022 see also Warwick-Evans et al., 2022) estimate peak potential competition (calculated as humpback whales’ per capita consumption multiplied by their abundance through the season, and krill catch through the season) in April, which corresponds with the highest values in our monthly spatial overlap index. Based on the assumption that whales spend 120 days in their Southern Ocean foraging areas (Lockyer, 1981), Johannessen et al. (2022) calculated very limited humpback whale-fishery competition by May, but tracking data in this study and Weinstein et al. (2017) shows that some individuals remain in the region as late as July. Further, minke whales may remain in the Western Antarctic Peninsula region much longer, and can overwinter in the area (Dominello and Širović, 2016). Thus, as vessels increasingly fish the region later into the year, it is unlikely that any overlap with baleen whales will be limited to just the summer. This will likely be exacerbated by the increasingly ice-free environment (e.g., Turner et al., 2016) that is likely to result in a longer season for fisheries and whales in future. Indeed, Trathan et al. (2022) show that peak fishing effort is now in May, highlighting the need for more intensive studies on predator-prey interactions at this time.
Minke whales occur within the foraging habitat of humpback whales (this study, Friedlaender et al., 2021). The seasonal movement of humpback whales closer inshore (this study, Curtice et al., 2015; Weinstein and Friedlaender, 2017) could thus increase potential competition between minke whales and humpback whales as the season progresses, congruent with increased fishing effort inshore.
Humpback whales, minke whales and krill fisheries are not the only consumers of krill in the study region, and as the humpback whale population that uses the Western Antarctic Peninsula region appears to be increasing (Pallin et al., 2018), it suggests that prey availability is not currently a limiting factor for baleen whales. However, changing populations of other krill consumers, suggest that species interactions should be considered as part of CCAMLR’s revised krill management approach, particularly given the ongoing expansion of the krill fishery. Further, as other baleen whale populations recover, competitive effects may increase, both amongst krill predators and with the krill fishery. Currently, catch levels in the study area remain low in real terms (~155,000 tonnes) compared with estimates of local krill biomass (~19.2 million tonnes in 2019; Krafft et al., 2021). However, the fishery regularly operates in favoured areas where krill biomass is predictable, but which are also areas used by krill predators (Trathan et al., 2022). Although Cox et al. (2018) argue that krill density was stable from 1976-2016, several studies have reported declines in krill density in the southwest Atlantic in the late 20th century (Atkinson et al., 2004; Atkinson et al., 2019; Hill et al., 2019), raising the possibility of increasing fishing of a declining resource. Future expansion of the krill fishery must therefore follow a precautionary approach to ensure krill predators are not disadvantaged. A key issue for managers will be ensuring replenishment of krill by ocean currents remains adequate for predator needs, and that retention and carry over of krill in certain areas is sufficient across time (Trathan et al., 2022). In the Western Antarctic Peninsula, many Adélie (Pygoscelis adeliae) and chinstrap (Pygoscelis antarcticus) penguin populations are decreasing (Cimino et al., 2016; Hinke et al., 2017b; Strycker et al., 2020). Understanding the drivers of these population changes, whether through increased competition with other krill predators, climate change or local fishery impacts is now required.
Recently, the international fishing fleet targeting Antarctic krill agreed to voluntarily avoid catches in the vicinity of large Pygoscelis penguin colonies situated in the Western Antarctic Peninsula region (e.g., Trathan et al., 2022; Godø & Trathan, 2022). This was based on the recognition that land-based predators are potentially most vulnerable to the competitive effects of fishing and any possible depletion of the resources predators need to provision both themselves and their offspring during the breeding season. The focus of the so-called ‘Voluntary Buffer Zones (VBZ)’ was penguin colonies, as these are the most numerous central-place foraging predators breeding on the Antarctic Peninsula. The VBZ are seasonal so only reduce competitive effects with the fishery during the penguin breeding season. Each VBZ extends from the penguin colony location to either 30 km or 40 km, depending upon the penguin species (see details in Trathan et al., 2022). The VBZ include the majority of the penguin foraging habitat during breeding for colonies within Subarea 48.1, including ~74.3% of chinstrap, ~97.5% of gentoo and ~91.4% of Adélie penguin colonies. Thus, approximately 74,160.8 km2 of ocean is protected: ~13,131.9 km2 within the Gerlache Strait; ~37,055.6 km2 around the South Shetland Islands; and ~23,973.3 km2 around the tip of the Antarctic Peninsula (Godø & Trathan, 2022). Inevitably, protection, though voluntary, also provides significant levels of protection for baleen whales, including both humpback and minke whales. Stricter protection would be provided by a proposed Marine Protected Area in the Western Antarctic Peninsula and South Scotia Arc (Delegations of Argentina and Chile, 2020). The proposed MPA includes protected zones encompassing areas in the Bransfield and Gerlache Straits and areas in the Bellingshausen Sea, important for krill, birds and marine mammals. The MPA would also include zones where commercial krill fishing is permitted (Delegations of Argentina and Chile, 2020). Still, there is at present no understanding about functional overlap between the fishery and krill predators, as opposed to simple spatial overlap. To determine levels of functional overlap, we need to better understand how predators (and the fishery) target krill.
As such, there is now a real need to examine the characteristics of krill patches targeted by the fishery, compared with patches targeted by natural krill consumers. Exploitation of large, dense patches of krill by the fishery may be similar to the patches required by large baleen whale species, or other predators. Minkes potentially can exploit less dense patches, but their habitat is restricted, and may become even more restricted as the polar regions continue to warm (Constable, 2022). Minkes may currently escape competition with fisheries (and humpbacks to some extent) due to their high affinity for sea ice and the capability to exploit less dense krill patches. However, decreasing sea ice means baleen whales (see discussion in Friedlaender et al., 2021) and fisheries (Krüger, 2019) are likely to experience increasing niche overlap. In contrast, Pickett et al. (2018) show that climate changes may not increase competition between gentoos and Adélies in the Western Antarctic Peninsula region, due to fine scale niche partitioning. This illustrates that increased potential competition is not a foregone outcome, given the fine-scale partitioning resulting from different foraging mechanics in humpback and minke whales and with other predators of krill. However, overall depletion of krill remains a concern despite any ability of consumers to partition resources at fine spatial scales in four dimensions, i.e., in space and time.
The primary aim of our modelling was spatial prediction, and we therefore used spatial random forests (Hengl et al., 2018), which incorporate distances among observations in the dataset. However, we included a limited number of environmental covariates to add some information on the physical environment and its correlation with whale behavioural state. In the humpback whale models, sea surface temperature variables were the most important environmental covariates. This likely reflects the transition of humpback whales from migratory (transit) to foraging (restricted) behaviour as they reach the South Shetland Islands and Peninsula. Sea surface temperature has been found to be an important covariate influencing the habitat selection of humpback whales in the Southern Ocean (e.g., Andrews-Goff et al., 2018; Bestley et al., 2019; Riekkola et al., 2019; Reisinger et al., 2021). Johannessen et al. (2022) observed a positive correlation between humpback whale presence and sea surface temperature in the Bransfield Strait region, and interpret this as a preference for the warm, nutrient-rich Circumpolar Deep Water that is delivered onto the continental shelf (Martinson & McKee, 2012; Wang et al., 2022), driving marine productivity (e.g., Ducklow et al., 2012).
In contrast, sea ice variables were more often among the most important environmental variables in the minke whale models, reflecting the species’ closer relationship with sea ice (Friedlaender et al., 2006; Ainley et al., 2012; Friedlaender et al., 2014; Williams et al., 2014; Ainley et al., 2017; Herr et al., 2019; Ainley et al., 2020; Friedlaender et al., 2021). For example, sightings and density of whales, mainly minkes, along surveys in the Amundsen and Bellingshausen Seas were best explained by sea ice concentration and distance to the marginal ice zone (Ainley et al., 2007) and Williams et al. (2014) observed most minke whales in the Weddell Sea near the sea ice edge. Similarly, Herr et al. (2019) found that minke whale distribution was best explained by distance to the sea ice edge – with most whales observed at the sea ice edge and through to medium sea ice concentrations. However, waters off the Western Antarctic Peninsula are comparatively ice-free (Ducklow et al., 2013), and becoming more so (Turner et al., 2016). Minke whale abundance off the Western Antarctic Peninsula is lower than in surveyed areas in the Weddell Sea (Herr et al., 2019) and minke whales tracked off the Western Antarctic Peninsula spent a great deal of time in open water (sea ice concentration <20%) with few locations in dense sea ice (>20% concentration) (Linsky et al., 2020). Minke whales thus have a facultative rather than obligate link with sea ice.
In the sea-ice zone there is a tight coupling between sea-ice melt and ocean productivity, but there is a weeks-to-months lag between sea ice retreat, phytoplankton increase and then the accumulation of zooplankton, including krill, which finally attracts birds and marine mammals (e.g., Fauchald et al., 2017). The movement of humpback whales in their Antarctic foraging areas has been linked to sea ice concentration one month prior (Andrews-Goff et al., 2018) and to distance to the sea ice edge two months prior (Riekkola et al., 2019). Our results indicate a lagged relationship in minke whales too. In both species, our results indicate a link with lagged sea surface temperature that may suggest water masses may also be influential in driving productivity and the distribution of krill.
Habitat selection modelling of some of the humpback whale and minke whale tracking data used by Friedlaender et al. (2021), and also included in our study, showed similar differences in the habitat selection of humpback and minke whales. Humpback whales showed decreasing preference as sea ice concentration increased, but also as distance from the sea ice edge increased (meaning they preferred areas near the sea ice edge but not in sea ice). They also preferred shallower, onshelf areas. Minke whales showed a comparatively flat preference-response for distance to the sea ice edge and depth. Both species had strong preferences for enclosed bays (Friedlaender et al., 2022). Such known habitat relationships informed by surveys and tracking can help to predict and project overlap between krill consumer and the krill fishery in adaptive management frameworks. This is increasingly urgent as the Western Antarctic Peninsula ecosystem changes (Clarke et al., 2007) with changing predator populations and rapid environmental change in this region, but also in the context of an expanding krill fishing (Trathan et al., 2022). An outlook is that continued decreases in sea ice (Turner et al., 2016) may in future increase favoured humpback whale habitat. Increases in chinstrap penguin populations have been attributed to decreases in the number of years with extensive winter sea ice (Fraser et al., 1992), raising the question of whether the same may happen for humpback whales, although more recently, chinstrap populations are now decreasing (Strycker et al., 2021). Foraging areas may also be accessible to whales for longer, as it will for fishing vessels and, ultimately, krill could become more accessible to consumers and fisheries in their key overwintering habitats. Conversely, habitat favoured by minke whales may decrease. However, the impacts of sea ice loss on krill abundance and distribution will be significant (Flores et al., 2012; Hill et al., 2013; Piñones & Fedorov, 2016; Veytia et al., 2020) with knock-on effects for consumers.
Broadly, our work illustrates the importance of integrating time as well as space when assessing overlap between fisheries and marine predators. Moreover, we show that overlap can be characterized by small spatial scales, and that fisheries should where possible be managed at these small scales, typical of predator foraging scales.
Data Availability Statement
The datasets presented in this article are not readily available because Antarctic krill fishing catch data should be requested from CCAMLR. Fishing effort data are publicly available from Global Fishing Watch. Whale tracking data are available from the authors without undue reservation.
Requests to access the datasets should be directed to CCAMLR (https://www.ccamlr.org/en/node/74767; catch data) and Ryan Reisinger (ci5yLnJlaXNpbmdlckBzb3V0aGFtcHRvbi5hYy51azsgdHJhY2tpbmcgZGF0YQ==).
Ethics Statement
The animal study was reviewed and approved by Duke University Institutional Animal Care and Use Committee (A049-122-01), the University of California Santa Cruz Institutional Animal Care and Use Committee (friea1706), Oregon State University Institutional Animal Care and Use Committee (4513) and the SWR/PIR Regional NMFS Institutional Animal Care and Use Committee. Research was conducted under NMFS Permits 14907, 14809, and 14856, ACA Permits 2009–013 and 2015–011, Duke University IACUC A049-122-01, UCSC IACUC friea1706, and OSU ACU 4513.
Author Contributions
Conceptualization: RR, PT, CJ, AF. Data curation: RR, TJ. Formal analysis: RR; Funding acquisition: RR, PT, CJ, AF, JD. Investigation: RR, PT, CJ, TJ, JD, RP, AF. Writing - original draft: RR, PT. Writing - review and editing: RR, PT, CJ, TJ, JD, RP, AF. All authors contributed to the article and approved the submitted version.
Funding
Funding for this work was provided by the Antarctic Wildlife Research Fund. Tracking work was supported by National Science Foundation Office of Polar Programs awards ANT-0823101, 1250208, and 1440435, by the Lindblad Expeditions−National Geographic Fund, the International Whaling Commission Southern Ocean Research Partnership and the Hogwarts Running Club. PT was supported by the UKRI/BAS Ecosystems programme.
Conflict of Interest
John Durban was employed by Southall Environmental Associates, Inc.
The remaining authors declare that the research was conducted in the absence of any commercial or financial relationships that could be construed as a potential conflict of interest.
Publisher’s Note
All claims expressed in this article are solely those of the authors and do not necessarily represent those of their affiliated organizations, or those of the publisher, the editors and the reviewers. Any product that may be evaluated in this article, or claim that may be made by its manufacturer, is not guaranteed or endorsed by the publisher.
Acknowledgments
We thank the CCAMLR Secretariat for providing access to C1 catch and effort data, and the International Whaling Commission for providing the whaling catch data. We also thank colleagues at the Australian Antarctic Division, including Mike Double, Virginia Andrews Goff, and Elanor Bell, for their support of this research as part of the International Whaling Commission Southern Ocean Research Partnership. We are grateful to Lindblad expeditions and the officers and crew of the M/V National Geographic for support of field operations. Holly Fearnbach assisted with these field operations.
Supplementary Material
The Supplementary Material for this article can be found online at: https://www.frontiersin.org/articles/10.3389/fmars.2022.914726/full#supplementary-material
References
Agnew D. J. (1997). The CCAMLR Ecosystem Monitoring Programme. Antarct. Sci. 9, 235–242. doi: 10.1017/S095410209700031X
Ainley D. G., Dugger K. M., Toniolo V., Gaffney I. (2007). Cetacean Occurrence Patterns in the Amundsen and Southern Bellingshausen Sea Sector, Southern Ocean. Mar. Mammal Sci. 23, 287–305. doi: 10.1111/j.1748-7692.2007.00109.x
Ainley D. G., Jongsomjit D., Ballard G., Thiele D., Fraser W. R., Tynan C. T. (2012). Modeling the Relationship of Antarctic Minke Whales to Major Ocean Boundaries. Polar Biol. 35, 281–290. doi: 10.1007/s00300-011-1075-1
Ainley D. G., Joyce T. W., Saenz B., Pitman R. L., Durban J. W., Ballard G., et al. (2020). Foraging Patterns of Antarctic Minke Whales in McMurdo Sound, Ross Sea. Antarct. Sci. 32, 454–465. doi: 10.1017/S0954102020000310
Ainley D. G., Lindke K., Ballard G., Lyver P. O. B., Jennings S., Toniolo V., et al. (2017). Spatio-Temporal Occurrence Patterns of Cetaceans Near Ross Island, Antarctica 2002–2015: Implications for Food Web Dynamics. Polar Biol. 40, 1761–1775. doi: 10.1007/s00300-017-2100-9
Allison C. (2016). IWC Individual Catch Database Version 6.1, 18 July 2016 (Cambridge: International Whaling Commission).
Andrews-Goff V., Bestley S., Gales N. J., Laverick S. M., Paton D., Polanowski A. M., et al. (2018). Humpback Whale Migrations to Antarctic Summer Foraging Grounds Through the Southwest Pacific Ocean. Sci. Rep. 8, 1–14. doi: 10.1038/s41598-018-30748-4
Atkinson A., Hill S. L., Pakhomov E. A., Siegel V., Anadon R., Chiba S., et al. (2017). KRILLBASE: A Circumpolar Database of Antarctic Krill and Salp Numerical Densities 1926-2016. Earth Syst. Sci. Data 9, 193–210. doi: 10.5194/essd-9-193-2017
Atkinson A., Hill S. L., Pakhomov E. A., Siegel V., Reiss C. S., Loeb V. J., et al. (2019). Krill (Euphausia Superba) Distribution Contracts Southward During Rapid Regional Warming. Nat. Clim. Change 9, 142–147. doi: 10.1038/s41558-018-0370-z
Atkinson A., Siegel V., Pakhomov E., Rothery P. (2004). Long-Term Decline in Krill Stock and Increase in Salps Within the Southern Ocean. Nature 10, 1–6. doi: 10.1038/nature02996
Atkinson A., Siegel V., Pakhomov E. A., Rothery P., Loeb V., Ross R. M., et al. (2008). Oceanic Circumpolar Habitats of Antarctic Krill. Mar. Ecol. Prog. Ser. 362, 1–23. doi: 10.3354/meps07498
Bamford C. C. G., Warwick-Evans V., Staniland I. J., Jackson J. A., Trathan P. N. (2021). Wintertime Overlaps Between Female Antarctic Fur Seals (Arctocephalus Gazella) and the Krill Fishery at South Georgia, South Atlantic. PLoS One 16, 1–20. doi: 10.1371/journal.pone.0248071
Benhamou S. (1992). Efficiency of Area-Concentrated Searching Behaviour in a Continuous Patchy Environment. J. Theor. Biol. 159, 67–81. doi: 10.1016/S0022-5193(05)80768-4
Bestley S., Andrews-Goff V., van Wijk E., Rintoul S. R., Double M. C., How J. (2019). New Insights Into Prime Southern Ocean Forage Grounds for Thriving Western Australian Humpback Whales. Sci. Rep. 9, 1–12. doi: 10.1038/s41598-019-50497-2
Bestley S., Ropert-Coudert Y., Bengtson Nash S., Brooks C. M., Cotté C., Dewar M., et al. (2020). Marine Ecosystem Assessment for the Southern Ocean: Birds and Marine Mammals in a Changing Climate. Front. Ecol. Evol. 8. doi: 10.3389/fevo.2020.566936
Branch T. A. (2011). Humpback Whale Abundance South of 60°S From Three Completed Sets of IDCR/SOWER Circumpolar Surveys. J. Cetacean Res. Manage. Special Issue 3, 53–69.
Capella J. E., Quetin L. B., Hofmann E. E., Ross R. M. (1992). Models of the Early Life History of Euphausia Superba – Part II. Lagrangian Calculations. Deep-Sea Res. 39, 1201–1220. doi: 10.1016/0198-0149(92)90064-Z
Cavalieri D. J., Parkinson C. L., Gloersen P., Zwally H. J. (1996). Updated Yearly. Sea Ice Concentrations From Nimbus-7 SMMR and DMSP SSM/I-SSMIS Passive Microwave Data, Version 1 (Boulder, Colorado USA: NASA National Snow and Ice Data Center Distributed Active Archive Center). doi: 10.5067/8GQ8LZQVL0VL
CCAMLR Secretariat (2021). “Summary of Incidental Mortality Associated With Fishing Activities Collected in Scientific Observer and Vessel Data During the 2020 and 2021 Seasons,” in Report of the Working Group on Fish Stock Assessment, Document WG-FSA-2021/04 Rev. 1 (Hobart: Scientific Committee for the Conservation of Antarctic Marine Living Resources). Available at: https://meetings.ccamlr.org/en/wg-fsa-2021/04-rev-1.
Cimino M. A., Lynch H. J., Saba V. S., Oliver M. J. (2016). Projected Asymmetric Response of Adélie Penguins to Antarctic Climate Change. Sci. Rep. 6, 28785. doi: 10.1038/srep28785
Clapham P. J (2018). “Humpback Whale: Megaptera novaeangliae,” in Encyclopedia of Marine Mammals, eds. Würsig B, Thewissen J. G. M., Kovacs K. M. (London: Academic Press), 489–492. doi: 10.1016/b978-0-12-804327-1.00154-0
Clarke A., Murphy E. J., Meredith M. P., King J. C., Peck L. S., Barnes D. K. A., et al. (2007). Climate Change and the Marine Ecosystem of the Western Antarctic Peninsula. Philos. Trans. R. Soc B Biol. Sci. 362, 149–166. doi: 10.1098/rstb.2006.1958
CLS (Collecte Localisation Satellites) (2016). Argos User’s Manual Version 1.6.6 (Toulouse: Collecte Localisation Satellites). Available at: https://www.argos-system.org/wp-content/uploads/2016/08/r363_9_argos_users_manual-v1.6.6.pdf.
Constable A. J., De LaMare W. K., Agnew D. J., Everson I., Miller D. (2000). Managing Fisheries to Conserve the Antarctic Marine Ecosystem: Practical Implementation of the Convention on the Conservation of Antarctic Marine Living Resources (CCAMLR). ICES J. Mar. Sci. 57, 778–791. doi: 10.1006/jmsc.2000.0725
Constable A. J. (2022). Imperatives for Integrated Science and Policy in Managing Greenhouse Gas Risks to the Southern Polar Region. Glob. Chang. Biol., 1–4. doi: 10.1111/gcb.16219
Cooke J. G. (2018)Megaptera Novaeangliae. In: The IUCN Red List of Threatened Species 2018. Available at: https://dx.doi.org/10.2305/IUCN.UK.2018-2.RLTS.T13006A50362794.en (Accessed 07 January 2022). e.T13006A50362794.
Cooke J. G., Zerbini A. N., Taylor B. L. (2018)Balaenoptera Bonaerensis. In: The IUCN Red List of Threatened Species 2018 (Accessed 07 January 2022). e.T2480A50350661.
Cox M. J., Candy S., de la Mare W. K., Nicol S., Kawaguchi S., Gales N. (2018). No Evidence for a Decline in the Density of Antarctic Krill Euphausia Superba Dana 1850, in the Southwest Atlantic Sector Between 1976 and 2016. J. Crustac. Biol. 38, 656–661. doi: 10.1093/jcbiol/ruy072
Curtice C., Johnston D. W., Ducklow H. W., Gales N. J., Halpin P. N., Friedlaender A. S. (2015). Modeling the Spatial and Temporal Dynamics of Foraging Movements of Humpback Whales (Megaptera Novaeangliae) in the Western Antarctic Peninsula. Mov. Ecol. 3, 13. doi: 10.1186/s40462-015-0041-x
Delegations of Argentina and Chile (2020). “Revised Proposal for a Conservation Measure Establishing a Marine Protected Area in Domain 1 (Western Antarctic Peninsula and South Scotia Arc),” in Thirty-ninth Meeting of the Commission, document CCAMLR-39/08 Rev. 1. (Hobart: Commission for the Conservation of Antarctic Marine Living Resources).
Delegations of Norway and the United Kingdom (2021). “Further Information Requested by WG-FSA-2021 on Humpback Whale (Megaptera Novaeangliae) Mortality Incidents Recorded by the Krill Fishery in Subareas 48.1 and 48.2 During the 2020/21 Season,” in Fortieth Meeting of the Scientific Committee, document SC-CAMLR-40/BG/27. (Hobart: Commission for the Conservation of Antarctic Marine Living Resources).
Dominello T., Širović A. (2016). Seasonality of Antarctic Minke Whale (Balaenoptera Bonaerensis) Calls Off the Western Antarctic Peninsula. Mar. Mammal Sci. 32 (3), 826–838. doi: 10.1111/mms.12302
Ducklow H., Clarke A., Dickhut R., Doney S. C., Geisz H., Huang K., et al. (2012). “The Marine System of the Western Antarctic Peninsula,” in Antarctic Ecosystems (Chichester, UK: John Wiley & Sons, Ltd), 121–159. doi: 10.1002/9781444347241.ch5
Ducklow H. W, Fraser W. R., Meredith M. P, Stammerjohn S. E., Doney S. C., Martinson D. G, et al (2013). West Antarctic Peninsula: An Ice-Dependent Coastal Marine Ecosystem in Transition. Oceanography 26, 190–203. doi:10.5670/oceanog.2013.62.
Evans J. S., Murphy M. A. (2021) Spatialeco. R Package Version 1.3-6. Available at: https://github.com/jeffreyevans/spatialEco.
Fauchald P., Erikstad K. E., Skarsfjord H. (2000). Scale-Dependent Predator-Prey Interactions: The Hierarchical Spatial Distribution of Seabirds and Prey. Ecology 81, 773. doi: 10.2307/177376
Fauchald P., Tarroux A., Tveraa T., Cherel Y., Ropert-Coudert Y., Kato A., et al. (2017). Spring Phenology Shapes the Spatial Foraging Behavior of Antarctic Petrels. Mar. Ecol. Prog. Ser. 568, 203–215. doi: 10.3354/meps12082
Fauchald P., Tveraa T. (2003). Using First-Passage Time in the Analysis of Area-Restricted Reports. Ecol. Soc Am. 84, 282–288. doi: 10.1890/0012-9658(2003)084[0282:UFPTIT]2.0.CO;2
Flores H., Atkinson A., Kawaguchi S., Krafft B. A., Milinevsky G., Nicol S., et al. (2012). Impact of Climate Change on Antarctic Krill. Mar. Ecol. Prog. Ser. 458, 1–19. doi: 10.3354/meps09831
Forcada J., Trathan P. N., Boveng P. L., Boyd I. L., Burns J. M., Costa D. P., et al. (2012). Responses of Antarctic Pack-Ice Seals to Environmental Change and Increasing Krill Fishing. Biol. Conserv. 149, 40–50. doi: 10.1016/j.biocon.2012.02.002
Fraser W. R., Trivelpiece W. Z., Ainley D. G., Trivelpiece S. G. (1992). Increases in Antarctic Penguin Populations: Reduced Competition With Whales or a Loss of Sea Ice Due to Environmental Warming? Polar Biol. 11, 525–531. doi: 10.1007/BF00237945
Friedlaender A. S., Goldbogen J. A., Nowacek D. P., Read A. J., Johnston D., Gales N. (2014). Feeding Rates and Under-Ice Foraging Strategies of the Smallest Lunge Filter Feeder, the Antarctic Minke Whale (Balaenoptera Bonaerensis). J. Exp. Biol. 217, 2851–2854. doi: 10.1242/jeb.106682
Friedlaender A., Halpin P., Qian S., Lawson G., Wiebe P., Thiele D., et al. (2006). Whale Distribution in Relation to Prey Abundance and Oceanographic Processes in Shelf Waters of the Western Antarctic Peninsula. Mar. Ecol. Prog. Ser. 317, 297–310. doi: 10.3354/meps317297
Friedlaender A. S., Johnston D. W., Fraser W. R., Burns J., Patrick N.H., Costa D. P. (2011). Ecological Niche Modeling of Sympatric Krill Predators Around Marguerite Bay, Western Antarctic Peninsula. Deep. Res. Part II Top. Stud. Oceanogr. 58, 1729–1740. doi: 10.1016/j.dsr2.2010.11.018
Friedlaender A. S., Johnston D. W., Tyson R. B., Kaltenberg A., Goldbogen J. A., Stimpert A. K., et al. (2016). Multiple-Stage Decisions in a Marine Central-Place Forager. R. Soc Open Sci. 3, 160043. doi: 10.1098/rsos.160043
Friedlaender A., Joyce T., Johnston D., Read A., Nowacek D., Goldbogen J., et al. (2021). Sympatry and Resource Partitioning Between the Largest Krill Consumers Around the Antarctic Peninsula. Mar. Ecol. Prog. Ser. 669, 1–16. doi: 10.3354/meps13771
Friedlaender A. S., Tyson R. B., Stimpert A. K., Read A. J., Nowacek D. P. (2013). Extreme Diel Variation in the Feeding Behavior of Humpback Whales Along the Western Antarctic Peninsula During Autumn. Mar. Ecol. Prog. Ser. 494, 281–289. doi: 10.3354/meps10541
GEBCO Compilation Group (2020). GEBCO 2020 Grid. (Southampton: British Oceanographic Data Centre). doi: 10.5285/a29c5465-b138-234d-e053-6c86abc040b9
Godø O. R., Trathan, (2022). Voluntary Actions by the Antarctic Krill Fishing Industry Help Reduce Potential Negative Impacts on Land-Based Marine Predators During Breeding, Highlighting the Need for CCAMLR Action. ICES J. Mar. Sci. doi: 10.1093/icesjms/fsac092
Grémillet D., Ponchon A., Paleczny M., Palomares M. L. D., Karpouzi V., Pauly D. (2018). Persisting Worldwide Seabird-Fishery Competition Despite Seabird Community Decline. Curr. Biol. 28, 4009–4013.e2. doi: 10.1016/j.cub.2018.10.051
Hedley S., Reilly S., Borberg J., Holland R., Hewitt R., Watkins J., et al. (2001). “Modelling Whale Distribution: A Preliminary Analysis of Data Collected on the CCAMLR-IWC Krill Synoptic Survey 2000,” in Document SC-CAMLR-XX/BG/30, (Hobart: CCAMLR).
Hengl T., Nussbaum M., Wright M. N., Heuvelink G. B. M., Gräler B. (2018). Random Forest as a Generic Framework for Predictive Modeling of Spatial and Spatio-Temporal Variables. PeerJ 6,e5518. doi: 10.7717/peerj.5518
Herr H., Kelly N., Dorschel B., Huntemann M., Kock K. H., Lehnert L. S., et al. (2019). Aerial Surveys for Antarctic Minke Whales (Balaenoptera Bonaerensis) Reveal Sea Ice Dependent Distribution Patterns. Ecol. Evol. 9, 5664–5682. doi: 10.1002/ece3.5149
Herr H., Viquerat S., Siegel V., Kock K. H., Dorschel B., Huneke W. G. C., et al. (2016). Horizontal Niche Partitioning of Humpback and Fin Whales Around the West Antarctic Peninsula: Evidence From a Concurrent Whale and Krill Survey. Polar Biol. 39, 799–818. doi: 10.1007/s00300-016-1927-9
Hewitt R. P., Watkins J., Naganobu M., Sushin V., Brierley A. S., Demer D., et al. (2004). Biomass of Antarctic Krill in the Scotia Sea in January/February 2000 and its Use in Revising an Estimate of Precautionary Yield. Deep. Res. Part II Top. Stud. Oceanogr. 51, 1215–1236. doi: 10.1016/j.dsr2.2004.06.011
Hijmans R. J. (2020). “Raster: Geographic Data Analysis and Modeling,” in R Package Version 3, 4–5. Available at: https://CRAN.R-project.org/package=raster.
Hill S. L., Atkinson A., Pakhomov E. A., Siegel V. (2019). Evidence for a Decline in the Population Density of Antarctic Krill Euphausia Superba. J. Crustac. Biol. 39, 316–322. doi: 10.1093/jcbiol/ruz004
Hill S. L., Phillips T., Atkinson A. (2013). Potential Climate Change Effects on the Habitat of Antarctic Krill in the Weddell Quadrant of the Southern Ocean. PLoS One 8, e72246. doi: 10.1371/journal.pone.0072246
Hill S. L., Atkinson A., Darby C., Fielding S., Krafft B. A., Godø O. R., et al. (2016). Is Current Management of the Antarctic Krill Fishery in the Atlantic Sector of the Southern Ocean Precautionary? CCAMLR Sci.23, 31–51.
Hindell M. A., Reisinger R. R., Ropert-Coudert Y., Hückstädt L. A., Trathan P. N., Bornemann H., et al. (2020). Tracking of Marine Predators to Protect Southern Ocean Ecosystems. Nature 580, 87–92. doi: 10.1038/s41586-020-2126-y
Hinke J. T., Cossio A. M., Goebel M. E., Reiss C. S., Trivelpiece W. Z., Watters G. M. (2017a). Identifying Risk: Concurrent Overlap of the Antarctic Krill Fishery With Krill-Dependent Predators in the Scotia Sea. PLoS One 12, 1–24. doi: 10.1371/journal.pone.0170132
Hinke J. T., Trivelpiece S. G., Trivelpiece W. Z. (2017b). Variable Vital Rates and the Risk of Population Declines in Adélie Penguins From the Antarctic Peninsula Region. Ecosphere 8 (1), e01666. doi: 10.1002/ecs2.1666
International Whaling Commission (2013). Reports of the Subcommittee on in-Depth Assessments. J. Cetacean Res. Manage. 14, 195–213.
International Whaling Commission (2016). Report of the Scientific Committee. Annex H. Report of the Sub-Committee on Other Southern Hemisphere Whale Stocks. J. Cetacean Res. Manage. 17 (Suppl.), 250–282.
Johannessen J. E. D., Biuw M., Lindstrøm U., Ollus V. M. S., Martín López L. M., Gkikopoulou K. C., et al. (2022). Intra-Season Variations in Distribution and Abundance of Humpback Whales in the West Antarctic Peninsula Using Cruise Vessels as Opportunistic Platforms. Ecol. Evol. 12, e8571. doi: 10.1002/ece3.8571
Johnston D. W., Friedlaender A. S., Read A. J., Nowacek D. P. (2012). Initial Density Estimates of Humpback Whales Megaptera Novaeangliae in the Inshore Waters of the Western Antarctic Peninsula During the Late Autumn. Endanger. Species Res. 18, 63–71. doi: 10.3354/esr00395
Jonsen I. (2016). Joint Estimation Over Multiple Individuals Improves Behavioural State Inference From Animal Movement Data. Sci. Rep. 6, 20625. doi: 10.1038/srep20625
Jonsen I., Flemming J., Myers R. (2005). Robust State-Space Modeling of Animal Movement Data. Ecology 86, 2874–2880. doi: 10.1890/04-1852
Jonsen I., Myers R., James M. (2007). Identifying Leatherback Turtle Foraging Behaviour From Satellite Telemetry Using a Switching State-Space Model. Mar. Ecol. Prog. Ser. 337, 255–264. doi: 10.3354/meps337255
Kareiva P., Odell G. (1987). Swarms of Predators Exhibit “Preytaxis” If Individual Predators Use Area-Restricted Search. Am. Nat. 130, 233–270. doi: 10.1086/284707
Krafft B. A., Macaulay G. J., Skaret G., Knutsen T., Bergstad O. A., Lowther A., et al. (2021). Standing Stock of Antarctic Krill (Euphausia Superba Dana 1850) (Euphausiacea) in the Southwest Atlantic Sector of the Southern Ocean 2018–19. J. Crustacean Biol. 41 (3), ruab046. doi: 10.1093/jcbiol/ruab071
Kroodsma D. A., Mayorga J., Hochberg T., Miller N. A., Boerder K., Ferretti F., et al. (2018). Tracking the Global Footprint of Fisheries. Science 359, 904–908. doi: 10.1126/science.aao5646
Krüger L. (2019). Spatio-Temporal Trends of the Krill Fisheries in the Western Antarctic Peninsula and Southern Scotia Arc. Fish. Manage. Ecol. 26, 327–333. doi: 10.1111/fme.12363
Krüger L., Huerta M. F., Santa Cruz F., Cárdenas C. A. (2021). Antarctic Krill Fishery Effects Over Penguin Populations Under Adverse Climate Conditions: Implications for the Management of Fishing Practices. Ambio 50, 560–571. doi: 10.1007/s13280-020-01386-w
Lascara C. M., Hofmann E. E., Ross R. M., Quetin L. B. (1999). Seasonal Variability in the Distribution of Antarctic Krill, Euphausia Superba, West of the Antarctic Peninsula. Deep. Res. Part I Oceanogr. Res. Pap. 46, 951–984. doi: 10.1016/S0967-0637(98)00099-5
Lewison R. L., Crowder L. B., Wallace B. P., Moore J. E., Cox T., Zydelis R., et al. (2014). Global Patterns of Marine Mammal, Seabird, and Sea Turtle Bycatch Reveal Taxa-Specific and Cumulative Megafauna Hotspots. Proc. Natl. Acad. Sci. U.S.A. 111, 5271–5276. doi: 10.1073/pnas.1318960111
Linsky J. M. J., Wilson N., Cade D. E., Goldbogen J. A., Johnston D. W., Friedlaender A. S. (2020). The Scale of the Whale: Using Video-Tag Data to Evaluate Sea-Surface Ice Concentration From the Perspective of Individual Antarctic Minke Whales. Anim. Biotelemetry 8, 31. doi: 10.1186/s40317-020-00218-8
Lockyer C. (1981) Growth and Energy Budgets of Large Baleen Whales From the Southern Hemisphere.FAO Fish. Ser. 5, 379–487.
Martinson D. G., McKee D. C.. (2012). Transport of Warm Upper Circumpolar Deep Water Onto the Western Antarctic Peninsula Continental Shelf. Ocean Sci. 8, 433–442. doi: 10.5194/os-8-433-2012
Nicol S., Foster J., Kawaguchi S. (2012). The Fishery for Antarctic Krill - Recent Developments. Fish Fish. 13, 30–40. doi: 10.1111/j.1467-2979.2011.00406.x
Nowacek D. P., Zhou M., Read A. J., Espinasse B., Zhu Y., Halpin P. N., et al. (2011). Super-Aggregations of Krill and Humpback Whales in Wilhelmina Bay, Antarctic Peninsula. PLoS One 6, e19173. doi: 10.1371/journal.pone.0019173
Pallin L. J., Baker C. S., Steel D., Kellar N. M., Robbins J., Johnston D. W., et al. (2018). High Pregnancy Rates in Humpback Whales (Megaptera Novaeangliae) Around the Western Antarctic Peninsula, Evidence of a Rapidly Growing Population. R. Soc Open Sci. 5, 180017. doi: 10.1098/rsos.180017
Pickett E. P., Fraser W. R., Patterson-Fraser D. L., Cimino M. A., Torres L. G., Friedlaender A. S. (2018). Spatial Niche Partitioning may Promote Coexistence of Pygoscelis Penguins as Climate-Induced Sympatry Occurs. Ecol. Evol. 8, 9764–9778. doi: 10.1002/ece3.4445
Piñones A., Fedorov A. V. (2016). Projected Changes of Antarctic Krill Habitat by the End of the 21st Century. Geophys. Res. Lett. 43, 8580–8589. doi: 10.1002/2016GL069656
Piñones A., Hofmann E. E., Daly K. L., Dinniman M. S., Klinck J. M. (2013b). Modeling the Remote and Local Connectivity of Antarctic Krill Populations Along the Western Antarctic Peninsula. Mar. Ecol. Prog. Ser. 481, 69–92. doi: 10.3354/meps10256
Queiroz N., Humphries N. E., Couto A., Vedor M., da Costa I., Sequeira A. M. M., et al. (2019). Global Spatial Risk Assessment of Sharks Under the Footprint of Fisheries. Nature 4, 461–466. doi: 10.1038/s41586-019-1444-4
Reisinger R. R., Friedlaender A. S., Zerbini A. N., Palacios D. M., Andrews-Goff V., Dalla Rosa L., et al. (2021). Combining Regional Habitat Selection Models for Large-Scale Prediction: Circumpolar Habitat Selection of Southern Ocean Humpback Whales. Remote Sens. 13, 2074. doi: 10.3390/rs13112074
Reiss C. S., Cossio A., Santora J. A., Dietrich K. S., Murray A., Greg Mitchell B., et al. (2017). Overwinter Habitat Selection by Antarctic Krill Under Varying Sea-Ice Conditions: Implications for Top Predators and Fishery Management. Mar. Ecol. Prog. Ser. 568, 1–16. doi: 10.3354/meps12099
Riekkola L., Andrews-Goff V., Friedlaender A., Constantine R., Zerbini A. N. (2019). Environmental Drivers of Humpback Whale Foraging Behavior in the Remote Southern Ocean. J. Exp. Mar. Bio. Ecol. 517, 1–12. doi: 10.1016/j.jembe.2019.05.008
Rocha R. C. Jr., Clapham P. J., Ivashchenko Y. (2015). Emptying the Oceans: A Summary of Industrial Whaling Catches in the 20th Century. Mar. Fish. Rev. 76, 37–48. doi: 10.7755/MFR.76.4.3
R Core Team (2022). R: A language and environment for statistical computing. Vienna, Austria: R Foundation for Statistical Computing. https://www.r-project.org/.
Santa Cruz F., Ernst B., Arata J. A., Parada C. (2018). ). Spatial and Temporal Dynamics of the Antarctic Krill Fishery in Fishing Hotspots in the Bransfield Strait and South Shetland Islands. Fish. Res. 208, 157–166. doi: 10.1016/j.fishres.2018.07.020
Santa Cruz F., Krüger L., Cárdenas C. A. (2022). Spatial and Temporal Catch Concentrations for Antarctic Krill: Implications for Fishing Performance and Precautionary Management in the Southern Ocean. Ocean Coast. Manage. 223, 106146. doi: 10.1016/j.ocecoaman.2022.106146
Santora J. A., Reiss C. S., Loeb V. J., Veit R. R. (2010). Spatial Association Between Hotspots of Baleen Whales and Demographic Patterns of Antarctic Krill Euphausia Superba Suggests Size-Dependent Predation. Mar. Ecol. Prog. Ser. 405, 255–269. doi: 10.3354/meps08513
Santora J. A., Schroeder I. D., Loeb V. J. (2014). Spatial Assessment of Fin Whale Hotspots and Their Association With Krill Within an Important Antarctic Feeding and Fishing Ground. Mar. Biol. 161, 2293–2305. doi: 10.1007/s00227-014-2506-7
Savoca M. S., Czapanskiy M. F., Kahane-Rapport S. R., Gough W. T., Fahlbusch J. A., Bierlich K. C., et al. (2021). Baleen Whale Prey Consumption Based on High-Resolution Foraging Measurements. Nature 599, 85–90. doi: 10.1038/s41586-021-03991-5
Scales K. L., Hazen E. L., Jacox M. G., Castruccio F., Maxwell S. M., Lewison R. L., et al. (2018). Fisheries Bycatch Risk to Marine Megafauna is Intensified in Lagrangian Coherent Structures. Proc. Natl. Acad. Sci115 7362–7367. doi: 10.1073/pnas.1801270115
SC-CCAMLR (2010) Report of the twenty-ninth meeting of the Scientific Committee. SC-CAMLR-XXIX, (Hobart: CCAMLR).
SC-CCAMLR (2016) in Report of the thirty-fifth meeting of the Scientific Committee. SC-CCAMLR-XXXV, (Hobart: CCAMLR).
SC-CCAMLR (2019) in Report of the thirty-eighth meeting of the scientific committee. SC-CAMLR-38, (Hobart: CCAMLR).
Siegel V. (1988). “A Concept of Seasonal Variation of Krill (Euphausia Superba) Distribution and Abundance West of the Antarctic Peninsula,” in Antarctic Ocean and Resources Variability. Ed. Sahrhage D. (Berlin: Springer-Verlag), 219–230.
Siegel V. (2005). Distribution and Population Dynamics of Euphausia Superba: Summary of Recent Findings. Polar Biol. 29, 1–22. doi: 10.1007/s00300-005-0058-5
Strycker N., Wethington M., Borowicz A., Forrest S., Witharana C., Hart T., et al. (2020). A Global Population Assessment of the Chinstrap Penguin (Pygoscelis Antarctica). Sci. Rep. 10, 19474. doi: 10.1038/s41598-020-76479-3
Strycker N., Borowicz A., Wethington M., Forrest S., Shah V., Liu Y., et al. (2021). Fifty-Year Change in Penguin Abundance on Elephant Island, South Shetland Islands, Antarctica: Results of the 2019–20 Census.Polar Biol. 44, 45–56. doi: 10.1007/s00300-020-02774-4
Sumner M. D. (2021). “Raadtools: Tools for Synoptic Environmental Spatial Data,” in R Package Version 0.6.0.9020. Available at: https://github.com/AustralianAntarcticDivision/raadtools.
Tarling G. A., Fielding S. (2016). “Swarming and Behaviour in Antarctic Krill,” in Biology and Ecology of Antarctic Krill. Advances in Polar Ecology. Ed. Siegel V. (Cham: Springer). doi: 10.1007/978-3-319-29279-3_8
Trathan P. N., Fielding S., Hollyman P. R., Murphy E. J., Warwick-Evans V., Collins M. A. (2021). Enhancing the Ecosystem Approach for the Fishery for Antarctic Krill Within the Complex, Variable, and Changing Ecosystem at South Georgia. ICES J. Mar. Sci. 78, 2065–2081. doi: 10.1093/icesjms/fsab092
Trathan P., Godø O. R., Hill S. (2016). “Possible Options for the Future Management of the Antarctic Krill Fishery in Subarea 48.2,” in Working Paper submitted to the CCAMLRWorking Group on Ecosystem Monitoring and Management (WG-EMM-16/18), (Hobart: CCAMLR).
Trathan P. N., Hill S. L. (2016). “The Importance of Krill Predation in the Southern Ocean,” in Biology and Ecology of Antarctic Krill. Ed. Siegel V. (Cham: Springer), 321–350. doi: 10.1007/978-3-319-29279-3_9
Trathan P. N., Priddle J., Watkins J. L., Miller D. G. M., Murray A. W. A. (1993). Spatial Variability of Antarctic Krill in Relation to Mesoscale Hydrography. Mar. Ecol. Prog.Ser. 98, 61–71. doi: 10.3354/meps098061
Trathan P. N., Warwick-Evans V., Hinke J. T., Young E. F., Murphy E. J., Carneiro A. P. B., et al. (2018). Managing Fishery Development in Sensitive Ecosystems: Identifying Penguin Habitat Use to Direct Management in Antarctica. Ecosphere 9, e02392 doi: 10.1002/ecs2.2392
Trathan P. N., Warwick-Evans V., Young E. F., Friedlaender A., Kim J. H., Kokubun N. (2022). The Ecosystem Approach to Management of the Antarctic Krill Fishery - the ‘Devils are in the Detail’ at Small Spatial and Temporal Scales. J. Mar. Syst. 225, 103598. doi: 10.1016/j.jmarsys.2021.103598
Trivelpiece W. Z., Hinke J. T., Miller A. K., Reiss C. S., Trivelpiece S. G., Watters G. M. (2011). Variability in Krill Biomass Links Harvesting and Climate Warming to Penguin Population Changes in Antarctica. Proc. Natl. Acad. Sci. 108, 7625–7628. doi: 10.1073/pnas.1016560108
Turner J., Scott Hosking J., Marshall G. J., Phillips T., Bracegirdle T. J. (2016). Antarctic Sea Ice Increase Consistent With Intrinsic Variability of the Amundsen Sea Low. Climate Dynamics 46 (7–8), 2391–2402. doi: 10.1007/s00382-015-2708-9
Tyson R. B., Friedlaender A. S., Nowacek D. P. (2016). Does Optimal Foraging Theory Predict the Foraging Performance of a Large Air-Breathing Marine Predator? Anim. Behav. 116, 223–235. doi: 10.1016/j.anbehav.2016.03.034
Van Etten J. (2017). R Package Gdistance: Distances and Routes on Geographical Grids. J. Stat. Software 76 (1), 1–21. doi: 10.18637/jss.v076.i13
Veytia D., Corney S., Meiners K. M., Kawaguchi S., Murphy E. J., Bestley S. (2020). Circumpolar Projections of Antarctic Krill Growth Potential. Nat. Clim. Change 10, 568–575. doi: 10.1038/s41558-020-0758-4
Wang X., Moffat C., Dinniman M. S., Klinck J. M., Sutherland D. A., Aguiar-González B. (2022). Variability and Dynamics of Along-Shore Exchange on the West Antarctic Peninsula (WAP) Continental Shelf. J. Geophys. Res. Ocean. 127, e2021JC017645. doi: 10.1029/2021JC017645
Warwick-Evans V., Kelly N., Dalla Rosa L., Friedlaender A. S., Hinke J. T., Kim J. H., et al. (2022). Using Seabird and Whale Distribution Models to Estimate Spatial Consumption of Antarctic Krill to Inform Fishery Management. Ecosphere 13, e4083. doi: 10.1002/ecs2.4083
Warwick-Evans V., Ratcliffe N., Lowther A. D., Manco F., Ireland L., Clewlow H. L., et al. (2018). Using Habitat Models for Chinstrap Penguins Pygoscelis Antarctica to Advise Krill Fisheries Management During the Penguin Breeding Season. Divers. Distrib. 24, 1756–1771. doi: 10.1111/ddi.12817
Watters G. M., Hinke J. T., Reiss C. S. (2020). Long-Term Observations From Antarctica Demonstrate That Mismatched Scales of Fisheries Management and Predator-Prey Interaction Lead to Erroneous Conclusions About Precaution. Sci. Rep. 10, 1–9. doi: 10.1038/s41598-020-59223-9
Weinstein B. G., Double M., Gales N., Johnston D. W., Friedlaender A. S. (2017). Identifying Overlap Between Humpback Whale Foraging Grounds and the Antarctic Krill Fishery. Biol. Conserv. 210, 184–191. doi: 10.1016/j.biocon.2017.04.014
Weinstein B. G., Friedlaender A. S. (2017). Dynamic Foraging of a Top Predator in a Seasonal Polar Marine Environment. Oecologia 185, 427–435. doi: 10.1007/s00442-017-3949-6
Williams R., Kelly N., Boebel O., Friedlaender A. S., Herr H., Kock K.-H., et al. (2014). Counting Whales in a Challenging, Changing Environment. Sci. Rep. 4, 4170. doi: 10.1038/srep04170
Keywords: humpback whale (Megaptera novaeangliae), Antarctic krill (Euphausia superba), Antarctic minke whale (Balaenoptera bonaerensis), competition, fishing, tracking
Citation: Reisinger RR, Trathan PN, Johnson CM, Joyce TW, Durban JW, Pitman RL and Friedlaender AS (2022) Spatiotemporal Overlap of Baleen Whales and Krill Fisheries in the Western Antarctic Peninsula Region. Front. Mar. Sci. 9:914726. doi: 10.3389/fmars.2022.914726
Received: 07 April 2022; Accepted: 10 June 2022;
Published: 21 July 2022.
Edited by:
Jaime Albino Ramos, University of Coimbra, PortugalReviewed by:
Andres De La Cruz, University of Cádiz, SpainLucas Krüger, Instituto Antártico Chileno (INACH), Chile
Copyright © 2022 Reisinger, Trathan, Johnson, Joyce, Durban, Pitman and Friedlaender. This is an open-access article distributed under the terms of the Creative Commons Attribution License (CC BY). The use, distribution or reproduction in other forums is permitted, provided the original author(s) and the copyright owner(s) are credited and that the original publication in this journal is cited, in accordance with accepted academic practice. No use, distribution or reproduction is permitted which does not comply with these terms.
*Correspondence: Ryan R. Reisinger, ci5yLnJlaXNpbmdlckBzb3V0aGFtcHRvbi5hYy51aw==