- 1School of Ocean and Earth Science, University of Southampton, Southampton, United Kingdom
- 2Centre for Resource Management and Environmental Studies (CERMES), University of the West Indies, Cave Hill, Barbados
- 3The University of Southern Mississippi, Center for Fisheries Research and Development, Gulf Coast Research Laboratory, Ocean Springs, MS, United States
Proliferation of sargassum across the tropical Atlantic since 2011 has motivated a range of forecasting methods. Statistical methods based on basin-scale satellite data are used to address seasonal timescales. Other methods involve explicit Lagrangian calculations of trajectories for particles that are representative of drifting sargassum over days-months. This computed sargassum drift is attributed to the combined action of surface currents, winds and waves, individually or in various combinations. Such calculations are undertaken with both observed surface drift and simulated currents, each involving strengths and weaknesses. Observed drift implicitly includes the action on sargassum of winds and waves, assumed equivalent between drifters and sargassum mats. Simulated currents provide large gridded datasets that facilitate computation of ensembles, enabling some quantification of the uncertainty inherent in an eddy-rich ocean, further subject to interannual variability. A more limited number of forecasts account for in situ growth or loss of sargassum biomass, subject to considerable uncertainty. Forecasts provide either non-dimensional indices or quantities of sargassum, accumulated in specified areas or counted across specified transects over a given time interval. Proliferation of different forecast methodologies may reduce uncertainty, if predictions for given seasons are consistent in broad terms, but there is scope to coordinate different approaches with common geographical foci and predicted variables, to facilitate direct inter-comparisons. In an example of forecasting westward sargassum flux into the Caribbean during the first half of 2022, challenges and opportunities are highlighted. In conclusion, prospects for closer alignment of complementary forecasting methods, and implications for sargassum management, are identified.
Introduction
Proliferation of sargassum across the tropical Atlantic is now an established seasonal phenomenon, since the first widespread appearance of previously rare sargassum variants in 2011 (Franks et al., 2012; Gower et al., 2013), and the subject of studies to explore drivers – both physical (Brooks et al., 2018; Johns et al., 2020; Skliris et al., 2022) and biogeochemical (Oviatt et al., 2019; Lapointe et al., 2021). The timing and severity of sargassum beaching events across the Caribbean is however highly variable, with record quantities present during 2018 (Wang et al., 2019). This underpins a leading objective, the focus here, to develop and improve forecasts of sargassum events at seasonal timescale.
Common to forecasting the oceanic transport of sargassum are satellite observations, briefly outlined here. This remote sensing of sargassum is based on the spectral reflectance in red, near infrared and shortwave infrared from floating vegetation; measurements are obtained per 1-km pixel in images from the Moderate Resolution Imaging Spectroradiometer (MODIS) instrument aboard the Terra and Aqua satellites, subsequently interpreted as the fractional area associated with floating algae (Hu, 2009). Now standardized and freely accessible are 7-day composite ‘FA_density’ maps of an ‘Alternative Floating Algae Index’ (AFAI), provided for selected sub-regions of the tropical Atlantic (Wang and Hu, 2016). Collecte Localisation Satellites (CLS, see https://www.cls.fr/en/) further use a Normalized Floating Algae Index (NFAI) derived from Sentinel satellite images that span the entire tropical Atlantic, not freely available at the present time.
A drifting sargassum mat is subject to winds and currents, highly variable over a wide range of space and time scales. Mats are further subject to the action of waves via ‘Stokes drift’, highly localized ‘Langmuir cells’ may also act to draw down a fraction of biomass, and the growth and mortality of sargassum will respond to variations in local temperatures and nutrient availability (Jouanno et al., 2021). With multiple processes acting on widely ranging spatial and temporal scales, forecasting of sargassum drift presents a substantial challenge. An ultimate forecasting challenge relates to the beaching process, which involves nearshore currents and transport processes, related in turn to local coastal geometry and bathymetry – all of which are typically unresolved with the observations and models used in large-scale, long-range forecasting (Oxenford et al., 2021).
A range of sargassum forecasts has emerged over the last 5 years. In the following sections, we first review these methods. We then illustrate the challenge of forecasting sargassum influx past the Lesser Antilles, with one of the newer methods, aligned with a more established forecast system. In discussion, we identify a number of key questions and issues arising from our review and analysis, leading to four recommendations, to focus the sargassum forecasting community.
Forecasting sargassum: Review of methods
Satellite observations alone underpin statistical forecasting (Wang and Hu, 2017), providing the basis for monthly Sargassum Outlook Bulletins, via the Satellite-based Sargassum Watch System (SaWS, https://optics.marine.usf.edu/projects/saws.html). Dynamical forecasts are obtained by calculating the drift of satellite-located sargassum with ocean surface currents. Short-range dynamical forecasts are based on operational ocean circulation models, e.g., SAMTool (Sargassum Monitoring Tool), which thus provides 5-day forecasts of sargassum drift (see https://datastore.cls.fr/products/samtool-sargassum-detection/). For longer-range forecasts, sargassum is advected using surface currents based on model hindcasts or climatological observations.
Building on an evaluation of observed and modelled ocean currents across the tropical Atlantic, Johnson et al. (2020) evaluated a superior skill level – for representing observed drift – with trajectories calculated using gridded climatological surface currents based on drift data sampled from the Global Drifter Program (GDP, see https://www.aoml.noaa.gov/phod/gdp/data.php), plus 0.5% ‘windage’ to represent surface flow. Due to extreme sensitivity of both modeled and observed currents to direct wind action and surface Ekman drift over the long distances and times, and uncertainty as to their validity, the simplest representation was chosen that tracked well-established drifter pathways.
Johnson et al. (2020) thus developed the tracking methodology now used to routinely obtain 3-month sargassum forecasts for the Lesser Antilles, published online in the Sargassum Sub-Regional Outlook Bulletin (https://www.cavehill.uwi.edu/cermes/projects/sargassum/outlook-bulletin.aspx), as the UWI-CERMES/USM-GCRL forecast system (henceforth referred to as the CERMES forecast). In this approach, each sargassum location in a satellite image, identified where pixels exceed a threshold value, is allocated an identification number and position. After complete trajectories have been obtained, for a given length of time, the first day of that sargassum crossing a selected meridian (60°W) is recorded in three zones that represent the three major inflow channels to the Caribbean. The total number of crossings per day provide the forecast. Improvements on this drifter forecast method are needed for transition from open ocean to island landings where neither the present drifter design nor models are adequate for guidance. As the forecast is extended to 6 months by incorporating basin-wide sargassum locations from CLS, growth and loss terms also need to be added to this simple forecast.
Sargassum forecasts are otherwise obtained from large ensembles of ‘particle’ trajectories, based on currents and winds from numerical ocean models. These model data are currently obtained from simulations with HYCOM (Chassignet et al., 2007) and NEMO (Madec, 2015) community codes, configured on eddy-resolving (typically 1/12°) global meshes, available at time frequencies ranging from daily to weekly. In early dynamical forecasts, satellite imagery and operational 7-day ocean current forecasts were used to predict sargassum drift on timescales of several days (Maréchal et al., 2017). To assess sources and pathways of sargassum, Putman et al. (2018) forward-tracked particles in ensembles released across the tropical Atlantic (0-10°N). These experiments strongly supported satellite evidence for a source region in the equatorial Atlantic and revealed how interannual variability in drift towards the Caribbean is related to dynamical changes in the North Brazil Current system. Putman et al. (2020) highlighted the strong influence on sargassum drift of the skin drag and form drag associated with prevailing winds, collectively termed ‘windage’, and the importance of correctly parameterizing this windage as a percentage of the local wind speed.
Forecast timescales of up to 6 months are becoming feasible, using a variety of drift observations and ocean model data, contingent on the availability of satellite images that detect sargassum across a sufficiently large ‘catchment area’, given timescales of advection across the tropical Atlantic. Berline et al. (2020) use satellite data from across the tropical Atlantic in 2017 to initialise sargassum distributions in an operational version of NEMO, subsequently tracked forwards with winds and currents for that year, to demonstrate the viability of drift as a determinant of sargassum distributions on timescale of up to 7 months. They find that transport processes best explain observed sargassum distributions in the eastern tropics, while distributions in the western tropics may be further explained by additional local growth.
Neglected in physical models of drift are a range of biological processes and local physical action on sargassum, likely to be important for predicting sargassum abundance on timescales beyond a few days. Depending on ambient conditions, sargassum can double in biomass on timescales of 9-20 days (Lapointe, 1986; Hanisak and Samuel, 1987). The mortality of sargassum increases with temperature above an upper threshold (around 27°C), while sinking is associated with both vertical motions in the surface layer and age/longevity of the individual sargassum thallus.
In the development of the physical-biogeochemical model, NEMO-Sarg1.0, Jouanno et al. (2021) simulate growth and loss of drifting sargassum as represented by a carbon pool, subject to surface temperature, dissolved inorganic nutrients (nitrate, phosphate) and local irradiance. Considerable uncertainty in model parameters is addressed with an ensemble approach, sampling 18 parameter values from plausible ranges about baseline values, and the system is tested for the 2017 season. Ensemble means and standard deviations of simulated sargassum biomass for selected regions compare reasonably with observations, although spring growth of biomass is early by 2-3 months in the tropical Atlantic at basin scale (10-98°W, 0-30°N) and across the Caribbean Sea at regional scale (55-85°W, 8-22°N). Some parameters are considerably more influential than others, hence their uncertainty adds more to ensemble standard deviation, and Jouanno et al. (2021) discuss scope for considerable improvement through increased model fidelity. A notable capability of NEMO-Sarg1.0 is the accommodation of wave-associated Stokes drift, neglected in most model-based forecasts, which may substantially influence sargassum distribution.
Marsh et al. (2021) combine ensemble particle tracking with the option of minimal biology, based on functions used in NEMO-Sarg1.0, relating growth and mortality to in situ temperature with baseline parameter values. In the SARTRAC-EFS system, surface winds and currents are sampled from an eddy-resolving (1/12°) NEMO hindcast spanning 1988-2010, providing a 23-member ensemble from which ensemble means and standard deviations are calculated. With a considerably simpler model system, and with much limited degree of freedom compared to NEMO-Sarg1.0, ensemble-mean hindcasts of the heavy sargassum inundations of 2015, 2018, 2019 and 2020, for the vicinity of Jamaica, closely reproduce observations at seasonal timescale. Extended to replicate CERMES forecasts, we highlight both the limits of SARTRAC-EFS and the challenges of validation.
Challenges and opportunities: Forecasting sargassum influxes to the eastern Caribbean
We focus now on the challenge and opportunity of forecasting sargassum influxes across the Lesser Antilles ‘gateway’ to the wider Caribbean and the Gulf of Mexico. In Figure 1, we illustrate some geographic contexts of the CERMES forecast system. In Figure 1A, we show March climatological surface currents, inferred from GDP drifter data, emphasizing the western boundary current system. Superimposed on these currents, we show the trajectories of four GPS trackers deployed in sargassum mats near Barbados and Jamaica in late summer and early fall of 2021, and five GDP drifters sampled across the Caribbean during 2021. While these drift data confirm general westward pathways, individual trajectories are somewhat convoluted, relative to the climatological currents, as trackers and drifters are entrained into mesoscale eddies on synoptic (days-weeks) timescales. We further annotate Figure 1A with the boxed ‘Central Atlantic’ region that provides the ‘initial’ distribution of sargassum (one of the Satellite Data Products provided by the University of South Florida, via https://optics.marine.usf.edu). This sargassum, shown here for mid-March of 2022, is tracked forwards with the CERMES forecast system.
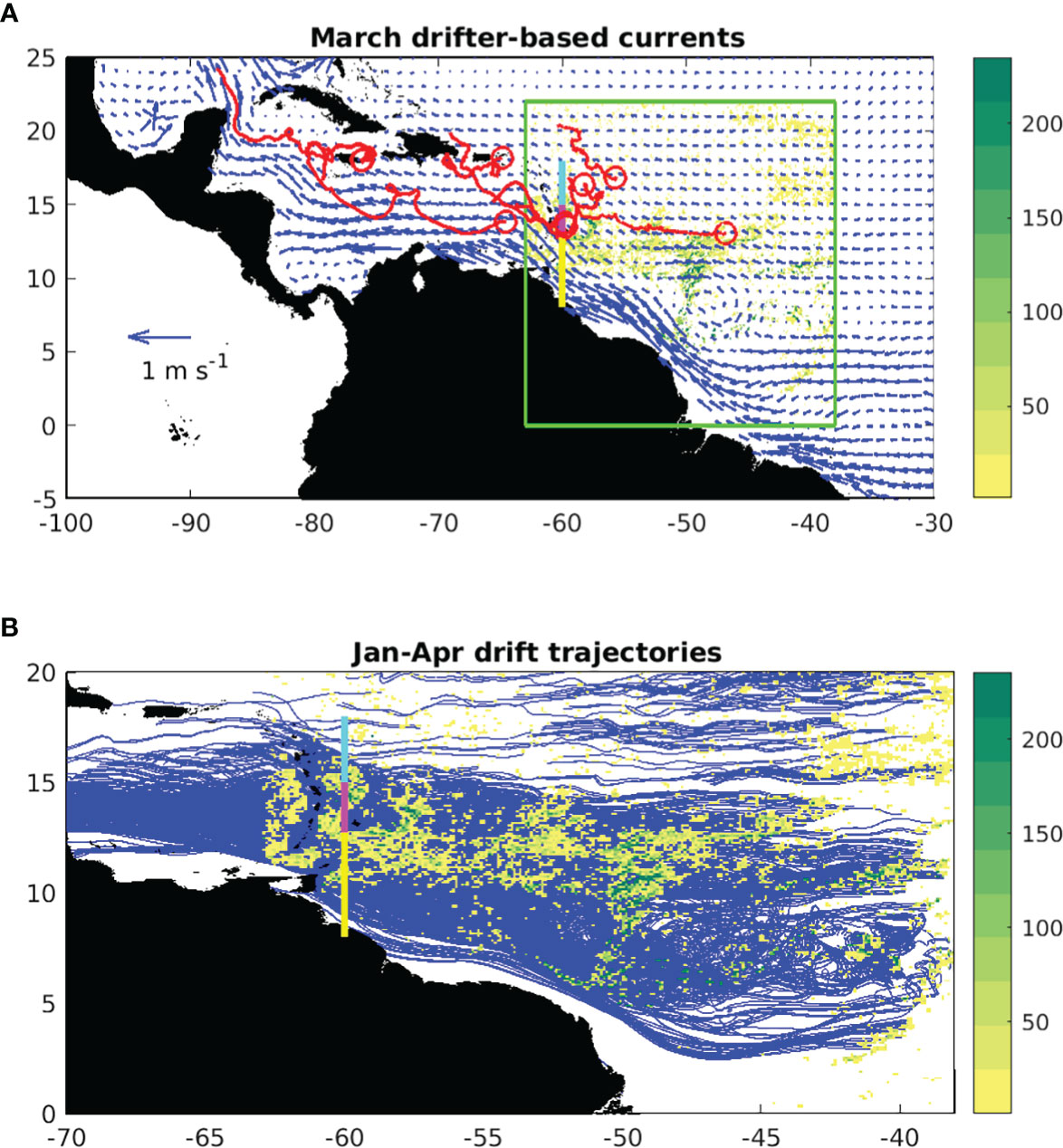
Figure 1 From the CERMES forecast system: (A) March surface currents inferred from GDP drift data, and nine example drift trajectories (in red, deployment locations indicated by circles) into and through the Caribbean, indicating the ‘Central Atlantic’ box (in green) for which week-averaged AFAI images are provided by SaWS, shown here for mid-March 2022 (non-dimensional units, colours); (B) Floating algae areal fraction from USF, observed by satellite for 12 January, from which 2188 trajectories are obtained (blue lines), each spanning 90 days. Also indicated in (A) and (B) are zones for forecasting sargassum fluxes across 60°W (‘South’, from the South American coast to 12.75°N, in yellow; ‘Middle’, 12.75-15°N, in magenta; ‘North’, 15-18°N, in cyan).
In Figure 1B, we show the drift trajectories used in the forecast spanning 12 January to 13 April (see Sargassum Sub-Regional Outlook Bulletin, Volume 2, Issue 2), indicating also the USF satellite data of 12 January, close to when the forecast is initialized. In proportion to the number of pixels in the satellite images used to initialise the January-April forecast, 2188 tracks are obtained. These tracks largely merge across the three zones at 60°W that are used for sargassum influx forecasts, also indicated in Figures 1A, B. In contrast to the convoluted and eddying drifter trajectories in Figure 1A, the forecast tracks associated with climatological currents are highly laminar.
In a step towards unifying forecasts, we align SARTRAC-EFS with the CERMES system, specifying the same 0.5% windage and without growth/loss terms, evaluated alongside satellite observations. As for CERMES, SARTRAC-EFS forecasts are obtained over 90 days, from successive mid-month initializations over January-May of 2022. In Figure 2, we map the floating algae areal fraction, observed by satellite (left panels) and in ensemble forecasts after 1 month (right panels). Note that satellite and forecast data are now aggregated in 0.5° grid cells, as outlined in Marsh et al. (2021). In so using satellite observations here, we note shortcomings associated with variable cloud cover and other factors, which limit comprehensive validation at the present time.
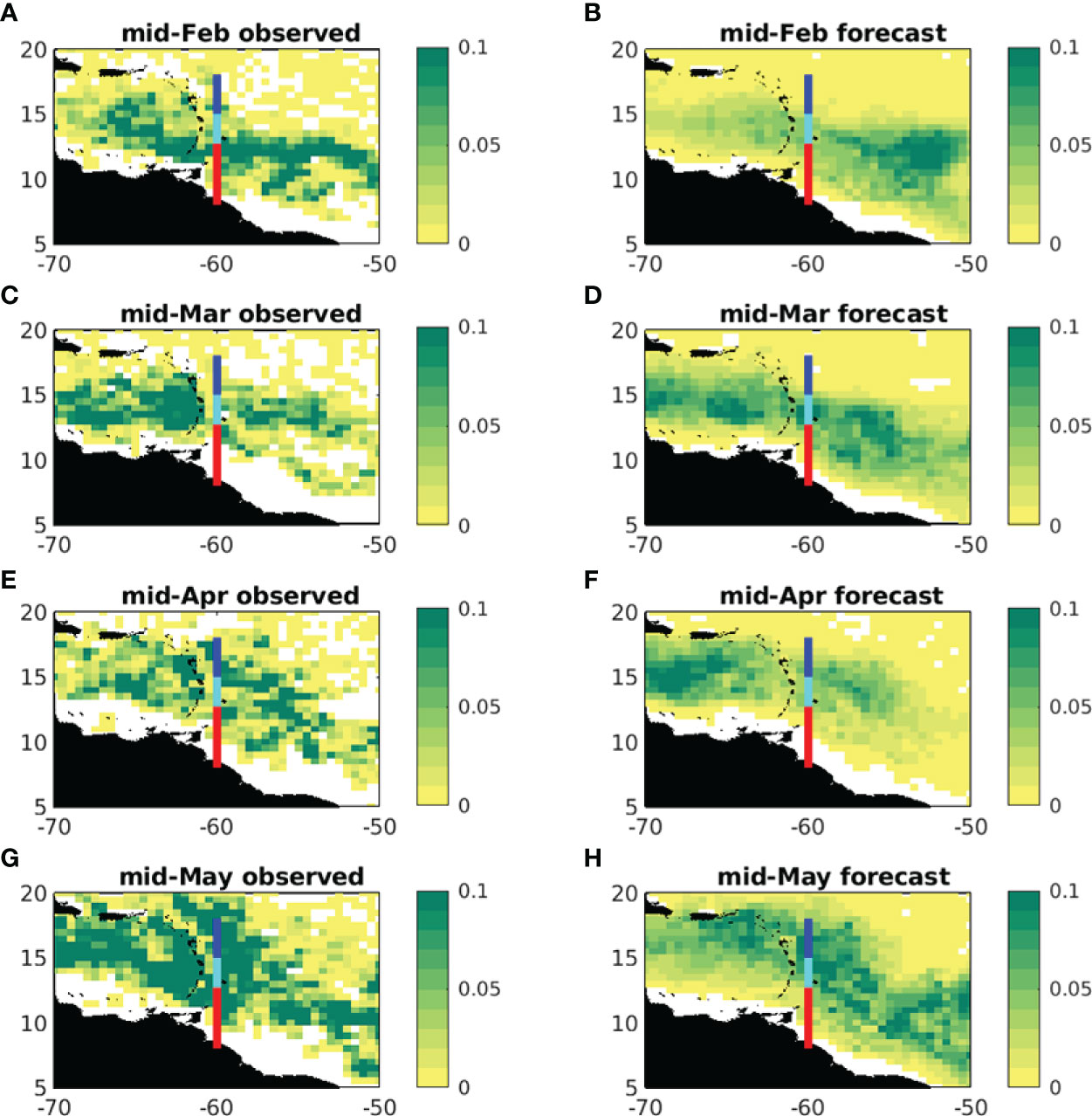
Figure 2 Floating algae areal fraction (aggregated in 0.5° grid cells), observed by satellite (left) and in ensemble forecasts after 1 month (right): (A, B) mid-February; (C, D) mid-March, (E, F) mid-April; (G, H) mid-May. Also indicated are zones for forecasting sargassum fluxes across 60°W (‘South’, from the South American coast to 12.75°N, in red; ‘Middle’, 12.75-15°N, in cyan; ‘North’, 15-18°N, in blue).
A clear contrast between observed and forecast distributions is sharper detail in the former, while an inevitable consequence of averaging 23 ensemble members is to ‘blur’ such detail in the forecasts. Considering large-scale observed features, these are reproduced with varying success in the ensemble forecasts. In mid-February, high areal fractions straddling the central part of the 60°W section (Figure 2A) are not well captured in the forecast (Figure 2B). The mid-March forecast (Figure 2D) bears closer resemblance to the observations (Figure 2C). Relatively higher areal fractions straddling the Middle and North zones in mid-April are reasonably well reproduced (Figures 2E, F). High areal fraction observed across the South zone (Figure 2G) is absent from the mid-May forecast (Figure 2H).
Aggregating SARTRAC-EFS particle fluxes across the South, Middle and North zones of 60°W, we further obtain forecasts of total sargassum flux (m2 day-1), as CERMES (not shown). Considering published CERMES forecasts issued in January, March and May of 2022 (Sargassum Sub-Regional Outlook Bulletin, Volume 2, Issues 2-4), it is evident that seasonal increases of sargassum fluxes are reliably forecast with both systems, but the CERMES forecast is better suited to prediction of major pulses. Preliminary analysis of CERMES forecasts with available field and satellite data provide some support for this tentative conclusion, although as noted above, we are currently unable to use satellite data in more comprehensive validation of these sub-regional forecasts. In summary, SARTRAC-EFS and CERMES forecasts are in reasonable qualitative agreement, although the CERMES forecasts are currently in closer agreement with the observed fluxes for this specific application.
Discussion
Our perspective on forecasting sargassum is informed by geographical contexts, processes and timescales. In reviewing different systems and addressing the prospects to refine and unify forecasts, we identify a number of key questions and issues, summarized as follows:
● Should we forecast quantities by sargassum area or influx?
● Which fixed locations, areas or transects might be defined for forecasts?
● The geographical extent and spatial resolution of satellite images determine limits on the forecast timescale for a given location, although there are prospects for improvement as basin-scale and higher resolution images become available.
● Ideally, we should include in situ growth/loss terms in Lagrangian calculations, although there is currently considerable uncertainty in the parameterization of these terms.
● Most model-based predictions lack details of beaching processes, involving tides, winds, waves and unresolved near-coastal drift related to small-scale bathymetry/geometry and residual flows
● A bespoke system such as CERMES is well designed to forecast major sargassum ‘pulses’, while SARTRAC-EFS (likewise implemented) naturally smooths these out, even within the forecast uncertainty
● The CERMES system is however currently limited to sub-regional application, largely due to shortcomings in the drifter database which compromises inferred flows through the Caribbean
● While parts of the CERMES forecast system are usefully explored and evaluated with observations, more comprehensive validation is elusive, due to limited inference of sargassum dynamics from the currently available satellite data
Elaborating on uncertainty, there is an analogue with climate forecasting, for which three sources of uncertainty have been identified: internal variability, sensitive to initial conditions; model (or structural) uncertainty; scenario uncertainty (Hawkins and Sutton, 2009). Sargassum forecasts are correspondingly subject to: uncertain initial distributions of sargassum, due to the limitations of remote sensing; uncertainty associated with limitations on the representation of processes that influence the movement, growth and loss of sargassum; uncertainty associated with the specified forcing (winds, currents, water properties), whether provided as forecasts or hindcasts.
‘Structural’ errors specific to missing processes are most notably associated with use of model data, whereas sargassum forecasts based on drift observations account for the integral effects (on drift) of multiple processes. However, on basin scales, drifter data coverage is compromised by stagnation zones created by undercurrents, river outflow and upwelling, all important to the upper ocean tropical Atlantic dynamics. In the Caribbean, a low density of drifter information in the southern portion of the basin has inhibited extension of drifter-based forecasts there. The present drifter-based forecast is also restricted to offshore waters, due to lack of understanding of the transition from deep water to coasts, which neither models nor the presently used drifters are capable of simulating.
Further structural uncertainty in forecasts based on model currents and winds to infer sargassum drift is associated with the windage factor that represents unresolved processes, including both the direct forces associated with the wind and, implicitly, the Stokes drift associated with the wave field. Several sources of uncertainty may be associated with the windage parameter:
● uncertainty in the ‘direct’ windage is related to the small extent to which a sargassum mat is exposed above the surface
● in not discriminating between wind direction and that of wave propagation, drift errors will grow with wind-wave misalignment
● sargassum mats may locally impact the wave field, of consequence for the size of Stokes drift
Apart from windage effects, a sargassum mat is subject to horizontal currents acting over the vertical extent of the largely submerged mat. While accounted for in simulations of more substantially submerged objects, such as icebergs (Merino et al., 2016), model-derived sargassum drift is typically based on surface level currents. Use of depth-integrated currents will be sensitive to the near-surface vertical resolution and hence current shear, in particular the Ekman spiral, that varies between models.
Drift (‘scenario’) uncertainty is naturally addressed with ensemble forecasts based on model data for different years (or ensemble members from an actual climate forecast). This uncertainty in forecasts based on drifter data may be quantified by sub-sampling the GDP data to obtain forecasts using an ensemble of alternative gridded currents.
While anticipated international research programmes may bring coordination to the sargassum forecasting community, some common practise may benefit both forecasters and stakeholders. We correspondingly propose four recommendations:
1. target a limited number of sargassum influxes across key meridians/sections, for local relevance
2. ideally quantify units of influx, as m2 of sargassum per day across a local transect upstream of an exposed coast, to inform deployment of local resources (for removal or harvesting)
3. where possible, provide measures of uncertainty associated with satellite data, drift, and missing processes
4. integrate forecast data with hazard exposure and vulnerability data to support impact forecasting
A concluding perspective is that forecast data should ideally be freely shared, in the public domain, to facilitate collaborative inter-comparison. Large-scale, long-range forecasts should further provide the input to local models that resolve the processes associated with beaching events, to ultimately provide more nuanced early warning that is tailored to key stakeholders in specific socio-economic sectors. Communicating the implication of forecasts is central to the successful uptake of this information by policy makers, to inform decision making.
Data availability statement
The raw data supporting the conclusions of this article will be made available by the authors, without undue reservation.
Author contributions
RM developed the manuscript and undertook the SARTRAC-EFS forecasts. DJ, SC and HO provided CERMES forecast data and contributed to the manuscript. JB developed software to auto-download FA density satellite data. All authors contributed to the article and approved the submitted version.
Funding
This publication is primarily supported by the UK Economic and Social Research Council through the Global Challenges Research Fund (GCRF) project, Teleconnected SARgassum risks across the Atlantic: building capacity for TRansformational Adaptation in the Caribbean and West Africa (SARTRAC), grant number ES/T002964/1. Further support is provided by the UK Natural Environment Research Council through the Urgency Grant project, Monitoring a large Sargassum bloom subject to a major volcanic eruption (MONISARG), grant number NE/W004798/1. We also acknowledge support from the Caribbean Biodiversity Fund (CBF) project, Adapting to a new reality: managing responses to influxes of sargassum seaweed in the Eastern Caribbean (SargAdapt), co-financed by the International Climate Initiative (IKI) of the German Federal Ministry for Environment, Nature Conservation, and Nuclear Safety through KfW.
Acknowledgments
We acknowledge the Optical Oceanography Laboratory at the University of South Florida, for freely providing FA_density images via https://optics.marine.usf.edu. In developing CERMES forecasts, we acknowledge the freely available GDP drifters from NOAA/AOML/PhOD, and winds from NOAA/OAR/ESRL PSL. The NEMO-ORCA12 hindcast simulation was carried out by the Marine Systems Modelling group at the National Oceanography Centre (NOC). The version of ARIANE particle tracking used in SARTRAC-EFS was developed by Dr George Nurser of NOC and implemented by Dr Jeff Blundell of the University of Southampton. We thank the reviewer for some insightful comments, helping us to clarify several key points.
Conflict of interest
The authors declare that the research was conducted in the absence of any commercial or financial relationships that could be construed as a potential conflict of interest.
Publisher’s note
All claims expressed in this article are solely those of the authors and do not necessarily represent those of their affiliated organizations, or those of the publisher, the editors and the reviewers. Any product that may be evaluated in this article, or claim that may be made by its manufacturer, is not guaranteed or endorsed by the publisher.
References
Berline L., Ody A., Jouanno J., Chevalier C., André J.-M., Thibaut T., et al. (2020). Hindcasting the 2017 dispersal of sargassum algae in the tropical north Atlantic. Mar. pollut. Bull. 158, 111431. doi: 10.1016/j.marpolbul.2020.111431
Brooks M. T., Coles V. J., Hood R. R., Gower J. F. R. (2018). Factors controlling the seasonal distribution of pelagic Sargassum. Mar. Ecol. Prog. Ser. 599, 1–18. doi: 10.3354/meps12646
Chassignet E. P., Hurlburt H. E., Smedstad O. M., Halliwell G. R., Hogan P. J., Wallcraft A. J., et al. (2007). The HYCOM (Hybrid coordinate ocean model) data assimilative system. J. Mar. Syst. 65, 60–83. doi: 10.1016/j.jmarsys.2005.09.016
Franks J. S., Johnson D.-S., Ko G., Sanchez-Rubio ,. R., Hendon, Lay M. (2012). Unprecedented influx of pelagic sargassum along Caribbean island coastlines during summer 2011. Proc. Gulf. Caribbean Fish. Institute 64, 6–8. Available at: http://hdl.handle.net/1834/36124
Gower J., Young E., King S. (2013). Satellite images suggest a new sargassum source region in 2011. Remote Sens. Lett. 4, 764–773. doi: 10.1080/2150704X.2013.796433
Hanisak M. D., Samuel M. A. (1987). Growth rates in culture of several species of Sargassum from Florida, USA. Hydrobiologia 151-152 (1), 399–404. doi: 10.1007/BF00046159
Hawkins E., Sutton R. (2009). The potential to narrow uncertainty in regional climate predictions. Bull. Amer. Met. Soc 90, 1095–1107. doi: 10.1175/2009BAMS2607.1
Hu C. (2009). A novel ocean color index to detect floating algae in the global oceans. Remote Sens. Environ. 113 (10), 2118–2129. doi: 10.1016/j.rse.2009.05.012
Johns E. M., Lumpkin R., Putman N. F., Smith R. H., Muller-Karger F. E., Rueda-Roa D. T., et al. (2020). The establishment of a pelagic Sargassum population in the tropical Atlantic: Biological consequences of a basin-scale long distance dispersal event. Prog. Oceanogr. 182, 102269. doi: 10.1016/j.pocean.2020.102269
Johnson D. R., Franks J. S., Oxenford H. A., Cox S. L. (2020). Pelagic sargassum prediction and marine connectivity in the tropical Atlantic. Gulf. Caribbean Res. 31 (1):GCF120-GCF130. doi: 10.18785/gcr.3101.15
Jouanno J., Benshila R., Berline L., Soulié A., Radenac M.-H., Morvan G., et al. (2021). A NEMO-based model of Sargassum distribution in the tropical Atlantic: description of the model and sensitivity analysis (NEMO-Sarg1.0). Geosci. Model. Dev. 14, 4069–4086. doi: 10.5194/gmd-14-4069-2021
Lapointe B. E. (1986). Phosphorus-limited photosynthesis and growth of Sargassum natans and Sargassum fluitans (Phaeophyceae) in the western north Atlantic. Deep-Sea Res. Part A. 33 (3), 391–399. doi: 10.1016/0198-0149(86)90099-3
Lapointe B. E., Brewton R. A., Herren L. W., Wang M., Hu C., McGillicuddy D. J. Jr., et al. (2021). Nutrient content and stoichiometry of pelagic sargassum reflects increasing nitrogen availability in the Atlantic basin. Nat. Commun. 12, 3060. doi: 10.1038/s41467-021-23135-7
Madec G. (2015). “NEMO reference manual, ocean dynamic component: NEMO-OPA. rep. 27,” in Note du ple de modlisation, institut Pierre simmon Laplace (IPSL)(France), 1288–1619.
Maréchal J. P., Hellio C., Hu C. (2017). A simple, fast, and reliable method to predict sargassum washing ashore in the lesser antilles. Remote Sens. Applications: Soc. Environ. 5, 54–63. doi: 10.1016/j.rsase.2017.01.001
Marsh R., Addo K. A., Jayson-Quashigah P.-N., Oxenford H. A., Maxam A., Anderson R., et al. (2021). Seasonal predictions of holopelagic sargassum across the tropical Atlantic accounting for uncertainty in drivers and processes: The SARTRAC Ensemble Forecast System. Front. Mar. Sci. 8. doi: 10.3389/fmars.2021.722524
Merino N., Le Sommer J., Durand G., Jourdain N. C., Madec G., Mathiot P., et al. (2016). Antarctic Icebergs melt over the southern ocean: Climatology and impact on sea ice. Ocean Model. 104, 99–110. doi: 10.1016/j.ocemod.2016.05.001
Oviatt C. A., Huizenga K., Rogers C. S., Miller W. J. (2019). What nutrient sources support anomalous growth and the recent sargassum mass stranding on Caribbean beaches? a review. Mar. pollut. Bull. 145, 517–525. doi: 10.1016/j.marpolbul.2019.06.049
Oxenford H. A., Cox S.-A., Tussenbroek B. I., Desrochers A. (2021). Challenges of turning the sargassum crisis into gold: Current constraints and implications for the Caribbean. Phycology 1 (1), 27–48. doi: 10.3390/phycology1010003
Putman N. F., Goni G. J., Gramer L. J., Hu C., Johns E. M., Trinanes J., et al. (2018). Simulating transport pathways of pelagic Sargassum from the equatorial Atlantic into the Caribbean Sea. Prog. Oceanography 165, 205–214. doi: 10.1016/j.pocean.2018.06.009
Putman N. F., Lumpkin R., Olascoaga M. J., Trinanes J., Goni G. J. (2020). Improving transport predictions of pelagic sargassum. J. Exper. Mar. Biol. Ecol. 529, 151398. doi: 10.1016/j.jembe.2020.151398
Skliris N., Marsh R., Appeaning Addo K., Oxenford H. (2022). Physical drivers of pelagic sargassum bloom interannual variability in the central West Atlantic over 2010–2020. Ocean Dynamics 72, 383–404. doi: 10.1007/s10236-022-01511-1
Wang M. Q., Hu C. M. (2016). Mapping and quantifying Sargassum distribution and coverage in the central West Atlantic using MODIS observations. Remote Sens. Environ. 183, 356–367. doi: 10.1016/j.rse.2016.04.019
Wang M. Q., Hu C. M. (2017). Predicting Sargassum blooms in the Caribbean Sea from MODIS observations. Geophysical Res. Lett. 44(7), 3265–3273. doi: 10.1002/2017GL072932
Keywords: seasonal, forecasting, sargassum, currents, winds, Antilles, Caribbean
Citation: Marsh R, Oxenford HA, Cox S-AL, Johnson DR and Bellamy J (2022) Forecasting seasonal sargassum events across the tropical Atlantic: Overview and challenges. Front. Mar. Sci. 9:914501. doi: 10.3389/fmars.2022.914501
Received: 06 April 2022; Accepted: 12 July 2022;
Published: 01 August 2022.
Edited by:
Youyu Lu, Bedford Institute of Oceanography (BIO), CanadaReviewed by:
Kai Christensen, Norwegian Meteorological Institute, NorwayCopyright © 2022 Marsh, Oxenford, Cox, Johnson and Bellamy. This is an open-access article distributed under the terms of the Creative Commons Attribution License (CC BY). The use, distribution or reproduction in other forums is permitted, provided the original author(s) and the copyright owner(s) are credited and that the original publication in this journal is cited, in accordance with accepted academic practice. No use, distribution or reproduction is permitted which does not comply with these terms.
*Correspondence: Robert Marsh, rm12@soton.ac.uk