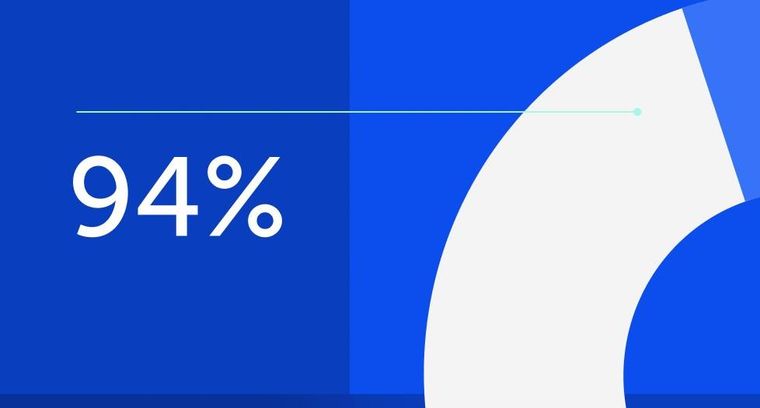
94% of researchers rate our articles as excellent or good
Learn more about the work of our research integrity team to safeguard the quality of each article we publish.
Find out more
ORIGINAL RESEARCH article
Front. Mar. Sci., 15 June 2022
Sec. Marine Biology
Volume 9 - 2022 | https://doi.org/10.3389/fmars.2022.913920
Many species, particularly marine organisms, are becoming more vulnerable to marine heatwaves due to climate change. Marine species anticipate perishing during marine heatwaves, but there is a growing interest in learning why some can resist. Using Pinctada maxima as a model species, we were able to clone a full-length cDNA encoding HSP90 with a calculated open reading frame of 2031 residues of amino acids and a molecular mass estimate of 78.08 kD to understand better the effects of marine heatwaves on the HSP90 gene expression in pearl oysters. The sequence of amino acids in P. maxima HSP90 was quite similar to the HSP90 families of Pinctada fucata martensii. At 32°C and 36°C, the expression of PmHSP90 significantly expressed and reached its highest level at 6 h, implying that in pearl oysters’ response to acute marine heatwaves, HSP90 expression rapidly increased. Pearl oysters’ temperature response was relieved, as best demonstrated by the dramatically reduced expression levels of PmHSP90 in the frequently reoccurring marine heatwaves event. Using these findings, it is possible to predict acute and repeated marine heatwaves in pearl oysters using P. maxima HSP90 as a molecular biomarker.
Since the early twentieth century, the frequency, intensity, and duration of marine heatwaves (MHWs), characterized as discrete, long-lasting, abnormally warm water episodes in the ocean, have increased significantly (Oliver et al., 2018). Amongst the most crucial elements influencing marine life and ecosystems is the combination of the atmosphere’s weather patterns and the ocean’s currents, waves, and current patterns (Hobday et al., 2016). “The blob” was a prime example of marine heatwaves in the Northeastern Pacific Ocean between 2014 and 2016. It is more than 3°C higher than the average sea surface temperature and exceeds annual SST records by four standard deviations (Miyama et al., 2021). There have been numerous MHWs events in the last decade, with devastating ecological and socioeconomic consequences, including off the coast of the northwest Atlantic, the Tasman Sea, and Western Australia (e.g., Chen et al., 2014; Oliver et al., 2017; Benthuysen et al., 2018). The shelf seas off the coast of China are among the world’s most decadent in marine life and fisheries (e.g., Belkin, 2009; Wu et al., 2012; Oliver et al., 2018; Li et al., 2019); The China’s marginal seas have been more vulnerable to and have been significantly affected by more frequent, intense, and longer-lasting sea surface temperature extremes in the past decade, as illustrated by Belkin (2009). The South China Sea, rich in fishing grounds, has recently been identified as a “hotspot” for warming oceans (e.g., Li et al., 2019; Deng et al., 2020; Yao et al., 2020). Benthic invertebrates have been decimated, and seagrass meadows (Marbà and Duarte, 2010) have been lost due to these MHWs events (Garrabou et al., 2009).
Among the most critical marine bivalve species in the tropical regions of Asia is the pearl oyster Pinctada maxima. P. maxima aquaculture and fishing have progressed slowly over the last three decades due primarily to high juvenile mortality rates (Xu et al., 2021). Furthermore, P. maxima’s pearl quality suffers greatly. Temperature, salinity, and pH fluctuations in seawater directly impact P. maxima’s biomineralization and growth rates (Deng et al., 2013; He et al., 2021). The Indo-Australian archipelago, the South China Sea, and other areas where P. maxima are found in large numbers are essential receptacles of marine biodiversity that are now under threat from MHWs (Lind et al., 2007; Yao et al., 2020).
Understanding how pearl oysters respond and adapt to pervasive seawater temperature anomalies like MHWs is critical in this era of increased ocean warming. Marine bivalves can activate various defense mechanisms in response to environmental stressors. Some of these defense mechanisms include heat shock proteins (HSPs), which can rapidly increase expression in response to stressful conditions (Gao et al., 2008). In more detail, HSPs are a protein family that cells produce in response to stress. This grouping is based on their molecular weight and sequence homology. These families include low molecular and high molecular weight HSPs (Lindquist and Craig, 1988; Heikkila, 2010). Initially, they were referred to as “heat shocks” (Ritossa, 1962). The heat shock factor is primarily responsible for inducing the dramatic regulation of HSPs during the heat shock response (Wu, 1995). As molecular chaperones, they help to prevent the deterioration of proteins and cells in the presence of stressful conditions. According to reports (Buchner, 1999), as much as 1% of the total soluble protein in some cells is HSP90. Heat stress necessitates the examination of HSP90 (Tanaka and Nakamoto, 1999). According to research (Obermann et al., 1998), HSP90’s ATPase activity is required to function in eukaryotes. It may be possible to understand the different responses of species to heatwaves by looking at how the molecular reactions allow them to acclimate to thermal stress. Some stress responses can increase the upregulation of heat shock proteins at the molecular level to protect biomolecules from thermal stress (Leung et al., 2019).
Therefore, to understand the physiological responses and protective mechanisms of P. maxima when exposed to MHWs, we explained the potential contributions of HSP90 against MHWs varying in duration, frequency, and intensity. These findings will significantly improve our knowledge of how pearl oysters react to MHWs with variable time, frequency, and severity.
Throughout the Indo-Pacific Ocean coastal regions, the pearl oyster, P. maxima, is an important bivalve species. Large-scale pearl cultivation has taken place in China and Australia in order to produce the sought-after South Sea pearl (FAO, 2014). The Indo-Australian Archipelago and the Beibu Gulf are home to a vast expanse of P. maxima, which is an important repository for marine biodiversity that is currently threatened by MHWs (Lind et al., 2007; Liu et al., 2014; Yao et al., 2020). Fishing grounds around the Beibu Gulf in the South China Sea have recently been identified as “hot spots”. Because of an increase in the severity and duration of MHWs as of late, the region has suffered greatly (Deng et al., 2020; Yao et al., 2020). There was a 26% and 14% increase in the reoccurrence and severity of MHWs impacting Beibu Gulf in 2017. As a result, the coral reefs and fisheries in the area suffered long-term damage (Zhang et al., 2020).
P. maxima were raised in cages of 30 cm x 30 cm and 0.5 m water depth 34.69, 5.25 mm length; 3.33 g weight). It was collected from Liusha Bay, South China Sea, and thoroughly cleaned off any shell surface epibionts when they arrived at the lab. On the next day, flow-through systems were used to acclimate them to lab conditions, seawater temperature of 24°C, the salinity of 31, pH 8.2, and dissolved oxygen concentration (6.0mg/L) all differed significantly between Liusha Bay’s seawater and that of the ocean. There was no death prior to the experiment.
Hobday et al. (2016) broad definition of MHWs was applied in this study to limit MHWs duration conditions. In our experiment, 24°C was used as the control group and 28, 32, and 36°C as the experimental groups. According to the report in the Beibu Gulf, the mean annual temperature is 24°C, and the other three temperature regimes are based on data collected by instruments during MHWs (Green et al., 2019; Xu et al., 2022). It was utilized through constructing two scenarios that mimicked MHWs occurrences’ ephemeral and intermittent character in the real world by employing acute and recurrent MHWs (Figure 1). 150 pearl oysters were randomly assigned to one treatment in three 50-L tanks. 50 pearl oysters were acclimated in 3 tanks and then distributed at random. Each tank was replenished every 2 or 3 days with pre-equilibrated seawater to maintain good water quality. A ratio of 20,000 Chlorella spp. cells were supplied to pearl oysters. Each tank was equipped with an electrical thermostat to maintain water temperature. The results of temperature monitoring and mortality during the experiment can be found in the study by Xu et al. (2021).
Figure 1 An illustration of the study design demonstrating MHWs events with varied frequencies and intensities. The green line represents the temperature regime specified in the control, whereas the grey, yellow, and blue lines represent the 28°C, 32°C, and 36°C temperature regimes specified under MHWs conditions, respectively.
The gills of 5 individuals from each group were chosen at random to be exposed to acute MHWs for 6 h, 12 h, 24 h, and 72 h. The same sample approach was applied for long-lasting MHWs at 3 days, 7 days, 10 days, and 14 days. After quick freezing in liquid nitrogen, store at -80°C before being analyzed.
Total RNA was extracted following the instructions of Trizol Reagent (Invitrogen, USA). A NanoDrop ND1000 UV light source spectrophotometer and a 1% agarose gel electrophoresis was used to measure the RNA’s concentration, purity, and integrity. Creating templates with (5’, 3’) RACE accompanying Amplification kit was the SMARTerTM RACE cDNA; RNaseH generates RT-qPCR cDNA templates. PmHSP90 partial sequence of was retrieved from database analyzing the transcriptome, and Primer Premier 5 software was used to designed customized primers (Table 1). Polymerase chain reaction amplification was performed to verify the intermediate fragment, and RACE technology was used to obtain 3’- and 5’-end sequences. Electrophoresis in 1% agarose gel was used to detect the amplified products. The purification and recovery kit isolated and purified target gene fragments then linked them to the, PMD19-T vector thereafter converted them to DH5 cells. LA (containing ampicillin Apm+) was used to select the positive cloned bacteria, and Sangon Biotech was used to sequence them. The PCR fragment (3’ 5’)-RACE sequences were aligned to construct the nucleotide with the complete sequence of the presumed HSP90 cDNA from pearl oysters. Before doing cluster analysis, the collected sequences were amplified to ensure accuracy.
DNAMAN spliced PmHSP90’s full-length sequence. Utilization of the NCBI ORF Finder (http://www.ncbi.nlm.nih.gov/orffinder/), amino acid sequence and ORF of PmHSP90 were predicted. Physicochemical parameters were decided using the Expasy-protparam (http://web.expasy.org/protparam/). It is used to analyze the hydrophilicity of amino acids using an online tool Expasy-ProtScale (http://web.expasy.org/protscale/). (PSITE-Search) Prosite patterns with statistical analysis can be used to predict amino acid sub-basic functional sites. SignalP4.1 predicted amino acid sequence of signal peptides, and SWISS-MODEL (https://swissmodel.expasy.org/interactive) generated the three-dimensional structure from which SMART (http://smart.embl-heidelberg.de/) was used to discover domains. PmHSP90 sequences were compared using Clustal W and NCBI BLAST was used determine the nucleotide and protein sequence similarity. The phylogenetic tree was constructed using the neighbor- joining method MEGA X software.
Total RNA was extracted using Trizol reagent according to the manufacturer’s instructions. PrimeScript RT Reagent Kit was used to generate cDNA. The RT-qPCR was performed using the Roche LightCycler 480 equipment and the SYBR(R) Ex TaqTM Premix. The comparative Ct method (2-ΔΔCt) was used to analyze the relative expression of these genes by taking β-actin as an endogenous control.
This study used SPSS 22.0 to validate the research data. Shapiro and Wilk employed these two F-tests to assess the data’s normality and homogeneity. We also utilized two-way ANOVA to investigate how different MHWs scenarios affect expression levels (P<0.05).
RACE technology cloned the cDNA of PmHSP90 gene with a 2383 bp length, a 2031 bp ORF, with 676 amino acids encoded, a (5’- 3’) UTR, 25 bp, and 352 bp respectively (Figure 2). PmHSP90 was predicted to have a 78.08 kDa molecular weight and a 4.94 theoretical isoelectric point. 76.26 as the PmHSP90 protein aliphatic index, and 34.69 as the instability index, suggesting that it is a stable protein, according to ExPASy-ProtParam analysis. PmHSP90 has an ATP/GTP-binding site (IGQFGVGFYSAYLVAD). According to predicted amino acid sub-basic functional sites, three HSP90 protein family signatures (LGTIAKSGT, IKLYVRRVFI, GVVDSEDLPL) and the characteristic cytoplasmic motif MEEVD were identified. A hydrophilic protein’s average coefficient of hydrophilicity (GRAVY) is -0.811. PmHSP90 has an HSP90 domain at positions 142- 667, according to SMART analysis (Figure 2).
Figure 2 Pm-HSP90 nucleotide sequence analysis. The 5’ and 3’ UTRs are denoted by small letters, while the deduced amino acid sequences are indicated by capital letters. The 5’-UTR start codon was in a box, and the stop codons (TAA) were labeled with an asterisk (*). The HSP90 characteristic motif was highlighted on a yellow background, and the underlined indicates the HSP90 domain.
Table 1s displays the species information from the various alignments and the phylogenetic tree. Based on amino acid sequence alignment, the multiple sequence alignment of PmHSP90 as well as other bivalves HSP90 reveals that HSP90 has substantial homology among bivalves. Pinctada fucata martensii HSP90 has 95.41 percent homology with PmHSP90 (Figure 3). According to the evolutionary tree, PmHSP90 patterns with bivalves mostly resembling P. fucata martensii and P. penguin, whereas mammals, and fish are further away (Figure 4).
Figure 3 The deduced amino acid sequences of HSP90 from P. maxima and other organisms were aligned multiple times. Black indicates complete homology, grey indicates homology more significant than 80%, and white indicates homology greater than 60%.
Figure 4 A phylogenetic tree based on amino acid sequences deduced from the HSP90 gene of Pinctada maxima and other species, constructed using the neighbor-joining method. “Pinctada maxima” was labeled “▲”. Table 2 displays the GenBank accession numbers for all HSP90.
PmHSP90 distribution and expression levels were assessed in the tissues using RT-qPCR in normal conditions. Hepatopancreas, and gills had the higher levels of PmHSP90 expression, respectively, in P. maxima, while the adductor muscle had the lowest levels of PmHSP90 expression (Figure 5).
Figure 5 PmHSP90 mRNA expression in different tissues. Significant differences (P<0.05) are indicated by other capital letters. Adductor muscle (A), gills (Gi), hepatopancreas (He), and mantle (M).
When comparing the experimental groups to the control groups during the acute exposure, the expression level in gills was significantly higher in the groups, notably those at 36°C.In the 28°C groups, the expression level of PmHSP90 reached its maximum at 24 h after being stimulated by the MHWs; in the 32°C groups, it reached its maximum at 6 h after being stimulated by the MHWs (Figure 6). The analysis of variance results demonstrated how respectively the temperature of the MHWs and the duration of stress had a significant impact on gene expression (P<0.05), and that their combination had a significant impact (P<0.05) as elaborated in (Table 2). Upon reoccurring MHWs exposure, revealed that the level of HSP90 expression was lower than that observed in the first MHWs, as well as the fold alterations between experimental and control groups were less than in the initial MHWs (Figure 7). The analysis of variance demonstrated, the MHWs’ temperature as well as the period of stress had a significant impact on gene expression (P<0.05), as did their interaction (P<0.05) (Table 2).
Figure 6 The expression of PmHSP90 mRNA in P. maxima gills after acute MHWs event. capital letters indicate significant differences between four temperature treatments (P<0.05), and small letters indicate significant differences between four temperature levels each time. The experiment was halted because pearl oysters exposed to a temperature anomaly of 36°C suffered mortality after 24 h.
Table 2 The relative expression of HSP90 in pearl oysters exposed to acute and recurrent MHWs scenarios was studied using two-way ANOVA.
Figure 7 The expression levels of PmHSP90 mRNA in P. maxima gills following repeated exposure to MHWs event. Capital letters represent significant variations between distinct periods within each temperature treatment, whereas small letters dnote significant differences within each interval of time, between four distinct temperatures (P<0.05). It was discontinued after 24 hours because pearl oysters exposed to a 36°C temperature anomaly suffered mortality.
Recently, the intense, frequent, and longer-lasting MHWs have been emerging as a significant threat to marine bivalves and the ecosystems they support (Deng et al., 2020). Previous studies have demonstrated the impacts of MHWs on pearl oysters and indicated the rapid acclimation of this species to various MHWs events (He et al., 2021; Xu et al., 2021; Xu et al., 2022). The rarity of heatwaves in nature has limited research into how they affect marine ecosystems, but what is known is that they have significant effects on marine life in a relatively short time. Nonetheless, organisms’ adaptive and recovery capacities, which allow them to cope with thermal stress such as oxygen consumption, and filtration rate (Viergutz et al., 2007; Zhao et al., 2019; Marroni et al, 2021), have been largely overlooked (Leung et al., 2019). In the current study, we used RACE technology to clone the full-length PmHSP90. The expression pattern of HSP90 molecular mechanisms responses of pearl oysters to MHWs was analyzed.
The quantification of the expression pattern analysis of HSP90 was revealed in the adductor muscle, gills, hepatopancreas, and mantle tissues of P. maxima under no MHWs condition (Figure 5). HSP90 was commonly expressed in all examined tissues, implying that HSP90 was necessary for cellular balance. However, the extent to which they were told differed by tissue (Rungrassamee et al., 2010). There was primarily an expression of HSP90 in the hepatopancreas and gills. The level of expression significantly increased, and this matched the study’s findings of Cheng et al. (2019), explaining that the gills are both respiratory organs and feeding organs because bivalves are filter feeders; as a result, they are better able to detect changes in their surroundings than other tissues. In addition to creating reactive oxygen species and xenobiotic detoxification, the hepatopancreas plays a vital role in immunological defense, digestion, absorption of nutrients, and the release of hormones (Chaurasia et al., 2016; Park et al., 2020). Because of its metabolic activity, the hepatopancreas is susceptible to environmental alterations (Rungrassamee et al., 2010; Qian et al., 2012). However, gill tissue was further explored because the gills are involved with both respiration and ciliary suspension feeding hence exploring the gills tissue under acute and recurrent MHWs could fully investigate HSP90 as a biomarker for MHWs.
In the current study, we examined the analysis of the expression pattern of HSP90 in P. maxima under acute (Figure 6) and recurrent (Figure 7) MHWs scenarios. We found a significant upregulation in the expression levels of HSP90 over the entire course of MHWs compared to the control period, revealing that HSP90 plays a vital role in indicating organisms that are going through stress. HSP90 is a chaperone that plays a role in several processes. The up-regulation of HSP90 is a defense mechanism because HSP90 could be attached to misfolded or damaged proteins to return them to their shape in their originality (Ramaglia, 2004; Gao et al., 2008).
Currently, there is HSP90 relative expression at different time points in gill tissue during acute MHWs. After exposure to thermal stress at 36°C, up-regulation of HSP90 was identified in the gills within the first 6 h, maximum expression level peaked at 6 h after the heat exposure, and in contrast, after the heat exposure at 12h–72h (Figure 6), HSP90 expression declined continuously in the gills, A similar study explains that it could be as a result of modifying biochemical reactions and energy resource allocations involved in protein synthesis, such as catalase and other HSP (Lin et al., 2018). This expression indicates that HSP90 may be vital in responding to acute temperature stress. Protein folding relies on HSP90, one of the systems that protect eukaryotic and prokaryotic cells from heatwave stress. (Wandinger et al., 2008). Previous research has also revealed similar trends in the data in crustacean heat shock proteins (HSPs) such as HSP90 of Fenneropenaeus chinensis, HSP90 of Penaeus monodon HSP40, and HSP90 of Marsupenaeus japonicus shown to have thermal tolerance (Ju-Ngam et al., 2021). Farcy et al. (2007) reported that environmental temperature fluctuations could activate stress responses in muscle cells. Kim et al. (2009) also explained that there were up-regulation patterns of HSP90 when subjected to acute temperature elevation. To the extent that heat stress reaction to MHWs was alleviated, implying that P. maxima can adapt quickly to heat stress after acute MHWs exposure (He et al., 2021; Xu et al., 2021; Xu et al., 2022).
A high-temperature environment stimulates a change in the relative expression of HSP90 in P. maxima. HSP90 in the gills of P. maxima showed to increase significantly after three days of repeated heat stress, but the HSP90 in the gills decreased rapidly when the duration of heat stress was prolonged after three days (Figure 7). The findings are consistent with those of Rungrassamee et al. (2010), which reported that HSP90 expression steadily decreased to the same level as in the untreated condition during the recovery phase following heatwave stress. The relative expression of LvHSP90 increased at 6 h, peaked at 24 h, and then fell progressively to the control at 96 h. (Qian et al., 2012). These results indicate that the heat tolerance of the gills of P. maxima is time-limited and that it induces HSP90 expression to alleviate heat stress. In a previous study, high temperatures could cause the synthesis of HSP90 mRNA and HSP90 protein to protect cells (Lei et al., 2009). However, this long-term stress may result in permanent cell damage. P. maxima response during constant exposure and recovery from MHWs suggest that metabolic exchange is essential in stress responses and acclimatization (He et al., 2021). However, it is pretty costly to maintain protein structure under pressure; increased HSP expression in long-term stress has repercussions (Sørensen et al., 2003). Its chronic heat stress has been shown to cause downregulation patterns detected in aposymbiotic larvae (Meyer et al., 2011).
Furthermore, heat stress accelerates metabolism. Thus, more ATP might have led to a continuation of HSP90 consumption. HSP90 protein expression is reduced when the consumption of HSP90 is greater than the synthesis of HSP90 (Yu et al., 2021). Interestingly, further research on the molecular basis of how HSP90 was activated under heat stress revealed that trans activations of heat shock proteins were mediated via the heat shock factor 1 (HSF1) and heat shock elements (HSE) interactions (Wu, 1995). Inactive HSF1 is localized in the cytosol, but upon heat stress (Kim et al., 2005), translocates to the nucleus and is directly coupled to large structural rearrangements in HSP90 regulated through inhibition, HSF1 activation increases HSP90 expression (Lackie et al., 2017). For some reason, HSF1 appears to be released from the activator by HSP90 when the temperature rises slightly (Fabbri et al., 2008). This demonstrates that HSP90 could be further explored under combined stressors such as MHWs and hypoxia.
The current study also opens up the possibility of a future investigation of transgenerational effects as a result of HSP90 expression in different tissues under MHWs being inherited as a result of persistent exposure, which is based on a recent review by Zhao et al. (2020), according to which acidic exposure causes a considerable increase in metabolism in clams with no parental exposure to low pH, possibly shifting the energy requirement for calcification and slowing shell growth (Leung et al., 2020). The current study paves the way for further research into the combined consequences of global warming, MHWs, and ocean acidification on bivalves (Wang et al., 2015; Zhao et al., 2018). More research is needed to understand better how MHWs and ocean acidification interacts to harm individual organisms and the entire ecosystem. This knowledge could also lead to further research on bivalve plasticity, particularly P. maxima, in the heat shock response to MHWs. In one of the few cases, Mytilus edulis transplanted from the Baltic to the North Sea showed stress protein levels similar to North Sea mussels (Tedengren et al., 1999). Experimenting with Nucella ostrina whelks in experimental cages exposed and protected from waves and a location with reduced quality of prey revealed HSP70 expression that changed over time and between different ecosystems and locations (Dahlhoff et al., 2001). The above mentioned future explorations could lead to a better understanding and new scientific discovery of how MHWs impacts bivalve species, P.maxima in particular.
The study concludes that HSP90 was expressed in different tissues of the pearl oyster P. maxima HSP90 was directly implicated in the sensitivity to temperature fluctuations. The analysis of the HSP90 expression pattern in P. maxima under acute and recurrent MHWs studies revealed the production of HSP90 in P. maxima gill tissues, which aids in acclimation and survival against MHWs. The distribution of the PmHSP90 expression provides essential information for analyzing its biological function in P. maxima. HSP90 expression in P. maxima is a possible biomarker because it can be utilized as a physical assessment in stress risk prediction. Early stress identification allows for better therapy selection and evaluation of therapeutic approaches. After all, MHWs will continue to influence ecosystems regardless of whether steps are taken to address some of their causes. It is critical to understand how pearl oysters will respond to future environmental stressors such as MHWs.
The datasets presented in this study can be found in online repositories. The names of the repository/repositories and accession number(s) can be found in the article/Supplementary Material.
FM: Methodology, Investigation, Writing- Original draft preparation YX: Methodology, Investigation. GH: Methodology. FL: Methodology. XL: Methodology, Formal analysis. KY: Resources, Formal analysis. RM: Methodology. JL: Methodology. YD: Conceptualization. LZ: Conceptualization, Writing-original draft preparation, Project administration. All authors contributed to the article and approved the submitted version.
The authors declare that the research was conducted without any commercial or financial relationships that could be construed as a potential conflict of interest.
All claims expressed in this article are solely those of the authors and do not necessarily represent those of their affiliated organizations, or those of the publisher, the editors and the reviewers. Any product that may be evaluated in this article, or claim that may be made by its manufacturer, is not guaranteed or endorsed by the publisher.
The present study has been made possible by research grants from the Guangxi Innovation Driven Development Specific Fund (AA19254032), the National Science Foundation of China (42076121, M-0163), the Department of Education of Guangdong Province (2020KTSCX050), the Starting Research Fund from Guangdong Ocean University (R20083), and the earmarked fund for Modern Agro-industry Technology Research System (CARS-49).
The Supplementary Material for this article can be found online at: https://www.frontiersin.org/articles/10.3389/fmars.2022.913920/full#supplementary-material
Belkin I. M. (2009). Rapid Warming of Large Marine Ecosystems. Prog. Oceanog. 81, 207–213. doi: 10.1016/j.pocean.2009.04.011
Benthuysen J. A., Oliver E. C., Feng M., Marshall A. G. (2018). Extreme Marine Warming Across Tropical Australia During Austral Summer 2015–2016. J. Geophys. Research: Ocean. 123, 1301–1326. doi: 10.1002/2017JC013326
Buchner J. (1999). HSP90 & Co.–a Holding for Folding. Trends Biochem. Sci. 24, 136–141. doi: 10.1016/S0968-0004(99)01373-0
Chaurasia M. K., Nizam F., Ravichandran G., Arasu M. V., Al-Dhabi N. A., Arshad A., et al. (2016). Molecular Importance of Prawn Large Heat Shock Proteins 60, 70 and 90. Fish. shellfish Immunol. 48, 228–238. doi: 10.1016/j.fsi.2015.11.034
Cheng D., Liu H., Zhang H., Soon T. K., Ye T., Li S., et al. (2019). Differential Expressions of HSP70 Gene Between Golden and Brown Noble Scallops Chlamys nobilis Under Heat Stress and Bacterial Challenge. Fish Shellfish Immuno. 94, 924–933. doi: 10.1016/j.fsi.2019.10.018
Chen F., Yang X., Zhu W. (2014). WRF Simulations of Urban Heat Island Under Hot-Weather Synoptic Conditions: The Case Study of Hangzhou City, China. Atmospher. Res. 138, 364–377. doi: 10.1016/j.atmosres.2013.12.005
Dahlhoff E. P., Buckley B. A., Menge B. A. (2001). Physiology of the Rocky Intertidal Predator Nucella Ostrina Along an Environmental Stress Gradient. Ecology 82, 2816–2829. doi: 10.1890/0012-9658(2001)082[2816:POTRIP]2.0.CO;2
Deng Y., Fu S., Liang F., Du X., Xie S. (2013). Growth and Survival of Pearl Oyster Pinctada Maxima Spat Reared Under Different Environmental Conditions. J. Shellfish Res. 32, 675–679. doi: 10.2983/035.032.0308
Deng K., Jiang X., Hu C., Chen D. (2020). More Frequent Summer Heatwaves in Southwestern China Linked to the Recent Declining of Arctic Sea Ice. Environ. Res. Lett. 15, 074011. doi: 10.1088/1748-9326/ab8335
Fabbri E., Valbonesi P., Franzellitti S. (2008). HSP Expression in Bivalves. Invertebrate Survival J. 5, 135–161.
FAO (2014). Fishery and Aquaculture Statistics Vol. 11 (Rome: Food and Agriculture Organization of the United Nations).
Farcy E., Serpentini A., Fiévet B., Lebel J. M. (2007). Identification of cDNAs Encoding HSP70 and HSP90 in the Abalone Haliotis Tuberculata: Transcriptional Induction in Response to Thermal Stress in Hemocyte Primary Culture. Comp. Biochem. Physiol. Part B: Biochem. Mol. Biol. 146, 540–550. doi: 10.1016/j.cbpb.2006.12.006
Gao Q., Zhao J., Song L., Qiu L., Yu Y., Zhang H., et al. (2008). Molecular Cloning, Characterisation, and Expression of Heat Shock Protein 90 Genes in the Haemocytes of Bay Scallop Argopecten Irradians. Fish. Shellfish Immunol. 24, 379–385. doi: 10.1016/j.fsi.2007.08.008
Garrabou J., Coma R., Bensoussan N., Bally M., Chevaldonné P., Cigliano M., et al. (2009). Mass Mortality in Northwestern Mediterranean Rocky Benthic Communities: Effects of the 2003 Heatwave. Global Change Biol. 15, 1090–1103. doi: 10.1111/j.1365-2486.2008.01823.x
Green T. J., Siboni N., King W. L., Labbate M., Seymour J. R., Raftos D. (2019). Simulated Marine Heat Wave Alters Abundance and Structure of Vibrio Populations Associated With the Pacific Oyster Resulting in a Mass Mortality Event. Microbial. Ecol. 77 (3), 736–747. doi: 10.1007/s00248-018-1242-9
Heikkila J. J. (2010). Heat Shock Protein Gene Expression and Function in Amphibian Model Systems. Comp. Biochem. Physiol. Part A: Mol. Integr. Physiol. 156, 19–33. doi: 10.1016/j.cbpa.2010.01.024
He G., Liu X., Xu Y., Liang J., Deng Y., Zhang Y., et al. (2021). Repeated Exposure to Simulated Marine Heatwaves Enhances the Thermal Tolerance in Pearl Oysters. Aquat. Toxicol. 239, 105959. doi: 10.1016/j.aquatox.2021.105959
Hobday A. J., Alexander L. V., Perkins S. E., Smale D. A., Straub S. C., Oliver E. C. J., et al. (2016). A Hierarchical Approach to Defining Marine Heatwaves. Prog. Oceanog. 141, 227–238. doi: 10.1016/j.pocean.2015.12.014
Ju-Ngam T., McMillan N., Yoshimizu M., Kasai H., Wongpanya R., Srisapoome P. (2021). Functional and Stress Response Analysis of Heat Shock Proteins 40 and 90 of Giant River Prawn (Macrobrachium Rosenbergii) Under Temperature and Pathogenic Bacterial Exposure Stimuli. Biomolecules 11, 1034. doi: 10.3390/biom11071034
Kim M., Ahn I. Y., Kim H., Cheon J., Park H. (2009). Molecular Characterisation and Induction of Heat Shock Protein 90 in the Antarctic Bivalve Laternula Elliptica. Cell Stress Chaperones 14, 363–370. doi: 10.1007/s12192-008-0090-9
Kim S. A., Yoon J. H., Lee S. H., Ahn S. G. (2005). Polo-Like Kinase 1 Phosphorylates Heat Shock Transcription Factor 1 and Mediates its Nuclear Translocation During Heat Stress. J. Biol. Chem. 280 (13), 12653–12657. doi: 10.1074/jbc.M411908200
Lackie R. E., Maciejewski A., Ostapchenko V. G., Marques-Lopes J., Choy W. Y., Duennwald M. L., et al. (2017). The Hsp70/HSP90 Chaperone Machinery in Neurodegenerative Diseases. Front. Neurosci. 11, 254. doi: 10.3389/fnins.2017.00254
Lei L., Yu J., Bao E. (2009). Expression of Heat Shock Protein 90 (HSP90) and Transcription of its Corresponding mRNA in Broilers Exposed to High Temperature. Br. poultry Sci. 50, 504–511. doi: 10.1080/00071660903110851
Leung J. Y. S., Bayden B. D., Connell S. D. (2019). Adaptive Responses of Marine Gastropods to Heatwaves. One Earth 1, 374–381. doi: 10.1016/j.oneear.2019.10.025
Leung J. Y. S., Russell B. D., Connell S. D. (2020). Linking Energy Budget to Physiological Adaptation: How a Calcifying Gastropod Adjusts or Succumbs to Ocean Acidification and Warming. Sci. Total Environ. 715, 136939. doi: 10.1016/j.scitotenv.2020.136939
Lind C. E., Evans B. S., Taylor J. J., Jerry D. R. (2007). Population Genetics of a Marine Bivalve, Pinctada Maxima, Throughout the Indo-Australian Archipelago Shows Differentiation and Decreased Diversity at Range Limits. Mol. Ecol. 16, 5193–5203. doi: 10.1111/j.1365-294X.2007.03598.x
Lindquist S., Craig E. A. (1988). The Heat-Shock Proteins. Annu. Rev. Genet. 22, 631–677. doi: 10.1146/annurev.ge.22.120188.003215
Lin X., Wu X., Liu X. (2018). Temperature Stress Response of Heat Shock Protein 90 (HSP90) in the Clam Paphia Undulata. Aquacult. fish. 3, 106–114. doi: 10.1016/j.aaf.2018.04.003
Li Y., Ren G., Wang Q., You Q. (2019). More Extreme Marine Heatwaves in the China Seas During the Global Warming Hiatus. Environ. Res. Lett. 14, 104010. doi: 10.1088/1748-9326/ab28bc
Liu G., Heron S. F., Mark Eakin C., Muller-Karger F. E., Vega-Rodriguez M., Guild L. S., et al (2014). Reef-Scale Thermal Stress Monitoring of Coral Ecosystems: New 5-km global products from NOAA coral reef watch. Remote Sens. 6, 11579–11606. doi: 10.3390/rs61111579
Marbà N., Duarte C. M. (2010). Mediterranean Warming Triggers Seagrass (Posidonia Oceanica) Shoot Mortality. Global Change Biol. 16, 2366–2375. doi: 10.1111/j.1365-2486.2009.02130.x
Marroni S., Mazzeo N., Iglesias C. (2021). Effects of Temperature and Food Availability on the Filtration and Excretion Rates of Diplodon Parallelopipedon (Hyriidae). Int. Rev. Hydrobio. 106 (5-6), 249–258. doi: 10.1002/iroh.202002066
Meyer E., Aglyamova G. V., Matz M. V. (2011). Profiling Gene Expression Responses of Coral Larvae (Acropora Millepora) to Elevated Temperature and Settlement Inducers Using a Novel RNA-Seq Procedure. Mol. Ecol. 20, 3599–3616. doi: 10.1111/j.1365-294X.2011.05205.x
Miyama T., Minobe S., Goto H. (2021). Marine Heatwave of Sea Surface Temperature of the Oyashio Region in Summer in 2010–2016. Front. Mar. Sci. 7, 576240. doi: 10.3389/fmars.2020.576240
Obermann W. M., Sondermann H., Russo A. A., Pavletich N. P., Hartl F. U. (1998). In Vivo Function of HSP90 is Dependent on ATP Binding and ATP Hydrolysis. J. Cell Biol. 143, 901–910. doi: 10.1083/jcb.143.4.901
Oliver E. C., Benthuysen J. A., Bindoff N. L., Hobday A. J., Holbrook N. J., Mundy C. N., et al. (2017). The Unprecedented 2015/16 Tasman Sea Marine Heatwave. Nat. Commun. 8 (1), 1–12. doi: 10.1038/ncomms16101
Oliver E. C. J., Lago V., Hobday A. J., Holbrook N. J., Ling S. D., Mundy C. N. (2018). Marine Heatwaves Off Eastern Tasmania: Trends, Interannual Variability, and Predictability. Prog. Oceanog. 161, 116–130. doi: 10.1016/j.pocean.2018.02.007
Park K., Kim W. S., Kwak I. S. (2020). Effects of Di-(2-Ethylhexyl) Phthalate on Transcriptional Expression of Cellular Protection-Related HSP60 and HSP67B2 Genes in the Mud Crab Macrophthalmus Japonicus. Appl. Sci. 10 (8), 2766. doi: 10.3390/app10082766
Qian Z., Liu X., Wang L., Wang X., Li Y., Xiang J., et al. (2012). Gene Expression Profiles of Four Heat Shock Proteins in Response to Different Acute Stresses in Shrimp, Litopenaeus Vannamei. Comp. Biochem. Physiol. Part C: Toxicol. Pharmacol. 156, 211–220. doi: 10.1016/j.cbpc.2012.06.001
Ramaglia V. (2004). Expression of Heat Shock Proteins 70 and 90 in Tissues of the Western Painted Turtle Following Bacterial Infection and Anoxia. Master thesis, University of Toronto.
Ritossa F. (1962). A New Puffing Pattern Induced by Temperature Shock and DNP in Drosophila. Experientia 18, 571–573. doi: 10.1007/BF02172188
Rungrassamee W., Leelatanawit R., Jiravanichpaisal P., Klinbunga S., Karoonuthaisiri N. (2010). Expression and Distribution of Three Heat Shock Protein Genes Under Heat Shock Stress and Under Exposure to Vibrio Harveyi in Penaeus Monodon. Dev. Comp. Immunol. 34, 1082–1089. doi: 10.1016/j.dci.2010.05.012
Sørensen J. G., Kristensen T. N., Loeschcke V. (2003). The Evolutionary and Ecological Role of Heat Shock Proteins. Ecol. Lett. 6, 1025–1037. doi: 10.1046/j.1461-0248.2003.00528.x
Tanaka N., Nakamoto H. (1999). HtpG is Essential for the Thermal Stress Management in Cyanobacteria. FEBS Lett. 458, 117–123. doi: 10.1016/S0014-5793(99)01134-5
Tedengren M., Olsson B., Bradley B., Zhou L. (1999). “Heavy Metal Uptake, Physiological Response, and Survival of the Blue Mussel (Mytilus Edulis) From Marine and Brackish Waters in Relation to the Induction of Heat-Shock Protein 70,” in Biological, Physical and Geochemical Features of Enclosed and Semi-Enclosed Marine Systems (Dordrecht: Springer), 261–269.
Viergutz C., Kathol M., Norf H., Arndt H., Weitere M. (2007). Control of Microbial Communities by the Macrofauna: A Sensitive Interaction in the Context of Extreme Summer Temperatures. Oecologia 151, 115–124. doi: 10.1007/s00442-006-0544-7
Wandinger S. K., Richter K., Buchner J. (2008). The HSP90 Chaperone Machinery. J. Biol. Chem. 283, 18473–18477. doi: 10.1074/jbc.R800007200
Wang Y., Li L., Hu M., Lu W. (2015). Physiological Energetics of the Thick Shell Mussel Mytilus Coruscus Exposed to Seawater Acidification and Thermal Stress. Sci. Total Environ. 514, 261–272. doi: 10.1016/j.scitotenv.2015.01.092
Wu C. (1995). Heat Shock Transcription Factors: Structure and Regulation. Annu. Rev. Cell Dev. Biol. 11, 441–469. doi: 10.1146/annurev.cb.11.110195.002301
Wu L., Cai W., Zhang L., Nakamura H., Timmermann A., Joyce T., et al. (2012). Enhanced Warming Over the Global Subtropical Western Boundary Currents. Nat. Climate Change 2, 161–166. doi: 10.1038/nclimate1353
Xu Y., Liang J., Liu X., Yang K., Masanja F., Deng Y., et al. (2022). Responses of Pearl Oysters to Marine Heatwaves as Indicated by HSP70. Front. Mar. Sci. 9, 847585. doi: 10.3389/fmars.2022.847585
Xu Y., Zhang Y., Liang J., He G., Liu X., Zheng Z., et al. (2021). Impacts of Marine Heatwaves on Pearl Oysters are Alleviated Following Repeated Exposure. Mar. Pollution Bull. 173, 112932. doi: 10.1016/j.marpolbul.2021.112932
Yao Y., Wang J., Yin J., Zou X. (2020). Marine Heatwaves in China’s Marginal Seas and Adjacent Offshore Waters: Past, Present, and Future. J. Geophys. Research: Ocean. 125, e2019JC015801. doi: 10.1029/2019JC015801
Yu Z. Q., Tian J. Y., Wen J., Chen Z. (2021). Effects of Heat Stress on Expression of Heat Shock Proteins in the Small Intestine of Wenchang Chicks. Braz. J. Poultry Sci. 23, 1–8. doi: 10.1590/1806-9061-2020-1430
Zhang W., Zheng Z., Zhang T., Chen T. (2020). Strengthened Marine Heatwaves Over the Beibu Gulf Coral Reef Regions From 1960 to 2017. Haiyang Xuebao 42, 41–49. doi: 10.3969/j.issn.0253-4193.2020.05.005
Zhao L., Liu L., Liu B., Liang J., Lu Y., Yang F. (2019). Antioxidant Responses to Seawater Acidification in an Invasive Fouling Mussel are Alleviated by Transgenerational Acclimation. Aquat. Toxicol. 217, 10533. doi: 10.1016/j.aquatox.2019.105331
Zhao L., Shirai K., Tanaka K., Milano S., Higuchi T., Murakami-Sugihara N., et al. (2020). A Review of Transgenerational Effects of Ocean Acidification on Marine Bivalves and Their Implications for Sclerochronology. Estuar. Coast. Shelf Sci. 235, 106620. doi: 10.1016/j.ecss.2020.106620
Keywords: extreme weather events, marine heatwaves, biomarker, heat shock proteins, bivalve mollusks
Citation: Masanja F, Xu Y, He G, Liang F, Liu X, Yang K, Mkuye R, Liang J, Deng Y and Zhao L (2022) Exploring HSP90 as a Biomarker for Marine Heatwaves in Pinctada maxima. Front. Mar. Sci. 9:913920. doi: 10.3389/fmars.2022.913920
Received: 06 April 2022; Accepted: 17 May 2022;
Published: 15 June 2022.
Edited by:
Menghong Hu, Shanghai Ocean University, ChinaCopyright © 2022 Masanja, Xu, He, Liang, Liu, Yang, Mkuye, Liang, Deng and Zhao. This is an open-access article distributed under the terms of the Creative Commons Attribution License (CC BY). The use, distribution or reproduction in other forums is permitted, provided the original author(s) and the copyright owner(s) are credited and that the original publication in this journal is cited, in accordance with accepted academic practice. No use, distribution or reproduction is permitted which does not comply with these terms.
*Correspondence: Liqiang Zhao, bHpoYW9AZ2RvdS5lZHUuY24=
†These authors contributed equally to this work
Disclaimer: All claims expressed in this article are solely those of the authors and do not necessarily represent those of their affiliated organizations, or those of the publisher, the editors and the reviewers. Any product that may be evaluated in this article or claim that may be made by its manufacturer is not guaranteed or endorsed by the publisher.
Research integrity at Frontiers
Learn more about the work of our research integrity team to safeguard the quality of each article we publish.