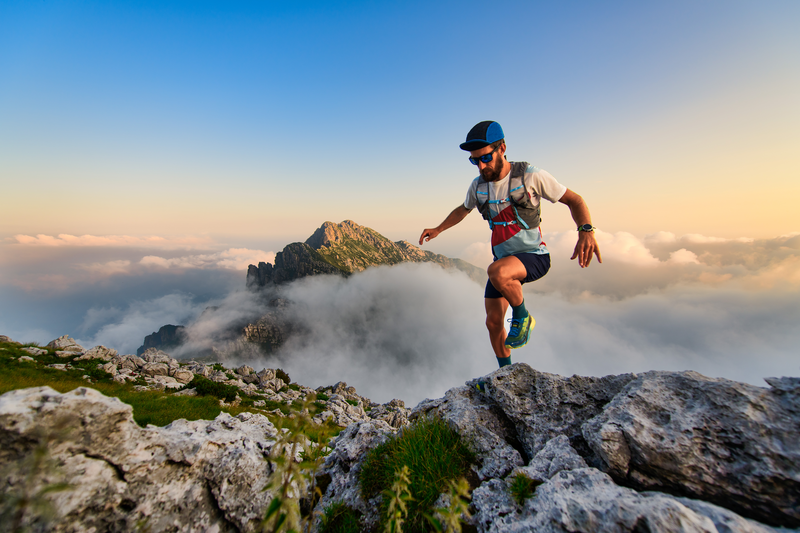
94% of researchers rate our articles as excellent or good
Learn more about the work of our research integrity team to safeguard the quality of each article we publish.
Find out more
ORIGINAL RESEARCH article
Front. Mar. Sci. , 16 June 2022
Sec. Marine Fisheries, Aquaculture and Living Resources
Volume 9 - 2022 | https://doi.org/10.3389/fmars.2022.912129
Stock enhancement based on hatchery-reared fish has become one of the most common forms of management practices in marine fisheries resource restoration. However, unnatural rearing environments may cause hatchery-reared fish to diverge phenotypically from wild conspecifics, with negative consequences for post-release performance in the natural environments. To better evaluate the suitability of releasing hatchery-reared fish, it is necessary to understand the phenotypic effects of captive rearing, through comparisons with wild conspecifics. In this study, we compared body morphology, swimming performance, and biochemical body composition between hatchery-reared and wild marbled rockfish (Sebastiscus marmoratus) from the same general gene pool. The results show that the overall body profile differed significantly between the groups, with hatchery-reared individuals having a deeper body (in particular in the head and trunk regions), narrower caudal peduncles, and higher condition factor, as compared to wild conspecifics. Hatchery-reared marbled rockfish also had relatively shorter fins, for a given size. In terms of swimming performance, the hatchery-reared marbled rockfish performed worse than the wild, with slower burst swimming speeds and poorer endurance. Wild rockfish had higher body protein content but lower lipid levels compared to the hatchery-reared individuals. These results suggest that hatchery rearing conditions have a great impact on the phenotypic development, with possibly high effects on their post-release performance of the hatchery-reared marbled rockfish. Modifications for the hatchery environment and operation should be investigated with an aim to minimize the divergence in phenotypic development for production of more wild-like fish for stocking.
With the development of modern aquaculture techniques, releases of hatchery-reared aquatic animals into natural waters has become one of the most common forms of enhancement practices in fisheries resource management and restoration around the world (Bell et al., 2008; Lorenzen et al., 2021). According to Kitada (2018), at least 187 marine species were released by 20 countries around the world between 2011 and 2016. In China alone, over 190 billion aquatic animal juveniles were released into natural waters for stock enhancement between 2016 and 2020 (Ministry of Agriculture and Rural Affairs of the PRC, 2022). While these aquaculture-based enhancement programs have been carried out widely, many concerns have been raised regarding the efficiency of these massive releases of hatchery-reared aquatic animals. One major issue concerns is the viability of hatchery-reared animals in natural environments, with high mortality rates being observed among released individuals, especially in marine fish (Brown and Laland, 2001; Araki et al., 2008; Johnsson et al., 2014).
Several studies have pointed out that the low survival rate of hatchery-reared fish can be attributed to the altered morphological, physiological and behavioral traits in hatchery-reared individuals, as compared to their wild conspecifics. Fishes generally exhibit substantial plasticity in their phenotypic development, as a consequence of several factors in their rearing environment (e.g. Ebbesson and Braithwaite, 2012; Johnsson et al., 2014; Venney et al., 2021). Since divergence in phenotypic expressions is common for hatchery-reared fish and can be detected even in the first hatchery-reared generation stemming from wild parents (Christie et al., 2012), maladaptive changes in the phenotype constitutes a possible cause for poor post-release performance (Araki et al., 2008; Fraser et al., 2018; Näslund, 2021). For example, rapid morphological divergence has been found between the cultured and wild Atlantic cod Gadus morhua from the same ancestral population (Wringe et al., 2015) and elevated rates of morphological defects (e.g. in lateral line morphology) have been observed in hatchery-reared steelhead trout Oncorhynchus mykiss (Brown et al., 2017) and turbot Scophthalmus maximus (Ellis et al., 1997). General body shape differences between hatchery- and wild-origin fish have been reported in many cultured species [e.g. steelhead trout (Pulcini et al., 2013); June sucker Chasmistes liorus (Belk et al., 2008); Chinook salmon Oncorhynchus tshawytscha (Tiffan and Connor, 2011) and Atlantic salmon Salmo salar (Von Cramon-Taubadel et al., 2005)].
Many studies suggest that body morphology has great impact on fish swimming efficiency and maneuverability (Ohlberger et al., 2006; Belk et al., 2008; Hatanpää et al., 2019; Zhao et al., 2020). The critical swimming speed (Ucrit) and burst swimming speed (Uburst) are two of the most fundamental parameters reflecting the fish swimming ability. Critical swimming speed often refers to the maximum aerobic swim speed of the fish, which can be used to indicate swimming endurance or determine the oxygen consumption capacity of the fish during swimming (Reidy et al., 2000). Burst swimming speed usually assesses the fast-start swimming performance (e.g. during the process of chasing prey or avoiding predators) of fish and can be used to evaluate the anaerobic ability (Marras et al., 2010). Hence, for hatchery-reared fish, the Ucrit and Uburst can be used as predictors to evaluate the immediate post-release predatory and anti-predation abilities, respectively (Lu et al., 2020; Zhao et al., 2020; Hou et al., 2022). In association to rearing effects on body morphology, lowered swimming abilities indicative of poor predatory ability and anti-predator performances have been documented in many fish species [e.g. European sea bass Dicentrarchus labrax (Handelsman et al., 2010; Benhaïm et al., 2012); Atlantic salmon (McDonald et al., 1998; Hammenstig et al., 2014)]. Accordingly, the poor swimming performance may result in the observed negative post-release fitness effects in captive-reared fishes (Araki et al., 2008; Johnsson et al., 2014; Lu et al., 2020; Hou et al., 2022).
Due to the large differences between hatchery- and wild-origin fish, it is necessary to make the comparisons of phenotypic differences between hatchery- and wild conspecifics prior to the initiation of massive stock-enhancement releases to optimize the performance of stocked fish in the wild (Huntingford, 2004; Johnsson et al., 2014). Such comparisons will not only provide a benchmark for assessing the general performance of hatchery-reared individual after release (Lorenzen, 2006; Näslund, 2021), but they also provide valuable information to help to redesign artificial rearing environments to optimize post-release performance, as well as to recognize the limitations of what can be achieved by modifying hatchery rearing practices (Johnsson et al., 2014). However, though substantial investment has been put into the culture of many marine fish species (Wringe et al., 2016), efforts are mostly restricted to commercially important taxa like salmonid fishes, owing to the long history of restocking and farming in this taxonomic group (Von Cramon-Taubadel et al., 2005; Näslund, 2021). These studies provide valuable information, but the lessons learned in salmonid rearing may not be applicable to other species (Salvanes and Braithwaite, 2006; Näslund, 2021). Additionally, most studies aiming to identify the phenotypic differences between hatchery- and wild-origin fish focus solely on a single aspect of the phenotype (e.g. Araki et al., 2007; Handelsman et al., 2010; Wringe et al., 2016) but combined comparison of morphological, behavioral and biochemical variables are very valuable, albeit scarce.
The marbled rockfish, Sebastiscus marmoratus (Cuvier, 1829), also known as ‘sea ruffe’, ‘false kelpfish’ and ‘dusky stingfish’, is an ecologically and economically important inshore species in eastern Asia, distributed within the coastal areas of China, Japan, Korea, and the Philippines (Kita et al., 1996). Its ecology resembles that of many of the Sebastini tribe members (which e.g. also includes commercially important rockfish species in the Sebastes genus), with a preference for demersal habitats in littoral rocky areas where it shows obvious territorial behavior associated to relatively small home ranges (Fujita and Kohda, 1998; Wu, 1999; Zhang et al., 2015). In China and Japan, marbled rockfish is one of the most popular recreational fish species (Zhang et al., 2015). Due to overfishing, the wild stock of this species has undergone a steep population decline (Zhang et al., 2015; Wang et al., 2020). In recent years, with the breakthrough of the aquaculture techniques for this species, small pilot-scale stocking projects have been conducted in eastern China, to evaluate the potential for supplementary rearing and stocking prior to initiating large-scale stocking efforts (Zhu, 2020). However, during the hatchery-rearing process, several visually distinguishable divergences from the wild phenotype were noted in the cultured fish (Figure 1). Considering the widely reported poor performance of hatchery-reared fish in the wild, it is crucial to carry out evaluations of potential phenotypic deficits of the hatchery-reared marbled rockfish before the full implementation of stock enhancement of this species commences.
Figure 1 Positions of the 20 landmarks used for measurement of morphology in wild (A) and hatchery-reared (B) marbled rockfish. 1: anterior tip of premaxilla; 2: most posterior point of maxilla; 3: insertion of the operculum on the ventral profile; 4: most posterior point of the operculum; 5: spine in front of orbit; 6: spine behind the orbit; 7: spine closest to the anterior base of the dorsal fin; 8: anterior base of the first dorsal fin; 9: vertex of the back; 10: anterior base of the second dorsal fin rays; 11: posterior base of dorsal fin; 12: deepest point of caudal peduncle at dorsal outline; 13: anterior attachment of dorsal membrane from caudal fin; 14: base of middle caudal rays; 15: anterior attachment of ventral membrane from caudal fin; 16: uppermost point of caudal peduncle at ventral outline; 17: posterior base of anal fin; 18: anterior base of anal fin; 19: anus; 20: semi-landmark collected along the ventral profile.
The purpose of the present study was to investigate effects of hatchery rearing on body morphology, swimming performance, and biochemical body composition in marbled rockfish, by comparisons of hatchery-reared and wild fish with similar genetic origin. We aimed to identify the effects of hatchery culture on (i) non-genetic morphometric variation, (ii) swimming performance, in terms of critical- and burst swimming capacity, and (iii) biochemical composition. The results will not only provide valuable baseline information for future assessments of general post-release performance of hatchery-reared individuals, but also provide fundamental information for further investigations of potential behavioral differences (e.g. acquisition and processing of food, antipredation behavior, shelter-seeking, habitat choice, inter-individual interactions, etc.) between the hatchery-reared and wild-origin marbled rockfish.
All the hatchery-reared marbled rockfish were the progeny of 350 wild fish, which were caught from the sea around Dongji Island, Zhoushan, China (30°12’ N, 122°40’ E). All these wild-caught broodstock fish had been reared in captivity for two years in concrete tanks (sand-filtered natural seawater; pH 8.0-8.3; dissolved oxygen ≥6.0 mg/L; temperature fluctuating seasonally between 12°C and 28°C; fed with forage fish). The hatchery-reared population used for this study were produced in January 2020 and then reared in nursery ponds (size: 5 m × 6 m × 1 m) in a commercial hatchery (Xixuan Technology Island) in Zhoushan, China, according to the standard methodology for the intensive culture of this species (Yan et al., 2018). The nursery ponds were provided with sand-filtered natural seawater (flow rate: 2 m3 h-1) and gently aerated with air-stone diffusers. After spawning, the larvae were gradually weaned from cladocerans and copepods to a commercial pellet diet over approximately 1 month. In early December 2020, a total of 500 hatchery-reared fish of uniform size (total body length: 8.02 ± 0.91cm, mean ± SD) were selected and transported to a single circular holding tank (water volume: 1780 L; inside diameter: 1.6 m; height: 0.8 m; recirculating seawater system) at the Laboratory of Marine Ranching Stock Enhancement (LMRSE), Zhejiang Ocean University. The water temperature in the holding tank was kept at 17°C, which was consistent with the temperature in the sea during the same period. The dissolved oxygen was ≥6.0 mg/L, unionized ammonia nitrogen maintained at <0.05 mg/L, salinity was 28‰, and pH kept at 8.0-8.3. The photoperiod followed an 11-h light/13-h dark cycle. From the third day after transport, fish were fed twice daily (at 9:00 and 18:00; to apparent satiation) with commercial dry pellets (Hayashikane Sangyo, Co., Ltd., Yamaguchi Prefecture, Japan; composition: crude protein ≥ 50.0%, lipid ≥ 6.0%, fiber ≤ 3.0%, and ash ≤ 17.0%).
Wild marbled rockfish were caught by angling in December 2020, in the same place as where the wild parents of the hatchery-reared fish had been caught. Thus, the hatchery and wild-caught fish are likely to be of the same stock and being genetically similar. After capture, the wild fish were transported to the LMRSE. In total, 450 wild marbled rockfish, size-matched with the cultured experimental fish (total body length: 8.01 ± 1.21 cm, mean ± SD), were selected and held in a holding tank for recovery, to mitigate potentially negative effects of transportation stress. The size and water conditions of the wild fish holding tank were similar with the one used for the hatchery-reared fish, since both two tanks shared one recirculating seawater system. Enrichment structures (plastic tube and rocks) were added into the wild fish holding tank to mimic some elements of the natural environment as well as possible. From the third day post-transport, wild fish were provided with live ridgetail white prawns Exopalaemon carinicauda every other day to make sure live preys were constantly available in the tank.
All animals were treated in accordance with the Guidelines for Use of Experimental Animals provided by Zhejiang Ocean University and all animal holding and experimental procedures were approved by the Institutional Animal Care and Use Committee at Zhejiang Ocean University.
On the second day after being transported to the laboratory facilities, 244 wild (total body length: 8.83 ± 1.53 cm, mean ± SD) and 276 hatchery-reared (total body length: 8.93 ± 1.03 cm, mean ± SD) marbled rockfish were randomly selected for morphometric assessment. The age of individuals was determined from their scales (microscope Olympus BX43; Olympus Life Science, Tokyo). All the selected individuals were around one year old. No significant difference was detected in total body length between these two groups (P = 0.362, independent sample t-test). After being anesthetized (tricaine methanesulfonate, MS-222; 50 mg · L−1), fish were arranged left side up on a white background panel and photographed with a tripod mounted digital single-lens reflex camera (Nikon D3400, Nikon, Minato City, Japan), with a 18-55 mm lens (AF-S DX 18-55mm F/3.5-5.6 G VR II, Nikon, Minato City, Japan). A millimeter ruler was included in each photograph for size calibration (Figure 1). Prior to the photography, all fish were fasted for 24 h to ensure that no gut contents affected body shape. The body shapes were examined using landmark-based geometric morphometrics (Rohlf and Marcus, 1993; Zelditch et al., 2004; Pulcini et al., 2013). Twenty landmarks were digitized from each photograph (Figure 1), using TpsDig2.0 software (Rohlf, 2015). Landmark configurations were aligned and superimposed using the Generalized Procrustes Analysis (GPA) to remove effects of differences in body size, position and orientation (Figure 2). Thereafter, residuals from the superimposition were analyzed with the thin-plate spline (TPS) interpolation function. To reduce the dimensionality of the data for each individual prior to comparative analyses, a principal component analysis (PCA) was run based on the covariance matrix (Zelditch et al., 2004) using the MorphoJ software (Klingenberg, 2011). In addition, sixty fish from each group (hatchery-reared and wild-origin) were randomly selected and weighted with an electronic balance (accurate to 0.01g; KFS-A, KaiFeng, Yongkang City, China) to calculate the condition factor (K = 100 × body mass × body length-3).
Figure 2 Superimposition of vectorized landmarks for all samples of (A) wild (n = 244), and (B) hatchery-reared (n = 276) marbled rockfish.
Pectoral-, pelvic-, anal-, caudal-, and ventral fin size were approximated by measures of the distance from the fin base to the tip of the longest fin ray, using ImageJ (Schneider et al., 2012) (Figure 1). Since all individuals were photographed with left side up, only the left pectoral fins were measured from the photographs. Fin size measurements were divided by fish total body length × 100 to obtain relative measures for comparison between the hatchery-and wild-origin experimental fish (Kindschi, 1987; Bosakowski and Wagner, 1994).
After 12 days of recovery from transportation and handling stress, thirty fish from each group (hatchery-reared and wild-origin) were randomly selected for the measurement of critical swimming speed Ucrit and burst swimming speed Uburst. The experimental fish were fasted for 24 hours before the tests. All swimming performance measurements were conducted using a flow-controlled recirculating swimming tunnel (Loligo Systems SW10150, Denmark, https://www.loligosystems.com; Figure 3). The volume of the testing chamber in the tunnel was 30 L (55 cm × 14 cm × 14 cm). The water flow in the tunnel was driven by a propeller powered by an electric motor, and the velocity of the water flow could be adjusted by turning the frequency converter. A honeycomb flow stabilizer was fixed in the left end of the testing chamber to induce laminar flow before entry into the testing chamber. The right end of the testing chamber was covered with 1 cm mesh.
Figure 3 (A) Design of the swim tunnel (Loligo Systems SW10150). Copyright: Loligo Systems, Viborg, Denmark, https://www.loligosystems.com/, published with permission. (B) Top view. a: dissolved oxygen probe; b: temperature probe; c: honeycomb flow stabilizer; d: testing chamber; e: water exchanging hole; f: propeller; g: electric motor; h: frequency converter; i: data collector module (DAQ-BT); j: experimental fish; k: acrylic plastic board; l: water flow deflectors.
Burst swimming speed Uburst was measured following the method described by Zhou et al. (2019). In brief, the experimental fish were allowed post-transfer recovery in the testing chamber for 30 min at a water velocity of 4 cm s−1 (i.e. 0.5 body lengths · s−1). Then, the water flow velocity in the testing chamber was gradually increased by 1 body length · s−1 at 20-second intervals until the experimental fish showed signs of fatigue (i.e. when fish could no longer maintain position against the current and failed to hold itself away from the mesh at the end of the testing chamber for more than 20 s) (Lu et al., 2020). The final flow velocity value was recorded as the burst swimming speed (body lengths · s−1; abbreviated for BL · s-1). The relative burst swimming speed (Ur-burst) was also calculated by dividing Uburst with the fish body length (cm).
Critical swimming speed Ucrit was measured according to the method described by Zhou et al. (2019). In brief, the experimental fish were allowed 1 h recovery in the testing chamber at a water velocity of 4 cm s−1 (i.e. 0.5 total body lengths · s−1) prior to the start of the measurements. Then, the water flow velocity in the testing chamber was gradually increased at an increment of 5 cm · s-1 every 20 min until the experimental fish showed signs of fatigue (as per the criteria described above for Uburst). The critical swimming speed (Ucrit) was calculated for each experimental fish by the formula described by (Brett, 1964):
where T is the time duration that the fish swam at the final flow velocity (min); ΔT is the time interval of each test flow velocity (i.e. 20 min); V is the maximum flow velocity (cm · s−1) against which the fish could swim for the entire 20 min; ΔV is the velocity increments (5 cm · s−1). In order to remove the size effect, we also calculated the relative critical swimming speed (Ur-crit) by dividing Ucrit with the fish body length (cm).
Directly after being transported to the laboratory facilities, thirty fish from each group (hatchery-reared and wild-origin) were randomly selected for the biochemical body composition analyses. All these experimental fish were fasted for 24 hours to ensure that no gut contents affected analyses and thereafter euthanized using an overdose of MS-222, followed by weighing using an electronic balance (accurate to 0.01g; KFS-A, KaiFeng, Yongkang City, China). Analyses were conducted according to the methods of the Association of Official Analytical Chemists (AOAC, 1995) and the procedures have described in detail in our previous work (Guo et al., 2020). In brief, the moisture content was measured by oven-dried at 105°C for 48 h until constant mass. Then the samples were ground to a powder. Ash content was determined by combusting samples in a muffle furnace set at 550°C for 24 h. Crude protein content was determined using the Kjeldahl method (FOSS Tecator KjelFlex TM 8400; FOSS, Hilleroed, Denmark) by measuring nitrogen (N×6.25). Crude lipid content was analyzed by ether extraction using Soxhlet method (B-801, BUCHI, Flawil, Switzerland).
Statistical analyses were performed using SPSS 17.0 (IBM Corp., Armonk, NY, USA). The data of condition factor, relative fin sizes, swimming performance and body biochemical composition were analyzed by independent sample t-tests. In all cases, differences were considered to be significant at P ≤ 0.05.
The direct graphical comparisons of the mean shapes show the body shape variation between the two groups (Figure 4), with an overall shape difference related to body profile. A general increase of the body depth is evident in the hatchery-reared group. The head region is thicker in the ventrolateral direction, with the jaws being angled more upwards (i.e. more horizontal along the anterio-caudal axis) than in the wild-origin fish. The trunk profile of hatchery-reared fish is also substantially thicker on average, as compared to wild conspecifics. The caudal peduncle of hatchery fish is slightly shorter and thinner at the base of the caudal fin, relative to the rest of the body. It is also evident that the jaws (when closed) were more angled in a downwards direction and that the caudal peduncle is slimmer in wild individuals. Comparing to the wild individuals, the hatchery-reared marbled rockfish had a generally more robust body, with a more rounded figure outline and a less streamlined body.
Figure 4 Comparisons of mean shape between hatchery-reared and wild marbled rockfish. Points relate to landmarks in Figure 1.
Shape variation along the discriminant axis is shown in Figure 5, with both splines and vectors. When looking at the direction and magnitude of the vectors, landmarks 1 (anterior tip of premaxilla), 3 (insertion of the operculum on the ventral profile), 4 (most posterior point of the operculum), 9 (vertex of the back), 10 (anterior base of the second dorsal fin rays), 17 (posterior base of anal fin), 19 (anus) and 20 (semi-landmark collected along the ventral profile) are responsible for the main overall body profile differences.
Figure 5 Deformation grid plots and visualization of body shape variation between hatchery-reared and wild marbled rockfish. The vectors showing shape variation along the discriminant axis. Points relate to landmarks in Figure 1.
The PCA biplot which summarizes overall body shape variation shows a very distinct and almost complete separation between the hatchery-reared and wild-origin groups (Figure 6). The first principal component (PC1) accounted for 24.0% of the variation and the second principal component (PC2) accounted for 17.74%, for a total of 41.74% of the variance being explained by these two components. Virtually all of the separation between the groups occurred along the PC1 axis (Figure 6).
Figure 6 Principal component biplot illustrating overall body shape variation for groups of wild (black dots) and hatchery-reared (grey dots) marbled rockfish.
Although the overall body length was similar among the two groups, the condition factor (K) of the cultured marbled rockfish (K = 3.86 ± 0.47, mean ± SD) was significantly higher (independent sample t-test, P = 0.042) compared with the wild marbled rockfish (K = 3.61 ± 0.79, mean ± SD).
Intraspecific comparison of relative fin length (fin length/total body length) for hatchery and wild fish showed that all fins in hatchery-reared marbled rockfish were generally shorter compared to the wild conspecific (P < 0.001; Table 1).
Table 1 Comparison of relative fin length between wild and hatchery-reared marbled rockfish (mean ± SD) (nwild = 244; nhatchery = 276).
The hatchery-reared marbled rockfish had significantly reduced swimming performance in all of the assessed variables (all P < 0.05; Table 2).
Comparison of biochemical body composition showed marked differences in whole body moisture-, ash-, and crude protein contents between wild and hatchery-reared marbled rockfish (all P < 0.05; Table 3). Moisture-, ash- and protein content were higher in the wild fish and fat content was higher in the hatchery-reared fish (Table 3).
Table 3 Comparison of the whole-body biochemical composition between the wild and hatchery-reared marbled rockfish.
Results from this study show that the morphology of hatchery-reared marbled rockfish differs substantially from that of wild conspecifics captured in the same area as where the parents of the hatchery fish originated. Hatchery-reared marbled rockfish have a generally higher body profile (except for the caudal peduncle), jaws that are angled more upwards, and shorter fins than the wild-origin conspecifics. In concordance with the higher body profile for a given length, the condition factor of the hatchery-reared fish was also higher than that of the wild-origin fish. Swimming ability was lower for hatchery-reared fish, in terms of both critical- and burst swimming speed. Finally, the biochemical body composition differed among the groups, with moisture-, ash- and protein content being higher in the wild fish and fat content being higher in the hatchery fish.
The difference in overall body morphology is dramatic in the marbled rockfish, as evidenced by the near complete separation between hatchery-reared and wild-origin fish along the first principal component axis. Similar strong morphological divergences have been observed in several other species. For instance, in Atlantic salmon juveniles the hatchery-reared individuals have been shown to have smaller fins and narrower caudal peduncles in comparison to wild-origin fish, with 100% accuracy when determining origin of the fish based on morphology (Fleming et al., 1994). Wringe et al. (2015) reported that farmed Atlantic cod were characterized by relatively smaller heads and fin measurements, while also having a higher body profile and higher condition index compared to wild conspecifics. Largely similar morphological differences have also been found in cultured Chinook salmon (Tiffan and Connor, 2011), gilthead seabream Sparus aurata (Fragkoulis et al., 2016) and rainbow trout (Pulcini et al., 2013). Thus, it can be concluded that the effects seen in hatchery-reared marbled rockfish in this study are in line with previous findings in other species.
Wringe et al. (2016) hypothesized that the homogenized rearing environment and procedures in modern aquaculture elicit generally similar plastic responses in the phenotypes in fishes, explaining why patterns in differences between cultured and wild fish tend to go in similar directions in many different species. These similar and readily identifiable morphological divergences from typical wild-type phenotypes found in cultured fish can thus be characterized as a “cultured fish phenotype”. This cultured fish phenotype is generally described by shorter but deeper heads, greater body depth and condition factor, and smaller fins, as compared to their wild conspecifics (Wringe et al., 2016). Our results provide further support for this hypothesis since many morphological characteristics in the hatchery-reared marbled rockfish are consistent with the synthesis of Wringe et al. (2016).
For hatchery-reared fish, the genotype and the rearing environment are two of the most fundamental factors underlying the morphological phenotypic expression (Araki et al., 2007; Chittenden et al., 2010; Wringe et al., 2016). However, although some studies have observed genetic influences on phenotypic differences between wild and hatchery populations (Riddell and Swain, 1991; Araki et al., 2007; Chittenden et al., 2010), the hatchery rearing environment seems to be the major cause of the phenotypic divergence (Swain et al., 1991; Fraser et al., 2019). In the present study, all the cultured marbled rockfish were the progeny of wild-caught parents, indicating that the genetics of the cultured experimental fish are likely unchanged relative to the wild caught experimental fish. Given the high survival rate of fish in hatcheries, the morphological phenotypic differences developed in the hatchery should be mainly attributed to the rearing environment, in contrast to the situation in the natural environment where selection also play a significant role, as argued by Stringwell et al. (2014). Several possible explanations have been raised for this divergence of overall body morphology for fish under artificial hatchery conditions (Fleming et al., 1994). In general, the restricted and protected rearing environment reduces the need for extensive activity and thereby allows for allocation of more energy to protein growth and lipid deposition, and several morphological changes may occur in association with this (Thorpe, 2004). In aquaculture, where the environment is barren and uniform with respect to structures and water flow, and where food pellets are plentiful and non-elusive, the reared marbled rockfish showed an inactive behavior where they spent most of the time motionless on the tank floor (pers. obs.). Thus, the increase in body depth is possibly induced as a response to the food-rich, low-exercise environment in the hatchery relative to variable natural marine environments. Under aquaculture conditions, fish generally tend toward a higher body fat content than wild fish (Bergström, 1989; Arechavala-Lopez et al., 2013; Powell et al., 2017), and the trunk is a site of fat deposition in many fishes e.g. salmonids (Robinson and Mead, 1973; Fleming et al., 1994). This was confirmed by the biochemical body composition analysis in this study, which shows a significant higher fat deposition in cultured marbled rockfish. Additionally, the food-pellet diet may result in changes in gut and intestinal morphology that influence girth positively (Weatherley and Gill, 1981; Fleming et al., 1994). In line with a generally higher body depth and fat content, the condition factor was higher in the cultured rockfish, which is characteristic for artificially reared fishes (Wringe et al., 2016).
Another characteristic feature found in hatchery-reared marbled rockfish in this study is the upward angling of the mouth, as indicated by the landmarks related to the upper jaw. This morphological difference between hatchery-reared and wild could be due to enforced differences in feeding habits in captivity related to provision of pellet-feed. In the wild, the marbled rockfish is a benthic carnivorous species, feeding on e.g. amphipods, decapods, cephalopods and fishes in littoral rocky areas (Yokogawa and Iguchi, 1992; Lee et al., 2012; Wang et al., 2017). Juveniles in particular feed mainly on crustaceans associated to benthic structures, and fish inclusion in the diet increasing with body size (Yokogawa and Iguchi, 1992; Lee et al., 2012). However, in artificial culture conditions, the pellet diet is supplied at the water surface and thereby appears above the fish head all the time. Continued exposure to a non-benthic food source during development may possibly lead to the abnormal development of the fish mouth structure, as a plastic response to these artificial feeding conditions (i.e. searching and swallowing pellet-feed from the surface rather than bottom). Few previous reports have described this particular effect on mouth morphology from cultured fish (but see Pulcini et al., 2013). However, general effects on head morphology are common (Wringe et al., 2016). Plastic changes in head morphology can even be seen in wild fish associated to aquaculture cages in natural waters, suggesting that food substitution from natural prey to pellets may underlie these plastic responses (Abaad et al., 2016). In general, environment dependent head and jaw apparatus plasticity is commonly observed in fishes outside of aquaculture conditions, and appear to often be related to food types (e.g. Mittelbach et al., 1999; Závorka et al., 2019; Lofeu et al., 2021).
Since the mouth morphology can be important for feeding efficiency, the observed divergence in hatchery-reared fish may have negative impacts on the post-release performance of hatchery-reared fish in the natural marine environment (Fleming et al., 1994; Belk et al., 2008; Pulcini et al., 2013; Johnsson et al., 2014). As far as we are aware, this is the first report of the abnormal development of the mouth structure in hatchery-reared marbled rockfish. The present findings demonstrate the need for continued research into differences in feeding behavior and foraging efficiency between the hatchery-reared and wild-origin marbled rockfish, to better assess the effects of artificial rearing on post-release performance in the context of stock enhancement strategies.
Hatchery-reared marbled rockfish were found to be poorer swimmers than their wild conspecifics, with lower sprint swimming speeds and endurance than wild conspecifics in terms of all investigated swimming parameters (Ucrit, Uburst, Ur-crit, and Ur-burst). Similar effects of artificial rearing on swimming performance have been found in other fish species. For example, Bellinger et al. (2014) reported that domesticated rainbow trout have larger body sizes but slower burst swimming speeds than wild rainbow trout. Cultured juvenile European seabass also showed slower burst swimming speeds compared to similar-sized wild fish in laboratory conditions (Handelsman et al., 2010). In Atlantic salmon, the burst swimming speed of hatchery-reared fish was found to be slower than that of wild conspecifics, at least within the first year of life (at the parr stage) (Hammenstig et al., 2014).
It has been widely recognized that performance is intimately linked to morphology (Ojanguren and Braña, 2003; Belk et al., 2008) and the swimming efficiency can be highly influenced by fish body morphology (Ohlberger et al., 2006; Belk et al., 2008; Zhao et al., 2020). Under cultured conditions, the lower and less variable water velocity has been shown to lead to the abnormal development of morphological phenotypes in salmonids, with stockier bodies and shorter fins as a result (Pakkasmaa and Piironen, 2000; Wringe et al., 2016). Also in wild salmonids, both fin- and body morphology respond plastically to water current velocity (Paéz et al., 2008). By using relative fin length, we show that all rayed fins of hatchery-reared marbled rockfish were significantly shorter than in wild conspecifics. The hatchery-reared individuals also had a less streamlined body profile. Hence, the poor swimming performance in the hatchery fish could probably be due to this morphological divergence from the wild phenotype.
Studies have also reported that swimming performance can be lowered in cultured fish with high level of muscle lipid content, due to the muscle glycolysis inhibition (Dyck et al., 1993; McDonald et al., 1998), and fish with high level of muscle protein content can have an increase in either muscle power or buffering capacity, which could improve swimming capacity (McDonald et al., 1998). Our results do not contrast these hypotheses, since wild marbled rockfish with higher muscle protein and lower lipid levels had better swim performance compared to hatchery-reared conspecific. However, more detailed studies, where lipid- and protein contents are investigated in the actual individuals going through the swim tests, would be necessary to show more direct evidence in support of the hypotheses. Together with the previous studies (Martinez et al., 2003; Anttila et al., 2010), it can be inferred that deficiency at muscular level is a candidate mechanism behind the observed poor swimming capacity in the hatchery-reared rockfish.
In conclusion, the results of this study indicate a discernible effect of captive rearing on the morphological phenotype of marbled rockfish. A general pattern emerged with the hatchery-reared fish developing a typical “cultured fish phenotype” (higher body profile, smaller fins and higher body condition with a higher lipid: protein ratio in the body), as compared to the wild conspecifics. These observed deviations from the wild phenotype are potentially causing the poor swimming performance of hatchery-reared fish relative to their wild counterparts. A difference in the positioning of the jaw apparatus was also observed. Both jaw morphology and swimming performance can likely affect the foraging- and antipredation abilities in natural environments, which would then possibly contribute to a greater mortality risk in hatchery-reared marbled rockfish released into the wild (Näslund, 2021). Thus, further investigations of these differences, as well as behavioral differences (e.g. predator avoidance, acquisition and processing of food, sheltering, etc.) are essential to better understand the fitness consequences of captive rearing in fish destined for release within stock enhancement programs. Such studies will also provide valuable information for making hatchery environment modifications in order to produce more wild-like marbled rockfish aimed for release.
The original contributions presented in the study are included in the article/supplementary material. Further inquiries can be directed to the corresponding author.
The animal study was reviewed and approved by Experimental Animal Ethics Committee, Zhejiang Ocean University.
HG conceived and designed the experiments, drafted the manuscript. XiaZ, LP, CL, and MT performed the experiments and analyzed the data. JN revised the manuscript. DZ and XC reared fish. XiuZ (*Correspondence) conceived the experiments. All authors contributed to the article and approved the submitted version.
This study was supported by the National Natural Science Foundation of China (32102755), Natural Science Foundation of Zhejiang Province (LGN22C190015), Research Program of Bureau of Science and Technology of Zhoushan (2020C43255), the Key Research and Development Program of Zhejiang Province (2021C02047), Scientific Research Project of Department of Education of Zhejiang Province (Y202147843) and National College Students Innovation and Entrepreneurship Training Program (202110340028).
The authors declare that the research was conducted in the absence of any commercial or financial relationships that could be construed as a potential conflict of interest.
All claims expressed in this article are solely those of the authors and do not necessarily represent those of their affiliated organizations, or those of the publisher, the editors and the reviewers. Any product that may be evaluated in this article, or claim that may be made by its manufacturer, is not guaranteed or endorsed by the publisher.
Abaad M., Tuset V. M., Montero D., Lombarte A., Otero-Ferrery J. L., Haroun R. (2016). Phenotypic Plasticity in Wild Marine Fishes Associated With Fish-Cage Aquaculture. Hydrobiologia 765, 343–358. doi: 10.1007/s10750-015-2428-5
Anttila K., Jäntti M., Mänttäri S. (2010). Effects of Training on Lipid Metabolism in Swimming Muscles of Sea Trout (Salmo trutta). J. Comp. Physiol. B. Biochem. Syst. Environ. Physiol. 180, 707–714. doi: 10.1007/s00360-010-0446-1
Araki H., Berejikian B. A., Ford M. J., Blouin M. S. (2008). Fitness of Hatchery-Reared Salmonids in the Wild. Evol. Appl. 1, 342–355. doi: 10.1111/j.1752-4571.2008.00026.x
Araki H., Cooper B., Blouin M. S. (2007). Genetic Effects of Captive Breeding Cause a Rapid, Cumulative Fitness Decline in the Wild. Science 318, 100–103. doi: 10.1126/science.1145621
Arechavala-Lopez P., Fernandez-Jover D., Black K. D., Ladoukakis E., Bayle-Sempere J. T., Sanchez-Jerez P., et al. (2013). Differentiating the Wild or Farmed Origin of Mediterranean Fish: A Review of Tools for Sea Bream and Sea Bass. Rev. Aquacult. 5, 137–157. doi: 10.1111/raq.12006
Association of Official Analytical Chemists (AOAC) (1995). Official Methods of Analysis. 16th (Arlington, VA: AOAC).
Belk M. C., Benson L. J., Rasmussen J., Peck S. L. (2008). Hatchery-Induced Morphological Variation in an Endangered Fish: A Challenge for Hatchery-Based Recovery Efforts. Can. J. Fish. Aquat. Sci. 65, 401–408. doi: 10.1139/f07-176
Bellinger K. L., Thorgaard G. H., Carter P. A. (2014). Domestication Is Associated With Reduced Burst Swimming Performance and Increased Body Size in Clonal Rainbow Trout Lines. Aquaculture 420-421, 154–159. doi: 10.1016/j.aquaculture.2013.10.028
Bell J. D., Leber K. M., Blankenship H. L., Loneragan N. R., Masuda R. (2008). A New Era for Restocking, Stock Enhancement and Sea Ranching of Coastal Fisheries Resources. Rev. Fish. Sci. 16, 1–9. doi: 10.1080/10641260701776951
Benhaïm D., Péan S., Lucas G., Blanc N., Chatain B., Bégout M. L. (2012). Early Life Behavioural Differences in Wild Caught and Domesticated Sea Bass (Dicentrarchus labrax). Appl. Anim. Behav. Sci. 141, 79–90. doi: 10.1016/j.applanim.2012.07.002
Bergström E. (1989). Effect of Natural and Artificial Diets on Seasonal Changes in Fatty Acid Composition and Total Body Lipid Content of Wild and Hatchery-Reared Atlantic Salmon (Salmo salar L.) Parr-Smolt. Aquaculture 82, 205–217. doi: 10.1016/0044-8486(89)90409-2
Bosakowski T., Wagner E. J. (1994). Assessment of Fin Erosion by Comparison of Relative Fin Length in Hatchery and Wild Trout in Utah. Can. J. Fish. Aquat. Sci. 51, 636–641. doi: 10.1139/f94-064
Brett J. R. (1964). The Respiratory Metabolism and Swimming Performance of Young Sockeye Salmon. J. Fish. Res. Board. Canada. 21, 1183–1226. doi: 10.1139/f64-103
Brown C., Laland K. (2001). Social Learning and Life Skills Training for Hatchery Reared Fish. J. Fish. Biol. 59, 471–493. doi: 10.1111/j.1095-8649.2001.tb02354.x
Brown A. D., Sisneros J. A., Jurasin T., Nguyen C., Coffin A. B. (2017). Differences in Lateral Line Morphology Between Hatchery-and Wild-Origin Steelhead. PLos One 8, e59162. doi: 10.1371/journal.pone.0059162
Chittenden C. M., Biagi C. A., Davidsen J. G., Davidsen A. G., Kondo H., McKnight A., et al. (2010). Genetic Versus Rearing-Environment Effects on Phenotype: Hatchery and Natural Rearing Effects on Hatchery-and Wild-Born Coho Salmon. PLos One 5, e12261. doi: 10.1371/journal.pone.0012261
Christie M. R., Marine M. L., French R. A., Blouin M. S. (2012). Genetic Adaptation to Captivity can Occur in a Single Generation. Proc. Natl. Acad. Sci. U.S.A. 109, 238–242. doi: 10.1073/pnas.1111073109
Dyck D. J., Putman C. T., Heigenhauser G. J. F., Hultman E., Spriet L. L. (1993). Regulation of Fat-Carbohydrate Interaction in Skeletal Muscle During Intense Aerobic Cycling. Am. J. Physiol.-Endocrinol. Metab. 265, E852–E859. doi: 10.1152/ajpendo.1993.265.6.E852
Ebbesson L., Braithwaite V. A. (2012). Environmental Effects on Fish Neural Plasticity and Cognition. J. Fish. Biol. 81, 2151–2174. doi: 10.1111/j.1095-8649.2012.03486.x
Ellis T., Howell B. R., Hayes J. (1997). Morphological Differences Between Wild and Hatchery-Reared Turbot. J. Fish. Biol. 50, 1124–1128. doi: 10.1111/j.1095-8649.1997.tb01637.x
Fleming I. A., Jonsson B., Gross M. R. (1994). Phenotypic Divergence of Sea-Ranched, Farmed, and Wild Salmon. Can. J. Fish. Aquat. Sci. 51, 2808–2824. doi: 10.1139/f94-280
Fragkoulis S., Christou M., Karo R., Ritas C., Tzokas C., Batargias C., et al. (2016). Scaling of Body-Shape Quality in Reared Gilthead Seabream Sparus aurata L. Consumer Preference Assessment, Wild Standard and Variability in Reared Phenotype. Aquacult. Res. 48, 2402–2410. doi: 10.1111/are.13076
Fraser D. J., Walker L., Yates M. C., Marin K., Wood J. L., Bernos T. A., et al. (2018). Population Correlates of Rapid Captive-Induced Maladaptation in a Wild Fish. Evol. Appl. 12, 1305–1317. doi: 10.1111/eva.12649
Fujita H., Kohda M. (1998). Timing and Sites of Parturition of the Viviparous Scorpionfish, Sebastiscus marmoratus. Environ. Biol. Fish. 52, 225–229. doi: 10.1023/A:1007471919373
Guo H. Y., Roques J. A., Li M., Zhang X. M. (2020). Effects of Different Feeding Regimes on Juvenile Black Rockfish (Sebastes schlegilii) Survival, Growth, Digestive Enzyme Activity, Body Composition and Feeding Costs. Aquacult. Res. 51, 4103–4112. doi: 10.1111/are.14753
Hammenstig D., Sandblom E., Axelsson M., Johnsson J. I. (2014). Effects of Rearing Density and Dietary Fat Content on Burst-Swim Performance and Oxygen Transport Capacity in Juvenile Atlantic Salmon Salmo salar. J. Fish. Biol. 85, 1177–1191. doi: 10.1111/jfb.12511
Handelsman C., Claireaux G., Nelson J. A. (2010). Swimming Ability and Ecological Performance of Cultured and Wild European Sea Bass (Dicentrarchus labrax) in Coastal Tidal Ponds. Physiol. Biochem. Zool. 83, 435–445. doi: 10.1086/651099
Hatanpää A., Huuskonen H., Kekäläinen J., Kortet R., Hyvärinen P., Vitelletti M. L., et al. (2019). Early Winter Foraging Success, Swimming Performance and Morphology of Juvenile Landlocked Atlantic Salmon Reared Under Semi-Wild and Hatchery Conditions. Can. J. Fish. Aquat. Sci. 77, 770–778. doi: 10.1139/cjfas-2019-0079
Hou Q. M., Fu S. J., Huang T. J., Li X. M., Shi X. T. (2022). Effects of Aerobic Exercise Training on the Growth, Swimming Performance, Antipredation Ability and Immune Parameters of Juvenile Rock Carp (Procypris rabaudi). Animals 12, 257. doi: 10.3390/ani12030257
Huntingford F. A. (2004). Implications of Domestication and Rearing Conditions for the Behaviour of Cultivated Fishes. J. Fish. Biol. 65, 122–142. doi: 10.1111/j.0022-1112.2004.00562.x
Johnsson J. I., Brockmark S., Näslund J. (2014). Environmental Effects on Behavioural Development Consequences for Fitness of Captive-Reared Fishes in the Wild. J. Fish. Biol. 85, 1946–1971. doi: 10.1111/jfb.12547
Kindschi G. A. (1987). Method for Quantifying Degree of Fin Erosion. Progr. Fish-Cult. 49, 314–315. doi: 10.1577/1548-8640(1987)49<314:MFQDOF>2.0.CO;2
Kitada S. (2018). Economic, Ecological and Genetic Impacts of Marine Stock Enhancement and Sea Ranching: A Systematic Review. Fish. Fish. 19, 511–532. doi: 10.1111/faf.12271
Kita J., Tsuchida S., Setoguma T. (1996). Temperature Preference and Tolerance, and Oxygen Consumption of the Marbled Rockfish, Sebastiscus marmoratus. Mar. Biol. 125, 467–471. doi: 10.1007/BF00353259
Klingenberg C. P. (2011). MorphoJ: An Integrated Software Package for Geometric Morphometrics. Mol. Ecol. Resour. 11, 353–357. doi: 10.1111/j.1755-0998.2010.02924.x
Lee S. J., Kim B. Y., Cha H. K. (2012). Feeding Habits of Sebastiscus marmoratus in the Coastal Waters of Jeju Island, Korea. J. Kor. Soc. Fish. Ocean. Technol. 48, 379–386. doi: 10.3796/KSFT.2012.48.4.379
Lofeu L., Anelli V., Straker L. C., Kohlsdorf T. (2021). Developmental Plasticity Reveals Hidden Fish Phenotypes and Enables Morphospace Diversification. Evolution 75, 1170–1188. doi: 10.1111/evo.14221
Lorenzen K. (2006). Population Management in Fisheries Enhancement: Gaining Key Information From Release Experiments Through Use of a Size-Dependent Mortality Model. Fish. Res. 80, 19–27. doi: 10.1016/j.fishres.2006.03.010
Lorenzen K., Leber K. M., Loneragan N. R., Schloesser R. W., Taylor M. D. (2021). Developing and Integrating Enhancement Strategies to Improve and Restore Fisheries. Bull. Mar. Sci. 97, 475–488. doi: 10.5343/bms.2021.0036
Lu Y., Wu H., Deng L. J., Li T. C., Yang K., Fu S. J., et al. (2020). Improved Aerobic and Anaerobic Swimming Performance After Exercise Training and Detraining in Schizothorax wangchiachii: Implications for Fisheries Releases. Comp. Biochem. Physiol. Part A.: Mol. Integr. Physiol. 245, 110698. doi: 10.1016/j.cbpa.2020.110698
Marras S., Claireaux G., McKenzie D. J., Nelson J. A. (2010). Individual Variation and Repeatability in Aerobic and Anaerobic Swimming Performance of European Sea Bass, Dicentrarchus labrax. J. Exp. Biol. 213, 26–32. doi: 10.1242/jeb.032136
Martinez M., Guderley H., Dutil J. D., Winger P. D., He P., Walsh S. J. (2003). Condition, Prolonged Swimming Performance and Muscle Metabolic Capacities of Cod Gadus morhua. J. Exp. Biol. 206, 503–511. doi: 10.1242/jeb.00098
McDonald D. G., Milligan C. L., McFarlane W. J., Croke S., Currie S., Hooke B., et al. (1998). Condition and Performance of Juvenile Atlantic Salmon (Salmo salar): Effects of Rearing Practices on Hatchery Fish and Comparison With Wild Fish. Can. J. Fish. Aquat. Sci. 55, 1208–1219. doi: 10.1139/cjfas-55-5-1208
Ministry of Agriculture and Rural Affairs of the People's Republic of China (2022). Guidance on Aquatic Stock Enhancement During the 14th Five Year Plan. Beijing: Fisheries and Fisheries Administration Press. Retrieved from: http://www.moa.gov.cn/govpublic/YYJ/202201/t20220127_6387852.htm
Mittelbach G. G., Osenberg C. W., Wainwright P. C. (1999). Variation in Feeding Morphology Between Pumpkinseed Populations: Phenotypic Plasticity or Evolution? Evol. Ecol. Res. 1, 111–128.
Näslund J. (2021). Reared to Become Wild-Like: Addressing Behavioral and Cognitive Deficits in Cultured Aquatic Animals Destined for Stocking Into Natural Environments-A Critical Review. Bull. Mar. Sci. 97, 489–538. doi: 10.5343/bms.2020.0039
Ohlberger J., Staaks G., Hölker F. (2006). Swimming Efficiency and the Influence of Morphology on Swimming Costs in Fishes. J. Comp. Physiol. B. 176, 17–25. doi: 10.1007/s00360-005-0024-0
Ojanguren A. F., Braña F. (2003). Effects of Size and Morphology on Swimming Performance in Juvenile Brown Trout (Salmo trutta L.). Ecol. Freshw. Fish. 12, 241–246. doi: 10.1046/j.1600-0633.2003.00016.x
Paéz D. J., Hedger R., Bernatchez L., Dodson J. J. (2008). The Morphological Plastic Response to Water Current Velocity Varies With Age and Sexual State in Juvenile Atlantic Salmon, Salmo salar. Freshw. Biol. 53, 1544–1554. doi: 10.1111/j.1365-2427.2008.01989.x
Pakkasmaa S., Piironen J. (2000). Water Velocity Shapes Juvenile Salmonids. Evol. Ecol. 14, 721–730. doi: 10.1023/A:1011691810801
Powell M. S., Hardy R. W., Hutson A. M., Toya L. A., Tave D. (2017). Comparison of Body Composition and Fatty Acid Profiles Between Wild and Cultured Rio Grande Silvery Minnows. J. Fish. Wildl. Manage. 8, 487–496. doi: 10.3996/072016-JFWM-055
Pulcini D., Wheeler P. A., Cataudella S., Russo T., Thorgaard G. H. (2013). Domestication Shapes Morphology in Rainbow Trout Oncorhynchus mykiss. J. Fish. Biol. 82, 390–407. doi: 10.1111/jfb.12002
Reidy S. P., Kerr S. R., Nelson J. A. (2000). Aerobic and Anaerobic Swimming Performance of Individual Atlantic Cod. J. Exp. Biol. 203, 347–357. doi: 10.1242/jeb.203.2.347
Riddell B. E., Swain D. P. (1991). Competition Between Hatchery and Wild Coho Salmon (Oncorhynchus kisutch): Genetic Variation for Agonistic Behaviour in Newly-Emerged Wild Fry. Aquaculture 98, 161–172. doi: 10.1016/0044-8486(91)90381-G
Robinson J. S., Mead J. F. (1973). Lipid Absorption and Deposition in Rainbow Trout (Salmo gairdnerii). Can. J. Biochem. 51, 1050–1058. doi: 10.1139/o73-137
Rohlf F. J. (2015). The Tps Series of Software. Hystrix. Ital. J. Mammal. 26, 9–12. doi: 10.4404/hystrix-26.1-11264
Rohlf F. J., Marcus L. F. (1993). A Revolution Morphometrics. Trends Ecol. Evol. 8, 129–132. doi: 10.1016/0169-5347(93)90024-J
Salvanes A. G. V., Braithwaite V. (2006). The Need to Understand the Behaviour of Fish Reared for Mariculture or Restocking. ICES J. Mar. Sci. 63, 346–354. doi: 10.1016/j.icesjms.2005.11.010
Schneider C. A., Rasband W. S., Eliceiri K. W. (2012). NIH Image to ImageJ: 25 Years of Image Analysis. Nat. Methods 9, 671–675. doi: 10.1038/nmeth.2089
Stringwell R., Lock A., Stutchbury C. J., Baggett E., Taylor J., Gough P. J., et al. (2014). Maladaptation and Phenotypic Mismatch in Hatchery-Reared Atlantic Salmon Salmo salar Released in the Wild. J. Fish. Biol. 85, 1927–1945. doi: 10.1111/jfb.12543
Swain D. P., Riddell B. E., Murray C. B. (1991). Morphological Differences Between Hatchery and Wild Populations of Coho Salmon (Oncorhynchus kisutch): Environmental Versus Genetic Origin. Can. J. Fish. Aquat. Sci. 48, 1783–1791. doi: 10.1139/f91-210
Thorpe J. E. (2004). Life History Responses of Fishes to Culture. J. Fish. Biol. 65, 263–285. doi: 10.1111/j.0022-1112.2004.00556.x
Tiffan K. F., Connor W. P. (2011). Distinguishing Between Natural and Hatchery Snake River Fall Chinook Salmon Subyearlings in the Field Using Body Morphology. Trans. Am. Fish. Soc. 140, 21–30. doi: 10.1080/00028487.2011.545003
Venney C. J., Wellband K. W., Heath D. D. (2021). Rearing Environment Affects the Genetic Architecture and Plasticity of DNA Methylation in Chinook Salmon. Heredity 126, 38–49. doi: 10.1038/s41437-020-0346-4
Von Cramon-Taubadel N., Ling E. N., Cotter D., Wilkins N. P. (2005). Determination of Body Shape Variation in Irish Hatchery-Reared and Wild Atlantic Salmon. J. Fish. Biol. 66, 1471–1482. doi: 10.1111/j.0022-1112.2005.00698.x
Wang K., Li C. W., Wang Z. H., Zhao J., Zhang S. Y. (2017). Feeding Habits of the Marbled Rockfish Sebastiscus marmoratus in the Marine Ranching Off Ma'an Archipelago, China. Chin. J. Appl. Ecol. 28, 2321–2326. doi: 10.13287/j.1001-9332.201707.035
Wang Y. B., Wang Y. C., Liu S. D., Liang C., Zhang H., Xian W. W. (2020). Stock Assessment Using LBB Method for Eight Fish Species From the Bohai and Yellow Seas. Front. Mar. Sci. 7. doi: 10.3389/fmars.2020.00164
Weatherley A. H., Gill H. S. (1981). Recovery Growth Following Periods of Restricted Rations and Starvation in Rainbow Trout Salmo gairdneri Richardson. J. Fish. Biol. 18, 195–208. doi: 10.1111/j.1095-8649.1981.tb02814.x
Wringe B. F., Fleming I. A., Purchase C. F. (2015). Rapid Morphological Divergence of Cultured Cod of the Northwest Atlantic From Their Source Population. Aquacult. Environ. Interact. 7, 167–177. doi: 10.3354/aei00145
Wringe B. F., Purchase C. F., Fleming I. A. (2016). In Search of a “Cultured Fish Phenotype”: A Systematic Review, Meta-Analysis and Vote-Counting Analysis. Rev. Fish. Biol. Fish. 26, 351–373. doi: 10.1007/s11160-016-9431-4
Wu C. W. (1999). Biological Studies on Sebastiscus marmomtus Off Zhoushan. J. Zhejiang. Ocean. Univ. 18, 185–190.
Yan Y. L., Liu Y. S., Shi Y. H., Xie Y. D., Deng P. P., Yuan X. C. (2018). Study on Indoor Artificial Breeding Technology of Sebastiscus marmoratus. Fish. Sci. Technol. Inf. 45 (06), 322–326. doi: 10.16446/j.cnki.1001-1994.2018.06.007(inChinese
Yokogawa K., Iguchi M. (1992). Food Habit and Maturation of Marbled Rockfish, Sebastiscus Marmoratus in Southern Coastal Waters of the Harima Sea. Aquacult. Sci. 40, 131–137. doi: 10.11233/aquaculturesci1953.40.131
Závorka L., Larranaga N., Lovén Wallerius M., Näslund J., Koeck B., Wengström N., et al. (2019). Within-Stream Phenotypic Divergence in Head Shape of Brown Trout Associated With Invasive Brook Trout. Biol. J. Linn. Soc. 129, 347–355. doi: 10.1093/biolinnean/blz192
Zelditch M. L., Swiderski D. L., Sheets H. D., Fink W. L. (2004). Geometric Morphometrics for Biologists (Amsterdam: Elsevier Academic Press).
Zhang X. G., Guo H. Y., Zhang S. Y., Song J. K. (2015). Sound Production in Marbled Rockfish (Sebastiscus marmoratus) and Implications for Fisheries. Integr. Zool. 10, 152–158. doi: 10.1111/1749-4877.12105
Zhao Z. L., Liang R. F., Wang Y. M., Yuan Q., Zhang Z. G., Li K. F. (2020). Study on the Swimming Ability of Endemic Fish in the Lower Reaches of the Yangtze River: A Case Study. Global Ecol. Conserv. 22, e01014. doi: 10.1016/j.gecco.2020.e01014
Zhou L. Y., Yan X. Y., Li X. M., Fu X., Xia J. G., Fu S. J. (2019). Effect of Exercise Training on Swimming Performance, Survival Under Predation and Hypoxia Tolerance in an Endangered Fish Species in China. Mar. Freshw. Behav. Physiol. 52, 67–82. doi: 10.1080/10236244.2019.1636653
Zhu Y. (2020). Study on the Suitability of Important Links of Reef Fish Breeding and Release [Master’s Thesis, Shanghai: Shanghai Ocean University] (Institutional Repository at the Shanghai Ocean University). Available at: http://cdmd.cnki.com.cn/Article/CDMD-10264-.
Keywords: morphological variation, geometric morphometrics, burst swimming speed, critical swimming speed, body composition
Citation: Guo H, Zhang X, Näslund J, Peng L, Liu C, Tian M, Chai X, Zhang D and Zhang X (2022) Differences in External Morphology, Body Composition and Swimming Performance Between Hatchery- and Wild-Origin Marbled Rockfish (Sebastiscus marmoratus). Front. Mar. Sci. 9:912129. doi: 10.3389/fmars.2022.912129
Received: 04 April 2022; Accepted: 09 May 2022;
Published: 16 June 2022.
Edited by:
Micheal S. Allen, University of Florida, United StatesCopyright © 2022 Guo, Zhang, Näslund, Peng, Liu, Tian, Chai, Zhang and Zhang. This is an open-access article distributed under the terms of the Creative Commons Attribution License (CC BY). The use, distribution or reproduction in other forums is permitted, provided the original author(s) and the copyright owner(s) are credited and that the original publication in this journal is cited, in accordance with accepted academic practice. No use, distribution or reproduction is permitted which does not comply with these terms.
*Correspondence: Xiumei Zhang, eGl1bWVpMTIyN0AxNjMuY29t
Disclaimer: All claims expressed in this article are solely those of the authors and do not necessarily represent those of their affiliated organizations, or those of the publisher, the editors and the reviewers. Any product that may be evaluated in this article or claim that may be made by its manufacturer is not guaranteed or endorsed by the publisher.
Research integrity at Frontiers
Learn more about the work of our research integrity team to safeguard the quality of each article we publish.