- 1Key Laboratory of East China Sea and Oceanic Fishery Resources Exploitation, Ministry of Agriculture, Shanghai, China
- 2East China Sea Fisheries Research Institute, Chinese Academy of Fishery Sciences, Shanghai, China
- 3Ocean College of Agriculture University of Hebei, Qinghuangdao, China
- 4Hebei Provincial Technology Innovation Center for Coastal Ecology Rehabilitation, Tangshan Marine Ranching Co. Ltd., Tangshan, China
- 5Yantai Institute of Coastal Zone Research, Chinese Academy of Sciences, Yantai, China
Aquaculture farms are known to attract wild organisms from nearby areas. Sea cucumber Apostichopus japonicus aquaculture farms in Bohai Bay, the Bohai Sea, China, might provide spawning and nursery grounds for wild black rockfish Sebastes schlegelii populations. To identify the above, we studied the variation in the numbers of S. schlegelii larvae (and length-categories as a percentage of the natural population) via ichthyoplankton surveys by months from January to October 2020, and assessed the food web structure and energy flow distribution of the farm and trophic links of S. schlegelii natural stock by using Ecopath modeling in July 2016 to August 2017. Larvae with a length between 3.90-5.80 mm were observed in the farm in May 2020, the juveniles with an estimated trophic level of 4.31. Juveniles used the farms as first-year nursery ground, and then they swam into deeper waters in August of the following year. MTI analysis showed increasing biomass of S. schlegelii might result in a decrease in the biomass of crustacea, and had positive and negative impacts on mollusk and Hexagrammos otakii biomass. We argued that aquacultural infrastructures constructed by artificial reefs could be used as a fisheries management tool to enhance S. schlegelii stocks and that monitoring of other marine organisms, such as oysters, Rapana venosa, Charybdis japonica, and Asterias spp. etc., was necessary to maintain sustainable exploitations of aquacultural farm ecosystems. A paucity of knowledge surrounding the interactions between aquaculture farms and wild organisms needs furthermore researches.
Highlights:
1. Spawning and first-year nursery grounds of S. schlegelii being in the farms.
2. Aquacultural infrastructures constructed by artificial reefs might enhance S. schlegelii populations.
Introduction
Aquaculture farms (for fishes, shellfishes, crustaceans, seaweeds, etc.) in coastal areas attract invertebrates (e.g. Pandalus borealis, Olsen et al., 2012; Homarus americanus, Drouin et al., 2015), various fishes (Callier et al., 2017), marine mammals (e.g. grey seals Halichoerus grypus on the Atlantic coast, Nash et al., 2000; monk seals Monachus monachus in the Turkish Aegean Sea, Güçlüsoy and Savas, 2003; sea lions Otavia flavescens in Chile, Sepulveda and Oliva, 2005; bottlenose dolphins Tursiops truncatus in the Mediterranean Sea and along the coast of Italy, Díaz-López and Bernal-Shirai, 2007, Bearzi et al., 2009) and various birds (e.g. Nycticorax nycticorax and Phalacrocorax auritus, Bradley et al., 2000) at both the individual and population levels. The distribution of organisms attracted to aquaculture farms varies over various temporal (day and season) and spatial scales (horizontal and vertical) (Valle et al., 2007; Fernandez-Jover et al., 2008; Sudirman et al., 2009; Uglem et al., 2009; Dempster et al., 2010; Goodbrand et al., 2013; Arechavala-Lopez et al., 2015; Bacher et al., 2015). Coastal aquacultural infrastructures such as fish cages, cement blocks, ropes, anchors, buoys, nets, stones, and other man-made submerged structures are thought to artificially aggregate fish by providing food and habitats (Bayle-Sempere et al., 2013; Kluger et al., 2017). Many studies have shown that the total biomass and abundance of wild fishes in aquaculture farms were dozens of times greater than in the surrounding area, e.g. coastal Atlantic salmon farms in Norway (Dempster et al., 2010), oyster aquaculture in Rhode Island (Tallman and Forrester, 2007). Thus, Aquacultural infrastructures might be a fisheries management tool to add fisheries resources and coastal aquaculture farms might be considered as artificial ecosystems.
In China, industrial aquaculture production of the sea cucumber Apostichopus japonicus was worth $ 46.38 billion USD in 2020. The area under aquaculture in China and its production reached 242,813 km2 and 196,564 t in 2020, respectively (Fisheries and Fisheries administration et al., 2021). Of this, 1,000 km2 (China Fisheries Association, 2021) of coastal aquaculture farms have been constructed using the deployment of artificial hard substrates, such as stones, to create so-called “sea ranching” (Yang, 2016). In 2013, a local community in the study area established a 2 km2 sea cucumber aquaculture farm by deploying stones and artificial reefs (Xu et al., 2021). These hard substrates were gradually colonized naturally by native pacific oysters (Crassostrea gigas), to create artificial marine oyster reefs. We observed wild Sebastes schlegelii juveniles between 30-60 mm long inside the farm in July 2016. However, sea cucumber aquaculture farms in China have been suggested to only attract these wild S. schlegelii individuals without increasing their production via enhancing natural stocks.
On the other hand, S. schlegelii, this species called ‘Kurosoi’ in Japanese, is an ovoviviparous fish that is widely distributed in the coastal waters of the western North Pacific, including the northern coastlines of China and the coastal areas of Japan (except for the Ryukyu islands and the Korean Peninsula) (Yamada et al., 2007). Owing to its high commercial value, fast growth, and limited migration, it is a promising species for aquaculture and recreational fisheries in northeastern Asia, including China, Japan, and Korea (Lee, 2002). In Japan, their adults mate in winter, after which the females migrate to shallow coastal waters to give birth to larvae in the spring (Takahashi et al., 1994). Juveniles use shallow Sargassum and eelgrass beds in the innermost part of the bay as a nursery area during their first year, and then start to migrate to deeper waters in around August of the following year, as water temperature decreases (Sasaki et al., 2002, Nakagawa, 2008). In China, whether juveniles of this species also use aquaculture farms as first-year nursery grounds is unclear.
In this study, we aim to: (1) verify whether sea cucumber aquaculture farms provide spawning and first-year nursery grounds for wild S. schlegelii populations by identifying larvae and the relative occurrence of length-categories of populations by month; (2) understand the food web structure and energy flow distribution of the farm and the trophic links of wild S. schlegelii by constructing a trophic model using Ecopath. The results bring benefits to understand ecosystem-based aquaculture farm management and the dynamic relationships between aquaculture farms and wild organisms. Also, we argue that it is possible to use aquacultural infrastructures constructed by artificial reefs as a fisheries management tool to enhance fishery resources.
Material and methods
Ethical statement
Samples, including larval collections in the study area, were authorized by the local fisheries community. All procedures were performed following the American Fisheries Society guidelines for the use of fishes and crustaceans in research (Jenkins et al., 2014). The study was approved by the ethics committee of the East China Sea Fisheries Research Institute, Chinese Academy of Fishery Sciences. The study did not involve any endangered or protected species as listed in the China Red Data Book of Endangered Animals.
Study site and sampling
The survey area (39°7’-39°11’N, 118°58.55’-119°2.55’E) is adjacent to Xiangyun Island, located inside the estuary of the Luanhe River, at the northeast part of Bohai Bay, the Bohai Sea (Figure 1). The Luanhe River is 1,200 km long and arises at the foot of the Yanshan Mountains and flows into Bohai Bay. In 2013, local fishermen deployed stones and artificial reefs (ARs) in the sea to create a ca. 2 km2 sea cucumber aquaculture farm, surrounded by a 4 km long, 8 m height breakwater to protect against wave damage (Figure 1).
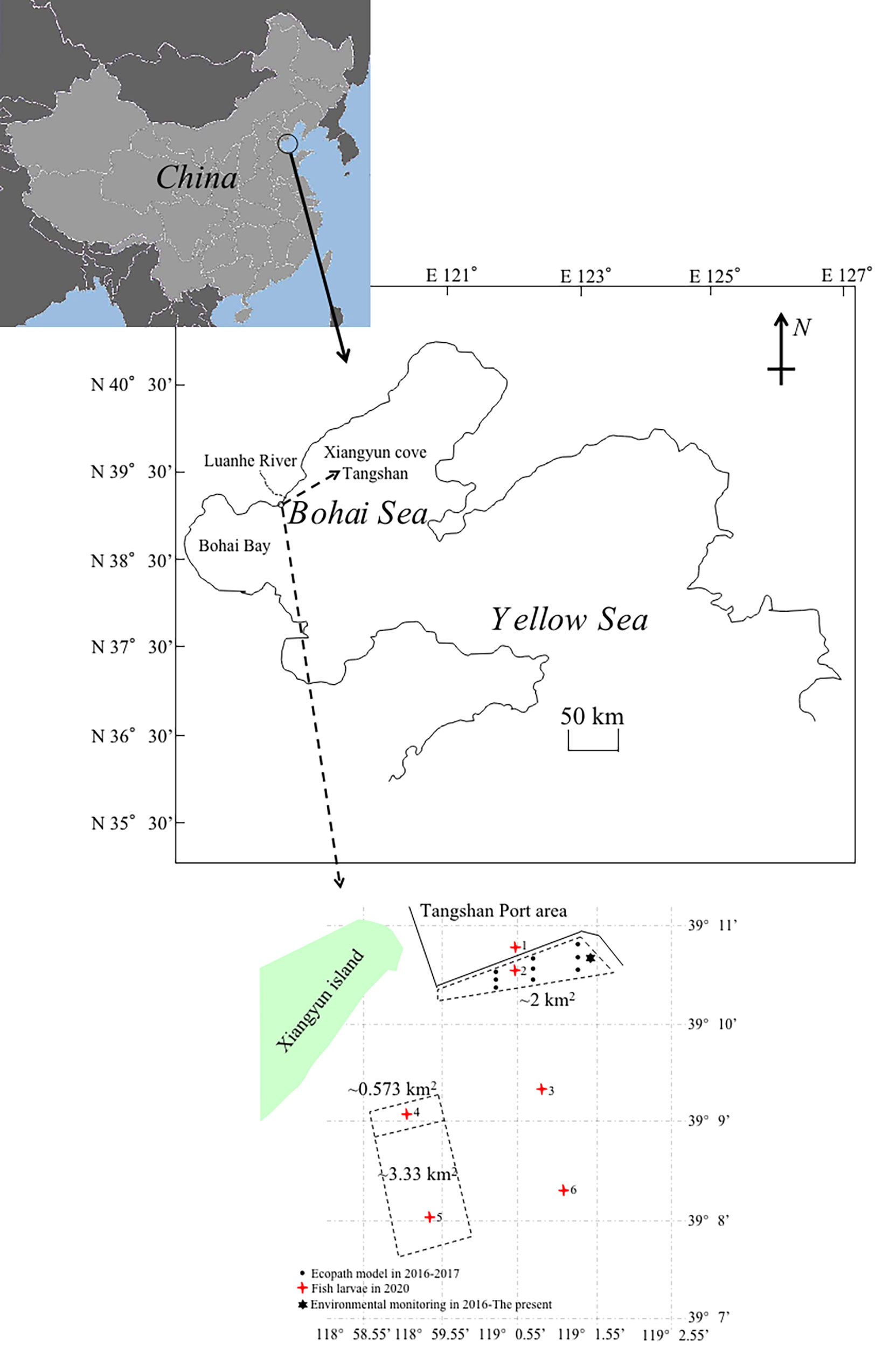
Figure 1 Schematic map showing the survey area in the northernmost part of Bohai Bay, the Bohai Sea, China (39°7’-39°11’N, 118°58.55’-119°2.55’E). Showing: 16 stations, including 12 sites inside the area of artificial reefs (ARs); 3 control sites (Nos. 1, 3 and 6); and 1 environmental monitoring station in Xiangyun cove, adjacent to Xiangyun Island and the Tangshan Port area. The white trapezoid and rectangles surrounded by black dashed lines represent: 3,000 mu (~2 km2) of ARs created in 2013; 860 mu (~0.573 km2) of ARs created in 2019; and 5,000 mu (~3.333 km2) of ARs created in 2020. One mu is approximately 666.667 m2. We established one environmental monitoring station (denoted by a black hexagon), operated from 2016 to the present, and nine sampling stations (denoted by black dots) in 2016 to 2017 to create the Ecopath model, and six sampling stations (denoted by red square stars) created in 2020 to sample Sebastes schlegelii larvae.
To identify whether aquaculture farms provide spawning grounds for the wild S. schlegelii population, we operated six stations to collect larval samples from January to October 2020 (Figure 1). We used a 0.505 mm mesh ichthyoplankton net (0.80 m diameter, 3.50 m length) to collect S. schlegelii larval samples. We sampled horizontally and vertically (raising the nets from the bottom to the surface) in 10 min sweeps from a vessel travelling at a speed of 1-2 knots. The samples were immediately preserved in seawater with 5% formalin in situ and identified to the lowest possible taxonomic level in the laboratory. The larvae of S. schlegelii were measured to the nearest 0.1 mm from the front of the snout to the terminal end of the crest bone using a light microscope (Sterm 2000, Karl Zeiss, Germany) (Pattrick and Strydom, 2014).
To describe the food web structure and energy flow distribution of the farm and trophic links among wild S. schlegelii adult stock, between July 2016 and August 2017 (sampled in July 2016, September 2016, November 2016, December 2016, January 2017, March 2017, April 2017, May 2017, June 2017, July 2017, and August 2017), we established nine sampling stations to collect biomass data for our Ecopath model precisely and from August 2016 to the present established one environmental monitoring station to measure water temperature (°C) and dissolved oxygen (DO) (mg L-1) in the farm (Xu et al., 2021). We used crab pots, gill nets, and trawl nets to collect samples and estimate the biomass of functional groups (measured in t km-2) in the farm. The samples were identified to the lowest possible taxonomic level, and species were counted and measured to an accuracy of ±1 mm using a 40 cm ruler, and weighed to an accuracy of ±0.1 g using a balance (JJ-Y series, SSWA).
Inputs, adjustments, and calibrations of the Ecopath model
The Ecopath approach and modeling software (Ecopath with Ecosim Version 6.5) (Christensen and Pauly, 1992; Christensen and Walters, 2004) were used in the study to assess food web structure and energy flow distribution of the farm and the trophic links of wild S. schlegelii. It is expressed by the mass-balance equation:
where Pi represents the production of group i, Bi represents biomass in tons (wet weight), and M2i represents mortality by predation. EEi represents ecotrophic efficiency and EXi represents export, and BAi represents the biomass accumulation of i during the study period.
The MTI was calculated by constructing an n x n matrix, where (Xu et al., 2019):
1. the i, jth element represents the interaction between the impacting group i and the impacted group j where MTI i,j=DCi,j-FCj,i;
2. DCi,j is the diet composition term denoting how much j contributes to the diet of i;
3. FCj,i is the host composition term giving the proportion of the predation on j that is due to i as a predator.
The composition of the functional groups of marine organisms were separated based on dietary similarity, preferred physical habitat, and other ecological similarities and were described in Table 1. The functional groups had to span all of the trophic system components and all the fishing targets in the study area. Marine fishes and crustaceans of high economic value (e.g. S. schlegelii, Pholis fangii, Hexagrammos otakii, Oratosquilla oratoria, Charybdis japonica, Rapana venosa, and A. japonicus) and large biomass oysters were assigned to separate distinct groups. We determined the input values of Biomass (B), Production/Biomass (P/B), Consumption/Biomass (Q/B), Ecotrophic efficiency (EE), and landing data for all functional groups using the Fishbase platform (Table 2). The P/B and Q/B values of fishes were determined using empirical regression of Pauly (1980) with the Fishbase platform. Input values were set equal to the averages of the parameters for the individual group members, weighted by their relative biomass. The Ecopath model generated the missing parameter values. The dietary composition of each functional group was then determined using data obtained from the literature and this study (Table 3). These data showed that each consumer group had a diet consisting of one or more other groups. Thus, each prey item was entered as a proportion of the consumer’s diet, and adjustments were made to obtain a balanced model. Most resident species fed both inside and outside of the aquaculture farm and could therefore be regarded as importing food items in their dietary composition (Xu et al., 2021). Flows were expressed in t wet weight km-2 year-1, and biomasses were expressed in t wet weight km-2. When a balanced model was established, Ecopath was used to calculate a trophic level (TL) for each group on the basis of its diet. Adjustments and calibrations of the parameters in the model followed the procedures of Xu et al., 2019 and Xu et al., 2021.
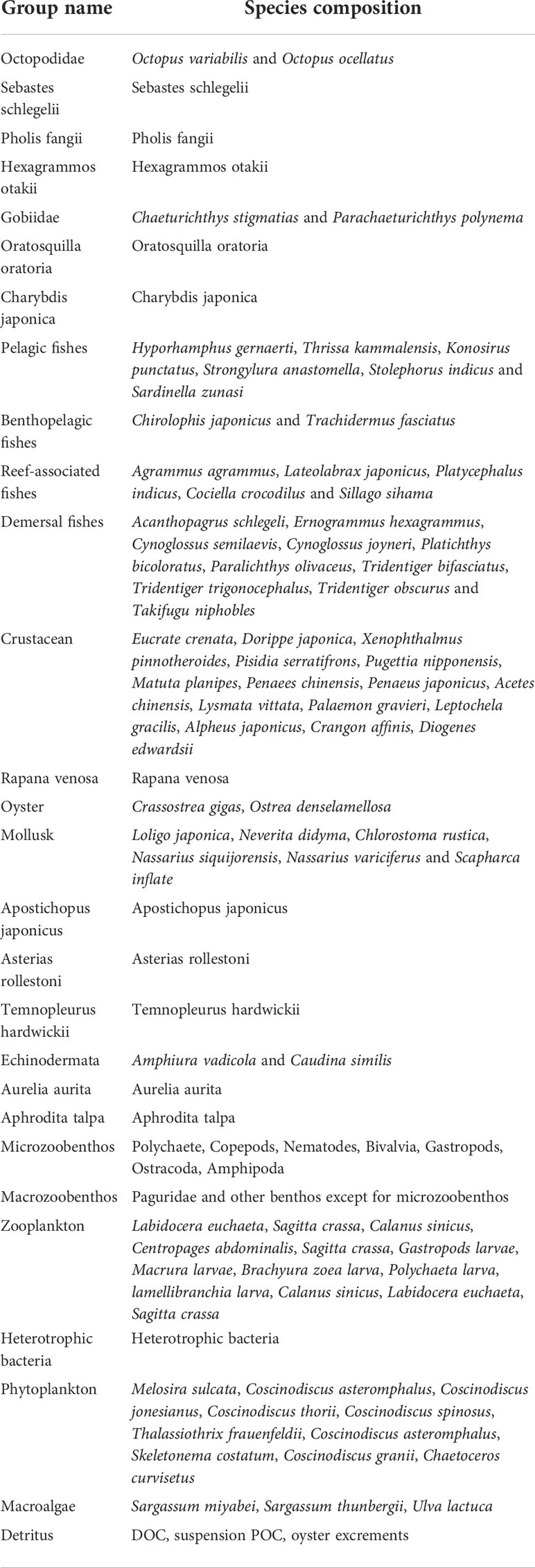
Table 1 Description of species composition of the function groups on the sea cucumber aquaculture farm (~2 km2) adjacent to the artificial breakwaters in Xiangyun cove, Bohai Bay, the Bohai Sea, China, between July 2016 and August 2017.
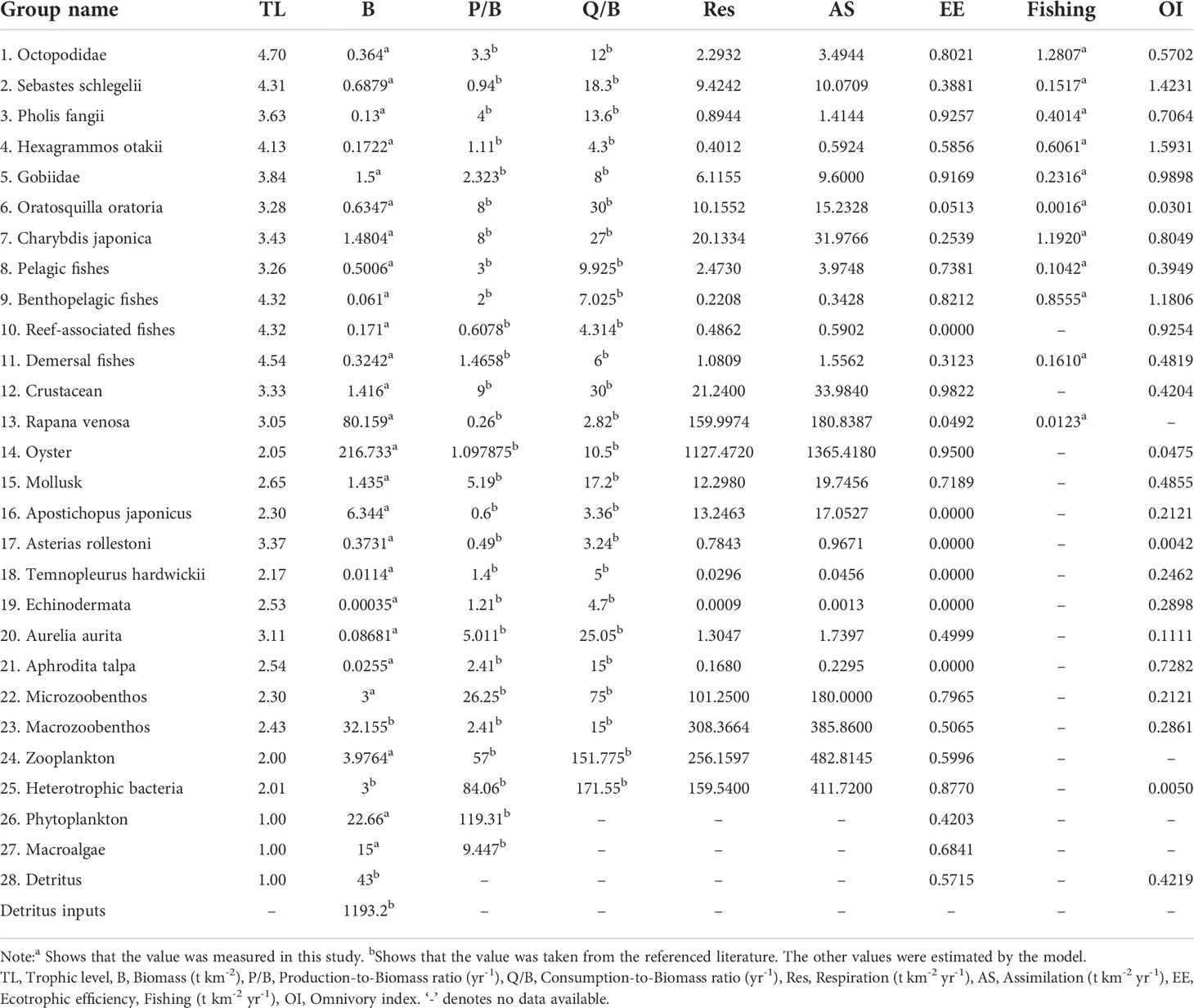
Table 2 The parameter values of each functional group in a sea cucumber farm (~2 km2) in Xiangyun cove, Bohai Bay, the Bohai Sea, China, from an Ecopath model from July 2016 to August 2017.
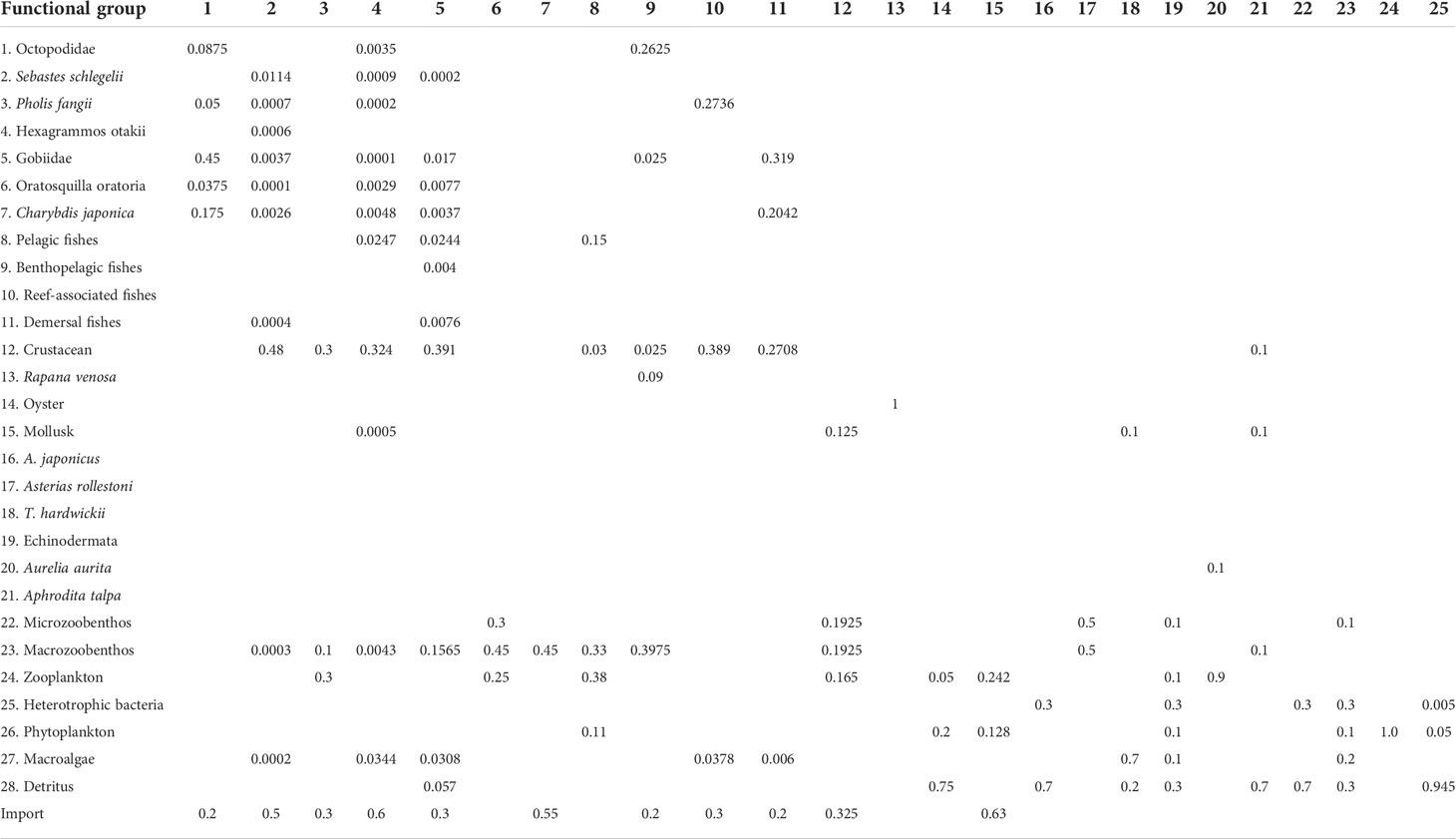
Table 3 The dietary composition of the functional groups in the sea cucumber aquaculture farm (~2 km2) in Xiangyun cove, Bohai Bay, the Bohai Sea, China, derived from July 2016 to August 2017.
Results
The variation in length of S. schlegelii larvae
Larvae of S. schlegelii with lengths range of 3.90-5.80 mm were found in May 2020 (Figure 2). Most were found inside the farm in early May at a water temperature of 12.73°C, but larger individuals swam outside the farm in late May (Figure 2). More individuals were found at the bottom of the water column than at the surface. The ranges of water temperature (increasing trend) and DO (decreasing trend) were 12.73-17.73°C and 4.65-9.40 mg L-1 in May 2020, respectively (Figure 2).
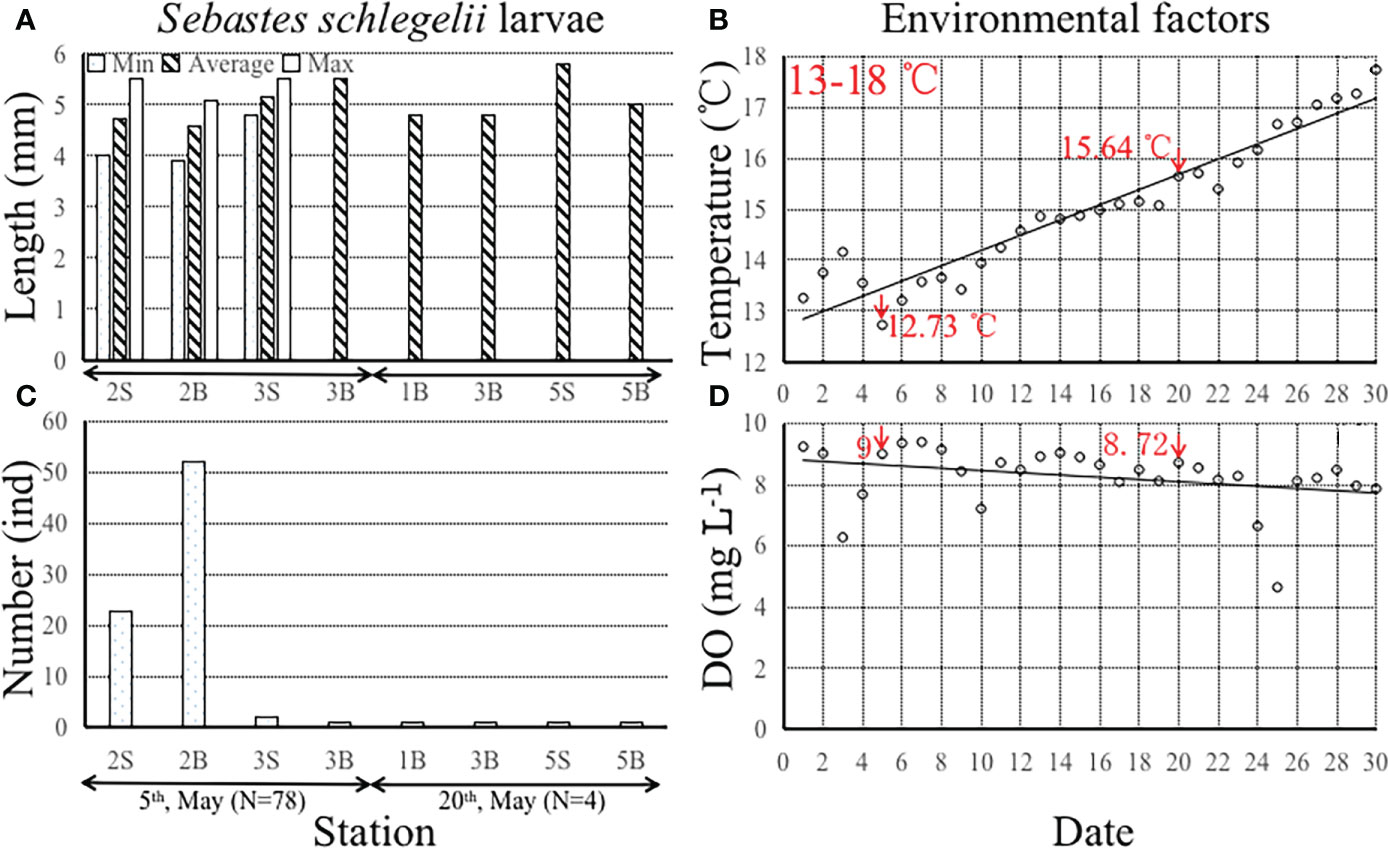
Figure 2 (A) The minimum, average and maximum length (mm) of Sebastes schlegelii larvae at the different sampling stations between 5th May and 20th May 2020 (upper-left); (B) The number (ind) of Sebastes schlegelii larvae at the different stations between 5th May (Total number=78 ind) and 20th May 2020 (Total number=4 ind) (bottom-left);(C) The water temperature increase (°C) from 1st May to 30th May 2020 (upper-right); (D) The dissolved oxygen content (DO, mg L-1) decreased from 1st May to 30th May 2020 (bottom-right). Notes: “S” denotes the sea surface; “B” denotes the sea bottom. Red arrowheads point to the sampling dates. All the measurements of water temperature and DO were taken at an environmental monitoring station at 10:00 am between 1st May and 30th May 2020.
The monthly variation in length-categories percentage of the population
In July 2016 and 2017, the majority of S. schlegelii were in the 30-59, 60-89, 90-119 mm length-categories, with very few older individuals of >120 mm length. They were similar in average length (56.34 versus 61.26 mm, respectively) at similar average water temperatures (25.81 ± 0.23 versus 25.36 ± 0.71°C respectively). Between August and December, the overall average length increased from 92.40 to 102.94 mm, while the average water temperature decreased from 26.58 ± 1.00 to 5.01 ± 0.80°C. The percentage of length-categories >90 mm increased from March to May. The 0-29 mm category was also observed in May 2017 (Figure 3). The total number and average length (77.50→88.45→94.30→106.59 mm) of fish increased from January→March→April→May, as average water temperature rose from 0.06→4.84→11.34→16.98°C. In particular, we found two especially large fish (L=225.00 mm, W=297.70 g; L=203.00 mm, W=270.00 g) about to spawn in March and two even larger ones (L=235.00 mm, W=395.60 g; L=215.00 mm, W=262.80 g) which finished spawning in the farm in May.
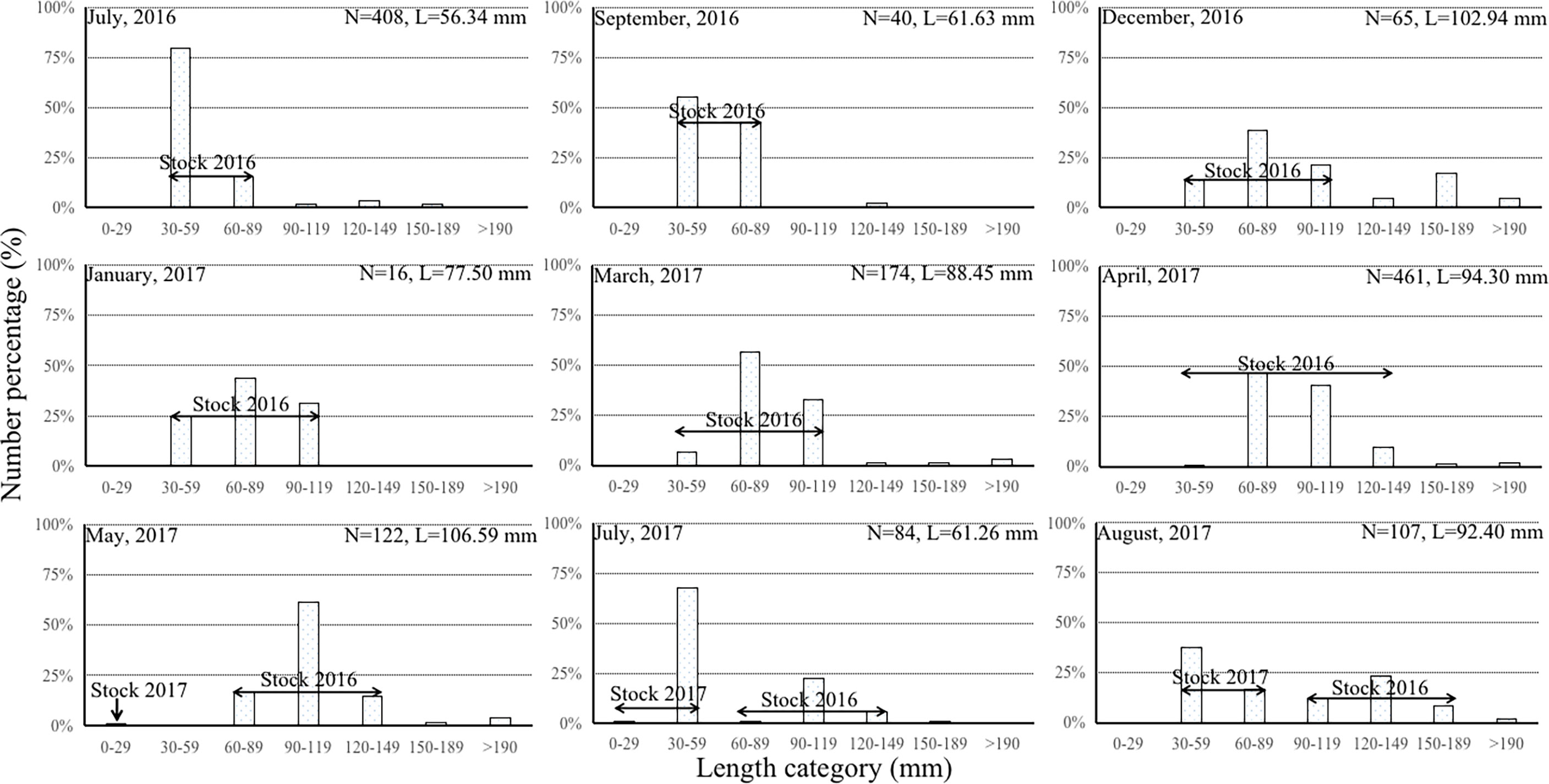
Figure 3 Percentage (%) of Sebastes schlegelii against length category (mm) from 0–29 mm to >190 mm in 30 mm length intervals from July 2016 to August 2017 in a ~2 km2 area of the sea cucumber farm. N denotes total number of individuals; L denotes average length of individuals.
MTI analysis and trophic links of S. schlegelii in the farm
We performed MTI analyses of the functional groups of organisms. The increasing biomass of detritus increased the biomass of benthic species and oysters, leading to an increasing biomass of O. oratoria, R. venosa, and A. rollestoni. The increasing biomass of oysters decreased the biomass of phytoplankton, zooplankton and detritus, which decreased the biomass of O. oratoria, A. japonicus, Echinodermata, A. aurita, A. talpa, microzoobenthos, A. rollestoni, and heterotrophic bacteria. The increasing biomass of R. venosa decreased the biomass of oysters, which led to an increase in biomass of zooplankton and detritus, and had a positive impact on O. oratoria, A. japonicus, Echinodermata, A. aurita, A. talpa, microzoobenthos, and heterotrophic bacteria. The increasing biomass of C. japonica decreased the biomass of macrozoobenthic species, thus increasing the biomass of T. hardwickii, microzoobenthos and macroalgae and decreasing the biomass of H. otakii, benthopelagic fishes and R. venosa. The increasing biomass of Gobiidae decreased the biomass of crustacea, which had a negative impact on fishes such as P. fangii, H. otakii, pelagic fishes, benthopelagic fishes, reef-associated fishes, and demersal fishes.
The trophic level of S. schlegelii in the farm was 4.31 compared with the Octopodidae, the highest ranked at 4.70. S. schlegelii and other fish groups such as benthopelagic fishes, reef-associated fishes, and demersal fishes were all top predators. The increasing biomass of S. schlegelii caused a decrease in the biomass of crustaceans, but had a positive and negative impact on mollusks and H. otakii, respectively (Figure 4).
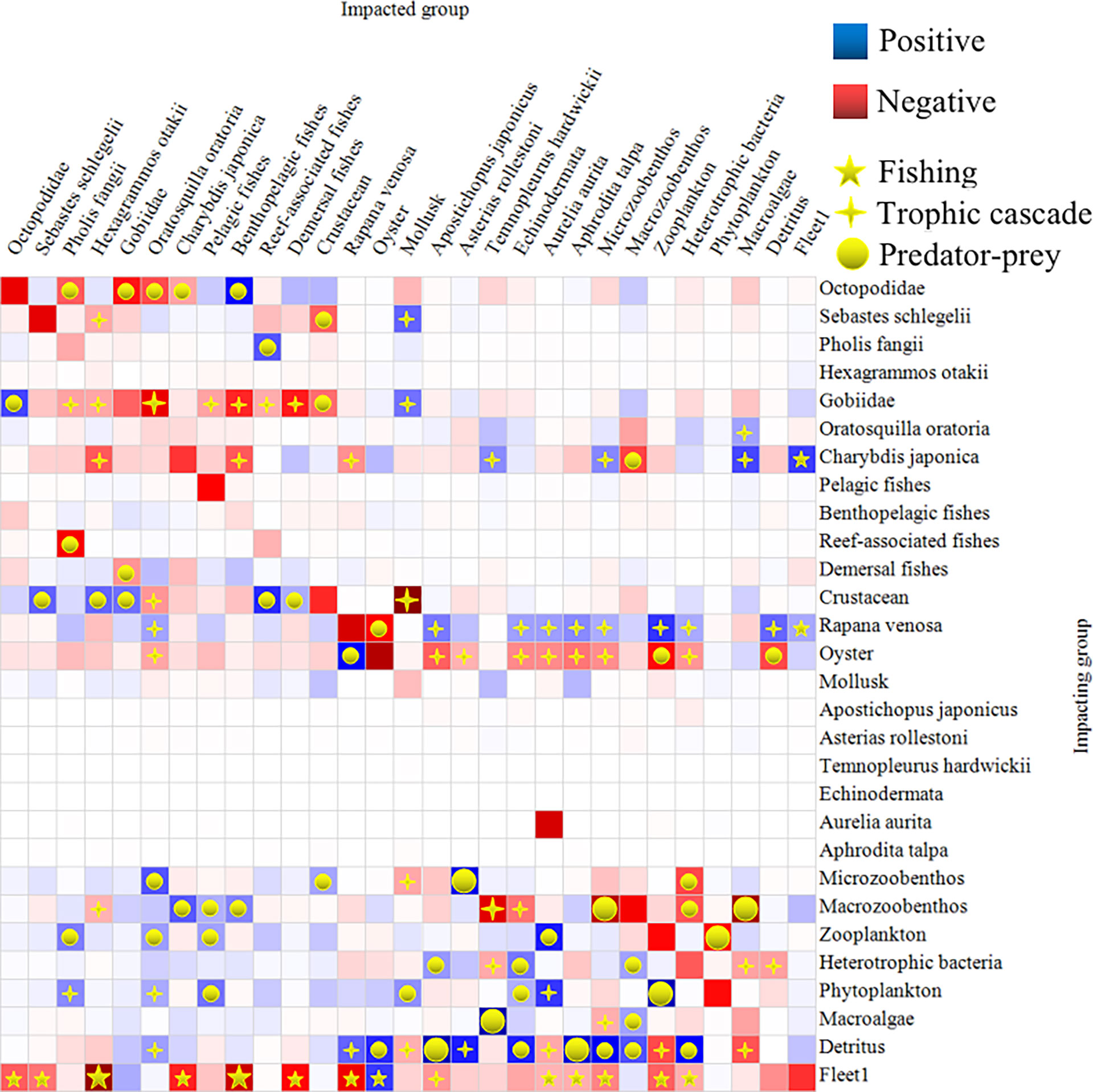
Figure 4 The result of a mixed trophic impact (MTI) analysis of the sea cucumber aquaculture farm ecosystem from 2016 to 2017. Impacting and impacted groups are shown along the vertical and horizontal axes, respectively. Positive and negative impacts are shown as blue and red squares, respectively. The squares should not be interpreted in an absolute sense: the impacts are relative, but comparable between groups. The darkness of the color indicates the degree of the impact. Trophic relationships of fishing, trophic cascades and predator-prey interaction are denoted by a yellow five-pointed star, a yellow cross-star and a yellow circular symbol. Impact values of 0.1–0.4 and > 0.4 are denoted by small and large symbols, respectively.
Food web structure and energy flow distribution of the farm
In terms of food web structure, phytoplankton and macroalgae were the primary producers. Oysters and A. japonicus relied largely on detritus and, in addition to marcoalgae and phytoplankton, were the main prey of zoobenthic and zooplanktonic species. Secondary consumers such as the Gobiidae, crustacea, and top predators such as Octopodidae, were the key functional groups (Figure 5).
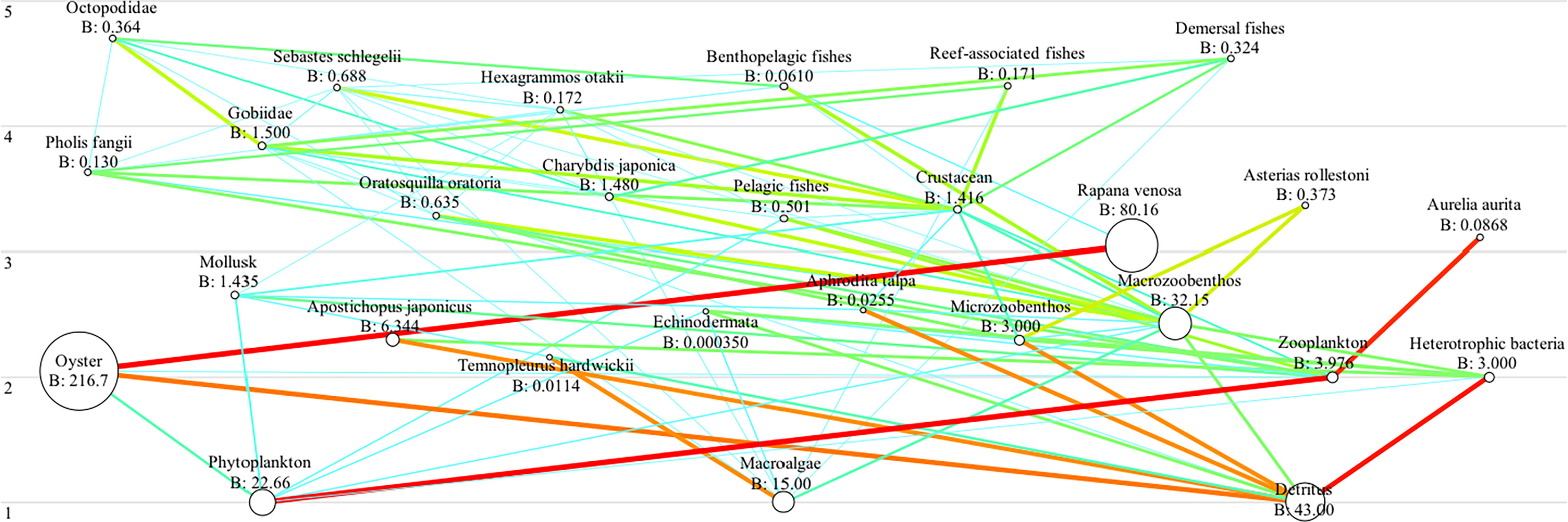
Figure 5 A flow diagram representing the food web structure in terms of functional groups and fractional trophic levels in the sea cucumber farm (~2 km2) in Xiangyun cove, Bohai Bay, the Bohai Sea, China in 2016-2017. Circles are distributed on the Y-axis according to trophic level (1-5), the size of the circle being proportional to each group’s biomass, the thickness of lines represented by different colors showing the strength of trophic links. Biomass is given in t km−2.
The transfer efficiency ranges in total flow, producer and detritus were 9.09-12.45%, 8.52-12.31% and 7.46-13.75%, respectively, varying among trophic levels II to VII. The transfer efficiency of producers in trophic levels II and III was higher than that of detritus, but an opposite result was observed in trophic levels IV and V. Most of the biomass in the ecosystem was in trophic levels I to III. The biomass in trophic levels II and III was 239.60 and 106.10 t km-2 year-1, respectively. The range of biomass in trophic levels V to VII was 0.006-0.86 t km-2 year-1. The first and second highest catches were 2.21 t km-2 year-1 in trophic level III and 1.25 t km-2 year-1 in trophic level IV. The energy flow value in the throughput item was equal to the sum of the import item, consumption item, export item, flow to detritus item and respiration item. In trophic level I, the first and second highest energy flow values were for the consumption item (3744.00 t km-2 year-1) and export item (1883.00 t km-2 year-1). In trophic levels II to VII, the energy flow value in the respiration item was highest, and the second highest item was the flow to detritus item. Energy flows in the throughtput item decreased with increasing trophic level (Table 4).
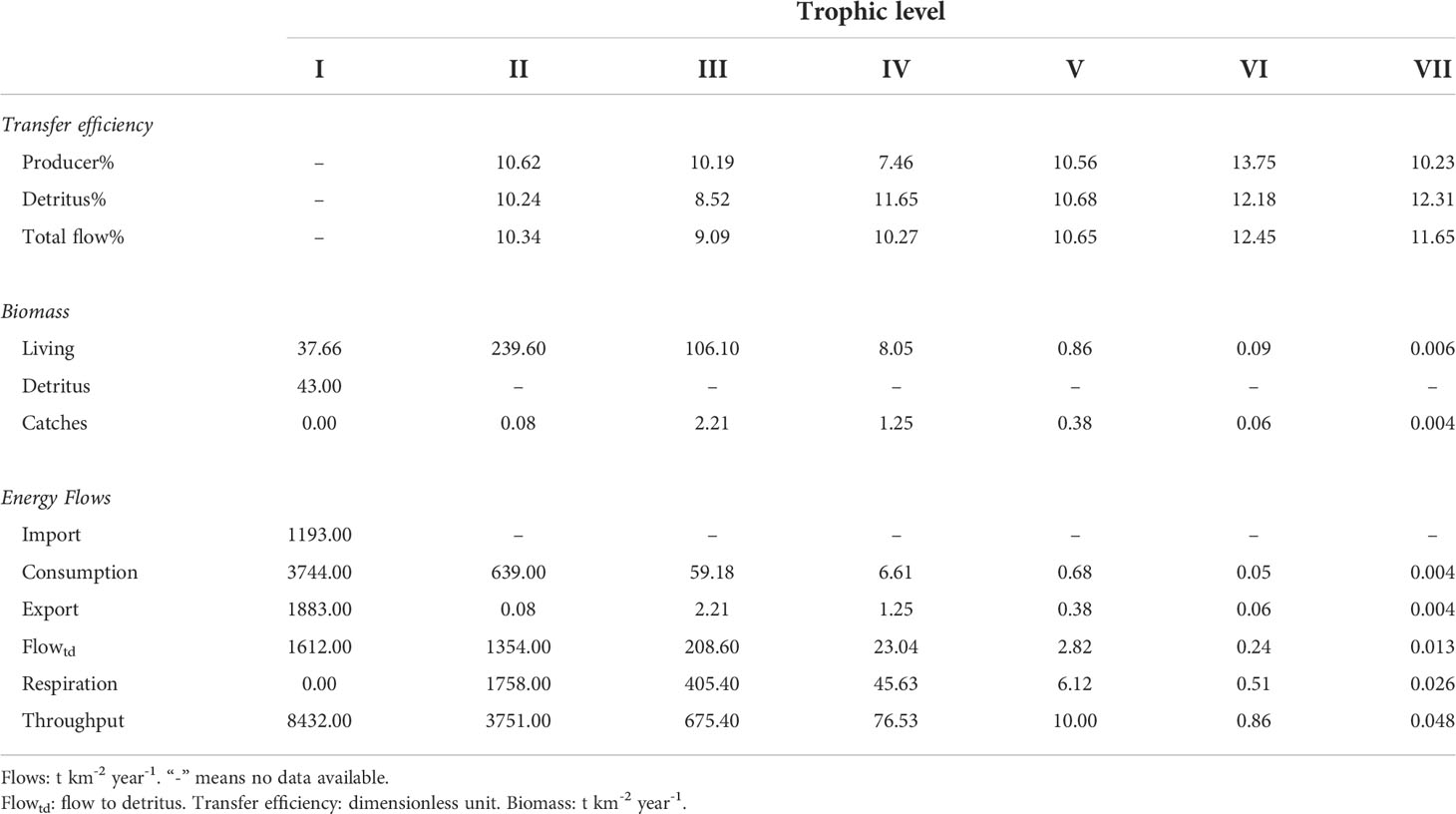
Table 4 Transfer efficiency, biomass and energy flows at various TLs (I-VII) showing the contribution of detritus and primary production to the trophic network in Xiangyun wan, Bohai Bay, Bohai Sea, China.
Discussion
Aquaculture farm infrastructures have been shown to enhance wild populations of sessile and mobile species, including bivalves, macroalgae, bryozoans, other mollusks, and tunicates. For example, The increased numbers of large crustaceans contribute perhaps 2.80 t ha-1 yr-1 to production on oyster reefs (zu Ermgassen et al., 2016). The productivity of invertebrates in the vicinity of Perna canaliculus mussel beds in New Zealand was 3.5 times greater than on nearby soft sediments (McLeod et al., 2013). The epifauna on an artificial reef in Delaware Bay, USA was found to be between 147 and 895 fold greater than in the surrounding area (Foster et al., 1994). However, there have been very few reports discussing the production increase and successful recruitment of wild S. schlegelii populations in sea cucumber farms. Fish production is defined as the net increase in biomass (Bohnsack and Sutherland, 1985), and recruitment is defined as the process of larval settlement and post-settlement survivorship to breeding age (Richards and Lindeman, 1987). In this study, we are the first to show that sea cucumber farms in the Bohai Sea are used as spawning grounds by economically important fishes such as S. schlegelii. Newborn larval S. schlegelii are reported to be 3.80-4.70 mm in length (Lin et al., 2014), and we obtained individuals 3.90-5.50 mm in the farm in early May 2020. The results provided an example of the successful coexistence of wildlife within sea cucumber farms.
Second, it was reported that communities on new artificial reefs might take 10-15 years to fully develop (Fager, 1971). However, Hueckele and Buckley (1987) found that new foraging species continued to appear even during the fourth and fifth years following reef construction. In this study, we obtained S. schlegelii larvae in the farm 3-5 years after its creation, but not on artificial reefs nearby created only 1-2 years previously. We argue that the presence of a wild S. schlegelii larvae indicates a healthy community on an artificial reef once the habitat changes from an unproductive barren substrate to a highly productive environment (Stone et al., 1979; Matthews, 1985). On this evidence, we speculate that a sea cucumber farm can reach community maturity within 3-5 years and that successful recruitment of S. schlegelii larvae can result from the creation of sea cucumber aquaculture farms in shallow waters.
Third, water temperature is one of the major factors influencing fish spawning (Wootton, 1990) such as S. schlegelii wild population, from the primordial germ-cell stage to the larval stages (Beldade et al., 2017). In the winter of 2020, the Bohai Sea suffered from more sea ice, over a longer period than usual (Xinhua News Agency, 2020). The average water temperature in the farm between December 2020 and March 2021 was 1.42-2.77°C (unpublished data), lower than during the same period from 2016 to 2017. Local fishermen reported very low production of S. schlegelii in the farm in 2021, and also we obtained very few number of larvae in this year.
Regarding the reason why that natural S. schlegelii population spawns and inhabits sea cucumber farm, aquaculture farms introduce a considerable number of hard physical structures (both at the sea bottom and in the water column), creating an environment which marine species such as Acetes chinensis find attractive. Pan et al. (2020) found that A. chinensis formed the major part of their diet in the study area. The A. chinensis population uses the shallow waters of Bohai Bay as a nursery ground in May, and remain there from May to October, after which the percentage of A. chinensis by weight in the diet of S. schlegelii decreases from 100.00% in June to 1.39% in November (Pan et al., 2020). Complex 3-D fixed structures of sea cucumber aquaculture farm may result in aggregation or high productivity of prey species (Coen et al., 2007; Mann and Powell, 2007; Powers et al., 2009) and therefore increase the food available for juvenile S. schlegelii. In addition, wild population in Haizhou Bay in the Yellow Sea preferred natural reefs, mixed sand/artificial reef bottoms, mixed bottoms of boulder, cobble, and gravel, and artificial reefs (Zhang et al., 2015). Thus, it is therefore reasonable that wild S. schlegelii choose sea cucumber aquaculture farms constructed using oyster reefs as spawning and nursery grounds.
Final, understanding food web structure is an important component of ecosystem-based aquaculture management. Oysters are major grazers of planktonic organisms and consumers of organic detritus suspended in the water. Bivalve species, including oysters, have the potential to affect the plankton community structure through their grazing activities (Loret et al., 2000). However, they can have a positive influence on phytoplankton population growth through their nutrient producing activities, releasing large amounts of ammonia and other inorganic compounds into estuarine ecosystems (Dame and Libes, 1993). Oysters may be important sources of nutrients for estuarine primary producers in the farm. The detritus consumer A. japonicus and bivalve predators such as R. venosa and C. japonica are economically important species and secondary consumers which support fishery production in our study. Asterias spp. in the farm can cause ecological catastrophes in coastal areas. For example, the excessive spread of Asterias amurensis caused massive death of cultured bivalves, including oysters and clams, in Jiaozhou Bay, the Bohai Sea in March 2021 (Zhao, 2021). A. amurensis ate 100,000 t of Ruditapes philippinarum in aquaculture farms in Qingdao in 2007 (Zhao, 2021). It is therefore important to understand the dynamic interactions of wild animals in aquacultural settings to promote the sustainable development of aquaculture farms. It is necessary for farm managers to weigh the close relationship between aquacultural practices and the benefits to the wild stocks through their provision of ecosystem structures. Aquacultural management decisions should not harm wild populations or the long-term sustainability of the ecosystem.
Conclusions
We summarize the main conclusions as follows.
1. Sea cucumber aquaculture farms in the Bohai Sea, China provided the spawning and first-year nursery grounds for populations of the economically important fish S. schlegelii.
2. Aquacultural infrastructures constructed by artificial reefs can be regarded as an effective fisheries management tool to enhance S. schlegelii populations.
In this study, we constructed an Ecopath model using high quality inputs to perform a mixed trophic impact analysis to understand the food web structure and energy flow distribution of a sea cucumber aquaculture farm constructed by oyster reefs. We argue that increasing the number of sea cucumber farms can increase the amount of spawning and nursery habitat for wild fishes. In future studies, we plan to compare the ecosystem attributes and quantitative indices of community maturity of such farms created in different years and estimate their environmental capacity for wild organisms. We will use stable isotope methods to verify the actual trophic levels of wild organisms in the farms to formulate medium- and long-term predictions of their ecosystem dynamics.
Data availability statement
The original contributions presented in the study are included in the article/Supplementary Material. Further inquiries can be directed to the corresponding author.
Author contributions
MX, Z-LQ, Z-LL, W-MQ, Y-LZ, QZ: contributed to the development, planning, data collection; Y-LZ, HL: contributed to data analysis and interpretation. All authors contributed to the writing of the manuscript.
Funding
The research was supported by the Key R&D Projects of Hebei Province (22373302D), National Natural Science Foundation of China (42030408), Tangshan Talent Project (A201905009), Tangshan Science and Technology Plan Project (19150239E), Hebei Province Modern Agricultural Industry Technical System “Special Seafood Innovation Team” (HBCT2018170204), the Key R&D Program of Zhejiang Province (2019C02056).
Acknowledgments
The authors wish to thank the members of Tangshan Sea Ranching Ltd. for their helps with field sampling. We thank reviewers for their insightful comments.
Conflict of interest
Authors QZ and Y-LZ are employed by Tangshan Marine Ranching Co. Ltd.
The remaining authors declare that the research was conducted in the absence of any commercial or financial relationships that could be construed as a potential conflict of interest.
Publisher’s note
All claims expressed in this article are solely those of the authors and do not necessarily represent those of their affiliated organizations, or those of the publisher, the editors and the reviewers. Any product that may be evaluated in this article, or claim that may be made by its manufacturer, is not guaranteed or endorsed by the publisher.
Supplementary Material
The Supplementary Material for this article can be found online at: https://www.frontiersin.org/articles/10.3389/fmars.2022.911399/full#supplementary-material
References
Arechavala-Lopez P., Izquierdo-Gomez D., Uglem I., Sanchez J. P. (2015). Aggregations of bluefish Pomatomus saltatrix (L.) at Mediterranean coastal fish farms: seasonal presence, daily patterns and influence of farming activity. Environ.Biol.Fish 98 (2), 499–510. doi: 10.1007/s10641-014-0280-5
Bacher K., Gordoa A., Sague O. (2015). Feeding activity strongly affects the variability of wild fish aggregations within fish farms: a sea bream farm as a case study. Aquac.Rec 46 (3), 552–564. doi: 10.1111/are.12199
Bayle-Sempere J. T., Arreguin-Sanchez F., Sanchez-Jerez P., Salcido-Guevara L. A., Fernandez-Jover D., Zetina-Rejon M. J. (2013). Trophic structure and energy fluxes around a mediterranean fish farm. Ecol.Model 248, 135–147. doi: 10.1016/j.ecolmodel.2012.08.028
Bearzi G., Fortuna C. M., Reeves R. R. (2009). Ecology and conservation of common bottlenose dolphins Tursiops truncatus in the Mediterranean Sea. Mammal.Rev 39 (2), 92–123. doi: 10.1111/j.1365-2907.2008.00133.x
Beldade R., Blandin A., O’Donnell R., Mills S. C. (2017). Cascading effects of thermally induced anemone bleaching on associated anemonefish hormonal stress response and reproduction. Nat.Commun 8 (1), 716–725. doi: 10.1038/s41467-017-00565-w
Bohnsack J. A., Sutherland D. L. (1985). Artificial reef research: a review with recommendations for future priorities. B.Mar.Sci 37 (1), 11–39. doi: 10.1038/s41467-017-00565-w
Bradley F. B., Richard A. D., Laura A. T. (2000). Lethal control of piscivorous birds at aquaculture facilities in the northeast united states: Effects on populations. N.Am.J.Aquacult 62 (4), 300–307. doi: 10.1577/1548-8454(2000)062<0300:LCOPBA>2.0.CO;2
Callier M. D., Byron C. J., Bengtson D. A., Cranford P. J., Cross S. F., Focken U., et al. (2017). Attraction and repulsion of mobile wild organisms to finfish and shellfish aquaculture: A review. Rev.Aquacult 10(4), 924–949 doi: 10.1111/raq.12208
China Fisheries Association (2021) The situation and future direction of the sea cucumber industry of 2020 in China. Available at: http://www.china-cfa.org/xwzx/xydt/2021/0105/524.html (Accessed 11 May 2022).
Christensen V., Pauly D. (1992). Ecopath-II–a software for balancing steady-state ecosystem models and calculating network characteristics. Ecol.Model 61 (3-4), 169–185. doi: 10.1016/0304-3800(92)90016-8
Christensen V., Walters C. J. (2004). Ecopath with ecosim: methods, capabilities, capabilities and limitations. Ecol.Model 172, 109–139. doi: 10.1016/j.ecolmodel.2003.09.003
Coen L. D., Brumbaugh R. D., Bushek D., Grizzle R., Luckenbach M., Posey M. H., et al. (2007). Ecosystem services related to oyster restoration. Mar.Ecol.-Prog.Ser 341, 303–307. doi: 10.3354/meps341303
Dame R., Libes S. (1993). Oyster reefs and nutrient retention in tidal creeks. J.Exp.Mar.Biol.Ecol 171 (2), 251–258. doi: 10.1016/0022-0981(93)90007-B
Dempster T., Sanchez-Jerez P., Uglem I., Bjørn P. A. (2010). Species specific patterns of aggregation of wild fish around fish farms. Estuar.Coast.Shelf.S 86 (2), 271–275. doi: 10.1016/j.ecss.2009.11.007
Díaz-López A. B., Bernal-Shirai J. A. (2007). Bottlenose dolphin (Tursiops truncatus) presence and incidental capture in a marine fish farm on the north-eastern coast of Sardinia (Italy). J.Mar.Biol.Assoc.UK 87, 113–117. doi: 10.1017/S0025315407054215
Drouin A., Archambault P., Clynick B. G., Richer K., McKindsey C. W. (2015). Influence of mussel aquaculture on the distribution of vagile benthic macrofauna in iles de la Madeleine, Eastern Canada. Aquacult.Env.Interac 6, 175–183. doi: 10.3354/aei00123
Fager E. W. (1971). Pattern in the development of a marine community. Limnol.Oceanogr 16, 241–253. doi: 10.4319/lo.1971.16.2.0241
Fernandez-Jover D., Sanchez-Jerez P., Bayle-Sempere J. T., Valle C., Dempster T. (2008). Seasonal patterns and diets of wild fish assemblages associated with Mediterranean coastal fish farms. ICES.J.Mar.Sci 65 (7), 1153–1160. doi: 10.1093/icesjms/fsn091
Fisheries and Fisheries administration, National Aquatic Product Technology Promotion General Station, Chinese society of aquaculture. (2021). China Fishery statistical yearbook 2021 (Beijing, China: China Agricultural Press).
Foster K. L., Steimle F. W., Muir W. C., Krapp R. K., Conlin B. E. (1994). Mitigation potential of habitat replacement: concrete artificial reef in Delaware bay-preliminary results. B.Mar.Sci 55 (2-31), 783–795.
Goodbrand L., Abrahams M. V., Rose G. A. (2013). Sea Cage aquaculture affects distribution of wild fish at large spatial scales. Can.J.Fish.Aquat.Sci 70, 1289–1295. doi: 10.1139/cjfas-2012-0317
Güçlüsoy H., Savas Y. (2003). Interaction between monk seals Monachus monachus (Herman) and marine fish farms in the Turkish Aegean and management of the problem. Aquac.Res 34 (9), 777–783. doi: 10.1046/j.1365-2109.2003.00884.x
Hueckele G. J., Buckley R. M. (1987). The influence of prey communities on fish species assemblages on artificial reefs in puget sound, Washington. Environ.Biol.Fish 19, 195–214. doi: 10.1007/BF00005349
Jenkins J. A., Bart H. L., Bowker J. D., Bowser P. R., MacMillan J. R., Nickum J. G., et al. (2014). Guidelines for use of fishes in research-revised and expanded. Fisheries 39 (9), 415–416. doi: 10.1080/03632415.2014.924408
Kluger L. C., Filgueira R., Wolff M. (2017). Integrating the concept of resilience into an ecosystem approach to bivalve aquaculture management. Ecosystems 20, 1364–1382. doi: 10.1007/s10021-017-0118-z
Lee S. M. (2002). Review of the lipid and essential fatty acid requirements of rockfish (Sebastes schlegeli). Aquac.Res 32 (s1), 8–17. doi: 10.1046/j.1355-557x.2001.00047.x
Lin Y. Z., Yu D. D., Wen H. S., Li J. F., Mu W. J., Liu Q., et al. (2014). Morphometrics development of ovoviparous Sebastes schlegeli hilgendorf larvae and juvenile. Trans. Oceanlog. Limnol. 2, 51–58. doi: 10.13984/j.cnki.cn37-1141.2014.02.009
Loret P., Pastoureaud A., Bacher C., Delesalle B. (2000). Phytoplankton composition and selective feeding of the pearl oyster Pinctada margaritifera in the takapoto lagoon (Tuamotu archipelago, French polynesia): in situ study using optical microscopy and HPLC pigment analysis. Mar.Ecol.-Prog.Ser 199, 55–67. doi: 10.3354/meps199055
Mann R., Powell E. N. (2007). Why oyster restoration goals in the Chesapeake bay are not and probably cannot be achieved. J.Shellfish.Res 26 (4), 905–917. doi: 10.2983/0730-8000(2007)26[905:WORGIT]2.0.CO;2
Matthews K. R. (1985). Species similarity and movement of fishes on natural and artificial reefs in Monterey bay, California. B.Mar.Sci 37 (1), 252–270. doi: 10.1515/botm.1985.28.11.501
McLeod I. M., Parsons D. M., Morrison M. A., Van Dijken S. G., Taylor R. B. (2013). Mussel reefs on soft sediments: a severely reduced but important habitat for macroinvertebrates and fishes in new Zealand. New.Zeal.J.Mar.Fresh 48, 48–59. doi: 10.1080/00288330.2013.834831
Nakagawa M. (2008). Studies on the stock enhancement technology of the black rockfish Sebastes schlegelii. Water Res. Center. Res. Bull. 25, 223–287.
Nash C. E., Iwamoto R. N., Mahnken C. V. W. (2000). Aquaculture risk management and marine mammal interactions in the pacific northwest. Aquaculture 183 (3-4), 307–323. doi: 10.1016/S0044-8486(99)00300-2
Olsen S. A., Ervik A., Grahl-Nielsen O. (2012). Tracing fish farm waste in the northern shrimp Pandalus borealis (Kroye) using lipid biomarkers. Aquacult.Env.Interac 2, 133–144. doi: 10.3354/aei00036
Pan X. W., Feng C., Yin Z. Q. (2020). Analysis of stomach contents of Sebastes schlegelii in tangshan marine ranch. Hebei. Fishe 3, 8–12.
Pattrick P., Strydom N. A. (2014). Larval fish variability in response to oceanographic features in a nearshore nursery area. J.Fish.Biol 85 (3), 857–881. doi: 10.1111/jfb.12477
Pauly D. (1980). On the interrelationships between natural mortality, growth parameters, and mean environmental temperature in 175 fish stocks. ICES.J.MAR.SCI 39 (2), 175–192. doi: 10.1093/icesjms/39.2.175
Powers S. P., Peterson C. H., Grabowski J. H., Lenihan H. S. (2009). Success of constructed oyster reefs in no-harvest sanctuaries: implications for restoration. Mar.Ecol.-Prog.Ser 389, 159–170. doi: 10.3354/meps08164
Richards W. J., Lindeman K. C. (1987). Recruitment dynamics of reef fishes planktonic processes settlement and demersal ecologies and fishes analysis. B.Mar.Sci 41 (2), 392–410.
Sasaki M., Nishiuchi S., Shiokawa F. (2002). Forecast on habitat and stomach contents of rockfish Sebastes schlegelii juveniles in central western Hokkaido. Cultivat. Giken. 30, 27–30.
Sepulveda M., Oliva D. (2005). Interactions between south American sea lions Otaria flavescens (Shaw) and salmon farms in southern Chile. Aquac.Res 36, 1062–1068. doi: 10.1111/j.1365-2109.2005.01320.x
Stone R. B., Pratt H. L., Parker R. O., Davis G. E. (1979). A comparison of fish populations on an artificial and natural reef in the Florida keys. Mar.Fish.Rev 41, 1–11.
Sudirman H. H., Jompa J., Zulfikar S. I., McKinnon A. D. (2009). Wild fish associated with tropical sea cage aquaculture in south sulawesi, Indonesia. Aquaculture 286 (3-4), 233–239. doi: 10.1016/j.aquaculture.2008.09.020
Takahashi K., Kumagai A., Asano K., Tomikawa N., Sato Y., Oikawa S., et al. (1994). Seedlings release of rockfish Sebastes schlegelii in shizugawa bay III. growth and distribution of fish caught in the bay. Miyaki. Brackish. Water Test. Rep. 9, 18–21.
Tallman J. C., Forrester G. E. (2007). Oyster grow-out cages function as artificial reefs for temperate fishes. T.Am.Fish.Soc 136, 790–799. doi: 10.1577/T06-119.1
Uglem I., Dempster T., Bjørn P. A., Sanchez-Jerez P., Økland F. (2009). High connectivity of salmon farms revealed by aggregation, residence and repeated movements of wild fish among farms. Mar.Ecol.-Prog.Ser 384, 251–260. doi: 10.3354/meps08001
Valle C., Bayle-Sempere J. T., Dempster T., Sanchez-Jerez P., Gimenez-Casalduero F. (2007). Temporal variability of wild fish assemblages associated with a sea-cage fish farm in the south-western Mediterranean Sea. Estuar.Coast.Shelf.S 72 (1-2), 299–307. doi: 10.1016/j.ecss.2006.10.019
Xinhua News Agency (2020) The sea ice condition of the yellow Sea and bohai Sea this winter is heavier than the previous two winters. Available at: https://baijiahao.baidu.com/s?id=1684145615532240461&wfr=spider&for=pc (Accessed 11 May 2022).
Xu M., Qi L., Zhang L. B., Zhang T., Yang H. S., Zhang Y. L. (2019). Ecosystem attributes of trophic models before and after construction of artificial oyster reefs using ecopath. Aquacult.Env.Interac 11, 111–127. doi: 10.3354/aei00284
Xu M., Yang X. Y., Song X. J., Xu K. D., Yang L. L. (2021). Seasonal analysis of artificial oyster reef ecosystems: implications for sustainable fisheries management. Aquacult.Int 29, 167–192. doi: 10.1007/s10499-020-00617-x
Yamada U., Tokimura M., Horikawa H., Nakabo T. (2007). Fishes and fisheries of the East China and yellow seas (Tokyo, Japan: Tokai University Press).
Yang H. S. (2016). Construction of marine ranching in China: reviews and prospects. Shuichan. Xuebao. 40, 1133–1140.
Zhang Y. Q., Xu Q., Josep A., Liu H., Xu Q. Z., Yang H. S. (2015). Short-term fidelity, habitat use and vertical movement behavior of the black rockfish Sebastes schlegelii as determined by acoustic telemetry. PloS One 10 (8), e0134381. doi: 10.1371/journal.pone.0134381
Keywords: Apostichopus japonicus, Sebastes schlegelii, aquaculture farm, ichthyoplankton, Ecopath modelling, spawn
Citation: Xu M, Qi Z-l, Liu Z-l, Quan W-m, Zhao Q, Zhang Y-l, Liu H and Yang L-l (2022) Coastal aquaculture farms for the sea cucumber Apostichopus japonicus provide spawning and first-year nursery grounds for wild black rockfish, Sebastes schlegelii: A case study from the Luanhe River estuary, Bohai bay, the Bohai Sea, China. Front. Mar. Sci. 9:911399. doi: 10.3389/fmars.2022.911399
Received: 02 April 2022; Accepted: 01 August 2022;
Published: 24 August 2022.
Edited by:
Fang Wang, Ocean University of China, ChinaReviewed by:
Haibo Yu, Northwest A&F University, ChinaJie Feng, Institute of Oceanology, Chinese Academy of Sciences (CAS), China
Copyright © 2022 Xu, Qi, Liu, Quan, Zhao, Zhang, Liu and Yang. This is an open-access article distributed under the terms of the Creative Commons Attribution License (CC BY). The use, distribution or reproduction in other forums is permitted, provided the original author(s) and the copyright owner(s) are credited and that the original publication in this journal is cited, in accordance with accepted academic practice. No use, distribution or reproduction is permitted which does not comply with these terms.
*Correspondence: Lin-lin Yang, c2Vhc3VuMTA3QDEyNi5jb20=
†These authors share first authorship