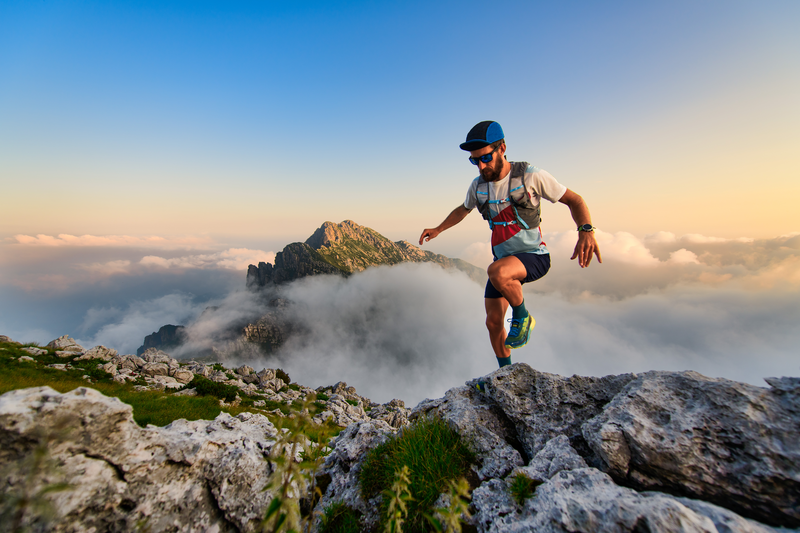
94% of researchers rate our articles as excellent or good
Learn more about the work of our research integrity team to safeguard the quality of each article we publish.
Find out more
ORIGINAL RESEARCH article
Front. Mar. Sci. , 07 July 2022
Sec. Physical Oceanography
Volume 9 - 2022 | https://doi.org/10.3389/fmars.2022.910941
Abyssal flow pathways in the Yap Trench and adjacent regions were investigated by computing geostrophic flows and analyzing water properties. Using boundary values of potential temperature, salinity, oxygen, and silicate, the upper and lower Circumpolar Deep Waters (UCPW, LCPW) were identified as the main abyssal water masses in the trench. At the western East Mariana Basin, some of the LCPW enters the southern Mariana Trench, and the remainder reaches the northern basin and then partly turns to the northern Mariana Trench, eventually joining the southern trench. At the Yap-Mariana Junction, some LCPW enters the West Mariana Basin, and the remainder passes the region east of the Yap Trench and then flows or subsides to the northern and southern trenches, respectively. In the northern trench, the LCPW flows north into the West Mariana Basin. The UCPW enters the northern Yap Trench via the East Mariana Basin and the Mariana Trench, reaches the sill of the Yap Trench via the East Caroline Basin and a narrow channel, and enters the southern trench via the East/West Caroline and Philippine Basins. The UCPW and LCPW circulate anticlockwise in the southern trench and in the northern region of it, respectively. The LCPW may be driven by the downwelling in the trench.
Global ocean circulation plays a crucial role in mediating climate change and in sustaining the marine environment by transporting water, heat, materials, and organisms. Clarifying the abyssal circulation in the Northwest Pacific is therefore an important task in oceanography. The abyss is the water zone exceeding 3000 m in depth (Macdonald et al., 2009; Hautala, 2018).
Johnson and Toole (1993) and Kawabe and Taira (1998) proposed that the abyssal water of the Northwest Pacific consists of Lower Circumpolar Deep Water (LCPW or LCDW) and North Pacific Deep Water (NPDW). The oxygen-rich LCPW is characterized by its relatively high salinity and low silicate content. The NPDW is characterized by high silicate concentration. The LCPW and NPDW correspond to a potential temperature θ <1.2°C at depths greater than ~3500 m and θ >1.2°C at depths less than ~3500 m, respectively (Kawabe and Fujio, 2010; Wang et al., 2020). After passing through the Samoan Passage, the LCPW enters the Central Pacific Basin and bifurcates into western and eastern branches (Kawabe and Fujio, 2010; Voet et al., 2015; Wang et al., 2020). The west branch passes through the Melanesian Basin and then the East Mariana Basin, ultimately flowing into the West Mariana Basin and the Philippine Basin (Kawabe and Fujio, 2010; Huang et al., 2018; Wang et al., 2020). The east branch enters the Northeast Pacific where it undergoes upwelling and is transformed into the NPDW (Kawabe and Fujio, 2010). The trench gyre may be generated by the upwelling/downwelling of the LCPW driven by the abyssal circulation and blockage by the ocean ridge (Kawabe and Fujio, 2010; De Lavergne et al., 2017; McDougall and Ferrari, 2017). In addition, the NPDW can be transported westward to the East Mariana Basin in a layer less than ~4000 m (Siedler et al., 2004; Kawabe and Fujio, 2010). After passing the eastern side of the Solomon Rise and flowing into the equatorial Pacific, an Upper Circumpolar Deep Water (UCPW) branch enters the Philippine Sea by way of the East Caroline Basins, the West Caroline Basins, and the East Mariana Basin (Kawabe et al., 2003; Kawabe et al., 2006; Kawabe and Fujio, 2010; Wang et al., 2020; Tian et al., 2021). These previous studies have provided a general picture of abyssal circulations of the Northwest Pacific.
The Yap Trench is an important segment of the arcuate trench system in the Northwest Pacific, linking adjacent basins and trenches (Figure 1). As mentioned above, previous studies have shown that abyssal water in the Mariana-Caroline regions (containing the Yap Trench) consists of LCPW, UCPW, and NPDW. Observations (e.g., Kawabe et al., 2003; Ma et al., 2019; Wang et al., 2020; Jiang et al., 2021) have shown that these waters flow into the West Mariana Basin through the Yap-Mariana Junction channel. Siedler et al. (2004) found that channels at the Yap-Mariana Junction and Caroline Ridge were essential for water transportation and that the water in the East Mariana Basin flows into the East Caroline Basin. Liu et al. (2018) suggested that abyssal water in the northern Yap Trench originates in the East Mariana Basin and East Caroline Basin. In the southern Yap Trench, observations (Liu et al., 2020) indicated that water flows southward in the western region of the trench and northward in the eastern region, and the overall transport is southward.
Figure 1 Bathymetry and sampling sections in the Yap Trench (YT) and adjacent regions. SYT, the southern YT; NYT, the northern YT; MT, Mariana Trench; YMJ, Yap-Mariana Junction; PT, Palau Trench; WCB, West Caroline Basin; ECB, East Caroline Basin; WMB, West Mariana Basin; EMB, East Mariana Basin; PB, Philippine Basin; SR, Solomon Rise. Nos. 37–38 cruise sections: NY, SY. WOD sections: zonal Y1 in SYT and WCB; zonal M1 (blue cross marks) and M2 (yellow dots) across the middle YT, the channel, and EMB; YM (black triangles), a zonal/meridional section across MT; YM1, at 140°E in MT and WMB; W1, at 134°E in PB; WM1 (black crosses), in WMB across SYT; WM2 (blue circles), at 137°E in WMB; WC1 (inverted black triangles) along 137°E across SYT and WCB; WC2 (red crosses), in WCB; WC3 (purple crosses) at 137°E in WCB; E1 (red crosses), in EMB and ECB; E2, at 144°E in ECB across MT; E3, in EMB across MT.
The complex bottom topography and immense size of the deep zones made it difficult to understand the abyssal circulation of the Yap Trench and its connection with adjacent basins and channels. However, over the last few decades, a number of investigations have been performed in this region, including several sections across the Yap Trench. Observations along the slopes of the northern and southern Yap Trench have been conducted using the submersible Jiaolong (Liu et al., 2018; Liu et al., 2020). This research vehicle can dive to more than 7000 m equipped with dozens of instruments including a conductivity-temperature-depth profiler (CTD) and sampling apparatus (Liu et al., 2010). The above observations along with historical observations provide the possibility to further clarify regional abyssal circulation and to construct an image of the pathways of abyssal water in the Yap Trench.
In the study presented here, we used available observational data to reveal the pathways of abyssal water in the Yap Trench and adjacent areas. The data and methods used in this study are firstly introduced. The section of results and analysis presents the computed abyssal geostrophic flows. And then, we discussed the water properties and flow paths in the Yap Trench and adjacent region. Finally, a brief summary is presented.
The study area includes the Yap Trench and adjacent trench and basins (Figure 1). The basins include the West Mariana Basin, the East Mariana Basin, the West Caroline Basin, and the East Caroline Basin. The adjacent trench is the Mariana Trench. The Yap Trench is ~700 km long with a maximum depth of ~8527 m (Fujioka et al., 2002), and separated into northern and southern parts by a sill. The northern part and the Mariana Trench are connected by the Yap-Mariana Junction, which is located at the south of the West Mariana Basin. The southern part is connected with the West Caroline Basin and forms an important segment of an arc trench system in the Western Pacific. At the sill, the Yap Trench is connected to the East Caroline Basin by a long channel between two ocean ridges.
The topography data we used in this study are from the General Bathymetric Chart of the Oceans (GEBCO_2014), which has a resolution of 30 arc-second and can be accessed over the internet (http://www.gebco.net/data_and_products/gridded_bathymetry_data/). In this paper, only abyssal water was studied.
We used observations collected during Chinese Ocean Cruises Nos. 37 and 38 led by China Ocean Mineral Resources R&D Association and supported by the National Key Basic Research Program of China. These applied oceanic scientific expeditions took place aboard the research vessel Xiangyanghong 9 with the deep-sea manned submersible Jiaolong. Cruise 37 was conducted during May 14–22, 2016 along the section across the northern Yap Trench at ~9.87°N (NY in Figures 1, 2D). Eight observation sites were selected around the trench slope. Three casts were conducted using ship-based CTD (SBE (Sea-Bird Equipment) 911 plus) and a dissolved oxygen sensor (JFE Alec Co. RINKO I). The submersible Jiaolong made five deep dives with a set of CTD (SBE 49 FastCAT) and a dissolved oxygen sensor (the RINKO I). The data were used to construct vertical profiles of temperature, salinity, and dissolved oxygen. The maximum depths were 3008–5917 m for the casts and 4950–6740 m for the dives. Cruise 38 took place during June 3–13, 2017, along a section that crossed the southern trench at 8.04°N (SY in Figures 1, 2D). Four casts and five dives were conducted using the same instruments as during Cruise 37. The maximum depth was 4487–5952 m for the casts and 4187–6685 m for the dives (more details can be referred in Liu et al., 2018, Liu et al., 2020). Before the cruises, the above instruments were calibrated at National Center of Ocean Standards and Metrology commissioned by the National Deep Sea Center. The raw data were processed using the standard procedures of SBE V7.2 software to obtain water temperature, salinity, density, and oxygen concentration in a 1-m interval grid. After that, the abnormal values were eliminated manually to ensure good quality of the data. The accuracy of SBE 49 FastCAT CTD is 0.002°C for temperature and 0.0003 S m-1 for conductivity. The accuracy of SBE 911 Plus CTD is 0.001°C for temperature and 0.0003 S m-1 for conductivity. The Thermodynamic Equation of Seawater 2010 (TEOS-10) was calculated using the Gibbs Sea Water (GSW) Oceanographic Toolbox (http://www.teos-10.org/). Concentrations of dissolved oxygen and silicate were manually analyzed on board by testing water samples according to the specifications for oceanographic survey (Standardization Administration of China, 2008). Dissolved oxygen was measured following the classic Winkler iodometric titration procedure: successively add 1-ml manganese chloride solution (2.4 mol l-1 MnCl2) and 1-ml alkaline potassium iodide (6.4 mol l-1 NaOH, 1.8 mol l-1 KI) into a 120-ml water sample, after dissolving solution by adding sulphuric acid, drop the required amount of standard sodium thiosulfate for titration of precipitated iodine, and finally obtain the concentration of dissolved oxygen by conversion. Silicate was determined by the silico-molybdenum spectrophotometry method using a continuous flow analyzer (SKALAR San++), after filtration of the 500-ml water sample through 0.45-μm cellulose acetate fiber filters, which were soaked in the 1% hydrochloric acid solution (HCl) for 12 hours and washed with distilled water to a neutral pH.
Figure 2 (A) Meridional geostrophic flows at section NY in northern Yap Trench. Positive and negative values correspond to northward and southward currents, respectively. Submersible Jiaolong dives at stations D109–D113 are denoted by dashed lines. (B) Potential density σ0 at NY. (C) Meridional geostrophic transport volume (from the reference level to a depth) at NY, the northward is positive. (D) Patterns of water transportation at sections NY, SY, M1, and M2 (black dots: Jiaolong diving; red dots: ship-CTD). AW, abyssal water; HW, hadal water. At NY, dashed arrow line: upper water flow; solid line: deeper water flow. The purple line is section A at the northern trench (Liu et al., 2018). M1 (black crosses) and M2 (purple circles) are two observation sections of WOD18. At the sill, the dashed arrow line denotes upper flow.
Historical CTD data were used for the region 133–150°E and 0–20°N in the west Pacific Ocean. The dataset was obtained from the World Ocean Database 2018 (WOD18) (https://www.nodc.noaa.gov/OC5/WOD/pr_wod.html). Fourteen cruise sections in the study region provided data on temperature, salinity, density, and oxygen. WOD data for silicate are described in the discussion section about water properties. The sites and dates of the investigations are shown in Figure 1 and Table 1.
Zonal or meridional geostrophic flows at observation sections were computed from observed CTD data using the thermal wind relation:
where (u, v) and (u0, v0) are geostrophic velocities at the depth z and at the reference level z0, respectively; g is the gravitational acceleration; f is the Coriolis parameter; ρ and ρ0 is the potential density and characteristic potential density, respectively. Because the near-bottom flow is usually faster than above for deep circulation (Wang et al., 2011), the level of no motion is chosen from a level which is well above seafloor with relatively weak pressure gradient (Siedler et al., 2004). Based on previous studies of the abyssal regions and adjacent trenches and basins (Johnson and Toole, 1993; Taira et al., 2005; Huang et al., 2018; Liu et al., 2018; Liu et al., 2020), the reference level for this study was chosen as 3000 m. At this weak circulation level, zonal and meridional velocities across the sections are set to be zero.
Geostrophic meridional flows along section NY in the northern Yap Trench were determined based on CTD data (Figure 2A). In the upper abyssal layer at depths less than ~3800 m, water flows southward with except for the region between D112 and D111, where potential density at D111 is higher than that at D112 at a same depth (Figure 2B). Below that layer, the flows travel northward with velocities at the west flank of the trench notably faster than at the east. Below 5000 m, the largest value is ~0.15 m s-1, where the large horizontal gradient of potential densities exists (Figure 2B). Nevertheless, between D111 and D112, the water at the depths of ~4000–5000 m flows southward, because the isopycnal of potential density is lower at D111. These flows are illustrated in Figure 2D. The net transport of abyssal water in the northern trench is northward (Figure 2C), mainly along the west slope of the trench. The total net transport across the section is estimated as ~4.5 Sv (1 Sv =106 m3 s-1).
Figures 3A, B show the geostrophic meridional flows relative to the reference level along sections M1 and M2 at the trench sill, respectively, which cross the sill between the northern and the southern trench (Figures 1, 2D). Water flows northward in the trench and southward at both flanks of the trench (Figure 2D). Two investigations in different seasons (February/winter and August/summer) basically show consistent patterns of water transport in the meridional direction.
Figure 3 Meridional geostrophic flows at sections M1 (A) and M2 (B) at the sill and the middle of Yap Trench. Salinity and potential density σ0 are presented.
Figure 4A illustrates the meridional flows at section Y1 across the southern trench. Generally, at the isobar levels the potential densities in the trench are lower than those in the east region, and thus the flows are northward in the trench and the adjacent region in the West Caroline Basin. In the abyssal layer above ~3800 m at the western region of the trench, the flow direction is southward (illustrated in Figure 4D). Figures 4B, C show the zonal flows at sections WM1 and WC1 across the southernmost region of the Yap Trench, respectively. Both sections indicate eastward flows in the abyssal layer above 4500 m where the isopycnal of potential density is lower. In the deeper zone, only one station was observed at each section, hence, the data is not enough to construct deeper flows in the southwest of the trench.
Figure 4 Meridional flows at section Y1 (A), zonal flows at sections WM1 (B) and WC1 (C) across the southern Yap Trench, and a schematic of water transportation at these sections (D). Potential density σ0 are presented. For zonal flows, a positive value represents the eastward direction. A brown arrow line denotes lower flows shown in (A, B), and a blue line denotes upper flows shown in (A). Symbols (black +) are observation sites of section WM1 across the trench.
Meridional and zonal flows at section YM along the southwestern Mariana Trench are shown in Figures 5A, B. In the region from ~138.7°E (the western side of the junction) to ~142.5°E, water generally flows southward, except for the upper layer (above ~3400 m) east of ~140.5°E. The flows are northward along the rest of the section, where the isolines of potential density are tilting and lift from west to east (Figure 5A). Along the trench section at ~145°E, water flows westward, except for the upper layer in the northern area. These results describe the water transportation pattern at the junction and trench, as shown in Figure 5D. The water in the Mariana Trench flows to the region east of the Yap Trench. In the region east of the junction, upper abyssal water moves to the West Mariana Basin. On the west flank of the junction connected to the basin, upper abyssal water flows southward to the Yap Trench.
Figure 5 Meridional flows and potential density σ0 at section YM along Yap-Mariana Junction channels (A), zonal flows across Mariana Trench (B), zonal flows at section YM1 along 140°E in a part of the Mariana Trench and the West Mariana Basin (C), and the schematic of flows in the junction region (D). Dashed arrow lines denote upper abyssal flows.
Zonal flows crossing section YM1 are shown in Figure 5C. Deeper abyssal water below ~3500 m travels westward in the northern part of the Mariana Trench channel and the region north of the channel (11–12°N) at ~140°E, while the water above 3500 m in this area flows eastward (illustrated in Figure 5D). This water may consist of returned water from the West Mariana Basin.
Figure 6A illustrates the zonal flows at section E1 in the East Caroline Basin. Spanning from 3.5°N to 6.5°N in the basin, the section is normal to the channel connecting the basin and the Yap Trench. It can be seen that water mainly flows westward across the section, especially in the zones of 4.5–5.5°N and 6–6.5°N, which are directly connected to the channel (Figure 6C). In the zones, the potential density at isobar levels is lower, corresponding to the fresh water (less saline) transported from the East Mariana Basin. Zonal flows along section E2 are illustrated in Figures 6B, C. Flows are westward at 6–8°N crossing the channel. Results at these two sections indicate that abyssal water moves to the East Caroline Basin, passing by the east slope of the Solomon Rise, and then partly flows to the channel (Figure 6C).
Figure 6 Zonal geostrophic flows at sections E1 (A) and E2 (B) in the East Caroline Basin, and flow pattern along the sections (C). Potential density σ0 are also presented.
Our results are supported by previous research about flow pathways of the UCPW in this region (Kawabe et al., 2003; Kawabe et al., 2006; Kawabe and Fujio, 2010; Liu et al., 2018; Wang et al., 2020). According to Kawabe et al. (2003) and Wang et al. (2020), some of UCPW flows westward to the West Caroline Basin and finally enters the Philippine Basin by way of equatorial regions. Figure 6C shows the western propagation from the East Caroline Basin to the West Caroline Basin.
Figures 7A–C show the zonal and meridional flows along section WC2 and zonal flows along WC3. At WC3, flows are mainly westward between ~3.0°N and ~5.0°N and eastward in the north region from ~5.0°N to the trench. This pattern agrees with the abyssal transport at section WC1 shown in Figure 4C. These two sections were observed during the summer of two different years. At WC2, zonal flows are generally westward between ~3.1°N and ~5.2°N but eastward between ~5.2°N and ~7°N, showing a similar pattern to sections WC3 and WC1. The direction of meridional flows along WC2 corresponds to the zonal flows at the same locations. In other words, the northward direction corresponds to the eastward flow in zonal direction and the southward direction corresponds to a westward flow. Thus, between ~137°E and ~138.8°E, the flows are mainly northeastward, and the water between ~138.8°N and ~141°E flows mainly in the southeastern direction. The abyssal water pathway in this region is summarized in Figure 7E.
Figure 7 Zonal (A) and meridional (B) flows at section WC2 in the West Caroline Basin, zonal flows at section WC3 (C) in the West Caroline Basin, zonal flows at section W1 (D) that crosses the Palau Trench in the West Caroline Basin and extends to the Philippine Basin, and flow pattern along observation sections in the region (E).
Figure 7D shows the zonal flows at section W1, which crosses the Palau Trench in the West Caroline Basin and extends to the Philippine Basin. In the channel south of the Palau Islands, zonal flows are eastward. Water enters the Palau Trench and the West Caroline Basin from the Philippine Basin (Figure 7E). Our results are supported by the previous studies that in the southern Philippine Basin, the main body of water circulates in the basin because of blockage of the Yap Ridge, while a small part of water enters the Western Caroline Basin (Zhai and Gu, 2020).
In summary, geostrophic flows at these sections indicate a general pattern of anticyclonic circulation in the West Caroline
Basin. Water flows westward in the southern region and eastward in the northern area. Abyssal water flows eastward to the West Caroline Basin and the Palau Trench and then partly enters the southernmost segment of the Yap Trench at the north of the West Caroline Basin.
Figure 8A shows the zonal flows across section E1 (around 149.5°E) in the East Mariana Basin. Between ~8.5°N and ~11.8°N, flows are mainly eastward, and between ~11.8°N and ~17°N, water generally moves to the west. Between ~17°N and ~18.5°N, flows are mainly eastward, and north of ~18.5°N, water generally flows westward. Therefore, abyssal water in the East Mariana Basin flows toward the Mariana Trench from the region of ~11.8–17°N and the region north of ~18.5°N along ~149.5°E at the basin (Figure 8E). In the southern area of the basin near the ridge, abyssal water flows away from the trench.
Figure 8 Zonal flows at sections E1 (A) and E3 (B) in the East Mariana Basin, meridional flows at sections M1 (C) and M2 (D) in the East Mariana Basin, and flow patterns (E).
Figure 8B show the zonal flows crossing section E3 at ~147°E (illustrated in Figure 8E). We can see that the abyssal water flows westward to the Mariana Trench between 11°N and 12°N. At 10–11°N and 12–14°N, the water flows eastward away from the trench. At 14–18°N, water in the area west of the trench flows westward. This pattern agrees with that suggested by Siedler et al. (2004) and Huang et al. (2018). With the obstruction of the ridge, this water flowing across the trench may turn southward and flow back to the trench, while the rest of the water will continue to flow eastward back to the basin.
Zonal flows across section E2 at ~144°E show that water is transported westward along the trench (Figure 6B, illustrated in Figures 6C, 8E). Between 9°N and 10°N and in the region north of the trench, flows are mainly eastward. Although the deeper flows in the trench are not constructed along E2 for only one observation station there, such flows are indicated to be westward at an adjacent section YM at ~145°E. As seen in Figure 5B, the water from the East Mariana Basin moves westward in the trench, except for the layer above ~4000 m in the northern region. In addition, at the area north of the trench, the upper water flows westward and the lower water flows eastward (illustrated in Figures 6C, 8E). The westward flows along section YM occur in the same meridional zone as that along sections E2 and E3. Huang et al. (2018) reported a similar pattern, that is, along a section at ~142°E, the abyssal water travels westward along the trench, but there are eastward flows on both sides of the trench channel. According to the study of Huang et al. (2018), the westward flow with a transport of 1.29 Sv dominates the deep layer of the Challenger Deep in the trench.
Figures 8C, D show the meridional flows across sections M1 and M2. At M1, which was observed in February of 1989, the general direction of abyssal flows was from north to south in areas between ~143.7°E and ~147°E, as well as in the deeper region east of ~149.2°E. At M2, which was observed in August of 2015, water was transported southward to the East Caroline Basin at ~142.3–145°E. The difference in meridional flows between the two observations reflects seasonal variations. However, both observations reveal the fact that there is flow entering the East Caroline Basin from the adjacent region of the Mariana Trench in the East Mariana Basin (Figure 8E). Furthermore, part of the water may flow to the Yap Trench via the channel north of the East and West Caroline Basins. This pathway for inflowing UCPW into the trench is supported by the results shown in former sections for the Yap Trench and East Caroline Basin and the study of Liu et al. (2018).
Along section YM1 at ~140°E, the water below 3,500 m flows westward in the region (~11–12°N) north of the Mariana Trench, while the upper water flows to the east (Figure 5C). Between 12°N and 14°N, abyssal water flows eastward from the west of the West Mariana Basin. Between 14°N and 17°N, the direction of zonal flows is westward. The general flows in the regions of 17–18°N and 18–19°N are eastward and westward, respectively. These flows are illustrated in Figure 5D.
Figures 9A, B show the zonal flows along two sections at ~137°E in the West Mariana Basin. Along WM1, water generally flows eastward between 9°N and 10°N and westward between 10°N and ~12.5°N and in the upper layer between 12.5°N and ~13.5°N. In areas north of ~18.5°N, the direction of zonal flows is generally eastward. Along section WM2, eastward flows appear in the southernmost region (~9–11°N, west of the Yap Islands) of the basin, and water flows to the west at 11–12°N. In the region from 12°N to 15°N, the direction of zonal flows is eastward. Although there are differences between two sections observed at different times, a common pattern (illustrated in Figure 9C) exists in the region near the Yap Trench. In the southernmost part of the West Mariana Basin, water flows eastward, with some water possibly turning north with the obstruction of the ridge west of the southern Yap Trench. In the region west of the northern Yap Trench, water flows from east to west, coming partly from the Mariana Trench and the Yap Trench via their junction. In the area of ~12.5–15°N, water moves from west to east. This water may flow from the circulated water in the West Mariana Basin.
Figure 9 Zonal flows at sections WM1 (A) and WM2 (B) in the West Mariana Basin, and their general pattern (C).
In a previous study (Liu et al., 2018), after reliability analysis of data, we used the outputs of an eddy-resolving OGCM for the Earth Simulator (OFES; Sasaki et al., 2008) to calculate geostrophic velocities at a wider section (Figure 2D, section A) and obtained a northward net abyssal transport (Figure 2D) volume of 0.42 Sv. This transport volume is much less than the westward transport at the Yap-Mariana Junction (5.80 Sv) or in the channel connecting the East Caroline Basin to the Yap Trench (4.55 Sv) calculated from OFES in the study. This suggests that there is a southward transport in the area east of the northern Yap Trench, which flows from the junction and supplies abyssal water for the trench. To some extent, the southward inflow (at the east part of section A) balances the northward transport (4.5 Sv) in the northern trench (the west part of section A).
According to Liu et al. (2018), the water flows to the Yap Trench from the East Caroline Basin via the channel between two ocean ridges and subsides along the east slope. As shown in Figures 3A, B, the salinity structure at the sill shows that downwelling fresh water reaches the east slope at the depth of ~3700 m, where the resulting barocline (Figure 3B) forces southward flows of abyssal water. As analyzed above and from the results at sections NY and SY (Figure 2D), we inferred that inflowing water from the East Caroline Basin bifurcates, with some water moving to the southern trench and the remainder moving westward to the northern trench.
Using ship-based CTD and Jiaolong CTD data collected during Cruise 38, we computed geostrophic flows along section SY in the southern trench (Liu et al., 2020). The results show that flows are southward in the western part and northward in the eastern slope (Figure 2D), and strong flows occur on the west flank. The turbulent mixing is enhanced in the slope and near-bottom zones, similar to the observed at the Mariana Trench (van Haren et al., 2017; Zhao et al., 2021). In the main region of the trench, the net transport of abyssal water is southward at ~1.74 Sv. In the hadal zone (below 6000 m), the southward transport in the western regions of the trench is balanced by the northward transport in the eastern region. The temperature, salinity, and dissolved oxygen data indicate that the deeper abyssal water in the southern trench comes from the LCPW and UCPW, but the hadal water seems to be isolated local water (Liu et al., 2020).
At the southernmost arc region of the trench, water flows eastward into the region of the southern Yap Trench from the adjacent basin or trench and then flows northward along the east slope of the trench. In the west region, water flows southward. Hence, a cyclonic circulation exists in the upper abyssal layer, similar to the pattern at section SY shown in Figure 2B (Liu et al., 2020). In the deeper layer, there are northward flows in the west region of the trench, whereas the direction of lower flows is not known. Thus, whether there exists a clockwise circulation for lower flows in this arc region is to be revealed.
At the west flank of the junction, our results indicate that upper abyssal water flows southward to the Yap Trench. This pattern is supported by Wang et al. (2020), who suggested that the return flow in the meridional direction above ~4200 m. Flow in the deeper zone of the junction channel is not presented due to the lack of data in our study. According to studies by Wang et al. (2020), the lower water (below ~4200 m) flows to the junction via the Mariana Trench from the East Mariana Basin and then flows northward into the West Mariana Basin (the lower northward flow is illustrated in Figure 5D).
Previous studies provide additional information for a comprehensive analysis of abyssal water transportation. Based on mooring data, Siedler et al. (2004) suggested that 0.36 Sv LCPW flows into the West Mariana Basin via the junction. Tian et al. (2021) proposed that since the junction is the only channel deeper than ~4000 m connecting to the Philippine Sea, the LCPW enters the sea through the junction area. Zhai and Gu (2020) suggested that because of the blockage of the Yap Ridge, the main body of the LCPW flowing from the East Mariana Basin enters the Philippine Sea via the Mariana Trench and the junction. In studies by Zhai and Gu (2020) and Tian et al. (2021), the LCPW incoming area of the Philippine Sea corresponds to the West Mariana Basin.
Based on previous studies and our results (Figure 5), we concluded that the LCPW moving through the Mariana Trench and Yap Trench is transported northward to the West Mariana Basin at the western channel of the junction. At the eastern part, the LCPW intrudes into the region east of the Yap Trench. In the upper layer, abyssal water flows southward to the trench at the junction.
Watermass properties were analyzed to further elucidate flow pathways and connections among abyssal water in the Yap Trench and adjacent basins and trench channels. Figure 10 shows the vertical profiles of potential temperature, salinity, and dissolved oxygen. Figure 11 shows the silicate concentrations in the regions using WOD designations for Ocean Station Data, which refer to measurements made from a stationary research ship measuring nutrients in seawater samples gathered using special bottles (Garcia et al., 2019).
Figure 10 Water temperature (A), salinity (B), and oxygen (C) properties in the Yap Trench (YT) and adjacent regions. WMB, West Mariana Basin; EMB, East Mariana Basin; WCB, West Caroline Basin; ECB, East Caroline Basin; MT, Mariana Trench; NYT, the northern YT; SYT, the southern YT; MYT, the middle sill of YT.
Figure 11 Silicate distributions in the Yap Trench and adjacent regions. Observation stations (A); silicate concentrations (B). PB, Philippine Basin.
Potential temperature θ =1.2°C is usually used as the boundary to separate the LCPW and UCPW, which corresponds to a depth of ~3500 m (e.g., Johnson and Toole, 1993; Johnson et al., 1994; Kawabe and Fujio, 2010; Wang et al., 2020) north of the mid-latitude in the south Pacific (Kawabe et al., 2003; Kawabe et al., 2006; Kawabe et al., 2009). Several studies have used other values such as 1.07°C (Wijffels et al., 1998) along 149°E as well as 1.1°C (Klatt and Holfort, 2000) and 1.18°C (Siedler et al., 2004) in the East Mariana Basin. We chose 1.2°C as the boundary value. The boundary between the two layers becomes deeper after the water reaches the northwestern Pacific. In our study region, the depth ranges between ~3650 and 4150 m (Figure 10A). The minimum depth occurs in the East Mariana Basin because the LCPW and UCPW from the south Pacific enter this basin after the LCPW passes the Melanesian Basin (Siedler et al., 2004; Kawabe and Fujio, 2010) and the UCPW passes east of the Solomon Rise (Kawabe et al., 2006; Kawabe and Fujio, 2010). In the East Mariana Basin, the maximum depth for the boundary (~4000 m) occurs in the southwestern-most part of the basin and in the Challenger Deep of the Mariana Trench. Studies by Siedler et al. (2004) reported that in the channel of the Yap-Mariana Junction, the LCPW with θ < 1.2°C is below ~4000 m. This fact leads to the conclusion that the LCPW and UCPW pass the East Mariana Basin, partly enter the Mariana Trench, and then continue to flow westward to the junction. As shown in Figures 2A, D, in the northern Yap Trench, meridional flows in the upper abyssal layer are mainly southward, while deeper water travels northward. Our results (Figures 5A, D) and observations by Wang et al. (2020) indicate that at the junction, the LCPW flows northward to the West Mariana Basin by the west flank of the junction channel but flows southward to the area of the Yap Trench, and the UCPW flows southward at the junction. As shown in Figure 10A, the properties of potential temperature in the northern Yap Trench and the Mariana Trench are highly consistent at the same depth level. In the lower abyssal zone, the Mariana Trench shows an almost similar vertical pattern of temperature as the East Mariana Basin. These results indicate that deeper abyssal water in the northern Yap Trench comes from the LCPW, and most of the upper abyssal water comes from the UCPW via the junction. In the southern Yap Trench, the level of 1.2°C is at ~3700–3950 m, almost the same as the level range in the northern Yap Trench and approximately overlapping the range in the West Caroline Basin. With respect to the UCPW, although there are no obvious differences in the vertical distribution of temperature within the study region, the vertical pattern in the southern Yap Trench is closest to that of the northern trench and the West Caroline Basin. However, the LCPW has a pattern similar to that found in the northern Yap Trench and the Mariana Trench. This indicates that deeper abyssal water in the southern Yap Trench is mainly LCPW. Approaching the hadal zone, temperatures in the southern Yap Trench are slightly higher than those in the northern trench at the same depths. Although the upper abyssal water has a similar temperature pattern throughout the study region, the lower zone shows similar patterns only in the Yap and Mariana Trenches and the East Mariana Basin, where the watermass is significantly colder than in the West and East Caroline Basins and the West Mariana Basin. This indicates that it is mainly the UCPW rather than the LCPW that enters the West and East Caroline Basins. This conclusion is supported by previous studies (Siedler et al., 2004; Kawabe and Fujio, 2010). After entering the West Mariana Basin, the LCPW gradually subsides in this less saline basin.
Previous studies (e.g., Siedler et al., 2004; Kawabe and Fujio, 2010) have shown that the LCPW is more saline and oxygen rich than the UCPW and NPDW (Figures 10B, C). The boundary values of salinity and oxygen for identifying the UCPW and LCPW are 34.683 psu and 153 μmol l-1, respectively. These values are very close to the values (34.685 psu and 155 μmol kg-1) used by Siedler et al. (2004); Talley (2007), and Kawabe and Fujio (2010). The salinity and dissolved oxygen in our study region have vertical distributions consistent with the potential temperature, the only difference being a lower temperature corresponding to a higher salinity and higher oxygen. In the upper layer above ~4000 m (generally UCPW or NPDW), differences in salinities are not obvious, but in the deeper zone, water masses are more saline in the Yap and Mariana Trenches and the East Mariana Basin than in the other basins. This further indicates that the deeper abyssal water in the Yap and Mariana Trenches and the East Mariana Basin is mainly of the LCPW. Although some LCPW enters the West Mariana Basin via the Yap-Mariana Junction, the water in the basin is strongly affected by water exchange with the Philippine Sea. In addition, after entering the basin, the LCPW gradually subsides because of baroclinicity. Therefore, the water is less saline in the deeper zone of the basin than at the same depths in other regions through which the LCPW passes. Dissolved oxygen of the deeper water is also relatively low in this basin. Since UCPW and NPDW occupy almost the same depth range, their boundary is difficult to identify locally (Kawabe and Fujio, 2010). Nevertheless, the NPDW is characterized by lower oxygen concentrations than UCPW (Wang et al., 2020). Figure 10C shows that in the upper abyssal water above ~3700 m, oxygen concentrations at some stations in the East Mariana Basin are lower than those in the other regions, indicating that the watermass at the stations is the NPDW. As shown in Figures 8A, B, westward flows occur at the northern regions of sections E1 and E3, indicating that the NPDW from the eastern part of the Pacific flows westward to the northern Mariana Trench through the northern part of the East Mariana Basin. With the topographical obstruction, the water turns partly southward and meets with the UPCW. Thus, some upper abyssal water over the Mariana Trench is mixed water of the UCPW and NPDW. The upper abyssal water in the Yap Trench is mostly of the UCPW, which communicates with adjacent basins and channels. At the sill of the trench (labeled MYT in Figure 10C), the oxygen concentrations of abyssal water above ~3700 m are essentially equivalent to those in the East Caroline Basin, indicating that some UCPW in this basin enters the Yap Trench through the channel.
The deeper water is relatively silica-poor in comparison with upper abyssal water, especially in the East Mariana Basin where the silicate of the LCPW is significantly lower than that of the UCPW or NPDW (Figure 11B). This is supported by previous observations (e.g., Siedler et al., 2004; Kawabe and Fujio, 2010). Klatt and Holfort (2000) and Siedler et al. (2004) selected silicate concentrations of 136 μmol kg-1 and 142 μmol kg-1, respectively, to distinguish between the LCPW and upper water in the East Mariana Basin. The observational results (Figure 11B) support 142 μmol kg-1 as the boundary values. The silicate of the LCPW in the northern Yap Trench is generally close to that in the basin. However, in the southern trench, the silicate concentration is higher than that in the basin, possibly due to enhanced turbulent mixing on the trench slope (Zhao et al., 2021; Liu et al., 2020). With respect to upper abyssal water, previous studies (Siedler et al., 2004; Kawabe and Fujio, 2010) proved that the NPDW is characterized by high silicate. Talley (2007) suggested that the silica concentration of the UCPW is ~140 μmol kg-1 in the Philippine Basin, and the NPDW is ~155 μmol kg-1 in the eastern Pacific. As the NPDW proceeds westward, its concentration is continually modified (Kawabe and Fujio, 2010). Siedler et al. (2004) reported that a high-silicate tongue with a maximum >144 μmol kg-1 marks the core of the NPDW (above ~3500 m), and the lower boundary of the tongue is ~4000 m, corresponding to an isoline of 142 μmol kg-1 in the East Mariana Basin. These data are consistent with the results shown in Figure 11B. This further indicates NPDW intruding into the basin.
By summarizing the analysis of geostrophic flows and watermass characteristics, we were able to determine the flow pathways of abyssal water in the Yap Trench and adjacent regions (Figures 12A, B).
Figure 12 Schematic pathways of abyssal flows in the Yap Trench and adjacent regions: LCPW (A), and UCPW and NPDW (B).
The western branches of the LCPW and UCPW, coming from the south Pacific (Roemmich et al., 1996) via the Melanesian Basin, enter the region of the East Mariana Basin (Siedler et al., 2004; Kawabe and Fujio, 2010). The LCPW proceeds to the west of the basin, some water flows directly toward the southern Mariana Trench, and the remainder travels in the northern direction. With the topography blockage in the northern area of the basin, the northward LCPW bifurcates into two branches. One branch flows out of the basin, and the other turns west to the northern Mariana Trench and then partly flows to the southern trench along the channel. Except for a part of the flow that returns back to the basin, the LCPW transported to the southern trench proceeds westward and arrives at the Yap-Mariana Junction after passing the Challenger Deep. At the junction, the LCPW flows partly southward into the region east of the Yap Trench. Along the east slope of the northern Yap Trench, the LCPW subsides into the trench and pushes the deep water in the trench northward to the west region of the junction channel connected with the West Mariana Basin. The westward LCPW in the Mariana Trench arrives at the western region of the junction, and this water, together with the northward water in the northern Yap Trench, enters the West Mariana Basin along the west part of the junction channel. These LCPW currents circulate in the West Mariana Basin, and some of them ultimately flow into the Philippine Basin. The downwelling LCPW (Liu et al., 2018) facilitates a cyclonic circulation in the northern area of the southern Yap Trench (Liu et al., 2020). In the southernmost of the trench, whether the LCPW circulates clockwise or flows into the West Caroline Basin in a small part is to be known. Based on the analysis of watermass properties and geostrophic flows (Liu et al., 2020), the hadal water in this southern trench seems to be isolated local water rather than the LCPW. Although the exchange of deeper water between the southern and northern trenches is obstructed by a sill with a depth of ~5000 m (Liu et al., 2020), the northward transport exists over the sill.
At the region north of the Solomon Rise, the UCPW bifurcates, with some water entering the East Caroline Basin and some proceeding westward to the West Caroline Basin where it finally enters the Philippine Basin. In the region south of the Palau Islands, a part of the UCPW in the Philippine Basin flows eastward to the West Caroline Basin and the Palau Trench. Most of the intruded water circulates in this basin, but some flows to the southern Yap Trench. The downwelling UCPW is a factor forcing water circulation in this arc trench, where the water circulates in a cyclonic direction. In the middle of the Yap Trench, the UCPW from the East Caroline Basin flows to the sill through the channel between the ocean ridges. With the isolation of the sill and the obstruction of the trench slope, the UCPW bifurcates, flowing mostly to the southern trench and partly into the northern trench. The downwelling UCPW at the sill (Liu et al., 2018) contributes to the northward propagation of the LCPW in the northern Yap Trench and the circulation in the southern trench. In the northern Yap Trench, downwelling water at the sill and downwelling LCPW at the east slope from the junction force the LCPW northward. Another arm of the UCPW that is bifurcated by the Solomon Rise flows northwestward in the East Mariana Basin, with some flowing westward to the southern Mariana Trench and the rest traveling northward. These pathways are both basically the same as the LCPW pathways. With the topography obstruction in the northern area of the East Mariana Basin, some of the UCPW flows northeastward and leaves the basin. The remainder flows westward to the northern Mariana Trench and then partly turns southwestward along the trench by the Mariana Islands. As the UCPW moves westward in the Mariana Trench, some water turns back to the East Mariana Basin, and some flows northward to the region west to the Mariana Islands. After arriving at the west end of the trench, one part of the UCPW leaves the trench area along the ridge slope south of the trench and partly enters the East Caroline Basin. Another part flows to the Yap-Mariana Junction and the Yap Trench area, and a third portion flows to the West Mariana Basin, with some turning southward to the junction and the trench. In general, the UCPW in the northern Yap Trench flows southward. Some of it flows to the western region of the southern Yap Trench and facilitates the local circulation.
The NPDW from the eastern part of the Pacific (Kawabe and Fujio, 2010) arrives at the northern East Mariana Basin after traveling a long distance. With the obstruction of the ocean ridge west of the Mariana Yap, some NPDW turns southward and is modified by mixing with the UCPW. The modified water proceeds to the southern Mariana Trench.
Moreover, cruises at NY and SY were in May and June, and seasonal variability of abyssal flows in the Yap Trench is not known. As analyzed above, previous observations in different times proved that abyssal water arrives at the Yap-Mariana Junction via the similar paths along the Mariana Trench, even though watermass properties vary seasonally (Huang et al., 2018). At the junction, although seasonal intrusions of the LCPW and UCPW were discovered (Wang et al., 2020), the general pattern of flow paths is annually common, as illustrated in Figure 12. At sections M1 (observed in February) and M2 (observed in August), the results show the consistent patterns of water transport at the middle Yap Trench. At the sections investigated in different periods in the West Caroline Basin and across the trench, the results also show the consistent feature of the paths. As analyzed above from adjacent regions, the transport paths of abyssal water may be the same annually in essentials, especially for the LCPW. In the future, temporal variations of abyssal flows and impacts on their paths need to be studied in the trench.
Using observations in the Yap Trench by the submersible Jiaolong system and WOD historical observations in adjacent basins and trenches, this study calculated geostrophic flows along specific sections and analyzed watermass properties to construct detailed pathways of abyssal water in the Yap Trench and its connections with adjacent regions.
By comparing vertical distributions of temperature, salinity, oxygen, and silicate and referring to previous results (Liu et al., 2018, Liu et al., 2020), we concluded that upper and deeper abyssal water in the trench mainly consists of the UCPW and LCPW, respectively. The NPDW is characterized by relatively high silicate and low oxygen. Originating with the upwelling LCPW in the eastern Pacific (Kawabe and Fujio, 2010), the NPDW arrives at the East Mariana Basin after traveling a long distance. It partly enters the region of the Mariana Trench. The NPDW is mixed with the UCPW, the dominant water in this region. Thus, the main upper abyssal water in the Yap Trench is the UCPW rather than NPDW. For identifying the UCPW and LCPW in the trench, we chose the boundary values of θ = 1.2°C for potential temperature, 34.683 psu for salinity, 153 μmol l-1 for oxygen, and 142 μmol kg-1 for silicate, which correspond to a maximum depth of ~3950 m for the UCPW. Liu et al. (2020) suggested that the hadal water in the southern Yap Trench is isolated, but cyclonic circulation exists in the north region. Hadal flows need further investigation with more observations.
After arriving at the East Mariana Basin from the south Pacific, some of the northwestward LCPW flows westward to the southern Mariana Trench. The remainder proceeds to the northern East Mariana Basin and then partly turns west to the northern Mariana Trench, eventually arriving at the southern trench. Next, the LCPW reaches the Yap-Mariana Junction, where it partly enters the West Mariana Basin. Other portions flow to the region east of the Yap Trench and then flow or subside into the northern and southern trench, respectively. In the northern trench, the LCPW flows northward to the junction and finally into the West Mariana Basin. In the southern Yap Trench, the LCPW circulates cyclonically in the northern region. Water downwelling may be important for the LCPW motion in the trench.
The UCPW arrives at the Yap Trench by three pathways, all from the East Mariana Basin. The southern pathway involves passing in succession the East and West Caroline Basins, the Philippine Basin, and the West Caroline Basin or the Palau Trench, finally entering the southern Yap Trench. The middle pathway involves passing the East Caroline Basin and the channel between two adjacent ridges, finally bifurcating into the southern (mainly) and northern (downwelling water) Yap Trench at the sill. The northern pathway consists of some water flowing directly westward to the southern Mariana Trench, some entering the trench via the northern East Mariana Basin and the northern trench, and at the junction, the water flows to the northern Yap Trench. At the sill, some water enters the southern trench over the west slope, whereas the transport is reversed in the east region.
This study contributes to a more comprehensive understanding of the Yap Trench and its connections with adjacent basins and trenches. Observational data are scarce, especially in the Yap Trench. As a result, our understanding of abyssal flows and connections within the region is very limited. Considering the complexity of topography and deep flows in the region, more observation is needed in the future. The investigation at the southwest of the Yap Trench is suggested for sketching the flow path in this arc region.
The datasets presented in this article are not readily available because the topography data is from General Bathymetric Chart of the Oceans (http://www.gebco.net/data_and_products/gridded_bathymetry_data). Historical CTD and chemical data are from World Ocean Database 2018 (https://www.nodc.noaa.gov/OC5/WOD/pr_wod.html). CTD data in Nos. 37 and 38 cruises are available from the National Deep Sea Center (NDSC), Ministry of Natural Resources of China (MNR) but restrictions apply to the data, which are used under license for this study, and are not publicly available. However, data are available from the first author of this paper upon reasonable request and with NDSC permission. Chemical data of Nos. 37–38 cruises are available under the permission by Dr. Chengjun Sun of First Institute of Oceanography (FIO), MNR. Requests to access the datasets should be directed to Xuehai Liu, bGl1eGhAZmlvLm9yZy5jbg==.
WC and DL conducted fieldwork. XL contributed to conception and design of the study. XL and YL prepared the data, conducted the computation, and analyzed the results. XL prepared the manuscript. All authors contributed to the article and approved the submitted version.
This work was supported by the National Key Basic Research Program of China (Program 973) (2015CB755904), National Natural Science Foundation of China (42106146), the Scientific Research Fund of the Second Institute of Oceanography, MNR (JG2011, JG2013), the Shandong Province Postdoctoral Innovation Project (201903048), the Qingdao Postdoctoral Application Project (QDBSH2019003), and the Research Startup Project of QNLM (JCZX202020).
The authors declare that the research was conducted in the absence of any commercial or financial relationships that could be construed as a potential conflict of interest.
All claims expressed in this article are solely those of the authors and do not necessarily represent those of their affiliated organizations, or those of the publisher, the editors and the reviewers. Any product that may be evaluated in this article, or claim that may be made by its manufacturer, is not guaranteed or endorsed by the publisher.
We acknowledge the crew and scientists of submersible Jiaolong and its mother ship for conducting observation. We thank Dr. Chengjun Sun and Dr. Fenghua Jiang (FIO) for providing chemical observation data and project management. We acknowledge all the reviewers for their valuable suggestions.
De Lavergne C., Madec G., Roquet F., Holmes R. M., McDougall T. J. (2017). Abyssal Ocean Overturning Shaped by Seafloor Distribution. Nature 551, 181–186. doi: 10.1038/nature24472
Fujioka K., Okino K., Kanamatsu T., Ohara Y. (2002). Morphology and Origin of the Challenger Deep in the Southern Mariana Trench. Geophys. Res. Lett. 29, 10–11. doi: 10.1029/2001GL013595
Garcia H. E., Boyer T. P., Locarnini R. A., Baranova O. K., Zweng M. M. (2019). World Ocean Database 2018: User’s Manual (Prerelease) (Silver Spring, Maryland: National Centers for Environmental Information). Available at: https://www.ncei.noaa.gov/sites/default/files/2020-04/wodreadme_0.pdf.
Hautala S. L. (2018). The Abyssal and Deep Circulation of the Northeast Pacific Basin. Prog. Oceanogr. 160, 68–82. doi: 10.1016/j.pocean.2017.11.011
Huang C., Xie Q., Wang D., Shu Y., Xu H., Xiao J., et al. (2018). Seasonal Variability of Water Characteristics in the Challenger Deep Observed by Four Cruises. Sci. Rep. 8, 1–7. doi: 10.1038/s41598-018-30176-4
Jiang H., Xu H., Vetter P. A., Xie Q., Yu J., Shang X., et al. (2021). Ocean Circulation in the Challenger Deep Derived From Super-Deep Underwater Glider Observation. Geophys. Res. Lett. 48, e2021GL093169. doi: 10.1029/2021GL093169
Johnson G. C., Rudnick D. L., Taft B. A. (1994). Bottom Water Variability in the Samoa Passage. J. Mar. Res. 52, 177–192. doi: 10.1357/0022240943077118
Johnson G. C., Toole J. M. (1993). Flow of Deep and Bottom Waters in the Pacific at 10°N. Deep Sea Res. Part I Oceanogr. Res. Pap. 40, 371–394. doi: 10.1016/0967-0637(93)90009-R
Kawabe M., Fujio S. (2010). Pacific Ocean Circulation Based on Observation. J. Oceanogr. 66, 389–403. doi: 10.1007/S10872-010-0034-8
Kawabe M., Fujio S., Yanagimoto D. (2003). Deep-Water Circulation at Low Latitudes in the Western North Pacific. Deep. Sea Res. Part I Oceanogr. Res. Pap. 50, 631–656. doi: 10.1016/S0967-0637(03)00040-2
Kawabe M., Fujio S., Yanagimoto D., Tanaka K. (2009). Water Masses and Currents of Deep Circulation Southwest of the Shatsky Rise in the Western North Pacific. Deep Sea Res. Part I Oceanogr. Res. Pap. 56, 1675–1687. doi: 10.1016/J.DSR.2009.06.003
Kawabe M., Taira K. (1998). Water Masses and Properties at 165°E in the Western Pacific. J. Geophys. Res. Ocean. 103, 12941–12958. doi: 10.1029/97JC03197
Kawabe M., Yanagimoto D., Kitagawa S. (2006). Variations of Deep Western Boundary Currents in the Melanesian Basin in the Western North Pacific. Deep Sea Res. Part I Oceanogr. Res. Pap. 53, 942–959. doi: 10.1016/J.DSR.2006.03.003
Klatt O., Holfort J. (2000). Bottom Water Circulation in the Western Equatorial Pacific as Inferred From Carbon Tetrachloride Observations. Geophys. Res. Lett. 27, 545–548. doi: 10.1029/1999GL010501
Liu F., Cui W. C., Li X. Y. (2010). China’s First Deep Manned Submersible, JIAOLONG. Sci. China Earth Sci. 53, 1407–1410. doi: 10.1007/S11430-010-4100-2
Liu X., Liu Y., Cao W., Sun C. (2020). Water Characteristics of Abyssal and Hadal Zones in the Southern Yap Trench Observed With the Submersible Jiaolong. J. Oceanol. Limnol. 38, 593–605. doi: 10.1007/S00343-019-8368-6
Liu Y., Liu X., Lv X., Cao W., Sun C., Lu J., et al. (2018). Watermass Properties and Deep Currents in the Northern Yap Trench Observed by the Submersible Jiaolong System. Deep Sea Res. Part I Oceanogr. Res. Pap. 139, 27–42. doi: 10.1016/J.DSR.2018.06.001
Macdonald A. M., Mecking S., Robbins P. E., Toole J. M., Johnson G. C., Talley L., et al. (2009). The WOCE-Era 3-D Pacific Ocean Circulation and Heat Budget. Prog. Oceanogr. 82, 281–325. doi: 10.1016/J.POCEAN.2009.08.002
Ma Q., Wang F., Wang J., Lyu Y. (2019). Intensified Deep Ocean Variability Induced by Topographic Rossby Waves at the Pacific Yap-Mariana Junction. J. Geophys. Res. Ocean. 124, 8360–8374. doi: 10.1029/2019JC015490
McDougall T. J., Ferrari R. (2017). Abyssal Upwelling and Downwelling Driven by Near-Boundary Mixing. J. Phys. Oceanogr. 47, 261–283. doi: 10.1175/JPO-D-16-0082.1
Roemmich D., Hautala S., Rudnick D. (1996). Northward Abyssal Transport Through the Samoan Passage and Adjacent Regions. J. Geophys. Res. Ocean. 101, 14039–14055. doi: 10.1029/96JC00797
Sasaki H., Nonaka M., Masumoto Y., Sasai Y., Uehara H., Sakuma H. (2008). “An Eddy-Resolving Hindcast Simulation of the Quasi-Global Ocean From 1950 to 2003 on the Earth Simulator,“ in High Resolution Numerical Modelling of the Atmosphere and Ocean. Eds. Hamilton K., Ohfuchi W. (New York, NY: Springer), 157–185. doi: 10.1007/978-0-387-49791-4_10
Siedler G., Holfort J., Zenk W., Müller T. J., Csernok T. (2004). Deep-Water Flow in the Mariana and Caroline Basins. J. Phys. Oceanogr. 34, 566–581. doi: 10.1175/2511.1
Standardization Administration of China (2008). Gb/T 12763-2007 Specifications for Oceanographic Survey (Beijing: Standards Press of China).
Taira K., Yanagimoto D., Kitagawa S. (2005). Deep CTD Casts in the Challenger Deep, Mariana Trench. J. Oceanogr. 61, 447–454. doi: 10.1007/S10872-005-0053-Z
Talley L. D. (2007). Hydrographic Atlas of the World Ocean Circulation Experiment (WOCE) Volume 2: Pacific Ocean (Southampton: WOCE International Project Office).
Tian Z., Zhou C., Xiao X., Wang T., Qu T., Yang Q., et al. (2021). Water-Mass Properties and Circulation in the Deep and Abyssal Philippine Sea. J. Geophys. Res. Ocean. 126, e2020JC016994. doi: 10.1029/2020JC016994
van Haren H., Berndt C., Klaucke I. (2017). Ocean Mixing in Deep-Sea Trenches: New Insights From the Challenger Deep, Mariana Trench. Deep Sea Res. Part I. Oceanogr. Res. Pap. 129, 1–9. doi: 10.1016/j.dsr.2017.09.003
Voet G., Girton J. B., Alford M. H., Carter G. S., Klymak J. M., Mickett J. B. (2015). Pathways, Volume Transport, and Mixing of Abyssal Water in the Samoan Passage. J. Phys. Oceanogr. 45, 562–588. doi: 10.1175/JPO-D-14-0096.1
Wang J., Ma Q., Wang F., Lu Y., Pratt L. J. (2020). Seasonal Variation of the Deep Limb of the Pacific Meridional Overturning Circulation at Yap-Mariana Junction. J. Geophys. Res. Ocean. 125, e2019JC016017. doi: 10.1029/2019JC016017
Wang G., Xie S.-P., Qu T., Huang R. X. (2011). Deep South China Sea Circulation. Geophys. Res. Lett. 38, L05601. doi: 10.1029/2010GL046626
Wijffels S. E., Hall M. M., Joyce T., Torres D. J., Hacker P., Firing E. (1998). Multiple deep gyres of the western North Pacific: A WOCE section along 149°E. J. Geophys. Res. 103, 12985–13009.
Zhai F., Gu Y. (2020). Abyssal Circulation in the Philippine Sea. J. Ocean. Univ. China 19, 249–262. doi: 10.1029/2020JC016502
Keywords: yap trench, geostrophic flows, lower circumpolar deep water, upper circumpolar deep water, abyssal flow pathway, basin and trench
Citation: Liu X, Liu Y, Cao W and Li D (2022) Flow Pathways of Abyssal Water in the Yap Trench and Adjacent Channels and Basins. Front. Mar. Sci. 9:910941. doi: 10.3389/fmars.2022.910941
Received: 01 April 2022; Accepted: 02 June 2022;
Published: 07 July 2022.
Edited by:
Robin Robertson, Xiamen University, MalaysiaReviewed by:
Zhenhua Xu, Chinese Academy of Sciences (CAS), ChinaCopyright © 2022 Liu, Liu, Cao and Li. This is an open-access article distributed under the terms of the Creative Commons Attribution License (CC BY). The use, distribution or reproduction in other forums is permitted, provided the original author(s) and the copyright owner(s) are credited and that the original publication in this journal is cited, in accordance with accepted academic practice. No use, distribution or reproduction is permitted which does not comply with these terms.
*Correspondence: Yongzhi Liu, eXpsaXVAZmlvLm9yZy5jbg==
Disclaimer: All claims expressed in this article are solely those of the authors and do not necessarily represent those of their affiliated organizations, or those of the publisher, the editors and the reviewers. Any product that may be evaluated in this article or claim that may be made by its manufacturer is not guaranteed or endorsed by the publisher.
Research integrity at Frontiers
Learn more about the work of our research integrity team to safeguard the quality of each article we publish.