- 1Laboratory for Marine Fisheries Science and Food Production Processes, Yellow Sea Fisheries Research Institute, Chinese Academy of Fishery Sciences, Qingdao, China
- 2China Ocean Press, Beijing, China
- 3Institute of Marine Research, Bergen, Norway
- 4Wageningen Marine Research, Yerseke, Netherlands
Bioirrigation is a process corresponding to animal-induced transport of porewater solute or exchange between interstitial and overlying waters. How and why the burrowing macrobenthos complete this process remain unclear. Here, we used two infaunal-behavior observation devices to investigate the ventilation behaviors of the benthic polychaete Perinereis aibuhitensis Grube, a species that is widespread along the Asian coast with a wide temperature range, at various temperatures (5°C, 10°C, 15°C, 20°C, and 25°C). The two typical movement behaviors of P. aibuhitensis, axial crawling and radial undulation, as well as associated parameters, were delimited and quantified. We found that the radial undulation frequency of P. aibuhitensis and the associated pumping rate (PR) increased significantly with temperature (T, 5-25°C); this relationship can be described by the regression equation PR = −0.0067T2 + 0.29T - 0.52 (R2 = 0.749, P < 0.05; n = 15). The relationship between axial crawling velocity (ACV) and temperature (T, 5-25°C) can be expressed by the regression equation ACV = -0.0001T3 + 0.0059T2 - 0.063T + 0.28 (R2 = 0.997, P<0.05; n = 15). In general, pumping efficiency increased as temperature decreased, implying that the polychaete increasingly conserved energy at lower temperatures. Peak pumping volume (4.36 L d-1) was observed at 25°C, as a result of radial undulations. Thus, we concluded that radial undulation was the primary movement that led to bioirrigation. The dissolved oxygen demand was the key factor driving the initiation of radial undulation, and the specific aim of radial undulation was to increase oxygen availability by pumping new seawater into the burrow. Thus, radial undulation is critical for polychaete survival. The dissolved oxygen threshold level at which pumping was initiated increased with temperature, suggesting more energy conserved at lower temperatures. This pumping strategy of P. aibuhitensis is consistent with optimality theory, and is here designated the “optimal dissolved oxygen obtainment strategy”.
Introduction
Infauna live in sediments and seldom migrate far away, so the sedimentary environment is critical for the survival of these animals (Tsujino and Tamai, 1996). However, sediments that have not been previously affected by organisms are not suitable for infaunal life without considering the micro-environment established by infauna. For example, dissolved oxygen penetrated the top <20 mm of sediments in the Skagerrak and only a few millimeters (within 10 mm) in unaffected sediments in other reported locations (Bakker et al., 1993; Jensen et al., 1993; Shi et al., 2015). In addition, in unaffected sediments, pH is usually less than 7.0 (Isaksen and Finster, 1996; Shi et al., 2015), and may even drop below 4.0 in these environments (Peine et al., 2000). Soluble sulfide content in the pore water of sediments around the burrow inhabited by the polychaetes Nereis succinea and N. diversicolor have been shown to reach 2000 μmol L-1 (Miron and Kristensen, 1993). It is thus clear that conditions in unaffected sediments are unsuitable for most aquatic animals, including infauna. Infauna, however, can affect sediments through bioturbation in order to develop tolerable micro-environments (Volkenborn et al., 2007b).
Bioturbation encompasses two distinct processes, namely sediment reworking (i.e., the movements of sediment particles and associated organic matter) and burrow ventilation, which causes bioirrigation (i.e., the exchange of water and solute between the overlying water column and porewater) (Pascal et al., 2019). The ecological functions of bioturbation have been well studied (Zhang et al., 2013; Yazdani Foshtomi et al., 2018; Mustajärvi et al., 2019), some of which showed that the bioturbation could accelerate the biogeochemistry process of the sediment. Infauna perform bioturbation through daily activities, such as feeding, excretion, burrowing, and movement; these activities strongly affect sediment biogeochemistry (Duport et al., 2006; Mustajärvi et al., 2019). For example, bioturbation change the biological, chemical, and physical properties of the sediment and accelerate substance exchange at the sediment-water interface (Duport et al., 2006; Turek and Hoellein, 2015). More specifically, through these processes it has been observed the enlargement of the area of sediment-water interface, irrigating the sediment with oxygen-rich water, promoting the aerobic metabolism of aerobic bacteria, and increasing the decomposition of harmful substances, inhibiting anaerobic metabolism in the sediment (Cheong et al., 1993; Howa and De Resseguier, 1994; Volkenborn et al., 2007a). Bioelements, such as carbon, nitrogen, and phosphorus, are also released from the sediment into the water (Pelegri and Blackburn, 1994; Kristensen and Mikkelsen, 2003; Fernandes et al., 2006; Cournane et al., 2010).
Although it is clear that bioturbation depend on the activities of benthic animals, the details of bioturbation processes remain unclear. Previous studies have generally focused on the effects of bioturbation processes, comparing properties of the water and/or sediment before and after such processes occur (Fenchel, 1996; Zorn et al., 2006; Sun and Zheng, 2010; Jia et al., 2017; Sun et al., 2019). Due to the lack knowledge on the relation bioturbation with infauna behaviors, some studies have quantified the behavioral parameters associated with bioturbation (Riisgård and Larsen, 2005; Murphy and Reidenbach, 2016) using pressure sensors (Wethey and Woodin, 2005; Wethey et al., 2008; Woodin et al., 2016), planar optodes (Timmermann et al., 2006; Volkenborn et al., 2012a; Volkenborn et al., 2012b) and image analysis (Grémare et al., 2004; Maire et al., 2007; Bernard et al., 2016). The new equipment and technologies promoted the study on infauna behaviors. And the methods and parameters were various depending on different infauna species. Because of the numerous infauna species, there are still difficulties in understanding the relationship between bioturbation and behaviors of infauna in the sediment and their biological meanings remain poorly understood.
For the burying species, such as bivalve, it is hard to observe and determine their movement in the sediment. Researchers still made great effort to find intermittent bioirrigation and oxygen dynamics in sediment disturbed by bivalves (Volkenborn et al., 2012a). And Maire et al. (2007) successfully quantified and linked feeding activity and sediment reworking of bivalve (Abra ovata) using image analysis, laser telemetry and luminophore tracers methods. For the burrowing species, their burrow gives people chance to observe and even determine their behaviors. Oxygen in the burrow is very important for the burrowing species. Bioirrigation behavior of polychaetes drives the oxygen dynamics in the burrow (Timmermann et al., 2006; Wethey et al., 2008; Murphy and Reidenbach, 2016). But there was still lack of the quantified and classified behaviors. Since then, 2020); Pascal et al. (2019 identified and quantified “Walking”, “Ventilating”, “Burrowing” and “Resting” of mud shrimp (Upogebia pusilla) behaviors linking bioturbation, which gave new perspective on bioturbation. The same time, those references showed that different behaviors induce different bioturbation rates and types, and different infauna species had various behaviors and needed specific research methods.
Polychaetes play crucial environmental recovery functions through bioturbation (Heilskov and Holmer, 2001; Fang et al., 2014): polychaetes bioturbation is thought to accelerate the environmental recovery of sediments (Kristensen, 2001; Fernandes et al., 2006; Cournane et al., 2010; Mustajärvi et al., 2019). They are usually major contributors to the benthic communities (Tomassetti and Porrello, 2005; Shimabukuro et al., 2016; Sivadas and Ingole, 2016). The polychaete Perinereis aibuhitensis Grube is widely distributed in the tidal zone on the west coast of the Pacific Ocean. It has a wide temperature adaption (1-28°C in the natural habitat sediment). In order to get suitable living micro-environment, P. aibuhitensis must ventilate in the burrow, which possibly induces series behaviors. However, we know little about the behaviors and the relationships between the behaviors and the micro-environment such as dissolved oxygen, nutrient concentration and water flowing, in its burrow. In this study, we aimed to link the behavior with bioturbation of infauna by investigating the relationships between quantified behaviors and environmental parameters in the P. aibuhitensis burrow at different temperatures. The work was in order to provide knowledge on the behavioral strategies of infauna adapting the micro-environment in the burrow, which gave a behavioral process perspective of infauna bioturbation.
Materials and methods
Polychaete Collection and Acclimation
Sediment and polychaetes (P. aibuhitensis, 1.45 ± 0.25 g WW) were collected in the natural habitat of P. aibuhitensis: Dingzi Bay, Shandong province, China (120°54’35 E, 36°38’51 N) in April, 2019. The original sediment without sieving to a depth of 10 cm was added to three aquaria (L × W × H = 60 cm × 60 cm × 35 cm) filled with recirculating seawater. Sediment was kept in the system for one week. After that, polychaetes were collected. The inactive and damaged animals were removed, and the remaining polychaetes (about 200 ind.) were acclimated for another week. The temperature, salinity, pH, and dissolved oxygen of the seawater in the circulating water system were maintained at 20°C ± 1°C, 29–31, 7.92-8.07, and 7.2–8.2 mg L-1, respectively. Each day, about 30% of the water in the system was replaced with clean seawater. According to the natural photoperiod in April in Shandong Province, China, a simulated natural photoperiod (12 h light: 12 h dark) was used throughout the period of the experiment.
Experimental Design
We measured the temperatures in the sediments inhabited by P. aibuhitensis in summer (Aug.) and winter (Feb.). At a depth of 20 cm, the temperature ranged from 0.9°C in the winter to 26.1°C in the summer. We selected five experimental temperatures within this range: 5°C, 10°C, 15°C, 20°C, and 25°C. Because the polychaetes were acclimated to a water temperature of 20°C in the artificial circulating system, all experiments began at this water temperature. The temperature of the circulating acclimation system was then adjusted about 1°C each day until the desired experimental temperature was reached. Once the target temperature had been reached, the animals were acclimated for another three days before observation.
The water temperatures in the infaunal-behavior observation devices (Figure S1, IBOD) and in the aquarium surrounding the artificial burrow devices (Figure S2, ABD) were adjusted to the experimental temperature using chillers (L-650, Risheng, China). Dissolved oxygen (DO) levels (Oxy-SMA-1, Presens, Germany) in the aquarium were maintained at 7.5–8.0 mg L-1. One polychaete was randomly selected and placed in the infaunal chamber of the IBOD at about 17:00. The polychaete was allowed to burrow into the sediment overnight. At about 7:30 the next morning, the burrow was checked and cleaned as described in the M1 part of Supporting Information. Before the experiment, we did a pre-experiment as a reference to decide the experimental video record duration. According to the result, the average interval duration including movement and stopping time was only 9-10 min. So the motions of each polychaete were then recorded two hours daily with the video system from 9:00 to 10:00 (daytime) and from 21:00 to 22:00 (nighttime). After the completion of the nighttime recording, a flat-headed iron wire was slowly inserted into the burrow to expel the polychaete, and the burrow was refilled with sediment.
The polychaete was transferred directly to the burrow of the ABD and allowed to acclimate overnight. The following day, the motions of the polychaete were again recorded with the video system from 9:00 to 10:00 (daytime) and from 21:00 to 22:00 (nighttime). DO levels at the tail end of the burrow were measured every 3 seconds during the observation period. In addition, during the radial undulating motion period, 4–6 ml of water were slowly removed from both the inflow (head direction) and outflow (tail direction) of the burrow (about 0.5-1.0 cm in the burrow opening) using different disposable droppers during the observation periods. Each water sample was filtered through a 0.45 μm glass fiber filter, and the filtrate was stored in a freezer at −80°C.
Next, the tracer beads (SM4) were used to measure the volume of water pumped by each P. aibuhitensis in the ABD. A tracer bead was placed at the inlet of the burrow, where it moved in response to the slight current caused by the moving polychaete. The movements of the tracer bead were then recorded using the video system.
Three replicates of this entire experiment were performed, using different animals, at each experimental temperature. There was no death during the experimental period.
Analysis
Typical Behaviors of P. aibuhitensis
Axial crawling motion is defined as movement forward or backward along the axis of the burrow (whether or not part of the polychaete exited the burrow). Radial undulating motion is defined as undulation along the body (from the head to the tail) without axial movement. Body undulations are always in the same direction as the radial direction of the burrow.
Motion Analysis
Recordings of polychaete movement were analyzed using ImageJ 2019 (National Institutes of Health, USA), which has a variety of image processing and analysis tools, in order to quantify axial crawling motion and radial undulating motion. Because polychaetes in both devices remained in burrows on a single plane perpendicular to the camera, motion parameters were easily determined. Parameters of polychaete motion were assessed using the ImageJ manual tracking plug-in module. In the ABD, the movements of the tracer beads were also recorded as a function of axial crawling motion and radial undulating motion. Tracer velocity was assumed to be equivalent to the current velocity in the borrow induced by pumping.
Behavioral and Chemical Analysis in ABD
The following behavioral and chemical parameters were measured in the ABD: pumping efficiency, pumping volume, DO concentration, and nutrient dissolution efficiency (Table S1). When calculating the surface area of the artificial burrow, the area of the transparent portions on both sides of the burrow were thus subtracted from the total surface area. The burrow surface area was used to calculate nutrient dissolution efficiency (Table S2).
The frozen water samples from the ABD were analyzed using a nutrient analyzer (CleverChem380, Germany) to determine the concentrations of phosphate, nitrite, ammonia nitrogen, and sulfide. Due to the short duration of each experiment, the amount of ammonia excreted by each P. aibuhitensis was negligible, which accounted for about 4% of the average ammonium concentration difference between inflow and outflow. Therefore, these trace amounts of ammonia were ignored.
Behavioral Parameters in IBOD
The following behavioral parameters were measured in the IBOD: radial undulation duration, radial undulation frequency, axial crawling duration, axial crawling velocity (Table S2).
Data Analysis
All statistical analyses were performed in SPSS Statistics 23.0 (IBM, USA). The effects of the interaction between temperature and time of day on radial undulation duration and axial crawling duration were analyzed using two-way analyses of variance (ANOVAs). If a given interaction between temperature and time of day had a significant effect on radial undulation duration or axial crawling duration, a one-way ANOVA followed by Duncan’s multiple range tests for post hoc pairwise comparisons was used to analyze the effect of each of the two interacting factors on radial undulation duration and axial crawling duration. A Bonferroni adjustment was applied to all parameters to avoid the magnification of statistical errors resulting from the repeated use of a one-way ANOVA. We also assessed the effects of temperature on radial undulation frequency, axial crawling velocity, pumping efficiency, pumping volume, DO concentration, and nutrient dissolution efficiency using one-way ANOVAs followed by Duncan’s multiple range tests for post hoc pairwise comparisons. Stepwise multiple regression analysis were used to visualize the relationships between temperature and radial undulating frequency, axial crawling velocity, pumping rate, and pumping volume. The assumption of homogeneity of variances was tested for all data. If normal distribution assumptions were not met, the data were (log-) transformed. Differences were considered statistically significant at P<0.05.
Results
Radial Undulation
Temperature significantly affected the radial undulating frequency (F=115.284, P<0.001, df=4) of P. aibuhitensis and the associated pumping rate (F=6.632, P=0.007, df=4). Both radial undulation frequency and pumping rate increased significantly as water temperature increased (P<0.05, Figure 1). The radial undulation frequency at 25°C was significantly greater than those at all other temperatures (P<0.05), while the radial undulation frequency at 5°C was significantly lower. The pumping rates at 15–25°C were significantly greater than those at 5–10°C (P<0.05). The relationship between temperature (T) and radial undulation frequency (RUF) could be expressed as RUF=1.03+1.54T (R2 = 0.98, P<0.05, n=15). The relationship between temperature (T) and pumping rate (PR) could be expressed as ln(PR)=0.45+0.72 ln(T) (R2 = 0.72, P<0.05, n=15).
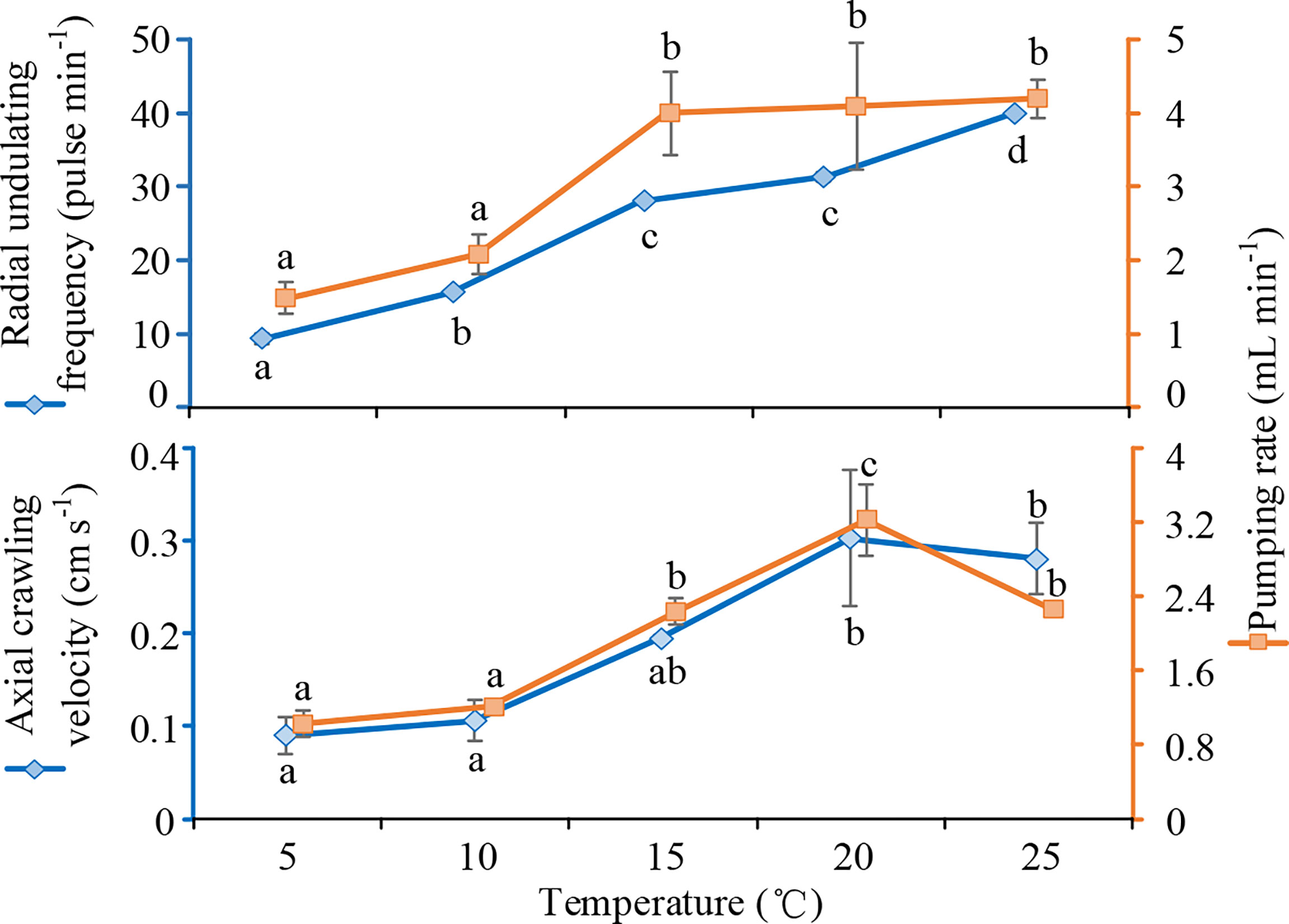
Figure 1 The radial undulation frequencies (pulse min-1), axial crawling velocities (cm s-1) and their corresponding associated pumping rates (mL min-1) of Perinereis aibuhitensis at various temperatures. Means labelled with different lowercase letters are significantly different (n=3, P<0.05). Error bars represent 1 S.E.
Axial Crawling Motion
Temperature significantly affected the axial crawling velocity of P.Baibuhitensis (F=6.136, P=0.009, df=4, one-way ANOVA), but not the associated pumping rate (F=2.027, P=0.166, df=4). The axial crawling velocities at 20–25°C were significantly greater than those at 5–10°C (P<0.05, Figure 1). The pumping rate associated with the axial crawling velocity of P. aibuhitensis was highest at 20°C; this rate was significantly greater than the rate at any of the other temperatures tested (P<0.05). The relationship between temperature (T) and pumping rate (PR) could be expressed as PR=−0.0067T2+0.29T−0.52 (R2 = 0.75, P<0.05, n=15). This equation predicted that pumping rate would peak at 20.7°C. The relationship between temperature (T) and axial crawling velocity (ACV) could expressed as ACV=−0.0001T3+0.0059T2−0.063T+0.28 (R2 = 0.997, P<0.05, n=15).
Pumping and Motion Duration
Temperature did not significantly affect the pumping efficiency (PE) of either radial undulation (F=0.759, P=0.575, df=4) or axial crawling (F=2.063, P=0.137, df=4) in P. aibuhitensis. However, PE had a tendency to decrease as temperature increased (Table 1).

Table 1 Dissolved oxygen of threshold initiating pumping behaviors, pumping efficiencies (PE) associated with radial undulation and axial crawling of the Perinereis aibuhitensis at different temperatures.
The total duration of the radial undulating motion was significantly affected by temperature (F=11.443, P=0.001, df=4), but the duration of the axial crawling motion was not (F=0.166, P=0.951, df=4, Figure 2; Table S3). The pumping volume associated with radial undulation also increased significantly as temperature increased (F=3.666, P=0.024, df=4, Figure 2; Figure S3). Pumping volume and radial undulation duration both peaked at 25°C; these figures were significantly greater than the corresponding figures at 5°C (Figure 2). Indeed, radial undulation time at 25°C was two times higher than at 5°C, while pumping volume at 25°C was nine times higher than at 5°C and almost six times that of axial crawling motion at 20°C.
The pumping volume associated with the axial crawling motion increased with temperature until 20°C, then decreased when temperature reached 25°C (F=3.743, P=0.041, df=4). Pumping volumes at 20°C and 25°C were significantly greater than those at 5°C and 10°C (Figure 2). The pumping volumes associated with the radial undulating motion were uniformly greater than those associated with the axial crawling motion at the same temperature (Figure 2).
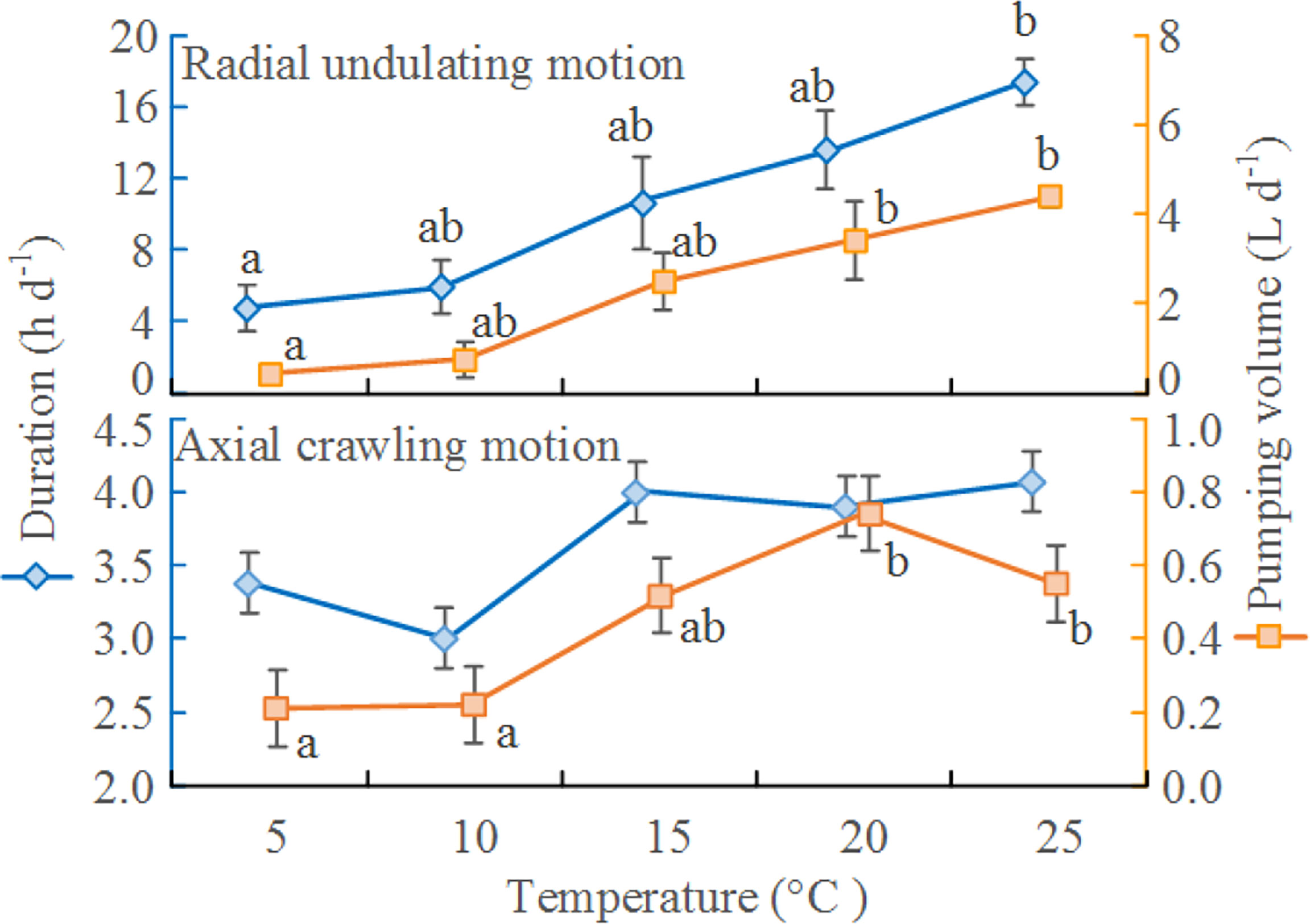
Figure 2 Estimated durations of radial undulation and axial crawling in Perinereis aibuhitensis over 24 h at different temperatures, with the associated volumes of water pumped. Means labelled with different lowercase letters are significantly different (n=3, P<0.05). Error bars represent 1 S.E.
The Efficiency of Nutrient Dissolution from the Sediment
The dissolution efficiencies of phosphate (F=3.532, P=0.032, df=4), ammonia nitrogen (F=3.314, P=0.035, df=4), nitrite (F=6.544, P=0.007, df=4), and sulfide (F=11.466, P=0.001, df=4) from the sediment in the polychaete burrow increased significantly between 5°C and 25°C (P<0.05). In general, dissolution efficiencies increased steadily with temperature, although the dissolution efficiency of phosphate was significantly greater at 20°C than at 25°C (Figure 3). The dissolution efficiencies of phosphate and ammonia nitrogen were noticeably greater than those of nitrite and sulfide (Figure 3).
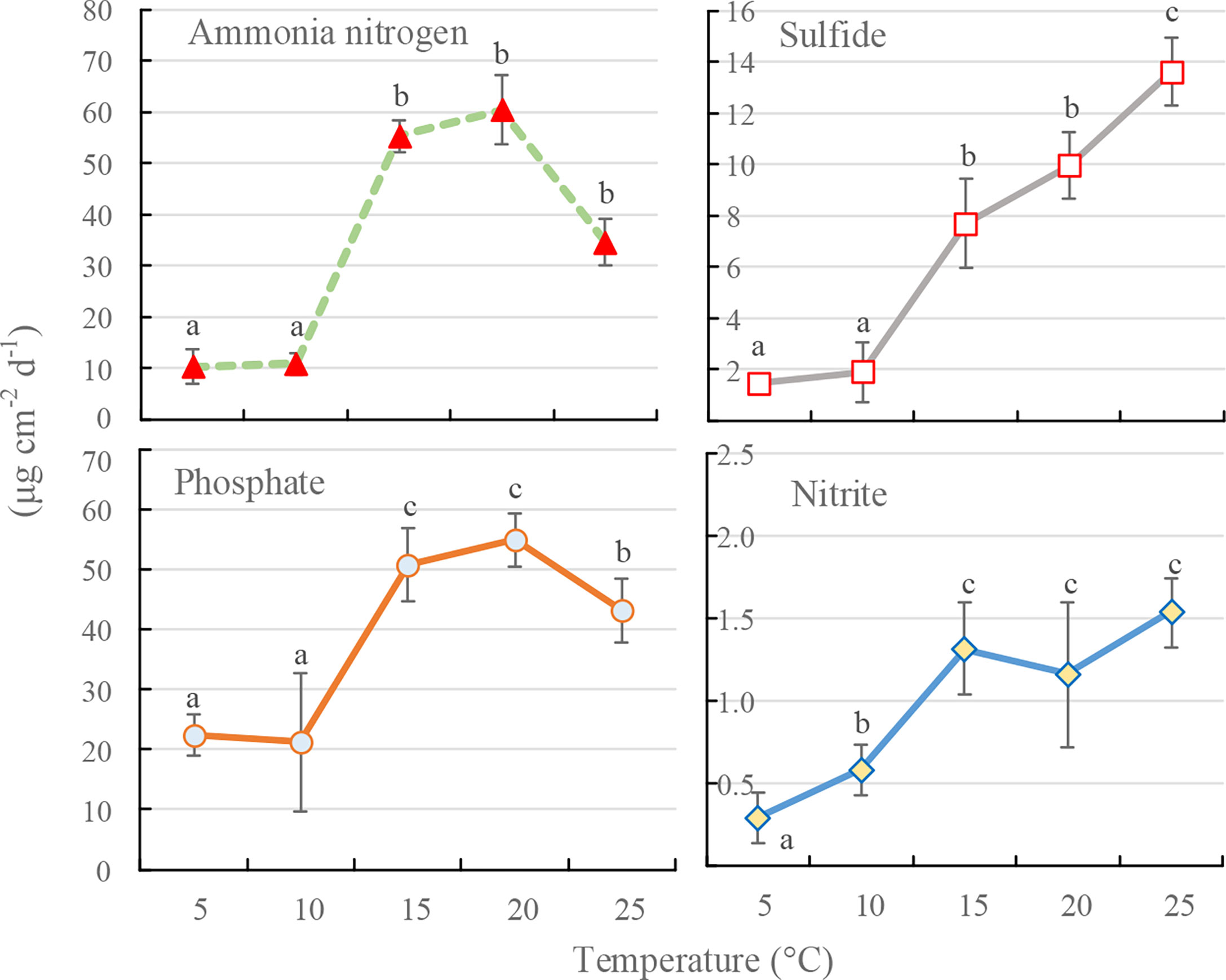
Figure 3 Dissolution efficiencies of various solutes in the burrows of Perinereis aibuhitensis over 24 h at different temperatures. Means labelled with different lowercase letters are significantly different (n=3, P<0.05). Error bars represent 1 S.D.
Fluctuations in DO
DO levels in the tail shaft of the burrow and the corresponding pumping behaviors were recorded continuously. In general, the polychaete initiated pumping when DO fell to very low levels (Figure S4). We considered these low levels the “DO threshold”, after which pumping behaviors were initiated. The DO threshold tended to increase with temperature (F=52.317, P<0.001, df=4): the DO thresholds at 5°C, 10°C, and 20°C were significantly lower than those at 15°C and 25°C (P<0.05, Table 1).
Discussion
Our results show that, in P. aibuhitensis, radial undulation frequency and the associated pumping rate, axial crawling velocity and the associated pumping rate, the duration of radial undulation, and the duration of axial crawling motion decreased significantly when the water temperature decreased from 25°C to 5°C. At a lower temperature, the poikilothermal animals including Perinereis aibuhitensis will reduce their activities (Dall, 1958; Zhou, 2005; Pan, 2015). This is in line with previous studies which have shown that temperature significantly affects the activities of poikilothermal aquatic animals. For example, it has previously been found that the activity of the shrimp Metapenaeus mastersii decreased at 16°C and improved at higher temperatures (Dall, 1958). Similarly, the swimming speed, scaphognathite intermittent beat rate, and activity of the shrimp Litopenaeus vannamei decreased as temperature was reduced from 36°C to 18°C (Zhou, 2005). In addition, Pan (2015) found that the sea cucumber Apostichopus japonicas moved less in the winter. However, quantitative studies of the effects of temperature on poikilothermal infauna in the sediment are rare. The range of temperature in the present study covered the natural temperature fluctuations in the habitat of Perinereis aibuhitensis. This range of temperatures reflects those experienced by P. aibuhitensis in its natural habitat. Interestingly, axial crawling velocity was similar at 20°C and 25°C, possibly because 20°C is the optimum growth temperature for this species (Fang et al., 2016). This suggests temperatures of 25°C and above are suboptimal for P. aibuhitensis.
The primary motions of P. aibuhitensis in the burrow were radial undulation and axial crawling. Axial crawling (common instinct) is initiated for several purposes, including movement, drilling, searching for food, and avoiding threats, which is a common knowledge. When a polychaete changes position in a burrow, the resulting movement of water was denoted “piston-pumping” by Forster and Graf (1995). In the polychaete Lanice conchilega, the pumping volume was only 0.04 L d-1 if the animal continuously pump water. Additionally, the effects of radial undulation were not assessed in Forster and Graf, 1995. In the present study, the maximum pumping volume of axial crawling motion in a day was much less than that pumped by radial undulating motion, both much more than Lanice conchilega, in a day. Thus, pumping water is not the aim of axial crawling, but only the result. In contrast, the main function of radial undulation in P. aibuhitensis seems to be water pumping resulting in burrow ventilation.
Infauna, including polychaetes, pump water to induce burrow ventilation, which could change the porewater pressure and flux in the sediment (Wethey et al., 2008). The porewater exchange rates were very slow and there were seasonal differences (Pascal et al., 2019). Infauna-driven ventilation accelerates the porewater exchange in the burrow. And the “Ventilating” behavior improves water exchange and accelerates the flux of certain solutes, such as oxygen, carbon, nitrogen, and other nutrients, at the sediment-water interface (Forster and Graf, 1995; Van Hoey, 2006; Duport et al., 2007; Na et al., 2008; Turek and Hoellein, 2015; Pascal et al., 2019). Substance flux tend to increase with temperature and the density of benthic animals at the sediment-water interface (Mahl et al., 2009; Zhang et al., 2013). However, bioirrigation-driven substance flux may reach a stable level at the individual level. Here, the pumping duration and total volume of water pumped by P. aibuhitensis increased with the increasing temperature. In addition, nitrogen, phosphorus, sulfide, and oxygen exchange between the sediment and the water increased due to P. aibuhitensis bioirrigation. However, the dissolution efficiencies of ammonia nitrogen, phosphate, and nitrite tended to remain stable above 15°C (although phosphate dissolution efficiency decreased significantly at 25°C). In contrast, sulfide dissolution efficiency increased steadily with temperature, reaching a significant peak at 25°C. This suggested that the degree of nutrient flux induced by the bioirrigation behaviors of an individual could reach limited level.
In addition, the strategy of bioirrigation, which is an energy-intensive process, are unclear. In particular, pumping water seems to be the sole aim of polychaete radial undulation in the present study. It is possible that water pumping helps to remove harmful solutes from the burrow. The maximum concentrations of ammonia nitrogen, nitrite, phosphate, and sulfide recorded in the P. aibuhitensis burrows in this study were 0.28 mg L-1, 0.007 mg L-1, 0.15 mg L-1, and 0.01 mg L-1, respectively. However, studies have indicated that P. aibuhitensis can tolerate much higher concentrations of these solutes. For example, P. aibuhitensis was shown to tolerate ammonia nitrogen levels above 3.31 mg L-1 (Lv et al., 2009) and sulfide levels above 0.031 mg L-1 (as H2S) (Wang et al., 2017). In addition, concentrations of nitrite below 0.504 mg L-1 did not significantly affect the ammonia excretion and oxygen consumption of P. aibuhitensis (Liu et al., 2016). Finally, the maximum phosphate concentration in our experiment was well within that of the seawater in the natural habitat of P. aibuhitensis (0.087–0.968 mg L-1) (Zhang et al., 2018). Several other studies have shown that P. aibuhitensis has a strong tolerance for various poisonous chemicals (Li et al., 2018a; Li et al., 2018b). Thus, the results show that it is unlikely that P. aibuhitensis pumped water with the purpose to remove harmful solutes from the burrow, it is possible that the removal of harmful solutes is a secondary result from water pumping.
Another possibility is that P. aibuhitensis pumps water to increase DO supply in the burrow. The DO dynamics in the burrow has been well studied in several burrowing species (Timmermann et al., 2006; Murphy and Reidenbach, 2016; Pascal et al., 2019). The fluctuations of DO levels were strong correlated with the irrigation behaviors. The DO decreases to a very low level when the animal “Resting” or “Walking” with almost no water pumping. Then they would initiate pumping behavior to get more oxygen and the DO fluctuated up and down in cycle. In this study, the minimum DO level in the burrow (at 5°C) was 0.80 mg L-1. It was previously shown that the polychaete Lumbrinereis latreilli was significantly negatively affected by DO levels below 1.0 mg L-1, but experienced no adverse effects at DO levels greater than 2.0 mg L-1 (Yang et al., 2019). Consistent with this, our results showed that when DO levels in the burrows of P. aibuhitensis decreased below a temperature-dependent threshold (0.80–2.19 mg L-1), radial undulation was initiated to pump new water into the burrow. In general, the DO threshold increased with water temperature (Table 1). In addition, radial undulation ceased when DO levels were equivalent to those in the surrounding seawater (Figure S4). Based on our results we concluded that DO was the key factor driving the initiation of radial undulation, and that the goal of radial undulation was to increase the DO in the burrow by pumping in new seawater. Thus, radial undulation is likely to be crucial for polychaete survival.
The radial undulating motion started because the DO was low in the burrow and stopped when the DO reached a similar level with overlying water. It was a recycling behavior to keep the DO level for living in the burrow. The initiation of radial undulation in response to low DO levels was consistent with the predictions of optimality theory, which is similar to optimal foraging theory (Shang, 2014), for two reasons: First, radial undulation was not initiated by P. aibuhitensis unless DO levels decreased below a threshold, which conserves energy. Second, the pumping efficiencies of both radial undulation (mL pulse-1) and axial crawling (mL cm-1) in P. aibuhitensis increased as temperature decreased. That is, radial undulation frequency and axial crawling velocity were significantly lower at 5°C than at 25°C. This implied that the lower the temperature, the more water was pumped due to the completion of a single action. In addition, water continued to flow after the completion of each pumping action due to inertia. The P. aibuhitensis pumping strategy was thus consistent with the predictions of optimality theory, because the polychaete conserved energy at low temperatures, when food consumption and energy income were reduced (Fang et al., 2016). We here designate this pumping strategy as the “optimal dissolved oxygen obtainment strategy”. Although the optimal dissolved oxygen obtainment strategy may be common among infauna, our study is one of the few studies that have examined how infaunal species obtain oxygen. Further study of activity modifications in response to DO demand is required.
According to the previous studies and the present study, the motion behaviors of infauna have at least two important ecological meanings: benefit the infauna living and induce bioturbation. The ventilation of animals could increase DO in the burrow, which was certificated in the present study and the cited articles (Hagerman and Uglow, 1985; Forster and Graf, 1995; Timmermann et al., 2006; Murphy and Reidenbach, 2016; Pascal et al., 2019). And DO is the key factor promotes ventilation behaviors (present study). The polychaete has “optimal dissolved oxygen obtainment strategy” to save living energy adapting sedimentary environment at different temperatures. Through motion behaviors, the infauna accelerates the porewater flux, water exchange and flutes flux at the sediment-water interface. The individual motion behaviors related with flutes flux were determined in the present study. It is possible to do similar work in other infauna such as Pascal et al. (2019) having done in U. pusilla. Due to the high density of infauna, P. aibuhitensis could reach about 300 ind. m-2 (Hu et al., 2021) and Capitella capitata could reach densities up to 46,000 ind. m-2 (Bannister et al., 2014), they play important roles in accelerating the biogeochemistry process of sediment through behavior. The ecological model of bioturbation effects, including flutes flux and sediment reworking, in a large scale habitat could be done according to the quantified individual behavior in the future, which would be more credible and accurate to explain and quantify the ecological function of bioturbation.
Data Availability Statement
The original contributions presented in the study are included in the article/Supplementary Material. Further inquiries can be directed to the corresponding author.
Author Contributions
JF obtained funding and directed the project. He designed the experiment. JF and SM planned the experiment and collected the data. WJ helped to carry out the experiment. SM and RL analyzed the data. JF and SM wrote the first draft of the manuscript supervised by JF and ØS. ZJ, YM, and HJ critically reviewed the initial manuscript and provided helpful input. JF and SM have equal contribution to the manuscript. All authors approved the final manuscript.
Funding
This study was funded by the National Natural Science Foundation of China (41876185), Central Public-interest Scientific Institution Basal Research Fund, YSFRI, CAFS (20603022022020), Environment and Aquaculture Governance Phase III (CHN 2152, 17/0033).
Conflict of Interest
The authors declare that the research was conducted in the absence of any commercial or financial relationships that could be construed as a potential conflict of interest.
Publisher’s Note
All claims expressed in this article are solely those of the authors and do not necessarily represent those of their affiliated organizations, or those of the publisher, the editors and the reviewers. Any product that may be evaluated in this article, or claim that may be made by its manufacturer, is not guaranteed or endorsed by the publisher.
Acknowledgments
We thank Bo Liang, Wei He and Chenggang Lin for helpful assistance in preparing the experiment.
Supplementary Material
The Supplementary Material for this article can be found online at: https://www.frontiersin.org/articles/10.3389/fmars.2022.910781/full#supplementary-material
References
Bakker J. F., Helder W., Liebezeit G., van Weering T. C. E., Rumohr J. (1993). Skagerrak (Northeastern North Sea) Oxygen Microprofiles and Porewater Chemistry in Sediments. Mar. Geol. 111, 299–321. doi: 10.1016/0025-3227(93)90137-K
Bannister R. J., Valdemarsen T., Hansen P. K., Holmer M., Ervik A. (2014). Changes in Benthic Sediment Conditions Under an Atlantic Salmon Farm at a Deep, Well-Flushed Coastal Site. Aquacult. Env. Interact. 5 (1), 29–47. doi: 10.3354/aei00092
Bernard G., Duchêne J.-C., Romero-Ramirez A., Lecroart P., Maire O., Ciutat A., et al. (2016). Experimental Assessment of the Effects of Temperature and Food Availability on Particle Mixing by the Bivalve Abra Alba Using New Image Analysis Techniques. PloS One 11, e0154270. doi: 10.1371/journal.pone.0154270
Cheong H., Shankar N. J., Radhakrishnan R., Toh A. (1993). Estimation of Sand Transport by Use of Tracers Along a Reclaimed Shoreline at Singapore Changi Airport. Coast. Eng. 19, 311–325. doi: 10.1016/0378-3839(93)90034-6
Cournane S., León Vintró L., Mitchell P. I. (2010). Modelling the Reworking Effects of Bioturbation on the Incorporation of Radionuclides Into the Sediment Column: Implications for the Fate of Particle-Reactive Radionuclides in Irish Sea Sediments. J. Environ. Radioactiv. 101, 985–991. doi: 10.1016/j.jenvrad.2010.07.006
Dall W. (1958). Observations on the Biology of the Green Tail Prawn, Metapenaeus Mastersii (Haswell) (Crustacea Decapoda: Penaeidae). Mar. Freshw. Res., 9 (1),111–134. doi: 10.1071/MF9580111
Duport E., Gilbert F., Poggiale J. C., Dedieu K., Rabouille C., Stora G. (2007). Benthic Macrofauna and Sediment Reworking Quantification in Contrasted Environments in the Thau Lagoon. Estuar. Coast. Shelf Sci. 72, 522–533. doi: 10.1016/j.ecss.2006.11.018
Duport E., Stora G., Tremblay P., Gilbert F. (2006). Effects of Population Density on the Sediment Mixing Induced by the Gallery-Diffusor Hediste (Nereis) Diversicolor O.F. Müller 1776. J. Exp. Mar. Biol. Ecol. 336, 33–41. doi: 10.1016/j.jembe.2006.04.005
Fang J., Zhang J., Jiang Z., Du M., Liu Y., Mao Y., et al. (2016). Environmental Remediation Potential of Perinereis Aibuhitensis (Polychaeta) Based on the Effects of Temperature and Feed Types on Its Carbon and Nitrogen Budgets. Mar. Biol. Res. 12, 583–594. doi: 10.1080/17451000.2016.1177653
Fang J., Zhang J., Wu W., Mao Y., Gao Y., Jiang Z., et al. (2014). Carbon and Nitrogen Budget and Environmental Optimization in an Integrated Cage Culture Model of Japanese Flounder With Perinereis Aibuhitensis. J.Fish. Sci. Chin. 21, 390–397. doi: 10.3724/sp.j.1118.2014.00390
Fenchel T. (1996). Worm Burrows and Oxic Microniches in Marine Sediments. 1. Spatial and Temporal Scales. Mar. Biol. 127, 289–295. doi: 10.1007/BF00942114
Fernandes S., Meysman F. J. R., Sobral P. (2006). The Influence of Cu Contamination on Nereis Diversicolor Bioturbation. Mar. Chem. 102, 148–158. doi: 10.1016/j.marchem.2005.12.002
Forster S., Graf G. (1995). Impact of Irrigation on Oxygen Flux Into the Sediment: Intermittent Pumping by Callianassa Subterranea and ‘Piston-Pumping’ by Lanice Conchilega. Mar. Biol. 123, 335–346. doi: 10.1007/BF00353625
Grémare A., Duchêne J.-C., Rosenberg R., David E., Desmalades M. (2004). Feeding Behaviour and Functional Response of Abra Ovata and A. Nitida Compared by Image Analysis. Mar. Ecol. Prog. Ser. 267, 195–208. doi: 10.3354/meps267195
Hagerman L., Uglow R. F. (1985). Effects of Hypoxia on the Respiratory and Circulatory Regulation of Nephrops Norvegicus. Mar. Biol. 87, 273–278. doi: 10.1007/BF00397805
Heilskov A. C., Holmer M. (2001). Effects of Benthic Fauna on Organic Matter Mineralization in Fish-Farm Sediments: Importance of Size and Abundance. ICES J. Mar. Sci. 58, 427–434. doi: 10.1006/jmsc.2000.1026
Howa H. L., De Resseguier A. (1994). Application of a Fluorescent Grain Detector/Counter for Sand Transport Evaluation in the Littoral Zone. Proc. Ocean OSATES Conference. 3, 254–257.
Hu F., Sun M., Fang J., Wang G., Li L., Gao F., et al. (2021). Carbon and Nitrogen Budget in Fish-Polychaete Integrated Aquaculture System. J. Ocean. Limnol. 39 (3), 1151–1159. doi: 10.1007/s00343-020-0218-z
Isaksen M. F., Finster K. (1996). Sulphate Reduction in the Root Zone of the Seagrass Zostera Noltii on the Intertidal Flats of a Coastal Lagoon (Arcachon, France). Mar. Ecol. Prog. Ser. 137, 187–194. doi: 10.3354/meps137187
Jensen K., Revsbech N. P., Nielsen L. P. (1993). Microscale Distribution of Nitrification Activity in Sediment Determined With a Shielded Microsensor for Nitrate. Appl. Environ. Microbiol. 59, 3287–3296. doi: 10.1002/yea.320091115
Jia X., Zhang T., Tian S. (2017). Particle Reworking by the Sediment-Living Polychaetes Perinereis Aibuhitensis. Mar. Sci. Bull. 19, 85–90. doi: 10.ssss/j.issn.1000-9620.2017.1.006
Kristensen E. (2001). Impact of Polychaetes (Nereis Spp. And Arenicola Marina) on Carbon Biogeochemistry in Coastal Marine. Geochem. T. 12, 1–12. doi: 10.1186/1467-4866-2-92
Kristensen E., Mikkelsen O. L. (2003). Impact of the Burrow-Dwelling Polychaete Nereis Diversicolor on the Degradation of Fresh and Aged Macroalgal Detritus in a Coastal Marine Sediment. Mar. Ecol. Prog. Ser. 265, 141–153. doi: 10.3354/meps265141
Li J., Tong Y., Cheng Q., Tian S. (2018a). Acute Toxicity and the Effects on Antioxidant Enzyme Activities of Polychaete Perinereis Aibuhitensis Exposed to Crude Oil in Seawater. Res. Envir. Sci. 31, 1972–1978. doi: 10.13198/j.issn.1001-6929.2018.03.10
Liu H., Guo W., Tan Z., Yang S., Sun C., Wu K. (2016). Effects of Ph, Ammonia and Nitrite on Oxygen Consumption and Ammonia Excretion of Perinereis Aibuhitensis. J. Guangdong Ocean Uni. 36, 52–56. doi: 10.3969/j.issn.1673-9159.2016.03.009
Li W., Xue S., Pang M., Yue Z., Yang D., Zhou Y., et al. (2018b). The Expression Characteristics of Vitellogenin (VTG) in Response to B(a)p Exposure in Polychaete Perinereis Aibuhitensis. J. Ocean. Lim. 36, 2297–2307. doi: 10.1007/s00343-019-7304-0
Lv F., Huang J., Lv L., Dong X. (2009). Study on the Toxicities of Ammonia-N to Perinereis Aibuhitensis Under Different Salinities. J. Anhui Agric. Sci. 37, 2986–2987. doi: 10.3969/j.issn.0517-6611.2009.07.076
Mahl U. H., Tyler A. C., Howarth R. W., Lenaghan N. M. (2009). “Effects of Macroinvertebrate Species Composition and Density on Benthic Oxygen and Nutrient Flux and Concentrations of Ammonium and Soluble-Sulfides in Sediment Porewater in Shallow Estuaries,” in The 94th ESA Annual Meeting, Albuquerque, New Mexico.
Maire O., Duchêne J.-C., Bigot L., Grémare A. (2007). Linking Feeding Activity and Sediment Reworking in the Deposit-Feeding Bivalve Abra Ovata With Image Analysis, Laser Telemetry, and Luminophore Tracers. Mar. Ecol. Prog. Ser. 351, 139–150. doi: 10.3354/meps07147
Miron G., Kristensen E. (1993). Factors Influencing the Distribution of Nereid Polychaetes: The Sulfide Aspect. Mar. Ecol. Prog. Ser. 93, 143–153. doi: 10.3354/meps093143
Murphy E. A. K., Reidenbach M. A. (2016). Oxygen Transport in Periodically Ventilated Polychaete Burrows. Mar. Biol. 163, 208. doi: 10.1007/s00227-016-2983-y
Mustajärvi L., Nybom I., Eriksson Wiklund A. K., Eek E., Cornelissen G., Sobek A. (2019). How Important Is Bioturbation for Sediment-to-Water Flux of Polycyclic Aromatic Hydrocarbons in the Baltic Sea? Environ. Toxicol. Chem. 38, 1803–1810. doi: 10.1002/etc.4459
Na T., Gribsholt B., Galaktionov O. S., Lee T., Meysman F. J. R. (2008). Influence of Advective Bio-Irrigation on Carbon and Nitrogen Cycling in Sandy Sediments. J. Mar. Res. 66, 691–722. doi: 10.1357/002224008787536826
Pan Y. (2015). Quantitative Research on Motor Behavioral Rhythms of Sea Cucumber Apostichopus Japonicas (Selenka) (Beijing: The University of Chinese Academy of Sciences). Doctoral Dissertation.
Pascal L., Grémare A., de Montaudouin X., Deflandre B., Romero-Ramirez A., Maire O. (2020). Parasitism in Ecosystem Engineer Species: A Key Factor Controlling Marine Ecosystem Functioning. J. Anim. Ecol. 89 (9), 2192–2205. doi: 10.1111/1365-2656.13236
Pascal L., Maire O., Deflandre B., Romero-Ramirez A., Gremare A. (2019). Linking Behaviours, Sediment Reworking, Bioirrigation and Oxygen Dynamics in a Soft-Bottom Ecosystem Engineer: The Mud Shrimp Upogebia Pusilla (Petagna 1792). J. Exp. Mar. Biol. Ecol. 516, 67–78. doi: 10.1016/j.jembe.2019.05.007
Peine A., Tritschler A., Küsel K., Peiffer S. (2000). Electron Flow in an Iron-Rich Acidic Sediment-Evidence for an Acidity-Driven Iron Cycle. Limnol. Oceanogr. 45, 1077–1087. doi: 10.4319/lo.2000.45.5.1077
Pelegri S. P., Blackburn T. H. (1994). Bioturbation Effects of the Amphipod Corophium Volutator on Microbial Nitrogen Transformations in Marine Sediments. Mar. Biol. 121, 253–258. doi: 10.1007/BF00346733
Riisgård H. U., Larsen P. S. (2005). Water Pumping and Analysis of Flow in Burrowing Zoobenthos: An Overview. Aquat. Ecol. 39, 237–258. doi: 10.1007/s10452-004-1916-x
Shi X., Li Y., Li D., Wang R., Deng M., Huang Y. (2015). Response of Sediment Micro Environment and Micro Interface to Physical Disturbance Intensity Under the Disturbance of Choronomus Plumosus. Environ. Sci. 36, 1622–1629. doi: 10.13227/j.hjkx.2015.05.015
Shimabukuro M., Bromberg S., Pires-Vanin A. M. S. (2016). Polychaete Distribution on the Southwestern Atlantic Continental Shelf. Mar. Biol. Res. 12 (3), 239–254. doi: 10.1080/17451000.2015.1131299
Sivadas S. K., Ingole B. S. (2016). Biodiversity and Biogeography Pattern of Benthic Communities in the Coastal Basins of India. Mar. Biol. Res. 12 (8), 797–816. doi: 10.1080/17451000.2016.1203949
Sun T., Liu C., Li X., An D., Yu H., Ma Z., et al. (2019). The Effect of Substrate Grain Size on Burrowing Ability and Distribution Characteristics of Perinereis Aibuhitensis. Acta Oceanol. Sin. 38, 52–58. doi: 10.1007/s13131-019-1348-z
Sun S., Zheng Z. (2010). Effect of Benthic Macro-Invertebrate Bioturbation on Sediment Environment: A Review. Acta Agric. Zhejiang. 22, 263–268. doi: 10.3724/SP.J.1231.2010.06781
Timmermann K., Banta G. T., Glud R. N. (2006). Linking Arenicola Marina Irrigation Behavior to Oxygen Transport and Dynamics in Sandy Sediments. J. Mar. Res. 64, 915–938. doi: 10.1357/002224006779698378
Tomassetti P., Porrello S. (2005). Polychaetes as Indicators of Marine Fish Farm Organic Enrichment. Aqua. Inter. 13 (1-2), 109–128. doi: 10.1007/s10499-004-9026-2
Tsujino M., Tamai K. (1996). Sediment Conditions and Meiobenthic Community in Osaka Bay, Japan. Bull. Nansei Regional Fisheries Res. Laboratory. 29, 87–100.
Turek K. A., Hoellein T. J. (2015). The Invasive Asian Clam (Corbicula Fluminea) Increases Sediment Denitrification and Ammonium Flux in 2 Streams in the Midwestern USA. Freshw. Sci. 34, 472–484. doi: 10.1086/680400
Van Hoey G. (2006). Spatio-Temporal Variability Within the Macrobenthic Abra Alba Community, With Emphasis on the Structuring Role of Lanice Conchilega. Belgium: PhD Thesis, Ghent University.
Volkenborn N., Hedtkamp S. I. C., van Beusekom J. E. E., Reise K. (2007b). Effects of Bioturbation and Bioirrigation by Lugworms (Arenicola Marina) on Physical and Chemical Sediment Properties and Implications for Intertidal Habitat Succession. Estuar. Coast. Shelf Sci. 74, 331–343. doi: 10.1016/j.ecss.2007.05.001
Volkenborn N., Meile C., Polerecky L., Pilditch C. A., Norkko A., Norkko J., et al. (2012a). Intermittent Bioirrigation and Oxygen Dynamics in Permeable Sediments: An Experimental and Modeling Study of Three Tellinid Bivalves. J. Mar. Res. 70, 794–823. doi: 10.1357/002224012806770955
Volkenborn N., Polerecky L., Hedtkamp S. I. C., van Beusekom J. E. E., de Beer D. (2007a). Bioturbation and Bioirrigation Extend the Open Exchange Regions in Permeable Sediments. Limnol. Oceanogr. 52, 1898–1909. doi: 10.4319/lo.2007.52.5.1898
Volkenborn N., Polerecky L., Wethey D. S., DeWitt T. H., Woodin S. A. (2012b). Hydraulic Activities by Ghost Shrimp Neotrypaea Californiensis Induce Oxic–Anoxic Oscillations in Sediments. Mar. Ecol. Prog. Ser. 455, 141–156. doi: 10.3354/meps09645
Wang H., Wang G., Fang J., Jiang Z., Du M., Gao Y., et al. (2017). Acute Sulphide Toxicity in Perinereis Aibuhitensis Under Different Salinities and Temperatures: LC50 and Antioxidant Responses. Aquat Biol. 26, 75–85. doi: 10.3354/ab00674
Wethey D. S., Woodin S. A. (2005). Infaunal Hydraulics Generate Porewater Pressure Signals. Biol. Bull. 209, 139–145. doi: 10.2307/3593131
Wethey D. S., Woodin S. A., Volkenborn N., Reise K. (2008). Porewater Advection by Hydraulic Activities of Lugworms, Arenicola Marina: A Field, Laboratory and Modeling Study. J. Mar. Res. 66, 255–273. doi: 10.1357/002224008785837121
Woodin S. A., Volkenborn N., Pilditch C. A., Lohrer A. M., Wethey D. S., Hewitt J. E., et al. (2016). Same Pattern, Different Mechanism: Locking Onto the Role of Key Species in Seafloor Ecosystem Process. Sci. Rep. 6, 26678. doi: 10.1038/srep26678
Yang L., Chen L., Li X., Zhou Z., Liu B., Song B., et al. (2019). Effect of Seasonal Hypoxia on Macrobenthic Communities in the Muping Marine Ranch, Yantai, China. Biod. Sci. 27, 200–210. doi: 10.17520/biods.2019012
Yazdani Foshtomi M., Leliaert F., Derycke S., Willems A., Vincx M., Vanaverbeke J. (2018). The Effect of Bio-Irrigation by the Polychaete Lanice Conchilega on Active Denitrifiers: Distribution, Diversity and Composition of nosZ Gene. PloS One 13, e192391. doi: 10.1371/journal.pone.0192391
Zhang A., Jiang H., Gu Z., Chen P. (2018). Composition, Distribution and Seasonal Variation of Surface Seawater Nutrients in Jiangsu Coastal Waters. Trans. Ocean. Lim. 2, 49–59. doi: 10.13984/j.cnki.cn37-1141.2018.02.007
Zhang L., Liao Q., He W., Shang J., Fan C. (2013). The Effects of Temperature on Oxygen Uptake and Nutrient Flux in Sediment Inhabited by Molluscs. J. Limnol. 72, e2. doi: 10.4081/jlimnol.2013.e2
Zhou X. (2005). The Effects of Environmental Stress on Behavior and Physiological Activity of Litopenaeus Vannamei (Guangzhou: Jinan University). Doctoral Dissertation.
Keywords: bioirrigation, bioturbation, polychaete behavior, burrow, micro-environment
Citation: Fang J, Meng S, Lux RHE, Jiang W, Jiang Z, Mao Y, Jansen H, Fang J and Strand Ø (2022) Why and How is Burrow Ventilation Initiated? A Case Study of Polychaete Behavior in the Burrow at Different Temperatures. Front. Mar. Sci. 9:910781. doi: 10.3389/fmars.2022.910781
Received: 01 April 2022; Accepted: 21 June 2022;
Published: 11 July 2022.
Edited by:
Menghong Hu, Shanghai Ocean University, ChinaReviewed by:
Hao Zhenlin, Dalian Ocean University, ChinaWei Li, Institute of Hydrobiology (CAS), China
Copyright © 2022 Fang, Meng, Lux, Jiang, Jiang, Mao, Jansen, Fang and Strand. This is an open-access article distributed under the terms of the Creative Commons Attribution License (CC BY). The use, distribution or reproduction in other forums is permitted, provided the original author(s) and the copyright owner(s) are credited and that the original publication in this journal is cited, in accordance with accepted academic practice. No use, distribution or reproduction is permitted which does not comply with these terms.
*Correspondence: Jinghui Fang, aHVpODYxQDE2My5jb20=
†The first two authors equally contributed to the work