- 1Centre for Sustainable Tropical Fisheries and Aquaculture, College of Science and Engineering, James Cook University, Townsville, QLD, Australia
- 2EcoDNA Group, Centre for Conservation Ecology and Genomics, University of Canberra, Canberra, ACT, Australia
- 3Aquatic Pest and Health Policy Section, Australian Government Department of Agriculture, Water and the Environment, Canberra, ACT, Australia
- 4Biodiversity & Geosciences Program, Queensland Museum, Brisbane, QLD, Australia
- 5The University of Sydney, School of Life and Environmental Sciences, Camden, NSW, Australia
- 6Aquaculture Group-Aquatic Animal Health Programme, Cawthron Institute, Nelson, New Zealand
The ornamental fish trade provides a pathway for the global translocation of aquatic parasites. Myxozoa is comprised of highly specialized metazoan parasites of aquatic hosts with a wide host range. Interest in the group has intensified along with the development of aquaculture due to emergent pathogenic myxozoan species in both freshwater and marine environments. However, little is known on myxozoan diversity in the ornamental fish trade. We examined 630 ornamental fish imported from Asia to Australia (representing 24 fish populations, including freshwater and wild caught marine fish species) for myxozoan parasites during 2015. Fish were sampled under Australian quarantine following veterinary certification that they showed no clinical signs of pests and diseases from the exporting country and visual inspection at Australian border control. Myxozoan parasites infected 8 of 12 freshwater populations and 8 of 12 marine fish populations. A total of 12 morphologically distinct Myxobolus spores were detected amongst all goldfish, Carassius auratus populations. Myxidium spores were detected in kissing gourami, Helostoma temminckii, and Ceratomyxa sp. spores were detected in cardinal fishes, Cheilodipterus quinquelineatus, Pterapogon kauderni, and Zoramia leptocantha. Kudoa sp. spores were detected in C. quinquelineatus, Sphaeramia nematoptera and Z. leptocantha. Results of this study show that Australian pre-export health requirements and visual inspections do not reliably detect myxozoan infections. Inspection prior to exportation and at border control should account for the highly cryptic nature of myxozoan parasites and consider alternative detection methods to complement inspections at border control.
Introduction
The live ornamental fish trade is a known pathway for the introduction of invasive fish, parasites, and pathogens, globally (Whittington and Chong, 2007; Lymbery et al., 2014; Becker et al., 2014; Rimmer et al., 2015; Trujillo-González et al., 2018a). Globalization, advances in technology and transport capability have facilitated the translocation of fish from remote locations and increased the number and volume of species traded. Trade of live animals at this scale presents the potential for introduction of invasive fish species and their associated parasites to endemic ecosystems (Lymbery et al., 2014; Sheath et al., 2015; Trujillo-González et al., 2018a), with important impacts on endemic wildlife, farmed fish species and natural resources (Knight, 2010; Lymbery et al., 2014; Gallardo et al., 2016). For this reason, governments may establish biosecurity protocols to detect and prevent the translocation of hazardous parasites and pathogens though the live ornamental trade (Whittington and Chong, 2007; Rimmer et al., 2015; Trujillo-González et al., 2018b).
Australia has stringent import conditions to manage risks associated with the importation of ornamental fish species. For instance, marine ornamental fish species must be sourced from wild populations 5 km away from any finfish aquaculture operation not associated with significant infectious disease or pest outbreaks during the six months prior to collection, and have not come into contact with water, equipment or fish associated with farmed food fish (DAWE, 2022). Freshwater ornamental fish species must have resided in approved export premises 14 days prior to export in systems separate from farmed food fish or koi carp and must have been inspected within seven days prior to export and show no clinical signs of infectious disease or pests (DAWE, 2022). There are additional health requirements for goldfish, Carassius auratus (Linnaeus, 1758), gouramis and paradise fish (Osphronemidae), Siamese fighting fish (Osphronemidae), cichlids, and poeciliids (DAWE, 2022). All ornamental fish species are inspected by the Department of Agriculture, Water and the Environment (DAWE) on arrival to Australia to ensure that fish are healthy, that the health certification is valid, that they are an approved species, and that they do not contain non-permitted material or material of biosecurity concern (DAWE, 2022). All ornamental species are then directed for isolation by the department at an ornamental fish Approved Arrangement site on arrival, where marine and freshwater fish are isolated for seven days except for goldfish, which must be isolated for 21 days (DAWE, 2022). Nonetheless, parasites and pathogens can be challenging to detect by visual inspections, microscopic life stages may be present in the transport water, and previous research has shown that ectoparasitic monogenean parasite species infecting imported ornamental fishes have gone undetected during pre-export quarantine periods and border control inspections in Australia (Trujillo-González et al., 2018).
Myxozoans comprise a species-rich clade of endoparasitic cnidarians with complex life cycles, exploiting invertebrate and vertebrate hosts in marine, freshwater, and terrestrial environments (Okamura et al., 2018). Available research on myxozoans has traditionally explored species of importance to aquaculture (Sayyaf Dezfuli et al., 2021; Born-Torrijos et al., 2022), with examples such as Ceratonova shasta (Noble, 1950) (Hallett et al., 2012), Enteromyxum leei (Diamant, Lom & Dyková, 1994) (Sekiya et al., 2016), multiple Kudoa species (Moran et al., 1999; Kristmundsson and Freeman, 2014; Marshall et al., 2016) and Myxobolus cerebralis Hofer, 1903 (Fetherman et al., 2011) known to cause severe mortalities and economic losses in food fish aquaculture. Indeed, whirling disease caused by infection with Myxobolus cerebralis, is a reportable aquatic disease in Australia (DAWE, 2021) and some Myxobolus spp. form plasmodia on the body surface of fish hosts and can cause severe disfigurement in farmed ornamental goldfish (Caffara et al., 2009; Zhang et al., 2018). Although over 2,500 myxosporean species have been described and known to infect freshwater and marine hosts (Okamura et al., 2018), the species richness and regularity with which myxozoan infections occur in imported fish in the ornamental fish trade remain largely unexplored, with few studies reporting myxozoan infections in imported freshwater (Caffara et al., 2009; Baska et al., 2009; Trujillo-González et al., 2018a) and marine (Sanil et al., 2016; Surendran et al., 2021) ornamental species. As such, the relative risk of myxozoan parasites being co-introduced through the ornamental trade remains poorly assessed.
The aim of this study was to document myxozoan parasite diversity infecting marine and freshwater ornamental fish species imported to Australia. Fish were imported from Asia and examined for myxozoan parasites under quarantine conditions using a combined morphological and molecular approach to determine the identity of myxozoan parasites undetected following inspection at border control.
Materials and Methods
Fish Importation and Collection
A repeated cross-sectional survey was conducted to examine imported ornamental fish under quarantine for the presence of nationally listed aquatic pathogens that are associated with at least one ornamental fish host imported to Australia. All fishes collected were considered pre-import and under quarantine at the time of testing. A total of 37 fish populations representing 11 species of freshwater fishes and seven species of marine fishes from 11 consignments were commissioned from Sri Lanka, Singapore, Indonesia, and Thailand for examination of parasite fauna (Supplementary S1, Table S1). A consignment was defined as all the ornamental fishes received from an international exporter on a specific day. A population was defined as a single fish species received from an international exporter on a specific day. Fish species were prioritized based on prior knowledge of potential for infection with nationally listed pathogens in consultation with the Australian Government Department of Agriculture, Water and the Environment (DAWE).
On arrival to Australia, all fish were subjected to quarantine practices, which involved clearance from Australian customs, followed by visual inspection and approval by Australian Quarantine Services from DAWE. Following release by Quarantine Services, fish were transported by road to an Approved Arrangement site at the Sydney School of Veterinary Sciences, The University of Sydney, Camden, Australia, for necropsy. It is important to note that all fish populations were still under quarantine authority, as Australian import conditions require all fish released by Quarantine Services to undergo a final quarantine period for 21 days in the case of goldfish, and seven days for all other fish in an Approved Arrangement site (DAWE, 2022). Thirty fish were randomly selected for sampling from each population to enable 95% confidence of detecting parasite prevalence ≥ 10%, assuming 100% sampling sensitivity (Post and Millest, 1991). Apparent prevalence was presented with 95% confidence intervals using the exact binomial approximation. When there were no observed parasites, the proportion was calculated with a one-sided upper 97.5% confidence limit. Fish were euthanized inside the original transport container used by the exporters for delivery (i.e., 20 L plastic bag for freshwater fish populations and individual 5 L plastic bags for marine fish) using benzocaine (100 mg/L) within 12 hours of receipt from Australian Quarantine Services, as per import conditions. To maximize the diversity of fish species available for examination within the 12-hour time limit, three large sampling events were completed in January, May, and October of 2015. Animal ethics approval was obtained from The University of Sydney Animal Ethics Committee (approval number: 720).
Tissue Sampling and Necropsy
Imported fish species were examined for protozoan and metazoan parasites via external macroscopic examination and necropsies of internal organs using wet mount microscopy preparations. Immediately following euthanasia, individual fish were placed in a disposable Petri dish to be photographed, weighed, and measured. Then, tissue samples from each fish were sequentially dissected, placed on glass slides under a large coverslip (with either saltwater or freshwater according to the origin of the fish, or saline solution for internal organs) and examined for the presence of parasites using a compound microscope (Olympus BX41). First, skin scrapes were collected from the left dorsal area of the fish. Then the gill basket was carefully removed and placed in a cavity block with either saltwater or freshwater according to the origin of the fish, and each gill arch carefully dissected. Tissue samples were collected in order from the brain, muscle tissue in the left dorsal muscle area, liver, spleen, and kidney. The gall bladder was excised onto a clean glass slide, immersed in saline solution under a large coverslip and carefully depressed, allowing bile to fill the glass slide. Lastly, the digestive tract was separated between the stomach and intestines, chopped with a scalpel blade, and immersed in saline on a glass slide under a coverslip, followed separately by the heart in the same manner. If microspores or plasmodia were detected, microphotographs were taken using an Olympus UC50 digital camera and NISElements Basic Research 3.0 software (Nikon Corporation, Japan). Samples from each organ were placed in individual Eppendorf tubes with 70% ethanol, labelled and stored for DNA extraction. Glass slides were left to air dry. Once dried, slides were held in slide holders with 70% ethanol for further examination.
All marine fish were examined fresh in this survey. Due to time constraints associated with the 12 h time limit of DAWE to euthanise fish, some freshwater fish were not able to be examined fresh following euthanasia. Fish not examined fresh were photographed, measured and weighed, and underwent external examinations. Subsequently, they were preserved whole with a ventral incision from the isthmus to the anus to permit more rapid fixation in 70% ethanol. These fish were shipped to the Marine Parasitology Laboratory (James Cook University, Australia), and dissected following the methods described above. Parasites were detected using a compound microscope (Olympus BX53) fitted with direct interference contrast, and a dissecting microscope (Leica M60). Microphotographs were taken using an Olympus UC50 mounted camera and Labsense image analysis software (Olympus v. 1).
DNA Extraction, PCR Amplification and Sequencing
DNA was extracted from myxozoan spores isolated from samples stored in 70% ethanol using a Qiagen DNeasy blood and tissue kit (QIAGEN Pty Ltd, Australia) according to the manufacturer’s instructions. Ribosomal DNA for myxozoan parasites was amplified using several primer combinations (Table 1). Polymerase Chain Reaction (PCR) assays (25 μL) contained 2 μL DNA, 1 μL of each corresponding primer combination (10 nM), 12.5 μL Qiagen Hotstart Taq Master Mix (Qiagen, Australia), 4.5 μL MilliQ® water, and 2 μL of Dimethyl Sulfoxide (DMSO, Sigma-Aldrich, Australia). Reactions were performed in a Veriti® Thermal Cycler (Sigma-Aldrich, Australia) under the following cycling conditions: initial denaturation at 95°C for 15 min, followed by 40 cycles of 95°C denaturation for 15 sec then primer-specific annealing temperature for 40 sec (Table 1), 65°C extension for 30 sec, and a final extension at 65°C for 5 min. Amplicons were visualised on agarose gels and selected amplicons were Sanger sequenced by the Australian Genome Sequencing Facility (Brisbane, Australia). Sequence identity was confirmed by BLAST in Geneious (v10.0.9).
Data Analysis
Measurements of myxozoan parasites were made from photomicrographs using the image analysis package Fiji from Image J (Schindelin et al., 2012). Measurements were only collected from spores positioned ventrally, with the aim of obtaining a minimum of 20 spores from each putative myxozoan species from each host/parasite combination. Measurements for Myxobolus spore ventral length and width were collected from each spore, as well as the length, width and number of ridges of each polar capsule within each spore, following protocols by Lom and Arthur (1989) and Burger and Adlard (2011). Measurements for Ceratomyxa spore length and thickness, and polar capsule length and width were collected following protocols by Heiniger et al. (2007). Principal component analysis was used to create Euclidean plots and differentiate myxozoan spores using S-PLUS (v 8.0). Factors were rotated to improve data interpretation when principal factors were equally loaded (Supplementary S1, Figure S1).
Results
Apparent Prevalence of Myxozoan Infections
Populations 1, 21 and 25 were seized at border control because of irregularities in their documentation and were excluded from the survey (Supplementary S1, Table S1). Fish from populations 12-20 were degraded despite ample fixation and deemed inadequate for recovery of optimal myxozoan parasites. Therefore, these populations were not examined in this study (Supplementary S1, Table S1).
Myxozoan parasites were detected in 66% (8 of 12) of freshwater fish populations (Table 2). All C. auratus populations were infected with Myxobolus sp. with apparent prevalence (95% CI) ranging from 16.7% (5.6-34.7) (Population 7) to 53.3% (34.3-71.7) (Population 5). Three Helostoma temminckii Cuvier, 1829, specimens imported from Singapore showed Myxidium sp. infections with an apparent prevalence = 10% (2.1–26.5). Parasites were not detected (apparent prevalence 0% (0-11.4)) in Beta splendens Regan, 1910, imported from Malaysia, Danio rerio (Hamilton, 1822) imported from Sri Lanka, Xiphophorus helleri Heckel, 1848, from Sri Lanka and Xiphophorus maculatus (Günther, 1866) imported from Thailand and Singapore (Table 2).
Similarly, 66% (8 of 12) of marine fish populations were infected with myxozoan parasites. All Pterapogon kauderni Koumans, 1933, populations imported from Singapore and Indonesia were infected with Ceratomyxa sp. with apparent prevalence ranging 50% (31.3–68.7) (Population 32) to 60% (40.6–77.3; Populations 33-34; Table 2). In the same way, Kudoa sp. was found infecting Cheilodipterus quinquelineatus Cuvier, 1828, Sphaeramia nematoptera (Bleeker, 1856) and Zoramia leptocantha Bleeker, 1856 imported from Indonesia with apparent prevalence ranging from 13.3% (3.8–30.7) (Population 37) to 40% (22.7–59.4l; Population 31; Table 2). Cheilodipterus quinquelineatus imported from Indonesia was also infected Ceratomyxa sp. (10 (2.1–26.5), while Zoramia leptocantha Bleeker, 1856, also imported from Indonesia, showed Myxidium sp. infections (10% (2.1–26.5); Table 2). Parasites were not detected (0% (0–11.4)) in any of the Amphiprion species imported for examination (populations 26 to 29; Table 2). Staining was inadequate for morphological diagnosis of Kudoa spores and no reliable measurements could be taken for comparison.
Morphometric Analysis
Twelve distinct Myxobolus spores were observed infecting imported goldfish. The principal component analysis (PCA) supported the morphological separation of Myxobolus spores infecting goldfish (Figure 1). Spore length contributed the most to differences in the morphometric analysis (Figure 1). Myxobolus spores 1, 2, 7, 9, 10, 11 and 12 were distinct in spore length and width while Myxobolus spores 3, 4 and 5, and 6 and 8 grouped within two similar clusters, respectively (Figure 1). All 12 Myxobolus spores displayed similar shape and capsule morphology consistent with 11 Myxobolus species reported for C. auratus in Asia (Table 3). Within these species, Myxobolus diversus Nie and Li, 1973, Myxobolus turpisrotundus Zhang, Wang, Gong 2010, and Myxobolus lentisuturalis Dyková, Fiala and Nie, 2002, have been reported infecting farmed ornamental C. auratus (Trujillo-González et al., 2018a) and have consistent measurements and myxosporean morphology as the spores reported in this study.
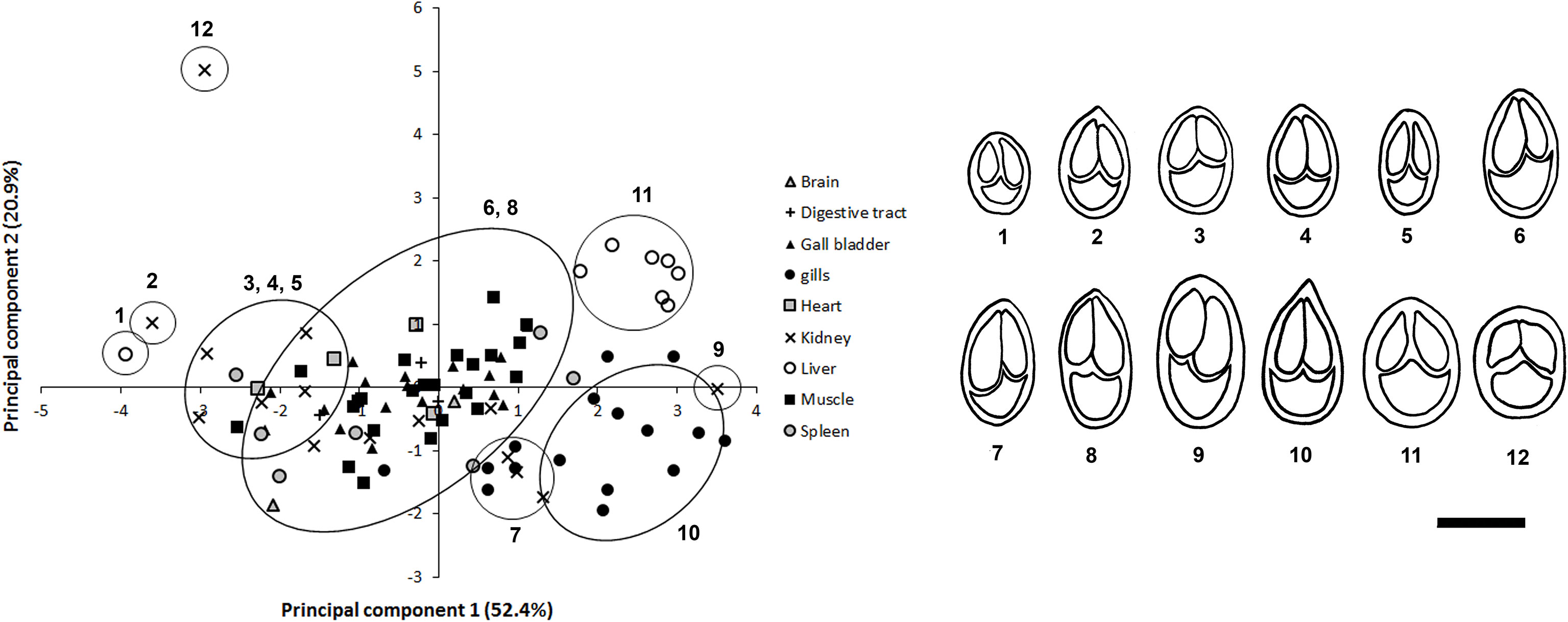
Figure 1 Principal Component analysis (PCA) of mature Myxobolus spores found in tissue samples from goldfish. Drawings for 12 morphologically distinct spores are provided in congruence with the PCA analysis. Principal Component 1 (spore length) explained 52.4% of variation, while Principal Component 2 (spore width) explained 20.9% of variation. Scale bar = 10 µm.
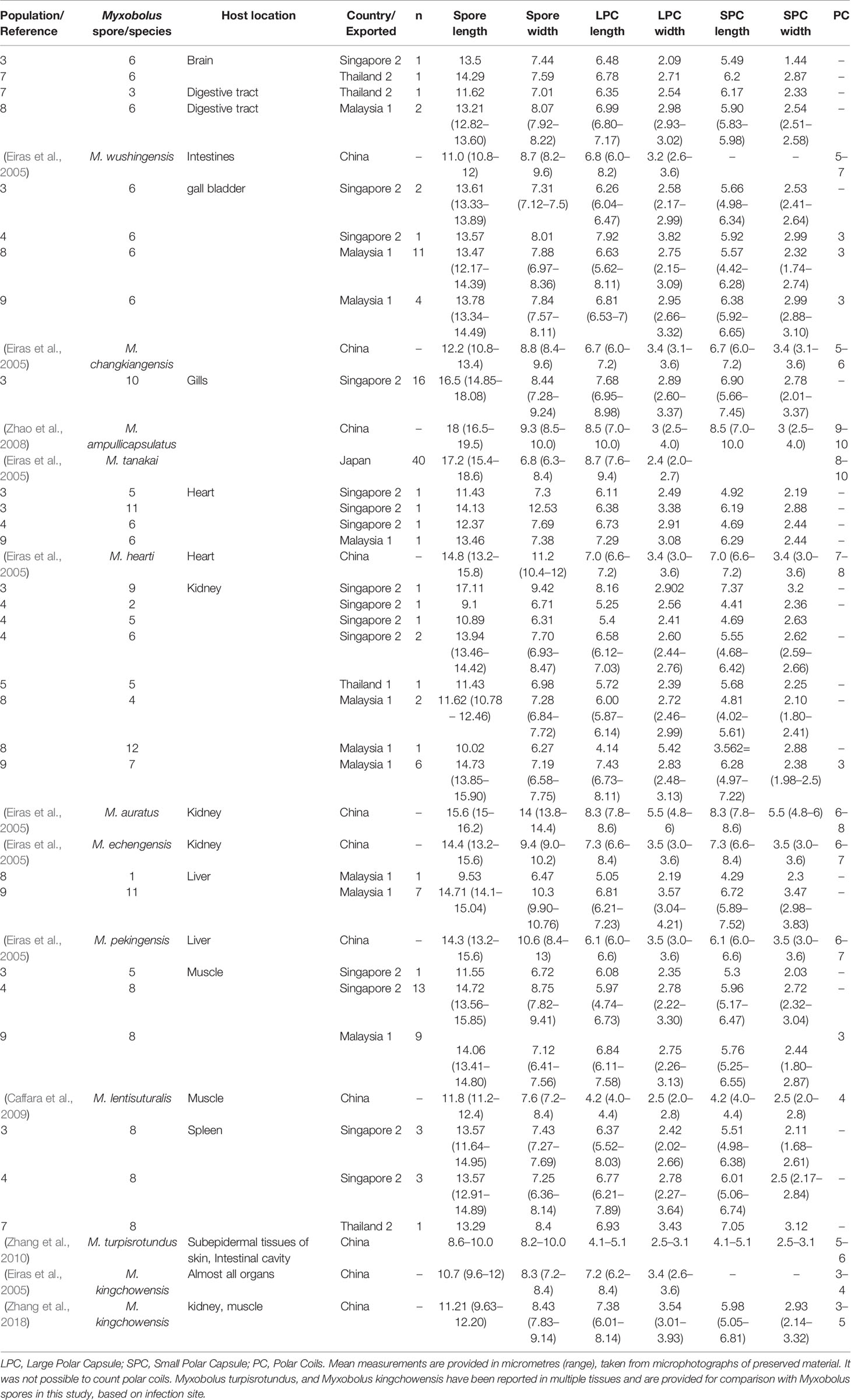
Table 3 Morphometric comparison of Myxobolus spores found infecting imported Carassius auratus populations and similar Myxobolus species.
Similarly, four apparently morphologically distinct Ceratomyxa spore were detected. Although the PCA for Ceratomyxa spores indicated that spore length contributed the most to differences in the morphometric analysis (Figure 2), it is important to consider that all samples for marine fishes were examined using fresh mounts, and spore length may have been affected by positioning of Ceratomyxa polar extensions (Figure 2). Ceratomyxa spores 1, 3 and 4 were detected infecting the gall bladder of P. kauderni imported from Singapore (Population 32, Figure 2 and Table 2), while spore 2 was detected infecting C. quinquelineatus (Population 31), and P. kauderni (Populations 32 and 34) (Figure 2 and Table 2). Ceratomyxa species infecting wild caught C. quinquelineatus and P. kauderni, had spore measurements and morphology consistent with previous records of Ceratomyxa cardinalis Heiniger & Adlard, 2013, Ceratomyxa talboti Gunter & Adlard, 2008, and Ceratomyxa ireneae Heiniger & Adlard, 2013 (Table 4).
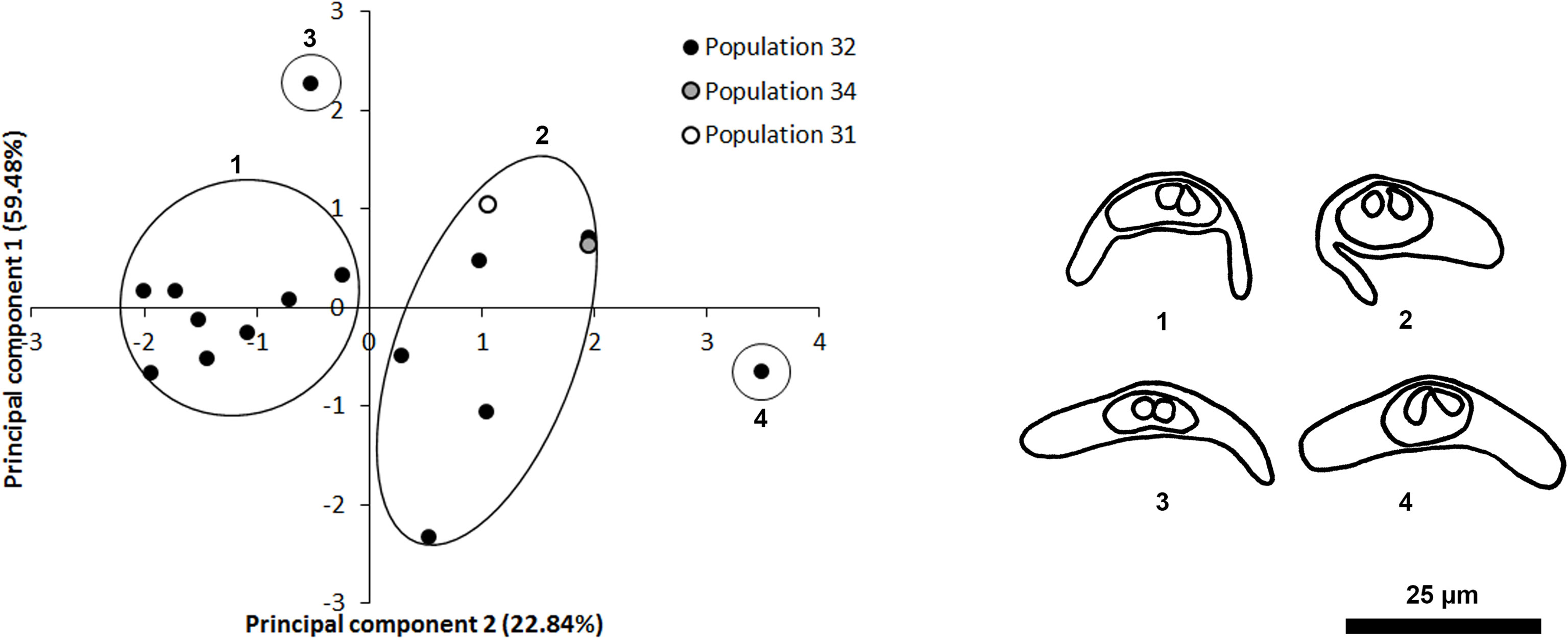
Figure 2 Principal Component Analysis (PCA) of Ceratomyxa spores found in marine fish populations. Drawings for four morphologically distinct Cetatomyxa spores are provided in congruence with the PCA analysis. Principal Component 1 (spore length) explained 59.48% of variation, while Principal Component 2 (polar capsule width) explained 22.84% of variation. Scale bar = 25 µm.
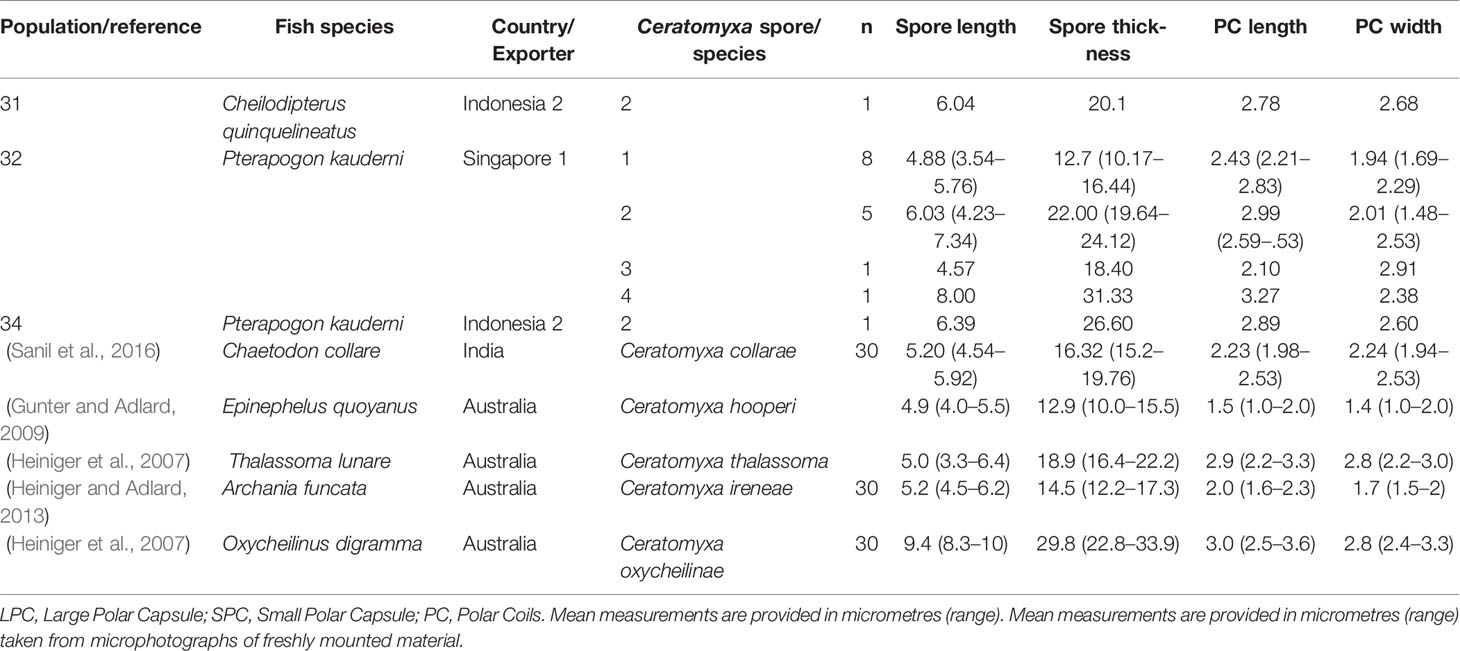
Table 4 Measurements from Ceratomyxa spores found infecting the gall bladder of marine fish imported to Australia and comparison to similar Ceratomyxa species.
Molecular Confirmation
A total of four separate 1225 bp fragments from the 18s gene region were generated from Myxobolus isolates infecting goldfish Populations 7-9. All fragments exhibited 98.3–99% similarity to Myxobolus kingchowensis Chen & Ma, 1998 (NCBI Accession KP400625). No sequences were recovered for any Myxidium species infecting H. temminckii.
A total of two 680 bp fragments in the 28s gene region and one 835 bp fragment from the 18s region were amplified from Kudoa isolates infecting C. quinquelineatus and Z. leptocantha (populations 31 and 36, respectively) using primer pair 5 for 28s and primer pair 6 for 18s (Table 1). Kudoa sp. fragments for the 28s and 18s gene regions showed 99% similarity to Kudoa cheilodipteri Heiniger, Cribb & Adlard, 2013, collected from C. quinquelineatus in Australia (NCBI Accession JX090299 AND JX090295 for 28s and 18s respectively). No sequences were recovered for any Ceratomyxa reported in this study and all curated sequences were accessioned in the National Centre for Biotechnology information (accession no. ON125902 and ON125905-ON125908).
Discussion
The endoparasitic nature of myxozoan parasites makes detection by visual inspection an almost impossible task unless symptoms are obvious. We detected myxozoan infections in 8 of 12 freshwater and 8 of 12 marine fish populations imported to Australia following veterinary certification from the exporting countries and inspection at the Australian border control. Myxobolus spores were detected infecting all C. auratus populations, within which M. kingchowensis was confirmed infecting in 3/7 C. auratus populations imported from Malaysia and Thailand. Myxidium spores were detected infecting H. temminckii imported from Singapore, while Ceratomyxa spores were detected in one C. quinquelineatus population imported from Indonesia and in all P. kauderni populations imported from Singapore, which also displayed high apparent prevalence of Ceratomyxa infections compared to all other infected marine populations. Kudoa cheilodipteri was confirmed infecting C. quinquelineatus and Z. leptocantha imported from Indonesia while unidentified Kudoa spores were detected in wild caught C. quinquelineatus S. nematoptera and Z. leptocantha imported from Indonesia. Detection of myxozoan infections in such a high number of fish populations highlights how these parasites can go undetected at border control in the absence of suitable health requirements for ornamental fish species imported to Australia (DAWE, 2022).
Detection of myxozoan infections in this study were mostly of mature spores present in internal organs with no instances of obvious external hyperplasia or superficial plasmodia. Myxozoan infections are considered emerging threats to ornamental aquaculture development associated with significant fish mortalities and economic losses in food fish aquaculture (Saha and Bandyopadhyay, 2017). Such association is commonly linked to intensive aquaculture conditions where tissue hyperplasia, macroscopic cysts and erosive necrotic lesions on the host fish associated to severe myxozoan infections are conspicuous (Kent et al., 2001; Morsy et al., 2012; Shinn et al., 2015; Saha and Bandyopadhyay, 2017). Considering the large diversity of myxozoan parasites reported in this study and the lack of obvious visual signs of infection, it remains unclear to what extent myxozoan infections are present in the ornamental trade. Multiple co-introduced and co-invasive myxozoan parasites have been reported globally associated with the goldfish trade (Trujillo-González et al., 2018a) and research has suggested marine host species could be important myxozoan reservoirs of importance to the development of aquaculture, both of food fish and ornamental species (Heiniger et al., 2007; Burger et al., 2008). As such, countries with developing ornamental industries and trade should consider assessing the parasite fauna of commercially important ornamental fish species within their jurisdictions, as this study showed that a high diversity of myxozoan parasites with an unassessed biosecurity risk to the Australian ornamental trade, were not detected at border control.
It is unclear to what extent myxozoan parasites reported in this study pose a risk to the ornamental trade and biosecurity. Despite their importance as disease-causing parasites in aquaculture and wild fish stock populations (Feist and Longshaw, 2006), the diversity and impact of myxozoan species of importance to the ornamental trade remains poorly understood. Research on myxozoans infecting commercially important species is available for some marine species (e.g. Kudoa nerophila (Cobcroft and Battaglene, 2013)), while several studies have explored the associated diversity of myxozoan parasites in wild marine species (e.g. Auerbachia, Ceratomyxa, Coccomyxa, and Kudoa (Whipps et al., 2003a; Whipps et al., 2003b; Whipps et al., 2004; Heiniger et al., 2007; Burger et al., 2008; Gunter and Adlard, 2009; Burger and Adlard, 2011; Heiniger et al., 2011; Heiniger and Adlard, 2013)), of which K. cheilodipteri, which was found infecting imported C. quinquelineatus and Z. leptocantha, has been reported infecting C. quinquelineatus in Australia (Heiniger and Adlard, 2013). Moreover, research on endemic and invasive myxozoan species infecting wild freshwater or marine species has far mostly targeted disease-causing Myxozoa (Moran et al., 1999; Hallett et al., 2012; Sekiya et al., 2016), or mechanisms used by myxozoan species to infect and thrive in their hosts (Sayyaf Dezfuli et al., 2021). As such, available risk assessments for myxozoans have been discussed and implemented within the context of risks associated to the importation of food fish, emerging species affecting farmed and wild food fish, and pathogen transmission from aquaculture to wild fish (Bartholomew and Kerans, 2015). Records in this study provide valuable baseline information for future assessments to determine the potential risks of myxozoan parasites co-imported via the ornamental trade, highlighting the need to better understand endemic and co-invasive species infecting fishes in the ornamental trade.
Similarly, management strategies for myxozoan infections are poorly studied beyond the scope of aquaculture and focus on avoidance through biosecurity measures. Infection intensities of the myxosporean stage of Kudoa neurophila (Grossel, 2003) in hatchery reared Latris lineata (Bloch & Schneider 1801) were reduced to 0% when treating source water with dose-controlled ultraviolet irradiation ≥ 44 mJ cm-2 UV (Cobcroft and Battaglene, 2013). Ozonating source water with > 700 mV Oxidation-Reduction Potential for 10 min prevented K. neurophila infections (Cobcroft and Battaglene, 2013). Sand and cartridge filtration of seawater (filtration< 5 μm), followed by ultraviolet (UV) irradiation at a dose of 46 mJ/cm2 was shown to prevent Kudoa septempunctata Matsukane, Sato, Tanaka, Kamata, and Sugita-Konishi, 2010, from infecting farmed Paralichthys olivaceus (Temminck & Schlegel, 1846) (Nishioka et al., 2016). Similarly, prior exposure to myxozoan infections in farmed fish could provide protective immunity and prevent reinfection (e.g. Kudoa thyrsites infecting Salmo salar (Braden et al., 2018)) and provide complementary management strategies to minimise the impact of myxozoan infections. Pre and post border biosecurity regulations as well as health certificate requirements could be implemented to minimize the risk of parasitic diseases. Such requirements could include the treatment and handling of fish during quarantine periods using suitable and approved methods, followed by health assessments by a recognised entity by the importing country. Future risk analyses should assess treatment conditions of water sources by exporting companies and consider the inclusion of source water treatment or rearing requirements prior to export overseas.
Species delineation of myxozoans is challenging through morphological features alone because of variation in measurements following preparation, few distinct morphological features and phenotypic plasticity. Similarities in spore measurements, size and morphology prevented delineation of Ceratomyxa and Myxobolus types to species level. Diagnostic features for Myxozoa have been recently questioned given the possibility of intra-specific phenotypic plasticity and the lack of genetic sequences available for comparison (Smothers et al., 1994; Sanil et al., 2016; Zhang et al., 2018). As such, studies have questioned the validity of many myxozoan species because of their incomplete and purely morphological descriptions, insufficient comparison with other known species, and recent revision of taxonomic criteria for myxozoans (Zhang et al., 2010). Although we identified Myxobolus kingchowensis infecting farmed C. auratus and Kudoa cheilodipteri infecting wild caught C. quinquelineatus and Z. leptocantha using both morphological and molecular approaches, our molecular approach (i.e., Sanger sequencing) failed to provide suitable molecular data to definitively identify myxozoan fauna as the targeted sequencing approach was affected by the wide array myxozoan species co-infecting sampled fish populations in this study (Zhang et al., 2010; Zhang et al., 2018). Future studies could aim to isolate myxozoan proliferative stages for accurate molecular identification (Born-Torrijos et al., 2022), or explore the use of high throughout sequencing methods to comprehensively explore myxozoan diversity (Alama-Bermejo and Holzer, 2021).
In conclusion, this study showed that despite stringent pre-export quarantine and border control requirements, a wide diversity of myxozoan parasites were undetected by visual inspections at border control. Here, we provide a baseline assessment to consider what myxozoan diversity can go undetected at border control and highlight that the commonly asymptomatic condition infections. Biosecurity measures should consider complementary detection methods and analyse the risks of exotic myxozoan parasites imported via the ornamental fish trade. For example, previous research has shown that the use of environmental DNA-based non-invasive sampling methods, coupled with high throughput sequencing was able to detect 107 distinct myxozoan Operational Taxonomic Units infecting otters, domestic and wild geese, and four species of wild birds by targeting water samples and faecal matter (Hartikainen et al., 2016). eDNA techniques have also been previously demonstrated to accurately detect and discriminate five different Dactylogyrus parasites (Trujillo-González et al., 2019) and could complement visual inspections in Australian biosecurity.
Data Availability Statement
The original contributions presented in the study are included in the article/Supplementary Materials. Further inquiries can be directed to the corresponding author.
Author Contributions
Conceptualization, KH, JB; methodology, KH, JB, ATG, TM; software, ATG; formal analysis, ATG, JA; investigation, ATG, JA; resources, KH, JB; data curation, ATG; writing–original draft preparation, ATG; writing–review and editing, all authors; visualization, ATG; project administration, JB; funding acquisition, KH, JB. All authors contributed to the article and approved the submitted version.
Funding
This study was funded by the Australian Government through the Fisheries Research and Development Corporation (FRDC) (Project No. 2014/001), The University of Sydney, James Cook University, and the New Zealand Ministry of Business, Innovation and Employment, under the programme Aquaculture Health to Maximise Productivity and Security (CAWX1707). Additional molecular analyses and publishing fees were funded by The University of Canberra.
Conflict of Interest
The authors declare no conflict of interest. The authors declare that the research was conducted in the absence of any commercial or financial relationships that could be construed as a potential conflict of interest.
Publisher’s Note
All claims expressed in this article are solely those of the authors and do not necessarily represent those of their affiliated organizations, or those of the publisher, the editors and the reviewers. Any product that may be evaluated in this article, or claim that may be made by its manufacturer, is not guaranteed or endorsed by the publisher.
Acknowledgments
We thank Dr Paul Hick and Mrs. Alison Tweedie for their assistance during sampling events.
Supplementary Material
The Supplementary Material for this article can be found online at: https://www.frontiersin.org/articles/10.3389/fmars.2022.910634/full#supplementary-material
Supplementary S1 | Complete list of surveyed fish populations, Morphometric data for Myxobolus and Ceratomyxa species, representative microphotographs of myxozoa parasites and PCA component weighting.
References
Alama-Bermejo G., Holzer A. S. (2021). Advances and Discoveries in Myxozoan Genomics. Trends Parasitol. 37 (6), 552–568. doi: 10.1016/j.pt.2021.01.010
Bartholomew J. L., Kerans B. (2015). “Risk Assessments and Approaches for Evaluating Myxozoan Disease Impacts,” in Myxozoan Evolution, Ecology and Development. Eds. Okamura B., Gruhl A., Bartholomew J. (Cham: Springer). doi: 10.1007/978-3-319-14753-6_20
Baska F., Voronin V. N., Eszterbauer E., Müller L., Marton S., Molnár K. (2009). Occurrence of Two Myxosporean Species, Myxobolus hakyi sp. n. and Hoferellus pulvinatus sp. n. in Pangasianodon hypophthalmus Fry Imported From Thailand to Europe as Ornamental Fish. Parasitol. Res. (1987) 105 (5), 1391–1398. doi: 10.1007/s00436-009-1567-x
Becker J. A., Tweedie A., Rimmer A., Landos M., Lintermans M., Whittington R. J. (2014). Incursions of Cyprinid Herpesvirus 2 in Goldfish Populations in Australia Despite Quarantine Practices. Aquaculture 432, 53–59. doi: 10.1016/j.aquaculture.2014.04.020
Born-Torrijos A., Kosakyan A., Patra S., Pimentel-Santos J., Panicucci B., Chan J. T. H., et al. (2022). Method for Isolation of Myxozoan Proliferative Stages From Fish at High Yield and Purity: An Essential Prerequisite for In Vitro, In Vivo and Genomics-Based Research Developments. Cells (Basel Switzerland) 11 (3), 377. doi: 10.3390/cells11030377
Braden L. M., Rasmussen K. J., Purcell S. L., Ellis L., Mahony A., Cho S., et al. (2018). Acquired Protective Immunity in Atlantic Salmon Salmo salar Against the Myxozoan Kudoa thyrsites Involves Induction of Mhiiβ + CD83 + Antigen-Presenting Cells. Infect Immun. 2018, 86(1). doi: 10.1128/IAI.00556-17
Burger M. A. A., Adlard R. D. (2011). Low Host Specificity in the Kudoidae (Myxosporea: Multivalvulida) Including Seventeen New Host Records for Kudoa thalassomi. Folia Parasitol. 58 (1), 1–16. doi: 10.14411/fp.2011.001
Burger M. A. A., Barnes A. C., Adlard R. D. (2008). Wildlife as Reservoirs for Parasites Infecting Commercial Species: Host Specificity and a Redescription of Kudoa amamiensis From Teleost Fish in Australia. J. Fish Dis. 31 (11), 835–844. doi: 10.1111/j.1365-2761.2008.00958.x
Caffara M., Raimondi E., Florio D., Marcer F., Quaglio F., Fioravanti M. L., et al. (2009). The Life Cycle of Myxobolus lentisuturalis (Myxozoa: Myxobolidae), From Goldfish (Carassius auratus auratus), Involves a Raabeia-Type Actinospore. Folia Parasitol. 56 (1), 6–12. doi: 10.14411/fp.2009.002
Cobcroft J. M., Battaglene S. C. (2013). Ultraviolet Irradiation is an Effective Alternative to Ozonation as a Sea Water Treatment to Prevent Kudoa neurophila (Myxozoa: Myxosporea) Infection of Striped Trumpeter, Latris lineata (Forster). J. Fish Dis. 36 (1), 57–65. doi: 10.1111/j.1365-2761.2012.01413.x
DAWE. Australian Goverment Department of Agriculture, Water and the Environment. (2021). Australia's National List of Reportable Diseases of Aquatic Animals. Animal Health Committee: Disease Surveillance and reporting. Available at: https://www.awe.gov.au/agriculture-land/animal/aquatic/reporting/reportable-diseases (Acessed 31st of May 2022).
DAWE. Department of Agriculture, Water and the Environment. (2022). Australian Biosecurity Import Conditions: Live Ornamental Fish. Available at: https://bicon. agriculture.gov.au/BiconWeb4.0 (Accessed 25th of May 2022).
Eiras J. C., Molnár K., Lu Y. S. (2005). Synopsis of the Species of Myxobolus Bütschli, 1882 (Myxozoa: Myxosporea: Myxobolidae). Syst. parasitol. 61 (1), 1–46. doi: 10.1007/s11230-004-6343-9
Feist S. W., Longshaw M. (2006). “Phylum Myxozoa,” in Fish Diseases and Disorders: Volume 1: Protozoan and Metazoan Infections. Ed. Woo P. T. K. (Wallingford, United Kingdom: CABI Publishing), 230–296.
Fetherman E. R., Winkelman D. L., Schisler G. J., Myrick C. A. (2011). The Effects of Myxobolus cerebralis on the Physiological Performance of Whirling Disease Resistant and Susceptible Strains of Rainbow Trout. J. Aquat. Anim. Health 23 (4), 169–177. doi: 10.1080/08997659.2011.630273
Gallardo B., Clavero M., Sánchez M. I., Vilà M. (2016). Global Ecological Impacts of Invasive Species in Aquatic Ecosystems. Global Change Biol. 22 (1), 151–163. doi: 10.1111/gcb.13004
Gunter N. L., Adlard R. D. (2009). Seven New Species of Ceratomyxa Thélohan, 1892 (Myxozoa) From the Gall-Bladders of Serranid Fishes From the Great Barrier Reef, Australia. Syst. Parasitol. 73 (1), 1–11. doi: 10.1007/s11230-008-9162-6
Hallett S. L., Adam Ray R., Hurst C. N., Holt R. A., Buckles G. R., Atkinson S. D., et al. (2012). Density of the Waterborne Parasite Ceratomyxa shasta and Its Biological Effects on Salmon. Appl. Environ. Microbiol. 78 (10), 3724–3731. doi: 10.1128/AEM.07801-11
Hartikainen H., Bass D., Briscoe A. G., Knipe H., Green A. J., Okamura B., et al. (2016). Assessing Myxozoan Presence and Diversity Using Environmental DNA. Int. J. Parasitol. 46 (12), 781–792. doi: 10.1016/j.ijpara.2016.07.006
Heiniger H., Adlard R. D. (2013). Molecular Identification of Cryptic Species of Ceratomyxa Thélohan, 1892 (Myxosporea: Bivalvulida) Including the Description of Eight Novel Species From Apogonid Fishes (Perciformes: Apogonidae) From Australian Waters. Acta. Parasitol. 58 (3), 342–360. doi: 10.2478/s11686-013-0149-3
Heiniger H., Gunter N. L., Adlard R. D. (2007). Relationships Between Four Novel Ceratomyxid Parasites From the Gall Bladders of Labrid Fishes From Heron Island, Queensland, Australia. Parasitol. Int. 57 (2), 158–165. doi: 10.1016/j.parint.2007.11.006
Heiniger H., Gunter N. L., Adlard R. D. (2011). Re-Establishment of the Family Coccomyxidae and Description of Five Novel Species of Auerbachia and Coccomyxa (Myxosporea: Bivalvulida) Parasites From Australian Fishes. Parasitology 138 (4), 501–515. doi: 10.1017/S0031182010001447
Kent M. L., Andree K. B., Bartholomew J. L., El-matbouli M., Desser S. S., Devlin R. H., et al. (2001). Recent Advances in Our Knowledge of the Myxozoa. J. Eukaryotic Microbiol. 48 (4), 395–413. doi: 10.1111/j.1550-7408.2001.tb00173.x
Knight J. D. M. (2010). Invasive Ornamental Fish: A Potential Threat to Aquatic Biodiversity in Peninsular India. J. Threatened Taxa 2 (2), 700–704. doi: 10.11609/JoTT.o2179.700-4
Kristmundsson A., Freeman M. A. (2014). Negative Effects of Kudoa islandica n. sp. (Myxosporea: Kudoidae) on Aquaculture and Wild Fisheries in Iceland. Int. J. Parasitol Parasites Wildlife 3 (2), 135–146. doi: 10.1016/j.ijppaw.2014.06.001
Lom J., Arthur J. R. (1989). A Guideline for the Preparation of Species Descriptions in Myxosporea. J. Fish Dis. 12 (2), 151–156. doi: 10.1111/j.1365-2761.1989.tb00287.x
Lymbery A. J., Morine M., Kanani H. G., Beatty S. J., Morgan D. L. (2014). Co-Invaders: The Effects of Alien Parasites on Native Hosts. Int. J. parasitol Parasites wildlife 3 (2), 171–177. doi: 10.1016/j.ijppaw.2014.04.002
Marshall W. L., Sitjà-Bobadilla A., Brown H. M., MacWilliam T., Richmond Z., Lamson H., et al. (2016). Long-Term Epidemiological Survey of Kudoa thyrsites (Myxozoa) in Atlantic Salmon (Salmo salar L.) From Commercial Aquaculture Farms. J. Fish Dis. 39 (8), 929–946. doi: 10.1111/jfd.12429
Moran J. D. W., Whitaker D. J., Kent M. L. (1999). A Review of the Myxosporean Genus Kudoa Meglitsch, 1947, and its Impact on the International Aquaculture Industry and Commercial Fisheries. Aquaculture 172 (1), 163–196. doi: 10.1016/S0044-8486(98)00437-2
Morsy K., Abdel-Ghaffar F., Bashtar A. R., Mehlhorn H., Al Quraishy S., Abdel-Gaber R.. (2012). Morphology and Small Subunit Ribosomal DNA Sequence of Henneguya suprabranchiae (Myxozoa), a Parasite of the Catfish Clarias gariepinus (Clariidae) From the River Nile, Egypt. Parasitol. Res. (1987) 111 (4), 1423–1435. doi: 10.1007/s00436-012-2976-9
Nishioka T., Satoh J., Mekata T., Mori K., Ohta K., Morioka T., et al. (2016). Efficacy of Sand Filtration and Ultraviolet Irradiation as Seawater Treatment to Prevent Kudoa septempunctata (Myxozoa: Multivalvulida) Infection in Olive Flounder Paralichthys olivaceus. Gyobyō Kenkyu 51 (1), 23–27. doi: 10.3147/jsfp.51.23
Okamura B., Hartigan A., Naldoni J. (2018). Extensive Uncharted Biodiversity: The Parasite Dimension. Integr. Comp. Biol. 58 (6), 1132–1145. doi: 10.1093/icb/icy039
Post R. J., Millest A. L. (1991). Sample Size in Parasitological and Vector Surveys. Parasitol. Today 7 (6), 141. doi: 10.1016/0169-4758(91)90279-W
Rimmer A. E., Becker J. A., Tweedie A., Lintermans M., Landos M., Stephens F. L., et al. (2015). Detection of Dwarf Gourami Iridovirus (Infectious Spleen and Kidney Necrosis Virus) in Populations of Ornamental Fish Prior to and After Importation Into Australia, With the First Evidence of Infection in Domestically Farmed Platy (Xiphophorus maculatus). Prev. Vet. Med. 122 (1), 181–194. doi: 10.1016/j.prevetmed.2015.09.008
Saha M., Bandyopadhyay P. K. (2017). Parasitological and Histological Analysis of a New Species of the Genus Thalohanellus and Description of a Myxozoan Parasite (Myxosporea: Bivalvulida) From Cultured Ornamental Goldfish, Carassius auratus L. Aquacult. Rep. 8 (C), 8–15. doi: 10.1016/j.aqrep.2017.07.001
Sanil N. K., Chandran A., Shamal P., Binesh C. P. (2016). Molecular and Morphological Descriptions of Ceratomyxa collarae n. sp. And Ceratomyxa leucosternoni n. sp. From Marine Ornamental Fishes of Indian Waters. Parasitol Res. (1987) 116 (2), 529–537. doi: 10.1007/s00436-016-5317-6
Sayyaf Dezfuli B., Giari L., Bosi G. (2021). “Chapter Three - Survival of Metazoan Parasites in Fish: Putting Into Context the Protective Immune Responses of Teleost Fish,” in Adv. Parasitol.. Eds. Rollinson D., Stothard R. (Academic Press) 112, 77–132. doi: 10.1016/bs.apar.2021.03.001
Schindelin J., Arganda-Carreras I., Frise E., Kaynig V., Longair M., Pietzsch T., et al. (2012). Fiji: An Open-Source Platform for Biological-Image Analysis. Nat. Methods 9 (7), 676–682. doi: 10.1038/nmeth.2019
Sekiya M., Setsuda A., Sato H., Song K., Han J.-K., Kim G.-J., et al. (2016). Enteromyxum leei (Myxosporea: Bivalvulida) as the Cause of Myxosporean Emaciation Disease of Farmed Olive Flounders (Paralichthys olivaceus) and a Turbot (Scophthalmus maximus) on Jeju Island, Korea. Parasitol. Res. (1987) 115 (11), 4229–4237. doi: 10.1007/s00436-016-5200-5
Sheath D. J., Williams C. F., Reading A. J., Britton J. R. (2015). Parasites of non-Native Freshwater Fishes Introduced Into England and Wales Suggest Enemy Release and Parasite Acquisition. Biol. invasions 17 (8), 2235–2246. doi: 10.1007/s10530-015-0857-8
Shinn A. P., Pratoomyot J., Bron J. E., Paladini G., Brooker E. E., Brooker A. J. (2015). Economic Costs of Protistan and Metazoan Parasites to Global Mariculture. Parasitology 142 (1), 196–270. doi: 10.1017/S0031182014001437
Smothers J. F., Von Dohlen C. D., Smith L. H., Spall R. D. (1994). Molecular Evidence That the Myxozoan Protists are Metazoans. Sci. (American Assoc. Advancement Sci) 265 (5179), 1719–1721. doi: 10.1126/science.8085160
Surendran S., Chandran A., Vijayagopal P., Sanil N. K. (2021). Morphological and Molecular Characterization of Ceratomyxa xanthopteri n. sp. (Myxosporea: Ceratomyxidae) From the Marine Ornamental Fish Acanthurus xanthopterus Valenciennes 1835 (Acanthuridae) Off Vizhinjam Coast, Kerala. Parasitol. Res. (1987) 120 (7), 2445–2453. doi: 10.1007/s00436-021-07168-x
Trujillo-González A., Becker J. A., Hutson K. S. (2018a). Parasite Dispersal From the Ornamental Goldfish Trade. Adv. Parasitol. 100, 239–281. doi: 10.1016/bs.apar.2018.03.001
Trujillo-González A., Becker J. A., Vaughan D. B., Hutson K. S. (2018b). Monogenean Parasites Infect Ornamental Fish Imported to Australia. Parasitol. Res. (1987) 117 (4), 995–1011. doi: 10.1007/s00436-018-5776-z
Trujillo-González A., Edmunds R. C., Becker J. A., Hutson K. S. (2019). Parasite Detection in the Ornamental Fish Trade Using Environmental DNA. Sci. Rep. 9 (1), 5173–5173. doi: 10.1038/s41598-019-41517-2
Whipps C. M., Adlard R. D., Bryant M. S., Kent M. L. (2003a). Two Unusual Myxozoans, Kudoa Quadricornis n. sp. (Multivalvulida) From the Muscle of Goldspotted Trevally (Carangoides Fulvoguttatus) and Kudoa Permulticapsula n. sp. (Multivalvulida) From the Muscle of Spanish Mackerel (Scomberomorus Commerson) From The Great Barrier Reef, Australia. J. Parasitol. 89 (1), 168–173. doi: 10.1645/0022-3395(2003)089[0168:TUMKQN]2.0.CO;2
Whipps C. M., Adlard R. D., Bryant M. S., Lester R. J., Findlav V., Kent M. L. (2003b). First Report of Three Kudoa Species From Eastern Australia: Kudoa thyrsites From Mahi Mahi (Coryphaena hippurus), Kudoa amamiensis and Kudoa minithyrsites n. sp. From Sweeper (Pempheris ypsilychnus). J. Eukaryotic Microbiol. 50 (3), 215–219. doi: 10.1111/j.1550-7408.2003.tb00120.x
Whipps C. M., Grossel G., Adlard R. D., Yokoyama H., Bryant M. S., Munday B. L., et al. (2004). Phylogeny of the Multivalvulidae (Myxozoa: Myxosporea) Based on Comparative Ribosomal DNA Sequence Analysis. J. Parasitol. 90 (3), 618–622. doi: 10.1645/GE-153R
Whittington R. J., Chong R. (2007). Global Trade in Ornamental Fish From an Australian Perspective: The Case for Revised Import Risk Analysis and Management Strategies. Prev. Vet Med. 81 (1), 92–116. doi: 10.1016/j.prevetmed.2007.04.007
Zhang J. Y., Wang J. G., Li A. H., Gong X. N. (2010). Infection of Myxobolus turpisrotundus sp. n. In Allogynogenetic Gibel Carp, Carassius Auratus gibelio (Bloch), With Revision of Myxobolus Rotundus (s. L.) Nemeczek Reported From C. Auratus Auratus (L.). J. Fish Dis. 33 (8), 625–638. doi: 10.1111/j.1365-2761.2010.01161.x
Zhang B., Zhai Y., Gu Z., Liu Y. (2018). Morphological, Histological and Molecular Characterization of Myxobolus Kingchowensis and Thelohanellus cf. Sinensis Infecting Gibel Carp Carassius auratus gibelio (Bloch, 1782). Acta. Parasitol. 63 (2), 221–231. doi: 10.1515/ap-2018-0026
Keywords: Myxozoa, surveillance, border control, aquatic parasites, ornamental fish
Citation: Trujillo-González A, Allas J, Miller TL, Becker JA and Hutson KS (2022) Myxozoan Diversity Infecting Ornamental Fishes Imported to Australia. Front. Mar. Sci. 9:910634. doi: 10.3389/fmars.2022.910634
Received: 01 April 2022; Accepted: 12 May 2022;
Published: 14 June 2022.
Edited by:
Archana Sinha, Central Inland Fisheries Research Institute (ICAR), IndiaReviewed by:
Sajina A. Muhammadali, Central Inland Fisheries Research Institute (ICAR), IndiaSagar Mandal, Central Agricultural University, India
Copyright © 2022 Trujillo-González, Allas, Miller, Becker and Hutson. This is an open-access article distributed under the terms of the Creative Commons Attribution License (CC BY). The use, distribution or reproduction in other forums is permitted, provided the original author(s) and the copyright owner(s) are credited and that the original publication in this journal is cited, in accordance with accepted academic practice. No use, distribution or reproduction is permitted which does not comply with these terms.
*Correspondence: Alejandro Trujillo-González, YWxlamFuZHJvLnRydWppbGxvZ29uemFsZXpAY2FuYmVycmEuZWR1LmF1