- 1Fisheries Resources Research Center, National Institute of Fisheries Science, Tongyeong, South Korea
- 2Department of Marine Ecology and Environment, Gangneung-Wonju National University, Gangneung, South Korea
Largehead hairtail (Trichiurus japonicus) is one of the most commercially important fishes in the western North Pacific. To identify the dietary changes across ontogeny and seasons, we investigated its stomach contents and stable isotope signatures (δ13C and δ15N) in the South Sea of Korea. The index of relative importance revealed that the dietary composition of largehead hairtail consisted mainly of Pisces (1.6%–84.8%) and Euphausiacea (3.0%–93.0%). However, the proportions of dietary items differed by season and anal length (ALs), suggesting a trophic adaptability response to seasonal food availability and size-related trophic variation, respectively. Our isotopic results showed that the largehead hairtail is placed intermediately between pelagic and benthic fishes and was more 13C and 15N-depleted than other carnivorous fishes, suggesting a high consumption of pelagic species. Similarly, seasonal variations were observed in the δ13C and δ15N values of the largehead hairtails (PERMANOVA, p= 0.001). Interestingly, their δ13C values in spring decreased significantly with ALs (r2 = 0.594, p< 0.001), suggesting an ontogenetic change due to the increased proportion of Euphausiacea to the nutrition of largehead individuals during this specific season. Based on a combination of stomach contents and stable isotope signatures, overall results provide new insights into the feeding characteristics of the largehead hairtail, which improves our understanding of its trophic ecology depending on season and body size.
1. Introduction
The family Trichiuridae, known as hairtail, is an economically important teleost fish group with a worldwide distribution generally inhabiting tropical and temperate continental shelves and shallow coastal waters (Nakamura and Parin, 1993). In 2016, the estimated annual catch of hairtail worldwide was approximately 1,280,000 t, making it one of the most caught marine fish species (FAO, 2019). In particular, the largehead hairtail (Trichiurus japonicus) is one of the most commercially important fishery resources in the western North Pacific (Lin et al., 2007). This species is widely distributed in the Bohai Sea, Yellow Sea, East China Sea, and South Sea of Korea. In Korea, its annual catch was approximately 120,000 t in the 1980s; since then, the annual catch has declined gradually from an average of approximately 60,000–80,000 t in the 2000s to an average of approximately 44,000 t in the last five years (KOSIS, 2019). Due to its increasing commercial value and decreasing catch volumes, the basic ecology, life history, population dynamics, habitat, and environmental conditions of the largehead hairtail are being widely studied (Kim et al., 2005; He et al., 2014; Kim et al., 2020; Sun et al., 2020; Mammel et al., 2022). In addition to basic biological knowledge, trophic ecology is essential for the effective management and conservation of this specific fish population.
In general, scientific data on the quality and quantity of diets available for fish populations are needed to evaluate the effects of environmental changes on biological processes, including growth and reproduction (Beverton and Holt, 1957; Darnaude, 2005; Persson and De Roos, 2006). Hairtails are high trophic level predators that play a key role in the marine food web, as they control lower trophic level species (e.g., fish, shrimp, and squid) through top-down processes (Martins et al., 2005; Yan et al., 2011). Since the survival and abundance of the hairtail can potentially affect the population dynamics of other fish, understanding their feeding ecology, based on energy flow within marine ecosystems, is critical for the assessment of commercial fisheries resources (Mammel et al., 2022). Accordingly, many studies have addressed the feeding characteristics, dietary composition, ontogenetic changes, and spatiotemporal variations in the diet of hairtails (Lee, 1978; Bakhoum, 2007; Liu et al., 2009; Yan et al., 2011; Hamaoka et al., 2014; Mammel et al., 2022). However, most of the research was limited to Chinese hairtail populations inhabiting China and the Yellow Sea. For the successful management of a given fish population, it is important to understand the spatial variability in its feeding characteristics resulting from the differences in prey composition in the feeding ground and habitats (Liu et al., 2009). Despite the importance of this information, very little is known about the feeding strategy of hairtail populations in Korean coastal waters (Huh, 1999).
Stomach content analysis has been commonly used to investigate the feeding habits of fish species and provide detailed information on their dietary composition (Hyslop, 1980; Buckland et al., 2017). This is the most widely accepted tool for generalizing the feeding ecology of consumer species (Baker et al., 2014). However, this technique can only reflect transient diet, and the digested and unidentifiable stomach contents may cause uncertainty in the taxonomic identification of the dietary items (Deb, 1997; Davenport and Bax, 2002). Stable isotope analysis has been adopted as an alternative method to overcome the limitations of stomach content analysis, providing a long-term view of feeding characteristics by evaluating actual assimilated dietary items among a variety of food sources during the feeding season (Michener and Schell, 1994; Layman et al., 2012). Stable carbon isotope (δ13C) can be used to infer the dietary source for consumer nutrition, which generally increases from prey to consumer by a slight enrichment of less than 1.0‰ (DeNiro and Epstein, 1978; Fry and Sherr, 1984). In contrast, stable nitrogen isotope (δ15N) can offer information on the trophic position (TP) of animals, which exhibits a high isotopic discrimination of 2–4‰ between prey and consumers (Minagawa and Wada, 1984; Post, 2002). Stable isotope analysis has been increasingly used to assess the trophic role of fish species and their ontogenetic dietary shifts (Jennings et al., 2002; Darnaude, 2005; Chiang et al., 2020; Estupiñán-Montaño et al., 2021). Stable isotope analysis supported by stomach content analysis is among the most powerful tools for improving the interpretation of feeding studies for fish species (Davis et al., 2012; Santos et al., 2013; Park et al., 2018).
The objective of this study was to examine the trophic ecology of the largehead hairtail in the South Sea of Korea. We investigated stable isotope signatures (δ13C and δ15N) and stomach contents of largehead hairtail. Specifically, considering its seasonal growth dynamics (Huh, 1999), we focused on the changes in its dietary composition and TP across ontogeny and seasons. A combination of stable isotope and stomach content analyses has rarely been applied to this species (Hamaoka et al., 2014). In addition, most studies have investigated the feeding characteristics of T. lepturus, whereas information on the trophic ecology of T. japonicus is very limited (Mammel et al., 2022). We anticipated that if the dietary composition of T. japonicus changes in association with its ontogeny and season, its tissue isotopic signatures would reflect this change. To the best of our knowledge, this is the first study to identify the trophic ecology of the largehead hairtail that may play an important role in the marine food web of Korean coastal waters.
2. Materials and methods
2.1. Study area and sample collection
Field surveys were performed on the continent shelf of the South Sea of Korea (Northern East China Sea, 33° 49´N, 127° 13´E) (Figure 1). The water temperature, salinity, and chlorophyll a levels in the sampling area were obtained using a CTD (SBE 911 Plus, Seabird Electronics Inc., Bellevue, WA, USA). The sampling area had low tidal amplitude (less than 30 cm) and water depth approximately 94–102 m. All specimens of largehead hairtail T. japonicus (a total of 422 individuals) and other fish were collected during February, May, August, and November 2021. Sampling was conducted using a trawl net (12 cm mesh in the main body, 8 cm mesh in the intermediate part, and 6 cm mesh in the cod end with a 2 cm cod end liner of the net) from a research vessel (R/V Tamgu 22) for over 30 min at 3 knots. The fishing depth for sampling ranged from 91.1 m to 98.2 m. The total length and weight of each individual was measured on board to the nearest 0.1 cm and 0.1 g, respectively. The collected fish samples were stored in a deep freezer (−60°C) and transported to the laboratory. For the collection of dominant zooplankton groups, sampling was conducted through oblique towing on each occasion using a Bongo net (2.0 m2 mouth opening, 500 μm mesh) equipped with a flowmeter. The collected zooplankton samples were frozen in 1 L polyethylene bottles in a deep freezer (−60°C) for processing.
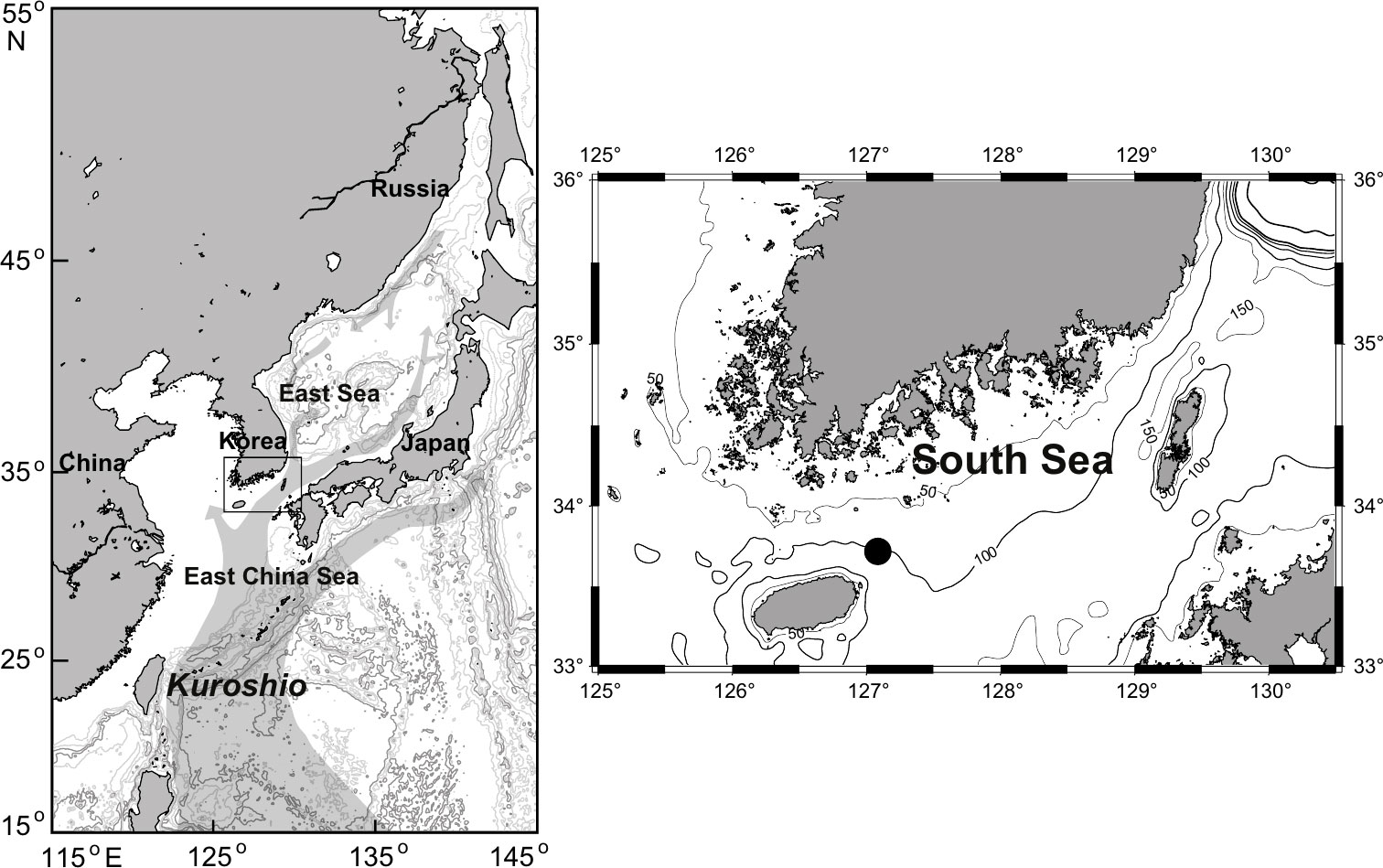
Figure 1 Map of the sampling area in the South Sea of Korea. Field circle indicates the sampling site collected for zooplankton, large-head hairtail (Trichiurus japonicus), and other fishes.
2.2. Laboratory processing
The collected fish samples for stable isotope analysis were dissected, and only muscle tissues were obtained from the anterior dorsal region. The stomachs were separated from the largehead hairtail individuals, and their contents were preserved in 10% formalin for stomach content analysis. For the zooplankton samples, two dominant groups of copepods and euphausiids for stable isotope analysis were identified under a dissecting microscope. The fish and zooplankton samples for isotope analysis were dried in an oven for 72 h at 50–60°C, ground into a homogeneous powder using a mortar and pestle, and kept frozen in a deep freezer (−70°C) to avoid contamination damage until the next stage.
2.3. Stomach content analysis
In the laboratory, the preserved stomach contents were identified to the lowest possible taxonomic level under a stereomicroscope (LEICA 12; Wetzlar, Germany). The wet weight of each food item was measured to the nearest 0.1 mg using an analytical balance (ME204TE/00; Mettler Toledo, Greifensee, Switzerland). Fresh and partially digested prey were used to reduce bias in the stomach content analysis. Food components were estimated by the percent frequency of occurrence (% F, number of stomachs in which a particular food item occurred as a percentage of the total number of stomachs examined), percent numerical frequency (% N) of each prey item to the total number of identifiable food items, and percent wet weight (% W) of each prey item to the total wet weight of the identifiable food items. An index of relative importance (IRI) for all food components was calculated for each prey type [IRI = (% N+% W)× % F] and expressed as a percentage (% IRI) as follows: (Pinkas et al., 1971), where n is the total number of food components categorized as class levels. Size-related dietary changes were examined by dividing the largehead specimens into three size classes:<10 cm, 10–15 cm, and ≥15 cm, based on their anal lengths (ALs).
2.4. Stable isotope analysis
Powdered zooplankton and fish samples (0.5–1.0 mg) were wrapped in tin combustion capsules (5 Ö9 mm, D– H). All sealed samples were combusted at 1020°C using a CNSOH elemental analyzer (EA Isolink, Bremen, Germany), and the resultant gases were analyzed using a linked continuous-flow isotope ratio mass spectrometer (CF-IRMS; DELTA V PLUS, Bremen, Germany). The stable isotope ratios are expressed in δ notation relative to conventional standard reference materials (Vienna Pee Dee Belemnite for carbon and atmospheric N2 for nitrogen) as follows: , where X is 13C or 15N, and R is 13C/12C or 15N/14N. The international standards of sucrose (NIST-8542, C12H22O11) and glucose (BCR-657, C6H12O6) for δ13C measurements and ammonium sulfate (NIST-8547, [NH4]2SO4) and L-glutamic acid (USGS-40, C5H9NO4) for δ15N measurements were used as reference standards to calibrate the analyzed isotope values. The analytical precision based on 20 urea (a laboratory reference) replicates was within 0.15‰ and 0.18‰ for δ13C and δ15N, respectively.
Due to bias in the δ13C values for fish species resulting from interspecific differences in the concentration of 13C-depleted lipids, we performed lipid correction by Post et al. (2007) if their C:N ratios were more than 3.5. The lipid correction was performed as follows: 13Cnormalized= 13Cuntreated −3.32+0.99 ×C:N (ratios) , where 13Cuntreated and 13Cnormalized are the measured and lipid-normalized values of the sample, respectively.
The TP for fish species was calculated using the equation: TPi=(δ15Ni− δ15Nbaseline)/Δ15N+2 , where δ15Ni represents the mean δ15N of the target species, δ15Nbaseline is the mean δ15N of trophic baseline consumers (i.e., copepod group), Δ15N is the enrichment factor (3.4‰) in δ15N per TP, and 2 represents the baseline TP (Post, 2002).
2.5. Data analysis
Data were analyzed using SPSS software (version 23.0, IBM, Armonk, NJ, United States) and PRIMER version 6 + PRIMER add-on (PRIMER-e, Auckland, New Zealand). Prior to statistical analyses, all data were tested for normality and homogeneity of variance using the Shapiro-Wilk and Levene’s tests, respectively. One-way analysis of variance (ANOVA) was used to test for significant differences in the TP values and ALs of largehead hairtails, followed by Tukey’s honest significant difference (HSD) multiple-comparison post hoc test to evaluate differences among variables. To examine the size-based trophic dynamics of the largehead hairtails, linear regressions were used to examine the relationships between their ALs and isotope values (δ13C and δ15N). All values are presented as the mean ± standard deviation. Significant differences in the δ13C and δ15N values of zooplankton, largehead hairtails, and other fish consumers among seasons were tested using permutational multivariate analysis of variance (PERMANOVA).
3. Results
3.1. Environmental conditions
The water temperature was lowest at the surface (16.2°C) in February and highest (27.6°C) in August (Table 1). In contrast, during the four seasons, the bottom layer water temperature ranged from 15.2°C in August to 15.9°C in February. The salinities in the surface layer varied between 30.6 in August and 34.8 in February. In the bottom layer, the salinity was fairly constant (34.4 in August and 34.8 in February). Chlorophyll a concentration of the surface water ranged from 0.8 μg/L in August to 1.2 μg/L in November.

Table 1 The environmental conditions (water temperature, salinity, and chlorophyll a) at the surface and bottom layers in the South Sea of Korea during February, May, August, and November 2021.
3.2. Dietary compositions
Of the 747 largehead hairtail stomachs sampled, 322 (43.1%) were empty. A total of 14 prey taxa groups were identified based on class (Figure 2). The diet of the largehead hairtail (based on IRI, %) consisted mainly of Pisces (1.6% in May to 84.8% in February) and Euphausiacea (3.0% in February to 93.0% in May) over the four seasons. The minor components of the stomach contents were Macrura (1.6% in May to 26.8% in November) and Amphipoda (0.1% in February to 7.4% in August). Size-related stomach compositions also showed that Pisces and Euphausiacea were preferred by the three AL groups (10 cm, 10–15 cm, and > 15 cm). While the small and mid-groups of largehead hairtails represented 5.5–93.4% and 22.1–94.6% for Pisces and 0–24.7% and 1.0–69.5% for Euphausiacea, respectively; the preferred prey composition of their largest group was 4.3 to 92.5% for Euphausiacea and 2.4 to 81.3% for Pisces.
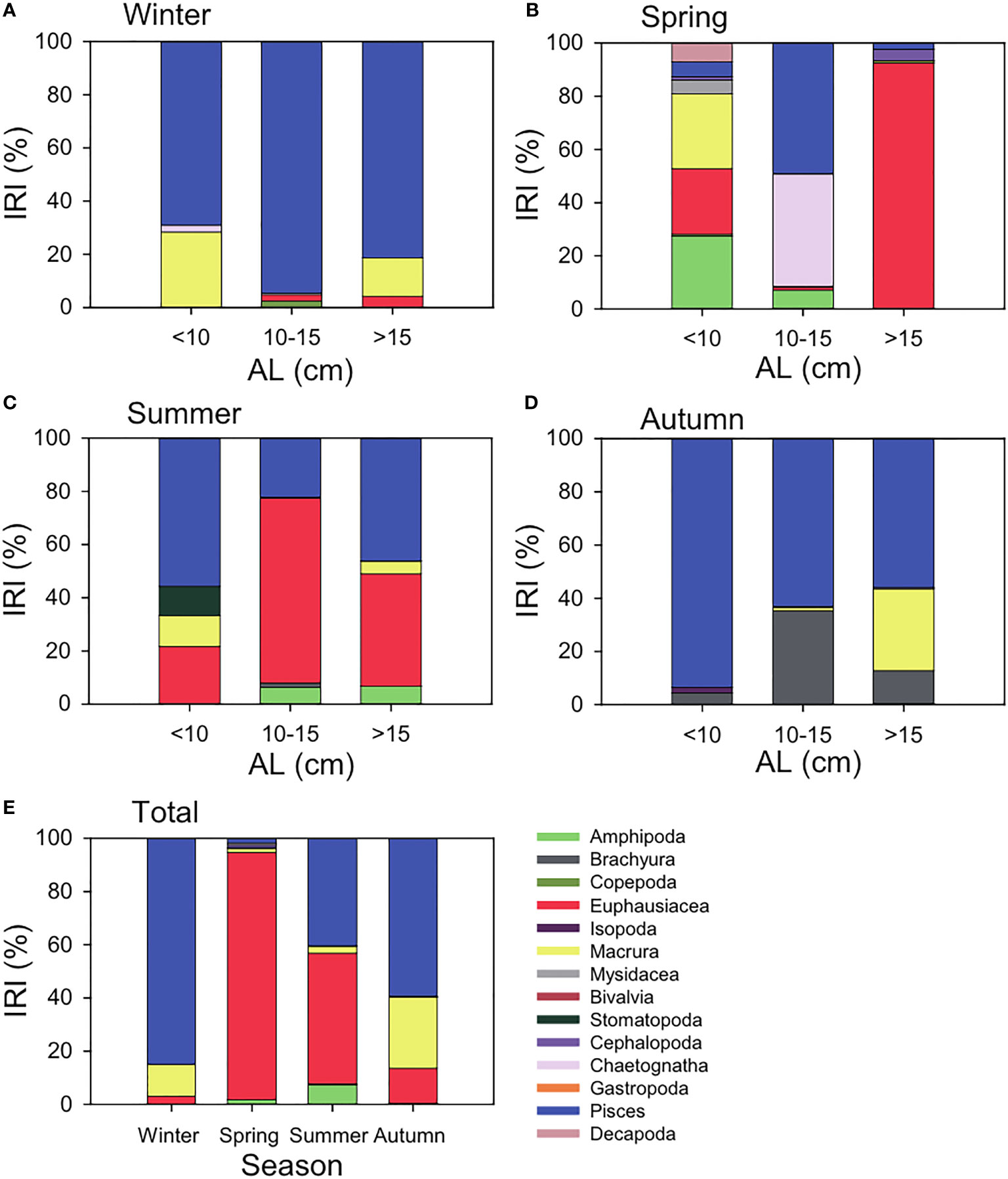
Figure 2 Size-related (anal length, AL; 10 cm, 10–15 cm, and > 15 cm) and total (E) compositions of the stomach contents of large-head hairtail (Trichiurus japonicus) by the index of relative importance (%IRI) expressed as a percentage of the sum of the IRI values in the South Sea of Korea during winter (A, February), spring (B, May), summer (C, August), and autumn (D, November) 2021.
3.3. Stable isotope ratios
3.3.1. Zooplankton
The δ13C and δ15N values of both zooplankton groups (calanoid copepods and euphausiids) differed significantly among the four seasons (PERMANOVA, pseudo-F3, 48 = 12.29, p = 0.001 and pseudo-F3, 35 = 9.36, p = 0.001, respectively) (Table 2). The mean δ13C and δ15N values of copepods ranged from −22.5 ± 0.5‰ (November) to −20.2 ± 1.2‰ (February) and from 6.4 ± 0.5‰ (February) to 8.0 ± 0.9‰ (August), respectively. The euphausiids showed mean δ13C and δ15N values of −21.3 ± 0.9‰ (May) to −20.4 ± 1.0‰ (February) and 6.1 ± 0.6‰ (November) to 8.5 ± 0.9‰ (August), respectively.

Table 2 δ13C and δ15N values of zooplankton (calanoid copepods and euphausiids) collected during February, May, August, and November 2021 in the South Sea of Korea. PERMANOVA test of δ13C and δ15N values for each zooplankton among seasons.
3.3.2. Largehead hairtail
Largehead hairtails of 18 (August) to 37 (November) individuals were prepared for stable isotope analysis during the four seasons (Figure 3). Seasonal variations were observed in δ13C and δ15N values of the largehead hairtail (pseudo-F3, 94 = 9.03, p = 0.001). The δ13C values of largehead hairtail ranged from −19.8 ± 0.8‰ (May) to −18.2 ± 0.3‰ (August), whereas the δ15N values varied between 10.8 ± 0.7‰ (May) and 11.3 ± 1.2‰ (February). The TPs of largehead hairtails, calculated based on the lowest δ15N values of zooplankton, showed significant differences among seasons (Tukey’s HSD test, p< 0.01), ranging from 2.8 ± 0.2 in August to 3.5 ± 0.2 in November.
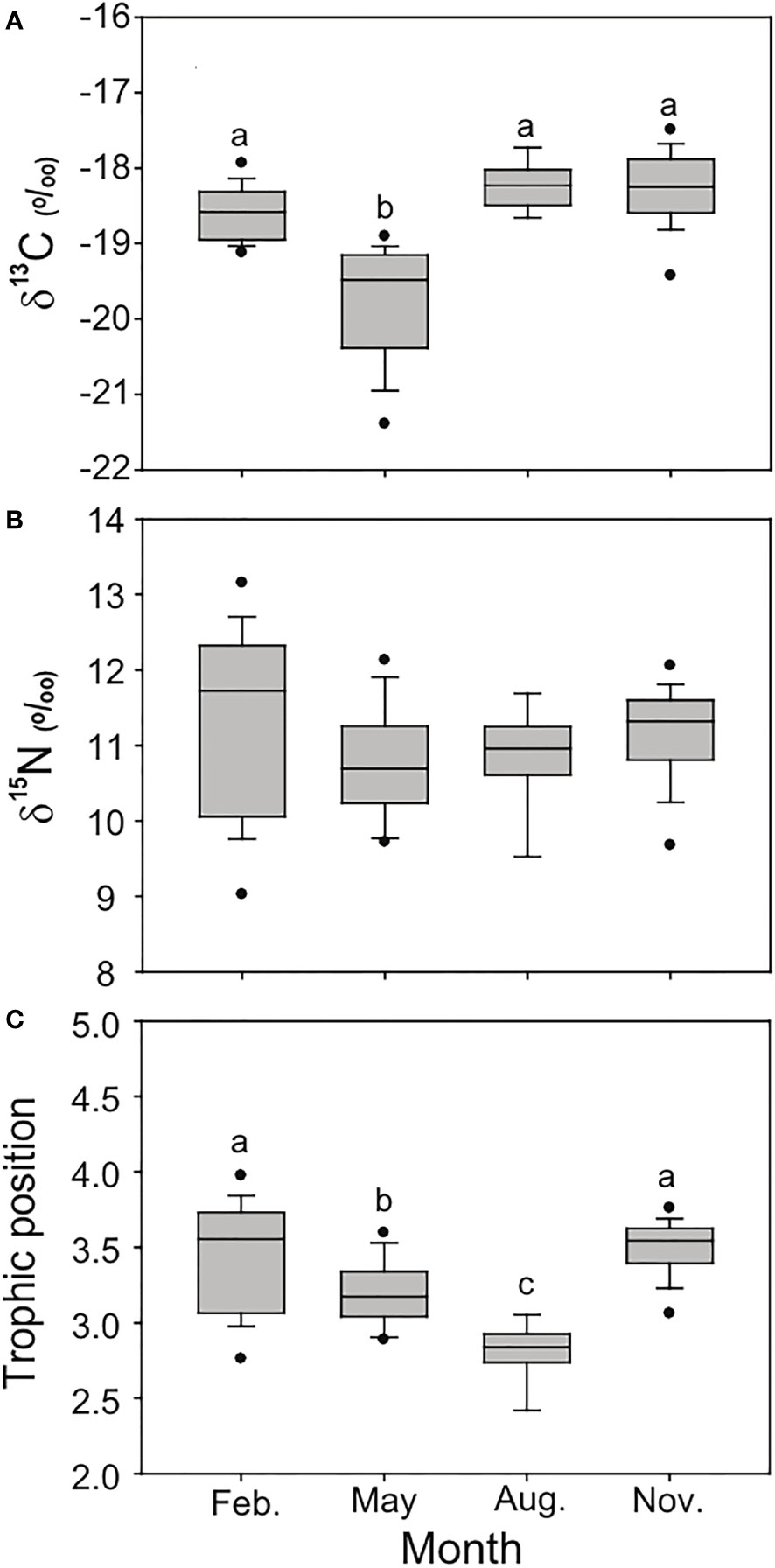
Figure 3 δ13C (A), δ15N (B), and trophic position (TP, C) values of large-head hairtail (Trichiurus japonicus) collected in the South Sea of Korea during February (Feb.), May, August (Aug.), and November (Nov.) 2021. One-way ANOVA and mean values of δ13C, δ15N, and TP values for large-head hairtail among four months.
There were significant differences in the mean ALs of analyzed largehead hairtails among seasons (one-way ANOVA, p = 0.001), ranging from 11.6 ± 4.0 cm (5.8 to 19.3 cm in February) to 17.9 ± 7.3 cm (6.8 to 28.4 cm in May). The δ13C values in May decreased significantly with AL (r 2 = 0.594, p< 0.001), whereas there were no significant correlations between ALs and δ13C in February, August, and November (r 2 = 0.033, p = 0.461; r 2 = 0.002, p = 0.929; r 2 = 0.006, p = 0.147, respectively; Figure 4). In contrast, significant positive relationships were found between ALs and δ15N in May, August, and November (r 2 = 0.441, p = 0.002; r 2 = 0.244, p = 0.033; r 2 = 0.420, p< 0.001, respectively). However, in February, there was no significant relationship between the AL and δ15N (r 2 = 0.115, p = 0.148).
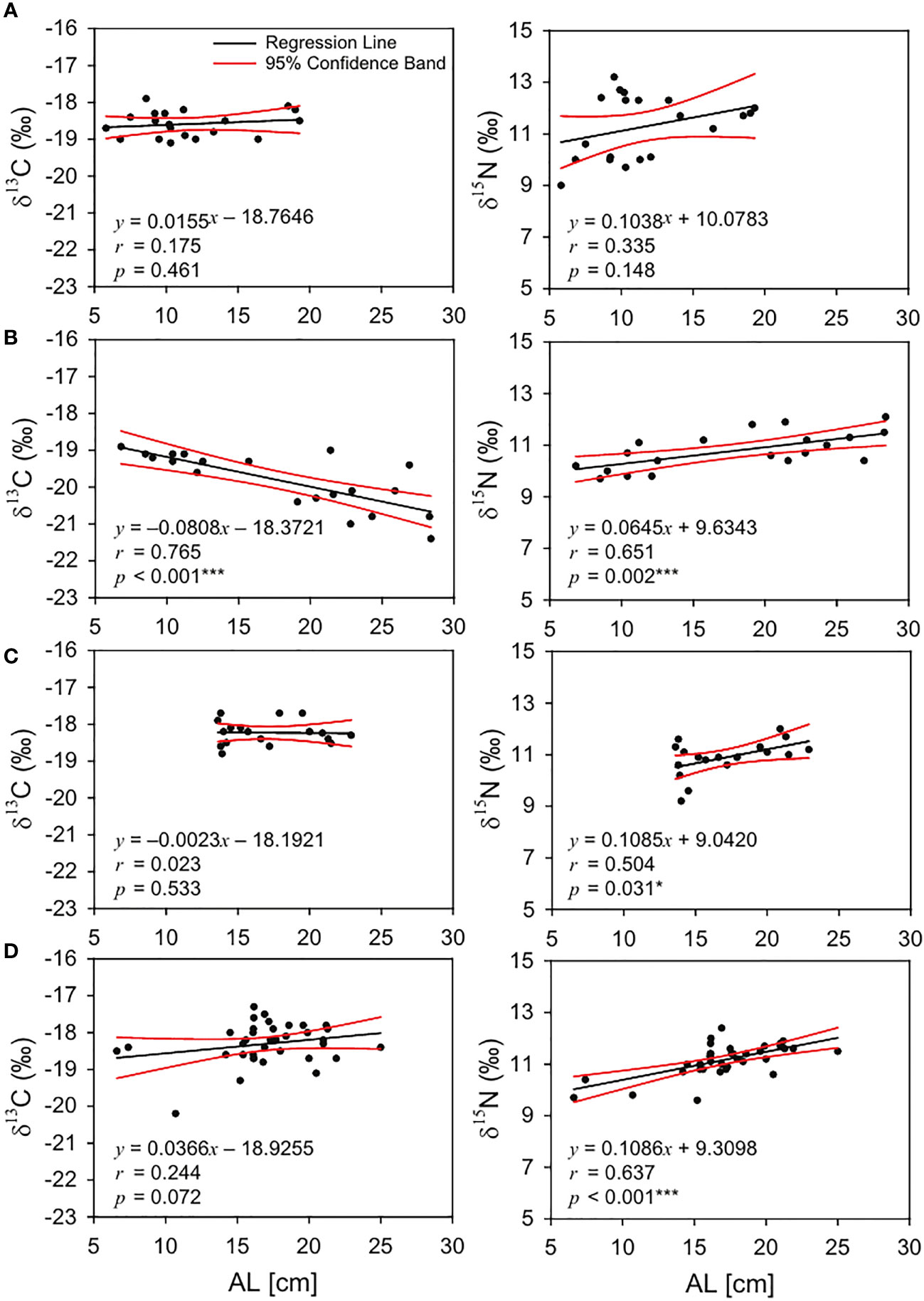
Figure 4 Regression relationships between δ13C – anal length (cm, left) and δ15N – anal length (cm, right) of large-head hairtail (Trichiurus japonicus) collected in the South Sea of Korea during February (A), May (B), August (C), and November (D) 2021, *, p < 0.05 and ***, p < 0.001.
3.3.3. Dual isotope plot for largehead hairtail and other fish consumers
Dual isotope plots for all fish consumers showed variable ranges of δ13C and δ15N values (Figure 5), which were significantly different among the seasons (PERMANOVA, pseudo-F3, 179 = 7.52, p = 0.001). The range of δ13C values of fish consumers during February (–21.8‰ to –16.6 ± 0.4‰) and May (–20.8‰ to –17.3 ± 0.3‰) were broader than those during August (–19.4‰ to –17.4 ± 0.2‰) and November (–18.6 ± 0.4‰ to –16.6 ± 0.2‰). Similarly, relatively wide ranges were observed for δ15N values during February (8.8‰ to 13.8 ± 0.7‰) and May (9.5‰ to 13.3 ± 0.4‰) compared with those during August (9.6‰ to 12.3 ± 0.2‰) and November (10.1‰ to 12.6 ± 0.3‰). The mean TP values of fish consumers (except for largehead hairtail) differed significantly among seasons (Tukey’s HSD test, p< 0.01), ranging from 2.90 ± 0.2 in August (2.46 − 3.27) to 3.59 ± 0.2 in November (3.20 − 3.93). Overall, the largehead hairtails showed intermediate δ13C and δ15N positions in the dual isotope plots during all seasons.
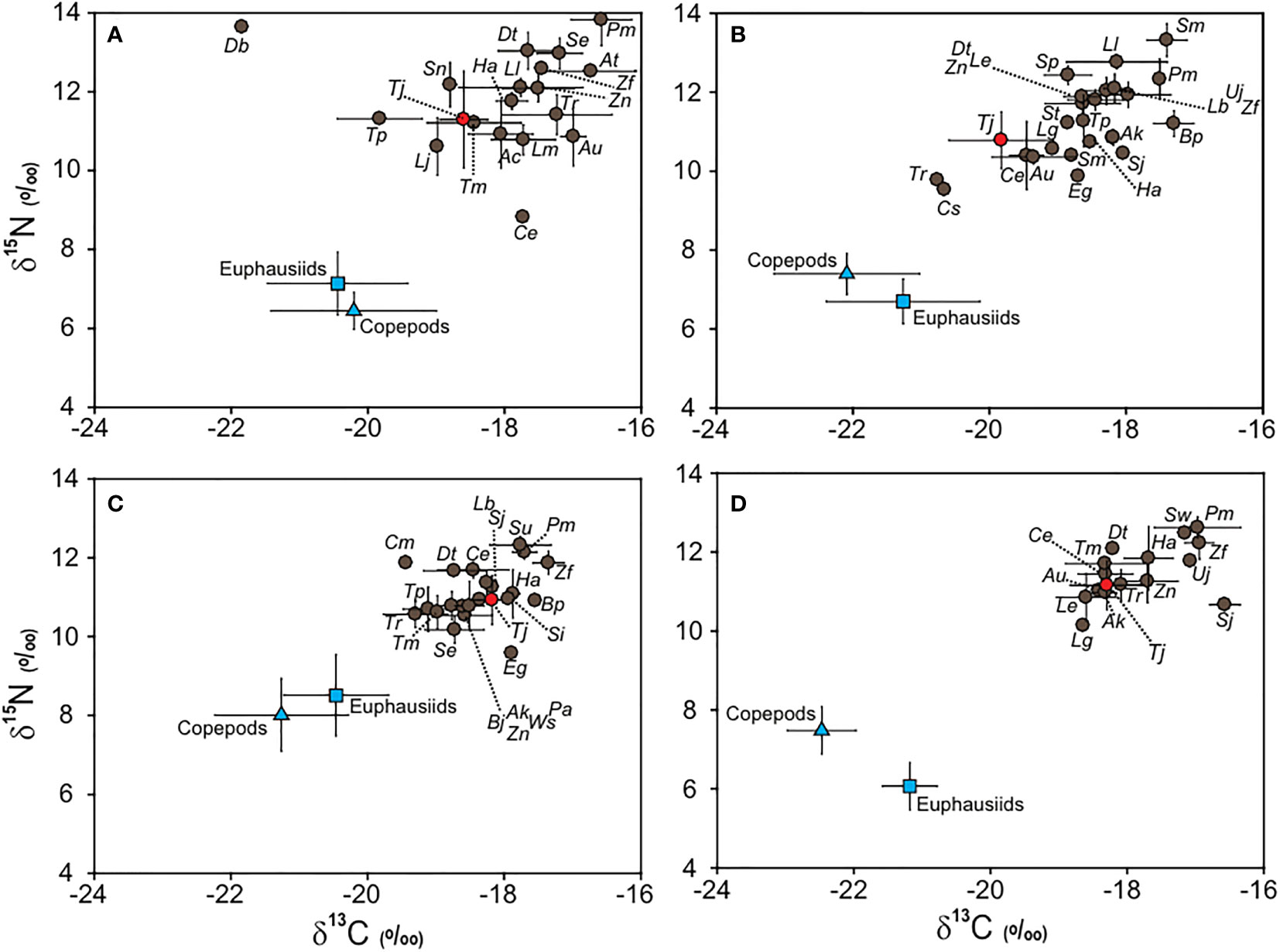
Figure 5 Bi-plots of δ13C and δ15N values of zooplankton (blue squares, euphausiids; blue triangles, copepods), large-head hairtail (red circles, Trichiurus japonicus), and other fishes (gray circles) in the South Sea of Korea during February (A), May (B), August (C), and November (D) 2021. Values are presented as mean δ13C and δ15N (‰ ± 1 SD). Species codes are presented in Table 3.
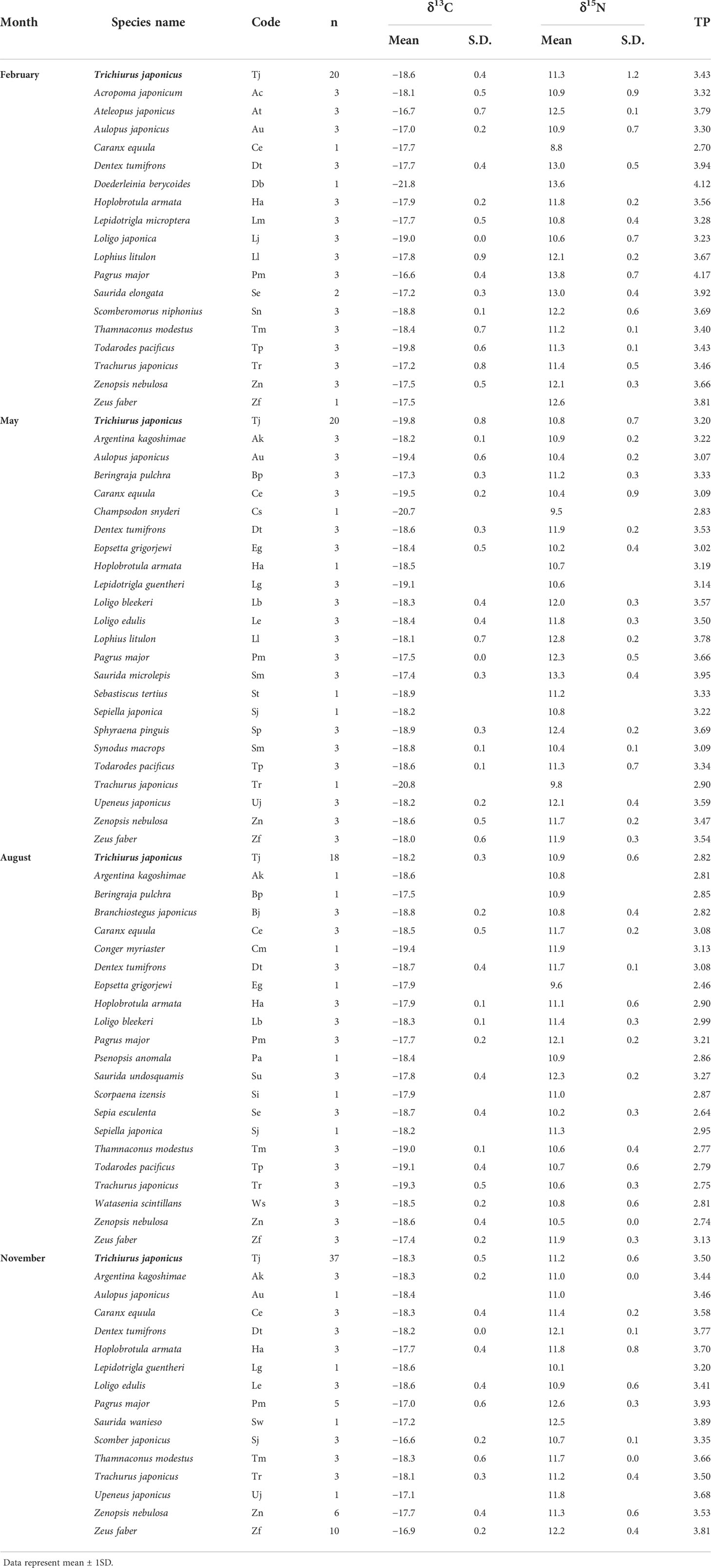
Table 3 δ13C and δ15N values and trophic position (TP) of large-head hairtail Trichiurus japonicus and other fish consumers collected during February, May, August, and November 2021 in the South Sea of Korea.
4. Discussion
This study revealed the feeding ecology of largehead hairtail (T. japonicus) in the southern coast of Korea through the combined use of stable isotope and stomach content analyses. The feeding characteristics observed in the species may be explained by seasonally varying patterns of feeding dynamics, ontogenetic dietary shifts, and dietary composition. A wide range of prey compositions for largehead hairtail as a carnivorous species has been reported from several other seas, identifying principal prey items, including zooplankton, shrimps, cephalopods, and fish, based on stomach content analysis (Martins et al., 2005; Mammel et al., 2022). Our study provides valuable information for understanding population variations in the dietary composition and trophic position (TP) of largehead hairtails in different foraging areas resulting from opportunistic and variable feeding behavior.
4.1. Seasonal effects on the dietary compositions of largehead hairtail
Overall, in the present study, the major diet of largehead hairtail was Pisces (1.6–84.8%) and Euphausiacea (3.0–93.0%) over the four seasons, regardless of the size effect. However, the proportions of dietary items differed by season and AL, as identified under microscopic observation. A clear seasonal distinction in the prey composition of the hairtail may be closely associated with its trophic adaptability response to food availability (Yan et al., 2012). Although we did not perform a seasonal analysis of the relationship between environmental variables and distributions of dietary items for the species, they have been identified as generalists due to their fluctuating feeding intensity as per prey availability (Hamaoka et al., 2014; Mammel et al., 2022). In addition, dietary preference shifts for largehead hairtails were likely related to their spawning season or maturation size. The major spawning periods for the species in the South Sea of Korea has been reported to be from May to September and the minimum ALs at sexual maturity is more than 25.0 cm (Cha and Lee, 2004; Kim et al., 2020). The seasonal and size-related prey compositions observed on stomach content analysis in our study may coincide with the known major spawning season. Most fish species, including hairtails, require additional nutritional energy for gonad development and maturation (Mohammed Koya et al., 2018). Accordingly, despite Pisces and Euphausiacea being the preferred diet of longhead hairtails, the diet composition showing contrasting results across seasons and size classes may be regulated by two important factors: seasonal food availability and size-related trophic variation.
In this study, stable isotope analysis revealed seasonal changes in the feeding ecology of largehead hairtail. Significant seasonal differences in the δ13C and δ15N values of the largehead hairtail suggest that its diet is flexible. Hairtails and largehead hairtails inhabiting the East Asian Marginal Seas are generally known to exhibit different spatiotemporal feeding habits due to differences in reliance on pelagic and benthic production, mainly through consuming pelagic and benthic fishes and Eupausia pacifica (Yan et al., 2011; Hamaoka et al., 2014). Several studies have reported that consumer species commonly discriminate isotopically between benthic and pelagic food webs, as shown by a higher number of 13C- and 15N-enriched benthic species than pelagic feeders (Davenport and Bax, 2002; Boyle et al., 2012; Park et al., 2020). Benthic consumers may display a tendency to demonstrate increasing δ13C and δ15N values with increasing depth due to the biogeochemical process of sinking phytoplankton-derived organic matter as the basal nutritional source supporting benthic food webs (Polunin et al., 2001; Park et al., 2018). The relatively lower δ13C values of the largehead hairtail in spring compared with those in other seasons were closely related to the higher reliance on pelagic production. These results were supported by the increased contribution of Euphausiacea to the diet of hairtails, as estimated by stomach content analysis. Furthermore, although hairtails are generally known as top predators, their relatively lower δ15N values than those of other carnivorous fishes were likely related to the higher contribution of pelagic sources to their diets. Dual isotope plots of fish consumers showed that hairtails had intermediate positions between pelagic and benthic fishes and was more 13C and 15N-depleted than other large carnivorous fishes. Thus, these results suggest that largehead hairtails may feed on both pelagic and benthic dietary sources and dietary items with a pelagic source affinity (Le Loc’h et al., 2008).
4.2. Size-related dietary compositions of largehead hairtail
Our isotopic results revealed a significant positive relationship between ALs and δ15N values of largehead hairtails, coinciding with higher δ15N values in larger individuals, except in February. Since higher δ15N values of carnivorous consumers are closely related to larger and higher TP dietary items, such ontogenetic dietary shifts are likely to be a general trend in the feeding characteristics of common fish species (Link and Garrison, 2002; Graham et al., 2007). The ecological function of increasing fish body and mouth size can allow individuals to capture a wider range of prey sizes and types for energy optimization (Gerking, 1994). Studies of the hairtail species T. margarites, T. lepturus, and T. japonicus show that with growth-related increasing body length, noticeable ontogenetic dietary changes have been observed by increasing the consumption of larger prey items (Yan et al., 2011; Mammel et al., 2022).
However, except in May, the δ13C values of largehead hairtails in our study did not reflect isotopic changes with body size. This result suggests that they may feed on similar dietary sources among size classes and that their dietary overlap may be relatively high. Although many fish species tend to have the potential for resource and trophic niche partitioning because of differences in their size-dependent distribution depths and feeding behaviors, no correlations in the δ13C values among size classes of largeheads were inconsistent with the stable isotope and/or stomach content results indicated by several previous studies (Pinnegar and Polunin, 2000; Bode et al., 2004; Collins et al., 2005; Park et al., 2018). This tendency may increase intraspecific competition and cannibalism of feeding behavior for largehead hairtails, with no size-dependent patterns in resource use. Cannibalism has been reported as a general phenomenon in the hairtail species in coastal waters to efficiently control population stock and species abundance (Lin et al., 2005; Yan et al., 2012; Mammel et al., 2022). However, in our study, such cannibalism was not observed in any size class during the stomach contents analysis of largehead hairtails (Chiou et al., 2006).
Interestingly, the significant negative correlation between the size and δ13C of largehead hairtails in May could disagree with the observations of common fishes that can feed on a variety of food types through opportunistic strategies by enhanced prey-catchability with growth (Le Loc’h et al., 2008; Boyle et al., 2012). Moreover, for some fish species, larger individuals may generally inhabit deeper waters than smaller individuals, to divide habitats and dietary resources (Adin and Mueter, 2007; Park et al., 2018). The enhanced swimming ability of largeheads with growth may allow large individuals to feed on pelagic fishes rather than on dominant zooplankton groups (Yan et al., 2011). However, the high contribution (approximately 93%) of Euphausiacea to the diet of the largest group (AL, > 15 cm) in May, observed by stomach content analysis, may support our isotopic results. Such a large proportion of Euphausiacea as a food source for largehead hairtails may result in a relationship that has lower δ13C values compared with the smaller groups and other benthic/benthopelagic feeders. In addition, the relatively high contribution and low diversity of Euphausiacea as a dietary source during a specific season can be explained by food availability due to their dense patch distribution (Martins et al., 2005), leading to a tight connection between pelagic prey sources and large individuals. Thus, despite the seasonal variability of prey composition and isotope values for largehead hairtails, Euphausiacea may be its most important dietary component in the South Sea of Korea.
4.3. Seasonal and size-related variability in the TPs of largehead hairtail
The TP value of a species is generally influenced by changes in prey items, feeding strategies, and environmental factors (Lorrain et al., 2015; Park et al., 2018; Wang et al., 2022). The δ15N values of fish consumers based on the enrichment of 15N due to prey-predator interactions have been used for the calculation of TP (Post, 2002). Most fish species commonly have a positive relationship between body length and TP, resulting from increased δ15N values as they grow (Pinnegar and Polunin, 2000). Our study also showed positive relationships between TPs and ALs in each season, except in winter, suggesting an ontogenetic change in largehead hairtail diets with increasing body length. However, the seasonal TPs of this species may not be affected by general size, because of the discrepancy in the AL distributions and TPs between February (11.6 ± 4.0 cm and 3.4 ± 0.4, respectively) and August (17.0 ± 3.2 cm and 2.8 ± 0.2, respectively). The relatively high TPs for largehead hairtails in February with small ALs likely resulted from the large contribution of Pisces to their diets, as observed by stomach analysis. Moreover, temporal variation in the δ15N values of trophic baselines (i.e., phytoplankton) has been previously reported in coastal waters of temperate regions (Fry and Sherr, 1984; Kang et al., 2009), likely resulting in seasonal variability in the δ15N values of dominant zooplankton groups due to trophic interactions, as shown by our isotopic results. The seasonal variability in the TPs of largehead hairtails in our study may be explained by differences in major prey items with seasonally different δ15N values.
5. Conclusion
Our study revealed the seasonal and size-related patterns in the feeding strategy and dietary composition of largehead hairtails in the South Sea of Korea, exhibiting temporal contrasting differences in the major prey items of Pisces and Euphausiacea, and ontogenetic dietary changes through the combination of stomach and stable isotope analyses. The feeding ecology of largehead hairtails may be opportunistic carnivorous feeding on available dietary species. Stomach content analysis showed no evidence of cannibalism throughout all seasons. Our isotopic results showed greater 13C- and 15N-depleted signatures in largehead hairtails than in other large carnivorous fishes, suggesting a high consumption of dietary items with pelagic source affinity. Similarly, this species had intermediate or lower TP values than pelagic and benthic fishes or other fishes. Overall, our study provides scientific evidence on the feeding characteristics of the highly commercially important fish species largehead hairtails in the western North Pacific, which improves our understanding of their conservation and ecological-based management.
Data availability statement
The original contributions presented in the study are included in the article/supplementary material. Further inquiries can be directed to the corresponding author.
Ethics statement
The animal study was reviewed and approved by Ministry of Oceans and Fisheries, South Korea.
Author contributions
DS and HJP designed the experiments and drafted the manuscript. DS, S-JL, and SK designed field collection and analyzed faunal assemblages. JMJ analyzed the fish stomach contents. THP and C-IL performed the statistical analyses. DS, THP, and HJP wrote the main manuscript text. HJP supervised all processes. All authors analyzed the data and reviewed the manuscript. All authors have read and agreed to the published version of the manuscript.
Funding
This research was supported by the National Institute of Fisheries Science, Korea (R2022030) and the National Research Foundation of Korea (NRF- 2021R1A2C1012537), Ministry of Science and ICT, Korea.
Conflict of interest
The authors declare that the research was conducted in the absence of any commercial or financial relationships that could be construed as a potential conflict of interest.
Publisher’s note
All claims expressed in this article are solely those of the authors and do not necessarily represent those of their affiliated organizations, or those of the publisher, the editors and the reviewers. Any product that may be evaluated in this article, or claim that may be made by its manufacturer, is not guaranteed or endorsed by the publisher.
References
Adin K., Mueter F. (2007). The Bering Sea−A dynamic food web perspective. Deep-Sea Res. Part II. 54, 25012525. doi: 10.1016/j.dsr2.2007.08.022
Baker R., Buckland A., Sheaves M. (2014). Fish gut content analysis: robust measures of diet composition. Fish Meter 15, 170177. doi: 10.1111/faf.12026
Bakhoum S. A. (2007). Diet overlap of immigrant narrow–barred Spanish mackerel scomberomorus commerson (Lac. 1802) and the largehead hairtail ribbonfish Trichiurus lepturus (L. 1758) in the Egyptian Mediterranean coast. Anim. Biodiv. Conserv. 30, 147–160.
Beverton R. J. H., Holt S. J. (1957). Fisheries investigations: Vol. 19. on the dynamics of exploited fish populations (London, UK: Ministry of Agriculture).
Bode A., Alvarez-Ossorio M. T., Carrera P., Lorenzo J. (2004). Reconstruction of trophic pathways between plankton and the north Iberian sardine (Sardina pilchardus) using stable isotopes. Sci. Mar. 68, 165–178. doi: 10.3989/scimar.2004.68n1165
Boyle M. D., Ebert D. A., Cailliet G. M. (2012). Stable-isotope analysis of a deep-sea benthic-fish assemblage: evidence of an enriched benthic food web. J. Fish Biol. 80, 1485–1507. doi: 10.1111/j.1095-8649.2012.03243.x
Buckland A., Baker R., Loneragan N., Sheaves M. (2017). Standardising fish stomach content analysis: The importance of prey condition. Fish. Res. 196, 126–140. doi: 10.1016/j.fishres.2017.08.003
Cha H. K., Lee D. W. (2004). Reproduction of hairtail, Trichiurus lepturus Linnaeus, in Korea waters. - maturation and spawning. J. Korean Soc Fish. Res. 6, 54–62.
Chiang W. C., Chang C. T., Madigan D. J., Carlisle A. B., Musyl M. K., Chang Y. C., et al. (2020). Stable isotope analysis reveals feeding ecology and trophic position of black marlin off eastern Taiwan. Deep-Sea Res. Part II. 175, 104821. doi: 10.1016/j.dsr2.2020.104821
Chiou W. D., Chen C. Y., Wang C. M., Chen C. T. (2006). Food and feeding habits of ribbonfish Trichiurus lepturus in coastal waters of south-western Taiwan. Fisheries Sci. 72, 373–381. doi: 10.1111/j.1444-2906.2006.01159.x
Collins M. A., Bailey D. M., Ruxton G. D., Priede I. G. (2005). Trends in body size across an environmental gradient: A differential response in scavenging and non-scavenging demersal deep-sea fish. Proc. R. Soc B: Biol. Sci. 272, 2051–2057. doi: 10.1098/rspb.2005.3189
Darnaude A. M. (2005). Fish ecology and terrestrial carbon use in coastal areas: implications for marine fish production. J. Anim. Ecol. 74, 864–876. doi: 10.1111/j.1365-2656.2005.00978.x
Davenport S., Bax N. J. (2002). A trophic study of a marine ecosystem off southeastern Australia using stable isotopes of carbon and nitrogen. Can. J. Fish. Aquat. Sci. 59, 514530. doi: 10.1139/f02-031
Davis A. M., Blanchette M. L., Pusey B. J., Jardine T. D., Pearson R. G. (2012). Gut content and stable isotope analyses provide complementary understanding of ontogenetic dietary shifts and trophic relationships among fishes in a tropical river. Freshw. Biol. 57, 2156–2172. doi: 10.1111/j.1365-2427.2012.02858.x
Deb D. (1997). Trophic uncertainty vs parsimony in food web research. Oikos 122, 191–194. doi: 10.2307/3545816
DeNiro M. J., Epstein S. (1978). Influence of diet on the distribution of carbon isotopes in animals. Geochim. Cosmochim. Acta 42, 495506. doi: 10.1016/0016-7037(78)90199-0
Estupiñán-Montaño C., Galván-Magaña F., Elorriaga-Verplancken F., Zetina-Rejón M. J., Sánchez-González A., Polo-Silva C. J., et al. (2021). Ontogenetic feeding ecology of the scalloped hammerhead shark Sphyrna lewini in the Colombian Eastern tropical pacific. Mar. Ecol. Prog. Ser. 663, 127–143. doi: 10.3354/meps13639
FAO (Food and Agriculture Organization) (2019). Food and agriculture organization of the united nations (Rome, Italy: Food and Agriculture Organization).
Fry B., Sherr E. B. (1984). δ13C measurements as indicators of carbon flow in marine and freshwater ecosystems. Cont. Mar. Sci. 27, 1347. doi: 10.1007/978-1-4612-3498-2_12
Graham B. S., Grubbs D., Holland K., Popp B. N. (2007). A rapid ontogenetic shift in the diet of juvenile yellowfin tuna from Hawaii. Mar. Biol. 150, 647–658. doi: 10.1007/s00227-006-0360-y
Hamaoka H., Miyazaki H., Nanko T., Akamatsu T., Shibata J., Omori K. (2014). Spatial variation in feeding habits and carbon source of cutlassfish Trichiurus japonicus in the Western seto inland Sea, Japan. Aquaculture Sci. 62, 243–251. doi: 10.11233/aquaculturesci.62.243
He L., Zhang A., Weese D., Li S., Li J., Zhang J. (2014). Demographic response of cutlassfish (Trichiurus japonicus and T. nanhaiensis) to fluctuating palaeo-climate and regional oceanographic conditions in the China seas. Sci. Rep. 4, 1–10. doi: 10.1038/srep06380
Hyslop E. J. (1980). Stomach contents analysis–a review of methods and their application. J. Fish Biol. 17, 411–429. doi: 10.1111/j.1095-8649.1980.tb02775.x
Jennings S., Greenstreet S. P. R., Hill L., Pet G. J., Pinnegar J. K., Warr K. J. (2002). Long-term trends in the trophic structure of the north Sea fish community: evidence from stable-isotope analysis, size-spectra and community metrics. Mar. Biol. 141, 10851097. doi: 10.1007/s00227-002-0905-7
Kang C. K., Choy E. J., Hur Y. B., Myeong J. I. (2009). Isotopic evidence of particle size-dependent food partitioning in cocultured sea squirt Halocynthia roretzi and pacific oyster Crassostrea gigas. Aquat. Biol. 6, 289–302. doi: 10.3354/ab00126
Kim J. Y., Kang Y. S., Oh H. J., Suh Y. S., Hwang J. D. (2005). Spatial distribution of early life stages of anchovy (Engraulis japonicus) and hairtail (Trichiurus lepturus) and their relationship with oceanographic features of the East China Sea during the 1997–1998 El niño event. Estuar. Coast. Shelf Sci. 63, 13–21. doi: 10.1016/j.ecss.2004.10.002
Kim H. J., Park J. H., Kwon D. H., Kim Y. (2020). Maturation and spawning of largehead hairtail Trichiurus japonicus near jeju island, Korea. Korean J. Fish. Aquat. Sci. 53, 1–8. doi: 10.5657/KFAS.2020.0001
KOSIS (Korean Statistical Information Service) (2019). Fishery production survey. (Daejeon, Korea: Korea Statistics)
Layman C., Araujo M. S., Boucek R., Hammerschlag-Peyer C. M., Harrision E., Jud Z. R., et al. (2012). Applying stable isotopes to examine food-web structure: an overview of analytical tools. Biol. Rev. 87, 545562. doi: 10.1111/j.1469-185X.2011.00208.x
Lee S. C. (1978). Food and feeding habits of ribbonfishes, Trichiurus japonicus and T. lepturus. Bull. Inst. Zool. Acad. Sinica. 17, 117124.
Le Loc’h F., Hily C., Grall J. (2008). Benthic community and food web structure on the continental shelf of the bay of Biscay (North Eastern Atlantic) revealed by stable isotopes analysis. J. Mar. Syst. 72, 1734. doi: 10.1016/j.jmarsys.2007.05.011
Lin L. S., Cheng J. H., Ling J. Z. (2007). Analysis on recent status of the bottom trawl fishery resources in the East China Sea region. Mar. Fish. 29, 371374. doi: 10.13233/j.cnki.mar.fish.2007.04.016
Link J. S., Garrison L. P. (2002). Trophic ecology of Atlantic cod Gadus morhua on the northeast US continental shelf. Mar. Ecol. Prog. Ser. 227, 109–123. doi: 10.3354/meps227109
Lin L. S., Yan L. P., Ling J. Z., Liu Y., Zhou R. K. (2005). Food habits of hairtail in the East China Sea region. Mar. Fish 27, 187192.
Liu Y., Cheng J., Chen Y. (2009). A spatial analysis of trophic composition: a case study of hairtail (Trichiurus japonicus) in the East China Sea. Hydrobiologia 632, 7990. doi: 10.1007/s10750-009-9829-2
Lorrain A., Graham B. S., Popp B. N., Allain V., Olson R. J., Hunt B. P. V., et al. (2015). Nitrogen isotopic baselines and implications for estimating foraging habitat and trophic position of yellowfin tuna in the Indian and pacific oceans. Deep-Sea Res. Part II. 113, 188198. doi: 10.1016/j.dsr2.2014.02.003
Mammel M., Wang Y. C., Lan Y. C., Hsu C. M., Lee M. A., Liao C. H. (2022). Ontogenetic diet shifts and feeding dynamics of Trichiurus japonicus temminck & schlegel 1844, off guishan island, southern East China Sea. Reg. Stud. Mar. Sci. 49, 102104. doi: 10.1016/j.rsma.2021.102104
Martins A., Haimovici M., Palacios R. (2005). Diet and feeding of the cutlassfish Trichiurus lepturus in the subtropical convergence ecosystem of southern Brazil. J. Mar. Biol. Assoc. U. K. 85, 1223. doi: 10.1017/S002531540501235X
Michener R. H., Schell D. M. (1994). “Stable isotope ratios as tracers in marine aquatic food webs,” in Stable isotopes in ecology and environmental science. Eds. Lajtha K., Michener R. H. (Oxford: Blackwell Scientific Publications), 138157.
Minagawa M., Wada E. (1984). Stepwise enrichment of 15N along food chains: further evidence and the relation between δ15N and animal age. Geochim. Cosmochim. Acta 48, 11351140. doi: 10.1016/0016-7037(84)90204-7
Mohammed Koya K., Vase V. K., Abdul Azeez P., Sreenath K. R., Dash G., Bharadiya Sangita A., et al. (2018). Diet composition and feeding dynamics of Trichiurus lepturus Linnaeus 1758 off Gujarat, north-west coast of India. Indian J. Fish. 65, 5057. doi: 10.21077/ijf.2018.65.2.69184-06
Nakamura I., Parin N. V. (1993). “Snake mackerels and cutlassfishes of the world,” in FAO fisheries synopsis, vol. 15. (Rome, Italy: Food and Agriculture Organization).
Park T. H., Lee C. I., Kang C. K., Kwak J. H., Lee S. H., Park H. J. (2020). Seasonal variation in food web structure and fish community composition in the East/Japan sea. Estuar. Coast. 43, 615629. doi: 10.1007/s12237-019-00530-4
Park H. J., Park T. H., Lee C. I., Kang C. K. (2018). Ontogenetic shifts in diet and trophic position of walleye pollock, Theragra chalcogramma, in the western East Sea (Japan Sea) revealed by stable isotope and stomach content analyses. Fish. Res. 204, 297304. doi: 10.1016/j.fishres.2018.03.006
Persson L., De Roos A. M. (2006). Food-dependent individual growth and population dynamics in fishes. J. Fish Biol. 69, 120. doi: 10.1111/j.1095-8649.2006.01269.x
Pinkas L., Oliphant M. S., Iverson I. L. K. (1971). Food habits of albacore, bluefin tuna and bonito in California waters. Fish. Bull. 152, 1–105.
Pinnegar J. K., Polunin N. V. C. (2000). Contributions of stable-isotope data to elucidating food webs of Mediterranean rocky littoral fishes. Oecologia 122, 399409. doi: 10.1007/s004420050046
Polunin N. V. C., Morales-nin B., Pawsey W. E., Cartes J. E., Pinnegar J. K., Moranta J. (2001). Feeding relationships in Mediterranean bathyal assemblages elucidated by stable nitrogen and carbon isotope data. Mar. Ecol. Prog. Ser. 220, 1323. doi: 10.3354/meps220013
Post D. M. (2002). Using stable isotopes to estimate trophic position: models, methods, and assumptions. Ecology 83, 703718. doi: 10.1890/0012-9658(2002)083[0703:USITET]2.0.CO;2
Post D. M., Layman C. A., Arrington D. A., Takimoto G., Quattrochi J., Montaña C. G. (2007). Getting to the fat of the matter: models, methods and assumptions for dealing with lipids in stable isotope analyses. Oecologia 152, 179189. doi: 10.1007/s00442-006-0630-x
Santos A. R., Trueman C., Connolly P., Rogan E. (2013). Trophic ecology of black scabbardfish, Aphanopus carbo in the NE Atlantic–assessment through stomach content and stable isotope analyses. Deep-Sea Res. Part I. 77, 110. doi: 10.1016/j.dsr.2013.02.009
Sun P., Chen Q., Fu C., Xu Y., Sun R., Li J., et al. (2020). Latitudinal differences in early growth of largehead hairtail (Trichiurus japonicus) in relation to environmental variables. Fish. Oceanogr. 29, 470483. doi: 10.1111/fog.12490
Wang L., Liu Y., Zhang X. (2022). Stable isotope analysis revealed ontogenetic changes in trophic ecology and migration patterns of Sepia esculenta in the northern coastal waters of China. Front. Mar. Sci. 9, 818088. doi: 10.3389/fmars.2022.818088
Yan Y., Chen J., Lu H., Hou G., Lai J. (2012). Feeding habits and ontogenetic diet shifts of hairtail, Trichiurus margarites, in the beibu gulf of the south China Sea. Acta Ecol. Sin. 32, 1825. doi: 10.1016/j.chnaes.2011.04.008
Keywords: family Trichiuridae, stomach contents, δ13C, δ15N, trophic position, ontogenetic dietary change
Citation: Shin D, Park TH, Lee C-Il, Jeong JM, Lee S-J, Kang S and Park HJ (2022) Trophic ecology of largehead hairtail Trichiurus japonicus in the South Sea of Korea revealed by stable isotope and stomach content analyses. Front. Mar. Sci. 9:910436. doi: 10.3389/fmars.2022.910436
Received: 01 April 2022; Accepted: 28 June 2022;
Published: 27 July 2022.
Edited by:
Johan Verreth, Wageningen University and Research, NetherlandsReviewed by:
Alberto Sánchez-González, Instituto Politécnico Nacional (IPN), MexicoChang-Keun Kang, Gwangju Institute of Science and Technology, South Korea
Copyright © 2022 Shin, Park, Lee, Jeong, Lee, Kang and Park. This is an open-access article distributed under the terms of the Creative Commons Attribution License (CC BY). The use, distribution or reproduction in other forums is permitted, provided the original author(s) and the copyright owner(s) are credited and that the original publication in this journal is cited, in accordance with accepted academic practice. No use, distribution or reproduction is permitted which does not comply with these terms.
*Correspondence: Hyun Je Park, phj13579@gwnu.ac.kr
†These authors have contributed equally to this work