- 1Department of Biological Sciences, National University of Singapore, Singapore, Singapore
- 2Department of Biosciences, Faculty of Science and Engineering, Swansea University, Swansea, United Kingdom
Increasing incidence of severe coral bleaching events caused by climate change is contributing to extensive coral losses, shifts in species composition and widespread declines in the physical structure of coral reef ecosystems. With these ongoing changes to coral communities and the concomitant flattening of reef structural complexity, understanding the links between coral composition and structural complexity in maintaining ecosystem functions and processes is of critical importance. Here, we document the impacts of the 2016 global-scale coral bleaching event on seven coral reefs in Singapore; a heavily degraded, turbid reef system. Using a combination of field-based surveys, we examined changes in coral cover, composition and structural complexity before, during and after the 2016 bleaching event. We also quantified differential bleaching responses and mortality among coral taxa and growth forms using a bleaching response index. Elevated SSTs induced moderate to severe coral bleaching across reefs in Singapore in July 2016, but low overall coral mortality (~12% of colonies). However, we observed high bleaching prevalence and post-bleaching mortality of the three most abundant coral genera (Merulina, Pachyseris and Pectinia), all generalists, declined significantly across reefs between March and November 2016. Four months post-bleaching (November 2016), small-scale structural complexity declined across all Singaporean reefs and no moderately complex reefs remained. Importantly, reductions in structural complexity occurred across reefs with a large range of live coral cover (19–62%) and was linked to the loss of dominant coral genera with low-profile foliose-laminar growth forms which resulted in flatter, less structurally complex reefs. And while generalist coral taxa remain highly competitive within Singapore’s reef environment, they may not have the capacity to maintain structural complexity or ensure the persistence of other reef functions, even within communities with high coral cover. The widespread loss of structurally complexity on Singapore’s degraded coral reefs may further impair ecosystem functioning, potentially compromising the long-term stability of reef biodiversity and productivity.
Introduction
Coral reefs, like many high-diversity tropical ecosystems, are threatened by a broad range of anthropogenic stressors (Barlow et al., 2018). Of greatest concern is increasing climate-driven thermal stress events that cause mass coral bleaching events, threatening coral reefs globally (Hughes et al., 2018a). Thermal stress events disrupt the relationship between corals and their photosynthetic endosymbionts (Symbiodinium spp.), causing corals to lose their colour or “bleach” (Glynn, 1983). Bleached corals are physiologically damaged and nutritionally comprised, and prolonged bleaching often leads to extensive coral mortality and reductions in live coral cover or abundance across large regions (e.g., Baird and Marshall, 2002; Hughes et al., 2018b). Impacts from coral bleaching events can be long lasting (e.g., Burt et al., 2011) and may alter species composition (Pratchett et al., 2011; Hughes et al., 2018b), recruitment (Burt and Bauman, 2020) and calcification rates (Perry and Morgan, 2017) resulting in the homogenization of coral assemblages (e.g., Bento et al., 2016) and loss of key ecological functions (Alvarez-Filip et al., 2013). Notably, climate induced bleaching events are already altering the structure and function of coral reefs (Hughes et al., 2018b) thereby jeopardizing critical ecosystem goods and services that reefs provide (Pratchett et al., 2014; Harris et al., 2018).
Severe coral bleaching events can also affect the structural complexity (or physical three-dimensional configuration) of coral reefs due to the loss of corals and shifts in community composition (e.g., Berumen and Pratchett, 2006; Alvarez-Filip et al., 2013). Structurally complex reefs typically support more diverse and abundant biological communities (Graham and Nash, 2013) by mediating competition and predation, and facilitating cohabitation of an increased number of species (e.g., Hixon and Beets, 1993; Syms and Jones, 2000). Maintaining structural complexity is also a key factoring determining reef recovery following a disturbance (Graham et al., 2015). However, as corals die and their skeletons erode, the structural complexity of reef habitats decline (Alvarez-Filip et al., 2009), which can have important consequences for the biodiversity (Graham and Nash, 2013) productivity (Darling et al., 2017) and associated ecosystem services of coral reefs (Alvarez-Filip et al., 2013; Perry and Morgan, 2017). Moreover, shifts in the composition and dominance of coral assemblages can result in rapid losses in coral community calcification rates and reef rugosity (Alvarez-Filip et al., 2013). Thus, even on reefs where corals remain the dominant taxa following a bleaching event, changes in the relative abundance of local coral taxa can impact the structural complexity and function of reefs (Berumen and Pratchett, 2006; Alvarez-Filip et al., 2011a).
Coral bleaching affects all scleractinian corals, however, susceptibility and mortality varies greatly among coral taxa (Marshall and Baird, 2000; Loya et al., 2001). Following a bleaching event, the proportion of colonies that bleach and die is invariably higher for some coral taxa (e.g. Acropora, Seriatopora, Stylophora) than others (e.g. Cyphastrea and Heliofungia) that rarely bleach and die (e.g., McClanahan et al., 2004; but see Pratchett et al., 2020). Taxonomic variation in bleaching susceptibility has generally been attributed to differences in physiological and morphological traits (e.g. Loya et al., 2001; Baird and Marshall, 2002) and in growth form (Darling et al., 2013) which directly dictate life history strategies (Jackson, 1979). Differential susceptibility among taxa and life history strategies, as well as variation in recovery potential, are causing shifts in community composition from competitive, reef-building species towards those with stress-tolerant and/or weedy life histories (van Woesik et al., 2011; Darling et al., 2013). These taxonomic shifts are resulting in structurally simplified (or flatter) reef assemblages (Alvarez-Filip et al., 2009) with lower carbonate production (Januchowski-Hartley et al., 2017) and vertical growth rates (Perry et al., 2018) that together impact reef functionality (e.g., Alvarez-Filip et al., 2013). Importantly, such community changes are predicted to persist and/or increase in the future as bleaching events become more frequent and intense (e.g., Hughes et al., 2017).
Reduction in and/or the loss of reef structural complexity following severe bleaching was initially reported to occur over timescales of 3–5 years (e.g., Graham et al., 2007; Alvarez-Filip et al., 2011b), but is now occurring more rapidly (Eakin et al., 2019). Recent bleaching studies report that the structure of both individual coral skeletons (Leggat et al., 2019) and entire reefs (Couch et al., 2017; Magel et al., 2019) are rapidly eroding over short timeframes (i.e., months to <1 year) causing abrupt shifts on reefs toward net erosion (Perry and Morgan, 2017). For example, Couch et al. (2017) reported a 99% reduction in structural complexity on bleached reefs in the Northwestern Hawaiian Islands <1 year after bleaching. Further, these structural losses are compounded by increases in the abundance of bioeroding organisms (Carballo et al., 2013). Leggat et al. (2019), for example, found rapid coral skeleton dissolution on the Great Barrier Reef (GBR) linked to bleaching conditions where endolithic cyanobacteria quickly grow, perforating and weakening the outer portion of coral skeleton within days to weeks after mortality. If these findings are representative, then the speed of structural changes driven by bleaching mortality, and the associated loss ecosystem functions, are likely being underestimated (Graham et al., 2015; Couch et al., 2017; Eakin et al., 2019).
In many regions, structural complexity is not regularly assessed or monitored on reefs prior to or following a bleaching event (Magel et al., 2019; Carlot et al., 2020) including highly diverse coral reef communities in Southeast Asia (Huang et al., 2015). Reefs in Southeast Asia are among the most heavily impacted in the world having experienced significant ecological declines (Burke et al., 2011). Regional declines in coral cover and species diversity from coastal development, pollution, and overfishing (Heery et al., 2018) are increasingly being compounded by coral bleaching events (e.g., Guest et al., 2012; Licuanan et al., 2019). As a result, community composition on many reefs across the region have shifted towards stress-tolerant and generalist taxa (e.g., Cleary et al., 2016; Guest et al., 2016), influencing rates of reef calcification and bioconstruction (Perry et al., 2018; Januchowski-Hartley et al., 2020), and driving increased vertical reef compression (Morgan et al., 2020). Yet, few studies have quantified how local taxonomic composition and associated growth forms affect reef structural complexity following a bleaching event (McCowan et al., 2012; Denis et al., 2017). Morphological diversity is the foundation of complex reef habitats (Graham and Nash, 2013) and a good predictor of coral assemblage responses to a disturbance (Darling et al., 2012) and recovery following a bleaching event (Graham et al., 2015). Coral growth forms contribute greatly to the overall structural complexity and resilience on reefs by influencing growth rates and demographic processes (e.g., Álvarez-Noriega et al., 2016). As such, coral growth forms are considered one of the most relevant indicators of reef condition and a major determinant of reef functioning (i.e., the coral’s ability to maintain a functional habitat; Denis et al., 2017).
Between 2015–2017, corals reefs around the world experienced high sea-surface temperatures that triggered the third global mass coral bleaching event (Hughes et al., 2017; Eakin et al., 2019). This study investigates the impacts of a severe bleaching event that occurred on reefs in the Southern Islands of Singapore subjected to unprecedented heat stress lasting three months from mid-April to mid-July 2016 (NOAA, 2016). Using a combination of field-based surveys, we examined changes in coral cover, community composition and structural complexity among coral assemblages before, during and after the 2016 event. We also quantified differential bleaching-responses and mortality amongst coral taxa and growth forms and examined their subsequent impacts on reef structural complexity.
Materials and Methods
Study Site
This study was conducted between March and November 2016 on seven offshore coral reefs along the southern coast of Singapore (Figures 1A, B). All reefs consist of a reef flat that leads seaward to the upper reef slope, which descends down to ~8 m depth. Coral cover is generally limited to a narrow strip between the lower reef flat and upper reef slope from 3 to 6 m depth (Guest et al., 2016). This narrow coral band (or ‘reef compression’ sensuHeery et al., 2018) is characteristic of most Singapore reefs and is primarily due to the dominance of fleshy macroalgae on the upper reef flats (~1–2 m, Low et al., 2019) and extreme light attenuation with increasing depth from chronic high sediment deposition (Morgan et al., 2020). Benthic community and coral bleaching surveys were initially conducted in March 2016 (pre-bleaching) as part of longer-term monitoring, and then in July 2016 (during bleaching) and November 2016 (post-bleaching) in response to the 2014–2017 global scale coral bleaching event (Eakin et al., 2019).
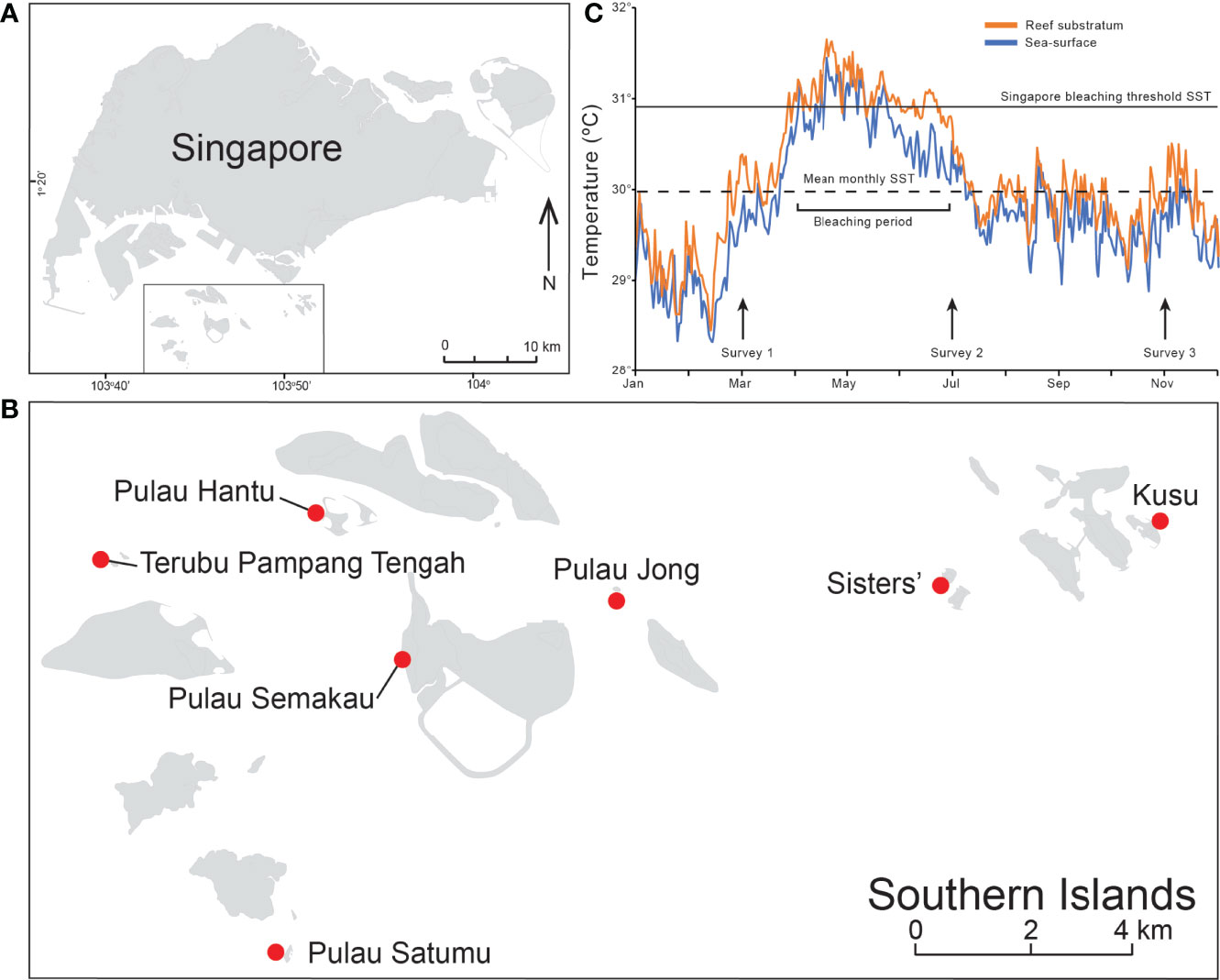
Figure 1 National setting showing (A) Singapore and (B) and the seven reefs sites in the Southern Islands (from west to east: Terumbu Pempang Tengah (TPT), Pulau Satumu, Pulau Hantu, Pulau Semakau, Pulau Jong, Sisters’ and Kusu. (C) In situ water temperature data (orange) and satellite derived sea-surface temperature (SST) data (blue) for Singapore recorded from 1/01/16 to 12/31/16). Data extracted from the NOAA Coral Reef Watch (https://coralreefwatch.noaa.gov/product/vs/gauges/singapore.php). Arrows indicate the three survey periods in March, July and November 2016.
The NOAA Coral Reef Watch 0.05° (5 km) resolution satellite monitoring sea-surface temperature (SST) in the northern Singapore Strait indicated that SST was above the climatological average for the entirety of 2016 (Figure 1C). The SST anomaly was highest (>1.8°C) during January, while the peak temperature (in May) exceeded the expected summertime maximum monthly mean (MMM) by more than 1.2°C. SST first exceeded the MMM in early April, just after the first set of benthic surveys and was at least 1°C higher for 40% of the period from mid-April to early-June, with accumulated heat stress reaching 3.1°C-weeks on 6 June, three weeks before the second surveys. This level of heat stress indicated from the current 5 km satellite products has been demonstrated to correspond with coral bleaching, but with little-to-no coral mortality (Hughes et al., 2018a). SST remained near the MMM value through until the end of November 2016, just after the third and final benthic surveys were conducted. Sea bottom temperatures were also recorded between January 2016 and December 2016 on each reef at hourly intervals using HOBO Water Temperature Pro V2. Temperature loggers were installed ~10 cm above the reef substratum on each reef. Daily averages were calculated and used to characterize the benthic thermal environment on each reef (Figure 1C).
Benthic and Coral Assemblage Surveys
Benthic and coral assemblage composition were estimated on each reef using six replicate 30 m point intercept transects in March, July and November 2016. Transects were laid parallel to the reef crest (3–4 m depth) spaced 5 m apart, and the substrate directly beneath the transect tape was surveyed every 50 cm. The substrate was categorized into the following major benthic groups: hard corals, macroalgae (> 10 mm in height), epilithic algal matrix (≤ 10 mm in height), other sessile invertebrates (e.g., soft corals, sponges, ascidians), dead coral, and abiotic substratum (sand, rubble and pavement). All hard corals were identified to genus and growth forms recorded as branching, digitate, encrusting, free-living, foliose, laminar, massive, or submassive (Madin et al., 2016).
Structural complexity was estimated along each transect using two complementary methods: small-scale rugosity (i.e. an estimate of structural complexity based on a single measurement of vertical relief or contours among benthic organisms) and large-scale reef topography (i.e. physical three-dimensional configuration of the larger structural features of a reef). Small-scale rugosity was estimated five times along each 30 m transect using a 3 m length of fine link chain that was fitted to the contours of the reef, and measuring the distance between the start and end point to the nearest cm (Risk, 1972). We calculated the rugosity index by dividing the total length of the 3 m chain by the same chain fitted to the reef surface (i.e. contours). A rugosity index of one is equivalent to a perfectly flat surface, with larger numbers indicating a greater degree of structural complexity. Based on the rugosity index we classified each reef into one of four rugosity categories (1.0–1.49, 1.5–1.99, 2.0–2.49 and greater than 2.5) from complex (rugosity greater than 2.0) to flatter (rugosity less than or equal to 1.5) following methods by Alvarez-Filip et al. (2009).
Larger scale reef topography was visually estimated using a 6-point scale following (Polunin and Roberts, 1993) in 5 areas along each transect. Each area separated by 6 m and was assigned a score ranging from 0 (no vertical relief) to 5 (exceptionally complex habitats with numerous caves and overhangs). This measure of structural complexity has been shown to correlate well with a range of other structural complexity measurement techniques (Wilson et al., 2007) including reef recovery potential following mass bleaching events (Graham et al., 2015). All visual observations of structural complexity were recorded by a single observer (AGB).
Bleaching Surveys
Six fixed 10 × 1 m belt transects were established at each reef site to measure the impacts of bleaching on coral genera and growth forms, and surveyed during each survey period. Due to Singapore’s small reef sizes (Huang et al., 2009) belt transects were positioned immediately adjacent to the reef areas where benthic and coral assemblage were surveyed (i.e. on the reef crest at 3–4 m depth). Within each belt transect, every coral colony >5 cm in diameter was counted and identified to genus following Veron (2000) and Huang et al. (2009). The level of bleaching was scored across a five-level categorical scale modified from Guest et al. (2012): (1) no bleaching; (2) pale; (3) 1–50% bleached; (4) 50–100% bleached; and (5) recently dead. A total of 5,258 individual coral colonies were surveyed in 126 belt transects across reefs and survey periods. A taxon-specific bleaching response index (BRI) for each reef was then calculated following Swain et al. (2016).
Statistical Analyses
We assessed changes in benthic composition, percent live coral cover and growth forms across reefs over the three survey periods (March, July and November 2016) using fitted multivariate generalized linear models in the mvabund package (Wang et al., 2012). All coral genera and growth forms with <3% relative abundance were excluded from analyses to reduce the effect of rare genera and growth forms on model outputs. All models were constructed using negative binomial error distributions based on residual plots. Independent correlation structures were used to reduce computation time and enable log-likelihood ratio tests for calculating p-values calculated through PIT-trap resampling (999 iterations). In addition to testing for the overall effects of time (survey period) and reefs, models were utilized to detect the effects of time and reefs on individual components within each set of survey data (e.g., univariate p-values for Merulina sp. in the coral assemblage data). Holm’s step-down multiple testing procedure was used to control for the family-wise error rate by adjusting the univariate p-values of the individual components. As the models do not support pair-wise comparisons between treatment levels for the univariate p-values by default, pair-wise comparisons were performed by rerunning the models with a subset of the data containing only the relevant levels of interest.
Notably, during the benthic surveys, some coral colonies with horizontally spreading growth forms (i.e., foliose and laminar corals) exhibited growth forms that were not easily discernible. In order to detect potential mistakenly categorized growth forms, we reanalysed the growth form composition of individual genera with fitted multivariate generalized models as described above (i.e., negative binomial error structure with time and reefs as fixed effects) and compared the results to changes in ambiguous growth form abundance (foliose-laminar when applicable) over time and reef using fitted separate generalized liner models (GLMs) with quasi-binomially distributed errors.
Variation in small-scale rugosity across the three time periods across reefs was analysed using crossed fixed factor orthogonal analysis of variance (ANOVA). Post-hoc multiple comparisons (using the TukeyHSD function) were conducted using single step Tukey adjustments to control for family wise error rates. Rugosity values were square root transformed to meet assumptions, and validated using standard residual, normal Q-Q and mean-variance plots. Reef topography values did not meet assumptions of normality after transformation, but were homoscedastic and individual Kruskal-Wallis tests were used to test the effect of reef site and of month.
To examine changes in coral assemblages and growth form composition across reefs over time, we used taxon-specific BRI values for July, and changes in BRI values between March and July from the bleaching surveys, to identify and rank genera and growth forms that exhibited the most severe bleaching responses. A linear mixed effects model with transect as the random effect was then used to examine relationships between growth form abundance and small-scale rugosity among reefs to identify which growth forms contributed significantly to small-scale rugosity. The BRI rankings, list of coral genera and growth forms that had significantly different abundances among reefs over time, and the list of dominant growth forms among reefs, were then cross referenced to identify genera and growth forms that exhibited bleaching induced mortality and changes in small scale rugosity across the reefs. The potential for genera BRI values, and their changes during bleaching, to be good predictors of post-bleaching colony loss was also examined. We used linear regressions to examine the relationship between both July BRI values and changes in BRI values between March and July with the proportional loss of colonies between March and November.
Results
Benthic Assemblages
Between March and November 2016 benthic assemblages changed across reefs in Singapore (Table 1), with marked changes in mean coral cover (F2,117 = 7.0773, p < 0.005, Figure 2A). Coral cover declined significantly between March (40.8% ± 2.4 SE) and November (33.5% ± 2.1 SE) (t-ratio = 3.75, p < 0.001). In March 2016, mean coral cover ranged from 19.4% ( ± 3.5 SE) at P. Jong to 62.1% ( ± 3.9 SE) at P. Satumu. Regardless of initial mean coral cover, the abundance of coral declined across all reefs between March and November 2016 (Figure 2A). Pulau Hantu was the worst impacted reef; coral cover declined from 43.9% ( ± 2.6 SE) in March to 32.8% ( ± 1.6 SE) by November, a 11.1% decline in coral cover (i.e. relative loss of 25.3% cover). By contrast, there were minimal changes in coral cover on P. Jong (19.9% ± 3.2 SE to 17.2% ± 2.8 SE), TPT (41.3% ± 3.8 SE to 39.9% ± 1.4 SE) and Kusu (48.9% ± 4.8 SE to 45.1% ± 2.8 SE) over the same period (Figure 2A).
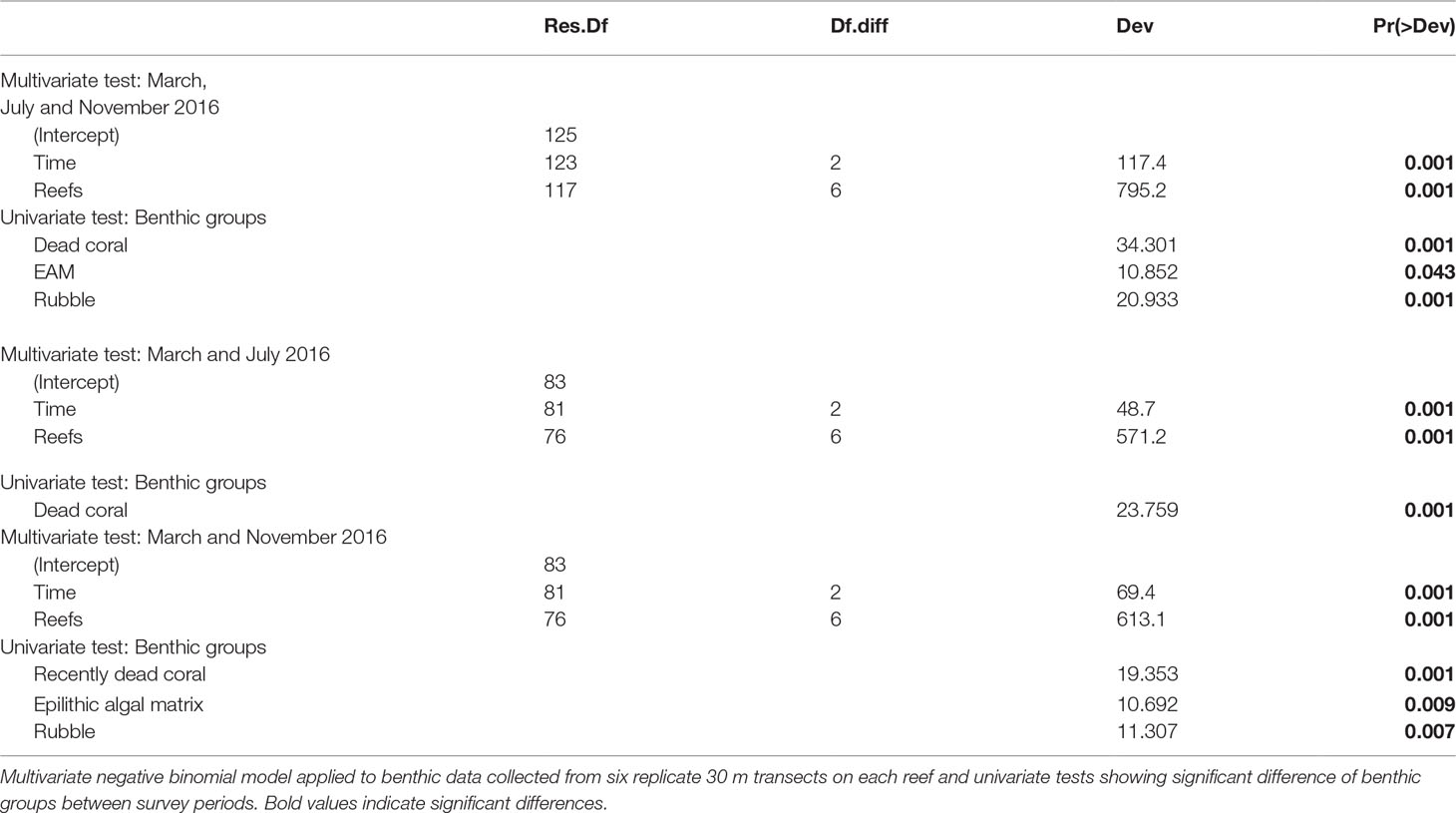
Table 1 Summary statistics for analysis of benthic assemblages pooled among reefs across months (March, July and November 2016).
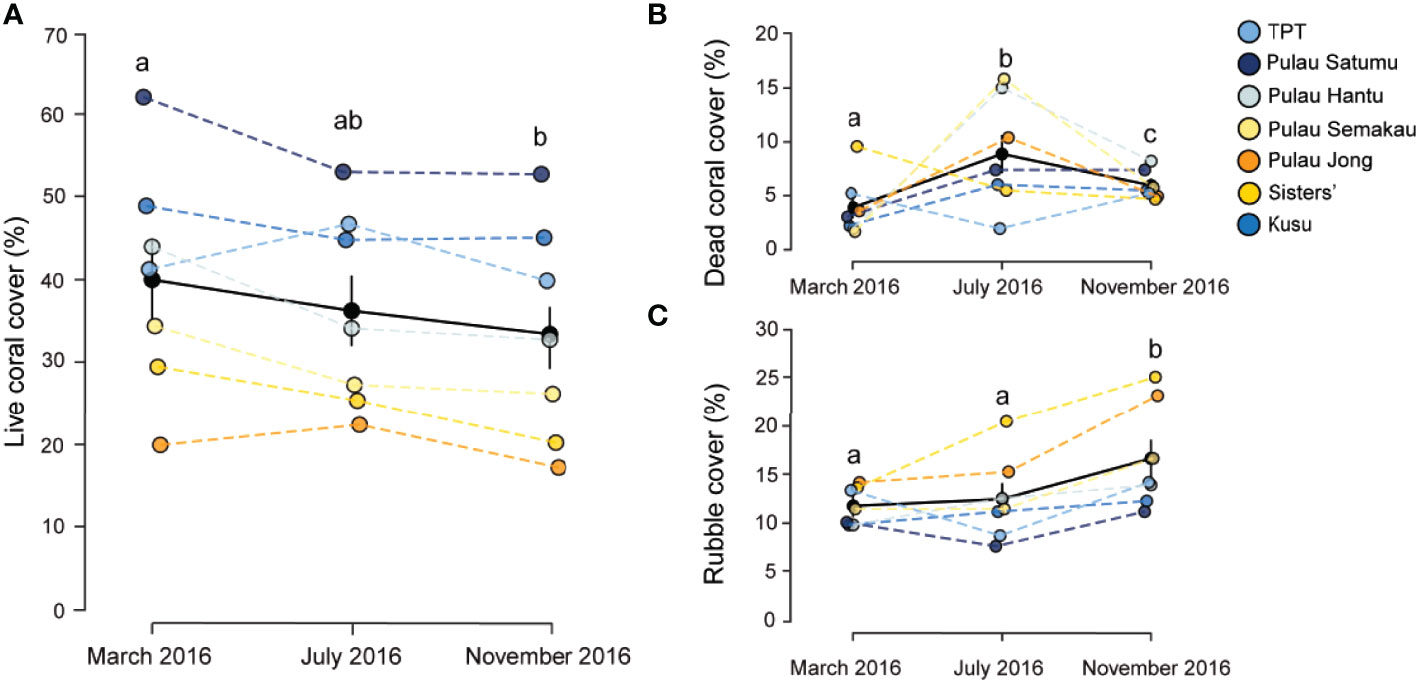
Figure 2 Mean percent cover of (A) live coral, (B) dead coral, and (C) rubble in March, July and November 2016 on the seven reefs in Singapore. Black circles represent the mean cover pooled among reefs and standard errors. Different letters above each month indicate significant differences (p < 0.05).
Concomitant with the loss of coral cover were marked and contrasting changes in dead coral and rubble (Table 1). Mean dead coral and rubble cover differed significantly across reefs among survey periods (F2,117 = 18.561, p < 0.001 and F2,117 = 19.057, p < 0.001, Figures 2B, C). Mean dead coral cover increased significantly between March and July (t-ratio = -6.063, p < 0.001) from 3.3% ( ± 0.5 SE) to 9.3% ( ± 0.8 SE) and then decreased to 5.9% ( ± 0.3 SE) in November (t-ratio = 3.553, p < 0.001). Dead coral increased at all sites except TPT and Sisters’ Island in July 2016 (Figure 2B), with highest cover of dead coral on P. Semakau (15.4% ± 0.9 SE) and P. Hantu (15.0% ± 2.1 SE) and lowest on TPT (5.2% ± 0.8 SE). Rubble significantly increased between March/July and November 2016 (t-ratio = 5.710, p < 0.001). Rubble cover across reefs in March and July 2016 was 11.2% (± 0.6 SE) and 13.1% ( ± 0.8 SE) respectively, and subsequently increased to 16.7% ( ± 1.0 SE) by November, five months after the bleaching event. Rubble cover was highest on Sisters (25.1% ± 3.4 SE) and P. Jong (23.2% ± 1.2 SE), while rubble at the other five reefs ranged from 12.3% to 16.7% (Figure 2C). Mean epilithic algal matrix cover in Singapore changed significantly between July and November (F2,117 = 69.778, p < 0.001), from 9.1% ( ± 1.6 SE) in July to 12.1% ( ± 1.4 SE), although there was no difference between cover in March (10.3% ± 1.7 SE) and either of these months. Epilithic algal matrix cover was consistently lowest at Kusu than all other reefs (Supplementary Figure 1).
Similar to coral cover there were marked changes in small-scale rugosity across reefs (F2,609 = 22.710, p < 0.001) with a significant decline in rugosity from March and July (1.69 ± 0.02) to November (1.55 ± 0.02; Figure 3). Small-scale rugosity varied significantly among reefs (F6,609 = 98.617, p < 0.001), ranging from 1.03 to 2.81. The highest initial mean rugosity was 2.17 (± 0.31) on P. Satumu and lowest rugosity was 1.44 ( ± 0.23) on P. Jong (Figure 3). There was no significant interaction between month and reef site (F12,609 = 0.412, p = 0.959). Significant positive correlations were found between small scale rugosity and the abundance of branching, digitate, foliose-laminar, massive (all p < 0.001) and plating (p < 0.05) growth forms indicating that changes in small scale rugosity were related to reductions in the abundance of foliose-laminar growth form. Reef topography did not differ across months (Kruskal Wallis χ2 = 5.155, p = 0.076), but did by reef (χ2 = 209.1, p < 0.001) with the same reefs (i.e. P. Satumu and P. Jong) having the most and least complex reef topography (2.45 ± 0.05 SE and 1.27 ± 0.05 SE, respectively).
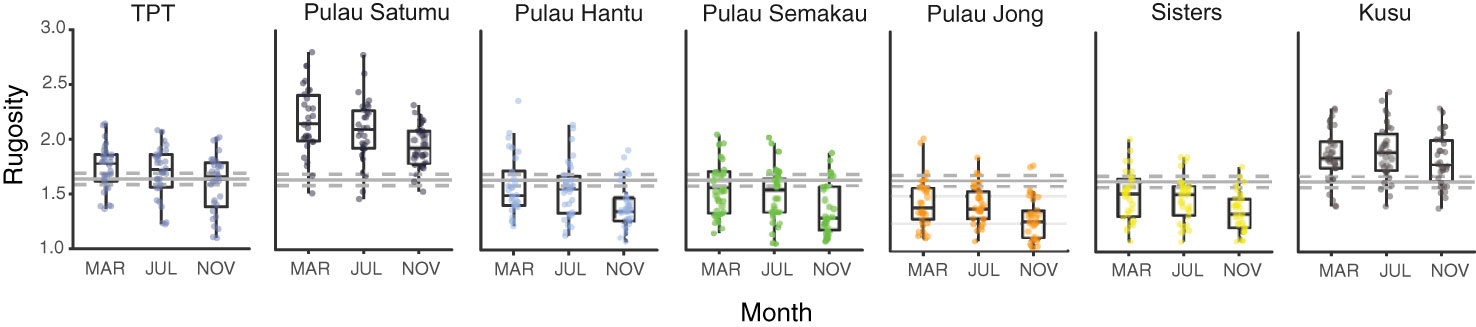
Figure 3 Box plots showing variation in small-scale rugosity among Singapore reefs in March, July and November 2016. Solid grey horizontal lines represent mean rugosity across all reefs, with dashed lines representing 95% confidence intervals over the entire study period. Rugosity estimates for each reef are based on 30 measurements each survey period.
Coral Assemblages
The 2016 bleaching event caused marked transformations in coral assemblages across reefs in Singapore (Figure 4A and Table 2), driven mainly by acute declines in the abundance of the three most abundant and dominant coral taxa, Pachyseries, Pectinia and Merulina (13.1%, 11.9% and 11.7% relative abundance, respectively; Table 2), which accounted for 36% of total coral cover across reefs. By November 2016, Pachyseris (8.6%), Pectinia (9.5%) and Merulina (3.2%) constituted 21% of the total abundance, a relative loss of 41.6%. Merulina was the worst affected coral genera across reefs (Figure 4A), declining in relative abundance by 72% (from 11.6% relative abundance in March to 3.2% by November 2016). Conversely, Montipora increased in relative abundance by 82% from 5.5% to 9.9%, while Porites abundance increased by 58% from 2.9% to 4.6% (Figure 4A), between March and November 2016. Notably, despite relatively low initial relative abundances of Acropora (1.4%) and Pocillopora (1.4%) there were marginal increases in the abundance of both genera between March and November 2016.
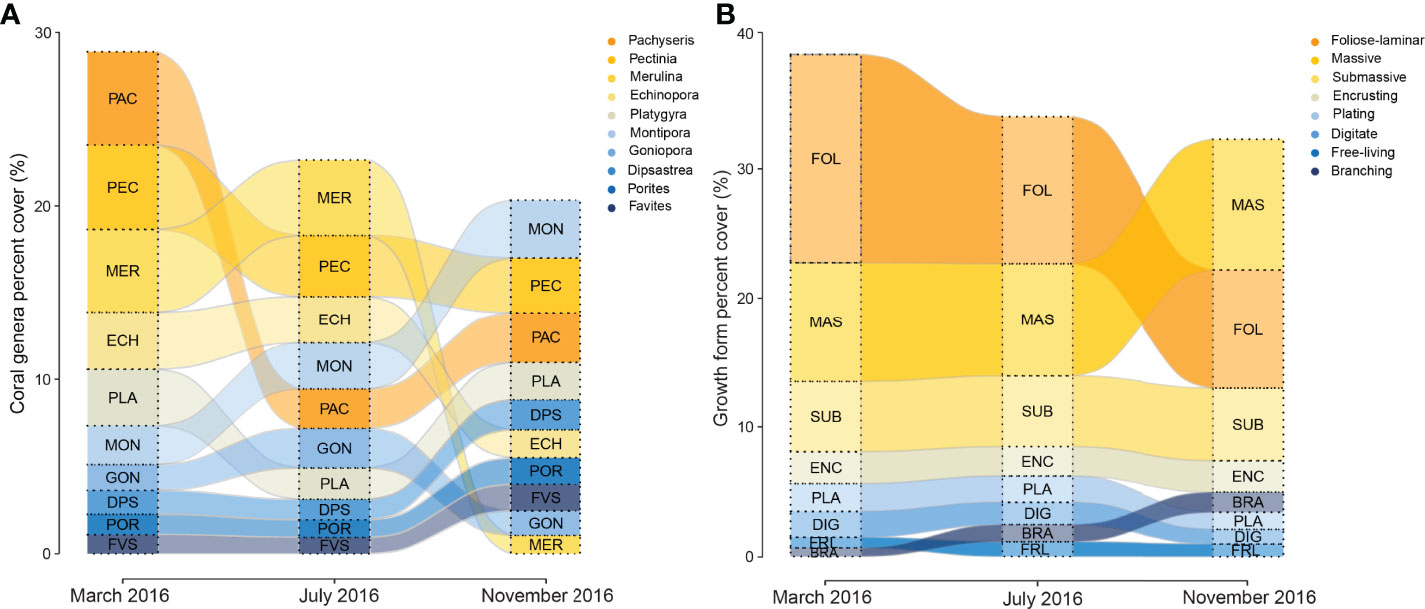
Figure 4 Variation in the proportion of the (A) 10 most abundant coral genera and, (B) eight most abundant growth forms pooled among Singapore reefs in March, July and November 2016. Genera and forms are sorted by relative abundance in each month, decreasing from top to bottom.
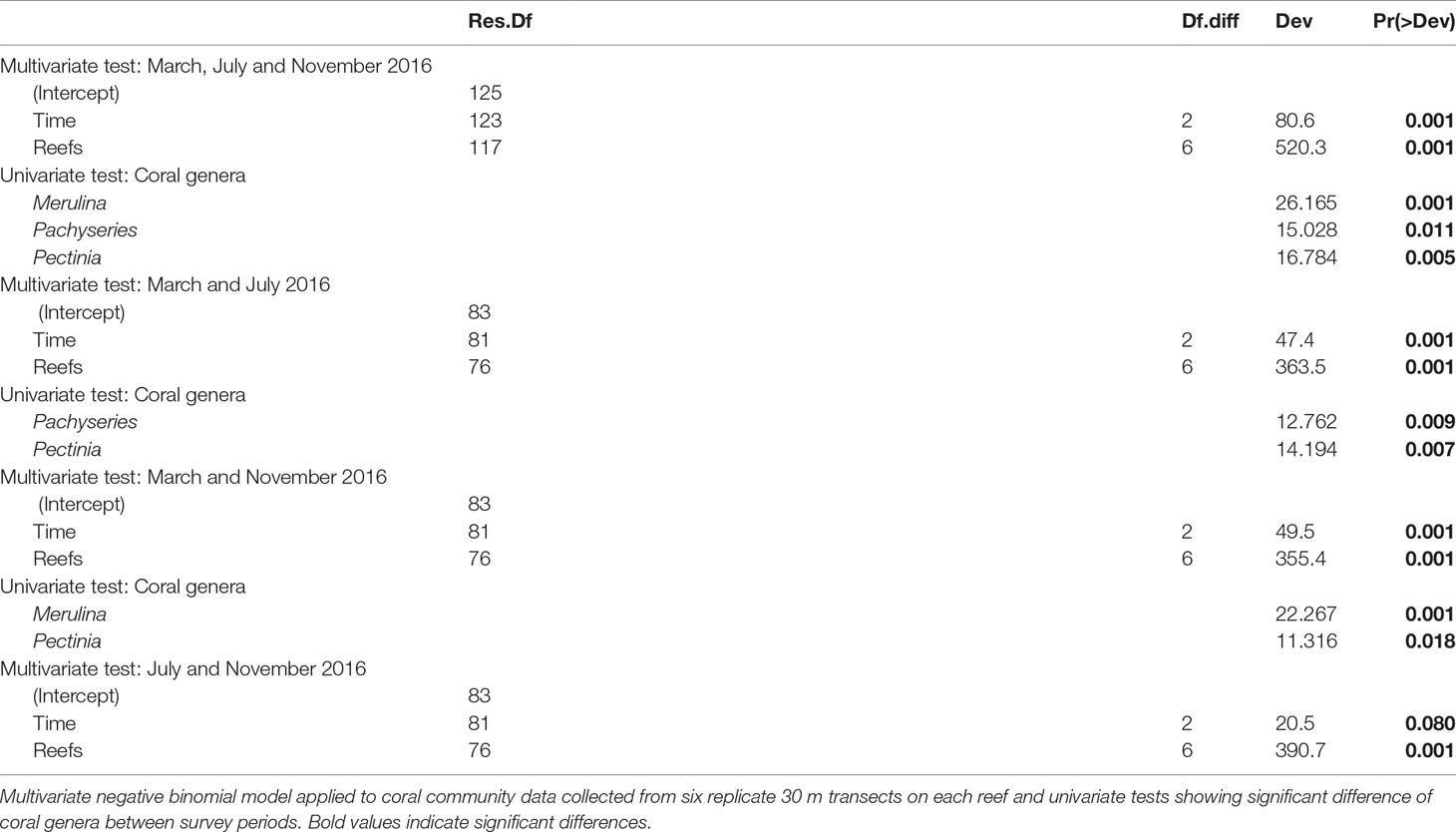
Table 2 Summary statistics for analysis of coral community composition pooled among reefs across months (March, July and November 2016).
Foliose-laminar corals were the dominant growth form across reefs, and showed marked declines from 41.3% in March to 28.3% in relative abundance by November 2016 (Figure 4B and Table 3), a loss of 31.5%. By contrast, there were moderate increases in massive, submassive and branching growth forms, which accounted for 37.6% relative abundance in March, and 51.7% in November 2016, an increase of 38%. Encrusting coral also increased slightly in abundance (from 6.0% to 7.2%), while plating, digitate and free-living corals showed little to no change across reefs during the study (Figure 4B).
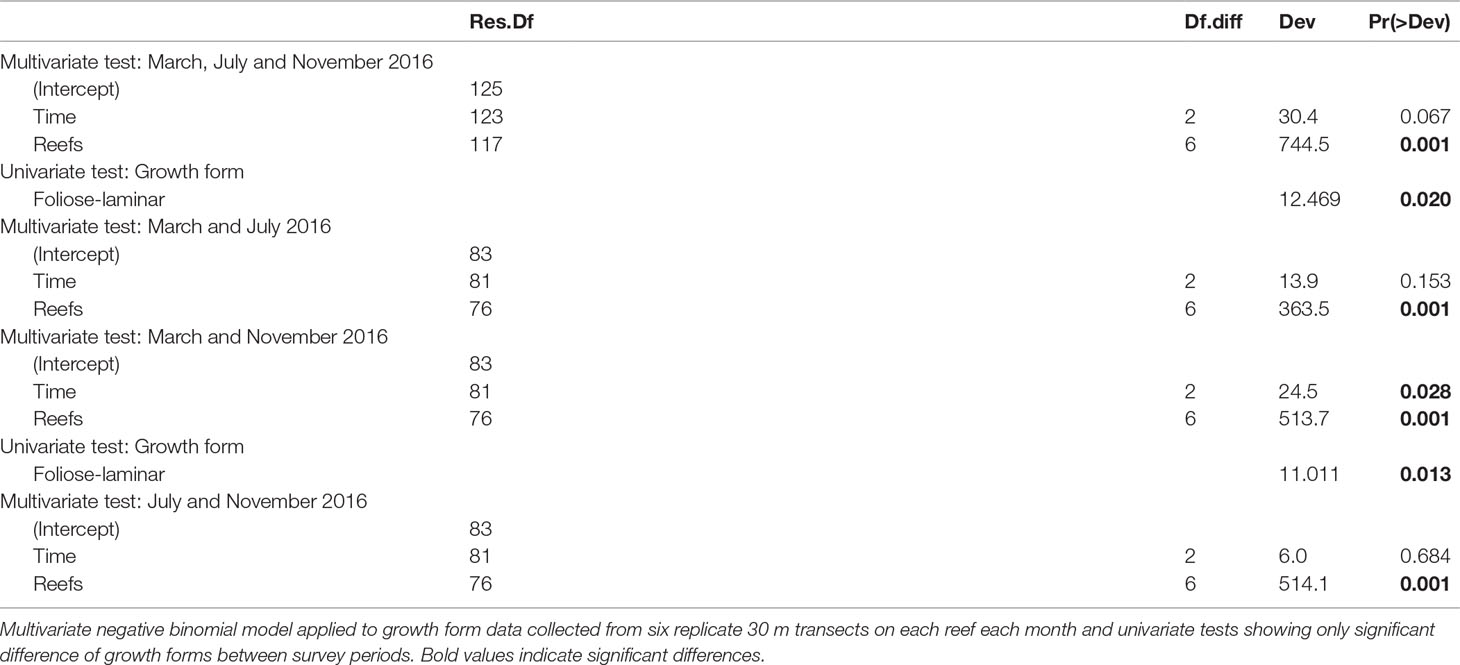
Table 3 Summary statistics for analysis of coral growth forms pooled among reefs across months (March, July and November 2016).
2016 Bleaching Event
Coral bleaching was recorded across all seven reefs in July 2016; however, the response of corals to the heat stress differed substantially between reefs, coral genera and growth forms (Figure 5 and Supplementary Figure 2). Bleaching surveys in July 2016 showed that 27% of 1648 colonies were partially or severely bleached (451 colonies) while 12% recently died (196 colonies). The proportion of bleached colonies ranged from 27% on TPT to 38% on P. Semakau (Figure 5A and Supplementary Table 1), with the highest coral mortality on P. Hantu (18% recently dead), and the lowest mortality on P. Satumu and TPT (both 9.4%, Figure 5A and Supplementary Figures 3, 4).
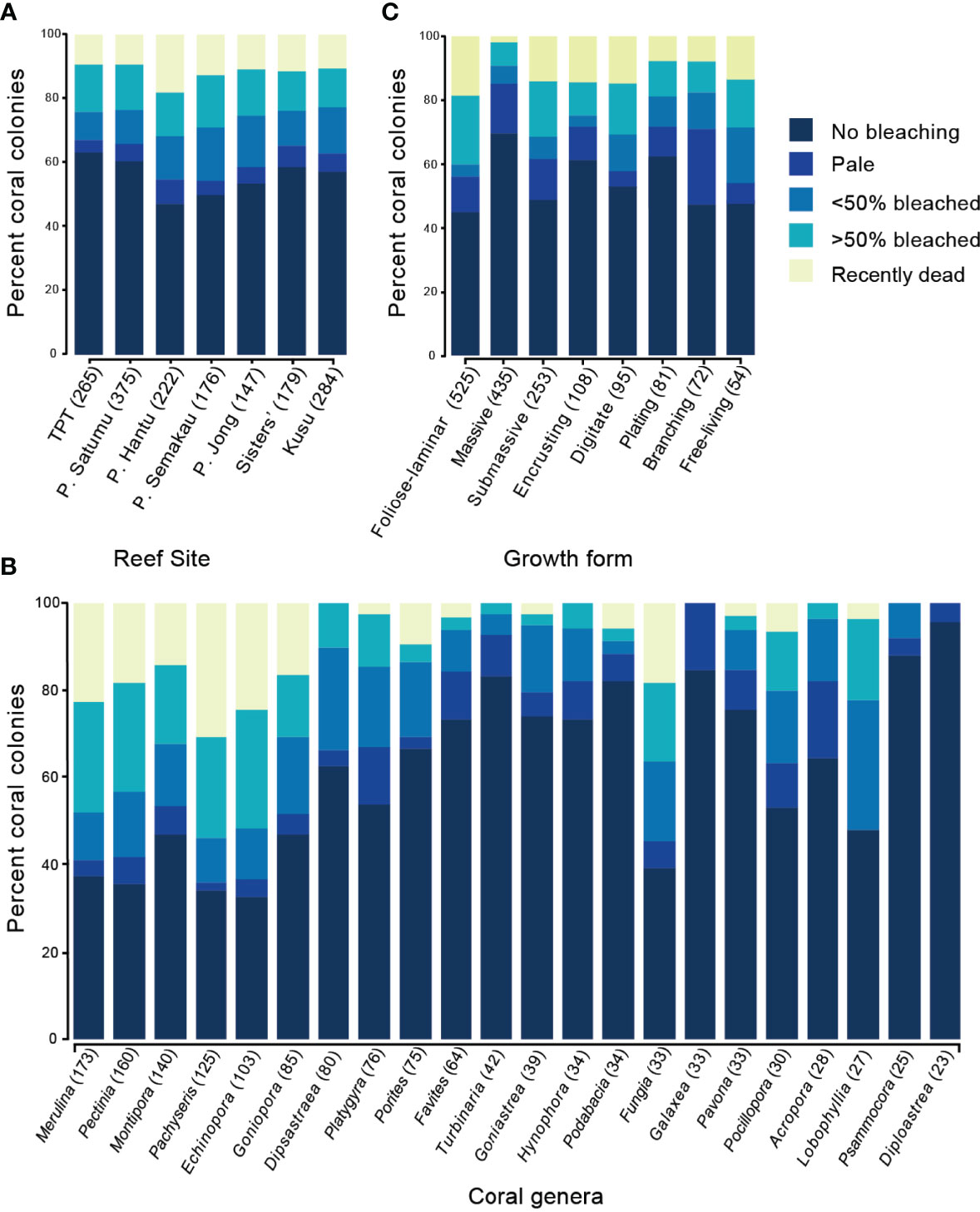
Figure 5 Proportion of coral colonies in each of the five bleaching categories (no bleaching to recently dead) across (A) seven reefs in Singapore ordered from westernmost to easternmost, (B) eight most abundant coral genera ordered from highest to lowest abundance, and (C) and coral growth form ordered highest to lowest abundance. Genera with <20 colonies were excluded.
Of the 46 genera recorded during the July 2016 bleaching surveys, 33 genera had bleached colonies, and 17 genera had colonies recently died (Supplementary Table 1). The five most abundant coral genera recorded during the bleaching surveys: Merulina (n = 173), Pectinia (160), Montipora (140), Pachyseris (125) and Echinopora (103), accounted for 52% of all bleached colonies (Figure 5B), 70% of colonies that were severely bleached and 77% of the colonies that died during or following the bleaching event (Supplementary Figure 2A). Pachyseris experienced the highest mortality in July 2016 among reefs with 30.4% of recorded colonies recently dead. Echinopora and Merulina also suffered relatively high mortality from the heat stress (>20% Supplementary Figure 2A). By contrast, only 14.3% of Montipora colonies were recently dead in July, and six less common genera Dipsastrea (80), Turbinaria (42), Hydnophora (34), Galaxea (33), Psammocora (25) and Diploastrea (23) ranged in bleaching incidence from 4.3% to 37.5% had no recently dead corals. Of the Acropora (28) and Pocillopora (30) colonies surveyed, 41% bleached, with the majority of these being branching growth forms, but we found no recently dead Acropora colonies, and only two recently dead Pocillopora colonies.
Foliose-laminar growth forms were the most commonly recorded growth form in bleaching surveys, comprising 30.5% of all colonies. However, 36.4% of these colonies bleached and 18.9% were recently dead (Figure 5C and Supplementary Figure 2B). Similarly, more than half of the submassive corals (15% of total colonies) were either bleached (36.7%) or dead (14.6%). Encrusting and digitate corals, which were considerably less abundant (6.4% and 5.5% of total colonies respectively), also showed substantial numbers of dead colonies in July 2016 (14.8% and 14.7% respectively, Figure 5C). Conversely, although massive corals accounted for over a quarter of colonies (25.3%), and bleaching incidence for massive corals was 28%, few massive colonies died (2.5%; Supplementary Figure 2B). The remaining growth forms plating (4.7% total colonies) and branching (4.3% total colonies) showed moderate mortality, with 4.7% and 8.3% dead colonies respectively (Figure 5C).
Bleaching Response Index
The bleaching response index (BRI) indicated marked variation in the severity of bleaching among the six most abundant coral genera and growth forms (Figures 6A, B). Changes in genera level BRI values from March to July ranged from -0.033 to +0.334 (0.112 ± 0.016 SE, Figure 6A) while changes in growth form BRI values ranged from 0 to +0.273 (0.145 ± 0.024 SE, Figure 6B). Proportional changes in the number of colonies between March and November were negatively correlated with both genera-level BRI in July (p < 0.001, Supplementary Figure 5) and change in genera-level BRI between March and July (p < 0.05, Supplementary Figure 5). The three most abundant genera recorded across bleaching surveys (Merulina, Pachyseris and Pectinia) decreased significantly in the number of colonies across reefs. All three genera had among the highest genera-level BRI values (Merulina BRI = 0.63; Pachyseris BRI = 0.69; Pectinia BRI = 0.63) in July and the largest changes in BRI from March to July (Merulina ΔBRI = +0.288; Pachyseris ΔBRI = +0.334; Pectinia ΔBRI = +0.303, Figure 6A).
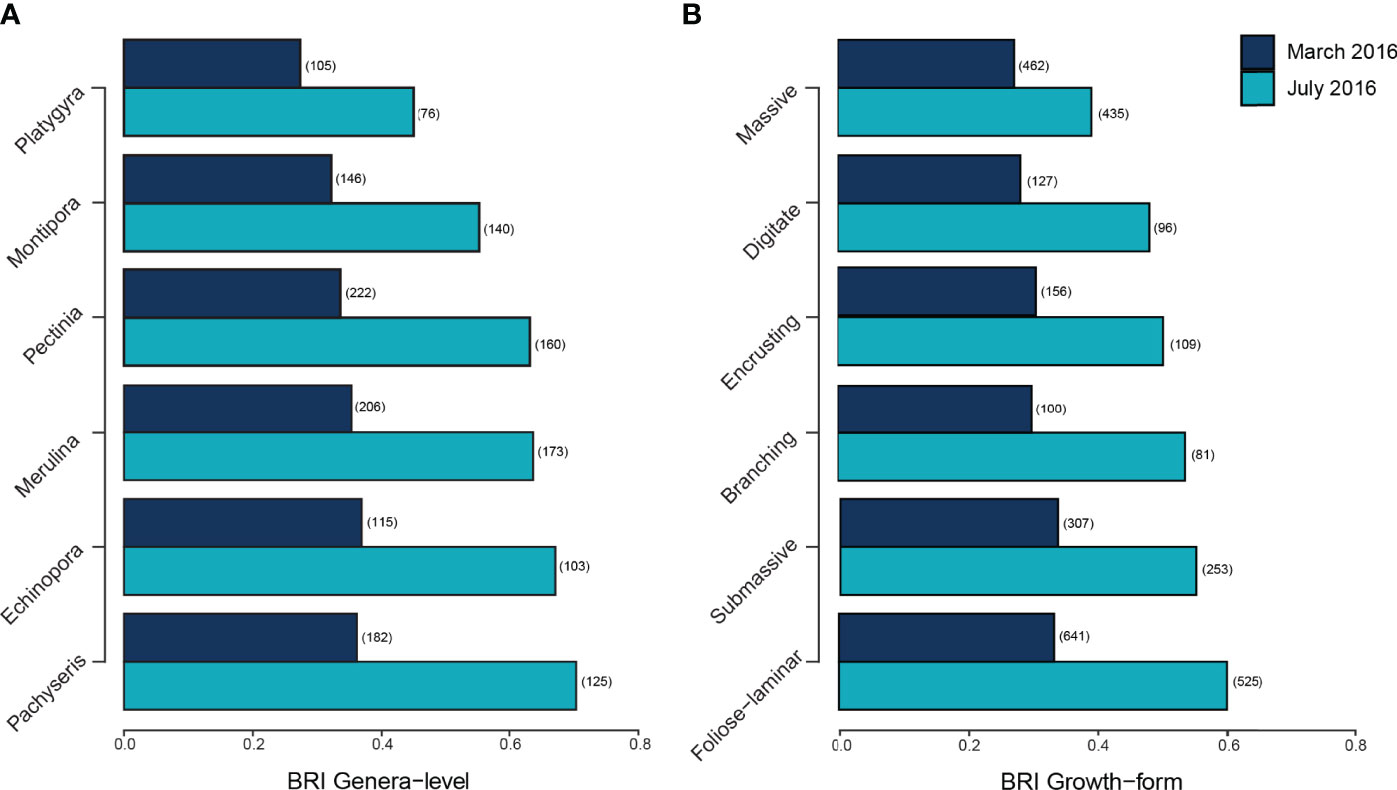
Figure 6 Bleaching Response Indices (A) highest genera-level BRI values and (B) highest growth form-level BRI value in July 2016 and their respective BRI values in March 2016. Numbers in parentheses represent the number of colonies for each coral genera.
Of the six most common coral growth forms recorded on Singapore’s reefs, colonies with foliose-laminar growth forms showed the greatest reduction in abundance between March and November 2016 (LRT = 11.01, p < 0.01, Figure 6B). This was reflected in BRI values, with foliose-laminar growth form having the highest BRI values in July (BRI = 0.605) and showing the largest ΔBRI from March to July (ΔBRI = +0.273, Figure 6B). The main growth forms recorded for Merulina and Pachyseris in March were foliose-laminar while Pectinia exhibited both submassive and foliose-laminar growth forms. The number of Pachyseris (LRT = 12.76, p < 0.01) and Pectinia (LRT = 14.19, p < 0.01) colonies declined significantly from March to July, while Merulina (LRT = 22.27, p < 0.001) and Pectinia (LRT = 11.32, p < 0.05) colony counts decreased significantly from March to November. By contrast, massive coral colonies had lowest BRI values in July (BRI = 0.392) and the smallest ΔBRI from March to July (ΔBRI = +0.119, Figure 6B)
Discussion
Severe coral bleaching events caused by climate driven marine heatwaves have impacted every coral reef region in the world. These recurring events have reduced live coral cover (Hughes et al., 2018a; Eakin et al., 2019), altered species composition (Hughes et al., 2018b), and are now undermining the functional importance of corals in maintaining three-dimensional complexity of the reef framework (Perry and Morgan, 2017). Similar to many other locations, extreme ocean temperatures in 2016 caused widespread coral bleaching across coral reefs in Southeast Asia (Kimura et al., 2018) including Singapore (Ng et al., 2020), with elevated temperatures occurring over three months and peak temperatures reaching well above established bleaching thresholds (30.8°C). Singapore’s bleaching event lasted two months from May 2016 (Ng et al., 2020) until July 2016 (this study) and surveys revealed moderate to severe coral bleaching across reefs in July 2016 but low coral mortality (~12% of colonies). However, subsequent benthic surveys showed marked reductions in coral cover and concomitant increases in dead coral and rubble likely reflecting delayed post-bleaching mortality and skeletal breakdown. Our findings also showed the three most abundant coral genera (Merulina, Pachyseris and Pectinia) recorded among reefs with the highest BRI levels in July declined significantly between March and November. Four months post-bleaching, small-scale structural complexity declined considerably across all reefs in this study and no moderately complex (>2 rugosity) reefs remained. Notably, this occurred across reefs with a large range of live coral cover (19–62%) and was linked to high mortality of foliose-laminar corals which resulted in flatter, less structurally complex reefs across Singapore.
Coral bleaching responses and subsequent mortality can be extremely patchy at local scales, where spatial differences in reef conditions that ameliorate or exacerbate the magnitude of the heat stress (e.g. water flow: Nakamura and van Woesik, 2001) can lead to differential bleaching and mortality (e.g., Anthony et al., 2007; Donovan et al., 2021). Similarly, spatial variation in coral bleaching and mortality across Singapore’s small reef system (~10 km2; Huang et al., 2009) may be associated with well-documented differences in local environmental gradients (Browne et al., 2015; Bauman et al., 2017; Morgan et al., 2020). Coral bleaching, mortality and the loss of coral cover was generally higher on Singapore’s nearshore reefs that persist under more turbid conditions (Browne et al., 2015) with lower light (Chen et al., 2005) and higher macroalgae cover (Bauman et al., 2017) relative to the offshore reefs (Guest et al., 2016; Januchowski-Hartley et al., 2020). Poor water quality (Wooldridge and Done, 2009), elevated nutrient levels (Burkepile et al., 2020) and high macroalgal abundance (Donovan et al., 2021) can act synergistically with thermal stress and magnify bleaching responses and mortality. While some turbid reefs in Singapore may be resistant to thermal stress (e.g. Guest et al., 2016; Ng et al., 2020), any potential protection afforded by turbidity (i.e., reduction in light stress; Cacciapaglia and van Woesik, 2015) during the 2016 bleaching event was likely limited given the intense heat stress and prolonged duration of the bleaching compared with previous events.
Spatial variation in bleaching and coral loss among reefs in Singapore may also vary according to local coral assemblage structure (Hughes et al., 2017), partly reflecting differences in the predominance of corals more susceptible to bleaching (Ng et al., 2020). Overall, bleaching prevalence and coral losses were related to the abundance of Merulina, Pachyseris, Pectinia and Echinopora which exhibited the greatest BRI changes between March and July 2016. Notably, reefs with relatively higher pre-bleaching cover of these taxa generally experienced greater bleaching and coral loss compared to other reefs. For example, on P. Hantu which had the greatest coral loss (18% mortality), these four taxa accounted for 50% of the total cover in March and subsequently declined to 31% of the cover by July 2016 (38% decline in relative abundance). Generalist coral taxa such as Merulina, Pachyseris and Pectinia are among the most dominant on Singaporean reefs (Guest et al., 2016) and tend to have traits that overlap life history groups (i.e., competitive, stress-tolerant and weedy; Darling et al., 2012) making them well adapted to marginal reef conditions and greater water depths (Darling et al., 2019). In Singapore, generalist taxa are found primarily on the shallow reef crest (~3–5 m depth; Huang et al., 2009) due to low-light conditions that vertically compresses the maximum depth of reef growth (Morgan et al., 2020). Moreover, many generalist species in Singapore are reported to be more resilient and less susceptible to coral bleaching (Ng et al., 2020). Yet, our results indicate that dominant generalist species found on Singaporean reefs are equally susceptible to high levels of bleaching but may have resisted bleaching for longer (Putnam et al., 2012) or bleached more slowly (McClanahan et al., 2001) than other more thermally sensitive corals suggesting a more complex pattern of stress and bleaching responses than observed during previous bleaching events (McClanahan et al., 2019). Such differences in bleaching patterns reported between studies highlight the need for caution when documenting bleaching susceptibility and resistance of corals, especially during more prolonged and intense bleaching events (Claar and Baum, 2019).
Our study also revealed marked differences in bleaching responses and mortality among coral growth forms. Overall, a higher proportion of corals with foliose-laminar, submassive and digitate growth forms bleached and died compared to massive, encrusting, plating (i.e. tabular), and branching corals growth forms. While it is generally assumed that slower-growing massive and encrusting coral growth forms are more resistant to bleaching than competitive branching, plating, and digitate forms (Marshall and Baird, 2000; Loya et al., 2001; but see McCowan et al., 2012; Swain et al., 2018) our results were not entirely consistent with these patterns. Notably, branching corals considered to be the most susceptible to bleaching had considerably lower BRI values compared to foliose-laminar corals, which had the highest BRI values in July, and exhibited the largest BRI changes between March and July 2016 compared to other growth forms. Moreover, branching corals experienced low mortality (8.3%) compared to foliose-laminar corals which suffered the highest mortality (19%). This result does not support the generally accepted notion that branching corals are more vulnerable to reductions in mass transfer than flatter colonies with lower aspect ratios, corresponding with less resistance to bleaching (Nakamura and van Woesik, 2001; van Woesik et al., 2012). Rather, it may be the over-representation of low-profile coral growth forms (e.g., foliose-laminar) on Singapore reefs that are better adapted to the poor light conditions. Flatter corals have larger surface-to-volume ratios which increases their ability to trap light for photosynthesis (e.g. Barnes, 1973). Alternatively, some corals with branching growth forms (e.g., Acropora and Pocillopora) in Singapore may have adapted and/or acclimatized to acute thermal stress (Guest et al., 2012). Together, these data suggest that many such generalities in patterns of bleaching susceptibility and mortality still lack empirical support (Baird and Marshall, 2002), emphasizing the need for further research on the vulnerability of different coral growth forms (McCowan et al., 2012).
Reductions in the abundance of dominant coral genera (Merulina, Pachyseris, Pectinia) and loss of foliose-laminar corals coincided with decreasing structural complexity across reefs in Singapore. Small-scale rugosity and reef topography both showed a high degree of spatial variation among reefs, that remained consistent up to one-year post survey (Januchowski-Hartley et al., 2020). However, overall reef rugosity in Singapore was low prior to bleaching (mean 1.7 ± 0.02) compared to other Indo-Pacific reefs including Kiribati (2.2 ± 0.5; Magel et al., 2019), Chagos (2.3 ± 0.2; Lang and Perry, 2019) and the Maldives (2.6 ± 0.1; Perry and Morgan, 2017), but comparable with Caribbean reefs (mean 1.5 ± 0.04) using similar methods (Alvarez-Filip et al., 2009; Alvarez-Filip et al., 2011b). Results showed small-scale reef rugosity did not change between March (pre-bleaching) and July (during bleaching), but that all reefs had undergone significant reductions in rugosity by November (post-bleaching) despite low overall bleaching mortality. Notably, four months post-bleaching no moderately complex (>2 rugosity) reefs remained in Singapore, and this pattern of decline was consistent across all reefs. These reductions were associated with increased dead coral cover and rubble suggesting delayed bleaching induced mortality (Brown and Phonguswan, 2012) and subsequent rapid skeletal dissolution (Leggat et al., 2019). In contrast, we detected no changes in reef topography among reefs in Singapore between March and November suggesting the bleaching event did not directly modify the larger physical reef framework. Without historical topographic complexity data, we could not determine whether the reef topography changed from previous bleaching events. Together, our results suggest that small-scale rugosity was already low on most Singaporean reefs prior to the onset of the study, and that additional reef flattening may occur with minimal changes in total coral cover (Alvarez-Filip et al., 2011b).
Notably, unlike other Indo-Pacific reefs, bleaching induced changes in small-scale structural complexity on Singapore reefs coincided with reductions in the abundance of generalist foliose-laminar taxa rather than competitive branching taxa such as Acropora, and/or stress-tolerant, massive taxa such as Porites (e.g., Perry and Morgan, 2017; Lang and Perry, 2019; Magel et al., 2019). Specifically, the loss of foliose-laminar Merulina and Pachyseries, and relative increases in the abundance of Montipora, Diploastrea, Porites and Favites with predominately massive or encrusting growth forms, suggest that reef flattening may be persistent across Singapore reefs. Throughout Singapore, most reef assemblages in Singapore have already shifted towards slower-growing generalist and stress-tolerant species with low-profile growth forms that provide less structural complexity (Guest et al., 2016), and contribute less to carbonate budgets (Januchowski-Hartley et al., 2020). Despite these shifts, overall mean coral cover has remained relatively high across most reefs, with over 30% cover on the reef crest (Huang et al., 2009; Guest et al., 2016; Wong et al., 2018). However, our results suggest these shifts in coral assemblage composition, do not ensure the persistence of critical reef functions such as maintaining structural complexity (Alvarez-Filip et al., 2013; Graham and Nash, 2013; Perry and Alvarez-Filip, 2019). Evidence from less-diverse Caribbean reefs, indicates that shifts in coral composition to dominance by opportunistic, non-framework building coral genera (e.g., Agaricia and Siderastrea) have substantially reduced the capacity of reefs to produce and maintain their structural complexity (Alvarez-Filip et al., 2011b), independent of changes in the total abundance of corals (Alvarez-Filip et al., 2013). Low reef carbonate budgets relative to the amount of coral cover on Singapore’s reefs can be attributed to the abundance of generalist and stress-tolerant taxa with encrusting and laminar growth forms (Januchowski-Hartley et al., 2020) resulting in structurally flatter reefs with lower vertical growth rates (Morgan et al., 2020). Under future climate change scenarios, these changes will likely further impair critical coral reef ecosystem functions, potentially compromising the long-term stability of reef-associated biodiversity and productivity on Singapore reefs.
Conclusion
While the 2016 global-scale coral bleaching event resulted in incredibly high coral mortality across many coral reefs around the world, our data show that the overall loss of live coral cover on Singapore’s reefs was comparatively low. Despite low coral loss, the high bleaching prevalence and post-bleaching mortality of dominant coral genera (mainly Merulina, Pachyseris and Pectinia) were associated with marked reductions in structural complexity across Singapore reefs with no moderately complex reefs (>2 rugosity) remaining after the 2016 bleaching event. Notably, this occurred across reefs with a large range of live coral cover (19–62%) and was linked to the loss of corals with low-profile foliose-laminar growth forms which resulted in flatter, less structurally complex reefs across Singapore. Importantly, while generalist and stress-tolerant coral taxa remain highly competitive within Singapore’s degraded, turbid reef environment, they may not have the capacity to maintain structural complexity or ensure the persistence of other critical reef functions, even within high coral cover communities (e.g. P. Satumu) due to their relatively thin, friable growth forms. If low-profile corals continue to dominate reef recovery in the long term, future reef complexity and functions in Singapore is unlikely to mirror any increases in coral cover. As reef communities move further from “healthy” coral assemblages, the links between bleaching susceptibility, growth form and ecosystem function will continue to evolve and warrant ongoing research.
Data Availability Statement
The original contributions presented in the study are included in the article/Supplementary Material. Further inquiries can be directed to the corresponding author.
Author Contributions
AB conceived and designed the study. AB carried out all the fieldwork. FJ-H, AB, and AT undertook the data analysis. AB and FJ-H wrote the manuscript, with contributions by AT and PT. All authors gave their final approval for publication.
Funding
This research was supported by the AXA Research Fellowship (154-000-649-507) awarded to AB, the European Regional Development Fund Sêr Cymru Fellowship (80761-SU-135) awarded to FJ-H and by the Singapore’s National Research Foundation (NRF) under the Marine Science Research and Development Programme (MSRDP-P03).
Conflict of Interest
The authors declare that the research was conducted in the absence of any commercial or financial relationships that could be construed as a potential conflict of interest.
Publisher’s Note
All claims expressed in this article are solely those of the authors and do not necessarily represent those of their affiliated organizations, or those of the publisher, the editors and the reviewers. Any product that may be evaluated in this article, or claim that may be made by its manufacturer, is not guaranteed or endorsed by the publisher.
Acknowledgments
We would like to thank Ian Chan, the Experimental Marine Ecology Laboratory, and the crew of the Dolphin Explorer for their assistance during fieldwork. Research was carried out under the Singapore National Parks Board permit (NP/RP15-009).
Supplementary Material
The Supplementary Material for this article can be found online at: https://www.frontiersin.org/articles/10.3389/fmars.2022.910085/full#supplementary-material
References
Alvarez-Filip L., Dulvy N. K., Gill J. A., Côté I. M., Watkinson A. R. (2009). Flattening of Caribbean Coral Reefs: A Region-Wide Declines in Architectural Complexity. Proc. R. Soc B. 276, 3019–3025. doi: 10.1098/rspb.2009.0339
Alvarez-Filip L., Dulvy N. K., Côté I. M., Watkinson A. R., Gill J. A. (2011a). Coral Identity Underpins Architectural Complexity on Caribbean Reefs. Ecol. Appl. 21, 2223–2231. doi: 10.1890/10-1563.1
Alvarez-Filip L., Côté I. M., Gill J. A., Watkinson A. R., Dulvy N. K. (2011b). Regional-Wide Temporal and Spatial Variation in Caribbean Reef Architecture: Is Coral Cover the Whole Story. Glob. Change Biol. 17, 2470–2477. doi: 10.1111/j.1365-2486.2010.02385.x
Alvarez-Filip L., Carricart-Ganivet J. P., Horta-Puga G., Iglesias-Prieto R. (2013). Shifts in Coral-Assemblage Composition do Not Ensure Persistence of Reef Functionality. Sci. Rep. 3, 3486. doi: 10.1038/srep03486
Álvarez-Noriega M., Baird A. H., Dornelas M., Madin J. S., Cumbo V. R., Connolly S. R. (2016). Fecundity and the Demographic Strategies of Coral Morphologies. Ecology 97, 3485–3493. doi: 10.1002/ecy.1588
Anthony K. R. N., Connolly S. R., Hoegh-Guldberg O. (2007). Bleaching, Energetics, and Coral Mortality Risk: Effects of Temperature, Light, and Sediment Regime. Limnol. Oceanogr. 52, 716–726. doi: 10.4319/lo.2007.52.2.0716
Baird A. H., Marshall P. A. (2002). Mortality, Growth and Reproduction in Scleractinian Corals Following Bleaching on the Great Barrier Reef. Mar. Ecol. Prog. Ser. 237, 133–141. doi: 10.3354/meps237133
Barlow J., França F., Gardner T. A., Hicks C. C., Lennox G. D., Berenguer E., et al. (2018). The Future of Hyperdiverse Tropical Ecosystems. Nature 559, 517–526. doi: 10.1038/s41586-018-0301-1
Bauman A. G., Hoey A. S., Dunshea G., Feary D. A., Low J., Todd P. A. (2017). Macroalgal Browsing on a Heavily Degraded, Urbanized Equatorial Reef System. Sci. Rep. 7, 8352. doi: 10.1038/s41598-017-08873-3
Bento R., Hoey A. S., Bauman A. G., Feary D. A., Burt J. A. (2016). The Implications of Recurrent Disturbance Within the World’s Hottest Coral Reef. Mar. Pollut. Bull. 105, 466–472. doi: 10.1016/j.marpolbul.2015.10.006
Berumen M. L., Pratchett M. S. (2006). Recovery Without Resilience: Persistent Disturbance and Long-Term Shifts in the Structure of Fish and Coral Communities at Tiahura Reef, Moorea. Coral Reefs 25, 647–653. doi: 10.1007/s00338-006-0145-2
Browne N. K., Tay J. K. L., Low J., Larson O., Todd P. A. (2015). Fluctuations in Coral Health of Four Common Inshore Reef Corals in Response to Seasonal and Anthropogenic Changes in Water Quality. Mar. Environ. Res. 105, 39–52. doi: 10.1016/j.marenvres.2015.02.002
Brown B. E., Phonguswan N. (2012). Delayed Mortality in Bleached Massive Corals on Intertidal Reef Flats Around Phuket, Andaman Sea, Thailand. Phuket Mar. Biol. Cent. Res. Bull. 71, 43–48.
Burkepile D. E., Shantz A. A., Adam T. C., Munsterman K. S., Speare K. E., Ladd M. C., et al. (2020). Nitrogen Identity Drives Differential Impacts of Nutrients on Coral Bleaching and Mortality. Ecosystems 23, 798–811. doi: 10.1007/s10021-019-00433-2
Burke L., Reytar K., Spalding M., Perry A. (2011). Reefs at Risk Revisited (Washington, DC: World Resources Institute).
Burt J. A., Al-Harthi S., Al-Cibahy A. (2011). Long-Term Impacts of Coral Bleaching Events on the World’s Warmest Reefs. Mar. Environ. Res. 72, 225–229. doi: 10.1016/j.marenvres.2011.08.005
Burt J. A., Bauman A. G. (2020). Suppressed Coral Settlement Following a Mass Bleaching in the Southern Persian/Arabian Gulf. Aquat. Ecosyst. Health Manage. 23, 166–174. doi: 10.1080/14634988.2019.1676024
Cacciapaglia C., van Woesik R. (2015). Climate-Change Refugia: Shading Reef Corals by Turbidity. Glob. Change Biol. 22, 1145–1154. doi: 10.1111/gcb.13166
Carballo J. L., Bautista E., Nava H., Cruz-Barraza J. A., Chavez J. A. (2013). Boring Sponges, an Increasing Threat for Coral Reefs Affected by Bleaching Events. Ecol. Evol. 3, 872–886. doi: 10.1002/ece3.452
Carlot J., Rovère A., Casella E., Harris D., Grellet-Muñoz C., Chancerelle Y., et al. (2020). Community Composition Predicts Photogrammetry-Based Structural Complexity on Coral Reefs. Coral Reefs 39, 967–975. doi: 10.1007/s00338-020-01916-8
Chen M., Murali K., Khoo B.-C., Lou J., Kumar K. (2005). Circulation Modelling in the Strait of Singapore. J. Coast. Res. 215, 960–972. doi: 10.2112/04-0412.1
Claar D. C., Baum J. K. (2019). Timing Matters: Survey Timing During Extended Heat Stress can Influence Perceptions of Coral Susceptibility to Bleaching. Coral Reefs 38, 559–565. doi: 10.1007/s00338-018-01756-7
Cleary D. F. R., Polónia A. R. M., Renema W., Hoeksema B. W., Rachello-Dolmen P. G., Moolenbeek R. G. (2016). Variation in the Composition of Corals, Fishes, Sponges, Echinoderms, Ascidians, Molluscs, Foraminifera and Macroalgae Across Pronounced in-to-Offshore Environmental Gradient in Jakarta Bay – Thousand Islands Coral Reef Complex. Mar. Pollut. Bull. 110, 701–717. doi: 10.1016/j.marpolbul.2016.04.042
Couch C. S., Burns J. H. R., Liu G., Steward K., Gutlay T. N., Kenyon J., et al. (2017). Mass Coral Bleaching Due to Unprecedented Marine Heatwave in Papahānaumokuākea Marine National Monument (Northwestern Hawaiian Islands). PloS One 12, e0185121. doi: 10.1371/journal.pone.0185121
Darling E. S., Alvarez-Filip L., Oliver T. A., McClanahan T. R., Côté I. M. (2012). Evaluating Life-History Strategies of Reef Corals From Species Traits. Ecol. Lett. 15, 1378–1386. doi: 10.1111/j.1461-0248.2012.01861.x
Darling E. S., Graham N. A. J., Januchowski-Hartley F. A., Nash K. L., Pratchett M. S., Wilson S. K. (2017). Relationships Between Structural Complexity, Coral Traits, and Reef Fish Assemblages. Coral Reefs 36, 561–575. doi: 10.1007/s00338-017-1539-z
Darling E. S., McClanahan T. R., Côté I. M. (2013). Life Histories Predict Coral Disassembly Under Multiple Stressors. Glob. Change Biol. 19, 1930–1940. doi: 10.1111/gcb.12191
Darling E. S., McClanahan T. R., Maina J., Gurney G. G., Graham N. A. J., Januchowski-Hartley F. A., et al. (2019). Social-Environmental Drivers Inform Strategic Management of Coral Reefs in the Anthropocene. Nat. Ecol. Evol. 3, 1341–1350. doi: 10.1038/s41559-019-0953-8
Denis V., Ribas-Deulofeu L., Sturaro N., Kuo C.-Y., Chen C. A. (2017). A Functional Approach to the Structural Complexity of Coral Assemblages Based on Colony Morphological Features. Sci. Rep. 7, 9849. doi: 10.1038/s41598-017-10334-w
Donovan M. K., Burkepile D. E., Kratochwill C., Shlesinger T., Sully S., Oliver T. A., et al. (2021). Local Conditions Magnify Coral Loss After Marine Heatwaves. Science 372, 977–980. doi: 10.1126/science.abd9464
Eakin C. M., Sweatman H. P. A., Brainhard R. E. (2019). The 2014–2017 Global-Scale Coral Bleaching Event: Insights and Impacts. Coral Reefs 38, 539–545. doi: 10.1007/s00338-019-01844-2
Glynn P. W. (1983). Extensive “Bleaching” and Death of Reef Corals on the Pacific Coast of Panamá. Environ. Conserv. 10, 149–154. doi: 10.1017/S0376892900012248
Graham N. A. J., Jennings S., MacNeil M. A., Mouillot D., Wilson S. K. (2015). Predicting Climate-Driven Regime Shifts Versus Rebound Potential in Coral Reefs. Nature 518, 94–97. doi: 10.1038/nature14140
Graham N. A. J., Nash K. L. (2013). The Importance of Structural Complexity in Coral Reef Ecosystems. Coral Reefs 32, 315–326. doi: 10.1007/s00338-012-0984-y
Graham N. A. J., Wilson S. K., Jennings S., Polunin N. V. C., Robinson J., Bijoux J. P., et al. (2007). Lag Effects in the Impacts of Mass Coral Bleaching on Coral Reef Fish, Fisheries, and Ecosystems. Conserv. Biol. 21, 1291–1300. doi: 10.1111/j.1523-1739.2007.00754.x
Guest J. R., Baird A. H., Maynard J. A., Muttaqin E., Edwards A. J., Campbell S. J., et al. (2012). Contrasting Patterns of Coral Bleaching Susceptibility in 2010 Suggest an Adaptive Response to Thermal Stress. PloS One 7, e33353. doi: 10.1371/journal.pone.0033353
Guest J. R., Tun K., Vergés A., Marzinelli E. M., Campbell A. H., Bauman A. G., et al. (2016). 27 Years of Benthic and Coral Community Dynamics on Turbid, Highly Urbanised Reefs Off Singapore. Sci. Rep. 6, 36260. doi: 10.1038/srep36260
Harris D. L., Rovere A., Casella E., Power H., Canavesio R., Collin A., et al. (2018). Coral Reef Structural Complexity Provides Important Coastal Protection From Waves Under Rising Sea Levels. Sci. Adv. 4, eaao4350. doi: 10.1126/sciadv.aao4350
Heery E. C., Hoeksema B. W., Browne N. K., Reimer J. D., Ang P. O., Huang D., et al. (2018). Urban Coral Reefs: Degradation and Resilience of Hard Coral Assemblages in Coastal Cities of East and Southeast Asia. Mar. Pollut. Bull. 135, 654–681. doi: 10.1016/j.marpolbul.2018.07.041
Hixon M. A., Beets J. P. (1993). Predation, Prey Refuges, and the Structure of Coral-Reef Fish Assemblages. Ecol. Monogr. 63, 77–101. doi: 10.2307/2937124
Huang D., Licuanan W. Y., Hoeksema B. W., Chen C. A., Ang P. O., Huang H., et al. (2015). Extraordinary Diversity of Reef Corals in the South China Sea. Mar. Biodiv. 45, 157–168. doi: 10.1007/s12526-014-0236-1
Huang D. H., Tun K. P. P., Chou L. M., Todd P. A. (2009). An Inventory of Zooxanthellate Scleractinian Corals in Singapore, Including 33 New Records. Raffles Bull. Zool. S22, 69–80. doi: 10.26107/NIS-2021-0091
Hughes T. P., Anderson K. D., Connolly S. R., Heron S. F., Kerry J. T., Lough J. M., et al. (2018a). Spatial and Temporal Patterns of Mass Bleaching of Corals in the Anthropocene. Science 359, 80–83. doi: 10.1126/science.aan8048
Hughes T. P., Kerry J. T., Álvarez-Noriega M., Álvarez-Romero J. G., Anderson K. D., Baird A. H., et al. (2017). Global Warming and Recurrent Mass Bleaching of Corals. Nature 543, 373–377. doi: 10.1038/nature21707
Hughes T. P., Kerry J. T., Baird A. H., Connolly S. R., Dietzel A., Eakin C. M., et al. (2018b). Global Warming Transforms Coral Reef Assemblages. Nature 556, 492–496. doi: 10.1038/s41586-018-0041-2
Jackson J. B. C. (1979). “Morphological Strategies of Sessile Animals” in Biology and Systematics of Colonial Animals. Eds. Larwood G., Rosen B. R. (New York: Academic Press), 499–555.
Januchowski-Hartley F. A., Bauman A. G., Morgan K. M., Seah J. C. L., Huang D., Todd P. A. (2020). Accreting Coral Reefs in a Highly Urbanized Environment. Coral Reefs 39, 717–731. doi: 10.1007/s00338-020-01953-3
Januchowski-Hartley F. A., Graham N. A., Wilson S. K., Jennings S., Perry C. T. (2017). Drivers and Predictions of Coral Reef Carbonate Budget Trajectories. Proc. R. Soc B. 25, 20162533. doi: 10.1098/rspb.2016.2533
Kimura T., Tun K., Chou L. M. (2018). Status of Coral Reefs in East Asian Seas Region: 2018 (Tokyo, Japan: Ministry of Environment of Japan and Japan Wildlife Research Center).
Lang I. D., Perry C. T. (2019). Bleaching Impacts on Carbonate Production in the Chagos Archipelago: Influence of Functional Coral Groups on Carbonate Budget Trajectories. Coral Reefs 38, 619–624. doi: 10.1007/s00338-019-01784-x
Leggat W. P., Camp E. F., Suggett D. J., Heron S. F., Fordyce A. J., Gardner S., et al. (2019). Rapid Coral Decay Is Associated With Marine Heatwave Mortality Events on Reefs. Curr. Biol. 29, 2723–2730. doi: 10.1016/j.cub.2019.06.077
Licuanan W. Y., Robles R., Reyes M. (2019). Status and Recent Trends in Coral Reefs of the Philippines. Mar. Pollut. Bull. 142, 544–550. doi: 10.1016/j.marpolbul.2019.04.013
Low J. K. Y., Fong J., Todd P. A., Chou L. M., Bauman A. G. (2019). Seasonal Variation of Sargassum Ilicifolium (Phaeophyceae) Growth on Equatorial Coral Reefs. J. Phycol. 55, 289–296. doi: 10.1111/jpy.12818
Loya Y., Sakai K., Yamazato K., Nakano Y., Sambali H., van Woesik R. (2001). Coral Bleaching: The Winners and Losers. Ecol. Lett. 4, 122–131. doi: 10.1046/j.1461-0248.2001.00203.x
Madin J. S., Hoogenboom M. O., Connolly S. R., Darling E. S., Falster D. S., Huang D., et al. (2016). A Trait-Based Approach to Advance Coral Reef Science. Trends Ecol. Evol. 31, 419–428. doi: 10.1016/j.tree.2016.02.012
Magel J. M., Burns J. H., Gates R. D., Baum J. K. (2019). Effects of Bleaching-Associated Mass Coral Mortality on Reef Structural Complexity Across a Gradient of Local Disturbance. Sci. Rep. 9, 2512. doi: 10.1038/s41598-018-37713-1
Marshall P. A., Baird A. H. (2000). Bleaching of Corals on the Great Barrier Reef: Differential Susceptibilities Among Taxa. Coral Reefs 19, 155–163. doi: 10.1007/s003380000086
McClanahan T. R., Baird A. H., Marshall P. A., Toscano M. A. (2004). Comparing Bleaching and Mortality Responses of Hard Corals Between Southern Kenya and the Great Barrier Reef, Australia. Mar. Pollut. Bull. 48, 327–335. doi: 10.1016/j.marpolbul.2003.08.024
McClanahan T. R., Darling E. S., Maina J. M., Muthiga N. A., D’gata S., Jupiter S. D., et al. (2019). Temperature Patterns and Mechanisms Influencing Coral Bleaching During the 2016 El Niño. Nat. Clim. Change 9, 845–851. doi: 10.1038/s41558-019-0576-8
McClanahan T. R., Muthiga N. A., Mangi S. (2001). Coral and Algal Changes After the 1998 Coral Bleaching: Interaction With Reef Management and Herbivores on Kenyan Reefs. Coral Reefs 19, 380–391. doi: 10.1007/s003380000133
McCowan D. M., Pratchett M. S., Baird A. H. (2012). “Bleaching Susceptibility and Mortality Among Corals With Differing Growth Forms,” in Proceedings of the 12th International Coral Reef Symposium, Cairns Vol. 9A. 1–6. QLD, Australia, 9–13 July 2012.
Morgan K. M., Moynihan M. A., Sanwlani N., Switzer A. D. (2020). Light Limitation and Depth-Variable Sedimentation Drives Vertical Reef Compression on Turbid Coral Reefs. Front. Mar. Sci. 7. doi: 10.3389/fmars.2020.571256
Nakamura T., van Woesik R. (2001). Water-Flow Rate and Passive Diffusion Partially Explain Differential Survival of Corals During the 1998 Beaching Event. Mar. Ecol. Prog. Ser. 212, 301–304. doi: 10.3354/meps212301
Ng C. S. L., Huang D., Toh K. B., Sam S. Q., Kikuzawa Y. P., Toh T. C., et al. (2020). Responses of Urban Reef Corals During the 2016 Mass Bleaching Event. Mar. Pollut. Bull. 154, 111111. doi: 10.1016/j.marpolbul.2020.111111
NOAA (2016). “Coral Reef Watch, Updated Daily,” in NOAA Coral Reef Watch Daily Global 5-Km Satellite Virtual Station Time Series. Data for the Singapore Strait 1/1/16 to 31/12/16(College Park, Maryland, USA: NOAA). Available at: http://coralreefwatch.noaa.gov/vs/index.php.
Perry C. T., Alvarez-Filip L. (2019). Changing Geo-Ecological Functions of Coral Reefs in the Anthropocene. Funct. Ecol. 33, 976–988. doi: 10.1111/1365-2435.13247
Perry C. T., Alvarez-Filip L., Graham N. A. J., Mumby P. J., Wilson S. K., Kench P. S., et al. (2018). Loss of Coral Reef Growth Capacity to Track Future Increases in Sea Level. Nature 558, 396–400. doi: 10.1038/s41586-018-0194-z
Perry C. T., Morgan K. M. (2017). Bleaching Drives Collapse in Reef Carbonate Budgets and Reef Growth Potential on Southern Maldives Reefs. Sci. Rep. 7, 40581. doi: 10.1038/srep40581
Polunin N. V. C., Roberts C. M. (1993). Greater Biomass and Value of Target Coral-Reef Fishes in Two Small Caribbean Marine Reserves. Mar. Ecol. Prog. Ser. 100, 167–176. doi: 10.3354/meps100167
Pratchett M. S., Hoey A. S., Wilson S. K. (2014). Reef Degradation and the Loss of Critical Ecosystem Goods and Services Provided by Coral Reef Fishes. Curr. Opin. Environ. Sustain. 7, 37–43. doi: 10.1016/j.cosust.2013.11.022
Pratchett M. S., McWilliam M. J., Riegl B. (2020). Contrasting Shifts in Coral Assemblages With Increasing Disturbances. Coral Reefs 39, 783–793. doi: 10.1007/s00338-020-01936-4
Pratchett M. S., Trapon M., Berumen M. L., Chong-Seng K. (2011). Recent Disturbances Augment Community Shifts in Coral Assemblages in Moorea, French Polynesia. Coral Reefs 30, 183–193. doi: 10.1007/s00338-010-0678-2
Putnam H. M., Stat M., Pochon X., Gates R. D. (2012). Endosymbiotic Flexibility Associates With Environmental Sensitivity in Scleractinian Corals. Proc. Biol. Sci. 279, 4352–4361. doi: 10.1098/rspb.2012.1454
Risk M. J. (1972). Fish Diversity on a Coral Reef in the Virgin Islands. Atoll Res. Bull. 153, 1–6. doi: 10.5479/si.00775630.153.1
Swain T. D., Bold E. C., Osborn P. C., Baird A. H., Westneat M. W., Backman V., et al. (2018). Physiological Integration of Coral Colonies is Correlated With Bleaching Resistance. Mar. Ecol. Prog. Ser. 586, 1–10. doi: 10.3354/meps12445
Swain T. D., Vega-Perkins J. B., Oestreich W. K., Triebold C., Dubois E., Henss J., et al. (2016). Coral Bleaching Response Index: A New Tool to Standardize and Compare Susceptibility to Thermal Bleaching. Glob. Change Biol. 22, 2475–2488. doi: 10.1111/gcb.13276
Syms C., Jones G. P. (2000). Disturbance, Habitat Structure, and the Dynamics of a Coral Reef Fish Community. Ecology 81, 2714–2729. doi: 10.2307/177336
van Woesik R., Irikawa A., Anzai R., Nakamura T. (2012). Effects of Coral Colony Morphologies on Mass Transfer and Susceptibility to Thermal Stress. Coral Reefs 31, 633–639. doi: 10.1007/s00338-012-0911-2
van Woesik R., Sakai K., Ganase A., Loya Y. (2011). Revisiting the Winners and the Losers a Decade After Coral Bleaching. Mar. Ecol. Prog. Ser. 434, 67–76. doi: 10.3354/meps09203
Veron J. E. N. (2000). Corals of the World Vol 1-3. Townsville, QLD: Australian Institute of Marine Science.
Wang Y., Naumann U., Wright S. T., Warton D. I. (2012). Mvabund – an R Package for Model-Based Analysis of Multivariate Abundance Data. Methods Ecol. Evol. 3, 471–474. doi: 10.1111/j.2041-210X.2012.00190.x
Wilson S. K., Graham N. A. J., Polunin N. V. C. (2007). Appraisal of Visual Assessments of Habitat Complexity and Benthic Composition on Coral Reefs. Mar. Biol. 151, 1069–1076. doi: 10.1007/s00227-006-0538-3
Wong J. S. Y., Chan Y. K. S., Ng C. S. L., Tun K. P. P., Darling E. S., Huang D. (2018). Comparing Patterns of Taxonomic Functional and Phylogenetic Diversity in Reef Coral Communities. Coral Reefs 37, 737–750. doi: 10.1007/s00338-018-1698-6
Keywords: coral reefs, coral bleaching, structural complexity, habitat loss, Southeast Asia
Citation: Bauman AG, Januchowski–Hartley FA, Teo A and Todd PA (2022) Further Flattening of a Degraded, Turbid Reef System Following a Severe Coral Bleaching Event. Front. Mar. Sci. 9:910085. doi: 10.3389/fmars.2022.910085
Received: 31 March 2022; Accepted: 09 June 2022;
Published: 22 July 2022.
Edited by:
Geórgenes Hilário Cavalcante, Federal University of Alagoas, BrazilReviewed by:
Maarten De Brauwer, Commonwealth Scientific and Industrial Research Organisation (CSIRO), AustraliaFrancoise Cavada-Blanco, Zoological Society of London, United Kingdom
Copyright © 2022 Bauman, Januchowski–Hartley, Teo and Todd. This is an open-access article distributed under the terms of the Creative Commons Attribution License (CC BY). The use, distribution or reproduction in other forums is permitted, provided the original author(s) and the copyright owner(s) are credited and that the original publication in this journal is cited, in accordance with accepted academic practice. No use, distribution or reproduction is permitted which does not comply with these terms.
*Correspondence: Andrew G. Bauman, YWJhdW1hbkBub3ZhLmVkdQ==
†Present address: Andrew G. Bauman, Department of Marine and Environmental Sciences, Nova Southeastern University, Dania Beach, FL, United States