- 1Institute of Estuarine and Coastal Research, School of Marine Engineering and Technology, Sun Yat-sen University, Guangzhou, China
- 2School of Marine Sciences, Sun Yat-Sen University, Zhuhai, China
- 3Southern Marine Science and Engineering Guangdong Laboratory (Zhuhai), Zhuhai, China
- 4Guangdong Provincial Key Laboratory of Marine Resources and Coastal Engineering, Sun Yat-sen University, Zhuhai, China
The lateral carbon export related to mangroves is of great scientific significance and ecological value in the global carbon cycle. The dissolved inorganic carbon (DIC), dissolved organic carbon (DOC), particulate organic carbon (POC), and stable isotopes (δ13CPOC) of water samples were quantified in the flood (September 2020) and dry (January 2021) seasons in Zhangjiang Estuary. The results revealed that the carbon compositions in the tidal channel of the Zhangjiang Estuary are as follows: DIC > DOC > POC in both seasons. Except for the POC in the site near the sluice, the contents of all carbon compositions were significantly larger in the flood season than those in the dry season (p< 0.05). In the flood season, the POC and DOC exhibited similar spatial characteristics that all sites from the lower sites to the mouth were significantly larger than the site near the sluice. The DIC had an increasing trend from the upper site to the mouth. In the dry season, DIC and DOC displayed patchy distribution under the influence of mariculture and the sluice, while the POC had a decreasing trend from the upper site to the mouth. The MixSIAR model indicates that the source of the POC is overwhelmingly the mariculture, averagely accounting for 42.7% in the flood season and 52.6% in the dry season, mainly in the form of microalgae. The average contribution of mangrove to POC was 33.1% in the flood season and 39.3% in the dry season. The DIC-δ13CPOC and DOC-POC relationships represent the biogeochemical process of microbial photosynthesis and the physical process of adsorption-desorption of organic carbon by redundancy analysis, respectively. This initial dataset for this region should be included in other studies to improve the mangrove outwelling estimate.
Introduction
Increasing carbon emissions from human activities and the resulting global climate change are of concern in both political and scientific communities (Rani et al., 2021). Thus, it is of great significance to explore the potentials of different types of ecosystems for mitigating global warming. As the largest carbon sink in the world, the ocean can absorb one-third of the global CO2 emissions per year, and it is defined as blue carbon (McLeod et al., 2011). Estuaries are transitional regions linking the terrestrial environment and continental shelf, and they play a vital role in the global carbon cycle, with an annual net carbon flux of 0.85 Pg C (Bauer et al., 2013) into the ocean. However, the carbon cycle and flux in estuaries are regulated by many factors owing to their complex hydrodynamic conditions. This also leads to the biogeochemical parameters, including carbon, nitrogen, phosphorus, salinity, temperature, and chlorophyll, having different spatial and temporal gradients in different regions. For example, with the largest runoff of 80,000–250,000 m3/s in the world, the Amazon Estuary mouth and the offshore displayed a CO2 sink by the dilution of seawater and high chlorophyll contents (Lefèvre et al., 2017). However, in the Altamaha Estuary, the amount of dissolved organic matter (DOM) decreases from the upstream area to the estuary, which is mainly driven by seasonal variations in the river runoff (Letourneau et al., 2021). In addition, anthropogenic activities have a strong influence on the spatiotemporal distribution of estuarine biogenic matter. Owing to anthropogenic perturbation, the carbon pool has decreased by 25%–50% in the past 100 years, and an extra 0.2 Pg C year−1 has been exported into the ocean (Regnier et al., 2013). However, the types and intensity of human activities vary in different regions, so it is necessary to focus on the spatiotemporal characteristics, sources, and biogeochemistry of the carbon pool based on the specific characteristics of each estuary to reveal the migration and transformation mechanisms.
Mangroves, a typical blue carbon ecosystem located in estuaries and coastal areas, occupy only 0.5% of the world’s coastal area, but they contribute 10%–15% to their total carbon sequestration (Alongi, 2014). Compared with salt marshes (593 Mg C ha−1), seagrass beds (142 Mg C ha−1), and other types of forest systems (200–400 Mg C ha−1), mangrove carbon stocks are remarkably high (956 Mg C ha−1) owing to the burying of organic carbon in anoxic sediment, and thus, mangroves play an irreplaceable role in mitigating climate change. Studies had quantified the carbon stock of mangroves and the carbon exchange (Bouillon et al., 2007a; Bauer et al., 2013; Feng et al., 2017; Jeffrey et al., 2018). The lateral exchange in mangroves is also particularly important to scientific issues related to the global carbon cycling (Donato et al., 2011; Alongi, 2014). However, the hydrodynamic and sedimentary processes change significantly. In particular, the mangrove ecosystem in small estuaries is very sensitive to anthropogenic perturbations. Owing to the complex conditions of the mangrove carbon exchange, the carbon source, carbon export, and controlling mechanisms require further investigation.
The vertical carbon accumulation in mangroves, such as the net primary productivity and biomass, carbon stocks, and storage rates between species or communities, has been frequently reported in previous studies (Donato et al., 2011; Alongi, 2014; Feng et al., 2017; Kamruzzaman et al., 2017; Zhang et al., 2021). It has been found that the vertical carbon sink formed by a mangrove forest can export half of its detritus to the adjacent water environment, accounting for 10%–11% of the total carbon (Odum and Heald, 1975; Alongi, 2014), and thus, it appears to be a lateral carbon source. The mangrove outwelling hypothesis proposes that most of the mangrove detritus is transported to the coastal area in the form of dissolved inorganic carbon (DIC), dissolved organic carbon (DOC), and particulate organic carbon (POC) and maintains the function and stability of the estuary ecosystem through a series of physical and biogeochemical processes. Bouillon et al. (2007b) found that organic carbon can be mineralized and converted into DIC in estuaries. As they reported, DIC is considered to be the main form of estuary carbon export, accounting for more than 50% (Cai et al., 2008) of the total carbon exported from mangrove ecosystems globally, which may contribute to the missing carbon part of the budget. Additionally, the export of mangrove detritus is an important part of the carbon cycle. The contribution of mangrove to POC has been challenged based on stable isotope analysis and has been reported to be very limited (Lee, 1995). Most estuaries have tremendous internal spatial and temporal heterogeneity in terms of carbon processing and fluxes, making it difficult to quantify the net carbon balance of even an individual estuary. Therefore, the contribution of and lateral export from mangrove in estuaries need to be determined to enable further regional and global extrapolation (Bauer et al., 2013).
Stable isotope analysis is an effective tracing method for inferring the relative contributions of different carbon sources to particulate organic matter in aquatic ecosystems, especially complex estuaries. Schulte et al. (2011) found that the δ13CPOC (13C/12C) value gradually increased from land to sea, indicating the transport distance of the terrestrial organic matter. On the Atlantic coast of the United States, the δ13CPOC values of the sediment suggest that terrestrial organic matter cannot be transported to the estuaries (Hunt, 1970). Stable isotopes are effective only when the isotopic values of each source are significantly different, and the utilization of multiple isotopic indices can produce more accurate results. For example, multiple isotopes (δ13CPOC, δ15N, and δ18O) have been combined to identify the sources of sewage (Cabral et al., 2019), organic matter in sediments, and consumers (Zhou et al., 2006). The C4Spartina alterniflora and phytoplankton have been found to be the main sources for filter-feeding mollusks based on δ13CPOC, δ15N, and δ34S data for mangrove ecosystems (Peterson et al., 1985).Anthropogenic perturbations have increased the flux of carbon to water bodies by as much as 1.0 Pg C year−1 since pre-industrial times (Regnier et al., 2013), which has altered the flux from land to sea and the global carbon budget. There is a large area of mangroves in the Zhangjiang Estuary (ZJE), which is also under the influence of strong human activities, including sluice construction, aquaculture, and invasion of S. alterniflora, making it even more complicated to determine the sources and contributors of the organic matter. The sluice built in 1966 prevents the saline sea water from retreating (Zhang and Deng, 2019) and decreases the discharge, transforming the ZJE into a typical tidally controlled area. In the past two decades, a large area of bare tidal flats has been invaded by S. alterniflora, as well as mangrove (Bouillon et al., 2008b; Alongi, 2014). The changes in the estuary’s hydrodynamics and vegetation definitely altered the biochemical processes and the lateral carbon flux and sources. Furthermore, the coastal mariculture ponds occupied 981 ha, accounting for 41% of the entire ZJE. Therefore, the increased organic matter input from the mariculture may greatly affect the estuarine carbon cycle.The objectives of this study were as follows: 1) to investigate the spatiotemporal features and sources of the DIC, DOC, and POC and the related estuarine processes under the effects of the sluice and mariculture; and 2) to determine the mangrove outwelling in the ZJE. Therefore, we attempted to reveal the fate of the laterally transported carbon in the ZJE and its critical mechanisms and to further understand the carbon cycle in small estuaries worldwide.
Materials and Methods
Study Area
The ZJE (23°54’00”–23°58’00”N, 117°22’00”–117°30’00”E) is located in Yunxiao, Fujian Province, on the southeastern coast of China (Figure 1). It has a subtropical monsoon climate with an annual average temperature of 21.2°C (27.1°C in summer and 18.6°C in winter), an average annual precipitation of 1,714.5 mm, which is concentrated from April to September, a relative humidity of 79%, and an annual discharge of 1.0 × 109 m3 in the estuary. The ZJE is a semi-enclosed, semi-diurnal tidal estuary, with a tidal range of 0.43–4.67 m (average of 2.32 m) and a salinity of 0‰–30‰. It occupies ~2,360 ha and is bordered by 117.9 ha of mangrove. The dominant species are Avicennia marina, Kandelia obovata, and Aegiceras corniculatum. S. alterniflora has been introduced into the region since the 1990s, has expanded at a rate of 4.28 m/year (Zhu et al., 2019), occupies 49.7 ha, and even has the potential to replace entire mangrove forests under the influence of strong human activities (Feng et al., 2017). In addition, the mariculture ponds distributed along the two sides of the ZJE and Dongshan Bay outside the estuary cover areas of 980 and 7,380 ha, respectively (Gao et al., 2021).
Sample Collection and Pretreatment
From the upper boundary to the mouth of the ZJE, eight sampling sites were chosen in two creeks. Sites N1, N2, N3, N4, and #5 were located in north Creek 1 from upstream to the estuarine mouth; and sites S1, S2, and S3 were located in south Creek 2 from the mangrove area to the bare flat (Figure 1).
Water samples and filter film samples were collected using Niskin bottles (1 L) from 10 to 20 cm below the surface during both ebb and flood in June 2020 (the flood season) and January 2021 (the dry season). The physicochemical parameters (temperature, salinity, and pH) of the water samples from each site were measured using a WTW Multi 3630 instrument (Weilheim, Germany). Total phosphorus (TP) was analyzed using the ammonium molybdate spectrophotometric method. The concentrations of nitrate−nitrogen (-N) and ammonia−nitrogen (-N) in the water were determined using the ultraviolet spectrophotometric method and the sodium salicylate-sodium hypochlorite spectrophotometric method, respectively. Before further treatment, referring to Call et al. (2019), the water samples were collected using a polypropylene syringe (previously rinsed with sample), filtered through 0.7-μm Whatman GF/F filters, and stored in a 4°C incubator. The suspended particulate matter (SPM) was weighed after drying at 60°C.The samples used to determine the POC content and for the stable carbon isotope analysis were then treated with 1 M HCl solution for 24 h to remove inorganic carbon. The organic carbon and total nitrogen contents were determined using an elemental analyzer (Elementar Macrocube, Germany). The stable carbon isotopes were measured using an elemental analyzer coupled with an isotope ratio mass spectrometer (Finnigan Delta V Advantage, Thermo Fisher Scientific, Inc.) in the stable isotope laboratory jointly operated by Shenzhen City Huake Precision Testing, Inc., and the Graduate School in Shenzhen, Tsinghua University. The stable isotope ratios are reported in delta notation as follows:
The DIC and DOC were collected in precombusted 50-ml borosilicate vials containing 100 μl of saturated HgCl2 solution, with no headspace or bubbles. These samples were stored at 4°C in a refrigerator (Call et al., 2019). The DIC and DOC were both analyzed using an organic carbon analyzer (TOCL CSH CSN; Shimadzu Corp., Japan) with precisions of 0.01 and 0.02 mM, respectively.
Statistical Analysis
For POC source analysis, firstly, one-way analysis of variance (ANOVA) revealed that the δ13CPOC and δ15N values of the mariculture organic matter, marine phytoplankton, mangrove plants, and S. alterniflora in the ZJE were all significantly different from each other (p< 0.05). A Bayesian-mixing isotope model, MixSIAR, was used to estimate the probability distributions of the contributions of the sources to the mixtures (Moore and Semmens, 2008). We set the isotope fractionation as 0‰. The δ13CPOC and δ15N of the sources are presented in Table 1. The spatiotemporal features of the carbon components were analyzed using kriging interpolation (Arcgis10.2, Esri USA). Two-way ANOVA was conducted to determine the seasonal and spatial effects on the physicochemical parameters, carbon contents, and stable isotope signatures using the SPSS 22 software (SPSS for Windows, SPSS, Inc.). Redundancy analysis (RDA) was also conducted to explore the relationships between the carbon content and environmental parameters using Canoco 4.5.
Results
Physicochemical Properties of the Water Samples
The salinity (8.3‰–26.7‰), SPM (17.3–36.9 mg/L), temperature (16.2°C–22.9°C), pH (7.8–8.7), dissolved oxygen (DO; 5.6–13.4 mg/L), particulate nitrogen (PN; 0.08–0.6 mg/L), dissolved inorganic nitrogen (DIN; 0.6–4.3 mg/L), and dissolved inorganic phosphorus (DIP; 0.5–10.9 μmol/L) all exhibited significant seasonal differences (p< 0.01, Table 2). Except for PN and DIP, all of the components exhibited spatial differences, which may be the result of the mariculture drainage and its low DIP content.
Spatial and Seasonal Variability of Carbon Composition
In this study, the proportions of the carbon components were found to be as follows: DIC (70%) > DOC (21%) > POC (9%) (Figure 2B). In terms of seasonal variations, except for POC in N1, the POC, DOC, and DIC contents of were significantly higher in the flood season than those in the dry season (p< 0.05, Table 3; Figure 2A).
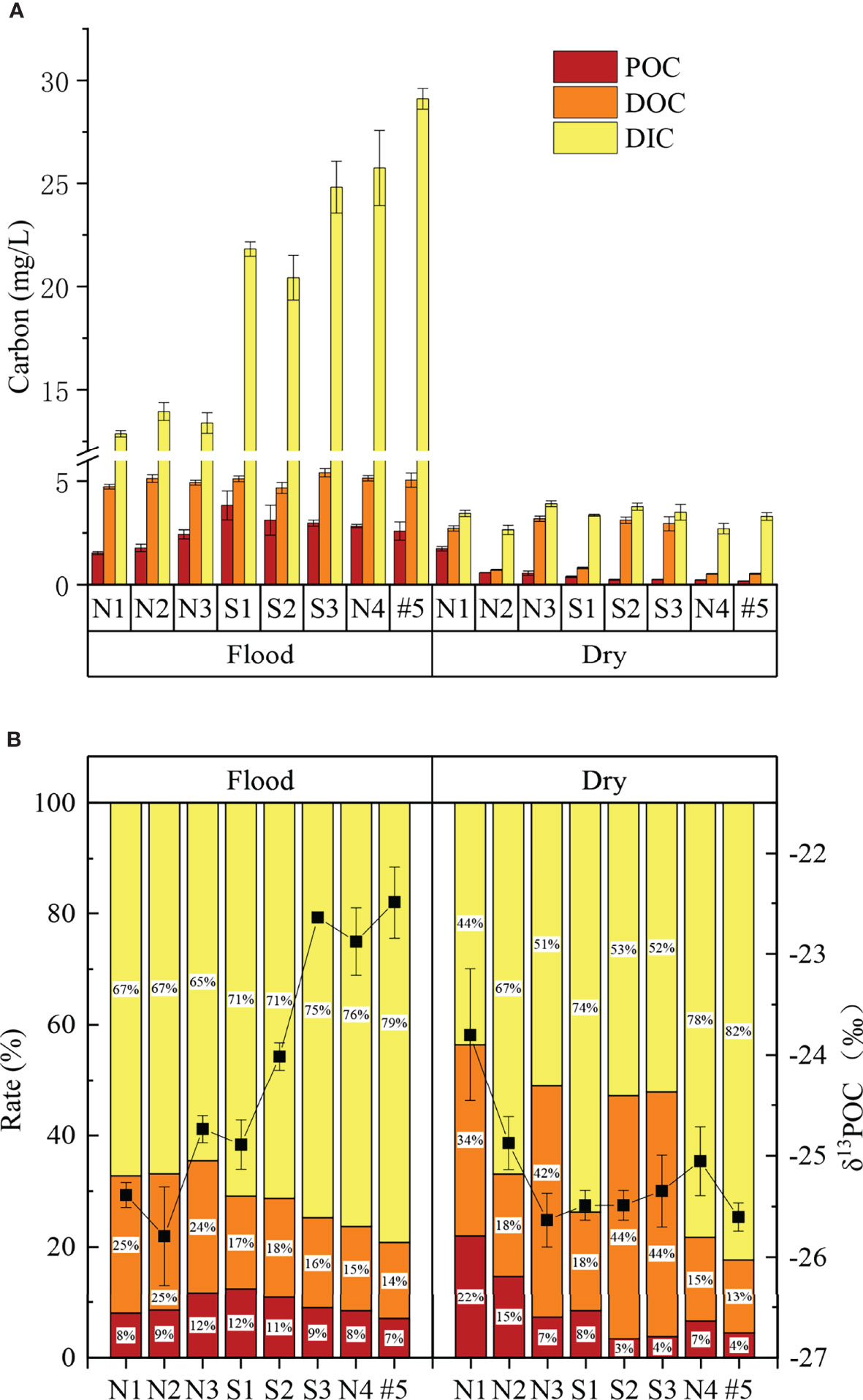
Figure 2 Carbon statistics (A, mean and standard error are shown), stacking plot of carbon rate and δ13POC (B, mean and standard error are shown) in the flood and dry seasons.
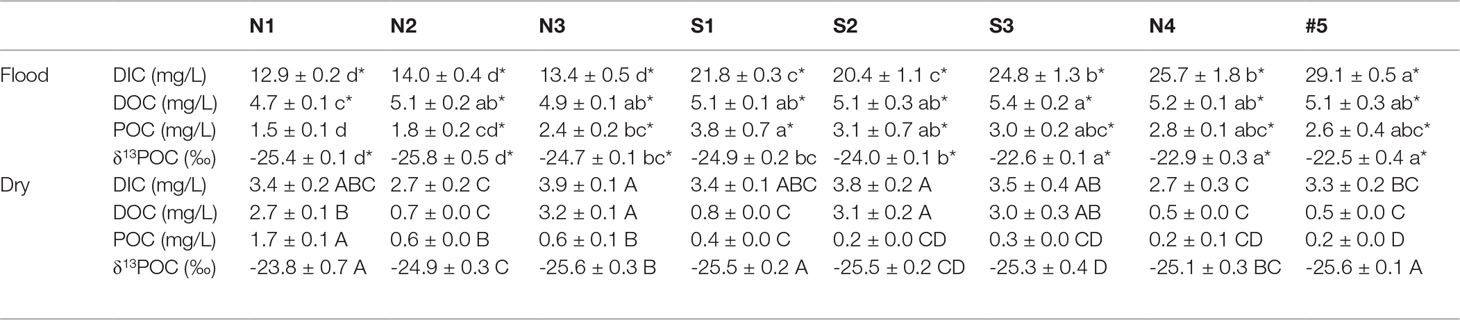
Table 3 Statistics of significant difference in the flood and dry seasons (mean ± SE, the uppercase and lowercase letters indicate the spatial difference in the flood season and dry season, respectively, and * indicates the seasonal difference at the same station, the differences are significant at p< 0.05).
The average DIC was 11.8 mg/L, accounting for 44%–82% of the total carbon. The DOC was significantly (about 2.8-fold) higher in the flood season than that in the dry season (Table 3). The DIC showed an increasing trend in both north Creek 1 (12.8–29.1 mg/L) and south Creek 2 (20.4–24.8 mg/L) in the flood season (Figure 3C; Table 3). In the dry season, the DIC contents ranged from 2.7 to 3.9 mg/L. The sites near the fringed mangrove (N3 and S1–S3) and the sluice (N1) showed significantly higher DOC contents than the other sites (Table 3; Figure 3F), indicating that the DIC may have been correlated with the mangrove in the dry season.
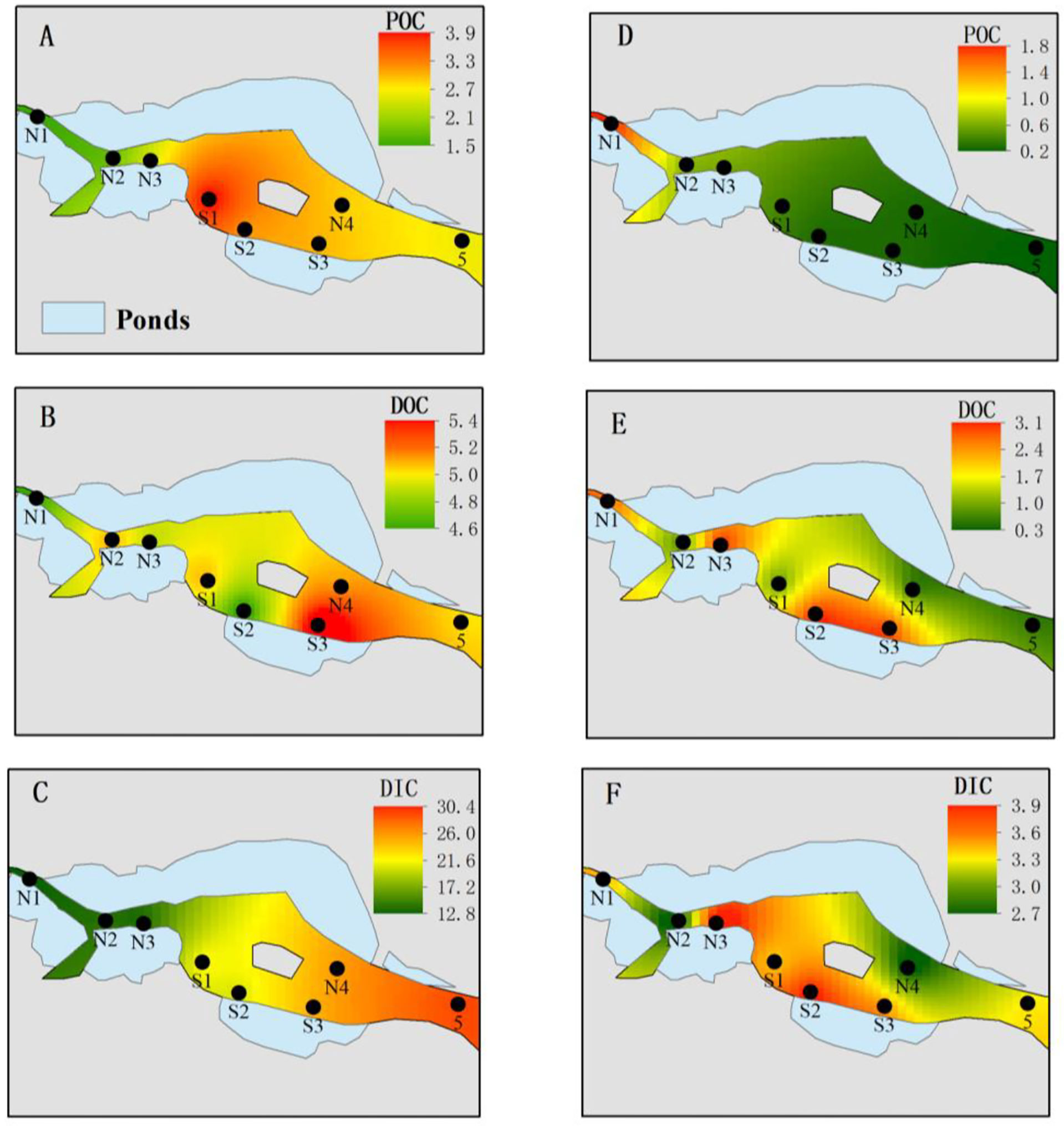
Figure 3 Spatial distribution of various carbons in ZJE during the flood (A–C) and dry (D–F) seasons. (A, D) Particulate organic carbon (POC; mg/L); (B, E) dissolved organic carbon (DOC; mg/L); (C, F) dissolved inorganic carbon (DIC; mg/L).
The average DOC content was 3.5 mg/L, accounting for 13%–44% of the total carbon. The DOC was significantly (about 2.8-fold) higher in the flood season than that in the dry season (Table 3). In the flood season, the DOC contents in all sites were significantly larger than that in N1 (p< 0.05), but no significant difference was found among them. The DOC exhibited a patchy distribution owing to the drainage from the mariculture ponds in the dry season.The average POC content was 1.57 mg/L, accounting for 3%–22% of the total carbon. The POC was significantly higher in the flood season than that in the dry season (about 5.1-fold that in the dry season; Table 3). The POC contents from N3 to the mouth #5 were significantly higher than that from N1 in the flood season (p< 0.05), while POC contents in the sites of N1–N3 were significantly higher than those in the other sites in the dry season (p< 0.05).
Stable Isotope Values and Sources of Particulate Organic Carbon
With average values of −25.4‰ to −22.5‰, the δ13CPOC values decreased successively from the upper reaches to the mouth. We divided the values into three groups according to the characteristics of the spatial differences, and the decreasing trend was as follows: Group 1 (S3, N4, #5) > Group 2 (N3, S1, S2) > Group 3 (N1, N2).
The contributions of the different sources to the POC in the ZJE in the flood season decreased in the following order: mariculture > mangrove > S. alterniflora > marine phytoplankton, with average values of 42.7%, 39.4%, 13.7%, and 4.1%, respectively. The contributions in the dry season changed to mariculture > mangrove > marine phytoplankton > S. alterniflora, with average values of 52.6%, 39.2%, 5.5%, and 2.5%, respectively. These results show that the POC mainly came from the mariculture ponds in the ZJE, mainly in the form of microalgae. As for the S. alterniflora and marine phytoplankton, both contributions exhibited seasonal variations. The decrease in the contribution from the mariculture and the increase in the contribution of the vegetation (mangrove, S. alterniflora) reflect the transition in the POC sources from the upper reaches to the mouth.
Relationship Between Carbon and Environmental Factors
The first two RDA dimensions were greater than 60% (Figure 4), so they can explain the carbon estuarine processes in the ZJE well. Negative relationships were observed between δ13CPOC and DIC and between POC and DOC (p< 0.05), reflecting the biochemical (such as microbial photosynthesis) and physical processes related to organic carbon.
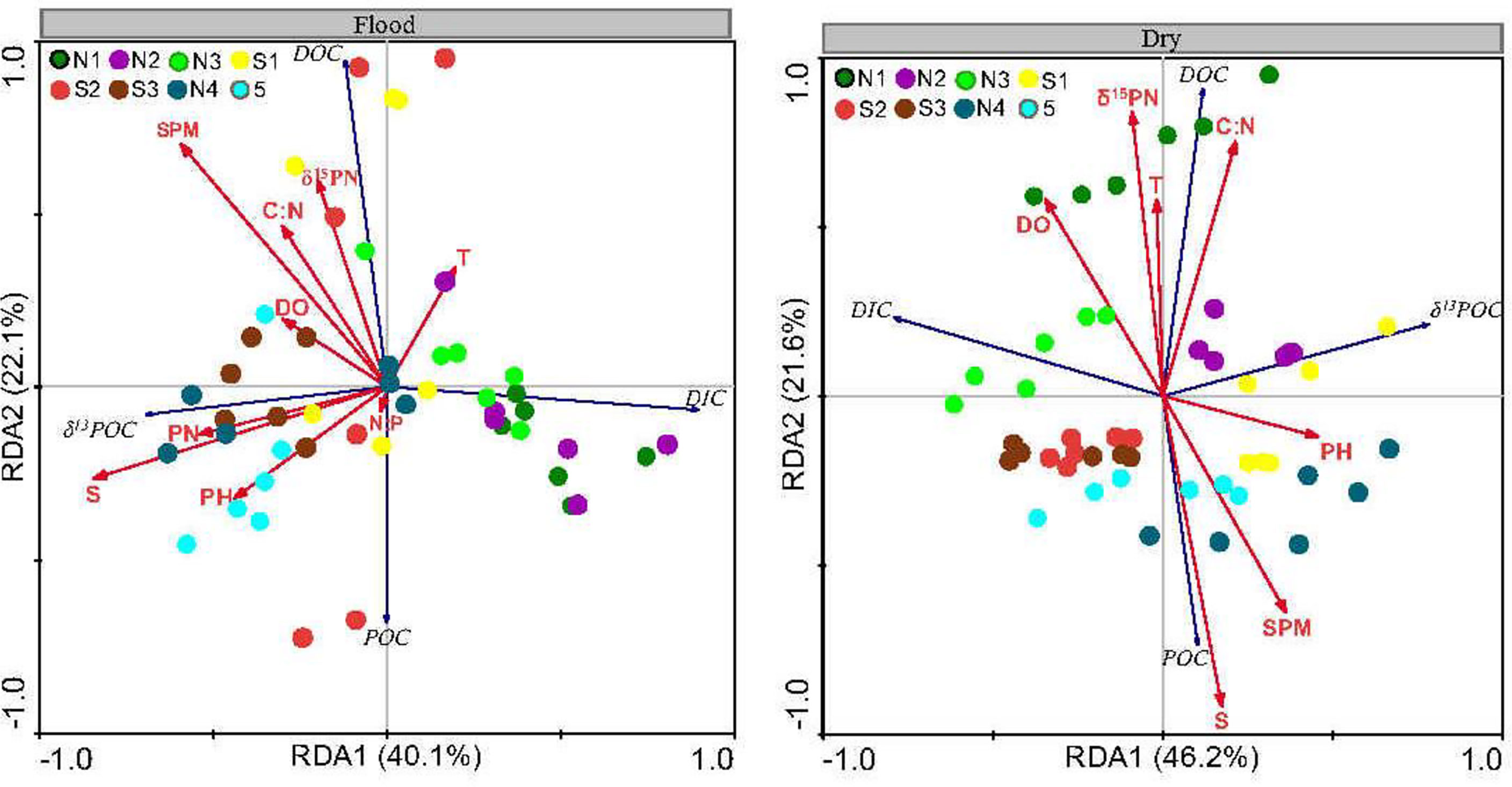
Figure 4 Redundancy analysis ordination showing the relationship between carbon elements and the environmental parameters in the flood and dry seasons (The colors mean different sites).
The temperature, salinity, DO, and DIN controlled the carbon composition. The highly negative correlation between the DIC and pH (p< 0.05), especially in the dry season, indicates that the pH directly affected the DIC in the water. The DOC was positively correlated with the DO, T, and C:N ratio in both the flood and dry seasons (p< 0.05). Moreover, the POC was positively correlated with the SPM and salinity (p< 0.05).
Discussion
Comparison With Carbon Compositions of Other Estuaries
The composition, spatial distribution, and transformation of carbon in the water in estuaries are closely related to the weathering degree of the basin, the dynamics, and the biochemical conditions (Adame and Lovelock, 2011; Bianchi and Bauer, 2011; Bauer et al., 2013; Borges et al., 2018; Pierre et al., 2018; Ray et al., 2018; Spivak et al., 2018). Globally, estuarine water is usually enriched in DIC (2.4–72 mg/L) (Lefèvre et al., 2017; Borges et al., 2018; Liu et al., 2018) owing to the net heterotrophic (Bauer et al., 2013) conditions, high pCO2, and correspondingly high rates of CO2 degassing in estuaries. Large estuaries, such as the Yangtze River Estuary and the Pearl River Estuary, have greater buffering capacities and retain CO2 in the water longer, leading to higher DIC contents (Cai, 2003). In this study, the water in the ZJE was found to have a relatively low DIC content (11.7 mg/L), which is similar to those of the Jiulong Estuary (13.85 mg/L), Sinnamary Estuary (13.38 mg/L), and Mekong Estuary (12.31 mg/L), but it is higher than that of the Hanjiang Estuary (7.96 mg/L), which does not contain mangrove (Figure 5). This indicates that the mangrove wetlands may act as an important net source of DIC. As the main form of carbon exported, DIC is mostly derived from mangrove mineralization and groundwater export (Bauer et al., 2013) in estuaries with mangrove ecosystems, contributing nearly 50% in the tidal channel (Bouillon et al., 2007a; Maher et al., 2013; Jeffrey et al., 2018).
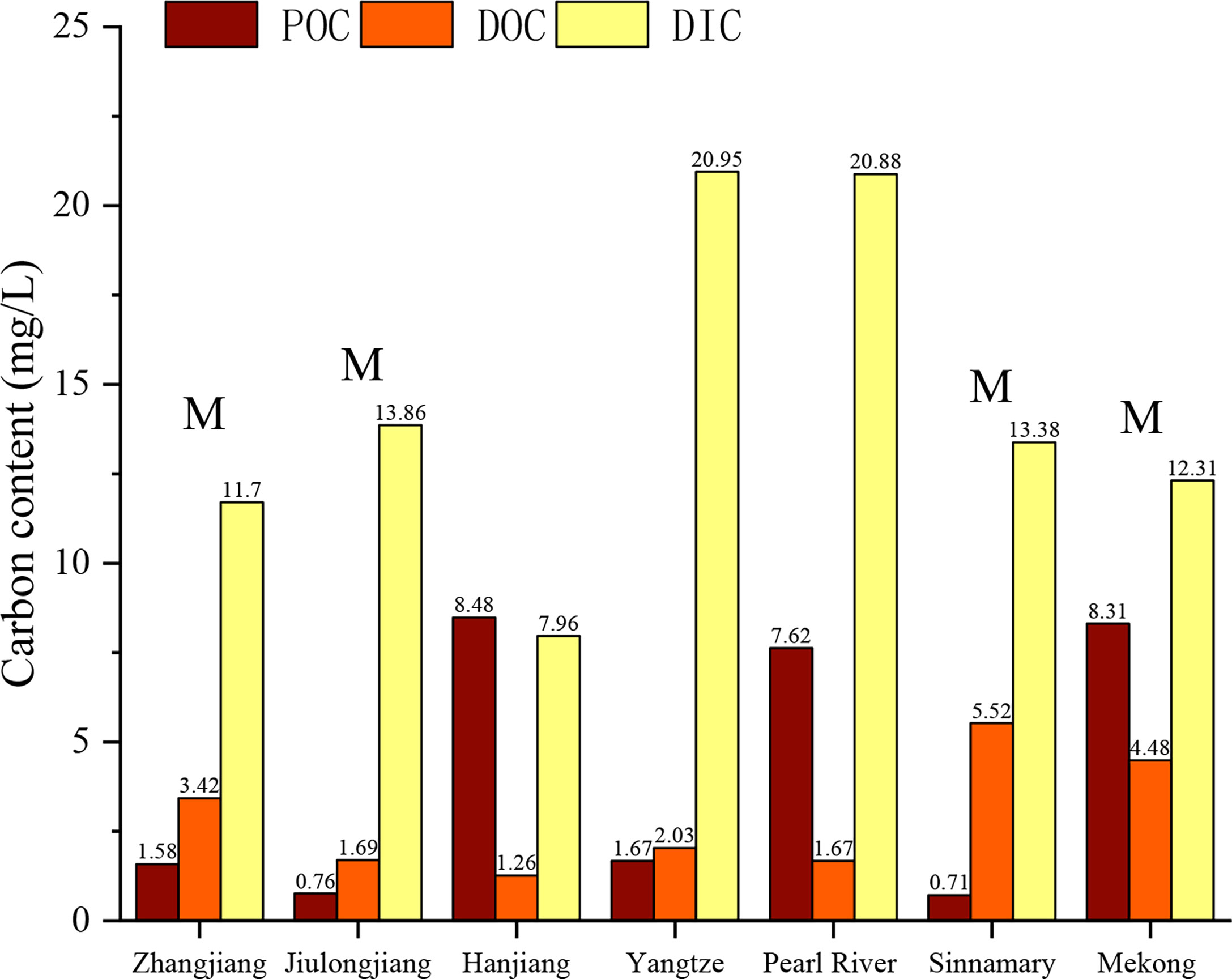
Figure 5 Comparison of carbon compositions in different estuarine regions including ZJE, Jiulong Estuary (Liu et al., 2018), Hanjiang Estuary (Liu et al., 2018), Yangtze, Pearl River Estuary (Liu et al., 2018), Sinnamary Estuary (Ray et al., 2018), and Mekong Estuary (Liu et al., 2018). M indicates that there are mangroves located in the estuary.
In terms of DOC, the DOC contents of tropical mangrove estuaries [such as the Sinnamary Estuary (5.52 mg/L) and Mekong Estuary (4.43 mg/L)] are much higher than those of other estuaries, regardless of the size of the estuary (Figure 5), indicating that the DOC content is sensitive to the climate zone of the estuaries. The Sinnamary Estuary is one of the mangrove areas with the highest growth rate (150 cm year−1) in the tropical regions (Ray et al., 2020). The hot and rainy climate accelerates leaching of the humus and organic detritus, and this included a series of processes (e.g., autotrophic activities, seawater mixing and dilution), resulting in the export of more DOC. The tidal dynamics and the mangrove area are secondary factors. The tidal range was found to influence the intensity of the pore water output because it controls the following: 1) the volume and surface area of the water in contact with the mangrove sediments at high tide and 2) the period and vertical extent of the pore water discharge based on the hydraulic advection phenomenon at low tide (Pierre et al., 2018). Thus, the larger the mangrove area is, the larger the contribution of the mangrove is. Under the same tidal amplitude, the water in the ZJE had a higher DOC content (3.42 mg/L) than that in the Jiulong Estuary (Liu et al., 2018) (1.69 mg/L) and the Hanjiang Estuary (Liu et al., 2018) (1.26 mg/L). This may be because the mangrove area is larger in the ZJE (660 ha) than those in the Jiulongjiang Estuary (360 ha) and the Hanjiang Estuary (0 ha).The POC supports microbial degradation and high trophic biological feeding. Earlier outwelling investigations have focused on detritus or POC and their uptake by consumers (Gattuso et al., 1998), and less attention has been paid to POC sources. Generally, primary producers (e.g., microalgae) wetland plants, and woody plants are the primary POC sources, so their relative abundances and compositions determine the amounts and forms of organic materials that persist and comprise the standing POC pool (Bianchi and Bauer, 2011). For estuaries with high runoffs (>270 km3 year−1), such as the Yangtze Estuary, the dominant input of terrestrial POC occurs during the high discharge period, and they account for 72%–86% total organic carbon (Bianchi and Bauer, 2011; Wang et al., 2012). This demonstrates the importance of allochthonous POC sources. However, the POC in the ZJE (1.58 mg/L) and the terrestrial-dominated POC in the Yangtze Estuary (1.67 mg/L) are approximately the same, indicating that POC derived from the coastal wetlands can be equal to that supplied by the river. For small estuaries with limited inflow of sediment, the coastal wetlands provide 90% of the organic carbon for the heterotrophic activities in these estuaries (Regnier et al., 2013), and thus, the larger the mangrove area is, the higher the organic detritus is, and the larger the POC content is. Additionally, the Jiulong Estuary is a small macro-tidal estuary (Wang et al., 2017), belonging to the same dynamic type with the ZJE that both estuaries have no riverine POC. Since 1995, the POC average content in Jiulong Estuary has little changed under normal conditions, and the in-suit POC production from phytoplankton can contribute 44% (Cai et al., 1998). While up to 86% POC was derived from mariculture activities in the ZJE, which may result in 2-fold higher POC content in ZJE water than that in Jiulong Estuary. However, the Sinnamary Estuary has one of the largest mangrove areas (1,134 ha), and the resulting high mineralization and respiration rate consume large amounts of POC (Bouillon et al., 2007a; Bouillon et al., 2007b; Liu et al., 2018), so the content is not as expected.
Carbon Dynamics in the Two Creeks in Zhangjiang Estuary
The two creeks in the ZJE have different hydrodynamics, which are reflected by their salinity (Pierre et al., 2018). North Creek 1, the main channel connecting the upper reaches to the downstream Dongshan Bay (a semi-enclosed bay), is heavily influenced by the input of seawater and mariculture effluents. From sites N1 to #5, the salinity ranges from 3‰ to 30‰, which is slightly below the salinity of marine water (35‰). This is likely because Dongshan Bay acts as a buffer, and it has been reported to have a water residence time, suggesting incomplete tidal flushing within this small bay (Call et al., 2019), and thus, the seawater salinity at site #5 is relatively low. The upper reaches, sites N1–N3, are distinctly influenced by the surrounding ponds. In addition, the adjacent steep topography may enhance the surface water runoff from the adjacent terrestrial environment into north Creek 1 (no rivers discharge into the upstream area). In contrast, the salinity of south creek 2 (S1–S3), representing the dynamic evolution of the tidal system, ranges from 6‰ to 22‰. Abundant evidence suggests that the composition of carbon in the creek water is controlled by the pore water input (Bouillon et al., 2007b; Sippo et al., 2017; Pierre et al., 2018; Call et al., 2019), despite the freshwater input during the flood season and the in situ biogeochemical processes.
The observations were conducted in both the flood and dry seasons. The POC distribution was found to be completely complementary despite the existing seasonal variations. In the flood season, the POC content was higher from the intertidal zone to both creeks (Creek 1: N4–#5, Creek 2: S1–S3). While in the dry season, the POC content had an decreasing trend from N1 to #5, and the POC content was significantly higher (p< 0.05) in the estuarine upper reaches (N1–N2). This indicates a high sediment load (associated with POC) from the mouth outside and that deposition-resuspension is also a very common phenomenon (María et al., 2016; Ray et al., 2020; Gao et al., 2021). This potential remobilization of sediments was observed to have a maximum velocity of 1 m/s, which is fast enough to exceed the threshold for the erosion of the sediments, resulting in an increase in the POC content. In addition, the vegetated tidal area (i.e., mangrove, S. alterniflora) had the maximum POC contents of 3.8 mg/L, which demonstrates the increase in the carbon storage capacity due to the interception and deposition of exogenous carbon (Li and Gao, 2013; Jiang et al., 2020; Zhang et al., 2021). In the dry season, the maximum POC content occurred in the upper reaches of Creek 1 (N1) (1.74 mg/L), but no seasonal difference was observed (p< 0.05). This indicates that the construction of the Beijiang sluice contributed to the change in the dynamic depositional conditions (Maavara et al., 2017; Pan et al., 2020; Gao et al., 2021). Before construction, the ZJE was affected by the fluvial runoff. After construction, the sediment dispersal was cut off, resulting in the accumulation of fine-grained sediments, i.e., a local POC sink.In this study, it was found that mariculture contributed a large amount of POC to the water in the coastal wetlands, i.e., 24.6%–86.7% based on the MixSIAR model. The POM in the ponds included microalga, fish bait residue, animal manure, and other substances from natural sources. The excessive food supply and metabolic by-products resulted in the increase in the POC content of the water (Li et al., 2016). In the Mahakam Delta in Indonesia, the carbon output from aquaculture ponds was found to be 525 Mg C ha−1, which is greater than that from mangrove and is equivalent to 266 years of carbon accumulation from natural mangrove (Arifanti et al., 2019). Feng et al. (2017) used MixSIR to analyze the food sources of the fish in the ZJE and found that the contribution of benthic microalgae was up to 80%. Baiting behavior is generally selective, and the contribution of POC to the food web is controversial. The suspended particles in ponds are similar to those of benthic microalgae in terms of their stable isotope compositions and C/N ratios (Spivak et al., 2018). Therefore, most of the POC in the ZJE may come from ponds, and the outwelling form could be microalgae.The DOC and DIC exhibited different spatial patterns in both seasons. In the flood season, except for N1, DOC in all sites had no significant differences, and DIC had an upward trend with salinity increasing. However, the DOC contents in both mangrove creeks, N3–#5 in Creek 1 and S1–S3 in Creek 2, had no significant differences (Table 3). This may be due to the input of pore water via the tidal pump. In mangrove creeks, many studies have shown that the DOC is controlled by the pore water (Bouillon et al., 2007a; Bouillon et al., 2007b; Maher et al., 2013; Pierre et al., 2018; Call et al., 2019; Ohtsuka et al., 2020). During ebb tides, the water that retreats from the tidal creek has a composition similar to that of the pore water, which explains >60% of the DOC and >90% of the DIC (Bouillon et al., 2007a; Maher et al., 2013; Pierre et al., 2018; Call et al., 2019; Ohtsuka et al., 2020).In the dry season, the effects of sluice construction and mariculture should be taken into account. The high DOC and DIC contents occurred near the Beijiang sluice (i.e., site N1) and the area with intensive mariculture (i.e., sites N3, S2, and S3). Under the closure effect of the Bejiang sluice, compared with the POC, the water exchange was limited and the residence time was longer, resulting in enrichment of terrestrial DOC and DIC near the sluice (i.e., 2.7 and 3.4 mg/L, respectively). The mariculture drainage resulted in the patchy distribution of the DOC. In southern China, aquaculture ponds are usually located adjacent to mangroves, and they are constructed by simply excavating mangrove forests. Sites N3, S2, and S3 were located close to aquaculture pond drainage outlets, resulting in DOC contents of 3.2, 3.1, and 2.9 mg/L, respectively, that is, 3.6–6.4-fold increases compared to the other sites. Following the decreasing trend of the nutrient concentration (Wu et al., 2014), the propagation distance of the DOC from the ponds was found to be about 3 km in the direction toward the sea. Although this is less than the distance to the coast, under certain conditions (such as typhoons, astronomical tides, and human activities), this carbon may be transported to the open sea. The short transport distance may be continuous and could lead to secondary pollution of the organic matter in the estuary.
Effects of Mangrove on Carbon Outwelling
Undoubtedly, mangrove forests have large organic carbon reserves, with a global mean total forest carbon reserve of 692. 8 ± 23.1 Mg C ha−1. However, sequestered carbon, especially at depths of 0–1 m, is susceptible to remineralization and tidal export of DOC and DIC, since nearly all of this carbon respired within the entire soil horizon is released in a dissolved form (Bouillon et al., 2007b; Borges et al., 2018; Pierre et al., 2018; Alongi, 2022). The export of leaves, bark, branches, twigs, and other plant litter represents the most obvious loss of POC from mangrove, accounting for roughly 50% of the mangrove litterfall (Alongi, 2014). This indicates that mangrove can act as an important POC source.
In this study, site S1, which was located near the mangrove outwelling outlet during the flood season, had DOC, DIC, and POC contents that were on average 6.3-, 6.6-, and 10-fold higher, respectively, than those of the other sites. Two different processes may explain the similar ranges of the seasonal concentrations. First, litterfall is expected to be higher during the flood season as a result of the higher mangrove-derived organic matter driven by rainfall and temperature (Alongi, 2014). This litter is rapidly decomposed and leached, resulting in the greater contribution of the mangrove during the flood season (52.2% in the flood season, >27.5% in the dry season; Table 4). Second, the significantly negative relationship between the δ13CPOC and DIC (r2 = 0.85; Figure 4) explains the δ13C of the microbial autotrophic activity under sufficient DIC. Identically, the highest δ13CPOC values and lowest DIC occurred during the cyanobacteria bloom (Chomicki, 2010). Even in arid mangrove, planktonic particles contributed mostly to the POC pool (10%–65%) (Ray and Weigt, 2018). In addition, the relationships between δ13CPOC and the other parameters (i.e., PN, DO, and C/N) illustrate the need for microbial autotrophic processes, implying the existence of this microbial activity.
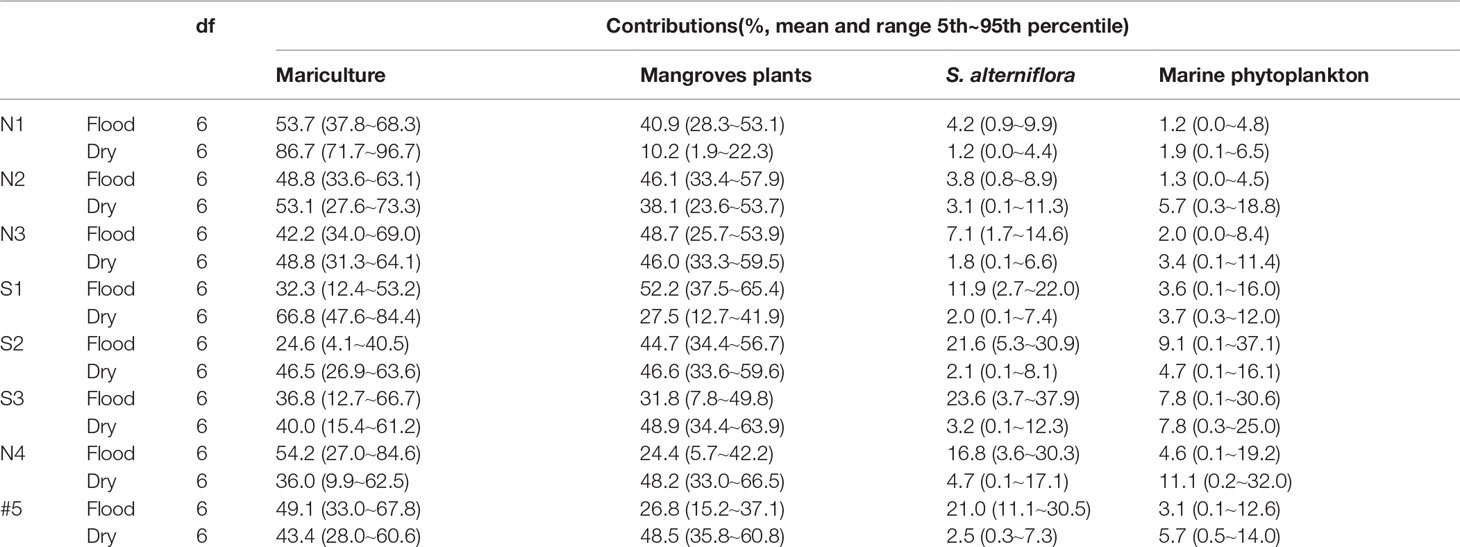
Table 4 Proportional contributions of different sources to POC in the flood and dry seasons (aquaculture, mangroves, S. alterniflora, and marine phytoplankton).
Mangroves cover only 0.3% of the global ocean coastal areas, but they contribute 41% of the DIC + DOC + POC export to the adjacent coastal areas of ocean globally (Alongi, 2020). The high concentrations of DIC and DOC in the pore water pool and the subsurface transport result in a significant export of DIC and DOC to the adjacent tidal waters of the ocean in many places (Maher et al., 2013; Ray et al., 2015; Liu et al., 2018; Pierre et al., 2018; Ray et al., 2018; Call et al., 2019; Santos et al., 2019). These results are consistent in revealing the high rates of export, and the mean DIC and DOC contents were found to be 339.6 and 229.1 mg/L, respectively. Large amounts of dissolved carbon may be exported from mangroves, possibly accounting for as much as 112–160 Tg a−1 of mangrove carbon, which could help to balance the budgets constructed by Bouillon et al. (2008a). As was reported, 41% of the litter produced is exported to the adjacent tidal waters, with 25.1 mmol m−2 d−1. In this study, the mangrove-derived POC was determined to be 10.2%–52.2%. Even in the world’s largest mangrove region (e.g., Sundarbans), the mangroves contribute ~60% (Ray et al., 2015), while in the Sinnamary Estuary, South America, the contribution is 70%, including terrestrial and benthic microalgae (Ray et al., 2018). Therefore, we confirm the viewpoint that mangrove contributions are conservative.In this study, we investigated the three types of carbon involved in estuary processes, the source contributions, and the contents of the carbon components in different regions. However, we prefer to obtain the net flux, that is, the total carbon outwelling to the ocean every year. We suggest that the tidal flux at the mouth of the cross section be estimated to measure the carbon flux in the tidal cycle, which may provide a scientific basis for understanding the carbon budget and outwelling estimates from the mangroves in the ZJE.
Conclusions
In this paper, the spatiotemporal variability and mangrove outwelling of DOC, DIC, and POC in a mangrove-fringed estuary in Zhangjiang, China, were examined. DIC was the dominant form in the water. The content of all of the types of carbon was significantly higher in the flood season than in the dry season. The POC exhibited seasonally complementary features, that is, the contents were high in the middle reaches and mouth in the flood season and in the upper reaches in the dry season. The DOC and DIC both increased with increasing salinity during the flood season, but they exhibited patchy and fringed-patchy distributions, respectively. The spatial characteristics of the DIC, DOC, and POC reveal the profound effects of the Beijiang sluice and mariculture. Specifically, an average of 47.7% of the POC was from the mariculture effluent based on the stable isotope compositions, mainly in the form of microalgae. Additionally, the contribution of the mangrove was relatively limited, with 36.2% on average. Thus, global carbon outwelling estimates from mangroves differ owing to differences in the methodologies and hydroclimatic settings. An overall understanding of mangrove outwelling on the semi-diurnal and spring-neap-spring timescales in the ZJE will be obtained in future studies.
Data Availability Statement
The original contributions presented in the study are included in the article/supplementary material. Further inquiries can be directed to the corresponding authors.
Author Contributions
RY: study conception, investigation, data analysis and manuscript draft preparation. JF, QY and LN: project administration, funding support, conceptualization, supervision, and writing—review and editing. RY, JF, YW, LF, XL: sampling support and methodology. All authors contributed to the article and approved the submitted version of the manuscript.
Funding
This study was financially supported by grants by the National Natural Science Foundation of China (Grant No. 41976160, 41706090, 51709289) and the Fundamental Research Funds for the Central Universities, Sun Yat-sen University (Grant No. 22qntd2202).
Conflict of Interest
The authors declare that the research was conducted in the absence of any commercial or financial relationships that could be construed as a potential conflict of interest.
Publisher’s Note
All claims expressed in this article are solely those of the authors and do not necessarily represent those of their affiliated organizations, or those of the publisher, the editors and the reviewers. Any product that may be evaluated in this article, or claim that may be made by its manufacturer, is not guaranteed or endorsed by the publisher.
Acknowledgments
We were sincerely grateful for the support provided by Shuai Hu, Hao Yang, Ping Zhang, Haiwei Li, Tao Zhang, Ou Chen, Jie Huang, Fangting He, Enmao Huang, Tao Fu, Da Wan, Jiamin Yuan etc. for the field work and data acquisition. We also appreciate the anonymous reviewers for their constructive suggestions.
References
Adame M. F., Lovelock C. E. (2011). Carbon and Nutrient Exchange of Mangrove Forests With the Coastal Ocean. Hydrobiologia 663, 23–50. doi: 10.1007/s10750-010-0554-7
Alongi D. M. (2014). Carbon Cycling and Storage in Mangrove Forests. Annu. Rev. Mar. Sci. 6, 195–219. doi: 10.1146/annurev-marine-010213-135020
Alongi D. M. (2020). Carbon Cycling in the World’s Mangrove Ecosystems Revisited: Significance of Non-Steady State Diagenesis and Subsurface Linkages Between the Forest Floor and the Coastal Ocean. Forests 11 (9), 977. doi: 10.3390/f11090977
Alongi D. M. (2022). Impacts of Climate Change on Blue Carbon Stocks and Fluxes in Mangrove Forests. Forests 13(2), 149. doi: 10.3390/f13020149
Arifanti V. B., Kauffman J. B., Hadriyanto D., Murdiyarso D., Diana R. (2019). Carbon Dynamics and Land Use Carbon Footprints in Mangrove-Converted Aquaculture: The Case of the Mahakam Delta, Indonesia. For. Ecol. Manage. 432, 17–29. doi: 10.1016/j.foreco.2018.08.047
Bauer J. E., Cai W.-J., Raymond P. A., Bianchi T. S., Hopkinson C. S., Regnier P. (2013). The Changing Carbon Cycle of the Coastal Ocean. Nature 504, 61–70. doi: 10.1038/nature12857
Bianchi T. S., Bauer J. E. (2011). “Particulate Organic Carbon Cycling and Transformation,” in Treatise on Estuarine and Coastal Science, vol. Vol 5. (Waltham: Academic Press), 69–117.
Borges A., Abril G., Bouillon S. (2018). Carbon Dynamics and CO2 and CH4 Outgassing in the Mekong Delta. Biogeosciences 15, 1093–1114. doi: 10.5194/bg-15-1093-2018
Bouillon S., Borges A. V., Castañeda-Moya E., Diele K., Dittmar T., Duke N. C., et al. (2008a). Mangrove Production and Carbon Sinks: A Revision of Global Budget Estimates. Global Biogeochem. Cycles. 22, GB2013. doi: 10.1029/2007GB003052
Bouillon S., Connolly R. M., Lee S. Y. (2008b). Organic Matter Exchange and Cycling in Mangrove Ecosystems: Recent Insights From Stable Isotope Studies. J. Sea. Res. 59, 44–58. doi: 10.1016/J.SEARES.2007.05.001
Bouillon S., Dehairs F., Velimirov B., Abril G., Borges A. V. (2007a). Dynamics of Organic and Inorganic Carbon Across Contiguous Mangrove and Seagrass Systems (Gazi Bay, Kenya). J. Geophys. Res.: Biogeosci. 112, GO2018. doi: 10.1029/2006JG000325
Bouillon S., Middelburg J. J., Dehairs F., Borges A. V., Abril G., Flindt M. R., et al. (2007b). Importance of Intertidal Sediment Processes and Porewater Exchange on the Water Column Biogeochemistry in a Pristine Mangrove Creek (Ras Dege, Tanzania). Biogeosciences 4, 311–322. doi: 10.5194/bg-4-311-2007
Cabral A. C., Wilhelm M. M., Figueira R. C. L., Martins C. C. (2019). Tracking the Historical Sewage Input in South American Subtropical Estuarine Systems Based on Faecal Sterols and Bulk Organic Matter Stable Isotopes (δ13c and δ15n). Sci. Total. Environ. 655, 855–864. doi: 10.1016/j.scitotenv.2018.11.150
Cai W.-J. (2003). Riverine Inorganic Carbon Flux and Rate of Biological Uptake in the Mississippi River Plume. Geophys. Res. Lett. 30 (2), 1032. doi: 10.1029/2002GL016312
Cai Y., Guo L., Douglas T. A. (2008). Temporal Variations in Organic Carbon Species and Fluxes From the Chena River, Alaska. Limnol. Oceanogr. 53, 1408–1419. doi: 10.4319/lo.2008.53.4.1408
Cai A., Li W., Chen Q., Wang X. (1998). Studies of Particulate Organic Carbon in the Xiamen Western Harbour and the Jiulong River Estuary. Mar. Sci. 2, 37–41.
Call M., Sanders C. J., Macklin P. A., Santos I. R., Maher D. T. (2019). Carbon Outwelling and Emissions From Two Contrasting Mangrove Creeks During the Monsoon Storm Season in Palau, Micronesia. Estuarine. Coast. Shelf. Sci. 218, 340–348. doi: 10.1016/j.ecss.2019.01.002
Chomicki K. (2010). The Use of Stable Carbon and Oxygen Isotopes to Examine the Fate of Dissolved Organic Matter in Two Small, Oligotrophic Canadian Shield Lakes. [dissertation/doctor’s thesis]. [Ontario (IL)]: University of Waterloo.
Donato D. C., Kauffman J. B., Murdiyarso D., Kurnianto S., Stidham M., Kanninen M. (2011). Mangroves Among the Most Carbon-Rich Forests in the Tropics. Nat. Geosci. 4, 293–297. doi: 10.1038/ngeo1123
Feng J., Zhou J., Wang L., Cui X., Ning C., Wu H., et al. (2017). Effects of Short-Term Invasion of Spartina Alterniflora and the Subsequent Restoration of Native Mangroves on the Soil Organic Carbon, Nitrogen and Phosphorus Stock. Chemosphere 184, 774–783. doi: 10.1016/j.chemosphere.2017.06.060
Gao C., Yu F., Chen J., Huang Z., Jiang Y., Zhuang Z., et al. (2021). Anthropogenic Impact on the Organic Carbon Sources, Transport and Distribution in a Subtropical Semi-Enclosed Bay. Sci. Total. Environ. 767, 145047. doi: 10.1016/j.scitotenv.2021.145047
Gattuso J. P., Frankignoulle M., Wollast R. (1998). Carbon and Carbonate Metabolism in Coastal Aquatic Ecosystems. Annu. Rev. Ecol. Systematics. 29, 405–434. doi: 10.1146/annurev.ecolsys.29.1.405
Huang Q. (2013). Stable Isotope Studies on Food Sources of the Razor Clams (Sinonovacula constricta) in Two Cultivation Modes in Zhangjiangkou Estuary, Fujian. [dissertation/master’s thesis]. [Xiamen (IL)]: Xiamen University
Hunt J. (1970). The Significance of Carbon Isotope Variations in Marine Sediments. Adv. Organic. Geochem., 27–35.
Jeffrey L. C., Maher D. T., Santos I. R., Call M., Reading M. J., Holloway C., et al. (2018). The Spatial and Temporal Drivers of Pco2, Pch4 and Gas Transfer Velocity Within a Subtropical Estuary. Estuarine. Coast. Shelf. Sci. 208, 83–95. doi: 10.1016/j.ecss.2018.04.022
Jiang R., Cheng P., Gao J., Wang A. (2020). Impacts of Mangrove on the Dynamic Process of Bottom Boundary Layer. Mar. Geo. Front. 36, 37–44. doi: 10.16028/j.1009-2722.2019.108
Kamruzzaman M., Ahmed S., Osawa A. (2017). Biomass and Net Primary Productivity of Mangrove Communities Along the Oligohaline Zone of Sundarbans, Bangladesh. For. Ecosyst. 4, 16. doi: 10.1186/s40663-017-0104-0
Lee S. Y. (1995). Mangrove Outwelling: A Review. Hydrobiologia 295, 203–212. doi: 10.1007/BF00029127
Lefèvre N., Flores M., Gaspar F., Rocha C., Jiang S., Araujo M., et al. (2017). Net Heterotrophy in the Amazon Continental Shelf Changes Rapidly to a Sink of CO2 in the Outer Amazon Plume. Front. Mar. Sci. 4. doi: 10.3389/fmars.2017.00278
Letourneau M. L., Schaefer S. C., Chen H., Mckenna A. M., Alber M., Medeiros P. M. (2021). Spatio-Temporal Changes in Dissolved Organic Matter Composition Along the Salinity Gradient of a Marsh-Influenced Estuarine Complex. Limnol. Oceanogr. 66, 3040–3054. doi: 10.1002/lno.11857
Li J., Gao S. (2013). Estimating Deposition Rates Using a Morphological Proxy of Spartina Alterniflora Plants. J. Coast. Res. 29, 1452–1463. doi: 10.2112/JCOASTRES-D-12-00186.1
Li R., Liu S., Zhang J., Jiang Z., Fang J. (2016). Sources and Export of Nutrients Associated With Integrated Multi-Trophic Aquaculture in Sanggou Bay, China. Aquacult. Environ. Interact. 8, 285–309. doi: 10.3354/aei00177
Liu Q., Guo X., Yin Z., Zhou K., Roberts E., Dai M. (2018). Carbon Fluxes in the China Seas: An Overview and Perspective. Sci. China Earth Sci. 61, 1564–1582. doi: 10.1007/s11430-017-9267-4
Maavara T., Lauerwald R., Regnier P., Van Cappellen P. (2017). Global Perturbation of Organic Carbon Cycling by River Damming. Nat. Commun. 8, 15347. doi: 10.1038/ncomms15347
Maher D. T., Santos I. R., Golsby-Smith L., Gleeson J., Eyre B. D. (2013). Groundwater-Derived Dissolved Inorganic and Organic Carbon Exports From a Mangrove Tidal Creek: The Missing Mangrove Carbon Sink? Limnol. Oceanogr. 58, 457–488. doi: 10.4319/lo.2013.58.2.0475
María C. M., Ana L. D., Anabela A. B., María C. P., Mónica S. H. (2016). Seasonal and Tidal Dynamics of Water Temperature, Salinity, Chlorophyll-A, Suspended Particulate Matter, Particulate Organic Matter, and Zooplankton Abundance in a Shallow, Mixed Estuary (Bahía Blanca, Argentina). J. Coast. Res. 32, 1051–1061. doi: 10.2112/JCOASTRES-D-14-00236.1
Mcleod E., Chmura G. L., Bouillon S., Salm R., Björk M., Duarte C. M., et al. (2011). A Blueprint for Blue Carbon: Toward an Improved Understanding of the Role of Vegetated Coastal Habitats in Sequestering CO2. Front. Ecol. Environ. 9, 552–560. doi: 10.1890/110004
Moore J. W., Semmens B. X. (2008). Incorporating Uncertainty and Prior Information Into Stable Isotope Mixing Models. Ecol. Lett. 11, 470–480. doi: 10.1111/j.1461-0248.2008.01163.x
Ohtsuka T., Onishi T., Yoshitake S., Tomotsune M., Kida M., Iimura Y., et al. (2020). Lateral Export of Dissolved Inorganic and Organic Carbon From a Small Mangrove Estuary With Tidal Fluctuation. Forests 11(10) 1041. doi: 10.3390/f11101041
Pan D., Wang Z., Zhan Q., Saito Y., Wu H., Yang S., et al. (2020). Organic Geochemical Evidence of Past Changes in Hydro- and Sediment-Dynamic Processes at River Mouths: A Case Study of Holocene Sedimentary Records in the Changjiang River Delta, China. Continent. Shelf. Res. 204, 104189. doi: 10.1016/j.csr.2020.104189
Peterson B., Howarth R., Garritt H. (1985). Multiple Stable Isotopes Used to Trace the Flow of Organic Matter in Estuarine Food Webs. Sci. (New. York. N.Y.) 227, 1361–1363. doi: 10.1126/science.227.4692.1361
Pierre T., Ziegler A. D., Friess D. A., David W., Vinh T. V., Frank D., et al. (2018). Carbon Dynamics and Inconstant Porewater Input in a Mangrove Tidal Creek Over Contrasting Seasons and Tidal Amplitudes. Geochimica. Cosmochimica. Acta 237, 32–48. doi: 10.1016/j.gca.2018.06.012
Rani V., Bijoy Nandan S., Schwing P. T. (2021). Carbon Source Characterisation and Historical Carbon Burial in Three Mangrove Ecosystems on the South West Coast of India. CATENA 197, 104980. doi: 10.1016/j.catena.2020.104980
Ray R., Michaud E., Aller R. C., Vantrepotte V., Gleixner G., Walcker R., et al. (2018). The Sources and Distribution of Carbon (DOC, POC, DIC) in a Mangrove Dominated Estuary (French Guiana, South America). Biogeochemistry 138, 297–321. doi: 10.1007/s10533-018-0447-9
Ray R., Rixen T., Baum A., Gleixner, Jana T. K. (2015). Distribution, Sources and Biogeochemistry of Organic Matter in a Mangrove Dominated Estuarine System (Indian Sundarbans) During the Pre-Monsoon. Estuar.Coast. Shelf. Sci. 167, 404–413. doi: 10.1016/j.ecss.2015.10.017
Ray R., Thouzeau G., Walcker R., Vantrepotte V., Gleixner G., Morvan S., et al. (2020). Mangrove-Derived Organic and Inorganic Carbon Exchanges Between the Sinnamary Estuarine System (French Guiana, South America) and the Atlantic Ocean. J. Geophys. Res.: Biogeosci. 125, e2020JG005739. doi: 10.1029/2020JG005739
Ray R., Weigt M. (2018). Seasonal and Habitat-Wise Variations of Creek Water Particulate and Dissolved Organic Carbon in Arid Mangrove (the Persian Gulf). Continent. Shelf. Res. 165, 60–70. doi: 10.1016/j.csr.2018.06.009
Regnier P., Friedlingstein P., Ciais P., Mackenzie F. T., Gruber N., Janssens I. A., et al. (2013). Anthropogenic Perturbation of the Carbon Fluxes From Land to Ocean. Nat. Geosci. 6, 597–607. doi: 10.1038/ngeo1830
Santos I. R., Maher D. T., Larkin R., Webb J. R., Sanders C. J. (2019). Carbon Outwelling and Outgassing vs. Burial in an Estuarine Tidal Creek Surrounded by Mangrove and Saltmarsh Wetlands. Limnol. Oceanogr. 64, 996–1013. doi: 10.1002/lno.11090
Schulte P., Van Geldern R., Freitag H., Karim A., Négrel P., Petelet-Giraud E., et al. (2011). Applications of Stable Water and Carbon Isotopes in Watershed Research: Weathering, Carbon Cycling, and Water Balances. Earth-Science. Rev. 109, 20–31. doi: 10.1016/j.earscirev.2011.07.003
Sippo J. Z., Maher D. T., Tait D. R., Ruiz-Halpern S., Sanders C. J., Santos I. R. (2017). Mangrove Outwelling is a Significant Source of Oceanic Exchangeable Organic Carbon. Limnol. Oceanogr. Lett. 2, 1–8. doi: 10.1002/lol2.10031
Spivak A. C., Gosselin K. M., Sylva S. P. (2018). Shallow Ponds are Biogeochemically Distinct Habitats in Salt Marsh Ecosystems. Limnol. Oceanogr. 63, 1622–1642. doi: 10.1002/lno.10797
Wang X., Ma H., Li R., Song Z., Wu J. (2012). Seasonal Fluxes and Source Variation of Organic Carbon Transported by Two Major Chinese Rivers: The Yellow River and Changjiang (Yangtze) River. Global Biogeochem. Cycles. 26, 1–10 GB2025. doi: 10.1029/2011GB004130
Wang A., Ye X., Cheng P., Wang L. (2017). Trapping Effect of Estuarine Turbidity Maximum on Particulate Organic Carbon and its Response to a Typhoon Event in a Macro-Tidal Estuary. in 19th EGU General Assembly, EGU2017: Proceedings from the Conference, 2017 April 23-28; Vienna, Austria: 2017. p. 115. URL: https://ui.adsabs.harvard.edu/abs/2017EGUGA.19.115W
Wu H., Peng R., Yang Y., He L., Wang W., Zheng T., et al. (2014). Mariculture Pond Influence on Mangrove Areas in South China: Significantly Larger Nitrogen and Phosphorus Loadings From Sediment Wash-Out Than From Tidal Water Exchange. Aquaculture 426-427, 204–212. doi: 10.1016/j.aquaculture.2014.02.009
Zhang Y. M., Deng H. H. (2019). Preliminary Analysis and Calculation of Runoff About Yunxiao Pumped Storage Hydropower Station in Fujian Province. Guangdong. Water Resour. Hydropowe. 04, 44–48. doi: 10.11905/j.issn.1008-0112.2019.04.011
Zhang Y., Yu C., Xie J., Du S., Feng J., Guan D. (2021). Comparison of Fine Root Biomass and Soil Organic Carbon Stock Between Exotic and Native Mangrove. CATENA 204, 105423. doi: 10.1016/j.catena.2021.105423
Zhou J., Wu Y., Zhang J., Kang Q., Liu Z. (2006). Carbon and Nitrogen Composition and Stable Isotope as Potential Indicators of Source and Fate of Organic Matter in the Salt Marsh of the Changjiang Estuary, China. Chemosphere 65, 310–317. doi: 10.1016/j.chemosphere.2006.02.026
Keywords: carbon pools, mangroves, spatiotemporal distribution, sources, anthropogenic activities
Citation: Yan R, Feng J, Wang Y, Fu L, Luo X, Niu L and Yang Q (2022) Distribution, Sources, and Biogeochemistry of Carbon Pools (DIC, DOC, and POC) in the Mangrove-Fringed Zhangjiang Estuary, China. Front. Mar. Sci. 9:909839. doi: 10.3389/fmars.2022.909839
Received: 31 March 2022; Accepted: 06 June 2022;
Published: 15 July 2022.
Edited by:
Meilin Wu, Chinese Academy of Sciences, ChinaReviewed by:
Jiafang Huang, Fujian Normal University, ChinaQian Huang, Johannes Gutenberg University Mainz, Germany
Copyright © 2022 Yan, Feng, Wang, Fu, Luo, Niu and Yang. This is an open-access article distributed under the terms of the Creative Commons Attribution License (CC BY). The use, distribution or reproduction in other forums is permitted, provided the original author(s) and the copyright owner(s) are credited and that the original publication in this journal is cited, in accordance with accepted academic practice. No use, distribution or reproduction is permitted which does not comply with these terms.
*Correspondence: Jianxiang Feng, ZmVuZ2p4MjNAbWFpbC5zeXN1LmVkdS5jbg==; Qingshu Yang, eWFuZ3FzaEBtYWlsLnN5c3UuZWR1LmNu