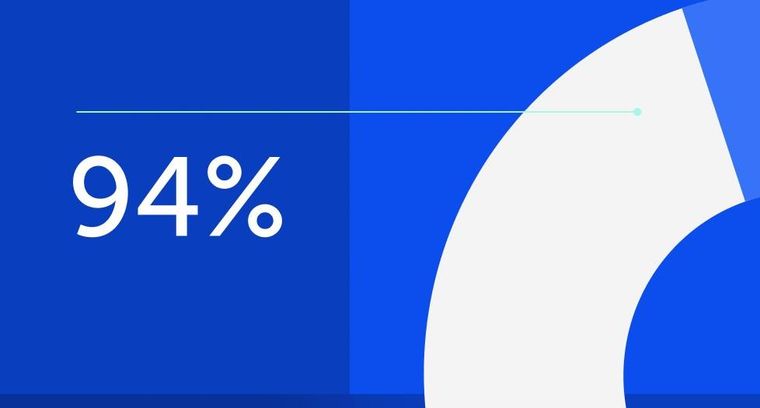
94% of researchers rate our articles as excellent or good
Learn more about the work of our research integrity team to safeguard the quality of each article we publish.
Find out more
ORIGINAL RESEARCH article
Front. Mar. Sci., 10 May 2022
Sec. Marine Conservation and Sustainability
Volume 9 - 2022 | https://doi.org/10.3389/fmars.2022.908195
This article is part of the Research TopicRemote Sensing for Coastal SustainabilityView all 23 articles
Small yellow croaker (Larimichthys polyactis), a benthic fish species widely distributed in the Northwest Pacific Ocean, plays an important role in the fishery catch of coastal countries. A large amount of dead small yellow croaker floated on the sea surface near 30.2°N, 125.3°E in the East China Sea on 19 February 2017, and more than 9,000 kilograms were caught by fishermen, which had never been recorded before. This study aims to investigate the dynamic causes of this sudden death event based on available in-situ, satellite and modelling data. The event occurred near the overwintering ground of small yellow croaker, where a majority of these fish assembled in winter. There were persistent ocean fronts at target site with average intensity of 0.05°C/km at sea surface and bottom in February 2017, which was the strongest in the past 5 years from 2015 to 2019. The fronts provided nutrients and food to fish, and the intense gradients prevented fish from crossing, thus forming “water barriers” to restrict the horizontal movement of fish. Due to enhanced convection, the water was well-mixed, enabling the demersal croaker to float to sea surface. The sea temperature was particularly warm in winter 2017, but then experienced a significant drop by more than 4.5°C in 40 days according to satellite and modelling datasets due to the northerly wind and the southeastward movement of cold eddy. The drop of temperature led to ‘cold shock’ of fish, affecting their physiological regulation and survival. Under the combined influence of these factors, a large number of small yellow croaker died and aggregated near the target site. This paper has a great reference value for further study on the living habits of small yellow croaker and their responses to marine dynamic changes.
Small yellow croaker (Larimichthys polyactis) is a demersal fish species widely distributed in the Bohai Sea, the Yellow Sea, and the East China Sea (ECS) in the Northwest Pacific Ocean (Li et al., 2011; Xiong et al., 2017; Ren et al., 2020). Small yellow croaker is a typical migratory species (Lin et al., 2018) that generally migrates for food and overwinters to warm waters (Li et al., 2011); and in springs, they return from the open sea to coastal waters (such as the Yangtze River Estuary and its adjacent areas) to spawn and grow up (Lin et al., 2018).
Small yellow croaker is one of the most important commercial fishery resources for coastal countries such as China, North Korea, and South Korea (Lin et al., 2011; Xiong et al., 2017), and a substantial number of fishermen in these countries depend on small yellow croaker fisheries. However, in recent years, the quantity and population structure of this fish have changed significantly due to overfishing (Shan et al., 2017; Ren et al., 2020). Moreover, environmental changes caused by marine hydrological factors and climate change have imposed a certain impact on its population (Li et al., 2011; Cheung et al., 2013b; Ren et al., 2020). Therefore, small yellow croaker resources are facing serious decline and the probability of achieving a high-density of fish population is decreasing.
At 3 p.m. (local time, GMT+8) on 19 February 2017, an unusual event happened: fishermen found more than 9,000 kilograms of small yellow croaker floating on the sea surface at approximately 30.2°N, 125.3°E over a short period, and most were dead or nearly dead (Figure 1B). The fishing vessel was surrounded by dead fish in an oval shape with a maximum radius of 60~70 m. According to the fishermen, this phenomenon had not been seen in past years. As red tide events had not been observed in the surrounding waters, and no red tide toxins or other toxins were found according to the toxicological tests by the Zhejiang Marine Fisheries Research Institute, the fish were approved for sale. The causes of death and gathering of small yellow croaker remain to be further explored.
Figure 1 (A) The East China Sea circulation in winter (TWWC: Taiwan Warm Current; ZMCC: Zhe-Min Coastal Current) and migration grounds and routes of small yellow croaker. The circulation is redrawn based on Liu et al. (2021b); the migration grounds and routes of small yellow croakers are after Ren et al. (2020). (B) The event: a large number of small yellow croaker floated on the sea surface around the fishing vessel.
Previous studies have shown that the survivals and spatial distributions of the fish are highly correlated with the changes of marine environmental factors. Finch (1917) described the destructive effects of a cold wave event on small fish (e.g. crabs and small shrimp) in Florida. Gunter (1941) found that along the coast of Texas in the United States in January 1940, different kinds of fish (e.g. pinfish, squid, eel, etc.) died due to cooling caused by severe northerly wind. The fishes were aerated and floated on the surface or washed ashore. Misund et al. (1998) found that the large amount death of herring occurred between cold-water fronts in the Icelandic Exclusive Economic Zone. Marti-Cardona et al. (2008) described several fish kills were related to strong winds and upwelling in the Salton Sea.
By summarizing previous studies, such fish death events may have been caused by the following factors associated with atmospheric and marine dynamics. Firstly, water temperature is the most important environmental factor affecting the spatial distribution of fishes (Lin et al., 2011). Temperature not only affects the metabolic rate of fishes by adjusting the activity of enzymes, thereby regulating the survival and growth of fishes (Hurst et al., 2012; Bergstad, 2013; Shan et al., 2017), but also influences the migration of fishes, and the locations of fishing grounds (Li, 1982; Perry et al., 2005; Cheung et al., 2013a; Shan et al., 2017). The abnormal change of water temperature, especially cooling, could cause discomfort of small yellow croaker and lead to the inflation of fish bladder and eventually death. Secondly, fish might encounter “water barriers”, or ocean fronts. Ocean fronts are vertical boundaries between water masses of different physical, chemical, and hydrodynamic properties (Wolanski and Hamner, 1988). Frontal zones play an important role in fisheries, ecology, and diffusion and concentration of nutrients (Belkin, 2021). Consequently, the fish would gather near fronts because there are high nutrients and they are unable to cross strong gradients. Seasonal hypoxia forms off the Yangtze River Estuary in the ECS under the massive anthropogenic eutrophication (Zhang et al., 2022), and is known to cause detrimental consequences to fish stock and other ecosystem elements. Generally, the seasonal hypoxia in this region starts to form in late spring and early summer, reaches its peak in August, and weakens in autumn (Chi et al., 2020; Wang et al., 2022). However, winter dissolved oxygen level is generally high due to fast oxygen exchange associated with active vertical mixing and lateral advection (Zuo et al., 2019; Zhang et al., 2022). Hence, hypoxia is unlikely to be a potential cause of this event. This paper is motivated to find out the possible reasons and relevant evidence for this event.
In this paper, oceanic numerical modelling data combined with satellite data and in-situ data were used to investigate the possible marine dynamic mechanisms underlying the sudden death event in winter 2017. The paper is organized as follows. The study area and datasets used in this paper are introduced in Section 2. Section 3 presents the analysis results based on multiple datasets. Section 4 discusses two main possible causes of this event, namely, cooling and fronts, and proposes some other possible reasons and deficiencies. A summary is given in Section 5.
The abnormal death event of small yellow croaker happened in the ECS adjacent to point 30.2°N, 125.3°E (hereinafter referred to as “target site”) (Figure 1A red spot). The ECS circulation in winter and migration routes of small yellow croaker are shown in Figure 1A.
The circulation of ECS is mainly composed of three parts: the Kuroshio in the southeast, the Taiwan Warm Current (TWWC) and Zhe-Min Coastal Current (ZMCC) in the middle, and the Yangtze River buoyant plume in the northwest (Isobe, 2008; Xu et al., 2018) (Figure 1A). Kuroshio is the strongest western boundary current in the Pacific Ocean, with characteristics of high temperature, high salinity, and high velocity. High concentrations of phosphorus are also carried by Kuroshio intrusive waters, supporting the primary production of the ECS shelf (Wang et al., 2018; Liu et al., 2021a). Kuroshio intrusion is divided into 3 branches (Yang et al., 2012; Yang et al., 2018): Kuroshio branch current (KBC), offshore Kuroshio branch current (OKBC), and nearshore Kuroshio branch current (NKBC). For demersal fishes, the NKBC may influence their spatial distribution (Xu et al., 2019). The intrusion of Kuroshio mainly occurs along the shelf break (usually 200 m isobath, Figure 1A), but can also occur at depth less than 200 m, and there is water exchange of the Kuroshio and these places (e.g. midshelf of 50-100 m) (Zhang et al., 2017). The intrusion may occur throughout the year but is more frequent in winter (Wu et al., 2014a) when the NKBC moves towards the Kuroshio mainstream (Yang et al., 2018). This event happened around 60 m isobath in February (Figure 1A); therefore, the target site would be affected by the intrusive water of Kuroshio. The Kuroshio intrusion is an important external factor of the ECS circulation, transporting nutrients from lower latitudes to higher latitudes, which plays a crucial role in the nutrient supply of the ECS and further affects marine creatures and ecology (Isobe, 2008; Guo et al., 2012; Guo et al., 2013; Yang et al., 2013).
TWWC flows northeastward along the ECS shelf and is characterized by high temperature (Yuan and Hsueh, 2010; Liu et al, 2021b). When northerly winds prevail in winter, the TWWC moves toward the shore (Liu et al., 2021b). Meanwhile, the buoyant plume from the Yangtze River transports southwestward toward the Taiwan Strait, and this current that carries cold and fresh waters is ZMCC (Liu et al., 2021b), which will reverse its direction in summer (Guan and Fang, 2006; Yang et al., 2018). All these currents control the hydrology of the ECS shelf. Ocean circulation combines nutrients with light, promoting primary productivity and interactions between higher nutrient levels (Weisberg et al., 2015), further affecting the survival of marine creatures.
The migration routes of small yellow croaker are inseparable from the pattern of ocean circulation in the ECS. In previous studies, three stocks were identified: North Yellow Sea stock, South Yellow Sea stock, and ECS stock (Liu, 1962; Han et al., 2009). The latter two stocks exist in our study area.
For South Yellow Sea stock, the overwintering ground is located at 32°-34°N, 123°-126°E (Han et al., 2009). The adults migrate westward to the Yangtze River estuary for spawning in March (Han et al., 2009; Lin et al., 2011), and form the largest spawning ground of small yellow croaker in China, namely, the Lvsi fishing ground (Xu and Chen, 2009) (Figure 1A).
For ECS stock, the southeastern coastal waters are overwintering ground (Han et al., 2009). From Figure 1A, the location is around 28°-30°N, 123°-125°E. In spring, the fish migrate to the Zhoushan Archipelago, Lvsi fishing ground, and Yushan fishing ground for spawning (Liu, 1990; Xu and Chen, 2009). Geographically, the death event occurred quite close to the overwintering ground, which indicated that small yellow croaker had gathered there in February. The fish can be brought to target site by ocean currents.
Daily sea surface temperature (SST) products of GHRSST-OSTIA (Group for High Resolution Sea Surface Temperature-Operational Sea Surface Temperature and Sea Ice Analysis) are provided with a spatial resolution of 0.05°, merging in-situ data and satellite data from both infrared and microwave radiometers. These data are distributed by NOAA’s National Centers for Environmental Information (https://www.ncei.noaa.gov/data/oceans/ghrsst/L4/GLOB/UKMO/OSTIA/2017/). This dataset is generated using optimal interpolation method. The in-situ temperature from 2 coastal stations near target site in February, 2017 are from Zhejiang Ocean and Fisheries Bureau with intervals of 1 hour, as shown in Figure 2A.
Figure 2 (A) Locations of observed data stations (blue dots) and the target site (red dot). (B) Temperature variation of in-situ data at 2 stations in February 2017. The data came from Zhejiang Ocean and Fisheries Bureau.
Two modelling datasets are used in this paper. The data of global HYCOM (HYbrid Coordinate Ocean Model) have a spatial resolution of 1/12° between 40°S-40°N and a temporal resolution of 3 hours after data assimilation (https://hycom.org/dataserver/gofs-3pt1/analysis). The spatial resolution of simulated data in Wu et al. (2011) is approximately 1/12° in the Kuroshio zone and 4 km in Zhejiang Coastal Waters, and the temporal resolution is 1 hour (hereinafter referred to as “regional model”). A detailed description can be found in Wu et al. (2011). This model was previously validated based on in-situ salinity, temperature, currents, and elevation data (Wu et al., 2011; Wu et al., 2014b; Yuan et al., 2016; Wu et al., 2018), and the results showed reasonable accuracy. The two modelling datasets are employed to perform a more comprehensive analysis. HYCOM is a global model and works well by using a distinctive vertical hybrid coordinate. It assimilates data from satellites, Argo, and other field observations (Helber et al., 2013). However, regional model has a finer resolution in our study area and involves tidal forcing. More importantly, it is refined in upper 5 m thickness, allowing to resolve the vertical structure of the Yangtze River plume (Wu et al., 2011).
The two modelling datasets are first compared with GHRSST to validate their credibility. From Figure 3, SST fields based on HYCOM and regional model show a great similarity with GHRSST. In addition to reflecting the high temperature of Kuroshio and southeast gradient of SST near this current, the modelling data can also distinguish the cold-water area along the Yellow Sea coast.
Figure 3 Comparison of sea surface temperature obtained from GHRSST (left panels: A, E, I), global HYCOM (the second panels: B, F, J), and the regional model (the third panels: C, G, K): note that the red line refers to the NE-SW transect and the blue line refers to the NW-SE transect; the bottom temperature obtained from global HYCOM (right panels: D, H, L). The selected time is 4, 13, and 18 February 2017.
The wind data is provided by CCMP (Cross-Calibrated Multi-Platform, https://www.remss.com/measurements/ccmp/), which assimilates inter-calibrated satellite data from numerous radiometers and scatterometers. The spatial and temporal resolutions are 1/4° and 6 hours, respectively.
Altimeter satellite gridded Sea Level Anomalies (SLA) of daily intervals are obtained from AVISO, which are distributed by Copernicus Marine Environment Monitoring Service (CMEMS; http://marine.copernicus.eu/). The spatial resolution is 1/4°.
Figure 4 demonstrates the temperature differences on the basis of 10 January 2017 from satellite data in the ECS. There was obvious and continuous cooling in February, which occurred near target site and its northwest region. The temperature has dropped by up to 5°C.
Figure 4 Temperature differences on the basis of 10 January (color shading) and daily average winds (vectors) of 4 (A), 8 (B), 12 (C), and 17 (D) February in the ECS. The SST were obtained from GHRSST and the wind data came from CCMP.
The spatial distribution of SLA shows that the cooling was partly caused by a cold eddy (Figure 5). The center of the warm eddy had a positive value with a relatively large SLA (Morrow et al., 2003), while the center of the cold eddy had a negative value with a relatively small SLA (Morrow et al., 2004). The cold eddy will lead to a decrease in ambient temperature. In early February of 2017, a cold eddy (CE-A) was near 32.5°N, 121.5°E (Figure 5A). Driven by the northerly wind, the cold eddy then moved southeastward and appeared at 32°N, 123.5°E on 7 February (Figure 5B), during which its position and size changed. Subsequently, from 8 February to 13 February, CE-A gradually merged with a cold eddy along the coast of Jiangsu Province, thus forming a new cold eddy (CE-B) (Figure 5C). On 14 February, CE-B merged with a southeastern cold eddy at 30°N, 124°E, forming a larger cold eddy (CE-C) (Figure 5D). On 15 February, a new cold eddy (CE-D) separated from CE-C, and its position was rather close to target site (Figure 5E). From 16 February to 19 February, CE-D actually persisted and gradually moved towards target site along with the southerly wind, as shown in Figures 5F–I, leading to further cooling of the area.
Figure 5 Changes of SLA (color shading) and wind (vectors) in 1 (A), 7 (B), 13-19 (C–I) of February 2017. Closed contour lines of SLA are cold eddies and warm eddies, and the negative centers are cold eddies. The SLA data were obtained from CMEMS and the wind data were from CCMP.
Another cause of cooling is the strong northerly wind (Figures 4, 5). The variation of wind at target site from January 10 was demonstrated in Figure 6A. The wind direction varied frequently: from 10 January to 14 February, the wind was basically from north and northwest except 28-30 January and 3-5 February, while from 15 February to 19 February, obvious wind direction alternations occurred. Furthermore, the strength of northerly and northwesterly wind (~10 m/s) was significantly stronger than the southerly winds (~5 m/s). The northwest wind brought cold and dry air from the continent, resulting in a huge loss of ocean heat from the ocean to the atmosphere (Kim et al., 2018), which could cause the cooling of February (Luis and Kawamura, 2000).
Figure 6 Changes of wind direction at the target site from 10 January 2017 (A). The data were from CCMP. Sea temperature variation from surface to bottom within 6 km (B) and 60 km (C) of the target site. The differences of surface (D) and bottom (F) temperatures and the differences of surface (E) and bottom (G) salinities between 18 February and 10 January 2017. The areas of decline have been marked. The temperature and salinity data were from HYCOM.
At target site, the temperature had decreased by 4.5°C within 6 km during about 40 days before the sudden death event. The cooling range can reach up to 60 km with a drop of 6.6°C, which can be found from both HYCOM and GHRSST at every depth (Figures 6B, C). Figures 6D–G demonstrate that the temperatures and salinities on 18 February were lower than those on 10 January within the nearby area of target site. However, the variation in salinity was less than 1, which was weaker than that in temperature. Wang et al. (2021) discussed the effects of salinity on small yellow croaker through controlled experiments on the enzyme activities in them after salinity changed. They found that when the salinity is reduced to 5, the croaker can adjust the physiological function by mobilizing the enzymes in the body in time, thus maintaining the osmotic pressure balance of the internal and external environment and surviving. Therefore, the slight change of less than 1 (Figures 6E, G) would not cause the death of small yellow croaker. Thus, more attention should be paid to temperature decrease (cooling).
The generation algorithm of HYCOM and GHRSST involves shift, interpolation, and assimilation of observed data, which may bring unavoidable estimation errors (Minnett and Kaiser-Weiss, 2012; Helber et al., 2013), especially the underestimation of sharp variations both in space and time domain. Due to the lack of directly in-situ data of target site, we analyzed the observed surface temperature at 2 coastal stations (Figure 2A blue dots). As shown in Figure 2B, the variation of surface temperature reflected by the in-situ data has a trend of decreasing, up to 15°C, during most of February, especially from 16 to 18 February. This is similar to the variation reflected by the HYCOM and GHRSST data (Figures 6B, C). Nevertheless, the magnitude of the variation is different. It is reasonable to speculate that the actual cooling at target site at the time of this event could be greater than that shown by the modelling datasets.
SST data can be used to explore the possible effect of the Kuroshio on this death event. The spatial distribution of daily mean SST of the ECS during February (Figure 3) shows that the warm Kuroshio was approximately 22°C and generally flowed northeastward, which could bring a wealth of nutrients. Figure 3 also illustrates the relatively cold Jiangsu Coastal Current, which has abundant inorganic nutrients (Zhang et al., 2020) and moves southeastward. The target site was in the middle of 14°C and 16°C isotherms on 4 February both at the surface and bottom. Later, it was between the isotherms of 12°C and 14°C and closer to 12°C on 18 February, which meant the temperature had decreased significantly. In addition, there were tongue-shaped temperature gradients between the Kuroshio intrusive path and target site, and a cold-water tongue was found north of it, which was easy to form thermal fronts.
Since the regional model has a higher vertical resolution near shore, we use this modelling dataset to analyze the vertical structure. The northwest-southeast and the northeast-southwest direction are represented by blue and red lines respectively in Figures 3C, G, K. As shown in Figure 7, the profiles also indicate the temperature decrease and temperature gradients, which will be further explored in the following sections. Besides, the temperature near target site shows obvious vertical uniform structures. From the surface to bottom, seawater was well-mixed and the temperature was relatively cold.
Figure 7 Upper left panels: the vertical temperature profile of the northwest-southeast direction (A, C, E). Upper right panels: the vertical temperature profile of the northeast-southwest direction (B, D, F). Bottom panels: the vertical variation of temperature at the target site (G). The temperature data were obtained from the regional model.
Ocean fronts can be seen in the vertical profile of temperature (Figure 7). The temperature in the southeast was higher than that in the northwest, showing a clear temperature gradient (Figures 7A, C, E). On 4 February, the vicinity of target site presented a temperature of approximately 13.5°C, followed by a gradual movement of the cold water from northwest gradually to southeast, resulting in a drop of 1.5°C in 2 weeks (Figure 7G). As cold water moved, the front around target site was also strengthened. This might be related to changes in the intrusive path of the cold-water tongue, which gradually moved southeastward.
In the northeast-southwest direction, the temperature profile presents a “sandwich” structure (Figures 7B, D, F), showing that warmer seawater was on both sides and relatively colder seawater was in the middle. The center of low temperature was located around 126°E, which indicated that there were fronts on both sides near target site, especially the southwest side. As the cold water gradually diffused, it also showed that the temperature of target site dropped, which is consistent with the results of section 3.2, whereas the fronts remained on both sides.
The horizontal gradients are commonly used to detect ocean fronts. The intensities of the temperature fronts at the surface and bottom are calculated by using the formula:
where x and y represent the distances of latitude and longitude respectively. The spatial distribution of intensities of temperature fronts shows that there were continuous and intense fronts at the surface and bottom near target site. The intensity of thermal fronts increased by 0.01°C/km at the bottom and 0.005 °C/km at the sea surface from 7 to 12 February 2017. The range of strong fronts near the target site expanded from 12 to 18 February (Figures 8A–F).
Figure 8 Spatial distribution of surface (A–C) and bottom (D–F) thermal fronts near the target site in February 2017. The fronts had been averaged between two isotherms. Daily variations of thermal front intensity near the target site in February from 2015 to 2019 (G, H), (G) refers to the surface and (H) refers to the bottom. The solid lines indicate the frontal intensity in 2015-2019, respectively, and black-dashed lines indicate the average front intensity in 2015-2019. The data were obtained from HYCOM.
Daily variations of ocean fronts in February from 2015 to 2019 (Figures 8G, H) indicate that the intensity of the fronts near target site in 2017 was the largest in the past 5 years from 2015 to 2019, both at the surface and bottom. The average fronts intensity at the surface and bottom was 0.05°C/km in 2017, 0.03°C/km in 2018, while 0.02°C/km in the other 3 years. In 2017, the intensities of the temperature fronts in the bottom layer increased significantly after 14 February. The variations of ocean fronts might have acted as a barrier, affecting the movement of small yellow croaker.
Based on comprehensive data analysis, the reasons for the sudden death event of small yellow croaker in the ECS during winter 2017 are illustrated in Figure 9.
Figure 9 Schematic diagram of the reasons for the sudden death event of small yellow croaker in the ECS in winter 2017. For more details: see [1] in Figure 1A; see [2] in Xu et al. (2018); see [3] in Figures 4–6A; see [4] in Figure 5; see [5] in Figure 7; see [6] in Figure 8.
The target site is near the overwintering ground of the ECS stock, between 60 m and 200 m isobaths within the continental shelf (Figure 1A). The intrusion of nutrient-rich NKBC could provide food and attract small yellow croaker to gather. Fish larvae will be transported onto the shelf by the northward intrusion of Kuroshio and its associated streamers and eddies (Sassa, 2019). The trawl investigation of demersal fish in the ECS carried out by Xu et al. (2019) also proved that the distribution of these fish closely matched intrusion of NKBC. Also, the tongue-shaped cold-water in Figure 3 and curved fronts in Figure 8 demonstrate that high-nutrients Jiangsu Coastal Current is moving to the target site, which can also provide nutrients.
There were continuous ocean fronts near the target site, especially temperature fronts (Figures 7, 8), which provided sufficient nutrition for small yellow croaker. In the early 20th century in Japan, the first scientific expression describing the relationship between fishes and fronts was so-called Kitahara’s law, which was put in fewer words: “fish shoals tend to be congregated in ocean fronts” (Sournia, 1994). A recent study discussed the relationship between fronts and skipjack tuna fishing grounds (Zainuddin et al., 2021). They found that skipjack gathered around the thermal fronts with a distance of 0-40 km. Ding et al. (2021) used satellite and reanalysis data to explore the causes of unusual fish assemblage in February and March 2017 in the ECS. They confirmed that fish are more likely to migrate to fishing areas with relatively high temperature, northerly wind, strong thermal fronts and high chlorophyll-a concentration. Based on their analysis, fish assemblage appeared when the front intensity was around 0.05°C/km in the 32°N, 125.5°E of the ECS in March 2017, which is consistent with front intensity at the target site. Moreover, several studies have confirmed that the abundance of most fish peaked at or near fronts (Tseng et al., 2014; Woodson and Litvin, 2015; Alemany et al., 2018; Belkin, 2021). This was because fronts were usually considered as locations of maximum biodiversity and elevated production in the ocean (Palacios et al., 2006; Woodson et al., 2012; Svendsen et al., 2020; Belkin, 2021). Besides, the fronts were beneficial to development and maturation of the gonads of small yellow croaker (Kucera et al., 2002). Consequently, the fronts were conducive to the gathering of small yellow croaker.
Meanwhile, ocean fronts formed “water barriers” for small yellow croaker that might restrict their horizontal movement (crossing the front) and hinder the range of movement, thus preventing the fish from escaping or prolonging their retention time. Such phenomena occurred because the gradients were large along the fronts, and fish tended to migrate along the boundaries rather than cross them (Iwatsuki et al., 1989; Hubbard et al., 2004). Furthermore, the most important prey of small yellow croaker are planktonic crustaceans (mainly krills and decapods) (Xue et al., 2005), which don’t have the ability to swim autonomously and can only rely on the flow of seawater to move. According to Iwatsuki et al. (1989), if the surface current moves toward the fronts and the current speed is 0.1 m/s or more, the fish larvae and crustaceans will follow the water masses toward the front and then convey along the frontal zone horizontally, further increasing fish population. In our case, the ocean fronts are basically in the northeast-southwest direction. From Figure 10, the speeds are larger than 0.1 m/s in most of the time, and the current was toward the fronts, especially on 10, 16, and 19 February. Therefore, a large number of small yellow croaker would gather and be confined near the target site, thus causing the large fishery catch in February 2017. In previous studies, the influence of the fronts had also been found in lots of areas. For example, Hidayat et al. (2019) carried out a survey to confirm that the distribution of skipjack tuna fishing grounds was at or near the detected thermal fronts in the Makassar Strait. Garcés-Rodríguez et al. (2021) found that the highest fish larvae abundance was related to the strongest thermal fronts in the Midriff Archipelago region, Gulf of California through cruises.
Fish are poikilothermic animals, meaning their body temperature changes with the ambient temperature (Lall and Tibbetts, 2009), and all of their physiological and behavioral parameters are controlled by temperature (Fry, 1947; Donaldson et al., 2008). Thus, changes of sea temperature due to wind and other factors will cause changes in the actions of fish. The small yellow croaker is warm-temperate demersal fish and prefers warmer water (Chen et al., 2010). As the temperature at the bottom of the target site continued to drop (Figures 6B, C), large numbers of small yellow croaker swim up from the bottom in search of a warmer environment. With the drop of temperature, seawater convection will be enhanced, leading to a more uniform structure (Killworth, 1983), making it possible for fish to swim up unimpededly (Figure 7). Meanwhile, high concentration of nutrients at the bottom can diffuse to the surface, which may better promote photosynthesis. In this case, the migration process of fish could occur in all water layers, which meant that the demersal yellow croaker could float to the sea surface without the limitations of thermoclines (Li, 1982). For example, the vertical isothermal structure caused by a cold wave in the Bohai Sea on 6 October 1977 led to a large number of prawns floating up to the surface, which greatly affected the catch (Li, 1982). During the process of migration, a decrease in sea temperature was caused by southerly movement of cold eddies and northerly wind (Figures 4–6A). Based on HYCOM and GHRSST datasets, the temperature had dropped at each depth by about 4.5°C in one and a half months at target site (Figures 6B, C). Moreover, we believe the actual cooling range would be greater than HYCOM and GHRSST show through the analysis of nearshore in-situ data (Figure 2).
Qi et al. (2017) studied the floating algae blooms in the ECS in 2017, in which they analyzed SST anomaly from 2010 to 2017 and found that the ECS experienced record-high temperature with a positive anomaly of 0.7-1.3°C in winter 2017. The temperature could as high as 18.21°C (Song et al., 2019). In this case, when the small yellow croaker had adapted to a specific warm water environment, the subsequent decrease of more than 4.5°C of living environment in around 40 days caused by the cold eddies and wind might cause a series of physiological and behavioral discomfort and even death. Donaldson et al. (2008) referred to the death of fish due to a decrease in temperature as “cold shock”. Cold shock is one of the stress responses of fish, and it affects the central nervous system, metabolic function, and ionic balance of fish (Donaldson et al., 2008). Furthermore, it leads to slow movement, compromised swimming and foraging ability, reduced responsiveness, shrinkage of gill, loss of equilibrium, the onset of cold coma, starvation, and respiratory failure (Fuiman and Batty, 1997; Smith and Hubert, 2003; Hurst, 2007; Donaldson et al., 2008). Several studies have focused on the effects of cold shock on fish and catches. For instance, Emery (1970) implicated declining temperatures from a seiche as a cause for high mortalities of sculpins and crayfish in Georgian Bay, Lake Huron, Canada. Lee et al. (2014) illustrated the effects of an unusual cold-water intrusion caused by a strong and continuous northeasterly wind in 2008 around Taiwan Islands. They noted that the cold water caused the death of fish but increased the catches from a set-net fishery, with the majority of the catch represented by migratory species. Through these studies, we confirmed the negative impacts of cooling on the living of small yellow croaker. Thus, the abnormal cooling caused by wind and cold eddies in February 2017 may be the main factor for the death of small yellow croaker.
This study has some limitations. We lack in-situ temperature, chlorophyll, dissolved oxygen measurements at target site. Moreover, due to the lack of higher-resolution datasets, we are unable to discuss the effects of smaller-scale motions, such as the submesoscale processes. For example, enhanced vertical and horizontal currents and high levels of shear can be produced by submesoscale fronts, which leads to nutrient flow into the surface water, and in turn causes more concentration of nutrients, phytoplankton and zooplankton (Genin et al., 2005; Lévy et al., 2012; Mahadevan, 2016; Snyder et al., 2017), affecting the migration of fish. Therefore, this abnormal event needs to be further explored from multiple aspects.
This paper discusses the possible causes of the abnormal sudden death event of small yellow croaker in the ECS during winter 2017. Satellite and modelling datasets are used to investigate the possible dynamic mechanisms of this event. The results indicate that small yellow croaker overwintered through the ECS in the winter, and the number of small yellow croaker would increase during this time. The pathways of NKBC intrusion and the development of ocean fronts might have provided nutrients, affecting the fish gathering. Moreover, the fronts formed “water barriers” that blocked the horizontal movement of the small yellow croaker. In the vertical direction, due to the vertical isothermal structure, the demersal croaker could freely swim to the sea surface. The analysis of multiple data shows that the wind directions switched several times in late February but mainly northerly, moreover, the cold eddies moved southward. There was more than 4.5°C drop of temperature caused by wind and the moving cold eddies in about 40 days, leading to “cold shock” of the fish, which finally resulted in discomfort and eventual death of small yellow croaker. Under the comprehensive influence of these factors, a large number of dead small yellow croaker emerged from the sea at the target site. In short, the impacts of changes in the marine dynamic environment on the abnormal death event of small yellow croaker are multifaceted, and the influences of other environmental factors, such as chlorophyll, dissolved oxygen, and submesoscale processes, need to be further studied. A comprehensive understanding of the relationships between marine dynamic factors and main creatures will be of great scientific significance in making fisheries policies and using marine fishery resources.
Publicly available datasets were analyzed in this study. This data can be found here: The wind data are obtained from CCMP, https://www.remss.com/measurements/ccmp/. The satellite temperature data of GHRSST can be found from NCEI, https://www.ncei.noaa.gov/data/oceans/ghrsst/L4/GLOB/UKMO/OSTIA/2017/. The HYCOM data used in this study are publicly available, which can be found in the https://hycom.org/dataserver/gofs-3pt1/analysis. The measured temperature data are from Zhejiang Ocean and Fisheries Bureau, which are not publicly available and further inquiries can contact the corresponding author. The SLA data are obtained from http://marine.copernicus.eu/. The bathymetry data near the coast of Zhejiang Province are provided by the Zhejiang Province Ocean and Fisheries Bureau, and data for the other areas were obtained from the ETOPO1 dataset (https://ngdc.noaa.gov/mgg/global/global.html). The model data of Wu et al. (2011) are not publicly available, further inquiries can be directed to the corresponding author.
ZW and XJ analyzed and visualized the data and wrote the manuscript. JZ conceived the study, provided the methods and resources, and reviewed and edited the manuscript. YD assisted with the formal analysis, and reviewed and edited the manuscript. ZR reviewed and edited the manuscript. HW investigated and edited the manuscript. YW revised the manuscript. All authors contributed to the article and approved the submitted version.
This work was supported by the National Natural Science Foundation of China [grant number 41876086], the National Key Research and Development Plan of China [grant number 2017YFA0604100], and the Innovation Program of Shanghai Municipal Education Commission [2019-01-07-00-05-E00027]. This research is supported by the HPC Center of ZJU (ZHOUSHAN CAMPUS).
We are very thankful to the Liangchao Ni of Ocean College, Zhejiang University to help to illustrate Figure 9. We are also grateful to the reviewers and editor.
The authors declare that the research was conducted in the absence of any commercial or financial relationships that could be construed as a potential conflict of interest.
All claims expressed in this article are solely those of the authors and do not necessarily represent those of their affiliated organizations, or those of the publisher, the editors and the reviewers. Any product that may be evaluated in this article, or claim that may be made by its manufacturer, is not guaranteed or endorsed by the publisher.
Alemany D., Iribarne O. O., Acha E. M. (2018). Marine Fronts as Preferred Habitats for Young Patagonian Hoki Macruronus Magellanicus on the Southern Patagonian Shelf. Mar. Ecol.: Prog. Ser. 588, 191–200. doi: 10.3354/meps12454
Belkin I. M. (2021). Review Remote Sensing of Ocean Fronts in Marine Ecology and Fisheries. Remote Sens. 13 (5), 1–22. doi: 10.3390/rs13050883
Bergstad O. A. (2013). North Atlantic Demersal Deep-Water Fish Distribution and Biology: Present Knowledge and Challenges for the Future. J. Fish Biol. 83 (6), 1489–1507. doi: 10.1111/jfb.12208
Chen J. J., Xu Z. L., Chen X. Z. (2010). The Spatial Distribution Pattern of Fishing Ground for Small Yellow Croaker in China Seas (in Chinese). J. Fish. China. 34 (2), 236–244. doi: 10.3724/SP.J.1231.2010.06371
Cheung W. W., Sarmiento J. L., Dunne J., Frölicher T. L., Lam V. W. Y., Palomares M. D., et al. (2013a). Shrinking of Fishes Exacerbates Impacts of Global Ocean Changes on Marine Ecosystems. Nat. Clim. Change. 3 (3), 254–258. doi: 10.1038/nclimate1691
Cheung W. W., Watson R., Pauly D. (2013b). Signature of Ocean Warming in Global Fisheries Catch. Nature 497 (7449), 365–368. doi: 10.1038/nature12156
Chi L., Song X., Yuan Y., Wang W., Cao X., Wu Z., et al. (2020). Main Factors Dominating the Development, Formation and Dissipation of Hypoxia Off the Changjiang Estuary (CE) and its Adjacent Waters, China. Environ. Pollu. 265, 115066. doi: 10.1016/j.marpolbul.2017.09.063
Ding W., Zhang C., Hu J., Shang S. (2021). Unusual Fish Assemblages Associated With Environmental Changes in the East China Sea in February and March 2017. Remote Sens. 13 (9), 1768. doi: 10.3390/rs13091768
Donaldson M. R., Cooke S. J., Patterson D. A., Macdonald J. S. (2008). Cold Shock and Fish. J. Fish Biol. 73 (7), 1491–1530. doi: 10.1111/j.1095-8649.2008.02061.x
Emery A. R. (1970). Fish and Crayfish Mortalities Due to an Internal Seiche in Georgian Bay, Lake Huron. J. Fish. Res. Board. Can. 27 (6), 1165–1168. doi: 10.1139/f70-136
Finch R. H. (1917). Fish Killed by the Cold Wave of February 2–4, 1917, in Florida. Mon. Weather. Rev. 45 (4), 171–172. doi: 10.1175/1520-0493(1917)45<171:FKBTCW>2.0.CO;2
Fry F. E. J. (1947). Effects of the Environment on Animal Activity. Publ. Out. Fish. Res. Lab. 55 (68), 1–62.
Fuiman L., Batty R. (1997). What a Drag it is Getting Cold: Partitioning the Physical and Physiological Effects of Temperature on Fish Swimming. J. Exp. Biol. 200 (12), 1745–1755. doi: 10.1242/jeb.200.12.1745
Garcés-Rodríguez Y., Sánchez-Velasco L., Parés-Sierra A., Jiménez-Rosenberg S. P. A., Márquez-Artavia A., Flores-Morales A. L. (2021). FISH Larvae Distribution and Transport on the Thermal Fronts in the Midriff Archipelago Region, Gulf of California. Cont. Shelf. Res. 218, 104384. doi: 10.1016/j.csr.2021.104384
Genin A., Jaffe J. S., Reef R., Richter C., Franks P. J. S. (2005). Swimming Against the Flow: A Mechanism of Zooplankton Aggregation. Science 308, 860–862. doi: 10.1126/science.1107834
Guan B. H., Fang G. H. (2006). Winter Counter-Wind Currents Off the Southeastern China Coast: A Review. J. Oceanogr. 62 (1), 1–24. doi: 10.1007/s10872-006-0028-8
Gunter G. (1941). Death of Fishes Due to Cold on the Texas Coast, January 1940. Ecology. 22 (2), 203–208. doi: 10.2307/1932218
Guo X. Y., Zhu X. H., Long Y., Huang D. J. (2013). Spatial Variations in the Kuroshio Nutrient Transport From the East China Sea to South of Japan. Biogeosciences. 10 (10), 6403–6417. doi: 10.5194/bg-10-6403-2013
Guo X. Y., Zhu X. H., Wu Q. S., Huang D. (2012). The Kuroshio Nutrient Stream and its Temporal Variation in the East China Sea. J. Geophys. Res.: Oceans. 117 (C1), 1978–2012. doi: 10.1029/2011JC007292
Han Z. Q., Lin L. S., Shui B. N., Gao T. X. (2009). Genetic Diversity of Small Yellow Croaker Larimichthys Polyactis Revealed by AFLP Markers. Afr. J. Agric. Res. 4 (7), 605–610. doi: 10.5897/AJAR.9000369
Helber R. W., Townsend T. L., Barron C. N., Dastugue J. M., Carnes M. R. (2013). Validation Test Report for the Improved Synthetic Ocean Profile (ISOP) System, Part I: Synthetic Profile Methods and Algorithm. Mississippi: NRL/MR/7320-13-9364. Naval Research Laboratory, Stennis Space Center. Available at: http://www7320.nrlssc.navy.mil/pubs/2013/helber1-2013.pdf.
Hidayat R., Zainuddin M., Safruddin S., Mallawa A., Farhum S. A. (2019). “Skipjack Tuna (Katsuwonus Pelamis) Catch in Relation to the Thermal and Chlorophyll-A Fronts During May-July in the Makassar Strait”. IOP Conf. Ser: Earth Environ. Sci. 253, 012045. doi: 10.1088/1755-1315/253/1/012045
Hubbard S., Babak P., Sigurdsson S. T., Magnússon K. G. (2004). A Model of the Formation of Fish Schools and Migrations of Fish. Ecol. Modell. 174 (4), 359–374. doi: 10.1016/j.ecolmodel.2003.06.006
Hurst T. P. (2007). Causes and Consequences of Winter Mortality in Fishes. J. Fish Biol. 71 (2), 315–345. doi: 10.1111/j.1095-8649.2007.01596.x
Hurst T. P., Munch S. B., Lavelle K. A. (2012). Thermal Reaction Norms for Growth Vary Among Cohorts of Pacific Cod (Gadus Macrocephalus). Mar. Biol. 159 (10), 2173–2183. doi: 10.1007/s00227-012-2003-9
Isobe A. (2008). Recent Advances in Ocean-Circulation Research on the Yellow Sea and East China Sea Shelves. J. Oceanogr. 64 (4), 569–584. doi: 10.1007/s10872-008-0048-7
Iwatsuki Y., Nakata H., Hirano R. (1989). The Thermohaline Front in Relation to Fish Larvae. Rapp. P.-v. Réun. Cons. Int. Explor. Mer. 191, 119–126.
Killworth P. D. (1983). Deep Convection in the World Ocean. Rev. Geophys. 21 (1), 1–26. doi: 10.1029/rg021i001p00001
Kim Y. S., Jang C. J., Yeh S. W. (2018). Recent Surface Cooling in the Yellow and East China Seas and the Associated North Pacific Climate Regime Shift. Cont. Shelf. Res. 156, 43–54. doi: 10.1016/j.csr.2018.01.009
Kucera C. J., Faulk C. K., Holt G. J. (2002). The Effect of Spawning Salinity on Eggs of Spotted Seatrout (Cynoscion Nebulosus, Cuvier) From Two Bays With Historically Different Salinity Regimes. J. Exp. Mar. Biol. Ecol. 272 (2), 147–158. doi: 10.1016/S0022-0981(02)00081-3
Lall S. P., Tibbetts S. M. (2009). Nutrition, Feeding, and Behavior of Fish. Vet. Clin. North Am. Exot. Anim. Pract. 12 (2), 361–372. doi: 10.1016/j.cvex.2009.01.005
Lee M. A., Yang Y. C., Shen Y., Chang Y., Tsai W. S., Lan K. W., et al. (2014). Effects of an Unusual Cold-Water Intrusion in 2008 on the Catch of Coastal Fishing Methods Around Penghu Islands, Taiwan. Terr. Atmos. Ocean. Sci. 25 (1), 107–120. doi: 10.3319/TAO.2013.08.06.01(Oc
Lévy M., Ferrari R., Franks P. J. S., Martin A. P., Riviére P. (2012). Bringing Physics to Life at the Submesoscale. Geophys. Res. Lett. 39, L14602. doi: 10.1029/2012GL052756
Li X. D. (1982). Studies on the Correlation Between the Temperature of Sea Water and Fishing Grounds (in Chinese). Acta Oceanol. Sin. (Chinese). 01), 103–113. doi: CNKI:SUN:SEAC.0.1982-0.-011
Lin N., Chen Y., Jin Y., Yuan X., Ling J., Jiang Y. (2018). Distribution of the Early Life Stages of Small Yellow Croaker in the Yangtze River Estuary and Adjacent Waters. Fish. Sci. 84 (2), 357–363. doi: 10.1007/s12562-018-1177-6
Lin L., Liu Z., Jiang Y., Huang W., Gao T. (2011). Current Status of Small Yellow Croaker Resources in the Southern Yellow Sea and the East China Sea. Chin. J. Oceanol. Limnol. 29, 547–555. doi: 10.1007/s00343-011-0182-8
Li Z., Shan X., Jin X., Dai F. (2011). Long-Term Variations in Body Length and Age at Maturity of the Small Yellow Croaker (Larimichthys Polyactis Bleeker 1877) in the Bohai Sea and the Yellow Sea, China. Fish. Res. 110 (1), 67–74. doi: 10.1016/j.fishres.2011.03.013
Liu X. S. (1962). The Research of Small Yellow Croaker (Larimichthys Polyactis) Geographic Race and Gonad (in Chinese) (Beijing: Science Press).
Liu X. S. (1990). Fishery Resources Investigation in the Yellow Sea and Bohai Sea (in Chinese) (Beijing: Ocean Press).
Liu Z., Gan J., Hu J., Wu H., Cai Z., Deng Y. (2021a). Progress of Studies on Circulation Dynamics in the East China Sea: The Kuroshio Exchanges With the Shelf Currents. Front. Mar. Sci. 8(4), 620910. doi: 10.3389/fmars.2021.620910
Liu Z., Gan J., Hu J., Wu H., Cai Z., Deng Y. (2021b). Progress on Circulation Dynamics in the East China Sea and Southern Yellow Sea: Origination, Pathways, and Destinations of Shelf Currents. Prog. Oceanogr. 193, 102553. doi: 10.1016/j.pocean.2021.102553
Luis A. J., Kawamura H. (2000). Wintertime Wind Forcing and Sea Surface Cooling Near the South India Tip Observed Using NSCAT and AVHRR. Remote Sens. Environ. 73 (1), 55–64. doi: 10.1016/S0034-4257(00)00081-X
Mahadevan A. (2016). The Impact of Submesoscale Physics on Primary Productivity of Plankton. Annu. Rev. Mar. Sci. 8, 161–184. doi: 10.1146/annurev-marine-010814-015912
Marti-Cardona B., Steissberg T. E., Schladow S. G., Hook S. J. (2008). Relating Fish Kills to Upwellings and Wind Patterns in the Salton Sea. Hydrobiologia. 604 (1), 85–95. doi: 10.1007/s10750-008-9315-2
Minnett P., Kaiser-Weiss A. (2012). Near-Surface Oceanic Temperature Gradients. Discussion Document, Group for High Resolution Sea-Surface Temperature. pp. 1–7. Available at: https://www.ghrsst.org/wp-content/uploads/2021/04/SSTDefinitionsDiscussion.pdf (accessed on 29 April 2022).
Misund O. A., Vilhjálmsson H., Jákupsstovu S.H.Í., Røttingen I., Belikov S., Asthorsson O., et al. (1998). Distribution, Migration and Abundance of Norwegian Spring Spawning Herring in Relation to the Temperature and Zooplankton Biomass in the Norwegian Sea as Recorded by Coordinated Surveys in Spring and Summer 1996. Sarsia. 83 (2), 117–127. doi: 10.1080/00364827.1998.10413677
Morrow R., Donguy J. R., Chaigneau A., Rintoul S. R. (2004). Cold-Core Anomalies at the Subantarctic Front, South of Tasmania. Deep. Sea. Res. Part I. 51 (11), 1417–1440. doi: 10.1016/j.dsr.2004.07.005
Morrow R., Fang F., Fieux M., Molcard R. (2003). Anatomy of Three Warm-Core Leeuwin Current Eddies. Deep. Sea. Res. Part II. 50 (12-13), 2229–2243. doi: 10.1016/S0967-0645(03)00054-7
Palacios D. M., Bograd S. J., Foley D. G., Schwing F. B. (2006). Oceanographic Characteristics of Biological Hot Spots in the North Pacific: A Remote Sensing Perspective. Deep. Sea. Res. Part II. 53 (3-4), 250–269. doi: 10.1016/j.dsr2.2006.03.004
Perry A. L., Low P. J., Ellis J. R., Reynolds J. D. (2005). Ecology: Climate Change and Distribution Shifts in Marine Fishes. Science 308 (5730), 1912–1915. doi: 10.1126/science.1111322
Qi L., Hu C., Wang M., Shang S., Wilson C. (2017). Floating Algae Blooms in the East China Sea. Geophys. Res. Lett. 44 (22), 11,501–11,509. doi: 10.1002/2017GL075525
Ren J. S., Jin X., Yang T., Kooijman S. A. L. M., Shan X. (2020). A Dynamic Energy Budget Model for Small Yellow Croaker Larimichthys Polyactis: Parameterisation and Application in its Main Geographic Distribution Waters. Ecol. Modell. 427, 109051. doi: 10.1016/j.ecolmodel.2020.109051
Sassa C. (2019). “Reproduction and Early Life History of Mesopelagic Fishes in the Kuroshio Region: A Review of Recent Advances”, in AGU Geophysical Monogr. Ser. Kuroshio Current: Physical, Biogeochemical and Ecosystem Dynamics. Eds. Nagai T., Saito H., Suzuki K., Takahashi M.. Hoboken, NJ and Washington, D.C.: John Wiley & Sons, Inc. pp. 273–294. doi: 10.1002/9781119428428.ch17
Shan X., Li X., Yang T., Sharifuzzaman S. M., Zhang G., Jin X., et al. (2017). Biological Responses of Small Yellow Croaker (Larimichthys Polyactis) to Multiple Stressors: A Case Study in the Yellow Sea, China. Acta Oceanol. Sin. 36 (10), 39–47. doi: 10.1007/s13131-017-1091-2
Smith M. A., Hubert W. A. (2003). Simulated Thermal Tempering Versus Sudden Temperature Change and Short-Term Survival of Fingerling Rainbow Trout. N. Am. J. Aquacult. 65 (1), 67–69. doi: 10.1577/1548-8454(2003)065<0067:STTVST>2.0.CO;2
Snyder S., Franks P. J., Talley L. D., Xu Y., Kohin S. (2017). Crossing the Line: Tunas Actively Exploit Submesoscale Fronts to Enhance Foraging Success. Limnol. Oceanogr. Lett. 2 (5), 187–194. doi: 10.1002/lol2.10049
Song C., Jiang H., Zhang S., Chen X., Wang H. (2019). Sea Surface Temperature Characteristics of the China Seas in 2017 (in Chinese). Marin. Forec. 36 (01), 1–9. doi: 10.11737/j.ssn.1003-0239.2019.01.001
Sournia A. (1994). Pelagic Biogeography and Fronts. Prog. Oceanogr. 34 (2-3), 109–120. doi: 10.1016/0079-6611(94)90004-3
Svendsen G. M., Reinaldo M. O., Romero M. A., Williams G., Magurran A., Luque S., et al. (2020). Drivers of Diversity Gradients of a Highly Mobile Marine Assemblage in a Mesoscale Seascape. Mar. Ecol.: Prog. Ser. 638, 149–164. doi: 10.3354/meps13264
Tseng C. T., Sun C. L., Belkin I. M., Yeh S. Z., Kuo C. L., Liu D. C. (2014). Sea Surface Temperature Fronts Affect Distribution of Pacific Saury (Cololabis Saira) in the Northwestern Pacific Ocean. Deep. Sea. Res. Part II. 107, 15–21. doi: 10.1016/j.dsr2.2014.06.001
Wang M., Chu T., Liu F., Zhan W., Lou B., Xu W. (2021). Effect of Salinity Stress on the Antioxidant Enzymes, non-Specific Immune Enzymes, and Na+/K+-ATPase Activities in Larimichthys Polyactis (in Chinese). Acta Oceanol. Sin. (Chinese). 43 (2), 59–66. doi: 10.12284/hyxb2021038
Wang W., Yu Z., Song X., Chi L., Zhou P., Wu Z., et al. (2022). Hypoxia Formation in the East China Sea by Decomposed Organic Matter in the Kuroshio Subsurface Water. Mar. Pollut. Bull. 177, 113486. doi: 10.1016/j.marpolbul.2022.113486
Wang W., Yu Z., Song X., Yuan Y., Wu Z., Zhou P., et al. (2018). Intrusion Pattern of the Offshore Kuroshio Branch Current and its Effects on Nutrient Contributions in the East China Sea. J. Geophys. Res. Oceans. 123 (3), 2116–2128. doi: 10.1002/2017JC013538
Weisberg R. H., Zheng L., Liu Y. (2015). “Basic Tenets for Coastal Ocean Ecosystems Monitoring”, in Coastal Ocean Observing Systems. Eds. Liu Y. G., Kerkering H., Weisberg R. H. (London: Academic Press), 40–57.
Wolanski E., Hamner W. M. (1988). Topographically Controlled Fronts in the Ocean and Their Biological Influence. Science. 241 (4862), 177–181. doi: 10.1126/science.241.4862.177
Woodson C. B., Litvin S. Y. (2015). Ocean Fronts Drive Marine Fishery Production and Biogeochemical Cycling. Proc. Natl. Acad. Sci. U. S. A. 112 (6), 1710–1715. doi: 10.1073/pnas.1417143112
Woodson C. B., McManus M. A., Tyburczy J. A., Barth J. A., Washburn L., Caselle J. E., et al. (2012). Coastal Fronts Set Recruitment and Connectivity Patterns Across Multiple Taxa. Limnol. Oceanogr. 57 (2), 582–596. doi: 10.4319/lo.2012.57.2.0582
Wu C. R., Hsin Y. C., Chiang T. L., Lin Y. F., Tsui I. F. (2014a). Seasonal and Interannual Changes of the Kuroshio Intrusion Onto the East China Sea Shelf. J. Geophys. Res.: Oceans. 119 (8), 5039–5051. doi: 10.1002/2013JC009748
Wu H., Shen J., Zhu J., Zhang J., Li L. (2014b). Characteristics of the Changjiang Plume and its Extension Along the Jiangsu Coast. Cont. Shelf. Res. 76, 108–123. doi: 10.1016/j.csr.2014.01.007
Wu H., Wu T., Bai M. (2018). Mega Estuarine Constructions Modulate the Changjiang River Plume Extension in Adjacent Seas. Estuar. Coasts. 41 (5), 1234–1252. doi: 10.1007/s12237-017-0357-4
Wu H., Zhu J., Shen J., Wang H. (2011). Tidal Modulation on the Changjiang River Plume in Summer. J. Geophys. Res.: Oceans. 116 (8), 1–21. doi: 10.1029/2011JC007209
Xiong Y., Yang J., Jiang T., Liu H., Zhong X., Tang J. (2017). Early Life History of the Small Yellow Croaker (Larimichthys Polyactis) in Sandy Ridges of the South Yellow Sea. Mar. Biol. Res. 13 (9), 993–1002. doi: 10.1080/17451000.2017.1319067
Xu Z. L., Chen J. J. (2009). Analysis on Migratory Routine of Larimichthy Polyactis (in Chinese). J. Fish. Sci. China 16 (6), 931–940. doi: 10.3321/j.issn:1005-8737.2009.06.014
Xue Y., Jin X., Zhang B., Liang Z. (2005). Seasonal, Diel and Ontogenetic Variation in Feeding Patterns of Small Yellow Croaker in the Central Yellow Sea. J. Fish Biol. 67 (1), 33–50. doi: 10.1111/j.0022-1112.2005.00677.x
Xu Y., Ma L., Sun Y., Li X., Wang H., Zhang H. (2019). Spatial Variation of Demersal Fish Diversity and Distribution in the East China Sea: Impact of the Bottom Branches of the Kuroshio Current. J. Sea. Res. 144, 22–32. doi: 10.1016/j.seares.2018.11.003
Xu L., Yang D., Benthuysen J. A., Yin B. (2018). Key Dynamical Factors Driving the Kuroshio Subsurface Water to Reach the Zhejiang Coastal Area. J. Geophys. Res.: Oceans. 123 (12), 9061–9081. doi: 10.1029/2018JC014219
Yang D., Yin B., Chai F., Feng X., Xue H., Gao G., et al. (2018). The Onshore Intrusion of Kuroshio Subsurface Water From February to July and a Mechanism for the Intrusion Variation. Prog. Oceanogr. 167, 97–115. doi: 10.1016/j.pocean.2018.08.004
Yang D., Yin B., Liu Z., Bai T., Qi J., Chen H. (2012). Numerical Study on the Pattern and Origins of Kuroshio Branches in the Bottom Water of Southern East China Sea in Summer. J. Geophys. Res.: Oceans. 117, C02014. doi: 10.1029/2011JC007528
Yang D., Yin B., Sun J., Zhang Y. (2013). Numerical Study on the Origins and the Forcing Mechanism of the Phosphate in Upwelling Areas Off the Coast of Zhejiang Province, China in Summer. J. Mar. Syst. 123, 1–18. doi: 10.1016/j.jmarsys.2013.04.002
Yuan D. L., Hsueh Y. (2010). Dynamics of the Cross-Shelf Circulation in the Yellow and East China Seas in Winter. Deep. Sea. Res. Part II. 57 (19-20), 1745–1761. doi: 10.1016/j.dsr2.2010.04.002
Yuan R., Wu H., Zhu J., Li L. (2016). The Response Time of the Changjiang Plume to River Discharge in Summer. J. Mar. Syst. 154, 82–92. doi: 10.1016/j.jmarsys.2015.04.001
Zainuddin M., Farhum S. A., Safruddin S., Hidayat R., Putri A. R. S., Ridwan M. (2021). “Dynamics of Thermal Fronts Distribution in the Flores Sea, Indonesia: An Implication for Locating Potential Skipjack Tuna Fishing Ground”. IOP Conf. Series: Earth Environ. Sci. 763, 012045. doi: 10.1088/1755-1315/763/1/012045
Zhang W., Dunne J. P., Wu H., Zhou F., Huang D. (2022). Using Timescales of Deficit and Residence to Evaluate Near-Bottom Dissolved Oxygen Variation in Coastal Seas. J. Geophys. Res.: Biogeosci. 127 (1), e2021JG006408. doi: 10.1029/2021JG006408
Zhang J., Guo X. Y., Zhao L., Miyazawa Y., Sun Q. (2017). Water Exchange Across Isobaths Over the Continental Shelf of the East China Sea. J. Phys. Oceanogr. 47, 1043–1060. doi: 10.1175/JPO-D-16-0231.1
Zhang H., Wang G., Zhang C., Su R., Shi X., Wang X. (2020). Characterization of the Development Stages and Roles of Nutrients and Other Environmental Factors in Green Tides in the Southern Yellow Sea, China. Harmf. Algae. 98, 101893. doi: 10.1016/j.hal.2020.101893
Keywords: fish kills, small yellow croaker, East China Sea, cooling, cold eddy, ocean fronts
Citation: Wei Z, Jiao X, Zhang J, Du Y, Rong Z, Wu H and Wang YP (2022) Dynamic Cause of the Sudden Death Event of Small Yellow Croaker (Larimichthys polyactis) in the East China Sea in Winter 2017. Front. Mar. Sci. 9:908195. doi: 10.3389/fmars.2022.908195
Received: 30 March 2022; Accepted: 12 April 2022;
Published: 10 May 2022.
Edited by:
Chao Chen, Zhejiang Ocean University, ChinaReviewed by:
Miaohua Mao, Yantai Institute of Coastal Zone Research (CAS), ChinaCopyright © 2022 Wei, Jiao, Zhang, Du, Rong, Wu and Wang. This is an open-access article distributed under the terms of the Creative Commons Attribution License (CC BY). The use, distribution or reproduction in other forums is permitted, provided the original author(s) and the copyright owner(s) are credited and that the original publication in this journal is cited, in accordance with accepted academic practice. No use, distribution or reproduction is permitted which does not comply with these terms.
*Correspondence: Jicai Zhang, amljYWlfemhhbmdAMTYzLmNvbQ==
†These authors have contributed equally to this work and share first authorship
Disclaimer: All claims expressed in this article are solely those of the authors and do not necessarily represent those of their affiliated organizations, or those of the publisher, the editors and the reviewers. Any product that may be evaluated in this article or claim that may be made by its manufacturer is not guaranteed or endorsed by the publisher.
Research integrity at Frontiers
Learn more about the work of our research integrity team to safeguard the quality of each article we publish.