- 1Nanjing Agricultural University, Wuxi, China
- 2Laboratory for Marine Fisheries Science and Food Production Processes, Pilot National Laboratory for Marine Science and Technology (Qingdao), Yellow Sea Fisheries Research Institute, Chinese Academy of Fishery Sciences, Qingdao, China
Astaxanthin (Axn) is a xanthophyll carotenoid that has previously been shown to suppress hepatic inflammation, reduce oxidative liver damage, and improve metabolic profiles. Exopalaemon carinicauda (E. carinicauda) is an economically important fishery species in China that has been found to exhibit increased body weight following Axn feeding as compared to a standard diet. In this study, dietary Axn can significantly decreased MDA content, T-AOC and significantly increased SOD, GSH and CAT activities in shrimp hepatopancreas. Moreover, transcriptome and metabolome of E. carinicauda after Axn feeding were investigated to identify the mechanism of the effect of Axn on E. carinicauda. The transcriptomic data revealed that a total 99 different expression genes (DEGs) were identified between the Axn and control groups, of which 47 and 52 were upregulated and downregulated, respectively. DEGs of E. carinicauda such as catherpsin, actin and PARP after Axn feeding were associated with apoptosis and immune system. The metabolomic analysis revealed that A total of 73 different expression metabolites (DEMs) were identified in both metabolites, including 30 downregulated metabolites and 43 upregulated metabolites. And Axn participate in metabolism processes in hepatopancreas of E. carinicauda, including the TCA cycle, amino acid metabolism and lipid metabolism. The multiple comparative analysis implicated that Axn can improve the antioxidant capacity of hepatopancreas and the energy supply of hepatopancreas mitochondria, and then improve the ability of anti-apoptosis. Collectively, all these results will greatly provide new insights into the molecular mechanisms underlying tolerance of adverse environment in E. carinicauda.
Introduction
Astaxanthin (Axn) is a xanthophyll carotenoid present in algae, yeast, and aquatic animals that has been linked to hepatoprotective activity (Yuan et al., 2011). Shrimp are unable to synthesize Axn and must instead obtain it from dietary sources (Diaz et al., 2014). Axn has previously been reported to accumulate in the liver, particularly in the mitochondrial and microsomal compartments therein (Takahashi et al., 2004). Oxidative stress can have adverse effects on affected organisms owing to increase reactive oxygen species (ROS) production, reduced ATP levels, membrane peroxidation, and morphological changes (McCarty, 2011). Axn can counteract such stress, and has been shown to reduce hepatic inflammation, decrease oxidative liver damage, and improve metabolic profiles (Bhuvaneswari et al., 2010; Curek et al., 2010; Islam et al., 2017). As an antioxidant, Axn has previously been shown to enhance tolerance for environmental stressors in Penaeus monodon, improving their recovery following thermal and osmotic stress exposure (Chien et al., 2003). Similarly, Axn protects against hepatopancreatic injury induced by nitrite stress in P. muelleri (Diaz et al., 2014). These results highly Axn as a promising therapeutic agent with the potential to be leveraged for treating hepatopancreatic diseases driven in part by oxidative stress.
Exopalaemon carinicauda (E. carinicauda) is an economically important fishery species in China, accounting for 30% of gross aquaculture production from polyculture ponds (Xu et al., 2010). E. carinicauda exhibit rapid growth and high reproductive performance (Ge et al., 2019), making it one of the most commercially valuable pond-raised species of shrimp. For these same reasons, it is also an ideal experimental model system that can be utilized to assess gene expression changes following Axn feeding.
The hepatopancreas is a multifunctional organ in crustaceans that regulates nutrient absorption, metabolism, and immune activity (Roszer, 2014), in addition to serving as a target organ diverse environmental stress responses, including osmotic stress, ammonia nitrogen stress, high temperature exposure, and heavy metal stress (Wang et al., 2008; Clark et al., 2013; Xie et al., 2014). While Axn has previously been reported to enhance stress resistance in crustaceans, the ability of its antioxidant activity to modulate stress resistance in E. carinicauda has not been thoroughly studied to date.
In this research, comparative transcriptomic analyses and metabolomic analysis were employed to assess changes in gene expression in E. carinicauda following Axn feeding. The overall goal of this analysis was to clarify the molecular effects of Axn on the hepatopancreas in E. carinicauda and to more fully elucidate the mechanisms whereby Axn contributes to stress resistance in this shrimp species.
Materials and Methods
Experimental Animals and Axn Sources
Post-larval prawns (day 10) were selected as experimental subjects. In total, 150 E. carinicauda were obtained from Haichen Aquatic Products Co. LTD in Rizhao, China. These E. carinicauda were allowed to acclimate for 1 week to temperature, salinity, dissolved oxygen, and pH conditions of 23 ± 0.5°C, 30 ± 1 psu, 7.5 ± 0.3 mgL−1, and 8.3 ± 0.1, respectively. During this period, shrimp were fed twice per day (8:00, 18:00) with first hatching brine shrimp (Artemia salina). To ensure consistent water quality, half of the seawater was replaced every other day.
Feed Preparation and Composition
Commercial shrimp feed (~1.0 mm in diameter) free of Axn [(Bioalgo, Shandong, China)] supplementation for L. vannamei served as a basal diet. For shrimp in the Axn treatment group, supplemental Axn was added at 0.1 g/kg, as this was identified as an optimal dose in our preliminary experiments (Table 1).
Experimental System and Feeding Trial
At the start of the feeding trial, 30 similarly-sized E. carinicauda were weighed by using an electronic scale ( ± 0.001 g), with the individual weight values of each shrimp being calculated (0.06 ± 0.01 g). After the completion of the acclimatization period, 6 water tanks were established, with 25 E. carinicauda being raised per tank. Feeding trials lasted for 56 days, and water quality was maintained as follows throughout the trials: temperature, 27 ± 1 °C; salinity, 30 ± 1 psu; dissolved oxygen, 7.4 ± 0.3 mg L−1; pH value, 8.2 ± 0.1.
E. carinicauda were fed thrice daily with appropriate experimental diets (8:00, 14:00, 20:00), with feeding amounts being regularly adjusted to ensure apparent satiation. Waste, including exuviates, feces, and dead bodies of experimental shrimp were siphoned out each morning, with half of the water being changed daily.
Sample Preparation and Analysis
All the tanks were used to pool samples that split into two replicate groups of three tanks. The muscles of the two groups were taken after eight weeks. At each of the two groups, three shrimps were taken from each tank (total = 18 shrimps) for transcriptome analysis and six shrimps were taken from each tank (total = 36 shrimps) for metabonomics analysis. Whole muscles samples were immediately flash frozen in liquid nitrogen, and stored at −80°C until further analysis.
Antioxidant Enzyme Analysis and Growth Performances
Three hepatopancreases per treatment were weighed and homogenized in the pre-chilled 0.9% saline solution (1:10, w/v) at a frequency of 60 Hz at 4°C for 30s(Tissuelyser-24, Shanghai Jingxin Technology, Shanghai, China). According to the manufacturer’s instructions using commercial assay kits (Nanjing Jiancheng Bioengineering Institute, Nanjing, China), the supernatant was collected after centrifuging at 4°C for 15 min(eppendorf, Germany) to measure total protein content, malondialdehyde(MDA) content, Total antioxidant capacity(T-AOC), the activities of superoxide dismutase(SOD), glutathion(GSH) and catalase(CAT). Differences in biochemical parameters were considered statistically significant at P<0.05 using a t test. Data are expressed as means ± SD (n=3).
After the experiment, 50 shrimp were randomly selected from each group for the measured body weight. Growth performance rate of shrimp were assessed in each group. FBW, final body weight (g); WG, weight gain (%) = [(final body weight − initial body weight)/initial body weight] × 100.SGR, specific growth rate (%) = [(loge final body weight − loge initial body weight)/days] × 100.
RNA Sequencing and Data Analysis
Trizol (Invitrogen, CA, USA) was utilized to extract RNA from hepatopancreas samples. RNA integrity and purity were assessed via 1% agarose gel electrophoresis. RNA purity and concentrations were assessed with a NanoPhotometer® spectrophotometer (IMPLEN, CA, USA). The Qubit® RNA Assay Kit was used to measure its integrity by Qubit® 2.0 Fluorometer (Life Technologies, CA, USA) and a Bioanalyzer 2100 instrument (Agilent Technologies, CA, USA).
RNA libraries were prepared from 3 μg RNA per sample with a NEB Next® Ultra™ RNA Library Prep Kit for Illumina® (NEB, MA, USA) that was used based on provided directions. Individual samples were identified using sequence barcodes. Library quality was then examined with an Agilent Bioanalyzer 2100 instrument following purification with an AMPure XP system. Index-coded sample clustering was performed using a TruSeq PE Cluster Kit v3-cBot-HS (Illumia) and a cBot Cluster Generation System based on provided directions. Following clustering, prepared libraries were sequenced using the Illumina Hiseq platform. After preprocessing to remove low-quality reads (quality score < 30) and those containing adaptor sequences, data were assembled using the Trinity software, with transcripts > 300 bp long being retained for further analysis. Predicted protein-coding sequence annotation was performed by querying the NCBI non-redundant (NR) protein (ftp://ftp.ncbi.nih.gov/blast/db/), SwissProt (http://www.uniprot.org/downloads), and eu-Karyotic Orthologous Groups (KOG) (ftp://ftp.ncbi.nih.gov/pub/COG/KOG/kyva) databases, with top hits being used to assign gene names. Gene Ontology (GO) (http://www.geneontology.org/) annotations were made based upon SwissProt annotations, with the WeGO software being used for functional classification (Ye et al., 2006). KEGG pathway (http://www.genome.jp/kegg/pathway.html) enrichment was used to evaluate the pathways in which these genes were involved.
Transcriptomic mapping of RNA-seq results was performed using bowtie2 (Langmead and Salzberg, 2012) and express (Roberts and Pachter, 2013), with unigene expression levels being calculated via the FPKM (fragments Per kb per Million reads) method (Trapnell et al., 2010). Differences in gene expression between groups were compared with DESeq (Anders and Huber, 2010). P < 0.05 and an absolute log2fold change > 1 were used to identify differentially expressed genes (DEGs), with biological replicates being assessed to establish sequence data quality.
Quantitative Real-Time Analysis
In total, 8 DEGs were selected for RT-qPCR analysis to validate the RNA-seq results. Briefly, cDNA was synthesized from prepared NRA using a PrimerScript™ RT Reagent Kit with gDNA Eraser (TaKaRa, Dalian, China). Appropriate RT-qPCR primers were designed using Premier Primer 5 (Table 2). Gene expression levels were assessed using SYBR® Premix Ex Taq (TaKaRa, Dalian, China) and in a 20 μL reaction with a LightCycler® 480 real-time PCR instrument (Roche, Switzerland). 18s served as a reference control for gene expression. Thermocycler settings were as follows: 95°C for 30 s; 40 cycles of 95°C for 5 s and 60°C for 34 s; 1 cycle of 95°C 15s, 60°C for 1 min and 95°C for 15s. Relative gene expression was assessed via the 2−△△Ct method, where ΔΔCt = (CtTarget gene – Ct18s) test group − (CtTarget gene – Ct18s) control group.
Metabolomic Analysis
To further investigate the changes in metabolites of hepatopancreas in response to feeding Axn, hepatopancrea samples (n = 6) from the two treatments were harvested and extracted for metabolomic analysis. Differentially expressed metabolites (DEMs) in the hepatopancrea tissues of the two treatments were analyzed using a gas chromatograph system coupled to a Pegasus HT time-of-flight mass spectrometer (GC-TOF-MS). Multivariate analyses including principal component analysis (PCA) and orthogonal partial least squares discrimination analysis (OPLS-DA) were conducted by using the SIMCA 14.1 software package (V14.1, MKS Data Analytics Solutions, Umea, Sweden). Also, the OPLS-DA models were validated using a permutation test with 200 as the permutation number. Student’s t-test (P <0.05) combined with the first principal component of variable importance in projection (VIP) values (VIP >1) were used to determine the species distribution models (SDMs) among the pairwise comparison groups. Comment HMDB database (http://www.hmdb.ca/), METLIN database (https://metlin.scripps.edu/) and KEGG database (https://www.genome.jp/kegg/) were used to search for metabolic pathways and conduct pathway analysis, which uses high-quality KEGG metabolic pathways as the backend knowledge base.
Results
The Impact of Dietary Astaxanthin Supplementation on Growth Performances and Antioxidant Capacity
Following a 56-day feeding trial period, the final body weight (FBW) of shrimp fed an Axn diet was significantly elevated as compared to shrimp fed a diet (Table 3). Consistently, the control group exhibited lower weight gain (WG) and specific growth rate (SGR) values as compared to those of Axn-fed E. carinicauda. *<0.05 compared to the control group.
In the hepatopancreas, Figure 1 was showed that the shrimp in the control gained significantly higher MDA contents than that of shrimp after Axn feeding (P < 0.05), T-AOC and the activity of GSH, SOD and CAT which was given the Axn diet were significantly higher than those in shrimp given the control diet.
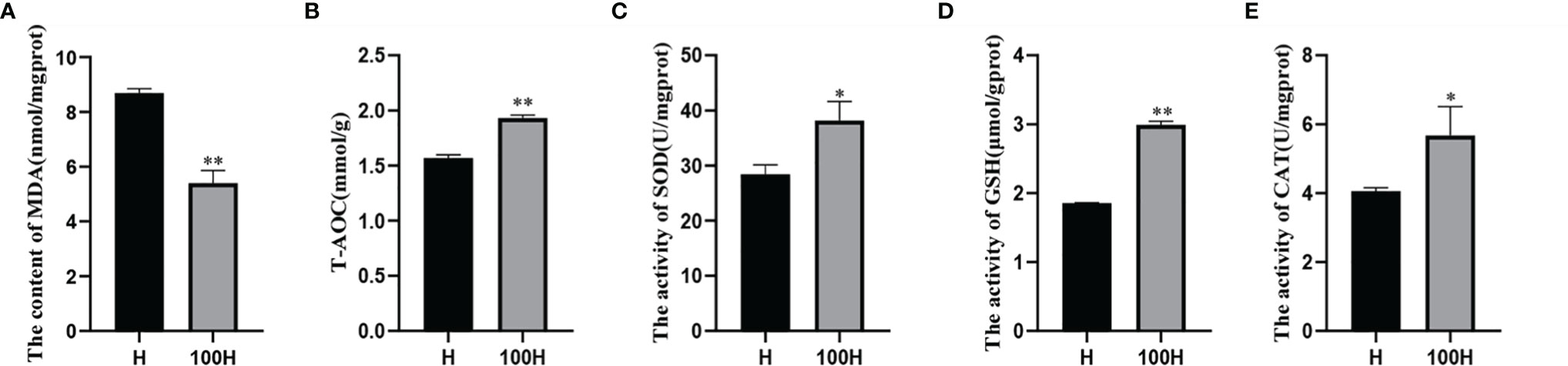
Figure 1 MDA and antioxidant enzyme activity. (A) The concent of MDA (B) T-AOC (C) The activity of SOD (D) The activity of GSH and (E) The activity of CAT. Data are expressed as means ± SD (n=3). *<0.05, **<0.01 compared to the control group.
Effects of Dietary Axn on Hepatopancreas Transcriptomics of E. carinicauda
Raw sequencing data have been deposited in the NCBI Short Read Archive (SRA) under the accession numbers SAMN26886889, SAMN26886890, SAMN26886891, SAMN26886892, SAMN26886893, SAMN26886894. In total, 99 DEGs were identified between the Axn and control groups, of which 47 and 52 were upregulated and downregulated, respectively (Figure 2A). Among them, the genes related to stress resistance, such as Catherpsin
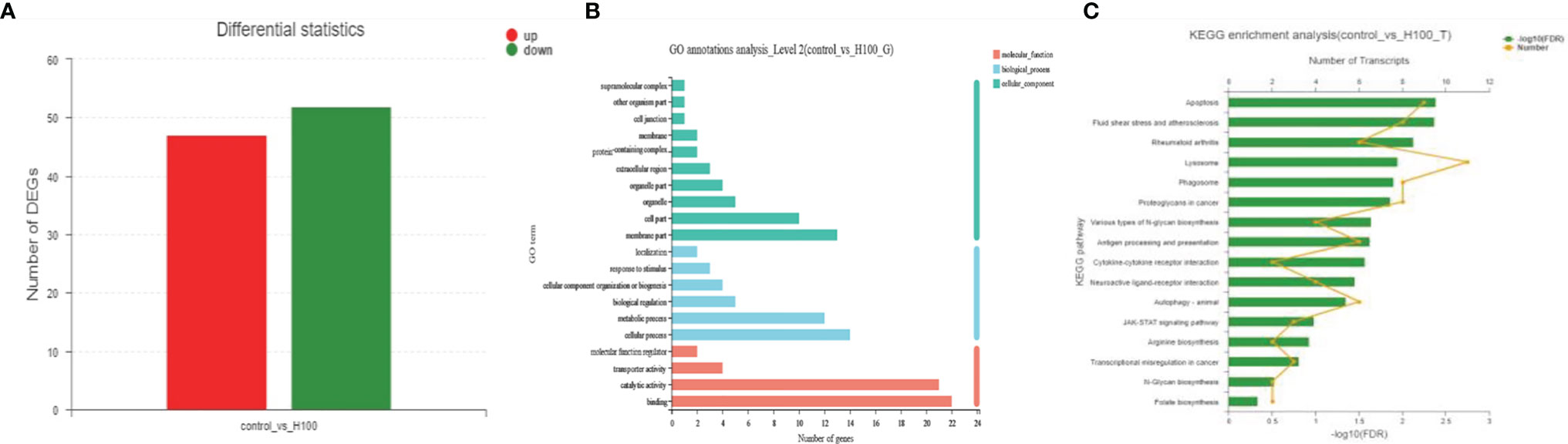
Figure 2 (A) Differential expression of the hepatopancreas of E. carinicauda following Axn feeding. Significantly upregulated and downregulated genes are respectively shown in red and green, whereas blue genes were not differentially expressed. (B) GO annotation analysis. Transcripts were assigned to GO terms in the cellular component, biological process, and molecular function categories. (C) Kyoto Encyclopedia of Genes and Genomes pathway analysis of the transcriptomic responses for E. carinicauda after feeding Axn.
Actin and PARP have significant changes in their gene expression level.
To understand the biological significance of the DEGs, GO analysis was used to annotate these DEGs with terms under biological process, cellular component, and molecular function categories. In the biological process category, the DEGs were mostly associated with cellular and metabolic processes. In the cellular component category, most of the DEGs were assigned to subcellular structures. In the molecular function category, most of the DEGs were categorized into binding and catalytic activities (Figure 2B).
To identify the biochemical pathways influenced by Axn feeding, the KEGG database was used to perform pathway enrichment analysis on the identified DEGs. Of the pathways identified, the most commonly represented class was related to stress and included several subclasses: “Apoptosis,” “Lysosome,” “Phagosome,” “Antigen processing and presentation,” “Cytokine-cytokine receptor interaction,” “JAK-STAT signaling pathway,” “Arginine biosynthesis” and “Autophagy” (Figure 2C).
Effects of Dietary Axn on Hepatopancreas Metabolomics of E. carinicauda
The UHPLC-Q-TOF-MS platform was used to analyze the hepatopancreas about untargeted metabolomic analysis to investigate the metabolic changes in E. carinicauda in response to Axn feeding. A total of 825 negative and 805 positive ion peaks were extracted from the analysis. A total of 73 DEMs were identified in both metabolites, including 30 downregulated metabolites and 43 upregulated metabolites (Table 4). The established OPLS-DA model indicated that the model was stable and reliable. Positive ion mode: R2Y = 0.99 cum, Q2Y = 0.75 cum, negative ion mode: R2Y = 0.99 cum, Q2Y = 0.79 cum (Figures 3A, B). Next, 200 OPLS-DA models were established by the permutation test in which the order of the categorical variables Y was changed randomly to obtain the R2 and Q2 values of the stochastic model (Figures 3C, D). All Q2 points were lower than the original red Q2 points on the right from left to right, which indicated a robust and reliable model without overfitting. Thus, it is reliable and stable for the test data and instrument analysis system for the experiment.
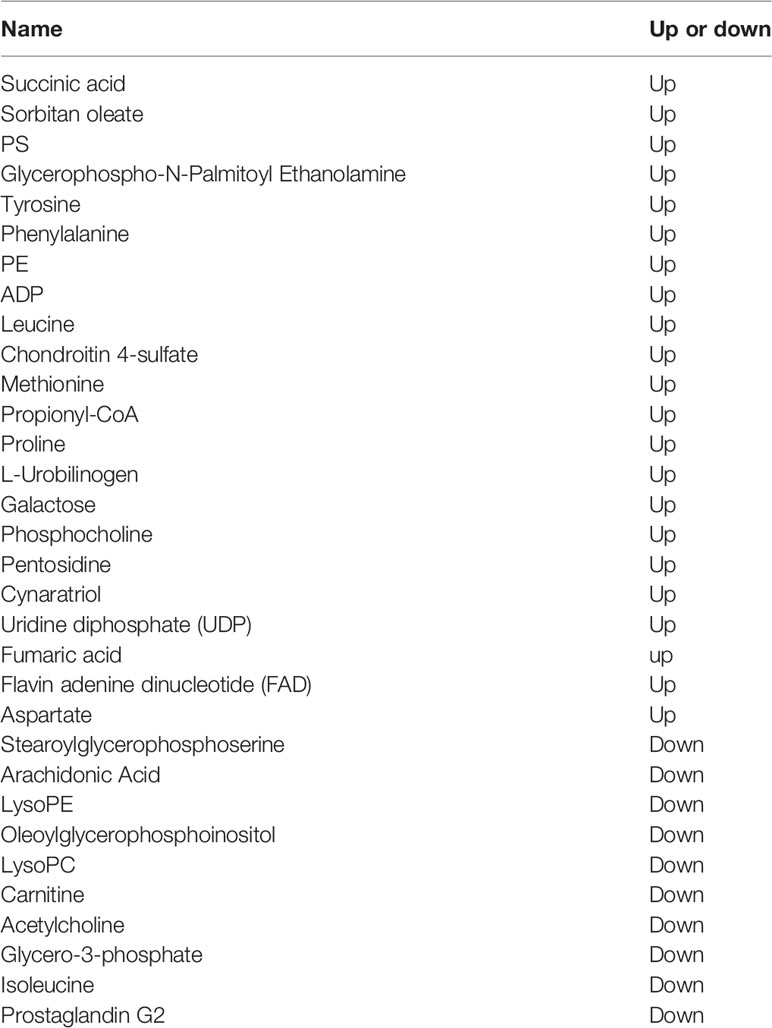
Table 4 Differentially expressed metabolites in the shrimp muscle tissue in response to Axn feeding.
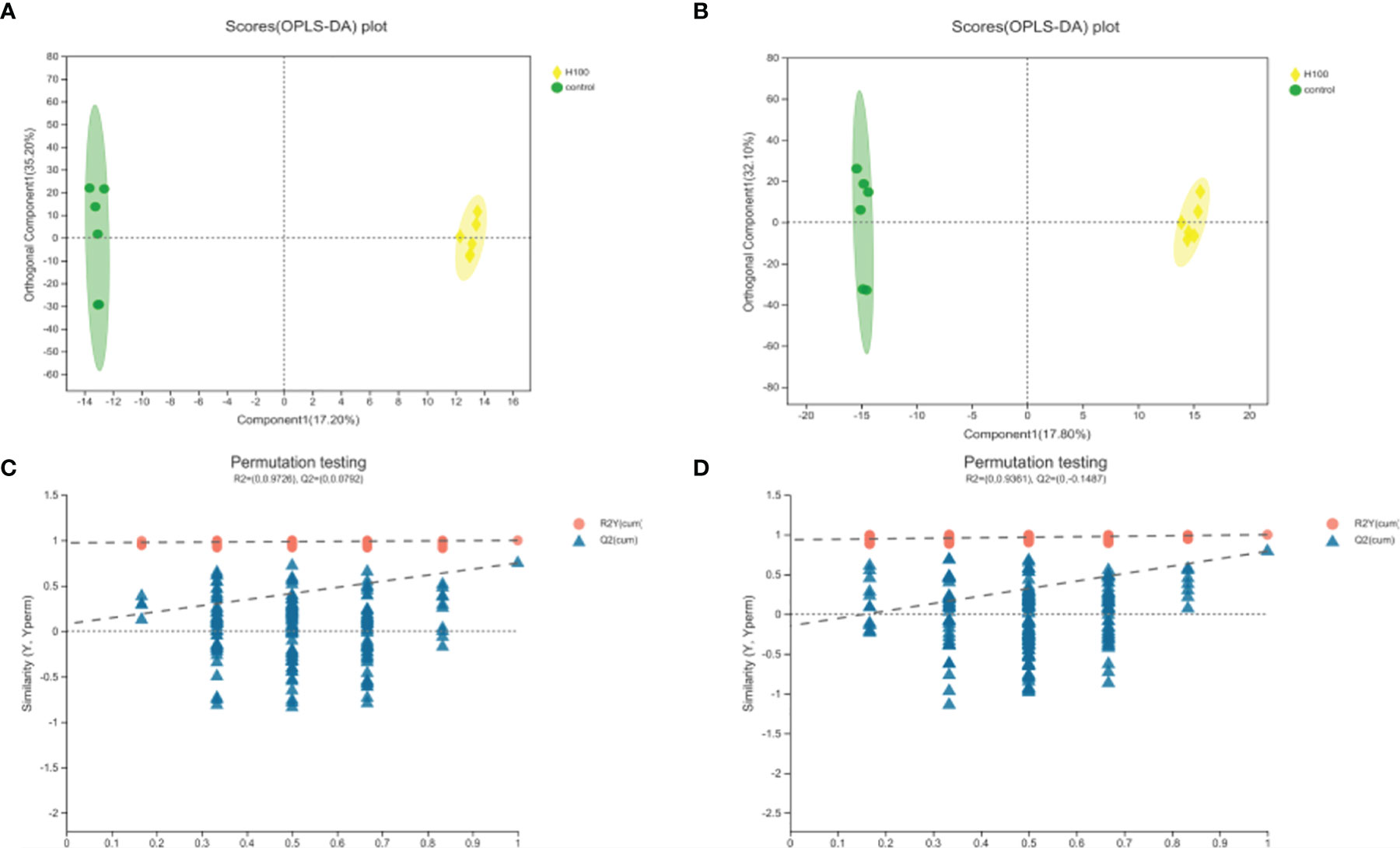
Figure 3 Quality analysis of metabolomic data. (A) OPLS-DA (orthogonal partial least-squares-discriminant analysis) score diagram for the positive ion mode. (B) OPLS-DA score diagram for the negative ion mode. (C) OPLS-DA permutation test for the positive ion mode. (D) OPLS-DA permutation test for the negative ion mode.
KEGG pathway analysis was used to assign these DEMs to metabolic pathways for exploring the metabolic pathways affected by Axn feeding. The pathway analysis results provided details of the changes in metabolic pathways related to Axn feeding. The most relevant pathways were identified based on a p-value < 0.05 and were “Thermogenesis,” “Taste transduction,” “Synaptic vesicle cycle,” “Regulation of actin cytoskeleton,” “Phenylalanine metabolism,” “Lysosome,” “Glycosaminoglycan degradation” “Glycerophospholipid metabolism,” and “FoxO signaling pathway” (Figure 4).
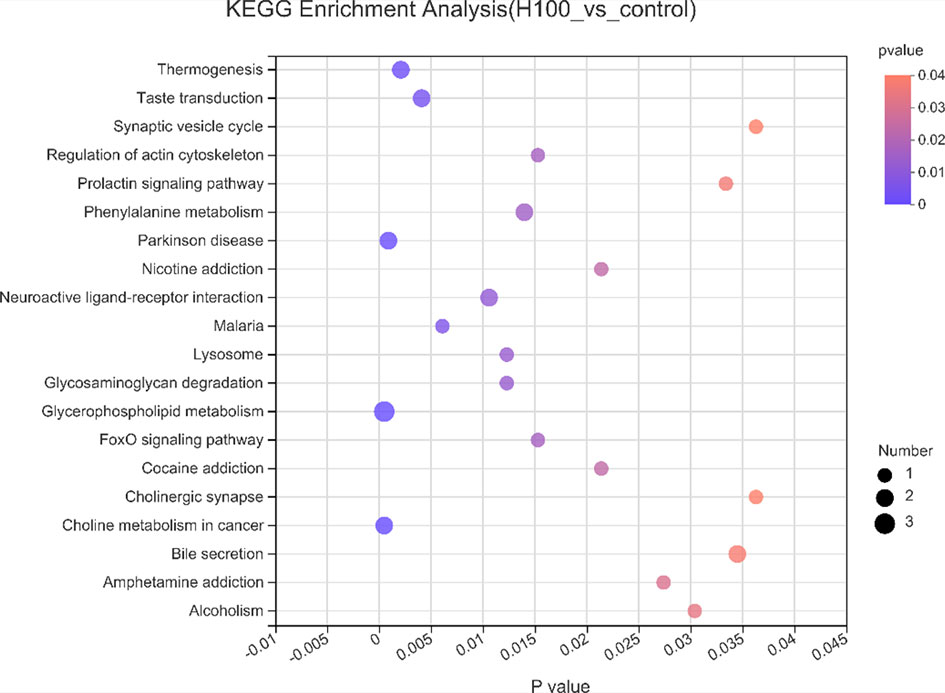
Figure 4 Kyoto Encyclopedia of Genes and Genomes pathway analysis of differentially expressed metabolites in response to Axn. The ordinate represents the top 20 KEGG terms significantly enriched by the DEMs, the abscissa indicates the rich factor between two sampling datasets.
RNA-seq Result Validation
To confirm the accuracy of our RNA-seq analyses, 8 genes were selected for an RT-qPCR-based validation assay, revealing significant changes in the expression of these genes consistent with the results of the RNA-seq analysis (Figure 5).
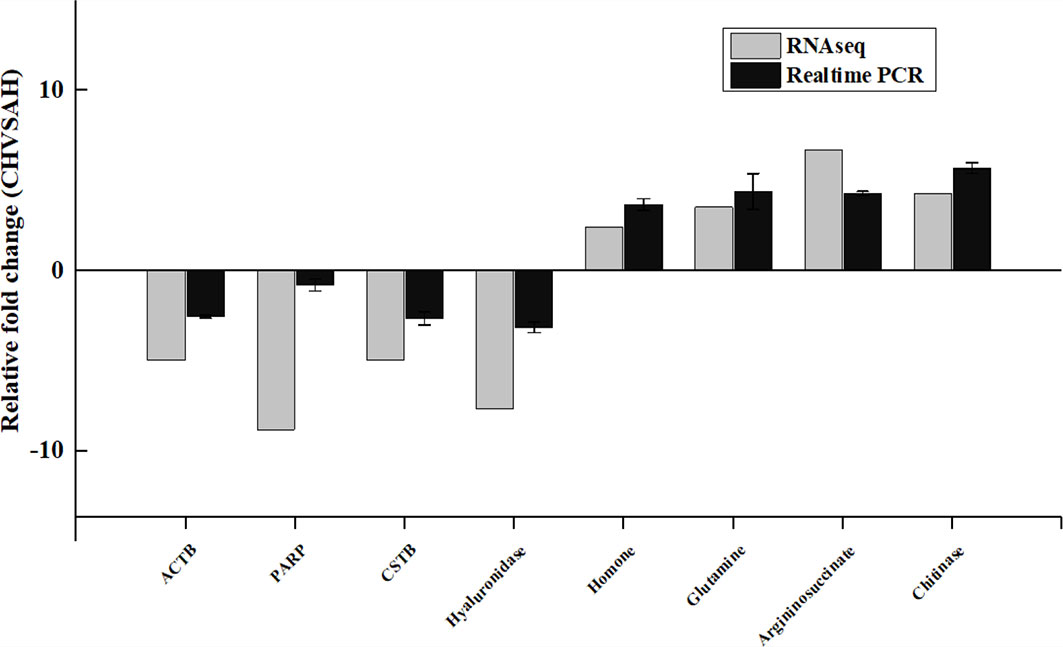
Figure 5 RT-qPCR-based RNA-seq result validation. Gene abbreviations: Catherpsin, Actin, poly ADP-ribose polymerase (PARP), Hyaluronidase, Homone, Glutamine, Argininosuccinate, Chitinase. Fold changes represent gene expression changes in each group relative to control.
Identification of Key Genes and Metabolites Using Multi-Omics Analysis
KEGG pathway analysis of genes and metabolomics was performed to determine correlations between the transcriptomic and metabolomic data (Figure 6). The analysis showed that the TCA cycle, carbohydrate metabolism, amino acid metabolism, fatty acid metabolism, energy metabolism and apoptosis signaling pathways were affected by Axn feeding. These pathways are important components of the metabolic pathways. Accordingly, the results showed that upregulation of succinic acid indicates vigorous metabolism of the TCA cycle. Similarly, it was observed that upregulation of most of the DEGs and DEMs was related to amino acid biosynthesis and fatty acid metabolism. Interestingly, the levels of fatty acids, such as arachidonic acid and carnitine, were downregulated while ADP was upregulated. These results indicate the importance of these metabolites in energy replenishment.
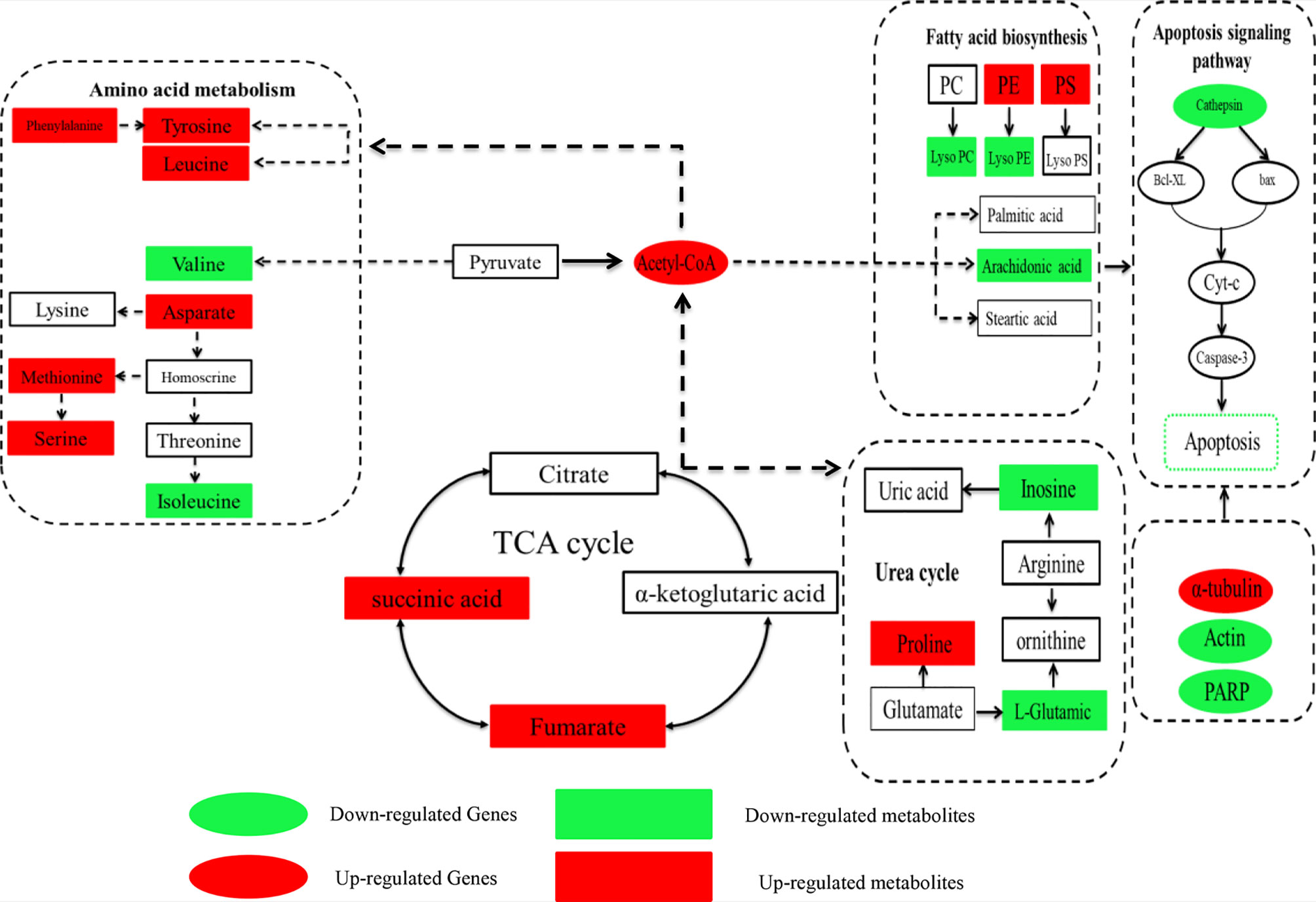
Figure 6 Correlation map of genes and metabolites regulated by Axn feeding in the muscle of E. carinicauda. The altered genes and metabolites are shown by marking the names in red (upregulated) or green (downregulated).
Discussion
The hepatopancreas functions as a multifunctional organ involved in nutrient uptake and intermediary carbon and nitrogen metabolism (Roszer, 2014). Some studies have also suggested that in mollusks and crustaceans, the hepatopancreas plays a central role in innate immunity (Huang et al., 2013; Liu et al., 2013; Zhang et al., 2014), contributing to antigen processing pathogen elimination, and infection-related changes in metabolic activity (Alday-Sanz et al., 2002). Metabolic regulation and nutrition can also affect immune response efficiency within the hepatopancreas (Mohankumar and Ramasamy, 2006; Nagaraju et al., 2011). Axn is a nutrient and an antioxidant that primarily accumulates within the mitochondria and microsomes of hepatopancreatic cells, thereby bolstering stress resistance in crustaceans (Diaz et al., 2014). And It had been found that combined transcriptomic and metabolomic data indicated that amino acid metabolic pathways and fatty acid metabolic pathway were changed accordingly in the muscles of E. carinicauda after Axn feeding (Li et al., 2022). The molecular mechanism of Axn feeding in the hepatopancrea of E. carinicauda remains unclear. In the current study, we analyzed antioxidant enzymes and changes in genes and metabolites involved in Axn feeding. Antioxidant enzyme analysis demonstrated that Axn can improve the antioxidant capacity of E. carinicauda. Furthermore, it was confirmed that Axn feeding affected amino acid, fatty acid, and energy metabolism, which may be related to the cytoskeleton and apoptosis.
Changes in Amino Acid Metabolism and the Cytoskeleton in Response to Dietary Astaxanthin
It was found that dietary Axn provoked a response involving changes in amino acid metabolism. Metabolomic data indicated that most amino acids (e.g. phenylalanine, tyrosine, leucine, asparate, methionine, serine and proline) were significantly more abundant in shrimp after Axn feeding, whereas, valine and isoleucine levels were significantly reduced. Succinic acid and fumaric acid are intermediates in the TCA cycle, while phenylalanine, tyrosine, leucine, asparate, methionine, serine and proline are the important intermediates in the TCA cycle as the precursors for oxaloacetic acid, acetyl-CoA andα-ketoglutarate and play important functions in many organisms (Wu et al., 2018). Valine and isoleucine are branched-chain amino acids (BCAAs) and are associated with a range of biological functions including protein synthesis, energy production, neurotransmission and immunity (Zhang et al., 2017). The observed results may suggest that Axn can enhance TCA cycle activity, with the significant increases in amino acid levels in E. carinicauda corresponding to improved resilience when faced with an adverse external environment.
Glutamate is a metabolic precursor of glutathione, proline, and arginine. Therefore, increased levels of glutamate may suggest the enhanced metabolism of these three factors. In the present study, although glutamate levels did not change significantly in response to Axn feeding, proline levels were significantly increased and L-glutamic levels were significantly decreased (Ren et al., 2020). The reason for the condition may be that E. carinicauda were not placed under specific stress conditions in this study, and glutamate is converted into proline as a reserve. When E. carinicauda are exposed to adverse environmental stimulation, it can be play serve to mitigate such stress.
Amino acids are an important part of the cytoskeleton (Xiao et al., 2020), and increases in the levels of various amino acids may thus make the cytoskeleton more resistant to adverse factors. Consistent changes in cytoskeleton-related genes were also observed.
Changes in Lipid Metabolism in Response to Dietary Astaxanthin
Fatty acids, particularly unsaturated fatty acids (UFAs) such as lecithin (PC), cephalin (PE), and phosphatidylserine (PS), are the basic components of cells and are involved in a variety of biological processes. Fatty acids were reported to play important roles in the immune system in response to stress (Zuo et al., 2017; Gao et al., 2018). Our metabolomics data suggested that the levels of most PCs and PSs were increased after Axn feeding. Interestingly, however, lysolecithin (LysoPC) and lysocephalin (LysoPE) levels were decreased after Axn feeding. LysoPC reportedly participates in cellular metabolism and energy metabolism, maintaining normal biofilm structure and physiological activity (Ren et al., 2020). These data may suggest that the hepatopancreas can store excess UFAs as reserve energy after astaxanthin feeding. The observed reductions in LysoPE and LysoPS levels may be attributable to the absence of any stress exposure for these shrimps such that there is no need for excess LysoPE and LysoPS to maintain the normal homeostasis.
Metabolomic analyses of the hepatopancreas indicated that the fatty acid biosynthesis and arachidonic acid metabolism pathways were enriched. Arachidonic acid (ARA) levels were decreased in the hepatopancreas in response to Axn feeding, and the levels of certain fatty acids, such as prostaglandin G2, which are related to the functional regulation of immune cells (Tallima and El Ridi, 2018), were also decreased in shrimp that were fed Axn relative to the control group. This may indicate that the immune activity of E. carinicauda is augmented in response to dietary Axn intake.
Energy Metabolism and Apoptosis Related Changes in Response to Dietary Astaxanthin
Oxidative stress is one of the key drivers of cellular toxicity resulting from environmental stress exposure. Several studies of juvenile crustaceans have shown that some adverse environmental stimuli can spur an increase in reactive oxygen species (ROS) production and can alter antioxidant defenses (Cheng et al., 2003; Li et al., 2017). Interestingly, these resutls suggested that SOD, CAT, GSH, and T-AOC activity were increased after Axn feeding, while MDA content was reduced. SOD catalyzes the dismutation of superoxide (O2-) into oxygen (O2) and hydrogen peroxide (H2O2), while CAT, T-AOC, and GSH decompose H2O2 into O2 and H2O. Increased SOD activity can lead to elevated levels of H2O2, while increases in CAT, GSH, CAT and T-AOC activity can mitigate the damage caused by H2O2. This decrease of H2O2 levels can have a protective effect on the hepatopancreas, as demonstrated by the increased levels of MDA after Axn feeding.
In addition, when crustaceans are exposed to adverse environmental stimuli, energy reserves are mobilized to meet increased energy expenditure needs in energy-consuming processes (Meng et al., 2021). Glycero-3-phosphate is an intermediate product of glycolysis and ADP is the product of ATP. Both are critical for mitochondrial ATP generation, as well as the TCA cycle. Decreases in their levels may suggest that E. carinicauda exhibit the accelerated activity of key energy metabolism pathways including the TCA cycle, providing sufficient ATP to support the stress responses induced by adverse environmental stimuli.
Among the identified DEGs, actin and poly ADP-ribose polymerase (PARP), which participates in apoptosis, was found to be downregulated by Axn feeding. Protection against stress was found to be associated with changes in the expression levels of genes related to apoptosis (Xiao et al., 2019). When the body is exposed to aversive stimuli, apoptosis-related genes are significantly upregulated. When the expression of these genes rises significantly, this in turn engages anti-stress mechanisms (Long et al., 2013). As such, the observed changes in actin and PARP expression may be related to Axn feeding, with their upregulation being linked to the ability of Axn to augment anti-apoptotic activity in E. carinicauda. In addition, the expression of cathepsin was significantly decreased after Axn feeding. Cathepsin, which induces the upregulation of proapoptotic genes and the downregulation of anti-apoptotic genes (Li et al., 2016), may contribute to the release of Cyt-c into the cytoplasm from the mitochondria, leading to apoptosis. In light of these results, we speculate that Axn can improve anti-apoptotic activity.
Conclusion
In this study, physiological and molecular differences were investigated in E. carinicauda after Axn feeding. The results were shown that Axn could improve the antioxidant capacity of hepatopancreas, so as to protect the body from damage brought about by oxidative stress. Most of DEGs were associated with apoptosis and immune system after Axn feeding. Furthermore, the combination of transcriptomic and metabolomic analysis revealed that Axn participated in metabolism processes in E. carinicauda, including amino acid metabolism, lipid metabolism and energy metabolism. All the results revealed that Astaxanthin can improve the ability of E. carinicauda to adapt to adverse environment.
Data Availability Statement
Publicly available datasets were analyzed in this study. This data can be found here: We have deposited the data in National Center for Biotechnology Information (NCBI). BioProject ID: PRJNA818883.
Ethics Statement
The animal study was reviewed and approved by Yellow Sea Fisheries Research Institute, Chinese Academy of Fishery Sciences, China.
Author Contributions
Conceptualization, JL and WL; methodology, JW, JTL and PL; software, WL; validation, WL; formal analysis, JW and WL; investigation, WL; resources, JL; data curation, WL; writing original draft preparation, WL; writing review and editing, JW, JL and FZ; visualization, WL; supervision, JL and PL; project administration, JL; funding acquisition, JL. All authors have read and agreed to the published version of the manuscript.
Funding
This project was financially supported by the earmarked fund for the National Key R&D Program of China (2019YFD0900403), the Modern Agro-industry Technology Research System (CARS-48), the National Natural Science Foundation of China (32072974) and Central Public-interest Scientific Institution Basal Research Fund, CAFS (2020TD46).
Conflict of Interest
The authors declare that the research was conducted in the absence of any commercial or financial relationships that could be construed as a potential conflict of interest.
Publisher’s Note
All claims expressed in this article are solely those of the authors and do not necessarily represent those of their affiliated organizations, or those of the publisher, the editors and the reviewers. Any product that may be evaluated in this article, or claim that may be made by its manufacturer, is not guaranteed or endorsed by the publisher.
References
Alday-Sanz V., Roque A., Turnbull J. F. (2002). Clearing Mechanisms of Vibrio Vulnificus Biotype I in the Black Tiger Shrimp Penaeus Monodon. Dis. Aquat. Organ 48 (2), 91–99. doi: 10.3354/dao048091
Anders S., Huber W. (2010). Differential Expression Analysis for Sequence Count Data. Genome Biol. 11 (10), R106. doi: 10.1186/gb-2010-11-10-r106
Bhuvaneswari S., Arunkumar E., Viswanathan P., Anuradha C. V. (2010). Astaxanthin Restricts Weight Gain, Promotes Insulin Sensitivity and Curtails Fatty Liver Disease in Mice Fed a Obesity-Promoting Diet. Process Biochem. 45 (8), 1406–1414. doi: 10.1016/j.procbio.2010.05.016
Cheng W., Chen S. M., Wang F. I., Hsu P. I., Liu C. H., Chen J. C. (2003). Effects of Temperature, Ph, Salinity and Ammonia on the Phagocytic Activity and Clearance Efficiency of Giant Freshwater Prawn Macrobrachium Rosenbergii to Lactococcus Garvieae. Aquaculture 219 (1-4), 111–121. doi: 10.1016/S0044-8486(03)00017-6
Chien Y. H., Pan C. H., Hunter B. (2003). The Resistance to Physical Stresses by Penaeus Monodon Juveniles Fed Diets Supplemented With Astaxanthin. Aquaculture 216 (1-4), 177–191. doi: 10.1016/S0044-8486(02)00056-X
Clark K. F., Acorn A. R., Greenwood S. J. (2013). A Transcriptomic Analysis of American Lobster (Homarus Americanus) Immune Response During Infection With the Bumper Car Parasite Anophryoides Haemophila. Dev. Comp. Immunol. 40 (2), 112–122. doi: 10.1016/j.dci.2013.02.009
Curek G. D., Cort A., Yucel G., Demir N., Ozturk S., Elpek G. O., et al. (2010). Effect of Astaxanthin on Hepatocellular Injury Following Ischemia/Reperfusion. Toxicology 267 (1-3), 147–153. doi: 10.1016/j.tox.2009.11.003
Diaz A. C., Velurtas S. M., Espino M. L., Fenucci J. L. (2014). Effect of Dietary Astaxanthin on Free Radical Scavenging Capacity and Nitrite Stress Tolerance of Postlarvae Shrimp, Pleoticus Muelleri. J. Agric. Food Chem. 62 (51), 12326–12331. doi: 10.1021/jf503754q
Gao Y., Zheng S. C., Zheng C. Q., Shi Y. C., Xie X. L., Wang K. J., et al. (2018). The Immune-Related Fatty Acids are Responsive to CO2 Driven Seawater Acidification in a Crustacean Brine Shrimp Artemia Sinica. Dev. Comp. Immunol. 81, 342–347. doi: 10.1016/j.dci.2017.12.022
Ge Q., Li J., Wang J., Li Z., Li J. (2019). Characterization, Functional Analysis, and Expression Levels of Three Carbonic Anhydrases in Response to pH and Saline-Alkaline Stresses in the Ridgetail White Prawn Exopalaemon Carinicauda. Cell Stress Chaperones 24 (3), 503–515. doi: 10.1007/s12192-019-00987-z
Huang M. M., Song X. Y., Zhao J. M., Mu C. K., Wang L. L., Zhang H., et al. (2013). A C-Type Lectin (AiCTL-3) From Bay Scallop Argopecten Irradians With Mannose/Galactose Binding Ability to Bind Various Bacteria. Gene 531 (1), 31–38. doi: 10.1016/j.gene.2013.08.042
Islam M. A., Al Mamun M. A., Faruk M., Ul Islam M. T., Rahman M. M., Alam M. N., et al. (2017). Astaxanthin Ameliorates Hepatic Damage and Oxidative Stress in Carbon Tetrachloride-Administered Rats. Pharmacognosy Res. 9 (Suppl 1), S84–S91. doi: 10.4103/pr.pr_26_17
Langmead B., Salzberg S. L. (2012). Fast Gapped-Read Alignment With Bowtie 2. Nat. Methods 9 (4), 357–359. doi: 10.1038/nmeth.1923
Li T. Y., Li E. C., Suo Y. T., Xu Z. X., Jia Y. Y., Qin J. G., et al. (2017). Energy Metabolism and Metabolomics Response of Pacific White Shrimp Litopenaeus Vannamei to Sulfide Toxicity. Aquat Toxicol. 183, 28–37. doi: 10.1016/j.aquatox.2016.12.010
Liu X. L., Ji C. L., Zhao J. M., Wu H. F. (2013). Differential Metabolic Responses of Clam Ruditapes Philippinarum to Vibrio Anguillarum and Vibrio Splendidus Challenges. Fish Shellfish Immun. 35 (6), 2001–2007. doi: 10.1016/j.fsi.2013.09.014
Li W., Wang J., Li J., Liu P., Li J., Zhao F. (2022). Antioxidant, Transcriptome and the Metabolome Response to Dietary Astaxanthin in Exopalaemon Carinicauda. Front. Physiol. 13, 859305. doi: 10.3389/fphys.2022.859305
Li Y. H., Wei L., Cao J. R., Qiu L. G., Jiang X., Li P., et al. (2016). Oxidative Stress, DNA Damage and Antioxidant Enzyme Activities in the Pacific White Shrimp (Litopenaeus Vannarnei) When Exposed to Hypoxia and Reoxygenation. Chemosphere 144, 234–240. doi: 10.1016/j.chemosphere.2015.08.051
Long Y., Song G. L., Yan J. J., He X. Z., Li Q., Cui Z. B. (2013). Transcriptomic Characterization of Cold Acclimation in Larval Zebrafish. BMC Genomics 14, 612. doi: 10.1186/1471-2164-14-612
McCarty M. F. (2011). Full-Spectrum Antioxidant Therapy Featuring Astaxanthin Coupled With Lipoprivic Strategies and Salsalate for Management of non-Alcoholic Fatty Liver Disease. Med. Hypotheses 77 (4), 550–556. doi: 10.1016/j.mehy.2011.06.029
Meng X. L., Jayasundara N., Zhang J. Y., Ren X. Y., Gao B. Q., Li J., et al. (2021). Integrated Physiological, Transcriptome and Metabolome Analyses of the Hepatopancreas of the Female Swimming Crab Portunus Trituberculatus Under Ammonia Exposure. Ecotox. Environ. Safe. 228, 113026. doi: 10.1016/j.ecoenv.2021.113026
Mohankumar K., Ramasamy P. (2006). Activities of Membrane Bound Phosphatases, Transaminases and Mitochondrial Enzymes in White Spot Syndrome Virus Infected Tissues of Fenneropenaeus Indicus. Virus Res. 118 (1-2), 130–135. doi: 10.1016/j.virusres.2005.12.002
Nagaraju G. P. C., Rajitha B., Borst D. W. (2011). Molecular Cloning and Sequence of Retinoid X Receptor in the Green Crab Carcinus Maenas: A Possible Role in Female Reproduction. J. Endocrinol. 210(3), 379–390. doi: 10.1530/JOE-11-0154
Ren X., Yu Z., Xu Y., Zhang Y., Mu C., Liu P., et al. (2020). Integrated Transcriptomic and Metabolomic Responses in the Hepatopancreas of Kuruma Shrimp (Marsupenaeus Japonicus) Under Cold Stress. Ecotoxicol. Environ. Saf. 206, 111360. doi: 10.1016/j.ecoenv.2020.111360
Roberts A., Pachter L. (2013). Streaming Fragment Assignment for Real-Time Analysis of Sequencing Experiments. Nat. Methods 10 (1), 71–73. doi: 10.1038/nmeth.2251
Roszer T. (2014). The Invertebrate Midintestinal Gland ("Hepatopancreas") Is an Evolutionary Forerunner in the Integration of Immunity and Metabolism. Cell Tissue Res. 358 (3), 685–695. doi: 10.1007/s00441-014-1985-7
Takahashi K., Watanabe M., Takimoto T., Akiba Y. (2004). Uptake and Distribution of Astaxanthin in Several Tissues and Plasma Lipoproteins in Male Broiler Chickens Fed a Yeast (Phaffia Rhodozyma) With a High Concentration of Astaxanthin. Brit Poult. Sci. 45 (1), 133–138. doi: 10.1080/00071660410001668950a
Tallima H., El Ridi R. (2018). Arachidonic Acid: Physiological Roles and Potential Health Benefits - A Review. J. Adv. Res. 11, 33–41. doi: 10.1016/j.jare.2017.11.004
Trapnell C., Williams B. A., Pertea G., Mortazavi A., Kwan G., van Baren M. J., et al. (2010). Transcript Assembly and Quantification by RNA-Seq Reveals Unannotated Transcripts and Isoform Switching During Cell Differentiation. Nat. Biotechnol. 28 (5), 511–U174. doi: 10.1038/nbt.1621
Wang L., Yan B., Liu N., Li Y. Q., Wang Q. (2008). Effects of Cadmium on Glutathione Synthesis in Hepatopancreas of Freshwater Crab, Sinopotamon Yangtsekiense. Chemosphere 74 (1), 51–56. doi: 10.1016/j.chemosphere.2008.09.025
Wu Z., Jin L., Zheng W., Zhang C., Zhang L., Chen Y., et al. (2018). NMR-Based Serum Metabolomics Study Reveals a Innovative Diagnostic Model for Missed Abortion. Biochem. Biophys. Res. Commun. 496 (2), 679–685. doi: 10.1016/j.bbrc.2018.01.096
Xiao J., Li Q. Y., Tu J. P., Chen X. L., Chen X. H., Liu Q. Y., et al. (2019). Stress Response and Tolerance Mechanisms of Ammonia Exposure Based on Transcriptomics and Metabolomics in Litopenaeus Vannamei. Ecotox Environ. Safe 180, 491–500. doi: 10.1016/j.ecoenv.2019.05.029
Xiao J., Liu Q. Y., Du J. H., Zhu W. L., Li Q. Y., Chen X. L., et al. (2020). Integrated Analysis of Physiological, Transcriptomic and Metabolomic Responses and Tolerance Mechanism of Nitrite Exposure in Litopenaeus Vannamei. Sci. Total Environ. 711, 134416. doi: 10.1016/j.scitotenv.2019.134416
Xie L., Hanyu T., Futatsugi N., Komatsu M., Steinman A. D., Park H. D. (2014). Inhibitory Effect of Naringin on Microcystin-LR Uptake in the Freshwater Snail Sinotaia Histrica. Environ. Toxicol. Pharmacol. 38 (2), 430–437. doi: 10.1016/j.etap.2014.07.006
Xu W. J., Xie J. J., Shi H., Li C. W. (2010). Hematodinium Infections in Cultured Ridgetail White Prawns, Exopalaemon Carinicauda, in Eastern China. Aquaculture 300 (1-4), 25–31. doi: 10.1016/j.aquaculture.2009.12.024
Ye J., Fang L., Zheng H., Zhang Y., Chen J., Zhang Z., et al. (2006). WEGO: A Web Tool for Plotting GO Annotations. Nucleic Acids Res. 34, W293–W297. doi: 10.1093/nar/gkl031
Yuan J. P., Peng J. A., Yin K., Wang J. H. (2011). Potential Health-Promoting Effects of Astaxanthin: A High-Value Carotenoid Mostly From Microalgae. Mol. Nutr. Food Res. 55 (1), 150–165. doi: 10.1002/mnfr.201000414
Zhang X. W., Wang X. W., Huang Y., Hui K. M., Shi Y. R., Wang W., et al. (2014). Cloning and Characterization of Two Different Ficolins From the Giant Freshwater Prawn Macrobrachium Rosenbergii. Dev. Comp. Immunol. 44 (2), 359–369. doi: 10.1016/j.dci.2014.01.009
Zhang S., Zeng X., Ren M., Mao X., Qiao S. (2017). Novel Metabolic and Physiological Functions of Branched Chain Amino Acids: A Review. J. Anim. Sci. Biotechnol. 8, 10. doi: 10.1186/s40104-016-0139-z
Keywords: E. carinicauda, astaxanthin, transcriptomics, metabolomics, antioxidant
Citation: Li W, Wang J, Li J, Liu P, Li J and Zhao F (2022) Antioxidant, Transcriptomic and Metabonomic Analysis of Hepatopancreatic Stress Resistance in Exopalaemon carinicauda Following Astaxanthin Feeding. Front. Mar. Sci. 9:906963. doi: 10.3389/fmars.2022.906963
Received: 05 April 2022; Accepted: 12 May 2022;
Published: 09 June 2022.
Edited by:
Samad Rahimnejad, University of South Bohemia in České Budějovice, CzechiaReviewed by:
Songlin Li, Shanghai Ocean University, ChinaShuyan Chi, Guangdong Ocean University, China
Copyright © 2022 Li, Wang, Li, Liu, Li and Zhao. This is an open-access article distributed under the terms of the Creative Commons Attribution License (CC BY). The use, distribution or reproduction in other forums is permitted, provided the original author(s) and the copyright owner(s) are credited and that the original publication in this journal is cited, in accordance with accepted academic practice. No use, distribution or reproduction is permitted which does not comply with these terms.
*Correspondence: Jian Li, YmlnYmlyZEB5c2ZyaS5hYy5jbg==; Fazhen Zhao, emhhb2Z6QHlzZnJpLmFjLmNu