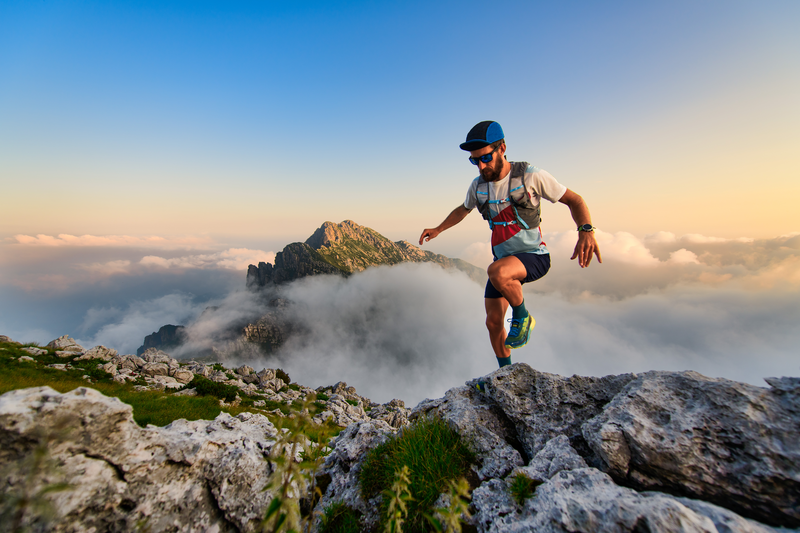
95% of researchers rate our articles as excellent or good
Learn more about the work of our research integrity team to safeguard the quality of each article we publish.
Find out more
ORIGINAL RESEARCH article
Front. Mar. Sci. , 19 May 2022
Sec. Marine Biotechnology and Bioproducts
Volume 9 - 2022 | https://doi.org/10.3389/fmars.2022.905147
This article is part of the Research Topic High Throughput Omics Technologies for Understanding Molecular Stress Response and Devising Mitigation Actions View all 8 articles
Ultraviolet B (UV-B) radiation is a potent environmental stressor and it severely affects the survival, growth, and physiology of aquatic organisms. The UV-B protective properties of three herbal ingredients enriched diets were evaluated in pre-exposed Catla catla. Fish (70.38 ± 1.18 g) were divided into five sets with three replicates each. Four sets were exposed to UV-B (80 µW/cm2) for an initial 10 days (20 min/day), and the fifth one remained unexposed (control). On day 11, feeding with enriched diets started. These diets were: diet 1 (D1) containing Withania somnifera (0.5%) root powder, diet 2 (D2) containing Emblica officinalis (1.25%) fruit powder, diet 3 (D3) containing seeds of Achyranthes aspera (0.5%), and diet 4 (D4) control. There were two batches of D4 diet-fed fish, D4a, UV-B exposed, and D4b unexposed. Blood and tissue samples were collected on days 0, 7, 14, and 21 of feeding. The average weight reduced 26.32% in UV-B exposed catla compared to the unexposed one on day 0. Among the exposed fish, average weight was significantly higher in D3 compared to others throughout the study period. Significantly lower nitric oxide synthase (NOS) and higher thiobarbituric acid reactive substances (TBARS), carbonyl protein, superoxide dismutase (SOD), and heat shock proteins (Hsp) 70 and 90 were observed in D4a compared to the enriched diet fed catla. The NOS level was significantly higher in D3 on day 21. The TBARS level was significantly lower in D3 on days 7 and 14 and in D2 and D3 on day 21. The carbonyl protein, SOD, and Hsp70 levels were always significantly lower in D3 compared to others, and D2 followed D3 treatment. Hsp 90 was significantly lower in D2 compared to others on day 7 and in D3 on days 14 and 21. All three enriched diets helped the fish overcome the harmful effect of UV-B radiation, and the D3 diet-fed fish showed the best performance.
The increased level of ultraviolet-B (UV-B) radiation is an environmental stressor. It is a potential threat to many aquatic organisms, viz., zooplankton, crustaceans, amphibians, fish, corals, etc. (Hader et al., 2011; Sucré et al., 2012). The penetration of UV-B in an aquatic ecosystem depends on two main factors: the depth of the water body and the levels of particulate and dissolved organic matter present in the water. UV-B can penetrate to significant depths in aquatic ecosystems as water itself absorbs a small amount of UV-B (Tedetti et al., 2007). In clean sea water, 10% of surface UV-R may penetrate up to 25 m depth, and in lakes with low dissolved organic carbon, the attenuation depth of UV may be more than 10 m (Fleischmann, 1989; Sayed et al., 2007; Behrendt et al., 2010). Freshwater fishes are vulnerable to UV-B exposure as they are cultured in clear water. UV radiation is harmful to organisms due to stress generation by reactive oxygen species (Carrasco-Malio et al., 2014). Reactive oxygen species (ROS) like O2, OH−, hydrogen peroxide (H2O2), peroxynitrite (ONOO−) and nitric oxide (NO-) are generated during UV-B light-stimulated photosensitization (Hakozaki et al., 2008; Terra et al., 2012). This causes oxidative damage in tissues and results in higher levels of oxidative stress, which degrades physiology, and the immune system, decreases disease resistance capacity, causes higher expression of heat shock proteins, and induces DNA damage (Lesser et al., 2001; Lesser and Barry, 2003; Jokinen et al., 2011; Terra et al., 2012; Carrasco-Malio et al., 2014). Proteins, nucleic acids, and lipids are the primary targets of UV-B radiation. Exposure to UV-B radiation increases the activities of enzymes that give protection against oxidative stress like superoxide dismutase, and it also reduces molecular defense mechanisms (Charron et al., 2000; Behrendt et al., 2010). Superoxide dismutase catalyzes the conversion of the anion superoxide into oxygen and molecular hydrogen peroxide. These enzymes function in cellular defense against UV-R induced oxidative stress and can also be used as biomarkers of oxidative stress (Sarkar, 2006).
In fish, UV-B irradiation affects both specific and non-specific branches of the immune system (Markkula et al., 2006; Markkula et al., 2009; Jokinen et al., 2011). In Atlantic cod, Salmo salar, UV radiation induced decreased hematocrit, plasma protein, and plasma immunoglobin levels (Salo et al., 2000). It affects the digestive enzyme activities and weakens the immune systems of catla larvae (Sharma et al., 2010). The reduced levels of myeloperoxidase, hemagglutination titer, and nitric oxide synthase, and enhanced levels of serum glutamic oxaloacetic transaminase and serum glutamate pyruvate transaminase are observed in UV-B exposed rohu Labeo rohita larvae (Singh et al., 2013a, b). Nitric oxide synthase is a family of enzymes that catalyze the production of the cellular signaling molecule nitric oxide. This nitric oxide plays a vital role in many biological processes. The inducible isoform iNOS produces a large amount of nitric oxide as a defense mechanism (Srivastava and Chakrabarti, 2012). Nitric oxide functions as an intracellular messenger, stimulating guanylate cyclase (Rochette et al., 2013).
All cellular components are susceptible to the action of reactive oxygen species, but biological membranes are the most severely affected (Podda et al., 1998; Eiberger et al., 2008; Perluigi et al., 2010; Terra et al., 2012). Fish tissue contains large quantities of polyunsaturated fatty acids (PUFAs) essential for membrane function (Martınez-Alvarez et al., 2005). Lipid peroxidation is the process of oxidative degradation of PUFA. Its occurrence in biological membranes causes impaired membrane function, structural integrity, and inactivation of several membrane-bound enzymes (Goel et al., 2005). Carbonyl protein is the most commonly measured end product of ROS-induced protein oxidation in biological samples. The products of lipid oxidation such as 4-hydroxy-2-nonenal and malonic dialdehyde could be involved in the formation of cross-linked proteins that are not degraded by proteases, and this might inhibit multicatalytic proteinase (Friguet et al., 1994; Lushchak et al., 2005). Stress proteins are being considered for their potential role as sensitive markers of cell injury, as they are related to the immune response and autoimmune diseases. Exposure to UV-B radiation damaged cellular components and caused oxidative stress, a potent trigger for Hsp gene activation. The chaperone function of Hsp70 appeared to be closely related to the stress tolerance capacity of the cells, and overexpression of Hsp70 enhanced anti-apoptotic activity against cellular stress (Li et al., 2000; Mosser et al., 2000a; Mosser et al., 2000b; Yamashita et al., 2010).
In a tropical country like India, aquatic organisms, especially those that remain at the surface of the water body, are exposed to the maximum amount of UV-B. A field study was conducted in Lake Naini, Delhi, where the highest intensity of UV-B (180.72–190.45 µW/cm2) has been recorded in June (Singh et al., 2015; Chakrabarti et al., 2019). The Indian major carp, Catla catla, is an economically important cultivable fish (ICAR, 2013). It is a surface-dwelling, zooplanktivorous fish and usually participates in its seeding migration moving towards the shallow water during monsoon (FAO, 2014). The fish is vulnerable to UV-B as it remains at the surface of the water body.
It is essential to determine the remedial measures that help fish overcome the harmful effects of UV-B irradiation. Oral administration of plant ingredients is one of the practical ways to improve the health status of fish. The application of natural herbal ingredients shows fewer (without) side effects on the organisms. There is mention of the use of ashwagandha, Withania somnifera (L.) Dunal (family: Solanaceae) and prickly chaff flower, Achyranthes aspera L. (family: Amaranthaceae) in Ayurveda as herbal medicines. The roots of ashwagandha are reputed to promote health and longevity by augmenting defense against disease, arresting the aging process, revitalizing the body in debilitated conditions, and increasing the capability of the individual to resist adverse environmental factors (Weiner and Weiner, 1994; Bhattacharya et al., 2001; Widodo et al., 2008). The fruits of amla (Emblica officinalis, Gaertn) (family: Euphorbiaceae) are extensively used to promote health and longevity by increasing the defense against disease and for health restorative purposes in most Asian countries (Bhattacharya et al., 1999; Bhattacharya et al., 2001). A. aspera is widely available in India. It is used for the treatment of numerous diseases such as inflammation, diabetes, hypertension, pneumonia, diarrhea, dysentery, asthma etc. (Baraik et al., 2014). The leaves and seeds of A. aspera show immunostimulatory and anti-stress effects in rohu Labeo rohita and common carp Cyprinus carpio (Chakrabarti and Srivastava, 2012; Chakrabarti et al., 2012; Singh et al., 2019; Sharma et al., 2021).
The aim of this study is to evaluate the UV-B protective properties of three traditional herbal ingredients, roots of ashwagandha (W. somnifera), fruits of amla (E. officinalis), and seeds of prickly chaff flower (A. aspera) in pre-exposed catla (C. catla). The fish are first exposed to UV-B and then the impacts of plant ingredients incorporated into diets on the physiology of fish are recorded.
C. catla was collected from a local fish farm. Fish were randomly distributed in fifteen aquaria (60 L each) and acclimated for 10 days. The stocking density was 4 fish/aquarium. Then, the fish (70.38 ± 1.18 g) were divided into five sets with three replicates each. Four sets of catla (twelve aquaria) were exposed to UV-B, and one set (three aquaria) remained unexposed. This unexposed fish served as control. Fish were exposed to UV-B radiation at an intensity of 80 µW/cm2 for 10 days. The exposure duration was 20 min/day and the fish received a cumulative dose of 960 mJ/cm2 of UV-B during the experimental period. The dose of UV-B was decided based on a field study conducted in Lake Naini, Delhi, India (Singh et al., 2015; Chakrabarti et al., 2019). All fish were fed with a control diet (40% protein) throughout the culture period.
The UV-B tubes were fixed at 20 cm above the aquaria. The source of UV-B (280–320 nm) was a Philips tube light (TL 20/12 RS, Holland). Aquaria were covered with black plastic sheets to avoid outside light and reflectance between the aquaria themselves. All tubes were pre-burned for 100 h to give a stable output. The spectral output of the tubes, as defined by the manufacturer, has a maximum emission at 313 nm, with negligible energy above 320 (Bertoni and Callieri, 1999). The UV-B tubes were coated with cellulose acetate, which absorbed light at <280 nm. Ultraviolet radiation (UV-B) penetrating into the water was measured using a Microtops II Sunphotometer (Solar Light Company, Philadelphia, USA). Both the treated and control sets were kept under fluorescent lamps (Philips 20 W) without UV components. The photoperiod was maintained as 12 h:12 h. The unexposed control set was covered with black plastic to avoid penetration of outside light.
After 10 days of UV-B exposure, exposed fish were fed with both enriched and control diets (Table 1). Three experimental diets were: diet 1 (D1) containing ashwagandha root powder (W. somnifera) (0.5%), diet 2 (D2) containing amla fruit powder (E. officinalis) (1.25%), and diet 3 (D3) containing prickly chaff flower seeds (A. aspera) (0.5%). Two sets of catla were fed with control diet, diet 4 (D4): one set of fish were exposed to UV-B (D4a), and the second set of fish were unexposed (D4b). Three replicates were used for each feeding regime. The dose of plant ingredients was selected based on the earlier study (Singh et al., 2013a; Singh et al., 2013b).
The water temperature, pH, and dissolved oxygen of the aquaria were monitored regularly using probes (HQ 40D, HACH, USA). For the culture of fish, dechlorinated tap water was used. Water temperature and dissolved oxygen levels were 28 ± 0.5°C and 5.5 ± 0.5 mg/l, respectively, pH ranged from 7.5 to 8.1 throughout the study period.
The culture of fish and their sampling were performed following the guidelines of the Institutional Animal Ethics Committee (DU/ZOOL/IAEC-R/2011/08).
The survival rate of fish was recorded. The weight of individual fish was measured on day 0 (start of feeding, first sampling), day 7 (second sampling), day 14 (third sampling), and day 21 (fourth sampling) of feeding with enriched diets.
Blood and tissue samples were collected on days 0, 7, 14, and 21 of feeding with enriched diets. Fish were anesthetized with 0.01% MS-222 (Sigma, USA). A blood sample was collected from the caudal vein of an individual fish using a 2 ml plastic syringe (NG: 24G × 1”, 0.56 × 25 mm), previously rinsed with 2.7% ethylenediaminetetraacetic acid (EDTA) solution. Samples were allowed to clot and were stored in a refrigerator at 4°C overnight. The clot was spun down at 400×g for 10 min. Then, the serum was stored in a sterile Eppendorf tube at −20°C until used for assays. The muscle and hepatopancreas were collected, pooled, and preserved at −80°C until further analyzed. The tissue was processed immediately for the assay of heat shock proteins 70 and 90.
The nitric oxide synthase (NOS) of the muscle was measured using the method of Lee et al. (2003). The tissue sample (100 mg) was homogenized in 1 ml of PBS (pH 7.4) and centrifuged at 10,000×g for 10 min at 4°C. Approximately 100 µl of supernatant was mixed with an equal volume of Griess reagent (1% sulfanilamide and 0.1% naphthylethylenediamine in 5% phosphoric acid) and incubated at room temperature for 10 min. The absorbance was recorded at 540 nm. The nitrite concentration was determined from the nitrite standard curve and was expressed as mol/mg tissue.
Tissue glutamate pyruvate transaminase (GPT) was determined in kinetic mode using diagnostic kits (Bayer Diagnostics, Baroda, India). Briefly, 100 µl reagent was added to either 10 µl of serum or tissue homogenate and mixed well. The initial absorbance was recorded immediately and four readings were taken at 1 min intervals against distilled water at 340 nm. GPT levels were expressed as IU/l.
Thiobarbituric acid reactive substances (TBARS) were measured following the method of Ohkawa et al. (1979). In this assay, malondialdehyde (MDA), the end product of lipid peroxidation, reacts with thiobarbituric acid (TBA). Muscle (1 g) was homogenized in 9 ml of KCl (1.15%). The sample was incubated in an acid medium containing 0.45% sodium dodecyl sulfate (SDS) and 0.6% TBA for 1 h at 100°C. After cooling, the sample was centrifuged at 800×g for 15 min at 4°C. The absorbance of the supernatant was measured at 532 nm. The standard was prepared using 1,1,3,3-tetramethoxy propane. The result was expressed as µmol MDA/mg protein.
Carbonyl protein (CP) was measured using the method of Lenz et al. (1989). Muscle (100 mg) was homogenized in 1 ml of potassium phosphate buffer (50 mM pH 7.0) containing 0.5 mM ethylenediaminetetraacetic acid (EDTA) and 100 µM of phenylmethylsulfonyl fluoride. A 250 µl aliquot of homogenate was mixed with 0.5 ml of 10% TCA and centrifuged at 13,000×g for 5 min. The pellet was collected for the assay and was mixed with 1 ml of dinitrophenyl hydrazine (10 mM DNPH) dissolved in 2 M HCl. The mixture was incubated at room temperature for 1 h. Then the mixture was centrifuged at 13,000×g for 5 min and the pellet was washed with 1 ml of ethanol-butylacetate (1:1, v/v). The process of washing of the pellet was repeated thrice. The pellet was dissolved in 1.5 ml of 6 M guanidine hydrochloride and centrifuged at 13,000×g for 5 min. The optical density of the collected supernatant was measured at 370 nm and expressed as µmoles of carbonyl protein/µg protein in the guanidine chloride solution. A blank was prepared using 1 ml of 2 M HCl instead of the DNPH solution. The amount of carbonyl protein was evaluated using a molar extinction coefficient of 22 × 103/M/cm.
The superoxide dismutase (SOD) of muscles was analyzed using the method of Kakkar et al. (1984). Hepatopancreas (200 mg) was homogenized in 1 ml chilled sodium phosphate buffer (0.1 M, pH 7.4). The homogenate was centrifuged at 10,000×g for 10 min and the resulting supernatant was centrifuged again at 12,500×g for 10 min at 4°C for the preparation of post mitochondrial supernatant (PMS). A reaction mixture was made with 1.2 ml (0.052 M) sodium pyrophosphate buffer, 0.2 ml post mitochondrial supernatant, 0.1 ml (186 mM) phenazine methosulfate, 0.3 ml (300 mM) nitroblue tetrazolium, and 1.0 ml of distilled water. To this, 0.2 ml of nicotinamide adenine dinucleotide (NADH, 780 mM) was added and incubated for 90 s. A 1 ml of glacial acetic acid was added to stop the reaction. The color intensity of the chromogen was measured at 560 nm. A control reaction was made in which the post-mitochondrial supernatant was replaced with sodium pyrophosphate buffer. SOD activity was expressed as units/min/µg of protein. One unit of enzyme was defined as the amount of enzyme inhibiting the rate of reaction by 50%.
Heat shock protein (Hsp 70) of the hepatopancreas was assayed using the Fish Heat Shock Protein 70 kit (ELISA, CUSABIO, Wuhan, China). The tissue (100 mg) was rinsed with 1× phosphate buffer saline (pH 7.4), homogenized in 1 ml PBS and stored overnight at −20°C. After two freeze-thawing cycles, the homogenate was centrifuged at 5,000×g for 5 min at 4°C. The supernatant was collected and stored at −80°C for assay. A sample (50 µl) was added to the ELISA plate (ninety six-well plate), which was pre-coated with antibody. Conjugate (50 µl) was added to each well except the blank and mixed well, and incubated at 37°C for 60 min. Each well was aspirated and washed with 200 µl of washing buffer three times. A 50 µl of HRP-avidin was added to each well, except the blank and mixed properly. It was incubated for 30 min at 37°C. The aspiration was repeated three times. Substrates A and B (each 50 µl) were added to each well and incubated for 15 min at 37°C. The stop solution (50 µl) was gently added to this and mixed properly. Absorbance was recorded at 450 nm using a microplate reader. The concentration of Hsp 70 was expressed in pg/ml.
The concentration of Hsp 90 was measured using an Hsp 90 ELISA kit (CUSABIO, Wuhan, China). All procedures were similar to the estimation of Hsp 70, except the type of antibody coated in the wells, conjugate, HRP-avidin, and constituents of substrates A and B. Absorbance was recorded at 450 nm. The concentration of Hsp 90 was expressed in pg/ml.
Total tissue protein was determined following the method of Lowry et al. (1951). The optical density of the sample was recorded at 750 nm. Distilled water was used as a blank.
Data were compiled as mean ± SE. All data were analyzed using one-way analysis of variance (ANOVA) with the help of SPSS 16.0 software (SPSS Inc., Chicago, IL), Duncan’s multiple range tests, DMR (Montgomery, 1984), and regression analysis. Statistical significance was accepted at the P <0.05 level.
All feeding regimes resulted in a 100% survival rate of the fish. There was no significant difference in the average weight of catla before UV-B exposure. After 10 days of exposure, the average weight of exposed fish was reduced by reduced 26.32% compared to the unexposed control fish. Fish were fed enriched diets from day 11 and the impact of diets was reflected in the growth of UV-B exposed catla. Among the exposed fish, a significantly higher average weight was found in the A. aspera seed-supplemented diet (D3) fed catla compared to the other diets fed fish throughout the culture period (Figure 1). On day 7, there was no significant difference in the average weight of fish in the D1, D2, and D4a treatments. On day 14, a significantly higher average weight was found in D1 diet-fed fish compared to D2 and D4a treatments. On this day, there was no significant difference between D2 and D4a treatments. On day 21, the average weight was minimal in D4a treatment. The highest average weight was always found in the unexposed and control diets (D4a) fed catla.
Figure 1 Average weight of four different diets fed UV-B exposed and unexposed Catla catla. Bars with different superscripts (a-e) are significantly different (n = 3).
A significantly lower nitric oxide synthase (NOS) level was observed in UV-B exposed and control diet (D4a) fed catla throughout the study period (Figure 2). On day 7 of feeding with enriched diets, the highest nitric oxide synthase level was found in D2 diet-fed fish compared to exposed fish fed with other diets. On day 7, there was no significant difference in nitric oxide synthase levels among enriched diets-fed exposed fish and control diets-fed unexposed fish. On day 14, nitric oxide synthase levels were 6.05–15.90% higher in D2 and D3 diets fed fish compared to the control diet-fed unexposed catla. On day 21, a significantly higher nitric oxide synthase level was found in the D3 diet-fed catla. A direct relationship was found between the NOS level and days of feeding in the D4a treatment.
Figure 2 Nitric oxide synthase level found in four different diets fed UV-B exposed and unexposed Catla catla. Bars with different superscripts (a-e) are significantly different (n = 3).
The glutamate pyruvate transaminase (GPT) level was significantly higher in the control diet-fed exposed fish (D4a) compared to other treatments on day 0 (Figure 3). Among the enriched diets-fed fish, it was always lowest in the D3 diet-fed fish.
Figure 3 Glutamate pyruvate transaminase level found in four different diets fed UV-B exposed and unexposed Catla catla. Bars with different superscripts (a-b) are significantly different (n = 3).
Significantly higher thiobarbituric acid reactive substance (TBARS) level was measured in exposed fish compared to the unexposed one on day 0 (Figure 4). Among the enriched diets-fed exposed fish, TBARS levels were significantly lower in the D3 treatment on days 7 and 14 and in D2 and D3 on day 21. In all these exposed fish, TBARS levels decreased with the progress of the culture period.
Figure 4 Thiobarbituric acid reactive substance level found in four different diets fed UV-B exposed and unexposed Catla catla. Bars with different superscripts (a-e) are significantly different (n = 3).
On day 0, the carbonyl protein level was significantly higher in exposed control diet-fed catla compared to the unexposed fish (Figure 5). Then fish were fed with enriched diets and the effect of the diet on the carbonyl protein level was recorded. The carbonyl protein level was significantly lower in the D3 diet-fed fish compared to others throughout the study period. The D2 diet-fed catla followed this D2 diet-fed one on days 7, 14, and 21 of feeding.
Figure 5 Carbonyl protein level found in four different diets fed UV-B exposed and unexposed Catla catla. Bars with different superscripts (a-e) are significantly different (n = 3).
On day 0, a 10-fold higher superoxide dismutase (SOD) level was observed in the hepatopancreas of catla in the D4a treatment compared to the fish in the D4b treatment (Figure 6). The positive impact of various feed ingredients was recorded on various sampling days. Significantly lower SOD activity was observed in D3 diet fed catla compared to others. D2 diet fed fish followed by D3 treatment. An inverse relationship was observed between the SOD levels and the days of culture.
Figure 6 Superoxide dismutase level found in four different diets fed UV-B exposed and unexposed Catla catla. Bars with different superscripts (a-e) are significantly different (n = 3).
Significantly higher heat shock proteins (Hsps) 70 and 90 were recorded in D4a treatment compared to D4b on day 0 (Figure 7). Then the fish were fed with enriched diets. A significantly lower Hsp 70 level was recorded in the hepatopancreas of D3 diet fed catla compared to others throughout the study period. On day 7, significantly lower Hsp 90 was recorded in the D2 diet fed catla compared to others (Figure 8). On days 14 and 21, significantly lower Hsp 90 was recorded in D3 diet fed catla compared to others.
Figure 7 Heat shock protein (Hsp) 70 level found in four different diets fed UV-B exposed and unexposed Catla catla. Bars with different superscripts (a-e) are significantly different (n = 3).
Figure 8 Heat shock protein (Hsp) 90 level found in four different diets fed UV-B exposed and unexposed Catla catla. Bars with different superscripts (a-e) are significantly different (n = 3).
In this study, catla were first exposed to UV-B radiation for 10 days, and then fish were fed with three indigenous plant ingredients like roots of ashwagandha, fruits of amla, and seeds of prickly chaff flower supplemented diets. There was no impact of UV-B exposure on the survival rate of catla, as all the fish survived. It affected the growth of fish. After 10 days of exposure, a 5% reduction in growth was found in exposed fish compared to the initial weight. On day 0, a higher average weight was found in the unexposed catla on day 0 compared to the initial weight of the fish. Among the exposed fish, a significantly higher average weight was found in D3 compared to other treatments throughout the study period. There was no significant difference in average weight among other diets fed exposed fish on day 7. A significant difference in average weight was recorded among four different diets fed exposed catla on day 21. Among exposed catla, the average weight was in the following order: D3 > D1 > D2 > D4a. Though ashwagandha and amla-supplemented diets-fed fish showed a slower growth rate compared to fish fed a seed-supplemented diet, the fish of these two former groups showed a 17.07–22.30% higher growth rate compared with the control diet-fed exposed fish on day 21. An earlier study also showed an increased growth rate of 0.5% in A. aspera seed-supplemented diet-fed rohu and common carp. The presence of ecdysterone and all essential and non-essential amino acids in the seeds of A. aspera influenced the growth of fish (Chakrabarti et al., 2012; Singh et al., 2013a; Singh et al., 2013b; Kumar et al., 2021).
Incorporation of plant ingredients improved the immune system as higher levels of nitric oxide synthase were found in enriched diets-fed exposed catla compared to the control diet fed exposed catla in the present study. On all three days of sampling, an even higher level of nitric oxide synthase was recorded in the D3 diet-fed exposed fish compared to the control diet-fed unexposed one. A similar result was found in 0.5% A. aspera seed-supplemented diet-fed rohu (Srivastava and Chakrabarti, 2012). The immunostimulating effect of seeds might be due to the presence of essential fatty acids like linolenic acid and oleic acid in the seeds (Chakrabarti et al., 2012). The presence of vitamin C in the seeds of A. aspera improved the health status of enriched diet-fed fish (Kumar et al., 2021). The antioxidant active compounds like emblicanin A, B, and tannins such as punigluconin and pedunculagin are present in amla (Bhattacharya et al., 1999; Bhattacharya et al., 2002). These compounds are known for their immunomodulating effects and gastroprotective properties (Sai Ram et al., 2002; Al-Rehaily et al., 2002). Amla also showed radiation protective ability (Scartezzini and Speroni, 2000). The presence of steroidal alkaloids and steroidal lactones, withanolides, withaferin A and withanolide D confirmed the anti-stress and antioxidant activity of ashwagandha in rat brain cortex (Bhattacharya et al., 1997a; Bhattacharya et al., 1997b; Matsuda et al., 2003; Widodo et al., 2008).
A higher level of GPT is an indicator of liver damage. The UV-B irradiated catla showed a higher level of GPT compared to the unexposed group. Significantly higher GPT was recorded in UV-B exposed catla larvae (Sharma et al., 2010). This was due to increased lipid peroxidation by the UV-B radiation, resulting in poor liver health conditions. Exposure of catla to UV-B radiation substantially increased the TBARS level compared to the unexposed control. The TBARS level also indicates lipid damage. Oxidation of lipid shows the free radical-induced damaged to aquatic organisms (Kubrak et al., 2011). UV-B induced oxidative damage of cell membranes both in vitro and in vivo was assessed by measuring the formation of lipid peroxidation products such as MDA or TBARS. The sea chub Girella laevifrons was exposed to 1, 4, and 5 h of UV-B radiation and lipid peroxidation was found to be maximal after 4 h of exposure (Carrasco-Malio et al., 2014). UV-B radiation increased the level of lipid peroxidation in freshwater prawn Macrobrachium olfersi (Nazari et al., 2010) and sea urchin Strongylocentrotus droebachiensis sperm (Lu and Wu, 2005). A. aspera seed-supplemented diet (D3) provided the highest protection to UV-B irradiated catla as lowest TBARS levels were found in all three days of sampling. The other two diets, D1 and D2, enriched with ashwagandha and amla, also showed reduced TBARS levels in the UV-B exposed fish compared to the D4a treatment. In this study, flavonoids, triterpenoids, polyphenolic compounds, and steroids were reported in the methanolic extract of aerial parts of A. aspera (Tahiliani and Kar, 2000; Varuna et al., 2010) and were related to the antioxidant activity in UV-B exposed catla. The tannoids, the active compounds in amla, gave protection to the fish against lipid peroxidation (Bhattacharya et al., 1999; Bhattacharya et al., 2001). The amla-supplemented diets helped to scavenge the oxidative stress induced by this harmful radiation. A. aspera seeds are a rich source of vitamin C: 166.40 ± 3.41 mg/100 g, dry weight (Kumar et al., 2021).
The reduced carbonyl protein levels were observed in plant ingredient-incorporated diets-fed catla compared to control diet-fed exposed catla. In this study, significantly lower carbonyl protein levels were found in D3 diet-fed exposed fish compared to the control diet-fed unexposed one. This showed the role of plant ingredients as natural antioxidant defenses against environmental stress like UV-B. The enhanced SOD level in the exposed fish compared to the unexposed one indicated the amount of superoxide radicals and other forms of ROS present in the system (Terra et al., 2012). UV radiation caused higher superoxide dismutase activity in Atlantic cod Gadus morhua larvae (Lesser et al., 2001). The exposure of embryos of sea urchins to UV-B radiation resulted in significantly higher concentrations of superoxide dismutase activity (Lesser and Barry, 2003). In sea chub, SOD levels were significantly higher in the 2 h exposed treatment compared to the 3, 4, and 5 h exposed treatments (Carrasco-Malio et al., 2014). The SOD level decreased in catla fed with plant ingredient-supplemented diets on day 7, and the level was further reduced on days 14 and 21. The enhanced antioxidant activity of the D3 diet was due to the presence of high phytoactive constituents, protein, and vitamin C in the seeds of A. aspera (Khan et al., 2010; Manjunatha et al., 2012; Kumar et al., 2021). Ashwagandha contained sitoindosides VII–X and withaferin-A and showed a significant antioxidant effect in rats (Bhattacharya et al., 1997a).
In this study, UV-B irradiated catla showed 2.50-fold and 6.97-fold higher Hsp70 and Hsp90 levels, respectively, compared to the control fish on day 0. A similar result was also observed in UV-B irradiated pike Esox lucius embryos whose level of Hsp/Hsc 70 was found higher after 3 h of exposure (Vehniainen et al., 2012). Higher concentrations of stress proteins could be toxic and therefore it can alter or interfere with the normal cellular functions, notably cell growth and development (Krebs and Feder, 1997). This resulted in the poor growth of catla exposed to UV-B. Many studies also reported that increased level of heat shock protein 70 (Hsp 70) could be associated with reduced individual fitness (Feder et al., 1997; Silbermann and Tatar, 2000; Sorensen et al., 2003; Tine et al., 2010). This was confirmed in this study, as a higher level of Hsp 70 was found in control diet-fed larvae. Feeding of three plant ingredient-supplemented diets reduced the level of Hsp 70 and 90 expressions in catla fish and showed anti-stress and bioprotective properties of the diets against harmful UV-B radiation.
Dietary supplementation of seeds of A. aspera (0.5%), root powder of W. somnifera (0.5%), and fruit powder of E. officinalis (1.25%) showed promising results in overcoming the harmful effect of UV-B radiation in pre-exposed C. catla. The enriched diets helped the fish in their recovery process. The seed-supplemented diet showed significantly higher UV-B protective properties compared to the other two plant-ingredient-enriched diets in fish.
The original contributions presented in the study are included in the article/supplementary material. Further inquiries can be directed to the corresponding author.
The animal study was reviewed and approved by the Institutional Animal Ethics Committee.
RC and JS designed the study. MS, RC, and JS cultured the fish and analyzed samples. JS, RC, and MS performed statistical analysis and wrote the manuscript. JS, RC, and MS prepared the graphs and table. All authors listed have made a substantial, direct, and intellectual contribution to the work and approved it for publication.
Authors are thankful to the Indian Council of Agricultural Research, ICAR, New Delhi for the financial support in the form of the NFBSFARA Project, AS-2001, dated 1.1. 2011.
The authors declare that the research was conducted in the absence of any commercial or financial relationships that could be construed as a potential conflict of interest.
All claims expressed in this article are solely those of the authors and do not necessarily represent those of their affiliated organizations, or those of the publisher, the editors and the reviewers. Any product that may be evaluated in this article, or claim that may be made by its manufacturer, is not guaranteed or endorsed by the publisher.
Al-Rehaily A. J., Al-Howiriny T. A., Al-Sohaibani M. O., Rafatullah S. (2002). Gastroprotective Effects of “Amla” Emblica officinalis on In Vivo Test Models in Rats. Phytomedicine 9, 515–522. doi: 10.1078/09447110260573146
Baraik B., Jain P., Sharma H. P. (2014). Achyranthes Aspera L.: As a Source of Bio-Fungicide. Am. J. Adv. Drug Deliv. 2, 686–696.
Behrendt L., Jönsson M. E., Goldstonea J. V., Stegeman J. J. (2010). Induction of Cytochrome P450 1 Genes and Stress Response Genes in Developing Zebrafish Exposed to Ultraviolet Radiation. Aquat. Toxicol. 98, 74–82. doi: 10.1016/j.aquatox.2010.01.008
Bertoni R., Callieri C. (1999). Effects of UVB Radiation on Freshwater Autotrophic and Heterotrophic Picoplankton in a Subalpine Lake. J. Plankton Res. 21, 1373–1388. doi: 10.1093/plankt/21.7.1373
Bhattacharya S. K., Bhattacharya D., Sairam K., Ghosal S. (2002). Effect of Bioactive Tannoid Principles of Emblica officinalis on Ischemia-Reperfusion Induced Oxidative Stress in Rat Heart. Phytomedicine 9, 171–174. doi: 10.1078/0944-7113-00090
Bhattacharya A., Ghosal S., Bhattacharya S. K. (1999). Antioxidant Activity of Active Tannoid Principles of Emblica officinalis (Amla). Indian J. Exp. Biol. 37, 676.
Bhattacharya A., Ghosal S., Bhattacharya S. K. (2001). Anti-Oxidant Effect of Withania somnifera Glycowithanolides in Chronic Footshock Stress-Induced Perturbations of Oxidative Free Radical Scavenging Enzymes and Lipid Peroxidation in Rat Frontal Cortex and Striatum. J. Ethnopharmacol. 74, 1–6. doi: 10.1016/S0378-8741(00)00309-3
Bhattacharya S. K., Satyan K. S., Chakrabarti A. (1997b). Effect of Trasina, an Ayurvedic Herbal Formulation, on Pancreatic, Islet Superoxide Dismutase Activity in Hyperglycaemic Rats. Indian J. Exp. Biol. 35, 297–299.
Bhattacharya S. K., Satyan K. S., Ghosal S. (1997a). Antioxidant Activity of Glycowithanolides From Withania somnifera. Indian J. Exp. Biol. 35, 236–239.
Carrasco-Malio A., Díaz M., Mella M., Montoya M. J., Miranda A., Landaeta M. F., et al. (2014). Are the Intertidal Fish Highly Resistant to UV-B Radiation? A Study Based on Oxidative Stress in Girella laevifrons (Kyphosidae). Ecotox Environ. Saf. 100, 93–98. doi: 10.1016/j.ecoenv.2013.07.030
Chakrabarti R., Srivastava P. K. (2012). Effect of Dietary Supplementation of Achyranthes aspera Seed on Labeo rohita Larvae Challenged with Aeromonas hydrophila. J. Aqua. Anim. Health 24, 213–218. doi: 10.1080/08997659.2012.694834
Chakrabarti R., Singh M. K., Sharma J. G., Mittal P. (2019). Dietary Supplementation of Vitamin C: An Effective Measure to Give Protection Against UV-B Irradiation Using Fish as Model Organism. Photochem. Photobiol. Sci. 18, 224–231. doi: 10.1039/C8PP00481A
Chakrabarti R., Srivastava P. K., Kundu K., Khare R. S., Banerjee S. (2012). Evaluation of Immunostimulatory and Growth Promoting Effect of Seed Fractions of Achyranthes aspera in Common Carp Cyprinus carpio and Identification of Active Constituents. Fish Shellfish Immun. 32, 839–843. doi: 10.1016/j.fsi.2012.02.006
Charron R. A., Fenwich J. C., Lean D. R. S., Moon T. W. (2000). Ultraviolet-B Radiation Effects on Antioxidant Status and Survival in the Zebra Fish Brachydanio rerio. Photochem. Photobiol. 72, 327–333. doi: 10.1562/0031-8655(2000)0720327UBREOA2.0.CO2
Eiberger W., Volkmer B., Amouroux R., Dherin C., Radicellac J. P., Epe B. (2008). Oxidative Stress Impairs the Repair of Oxidative DNA Base Modifications in Human Skin Fibroblasts and Melanoma Cells. DNA Repair 7, 912–921. doi: 10.1016/j.dnarep.2008.03.002
FAO. (2014). The State of the World Fisheries and Aquaculture Opportunities and Challenges. Rome, Italy: Food and Agriculture Organization of the United Nations (FAO). Opportunities and Challenges. Available at: https://www.fao.org/3/i3720e/i3720e.pdf.
Feder M. E., Blair N., Figueras H. (1997). Natural Thermal Stress and Heat-Shock Protein Expression in Drosophila Larvae and Pupae. Funct. Ecol. 11, 90–100. doi: 10.1046/j.1365-2435.1997.00060.x
Fleischmann E. M. (1989). The Measurement and Penetration of Ultraviolet Radiation Into Tropical Marine Water. Limonol. Oceanogr. 34, 1623–1629. doi: 10.4319/lo.1989.34.8.1623
Friguet B., Szweda L. I., Stadtman E. R. (1994). Susceptibility of Glucose-6-Phosphate Dehydrogenase Modified by 4-Hydroxy-2-Nonenal and Metal-Catalyzed Oxidation to Proteolysis by the Multicatalytic Protease. Arch. Biochem. Biophys. 311, 168–173. doi: 10.1006/abbi.1994.1222
Goel A., Dani V., Dhawan D. K. (2005). Protective Effects of Zinc on Lipid Peroxidation, Antioxidant Enzymes and Hepatic Histoarchitecture in Chlorpyrifos-Induced Toxicity. Chem-Biol. Interact. 156, 13–141. doi: 10.1016/j.cbi.2005.08.004
Hader D. P., Elbling E. W., Williamson C. E., Worrest R. C. (2011). Effects of UV Radiation on Aquatic Ecosystems and Interactions With Climate Change. Photochem. Photobiol. Sci. 10, 242–260. doi: 10.1039/C0PP90036B
Hakozaki T., Akira D., Yoshii T., Toyokuni S., Yasui H., Sakurai H. (2008). Visualization and Characterization of UVB-Induced Reactive Oxygen Species in a Human Skin Equivalent Model. Arch. Dermatol. Res. 300, 51–56. doi: 10.1007/s00403-007-0804-3
ICAR. (2013). “Handbook of Fisheries and Aquaculture”, 3rd Edn (New Delhi, India: Directorate of Knowledge Management in Agriculture, Indian Council of Agricultural Research (ICAR).
Jokinen I. L., Salo H. M., Markkula E., Rikalainen K., Arts M. T., Browman H. I. (2011). Additive Effects of Enhanced Ambient Ultraviolet B Radiation and Increased Temperature on Immune Function, Growth and Physiological Condition of Juvenile (Parr) Atlantic Salmon, Salmo salar. Fish Shellfish Immun. 30, 102–108. doi: 10.1016/j.fsi.2010.09.017
Kakkar P., Das B., Viswanathan P. N. (1984). A Modified Spectrophotometric Assay of Superoxide Dismutase. J. Biochem. Biophys. 21, 130–131.
Khan M. T. J., Ahmad K., Alvi M. N., Noor-ul- A., Mansoor B., Saeed M. A., et al. (2010). Antibacterial and Irritant Activities of Organic Solvent Extracts of Agave americana Linn. Albizzia lebek Benth. Achyranthes aspera Linn. And Abutilon indicum Linn. A Preliminary Investigation. Pak. J. Zool. 42, 93–97.
Krebs R. A., Feder M. E. (1997). Deleterious Consequences of Hsp70 Overexpression in Drosophila melanogaster Larvae. Cell Stress Chaperone. 2, 60–71. doi: 10.1379/1466-1268(1997)002<0060:DCOHOI>2.3.CO;2
Kubrak O. I., Husak V. V., Rovenko B. M., Storey J. M., Storey K. B., Lushchak V. I. (2011). Cobalt-Induced Oxidative Stress in Brain, Liver and Kidney of Goldfish Carassius auratus. Chemosphere 85, 983–989. doi: 10.1016/j.chemosphere.2011.06.078
Kumar N., Sharma J. G., Kumar G., Shrivastav A. K., Tiwari N., Begum A., et al. (2021). Evaluation of Nutritional Value of Prickly Chaff Flower Achyranthes aspera (L.) as Fish Feed Ingredient. Indian J. Anim. Sci. 91, 239–244.
Lee D. U., Kang Y. J., Park M. K. (2003). Effects of 13-Alkyl-Substituted Berberine Alkaloids on the Expression of COX-II, TNF-α, iNOS and IL-12 Production in LPS Stimulated Macrophages. Life Sci. 73, 1401–1412. doi: 10.1016/S0024-3205(03)00435-1
Lenz A.-G., Costabel U., Shaltiel S., Levine R. L. (1989). Determination of Carbonyl Groups in Oxidatively Modified Proteins by Reduction With Tritiated Sodium Borohydride. Anal. Biochem. 177, 419-25. doi: 10.1016/0003-2697(89)9007FAO2014
Lesser M. P., Barry T. M. (2003). Survivorship, Development, and DNA Damage in Echinoderm Embryos and Larvae Exposed to Ultraviolet Radiation (290-400 Nm). J. Exp. Mar. Biol. Ecol. 292, 75–91. doi: 10.1016/S0022-0981(03)00141-2
Lesser M. P., Farrel J. H., Walker C. W. (2001). Oxidative Stress and P53 Expression in the Larvae of Atlantic Cod (Gadus morhua) Exposed to Ultraviolet (290-400 Nm). J. Exp. Biol. 204, 157–164. doi: 10.1242/jeb.204.1.157
Li C. Y., Lee J. S., Ko Y. G., Kim J. I., Seo J. S. (2000). Suppression of Heat Shock Protein-70 by Ceramide in Heat Shock-Induced HL-60 Cell Apoptosis. J. Biol. Chem. 275, 8872–8879. doi: 10.1074/jbc.275.12.8872
Lowry O. H., Rosebrough N. J., Farr F. R. (1951). Protein Measurement With the Folin Phenol Reagent. J. Biol. Chem. 193, 265–275. doi: 10.1016/S0021-9258(19)52451-6
Lushchak I. L., Bagnyukova V. T., Victor V., Husak V., Luzhna L. I., Lushchak O. V., et al. (2005). Hyperoxia Results in Transient Oxidative Stress and an Adaptive Response by Antioxidant Enzymes in Goldfish Tissues. Int. J. Biochem. Cell Biol. 37, 1670–1680. doi: 10.1016/j.biocel.2005.02.024
Lu X. Y., Wu R. S. S. (2005). UV Induces Reactive Oxygen Species, Damages Sperm, and Impairs Fertilisation in the Sea Urchin Anthocidaris crassispina. Mar. Biol. 148, 51–57. doi: 10.1007/2Fs00227-005-0049-7
Manjunatha B. K., Abhilash N., Hegde V., Suchitra M. N., Vidya S. M. (2012). Hepatoprotective Potency of Achyranthes aspera: An In Vivo Study. Int. J. Pharm. Phytopharmacol. Res. 1, 387–390.
Markkula S. E., Salo H. M., Rikalainen A. K., Jokinen E. I. (2006). Different Sensitivity of Carp (Cyprinus carpio) and Rainbow Trout (Oncorhyncus mykiss) to the Immunomodulatory Effects of UV-B Irradiation. Fish Shellfish Immun. 21, 70–79. doi: 10.1016/j.fsi.2005.10.007
Markkula E. S., Salo H. M., Rikalainen A. K., Jokinen E. I. (2009). Long-Term UVB Irradiation Affects the Immune Functions of Carp (Cyprinus carpio) and Rainbow Trout (Oncorhynchus mykiss). Photochem. Photobiol. 85, 347–352. doi: 10.1111/j.1751-1097.2008.00446.x
Martınez-Alvarez R. M., Morales A. E., Sanz A. (2005). Antioxidant Defenses in Fish: Biotic and Abiotic Factors. Rev. Fish. Biol. Fisher. 15, 75–88. doi: 10.1007/s11160-005-7846-4
Matsuda H., Murakami T., Kishi A., Yoshikawa M. (2003). Structures of Withanosides I, II, III, IV, V, VI, and VII, New Withanolide Glycosides, From the Roots of Indian Withania somnifera DUNAL. And Inhibitory Activity for Tachyphylaxis to Clonidine in Isolated Guinea-Pig Ileum. Bioorg. Med. Chem. 9, 1499–1507. doi: 10.1016/S0968-0896(01)00024-4
Mosser D. D., Caron A. W., Bourget L., Denis-Larose C., Massie B. (2000b). The Chaperone Function of Hsp70 is Required for Protection Against Stress-Induced Apoptosis. Mol. Cell Biol. 20, 7146–7159. doi: 10.1128/MCB.20.19.7146-7159.2000
Mosser D. D., Caron A. W., Bourget L., Meriin A. B., Sherman M. Y., Morimoto R. I., et al. (2000a). Heat Shock Protein 70 Inhibits Apoptosis Downstream of Cytochrome C Release and Upstream of Caspase-3 Activation. J. Biol. Chem. 275, 25665–25671. doi: 10.1074/jbc.M906383199
Nazari E. M., Ammar D., de Bem A. F., Litini A., Muller Y. M. R., Allodi S. (2010). Effects of Environmental and Artificial UV-B on Freshwater Prawn, Macrobrachium olfersi Embryos. Aquat. Toxicol. 98, 25–33. doi: 10.1016/j.aquatox.2010.01.010
Ohkawa H., Ohishi N., Yagi K. (1979). Assay for Lipid Peroxides in Animal Tissues by Thiobarbituric Acid Reaction. Anal. Biochem. 95, 351–358. doi: 10.1016/0003-2697(79)90738-3
Perluigi M., Domenico F. D., Blarzino C., Foppoli C., Cini C., Giorgi A., et al. (2010). Effects of UV-B Induced Oxidative Stress on Protein Expression and Specific Protein Oxidation in Normal Human Epithelial Keratinocytes: A Proteomic Approach. Proteome Sci. 18, 8–13. doi: 10.1186/1477-5956-8-13
Podda M., Traber M. G., Weber C., Yan L. J., Packer L. (1998). UV-Irradiation Depletes Antioxidants and Causes Oxidative Damage in a Model of Human Skin. Free Radical. Biol. Med. 24, 55–65. doi: 10.1016/S0891-5849(97)00142-1
Rochette L., Lorin J., Zeller M., Guilland J. C., Lorgis L., Cottin Y., et al. (2013). Nitric Oxide Synthase Inhibition and Oxidative Stress in Cardiovascular Diseases: Possible Therapeutic Targets? Pharmacol. Ther. 140, 239–257. doi: 10.1016/j.pharmthera.2013.07.004
Sai Ram M., Neetu D., Yogesh B., Anju B., Dipti P., Pauline T., et al. (2002). Cyto-Protective and Immunomodulating Properties of Amla (Emblica officinalis) on Lymphocytes: An In Vitro Study. J. Ethnopharmacol. 81, 5–10. doi: 10.1016/S0378-8741(01)00421-4
Salo H. M., Jokinen E., Markuula S. E., Aaltonen T. M., Penttila H. T. (2000). Comparative Effects of UVA and UVB Irradiation on the Immune System of Fish. J. Photochem. Photobiol. B. 56, 154–162. doi: 10.1016/S1011-1344(00)00072-5
Sarkar A. (2006). Biomarkers of Marine Pollution and Bioremediation. Ecotoxicology 15, 331–332. doi: 10.1007/s10646-006-0073-5
Sayed A. H., Ibrahim A. T., Mekkawy I. A. A., Mahmoud U. M. (2007). Acute Effects of Ultraviolet-A on Some Biochemical and Histological Parameters of African Catfish C. gariepinus (Burchel). Photochem. Photobiol. 89, 170–174. doi: 10.1016/j.jphotobiol.2007.09.010
Scartezzini P., Speroni E. (2000). Review on Some Plants of Indian Traditional Medicine With Antioxidant Activity. J. Ethnopharmacol. 71, 23–43. doi: 10.1016/S0378-8741(00)00213-0
Sharma J. G., Rao Y. V., Kumar S., Chakrabarti R. (2010). Impact of UV-B Radiation on the Digestive Enzymes and Immune System of Larvae of Indian Major Carp Catla catla. Int. J. Radiat. Biol. 3, 181–186. doi: 10.3109/09553000903419312
Sharma J. G., Singh A., Begum A., Har Krishna V., Chakrabarti R. (2021). The Impact of Achyranthes aspera Seeds and Leaves Supplemented Feeds on the Survival, Growth, Immune System and Specific Genes Involved in Immunostimulation in Clarias batrachus Fry Challenged With Aeromonas hydrophila in Pond Conditions. Fish Shellfish Immunol. 118, 11–18. doi: 10.1016/j.fsi.2021.08.026
Silbermann R., Tatar M. (2000). Reproductive Costs of Heat Shock Protein in Transgenic Drosophila melanogaster. Evol. Ecol. 54, 2038–2045. doi: 10.1111/j.0014-3820.2000.tb01247.x
Singh K. M., Sharma J. G., Chakrabarti R. (2013a). Effect of UV-B Radiation on the Defense System of Labeo rohita (Cyprinidae) Larvae and its Modulation by Seed of Achyranthes aspera. Acta Ichthyol. Piscat. 43, 119–126. doi: 10.3750/AIP2013.43.2.04
Singh K. M., Sharma J. G., Chakrabarti R. (2013b). Impact of UV-B Radiation on the Physiology of Freshwater Carp Labeo rohita Larvae and Evaluation of UV-B Protective Properties of Seeds of Achyranthes aspera and Vitamin C. Agric. Res. 2, 166–171. doi: 10.1007/s40003-013-0060-z
Singh K. M., Sharma J. G., Chakrabarti R. (2015). Simulation Study of Natural UV-B Radiation on Catla catla and its Impact on Physiology, Oxidative Stress, Hsp70 and DNA Fragmentation. Photochem. Photobiol. B. Biol. 149, 156–163. doi: 10.1016/j.jphotobiol.2015.05.019
Singh A., Sharma J. G., Paichha M., Chakrabarti R. (2019). Achyranthes aspera (Prickly Chaff Flower) Leaves and Seeds Supplemented Diets Regulate the Growth, Innate Immunity and Oxidative Stress in Aeromonas hydrophila Challenged Labeo rohita. J. Appl. Aquac. 32, 250–267. doi: 10.1080/10454438.2019.1615594
Sorensen J. G., Kristensen T. N., Loeschcke V. (2003). The Evolutionary and Ecological Role of Heat Shock Proteins. Ecol. Lett. 6, 1025–1037. doi: 10.1046/j.1461-0248.2003.00528.x
Srivastava P. K., Chakrabarti R. (2012). Effect of Dietary Supplementation of Seed of Achyranthes aspera on the Immune System of Labeo rohita Fry. Isr. J. Aquacult. Bamid. 64.2012, 786. doi: 10.46989/001c.20615
Sucré E., Vidussi F., Mostajir B., Charmantier G., Lorin-Nebel C. (2012). Impact of Ultraviolet-B Radiation on Planktonic Fish Larvae: Alteration of the Osmoregulatory Function. Aquat. Toxicol. 109, 194–201. doi: 10.1016/j.aquatox.2011.09.020
Tahiliani P., Kar A. (2000). Achyranthes aspera Elevates Thyroid Hormone Levels and Decreases Hepatic Lipid Peroxidation in Male Rats. J. Ethnopharmacol. 71, 527–532. doi: 10.1016/S0378-8741(00)00170-7
Tedetti M., Sempere R., Vasilkov A., Charriere B., Nerini D., Miller W. L., et al. (2007). High Penetration of Ultraviolet Radiation in the South East Pacific Water. Geophys. Res. Lett. 34, L12610. doi: 10.1029/2007GL029823
Terra V. A., Souza-Neto F. P., Pereira R. C., Silva T. N. X., Costa A. C., Luiz R. C., et al. (2012). Time-Dependent Reactive Species Formation and Oxidative Stress Damage in the Skin After UV-B Irradiation. J. Photochem. Photobiol. B. 109, 34–41. doi: 10.1016/j.jphotobiol.2012.01.003
Tine M., Bonhomme F., Mckenzie D. J., Durand J. D. (2010). Differential Expression of the Heat Shock Protein Hsp70 in Natural Populations of the Tilapia, Sarotherodon melanotheron, Acclimatised to a Range of Environmental Salinities. BMC Ecol. 10, 1–8. doi: 10.1186/1472-6785-10-11
Varuna K. M., Khan M. U., Sharma P. K. (2010). Review on Achyranthes aspera. J. Pharm. Res. 3, 714–717.
Vehniainen E. R., Vahakangas K., Oikari A. O. J. (2012). UV-B Exposure Causes DNA Damages and Changes in Protein Expression in Northern Pike (Esox lucius) Posthatched Embryo. Photochem. Photobiol. 88, 363–370. doi: 10.1111/j.1751-1097.2011.01058.x
Weiner M. A., Weiner J. (1994). “Ashwagandha (India Ginseng).” in Herbs That Heal (Mill Valley, CA: Quantum Books).
Widodo N., Takagi Y., Shrestha B. G., Ishii T., Kaul S. C., Wadhwa R. (2008). Selective Killing of Cancer Cells by Leaf Extract of Ashwagandha: Components, Activity and Pathway Analyses. Cancer Lett. 262, 37–47. doi: 10.1016/j.canlet.2007.11.037
Keywords: Achyranthes aspera, Catla catla, carbonyl protein, Emblica officinalis, heat shock proteins 70 and 90, nitric oxide synthase, ultraviolet B, Withania somnifera
Citation: Sharma J, Singh MK and Chakrabarti R (2022) Evaluation of UV-B Ameliorating Properties of Indigenous Plants Ashwagandha Withania somnifera (Dunal), Amla Emblica officinalis (Gaertn), and Prickly Chaff Flower Achyranthes aspera (L.) Supplemented Diets in Prior UV-B Exposed Catla catla. Front. Mar. Sci. 9:905147. doi: 10.3389/fmars.2022.905147
Received: 26 March 2022; Accepted: 18 April 2022;
Published: 19 May 2022.
Edited by:
Arabinda Mahanty, National Rice Research Institute (ICAR), IndiaReviewed by:
Prem Kumar Indraganti, Institute of Nuclear Medicine & Allied Sciences (DRDO), IndiaCopyright © 2022 Sharma, Singh and Chakrabarti. This is an open-access article distributed under the terms of the Creative Commons Attribution License (CC BY). The use, distribution or reproduction in other forums is permitted, provided the original author(s) and the copyright owner(s) are credited and that the original publication in this journal is cited, in accordance with accepted academic practice. No use, distribution or reproduction is permitted which does not comply with these terms.
*Correspondence: Rina Chakrabarti, YXF1YXJlc2VhcmNobGFiQHlhaG9vLmNvLmlu
Disclaimer: All claims expressed in this article are solely those of the authors and do not necessarily represent those of their affiliated organizations, or those of the publisher, the editors and the reviewers. Any product that may be evaluated in this article or claim that may be made by its manufacturer is not guaranteed or endorsed by the publisher.
Research integrity at Frontiers
Learn more about the work of our research integrity team to safeguard the quality of each article we publish.