- 1Institute of Geology and Mineralogy, University of Cologne, Cologne, Germany
- 2Institute of Earth Sciences, Heidelberg University, Heidelberg, Germany
- 3School of Arts, Sciences and Humanities, University of São Paulo, São Paulo, Brazil
- 4Departamento de Geoquímica, University Federal Fluminense, Niterói, Brazil
This study presents new quantitative data on benthic foraminifera from three bathymetric transects of the Brazil (11-14°S, 420-1900 m) and Campos (22°S, 430-2000 m) basins. The quantity and quality of organic matter flux as well as substrate properties and hydrodynamic conditions at the sediment-water interface are identified as key parameters controlling assemblage distribution. Based on the total (stained and unstained) fauna, a distinct biogeographic divide between a Globocassidulina subglobosa/crassa assemblage in the Campos Basin and a rosalinid/bolivinid assemblage in the Brazil Basin occurs across the bifurcation of the South Atlantic Central Water into its southward subtropical and northward tropical branches. In the Campos Basin, coarser sediments, increased bottom current activity, and variable nutrient supply favor an assemblage of Globocassidulina subglobosa/crassa, Nuttalides umbonifer and Alabaminella weddellensis. Occurrences of cold-water coral mounds in 870 m provide an ecological niche favoring species such as Alabaminella weddellensis which benefit from trapped nutrients. The Brazil Basin is characterized by increased abundances of Rosalina and Bolivina, while Globocassidulina subglobosa/crassa is comparatively less frequent. Assemblages with G. subglobosa/crassa, Rosalina spp., Bolivina variabilis and Bolivina subreticulata are favored by a relatively high nutrient input at 14°S. Further north, assemblages with Bolivina subreticulata, Bolivina variabilis, Epistominella exigua, G. subglobosa/crassa are located beneath the velocity core of the North Brazil Undercurrent (NBUC), coinciding with more clayey sediments rich in TOC. Occurrences of delicate branching forms such as Saccorhiza ramosa indicate a more stable setting, distal to the main current. Rose Bengal stained (living) specimens are scarce in all three regions, as is typical for deep-sea foraminiferal faunas. Their patterns of species distribution largely reflect those observed for the total fauna.
1 Introduction
Benthic deep-sea foraminifera are a diverse group of shell-bearing unicellular eukaryotes adapted to a wide range of marine microhabitats (e.g., Sen Gupta, 2002). Their distribution is largely determined by the interplay of organic carbon influx, physical and chemical properties of major water masses, and the hydrodynamic regime at the sediment-water interface (Mackensen et al., 1995; Schmiedl et al., 1997; Gooday and Jorissen, 2012). At the extensive Brazilian continental margin, the impact of such environmental factors on the benthic foraminiferal fauna is poorly understood as previous studies have mainly focused on shelf areas south of 20°S (Oliveira-Silva et al., 2005; de Mello e Sousa et al., 2006; Eichler et al., 2008; Burone et al., 2011; Eichler et al., 2012; Vieira et al., 2015; Yamashita et al., 2018; Yamashita et al., 2020). Studies on benthic foraminiferal assemblages on the continental slope are particularly rare and limited to the Campos Basin (~ 22°S; de Mello e Sousa et al., 2006; Yamashita et al., 2018; de Almeida et al., 2022). Analyses of modern deep-sea foraminifera from the continental shelf and slope north of 20°S off Eastern Brazil are lacking (Murray, 2006). Environmental conditions vary greatly along the Brazilian continental slope due to a complex water mass system and varied local seafloor topographies. Diverse microhabitats occupied by a wide range of different foraminiferal associations are thus to be expected to the North of the Campos Basin. Filling this gap in biogeographic documentation is essential to fully understand the controls on foraminiferal distribution off Brazil.
During R/V METEOR expedition M125, the Brazilian continental shelf and slope were sampled between 10°S and 23°S (Bahr et al., 2016), offering a unique opportunity to document benthic foraminiferal distribution patterns north of 20°S. Here we investigate recent benthic foraminiferal assemblages from three bathymetric transects in the Brazil (11°S, 14°S) and Campos (22°S) basins. Through integration of the observed faunal patterns with environmental parameters we gain new insights into the drivers of diversity and biogeographic patterns of deep-sea benthic foraminifera along the Brazilian continental margin. This study will serve as a baseline for future biogeographic and palaeoceanographic investigations on benthic foraminiferal faunas in the area.
2 Study Area
The Brazilian margin, with a length of 7400 km, can be divided into different physiographical provinces (Martins and Coutinho, 1981). The north-eastern part stretches from Cabo Sao Roque to Belmonte and includes the Brazil Basin (5-16°S; Figure 1; Martins and Coutinho, 1981; da Silveira et al., 2020). Reduced continental erosion and low marine sedimentation rates result in a very narrow continental shelf (Figures 2A, B) less than 10 km in width (Summerhayes et al., 1976; Knoppers et al., 1999; Bahr et al., 2016). Extensive canyon systems are common and determine seafloor topography along the continental slope (Bahr et al., 2016). Further south (16-23°S), the east coast extends to Cabo Frio including the Campos Basin. The shelf area is considerably wider compared to the north and may reach a width of up to 90 km (Figure 2C; Martins and Coutinho, 1981; Knoppers et al., 1999). The upper slope is characterized by bottom current-induced and contour-parallel erosional ridges (Viana et al., 2002; Bahr et al., 2016). Contouritic deposits shaped by bottom currents characterize the middle and lower slope of the Campos Basin (Viana, 2001).
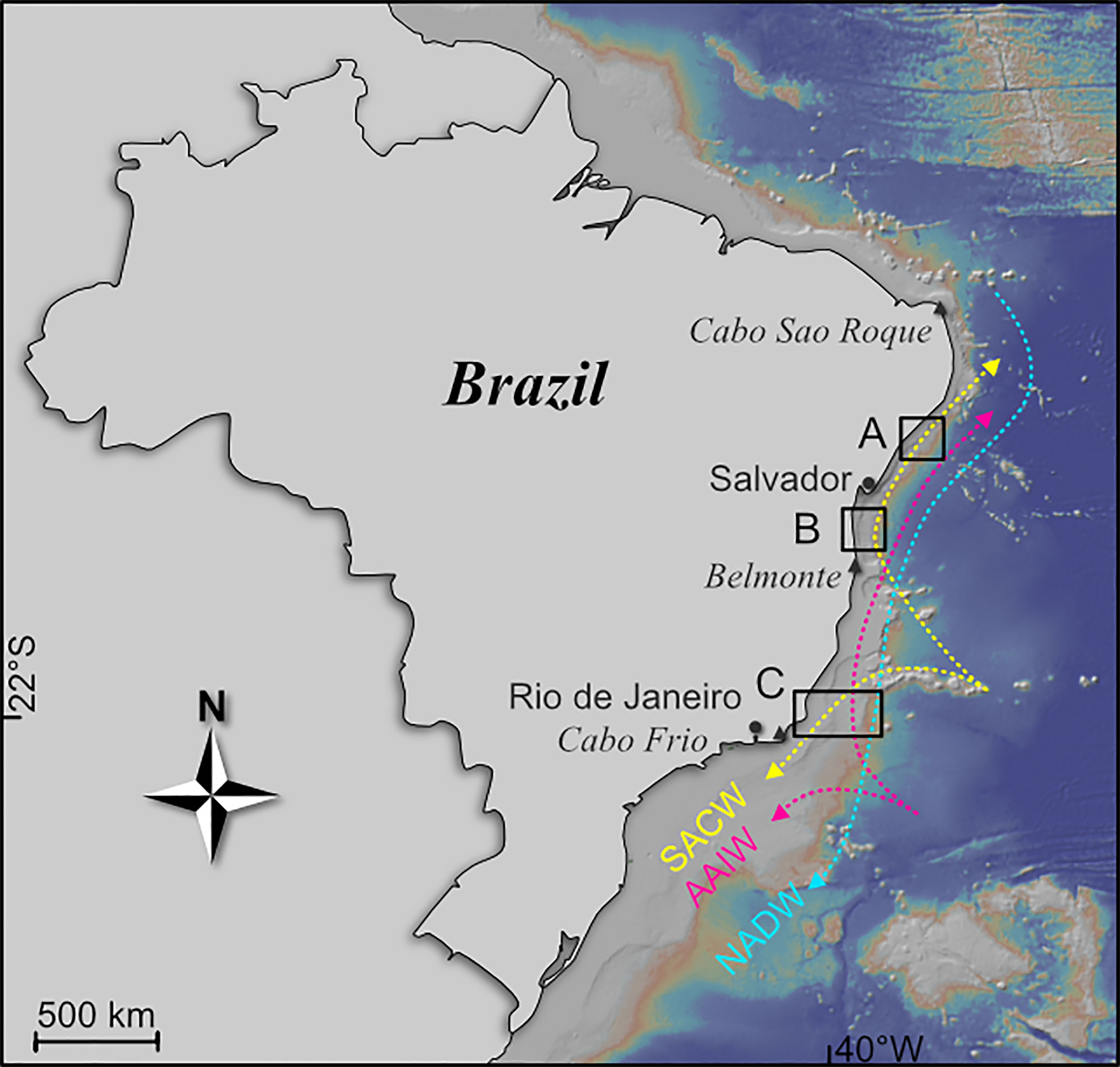
Figure 1 General map of the study area along the Brazilian continental margin. A, B, and C indicate the three investigated areas at 11°S, 14°S, and 22°S, respectively. South Atlantic Central Water (SACW), Antarctic Intermediate Water (AAIW) and North Atlantic Deep Water (NADW) are the major water masses. The map was created with GeoMapApp (www.geomapapp.org), using the base map of Ryan et al. (2009).
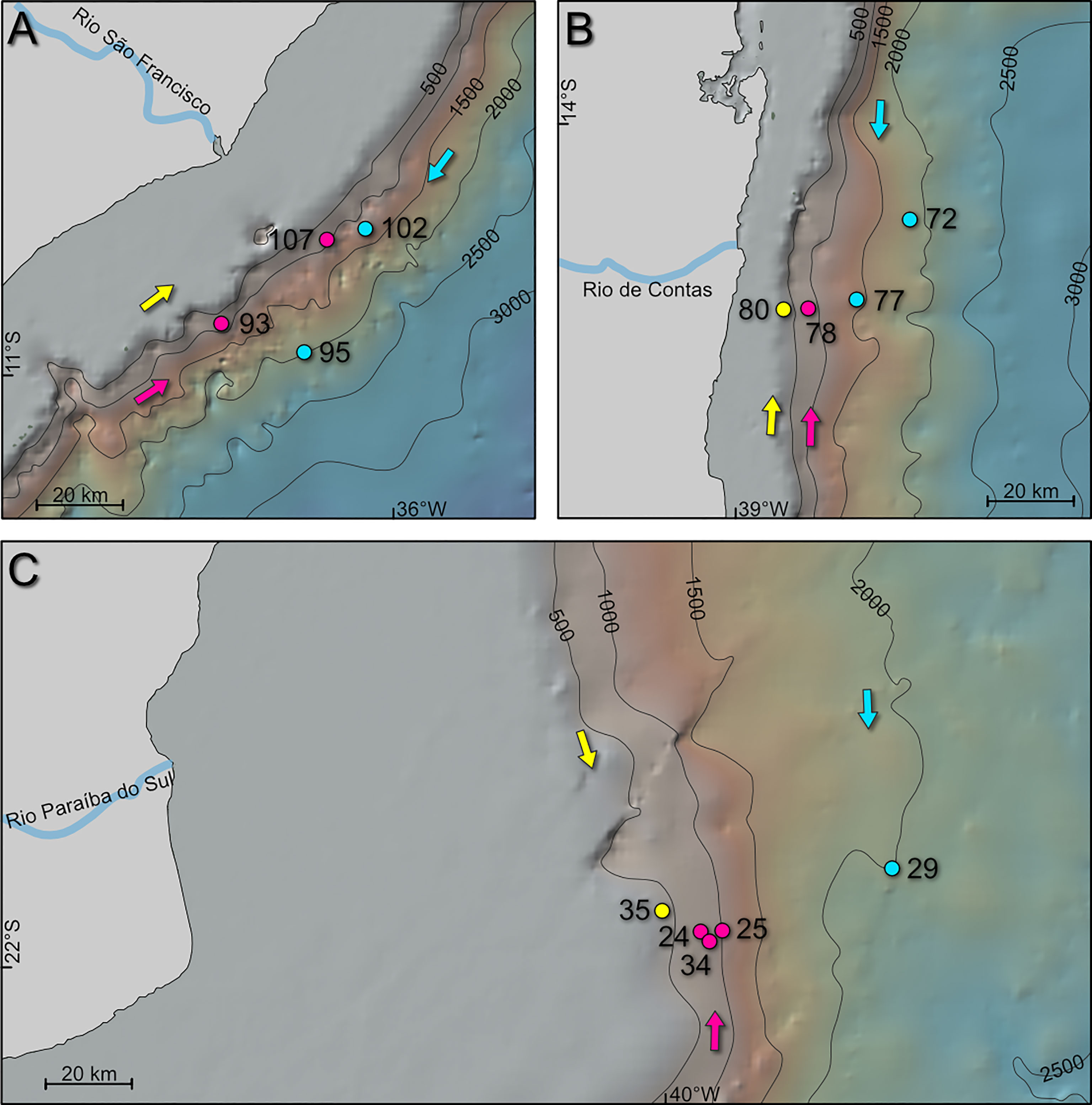
Figure 2 Sample sites of the studied transects of R/V Meteor cruise M125: (A) Transect 7 off Rio Sao Francisco at 11°S; (B) Transect 6 off Rio de Contas at 14°S; (C) Transect 2 off Rio Paraíba do Sul at 22°S. Arrows indicate direction of South Atlantic Central Water (SACW; yellow), Antarctic Intermediate Water (AAIW; pink), and North Atlantic Deep Water (NADW; blue), respectively. Maps were created with GeoMapApp (www.geomapapp.org), using the base map of Ryan et al. (2009).
Four major water masses strongly affect the Brazilian continental margin to a water depth of 3500 m: the Tropical Water (TW), the South Atlantic Central Water (SACW), the Antarctic Intermediate Water (AAIW) and the North Atlantic Deep Water (NADW; Figure 1; Stramma and England, 1999; da Silveira et al., 2020). The warm and saline TW represents the uppermost layer and extends to a depth of 150 m. Beneath the TW, the nutrient-rich SACW with salinities around 35.8 prevails to a depth of 500 m. Sediments transported from the shelf, facilitated by the complex channel system, are considered a major source of organic matter input to the continental margin and contribute to an enhanced nutrient availability within the SACW (Braga et al., 2008). SACW is generated in the South Atlantic at about 40°S by the Brazil- and Falkland Currents (Stramma and England, 1999). It circulates as subtropical SACW within the Southern Subtropical Gyre and is largely driven by the Brazil Current (BC) (Peterson and Stramma, 1991; Stramma and England, 1999). The BC reaches current velocities between 50-80 cm s-1 in the subtropical SACW at the Campos Basin (de Mello e Sousa et al., 2006). At about 20°S, part of the SACW is deflected northwards (Stramma and England, 1999; da Silveira et al., 2020). Slightly lower salinities distinguish it from the subtropical SACW (Stramma and England, 1999). The tropical branch of the SACW, as well as the underlying AAIW, are controlled by the North Brazil Undercurrent (NBUC), which is formed mainly in the northern Drake Passage and the Falkland Current Loop at about 40°S and flows towards the north along the eastern continental margin of South America (Stramma and England, 1999). The AAIW, present at water depths between 500 and 1100 m, is distinguishable from SACW by lower salinities of about 34.2 (Mémery et al., 2000; da Silveira et al., 2020; Raddatz et al., 2020). The equatorward flowing NBUC reaches current velocities of up to 80 cm s-1 in the tropical SACW (da Silveira et al., 2020) and up to 30 cm s-1 in the underlying AAIW (Viana, 2001). The layer of the AAIW at the Brazilian continental slope provides an ideal habitat for cold water coral (CWC) mounds which are mainly known from the Campos Basin at depths of 800-900 m (Viana et al., 1998; Bahr et al., 2016; Raddatz et al., 2020). Below the AAIW lies the upper North Atlantic Deep Water (UNADW), which is carried by the Deep Western Boundary Current (DWBC) from the Northern Hemisphere into the South Atlantic (Stramma and England, 1999; da Silveira et al., 2020). It achieves current speeds up to 20 cm s-1 in the study area (De Madron and Weatherly, 1994) and is characterized by lower nutrient levels compared to its overlying water mass layers (da Silveira et al., 2020; Raddatz et al., 2020).
3 Material & Methods
3.1 Stations and Sample Material
All samples used in this study were obtained during R/V METEOR expedition M125 ‘SAMBA’ along the Brazilian Margin (Bahr et al., 2016). They were taken as multicores or box cores, from which the upper 0.5 cm or 1 cm of sediment were used for foraminiferal analysis, respectively. The internal diameter of the multicore tubes was 10 cm. For the box corer samples, ~78.5 cm3 of surface sediment (1cm depth) was removed using the MUC tube. Samples were partitioned for the measurement of various parameters (e.g., benthic foraminifera, grain size, bulk geochemistry). For calculating the benthic foraminiferal density, the analyzed ‘real’ sediment volume was calculated based on sediment density and analyzed dry weight. A total of 13 surface samples, stained with Rose Bengal, were selected from bathymetric transects 2, 6 and 7 of the cruise. They range from the upper to lower slope between 400 and 2000 m water depth. Transect 2 represents the southernmost transect at approximately 22°S, consisting of 5 stations (24, 25, 29, 34, 35) in water depths ranging from 430 to 2019 m. Transects 6 and 7 are located about 800 km and 1100 km further north, respectively. At 14°S, transect 6 spans four stations (72, 77, 78, 80) at water depths between 420 and 1738 m. Four stations from transect 7 (93, 95, 102, 107) are located at 11°S between 924 and 1900 m (Table 1).
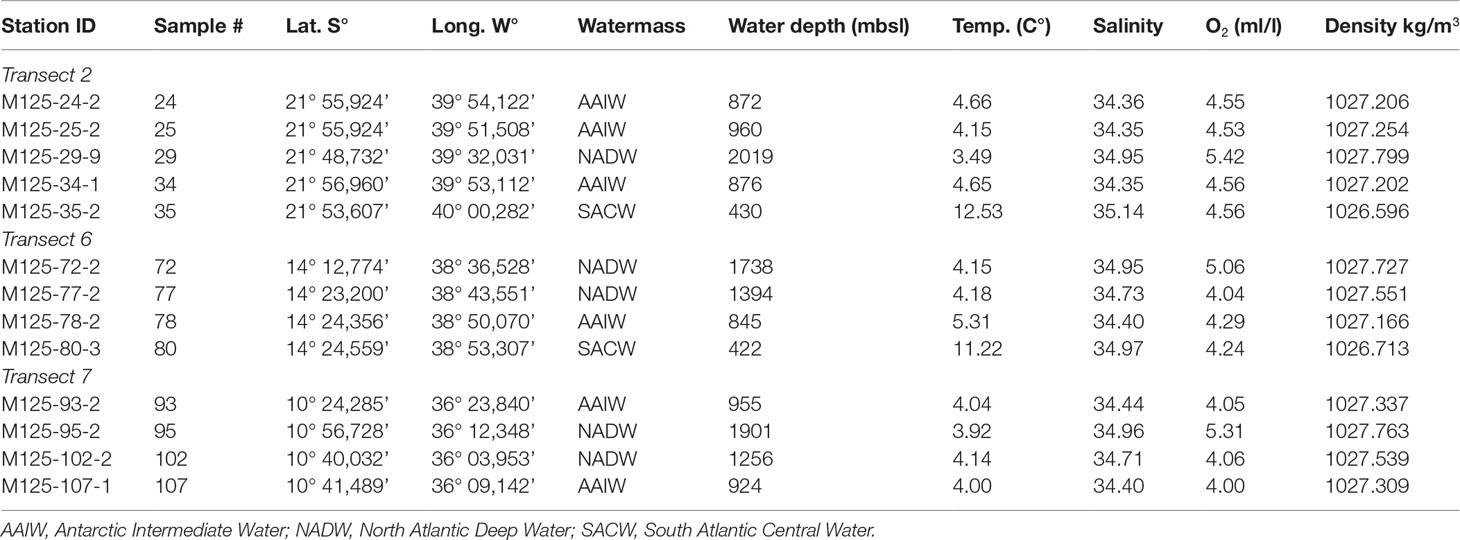
Table 1 Position and hydrological parameters of the selected transects and sample sites of Meteor cruise M125.
3.2 Sedimentological Parameters
Grain size analysis (Table 2; Figure 3) was performed with a particle analyzer via laser diffraction (CILAS 1064) at the Institute of Chemistry, Department of Geochemistry, Universidade Federal Fluminense (UFF), Brazil. The analysis was conducted with decarbonized samples free of organic matter by adding 1M HCl and 30% hydrogen peroxide. For particle dispersion sodium hexametaphosphate solution (4%) was added before the laser analysis.
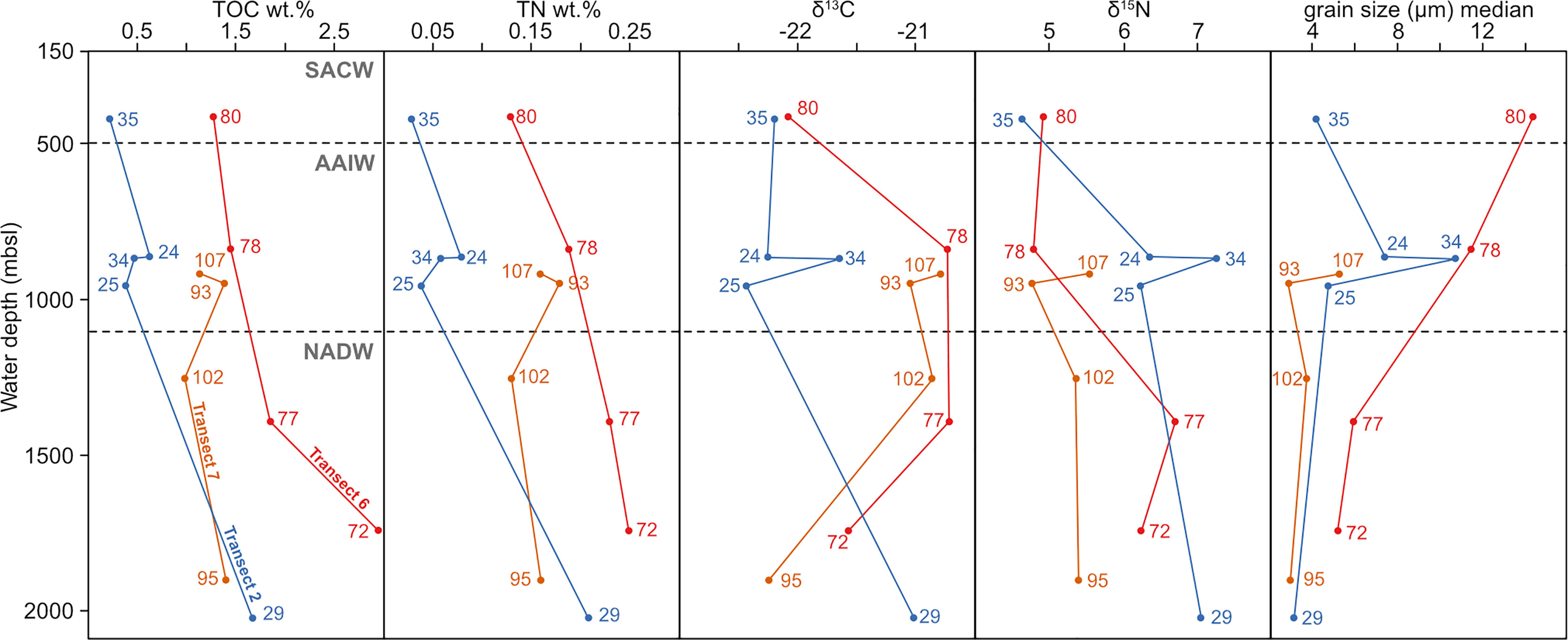
Figure 3 Main sedimentological properties of transects 2, 6 and 7 plotted with increasing water depth. The numbers refer to the samples of the respective transect. Transect 2 is shown in blue, transect 6 in red and transect 7 in orange.
Total organic carbon (TOC) and total nitrogen (TN) contents, and their stable carbon (δ13Corg) and nitrogen (δ15N) isotopes were measured in approximately 60 mg of decarbonized, macerated sediment samples. They were analyzed in the PDZ Europa ANCA-GSL elemental analyzer at the UFF, coupled to a 20-20 PDZ Europa isotope ratio mass spectrometer (SERCON Ltd. Cheshire UK). Isotope ratios were reported relative to the Vienna Pee Dee Belemnite (V-PDB) international standard for carbon and atmospheric N2 for nitrogen. The analytical precision for standards was within ±0.14‰ for δ13Corg and ±0.13‰ for δ15N.
3.3 Benthic Foraminifera
All samples were stored between April 2016 to March 2017 in buffered 4% formaldehyde solution to which had been added Rose Bengal with a concentration of 1g/L. The samples were wet sieved with water over a 63 µm sieve and then dried at 30°C. A dry splitter was used for sample partitioning. All benthic foraminiferal tests > 63 µm of the whole sample or split were counted, yielding at least 400 individuals per sample. Stained (Rose Bengal) and unstained specimens were counted separately, allowing to differentiate the living and dead assemblages in the total faunas (see Bernhard, 2000 for review). Only brightly stained specimens were counted as living. For porcelaneous tests, pale pink stained specimens were distinguished from white ones. Due to very low amounts of stained foraminifera, their evaluation is approached with caution.
Epi- and infaunal microhabitats were assigned according to Schönfeld (1997); Sen Gupta (2002); Murray (2006); Jorissen et al. (2007) and Rasmussen and Thomsen (2017).
The assessment of tubular agglutinated species is challenging as almost all tests are fragmented. Tubular fragments were thus counted individually, but excluded from the total species dataset to avoid over-representation (Goineau and Gooday, 2017). Tubular fragments comprise the species Rhabdammina abyssorum, Rhabdamminella cylindrica, Rhizammina algaeformis and Saccorhiza ramosa. Likewise, species that were not deposited autochthonously but had clearly been transported were removed, including Cribroelphidium spp. and Discorbis williamsoni (see also Appendix 2). To document and assess the role of these excluded taxa, they are presented in Table 3, referred to an expanded matrix that includes tubular fragments and transported specimens.
The species Globocassidulina subglobosa and G. crassa were often not distinguishable from each other. We therefore combined them under the name G. subglobosa/crassa.
For diversity analysis, Fisher’s α and Shannon H indices were calculated (Fisher et al., 1943; Shannon and Weaver, 1949). Due to the very low numbers of stained foraminiferal tests, these calculations were limited to the total assemblages. The dimensionless Fisher’s α, based on logarithmic series, facilitates the comparison of species richness between different habitats as well as samples of different size (Peet, 1974; Murray, 2006). Species diversity, expressed via Shannon H, incorporates rare species that make a smaller contribution (Peet, 1974; Murray, 2006). To estimate foraminiferal densities, the total absolute number of foraminifera for each sample was normalized to 10 cubic centimeter (ccm).
3.4 Multivariate Statistical Analysis
The software package Past 4.02 (Hammer et al., 2001) was used for multivariate statistical analysis. Since abundances of living specimens were very low, statistical analyses were limited to the total fauna (live + dead specimens), as previously implemented by e.g., de Mello e Sousa et al. (2006) for the Campos Basin.
A hierarchical Q-mode cluster analysis (HCA; UPGMA algorithm, Bray-Curtis similarity index) was performed to identify groups of samples based on their similarity and dissimilarity. Based on a similarity percentage analysis (=SIMPER analysis, Bray-Curtis similarity index), the contribution of each species to the dissimilarity of each cluster is obtained for the total fauna (Table 4).
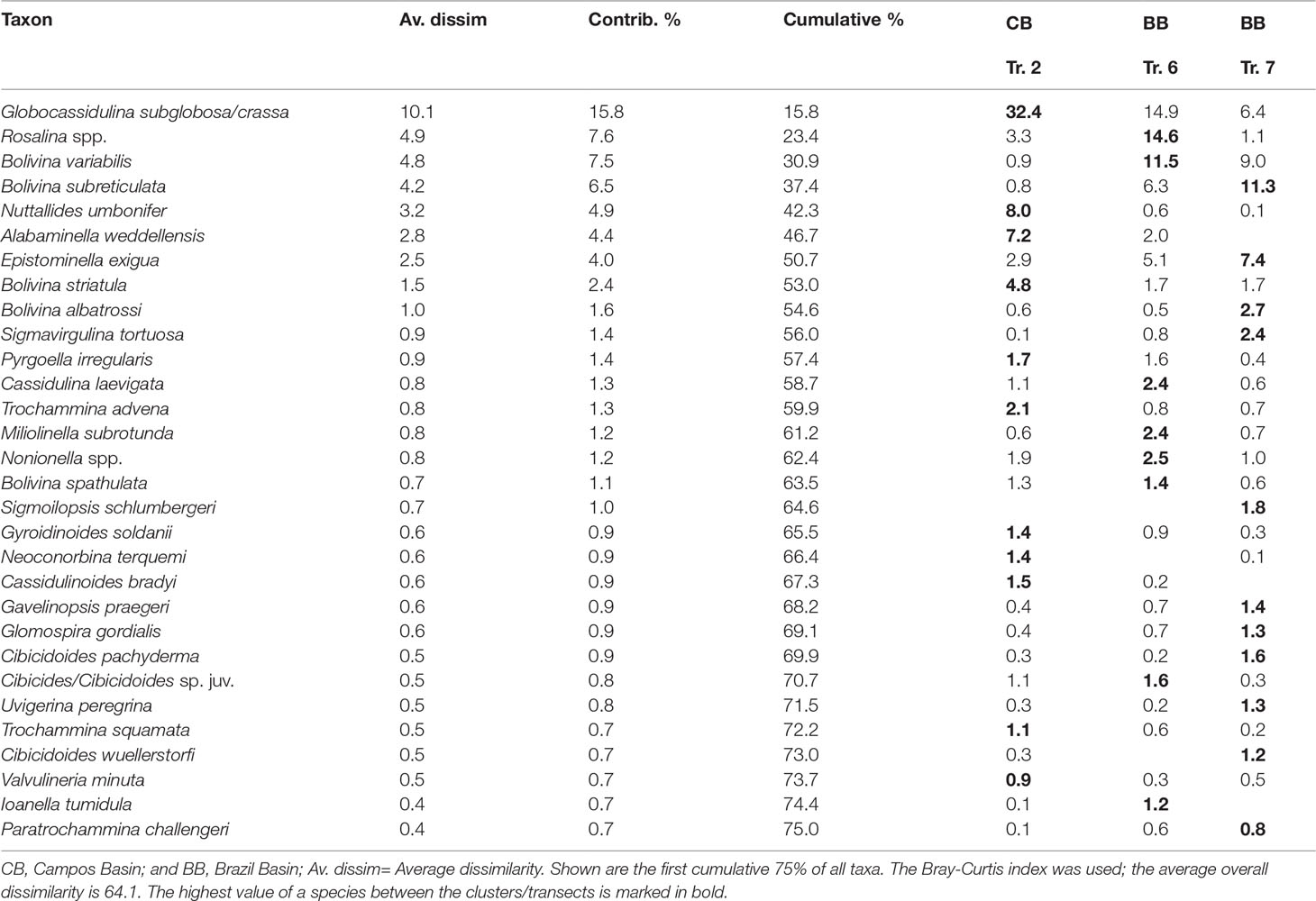
Table 4 Composition of the total benthic foraminiferal community via a SIMPER analysis based on clusters, see Figure 4.
To contextualize foraminiferal data and environmental parameters, a canonical correspondence analysis (CCA) was conducted. A CCA is based on unimodal relationships between species abundances and site-specific environmental variables via synthetic ordination axes (ter Braak and Verdonschot, 1995; Ramette, 2007; Paliy and Shankar, 2016). Hydrographic properties such as salinity and oxygen are excluded as limiting factors as the faunal composition is unlikely to be affected by the rather uniform and stable values for salinity and relatively high oxygen concentrations (Table 1; de Mello e Sousa et al., 2006). However, parameters reflecting export productivity and sedimentation show considerably greater variability. We therefore included six parameters in the CCA that exhibit the greatest impact on the benthic fauna (TOC, TN, δ13Corg, δ15N, proportion of silt and clay; Table 2).
Since rare species tend to be overestimated in a CCA (Legendre and Legendre, 1998; Ramette, 2007), only species that occur to > 3% in at least one sample were included; indeterminate taxa were removed, tubular fragments and transported specimens were excluded.
4 Results
4.1 Environmental Characteristics
4.1.1 Grain Size Distribution
In the Campos Basin at transect 2 (22°S), mean grain sizes between 4.3 and 19.5 µm were recorded. A wider range of 6.5 to 30.5 µm is observed in the samples of transect 6 in the Brazil Basin (14°S). Finer sediment was encountered in transect 7 (11°S) with mean grain sizes between 3.8 and 7 µm. Sand contents were only recorded in sample 34 from transect 2 (12.5%, AAIW in 876 m) as well as samples 78 (11.5%, AAIW in 845 m) and 80 (29.9%, SACW in 422 m) from transect 6, while the remainder consist of silt and clay only (Table 2).
In the Campos Basin, sample 34 was taken within a cold-water coral (CWC) mound sequence, and sample 24 was retrieved from the marginal mound facies, covered with corals only at the surface (Bahr et al., 2016). Both samples contain the highest mean grain sizes within transect 2 of 19.5 µm (sample 34) and 8.6 µm (sample 24), as well as the highest silt contents ranging from 76 to 88%, and lowest clay contents at about 12% in each case (Table 2). Coarsest mean grain sizes within transect 6 are present in SACW sample 80 (422 m) with 30.5 µm and AAIW sample 78 (845 m) with 21 µm on average (Table 2). In transect 7, mean grain sizes reach their maximum in AAIW sample 107 (924 m) with only 7 µm. Clay contents are highest in this transect and vary between 26 and 37% (Table 2).
4.1.2 Total Organic Carbon, Total Nitrogen Contents and Their Stable Isotopes δ13Corg and δ15N
The range of total organic carbon within the sediment is 0.2-3.0 wt.% with the maximum being measured in sample 72 (transect 6, NADW, 1738 m). Total nitrogen contents also reach their maximum of 0.3 wt. % in sample 72. In the Campos Basin (22°S) TN levels are 0.03-0.2, whereas they are 0.1-0.3 wt. % in the Brazil Basin transects (11-14°S). The C:N ratios range from 7 to 12 for the entire data set, with only sample 72 yielding a value exceeding 10 (Table 2).
The stable carbon isotope δ13Corg within the bulk organic matter spans values between -22.4 and -20.7 ‰ for the entire data set. δ15N content ranges from 4.7 to 7.3 ‰ of the bulk sediment (Table 2).
4.2 Benthic Foraminifera
4.2.1 Total Assemblage Composition
A total of 278 species was identified, of which 139 have hyaline tests, 104 are agglutinated, and 35 have a porcelaneous test.
Densities of benthic foraminifera per 10 ccm are higher in transect 2 and 6 (44 000-800 000 specimens/10 ccm) than in transect 7 (5 000-13 000 specimens/10 ccm). The highest density in transect 2 in the Campos Basin was observed in sample 35 associated with subtropical SACW (321 000 specimens/10 ccm). Population density is even higher in sample 80 associated with tropical SACW in the Brazil Basin (799 000 specimens/10 ccm; Table 3). A comparison of samples associated with AAIW and NADW reveals that transect 7, with a maximum of 13 000 specimens/10 ccm, has generally reduced population densities than transect 2 (max. 165 000 s/10 ccm for AAIW and NADW) and 6 (max. 359 000 s/10 ccm for AAIW and NADW; Table 3). However, species richness, indicated by Fisher’s α, is lower in transects 2 and 6 (α=6-12) than in the northern transect 7 (α=13-23; Table 3). This is also reflected by the Shannon H index, where rare species contribute less (Murray, 2006). Smaller values were calculated for transect 2 (H=2.6-3.1), they increased slightly in transect 6 (H=2.8-3.2) and highest in transect 7 (H=3.5-4.0; Table 3; see also Appendix 1).
Calcareous-hyaline species predominate all investigated transects, with the highest abundances of > 86% recorded in transect 2 (samples 24, 25, 34, 35) and transect 6 (sample 77; Table 3). Globocassidulina subglobosa/crassa is abundant across all studied transects. However, it is more frequent along the Campos Basin (32%) than along the Brazil Basin transects (6-15%; result of the SIMPER analysis see Table 4). Important hyaline taxa that further define the dataset are Rosalina spp., Bolivina variabilis and B. subreticulata, which are more abundant in the Brazil Basin (each taxon occurs at > 11% in transect 6 or 7) than in the Campos Basin (each taxon occurs < 4%; according to the SIMPER analysis in Table 4). Agglutinated tests are more frequent in transect 7 (17-28%) than in transects 2 and 6 (6-16%; Table 3), with a broad range of taxa being responsible (e.g., cribrostomoidids, trochamminids; see Appendix 2). Additionally, the proportion of tubular fragments is increased in transect 7 (2-9%) compared to the southern sample sites (0-1%), with Saccorhiza ramosa fragments being most abundant (Table 3 and Figure 5). Frequently occurring porcelaneous taxa include Pyrgoella irregularis and Miliolinella subrotunda in all transects (Tables 3, 4).
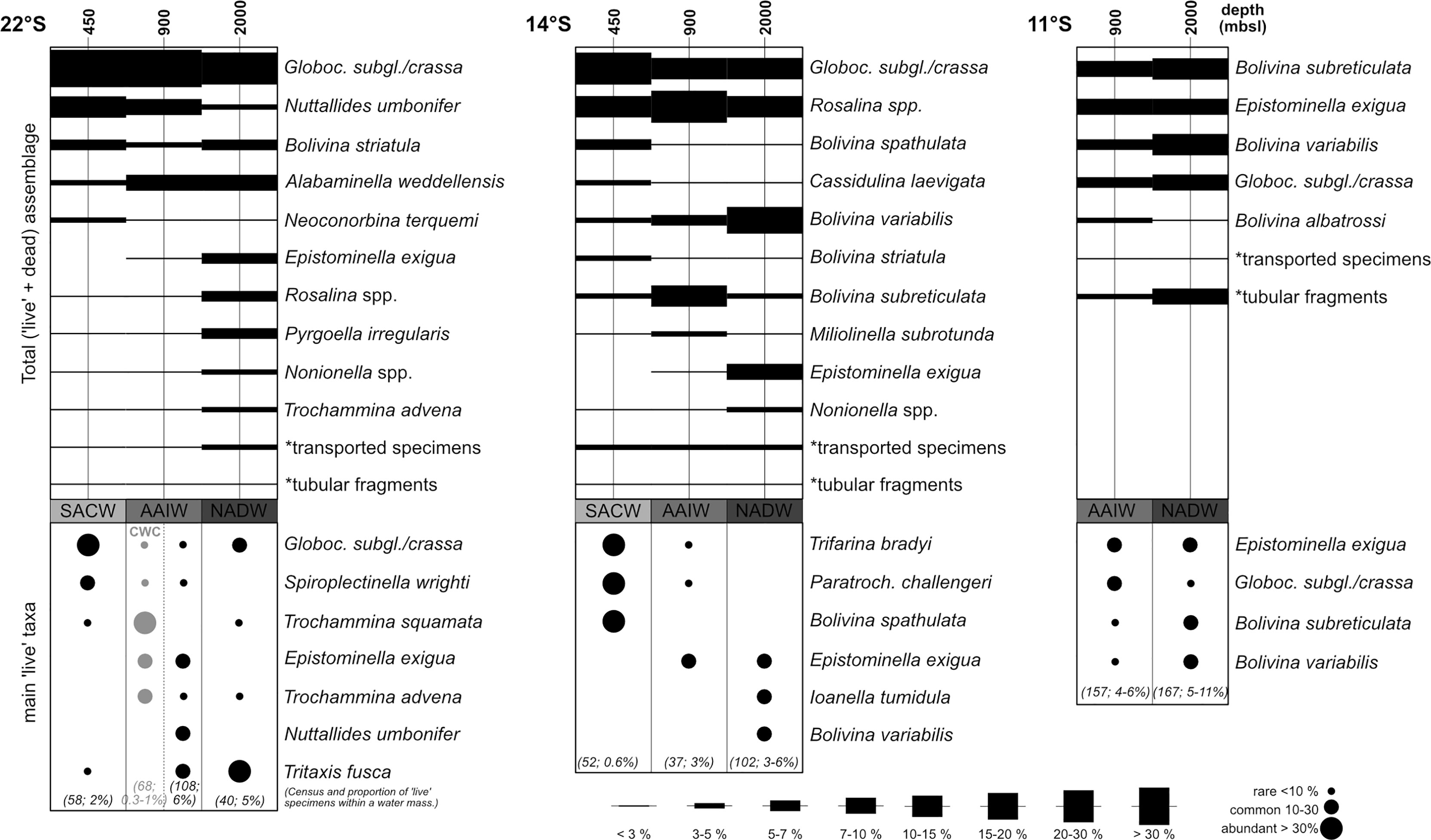
Figure 5 Abundances of benthic foraminiferal taxa > 63 µm in the studied areas at 22°S, 14°S and 11°S relative to water masses. Proportions of transported specimens and tubular fragments indicated by asterisks have been calculated with reference to the whole assemblages (Table 3). All taxa that occur with at least 3% in one water mass are plotted. Bars indicate abundances based on the total (live+dead) assemblage. Stained (living) specimens are indicated by circles and given as rare (< 10 %), common (10-30 %), and abundant (> 30 %). The values in parentheses indicate the absolute analyzed census counts and proportions of living specimens.
Infaunal species dominate the data set. In the Campos Basin they make up 49-62% of the total fauna, while they range between 42 and 57% in the Brazil Basin (Table 3).
4.2.2 Total (Live + Dead) Assemblage Distributions
According to the hierarchical cluster analysis (HCA; cophenetic correlation coefficient: 0.88), the data set of the total assemblage can be divided into two major clusters representing the Campos Basin (cluster ‘CB’) and the Brazil Basin (cluster ‘BB’), respectively. Cluster ‘CB’ comprises all transect 2 samples, while Cluster ‘BB’ is internally subdivided into two groups of samples corresponding to transects 6 and 7 (Figure 4). SIMPER analysis reveals that 30 taxa determine 75% of the cumulative dissimilarity between clusters and groups (Table 4). Cluster ‘CB’ is distinguished by increased abundances of Globocassidulina subglobosa/crassa associated with Nuttallides umbonifer and Alabaminella weddellensis. In contrast, Cluster ‘BB’ is mainly determined by an assemblage comprising various bolivinids and Rosalina species (Table 4).
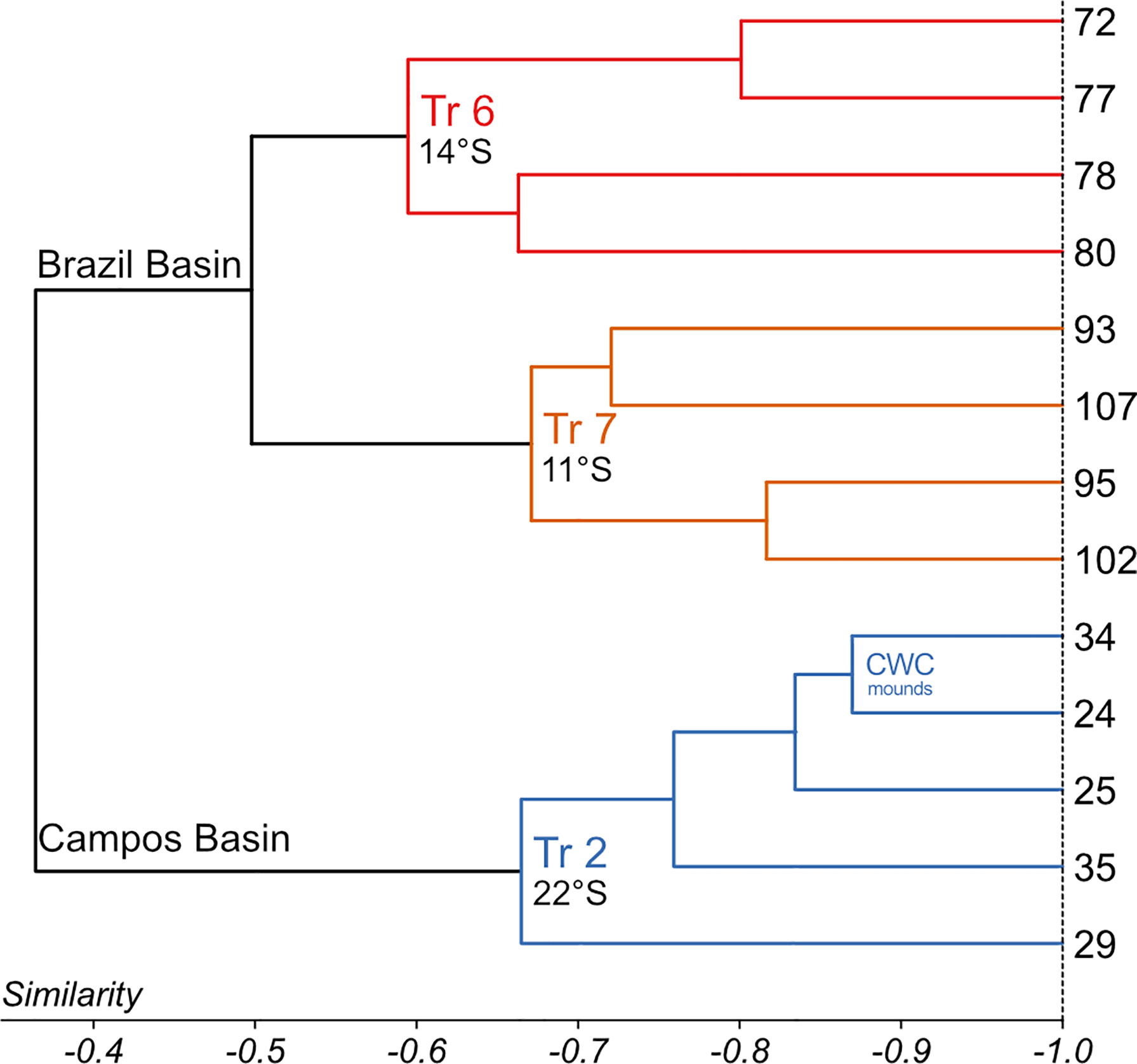
Figure 4 Cluster analysis of the total assemblages of benthic foraminifera > 63 µm. The UPGMA algorithm and Bray-Curtis dissimilarity were used, cophenetic correlation is 0.88. Three clusters could be distinguished, reflecting the surveyed transects (Tr. 2, Tr. 6, and Tr. 7).
A Globocassidulina subglobosa/crassa assemblage prevails in the Campos Basin. According to the SIMPER analysis the transect is characterized by G. subglobosa/crassa (32%), Nuttalides umbonifer (8%), Alabaminella weddellensis (7%), Bolivina striatula (5%) and Rosalina spp. (3%; Table 4). Globocassidulina subglobosa/crassa and N. umbonifer decrease slightly with increasing depth, while A. weddellensis increases in the AAIW and NADW samples 24, 25, 29, 34 (Figure 5). Epistominella exigua, Rosalina spp. and Pyrgoella irregularis are associated taxa in the deeper sample 29 within the NADW (Figure 5). Within the ‘CB’ cluster, both CWC mound samples 24 and 34 form a distinct subgroup, plotting closest to cluster ‘BB’.
The total assemblage of transect 6 at 14°S is mainly determined by G. subglobosa/crassa (15%), Rosalina spp. (15%), Bolivina variabilis (12%), Bolivina subreticulata (6%) and Epistominella exigua (5%; Table 4). Occurrences of G. subglobosa/crassa are mainly concentrated on the upper slope within SACW (sample 80) and abundances are substantially reduced when compared to the Campos Basin (Figure 5). With increasing depth, assemblages with various species of Rosalina determine the benthic fauna of transect 6. In samples 72 and 77, which are associated with NADW, bolivinids become predominant, with Bolivina variabilis being most abundant (Figure 5).
In transect 7 at 11°S, a Bolivina assemblage characterizes the benthic foraminiferal association. The SACW layer was not investigated in this area, so the association only reflects AAIW and NADW samples. The assemblage is composed of Bolivina subreticulata (11%), Bolivina variabilis (9%), Epistominella exigua (7%) and Globocassidulina subglobosa/crassa (6%; Table 4). Tubular fragments are common in transect 7 and increase with depth (Table 3; Figure 5). Bolivinids are slightly more common in deeper NADW samples 95 and 102 than in AAIW samples 93 and 107, where they are accompanied by increased occurrences of Saccorhiza ramosa fragments. Associated Epistominella exigua occurs equally in both water masses (Figure 5). Thus, the assemblages in the Brazil Basin are strongly determined by rosalinids and bolivinids, contrasting a Globocassidulina assemblage in the Campos Basin.
4.2.3 Key Environmental Factors and Total Assemblage Distribution
A canonical correspondence analysis (CCA) based on scale type 1 after Legendre and Legendre (1998) explains the relationship between foraminiferal species, environmental variables and sample sites (Figure 6). As a function of distance between the variables TOC, TN, δ13Corg, δ15N, silt and clay, the canonical axes CCA1 (eigenvalue = 0.28, variance = 58.4%) and CCA2 (eigenvalue = 0.09, variance = 18.1%) explain > 75% of the entire data set.
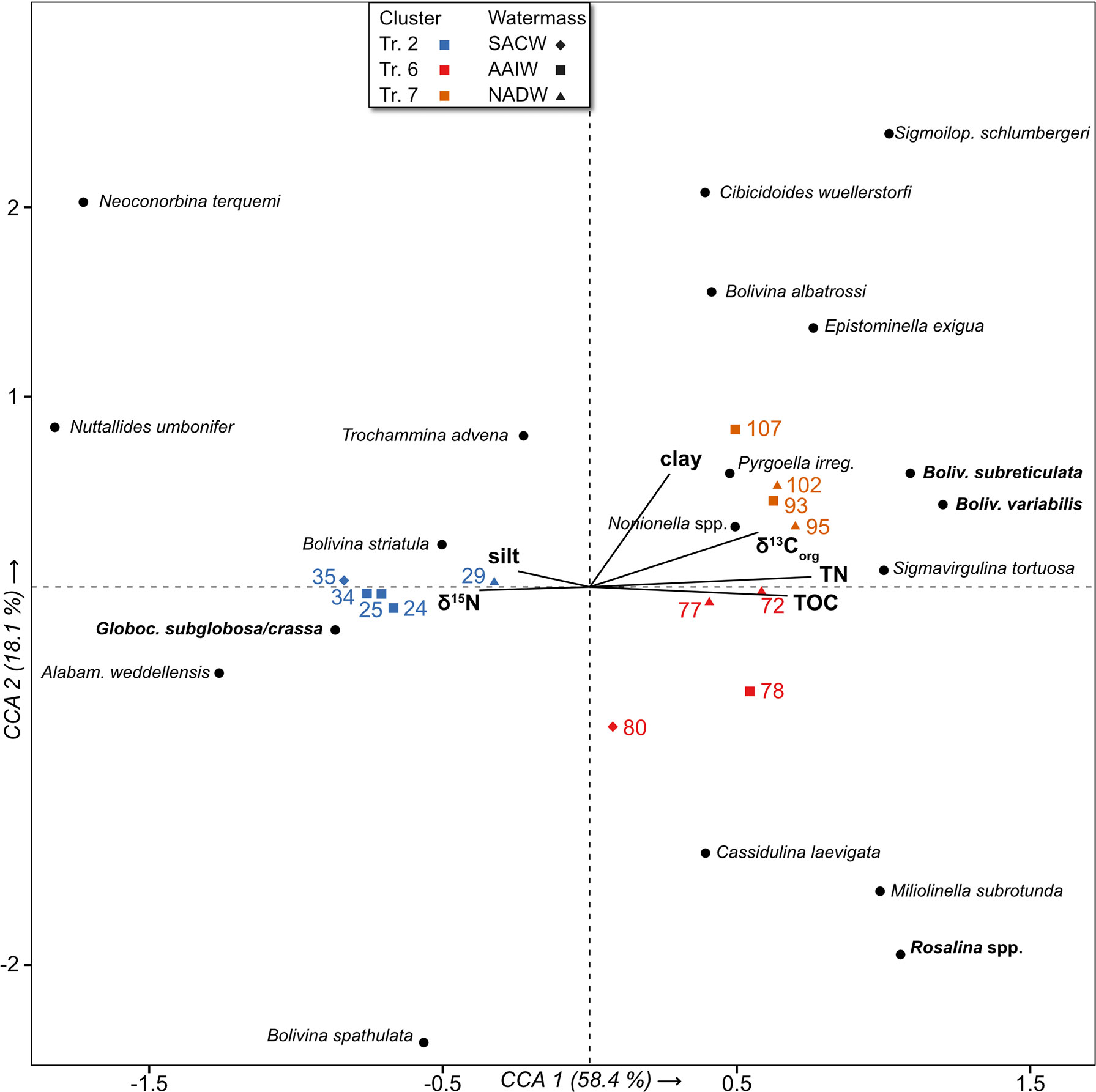
Figure 6 Canonical correspondence analysis, scale type 1, with environmental parameters δ15N, δ13Corg, clay and silt contents, total organic carbon (TOC) and total nitrogen (TN). Taxa with abundances > 3% in at least one sample are shown. Colour coding represents affiliation to a cluster; symbols indicate the water mass of a sample.
The sample sites are separated along the axes in terms of their assignment to the ‘CB’ and ‘BB’ clusters. Cluster ‘CB’ (transect 2) plots on negative CCA1 together with the δ15N and silt vectors. Sample 35 from the upper slope (SACW) and deepest sample 29 (NADW) plot in the positive range of CCA2, more strongly associated with silt. All AAIW samples (24, 25, 34) plot in the negative range and are more strongly associated with δ15N. All caluster ‘BB’ samples (transects 6 and 7) plot on positive CCA1 in the range of TOC, TN, δ13Corg and clay vectors. Within cluster ‘BB’, all transect 6 samples plot on negative CCA2, where they are associated with TOC. The samples of transect 7 fall in the positive range of CCA2, within the quadrant of TN, clay and δ13Corg vectors (Figure 6).
The Globocassidulina subglobosa/crassa assemblage of the Campos Basin falls within the negative range of CCA1. Globocassidulina subglobosa/crassa, and Alabaminella weddellensis occur rather close to δ15N, while Trochammina advena and Bolivina striatula plot between the silt and clay vectors (Figure 6). Bolivina spathulata is the only bolivinid species that contrasts with the clay vector, as it is slightly enhanced in the samples close to the CWC mounds (24, 25). The CCA illustrates that the G. subglobosa/crassa assemblage is mainly associated with Nuttalides umbonifer at the upper slope (sample 35), while Alabaminella weddellensis is predominant in the deeper areas (samples 24, 25, 34; Figures 5, 6).
The Rosalina assemblage of transect 6 plots on positive CCA1 and negative CCA2. Rosalina spp., Miliolinella subrotunda and Cassidulina laevigata are associated with relatively higher organic carbon levels (Figure 6).
The CCA shows that various infaunal species of the Bolivina assemblage, such as Bolivina subreticulata, Sigmavirgulina tortuosa, Bolivina albatrossi and Sigmoilopsis schlumbergeri occur preferentially in the clay-rich samples of transect 7, all plotting in the positive range of CCA1 and CCA2. The associated species Epistominella exigua, Pyrgoella irregularis and Nonionella spp. also show good concordance with higher clay contents.
4.2.4 Stained (Living) Assemblage Composition
Between 22 to 128 stained specimens were picked per sample, exceeding 100 specimens in only three samples (25, 93, 102). The contribution of living individuals to the total assemblage ranges from 0.3% to 11%, being highest in transect 7 (Table 3). Similar to the total assemblages, the number of living foraminifera per 10 ccm is the lowest in transect 7 (200-800 specimens/10 ccm); it is slightly increased at transect 2 in the Campos Basin (500-7000 specimens/10 ccm) and highest in transect 6 in the Brazil Basin (3000-11 000 specimens/10 ccm; Table 3).
Stained specimens belong to 147 species (63 hyaline, 61 agglutinated, 23 porcelaneous) all of which are represented among dead tests. The biocoenosis of transect 2 from the Campos Basin consists primarily of G. subglobosa/crassa, Trochammina squamata, and Tritaxis fusca. Stained specimens of G. subglobosa/crassa are abundant on the upper slope within the SACW in sample 35, associated with common occurrences of Spiroplectinella wrighti (Figure 5). AAIW samples 34 and 24, which were retrieved directly from, or very close to, a cold-water coral mound, contain mainly stained Trochammina squamata with Epistominella exigua and Trochammina advena (Figure 5). In adjacent AAIW sample 25, located distal to the coral mounds, E. exigua, Nuttallides umbonifer and Tritaxis fusca occur commonly within the biocoenosis. In sample 29, associated with NADW, Tritaxis fusca was the most common species in the live assemblage (Figure 5).
In the Brazil Basin at transect 6, the living assemblage within SACW (sample 80) consists of abundant Trifarina bradyi, Paratrochammina challengeri and Bolivina spathulata. Stained specimens of Epistominella exigua are common in sample 78 associated with AAIW. With increasing depth, live individuals of E. exigua are associated with live Ioanella tumidula and Bolivina variabilis in the NADW (Figure 5). The biocoenosis of transect 7 is mainly determined by E. exigua, G. subglobosa/crassa, Bolivina subreticulata and B. variabilis and shows the highest resemblance to the total assemblage (Figure 5; see also Appendix 2).
5 Discussion
5.1 Patterns of Foraminiferal Diversity, Density, and Distribution
Diversity analyses indicate that the benthic foraminiferal faunas correspond to typical assemblages from well-oxygenated deep-sea environments (Gooday, 2003; Murray, 2006). Calculated Fisher’s α and Shannon H indices are comparable to the species diversity for the South Atlantic (Murray, 2006). Lower diversity indices in transect 2 (α=6-12; H=2.6-3.1), slightly increased indices in transect 6 (α=8-10; H=2.8-3.2), followed by higher indices in transect 7 (α=13-23; H=3.5-4.0) may reflect a general increase in foraminiferal diversity along the Brazilian Margin from South to North (Table 3; Appendix 1). Clearly, more studies with a higher spatial resolution are needed to test the robustness of this pattern.
No direct relation is observed between benthic foraminiferal diversity and density. The latter is substantially lower in transect 7 (5 000-13 000 specimens/10 ccm) than in transects 2 and 6 (44 000-800 000 specimens/10 ccm; Table 3) for both total and living faunas. Thus, the population density of transect 7 is more similar to the eastern South Atlantic (cf. Schmiedl et al., 1997). The densities of transect 2 agree well with those measured in the Campos Basin by de Mello e Sousa et al. (2006), while transect 6 even exceeds these values.
Lower numbers of foraminiferal tests per 10 cubic centimeter in transect 7 do not result in a lower diversity, as might be expected from other faunal studies, e.g., Jorissen et al. (1995) and Fontanier et al. (2002). A low number of specimens per 10 ccm coupled with a high species heterogeneity could be linked to increased inputs of phytodetritus (Lambshead and Gooday, 1990; Gooday and Rathburn, 1999). This is supported by occurrences of species sometimes associated with phytodetritus such as Pyrgoella irregularis in transect 7 (Figure 6 and Appendix 2; Gooday, 1988). A higher sampling resolution would be necessary to gain more detailed insight into the observed patterns of species density and diversity.
Percentages of stained specimens in all transects (0.3-11%; Table 3) are consistent with the observations of de Mello e Sousa et al. (2006), who describe similarly low values from the Campos Basin. It is a common observation in deep-sea samples that only a small proportion of benthic foraminiferal specimens consists of living individuals (Murray, 2006). Great variation in the volume of protoplasm (e.g., during periods of low food influx) in certain species further complicates the recognition of stained tests and thus limits the application of Rose Bengal (Linke and Lutze, 1993; Murray, 2006).
Regarding the distribution of different test types, a northward trend can be observed. Agglutinated tests are relatively more abundant in transect 7 (17-28%) compared to both southern transects (6-16%; tab. 3). This is further underlined when comparing the proportions of tubular fragments between transect 7 (2-9%) and transects 2 and 6 (0-1%; Table 3). In transect 7, tubular fragments mainly include Saccorhiza ramosa (Figure 7), which prefers areas with less pronounced bottom current activity (Altenbach et al., 1988; Gooday, 2003). Individual species of each test type will be examined in more detail in the following chapters.
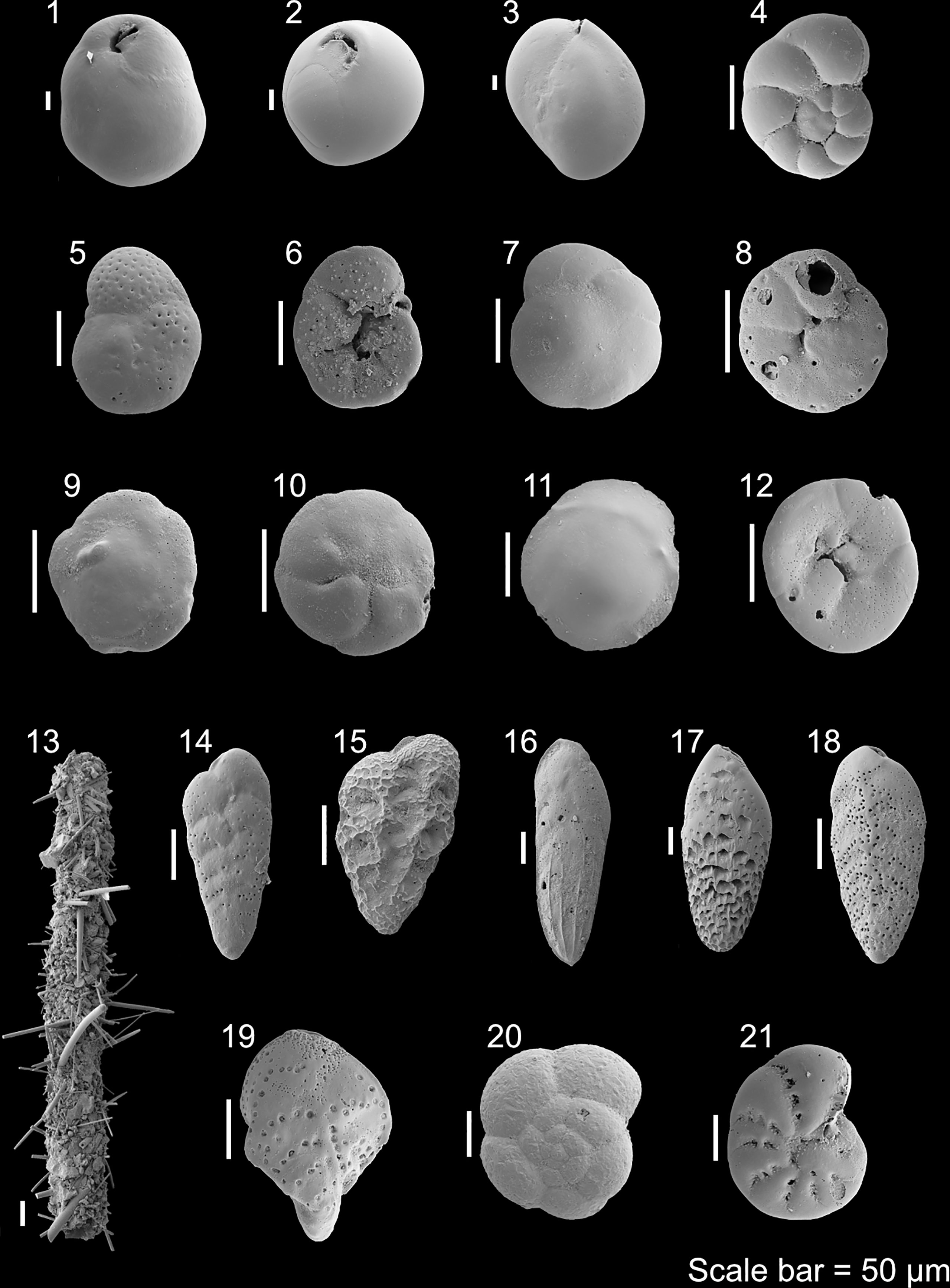
Figure 7 The main taxa of the Brasil and Campos basin records: 1 Globocassidulina subglobosa (Brady, 1881); 2 Pyrgoella irregularis (d’Orbigny, 1839); 3 Miliolinella subrotunda (Montagu, 1803); 4 Nonionella sp.; 5 Rosalina globularis (d’Orbigny, 1826), spiral side; 6 R. globularis (d’Orbigny, 1826), umbilical side; 7 Nuttallides umbonifer (Cushman, 1933), spiral side; 8 N. umbonifer (Cushman, 1933), umbilical side; 9 Alabaminella weddellensis (Earland, 1936), spiral side; 10 A. weddellensis (Earland, 1936), umbilical side; 11 Discorbis williamsoni (Chapman & Parr, 1932), spiral side; 12 D. williamsoni (Chapman & Parr, 1932), umbilical side; 13 Fragment of Saccorhiza ramosa (Brady, 1879); 14 Bolivina variabilis (Williamson, 1858); 15 Bolivina subreticulata (Parr, 1932) 16 Bolivina striatula (Cushman, 1922) 17 Bolivina albatrossi (Cushman, 1922) 18 Bolivina spathulata (Williamson, 1858); 19 Sigmavirgulina tortuosa (Brady, 1881); 20 Trochammina advena Cushman, 1922, spiral side; 21 Cribroelphidium incertum (Williamson, 1858), side view.
5.2 Controlling Factors of Faunistic Differences Between the Campos and Brazil Basins
Multivariate statistical analyses of the total assemblages suggest that the observed patterns of benthic foraminiferal distribution are explained by the quantity and quality of organic matter exported to the seafloor, properties of the substrate and the hydrodynamic regime at the sediment/water interface. δ15N values in the range of 4.7-7.3 ‰, together with δ13Corg between -20 to -22 ‰ indicate a marine origin of the organic matter deposited along the investigated transects in the Campos and Brazil Basins (Nagel et al., 2009; Rumolo et al., 2011; Gaye et al., 2018). This interpretation is further validated by C:N ratios between 4 and 10, indicating that nitrogen is remineralized and nutrients are released (Table 2; Anderson, 1992; Rumolo et al., 2011). The main food source on the continental slope in the Campos Basin was previously reported to be autochthonous organic material derived from primary production (Carreira et al., 2010; Yamashita et al., 2018). Despite the exceedingly narrow shelf at the Rio de Contas and the Rio Sao Francisco (11-14°S), the southward drift of sediments delivered by these rivers prevents their transport to the continental slope (Rebouças et al., 2011; Cavalcante et al., 2020). Terrestrial organic material thus seems to play a minor role in the sediments despite nearby river discharges, implying a predominance of marine organic material (Cordeiro et al., 2018). In addition to primary production, along- and downslope transport due to major current systems and local canyons and channel networks delivers more refractory organic matter to all three study areas (Bahr et al., 2016; da Silveira et al., 2020). The Campos Basin in particular is characterized by contourite deposition and coarser sediments indicating increased bottom currents in this area (Viana, 2001; de Mello e Sousa et al., 2006). Constant bottom flow velocities reflected in increased silt proportions (Table 2) as well as reworked sediments result in relatively reduced organic matter concentrations in the surface substrates (de Mello e Sousa et al., 2006; Gaye et al., 2018). Towards the north, where the subtropical gyre becomes less vigorous, calmer settings and more clayey sediments predominate (Table 2; Stramma and England, 1999; Mémery et al., 2000; da Silveira et al., 2020).
5.2.1 Campos Basin
High abundances of Globocassidulina subglobosa/crassa are typical for the shelf and upper slope of the Campos Basin (Lohmann, 1978; de Mello e Sousa et al., 2006; Eichler et al., 2008; Burone et al., 2011; Dias et al., 2018). Their high abundances coincide with increased grain sizes (Figure 6), suggesting they prefer constant bottom flow velocities, as already suggested in previous studies (e.g., Schmiedl et al., 1997; de Mello e Sousa et al., 2006). Local occurrences of upper slope contourites as well as CWC mounds in the Campos Basin document an increased bottom current activity in this area as well (Viana, 2001; Viana et al., 2002; Bahr et al., 2020; Raddatz et al., 2020). On the upper slope in the subtropical part of the SACW at 430 m (sample 35) a G. subglobosa/crassa-Nuttalides umbonifer assemblage is adapted to lower nitrogen levels and reduced primary productivity, highlighted in the CCA by TN plotting contrary to it (Figure 6; Gooday, 1994; Gooday, 2003; Margreth et al., 2009; De and Gupta, 2010). The assemblage is complemented by Alabaminella weddellensis with increasing depth beyond 850 m in the AAIW. Similar to G. subglobosa/crassa, A. weddellensis can be considered as an r-strategist that responds opportunistically to the input of organic material (e.g., Gooday, 1988; Gooday, 1993; Dias et al., 2018). Bolivinids contribute less to the Campos Basin than to the Brazil Basin samples, with Bolivina striatula and B. spathulata being the most prevalent species (Table 4 and Figure 6). Bolivina striatula is mainly recorded from the upper slope (450 m, SACW sample 35; Figure 5). However, it is well known from the continental shelf down to a depth of 200 m and can serve as an indicator for freshwater input in estuarine areas (Boltovskoy et al., 1980; Eichler et al., 2008; Mendes et al., 2012). Since no stained B. striatula were found in sample 35, downslope transport from the shelf, favored by the pronounced canyon system on the slope of the Campos Basin (Bahr et al., 2016), might explain the occurrences of B. striatula at greater water depths. Support for downslope transport comes from occurrences of shelf-dwelling Discorbis williamsoni as well as some occurrences of elphidiids, mainly represented by Cribroelphidium incertum (Figure 7; Boltovskoy et al., 1980; Murray, 2006).
The surveyed transect in the Campos Basin (430-2000 m, 22°S) agrees well in its faunal composition with the former study by de Mello e Sousa et al. (2006; 750-2000 m, 22-23°S) near the Sao Tomé and Itapemirim Canyons. The authors describe a Globocassidulina subglobosa assemblage, inhabiting sandier sediments and being adapted to oligotrophic conditions and increased bottom currents (de Mello e Sousa et al., 2006). Opportunistic species such as G. subglobosa, Nuttalides umbonifer and Alabaminella weddellensis thrive in more unstable substrates due to local bottom currents and cope with high fluctuations in food availability (Schmiedl et al., 1997; de Mello e Sousa et al., 2006; Fentimen et al., 2018; Fentimen et al., 2020). These species decline northwards and are clearly under-represented in the northern study areas.
The CWC mounds of the Campos Basin represent a distinct facies not addressed in previous studies and yielding a slightly divergent foraminiferal association. The high abundances of A. weddellensis in the AAIW are mainly linked to the CWC mound samples (Figure 6; Appendix 2). As coral scaffolds capture phytodetritus, increased A. weddellensis occurrences seem to be favored here (Fentimen et al., 2018). Attached species such as Trochammina advena use the constant near-bottom current for suspension feeding (Schönfeld, 2002a). It was also noticeable that particularly large specimens of Cibicidoides wuellerstorfi occur exclusively in CWC mounds (they occur in samples 24 and 34 almost exclusively in the > 250 µm fraction). Although C. wuellerstorfi appears to be more numerous in the samples from the Brazil Basin (Figure 6), the examined specimens were clearly smaller there. Probably C. wuellerstorfi benefits from a better nutrient supply in the increased current regime within the CWC mounds, which is reflected in its size. The increased proportion of attached Trochammina squamata in the living stock of the CWC samples, coinciding with the lack of stained globocassidulinids, demonstrates that attached suspension feeders use the elevated substrates of coral reefs as a niche to benefit from trapped suspended nutrients (Lutze and Thiel, 1989; Linke and Lutze, 1993; Schönfeld, 1997). Occurrences of B. spathulata close to the CWC mounds (samples 24, 25) imply that this particular bolivinid species prefers to live in silty environments near a coral facies, which has already been shown by Fentimen et al. (2018); Fentimen et al., 2020) for the CWC mounds of the Porcupine Seabight in the northeast Atlantic Ocean. The preference of B. spathulata for silty substrates is further reflected in its occurrences within the SACW (sample 35 in transect 2 and sample 80 in transect 6; Table 2 and Figure 6), which exhibits relatively higher current velocities (de Mello e Sousa et al., 2006; da Silveira et al., 2020).
5.2.2 Brazil Basin
The bolivinid-dominated assemblages of transects 6 and 7 coincide with comparably higher total nitrogen and organic carbon contents, as implied in the CCA (Figure 6). This association suggests an increased nutrient input and thus slightly higher food availability in these areas (Corliss, 1985; Schmiedl et al., 1997; de Mello e Sousa et al., 2006). The consistent presence of Epistominella exigua in all samples > 900 m depth (Figure 5) further indicates increased primary production, as this species is often associated with phytodetrital input (Gooday, 1988; Gooday, 1993; Mackensen et al., 1995; Gooday, 1996; Kurbjeweit et al., 2000; De and Gupta, 2010). Seasonal variability of the NBUC at about 10°S (Stramma et al., 1995) seems to be associated with increased E. exigua occurrences, as this species prefers seasonal inputs of phytodetritus (Gooday, 1988; Mackensen et al., 1990; Schmiedl et al., 1997).
In transect 6 increased occurrences of Cassidulina laevigata, generally linked to enhanced influx of organic matter (Schmiedl et al., 1997; de Mello e Sousa et al., 2006; De and Gupta, 2010), are mainly observed in SACW sample 80, where it coincides within the same quadrant as the TOC vector in the CCA (Figure 5). Downslope transport in this region is indicated by occurrences of elphidiids which are present in the total assemblage in all samples of transect 6, extending into the areas of NADW (sample 72, 1700 m; represented as ‘transported species’ in Figure 5). The steep slope with deeply incised canyons characterized by a network of meandering and straight channels, together with a very narrow continental shelf, facilitates downslope transport of entrained material (Bahr et al., 2016). Rich Rosalina spp. occurrences are mainly documented from the middle slope (Figure 5). Rosalina species favor more distal settings in terms of increased bottom current activity and prefer to inhabit slightly elevated substrates that are exposed to light currents (Corliss, 1985; Schönfeld, 2002b). They can move from their clinging position to a motile situation when food is scarce (DeLaca and Lipps, 1972). Rosalina species are thus able to search for areas with an increased nutrient supply, where they can stretch out their pseudopodia to ingest food (DeLaca and Lipps, 1972). A high Rosalina density in transect 6 is likely linked to sufficient food availability, as suggested by the correspondence with TOC and TN contents (depicted in the CCA, Figure 6). Slightly increased occurrences of Miliolinella subrotunda within the AAIW (sample 78) coincide with the highest occurrences of rosalinids within transect 6 (Figure 5). Suspension-feeding M. subrotunda also prefers rather gentle currents as this protist uses flexible agglutinated tubes to support its pseudopodial system that it deploys into the water to feed. These tubes may be distorted or damaged by bottom flow speeds that were too high (Altenbach et al., 1993). Along the slope in NADW samples 72 and 77, the Rosalina assemblage is complemented by occurrences of various Nonionella species (N. turgida, N. iridea, Nonionella spp. juv.; Figure 5). Nonionella is associated in several studies with the input of fresh organic matter and phytodetritus (Mackensen et al., 1990; Gooday and Hughes, 2002; Duffield et al., 2015; Alve et al., 2016). Transect 6 is thus characterized by a comparably higher nutrient input, corroborated by the positive association with TOC and TN contents in the CCA (Figure 6; Gooday, 2003; Alve et al., 2016).
Substrates sampled on the slope along transect 7 at 11°S are much finer compared to the study sites at 22°S and 14°S (Figure 6 and Table 2). The fauna defined by various Bolivina species is complemented by occurrences of Pyrgoella irregularis, which is sometimes associated with phytodetritus and inhabits more clayey areas (Gooday, 1988; Gooday, 1993). Saccorhiza ramosa colonizes the NADW samples 95 and 102 on transect 7 (shown as ‘tubular fragments’ in Figure 5), each of which was collected at elevated positions within the canyon system and thus located distal to the main downslope transport (Figure 5; Bahr et al., 2016). This tubular and fragile suspension feeder requires stable substrates to dwell upright in the sediment and indicates moderate to low current intensities and poor reworking by detritus feeders (Altenbach et al., 1987; Mackensen, 1987; Linke and Lutze, 1993). Occurrences of S. ramosa are already known from similar settings in the Gulf of Cadiz where they live distal to the main current of the Mediterranean outflow water (Schönfeld, 1997; Schönfeld, 2002a; Schönfeld 2002b). The coincidence of enhanced silt contents with all samples from transect 7 in the CCA emphasizes a generally calmer setting in this study area (Figure 6). The current velocity of the northward flowing North Brazilian Undercurrent (NBUC) appears to be weaker at 11°S compared to the southern study areas (Stramma et al., 1995). This can be explained by the sampling depth far below the velocity core of the NBUC, which is located at the pycnocline between BC and SACW in this area (150-200 m; Stramma et al., 1995; da Silveira et al., 2020).
De Mello e Sousa et al. (2006) describe a Bolivina assemblage with reduced G. subglobosa abundances, from the deeper and muddier areas of the Campos Basin, where more stable substrates and reduced bottom currents prevail. In some respects, their Bolivina assemblage resembles that found between 11 and 14°S in the present study. However, the abundance of G. subglobosa/crassa on transects 6 and 7 is even lower than reported by de Mello e Sousa et al. (2006). The faunal data from 11-14°S suggests that the northward directed branch of tropical SACW, strongly controlled by the NBUC, favors an assemblage that prefers high organic matter fluxes and finer sediments (Stramma and England, 1999; Gooday, 2003; da Silveira et al., 2020). In transect 6, G. subglobosa/crassa is still the most common taxon, but accompanied by a rich Rosalina association, which is missing at the Campos Basin (de Mello e Sousa et al., 2006). Globocassidulina subglobosa/crassa plays a very minor role at 11°S in transect 7 (Table 4). Alabaminella weddellensis is much less abundant in the northern sample sites compared to the Campos Basin; in transect 7 it is not recorded at all (Table 4). This could be due to the lack of CWC mounds in the Brazil Basin as well as finer sediments in transect 7 (Fentimen et al., 2018; Fentimen et al., 2020). High organic matter fluxes considerably influence the bolivinid-determined assemblage in these areas (Gooday, 2003). The additional occurrence of the opportunistic species Epistominella exigua further distinguishes the northern assemblage from the deeper Campos Basin, where this species is under-represented (de Mello e Sousa et al., 2006).
Regarding the sparse living assemblage, stained specimens such as attached Tritaxis fusca and Trochammina squamata in transect 2 contrast with the few living foraminifera at transects 6 and 7, some of which are infaunal bolivinids (transect 6) and vulnerable tube-shaped tests of Saccorhiza ramosa (transect 7). Tritaxis fusca and Trochammina squamata have already been mentioned in relation to increased bottom currents, confirming an increased hydrodynamic energy level in the Campos Basin (e.g., Schönfeld, 1997; Schönfeld and Zahn, 2000; Schönfeld 2002a), whereas the biocenosis of the Brazil Basin transects well reflects calmer environments (Figure 5).
6 Conclusions and Future Directions
New microfaunal and sedimentological data indicate that the benthic foraminiferal fauna at the Brazilian continental margin between 11 and 22°S is controlled by the quantity and quality of organic matter fluxes as well as substrate properties and hydrodynamic conditions at the sediment water interface. This study reveals that a Globocassidulina subglobosa/crassa assemblage in the Campos Basin is contrasted by a rosalinid/bolivinid assemblage in the Brazil Basin. This biogeographic divide occurs across the bifurcation of the SACW into its southward subtropical and northward tropical branches. The subtropical transect at 22°S reveals slightly lower diversity indices but increased population densities compared to the tropical study areas (11-14°S). The proportion of stained (living) specimens is somewhat lower at 22°S compared to 11-14°S. However, typically for deep-sea samples, their proportion is rather small for all three sample areas.
The Campos Basin is characterized by a Globocassidulina subglobosa/crassa assemblage, further defined by Nuttalides umbonifer and Alabaminella weddellensis. This assemblage reflects a variable nutrient supply and coarser substrates due to increased bottom current activity. Alabaminella weddellensis occupies an ecological niche in CWC mounds to benefit from trapped phytodetritus. Occurrences of elphidiids and Bolivina striatula indicate downslope transport due to the large canyon network on the continental slope of the Campos Basin.
A Rosalina-Bolivina assemblage characterizes the continental slope in the Brazil Basin. At 14°S, the assemblage composition is defined by G. subglobosa/crassa, Rosalina spp., Bolivina variabilis and Bolivina subreticulata, with a lower proportion of G. subglobosa compared to the Campos Basin. Enhanced abundances of Rosalina spp. are associated with increased TOC and TN levels. In transect 7, a faunal association of Bolivina subreticulata, Bolivina variabilis, Epistominella exigua, G. subglobosa/crassa characterises the upper to lower continental slope, coinciding with more clayey sediments. The bolivinid dominated assemblage is associated with an increased nutrient supply, as reflected by its coincidence with relatively higher TOC values in the CCA. Occurrences of Saccorhiza ramosa, especially at the lower slope, are linked to more stable substrates.
The new data set implies distinct contrasts in foraminiferal densities, diversities and assemblage composition between the Campos and Brazil basins. The quantity, quality, and seasonality of organic matter influx together with substrate properties and hydrodynamic conditions are identified as the main drivers of the observed patterns. However, the present study, while presenting the first quantitative data for the Brazilian continental margin 11-14°S, is limited in its ability to predict large-scale biogeographic patterns due to its coarse spatial resolution. It should thus be considered a base line and starting point for further faunistic studies along the Brazilian continental margin in the future. Only a significantly higher spatial resolution will allow a fuller understanding of patterns of foraminiferal densities, diversity, and biogeography in this vast area. Investigations of faunal distributions across the potentially critical water mass divide of the northern and southern SACW branches might prove particularly informative in this endeavor.
Data Availability Statement
The original contributions presented in the study are included in the article/Supplementary Material. Further inquiries can be directed to the corresponding author.
Author Contributions
AS performed the statistical analyses and wrote the manuscript. AS, JP and PG drafted the concept of the study and contributed to the evaluation of the statistical analyses. AS and JS conducted the taxonomic evaluation. AB and BD contributed to the collection of samples and interpretation of sedimentological parameters. BD, AA and RD performed the chemical analyses on the sediment samples. All authors contributed to the article and approved the submitted version.
Funding
This study was financially supported and enabled by the Deutsche Forschungsgemeinschaft (DFG, German Research Foundation) through project GR52851/1-1.
Conflict of Interest
The authors declare that the research was conducted in the absence of any commercial or financial relationships that could be construed as a potential conflict of interest.
Publisher’s Note
All claims expressed in this article are solely those of the authors and do not necessarily represent those of their affiliated organizations, or those of the publisher, the editors and the reviewers. Any product that may be evaluated in this article, or claim that may be made by its manufacturer, is not guaranteed or endorsed by the publisher.
Acknowledgments
We acknowledge Hanna Cieszynski (University of Cologne) for her support with the SEM. Marie Scheel (University of Cologne) is thanked for her assistance in washing and picking the samples. We thank Tobias Walla who contributed to the sample preparation. BD acknowledges the financial support from FAPESP (grants 2020/11452-3, 2019/24349-9, and 2018/15123-4). We further thank the three reviewers Andrew John Gooday, Michael Martínez-Colón, and Bryan O’Malley for their helpful comments.
Supplementary Material
The Supplementary Material for this article can be found online at: https://www.frontiersin.org/articles/10.3389/fmars.2022.901224/full#supplementary-material
References
Altenbach A. V., Heeger T., Linke P., Spindler M., Thies A. (1993). Miliolinella Subrotunda (Montagu), a Miliolid Foraminifer Building Large Detritic Tubes for a Temporary Epibenthic Lifestyle. Mar. Micropaleontol. 20, 293–301. doi: 10.1016/0377-8398(93)90038-Y
Altenbach A. V., Lutze G. F., Weinholz P. (1987). Beobachtungen an Benthos-Foraminiferen (Teilprojekt A3). Berichte. aus. dem. Sonderforschungsbereich. 313 (6), 86.
Altenbach A. V., Unsöld G., Walger E. (1988). The Hydrodynamic Environment of Saccorhiza Ramosa (BRADY). Meyniana 40, 119–132.
Alve E., Korsun S., Schönfeld J., Dijkstra N., Golikova E., Hess S., et al. (2016). Foram-AMBI: A Sensitivity Index Based on Benthic Foraminiferal Faunas From North-East Atlantic and Arctic Fjords, Continental Shelves and Slopes. Mar. Micropaleontol. 122, 1–12. doi: 10.1016/j.marmicro.2015.11.001
Anderson T. R. (1992). Modelling the Influence of Food C:N Ratio, and Respiration on Growth and Nitrogen Excretion in Marine Zooplankton and Bacteria. J. Plankton Res. 14, 1645–1671. doi: 10.1093/plankt/14.12.1645
Bahr A., Doubrawa M., Titschack J., Austermann G., Koutsodendris A., Nürnberg D., et al. (2020). Monsoonal Forcing of Cold-Water Coral Growth Off Southeastern Brazil During the Past 160&Thinsp;Kyr. Biogeosciences 17, 5883–5908. doi: 10.5194/bg-17-5883-2020
Bahr A., Spadano Albuquerque A. L., Ardenghi N., Batenburg S. J., Bayer M., Catunda M. C., et al. (2016). METEOR-Berichte: South American Hydrological Balance and Paleoceanography During the Late Pleistocene and Holocene (SAMBA) - Cruise No. M125. (Bremen, Germany: MARUM – Zentrum für Marine Umweltwissenschaften der Universität Bremen) doi: 10.2312/cr_m125
Bernhard J. M. (2000). Distinguishing Live From Dead Foraminifera: Methods Review and Proper Applications. Micropaleontology 46, 38–46.
Boltovskoy E., Giussani G., Watanabe S., Wright R. C. (1980). Atlas of Benthic Shelf Foraminifera of the Southwest Atlantic 1st ed. Ed. Junk W. (The Netherlands: bv. publishers, The Hague). doi: 10.1016/0377-8398(81)90028-1
Brady H. B., (1879). Notes on some of the Reticularian Rhizopoda of the "Challenger" Expedition; Part I. On New or Little Known Arenaceous Types.Quarterly Journal of Microscopical Sciences 19, 20–67.
Brady H. B., (1881). Notes on some of the Reticularian Rhizopoda of the "Challenger" Expedition. Part III. Quarterly Journal of Microscopical Science 21, 31–71.
Braga E. S., Chiozzini V. C., Berbel G. B. B., Maluf J. C. C., Aguiar V. M. C., Charo M., et al. (2008). Nutrient Distributions Over the Southwestern South Atlantic Continental Shelf From Mar Del Plata (Argentina) to Itajaí (Brazil): Winter-Summer Aspects. Cont. Shelf Res. 28, 1649–1661. doi: 10.1016/j.csr.2007.06.018
Burone L., de e Sousa S. H. M., de Mahiques M. M., Valente P., Ciotti A., Yamashita C. (2011). Benthic Foraminiferal Distribution on the Southeastern Brazilian Shelf and Upper Slope. Mar. Biol. 158, 159–179. doi: 10.1007/s00227-010-1549-7
Carreira R. S., Araújo M. P., Costa T. L. F., Ansari N. R., Pires L. C. M. (2010). Lipid Biomarkers in Deep Sea Sediments From the Campos Basin, SE Brazilian Continental Margin. Org. Geochem. 41, 879–884. doi: 10.1016/j.orggeochem.2010.04.017
Cavalcante G., Vieira F., Campos E., Brandini N., Medeiros P. R. P. (2020). Temporal Streamflow Reduction and Impact on the Salt Dynamics of the São Francisco River Estuary and Adjacent Coastal Zone (NE/Brazil). Reg. Stud. Mar. Sci. 38, 101363. doi: 10.1016/j.rsma.2020.101363
Chapman F., Parr W. J. (1932). Victorian and South Australian Shallow-Water Foraminifera. Part II.Proceedings of the Royal Society of Victoria 44, 218–234.
Cordeiro L. G. M. S., Wagener A. L. R., Carreira R. S. (2018). Organic Matter in Sediments of a Tropical and Upwelling Influenced Region of the Brazilian Continental Margin (Campos Basin, Rio De Janeiro). Org. Geochem. 120, 86–98. doi: 10.1016/j.orggeochem.2018.01.005
Corliss B. H. (1985). Microhabitats of Benthic Foraminifera Within Deep-Sea Sediments. Nature 314, 435–438. doi: 10.1038/314435a0
Cushman J. A. (1922). Shallow-Water Foraminifera of the Tortugas Region. Publications of the Carnegie Institution of Washington 311.Department of Marine Biology of the Carnegie Institution of Washington 17, 1–85.
Cushman J. A., (1933). Some New Recent Foraminifera from the Tropical Pacific. Contributions from the Cushman Laboratory for Foraminiferal Research 9(4), 77–95.
da Silveira I. C. A., Napolitano D. C., Farias I. U. (2020). Water Masses and Oceanic Circulation of the Brazilian Continental Margin and Adjacent Abyssal Plain . In: Sumida P.Y.G., Bernardino A.F., De Léo F.C. (eds) Brazilian Deep-Sea Biodiversity. Brazilian Marine Biodiversity. Springer:Cham. 7–36. doi: 10.1007/978-3-030-53222-2_2
de Almeida F. K., de Mello R. M., Rodrigues A. R., Bastos A. C. (2022). Bathymetric and Regional Benthic Foraminiferal Distribution on the Espírito Santo Basin Slope, Brazil (SW Atlantic). Deep. Res. Part I Oceanogr. Res. Pap. 181, 103688. doi: 10.1016/j.dsr.2022.103688
De S., Gupta A. K. (2010). Deep-Sea Faunal Provinces and Their Inferred Environments in the Indian Ocean Based on Distribution of Recent Benthic Foraminifera. Palaeogeogr. Palaeoclimatol. Palaeoecol. 291, 429–442. doi: 10.1016/j.palaeo.2010.03.012
DeLaca T. E., Lipps J. H. (1972). The Mechanism and Adaptive Significance of Attachment and Substrate Pitting in the Foraminiferan Rosalina Globularis d’Orbigny. J. Foraminifer. Res. 2, 68–72. doi: 10.2113/gsjfr.2.2.68
De Madron X. D., Weatherly G. (1994). Circulation, Transport and Bottom Boundary Layers of the Deep Currents in the Brazil Basin. J. Mar. Res. 52, 583–638. doi: 10.1357/0022240943076975
de Mello e Sousa S. H., Passos R. F., Fukumoto M., da Silveira I. C. A., Figueira R. C. L., Koutsoukos E. A. M., et al. (2006). Mid-Lower Bathyal Benthic Foraminifera of the Campos Basin, Southeastern Brazilian Margin: Biotopes and Controlling Ecological Factors. Mar. Micropaleontol. 61, 40–57. doi: 10.1016/j.marmicro.2006.05.003
Dias B. B., Barbosa C. F., Faria G. R., Seoane J. C. S., Albuquerque A. L. S. (2018). The Effects of Multidecadal-Scale Phytodetritus Disturbances on the Benthic Foraminiferal Community of a Western Boundary Upwelling System, Brazil. Mar. Micropaleontol. 139, 102–112. doi: 10.1016/j.marmicro.2017.12.003
d’Orbigny A.D., (1826). Tableau méthodique de la classe des Céphalopodes.Annales des Sciences Naturelles 7, 245–314.
d’Orbigny A. D., (1839).Voyage dans l’Amérique méridionale. ForaminifèresPitoit-Levrault, Paris. 1–86. doi: 10.5962/bhl.title.110540
Duffield C. J., Hess S., Norling K., Alve E. (2015). The Response of Nonionella Iridea and Other Benthic Foraminifera to “Fresh” Organic Matter Enrichment and Physical Disturbance. Mar. Micropaleontol. 120, 20–30. doi: 10.1016/j.marmicro.2015.08.002
Earland A., (1936). Foraminifera, Part IV. Additional Records from the Weddell Sea Sector from Material obtained by the S.Y 'Scotia'. Discovery Reports 13. 1–76.
Eichler P. P. B., Rodrigues A. R., Eichler B. B., Braga E. S., Campos E. J. D. (2012). Tracing Latitudinal Gradient, River Discharge and Water Masses Along the Subtropical South American Coast Using Benthic Foraminifera Assemblages. Braz. J. Biol. 72, 723–759. doi: 10.1590/s1519-69842012000400010
Eichler P. P. B., Sen Gupta B. K., Eichler B. B., Braga E. S., Campos E. J. (2008). Benthic Foraminiferal Assemblages of the South Brazil: Relationship to Water Masses and Nutrient Distributions. Cont. Shelf Res. 28, 1674–1686. doi: 10.1016/j.csr.2007.10.012
Fentimen R., Lim A., Rüggeberg A., Wheeler A. J., Van Rooij D. (2020). Impact of Bottom Water Currents on Benthic Foraminiferal Assemblages in a Cold-Water Coral Environment: The Moira Mounds (NE Atlantic). Mar. Micropaleontol. 154, 1–14. doi: 10.1016/j.marmicro.2019.101799
Fentimen R., Rüggeberg A., Lim A., Kateb A., Foubert A., Wheeler A. J., et al. (2018). Benthic Foraminifera in a Deep-Sea High-Energy Environment: The Moira Mounds (Porcupine Seabight, SW of Ireland). Swiss. J. Geosci. 111, 533–544. doi: 10.1007/s00015-018-0317-4
Fisher R. A., Corbet A. S., Williams C. B. (1943). The Relation Between the Number of Species and the Number of Individuals in a Random Sample of an Animal Population. J. Anim. Ecol. 12, 42. doi: 10.2307/1411
Fontanier C., Jorissen F. J., Licari L., Alexandre A., Anschutz P., Carbonel P. (2002). Live Benthic Foraminiferal Faunas From the Bay of Biscay: Faunal Density, Composition, and Microhabitats. Deep. Res. Part I Oceanogr. Res. Pap. 49, 751–785. doi: 10.1016/S0967-0637(01)00078-4
Gaye B., Böll A., Segschneider J., Burdanowitz N., Emeis K. C., Ramaswamy V., et al. (2018). Glacial-Interglacial Changes and Holocene Variations in Arabian Sea Denitrification. Biogeosciences 15, 507–527. doi: 10.5194/bg-15-507-2018
Goineau A., Gooday A. J. (2017). Novel Benthic Foraminifera are Abundant and Diverse in an Area of the Abyssal Equatorial Pacific Licensed for Polymetallic Nodule Exploration. Sci. Rep. 7, 1–15. doi: 10.1038/srep45288
Gooday A. J. (1988). A Response by Benthic Foraminifera to the Deposition of Phytodetritus in the Deep Sea. Nature 332, 70–73. doi: 10.1038/332070a0
Gooday A. J. (1993). Deep-Sea Benthic Foraminiferal Species Which Exploit Phytodetritus: Characteristic Features and Controls on Distribution. Mar. Micropaleontol. 22, 187–205. doi: 10.1016/0377-8398(93)90043-W
Gooday A. J. (1994). The Biology of Deep-Sea Foraminifera: A Review of Some Advances and Their Applications in Paleoceanography. PALAIOS 9, 14–31. doi: 10.2307/3515075
Gooday A. J. (1996). Epifaunal and Shallow Infaunal Foraminiferal Communities at Three Abyssal NE Atlantic Sites Subject to Differing Phytodetritus Input Regimes. Deep. Res. Part I Oceanogr. Res. Pap. 43, 1395–1421. doi: 10.1016/S0967-0637(96)00072-6
Gooday A. J. (2003). Benthic Foraminifera (Protista) as Tools in Deep-Water Palaeoceanography: Environmental Influences on Faunal Characteristics. Adv. Mar. Biol. 46, 1–90. doi: 10.1016/S0065-2881(03)46002-1
Gooday A. J., Hughes J. A. (2002). Foraminifera Associated With Phytodetritus Deposits at a Bathyal Site in the Northern Rockall Trough (NE Atlantic): Seasonal Contrasts and a Comparison of Stained and Dead Assemblages. Mar. Micropaleontol. 46, 83–110. doi: 10.1016/S0377-8398(02)00050-6
Gooday A. J., Jorissen F. J. (2012). Benthic Foraminiferal Biogeography: Controls on Global Distribution Patterns in Deep-Water Settings. Ann. Rev. Mar. Sci. 4, 237–262. doi: 10.1146/annurev-marine-120709-142737
Gooday A. J., Rathburn A. E. (1999). Temporal Variability in Living Deep-Sea Benthic Foraminifera: A Review. Earth Sci. Rev. 46, 187–212. doi: 10.1016/S0012-8252(99)00010-0
Hammer Ø., Harper D. A. T., Ryan P. D. (2001). Past: Paleontological Statistics Software Package for Education and Data Analysis. Palaeontol. Electron. 4, 9.
Jorissen F. J., de Stigter H. C., Widmark J. G. V. (1995). A Conceptual Model Explaining Benthic Foraminiferal Microhabitats. Mar. Micropaleontol. 26, 3–15. doi: 10.1016/0377-8398(95)00047-X
Jorissen F. J., Fontanier C., Thomas E. (2007). “Paleoceanographical Proxies Based on Deep-Sea Benthic Foraminiferal Assemblage Characteristics,” in Proxies in Late Cenozoic Paleoceanography: Pt. 2: Biological Tracers and Biomarkers. Eds. Hillaire-Marcel C., de Vernal A., (Amsterdam, The Netherlands: Elsevier) 263–325. doi: 10.1016/S1572-5480(07)01012-3
Knoppers B., Ekau W., Figueiredo A. G. (1999). The Coast and Shelf of East and Northeast Brazil and Material Transport. Geo-Marine. Lett. 19, 171–178. doi: 10.1007/s003670050106
Kurbjeweit F., Schmiedl G., Schiebel R., Hemleben C., Pfannkuche O., Wallmann K., et al. (2000). Distribution, Biomass and Diversity of Benthic Foraminifera in Relation to Sediment Geochemistry in the Arabian Sea. Deep. Res. Part II Top. Stud. Oceanogr. 47, 2913–2955. doi: 10.1016/S0967-0645(00)00053-9
Lambshead P. J. D., Gooday A. J. (1990). The Impact of Seasonally Deposited Phytodetritus on Epifaunal and Shallow Infaunal Benthic Foraminiferal Populations in the Bathyal Northeast Atlantic: The Assemblage Response. Deep Sea Res. Part A Oceanogr. Res. Pap. 37:1263-1283. doi: 10.1016/0198-0149(90)90042-T
Legendre L., Legendre P. (1998). Numerical Ecology 2nd ed Volume 24 (Amsterdam, The Netherlands: Elsevier Science).
Linke P., Lutze G. F. (1993). Microhabitat Preferences of Benthic Foraminifera – a Static Concept or a Dynamic Adaptation to Optimize Food Acquisition? Mar. Micropaleontol. 20, 215–234. doi: 10.1016/0377-8398(93)90034-U
Lohmann G. P. (1978). Abyssal Benthonic Foraminifera as Hydrographic Indicators in the Western South Atlantic Ocean. J. Foraminifer. Res. 8, 6–34. doi: 10.2113/gsjfr.8.1.6
Lutze G. F., Thiel H. (1989). Epibenthic Foraminifera From Elevated Microhabitats; Cibicidoides Wuellerstorfi and Planulina Ariminensis. J. Foraminifer. Res. 19, 153–158. doi: 10.2113/gsjfr.19.2.153
Mackensen A. (1987). Benthische Foraminiferen Auf Dem Island-Schottland Rücken: Umwelt-Anzeiger an Der Grenze Zweier Ozeanischer Räume. Paläontologische. Z. 61, 149–179. doi: 10.1007/BF02985902
Mackensen A., Grobe H., Kuhn G., Fütterer D. K. (1990). Benthic Foraminiferal Assemblages From the Eastern Weddell Sea Between 68 and 73°S: Distribution, Ecology and Fossilization Potential. Mar. Micropaleontol. 16, 241–283. doi: 10.1016/0377-8398(90)90006-8
Mackensen A., Schmiedl G., Harloff J., Giese M. (1995). Deep-Sea Foraminifera in the South Atlantic Ocean: Ecology and Assemblage Generation. Micropaleontology 41, 342–358. doi: 10.2307/1485808
Margreth S., Rüggeberg A., Spezzaferri S. (2009). Benthic Foraminifera as Bioindicator for Cold-Water Coral Reef Ecosystems Along the Irish Margin. Deep. Res. Part I Oceanogr. Res. Pap. 56, 2216–2234. doi: 10.1016/j.dsr.2009.07.009
Martins L. R., Coutinho P. N. (1981). The Brazilian Continental Margin. Earth Sci. Rev. 17, 87–107. doi: 10.1016/0012-8252(81)90007-6
Mémery L., Arhan M., Alvarez-Salgado X. A., Messias M. J., Mercier H., Castro C. G., et al. (2000). The Water Masses Along the Western Boundary of the South and Equatorial Atlantic. Prog. Oceanogr. 47, 69–98. doi: 10.1016/S0079-6611(00)00032-X
Mendes I., Dias J. A., Schönfeld J., Ferreira O. (2012). Distribution of Living Benthic Foraminifera on the Northern Gulf of Cadiz Continental Shelf. J. Foraminifer. Res. 42, 18–38. doi: 10.2113/gsjfr.42.1.18
Montagu G., (1803). Testacea Britannica, or Natural History of British Shells, Marine, Land and Fresh Water, Including the Most Minute: Systematically Arranged and Embellished with Figures.J. White, London, England, 606 pp. doi: 10.5962/bhl.title.33927
Murray J. W. (2006). Ecology and Applications of Benthic Foraminifera. Cambridge University Press, Cambridge. 426 pp.
Nagel B., Gaye B., Kodina L. A., Lahajnar N. (2009). Stable Carbon and Nitrogen Isotopes as Indicators for Organic Matter Sources in the Kara Sea. Mar. Geol. 266, 42–51. doi: 10.1016/j.margeo.2009.07.010
Oliveira-Silva P., Fernandes Barbosa C., Soares-Gomes A. (2005). Distribution of Macrobenthic Foraminifera on Brazilian Continental Margin Between 18°S – 23°S. Rev. Bras. Geociências. 35, 209–216. doi: 10.25249/0375-7536.2005352209216
Paliy O., Shankar V. (2016). Application of Multivariate Statistical Techniques in Microbial Ecology. Mol. Ecol. 25, 1032–1057. doi: 10.1111/mec.13536
Parr W. J. (1932). Victorian and South Australian Shallow-Water Foraminifera. Part I.Proceedings of the Royal Society of Victoria 44, 1–14.
Peet R. K. (1974). The Measurement of Species Diversity. Annu. Rev. Ecol. Syst. 5, 285–307. doi: 10.2110/scn.79.06.0003
Peterson R. G., Stramma L. (1991). Upper-Level Circulation in the South Atlantic Ocean. Prog. Oceanogr. 26, 1–73. doi: 10.1016/0079-6611(91)90006-8
Raddatz J., Titschack J., Frank N., Freiwald A., Conforti A., Osborne A., et al. (2020). Solenosmilia Variabilis-Bearing Cold-Water Coral Mounds Off Brazil. Coral. Reefs 39, 69–83. doi: 10.1007/s00338-019-01882-w
Ramette A. (2007). Multivariate Analyses in Microbial Ecology. FEMS Microbiol. Ecol. 62, 142–160. doi: 10.1111/j.1574-6941.2007.00375.x
Rasmussen T. L., Thomsen E. (2017). Ecology of Deep-Sea Benthic Foraminifera in the North Atlantic During the Last Glaciation: Food or Temperature Control. Palaeogeogr. Palaeoclimatol. Palaeoecol. 472, 15–32. doi: 10.1016/j.palaeo.2017.02.012
Rebouças R. C., Dominguez J. M. L., da Silva Pinto Bittencourt A. C. (2011). Provenance, Transport and Composition of Dendê Coast Beach Sands in Bahia, Central Coast of Brazil. Braz. J. Oceanogr. 59, 339–347. doi: 10.1590/S1679-87592011000400004
Rumolo P., Barra M., Gherardi S., Marsella E., Sprovieri M. (2011). Stable Isotopes and C/N Ratios in Marine Sediments as a Tool for Discriminating Anthropogenic Impact. J. Environ. Monit. 13, 3399–3408. doi: 10.1039/c1em10568j
Ryan W.B.F., Carbotte S.M., Coplan J.O., O’Hara S., Melkonian A, Arko R, et al. (2009).Global Multi-Resolution Topography Synthesis. Geochemistry, Geophys. Geosystems 10, 1–9. doi:10.1029/2008GC002332
Schmiedl G., Mackensen A., Müller P. J. (1997). Recent Benthic Foraminifera From the Eastern South Atlantic Ocean: Dependence on Food Supply and Water Masses. Mar. Micropaleontol. 32, 249–287. doi: 10.1016/S0377-8398(97)00023-6
Schönfeld J. (1997). The Impact of the Mediterranean Outflow Water (MOW) on Benthic Foraminiferal Assemblages and Surface Sediments at the Southern Portuguese Continental Margin. Mar. Micropaleontol. 29, 21–236. doi: 10.1016/S0377-8398(96)00050-3
Schönfeld J. (2002a). Recent Benthic Foraminiferal Assemblages in Deep High-Energy Environments From the Gulf of Cadiz (Spain). Mar. Micropaleontol. 44, 141–162. doi: 10.1016/S0377-8398(01)00039-1
Schönfeld J. (2002b). A New Benthic Foraminiferal Proxy for Near-Bottom Current Velocities in the Gulf of Cadiz, Northeastern Atlantic Ocean. Deep. Res. I 49, 1853–1875. doi: 10.1016/S0967-0637(02)00088-2
Schönfeld J., Zahn R. (2000). Late Glacial to Holocene History of the Mediterranean Outflow. Evidence From Benthic Foraminiferal Assemblages and Stable Isotopes at the Portuguese Margin. Palaeogeogr. Palaeoclimatol. Palaeoecol. 159, 85–111. doi: 10.1016/S0031-0182(00)00035-3
Sen Gupta B. K. (Ed.) (2002). Modern Foraminifera (Dordrecht, The Netherlands: Kluwer Academic Publishers), 384.
Shannon C. E, Weaver W. (1949). The Mathematical Theory of Communication. University of Illinois Press, Urbana, pp. 144.
Stramma L., England M. (1999). On the Water Masses and Mean Circulation of the South Atlantic Ocean. Geophys. Res. 104, 20,863–20,883. doi: 10.1029/1999JC900139
Stramma L., Fischer J., Reppin J. (1995). The North Brazil Undercurrent. Deep. Res. Part I 42, 773–795. doi: 10.1016/0967-0637(95)00014-W
Summerhayes C. P., Fainstein R., Ellis J. P. (1976). Continental Margin Off Sergipe and Alagoas, Northeastern Brazil: A Reconnaissance Geophysical Study of Morphology and Structure. Mar. Geol. 20, 345–361. doi: 10.1016/0025-3227(76)90112-2
ter Braak C. J. F., Verdonschot P. F. M. (1995). Canonical Correspondence Analysis and Related Multivariate Methods in Aquatic Ecology. Aquat. Sci. 57, 255–289. doi: 10.1007/BF00877430
Viana A. R. (2001). Seismic Expression of Shallow- to Deep-Water Contourites Along the South-Eastern Brazilian Margin. Mar. Geophys. Res. 22, 509–521. doi: 10.1023/A:1016307918182
Viana A. R., Almeida W. Jr., Almeida C. W. (2002). Upper Slope Sands: Late Quaternary Shallow-Water Sandy Contourites of Campos Basin, SW Atlantic Margin. Geol. Soc London. Mem. 22, 261–270. doi: 10.1144/GSL.MEM.2002.022.01.19
Viana A. R., Faugères J. C., Kowsmann R. O., Lima J. A. M., Caddah L. F. G., Rizzo J. G. (1998). Hydrology, Morphology and Sedimentology of the Campos Continental Margin, Offshore Brazil. Sediment. Geol. 115, 133–157. doi: 10.1016/S0037-0738(97)00090-0
Vieira F. S., Koutsoukos E. A. M., Machado A. J., Dantas M. A. T. (2015). Biofaciological Zonation of Benthic Foraminifera of the Continental Shelf of Campos Basin, SE Brazil. Quat. Int. 377, 18–27. doi: 10.1016/j.quaint.2014.12.020
Williamson W.C., (1858). On the Recent Foraminifera of Great Britain.The Ray Society, London. 1–107.
Yamashita C., Mello e Sousa S. H., Vicente T. M., Martins M. V., Nagai R. H., Frontalini F., et al. (2018). Environmental Controls on the Distribution of Living (Stained) Benthic Foraminifera on the Continental Slope in the Campos Basin Area (SW Atlantic). J. Mar. Syst. 181, 37–52. doi: 10.1016/j.jmarsys.2018.01.010
Yamashita C., Omachi C., Santarosa A. C. A., Iwai F. S., Araujo B. D., Disaró S. T., et al. (2020). Living Benthic Foraminifera of Santos Continental Shelf, Southeastern Brazilian Continental Margin (SW Atlantic): Chlorophyll-a and Particulate Organic Matter Approach. J. Sediment. Environ. 5, 17–34. doi: 10.1007/s43217-019-00001-7
Keywords: benthic foraminifera, Brazilian margin, continental slope, deep-sea, South Atlantic
Citation: Saupe A, Schmidt J, Petersen J, Bahr A, Dias BB, Albuquerque ALS, Díaz Ramos RA and Grunert P (2022) Controlling Parameters of Benthic Deep-Sea Foraminiferal Biogeography at the Brazilian Continental Margin (11-22°S). Front. Mar. Sci. 9:901224. doi: 10.3389/fmars.2022.901224
Received: 21 March 2022; Accepted: 01 June 2022;
Published: 20 July 2022.
Edited by:
Jeroen Ingels, Florida State University, United StatesReviewed by:
Andrew John Gooday, National Oceanography Centre, Southampton, United KingdomMichael Martínez-Colón, Florida Agricultural and Mechanical University, United States
Bryan O’ Malley, Eckerd College, United States
Copyright © 2022 Saupe, Schmidt, Petersen, Bahr, Dias, Albuquerque, Díaz Ramos and Grunert. This is an open-access article distributed under the terms of the Creative Commons Attribution License (CC BY). The use, distribution or reproduction in other forums is permitted, provided the original author(s) and the copyright owner(s) are credited and that the original publication in this journal is cited, in accordance with accepted academic practice. No use, distribution or reproduction is permitted which does not comply with these terms.
*Correspondence: Anna Saupe, YW5uYS5zYXVwZUB1bmkta29lbG4uZGU=