- 1Marine Laboratory, University of Guam, Mangilao, GU, United States
- 2School of Science, Technology, and Engineering, University of the Sunshine Coast, Sippy Downs, QLD, Australia
In the tropics, crustose calcifying red algae (Corallinophycidae and Peyssonneliales; CCRA) are dominant and important reef builders that serve a suite of ecological functions affecting reef health. However, CCRA taxa have historically been overlooked in floristic and ecological studies because of their high degrees of phenotypic plasticity and morphological convergence that impede reliable identifications based on morphology. This study provides an update of the CCRA diversity of Guam (Mariana Islands) based on a recent DNA barcoding effort. This account of CCRA taxa is compared to (1) the most current species inventories for Guam based on morphological identifications and (2) similar floristic accounts of CCRA from other regions using DNA barcoding. 492 CCRA specimens were collected from Guam for which two markers, COI-5P and psbA, were used for phylogenetic analysis and species delimitation. Phylogenetic relationships were inferred using maximum likelihood. Species richness estimates were obtained through a conservative approach using the Automatic Barcode Gap Discovery method for species delimitation. A total of 154 putative CCRA species were identified, with 106 representatives of the subclass Corallinophycidae and 48 belonging to the order Peyssonneliales. When compared to previous studies based on morphological identification, molecular data suggests that all but one of the CCRA species reported for Guam were incorrectly identified and CCRA species richness is more than six times higher than previously assumed. Species accumulation curves show that CCRA species richness will continue to rise with increased sampling effort and the exploration of new (micro)habitats before reaching a plateau. Guam’s true CCRA richness might eventually exceed the currently reported species richness of all marine red algae for the island. Of the 154 putative species documented in this study, only ten closely match (≥ 98% COI-5P sequence similarity) previously described species, implying that many are probably new species to science. The here-reported CCRA diversity for Guam as a small, remote tropical island in the Western Pacific Ocean is greater than those of well-documented CCRA floras for much larger nearshore ecosystems in Brazil and New Zealand, emphasizing the value of tropical islands as hotspots of marine biodiversity.
Introduction
In ecology, biodiversity has been the subject of extensive study, and has been shown to affect the stability, health, ecosystem processes, and overall performance of ecosystems (Naeem et al., 1994; Loreau et al., 2002; Tilman et al., 2006). Moreover, the implementation of new technologies (Mora et al., 2011) and the rapid changes that many ecosystems are experiencing (Hughes et al., 2003; Hughes et al., 2007) emphasize the need to further examine biodiversity worldwide, especially in the tropics (Paulay, 2003). Macroalgae are an integral component of tropical reefs and contribute significantly to the biodiversity of tropical reef ecosystems in the Pacific (Vroom, 2011). Of these, crustose calcifying red algae (CCRA) provide major ecological functions for tropical reefs. In this study, CCRA are comprised of the non-geniculate, calcified members of the Corallinophycidae and the Peyssonneliales. CCRA have long been considered as essential components of tropical reefs due to their role as reef builders and cementers (Gordon et al., 1976; Adey, 1998), as well as their significant contribution to the carbonate budget on tropical reefs (Lee and Carpenter, 2001). Some CCRA species can also act as suppressors of potentially harmful nutrient indicator algae (Vermeij et al., 2011; O’Leary et al., 2017), as well as remove nitrogenous compounds, thereby reducing the mortality of reef-building corals (Yuen et al., 2009). Moreover, some CCRA are particularly important in the early stages of colonization of bare reef substrates, as they are the dominant organisms that colonize such habitats and have been shown to serve as the preferred settlement substrates for many invertebrate larvae (Tebben et al., 2015; Vargas-Ángel et al., 2015; Deinhart et al., 2022). Other CCRA taxa, however, can have vastly different ecological roles on reefs. Some members of the order Peyssonneliales, for example, flourish on disturbed reefs, outcompeting and overgrowing entire reef communities (Verlaque et al., 2000; Pueschel and Saunders, 2009; Eckrich et al., 2011; Eckrich and Engel, 2013; Nieder et al., 2019; Williams and García-Sais, 2020).
CCRA are thought to be some of the most sensitive organisms to the effects of climate change, ocean acidification, and global warming (Vásquez-Elizondo and Enríquez, 2016). Since CCRA can be more sensitive to chronic disturbances than scleractinian corals, they could be considered as sentinel taxa of tropical marine ecosystems (Fabricius and De'ath, 2001; Ries, 2011; Yamamoto et al., 2012; Mallela, 2013). This does not apply to all CCRA, however. Recent evidence suggests that some CCRA species, particularly those with shorter generation times, high phenotypic plasticity, or broad thermal tolerance, could be more capable of acclimatizing and adapting to increasing ocean temperatures (Cornwall et al., 2019). Further research demonstrated that the reef-cementing and reef-accreting Hydrolithon boergesenii (Foslie) Foslie gained tolerance to ocean acidification over six generations of exposure, suggesting that some CCRA species could maintain their ecological roles in the face of ocean acidification (Cornwall et al., 2020). It is unclear, however, if such findings can be extended to other CCRA species and how this response is influenced by other environmental factors (Cornwall et al., 2020).
Historically, CCRA have often been overlooked by phycologists and ecologists, despite their abundance and ecological importance on reefs. This can largely be attributed to their phenotypic plasticity and convergent morphologies that complicate identifications based on morphology, even at family or genus level (Steneck, 1986; Hernández-Kantún et al., 2014). Molecular phylogenies of CCRA have shown that morpho-anatomical features alone often fail to correctly identify CCRA to species and sometimes to genus-level (Sissini et al., 2014; Gabrielson et al., 2018). However, DNA-based identification has proven to be an effective method to identify CCRA species in diversity, biogeographic, and ecological studies (Sherwood et al., 2010; Hernández-Kantún et al., 2014; Hind et al., 2014; Peña et al., 2014; Sissini et al., 2014; Gabrielson et al., 2018; Manghisi et al., 2019; Sherwood et al., 2020).
CCRA are among the most dominant organisms on reefs in the Mariana Islands and throughout the Pacific Islands (Schils et al., 2013), yet little is known about their diversity and the communities they form. Studies on fossil and living CCRA from Guam date back to 1964 (Johnson, 1964; Gordon, 1975; Gordon et al., 1976; Tsuda, 2003). The first study to provide a floristic account of extant CCRA in Guam was a master’s thesis by Gordon (1975) and the resulting publication (Gordon et al., 1976). In the study, CCRA specimens were collected from sites around Guam and 15 CCRA species were identified through detailed morphological and anatomical observations. The most recent inventory of CCRA was part of a checklist and bibliography on the seaweed flora of Guam and the Mariana Islands (Tsuda, 2003) and the resulting publication (Lobban and Tsuda, 2003). As it stands, 24 extant species of CCRA have been reported for Guam. Of those 24 species, two are representatives of the Peyssonneliales, one belongs to the Sporolithales, four belong to the Hapalidiales, and 17 are Corallinales. Since Lobban and Tsuda (2003), four of the reported species have been transferred to another genus, two of the species have been synonymized, and Peyssonnelia corallis Tsuda is an erroneously used, invalid name for the brown alga Lobophora variegata (J.V.Lamouroux) Womersley ex E.C.Oliveira (Guiry and Guiry, 2022). Most of the previously published CCRA records for Guam relied on thorough morphological and anatomical examination but did not use DNA-based identification. The technical challenges associated with morphological studies of these limestone-encrusted algae, their taxonomic diversity, and their high levels of cryptic diversity warrant a re-examination of Guam’s CCRA flora as these algae have played an important role in benthic community changes on Guam’s reefs in the last decade. Studies on red seaweeds in other Pacific islands, such as Hawaii and Easter Island, report endemism levels of 14-24% (Santelices and Abbott, 1987; Tsuda, 2014) and suggest that many species have much smaller distribution ranges than previously believed. Many of the 24 reported CCRA species for Guam have type localities in far off regions like the Caribbean Sea, Atlantic Ocean, or Mediterranean Sea, stressing the need for a floristic update. As such, the intent of this study is to provide a much-needed review of the taxonomic diversity of CCRA on Guam, as well as a revision of the CCRA flora as reported by Gordon et al. (1976) based on morphology.
Methods
Study area
The island of Guam (13°28’N, 144°46’E) is an organized, unincorporated territory of the United States of America and it is the southernmost island of the Mariana Archipelago (Figure 1). Guam has a diverse geology and topography, with northern Guam comprised primarily of flat, uplifted limestone and southern Guam consisting of volcanic hills. The island is surrounded by fringing reefs that have developed into a barrier reef at its southern tip (i.e., Cocos Lagoon). Guam’s nearshore marine ecosystems also contain patch reefs, seagrass beds, and mangrove stands. Habitats differ distinctly between the east and west coasts, largely due to differences in current, wind, and wave exposure. Prevailing winds and typhoons originate from the east, making eastern reefs more exposed than western reefs (Paulay, 2003). The north equatorial current is thought to split around Guam such that one part sweeps along the east coast, travelling around the southern tip of the island, and flowing up the bottom half of the west coast before joining with the other stream roughly halfway up the coast line (Emery, 1962). Despite the increased exposure, reefs on the east coast have higher rugosity, habitat complexity, live coral cover, and fish diversity and abundance than western reefs (Randall and Holloman, 1974; Randall and Eldredge, 1976; Burdick et al., 2008).
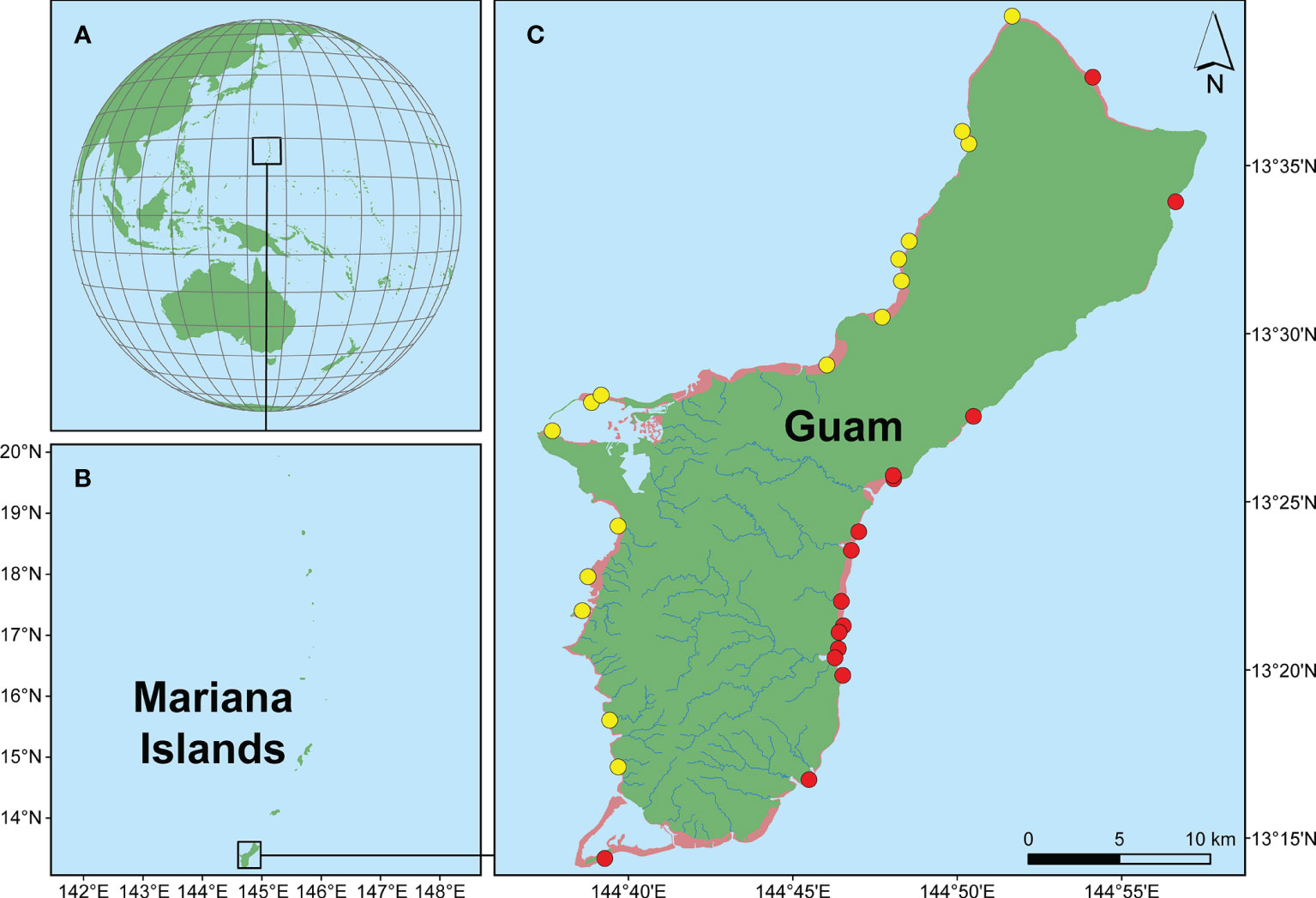
Figure 1 Maps indicating the study area and sample collection sites. (A) Pacific-centered map showing the location of the Mariana Islands. (B) Map of the Mariana Islands with Guam as the largest and southernmost island. (C) Map of Guam identifying the 31 sites from which CCRA were collected, separated into eastern sites (red dots) and western sites (yellow dots). Scale bar = 10 km.
Specimen collection and preservation
492 CCRA specimens were selectively and opportunistically collected from 31 sites around Guam (Figure 1; Supplementary Table 1). Sampling was directed toward maximizing the number of different CCRA morphotypes in an attempt to cover a broad range of CCRA taxa. Because the initial study of Guam’s CCRA (Gordon et al., 1976) contained detailed collection information of the studied specimens, 12 of the 15 reported species were re-collected based on the habit and gross morphology as described in Gordon et al. (1976). Most species were re-collected from the same locations as reported by Gordon et al. (1976), with only two species that were collected from other sites (Table 1). The specimens were collected by hand or with a hammer and chisel while SCUBA diving or freediving (<25 m depth). Photographs of each specimen were taken in situ prior to collection, as well as ex situ following the removal of tissue used for DNA extraction. In addition to these photographs, all other appropriate metadata (e.g., herbarium accession number, site information, GPS coordinates, etc.) were recorded for each specimen. Specimens were stored separately upon collection and transferred to holding tanks with running seawater to keep them alive until DNA extraction could be performed. Upon completion of DNA extractions, all samples were added to the herbarium collection of the University of Guam (GUAM; Thiers, 2016) as air-dried, silica-dried, and formalin-preserved specimens and were individually stored in custom-made clear acrylic boxes.
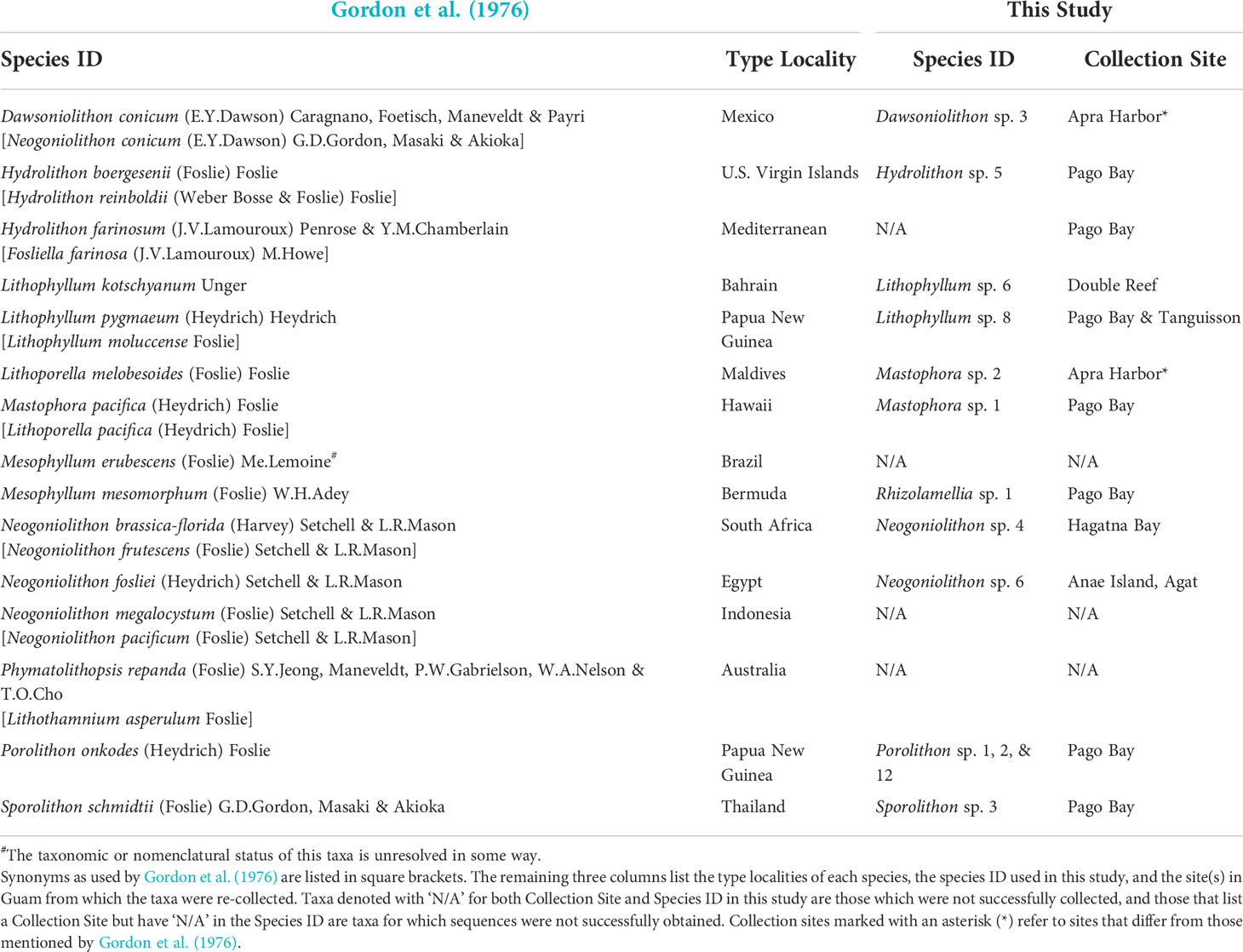
Table 1 Currently accepted names of CCRA species (Guiry and Guiry, 2022) reported for Guam by Gordon et al. (1976).
DNA extraction
Each CCRA specimen was carefully examined to find an area visibly free of epiphytes, which was then swabbed using 10% bleach or 95% ethanol to remove potential surface contaminants. CCRA tissue was collected by using a Dremel rotary tool, a pair of tweezers, or a single-edged razor blade (single use) to scrape off minimal amounts of tissue from specimens. To prevent sample contamination, the Dremel drill bit (or tweezers) were soaked in 10% bleach, 100% ethanol, and burned between each use. Total genomic DNA from CCRA tissue was extracted using either the QIAGEN DNeasy Blood & Tissue Kit (Qiagen Inc., Valencia, CA) or the GenCatch Blood & Tissue Genomic Mini Prep Kit (Epoch Life Science Inc., Missouri City, TX) following the manufacturer’s bench protocol. The extracted DNA was stored at 4°C until the polymerase chain reaction (PCR) was successful, after which it was stored at -20°C.
Polymerase chain reaction (PCR)
Two genetic markers were PCR amplified for DNA barcoding (species delimitation and taxon identification). The mitochondrial cytochrome c oxidase subunit 1 DNA barcode region, or COI-5P (roughly 664 bp), was the primary marker used for CCRA barcoding and species delimitation due to its proven accuracy for species identification (Sherwood et al., 2010; Dixon and Saunders, 2013; Hind and Saunders, 2013; Manghisi et al., 2019). COI-5P is the primary marker used in the barcode of life database (BOLD) but can be difficult to amplify for CCRA. Therefore, different primer combinations were tested to amplify COI-5P for every specimen. The primers used include GWSFn and GWSRx, the primers utilized by Saunders and McDevit (2012), as well as TS_COI_F01_10 (Mills and Schils, 2021). COI-5P was amplified following the amplification profile 95°C for 3 minutes; 35 cycles of 94°C for 40 seconds, annealing at 48°C for 40 seconds, extension at 72°C for 1:40 minutes; and a final extension at 72°C for 10 minutes.
The chloroplast photosystem II thylakoid membrane protein D1, or psbA (roughly 950 bp) was also used for CCRA barcoding and species delimitation due to its high amplification success. This marker is often used in CCRA barcoding and identification studies (Broom et al., 2008; Carro et al., 2014; Maneveldt et al., 2019). PsbA is more conserved than COI-5P and provides additional support for deeper nodes of the CCRA phylogeny. The gene was amplified using the primers psbAF and psbAR2, developed by Yoon et al. (2002) following the amplification profile 95°C for 3 minutes; 35 cycles of 94°C for 40 seconds, annealing at 50°C for 40 seconds, extension at 72°C for 1:40 minutes; a final extension step at 72°C for 10 minutes.
DNA sequencing and sequence analysis
PCR products were sent to Macrogen Inc. (Seoul, Republic of Korea) for DNA sequencing. Consensus sequences were generated from the forward and reverse reads, which were then compared to a database of available CCRA specimens via BLAST search or via the Barcode of Life Database (BOLD; Ratnasingham and Hebert, 2007). All sequence data were archived and analyzed using the Geneious Pro 11.0.5 computer software (https://www.geneious.com; Kearse et al., 2012).
CCRA specimens belonging to the order Peyssonneliales and the subclass Corallinophycidae were analyzed separately, and alignments for each of the gene regions were created using the MUSCLE plugin (Edgar, 2004) in Geneious Pro 11.0.5. Partitioned maximum likelihood analyses of the concatenated two-locus alignments (COI-5P and psbA) of the Corallinophycidae and Peyssonneliales were run in IQ-TREE (Minh et al., 2020). ModelFinder (Kalyaanamoorthy et al., 2017) was used to identify the best-fit substitution model for each locus. Ultrafast bootstrap approximations (UFBoot; 1,000 bootstrap replicates) were calculated to evaluate branch support in a single IQ-TREE run. Two independent single-locus methods were used to assess CCRA diversity and delimitate putative species: distance-based Barcode-Gap analyses (Hebert et al., 2003; Meier et al., 2008) and the tree-based Bayesian implementation of the Poisson Tree Processes (bPTP) model (Zhang et al., 2013). Recent studies investigating cryptic diversity in CCRA, and other red algae have often reported a 2-4% COI-5P barcode-gap between species (Saunders, 2008; Dixon and Saunders, 2013; Hind and Saunders, 2013; Hind et al., 2014). Automatic Barcode Gap Discovery (ABGD; Puillandre et al., 2012) was performed using the default parameters for both COI-5P and psbA to help delimitate putative species in this study. Taxa that matched sequences of described species (≥ 98% COI-5P similarity) were identified as those species. All other taxa were identified to the highest possible taxonomic resolution using BOLD, GenBank, and by comparing the sequences generated for this study against those used in recent large-scale taxonomic and phylogenetic investigations of the Corallinophycidae (Peña et al., 2020) and the Peyssonneliales (Pestana et al., 2021) based on the phylogenetic species concept.
Rarefaction and extrapolation curves
Sample‐size‐based rarefaction-and-extrapolation curves, which depict the relationship between species richness estimates and sample size (number of specimens sequenced), were computed in the statistical software environment R (R Core Team, 2022) using the R package iNEXT (Hsieh et al., 2016). Hill number of order zero (q = 0) were used to interpolate and predict species richness based on the number of specimens sequenced. The function ggiNEXT was used to plot the rarefaction-and-extrapolation curves.
Results
DNA sequences were obtained for 492 CCRA specimens. Of those, 341 specimens belonged to the subclass Corallinophycidae, and 151 specimens belonged to the order Peyssonneliales (Table 2). ModelFinder identified GTR+F+I+G4 as the best-fit model for both partitions in the Corallinophycidae alignment. GTR+F+G4 (COI-5P) and GTR+F+I+G4 (psbA) was the best-fit partition model for the Peyssonneliales. Both ABGD analyses of COI-5P and psbA alignments resolved a total of 122 and 125 distinct species respectively, with a barcode gap of 2-3% interspecific sequence divergence for COI-5P and ~1.5% for psbA. Species delimitation based on the bPTP model was largely concordant with the ABGD results but resolved a few more cryptic species for both COI-5P (135 species) and psbA (134 species) than the distance-based approach. The species delimitation results of the ABGD and bPTP analyses of COI-5P and psbA supported each other, though there were rare disagreements when the bPTP model identified cryptic sister species or species complexes (Mastophora rosea (C.Agardh) Setchell and Hydrolithon sp. 5) where ABGD identified a single species (Figures 2, 3, in bold type). On the few occasions where the two analyses disagreed, the more conservative ABGD results were used to define species boundaries. Both genes were not successfully sequenced for all specimens, so a combined analysis of both genes resulted in the recognition of 154 putative CCRA species. DNA sequence data suggested that the 341 specimens belonging to the Corallinophycidae comprised 106 putative species (Figure 2), while the 152 Peyssonneliales specimens represented 48 putative species (Figure 3). However, there were a few instances where the ABGD and bPTP analyses of COI-5P resolved a group of closely related taxa as separate sister-species, while the analysis of psbA resolved them as a species complex. In the few cases where the two analyses did not agree, COI-5P results were followed because this marker is less conserved than psbA and serves as a benchmark for species delimitation in red algae (Sherwood et al., 2010; Dixon and Saunders, 2013; Manghisi et al., 2019). For all trees (Figures 2, 3), bootstrap support values are shown on nodes.
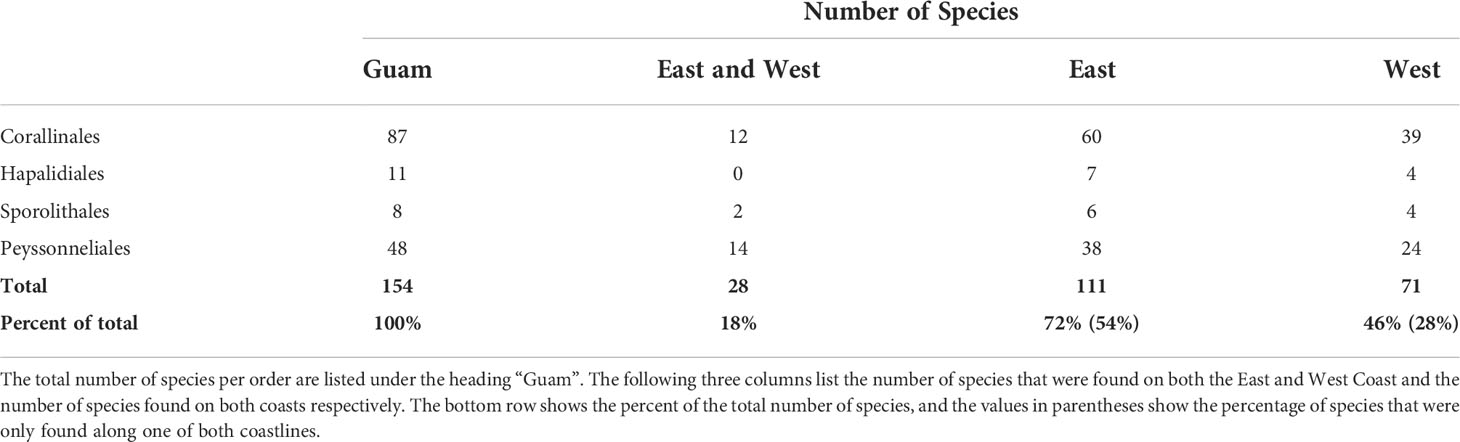
Table 2 Breakdown of the number of CCRA species at ordinal level and the coastline that they were collected from.
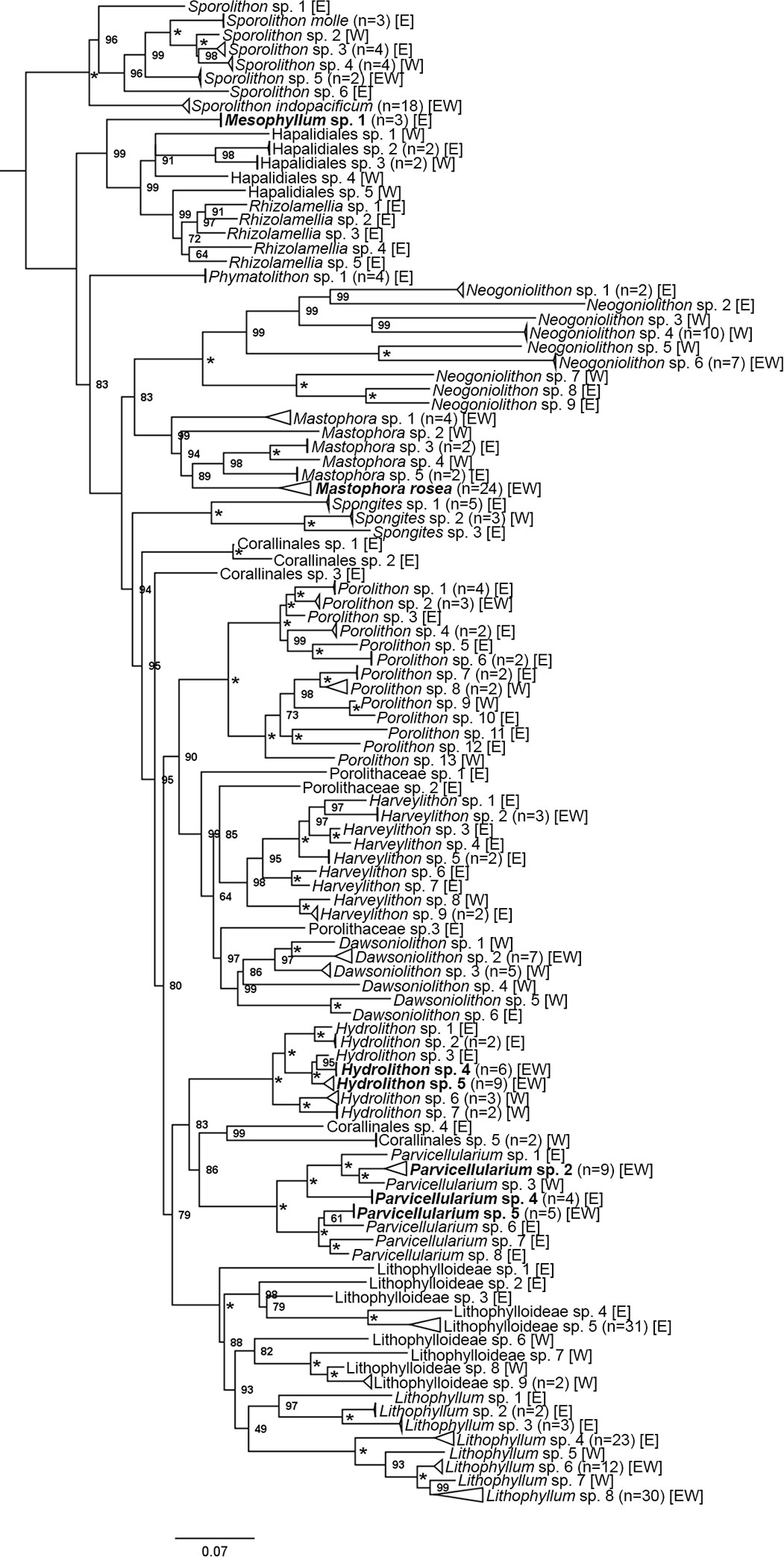
Figure 2 Maximum likelihood phylogenetic tree of concatenated COI-5P and psbA sequences for all specimens in the subclass Corallinophycidae collected during this study with bootstrap support values located at the nodes. Asterisks (*) denote nodes with full support. The side of the island that each species was found is shown using [E] for east side, [W] for west side, and [EW] for both sides. Tips denote putative species (n=106). Species recognized by ABGD, but split into one or two additional sister species by bPTP species delimitation are in bold type. Batrachospermum gelatinosum (Linnaeus) De Candolle was used as the outgroup and pruned from the depicted tree.
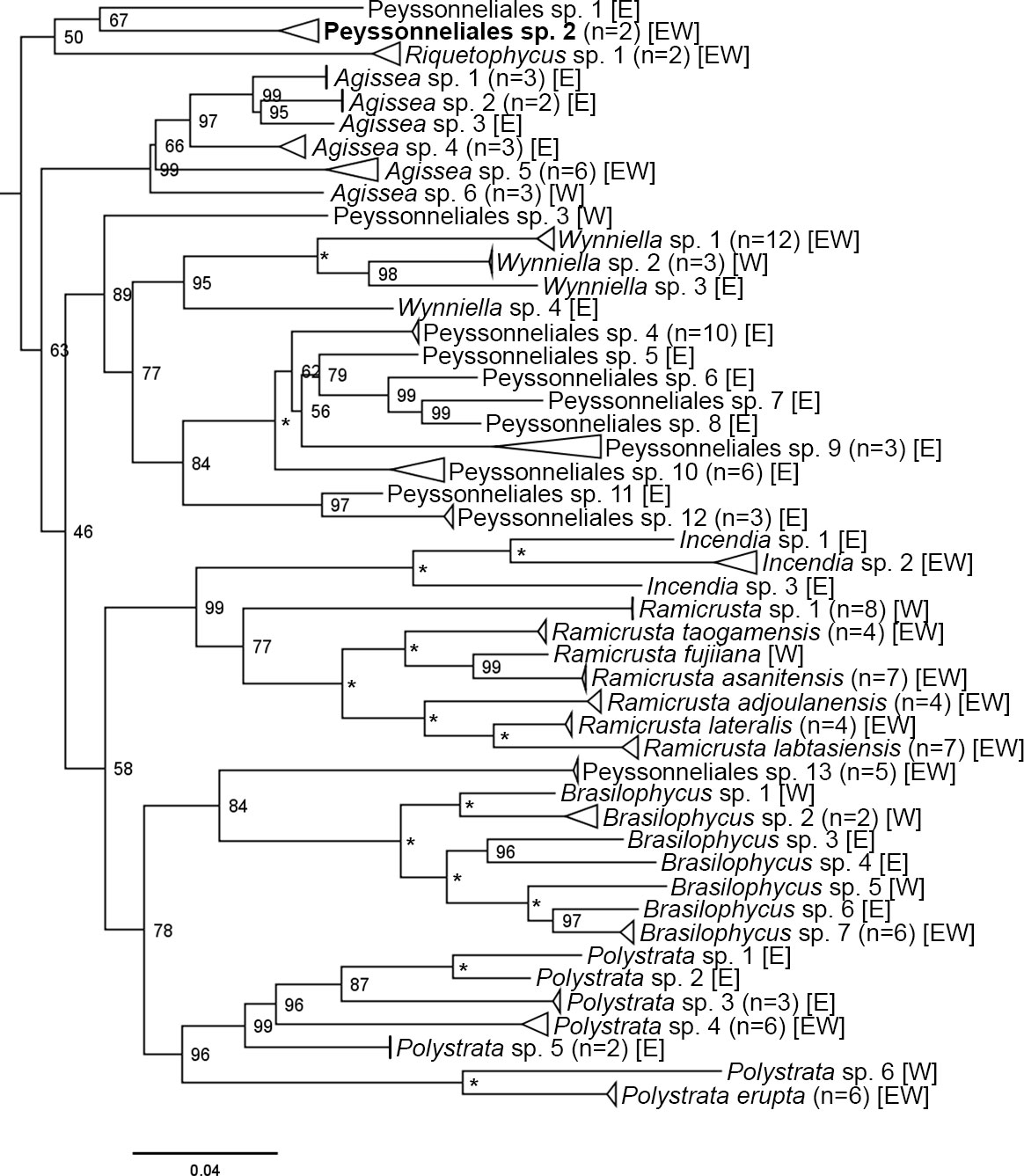
Figure 3 Maximum likelihood phylogenetic tree of concatenated COI-5P and psbA sequences for all specimens collected during this study belonging to the order Peyssonneliales with bootstrap support values located at the nodes. Asterisks (*) denote nodes with full support. The side of the island that each species was found is shown using [E] for east side, [W] for west side, and [EW] for both sides. Tips denote putative species (n=48). Species recognized by ABGD, but split into one or two additional sister species by bPTP species delimitation are in bold type. Plocamium cartilagineum (Linnaeus) P.S.Dixon was used as the outgroup and pruned from the depicted tree.
Discussion
Specimens of the same apparent taxa from largely the same locations as in Gordon et al. (1976) were collected to compare morphospecies identifications with molecular species identifications. Of the 15 species, 12 were able to be re-collected, 10 of which were collected from the same locations as in Gordon et al. (1976), and sequence data were obtained for 11 of the 12 species. Porolithon onkodes (Heydrich) Foslie, thought to be the one of Guam’s most dominant and important CCRA species identified by Gordon et al. (1976), was found to be comprised of three separate cryptic species based on molecular data. In fact, DNA sequence analysis suggested that none of the 11 re-collected and sequenced CCRA species initially identified morphologically by Gordon et al. (1976) matched any of the molecularly-identified species of this DNA barcoding effort (Table 1). DNA sequence analysis validated only one of the 24 CCRA species listed in the most recent checklist of Guam’s seaweed flora (Lobban and Tsuda, 2003). This species is Mastophora rosea (C.Agardh) Setchell, for which Guam is the type locality. The differences between molecular and morphospecies identification observed further reiterate the unreliability of morphoanatomical identification and the utility of DNA based identification in revealing cryptic diversity among CCRA (Carro et al., 2014; Sissini et al., 2014; Pezzolesi et al., 2016; Gabrielson et al., 2018; Maneveldt et al., 2019; Peña and Torres, 2021). The 106 Corallinophycidae species detected in this study represent members of the orders Corallinales (87 spp.), Hapaladiales (11 spp.), and Sporolithales (8 spp.). This is more than a quadruple increase of the currently reported 22 species of crustose calcifying representatives of the Corallinophycidae from Guam. In addition, the 48 detected Peyssonneliales species represent a more than 20-fold increase of the previous two species reported for Guam. Overall, the 154 species recognized in this study represent more than a six-fold increase in CCRA species previously listed for Guam. However, the sample-size-based rarefaction-and-extrapolation curves (Figure 4) show that the true CCRA species richness of Guam is probably substantially higher as the gain in CCRA species has not begun to level off with increased sampling effort. If the species richness of Guam’s CCRA would approach 300 as predicted by the extrapolation curve, the red algal diversity of Guam (currently at 356 species; Lobban and Tsuda, 2003) would almost double.
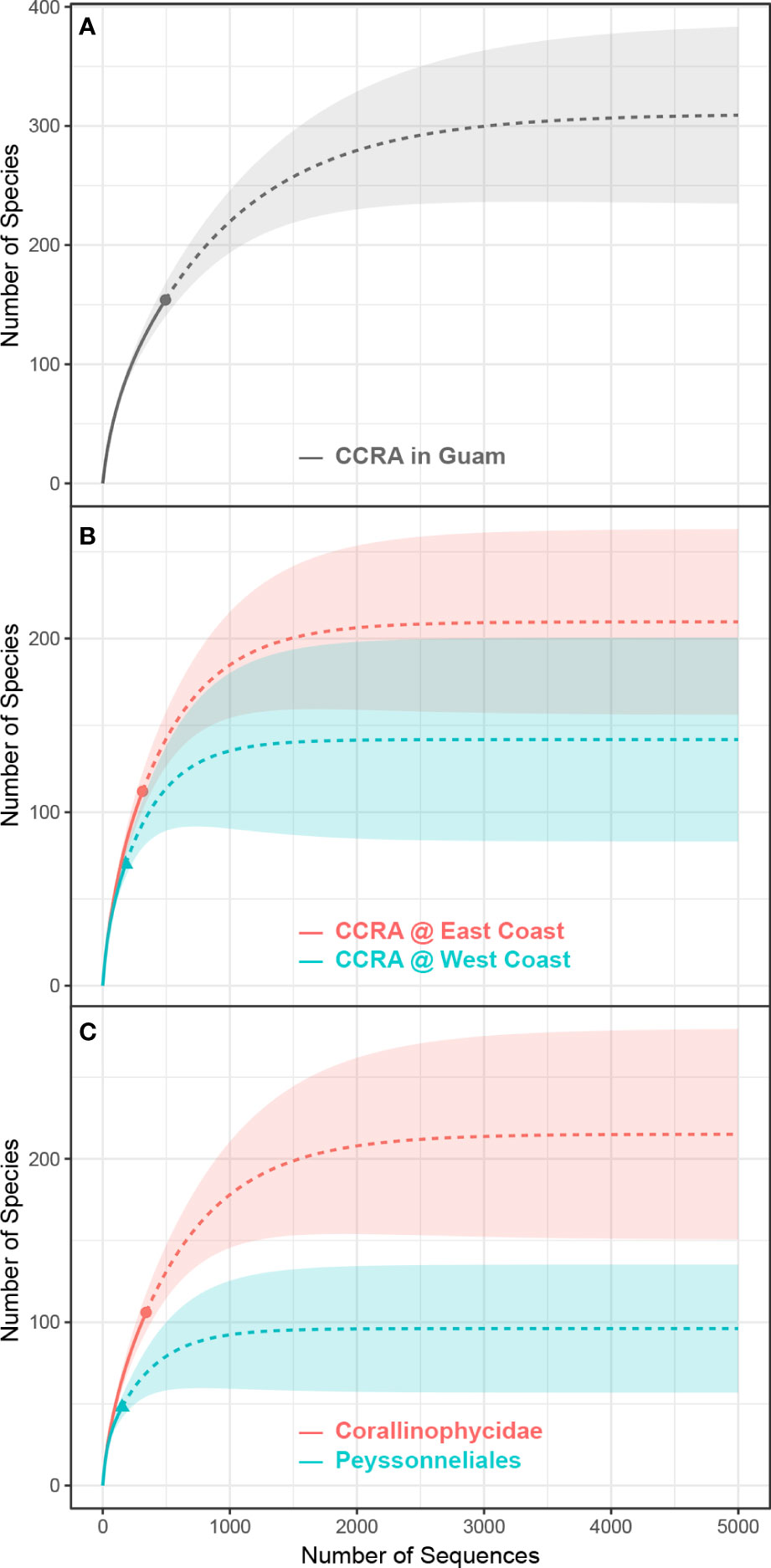
Figure 4 Sample-size-based rarefaction (solid line) and extrapolation (dashed line) curves. (A) Rarefaction-and-extrapolation curve of the number of sequenced specimens (x-axis) versus CCRA species richness (y-axis) for the whole of Guam. The grey dot indicates the total number of specimens sequenced and the overall species richness. (B) Rarefaction-and-extrapolation curve of the number of sequenced specimens (x-axis) versus CCRA species richness (y-axis) for the east and west coast of Guam. The red and green dots indicate the total number of specimens sequenced and the species richness for the east and west coast, respectively. (C) Rarefaction-and-extrapolation curve of the number of sequenced specimens (x-axis) versus CCRA species richness (y-axis) of the Corallinophycidae and Peyssonneliales. The red and green dots indicate the total number of specimens sequenced and the species richness of the Corallinophycidae and Peyssonneliales, respectively.
Of the 154 species found in this study, only ten of them matched (≥ 98% COI-5P similarity) sequences of described species, four of those were only described from Guam last year (Mills and Schils, 2021), suggesting that many of these CCRA species are possibly new to science. These findings are also consistent with recent studies that revealed extensive degrees of endemism and cryptic diversity within Guam’s seaweed flora (Verbruggen and Schils, 2012; Gabriel et al., 2017; Leliaert et al., 2018; Gabriel et al., 2019; Mills and Schils, 2021; Vieira et al., 2022). Additionally, the CCRA diversity reported herein is greater than those of much larger coastal and oceanic ecosystems, including those documented in recent studies in New Zealand and Brazil (Twist et al., 2019; Sissini et al., 2021), in other barcoding blitzes in Hawaii, Taiwan, Tunisia, and northern Madagascar (Sherwood et al., 2010; Liu et al., 2018; Manghisi et al., 2019; Vieira et al., 2021), and in comprehensive checklists of seaweeds from México and the Philippines (Lastimoso and Santiañez, 2020; Pedroche and Sentíes, 2020). The comparatively higher CCRA diversity for the small, remote western Pacific island of Guam emphasizes the conservation value of tropical islands as hotspots of marine biodiversity. Although based on different taxonomic concepts (phylogeny versus morphology), the high cryptic diversity and the potentially high levels of endemism in CCRA corroborate ecological studies that revealed pronounced differences in (1) the community composition of seaweed assemblages (Schils et al., 2013) and (2) the floristic diversity (Schils and Guiry, 2016) between islands of different marine ecoregions in the tropical Pacific. Even at a smaller geographical scale, the distribution of CCRA taxa seems to be structured. This structure could be influenced by any number of factors, including habitat diversity and availability, species interactions, environmental factors, macro-ecological forces, and geographic isolation (Schils et al., 2013; Selkoe et al., 2016). Despite being similar in length (~70 km as a smoothened contour line), the east (windward) coast of Guam appears to have a higher CCRA species richness than the west (leeward) coast, possibly due to a higher (micro)habitat diversity along the east coast (Randall and Holloman, 1974; Randall and Eldredge, 1976; Paulay, 2003; Burdick et al., 2008). In addition, a high number of CCRA species seems to be restricted to either coast (82%, or 126 out of 154 species), with only a small number of shared species that were collected along the east and west coast (18%, or 28 out of 154 species). Further sampling is necessary to validate these hypotheses. Approximately 50% of the 154 species were represented by a single specimen. Similar results have been reported in recent studies of CCRA diversity in New Zealand and Brazil, indicating the need to increase sampling effort to detect more and to better document the distribution of these potentially rare species (Twist et al., 2019; Sissini et al., 2021).
While this study comprised 492 CCRA specimens collected from 31 sites surrounding the rather small island of Guam (549 km2), it represents a modest sampling effort at best. Because of the opportunistic nature of specimen collection, long stretches of reef were not sampled. Moreover, despite being found as deep as the mesophotic and rariphotic zones (Littler et al., 1985; Richards et al., 2018), all CCRA specimens were collected from reef flats and shallow (<25 m) nearshore reef ecosystems. Species accumulation curves (Figure 4) show that Guam’s CCRA species richness will continue to grow with increased sampling effort and the exploration of new (micro)habitats. However, it is expected that more complete sampling of Guam’s shallow reefs and expanding sampling to greater depths will result in even higher CCRA species richness than currently predicted in the species extrapolation curves. On a global scale, the discovery of 154 CCRA species corresponds to 27% of all currently described and accepted non-geniculate members of the Corallinophycidae (449 spp.) and Peyssonneliales (134 spp.; Guiry and Guiry, 2022). Based on the current data, the species discovery curve is expected to rise to about 300 species for Guam. Guam is a rather small island with a total shoreline length of 244 km. Considering this, the global CCRA species diversity is expected to be orders of magnitude higher than the currently known 538 species, emphasizing the need to further investigate the diversity of this ecologically important group.
The results of this study serve to emphasize just how little is known about the diversity of Guam’s CCRA flora, despite their ecological significance and dominant benthic cover on tropical reefs. A better understanding of Guam’s CCRA communities could improve the overall understanding of ecosystem processes and community dynamics of tropical reef systems. More accurate estimates of Guam’s CCRA diversity can only be reached through increased collection from more collection sites and depth zones to obtain a broader geographical and more complete (micro)habitat representation. This study serves not only as a testament to the utility of DNA-based identification in examining CCRA diversity, but also as an important first step toward better understand this dominant, ecologically important, and often overlooked group of reef builders and cementers.
Data availability statement
The datasets presented in this study can be found in online repositories. The names of the repository/repositories and accession number(s) can be found in the article/Supplementary Material.
Author contributions
Conceptualization: MM and TS. Data curation: MM, TS, MD, and MH. Formal analysis: MM, TS. Funding acquisition: MM and TS. Investigation: MM, TS, MD, and MH. Methodology: MM and TS. Project administration: MM and TS. Resources: MM and TS. Supervision: MM and TS. Validation: MM and TS. Visualization: MM and TS. Writing – original draft: MM and TS. Writing – review and editing: MM, TS, and MD. All authors contributed to the article and approved the submitted version.
Funding
This research is based upon work supported by the National Aeronautics and Space Administration (NASA; nasa.gov) and the National Science Foundation (NSF; nsf.gov) under grant numbers 80NSSC17M0052 and OIA-1946352 awarded to TS and managed through the Guam EPSCoR offices of NASA and NSF. The work for this paper was also partly funded as an award to MM by a cooperative agreement with the National Oceanic and Atmospheric Administration (NOAA; noaa.gov), Project NA14OAR4170116, which is sponsored by the University of Guam Sea Grant from NOAA Office of Sea Grant, Department of Commerce. Any opinions, findings, and conclusions or recommendations expressed in this manuscript are those of the authors and do not necessarily reflect the views of NASA, NSF, NOAA or any of their subagencies. The funders had no role in study design, data collection and analysis, decision to publish, or preparation of the manuscript.
Acknowledgments
We are grateful to the University of Guam for supporting studies to document and conserve the natural heritage of Guam and the larger Micronesian region. MM is indebted to the University of Guam and the University of the Sunshine Coast for supporting him during much of this study.
Conflict of interest
The authors declare that the research was conducted in the absence of any commercial or financial relationships that could be construed as a potential conflict of interest.
Publisher’s note
All claims expressed in this article are solely those of the authors and do not necessarily represent those of their affiliated organizations, or those of the publisher, the editors and the reviewers. Any product that may be evaluated in this article, or claim that may be made by its manufacturer, is not guaranteed or endorsed by the publisher.
Supplementary material
The Supplementary Material for this article can be found online at: https://www.frontiersin.org/articles/10.3389/fmars.2022.898308/full#supplementary-material
References
Adey W. H. (1998). Coral reefs: algal structured and mediated ecosystems in shallow, turbulent, alkaline waters. J. Phycol. 34, 393–406. doi: 10.1046/j.1529-8817.1998.340393.x
Broom J. E. S., Hart D. R., Farr T. J., Nelson W. A., Neill K. F., Harvey A. S., et al. (2008). Utility of psbA and nSSU for phylogenetic reconstruction in the corallinales based on new Zealand taxa. Mol. Phylogenet. Evol. 46, 958–973. doi: 10.1016/j.ympev.2007.12.016
Burdick D., Brown V., Asher J., Caballes C., Gawel M., Goldman L., et al. (2008). Status of the coral reef ecosystems of Guam (Guam, U.S.A.: Bureau of Statistics and Plans, Guam Coastal Management Program).
Carro B., Lopez L., Peña V., Bárbara I., Barreiro R. (2014). DNA Barcoding allows the accurate assessment of European maerl diversity: a proof-of-Concept study. Phytotaxa 190 (1), 176–189. doi: 10.11646/phytotaxa.190.1.12
Cornwall C. E., Comeau S., DeCarlo T. M., Larcombe E., Moore B., Giltrow K., et al. (2020). A coralline alga gains tolerance to ocean acidification over multiple generations of exposure. Nat. Clim. Change 10 (2), 143–146. doi: 10.1038/s41558-019-0681-8
Cornwall C. E., Diaz-Pulido G., Comeau S. (2019). Impacts of ocean warming on coralline algal calcification: meta-analysis, knowledge gaps, and key recommendations for future research. Front. Mar. Sci. 6, 186. doi: 10.3389/fmars.2019.00186
Deinhart M. E., Mills M. S., Schils T. (2022). Community assessment of crustose calcifying red algae as coral recruitment substrates. PLoS One, in press.
Dixon K. R., Saunders G. W. (2013). DNA Barcoding and phylogenetics of Ramicrusta and Incendia gen. nov., two early diverging lineages of the peyssonneliaceae (Rhodophyta). Phycologia 52, 82–108. doi: 10.2216/12-62.1
Eckrich C. E., Engel M. S. (2013). Coral overgrowth by an encrusting red alga (Ramicrusta sp.) overgrowing scleractinian corals, gorgonians, a hydrocoral, sponges, and other algae in lac bay, bonaire, Dutch Caribbean. Coral Reefs 32, 81–84. doi: 10.1007/s00338-012-0961-5
Eckrich C. E., Engel M. S., Peachey R. B. J. (2011). Crustose, calcareous algal bloom (Ramicrusta sp.) overgrowing scleractinian corals, gorgonians, a hydrocoral, sponges, and other algae in lac bay, bonaire, Dutch Caribbean. Coral Reefs 30, 131. doi: 10.1007/s00338-010-0683-5
Edgar R. C. (2004). MUSCLE: multiple sequence alignment with high accuracy and high throughput. Nucleic Acids Res. 32 (5), 1792–1797. doi: 10.1093/nar/gkh340
Emery K. O. (1962). Marine geology of Guam. Geol. Surv. Prof. Pap. 403-B, B1–B76. doi: 10.3133/pp403B
Fabricius K., De'ath G. (2001). Environmental factors associated with the spatial distribution of crustose coralline algae on the great barrier reef. Coral Reefs 19, 303–309. doi: 10.1007/s003380000120
Gabriel D., Draisma S. G. A., Schils T., Schmidt W. E., Sauvage T., Harris D. J., et al. (2019). Quite an oddity: new worldwide records of Renouxia (Rhodogorgonales, rhodophyta), including r. marerubra sp. nov. Eur. J. Phycol. 55 (2), 197–206. doi: 10.1080/09670262.2019.1670362
Gabriel D., Draisma S. G. A., Schmidt W. E., Schils T., Sauvage T., Maridakis C., et al. (2017). Beneath the hairy look: the hidden reproductive diversity of the Gibsmithia hawaiiensis complex (Dumontiaceae, rhodophyta). J. Phycol. 53 (6), 1171–1192. doi: 10.1111/jpy.12593
Gabrielson P. W., Hughey J. R., Diaz-Pulido G. (2018). Genomics reveals abundant speciation in the coral reef building alga Porolithon onkodes (Corallinales, rhodophyta). J. Phycol. 54 (4), 429–434. doi: 10.1111/jpy.12761
Gordon G. D. (1975). Floristic and distributional account of the common crustose coralline algae of Guam. [master's thesis]. [Mangilao (GU)]: University of Guam.
Gordon G. D., Masaki T., Akioka H. (1976). Floristic and distributional account of the common crustose coralline algae on Guam. Micronesica 12 (2), 247–277.
Guiry M. D., Guiry G. M. (2022) AlgaeBase (Galway: World-wide electronic publication, National University of Ireland). Available at: https://www.algaebase.org (Accessed June 4, 2022).
Hebert P. D. N., Cywinska A., Ball S. L., deWaard J. R. (2003). Biological identifications through DNA barcodes. Proc. R. Soc B 270, 313–321. doi: 10.1098/rspb.2002.2218
Hernández-Kantún J. J., Riosmena-Rodriguez R., Adey W. H., Rindi F. (2014). Analysis of the cox2-3 spacer region for population diversity and taxonomic implications in rhodolith-forming species (Rhodophyta: Corallinales). Phytotaxa 190 (1), 331–354. doi: 10.11646/phytotaxa.190.1.20
Hind K. R., Gabrielson P. W., Saunders G. W. (2014). Molecular-assisted alpha taxonomy reveals pseudocryptic diversity among species of Bossiella (Corallinales, rhodophyta) in the eastern pacific ocean. Phycologia 53 (5), 443–456. doi: 10.2216/13-239.1
Hind K. R., Saunders G. W. (2013). A molecular phylogenetic study of the tribe corallineae (Corallinales, rhodophyta) with an assessment of genus-level taxonomic features and descriptions of novel genera. J. Phycol. 49, 103–114. doi: 10.1111/jpy.12019
Hsieh T. C., Ma K. H., Chao A. (2016). iNEXT: an r package for rarefaction and extrapolation of species diversity (Hill numbers). Methods Ecol. Evol. 7 (12), 1451–1456. doi: 10.1111/2041-210X.12613
Hughes T. P., Baird A. H., Bellwood D. R., Card M., Connolly S. R., Folke C., et al. (2003). Climate change, human impacts, and the resilience of coral reefs. Science 301, 929–933. doi: 10.1126/science.1085046
Hughes T. P., Rodrigues M. J., Bellwood D. R., Ceccarelli D., Hoegh-Guldberg O., McCook L., et al. (2007). Phase shifts, herbivory, and the resilience of coral reefs to climate change. Curr. Biol. 17 (4), 360–365. doi: 10.1016/j.cub.2006.12.049
Johnson J. H. (1964). “Fossil and recent calcareous algae from Guam,” in Geol. surv. Prof. pap, 403–40G. Washington, DC, U.S.A.; United States Geological Survey (USGS).
Kalyaanamoorthy S., Minh B. Q., Wong T. K. F., von Haeseler A., Jermiin L. S. (2017). ModelFinder: fast model selection for accurate phylogenetic estimates. Nat. Methods 14, 587–589. doi: 10.1038/nmeth.4285
Kearse M., Moir R., Wilson A., Stones-Havas S., Cheung M., Sturrock S., et al (2012). Geneious Basic: an integrated and extendable desktop software platform for the organization and analysis of sequence data. Bioinformatics 28 (12) 1647–1649. doi: 10.1093/bioinformatics/bts199
Lastimoso J. M. L., Santiañez W. J. E. (2020). Updated checklist of the benthic marine macroalgae of the Philippines. Phillipp. J. Sci. 150 (S1), 29–92.
Lee D., Carpenter S. J. (2001). Isotopic disequilibrium in marine calcareous algae. Chem. Geol. 172 (3–4), 307–329. doi: 10.1016/S0009-2541(00)00258-8
Leliaert F., Payo D. A., Gurgel C. F. D., Schils T., Draisma S. G. A., Saunders G. W., et al. (2018). Patterns and drivers of species diversity in the indo-pacific red seaweed Portieria. J. Biogeogr. 45 (10), 2299–2313. doi: 10.1111/jbi.13410
Littler M. M., Littler D. S., Blair S. M., Norris J. N. (1985). Deepest known plant life discovered on an uncharted seamount. Science 227, 57–59. doi: 10.1126/science.227.4682.57
Liu L.-C., Lin S.-M., Caragnano A., Payri C. (2018). Species diversity and molecular phylogeny of non-geniculate coralline algae (Corallinophycidae, rhodophyta) from taoyuan algal reefs in northern Taiwan, including Crustaphytum gen. nov. and three new species. J. Appl. Phycol. 30, 3455–3469. doi: 10.1007/s10811-018-1620-1
Lobban C. S., Tsuda R. T. (2003). Revised checklist of benthic marine macroalgae and seagrasses of Guam and Micronesia. Micronesica 35, 54–99.
Loreau M., Naeem S., Inchausti P. (2002). Biodiversity and ecosystem functioning: synthesis and perspectives (Oxford, UK: Oxford University Press).
Mallela J. (2013). Calcification by reef-building sclerobionts. PloS One 8, e60010. doi: 10.1371/journal.pone.0060010
Maneveldt G. W., Gabrielson P. W., Townsend R. A., Kangwe J. (2019). Lithophyllum longense (Corallinales, rhodophyta): a species with a widespread Indian ocean distribution. Phytotaxa 49 (2), 149–168. doi: 10.11646/phytotaxa.419.2.2
Manghisi A., Miladi R., Armeli Minicante S., Genovese G., Le Gall L., Abdelkafi S., et al. (2019). DNA Barcoding sheds light on novel records in the Tunisian red algal flora. Cryptogam. Algol. 40 (3), 5–27. doi: 10.5252/cryptogamie-algologie2019v40a3
Meier R., Zhang G., Ali F. (2008). The use of mean instead of smallest interspecific distances exaggerates the size of the “Barcoding gap” and leads to misidentification. Syst. Biol. 57 (5), 809–813. doi: 10.1080/10635150802406343
Mills M. S., Schils T. (2021). The habitat-modifying red alga Ramicrusta on pacific reefs: a new generic record for the tropical northwestern pacific and the description of four new species from Guam. PloS One 16 (11), e0259336. doi: 10.1371/journal.pone.0259336
Minh B. Q., Schmidt H. A., Chernomor O., Schrempf D., Woodhams M. D., von Haeseler A., et al. (2020). IQ-TREE 2: new models and efficient methods for phylogenetic inference in the genomic era. Mol. Biol. Evol. 37 (5), 1530–1534. doi: 10.1093/molbev/msaa015
Mora C., Tittensor D. P., Adl S., Simpson A. G. B., Worm B. (2011). How many species are there on earth and in the ocean? PloS Biol. 9 (8), e1001127. doi: 10.1371/journal.pbio.1001127
Naeem S., Thompson L. J., Lawler S. P., Lawton J. H., Woodfin R. M. (1994). Declining biodiversity can alter the performance of ecosystems. Nature 368, 734–737. doi: 10.1038/368734a0
Nieder C., Chen P.-C., Chen C. A., Liu S.-L. (2019). New record of the encrusting alga Ramicrusta textilis overgrowing corals in the lagoon of dongsha atoll, south China Sea. Bull. Mar. Sci. 95 (3), 459–462. doi: 10.5343/bms.2019.0010
O’Leary J. K., Barry J. P., Gabrielson P. W., Rogers-Bennett L., Potts D. C., Palumbi S. R., et al. (2017). Calcifying algae maintain settlement cues to larval abalone following algal exposure to extreme ocean acidification. Sci. Rep. 7, 5774. doi: 10.1038/s41598-017-05502-x
Pedroche F. F., Sentíes A. (2020). Diversidad de macroalgas marinas en méxico. una actualización florística y nomenclatural. Cymbella 6 (1), 4–55.
Peña V., Rousseau F., De Reviers B., Le Gall L. (2014). First assessment of the diversity of coralline species forming maerl and rhodoliths in Guadeloupe, Caribbean using an integrative systematic approach. Phytotaxa 190 (1), 190–215. doi: 10.11646/phytotaxa.190.1.13
Peña V., Torres T. G. (2021). Lithophyllum artabricum V.Peña, sp. nov. (Corallinales, rhodophyta): a cryptic species in the Atlantic Iberian peninsula hitherto assigned to Lithophyllum stictiforme (Areschoug) hauck. Cryptogam. Algol. 42 (11), 153–172. doi: 10.5252/cryptogamie-algologie2021v42a11
Peña V., Vieira C., Braga J. C., Aguirre J., Rösler A., Baele G., et al. (2020). Radiation of the coralline red algae (Corallinophycidae, rhodophyta) crown group as inferred from a multilocus time-calibrated phylogeny. Mol. Phylogenet. Evol. 150, 106845. doi: 10.1016/j.ympev.2020.106845
Pestana E. M. D. S., Nunes J., Cassano V., Lyra G. (2021). Taxonomic revision of the peyssonneliales (Rhodophyta): circumscribing the authentic Peyssonnelia clade and proposing four new genera and seven new species. J. Phycol. 57, 1749–1767. doi: 10.1111/jpy.13207
Pezzolesi L., Falace A., Kaleb S., Hernández-Kantún J. J., Cerrano C., Rindi F. (2016). Genetic and morphological variation in an ecosystem engineer, Lithophyllum byssoides (Corallinales, rhodophyta). J. Phycol. 53 (1), 146–160. doi: 10.1111/jpy.12488
Pueschel C. M., Saunders G. W. (2009). Ramicrusta textilis sp. nov. (Peyssonneliaceae, rhodophyta), an anatomically complex Caribbean alga that overgrows corals. Phycologia 48 (6), 3–25. doi: 10.2216/09-04.1
Puillandre N., Lambert A., Brouillet S., Achaz G. (2012). ABGD, automatic barcode gap discovery for primary species delimitation. Mol. Ecol. 21, 1864–1877. doi: 10.1111/j.1365-294X.2011.05239.x
Randall R. H., Eldredge L. G. (1976). Atlas of the reefs and beaches of Guam (Mangilao (GU: University of Guam Marine Laboratory).
Randall R. H., Holloman J. (1974). Coastal survey of Guam (Mangilao (GU: University of Guam Marine Laboratory).
Ratnasingham S., Hebert P. D. N. (2007). BOLD: The barcode of life data system (www.barcodinglife.org). Mol. Ecol. Notes 7, 355–364. doi: 10.1111/j.1471-8286.2006.01678.x
R Core Team (2022). R: a language and environment for statistical computing (Vienna, Austria: R Foundation for Statistical Computing). Available at: https://www.R-project.org/.
Richards J. L., Gabrielson P. W., Schneider C. W. (2018). Sporolithon mesophoticum sp. nov. (Sporolithales, rhodophyta) from plantagenet bank off Bermuda at a depth of 178 m. Phytotaxa 385 (2), 67–76. doi: 10.11646/phytotaxa.385.2.2
Ries J. B. (2011). Skeletal mineralogy in a high-CO2 world. J. Exp. Mar. Bio.Ecol. 403, 54–64. doi: 10.1016/j.jembe.2011.04.006
Santelices B., Abbott I. A. (1987). Geographic and marine isolation: an assessment of the marine algae of Easter island. Pac. Sci. 41 (1), 1–20.
Saunders G. W. (2008). A DNA barcode examination of the red algal family dumontiaceae in Canadian waters reveals substantial cryptic species diversity. 1. the foliose Dilsea-Neodilsea complex and Weeksia. Botany 86, 773–789. doi: 10.1139/B08-001
Saunders G. W., McDevit D. C. (2012). Methods for DNA barcoding photosynthetic protists emphasizing the macroalgae and diatoms. Methods Mol. Biol. 858, 207–222. doi: 10.1007/978-1-61779-591-6_10
Schils T., Guiry M. D. (2016). Donor-recipient relationships of non-indigenous marine macroalgae between tropical pacific islands. Cryptogam. Algol. 37 (3), 1–14. doi: 10.7872/crya/v37.iss3.2016.199
Schils T., Vroom P. S., Tribollet A. D. (2013). Geographical partitioning of marine macrophyte assemblages in the tropical pacific: a result of local and regional diversity processes. J. Biogeogr. 40, 1266–1277. doi: 10.1111/jbi.12083
Selkoe K. A., Gaggiotti O. E., Treml E. A., Wren J. L. K., Donovan M. K., Connectivity Consortium H. R., et al. (2016). The DNA of coral reef biodiversity: predicting and protecting genetic diversity of reef assemblages. Proc. R. Soc B 283, 20160354. doi: 10.1098/rspb.2016.0354
Sherwood A. R., Kurihara A., Conklin K. Y., Sauvage T., Presting G. G. (2010). The Hawaiian rhodophyta biodiversity survey (2006-2010): a summary of principal findings. BMC Plant Biol. 10, 1–29. doi: 10.1186/1471-2229-10-258
Sherwood A. R., Paiano M. O., Spalding H. L., Kosaki R. K. (2020). Biodiversity of Hawaiian peyssonneliales (Rhodophyta): Sonderophycus copusii sp. nov., a new species from the northwestern Hawaiian islands. Algae 35 (2), 145–155. doi: 10.4490/algae.2020.35.5.20
Sissini M. N., Koerich G., de Barros-Barreto M. B., Coutinho L. M., Gomes F. P., Oliveira W., et al. (2021). Diversity, distribution, and environmental drivers of coralline red algae: the major reef builders in the southwestern Atlantic. Coral Reefs 41, 711–725. doi: 10.1007/s00338-021-02171-1
Sissini M. N., Oliveira M. C., Gabrielson P. W., Robinson N. M., Okolodkov Y. B., Riosmena-Rodríguez R., et al. (2014). Mesophyllum erubescens (Corallinales, rhodophyta)–so many species in one epithet. Phytotaxa 190 (1), 299–319. doi: 10.11646/phytotaxa.190.1.18
Steneck R. S. (1986). The ecology of coralline algal crusts: convergent patterns and adaptative strategies. Annu. Rev. Ecol. Syst. 17, 273–303. doi: 10.1146/annurev.es.17.110186.001421
Tebben J., Motti C. A., Siboni N., Tapiolas D. M., Negri A. P., Schupp P. J., et al. (2015). Chemical mediation of coral larval settlement by crustose coralline algae. Sci. Rep. 5, 10803. doi: 10.1038/srep10803
Thiers B. (2016) Index herbariorum: a global directory of public herbaria and associated staff. new York botanical garden’s virtual herbarium. Available at: http://sweetgum.nybg.org/science/ih/.
Tilman D., Reich P. B., Knops J. M. H. (2006). Biodiversity and ecosystem stability in a decade-long grassland experiment. Nature 441 (6), 629–632. doi: 10.1038/nature04742
Tsuda R. (2003). Checklist and bibliography of the marine benthic algae from the Mariana islands (Guam and CNMI) (Mangilao (GU: University of Guam Marine Laboratory).
Tsuda R. (2014). Endemism of marine algae in the Hawaiian islands. Bishop Mus. Occas. Pap. 115, 23–27.
Twist B. A., Neill K. F., Bilewitch J., Jeong S. Y., Sutherland J. E., Nelson W. A. (2019). High diversity of coralline algae in new Zealand revealed: Knowledge gaps and implications for future research. PloS One 14 (12), e0225645. doi: 10.1371/journal.pone.0225645
Vargas-Ángel B., Richards C. L., Vroom P. S., Price N. N., Schils T., Young C. W., et al. (2015). Baseline assessment of net calcium carbonate accretion rates on U.S. pacific reefs. PloS One 10 (12), 1–25. doi: 10.1371/journal.pone.0142196
Vásquez-Elizondo R. M., Enríquez S. (2016). Coralline algal physiology is more adversely affected by elevated temperature than reduced pH. Sci. Rep. 6 (1), 1–14. doi: 10.1038/srep19030
Verbruggen H., Schils T. (2012). Rhipilia coppejansii, a new coral reef-associated species from Guam (Bryopsidales, chlorophyta). J. Phycol. 48 (5), 1090–1098. doi: 10.1111/j.1529-8817.2012.01199.x
Verlaque M., Ballesteros E., Antonius A. (2000). Metapeyssonnelia corallepida sp. nov. (Peyssonneliaceae, rhodophyta), an Atlantic encrusting red alga overgrowing corals. Botanica Marina 43, 191–200. doi: 10.1515/BOT.2000.020
Vermeij M. J. A., Dailer M. L., Smith C. M. (2011). Crustose coralline algae can suppress macroalgal growth and recruitment on Hawaiian coral reefs. Mar. Ecol. Prog. Ser. 422, 1–7. doi: 10.3354/meps08964
Vieira C., N’Yeurt A. D. R., Rasomanendrika F. A., D’Hondt S., Tran L. A. T., den Spiegel D. V., et al. (2021). Marine macroalgal biodiversity of northern Madagascar: morpho-genetic systematics and implications of anthropic impacts for conservation. Biodivers. Conserv. 30, 1501–1546. doi: 10.1007/s10531-021-02156-0
Vieira C., Schils T., Kawai H., D’hondt S., Paiano M. O., Sherwood A. R., et al. (2022). Phylogenetic position of Newhousia (Dictyotales, phaeophyceae) and the description of N. sumayensis sp. nov. from Guam. Phycologia. 61 (3), 255–264. doi: 10.1080/00318884.2022.2034364
Vroom P. S. (2011). “Coral dominance”: a dangerous ecosystem misnomer? J. Mar. Biol. 2011, 164127. doi: 10.1155/2011/164127
Williams S. M., García-Sais J. R. (2020). A potential new threat on the coral reefs of Puerto Rico: The recent emergence of Ramicrusta spp. Mar. Ecol. 41 (4), e12592. doi: 10.1111/maec.12592
Yamamoto S., Kayanne H., Terai M., Watanabe A., Kato K., Negishi A., et al. (2012). Threshold of carbonate saturation state determined by CO2 control experiment. Biogeosciences 9, 1441–1450. doi: 10.5194/bg-9-1441-2012
Yoon H. S., Hackett J. D., Bhattacharya D. (2002). A single origin of the peridinin- and fucoxanthin-containing plastids in dinoflagellates through tertiary endosymbiosis. Proc. Natl. Acad. Sci. U.S.A. 99 (18), 11724–11729. doi: 10.1073/pnas.172234799
Yuen Y. S., Yamazaki S. S., Nakamura T., Tokuda G., Yamasaki H. (2009). Effects of live rock on the reef-building coral Acropora digitifera cultured with high levels of nitrogenous compounds. Aquac. Eng. 41, 35–43. doi: 10.1016/j.aquaeng.2009.06.004
Keywords: Corallinophycidae, Peyssonneliales, DNA barcoding, biodiversity, cryptic species, COI-5P, psbA
Citation: Mills MS, Deinhart ME, Heagy MN and Schils T (2022) Small tropical islands as hotspots of crustose calcifying red algal diversity and endemism. Front. Mar. Sci. 9:898308. doi: 10.3389/fmars.2022.898308
Received: 17 March 2022; Accepted: 04 July 2022;
Published: 26 July 2022.
Edited by:
Daniela Basso, University of Milano-Bicocca, ItalyReviewed by:
Ricardo Bahia, Rio de Janeiro Botanical Garden, BrazilMarina Nasri Sissini, Fluminense Federal University, Brazil
Copyright © 2022 Mills, Deinhart, Heagy and Schils. This is an open-access article distributed under the terms of the Creative Commons Attribution License (CC BY). The use, distribution or reproduction in other forums is permitted, provided the original author(s) and the copyright owner(s) are credited and that the original publication in this journal is cited, in accordance with accepted academic practice. No use, distribution or reproduction is permitted which does not comply with these terms.
*Correspondence: Matthew S. Mills, TWF0dGhldy5NaWxsc0ByZXNlYXJjaC51c2MuZWR1LmF1
†These authors have contributed equally to this work