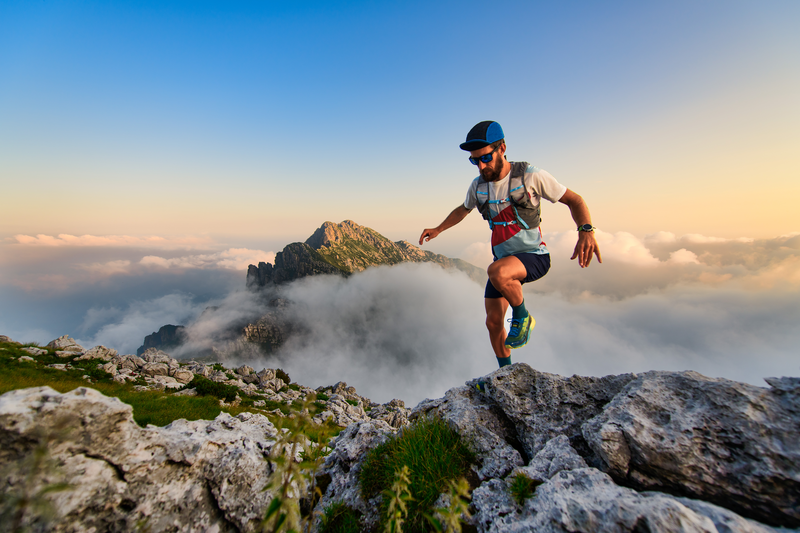
95% of researchers rate our articles as excellent or good
Learn more about the work of our research integrity team to safeguard the quality of each article we publish.
Find out more
ORIGINAL RESEARCH article
Front. Mar. Sci. , 01 August 2022
Sec. Marine Fisheries, Aquaculture and Living Resources
Volume 9 - 2022 | https://doi.org/10.3389/fmars.2022.895061
This article is part of the Research Topic Aquaculture of Emergent Marine Invertebrates: Advances in Nutrition, Rearing Technology and End-Product Quality View all 11 articles
The global demand for sea urchin as seafood is currently unmet. Despite exploitation of > 40 species across the world, there is a need to identify other candidate species, especially in regions where diversification in production is sought where species are considered native. The Eastern US presents an opportunity to determine the marketability of the currently unexploited Arbacia punctulata which is naturally distributed from Massachusetts and southwards into the Gulf of Mexico. To determine whether A. punctulata had market potential, it was fed one of the following diets to determine whether the gonad tissue (uni) could be manipulated to increase gonad mass and improve gonad color for the market: dried Ulva lactuca, Salmon pellets (Skretting), Tilapia pellets (Ziegler) or an Urchinomics diet designed for sea urchins either fed for 8 weeks or 12 weeks. All of the pelleted feeds (Salmon, Tilapia and Urchinomics) increased gonad mass and altered the color. The colors of the uni were generally darker than the colors that the market would typically prefer but some individuals did exhibit colors which have been classed as acceptable to the European market. This work highlights that further research is worthwhile to assess the market potential of A. punctulata.
There is a globally unmet demand for the luxury seafood product, sea urchin gonads (termed roe by the industry or uni in Japan). This is largely driven by the Asian market, but urchins are grown, sold, and consumed across the world, including regions such as North America (Eddy et al., 2015; Sun and Chiang, 2015; Stefánsson et al., 2017). Regional demand can range from local restaurants serving uni raw as sushi or incorporating it into a sauce served with cooked pasta, to regional processors who can remove and prepare uni into aesthetically appealing packaged trays in preparation for shipping. Marketable uni should ideally have a firm and non-gamete-shedding texture and bright orange or yellow in color with a pleasant sweet-salty flavor (Sun and Chiang, 2015). Sea urchins have been wild harvested for at least six decades, with highest yields produced during the times when sushi became popular during the 1960s, however, most catches dropped dramatically following this period due to overexploitation (Stefánsson et al., 2017). Harvesting pressure to meet market demand led to over 40 species populations to overexploitation (Andrew et al., 2002; McBride, 2005) and there is pressure to identify other candidate species and to also rely more heavily on aquaculture production to meet this demand. In recent years new or emerging species have included the European Sea urchin species, Psammechinus miliaris (Suckling et al., 2011; Suckling et al., 2018; Suckling et al., 2020a; Suckling, 2021) and Sphaerechinus granularis (José et al., 2019), but there remain many species which have not yet been investigated for market potential.
The Atlantic purple sea urchin, Arbacia punctulata (Lamarck, 1816) is a common model species for toxicology studies (Ward et al., 2006; Nelson et al., 2010; Barron et al., 2020) but is not fished commercially at all and little is known about its market potential. It is a regular echinoid with a similar morphology to other echinoids which are currently commercially exploited, and has a wide distribution in the western Atlantic Ocean, from Massachusetts through the Gulf of Mexico and along the coast of Central and South America towards Belize, from the low tide line down to approximately 230 m depth (Kier, 1975; Serafy, 1979; Hendler & Pawson, 2000). A combination of literature reviewing and pilot sampling (Suckling, unpublished data) of sea urchins collected in the Cape Cod and Narragansett Bay regions (Rhode Island, USA) have highlighted that A. punctulata are gonochoristic and generally spawn in the Summer (Harvey, 1956) with firm non-gamete-shedding gonads during the austral winter indicating a lack of distinct gametes (Suckling et al., 2011). While the broad reproductive stages for A. punctulata need further investigation this indicates a similar reproductive stage pattern to many other temperate/sub-tropical sea urchin species (e.g. Psammechinus miliaris and Paracentrotus lividus; Byrne, 1990; Kelly et al, 2000) where firm roe are marketable within the late fall through to early spring months (Figure 1). Pilot sampling of sea urchins sampled Vineyard Sound (Massachusetts) and Narragansett Bay (Rhode Island, U.S.A) also identified that A. punctulata uni were small and undesirable in market color indicating that fishing alone would not meet market demand (Suckling, unpublished data) and is typical for animals found within habitats with low food supplies (Hughes et al., 2006; Symonds et al., 2009; Suckling et al., 2011). Their omnivorous feeding habits on a range of animal and algal food sources (Lawrence, 1975; Wahl & Hay, 1995) indicate a strong ability to digest various compounds. This therefore strongly suggests that intervention using formulated feeds could be feasible, an approach used to influence and yield uni with marketable attributes for some existing commercially exploited sea urchin species (e.g. large size, firm texture and appealing bright colors; Robinson et al., 2002; Pearce et al., 2002; McBride et al., 2004; Shpigel et al., 2005; Symonds et al. 2007; Suckling et al., 2011).
Figure 1 Schematic of the reproductive stages of a mature sea urchin which are best suited for the seafood market (light grey lines and text) when uni are firm and not seeping gametes, and those that are not suited for the market (black lines and text) when near their reproductive peak and uni readily seep gametes. Based on Northern hemisphere species such as Arbacia punctulata, Psammechinus miliaris and Paracentrotus lividus (adapted from Fuji, 1960; Byrne, 1990; Kelly et al., 2000; Suckling et al., 2011; Suckling, 2012). This schematic presents a general overview only, to illustrate how the reproductive cycle can be linked to the market and season, and does not align exactly with seasonal timings for A. punctulata.
A proprietary diet specifically designed for sea urchins has been emerging in recent years. This was initially developed by and referred to as the ‘Nofima’ diet [Norway (e.g. Siikavuopio and Mortensen, 2015)], but has since been globally licensed to Urchinomics (www.urchinomics.com) and undergone further formulation developments and is now a different diet. This diet has shown extremely promising results, yielding marketable uni in wild collected sea urchins collected to protect diminishing kelp forests within as little as 8-12 weeks. At present this feed is not available as an off the shelf diet with selective commercial agreements with the company required. Determining the suitability of alternative off the shelf options would therefore be valuable for growers. A range of available high protein (e.g. fishmeal and or soya bean protein sources) formulated feeds designed for other aquaculture species such as salmon have shown to be palatable by many sea urchin species and to yield large uni (Brown and Eddy, 2015; Fernandez and Boudouresque, 2000; Suckling et al., 2011). These feeds often incorporate natural carotenoids such as astaxanthin to promote the red/pink salmon flesh color prized by consumers, but these do not always translate well to promote marketable uni colors in sea urchins (Suckling et al., 2020b), often instead needing β-carotene supplementation through the provision of macroalgae (e.g. Shpigel et al., 2005; Carrier et al., 2017) and microalgae (McLaughlin and Kelly, 2001; Shpigel et al., 2006). Commercially available proprietary formulated feeds designed for Tilapia (e.g. Zeigler) incorporate both high protein inclusions as well as algae which would likely include desirable carotenoids which could positively influence uni color (Shpigel et al., 2005; Suckling et al., 2020b), but these remain untested in sea urchins to date.
With species such as A. punctulata where the effect of formulated feeds on uni development is currently unknown, some of the initial steps are to determine palatability and whether uni size and color can be influenced. Pilot trials indicate that the above listed formulated feeds are palatable and ingested and processed by A. punctulata (Suckling, unpublished data) and therefore the next step is to determine whether prolonged feeding can enhance uni marketable attributes. The aim of this study was to therefore assess the potential commercial prospects of A. punctulata by providing a range of food treatments for a period of up to 3 months, a period known to be sensitive enough to measure nutritional influences on the development of the uni (e.g. Suckling et al., 2011).
Adult Arbacia punctulata were collected in early September 2019 off the coast of Falmouth, Massachusetts, U.S.A in Vineyard Sound (41°31’38.1”N 70°38’08.1”W). Specimens were collected by bottom dredge using a 5-foot-wide scallop dredge at a depth of 9 meters and stored in coolers with aeration until their return to land within approximately 1-2 hours. Following collection, specimens were housed at the Marine Biological Laboratory (MBL), Marine Resource Center in Woods Hole (Massachusetts, USA) for 48 hours within a flow through system and fed Ulva sp. and Saccharina latissima. Specimens were then transported in insulated 95 L coolers containing ~ 56 L of seawater (~ 23°C) with aeration to the University of Rhode Island’s (URI) Bay Campus aquarium facilities, Narragansett Rhode Island, U.S.A. Upon arrival at the URI Bay Campus (within approximately 2 hours), inspection of the coolers showed no sign of spawning had occurred during transportation (e.g. no milky appearance from sperm release). Seawater from the URI Bay Campus seawater supply was gradually added to the coolers across a 30-minute period to acclimate the animals to the new seawater supply. Seawater provided to the URI Bay Campus aquarium facilities was ambient with Southeastern Narragansett Bay (ambient temperature ~ 23.1°C, salinity 34 psu, pH ~ 7.9). Specimens were then held across eight 60 L (60.5 x 30.5 x 40 cm) holding tanks (25 specimens per aquaria) for two weeks supplied with ambient sand filtered flow through seawater (flow rate ~ 560 ml/min; 23.1°C; 34 psu from southern Narragansett Bay) and with aeration and under an ambient photoperiod with fluorescent lighting. During this period sea urchins were fed a combination of Ulva sp., Palmaria palmata and Grateloupia turuturu ad libitum.
The sea urchins were randomly allocated across eighteen 60 L glass experimental tanks (60.5 x 30.5 x 40 cm) flow through aquaria (seawater flow rate ~ 560 ml/min) until 10 specimens per aquarium was achieved. These animals were then starved for a period of two weeks to assure empty alimentary canals and to standardize their nutritional state (Vadas, 1977). Animal test diameters and whole animal wet mass were measured at the experimental start and were found to be homogenous across all experimental tanks thus showing strong initial experimental control (test diameter (mean ± SD) = 31.92 ± 3.53 mm, F17, 179 = 0.26, p = 0.999; wet mass = 17.24 ± 5.67g, F17, 179 = 0.454, p = 0.501).
The tanks (n = 10 sea urchins per replicate) were randomly allocated to baseline sampling or one of five diet treatments (3 replicate tanks per treatment and baseline group). One diet comprised of Ulva lactuca (“Ulva”) was used due to various Ulva species having shown to act as a feeding stimulant and enhance gonad growth and quality in sea urchins when added as an additive to pelleted feeds (Cyrus et al., 2015a; Cyrus et al., 2015b; Shpigel et al., 2018; Cyrus et al., 2019). Due to its abundance in coastal areas around the globe U. lactuca may be a cheap and easily available alternative to other diets for raising sea urchins and is easily dried as a supplement for pelleted feeds (Cyrus et al., 2014). U. lactuca was collected from the east passage of Narragansett Bay at Beavertail State Park (41.4535°N, 71.3976°W) at low tide once a week throughout September and October of 2019. All epiphytes were removed from the surface and U. lactuca was then oven dried at 60°C for one week and then frozen at -20°C. U. lactuca was dried to enable medium term storage. High protein diets were also assessed because, like other sea urchin species, A. punctulata is omnivorous with which include carnivory habits (Lawrence, 1975; Wahl & Hay, 1995; Gianguzza, 2020). Furthermore, previous studies have highlighted that gonad index can be substantially enhanced with high protein diets (e.g. Pearce et al., 2002; Robinson et al., 2002; Suckling et al., 2011). Sea urchins were fed a commercially available “Salmon” pelleted diet treatment (Skretting Salmon Sink 1.6 mm, Tooele, UT, USA; Table 1) with the primary sources of protein comprising of fish meal/oil and poultry meal/oil. A “Tilapia” diet treatment (Zeigler Finfish Broodstock 38-10, Gardners, PA, USA; Table 1) was also used with proteins comprising primarily of fish and poultry meal as well as wheat, corn, and soybeans. This diet also contains a proprietary mix of the carotenoids (Zeigler Bros., Inc, personal communication) due to the inclusion of algae in this pelleted feed, known to be important in immunity defense (Ito et al., 1992). Furthermore, carotenoids (e.g. β-carotene) have been widely shown to enhance the color of the gonads for the market (Robinson et al., 2002; Shpigel et al., 2005; Symonds et al. 2007; Symonds et al., 2009; Suckling et al., 2011; Suckling et al., 2020b).
Table 1 Percent proximate analysis and caloric value, of the diets provided throughout the feeding trial.
The final diet treatments comprised of an “Urchinomics” diet. This is a proprietary sea urchin diet currently globally licensed by Urchinomics (https://www.urchinomics.com/). It has previously been identified as the “NOFIMA” diet (Siikavuopio and Mortensen, 2015) but has since undergone further development. During its identity as the Nofima diet, it was shown to successfully enhance somatic and gonad growth within commercial sea urchin species such as Strongylocentrotus droebachiensis and Paracentrotus lividus and enhanced gonad color due to its high inclusion rate of macroalgae containing β-carotene (e.g. Siikavuopio and Mortensen, 2015; Prato et al., 2018). Since these studies and licensing to Urchinomics, this diet has undergone further changes through research and development, thus meaning it now has a different composition to its previous identity as the NOFIMA diet and its current status will now be referred to as the “Urchinomics” diet. Wild caught sea urchins can be fed the Urchinomics diet for a period of at least eight weeks to show gonad enhancement for the commercial market, but most food trials have been conducted for 12 weeks (e.g. Pearce et al., 2007; Suckling et al., 2011; Shpigel and Erez, 2020). This study incorporated both time frames for the Urchinomics diet (8 and 12 weeks; ‘Urchinomics-8’ and “Urchomics-12” respectively) and started feeding sea urchins from the start of the experimental period, with the ‘Urchinomics-8’ treatment group ending 4 weeks earlier than all other diet treatments. The start of these feed trials conformed to the start of the reproductive cycle and both time frames (8 and 12 weeks) were within what would be considered as the harvesting period, when gonad is firm and not leaching gametes.
Sea urchins were fed diet treatments at 3% wet body mass, three times a week for three months following the protocols outlined in Suckling et al. (2011). Aquaria were cleaned and siphoned three times a week and allowed to refill before the sea urchins were fed. Frozen U. lactuca was defrosted before being fed. Proximate analyses of the diets were provided by the manufacturer except for Ulva (Table 1). Ulva for proximate analysis was homogenized into a fine powder and three 50 mg samples were sent to New Jersey Feed Lab Inc (Trenton, NJ, USA) for a proximate and caloric analysis. The feed trials were conducted at ambient temperature and a 12L:12D photoperiod with fluorescent lighting was used. Tank seawater parameters (salinity and temperature) were monitored and measured twice a week (Mettler Toledo Portable SG3 pH Meter and TMC Aquarium V2 Handheld Refractometer). The seawater salinity and temperature remained similar throughout the experiment (Table 2).
Thirty sea urchins were dissected at the start of the experiment for baseline samples (3 replicates of 10 urchins, total = 30) and an additional 30 sea urchins (3 replicates of 10 urchins, total = 30) were sampled at the end of the experiment for each food treatment group following a ten-day starvation period to empty the digestive tract from remaining food and fecal materials to allow for tissue comparisons (Vadas, 1977). Prior to dissection, excess seawater was removed from the sea urchin body by briefly drip drying on clean paper towels. Test diameter (mm ± 0.01) was measured three times using vernier calipers and the mean test diameter was used for analysis. Whole animal wet mass was then measured (g ± 0.01) after which they were dissected in half using dissection scissors and tweezers to remove the gonad segments and alimentary canal. Immediately following dissection, gonads were qualitatively assessed by compared against a gonad color chart comprising of Pantone color chips for assessing the market value developed by Cook (1999); Symonds et al. (2009) and Suckling et al. (2011). In summary, colors ranked as acceptable (bright orange to yellow and pale colors) and unacceptable (dark brown). The gonad tissue was removed and following recording the wet gonad mass (g ± 0.01), one segment was selected at random and removed, and CIE L*a*b color values were measured using a chromometer (Minolta Chroma Meter CR-300; Suckling et al., 2011). The L*a*b color space is a way to quantifiably define colors (C.I.E., 1931) and is commonly used in the food industry (Hutchings, 1994) and used to define sea urchin gonads (Agatsuma, 1998; Robinson et al., 2002; McBride et al., 2004). L* represents the intensity or lightness of a samples (L* = 60 is white), while a* represents the hue or redness in a sample (+0 – 60), and b is the chroma or yellowness in a sample (+0 -60; McBride et al., 2004; Suckling et al., 2020b). Gonad index (GI) was calculated by dividing the wet gonad mass by the whole animal wet mass and expressed as a percentage.
One of the gonad segments was fixed in a 4% formalin solution until it was dehydrated, stained with a hematoxylin and eosin dye (H/E) which stained the gametogenic cells and nutritive phagocytes differently (Byrne, 1990), sectioned, and placed on positively charged slides (MAS Histology Services, Worcester, Massachusetts, USA). Upon return the samples were photographed using a compound trinocular microscope with mounted Omax (A35180U3) 18 mp digital camera. Each sample was photographed at 4x magnification. The images were then used to determine the sex and reproductive stage following the descriptions outlined by Fuji (1960) and Byrne (1990). “Stage I” is the recovering period and the follicle is contracted with “rumples”, “Stage II” is the growing period with oocytes between 40-60 µm and many spermatocytes in testes, “Stage III” is the pre-mature stage and in females the follicle is occupied by the primary oocyte and in males there are “sperm patches”, “Stage IV” are mature and there is no empty space in the follicle with numerous secondary oocytes while male follicles are filled with spermatozoa, finally “Stage V” is spent and in both sexes there is a large empty space in the follicle.
The alimentary canal was removed and placed onto tissue paper briefly to remove excess moisture, and weighed (g ± 0.01). The alimentary index was then calculated by dividing the alimentary mass by the whole animal wet mass and expressed as a percentage.
Data was stored in Microsoft Excel (V.16.0.12730.20188), analyzed in Minitab (v17). Proportional data were arcsine transformed before analysis (Kelly et al., 2000). The data were tested for homogeneity of variance (Levene’s) and if these assumptions were met then a general linear model was used to assess the factor of treatment and nesting replicate tanks for test diameter, whole animal wet mass, alimentary index, and color measurements (CIE L*a*b values). A one-way ANOVA was conducted for survival and marketable gonad color data. After significant results, Tukey’s pairwise comparisons were conducted to determine treatment differences. If data did not fit the assumptions of ANOVA following either a log or square root transformation, then a nonparametric Kruskal Wallis test was conducted (gonad index, reproductive stage). Where significant differences occurred, a Mann Whitney post-hoc comparison test was conducted to identify the treatment differences. Due to multiple testing, a Bonferroni correction was applied to reduce the occurrence of type I errors.
No significant differences in survival were found between the food treatments (F4,14 = 2.00, p = 0.171; Figure 2A). With respect to somatic growth, test diameters of A. punctulata fed the Urchinomics diet for 8 weeks (Urchinomics-8) was significantly lower than the Ulva, Tilapia and Urchinomics diet for 12 weeks (Urchnomics-12) treatments, likely due to the shorter experimental time utilized for this treatment group (i.e. 8 vs 12 weeks) (Treatment: F4, 147 = 7.62, p <0.001; Treatment (Tank): F10,147 = 3.15, p = 0.001; Figure 2B). The provision of different diet treatments did not significantly impact whole animal wet mass (Treatment: F4, 147 = 0.62, p = 0.647; Treatment (Tank): F4,147 = 3.05, p = 0.002; Figure 2C). Specimens fed the pelleted diets had significantly higher alimentary indices (A.I.) compared to the baselines and those fed Ulva (F5,177 = 12.23, p < 0.001; Treatment (Tank): F12,177 = 2.90, p = 0.179; Figure 2D).
Figure 2 Mean (± SE) survival (%; A), test diameter (mm; B), whole animal wet mass (g; C), and alimentary index (%; D) of Arbacia punctulata fed different diet treatments. Letters above bars represent groups that are significantly different from each other, and where there are no letters indicate no significant treatment effect.
The pelleted diet treatments (Salmon, Tilapia, Urchinomics-8 and Urchinomics-12) gave rise to the largest GI and were significantly greater compared to the Ulva diet and baseline samples (Treatment: F5,177 = 18.71, p < 0.001; Treatment (Tank): F12,177 = 1.48, p = 0.135; Figure 3A). A significant lightening (CIE L*) of gonad color was seen from the baseline samples for sea urchins fed the salmon diet diets (F5, 177 = 5.55, p < 0.001; Treatment (Tank): F12,177 = 2.90, p = 0.001; Figure 3B). A decrease in redness (CIE a*) was observed for sea urchins fed Ulva compared to the baseline samples (F5, 177 = 7.25, p < 0.001; Treatment (Tank): F12,177 = 1.08, p = 0.382; Figure 3C). Compared to baseline samples, a decrease in yellowness (CIE b) of gonad color was observed in sea urchins fed the Ulva diet, and an increase in yellowness was observed in sea urchins fed the Tilapia diet (F5, 177 = 6.62, p < 0.001; Treatment (Tank): F12,177 = 2.12, p = 0.019; Figure 3D). There were no significant differences in the percentage of sea urchins with gonad colors acceptable for the market across the experiment (F5,17 = 2.83, p = 0.065; Figure 3E). The reproductive stage of the sea urchins remained statistically similar throughout the experimental period, regardless of the diet provided (H5 = 9.69, p = 0.140; Figure 3F).
Figure 3 Mean (± SE) wet gonad index (%; A), CIE L* (lightness; B), CIE a* (redness; C), CIE b (yellowness; D), acceptable market gonad colors (%; E) and median (± IQR) reproductive stage (F) of Arbacia punctulata fed different diet treatments. Letters above bars represent groups that are significantly different from each other, and where there are no letters indicate no significant treatment effect.
This study highlights that the gonad index of A. punctulata can be positively enhanced using pelleted feed which is in agreement with a wide number of studies (e.g. de Jong-Westman et al., 1995; Fernandez et al., 1997; Lawrence et al., 1997; Akiyama et al., 2001; Robinson et al, 2002; Suckling et al., 2011; Zupo et al., 2018). While there was no significant improvement on marketable gonad colors, there was a subtle increase which highlights potential to manipulate gonad color through diet in this species and would need further trials to elucidate this. The colors of the uni were a darker range of colors (i.e. dark shades of purple, brown, red, orange and yellow) than the market would typically prefer from established marketable species (Suckling and Zavell, personal observation). At present we do not know for certain whether this species can achieve the familiar and preferred market uni colors typically being bright orange or yellow. But some individuals did exhibit darker pink/cream/red colors which have been classed as acceptable to the European market for P. miliaris and may provide an interesting new color aesthetic experience for the sushi market (Suckling et al., 2011). It is possible that the carotenoids included within these diets might not be compatible for biochemical conversion to color in the uni, and this has been shown to differ across species (Tsushima et al., 1997; Suckling et al., 2020b). Further work assessing the influence of differing carotenoids included into a base diet such those described by Robinson et al. (2002) and Suckling et al. (2011) would be required to determine this. Despite the darker uni colors, this study highlights that there was a clear influence of diet on gonad coloration. Additionally, the uni were observed to be at reproductive stages which would be conducive to typical late harvesting periods (Figure 1), thus showing that A. punctulata has potential for the market.
While it was clear that diet could influence and enhance gonadal growth in A. punctulata, in comparison to commercially exploited or emerging species, this growth was markedly slow (e.g. de Jong-Westman et al., 1995; Fernandez et al., 1997; Lawrence et al., 1997; Akiyama et al., 2001; Robinson et al, 2002; Suckling et al., 2011; Zupo et al., 2018). This could in part be explained by the collection method, scallop dredging. During this collection method, the harvested animals are caught within a mesh collection bag and dragged some distance with other dredged materials which can incur injury and high stress. Scallop dredging has been used in the past to collect Strongylocentrotus droebachiensis for the US market with some success (Scattergood, 1961), but the animals were sold and consumed within a short period of time after harvesting, thus omitting the longer-term impacts of this method. Dredging is used in other sea urchin fisheries across the world (e.g. Greenland and Iceland) but can be modified to reduce the stress and damage on the harvested sea urchins (James and Hannon, 2017). It is therefore possible that the harvested A. punctulata could have been highly stressed and therefore physiologically compromised and/or needing to allocate energy towards repair, despite efforts to use only healthy intact and undamaged looking animals in this study. Although survival data was not significantly different, this could also explain the mortalities recorded for both Urchinomics diet treatments (Figure 2), however, only a single animal died in a replicate tank thus representing 10% of that population and therefore was overall a low number. The Urchinomics diet has shown strong unanimous successes in its use (www.urchinomics.com) and would unlikely have been the cause of mortality in this trial.
Slow gonadal growth could also be explained by the geographical distribution and sourcing of this species. These animals were collected from and reared within the coldest northernmost range of their natural distribution (Serafy, 1979). This study was also carried out during the seasonally low winter temperatures (Table 2) meaning that this species was likely to be functioning within its lowest metabolic scope (Clarke and Johnston, 1999; Addo-Bediako et al., 2000; Suckling et al., 2020a). In turn the collection method or cooler temperatures could have limited the carotenoid utilization from the diets thus leading to the darker uni colors. Therefore, it is recommended that more trials be conducted using less impactful harvesting methods and within warmer temperatures than those used in the current study and/or across longer periods of time and using different carotenoids (e.g., lutein and zeaxanthin; Suckling et al., 2011; Suckling et al., 2020b) to determine whether gonad growth and color in A. punctulata can be further enhanced. While these remain the most likely factors of influence, there is also the possibility the diets provided within this study were not optimized for this species, thus warranting further investigations on differing food supplies.
Numerous sea urchin species (e.g. Arbacia lixula, Strongylocentrotus droebachiensis, Psammechinus miliaris), have been shown to be resilient to projected variability in ocean conditions expected within the next few decades (e.g. alteration to CO2 and temperature; Wangensteen et al., 2013; Suckling et al., 2014; Ross et al., 2015). The cellular processes of acid base buffering, that counteract increased CO2, can be achieved within 7-10 days (Stumpp et al., 2012) and they have shown to be reproductive and marketable under medium to long term exposures to laboratory simulated climate change scenarios (Dupont et al., 2013; Suckling et al., 2014; Suckling et al., 2020a) across several generations (Suckling, unpublished data). Therefore, the production of sea urchins presents a potential sustainable option for growers, and to support this industry it is important that new (e.g. A. punctulata) and emerging species (e.g. P. miliaris, and S. granularis;Suckling et al., 2011; Suckling et al., 2018; José et al., 2019; Suckling et al., 2020a), are identified and investigated. This mitigation strategy of diversifying production (i.e. with tolerant sea urchin species) is increasing in interest and uptake by growers in the US (Reid et al., 2019) allowing for contingency against losses already being observed from climate change (e.g. shellfish production; Barton et al., 2012; Clements & Chopin, 2016).
At present on the Eastern US coast, the green sea urchin (Strongylocentrotus droebachiensis) is one of the most valuable sea urchin species. It is a cold-water species and is currently produced through wild fisheries capture and is emerging through aquaculture in the State of Maine (ME). But recent work funded by the Northeastern Regional Aquaculture Center led by lead author Suckling shows that there is interest to expand this production into other New England states (New Hampshire, Massachusetts, and Rhode Island). At present the Rhode Island governing bodies are uncertain of the native status of S. droebachiensis with unconfirmed reports provided from regional recreational scuba divers and one confirmed report in 1998 in a coastal pond (Rhode Island Department of Environmental Management). Therefore, there is a potential niche and interest for A. punctulata production in areas where sea urchin cultivation is not yet practiced expanding southwards comfortably within this species’ natural distribution range. However, further work is first needed to fully determine the economic potential for A. punctulata.
The raw data supporting the conclusions of this article will be made available by the authors, without undue reservation.
CS, MZ, and BT contributed to conception and design of the study. CS and MZ performed the statistical analysis, and wrote the first draft of the manuscript. AB conducted and contributed sex and reproductive stage determination data. AB and BT wrote sections of the manuscript. BT contributed the Urchinomics diet for the trial. All authors contributed to manuscript revision, read, and approved the submitted version.
This work was partly funded by the United States Department of Agriculture, National Institute of Food and Agriculture (Project #RI0019-H020), Northeastern Regional Aquaculture Center and the University of Rhode Island Department of Undergraduate Research and Innovation (URI2).
Thank you to Urchinomics and Yuma Yamamoto for supplying the diet for use in this study. Thank you to Alli McKenna and Tyler Pelletier for animal husbandry assistance and to Sara Lacourciere, Ellie Mulligan, and Kelsey Mudry for assistance in the laboratory. This work was generated through an undergraduate research skills course (AFS491) within the University of Rhode Island.
Author BT was employed by Urchinomics BV.
The remaining authors declare that the research was conducted in the absence of any commercial or financial relationships that could be construed as a potential conflict of interest.
All claims expressed in this article are solely those of the authors and do not necessarily represent those of their affiliated organizations, or those of the publisher, the editors and the reviewers. Any product that may be evaluated in this article, or claim that may be made by its manufacturer, is not guaranteed or endorsed by the publisher.
Addo-Bediako A., Chown S. L., Gaston K. J. (2000). Thermal tolerance, climatic variability and latitude. Proc. Biol. Sci. 267, 739–745. doi: 10.1098/rspb.2000.1065
Agatsuma Y. (1998). Aquaculture of the sea urchin (Strongylocentrotus nudus) transplanted from coralline flats in hoikkaido Sea. J. Shellfish. Res. 17, 1541–1547.
Akiyama T., Uhuma T., Yamamota T. (2001). Optimum protein level in a purified diet for young red sea urchin pseudocentrotus depressus. Fish. Sci. 67, 361–363. doi: 10.1046/j.1444-2906.2001.00252.x
Andrew N. L., Agastusma Y., Ballesteros E., Bazhin A. G., Creaser E. P., Barnes D. K. A., et al. (2002). Status and management of world sea urchin fisheries. Oceanogr. Mar. Biol. 40, 343–425. doi: 10.1201/9780203180594-26
Barron M. G., Bejarano A. C., Conmy R. N., Sundaravadivelu D., Meyer P. (2020). Toxicity of oil spill response agents and crude oils to five aquatic test species. Mar. pollut. Bull. 153, 110954. doi: 10.1016/j.marpolbul.2020.110954
Barton A., Hales B., Waldbusser G. G., Langdon C., Feely R. A. (2012). The pacific oysters, crassostrea gigas, shows negative correlation to naturally elevated carbon dioxide levels: implications for near-term ocean acidification effects. Limnol. Ocean. 57, 698–710. doi: 10.4319/lo.2012.57.3.0698
Byrne M. (1990). Annual reproductive cycles of the commercial sea urchin paracentrotus lividus from an exposed intertidal and a sheltered subtidal habitat on the west coast of Ireland. Mar. Biol. 104, 275–289. doi: 10.1007/BF01313269
Carrier T. J., Eddy S. D., Redmond S. (2017). Solar-dried kelp as potential feed in sea urchin aquaculture. Aquac. Intl. 25, 355–366. doi: 10.1007/s10499-016-0033-x
C.I.E (1931). Comission internationale de l’Eclairage: Proceedings 8th session. Cambridge, England, 19 to 29 September 1931 (Paris, France: Bureau Central de la C.I.E.).
Clarke A., Johnston N. M. (1999). Scaling of metabolic rate with body mass and temperature in teleost fish. J. Anim. Ecol. 68, 893–905. doi: 10.1046/j.1365-2656.1999.00337.x
Clements J. C., Chopin T. (2016). Ocean acidification and marine aquaculture in north America: potential impacts and mitigation strategies. Rev. Aqua. 9, 326–341. doi: 10.1111/raq.12140
Cook E. J. (1999). Psammechinus miliaris (Gmelin) (Echinodermata: Echinoidea): factors affecting its somatic growth and gonadal growth and development (Edinburgh, UK: Napier University).
Cyrus M. D., Bolton J. J., De Wat L., Macey B. M. (2014). The development of a formulated feed containing ulva (Chlorophyta) to promote rapid growth and enhanced production of high quality roe in the sea urchin tripneustes gratilla (Linnaeus). Aquac. Res. 45, 159–176. doi: 10.1111/j.1365-2109.2012.03219.x
Cyrus M. D., Bolton J. J., Macey B. M. (2015a). The role of green seaweed ulva as a dietary supplement for full life cycle grow-out of tripneustes gratilla. Aquaculture 446, 187–197. doi: 10.1016/j.aquaculture.2015.05.002
Cyrus M. D., Bolton J. J., Macey B. M. (2019). The use of stable isotope ratios δ13C and δ15N to track the incorporation of ulva and other important dietary ingredients into the gonads of the sea urchin tripneustes gratilla. Aquac. Nutr. 26, 174–1185. doi: 10.1111/anu.12979
Cyrus M. D., Bolton J. J., Scholtz R., Macey B. M. (2015b). The advantages of ulva (Chlorophyta) as an additive in sea urchin formulated feeds: effects on palatability, consumption and digestibility. Aquac. Nutr. 21, 578–591. doi: 10.1111/anu.12182
de Jong-Westman M., March B. E., Carefoot T. H. (1995). The effect of different nutrient formulations in artificial diets on gonad growth in the sea urchin strongylocentrotus droebachiensis. Can. J. Zool. 73, 1495–1502. doi: 10.1139/z95-177
Dupont S., Dorey N., Stumpp M., Melzner F., Thorndyke M. (2013). Long-term & trans-life-cycle effects of exposure to ocean acidification in the green sea urchin strongylocentrotus droebachiensis. Mar. Biol. 160, 1835–1843. doi: 10.1007/s00227-012-1921-x
Eddy S. D., Brown N. P., Harris L. G. (2015). “Aquaculture of the green Sea urchin strongylocentrotus droebachiensis in north America,” in Echinoderm aquaculture. Eds. Brown N. P., Eddy S. D. (Hoboken, NJ: Wiley-Blackwell), 175–209. doi: 10.1002/9781119005810.ch8
Fernandez C., Boudouresque C.-F. (2000). Nutrition of the sea urchin paracentrotus lividus (Echinodermata: Echinoidea) fed different artificial food. Mar. Ecol. Prog. Ser. 204, 131–141. doi: 10.3354/meps204131
Fernandez C., Dombrowski E., Caltagirone A. (1997). “Gonadic growth of adult sea urchins, paracentrotus lividus (Echinodermata: Echinoidea) in rearing: the effect of different diet types,” in Echinoderm research. Eds. Emson R., Smith A., Campbell A. (Rotterdam: Balkema), 269–275.
Fuji A. (1960). Studies on the biology of the sea urchin: I. superficial and histological gonadal changes in gametogenic process of two sea urchins, strongylocentrotus nudus and s. intermedius Vol. 11 (Bulletin of Faculty of Fishes, Hokkaido University), 1–14. Available at: https://eprints.lib.hokudai.ac.jp/dspace/bitstream/2115/23091/1/11(1)_P1-14.pdf.
Gianguzza P. (2020). “Chapter 24: Arbacia,” in Sea Urchins: Biology and ecology, 4th ed. Ed. Lawrence J. M. (Amsterdam: Elsevier B.V.), 419–429. doi: 10.1016/B978-0-12-819570-3.00024-X
Harvey E. B. (1956). The American arbacia and other sea urchins (Princeton, New Jersey: Princeton University Press). doi: 10.5962/bhl.title.7234
Hendler G., Pawson D. L. (2000). Echinoderms of the rhomboidal cays, Belize: Biodiversity, distribution, and ecology. Atoll. Res. Bull. 479, 275–299.
Hughes A. D., Kelly M., Barnes D. K. A., Catarino A. I., Black K. D. (2006). The dual function of sea urchin gonads are reflected in the temporal variations of their biochemistry. Mar. Biol. 148, 789–798. doi: 10.1007/s00227-005-0124-0
Hutchings J. B. (1994). Food colour and appearance (New York: Springer US). doi: 10.1007/978-1-4615-2123-5
Ito T., Matsutani T., Mori K., Nomura T. (1992). Phagocytosis and hydrogen peroxide production by phagocytes the sea urchin strongylocentrotus nudus. Dev. Comp. Immunol. 16, 287–294. doi: 10.1016/0145-305x(92)90003-u
James P., Hannon C. (2017). “Cost/benefit analysis of sea urchin fishing techniques,” in Technical report activity A3.2.2 of the NPA URCHIN project (Tromsø: Nofima). doi: 10.13140/RG.2.2.10724.78728
José R., Ribeiro C., Neves P., Lourenço S. (2019). “First assessment of the population structure and reproductive cycle of the sea urchin sphaerechinus granularis (Lamarck 1816) in Madeira island: a potential new candidate to echinoculture,” in IMMR'18 | International Meeting on Marine Research, Peniche, 2018. doi: 10.3389/conf.FMARS.2018.06.00133
Kelly S. M., Hunter A. J., Scholfield C. L., McKenzie J. D. (2000). Morphology and survivorship of larval psammechinus miliaris (Gmelin) (Echinodermata: Echinoidea) in response to varying food quantity and quality. Aquaculture 183, 223–240. doi: 10.1016/S0044-8486(99)00296-3
Kier P. M. (1975). The echinoids of Carrie bow cay, belize. smithson. contrib. zool. NO. 206, 1–45. Smithsonian Institute.
Lawrence J. M. (1975). On the relation between marine plants and sea urchins. Oceanogr. Mar. Biol. 13, 213–286.
Lawrence J. M., Olave S., Otaiza R., Lawrence A. L., Bustos E. (1997). Enhancement of gonad production in the sea urchin loxechinus albus in Chile fed extruded feeds. J. World Aquac. Soc 28, 91–96. doi: 10.1111/j.1749-7345.1997.tb00966.x
McBride S. C., Price R. J., Tom P. D., Lawrence J. M., Lawrence A. L. (2004). Comparison of gonad quality factors: color, hardness, and resilience, of strongylocentrotus franciscanus between sea urchins fed prepared feed or algal diets and sea urchins harvested from the northern California fishery. Aquaculture 231, 405–422. doi: 10.1016/j.aquaculture.2003.10.014
McLaughlin G., Kelly S. M. (2001). Effect of artificial diets containing carotenoid-rich microalgae on gonad growth and colour in the sea urchin psammechinus miliaris (Gmelin). J. Shellfish. Res. 20, 377–382.
Nelson R., Nipper M., Lawrence A., Watts S. (2010). Parental dietary effect on embryological development response to toxicants with the sea urchin arbacia punctulata. Bull. Environ. Contam. Toxicol. 84, 71–75. doi: 10.1007/s00128-009-9909-z
Pearce C. M., Daggett T. L., Robinson S. M. C. (2002). Effect of binder type and concentration on prepared feed stability and gonad yield and quality of the green sea urchin, strongylocentrotus droebachiensis. Aquaculture 205, 301–323. doi: 10.1016/s0044-8486(01)00685-8
Pearce C. M., Daggett T., Robinson S. M. C. (2007). Optimizing prepared feed ration for gonad production of the green sea urchin strongylocentrotus droebachiensis. J. World Aquac. Soc 33, 268–277. doi: 10.1111/j.1749-7345.2002.tb00503.x
Prato E., Chiantore M., Kelly M. S., Hughes A. D., James P., Ferranti M. P., et al. (2018). Effect of formulated diets on the proximate composition and fatty acid profiles of sea urchin paracentrotus lividus gonad. Aquac. Int. 26, 185–202. doi: 10.1007/s10499-017-0203-5
Reid G. K., Gurney-Smith H. J., Flaherty M., Garber A. F., Forster I., Brewer-Dalton K., et al (2019). Climate change and aquaculture: considering adaptation potential. Aquac. Environ. Interact. 11, 603–624. doi: 10.3354/aei00333
Robinson S. M. C., Castell J. D., Kennedy E. J. (2002). Developing suitable colour in the gonads of cultured green sea urchins (Strongylocentrotus droebachiensis). Aquaculture 206, 289–303. doi: 10.1016/S0044-8486(01)00723-2
Ross P., Parker L., Byrne M. (2015). Transgenerational responses of molluscs and echinoderms to changing ocean conditions. ICES J. Mar. Sci. 73, 537–549. doi: 10.1093/icesjms/fsv254
Scattergood L. W. (1961). The sea urchin fishery (Washington D.C: The United States Department of the Interior, Fish and Wildlife Service Bureau of Commercial Fisheries), 20240.
Serafy D. K. (1979). Memoirs of the hourglass cruises: Echinoids (Echinodermata: Echinoidea), volume 5 (St. Petersberg, Florida: Florida Department of Natural Resources, Marine Research Laboratory). Available at: http://hdl.handle.net/1834/18639.
Shpigel M., Erez J. (2020). Effect of diets and light regimes on calcification and somatic growth of the sea urchin tripneustes gratilla elantensis. Aquaculture 529, 735547. doi: 10.1016/j.aquaculture.2020.735547
Shpigel M., McBride S. C., Marciano S., Ron S., Ben-Amotz A. (2005). Improving gonad color and somatic index in the European sea urchin paracentrotus lividus. Aquaculture 318, 335–342. doi: 10.1016/j.aquaculture.2004.11.043
Shpigel M., Schlosser S. C., Ben-Amotz A., Lawrence A. L., Lawrence J. M. (2006). Effects of dietary carotenoid on the gut and the gonad of the sea urchin paracentrotus lividus. Aquaculture 261, 1269–1280. doi: 10.1016/j.aquaculture.2006.08.029
Shpigel M., Shauli L., Odintsov V., Ashkenazi N., Ben-Ezra D. (2018). Ulva lactuca biofilter from a land-based integrated multi trophic aquaculture (IMTA) system as a sole food source for the tropical sea urchin tripneustes gratilla elatensis. Aquaculture 496, 221–231. doi: 10.1016/j.aquaculture.2018.06.038
Siikavuopio S. I., Mortensen A. (2015). “Sea Urchin aquaculture in Norway,” in Echinoderm aquaculture. Eds. Brown N., Eddy S. (Hoboken, New Jersey: Wiley Blackwell), 147–174.
Stefánsson G., Krisinsson H., Ziemer N., Hannon C., James P. (2017). “Markets for sea urchins: a review of lobal supply and markets,” in Matís report(Reykjavik: Skýrsluágrip Matís ohf - Icelandic Food and Biotech R&D). doi: 10.13140/RG.2.2.12657.99683
Stumpp M., Trubenbach K., Brennecke D., Hu M. Y., Melzner F. (2012). Resource allocation and extracellular acid-base status in the sea urchin strongylocentrotus droebachiensis in response to CO2 induced seawater acidification. Aquat. Toxicol. 110-111, 194–207. doi: 10.1016/j.aquatox.2011.12.020
Suckling C. C. (2012). Calcified marine invertebrates: the effects of ocean acidification (Cambridge: University of Cambridge, UK). doi: 10.1016/j.aquaculture.2011.05.042
Suckling C. C. (2021). Responses to environmentally relevant microplastics are species-specific with dietary habit as a potential sensitivity indicator. Sci. Total Environ. 751, 142341. doi: 10.1016/j.scitotenv.2020.142341
Suckling C. C., Clark M. S., Beveridge C., Brunner L., Hughes A., Cook E., et al. (2014). Experimental influence of pH on the early life-stages of sea urchins II: increasing parental exposure times gives rise to different responses. Invertebr. Reprod. Dev. 58, 161–175. doi: 10.1080/07924259.2013.875951
Suckling C. C., Czachur M. V., Ellis J. E., Davies A. J. (2020a). European Sea urchin somatic and gonadal growth responses to differing stocking densities and seawater flow rates: A case study for experimental design consideration. J. Shellfish. Res. 39, 159–171. doi: 10.2983/035.039.0115
Suckling C. C., Kelly M. S., Symonds R. C. (2020b). “Chapter 11: Carotenoids in sea urchins,” in Sea Urchins: Biology and ecology, 4th ed. Ed. Lawrence J. M. (Amsterdam: Elsevier B.V.), 209–217. doi: 10.1016/B978-0-12-819570-3.00011-1
Suckling C. C., Symonds R. C., Kelly M. S., Young A. J. (2011). The effect of artificial diets on the gonad colour and biomass in the edible sea urchin psammechinus miliaris. Aquaculture 318, 355 – 342.
Suckling C. C., Terrey D., Davies A. J. (2018). Optimising stocking density for the commercial cultivation of sea urchin larvae. Aquaculture 488, 96–104. doi: 10.1016/j.aquaculture.2018.01.022
Sun J., Chiang F.-S. (2015). “Use and exploitation of sea urchin,” in Echinoderm aquaculture. Eds. Brown N. P., Eddy S. D. (Hoboken, New Jersey: Wiley-Blackwell), 25–45.
Symonds R. C., Kelly M., Caris-Veryat C., Young A. (2007). Carotenoids in the sea urchin paracentrotus lividus: occurrence of 9’-cis-echinenone as the dominant carotenoid in gonad colour determination. Comp. Biochem. Physiol. B Biochem. Mol. Biol. 148, 432–444. doi: 10.1016/j.cbpb.2007.07.012
Symonds R. C., Kelly M. S., Suckling C. C., Young A. J. (2009). Carotenoids in the gonad and gut of the edible sea urchin psmamechinus miliaris. Aquaculture 288, 120–125. doi: 10.1016/j.aquaculture.2008.11.018
Tsushima M., Kawakami T., Mine M., Matsuno T. (1997). The role of carotenoids in the development of the sea urchin pseudocentrotus depressus. Invertebr. Reprod. Dev. 32, 149–153. doi: 10.1080/07924259.1997.9672616
Vadas R. L. (1977). Preferential feeding: an optimization strategy in sea urchins. Ecol. Monogr. 47, 337–371. doi: 10.2307/1942173
Wahl M., Hay M. E. (1995). Associational resistance and shared doom: effects of epibosis on herbivory. Oecologia. 102, 329–340. doi: 10.1007/bf00329800
Wangensteen O. S., Turon X., Casso M., Palacín C. (2013). The reproductive cycle of the sea urchin arbacia lixula in Northwest Mediterranean: potential influence of temperature and photoperiod. Mar. Biol. 160, 3157–3168. doi: 10.1007/s00227-013-2303-8
Ward T. J., Kramer J. R., Boeri R. L., Gorsuch J. W. (2006). Chronic toxicity of silver to the sea urchin (Arbacia punctulata). Environ. Toxicol. Chem. 25, 1568–1573. doi: 10.1897/05-299r.1
Keywords: aquaculture, echinoderm, economic, emerging species, low-trophic, novel species, sustainability
Citation: Suckling CC, Zavell MD, Byczynski AL and Takeda BT (2022) Assessing the potential of the unexploited Atlantic purple sea urchin, Arbacia punctulata, for the edible market. Front. Mar. Sci. 9:895061. doi: 10.3389/fmars.2022.895061
Received: 12 March 2022; Accepted: 29 June 2022;
Published: 01 August 2022.
Edited by:
Luisa M. P. Valente, ICBAS - Universidade do Porto, PortugalReviewed by:
Adriana Giangrande, University of Salento, ItalyCopyright © 2022 Suckling, Zavell, Byczynski and Takeda. This is an open-access article distributed under the terms of the Creative Commons Attribution License (CC BY). The use, distribution or reproduction in other forums is permitted, provided the original author(s) and the copyright owner(s) are credited and that the original publication in this journal is cited, in accordance with accepted academic practice. No use, distribution or reproduction is permitted which does not comply with these terms.
*Correspondence: Coleen C. Suckling, Y29sZWVuc3Vja2xpbmdAdXJpLmVkdQ==
†Present address: Max Zavell, Department of Marine Sciences, The University of Connecticut, Groton, CT, United States
Disclaimer: All claims expressed in this article are solely those of the authors and do not necessarily represent those of their affiliated organizations, or those of the publisher, the editors and the reviewers. Any product that may be evaluated in this article or claim that may be made by its manufacturer is not guaranteed or endorsed by the publisher.
Research integrity at Frontiers
Learn more about the work of our research integrity team to safeguard the quality of each article we publish.