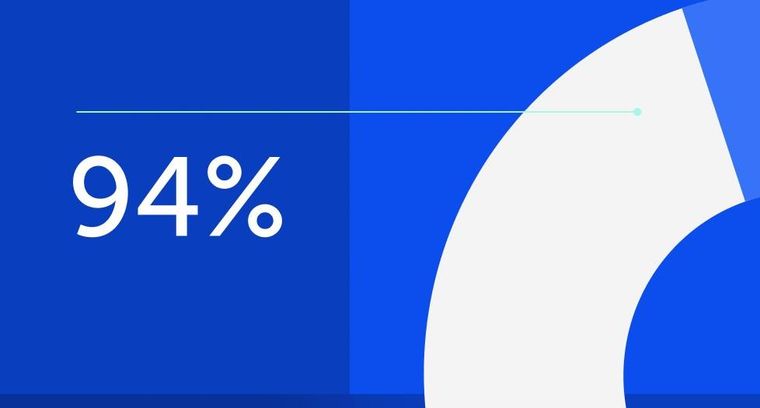
94% of researchers rate our articles as excellent or good
Learn more about the work of our research integrity team to safeguard the quality of each article we publish.
Find out more
ORIGINAL RESEARCH article
Front. Mar. Sci., 15 July 2022
Sec. Marine Biology
Volume 9 - 2022 | https://doi.org/10.3389/fmars.2022.893539
Bioturbation of infauna plays an important role in the biogeochemical processing of sediments. Infaunal animals build burrows and enlarge the sediment-water interface by their activities and so bioturbation is closely related with burrow structure and animal behavior in the sediment. The purpose of this study is to explore the characteristics of Perinereis aibuhitensis burrow structures with the factors of months and animal sizes (0-1g, 1-2g, 2-3g, 3-4g, and >4g), which would also provide useful knowledge of infauna behavioral ecology. The dimension and complexity of the burrows of P. aibuhitensis were measured by dissecting sediments. The results showed that there were three burrow shapes of P. aibuhitensis, i.e., I, Y and U shapes. Overall, the order of abundance of each of the three burrow shapes were I > Y > U. Larger P. aibuhitensis are inclined to build Y- and U-shaped burrows in June and August. There were significant differences in the tunnel diameter, burrow depth and burrow length separately between different polychaete size classes (P< 0.001). In February and August, the burrow depths and burrow lengths of P. aibuhitensis individuals with body weights of 1-2 g and 2-3 g were significantly greater than in other months (P< 0.001). P. aibuhitensis individuals of 1-2 g and 3-4 g body weight had significantly more burrow openings and branches in August than in February (P< 0.001). Within the same month, the burrow HEindex increased with increasing polychaete size, and when the sizes were 1-2 g, 2-3 g and 3-4 g, the complexity in August was higher than that in other months. This study suggests that I-shaped burrow dominants the burrow architecture of P. aibuhitensis. The polychaete with large size has a higher HEindex (burrow complexity) indicating a strong bioturbation ability. Y-shaped burrows are more conducive to the survival of P. aibuhitensis in hot weather. In order to adapt to environmental stresses outside, P. aibuhitensis usually builds deeper burrows.
The ecological functions of bioturbation have been well studied (Yazdani-Foshtomi et al., 2018; Mustajärvi et al., 2019). Bioturbation can promote biogeochemical processes (Fang et al., 2016; Hu et al., 2021). The biological, chemical, and physical properties of sediment were changed by bioturbation. It could also accelerate substance exchange at the sediment-water interface including the flux of dissolved oxygen in the sediment (Forster and Graf, 1995; Duport et al., 2006; Turek and Hoellein, 2015). During the processes of bioturbation, the infauna plays very important role through building burrows, foraging, pumping water and other behaviors (Duport et al., 2006; Mustajärvi et al., 2019). However, little is currently known about the behavior of infauna in the burrow, mainly due to the difficulty of direct observation and the lack of knowledge of aspects such as burrow architecture and food sources.
In previous studies, transparent tubes have been used to observe the behavior of infauna (Kristensen, 1983; Miron and Kristensen, 1993). However, the burrow structure of infauna is diverse (Miron et al., 1991; Kristensen and Kostka, 2005). There are many infauna species which have many different burrow shapes (Miron et al., 1991; Kristensen and Kostka, 2005), and it is difficult to explore the complexity of every species. As with the burrow shapes, the burrow complexity of the infauna varied across species and sediment types. There were only some general descriptions about burrow complexity or some descriptions only with several structural parameters (Correia and Ferreira, 1995; Baird, 2014; Wang et al., 2014). The integration of structural parameters and the use of suitable methods to quantify burrow complexity can promote the research of the infauna biology. Until now, the burrow structure of many dominant species in the sediment are still unknown. Additionally, the composition of the substrate of infauna burrow structure is diverse, including soft mud, sand, and gravel. Suitable sampling methods are helpful to reveal the burrow structure of different substrata and increase the reliability of data. In this study, dissecting is suitable for soft mud.
Polychaetes are common infauna of the coastal intertidal zone and contribute to the remediation of sediment and ecological recovery in natural waters (Koo and Seo, 2017; Chouikh et al., 2020; Gopal et al., 2020; Fang et al., 2021b). Many studies have been conducted on the relationship between sediment and polychaete from several aspects, such as sediment selectivity, irrigation, burrowing, dissolved oxygen and microbial community (Papaspyrou et al., 2006; Shull et al., 2009; Crane and Merz, 2017; Fang et al., 2021a). However, burrow architecture is still not clearly understood. Much of the morphological description is not clear and it is common practice to name burrow shapes based on the similarity between burrow architecture and English-language letter shapes (Davey, 1994; Liu and Hsieh, 2000). Understanding burrow architecture is needed in order to understand the process of polychaetes adapting to the sediment. Burrow architecture may be closely linked to the season, density and species of polychaete (Miron et al., 1991; Kristensen and Kostka, 2005; Koo et al., 2007). In addition, the size of polychaete individuals may also have an influence on the burrow architecture (Davey, 1994).
Perinereis aibuhitensis (Grube, 1878), as one of the important aquaculture species widely distributed in estuarine and tidal zones along the west coast of the Pacific Ocean (Sun and Yang, 2004), has a significant disturbance effect on sediments by burrowing (Fang et al., 2017). After inhabiting for a period of time, there are many openings and branches left by P. aibuhitensis in sediments (Lee and Koh, 1994). These structures can significantly increase the surface area of sediment-water interface, and then increase the occurring of biogeochemical processes and the total content of dissolved oxygen in the burrow (Koo et al., 2005). Different burrow shapes could induce different bioturbation function and biogeochemical processes. In this study, factors influencing P. aibuhitensis burrow architectures were explored, which could provide useful method and data support for the study of infauna behavioral ecology and expand the knowledge of polychaete biology.
The experiment was conducted in an outdoor rectangular cement pool (2.0 m×1.4 m×0.8 m). A flexible detachable wooden frame (1.0 m×0.5 m×0.5 m) was placed in one corner of the pool as an experimental operating space. Sediment (clayey silt) obtained from the natural polychaete habitat was placed into the pool to a depth of 50 cm, then mixed with appropriate amounts of seawater. The pool was then left to settle for 1 month with continuous flowing sea water passing through it. The pool was about 50m away from the seashore. The sediment, water situation, and tide were simulated similar with the natural habitat.
P. aibuhitensis were collected from a polychaete farm in Shandong Province, China (36°50′52″ N, 121°28′31″ E) on 1st, August, 2020. Active animals with different sizes (0.01g - 4.0g) were selected and separated to three groups (>3 g, 1-3 g, and 0.01-1 g) according to the wet weight. The ranges of the animal size covered most sizes of the natural P. aibuhitensis (Choi and Lee, 1997). Then, they were placed on the sediment surface (the flowing water was stopped in advance) in a fan-shaped distribution along the edge of the operating space in the order of wet weight (Figure 1). Fan-shaped placement enabled us to get P. aibuhitensis with different weights more easily. There were about 300 animals in each group. When all P. aibuhitensis had drilled into the sediment, the flowing natural seawater (pumped from seashore, 1.5 - 3 L/min) was restarted, maintaining a water depth of 4-5 cm over the sediment. During the experiment, the flowing seawater was stopped in the morning for six hours then continued in the afternoon every day. The polychaete were fed with 50g artificial shrimp feed (size 1mm) every two days.
Figure 1 The layout of experiment and the placing of P. aibuhitensis. The inlet of water tap was immersed on the bottom of the operating frame.
Sampling was conducted every two months: October and December in 2020 and February, April, June and August in 2021. About 30 burrows were investigated each time. The temperature was measured with a glass thermometer (0.1°C). Then, the flowing seawater in the pool was stopped one hour before sampling. Each sampling was randomly carried out from the edge of the operating frame to further area of the pool. In order to well track a burrow in the soft sediment, edible pigment solution (China, FleurCouleur, main ingredients: water, sorbitol, carmine, brilliant blue, tartrazine, glycerol) with a specific color was injected into the opening of a burrow. Within one minute, the color would be well spread through the whole burrow. Then, the sediment around the burrow was gently removed using a spoon tracking the pigment color, until all the burrow and its branches were exposed. The adjacent burrows were colored with different pigments (green, purple, blue and red) to avoid burrow confusion. Half of burrow along the burrow axis was left in the original sediment, and the other half was removed. During this process, the tunnel diameter (W mm) and depth (D cm) of the burrow was measured with a vernier caliper and a steel ruler, respectively. The length (L cm) of the burrow was traced with a soft cord, which was then measured using a steel ruler. The polychaete in the measured burrow was taken out and weighed using an electronic balance (m, 0.01g) and they were not putted back to the experimental pool. The side view of the burrow architecture was recorded by being hand drawn on paper. At the same time, the number of burrow openings (N1) and burrow branches (N2) were recorded. After each sampling, all the sediment was backfilled. The definitions of burrow shape and burrow architectural parameters are presented in Table 1.
Table 1 Definitions of burrow shapes and burrow architectural parameters (Lee and Koh, 1994; Davey, 1994; Kristensen and Kostka, 2005).
In order to quantify the complexity of an animal’s burrow inside the sediment, the burrow complexity index (HEindex) was designed. In this study, HEindex integrated both burrow dimension (burrow depth, burrow length) and complexity parameters (number of openings and number of branches) of P. aibuhitensis. The burrow structures of infauna may differ from species (Kristensen and Kostka, 2005). Therefore, the parameters used to calculate the HEindex of infauna could be adjusted. A general HEindex is calculated as follows:
Where Xn is the value of the nth parameter, and Xnmax is the maximum value of the nth parameter in samples. Xi is the Xn.
According to the burrow structure of P. aibuhitensis, the HEindex’s formula for P. aibuhitensis burrows is calculated as follows in the present study:
Where N1, N2, D and L represent the number of burrow openings, the number of burrow branches, the burrow depth, and the burrow length, respectively; N1maxN2maxDmax and Lmax are the maximum value of burrow openings, the number of burrow branches, the burrow depth, and the burrow length, respectively.
Structural parameters, including tunnel diameters, burrow depths, burrow lengths, number of burrow openings, number of burrow branches and HEindex, were subjected to two-way analyses of variance (ANOVA) to compare the interaction between size classes and sampling time (two factors). The size classes were ranged as 0-1g, 1-2g, 2-3g, 3-4g, and >4g (five classes) according to the collected wet weight data of polychaete. Performing a log transformation for some data was necessary, which were found not to exhibit normal distributions with homogeneous variance. Subsequently, means with significant difference from each other in the ANOVA tests were determined by least significant differences (LSD) comparisons. A Bonferroni adjustment was used to all structural parameters to avoid the magnification of statistical errors resulting from the repeated uses of ANOVA. Regression analysis was used to analyze the relationship between P. aibuhitensis’s wet weight and tunnel diameter, depth and length. The proportions of different burrow shapes were calculated in different size classes and months, respectively. All analyses were performed using the software language R (Version 3.6.3). Differences were considered statistically significant if P< 0.05.
The average temperature of the overlying water in February, April, June, August, October, and December were 4.7°C, 17.0°C, 22.6°C, 27.2°C, 23.1°C, and 8.3°C, respectively.
The three burrow shapes (I, Y and U) found in this study are shown in Figure 2. The abundances of each of the three burrow shapes were in the order I > Y > U. The proportion of I-shaped burrows was 52%, which was the dominant burrow shape of P. aibuhitensis. The number of I-shaped burrows decreased gradually with increasing polychaete size class, while the number of Y-shaped burrows and U-shaped burrows increased with increasing polychaete size class (Figure 3A). The proportion of I-shaped burrows in February, April, October and December was more than 60%. The Y-shaped burrows and U-shaped burrows accounted for the largest proportions in August, accounting for 53% and 30%, respectively (Figure 3B).
Figure 3 The proportions of the three burrow shapes of P. aibuhitensis created by polychaete of different size classes (A) and at different sampling months (B). The compositions of all burrow shapes are shown in the first pie chart in (A).
Two-way ANOVA tests showed that tunnel diameters were significantly affected by the size class of the P. aibuhitensis and the sampling month (size class: F = 153.901, P< 0.001; sampling month: F = 7.208, P< 0.001). The interaction between size class and sampling month was not significant (F = 1.851, P = 0.0261). There was a significant difference in the tunnel diameters of worms between different size classes in the same month (P< 0.001, Figure 4A). The tunnel diameter increased with increasing polychaete size class. However, there was no significant difference between different sampling months in the same size. The regression equation of the relationship between the mass and the tunnel diameter of P. aibuhitensis was obtained by regression analysis: W = 4.601m0.231 (R2adj = 0.8245, P< 0.001, Figure 5A).
Figure 4 Variation of P. aibuhitensis tunnel diameter (A), burrow depth (B), burrow length (C) with size class and sampling month. Different capital letters represent significant differences between size classes with the same month (P< 0.05). Different lowercase letters represent significant differences between sampling months within the same size class (P< 0.05). Error bars represent SE.
Figure 5 Relationship between mass and tunnel diameter (A) and burrow depth (B) and burrow length (C) of P. aibuhitensis. The lines represent the least-squares linear regression of the filled symbols according to the inset equations.
Two-way ANOVA showed that the burrow depth was significantly affected by size class, sampling month and their interaction (size class: F = 442.3, P< 0.001; sampling month: F = 35.95, P< 0.001; interaction: F = 4.041, P< 0.001). There was a significant difference in the burrow depth of worms between different size classes in the same month (P< 0.001). The burrow depth increased with increasing size class. The burrow depths of February and August were significantly deeper than other months with the same size class (P< 0.001), including 1-2 g, 2-3 g, 3-4 g (Figure 4B). The regression equation of the relationship between the mass and the burrow depth of P. aibuhitensis was obtained by regression analysis: D = 17.986m0.402 (R2adj = 0.7139, P< 0.001, Figure 5B).
Two-way ANOVA showed that the burrow length was significantly affected by size class, sampling month and their interaction (size: F = 88.138, P< 0.001; month: F = 15.535, P< 0.001; interaction: F = 2.964, P< 0.001). There was a significant difference in the burrow length of worms between different size classes in the same month (P< 0.001). The burrow length increased with increasing size class. The burrow length in both June and August was longer than those in February and December in the 1-2 g, 2-3 g, 3-4 g and >4 g size classes (Figure 4C). The regression equation of the relationship between the mass and the burrow length of P. aibuhitensis was obtained by regression analysis: L=33.223m0.366 (R2adj = 0.5786, P< 0.001, Figure 5C).
Two-way ANOVA showed that sampling month significantly affected the number of burrow openings (N1, month: F = 8.476, P< 0.001), but the size class and interaction were not significant (size class: F = 3.083, P = 0.0186; interaction: F = 0.649, P = 0.8456). The number of burrow openings in February and December were significantly less than that in August (P< 0.001), including in the 1-2 g, 2-3 g and 3-4 g size classes (Table 2), and tended to decrease compared with those in other months.
Table 2 The number of P. aibuhitensis burrow openings (N1) and burrow branches (N2) for different size classes and sampling months (mean ± S.E).
Two-way ANOVA showed that sampling month significantly affected the number of burrow branches (N2, F = 8.653, P< 0.001), but the size class did not (F = 1.68, P = 0.159). There was no significant interaction with size class (F = 1.179, P = 0.292). The number of burrow branches in August was significantly higher than in February and October (P< 0.001), including in the 1-2 g and 3-4 g size classes (Table 2). With the same size class, the number of burrow branches in February and December tended to decrease compared with those in other months (Table 2). The number of burrow branches in the 0-1 g size class was significantly lower than in the 1-2 g, 3-4 g and > 4g size classes (P< 0.001) in August.
The mean values of the HEindex were lower than 0.2. Two-way ANOVA showed that the HEindex was significantly affected by size class, sampling month and their interaction (size class: F = 4.442, P = 0.00236; sampling month: F = 9.216, P< 0.001; interaction: F = 2.663, P = 0.00124). There was a significant difference in the HEindex between different size classes including in February and April (P< 0.001, Figure 6). The HEindex increased with the increasing size class. In April, the HEindex in 2-3 g, 3-4 g and >4 g was significantly higher than in 0-1 g and 1-2 g (P< 0.001). The HEindex tended to increase with the increasing size class in October. In the size class 1-2 g, the HEindex in August was significantly higher than other sampling months (P< 0.001). In the size class >4 g, the HEindex in June was significantly higher than in December (P< 0.001).
Figure 6 The P. aibuhitensis burrow HEindex for different size classes (A) and sampling months (B). The black dots in the center of each box chart represent the mean values. Different lowercase letters represent significant differences between different treatments (P < 0.05).
We specifically defined burrow shapes for P. aibuhitensis according to the length of the burrow branch. This definition may also be suitable for burrows of other benthic invertebrates. Kristensen and Kostka (2005) provided definitions of I-, J-, U- and Y-shaped burrows for benthic invertebrates based on the number of burrow openings and of horizontal and vertical axes. Kristensen’s classifications of I- and Y-shaped burrows were consistent with those used in this study if the burrow had 1-2 openings, but were not completely consistent when there were more than two openings and branches in a burrow. Burrow characteristics, such as burrow depth, number of openings, number of branches, vary by species (Kristensen and Kostka, 2005). Species specificity is one of reasons for the discrepancy between this study and Kristensen’s classification of burrow shapes.
In this study, we divided the burrow shapes into three classes: I, Y, and U shapes. Building complex biogenic structures is an expression of animal adaptation to the environment. From the perspective of structural composition, the early construction and later maintenance of the I-shaped burrow is easier than that of Y- and U-shaped burrows. The Y- and U-shaped burrows have a wide range of space extension and more complex structures. The characteristics of Y- and U-shaped burrows may contribute to cooling and storing water (Koo et al., 2005). Thus, Y- and U-shaped burrows may have a survival advantage over I-shaped burrows in the face of extreme environments.
In our first four samplings, more than 60% of burrows were I-shaped. In the last sampling month, i.e. in August, the I-shaped burrows lost dominance and the proportion occupied by Y- and U-shaped burrows was at a maximum. The shapes of Nereis diversicolor burrows often changed from the simpler I- and U-shapes to the more complex Y-shapes (Davey, 1994) over periods of weeks. Michaud et al. (2010) suggested that the burrow structure of polychaetes tends to stabilize over time. These results were obtained by X-ray photography in the laboratory and their experiment was carried out for a few days to one month. Therefore, P. aibuhitensis’s burrow shape would change from I-shaped burrow to more complex shapes over time. In addition, the natural in situ environment is complex, and there may be many conditions affecting the evolution of burrow architecture. The average temperature of the overlying water was maximum in August, minimum in February. This result indicates that the behavior of building Y- and U-shaped burrows of P. aibuhitensis may be triggered by certain environmental conditions. However, more research on an extensive thermal profile is needed before inferring on the role of temperature.
We found that there were differences in burrow shapes and the burrow dimensions among the different size classes of P. aibuhitensis. The activities of foraging, building burrows and water exchange in the sediments of infauna constantly change the structure of the burrow (Hertweck et al., 2007). The change of burrow structure can reflect the intensity of this activity. This activity intensity may be affected by animal size, temperature, tide level and vegetation density (Zheng and Fan, 1986; Davey, 1994; Koo et al., 2005; Koo et al., 2007). In this study, when the animal mass was less than 2 g, I-shaped burrows dominated. More than half of burrows built by animals over 2 g were Y- and U-shaped burrows. Within the same month, the HEindex had a trend of increasing with increase of animal size class. In addition, the tunnel diameter, depth and length of the burrows increased with the increase of animal size. It is a common ecological phenomenon that living space increases with increasing animal size (e.g., Davey, 1994). Reise (1979) found that polychaetes present size stratification, and the habitat depth of adults is greater than that of juveniles. Thus, the bigger polychaete requires a larger living space. Moreover, Kristensen and Kostka (2005) inferred that only organisms with greater muscle capacity could effectively build deep burrows. The larger the size of P. aibuhitensis, the stronger the capability of bioturbation in the burrow. This capability is often of great importance in burrowing progress. As the size of P. aibuhitensis increases, the burrows will be more complex. Driven by need, it will be an inevitable result that burrow dimension of P. aibuhitensis increases with the increase of body size.
The tunnel diameters of P. aibuhitensis’s burrows tended to increase in the winter months such as February and December for the large size classes, i.e., 2-3 g, 3-4 g, >4 g. It is now known that the tunnel diameter of benthos is closely related to body width (Nickell and Atkinson, 1995). When we sampled in February and December, many worms stayed in the burrows in a curled-up position with thin mucus and responded slowly to being disturbed. The tunnel diameter of curled-up position was enlarged. This behavior is similar to the sea cucumber (Apostichopus japonicus) during hibernation (Yu and Chang, 2008). Curling in thin mucus might keep be moist, which might be a manifestation of benthos adapting to a low temperature environment.
According to our result, in the size classes 1-2 g, 2-3 g and 3-4 g, the burrow depths in both February and August were significantly greater than those in other months (P< 0.001). In winter months, polychaete burrows were generally deeper (Zheng and Fan, 1986; Esselink and Zwarts, 1989; Hertweck et al., 2007). In addition, Koo et al. (2007) found that the burrow depths of large polychaetes, such as Periserrula leucophryna and P. aibuhitensis, in summer were significantly greater than those in spring when they conducted the sampling in the upper intertidal zone. The same time, they also observed that the sediment temperature in summer generally decreased with increasing depth. Our measurements showed that the sediment temperature increased with depth in cold months. An inversion of temperature-depth patterns happens in summer and winter. The polychaetes could choose a suitable habitat temperature by burrowing to avoid extreme temperature outside of the sediment, which is an adaptive behavior of polychaetes to the external environment. Hence, our observations suggest that the P. aibuhitensis adapts to extreme ambient temperature conditions by building deeper burrows.
Most P. aibuhitensis inhabit the upper layers of the tidal zone. In order to obtain more food and renew water when the tide comes, they build burrows with very complex structures (Lee and Koh, 1994). In addition to the dense openings on the sediment surface, they also build many branches beneath the sediment. As a subsurface deposit-feeder, the burrow opening is its window to the outside world, and its complex branches are the result of the animal crawling and devouring sediments (Lee and Koh, 1994; Hertweck et al., 2007). Burrows openings and burrow branches can facilitate the ability of the P. aibuhitensis to forage, evade predators, and exchange water in the burrow and are good indicators of the complexity of burrows. Moreover, the HEindex, integrating both dimension and complexity parameters, was used to make a quantitative description for complexity of P. aibuhitensis burrow. The same applies to intraspecific comparisons of burrow complexity among other infauna. This study found that the number of burrow opening, branches and HEindex were mostly higher in August than in other months. Some researchers found that the high temperature in summer promotes the growth of benthic algae, and the abundance of food increases the feeding intensity of P. aibuhitensis. Nevertheless, in summer, the lower concentration of dissolved oxygen in the water can restrict the growth of polychaetes (Forbes and Lopez, 1990) and change the bacteria in the burrow from aerobic to anaerobic (Guo et al., 2022). Therefore, frequent activity of polychaete induced more openings and branches in summer.
Shinn (1968) developed a resin casting method to study the burrow structure of benthos. Following that study, many researchers carried out a series of behavioral ecological studies using the same method. Katz (1980) found that plaster of paris can also be used to cast burrows, which was rarely applied. Davey (1994) used resin castings with X-rays to study the behavior and burrow structure of Nereis diversicolor. Hertweck et al. (2007) not only used resin casts but also dissected dried deposit cores. This study draws on Hertweck’s experimental method. Compared with fixing with chemical materials, the burrow directly excavated on site can better reflect its real appearance, as site excavation can examine a burrow without being affected by the nature of the sediment. Moreover, resin and plaster of paris may not be able to enter every space of a burrow after pouring due to the viscosity of the material as well as the burrow contents. Some researchers have suggested that resin casting is difficult to relate an individual animal to an individual burrow (Davey, 1994). We can ensure the matching of burrow to animal in the method used here, and obtain enough samples to be statistically meaningful. However, some shortcomings are present in the live dissection method used here. For example, all data were collected in one single experimental pool (no replication), which might induce a pseudo-replication issue. Furthermore, the surface area of the burrow wall cannot be measured, nor the three-dimensional burrow shape. The sediment in this experiment primarily consisted of clayey silt, so handling required special care.
There are many burrow shapes of P. aibuhitensis, which were classified into three types of I, U, Y in the present study. The I-shaped burrow is the dominant burrow shape of P. aibuhitensis. The Y-shaped burrows accounted for a large proportion in summer or in larger individuals of P. aibuhitensis. The bioturbation ability of large P. aibuhitensis was strong as their burrows were more complex showing higher HEindex which could well reflex the burrow complexity of infauna. The changes of burrow dimension could also reflect the ecological habits of worms. The burrow depth of P. aibuhitensis was significantly greater in February and August than in other months, which indicated that increasing burrow depth may be a way to adapt to outside environmental stresses for P. aibuhitensis. Therefore, it should increase the sediment depth for P. aibuhitensis aquaculture as they were cultured in about 20cm depth sediment. In addition, in winter, the tunnel diameter of P. aibuhitensis’s burrow was large and P. aibuhitensis mostly curled up in the burrow. Hence, P. aibuhitensis might exhibit hibernation, but further research is needed to confirm this.
The original contributions presented in the study are included in the article/supplementary material. Further inquiries can be directed to the corresponding author.
JinF, JiaF, and ZJ conceptualized the study. JinF and WH was responsible for the experimental design. WH, YZ, JH, JinF, and YY were in charge of the field sampling. WH conducted the analysis of data. WH prepared and wrote the original draft. JinF, WH, YM, and JW reviewed, edited, and wrote the manuscript. All authors read and approved the final manuscript.
This study was funded by the National Natural Science Foundation of China (41876185), Central Public-interest Scientific Institution Basal Research Fund, YSFRI, CAFS (20603022022020). No conflicts of interest exist for authors.
Authors YZ and JW are employed by Rongcheng Chudao Aquatic Products Co., Ltd.
The remaining authors declare that the research was conducted in the absence of any commercial or financial relationships that could be construed as a potential conflict of interest.
All claims expressed in this article are solely those of the authors and do not necessarily represent those of their affiliated organizations, or those of the publisher, the editors and the reviewers. Any product that may be evaluated in this article, or claim that may be made by its manufacturer, is not guaranteed or endorsed by the publisher.
Baird I. R. (2014). Larval Burrow Morphology and Groundwater Dependence in a Mire-Dwelling Dragonfly, Petalura Gigantea (Odonata: Petaluridae). Int. J. Odonatol. 17, 101–121. doi: 10.1080/13887890.2014.932312
Choi J. W., Lee J. H. (1997). Secondary Production of a Nereid Species, Perinereis Aibuhitensis in the Intertidal Mudflat of the West Coast of Korea. B. Mar. Sci. 60, 517–528. doi: 10.1029/96JC02430
Chouikh N., Gillet P., Langston W. J., Cheggour M., Maarouf A., Mouabad A. (2020). Spatial Distribution and Structure of Benthic Polychaete Communities of Essaouira Intertidal Rocky Shores (Atlantic Coast of Morocco). J. Oceanol. Limnol. 38, 143–155. doi: 10.1007/s00343-019-8286-7
Correia A. M., Ferreira Ó. (1995). Burrowing Behavior of the Introduced Red Swamp Crayfish Procambarus Clarkii (Decapoda: Cambaridae) in Portugal. J. Crustacean. Biol. 15, 248–257. doi: 10.1163/193724095X00262
Crane R. L., Merz R. A. (2017). Mechanical Properties of Sediment Determine Burrowing Success and Influence Distribution of Two Lugworm Species. J. Exp. Biol. 220, 3248–3259. doi: 10.1242/jeb.156760
Davey J. T. (1994). The Architecture of the Burrow of Nereis Diversicolor and its Quantification in Relation to Sediment-Water Exchange. J. Exp. Mar. Biol. Ecol. 179, 115–129. doi: 10.1016/0022-0981(94)90020-5
Duport E., Stora G., Tremblay P., Gilbert F. (2006). Effects of Population Density on the Sediment Mixing Induced by the Gallery-Diffusor Hediste (Nereis) Diversicolor O.F. Müller 1776. J. Exp. Mar. Biol. Ecol. 336, 33–41. doi: 10.1016/j.jembe.2006.04.005
Esselink P., Zwarts L. (1989). Seasonal Trend in Burrow Depth and Tidal Variation in Feeding Activity of Nereis Diversicolor. Mar. Ecol. Prog. Ser. 56, 243–254. doi: 10.3354/meps056243
Fang J., Zhang J., Jiang Z., Du M., Liu Y., Mao Y., et al. (2016). Environmental Remediation Potential of Perinereis Aibuhitensis (Polychaeta) Based on the Effects of Temperature and Feed Types on its Carbon and Nitrogen Budgets. Mar. Biol. Res. 12, 1–12. doi: 10.1080/17451000.2016.1177653
Fang J., Wang H., Fang J., Jiang Z., Du M., Gao Y., et al. (2017). The Characteristics of the Sedimentary Environment in a Typical Habitat of Perinereis Aibuhitensis Grube. Prog. Fis. Sci. 38, 1–8. doi: 10.11758/yykxjz.20160801001
Fang J., He W., Meng S., Jiang Z., Fang J., Mao Y., et al. (2021a). The Sediment Selectivity of Perinereis Aibuhitensis Larvae: Active or Passive? Front. Mar. Sci. 8 , 697736. doi: 10.3389/fmars.2021.697736
Fang X., Cozzoli F., Smolders S., Knights A., Moens T., Soetaert K., et al. (2021b). Hindcasting Ecosystem Functioning Change in an Anthropogenized Estuary. Front. Mar. Sci. 8. doi: 10.3389/fmars.2021.747833
Forbes T. L., Lopez G. R. (1990). The Effect of Food Concentration, Body Size, and Environmental Oxygen Tension on the Growth of the Deposit-Feeding Polycheate, Capitella Species 1. Limnol. Oceanogr. 35, 1535–1544. doi: 10.4319/lo.1990.35.7.1535
Forster S., Graf G. (1995). Impact of Irrigation on Oxygen Flux Into the Sediment: Intermittent Pumping by Callianassa Subterranea and “Piston-Pumping” by Lanice Conchilega. Mar. Biol. 123, 335–346. doi: 10.1007/BF00353625
Gopal A., Abdul Jaleel K. U., Parameswaran U. V., Sanjeevan V. N., Saramma A. V., Vijayan A., et al. (2020). Distinctive Community Patterns With Exceptional Diversity of Polychaetes Around a Tectonically Active Archipelago in the Tropical Indian Ocean. Front. Mar. Sci. 7. doi: 10.3389/fmars.2020.00710
Guo X., Song G., Li Y., Zhao L., Wang J. (2022). Switch of Bacteria Community Under Oxygen Depletion in Sediment of Bohai Sea. Front. Mar. Sci. 9. doi: 10.3389/fmars.2022.833513
Hertweck G., Wehrmann A., Liebezeit G. (2007). Bioturbation Structures of Polychaetes in Modern Shallow Marine Environments and Their Analogues to Chondrites Group Traces. Palaeogeogr. Palaeocl. 245, 382–389. doi: 10.1016/j.palaeo.2006.09.001
Hu F., Sun M., Fang J., Wang G., Li L., Gao F., et al. (2021). Carbon and Nitrogen Budget in Fish-Polychaete Integrated Aquaculture System. J. Oceanol. Limnol. 39, 1151–1159. doi: 10.1007/s00343-020-0218-z
Katz L. C. (1980). Effects of Burrowing by the Fiddler Crab, Uca Pugnax (Smith). Estuar. Coast. Mar. Sci. 11, 233–237. doi: 10.1016/S0302-3524(80)80043-0
Koo B. J., Kwon K. K., Hyun J. H. (2005). The Sediment-Water Interface Increment Due to the Complex Burrows of Macrofauna in a Tidal Flat. Ocean. Sci. J. 40, 221–227. doi: 10.1007/BF03023522
Koo B. J., Kwon K. K., Hyun J. H. (2007). Effect of Environmental Conditions on Variation in the Sediment-Water Interface Created by Complex Macrofaunal Burrows on a Tidal Flat. J. Sea. Re. 58, 302–312. doi: 10.1016/j.seares.2007.07.002
Koo B. J., Seo J. (2017). Sediment Reworking by a Polychaete, Perinereis Aibuhitensis, in the Intertidal Sediments of the Gomso Bay, Korea. Ocean. Sci. J. 3, 1–8. doi: 10.1007/s12601-017-0037-0
Kristensen E. (1983). Comparison of Polychaete (Nereis Spp.) Ventilation in Plastic Tubes and Natural Sediment. Mar. Ecol. Prog. Ser. 12, 307–309. doi: 10.3354/meps012307
Kristensen E., Kostka J. E. (2005). Macrofaunal Burrows and Irrigation in Marine Sediment: Microbiological and Biogeochemical Interactions. In Interactions Between Macro- and Microorganisms in Marine Sediments (Eds E. Kristensen, R. R. Haese and J. E. Kostka). American Geophysical Union. Washington, D. C. Coast. Estuar. Stud. 60, 125–157. doi: 10.1029/CE060p0125
Lee Y. H., Koh C. H. (1994). Biogenic Sedimentary Structures on a Korean Mud Flat: Spring-Neap Variations. Neth. J. Sea. Res. 32, 81–90. doi: 10.1016/0077-7579(94)90030-2
Liu P. J., Hsieh H. L. (2000). Burrow Architecture of the Spionid Polychaete Polydora Villosa in the Corals Montipora and Porites. Zool. Stud. 39, 47–54. doi: 10.2108/zsj.17.123
Michaud E., Robert C. A., Stora G. (2010). Sedimentary Organic Matter Distributions, Burrowing Activity, and Biogeochemical Cycling: Natural Patterns and Experimental Artifacts. Estuar. Coast. Shelf. S. 90, 21–34. doi: 10.1016/j.ecss.2010.08.005
Miron G., Desrosiers G., Retière C., Lambert R. (1991). Évolution Spatio-Temporelle Du Réseau De Galeries Chez Le Polychète Nereis Virens (Sars) En Relation Avec La Densité. Can. J. Zool. 69, 39–42. doi: 10.1139/z91-006
Miron G., Kristensen E. (1993). Factors Influencing the Distribution of Nereid Polychaetes: The Sulfide Aspect. Mar. Ecol. Prog. Ser. 93, 143–153. doi: 10.3354/meps093143
Mustajärvi L., Nybom I., Eriksson-Wiklund A. K., Eek E., Cornelissen G., Sobek A. (2019). How Important is Bioturbation for Sediment-to-Water Flux of Polycyclic Aromatic Hydrocarbons in the Baltic Sea? Environ. Toxicol. Chem. 38, 1803–1810. doi: 10.1002/etc.4459
Nickell L. A., Atkinson R. J. A. (1995). Functional Morphology of Burrows and Trophic Modes of Three Thalassinidean Shrimp Species, and a New Approach to the Classification of Thalassinidean Burrow Morphology. Mar. Ecol. Prog. Ser. 128, 181–197. doi: 10.3354/meps128181
Papaspyrou S., Gregersen T., Kristensen E., Christensen B., Cox R. P. (2006). Microbial Reaction Rates and Bacterial Communities in Sediment Surrounding Burrows of Two Nereidid Polychaetes (Nereis Diversicolor and N. Virens). Mar. Biol. 148, 541–550. doi: 10.1007/s00227-005-0105-3
Reise K. (1979). Spatial Configurations Generated by Motile Benthic Polychaetes. Helgolnder. Wiss. Meeresunters. 32, 55–72. doi: 10.1007/BF02189892
Shinn E. A. (1968). Burrowing in Recent Lime Sediments of Florida and the Bahamas. J. Paleontol. 42, 879–894. doi: 10.2307/1302395
Shull D., Benoit J., Wojcik C., Senning J. (2009). Infaunal Burrow Ventilation and Pore-Water Transport in Muddy Sediments. Estuar. Coast. Shelf. S. 83, 277–286. doi: 10.1016/j.ecss.2009.04.005
Sun R., Yang D. (2004). Fauna Sinica. Invertebrata 33. Annelida. Polychaete II Neridida (Beijing: Science Press), 180183.
Turek K. A., Hoellein T. J. (2015). The Invasive Asian Clam (Corbicula Fluminea) Increases Sediment Denitrification and Ammonium Flux in 2 Streams in the Midwestern USA. Freshw. Sci. 34, 472–484. doi: 10.1086/680400
Wang M., Gao X., Wang W. (2014). Differences in Burrow Morphology of Crabs Between Spartina Alterniflora Marsh and Mangrove Habitats. Ecol. Eng. 69, 213–219. doi: 10.1016/j.ecoleng.2014.03.096
Yazdani-Foshtomi M., Leliaert F., Derycke S., Willems A., Vincx M., Vanaverbeke J. (2018). The Effect of Bio-Irrigation by the Polychaete Lanice Conchilega on Active Denitrifiers: Distribution, Diversity and Composition of nosZ Gene. PLos One 13. doi: 10.1371/journal.pone.0192391
Yu M., Chang Y. (2008). The Effects of Low Temperature on Some Physiological Phenomena in Different Population of Sea Cucumber Apostichopus Japonicus Juveniles. J. DaLian. Fish. Uni. 23, 31–36. doi: 10.16535/j.cnki.dlhyxb.2008.01.012
Keywords: benthos, infauna, tidal zone, burrow architecture, burrow complexity
Citation: He W, Fang J, Zhang Y, Yan Y, Zhang Z, Huang J, Fang J, Mao Y, Jiang Z and Wang J (2022) Factors Affecting Burrow Architecture of the Polychaete Perinereis Aibuhitensis. Front. Mar. Sci. 9:893539. doi: 10.3389/fmars.2022.893539
Received: 11 March 2022; Accepted: 17 June 2022;
Published: 15 July 2022.
Edited by:
Francesca Porri, South African Institute for Aquatic Biodiversity, South AfricaReviewed by:
Er Hua, Ocean University of China, ChinaCopyright © 2022 He, Fang, Zhang, Yan, Zhang, Huang, Fang, Mao, Jiang and Wang. This is an open-access article distributed under the terms of the Creative Commons Attribution License (CC BY). The use, distribution or reproduction in other forums is permitted, provided the original author(s) and the copyright owner(s) are credited and that the original publication in this journal is cited, in accordance with accepted academic practice. No use, distribution or reproduction is permitted which does not comply with these terms.
*Correspondence: Jinghui Fang, aHVpODYxQDE2My5jb20=
Disclaimer: All claims expressed in this article are solely those of the authors and do not necessarily represent those of their affiliated organizations, or those of the publisher, the editors and the reviewers. Any product that may be evaluated in this article or claim that may be made by its manufacturer is not guaranteed or endorsed by the publisher.
Research integrity at Frontiers
Learn more about the work of our research integrity team to safeguard the quality of each article we publish.