- 1Department of Forestry, Fisheries and Environment, Cape Town, South Africa
- 2Department of Biological Sciences and Marine Research Institute, University of Cape Town, Cape Town, South Africa
- 3Cape Research Centre, SANParks Scientific Services, Cape Town, South Africa
This study assessed the effectiveness of no-take areas in the Table Mountain National Park MPA in protecting the biodiversity of intertidal rocky shores from impacts of harvesting. Surveys were conducted in areas of controlled harvesting and in no-take areas to compare the densities and sizes of exploited species and the community composition between shores experiencing these two levels of protection, in two ecoregions and on two types of rock substrate. Clear patterns emerged. Firstly, no-take areas had significantly greater densities of the exploited limpets Cymbula granatina, C. oculus and Scutellastra argenvillei, particularly on sandstone shores, relative to their abundance in harvested areas. Conversely, densities of the rarely harvested limpets S. cochlear, S. longicosta and S. granularis did not differ in any manner consistent with protection levels. Secondly, C. granatina and S. argenvillei were significantly larger in no-take areas, although C. oculus displayed the opposite pattern. None of the rarely harvested limpets showed consistent differences in sizes between protection levels. Thirdly, community composition differed between protection levels, particularly on sandstone shores and in the lower intertidal zones where limpets are common. No-take areas were distinguished by greater abundances of harvested limpets and mussels, while harvested areas were dominated by ephemeral and corticated algae, which flourished under reduced grazing pressure by limpets. Our study provides congruent evidence that no-take areas within this MPA are effective in maintaining a natural rocky-shore community composition, and natural densities and size structures of exploited species, testifying to the management success of no-take areas in the Table Mountain National Park, especially on sandstone shores of the Southern Benguela, where densities of harvested species are high, and harvesting is concentrated.
1. Introduction
Harvesting is a major threat to rocky shore biodiversity worldwide. Stocks of many species have been depleted and some face extinction due to overexploitation (Lasiak, 1991a; Espinosa et al., 2006; Espinosa et al., 2009; Coppa et al., 2012; Espinosa et al., 2014; Coppa et al., 2015). To reduce harvesting pressure and allow recovery of overexploited species, Marine Protected Areas (MPAs) have been established and efforts to develop more MPAs have intensified, to the extent that over 6800 MPAs exist worldwide (López et al., 2012; Bennett and Dearden, 2014; Marra et al., 2017). As of 2000, 19 MPAs had been proclaimed on the coast of South Africa (Chadwick et al., 2014); but an additional 21 Offshore Marine Protected Areas were proposed in 2018 (Sink, 2016) and gazetted in 2019 (Findlay, 2020), bringing the total area inside MPAs up to 5% of the Exclusive Economic Zone of South Africa.
As a result of growing numbers of MPAs, questions have been asked worldwide about their effectiveness in mitigating anthropogenic impacts and allowing recovery of depleted populations (Benedetti-Cecchi et al., 2003; García-Charton et al., 2008; Abecasis et al., 2015; Coppa et al., 2015). In South Africa, critical examination of the performance of MPAs has often focused on particular species and specific reserves, such as Langebaan Lagoon in the West Coast National Park (Kerwath et al., 2009), Dwesa-Cwebe Nature Reserve (Lasiak, 1993a; Lasiak, 1993b; Lasiak, 1998, Lasiak, 2006, Branch and Odendaal, 2003; Nakin et al., 2012; Nakin and McQuaid, 2014; Nakin and McQuaid, 2016), Tsitsikamma (Buxton and Smale, 1989; Buxton, 1993; Cowley et al., 2002; Brouwer et al., 2003), de Hoop (Bennett and Attwood, 1991; Bennett and Attwood, 1993; Attwood and Bennett, 1994; Attwood and Bennett, 1995), Goukamma (Kerwath et al., 2008; Götz et al., 2009a; Götz et al., 2009b; Kerwath et al., 2013), Pondoland (Maggs et al., 2013; Mann et al., 2016) and Maputaland (Currie et al., 2012; Floros et al., 2012; Floros et al., 2013; Nel et al., 2013). In addition, two recent overviews of the effectiveness of all South African MPAs in terms of their ecological and socio-economic performances have been undertaken (Kirkman et al., 2021; Mann-Lang et al., 2021). Relatively little attention has been paid to the Table Mountain National Park (TMNP), although Lechanteur (1999) and Lechanteur and Griffiths (2002) demonstrated that reef fish were more abundant inside the Castle Rocks Reserve no-take section than outside, despite this reserve being quite small.
Such evaluations are important for adaptive management of MPAs, particularly as authorities are increasingly moving to an ecosystem-based approach (Crowder and Norse, 2008) and rely on scientific evidence from community-level surveys rather than focusing on individual species alone (Benedetti-Cecchi et al., 2003; García-Charton et al., 2008; Abecasis et al., 2015; Coppa et al., 2015).
Harvesting has direct and indirect impacts on rocky shore organisms. Humans tend to selectively remove preferred species and larger individuals, reducing densities, average sizes, and ages of target species (Durán and Castilla, 1989; Branch and Odendaal, 2003; Micheli et al., 2005; Coppa et al., 2012), altering genetic composition (Fenberg and Roy, 2008), and driving some species to the point of extinction (Espinosa and Rivera-Ingraham, 2017, Carballo et al., 2019). As a result, greater densities and larger sizes of frequently harvested organisms are commonly recorded inside no-take MPAs compared to outside. Examples of the effects of protection include the kelp Durvillaea antarctica (Castilla and Bustamante, 1989), the limpets Helcion concolor (Branch, 1975), Cymbula oculus (Branch and Odendaal, 2003), Fissurella crassa, F. limbata (Godoy and Moreno, 1989; Durán and Castilla, 1989), Lottia gigantea (Sagarin et al., 2007; Fenberg and Roy, 2012), several European species of Patella (Espinosa et al., 2009; López et al., 2012; Zarrouk et al., 2016; Marra et al., 2017), the mussel Perna perna (Lasiak, 1998; Rius et al., 2006) and the urchins Strongylocentrotus franciscanus (Tuya et al., 2000) and Paracentrotus lividus (Bertocci et al., 2014).
However, the establishment of MPAs can also be associated with declines in the densities of some species through the indirect effects of protecting their predators or competitors (McClanahan and Muthiga, 1988; McClanahan and Arthur, 2001; Guidetti, 2006; Giakoumi and Pey, 2017). This can neutralize or even reverse protection effects for some species: as their predators or competitors increase in abundance, subordinate competitors or prey become depleted and fail to respond positively to protection. For example, Nakin and McQuaid (2014) recorded similar densities for Helcion concolor and Scutellastra longicosta inside and outside Dwesa-Cwebe Nature Reserve on the east coast of South Africa, despite both species being harvested in that region. Similarly, community composition did not differ in different zones of the Tuscan Archipelago National Park (Benedetti-Cecchi et al., 2003) or in Torre Guaceto MPA in the Mediterranean (Fraschetti et al., 2005). Therefore, the effects of MPAs can be area-specific and species-specific, or reflect the efficiency of enforcement, thus indicating a need for evaluation on a case-by-case basis (Benedetti-Cecchi et al., 2003; Nakin et al., 2012).
Reduction in the abundance of large individuals of herbivorous species caused by harvesting can alter ecosystem functioning by weakening their effects on other species (Underwood and Jernakoff, 1984; Lasiak and Field, 1995; Lasiak, 1998; Benedetti-Cecchi et al., 2003; Fraschetti et al., 2005; Sagarin et al., 2007). Conversely, such interactions among species may be strengthened when target species that are protected inside MPAs increase in abundance, leading to changes in community composition as prey or competitors are driven to lower levels (Durán and Castilla, 1989; Lasiak and Field, 1995; Lasiak, 1998; Benedetti-Cecchi et al., 2003; Fraschetti et al., 2005; Rius et al., 2006; Martins et al., 2008). Consequently, the community composition of harvested and no-take areas often differ, as reflected by the prevalence of species such as limpets, abalone and mussels in the absence of harvesting in protected areas, whereas harvested areas tend to be dominated by algae as a result of the removal of grazers or species that compete with algae for space (Hockey and Bosman, 1986; Lasiak and Field, 1995; Martins et al., 2008). For example, depletion of the keystone predator Concholepas concholepas on exploited rocky shores in Chile has led to a domination by its prey, the mussel Perumytilus purpuratus (Durán and Castilla, 1989). In the light of these direct and indirect effects of protection it is important to evaluate the effectiveness of MPAs in conserving the community composition of ecosystems as a whole, as well as in preserving the demographics and dynamics of individual populations (Henriques et al., 2017).
Our study examines the effects of the Table Mountain National Park (TMNP) MPA on, firstly, densities and sizes of key intertidal limpets and, secondly, rocky-shore community composition, taking into account differences between the two ecoregions that our study spanned, and between rocky substrate types that are present in the park. Our primary comparisons were between the densities and sizes of targeted species, and the community compositions, of sites that are fully protected (i.e., ‘restricted’ or ‘no-take’ areas) versus corresponding areas where harvesting is permitted but controlled (termed ‘controlled’ or ‘harvested’ areas). However, we sampled a sufficient number of replicate sites also to be able to examine the effects of biogeography and rock type. These are novel steps, for few studies of the efficacy of MPAs take into account the complicating effects of biogeography and rock type (see, for example, Barrett et al., 2009; Coppa et al., 2015; Dimitriadis et al., 2018). Bertocci et al. (2012) have demonstrated the difficulties of detecting protection effects in the face of natural spatial and temporal variability of populations.
The following hypotheses were tested: (1) There is a selective removal of large individuals of the targeted limpets Cymbula granatina and Scutellatra argenvillei in areas where harvesting takes place. (2) In situ Densities and average sizes of the frequently harvested limpets C. granatina, C. oculus and Scutellastra argenvillei will be greater in no-take areas than in harvested areas. (3) For limpets that are rarely harvested, i.e., Scutellastra cochlear, S. longicosta and S. granularis, protection will not have an effect: their densities and sizes will not differ consistently between no-take and harvested areas. (4) Differences will exist in community composition between no-take and harvested areas, with functional groups that are susceptible to harvesting being more abundant in no-take than harvested areas. (5) Functional groups that are not harvested may proliferate in exploited areas due to the removal of groups that normally control their abundance. (6) Protection effects will differ between ecoregions and rock types, as species assemblages differ between the ecoregions, and the abundances of some species are influenced by rock type.
2. Materials and methods
2.1. Study design and sites
The TMNP MPA was established in 2004 and occupies a transition between the Agulhas and Southern Benguela ecoregions, with Cape Point constituting a well-known coastal biogeographic break (Emanuel et al., 1992; Sink et al., 2019), across which gene flow is reduced (Von der Heyden, 2009; Wright et al., 2015). We surveyed shores on both the east and west sides of the Cape Peninsula, thus covering both ecoregions. Diverse rock types are present, but Cape Granite and Table Mountain Sandstone (TMS) predominate (McQuaid and Branch, 1985; Pfaff et al., 2019). Both rock types are represented in no-take ‘restricted’ areas and in harvested ‘controlled’ areas (Figure 1). Site names, protection level, rock type, ecoregion and GPS coordinates are recorded in Supplementary Table A1.
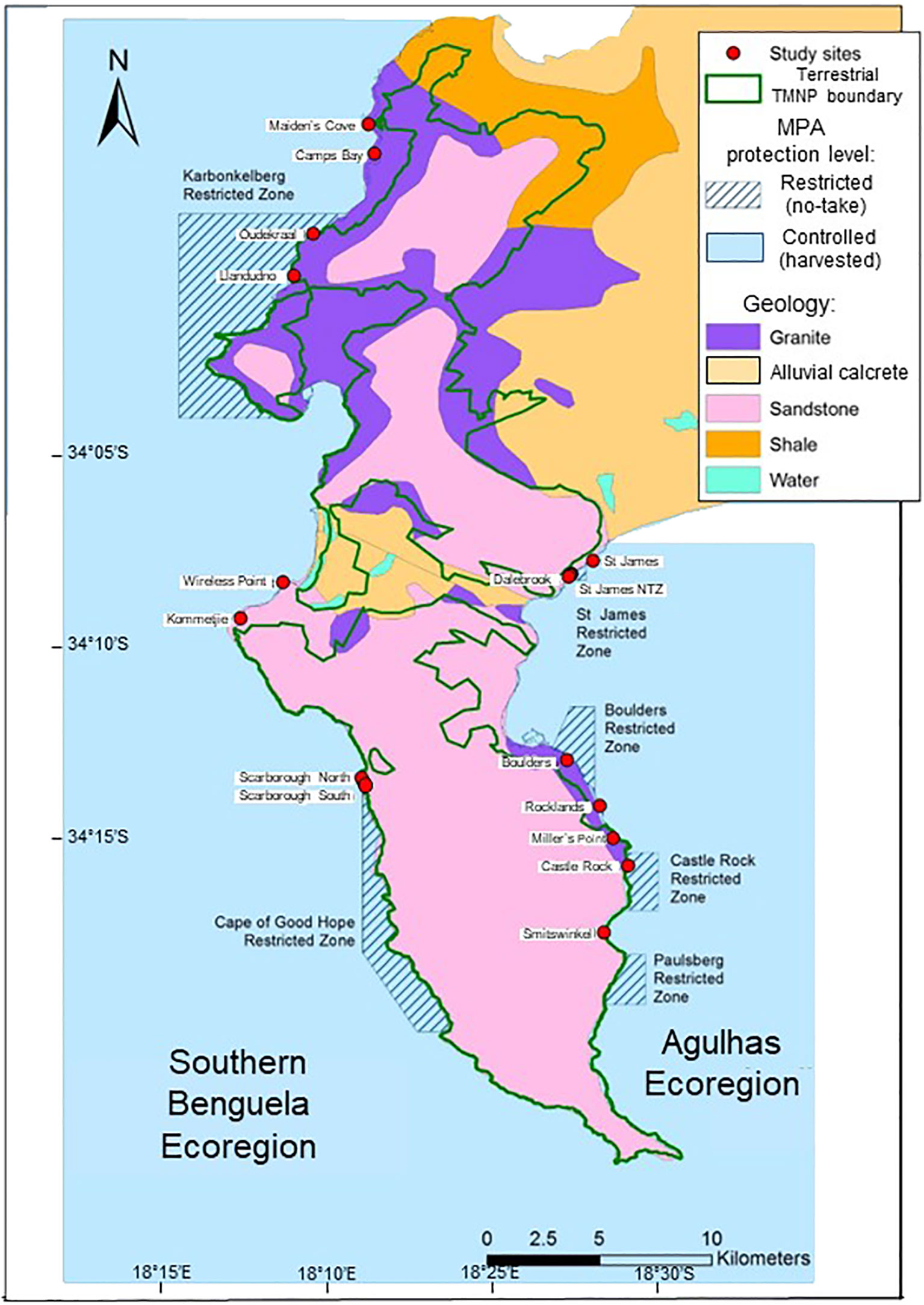
Figure 1 Map of the Cape Peninsula showing the Table Mountain National Park Marine Protected Area (TMNP MPA), with Controlled zones where harvesting is permitted (blue unmarked areas) and Restricted ‘no-take’ zones (blue hatched areas). Study sites are marked with red dots, and colour coding of the land indicates different geological formations.
Sixteen sites were selected for study, representing two protection levels, two ecoregions and two rock types, with two replicate sites for each combination. Site selection was spatially randomized by placing replicate sites in different no-take areas. Wave action was standardized by selecting sites that fell in the range defined as ‘semi-exposed’ to ‘exposed’ by Steffani and Branch (2003), thus avoiding ‘sheltered’ or ‘extremely exposed’ shores.
2.2. Sampling procedure
Surveys of community composition were conducted at all 16 sites. Sampling was undertaken under permits issued by the Department of Forestry Fisheries and Environment and SANParks. For each site, four intertidal zones were identified based on their relative level and biotic indicators, termed the low, mid, high and top shore, at respective heights of approximately +0.2, +0.8, +1.2 and +1.5 m above low water spring tide (mean spring tidal range being 1.87 m). In each zone, 15 replicate 50×50-cm quadrats (divided into 25 cells of 4% cover each) were randomly placed and visually non-destructively sampled. All macro-organisms were identified and quantified as percentage cover for sessile organisms and as counts for mobile fauna (Bustamante and Branch, 1996; Blamey and Branch, 2009). To standardize, counts were later converted to percentage cover (Wieters et al., 2009).
Densities and sizes (shell lengths) of three limpet species commonly harvested in the region, Cymbula granatina, C. oculus and Scutellastra argenvillei (Eekhout et al., 1992; Bustamante et al., 1995; Branch and Odendaal, 2003), and three species of limpet rarely harvested there, S. cochlear, S. granularis and S. longicosta, were compared between harvested and no-take areas. Classification of species as ‘commonly harvested’ or ‘rarely harvested’ was based on our own observations, as reported in the results, and those of Lasiak (1991b) and Nakin and McQuaid (2014). These authors did report Scutellastra longicosta as being harvested on the south-east coast of South Africa, but there was no evidence of this in our study area. Measurements of densities and sizes were made separately in all four intertidal zones within the 15 replicate quadrats, with a minimum of 50 individuals being measured per species per zone per site.
Data for the shell lengths (measured with calipers to 0.1 mm accuracy) and relative abundances of shellfish (from counts of material that had been harvested) were obtained from South African National Parks field rangers’ records, and opportunistically from confiscated material and discarded shucked shells. These data allowed assessment of which species are most often harvested, and whether harvesters are selectively collecting larger individuals relative to the size spectrum available. The latter comparisons were restricted to C. granatina at two sites, and S. argenvillei at one site, as it was only there that sample sizes of harvested limpets were large enough for rigorous statistical comparisons.
2.3. Data analyses
To compare the mean sizes of Cymbula granatina, individuals collected by harvesters at Wireless Point and Kommetjie with those present on the shore at these respective sites and, likewise, for Scutellastra argenvillei at Wireless Island, Student’s t-tests were used. If assumptions of normality and equality of variance were not met, Mann-Whitney U tests were applied.
Univariate GLMs were employed to test for harvesting effects on densities and sizes (i.e., shell lengths) of individual limpet species. Not all species were present in both ecoregions or for some ecoregion-rock type combinations, therefore the data were analyzed separately per ecoregion and rock type. Individual GLMs included factors Protection level (as proxy for harvesting effects) and Site nested within Protection level. Significance levels were Bonferroni-adjusted to account for type-I error inflation associated with multiple tests of a variable.
Multivariate analyses to determine harvesting effects on the entire rocky shore community were conducted separately for each intertidal zone representing a unique biotope, i.e. low, mid, high and top zones. To meaningfully compare community composition between the two ecoregions comprising different species but comparable functional groups, species within each quadrat were grouped into functional groups based on criteria specified below (Supplementary Table A2). Grouping may obscure the effects for rare species, but reduces statistical challenges associated with numerous dependent variables (Warton et al., 2012; Warton et al., 2015). Algal grouping was based on morphology, productivity and susceptibility to herbivory (Steneck and Dethier, 1994). Invertebrates were grouped by trophic category and foraging strategy (Wieters et al., 2009). Herbivores were divided into ‘grazers’ that crop algae, ‘trappers’ snaring drift algae or fronds (individuals of the limpets Cymbula granatina > 30 mm and Scutellastra argenvillei > 40 mm), and ‘gardeners’ cultivating patches of algae.
Percentage cover data of functional groups were analyzed with four-way multivariate GLMs using the mvabund package in R (Wang et al., 2012). The factors included in the models were Ecoregion, Rock Type, Protection (all fixed and crossed) and Site (random, nested in Rock Type). The nature of any significant differences was statistically explored using post-hoc pairwise multivariate comparisons included in the PERMANOVA toolbox in PRIMER; multidimensional scaling (MDS) ordination plots were used to visualize and interpret the community patterns. SIMPER analyses explored which functional groups were responsible for observed differences between harvested and no-take areas of the MPA.
Statistical analyses were performed in R (R Core Team, 2019), using the car package (Fox and Weisberg, 2019) and mvabund package (Wang et al., 2012). Pairwise PERMANOVA, MDS and SIMPER analyses were conducted with PRIMER V7 (Clarke and Gorley, 2015).
3. Results
3.1. Abundances and sizes of limpets collected by harvesters
Harvesters at Kommetjie, Wireless Point and Wireless Island targeted three species of limpets, together with the mussels Choromytilus meridionalis and Mytilus galloprovincialis (pooled as ‘mussels’ as rangers’ records treated them as a group) (Table 1). Cymbula granatina was the most harvested limpet, followed by C. oculus. Scutellastra argenvillei was collected at Wireless Island only, while S. cochlear and S. granularis were never taken.
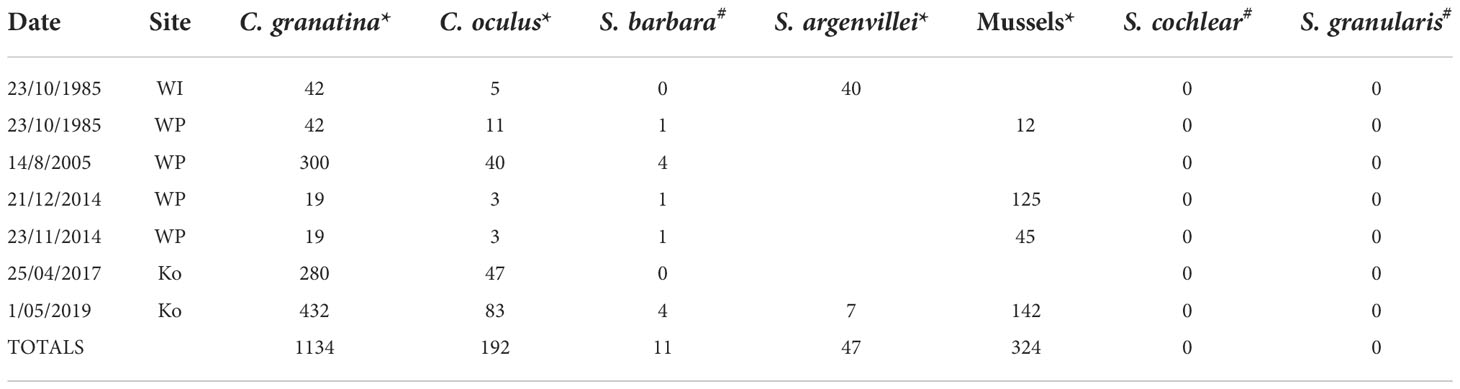
Table 1 Number of individuals harvested for seven species of commonly harvested (*) and rarely harvested (*) species at Ko, Kommetjie, WI, Wireless Island and WP, Wireless Point, the three most heavily harvested sites.
Harvesters focused on large individuals of C. granatina at both sites examined, with the difference between the mean sizes of harvested individuals and those in the natural populations being significant (Mann Whitney U test, p ≤ 0.001 in both cases; Figures 2A, B). The smallest harvested individuals were respectively 40-45 mm and 60 mm, whereas natural populations had individuals as small as 5-20 mm. Although a similar pattern emerged for S. argenvillei, with harvested individuals falling in the range 60–100 mm and the natural population spanning 5–100 mm, the difference in mean sizes was in this case not significant (Figure 2C; Mann Whitney U test, p = 0.3094).
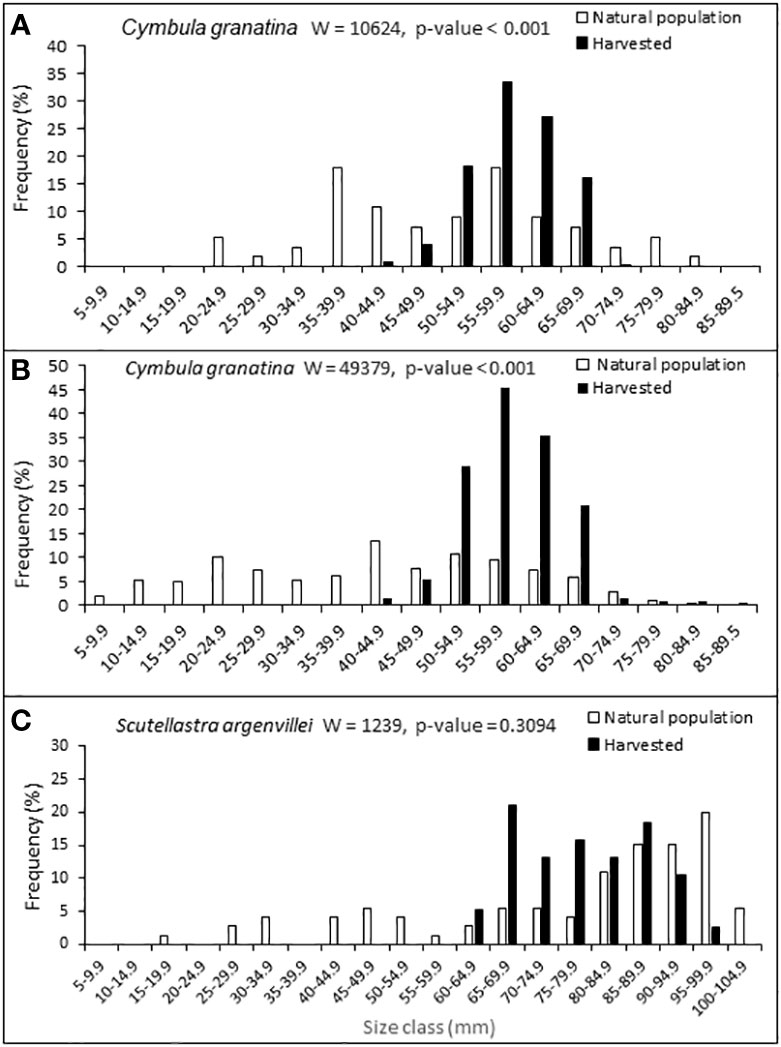
Figure 2 Comparisons of shell lengths of harvested individuals and those in natural populations, for (A) C granatina at Kommetjie, (B) C granatina at Wireless Point and (C) S. argenvillei at Wireless Island. Mann Whitney U tests (W) and probabilities of differences between sizes of harvested and natural samples are shown. Sample sizes for respectively harvested and natural samples were n=280 and n=56 for Kommetjie, n=298 and n=209 for Wireless Point, n=38 and n=72 for Wireless Island.
3,2. Densities of limpets
On sandstone (TMS), all three commonly harvested limpets Cymbula granatina, C. oculus and Scutellastra argenvillei had significantly lower densities in harvested areas than in no-take areas. Harvesting effects were significant despite significant variability between replicate sites in most cases and the reduced power of Bonferroni-adjusted analyses. Densities on granite were low regardless of the level of protection and showed no harvesting effects or - in the case of C. oculus - greater densities in Agulhas harvested areas (Supplementary Table A3 post-hoc ; Figures 3A–C).
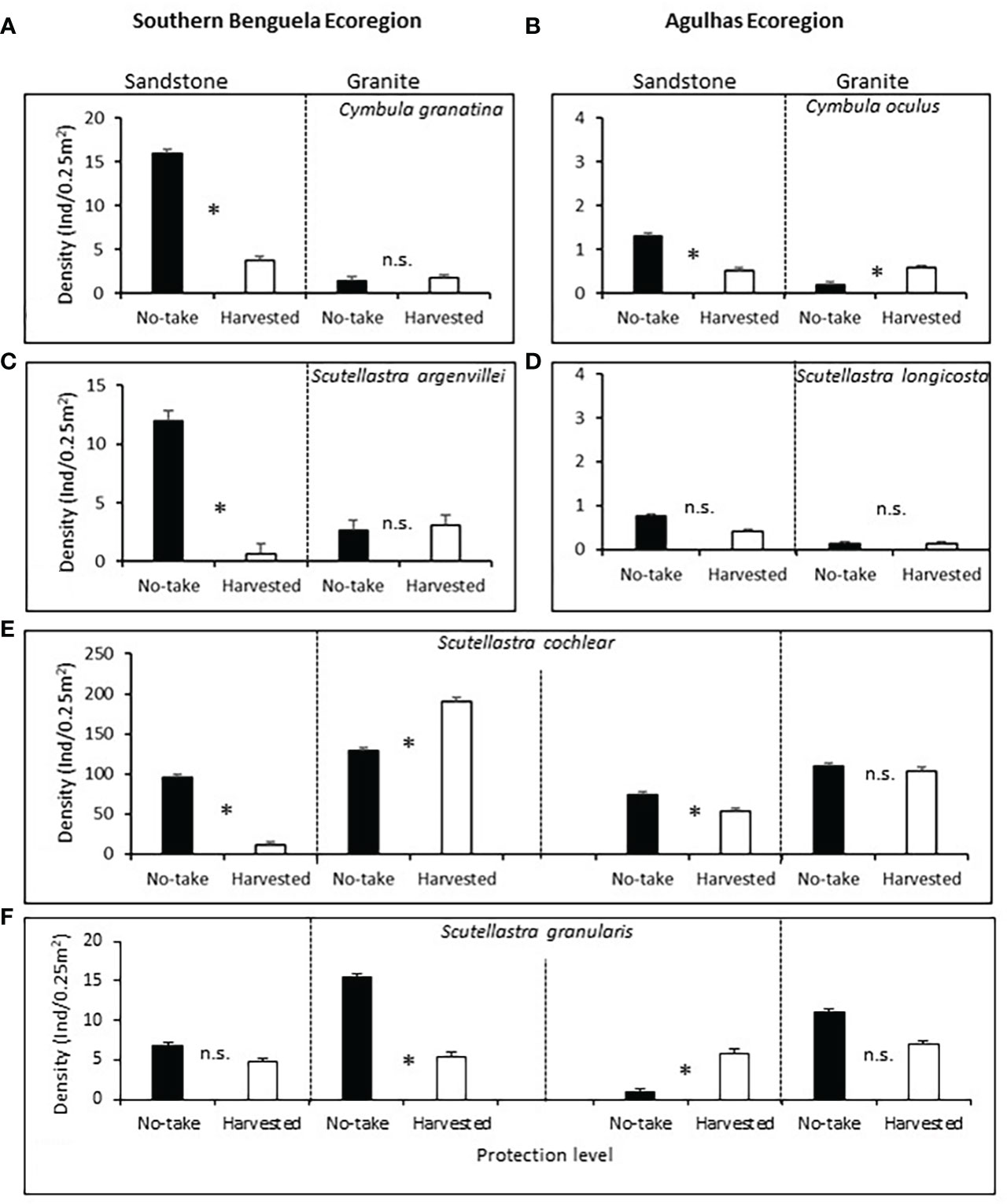
Figure 3 Mean densities (+1SE) of the frequently harvested limpets (A) Cymbula granatina, (B) C oculus and (C) Scutellastra argenvillei, and the rarely harvested limpets (D) S. longicosta, (E) S. cochlear and (F) S. granularis, with regard to protection level and rock type. The first four species (A-D) occur predominantly in only one ecoregion, so this factor was not included for their analyses, but was included in the analyses for the fifth and sixth species), which occur in both ecoregions. Ind, individuals. Asterisks between bars, Bonferroni-corrected significant differences; n.s., non significant.
In contrast, densities of the rarely harvested limpets showed inconsistent or no effects of protection. Scutellastra longicosta densities did not differ significantly between levels of protection on either rock type (Figure 3D). Scutellastra cochlear densities were lower in harvested areas on TMS in both ecoregions, while no or reversed protection effects existed on granite (Figure 3E). Scutellastra granularis also showed inconsistent harvesting effects, with greater densities in protected than harvested sites on granite in the Southern Benguela, whereas no significant or negative effects of protection were evident at the other three combinations of ecoregion and rock type (Figure 3F).
3.3. Sizes of limpets
Of the commonly harvested limpets, Cymbula granatina was significantly larger inside the no-take than the harvested areas, regardless of rock type (Supplementary Table A4; Figure 4A). Cymbula oculus was counterintuitively larger in the harvested areas, but only on sandstone shores (Figure 4B). For Scutellastra argenvillei, post-hoc sizes were larger in the no-take than harvested areas, but on sandstone shores only; protection had no effect on granite, where its densities were low (Figure 4C).
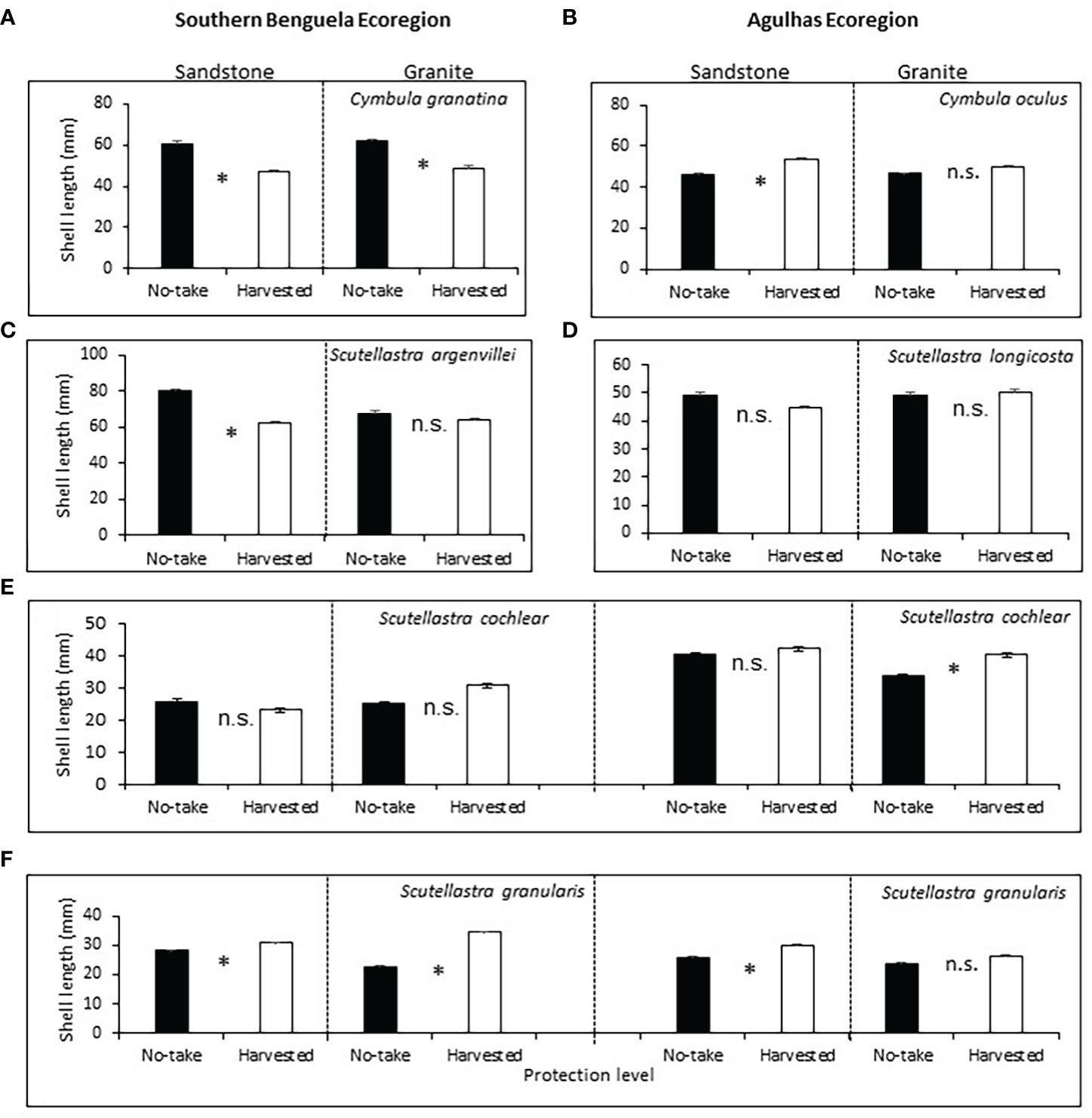
Figure 4 Mean shell lengths (+SE) of limpets, in relation to protection levels, rock types and ecoregions (in the case of species that occurred in both ecoregions). Asterisks between bars indicate Bonferroni-corrected significant differences. For further details see caption to Figure 3.
Of the rarely or never harvested limpets (Supplementary Table A4), the size of S. longicosta, was not affected by either protection level or rock type (Figure 4D). For S. cochlear, sizes were greater in harvested than no-take areas in the Agulhas ecoregion on granite, but protection had no effect elsewhere (Figure 4E). For S. granularis, sizes were significantly larger in harvested areas in the Benguela on both TMS and granite and on Agulhas TMS, but not significantly different on Agulhas sandstone (Figure 4F).
3.4. Community effects
MDS plots of the four zones on the shore exhibited a clear biogeographic distinction between the Southern Benguela and Agulhas ecoregions (Figure 5). Differences also existed between replicate sites (Supplementary Table A5). The distinctions between protection levels and sites emerged more clearly when the data were disaggregated to separate the ecoregions and rock types and bring to the fore the protection and site effects (Supplementary Figure A1). Over and above the regional differences, clear harvesting effects existed, but they differed among intertidal zones, ecoregions and rock types. In the low intertidal zone (Supplementary Figure A1A), dissimilarities between harvested and no-take areas existed for TMS but not granite ledges where site differences dominated; and were more pronounced in the Southern Benguela than the Agulhas ecoregion. Similarly, in the mid intertidal zone (Supplementary Figure A1B) harvesting effects existed only for TMS and not granite shores, in both ecoregions (Supplementary Figure A1B). In the high intertidal zone, harvesting effects were most prominent on Southern Benguela TMS, while site differences overrode harvesting differences in all other cases (Supplementary Figure A1C). On the top intertidal zone, site differences were pronounced, overriding harvesting effects in all cases (Supplementary Figure A1D).
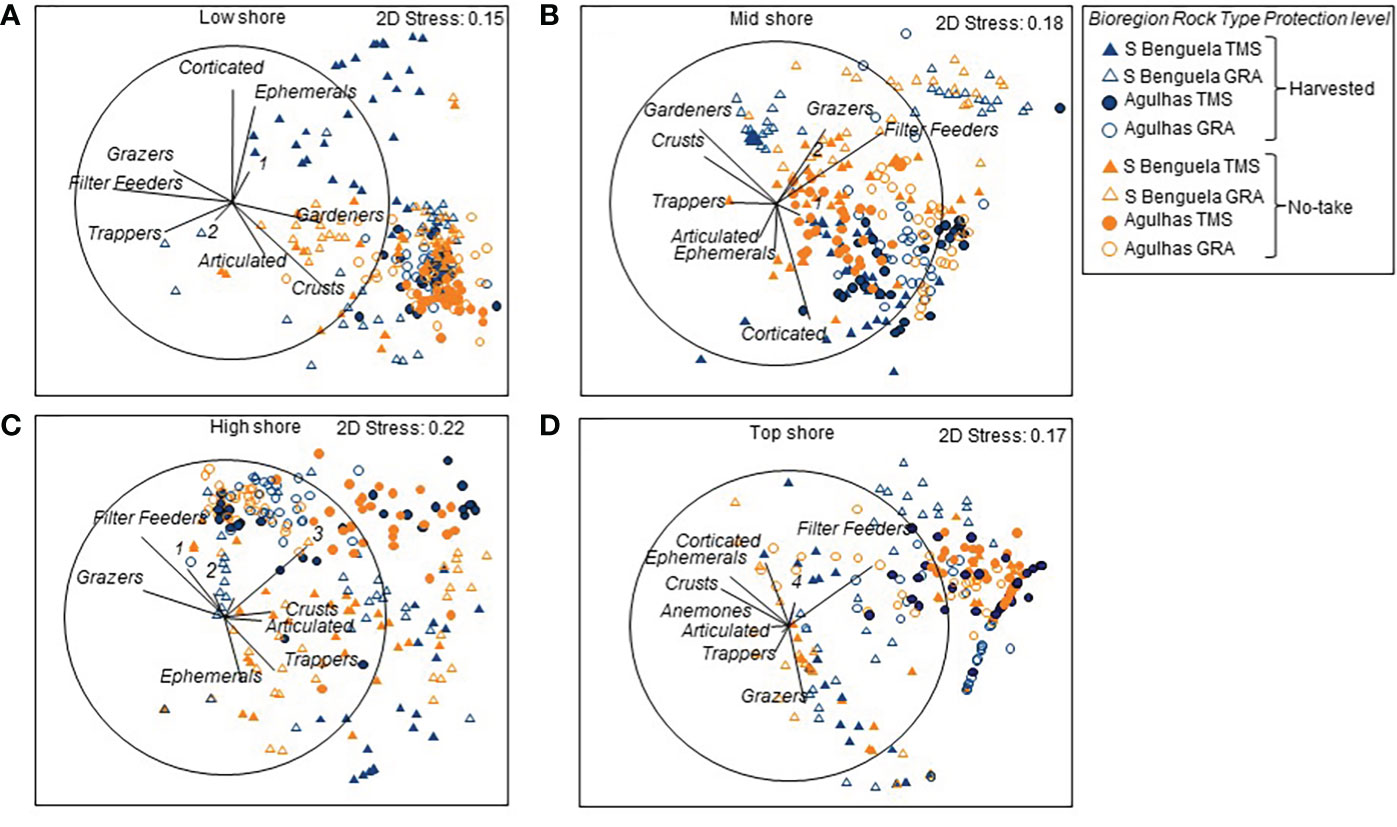
Figure 5 MDS plots showing differences in community composition among quadrats reflecting the effects of Protection, Ecoregion and Rock type for (A) low, (B) mid, (C) high and (D) top zones of 16 sites within the Table Mountain national `park MPA, which spans the Southern Benguela (S Benguela) and Agulhas ecoregions and features Table Mountain sandstone (TMS) and granite (GRA) For clarity some functional groups are numbered: 1 – Predators ad scavengers; 2 = anemones; 3 = Corticated alage; 4 – Gardeners.
3.5. Functional groups distinguishing between harvested and no-take areas
SIMPER analyses (Figures 6–9), coupled with the MDS ordinations discussed above, indicated that marked dissimilarities existed between no-take and harvested areas, but varied among ecoregions, rock types and intertidal zones. On the low shore (Figure 6), ephemeral algae were consistently more abundant on harvested shores, most obviously in the Southern Benguela ecoregion. Corticated algae followed suit and distinguished harvested sites in all cases except Southern Benguela granite. Algal crusts were more prevalent on harvested shores on granite in both ecoregions, but on sandstone the reverse was true. Herbivores (particularly trappers and gardeners) were more abundant at no-take sites.
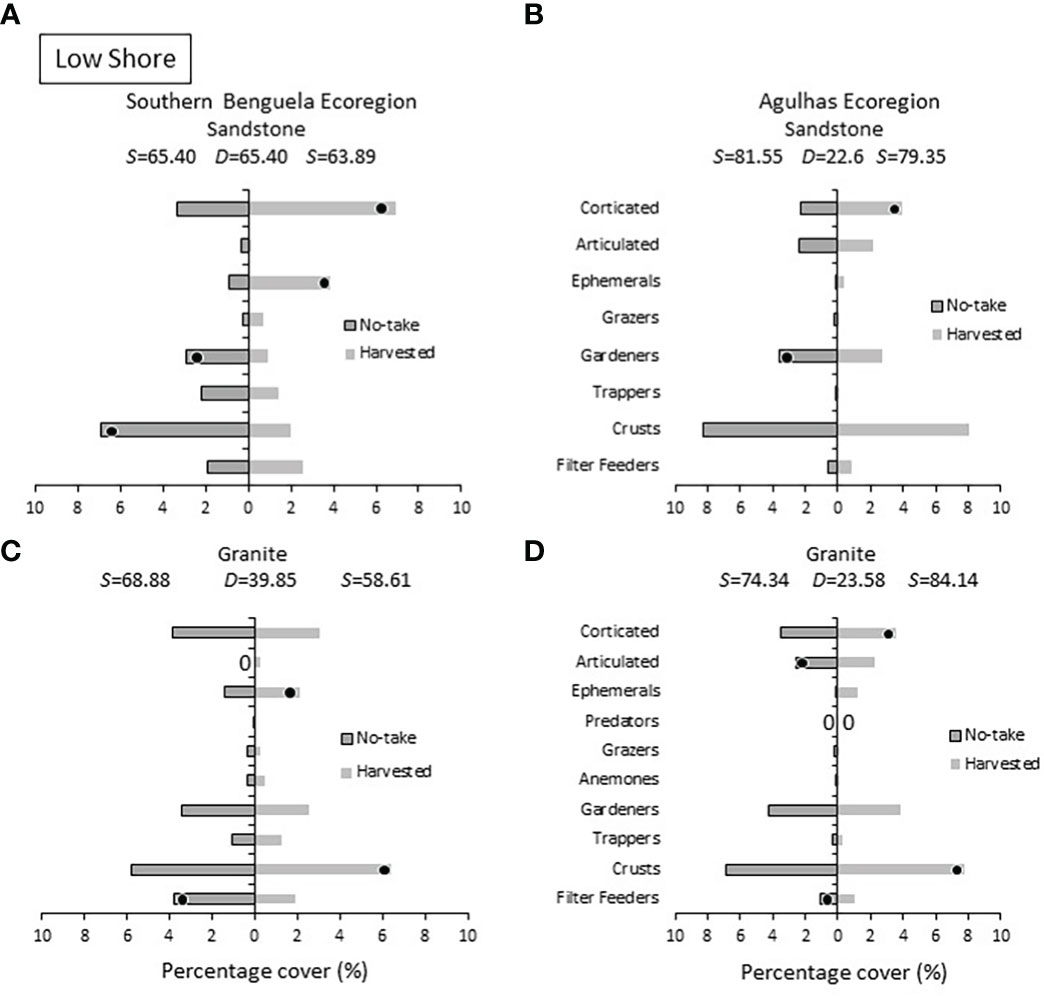
Figure 6 Low shore: Percentage cover of groups responsible for the similarities (S) within harvested or no-take areas and the dissimilarities (D) between them, on sandstone in (A) the Southern Benguela and (B) in the Agulhas Ecoregion, and on granite in (C) the southern Benguela and (D) Agulhas. Black dots identify groups distinguishing between harvesting and no-take zones and are placed in the zone with the greatest abundance; 0 = absence.
Within the mid intertidal zone (Figure 7), corticated algae were more abundant on harvested shores (with the exception of Southern Benguela granite), and harvested sites housed larger amounts of ephemeral algae than no-take sites in the Southern Benguela ecoregion. Algal crusts were, contrary to the low-shore pattern, more abundant on no-take shores, being distinguishing members of no-take shores in three cases. Filter feeders also distinguished no-take sites in three of the four comparisons.
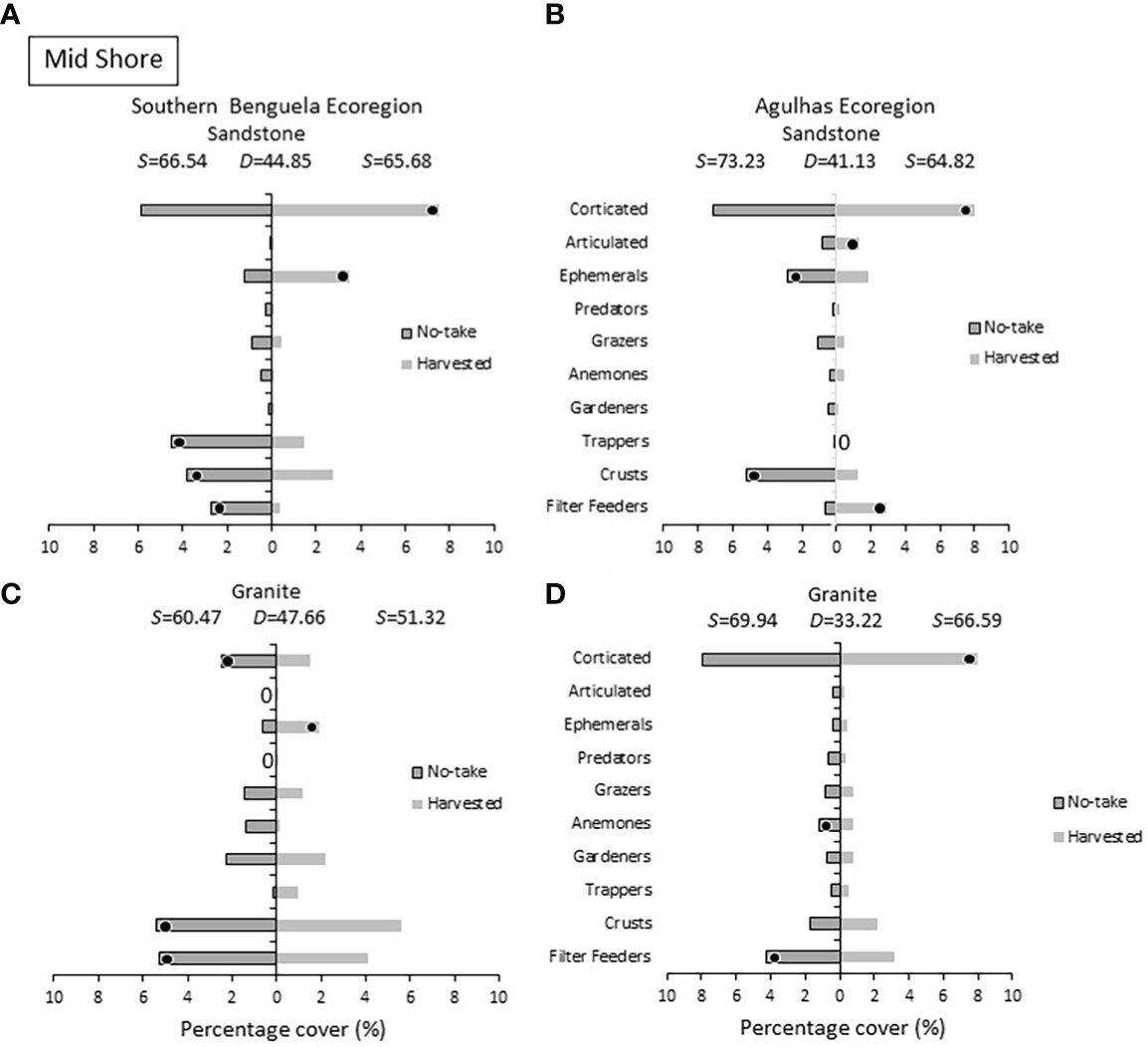
Figure 7 Mid shore: Percentage cover of groups responsible for similarities (S) within harvested or no-take areas and dissimilarities (D) between them, on (A) sandstone in the Southern Benguela and (B) in the Agulhas ecoregions, and on granite in (C) the southern Benguela and (D) Agulhas. See caption for Figure 6 for further interpretation.
In the high shore (Figure 8), ephemeral algae were prevalent at the majority of harvested sites, distinguishing these shores in three of four cases. Corticated algae, however, reversed the trend evident lower on the shore and were more abundant at, and distinguished, no-take sites in two instances. Of the herbivores, trappers yielded no consistent results with respect to protection level, but greater grazer abundances were found on protected shores in three cases, two of which diagnostic of no-take shores. Filter feeders yielded mixed results, being more abundant on no-take shores in two cases, but less abundant there in the other two cases.
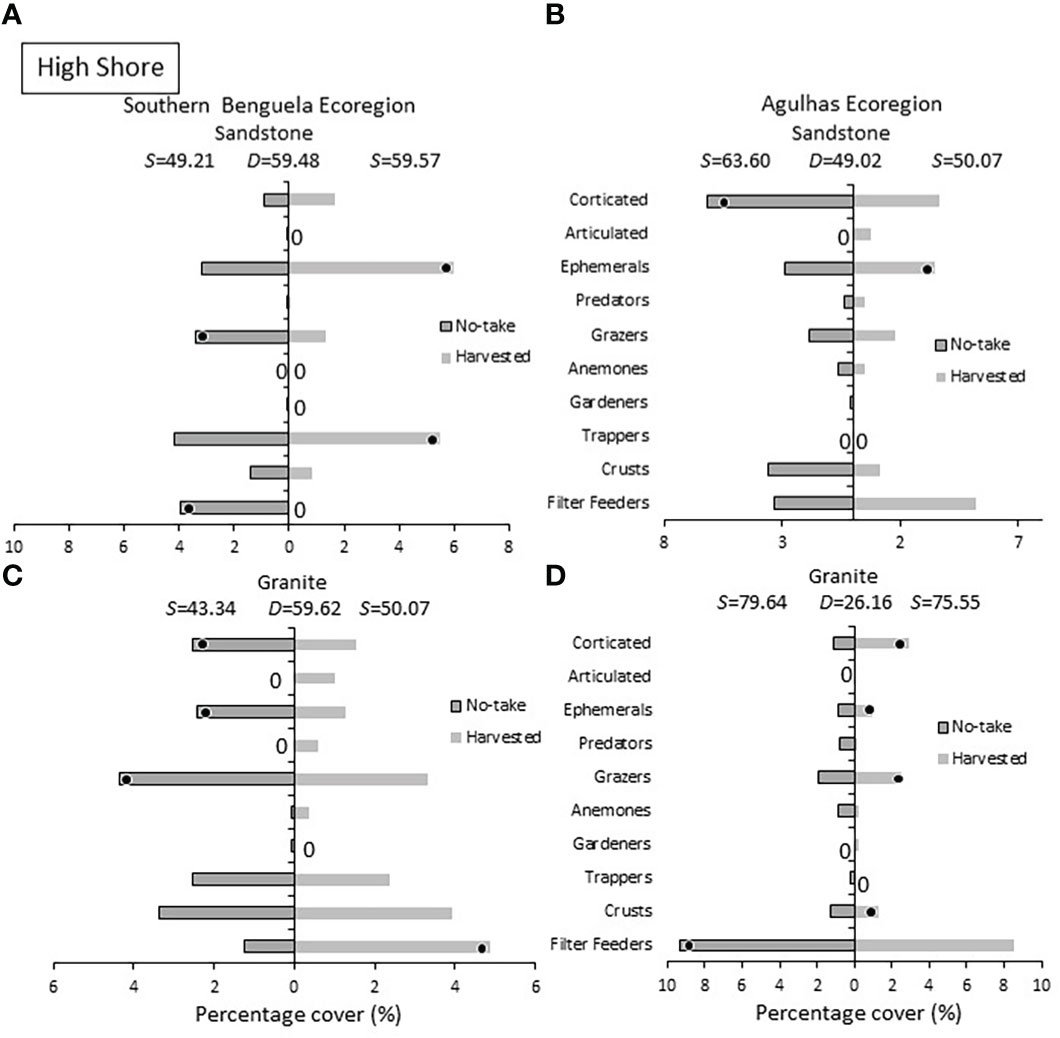
Figure 8 High shore: Percentage cover of groups responsible for similarities (S) within harvested or no-take areas and dissimilarities (D) between them, on (A) sandstone in the Southern Benguela and (B) Agulhas ecoregions, and on granite in (C) the southern Benguela and (D) Agulhas. See caption for Figure 6 for further interpretation.
On the top shore (Figure 9) ephemeral algae were more abundant in no-take areas, with one exception. Corticated algae remained more common in harvested sites in three out of four cases. Grazers– dominated by the rarely harvested limpet S. granularis - attained greater densities on harvested shore in all cases bar Southern Benguela granite. In three of four instances, filter feeders distinguished and achieved greater abundance in no-take areas.
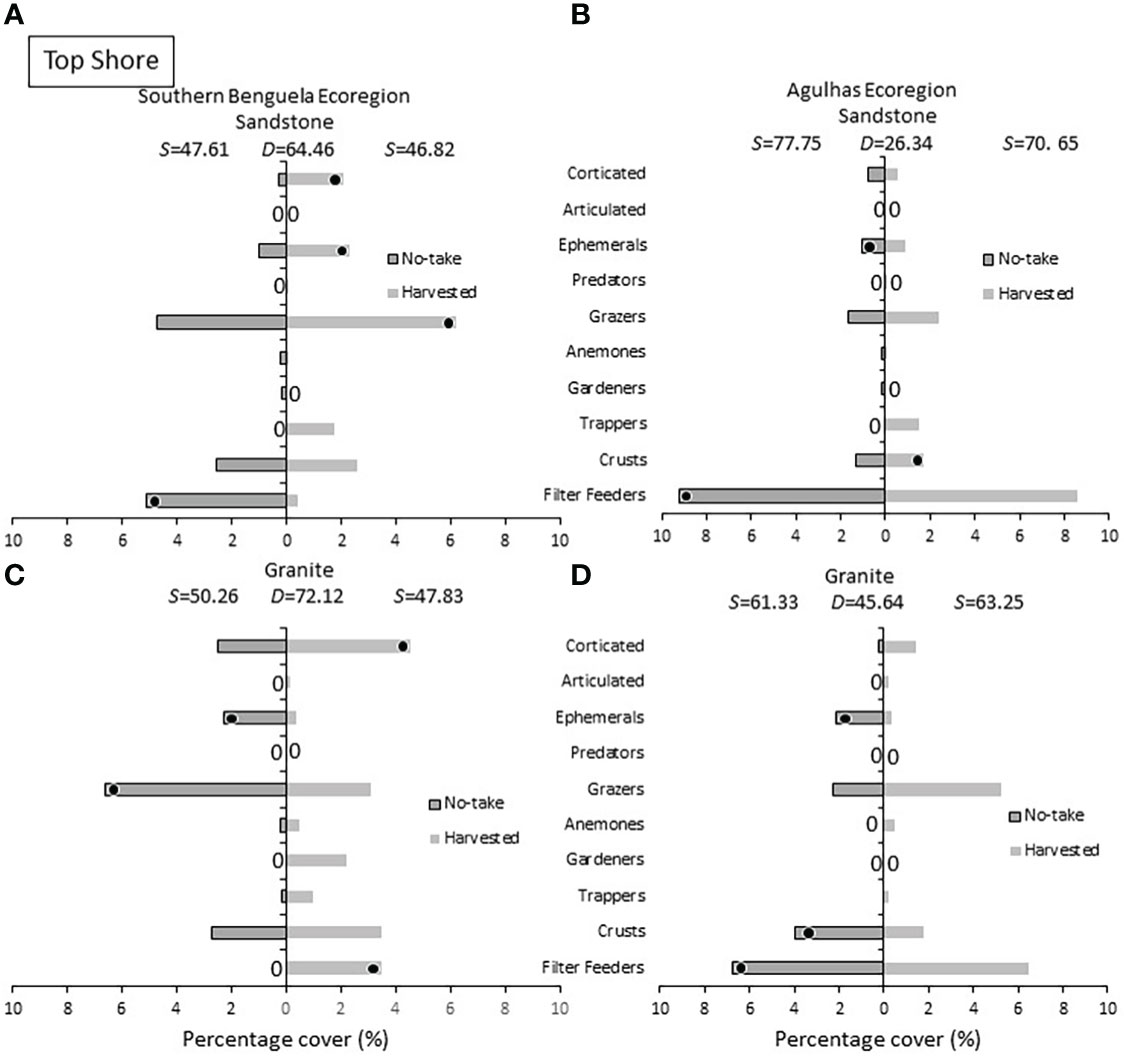
Figure 9 Top shore: Percentage cover of groups responsible for similarities (S) within harvested or no-take areas and dissimilarities (D) between them, on (A) sandstone in the Southern Benguela and (B) Agulhas ecoregions, and on granite in (C) the southern Benguela and (D) Agulhas. See caption for Figure 6 for further interpretation.
3.6. Functional groups distinguishing between ecoregions
SIMPER identified groups that distinguished ecoregions (Figure 10). Most differences were zone-specific, but ephemeral algae consistently distinguished the Southern Benguela, as did crustose algae in all zones bar the low shore, where they distinguished the Agulhas ecoregion, associated with a greater abundance of gardening limpets in that zone and region. Grazers were abundant in only the high and top zones, where they distinguished the Southern Benguela. Articulated coralline algae were abundant only in the low shore, where they distinguished the Agulhas. Corticated algae distinguished the Agulhas in the mid and high shore where their abundance was greatest but distinguished the Southern Benguela in the low and top zones. Filter feeders distinguished the Agulhas in the high and top zones where they were most abundant, but the Southern Benguela in the low and mid zones.
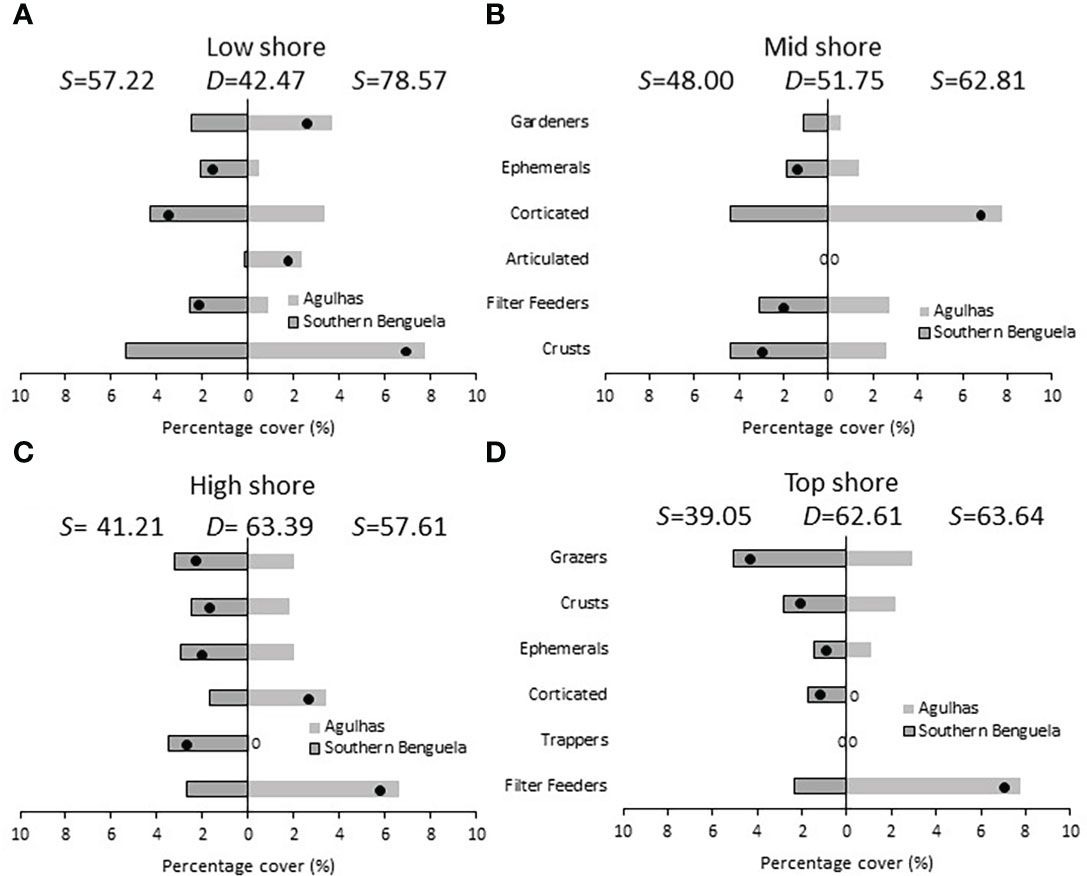
Figure 10 Percentage cover of groups responsible for the similarities (S) within ecoregions and the dissimilarities (D) between ecoregions at the four intertidal zones: Low shore, mid shore, high shore and top shore (A–D). Black dots identify groups distinguishing between ecoregions, and are placed in the ecoregion with the greatest abundance; 0 = absence.
4. Discussion
Exploitation is a major factor driving communities on intertidal rocky shores, but its impacts vary among rock types and ecoregions (Hockey et al., 1988; Lasiak and Field, 1995; Lasiak, 1999; Airoldi et al., 2005; Jimenez et al., 2011; Jimenez et al., 2015; Jimenez et al., 2016). The key findings of our study supported our hypotheses that (1) shellfish gatherers selectively harvest large individuals of limpets, (2) reducing the densities and usually the mean sizes of frequently harvested species, but (3) not those of rarely harvested species. (4) Protection influenced the community composition of rocky shores; but the magnitude of its effects was specific to functional groups and depended on ecoregion, rock type and zone. (5) Functional groups that are not harvested did proliferate in harvested areas where species normally controlling them were depleted. (6) Protection effects did differ between ecoregions and rock types. Overall, these outcomes confirmed that (a) protection inside no-take areas in the Table Mountain National Park MPA has significant beneficial effects on commonly harvested invertebrates, but less influence (or none at all) on rarely harvested ones and (b) community composition did respond to protection.
4.1. Selection of species and sizes by harvesters
Previous studies have demonstrated that shellfish gatherers have preferences for particular species (Durán et al., 1987; Airoldi et al., 2005; Nakin and McQuaid, 2014). This leads to a small number of species dominating the catches (Hockey and Bosman, 1986; Lasiak, 1991b; Lasiak, 1993b; García-Escárzaga et al., 2017). For example, Durán et al. (1987) showed that in Chile shellfish gatherers concentrate their harvest on Concholepas concholepas and Fissurella crassa. Our study revealed that, unlike the east coast of South Africa where the mussel Perna perna dominates catches by shellfish gatherers (Hockey and Bosman, 1986; Hockey et al., 1988; Lasiak, 1991a; Lasiak, 1992), the limpet Cymbula granatina was the most exploited intertidal shellfish in Table Mountain National Park. This could be attributed to its large size, high densities, accessibility on relatively sheltered shores (Eekhout et al., 1992; Bustamante et al., 1995; Blamey and Branch, 2009), and comparatively low strength of attachment (Branch and Marsh, 1978). All these characteristics lead to easy harvesting of this species on rocky shores, making it vulnerable to overfishing (Lasiak, 1991b). Indeed, there is a long history of exploitation of species such as this in southern Africa, extending back at least 100 000 years in the archaeological record (Jerardino et al., 2008; Parkington et al., 2013; Will et al., 2016; Jerardino, 2021). Cymbula oculus was also harvested at three Southern Benguela sites, but in smaller amounts, reflecting the fact that its densities there are lower than those of C. granatina, reflecting its prevalence in the warmer waters of the Agulhas Ecoregion (Branch, 1971). By contrast, Scutellastra argenvillei, which inhabits semi-exposed to exposed areas (Branch and Steffani, 2004), was recorded as being harvested at only one site in any numbers. The absence of S. longicosta, S. cochlear and S. granularis from any catch records supports the view that they are rarely harvested in the region we investigated, although S. longicosta is harvested on the more easterly shores of South Africa (Hockey et al., 1988; Nakin et al., 2012; Nakin and McQuaid, 2014; Nakin and McQuaid, 2016).
The selection of larger individuals of C. granatina by shellfish gatherers upholds our first hypothesis to this effect and supports other findings that shellfish gatherers target large individuals (Durán et al., 1987; Lasiak, 1991a; Lasiak, 1992; Jimenez et al., 2011; Alexander and Gladstone, 2012). This usually leads to reductions in the mean sizes of the individuals in the natural populations (Eekhout et al., 1992; Jimenez et al., 2011), with profound effects on reproductive output (Branch, 1975; Branch and Odendaal, 2003).
4.2. Densities of key species
Exclusion of shellfish gatherers from MPAs often leads to greater densities of commonly harvested species inside no-take areas relative to harvested areas (Moreno et al., 1984; Hockey and Bosman, 1986; Moreno et al., 1986; Hockey et al., 1988; Edgar and Barrett, 1999; Alexander and Gladstone, 2012; Bednar and Trulio, 2017). For example, higher densities of the limpet Cellana tramoserica were found in the Bouddi National Park Marine Extension relative to unprotected shores (Alexander and Gladstone, 2012). The same was true for Patella ulyssiponensis in La Palma MPA (López et al., 2012), for Cymbula oculus in Dwesa Nature Reserve in South Africa (Branch and Odendaal, 2003), and for Fissurella picta and Fissurella limbata in the Marine Reserve of Mehuin in Chile (Duarte et al., 1996). These increased densities were all attributed to protection. Our results produced similar patterns for the commonly harvested limpets C. granatina, C. oculus and S. argenvillei, particularly on sandstone where these species are most abundant.
The conclusion that protection is responsible for increased densities of harvested species is strengthened by the fact that the rarely harvested limpets S. granularis, S. longicosta and S. cochlear had equivalent densities at both protection levels in most instances (six out of ten comparisons). This difference is what would be expected if it is harvesting, rather than any other factors, that accounts for the decline in harvested species in sites that are not protected. Of the species that are not harvested, in the case of S. longicosta, no differences in density existed inside and outside no-take areas. For the other two species that are not harvested, there were, however, departures from this pattern, but they still amounted to an absence of any protection effect, or at least inconsistencies in its effects. For S. cochlear, densities inside and outside no-take MPAs were the same in two cases, greater in no-take areas in one case, and greater in harvested areas in one case. Scutellastra granularis attained high densities inside no-take areas in only one instance. It is possible that that its abundance is influenced more by the arrival of the alien mussel M. galloprovincialis (Griffiths et al., 1992; Branch et al., 2008; Branch et al., 2010) than any other factor, as this mussel greatly boosts this limpet’s recruitment (Van Erkom Schurink and Griffiths, 1990). In the Agulhas Ecoregion, S. granularis density is also often restricted by barnacles such as Tetraclita serrata and Chthamalus dentatus (Branch, 1976; Nakin and McQuaid, 2014), which are substantially less abundant in the Southern Benguela than the Agulhas (Boland, 1997). There were significant and complex interactions among ecoregion, rock types and protection level for S. granularis and S. cochlear as a result of these variable outcomes, which was not unexpected in the absence of any likely direct effect of protection. The absence or inconsistent outcomes of any protection effect observed for rarely harvested species also concurs with previous studies. For example, another rarely harvested species, Cellana capensis, had higher densities in harvested than no-take areas on the southeast coast of South Africa (Lasiak, 1993a).
Protection of even harvested species by way of no-take areas is, however, not always effective. An absence of any protection effect was reported by Nakin and McQuaid (2014) for limpets in Dwesa Nature Reserve, attributable to illegal harvesting in the reserve and differences in the intensity of harvesting outside the reserve. Similarly, Coppa et al. (2012; 2015) associated the decline of Patella ferruginea in Italy’s Mal di Ventre MPA with ineffective enforcement of regulations. Although C. oculus had high densities inside sandstone no-take areas, its overall abundance appears to have dramatically decreased in the TMNP MPA over the past 11 years, from 12.35 m-2 to 8 m-2 (Maneveldt et al., 2009). The lack of protection effect on the granite rocky shore might be due to higher mortality associated with dislodgement of limpets from granite rocks, as it has been suggested that they cannot attach as firmly to this rock type (McQuaid et al., 1985). Thus, low densities of C. oculus on granite rocky shores may not reflect a failure of protection inside no-take areas of the MPA, but rather an influence of habitat type on the density of the species.
We had anticipated that there would be differences in the abundances of limpets between the two ecoregions spanned by our study. This manifested itself in two ways. First, some species were either confined to, or substantially more abundant, in one of the two ecoregions. That was the case for C. granatina and S. argenvillei (predominantly Southern Benguela), and C. oculus and S. longicosta (predominantly Agulhas). For those species that occurred in both ecoregions, we had expected that there might be greater abundances in the Southern Benguela than the Agulhas Ecoregion because of the greater productivity on the west coast and the fact that greater biomasses of grazers have been recorded there (Bustamante et al., 1995). That was, however, not the case. There were no obvious differences in abundances of either S. granularis or S. cochlear between ecoregions.
The densities of S. longicosta that we recorded were magnitudes less than those recorded in Dwesa Nature Reserve (Nakin and McQuaid, 2014). This might reflect that the species is reaching the western limit of its distribution range in the TMNP, resulting in low densities (Hidas et al., 2010).
4.3. Sizes of key species
Shellfish gatherers generally target large individuals, as noted above for Cymbula granatina and Scutellastra argenvillei. This can truncate the size distribution of populations in harvested areas (Sagarin et al., 2007; Jimenez et al., 2011; Alexander and Gladstone, 2012; Coppa et al., 2012; Fenberg et al., 2012; Coppa et al., 2015; Bednar and Trulio, 2017), as has been reported for Cymbula oculus (Branch and Odendaal, 2003), Cellana capensis (Lasiak, 1993a), Lottia gigantea (Sagarin et al., 2007; Lucas and Smith, 2016), and many other species, including fish (Marra et al., 2017; Heyns-Veale et al., 2019).
In our results, harvesting had variable effects on the sizes of key species. The most frequently harvested species, C. granatina, consistently had larger sizes inside protected vs. harvested areas, on both sandstone and granite rocks. The second frequently harvested species, Scutellastra argenvillei, yielded the same outcome on sandstone, but not on granite, possibly because densities there were much lower, and no harvesting of this species was recorded at sites with granite rocks. However, C. oculus, which is also harvested, displayed a weak effect of protection on size, being larger outside no-take areas than inside on sandstone, and not significantly different on granite. Effects of protection may thus be species-specific, habitat-specific and region-specific (Nakin and McQuaid, 2014). The existence of larger individuals in protected areas enhances reproductive output because of their greater fecundity (Branch, 1975; Branch and Odendaal, 2003; Orozco et al., 2013; Zarrouk et al., 2016). For species like C. oculus that are protandric hermaphrodites and change sex from male to female at an intermediate size, a reduction of mean size will also affect population reproductive output as it will skew sex ratios in favor of males (Branch and Odendaal, 2003).
In contrast to the three harvested species of limpets, all three rarely harvested species, S. longicosta, S. granularis and S. cochlear, conformed to expectation and showed either no effects or no consistent effects of protection on their mean sizes. The size of S. longicosta was not related to the level of harvesting, and the same was true in three of the four comparisons possible for S. cochlear. For S. granularis, size was larger at harvested sites – significantly so in three of the four cases.
Similar findings were reported for S. granularis at Dwesa Nature Reserve, with larger sizes outside than inside the reserve (Nakin and McQuaid, 2014). The occurrence of larger individuals in harvested areas may reflect reduced competition due to the removal of harvested competitors, although that has not yet been demonstrated empirically. Lasiak and White (1993) have, however, shown that high densities of the limpet Cellana capensis reduce microalgal availability, with implied competitive effects for other species.
The sizes and densities of key limpet species were forecast to be greater in the Southern Benguela than the Agulhas Ecoregion because of greater productivity there as a result of upwelling (Bustamante et al., 1995). This was, however, not the case.
4.4. Community composition
There were considerable differences in community composition between the harvested and no-take areas, with relatively greater abundances of filter feeders, grazers and trappers inside no-take areas. Reductions of these groups in harvested areas have frequently been reported (Moreno et al., 1984; Hockey and Bosman, 1986; Lasiak and Field, 1995; Sharpe and Keough, 1998; Lasiak, 1998; Lasiak, 1999; Ceccherelli et al., 2005; Sink et al., 2010; Ceccherelli et al., 2011; Barbiero et al., 2011). Their depletion at harvested sites was likely responsible for the increased abundances of algae due to diminished grazing and/or competition for space. Similar findings have been reported for Dwesa-Cwebe, Hluleka and Mkambati Nature Reserves, and in Tsitsikamma MPA, on the east and south coasts of South Africa respectively, where community composition inside these protected areas is dominated by commonly harvested species such as Perna perna, Haliotis spadicea, Scutellastra barbara, Cymbula oculus and Cymbula miniata (Hockey and Bosman, 1986; Lasiak and Field, 1995; Dye, 1998; Lasiak, 1998; Lasiak, 1999; Hanekom, 2011). Comparable outcomes have been noted worldwide: the commonly harvested urchin Heliocidaris erythrogramma is a characteristic and distinguishing taxon for no-take areas in Tasmanian marine reserves (Barrett et al., 2009). Three other harvested taxa, Tridacna spp., Trochus niloticus and Turbo spp., are the major groups distinguishing between protected and no-take areas in New Caledonia (Jimenez et al., 2015; Jimenez et al., 2016). The fact that harvested filter feeders, grazers and trappers were the major functional groups distinguishing between harvested and no-take areas in our data strengthens our conclusion that protection influenced the community composition, as reflected in other studies (Lasiak and Field, 1995; Lasiak, 1998; Lasiak, 1999). Protection in no-take areas often results in a mosaic of communities, with patches of filter feeders, grazers and trappers, while adjacent harvested areas tend to have extensive uniform mats of algae, as described for Dwesa-Cwebe and Hluleka Nature Reserves and Tsitsikamma MPA (Hockey and Bosman, 1986; Lasiak and Field, 1995; Dye, 1998; Lasiak, 1998; Hanekom, 2011).
Whilst not the primary focus of this study, community composition also differed in a consistent and significant manner between ecoregions, which led to frequent interaction effects between protection and ecoregion. Our results agree with the biogeographic findings of Bustamante et al. (1995) and Bustamante and Branch (1996) that community composition is markedly different between the Agulhas and Southern Benguela ecoregions, which is the most clear-cut biogeographic break along the coast of southern Africa (Emanuel et al., 1992). This is associated with greater nutrient availability and lower temperatures in the Southern Benguela (McQuaid et al., 1985; Emanuel et al., 1992; Bustamante et al., 1995). In our results, the Southern Benguela displayed higher abundances of ephemeral algae, grazers and trappers, probably as a result of upwelling boosting nutrient availability and primary productivity (Bustamante et al., 1995; Bustamante and Branch, 1996).
The presence of larger quantities of algal crusts inside some of the no-take areas probably reflects trophic cascades and competitive interactions among functional groups, since this group has been shown to positively related to the abundance of grazers, which deplete macroalgae (Blamey and Branch, 2009) that would otherwise overgrow crusts. Harvested areas frequently had the highest percentage cover of corticated and ephemeral algae, which signals an altered community composition associated with lower densities of grazers (Bustamante et al., 1997; Lasiak, 1998; Barbiero et al., 2011).
The average levels of similarity (70.9%) in the Agulhas Ecoregion were equivalent to those considered to be converged communities (Sink et al., 2010; Jimenez et al., 2012). In addition, both protection levels were characterized by similar functional groups and dominated by algae, which are usually typical of areas experiencing high harvesting pressure (Hockey and Bosman, 1986; Lasiak and Field, 1995; Lasiak, 1998; Barrett et al., 2009; Sink et al., 2010). Thus, the dominance of some groups of algae in no-take areas in the Agulhas Ecoregion contradicts early findings in other MPAs along the south and east coasts of South Africa, such as Dwesa, Hluleka and Mkambati Nature Reserves (Hockey and Bosman, 1986; Lasiak and Field, 1995; Lasiak, 1998) and Tsitsikamma MPA (Hanekom, 2011), where commonly harvested consumers dominate and characterize no-take areas, with algae being less abundant (Lasiak and Field, 1995; Lasiak, 1998; Hanekom, 2011). This may, however, reflect the influence of upwelling in the Table Mountain National Park MPA, which supplies nutrients and thus facilitates algal growth and productivity, while the contrary findings were from more oligotrophic regions of the country, where grazing may have a more severe effect on algal abundances.
In the Southern Benguela Ecoregion, differences between harvested and no-take areas were more obvious (D = 39.85–72.12%), reflecting greater divergence (Sink et al., 2010; Jimenez et al., 2012) than in the Agulhas Ecoregion. There, communities reflected conventional expectations to a much greater degree, especially on sandstone shores, where corticated and ephemeral algae were prevalent in harvested situations, associated with the depletion of herbivores.
In terms of individual species, one striking absentee from the list of characteristic species of the Agulhas Ecoregion was the indigenous brown mussel Perna perna, which was abundant in False Bay at the southeast limits of the Agulhas Ecoregion in the 1980s but had diminished by 2011 (Griffiths and Mead, 2011; Reimers et al., 2014), and was never recorded in our study. Changes in its abundance and presence in this region are likely associated with cooling of the waters and a southeasterly contraction of this species’ range (Pfaff et al., 2019). Replacing it, the alien Mediterranean mussel Mytilus galloprovincialis has become a dominant element of all the shores studied.
5. Conclusions
Our study was both enriched and complicated by the fact that our comparisons of the effects of protection spanned two ecoregions and two rock types. Had we confined analyses to the Southern Benguela, where harvesting is most intense, and to sandstone, where the limpets we examined are most abundant, the effects of protection would have been unambiguous: protection increases the abundance of grazers and mussels, but the abundance of algae is decreased due to greater grazing and competition for space. However, expanding the study to cross ecoregional boundaries and rock types yielded a much richer understanding . Ecoregions differed in ways we had not expected, and rock type introduced interactions that muted the effects of protection on granite where many of the harvested species were less abundant.
In summary, shellfish gatherers collected mainly the limpets C. granatina, C. oculus and S. argenvillei and mussels, and targeted large individuals, supporting our first hypothesis. Our second hypothesis, that protection would result in greater densities and sizes of harvested limpets, was supported on sandstone shores, but not on granite rocky shores where their densities were uniformly low. Our third hypothesis, that rarely harvested species would show no differences in densities and sizes attributable to protection gained partial support: S. longicosta conformed to the hypothesis, whereas S. granularis provided only partial support, being larger in harvested areas in three out of four cases, and only once having greater densities in no-take zones; S. cochlear yielded sizes that were larger in harvested than no-take areas in one instance, and its densities were ambivalent with respect to protection level.
Differences in protection did affect rocky shore community composition, supporting our fourth hypothesis. The depletion of limpets and filter feeders was associated with dramatic increases in algal domination in the harvested areas of Southern Benguela sandstone shores, upholding our fifth hypothesis. Effects of harvesting on community composition were thus specific to region, zone and rock type, supporting our sixth hypothesis.
Likely causes of these differences include (a) differences in the intensity of harvesting in different areas; (b) targeting of different species; (c) effectiveness of enforcement of protection, (d) differences in abundance between rock types, and (e) differences in the abundances of alien species, particularly Mytilus galloprovincialis. Influences of protection were most clear for sandstone rocks in the Southern Benguela, where harvesting was concentrated and target species most abundant.
Due to their accessibility or vicinity to coastal communities, some sites are harvested more intensely than others. In addition, the effectiveness of law enforcement varies among the sites. The management of TMNP MPA would benefit from data of actual harvesting levels and measures of compliance, as well as experimental tests of the impacts of different harvesting intensities on rocky shore biodiversity. We would urge all three measures to be pursued.
Overall, this study supports the effectiveness of no-take areas in the TMNP MPA in maintaining densities and average sizes of harvested limpets. With the removal of these keystone grazers, communities will transform towards algal-dominated systems, which demonstrates the ecosystem effects of the MPA’s conservation measures.
Data availability statement
The raw data supporting the conclusions of this article will be made available by the authors, without undue reservation.
Author contributions
All authors contributed remarkable in the production of this manuscript from conceptualization, data collection, data analysis and writing.
Conflict of interest
The authors declare that the research was conducted in the absence of any commercial or financial relationships that could be construed as a potential conflict of interest.
Publisher’s note
All claims expressed in this article are solely those of the authors and do not necessarily represent those of their affiliated organizations, or those of the publisher, the editors and the reviewers. Any product that may be evaluated in this article, or claim that may be made by its manufacturer, is not guaranteed or endorsed by the publisher.
Supplementary material
The Supplementary Material for this article can be found online at: https://www.frontiersin.org/articles/10.3389/fmars.2022.893260/full#supplementary-material
References
Abecasis R. C., Afonso P., Colaço A., Longnecker N., Clifton J., Schmidt L., et al. (2015). Marine conservation in the Azores: Evaluating marine protected area development in a remote island context. Front. Mar. Sci. 2. doi: 10.3389/fmars.2015.00104
Airoldi L., Bacchiocchi F., Cagliola C., Bulleri F., Abbiati M. (2005). Impact of recreational harvesting on assemblages in artificial rocky habitats. Mar. Ecol. Prog. Ser. 299, 55–66. doi: 10.3354/meps299055
Alexander T. J., Gladstone W. (2012). Assessing the effectiveness of a long-standing rocky intertidal protected area and its contribution to the regional conservation of species, habitats and assemblages. Aquat. Conserv.: Mar. Freshw. Ecosyst. 23, 111–123. doi: 10.1002/aqc.2284
Attwood C. G., Bennett B. A. (1994). Variation in dispersal of galjoen (Coracinus capensis) (Teleostei: Coracinidae) from a marine reserve. Can. J. Fish. Aquat. Sci. 51, 1247–1257. doi: 10.1139/f94-124
Attwood C. G., Bennett B. A. (1995). Modelling the effect of marine reserves on the recreational shore-fishery of the south-Western cape, south Africa. South Afr. J. Mar. Sci. 16, 227–240. doi: 10.2989/025776195784156458
Barbiero D. C., Macedo I. M., Masi B. P., Zalmon I. R. (2011). A removal disturbance effect on the ecological succession of a benthic intertidal community-south-eastern Brazil. Mar. Biodivers. Records 4, 1–8. doi: 10.1017/S1755267210000813
Barrett N. S., Buxton C. D., Edgar G. J. (2009). Changes in invertebrate and macroalgal populations in Tasmanian marine reserves in the decade following protection. J. Exp. Mar. Biol. Ecol. 370, 104–119. doi: 10.1016/j.jembe.2008.12.005
Bednar C., Trulio L. (2017). Reducing human impacts on central Californian intertidal gastropods: are marine protected areas effective? Molluscan Res. 37, 148–152. doi: 10.1080/13235818.2017.1278957
Benedetti-Cecchi L., Bertocci I., Micheli F., Maggi E., Fosella T., Vaselli S. (2003). Implications of spatial heterogeneity for management of marine protected areas (MPAs): Examples from assemblages of rocky coasts in the northwest Mediterranean. Mar. Environ. Res. 55, 429–458. doi: 10.1016/S0141-1136(02)00310-0
Bennett B. A., Attwood C. G. (1991). Evidence for recovery of a surf-zone fish assemblage following the establishment of a marine reserve on the southern coast of south Africa. Mar. Ecol. Prog. Ser. 75, 173–181. doi: 10.3354/meps075173
Bennett B. A., Attwood C. G. (1993). Shore-angling catches in the de hoop nature reserve, south Africa, and further evidence for the protective value of marine reserves’. South Afr. J. Mar. Sci. 13, 213–222. doi: 10.2989/025776193784287202
Bennett N. J., Dearden P. (2014). Why local people do not support conservation: Community perceptions of marine protected area livelihood impacts, governance and management in Thailand. Mar. Policy 44, 107–116. doi: 10.1016/j.marpol.2013.08.017
Bertocci I., Dominguez R., Freitas C., Sousa-Pinto I. (2012). Patterns of variation of intertidal species of commercial interest in the parque litoral norte (north Portugal) MPA: Comparison with three reference shores. Mar. Environ. Res. 77, 60–70. doi: 10.1016/j.marenvres.2012.02.003
Bertocci I., Dominguez R., Machado I., Freitas C., Domínguez Godino J., Sousa-Pinto I., et al. (2014). Multiple effects of harvesting on populations of the purple sea urchin paracentrotus lividus in north Portugal. Fish. Res. 150, 60–65. doi: 10.1016/j.fishres.2013.10.010
Blamey L. K., Branch G. M. (2009). Habitat diversity relative to wave action on rocky shores: Implications for the selection of marine protected areas. Aquat. Conserv.: Mar. Freshw. Ecosyst. 19, 645–657. doi: 10.1002/aqc
Boland J. M. (1997). Is the geographic pattern in the abundance of south African barnacles due to pre-recruitment or post-recruitment factors? South Afr. J. Mar. Sci. 18, 63–73. doi: 10.2989/025776197784161036
Branch G. M. (1971). The ecology of Patella Linnaeus from the cape peninsula, south africa. i. zonation, movements and feeding. Zoologica Africana 6, 1–38. doi: 10.1080/00445096.1971.11447402
Branch G. M. (1975). Notes on the ecology of Patella concolor and Cellana capensis, and the effects of human consumption of limpet populations. Zoologica Africana 10, 75–85. doi: 10.1080/00445096.1975.11447494
Branch G. M. (1976). Interspecific competition experienced by south African Patella species. J. Anim. Ecol. 45, 507–529. doi: 10.2307/3888
Branch G. M., Marsh A. C. (1978). Tenacity and shell shape in six Patella species: Adaptive features. J. Exp. Mar. Biol. Ecol. 34, 111–130. doi: 10.1016/0022-0981(78)90035-7
Branch G. M., Odendaal F. (2003). The effects of marine protected areas on the population dynamics of a south African limpet, Cymbula oculus, relative to the influence of wave action. Biol. Conserv. 114, 255–269. doi: 10.1016/S0006-3207(03)00045-4
Branch G. M., Odendaal F., Robinson T. B. (2008). Long-term monitoring of the arrival, expansion and effects of the alien mussel Mytilus galloprovincialis relative to wave action. Mar. Ecol. Prog. Ser. 370, 171–183. doi: 10.3354/meps07626
Branch G. M., Odendaal F., Robinson T. B. (2010). Competition and facilitation between the alien mussel Mytilus galloprovincialis and indigenous species: Moderation by wave action. J. Exp. Mar. Biol. Ecol. 383, 65–78. doi: 10.1016/j.jembe.2009.10.007
Branch G. M., Steffani C. N. (2004). Can we predict the effects of alien species? a case-history of the invasion of south Africa by Mytilus galloprovincialis (Lamarck). J. Exp. Mar. Biol. Ecol. 300, 189–215. doi: 10.1016/j.jembe.2003.12.007
Brouwer S. L., Griffiths M. H., Roberts M. J. (2003). Adult movement and larval dispersal of Argyrozona argyrozona (Pisces: Sparidae) from a temperate marine protected area. Afr. J. Mar. Sci. 25, 395–402. doi: 10.2989/18142320309504028
Bustamante R. H., Branch G. M. (1996). Large Scale patterns and trophic structure of southern African rocky shores: The role of geographic variation and wave exposure. J. Biogeogr. 23, 339–351. doi: 10.1046/j.1365-2699.1996.00026.x
Bustamante R. H., Branch G. M., Eekhout S. (1997). The influences of physical factors on the distribution and zonation patterns of south African rocky-shore communities. South Afr. J. Mar. Sci. 18, 119–136. doi: 10.2989/025776197784160901
Bustamante R. H., Branch G. M., Eekhout S., Robertson B., Schleyer M., Dye A., et al. (1995). Gradients of intertidal primary productivity around the coast of south Africa and their relationships with consumer biomass. Oecologia 102, 189–201. doi: 10.1007/BF00333251
Buxton C. D. (1993). Life-history changes in exploited reef fishes on the east coast of south Africa. Environ. Biol. Fishes 36, 47–63. doi: 10.1007/BF00005979
Buxton C. D., Smale M. J. (1989). Abundance and distribution patterns of three temperate marine reef fish (Teleostei: Sparidae) in exploited and unexploited areas off the southern cape coast. J. Appl. Ecol. 26, 441–451. doi: 10.2307/2404072
Carballo J. L., Yáñez B., Bautista-Guerrero E., García-Gómez J. C., Espinosa F., Tortolero-Langarica J. J. A., et al. (2019). Decimation of a population of the endangered species Scutellastra mexicana (Broderip and sowerb) (Mollusca, Gastropoda) in the marías island (Eastern ocean pacific) biosphere reserve. Aquat. Conserv.: Mar. Freshw. Ecosyst. 30, 1–11. doi: 10.1002/aqc.3239
Castilla J. C., Bustamante R. H. (1989). Human exclusion from rocky intertidal of las cruces, central Chile: effects on Durvillaea antarctica (Phaeophyta, durvilleales). Mar. Ecol. Prog. Ser. 50, pp. doi: 10.3354/meps008203
Ceccherelli G., Casu D., Sechi N. (2005). Spatial variation of intertidal assemblages at tavolara-capo coda cavallo MPA (NE sardinia): Geographical vs. protection effect. Mar. Environ. Res. 59, 533–546. doi: 10.1016/j.marenvres.2004.09.002
Ceccherelli G., Pinna S., Navone A., Sechi N. (2011). Influence of geographical siting and protection on rocky-shore gastropod distribution at a Western Mediterranean marine protected area. J. Coast. Res. 27, 882–889. doi: 10.2112/JCOASTRES-D-10-00027.1
Chadwick P., Duncan J., Tunley K. (2014). “State of management of south africa’s marine protected areas,” in WWF south Africa report series - 2014/Marine/001. (South Africa: WWF)
Coppa S., De Lucia G. A., Massaro G., Camedda A., Marra S., Magni P., et al. (2015). Is the establishment of MPAs enough to preserve endangered intertidal species? the case of Patella ferruginea in mal di ventre island (W Sardinia, Italy). Aquat. Conserv.: Mar. Freshw. Ecosyst. 26, 623–638. doi: 10.1002/aqc.2579
Coppa S., De Lucia G. A., Massaro G., Magni P. (2012). Density and distribution of Patella ferruginea in a marine protected area (western Sardinia, italy): Constraint analysis for population conservation. Mediterr. Mar. Sci. 13, 108–117. doi: 10.12681/mms.27
Cowley P. D., Brouwer S. L., Tilney R. L. (2002). The role of the tsitsikamma national park in the management of four shore-angling fish along the south-eastern cape coast of south Africa. South Afr. J. Mar. Sci. 24, 27–35. doi: 10.2989/025776102784528664
Crowder L., Norse E. (2008). Essential ecological insights for marine ecosystem-based management and marine spatial planning. Mar. Policy 32, 722–778. doi: 10.1016/j.marpol.2008.03.012
Currie J. C., Sink K. J., Le Noury P., Branch G. M. (2012). Comparing fish communities in sanctuaries, partly protected areas and open-access reefs in south-East Africa. Afr. J. Mar. Sci. 34, 269–281. doi: 10.2989/1814232X.2012.709963
Dimitriadis C., Sini M., Trygonis V., Gerovasileiou V., Sourbs L., Koutsabis D. (2018). Assessment of fish communities in a Mediterranean MPA: Can a seasonal no-take zone provide effective protection? Estuar. Coast. Shelf Science 207 pp, 223–231. doi: 10.1016/j.ecss.2018.04.012
Duarte W. E., Asencio G., Moreno C. A. (1996). Long-term changes in population density of Fissurella picta and Fissurella limbata (Gastropoda) in the marine reserve of mehuin, Chile. Rev. Chil. Hist. Natural 69, 45–56. doi: 10.1098/rspb.1975.0109
Durán L. R., Castilla J. C. (1989). Variation and persistence of the middle rocky intertidal community of central Chile, with and without human harvesting. Mar. Biol. 103, 555–562. doi: 10.1038/470444a
Durán L. R., Castilla J. C., Oliva D. (1987). Intensity of human predation on rocky shores at las cruces in central Chile. Environ. Conserv. 14, 143–149. doi: 10.1017/S0376892900011504
Dye A. H. (1998). Community-level analyses of long-term changes in rocky littoral fauna from south Africa. Mar. Ecol. Prog. Ser. 164, 47–57. doi: 10.3354/meps164047
Edgar G. J., Barrett N. S. (1999). Effects of the declaration of marine reserves on Tasmanian reef fishes, invertebrates and plants. J. Exp. Mar. Biol. Ecol. 242, 107–144. doi: 10.1016/S0022-0981(99)00098-2
Eekhout S., Raubenheimer C. M., Branch G. M., Bosman A. L., Bergh M. O. (1992). A holistic approach to the exploitation of intertidal stocks: Limpets as a case study. South Afr. J. Mar. Sci. 12, 1017–1029. doi: 10.2989/02577619209504759
Emanuel B. P., Bustamante R. H., Branch G. M., Eekhout S., Odendaal F. J. (1992). A zoogeographic and functional approach to the selection of marine reserves on the west coast of south Africa. South Afr. J. Mar. Sci. 12, 341–354. doi: 10.2989/02577619209504710
Espinosa F., Guerra-García J. M., Fa D., García-Gómez J. C. (2006). Aspects of reproduction and their implications for the conservation of the endangered limpet, Patella ferruginea. Invertebrate Reprod. Dev. 49, 85–92. doi: 10.1080/07924259.2006.9652197
Espinosa F., Rivera-Ingraham G. A. (2017). Biological conservation of giant limpets: The implications of large size. Adv. Mar. Biol. 76, 105–155. doi: 10.1016/bs.amb.2016.10.002
Espinosa F., Rivera-Ingraham G. A., Fa D., García-Gómez J. C. (2009). Effect of human pressure on population size structures of the endangered ferruginean limpet: Toward future management measures. J. Coast. Res. 254, 857–863. doi: 10.2112/08-1005.1
Espinosa F., Rivera-Ingraham G. A., Maestre M., González A. R., Bazairi H., García-Gómez J. C. (2014). Updated global distribution of the threatened marine limpet Patella ferruginea (Gastropoda: Patellidae): An example of biodiversity loss in the Mediterranean. Oryx 48, 266–275. doi: 10.1017/S0030605312000580
Fenberg P. B., Caselle J. E., Claudet J., Clemence M., Gaines S. D., García-Charton J. A., et al. (2012). The science of European marine reserves: Status, efficacy, and future needs. Mar. Policy 36, 1012–1021. doi: 10.1016/j.marpol.2012.02.021
Fenberg P. B., Roy K. (2008). Ecological and evolutionary consequences of size-selective harvesting: How much do we know? Mol. Ecol. 17, 209–220. doi: 10.1111/j.1365-294X.2007.03522.x
Fenberg P. B., Roy K. (2012). Anthropogenic harvesting pressure and changes in life history: Insights from a rocky intertidal limpet. Am. Nat. 180, 200–210. doi: 10.1086/666613
Findlay K. (2020). “Challenges facing marine protected areas in southern African countries in light of expanding ocean economies across the subregion,” in Marine protected areas, vol. pp . Eds. Humphreys J., Clark R. W. E. (Elsevier), 37–65. (Amsterdam: Elsevier)
Floros C., Schleyer M. H., Maggs J. Q. (2013). Fish as indicators of diving and fishing pressure on high-latitude coral reefs. Ocean Coast. Manage. 84, 130–139. doi: 10.1016/j.ocecoaman.2013.08.005
Floros C., Schleyer M. H., Maggs J. Q., Celliers L. (2012). Baseline assessment of high-latitude coral reef fish communities in southern Africa. Afr. J. Mar. Sci. 34, 55–69. doi: 10.2989/1814232X.2012.673284
Fox J., Weisberg S. (2019). An {R} companion to applied regression, third edition (Thousand Oaks CA: Sage). Available at: https://socialsciences.mcmaster.ca/jfox/Books/Companion.
Fraschetti S., Terlizzi A., Bussotti S., Guarnieri G., D’ambrosio P., Boero F. (2005). Conservation of Mediterranean seascapes: Analyses of existing protection schemes. Mar. Environ. Res. 59, 309–332. doi: 10.1016/j.marenvres.2004.05.007
García-Charton J. A., Pérez-Ruzafa A., Marcos C., Claudet J., Badalamenti F., Benedetti-Cecchi L., et al. (2008). Effectiveness of European atlanto-Mediterranean MPAs: Do they accomplish the expected effects on populations, communities and ecosystems? J. Nat. Conserv. 16, 193–221. doi: 10.1016/j.jnc.2008.09.007
García-Escárzaga A., Gutiérrez-Zugasti I., González-Morales M. R., Cobo-García A. (2017). Shells and humans: Molluscs and other coastal resources from the earliest human occupations at the mesolithic shell midden of El mazo (Asturias, northern Spain). Papers Institute Archaeol. 27, 1–17. doi: 10.5334/pia-481
Giakoumi S., Pey A. (2017). Assessing the effects of marine protected areas on biological invasions: A global review. Front. Mar. Sci. 4. doi: 10.3389/fmars.2017.00049
Godoy C., Moreno C. A. (1989). Indirect effects of human exclusion from the rocky intertidal in southern Chile: A case of cross-linkage between herbivores. Oikos 54, 101–106. doi: 10.2307/3565902
Götz A., Kerwath S. E., Attwood C. G., Sauer W. H. H. (2009a). Effects of fishing on a temperate reef community in south Africa 1: Ichthyofauna. Afr. J. Mar. Sci. 31, 241–251. doi: 10.2989/AJMS.2009.31.2.12.884
Götz A., Kerwath S. E., Attwood C. G., Sauer W. H. H. (2009b). Effects of fishing on a temperate reef community in south Africa 2: Benthic invertebrates and algae. Afr. J. Mar. Sci. 31, 253–262. doi: 10.2989/AJMS.2009.31.2.13.885
Griffiths C. L., Hockey P. A., Van Erkom Schurink C., Le Roux P. J. (1992). Marine invasive aliens on south African shores: Implications for community structure and trophic functioning. South Afr. J. Mar. Sci. 12, 713–722. doi: 10.2989/02577619209504736
Griffiths C. L., Mead A. (2011). “Human activities as drivers of change on south African rocky shores,” in Observations on environmental change in south Africa. Ed. Zietsman L. (Stellenbosch: Sun Press), 242–246.
Guidetti P. (2006). Marine reserves reestablish lost predatory interactions and cause community changes in rocky reefs. Ecol. Appl. 16, 963–976. doi: 10.1890/1051-0761(2006)016[0963:MRRLPI]2.0.CO;2
Hanekom N. (2011). Trophic structure and biomass distribution of macrobenthos on sheltered and semi-exposed rocky shores of tsitsikamma marine protected area. Afr. Zool. 46, 224–238. doi: 10.3377/004.046.0203
Henriques P., Delgado J., Sousa R. (2017). Patellid limpets: an overview of the biology and conservation of keystone species of the rocky shores. In: Ray S (Ed.) Organismal Mol. Malacol. InTech Croatia pp, 71–95. doi: 10.5772/67862
Heyns-Veale E. R., Bernard A. T. F., Götz A., Mann B. Q., Maggs J. Q., Smith M. K. S. (2019). Community-wide effects of protection reveals insights into marine protected area effectiveness for reef fish. Mar. Ecol. Prog. Ser. 620, 99–117. doi: 10.3354/meps12970
Hidas E. Z., Ayre D. J., Minchinton T. E. (2010). Patterns of demography for rocky-shore, intertidal invertebrates approaching their geographical range limits: Tests of the abundant-centre hypothesis in south-eastern Australia. Mar. Freshw. Res. 61, 1243–1251. doi: 10.1071/MF09317
Hockey P. A. R., Bosman A. L. (1986). Man as an intertidal predator in transkei: Disturbance, community convergence and management of a natural food resource. Oikos 46, 3–14. doi: 10.2307/3565373
Hockey P. A. R., Bosman A. L., Siegfried W. R. (1988). Patterns and correlates of shellfish exploitation by coastal people in transkei: An enigma of protein production. J. Appl. Ecol. 25, pp. doi: 10.2307/2403631
Jerardino A. (2021). Coastal foraging and transgressive sea levels during the terminal pleistocene: Insights from the central west coast of south African. J. Anthropological Archaeol. 64, 101351. doi: 10.1016/j.jaa.2021.101351
Jerardino A., Branch G. M., Navarro R. (2008). “Human impact on the pre-colonial west coast marine environments of south Africa,” in Human impacts on ancient marine ecosystems. a global perspective. Eds. Erlandson J. M., Rick T. C. (Berkeley: University of California Press), 279–296.
Jimenez H., Dumas P., Bigot L., Ferraris J. (2015). Harvesting effects on tropical invertebrate assemblages in new Caledonia. Fish. Res. 167, 75–81. doi: 10.1016/j.fishres.2015.02.001
Jimenez H., Dumas P., Leopold M., Ferraris J. (2011). Invertebrate harvesting on tropical urban areas: Trends and impact on natural populations (New Caledonia, south pacific). Fish. Res. 108, 195–204. doi: 10.1016/j.fishres.2010.12.021
Jimenez H., Dumas P., Mouillot D., Bigot L., Ferraris J. (2016). Harvesting effects on functional structure and composition of tropical invertebrate assemblages. ICES J. Mar. Sci. 73, 420–428. doi: 10.1093/icesjms/fsv179
Jimenez H., Dumas P., Ponton D., Ferraris J. (2012). Predicting invertebrate assemblage composition from harvesting pressure and environmental characteristics on tropical reef flats. Coral Reefs 31, 89–100. doi: 10.1007/s00338-011-0820-9
Kerwath S. E., Götz A., Attwood C. G., Sauer W. H. H. (2008). The effect of marine protected areas on an exploited population of sex-changing temperate reef fish: An individual-based model. Afr. J. Mar. Sci. 30, 337–350. doi: 10.2989/AJMS.2008.30.2.10.560
Kerwath S. E., Thorstad E. B., Næsje T. F., Cowley P. D., Økland F., Wilke C., et al. (2009). Crossing invisible boundaries: The effectiveness of the langebaan lagoon marine protected area as a harvest refuge for a migratory fish species in south Africa. Conserv. Biol. 23, 653–661. doi: 10.1111/j.1523-1739.2008.01135.x
Kerwath S. E., Winker H., Götz A., Attwood C. G. (2013). Marine protected area improves yield without disadvantaging fishers. Nat. Commun. 4, 2347. doi: 10.1038/ncomms3347
Kirkman S. P., Mann B. Q., Sink K. G., Adams R., Livingstone T.-C., Mann-Lang J. B., et al. (2021). Evaluation the evidence for ecological effectiveness of south africa's marine protected areas. Afr. J. Mar. Sci. 43, 389–412. doi: 10.2989/1814232X.2021.1962975
Lasiak T. (1991a). Is there evidence of over-exploitation of mussel stocks on the transkei coast? South Afr. J. Mar. Sci. 10, 299–302. doi: 10.2989/02577619109504640
Lasiak T. (1991b). The susceptibility and/or resilience of rocky littoral molluscs to stock depletion by the indigenous coastal people of transkei, south Africa. Biol. Conserv. 56, 245–264. doi: doi.org/10.1016/0006-3207(91)90060-M
Lasiak T. (1992). Contemporary shellfish-gathering practices of indigenous coastal people in transkei: Some implications for interpretation of the archaeological record. South Afr. J. Sci. 88, 19–28. doi: doi/pdf/10.10520/AJA00382353_9423
Lasiak T. (1993a). Temporal and spatial variations in exploited and non-exploited populations of the intertidal limpet Cellana capensis. J. Molluscan Stud. 59, 295–307. doi: doi.org/10.1093/mollus/59.3.295
Lasiak T. (1993b). The shellfish-gathering practices of indigenous coastal people in transkei: Patterns, preferences and perceptions. South Afr. J. Ethnol. 16, 115–120. doi: doi/pdf/10.10520/AJA02580144_669
Lasiak T. (1998). Multivariate comparisons of rocky infratidal macrofaunal assemblages from replicate exploited and non-exploited localities on the transkei coast of south Africa. Mar. Ecol. Prog. Ser. 167, 15–23. doi: 10.3354/meps167015
Lasiak T. (1999). The putative impact of exploitation on rocky infratidal macrofaunal assemblages: A multiple-area comparison. J. Mar. Biol. Assoc. United Kingdom 79, 23–34. doi: 10.1017/S0025315498000022
Lasiak T. (2006). Spatial variation in density and biomass of patellid limpets inside and outside a marine protected area. Journal of Molluscan Studies, 72(2), 137–142. doi: doi.org/10.1093/mollus/eyi058
Lasiak T. A., Field J. G. (1995). Community-level attributes of exploited and non-exploited rocky infratidal macrofaunal assemblages in transkei. J. Exp. Mar. Biol. Ecol. 185, 33–53. doi: 10.1016/0022-0981(94)00130-6
Lasiak T. A., White D. R. (1993). Microalgal food resources and competitive interactions among the intertidal limpets Cellana capensis (Gmelin 1791) and Siphonaria concinna sowerby 1824. South Afr. J. Mar. Sci. 13, 97–108. doi: 10.2989/025776193784287419
Lechanteur Y. A. R. G. (1999). The ecology and management of reef fishes in false bay, southwestern cape South Africa (South Africa: University of Cape Town). PhD Thesis.
Lechanteur Y. A. R. G., Griffiths C. L. (2002). Composition and seasonal variability of the suprabenthic reef-fish assemblage in false bay, south Africa. Afr. Zool. 37, 171–184. doi: 10.1080/15627020.2002.11657173
López C., Poladura A., Hernández J. C., Martín L., Concepción L., Sangil C., et al. (2012). Contrasting effects of protection from harvesting in populations of two limpet species in a recently established marine protected area. Scientia Marina 76, 799–807. doi: 10.3989/scimar.03601.15C
Lucas B. J., Smith J. R. (2016). Alterations in human visitation patterns and behaviors in southern California rocky intertidal ecosystems over two-decades following increased management efforts. Ocean Coast. Manage. 121, 128–140. doi: 10.1016/j.ocecoaman.2015.12.014
Maggs J., Mann B., Cowley P. (2013). Reef fish display station-keeping and ranging behaviour in the pondoland marine protected area on the east coast of south Africa. Afr. J. Mar. Sci. 35, 183–193. doi: 10.2989/1814232X.2013.798152
Maneveldt G. W., Eager R. C., Bassier A. (2009). Effects of long-term exclusion of the limpet Cymbula oculus (Born) on the distribution of intertidal organisms on a rocky shore. Afr. J. Mar. Sci. 31 (2), 171–179. doi: 10.2989/AJMS.2009.31.2.5.877
Mann B. Q., Cowley P. D., Kyle R. (2016). Estimating the optimum size for inshore no-take areas based on movement patterns of surf-zone fishes and recommendations for rezoning of a world heritage site in south Africa. Ocean Coast. Manage. 125, 8–19. doi: 10.1016/j.ocecoaman.2016.03.006
Mann-Lang J. B., Branch G. M., Mann B. Q., Sink K., Kirkman S., Adams R. (2021). A review of the social and economic effects of marine protected areas in south Africa with recommendations for future assessments. Afr. J. Mar. Sci. 43, 367–387. doi: 10.2989/1814232X.2021.1961166
Marra S., Coppa S., Camedda A., Massaro G., De Lucia G. A. (2017). The exploitation of limpets in a Mediterranean marine protected area: Assessing the effectiveness of protection in the intertidal zone. Mediterr. Mar. Sci. 18, 406–423. doi: 10.12681/mms.2087
Martins G. M., Thompson R. C., Neto A. I., Hawkins S. J., Jenkins S. R. (2008). Exploitation of rocky intertidal grazers: Population status and potential impacts on community structure and functioning. Aquat. Biol. 3, 1–10. doi: 10.3354/ab00072
McClanahan T. R., Arthur R. (2001). The effect of marine reserves and habitat on populations of East African coral reef fishes. Ecol. Appl. 11, 559–569. doi: 10.1890/1051-0761(2001)011[0559:TEOMRA]2.0.CO;2
McClanahan T. R., Muthiga N. A. (1988). Changes in Kenyan coral reef community structure and function due to exploitation. Hydrobiologia 166, 269–276. doi: 10.1007/BF00008136
McQuaid C., Branch G. (1985). Trophic structure of rocky intertidal communities’ response to wave action and implications for energy flow. Mar. Ecol. Prog. Ser. 22, 153–161. doi: 10.3354/meps022153
McQuaid C. D., Branch G. M., Crowe A. A. (1985). Biotic and abiotic influences on rocky intertidal biomass and richness in the southern benguela region. South Afr. J. Zool. 20, 115–122. doi: 10.1080/02541858.1985.11447923
Micheli F., Benedetti-Cecchi L., Gambaccini S., Bertocci I., Borsini C., Osio G. C., et al. (2005). Cascading human impacts, marine protected areas, and the structure of Mediterranean reef assemblages. Ecol. Monogr. 75, 81–102. doi: 10.1890/03-4058
Moreno C. A., Lunecke K. M., Lepez M. I. (1986). The response of an intertidal Concholepas concholepas population to protection from man in southern (Gastropoda) Chile and the effects on benthic sessile assemblages. Oikos 46, 359–364. doi: 10.2307/3565835
Moreno C. A., Sutherland J. P., Jara H. F. (1984). Man as a predator in the intertidal zone of southern Chile. Oikos 42, 155–160. doi: 10.2307/3544787
Nakin M. D. V., Booth A. J., McQuaid C. D. (2012). Species-specific effects of marine reserves: Mortality and growth differ within and among heavily exploited and rarely exploited limpets. Mar. Ecol. Prog. Ser. 445, 53–63. doi: 10.3354/meps09470
Nakin M. D. V., McQuaid C. D. (2014). Marine reserve effects on population density and size structure of commonly and rarely exploited limpets in south Africa. Afr. J. Mar. Sci. 36, 303–311. doi: 10.2989/1814232X.2014.946091
Nakin M. D. V., McQuaid C. D. (2016). Effects of marine reserves on the reproductive biology and recruitment rates of commonly and rarely exploited limpets. Afr. J. Mar. Sci. 38, 549–561. doi: 10.2989/1814232X.2016.1255255
Nel R., Punt A. E., Hughes G. R. (2013). Are coastal protected areas always effective in achieving population recovery for nesting sea turtles? PloS One 8, e63525. doi: 10.1371/journal.pone.0063525
Orozco Á., Guallart J., Templado J. (2013). Population status of the endangered limpet Patella ferruginea (Mollusca: Patellidae) in the peñón de vélez de la gomera (south-west Mediterranean Sea). Mar. Biodivers. Records 6, 1–6. doi: 10.1017/S1755267213001024
Parkington J., Fisher J. W. Jr, Kyriacou K. (2013). Limpet gathering strategies in the later stone age along the cape West coast, south Africa. J. Island Coast. Archaeol. 39 pp, 91–107. doi: 10.1080/15564894.2012.756084
Pfaff M. C., Logston R. C., Raemaekers S. J. P. N., Hermes J. C., Blamey L. K., Cawthra H. C., et al. (2019). A synthesis of three decades of socio-ecological change in false bay, south Africa: Setting the scene for multidisciplinary research and management. Elementa Sci. Anthropocene 7, 32. doi: 10.1525/elementa.367
R Core Team (2019). R: A language and environment for statistical computing (Vienna, Austria: R Foundation for Statistical Computing). Available at: https://www.R-project.org/.
Reimers B., Griffiths C. L., Hoffman M. T. (2014). Repeat photography as a tool for detecting and monitoring historical changes in south African coastal habitats. Afr. J. Mar. Sci. 36, 387–398. doi: 10.2989/1814232X.2014.954618
Rius M., Kaehler S., McQuaid C. D. (2006). The relationship between human exploitation pressure and condition of mussel populations along the south coast of south Africa. South Afr. J. Sci. 102, 130–136. doi: 10.10520/EJC96520
Sagarin R. D., Ambrose R. F., Becker B. J., Engle J. M., Kido J., Lee S. F., et al. (2007). Ecological impacts on the limpet Lottia gigantea populations: Human pressure over a broad scale on island and mainland intertidal zones. Mar. Biol. 150, 399–413. doi: 10.1007/s00227-006-0341-1
Sharpe A. K., Keough M. J. (1998). An investigation of the indirect effects of intertidal shellfish collection. J. Exp. Mar. Biol. Ecol. 223, 19–38. doi: 10.1016/S0022-0981(97)00150-0
Sink K. (2016). The marine protected areas debate: Implications for the proposed phakisa marine protected areas network. South Afr. J. Sci. 112, 1–4. doi: 10.17159/sajs.2016/a0179
Sink K. J., Branch G. M., Harris J. M. (2010). Biogeographic patterns in rocky intertidal communities in KwaZulu-natal, south Africa. Afr. J. Mar. Sci. 27, 81–96. doi: 10.2989/18142320509504070
Sink K., Harris L., Skowno A., Livingstone T., Franken M., Porter S., et al. (2019). “Chapter 3: Marine ecosystem classification and mapping,” in 2019. south African national biodiversity assessment 2018 technical report, vol. 4 . Eds. Sink K. J., van der Bank M. G., Majiedt P. A., Harris L. R., Atkinson L. J., Kirkman S. P., Karenyi N. (Pretoria. South Africa: Marine Realm. South African National Biodiversity Institute). Available at: http://hdl.handle.net/20.500.12143/6372.
Steffani C. N., Branch G. M. (2003). Temporal changes in an interaction between an indigenous limpet Scutellastra argenvillei and an alien mussel Mytilus galloprovincialis: Effects of wave exposure. Afr. J. Mar. Sci. 25, 213–229. doi: 10.2989/18142320309504011
Steneck R. S., Dethier M. N. (1994). A functional group approach to the structure of algal-dominated communities. Oikos 69, 476–498. doi: 10.2307/3545860
Tuya F. C., Soboil M. L., Kido J. (2000). An assessment of the effectiveness of marine protected areas in the San Juan islands, Washington, USA. ICES J. Mar. Sci. 57, 1218–1226. doi: 10.1006/jmsc.2000.0808
Underwood A. J., Jernakoff P. (1984). The effects of tidal height, wave exposure, seasonality and rock pools on grazing and the distribution of intertidal macroalgae in new south Wales. J. Exp. Mar. Biol. Ecol. 75, 71–96. doi: 10.1016/0022-0981(84)90024-8.
Van Erkom Schurink C., Griffiths C. L. (1990). Marine mussels of southern Africa–their distribution patterns, standing stocks, exploitation and culture. J. Shellfish Res. 9, 75–85. Available at: cir.nii.ac.jp/crid/1573387449010334976
Von der Heyden S. (2009). Why do we need to integrate population genetics into south African marine protected area planning? Afr. J. Mar. Sci. 31, 263–269. doi: 10.2989/AJMS.2009.31.2.14.886
Wang Y. I., Naumann U., Wright S. T., Warton D. I. (2012). Mvabund–an r package for model-based analysis of multivariate abundance data. Methods Ecol. Evol. 3 (3), 471–474. doi: 10.1111/j.2041-210X.2012.00190.x
Warton D. I., Blanchet F. G., O’Hara R. B., Ovaskainen O., Taskinen S., Walker S. C., et al. (2015). So many variables: Joint modeling in community ecology. Trends Ecol. Evol. 30, 766–779. doi: 10.1016/j.tree.2015.09.007
Warton D. I., Wright S. T., Wang Y. (2012). Distance-based multivariate analyses confound location and dispersion effects. Methods Ecol. Evol. 3, 89–101. doi: 10.1111/j.2041-210X.2011.00127.x
Wieters E. A., Broitman B. R., Branch G. M. (2009). Benthic community structure and spatiotemporal thermal regimes in two upwelling ecosystems: Comparisons between south Africa and Chile. Limnology Oceanogr. 54, 1060–1072. doi: 10.4319/lo.2009.54.4.1060
Will M., Kandel A. W., Kyriacou K., Connard N. C. (2016). An evolutionary perspective on coastal adaptations by modern humans during the middle stone age of Africa. Quaternary International 404 pp, 68–86. doi: 10.1016/j.quaint.2015.10.021
Wright D., Bishop J. M., Matthee C. A., von der Heyden S. (2015). Genetic isolation by distance reveals restricted dispersal across a range of life histories: Implications for biodiversity conservation planning across highly variable marine environments. Diversity Distributions 21, 698–710. doi: 10.1111/ddi.12302
Keywords: algae, ecosystem effects of fishing, harvesting, limpets, MPA, Table Mountain National Park
Citation: Baliwe NG, Pfaff MC and Branch GM (2022) Assessing the effects of no-take zones in a marine protected area spanning two ecoregions and rock substrate types. Front. Mar. Sci. 9:893260. doi: 10.3389/fmars.2022.893260
Received: 10 March 2022; Accepted: 28 November 2022;
Published: 16 December 2022.
Edited by:
Trevor Willis, Stazione Zoologica Anton Dohrn Napoli, ItalyReviewed by:
Iacopo Bertocci, University of Pisa, ItalyCharalampos Dimitriadis, National Marine Park of Zakynthos, Greece
Copyright © 2022 Baliwe, Pfaff and Branch. This is an open-access article distributed under the terms of the Creative Commons Attribution License (CC BY). The use, distribution or reproduction in other forums is permitted, provided the original author(s) and the copyright owner(s) are credited and that the original publication in this journal is cited, in accordance with accepted academic practice. No use, distribution or reproduction is permitted which does not comply with these terms.
*Correspondence: Ndiviwe Gabriel Baliwe, bmJhbGl3ZUBnbWFpbC5jb20=